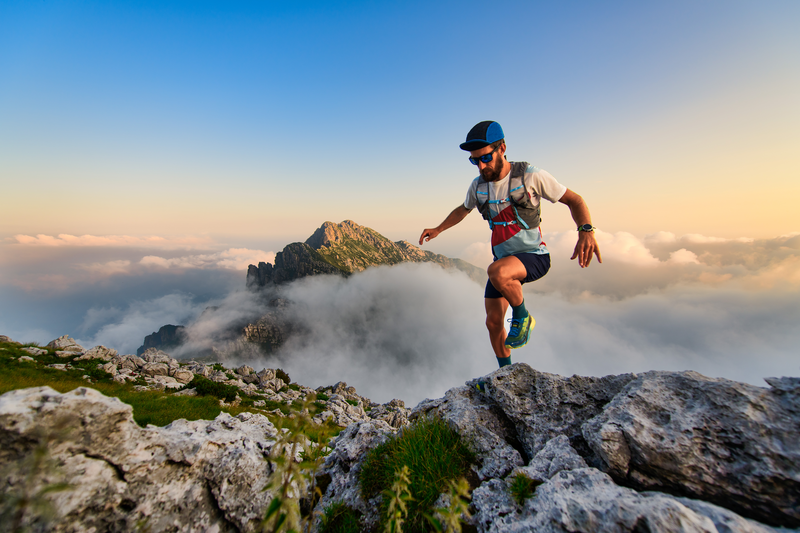
95% of researchers rate our articles as excellent or good
Learn more about the work of our research integrity team to safeguard the quality of each article we publish.
Find out more
ORIGINAL RESEARCH article
Front. Nutr. , 28 September 2023
Sec. Nutrition and Food Science Technology
Volume 10 - 2023 | https://doi.org/10.3389/fnut.2023.1254624
This article is part of the Research Topic Adaptation of Traditional Crop Cultivars to Climate Change in Terms of Nutritional Aspects View all 5 articles
Traditional rice is gaining popularity worldwide due to its high nutritional and pharmaceutical value, as well as its high resistance to abiotic and biotic stresses. This has attracted significant attention from breeders, nutritionists, and plant protection scientists in recent years. Hence, it is critical to investigate the grain metabolome to reveal germination and nutritional importance. This research aimed to explore non-targeted metabolites of five traditional rice varieties, viz., Chinnar, Chithiraikar, Karunguruvai, Kichili samba, and Thooyamalli, for their nutritional and therapeutic properties. Approximately 149 metabolites were identified using the National Institute of Standards and Technology (NIST) library and Human Metabolome Database (HMDB) and were grouped into 34 chemical classes. Major classes include fatty acids (31.1–56.3%), steroids and their derivatives (1.80–22.4%), dihydrofurans (8.98–11.6%), prenol lipids (0.66–4.44%), organooxygen compounds (0.12–6.45%), benzene and substituted derivatives (0.53–3.73%), glycerolipids (0.36–2.28%), and hydroxy acids and derivatives (0.03–2.70%). Significant variations in metabolite composition among the rice varieties were also observed through the combination of univariate and multivariate statistical analyses. Principal component analysis (PCA) reduced the dimensionality of 149 metabolites into five principle components (PCs), which explained 96% of the total variance. Two clusters were revealed by hierarchical cluster analysis, indicating the distinctiveness of the traditional varieties. Additionally, a partial least squares-discriminant analysis (PLS-DA) found 17 variables important in the projection (VIP) scores of metabolites. The findings of this study reveal the biochemical intricate and distinctive metabolomes of the traditional therapeutic rice varieties. This will serve as the foundation for future research on developing new rice varieties with traditional rice grain metabolisms to increase grain quality and production with various nutritional and therapeutic benefits.
Rice is the most important staple crop after wheat, and two-thirds of the world's population relies on rice as their main food supply (1). Globally, rice is cultivated on 167.2 million acres, and the current rice production is 769.4 million tons (2). In India, rice is cultivated on 45 million hectares of land with a production of 125 million tons (3), and West Bengal, Uttar Pradesh, Punjab, Odisha, Andhra Pradesh, Telangana, Chhattisgarh, Bihar, Tamil Nadu, Assam, and Madhya Pradesh are the major rice growing states in India. In India, rural communities typically eat traditional rice varieties with coarse grains, which have lower production costs than newer rice varieties with high-quality grains. After COVID-19, the eating habits of humans have been changed much, and all want to have quality and immune-boosting food grains. One of the food grains gaining attention in our diet is traditional rice varieties as they are rich in nutrients and bioactive compounds such as polyphenols, phytochemicals, antioxidants, vitamins, and minerals. Hence, the demand for traditional rice varieties in India has increased due to their higher nutritional qualities, which also help protect us from lifestyle disorders (4).
Tamil Nadu, a southern state of India, is home to ~400 traditional rice varieties, and very specifically, the Cauvery deltaic region is known for the collection of traditional rice germplasm. The varieties such as Kichili samba, Poongar, Thooyamalli, Chinnar, Karunguruvai, Milagu samba, Seeraga samba, Kaiviral samba, Mappillai samba, Karuppu kavuni, Kattuyanam, and Kuzhiyadichan are popular among them. These varieties have been especially preferred for their diuretic and anti-inphemmatory effects (5). Along with being an infant's first solid food, rice is also crucial to the baby shower celebration (6). For instance, in Tamil Nadu, a specific variety of rice called Mappillai samba is provided to the groom to increase fertility (7). The antioxidant potential of some medicinal rice varieties such as Kattuyanam, Mappillai samba, Navara, Karunguruvai, Kavuni, Kichadi samba, Illupai poo samba, Kalanamak, Garudan samba, and Seeraga samba can treat human ailments and cause various physiological changes in the human body (4). Karunguruvai is used for healing elephantiasis joint pain, chickenpox, Hansen's illness, cholera, skin sickness, venomous bites, urinary infections, and canine nibbles, and weakens bad cholesterol (8). However, the pharmaceutical values of these varieties may vary based on their individual genetic makeup.
The bioactive compounds present in rice include primary metabolites (sugars, lipids) and secondary metabolites (terpenoids, steroids, hydrocarbons, etc.) which are known to possess pharmaceutical and health-promoting values in addition to anticancer, anti-atherogenic, and blood sugar and cholesterol-regulating activities (9). However, the composition, characteristics, and functions of these phytochemicals in traditional rice varieties have not yet been completely explored and documented. This highlights the need for further study to describe these bioactive substances and enable their potential use.
Untargeted metabolomics provides comprehensive metabolite coverage, and the generated data can be utilized to generate or test hypotheses. It is not necessary to have prior knowledge of treatment-responsive compounds, and the findings can be utilized as a screening tool to direct further focused investigation of compounds and/or interest pathways (10). For instance, Rajagopalan et al. (11) found phytosterols in Mappillai samba that possess cholesterol-lowering, antioxidant, and anticancer properties. Ashokkumar et al. (12, 13) reported linoleic and oleic acids in Kaiviral samba. Sukhonthrea et al. (14) identified myristic acid, nonanal, (E)-β-ocimene, and 6,10,14-trimethyl-2-pentadenone in red rice and myristic acid, nonanal, caproic acid, pentadecanal, and pelargonic acid in black pigmented rice. Kotamreddy et al. (15) identified l-threonine, l-aspartic acid, tyrosol, hydroxytyrosol, 4-hydroxybenzoic acid, 4-coumaric acid, isoferulic acid, azelaic acid, and galactitol in rice. The bioactive features in rice bran include nutritional components such as hemicellulose, cellulose, arabinoxylan, polyphenolics, pectin, lignin, β-glucan, β-sitosterol, γ-oryzanol, vitamin E isomers, vitamin B9, essential amino acids, and micronutrients (16, 17). The beneficial secondary metabolites found in rice bran include dietary fiber, phytosteroids, oryzanol, tocopherol, tocotrienols, ferulic acids, and other phenolic compounds. Hence, in this study, we used brown rice to retain all bioactive compounds. According to reports, rice bran lowers blood and liver cholesterol, inhibits platelet aggregation, increases skin microcirculation, and prevents the development of ulcers (18).
In the current study, a triple quadrupole mass spectrometry-based gas chromatography (GC-MS/MS) metabolomics technique was used to examine the kinds and relative amounts of non-targeted-based primary and secondary metabolites in five traditional varieties, viz., Chinnar, Chithiraikar, Karunguruvai, Kichili samba, and Thooyamalli. Chemometric analyses such as principle component and partial least square discrimination analysis were used to classify the sample as diverse. Hierarchical cluster analysis and metabolic pathway identification were carried out to categorize the functional metabolites that possess a health advantage. The findings will offer a theoretical framework for the development of functional foods using traditional rice and identify molecular markers for deciding breeding programs.
The traditional rice varieties, viz., Chinnar, Chithiraikar, Karunguruvai, Kichili samba, and Thooyamalli, were selected for the study, and a legal permission letter for the collection of plant material adhering to institutional, national, and international guidelines and legislation was obtained from the Director of Research of the Tamil Nadu Agricultural University, Coimbatore, Tamil Nadu, India. The varieties were obtained from the local farmers of the Cauvery delta region of Tamil Nadu, India. They were grown with canal irrigation during the Samba season (July–December 2021) by adopting the required agronomic practices and harvested at physiological maturity. The growth location, agronomic practices, and harvest time were similar in the entire sampled farmer's field. These was given high significance as they will directly alter the chemical composition of grains. Detailed agronomical characteristics of the selected rice varieties are given in Table 1. Three biological replicates of samples were collected manually. Approximately 500 g of each variety was cleaned by removing foreign matter such as stones, straw, and dirt from each replication and sun-dried until the moisture content reached 12.0%. All the lab experiments were carried out in the Laboratory of the Center of Excellence in Soil Health, Anbil Dharmalingam Agricultural College and Research Institute, Tamil Nadu Agricultural University Campus, Trichy, Tamil Nadu, India, in January 2022.
One gram of the finely ground rice sample was placed in a 20 ml centrifuge tube and 10 ml of HPLC-grade ethanol was added. The tube was then vortexed (LABOID International, Himachal Pradesh, India) at 2,000 rpm for 10 min. The mixture was centrifuged at 5,000 rpm for 20 min (19). The supernatant was concentrated in a rotary evaporator and filtered using a 0.2 μm PVDF syringe filter. The filtrate was kept in an airtight glass vial at 4°C for chromatographic analysis.
The filtered ethanolic extract was subjected to metabolite analysis in a Thermo Fisher ISQ triple quadrupole gas chromatograph-mass spectrometer (Thermo Fisher TSQ 8000 Duo Triple Quadrupole GC-MS/MS). The GC was equipped with a fused silica capillary column DB-5 ms (30 m, 0.25 mm ID) with a film thickness of 0.25 μm. Helium was used as a carrier gas at a flow rate of 1.0 ml/min. Then, 1 ml of the sample was kept in a 2-ml screw-top vial in an auto-injector, and 1 μl of the sample was injected in split mode (1:10). The detector and injector temperature were maintained at 250°C. The oven temperature was programmed as 70°C for 15 min to 280°C at 30°C/min (10 min hold), up to 250°C at 10°C per min. The MS conditions were full scan mode, electron impact spectra at 70 eV, ion source temperature of 260°C, and transmission line temperature of 280°C. The mass scan range (m/z) was 50–650 amu with a solvent delay of 3 min (12). The bioactive molecules were identified by comparing mass spectra with the NIST 08 Mass Spectra Library (National Institute of Standards and Technology). The name, molecular weight, and structure were ascertained from the NIST, PubChem, and HMDB databases.
All the experiments were performed in triplicate. The online MetaboAnalyst 5.0 (20) software was used for the statistical analysis of the data. For univariate and multivariate analyses, the mean data were used to normalize the relative amounts of data for identified metabolites. A one-way ANOVA was performed on the dataset, followed by post-hoc Tukey's honestly significant difference (HSD) test with an adjusted p-value of 0.05 and a Student's t-test (P ≤ 0.05). The unsupervised approach of pattern identification known as PCA was first used to investigate the data matrix's inherent variation. Following that, the dataset's discriminating molecular characteristics were removed using PLS-DA, a supervised classification method.
These data were ordered according to their abundance value, and the most distinctive features were chosen for additional data clustering by extracting the most information from potentially discriminating features. In order to analyze and illustrate the similarities between sample replicates and to better understand the differences in metabolite compounds among different sample types, a hierarchical clustering analysis (HCA) dendrogram with heatmap representation was built. The dissimilarity was measured using the Euclidean distance, and the clusters were described using Ward's approach.
The five traditional rice varieties currently grown in Tamil Nadu, viz., Chinnar, Chithiraikar, Karunguruvai, Kichili samba, and Thooyamalli, were subjected to metabolome analysis using GC-MS/MS. Chithiraikar and Karunguruvai are pigmented types, while Chinnar, Kichili samba, and Thooyamalli are white-colored rice varieties (Figure 1). Non-targeted metabolomic analysis was employed to detect the metabolites in these rice varieties to fully understand the variations in their chemical composition. Chromatographic analysis of the grain metabolome detected about 149 compounds (Supplementary Figures S1–S5 and Supplementary Table S1) from the five traditional rice varieties, which were identified and categorized into 34 chemical classes using the NIST library and HMDB database. The identified 149 metabolites comprise 7 benzene and its substituted derivatives, 9 saturated hydrocarbons, 14 prenol lipids, 20 organooxygen compounds, 25 steroids and steroid derivatives, and 39 fatty acyls. The classes such as hydroxy acids and derivatives, phenols, purine nucleosides, quinoline derivatives, and unsaturated hydrocarbons hold two metabolites under each class (Table 2), and the remaining 22 classes share one metabolite under each category.
Table 2. Major classes of metabolites identified in the five traditional rice varieties and its concentration (area %).
Fatty acids shared 26.0% of the detected metabolites. Other contributions are made by steroids and steroid derivatives (17.0%), organooxygen compounds (14.0%), prenol lipids (9.0%), benzene and substituted derivatives (5.0%), carboxylic acids and derivatives (2.0%), and saturated hydrocarbons (6.0%) (Figure 2). The classes that predominate include fatty acyls (31.1–56.3%), steroids and steroid derivatives (1.80–22.4%), dihydrofurans (8.98–11.6%), prenol lipids (0.66–4.44%), organooxygen compounds (0.12–6.45%), benzene and substituted derivatives (0.53–3.73%), glycerolipids (0.36–2.28%), hydroxy acids and derivatives (0.03–2.70%), and benzothiazoles (1.23–3.17%) (Supplementary Table S1).
Figure 2. Pie chart illustrating the percentage of identified metabolites for each metabolite class. The various colors in each part of pie chart indicate various HMDB classifications.
Elaidic acid (0.32–21.5%), cis-vaccenic acid (0.10–21.4%), pentadecanoic acid (0.37–0.86%), and oleic acid (0.10–22.3%) were found to be the primary fatty acid components in the five rice varieties. The other fatty acids found in significant concentrations were tetracosanoic acid (0.46–0.86%), pelargonic acid (0.06–0.07%), and methyl stearic acid (0.25–3.19%). While a higher amount of palmitic acid was found in Chithiraikar (10.23%), capric acid (1.08%), and panaxydol (0.03%) were found only in Chinnar and Kichili samba, respectively.
Among the 25 steroid compounds, digitoxin (0.03–0.13%), cholestan-3-ol (0.01–0.33%), campesterol (0.01–1.13%), alpha-sitosterol (2.13–6.32%), and (22E, 24R)-stigmasta-4, 22-diene-3,6-dione (0.13–4.57%) were commonly detected in all the rice varieties. Predominate and specific metabolites identified in each rice variety are as follows: 16b-hydroxyestradiol, desmosterol, glycocholic acid in Thooyamalli; 9,19-cyclolanost-24-en-3-ol, (3á)-, calcitriol, á-D-mannofuranoside, 2,3:5,6-di-O-ethylboranediyl-1-O-(stigmasta-5, 22-dien-3-yl)-, and ergosta-5,22-dien-3-ol, acetate, (3á,22E) in Karunguruvai; cycloartenol in Kichili samba; and vitamin D3 in Kichili samba and Chithiraikar.
D-fructose, D-glucose, L-galactose, melezitose, melibiose, and nonanal were the commonly found carbohydrates and their conjugate-based organooxygen compounds (21 metabolites) among the five rice varieties. Out of 13 prenol lipids, cis-sesquisabinene hydrate, cymbopogonol, dehydroabietic acid, gamma-tocopherol, geranyl-PP, longispinogenin, Petasalbin, rhodopin, spirost-8-en-11-one, 3-hydroxy-, (3á,5à,14á,20á,22á,25R)-, and sugiol are the unique ones. All the saturated hydrocarbons (9) found in rice are alkanes. N-dodecane, 2-methylhexacosane, isopentacosane, and 3-methyltetradecane were found only in Chinnar, Thooyamalli, Karunguruvai, and Chithiraikar, respectively. However, a major olefin compound, azulene (0.38%), was accumulated only in Kichili samba. Though the benzene and substituted derivative classes were not detected in Kichili samba and Thooyamalli, the predominant of benzene compound 2,4-di-tert-butylphenol was detected among the other three varieties. Ascaridole was the only dioxane class present in Chinnar and Kichili samba. Among the two phenolic metabolites, viz., o-cresol and 2-methoxy-4-vinylphenol, the former was only recorded in Karunguruvai.
The number of metabolites in traditional rice grain metabolomes commonly present or differing among the five varieties was studied and is shown using a Venn diagram (Figure 3 and Supplementary Table S2). All five rice varieties showed 23 metabolites in common, and each variety had its own special metabolites. Out of the 149 metabolites, 23 (7 upregulated and 6 downregulated) were commonly found among the five rice varieties belonging to Aspidosperma tan-type alkaloids, dihydrofurans, fatty acyls, hydroxy acids and derivatives, organooxygen compounds, prenol lipids, saturated hydrocarbons, and steroids and steroid derivatives (Supplementary Figure S6). The remaining nine compounds were either increased or decreased in one of the rice varieties. For instance, (22E, 24R)-stigmasta-4,22-diene-3,6-dione was decreased in Chinnar, Karunguruvai, and Thooyamalli. However, the concentration of 1,25-dihydroxyvitamin D3-26,23-lactone was reduced in Kichili samba. Metabolomes such as 14-methylicosanoic acid, heptadecanoic acid, pentadecanoic acid, and tetradecanoic acid declined in Karunguruvai compared to other rice samples. A Venn diagram showed that 33 metabolites were specific to Karunguruvai, 22 to Chinnar, 9 to Chithiraikar, and each 13 to Kichili samba and Thooyamalli. It was found that 13 fatty acids and 4 steroids were common to all five varieties.
Figure 3. Venn diagram showing number of the commonly shared and differing metabolites among the five traditional rice varieties.
Both univariate and multivariate statistical analyses were carried out to assess the changes in metabolic profiles among the rice varieties. The one-way analysis of variance (ANOVA) statistical test illustrated how the identified metabolites varied among the five rice varieties (Figure 4). While the red color indicates the significant metabolites among the rice varieties, the green color indicates the metabolites of non-significance. Out of the 149 metabolites, 94 distinct metabolites were found to vary significantly (P ≤ 0.05) across all the varieties. The precise identities and P-value of the metabolites are shown in Supplementary Table S3.
Figure 4. Analysis of variance (ANOVA) plot showing significantly (P < 0.05) detected metabolites in traditional varieties. Each red dot represents one metabolite with significance, while the green dot represents without statistical significance. The detailed metabolite identities are given in Supplementary Table S2.
The processed grain metabolite list was subjected to chemometric analysis techniques such as principal components analysis (PCA), partial least squares-discriminant analysis (PLS-DA), and hierarchical clustering analysis (HCA) to reduce the data dimensionality and enhance data interpretability. All the identified 149 metabolites were subjected to PCA analysis and were reduced to five major principal components (PCs) using the normalized data that indicates major differences between the metabolite data. The PCs with eigenvalues >1 were retained in the study. Figure 5 and Supplementary Figure S6A show the score plots for the five rice varieties. According to the PCA score graph, the metabolite compositions among the five rice varieties differed statistically and significantly from one another. In the PCA score plot, Kichili samba and Chinnar are closely located, which shows a high degree of metabolite similarity among them. The chosen PCs between the score plots indicated that the metabolite loadings of Kichili samba and Chinnar were on the negative side and the others remained on the positive side.
The extracted five PCs explained 96% of the total variance as depicted in the scree plot (Supplementary Figure S6B and Supplementary Table S4). The 36% of variance was explained by PC1 and was correlated with dimethylcycloergostenol, decenal, methyl decenol, dimethoxynonane, dihydroxyvitamin lactone, methylicosanoic acid, methylnonadecanoic acid, methylenecycloartanol, hydroxymethyl furancarboxaldehyde, elaidic acid, heptadecanoic acid, oleic acid, palmitic acid, and tetracosanoic acid. Similarly, the PC2 (25.2% variance) was associated with dioxolane, hydroxyestradiol, di-tert-butylphenol, benzothiazole, and dodecanoic acid in addition to the PC1 metabolites. Similarly, 16.8% of the variance contributed to PC3 was correlated with cis-vaccenic acid, eicosenoic acid, methoxy vinylphenol, hydroxydodecanoic acid, ascorbic acid, and pentadecanoic acid. The PC4 with 14.6% variance was attributed by hexadecanethiol, hexadecane, and octadecane, and PC5 (3.4%) was majorly highlighted by methylenecycloartanol, methoxy vinylphenol, and hydroxymethyl furancarboxaldehyde. The PC analysis showed that the rice varieties differed significantly in terms of both individuals and combinations (Supplementary Figure S6C).
A pathway topology analysis was carried out to identify the major metabolic pathways in rice varieties, and the outcome depicting the pathway of distinct metabolites from the five traditional rice varieties is presented as a bubble chart in Figure 6. Approximately 18 important metabolic pathways were identified by pathway topology and impact values (cumulative percentage of the metabolite nodes). Four significant metabolic pathways with false discovery rate (FDR) values ≤0.05 were found when the Kyoto Encyclopedia of Genes and Genomes (KEGG) database was used to map the pathways of significant metabolites. The steroid biosynthesis pathway had the highest log (p) value of 3.6682 and was followed by the fatty acid biosynthesis pathway, which had a value of 2.4391. Furthermore, the unsaturated fatty acid biosynthesis pathway with a value of 1.8831 and primary bile acid biosynthesis (1.5955) were mapped (Supplementary Table S5 and Figure 5).
Figure 6. Metabolic pathways identified among the five traditional rice varieties. Each bubble in the plot represents a metabolic pathway whose abscissa and bubble size jointly indicate the magnitude of the impact factors of the pathway in the topological analysis. A larger bubble size indicates a larger impact factor.
The pathways that have greater impact values are glyoxylate and dicarboxylate metabolism, glycine, serine and threonine metabolism, steroid biosynthesis, fatty acid biosynthesis, primary bile acid biosynthesis, histidine metabolism, retinol metabolism, glutathione metabolism, and steroid hormone biosynthesis.
The differential metabolites found in the present investigation demonstrated numerous biologically identical or complimentary roles, and they were positively or negatively controlled by the same metabolic pathway. In order to further investigate this metabolite dataset, an agglomerative hierarchical clustering analysis model was built based on the metabolites to assess the similarities in the detection of inter-group variance in metabolite features of the grain samples. The differential metabolites were grouped using a complete-linkage method and shown as thermograms after the Euclidean distance matrix was calculated for the quantitative values of each group of comparisons. A dendrogram was used to display the cluster memberships of the traditional rice samples along with heatmap visualization.
The pattern of the top 35 metabolite distributions among the samples is visualized as a heatmap (Figure 7). Metabolites are shown in decreasing order of content by the color gradation from red to yellow. More than 50% of the highlighted metabolites are present in the samples with high relative abundances. While cluster 1 consisted of two varieties, namely Chithiraikar and Kichili samba, cluster 2 was found to consist of Chinnar, Karunguruvai, and Thooyamalli. These two clusters are distributed equally with similar distances; however, the varieties in cluster 1 are chemically similar to each other.
Figure 7. Heatmap representing the changes in soluble metabolites related to five traditional rice varieties which were drawn in MetaboAnalyst 5.0 platform. Each rice sample is shown as a single column in the heatmap, and each metabolite is shown as a single row. Different shades represent metabolite accumulation, whereas red represents the trend of decrease and yellow represents a rising trend (color key scale on the right of heatmap).
Unsupervised models such as PCA analysis are used to separate the sample based on the variations in factors, whereas supervised analysis explains how far the samples can be discriminated by a particular factor. Partial least squares-discriminant analysis (PLS-DA) is a supervised classification of multivariate analysis that identifies sample variation through dimension reduction and correlation among the variables and is a projection method.
To assess the compounds responsible for the major differences between the traditional rice varieties, a variable importance in projection (VIP) plot by PLS-DA was employed. Fold changes of >2 or 0.5 were fixed as screening thresholds in combination with PLS-DA model analysis (VIP > 1.0) to identify significantly different metabolites among the five rice varieties (Supplementary Table S6). Seventeen compounds that had a VIP score higher than 1.0 are Tetracosanoic acid, dimethoxynonane, dihydroxyvitamin lactone, di-tert-butylphenol, decenal, hydroxyestradiol, methyl decenol, methoxy vinylphenol, oleic acid, methylenecycloartanol, dodecanoic acid, benzothiazole, elaidic acid, methylnonadecanoic acid, eicosenoic acid, hydroxymethyl furancarboxaldehyde, and cis-vaccenic acid (Figure 8).
Figure 8. VIP score plot showing the top 17 (score >1) most important metabolite features identified by PLS-DA. The statistical analysis was carried out with the same dataset as in Figure 5.
We conducted Person's correlation coefficient analysis of the five rice varieties to better comprehend the relationship between metabolite composition and discovered 2,438 significant correlation coefficients (p < 0.01, r2 ≥ 0.49) in the study, which included 1,976 and 463 positive and negative significant correlations, respectively (Figure 9). The findings revealed that certain sugars, fatty acids, and amino acids, including proline, norleucine, and phenylalanine, had the greatest concentration fluctuations. These sugars included fructose, glucopyranose, and raffinose. Correlation clustering divided the 149 metabolites into six clusters that took part in closely associated metabolic pathways. Fatty acids, sugars, hydrocarbons, and cresol were placed in cluster 1. Cluster 2 contained 11 compounds, viz, fatty acids, steroids, sugar, and alkaloids, which are positively linked. Of the 22 compounds found in cluster 3, 10 were steroids, 8 were fatty acids, isosorbide, phosphonoacetate, and ethoxyquin. Cluster 4 claimed 20 metabolic features, and the majority of them were fatty acids and prenols. Clusters 5 and 6 were dominated by primary metabolites with few secondary metabolites.
Figure 9. Correlation matrix and cluster analysis of data for 149 metabolites of traditional rice varieties.
The present investigation was carried out to elucidate the complex grain metabolome of the well-known nutritious and medicinal rice varieties of the Cauvery deltaic region of Tamil Nadu. The primary and secondary metabolites are abundantly found in rice grains. The synthesis of macromolecules that are important for yield and quality, such as starch, is facilitated by primary metabolites. Rice has the capacity to amass an extensive array of secondary metabolites, encompassing phenolic acids, flavonoids, terpenoids, steroids, and alkaloids. These molecules showcase attributes advantageous to humans (Table 3), such as cytotoxic, antitumor, anti-inflammatory, antioxidant, and neuroprotective qualities. As an illustration, numerous antioxidant activities are attributed to elements such as phenolic acids, flavonoids, tocopherols, tocotrienols, γ-oryzanol, and phytic acid found within rice (43).
Table 3. Detailed information about important metabolites and their corresponding biological significance.
Additionally, several secondary metabolites have dietary purposes. For instance, the outer layer of rice grain has a distinctive profile of phytochemicals with therapeutic and dietary benefits for human health. A few researchers have attempted to quantify the biochemical constituents in the traditional rice grain varieties and the metabolomics of one or two in comparison with modern varieties (11, 12). However, no comprehensive study has examined the metabolite profiling of the nutritionally and therapeutically rich traditional rice varieties belonging to the Cauvery delta region of southern India. After COVID-19, traditional rice has drawn increased interest from nutritionists, consumers, and health advisors because of its higher significance in terms of its biological activity, nutritional content, and significant impact on human health. They are more concentrated in fiber, iron, calcium, vitamins, minerals, and other bioactive compounds than modern and hybrid rice varieties. Furthermore, Indian traditional rice helps increase the milk secretion of lactating mothers and is used to cure a variety of illnesses, including rheumatism, leukorrhea, gastrointestinal problems, and skin conditions (12).
In addition to being significant energy sources, carbohydrates serve as the building blocks for the production of starch and maintain metabolic homeostasis, as well as a number of other processes, including the pathology and physiology of the large intestine, dental health, and the prevention of the onset of chronic non-communicable diseases. These mechanisms are contingent upon the specific carbohydrates ingested, their quantities, and their equilibrium with other essential nutrients. The current study confirms the presence of monosaccharides, disaccharides, oligosaccharides, aldehydes, alcohols, and polyols in all the traditional rice varieties. Paromomycin, an antibiotic belonging to the aminoglycoside class found only in Thooyamalli, has demonstrated dose-dependent effectiveness in addressing visceral leishmaniasis (22). The compound 9-undecenal, identified within Chinnar, has been established to exhibit odorant receptor properties, as evidenced by the research of Trimmer et al. (44). Other organooxygen compounds found in this investigation are sinigrin (antiproliferative activity) (45) and nonanal (olfactory simulation effect) (40).
Fatty acids are a group of lipids that play a crucial structural, functional, and biological role in addition to serving as a significant energy source. Most of these fatty acids can be produced by the human body, with the exception of important polyunsaturated fatty acids (PUFAs), viz., omega-3, omega-6, and omega-9, which are found in traditional rice varieties. Omega-3 and omega-6 fatty acids can also lower blood cholesterol levels and the risk of cardiovascular diseases (23). Eicosapentaenoic acid (EPA) is one of the significant omega-3 fatty acids present only in Kichili samba. It plays an essential role in the anti-inflammatory process (34) and proper fetal brain and retina development (35). EPA enhances plaque stability, lessens endothelial activation, and increases vascular permeability, which lowers the risk of suffering a cardiovascular issue (46). By influencing important blood glucose metabolism enzymes, palmitoleic acid lowers insulin resistance in diabetics and controls insulin secretion in patients (47). According to earlier research findings (48), the two fatty acids, namely oleic acid and linoleic acid, which make up 75% of the total unsaturated fats in rice bran oil, are primarily responsible for decreasing cholesterol levels. Oleic acid is a monounsaturated fatty acid that can reduce blood cholesterol levels, improve cell membrane permeability, and prevent myocardial block and arteriosclerosis (49). Our study agrees with the literature that the Garudan Samba possesses 9,12-octadecadienoic acid (Z, Z), which is responsible for anti-arthritic, 5-alpha-reductase inhibiting, hypocholesterolemic, hepatoprotective, anti-androgenic, anti-coronary, and anti-histaminic effects (50).
Rice terpenoids include monoterpenoids, sesquiterpenoids, diterpenoids, and triterpenoids. 1,4-Cineole is one of the monoterpenoids that has anti-inflammatory activity (51). Squalene, a triterpenoid molecule with pharmacological significance that exhibits anti-inflammatory, immunostimulant, anticancer, and antioxidant properties (52), is also present in all five rice varieties. Squalene's main role is to shield the surface of human skin from lipid peroxidation brought on by exposure to UV and other ionizing radiation (53). Sesquiterpenoids are another group of secondary metabolites that influence the rice aroma (43). To the best of our knowledge, this is the first time that gamma-tocopherol has been reported in the grains of Chithiraikar which is a quinone and hydroquinone lipid-based metabolite. This has an antioxidant function (54), encourages the healing of plasma membranes (55), enhances cardiovascular performance (56), and reduces the expansion of cancer cells. Our study corroborates previous findings, which also detected the presence of alpha-tocopherol in Mappillai samba (11). Sugiol, a diterpenoid present in Chinnar, has the potential to function as an antimicrobial, antioxidant, anti-inflammatory, anti-carcinoma, antiviral, and cardiovascular agent (21).
In the course of this investigation, we identified two previously undiscovered carotenoids, namely, 7,7′,8,8′-tetrahydrolycopene and 1-hydroxylycopene, present in all five rice varieties. These carotenoids are renowned antioxidants known for safeguarding DNA, proteins, and lipids against oxidative damage (32). All-trans-retinoic acid (ATRA) is a biologically active form of vitamin A and serves as a crucial signaling molecule in various physiological processes. ATRA assumes pivotal roles across a diverse spectrum of biological processes, encompassing embryonic morphogenesis, organogenesis, cell proliferation, differentiation, apoptosis, homeostasis, and their associated disorders (57). Dehydroabietic acid is a prominent example within the aromatic abietane category. Similar to many other diterpenoids, aromatic abietanes are predominantly recognized for their roles as chemical defense agents. This group has been attributed with a range of biological activities, including antimicrobial, antileishmanial, antiplasmodial, antifungal, antitumor, cytotoxic, antiviral, antiulcer, cardiovascular, antioxidant, and anti-inflammatory activities, as documented in existing reports (58).
Inosine is a purine nucleoside found in Karunguruvai and has been under investigation in clinical trials for its potential in treating neurological disorders, including Parkinson's disease (59).
Nine alkanes were present in the studied varieties, which are in agreement with earlier observations by Krishnanunni et al. (19) and Ashokkumar et al. (12, 13) in rice. Eicosane has antimicrobial activity (60) that is found only in Chinnar and Chithiraikar. Tetradecane, hexadecane, and pentadecane are known to possess antifungal and antibacterial effects (61). Azulene is the only unsaturated carbon-based olefin present in Kichili samba, and Chithiraikar possesses antineoplastic, analgesic, antidiabetic, and antiretroviral properties in the treatment of HIV-1 and antifungal properties. Regular intake of Kichili samba builds muscles and increases the immune system (4).
Trigonelline is an alkaloid present in Kichili samba and has been shown to alleviate diabetic auditory neuropathy and platelet aggregation. It also exhibits hypoglycemic, hypolipidemic, neuroprotective, antimigraine, sedative, memory-improving, antibacterial, antiviral, and antitumor properties (39).
Plants' membrane lipid bilayer is made up in large part by plant steroids, also known as phytosterols. They control membrane fluidity, affecting the structure, features, and functions of the membrane (62). In this investigation, all five crucial phytosterols, namely, campesterol, stigmasterol, desmosterol, brassicasterol, and alpha-sitosterol, were observed to be prevalent in all the studied varieties and reported to have a variety of physiological impacts. This contradicts earlier findings concerning conventional rice, as noted by Ashokkumar et al. (12, 13). Campesterol which has antioxidant and hypocholesterolemic properties (19), β-sitosterol is known for its hypocholesterolemic, antisterility, and anticancer properties (63), whereas stigmasterol is being used as a precursor in the synthesis of semi-synthetic progesterone (33). Stigmasterol has additionally been documented to display antihepatotoxic, anti-inflammatory, antioxidant, antiviral, anticancer, and antihypercholesterolemic effects (19). Therefore, consuming pigmented rice as part of a healthy diet is one of the long-term methods to prevent the spread of breast cancer. However, the studied five varieties (white and red) possess all the phytosterols that make them one of the most balanced diets to prevent the metastasis of breast cancer. Traditional pigmented rice cultivars have the ability to sustain glucose homeostasis, making them helpful for the management of diabetes mellitus (64). Consequently, these traditional varieties (unpolished and hand-milled) are popular among local consumers and thought to be the best for diabetes patients due to the presence of significant phytosterols.
The secosteroid vitamin D3 is inversely related to respiratory infection (65) by producing cathelicidin and defensin peptides (62) that have antimicrobial activities against various microorganisms, including bacteria, viruses, and fungi (66). A study conducted by Pinzon et al. (67) found that a vitamin D shortage might be a risk factor for causing viral-related infections. In our study, vitamin D3 was possessed by Chithiraikar and Kichili samba. The popularity of eating these traditional varieties has increased after the COVID-19 pandemic. Hence, we first report here that the presence of seco-steroids in traditional varieties might be the reason for resistance to respiratory-related viral infections. Calcitriol is one of the hormonally active forms of vitamin D present only in Karunguruvai, exerts antiproliferative impacts in various cancers and malignant cells, including prostate cancer, raising the significance of its utilization as an anticancer agent (68). Therefore, this variety can be recommended to people to prevent the formation of malignant cells and also to those having treatment for the same. In spite of this, a detailed study is required to explore the antiproliferative properties of Karunguruvai.
A bile acid derivative, namely glycocholic acid, is present in Karunguruvai, and Thooyamalli is reported to have clinical importance in absorbing fat- and fat-soluble vitamins and reducing the risk of bile acid amidation defects (69). Neurosteroid found only in Thooyamalli is pregnenolone, which exerts anxiety and depression-regulatory mechanisms (70).
Phenolic acids are key auxiliary metabolites generally found in plant-based food varieties, such as grains, organic products, and vegetables, and provide different biological activities, including cancer prevention agents, antimicrobial, antitumor, and mitigating inflammatory issues (71). The main phenolic acids in Cauvery deltaic traditional rice are o-cresol and 2-methoxy-4-vinylphenol. Ethylbenzoic acid was found in the Karunguruvai rice. Ribavirin is a ribonucleoside found only in Chinnar. While exhibiting activity against a wide range of viruses, ribavirin finds its prominent clinical utility in treating respiratory syncytial virus among pediatric patients, along with managing chronic hepatitis C virus (HCV) infection in both the children and adult populations (72).
More than 200 volatile substances, including (E,E)-2,4-decadienal, hexanal, octanal, nonanal, 4-vinylphenol, and 4-vinyl guaiacol, are considered to influence the aroma of rice, with 2-acetyl-1-pyrroline (2-AP) serving as the main volatile aromatic molecule (10). We report the presence of nonanal for the first time in Chithiraikar, Karunguruvai, and Kichili samba.
The intricate metabolic reactions within living organisms are tightly interconnected and often orchestrated by a multitude of genes and proteins. These components collaboratively construct intricate pathways and networks, intertwining and governing each other's functions. Consequently, this interconnected process culminates in comprehensive modifications to the metabolome, as outlined by Töpfer et al. (73). In our investigation, we meticulously delineated and organized pathways to elucidate the differentially expressed metabolites in the individual traditional varieties.
To gather pertinent data about the similarities and differences in the metabolite profiles between the grain samples, HCA was built based on the metabolites. The metabolite profiles' diversity was shown through cluster analysis. The clustering of the samples based on the top 35 secondary metabolites demonstrates the analytical parameters' capacity to discriminate in this situation. A single linkage with correlation as a measure of similarity was used to create the dataset. More than 50% of the highlighted metabolites present in the samples have high relative abundances, as demonstrated in Figure 6 (represented in red or yellow).
According to the heatmap-based dendrogram, cluster 1 (Chithiraikar and Kichili samba) is more compact, which implies that the metabolites are comparable with each other within the cluster group. In contrast, cluster 2′s (Chinnar, Karunguruvai, and Thooyamalli) metabolites are comparatively spaced out, thus indicating that they are more chemically diverse than the cluster 1 samples. The metabolite variation within the cluster and between the clusters might be due to the genetic makeup of the individual rice variety and environment (74, 75). Therefore, unequivocally, the HCA results might be affected by genetic differences (76). We also found that this difference was not altered by the growth location, management practice, or harvest time, as they were similar to each other.
The metabolic features present in the clusters were positively correlated with each other. Fatty acids and sugars present in clusters 1 and 2 are positively correlated with each other, except dioxalones, hydroxylycopene, aspidospermidine, and undecanoic acid which are negatively correlated.
The variable importance in the projection (VIP >1) and P-value of Student's t-test (p > 0.05) were employed in this experiment to identify the metabolites with differential expression (Figure 7). The 17 differential metabolites with substantial variations were selected from five rice varieties. These metabolites include nine different types of fatty acids, three different kinds of steroids, two benzenes and substituted derivatives, a type of benzothiazole, a type of phenol, and hydroxy acids and derivatives. Out of 17 VIP metabolites, 7 (4 upregulated, 3 downregulated) were common to all the rice varieties. Phenolic acids are viewed as natural antioxidants, having the potential to scavenge free radicals that might increment oxidative pressure (77); hence, rice phenols are always correlated with antioxidant capacity (78). One such phenolic metabolite, 2,4-di-tert-butylphenol (DTBP), present in studied rice varieties has been shown to have a variety of biological properties, including antibacterial (79), antifungal (80), and anticancer activity (31, 81), which was not present in Kichili samba. A methoxy vinylphenol that acts as an anti-cell proliferative agent (82) was not associated with Karunguruvai.
Traditional rice boasts inherent antioxidant content and therapeutic attributes, enabling its integration into an array of functional foods, food additives, nutraceuticals, pharmaceuticals, and cosmetics. Consequently, the study underscores the potential for enhanced health benefits through the regular consumption of traditional rice (Figure 10). To capitalize on this potential, the study recommends an augmentation of production in the processing industry, particularly focusing on the creation of convenient food items such as ready-to-eat, ready-to-cook, and instant foods.
Figure 10. Therapeutic properties of five traditional rice varieties and their functions on human health.
The present study is the first to provide extensive data on the variation of bioactive metabolomes in the traditional rice varieties (except Kichili samba) of the Cauvery deltaic region, South India. Untargeted metabolomics of five traditional rice varieties showed 149 metabolites under 34 chemical classes exhibiting pharmaceutical and health functions. Further metabolic pathway analysis shows that these traditional rice grain metabolomes have a tremendous role in physiological pathways and hence could be a reference for future breeding programs. The pharmaceutically significant traits of the identified therapeutic/nutraceutical compounds in the grains of these varieties may serve as potential leads for the genetic mapping of these traits, accelerating the development of high-yielding rice varieties with enhanced therapeutic/nutritional qualities. In the current study, it was discovered that the traditional rice cultivars from Tamil Nadu have a wide range of phytochemicals or bioactive compounds, which may help scientists, policymakers, and rice producers to enhance the acreage of cultivation. In Tamil Nadu, roughly more than 400 traditional varieties are available for cultivation. However, only a few of these landraces have undergone a thorough investigation of their grain end-use quality, nutritional characteristics, and possible therapeutic advantages. Therefore, there is an urgent need to validate the science and confirm the traditional knowledge connected to these varieties.
The original contributions presented in the study are included in the article/Supplementary material, further inquiries can be directed to the corresponding authors.
SV: Conceptualization, Data curation, Formal Analysis, Investigation, Methodology, Resources, Software, Writing—original draft, Writing—review and editing. DU: Conceptualization, Data curation, Formal Analysis, Investigation, Methodology, Resources, Software, Writing—original draft, Writing—review and editing. KS: Investigation, Resources, Validation, Writing—review and editing. PJ: Conceptualization, Investigation, Methodology, Resources, Supervision, Visualization, Writing—review and editing. BP: Investigation, Resources, Validation, Writing—review and editing. SS: Investigation, Resources, Validation, Writing—review and editing. SV: Investigation, Resources, Validation, Writing—review and editing. SM: Investigation, Resources, Supervision, Validation, Visualization, Writing—review and editing. NB: Investigation, Resources, Supervision, Validation, Visualization, Writing—review and editing. MR: Validation, Visualization, Writing—review and editing, Investigation, Resources, Supervision. VG: Investigation, Resources, Supervision, Validation, Visualization, Writing—review and editing. ES: Investigation, Resources, Supervision, Validation, Visualization, Writing—review and editing.
The authors are indebted to the Center of Excellence in sustaining Soil Health, Anbil Dharmalingam Agricultural College and Research Institute, Tamil Nadu Agricultural University, Trichy, for providing the necessary laboratory and instrumentation facilities to support the study.
The authors declare that the research was conducted in the absence of any commercial or financial relationships that could be construed as a potential conflict of interest.
All claims expressed in this article are solely those of the authors and do not necessarily represent those of their affiliated organizations, or those of the publisher, the editors and the reviewers. Any product that may be evaluated in this article, or claim that may be made by its manufacturer, is not guaranteed or endorsed by the publisher.
The Supplementary Material for this article can be found online at: https://www.frontiersin.org/articles/10.3389/fnut.2023.1254624/full#supplementary-material
2. Food and Agriculture Organization. The State of Food Security and Nutrition in the World 2017: Building Resilience for Peace and Food Security International Fund for Agricultural Development, UNICEF, World Food Programme, WHO, Rome.
3. IPAD. Available online at: https://ipad.fas.usda.gov/country~summary/Default.aspx?idINandCropRice (accessed March 23, 2023).
4. Kowsalya P, Sharanyakanth P, Mahendran R. Traditional rice varieties: a comprehensive review on its nutritional, medicinal, therapeutic and health benefit potential. J Food Comp Anal. (2022) 114:104742. doi: 10.1016/j.jfca.2022.104742
5. Umadevi M, Pushpa R, Sampathkumar KP, Bhowmik D. Rice traditional medicinal plant in India. J Pharmacogn Phytochem. (2012) 1:6–12.
6. Rathna Priya T, Eliazer Nelson ARL, Ravichandran K. Nutritional and functional properties of coloured rice varieties of South India: a review. J Ethn Food. (2019) 6:11. doi: 10.1186/s42779-019-0017-3
7. Sulochana S, Singaravadivel K. A study on phytochemical evaluation of traditional rice variety of Tamil Nadu -'Maappillai Samba' by GC-MS. Int J Pharma Biosci. (2015) 6:606–11.
8. Pushpam R, Mythili SR, Nikitha TC. Medicinal rice and its medicinal values. Int J Curr Microbiol Appl Sci. (2019) 8:2090–5. doi: 10.20546/ijcmas.2019.810.243
9. MohdEsa N, Ling TB. By-products of rice processing: an overview of health benefits and applications. Rice Res. (2016) 4:1–11. doi: 10.4172/jrr.1000107
10. Lee DE, Lee S, Singh D. Time-resolved comparative metabolomes for Koji fermentation with brown-, white-, and giant embryo-rice. Food Chem. (2017) 231:258–66. doi: 10.1016/j.foodchem.2017.03.119
11. Rajagopalan VR, Manickam S, Muthurajan RA. Comparative metabolomic analysis reveals the nutritional and therapeutic potential of grains of the traditional rice variety mappillai samba. Plants. (2022) 11:543. doi: 10.3390/plants11040543
12. Ashokkumar K, Govindaraj M, Vellaikumar S, Shobhana VG, Karthikeyan A, Akilan M, et al. Comparative profiling of volatile compounds in popular south indian traditional and modern rice varieties by gas chromatography-mass spectrometry analysis. Front Nutr. (2020) 7:599119. doi: 10.3389/fnut.2020.599119
13. Ashokkumar K, Murugan M, Dhanya MK, Raj S, Kamaraj D. Phytochemical variations among four distinct varieties of Indian cardamom Elettaria cardamomum (L.) Maton. Nat Prod Res. (2020) 34:191922. doi: 10.1080/14786419.2018.1561687
14. Sukhonthrea S, Theerakulkait C, Miyazawa M. Characterization of volatile aroma compounds from red and black rice bran. J Oleo Sci. (2009) 58:155–61. doi: 10.5650/jos.58.155
15. Kotamreddy JNR, Hansda C, Mitra A. Semi-targeted metabolomic analysis provides the basis for enhanced antioxidant capacities in pigmented rice grains. J Food Measur Character. (2020) 14:1183–91. doi: 10.1007/s11694-019-00367-2
16. Goffman FD, Bergman CJ. Rice kernel phenolic content and its relationship with antiradical efficiency. J Sci Food Agric. (2004) 84:1235–40. doi: 10.1002/jsfa.1780
17. Hudson EA, Dinh PA, Kokubun T, Simmonds MS, Gescher A. Characterization of potentially chemopreventive phenols in extracts of brown rice that inhibit the growth of human breast and colon cancer cells. Cancer Epidemiol Prevent Cancer Epidemiol Prevent Biomark. (2000) 9:1163–70.
18. Ahmed F, Platel K, Vishwanatha S, Puttara S, Srinivasan K. Improved shelf-life of rice bran by domestic heat processing and assessment of its dietary consumption in experimental rats. J Sci Food Agric. (2007) 87:60. doi: 10.1002/jsfa.2670
19. Krishnanunni K, Senthilvel P, Ramaiah S, Anbarasu A. Study of chemical composition and volatile compounds along with in-vitro assay of antioxidant activity of two medicinal rice varieties: Karungkuravai and Mappilai samba. J Food Sci Technol. (2015) 52:2572–84. doi: 10.1007/s13197-014-1292-z
20. Pang Z, Chong J, Zhou G, de Lima Morais DA, Chang L, Barrette M, et al. MetaboAnalyst 5.0: narrowing the gap between raw spectra and functional insights. Nucleic acids research. (2021) 49, 388–396. doi: 10.1093/nar/gkab382
21. Bajpai VK, Sonwal S, Hwang SK, Shukla S, Khan I, Dey DK, et al. Sugiol, a diterpenoid: therapeutic actions and molecular pathways involved. Pharmacol Res. (2020) 163:105313. doi: 10.1016/j.phrs.2020.105313
22. Sundar S, Jha TK, Thakur CP, Sinha PK, Bhattacharya SK. Injectable paromomycin for visceral leishmaniasis in India. N Eng J Med. (2007) 356:2571–81. doi: 10.1056/NEJMoa066536
23. Kris-Etherton PM, Harris WS, Appel L. Fish consumption, fish oil, omega-3 fatty acids, and cardiovascular disease. Circulation. (2002) 106:2747–57. doi: 10.1161/01.CIR.0000038493.65177.94
24. Guarrera M, Turbino L, Rebora A. The anti-inflammatory activity of azulene. J Eur Acad Dermatol Venereol. (2001) 15:486–7. doi: 10.1046/j.1468-3083.2001.00340.x
25. Hinai EAA, Kullamethee P, Rowland IR, Swann J, Walton GE, Commane DM, et al. Modelling the role of microbial p-cresol in colorectal genotoxicity. Gut Microbes. (2019) 10:398–411. doi: 10.1080/19490976.2018.1534514
26. Fattore E, Fanelli R. Palm oil and palmitic acid: a review on cardiovascular effects and carcinogenicity. Int J Food Sci Nutr. (2013) 64:648–59. doi: 10.3109/09637486.2013.768213
27. Bergman AM, Kuiper CM, Myhren F, Sandvold ML, Hendriks HR, Peters GJ, et al. Antiproliferative activity and mechanism of action of fatty acid derivatives of arabinosylcytosine (ara-C) in leukemia and solid tumor cell lines. Nucleos Nucleot Nucl. (2004) 23:1523–6. doi: 10.1081/NCN-200027735
28. Priore P, Gnoni A, Natali F, Testini M, Gnoni GV, Siculella L, et al. Oleic acid and hydroxytyrosol inhibit cholesterol and fatty acid synthesis in C6 glioma cells. Oxid Med Cell Longev. (2017) 2017:9076052. doi: 10.1155/2017/9076052
29. Cho S, Choi CW, Lee DH, Won CH, Kim SM, Lee S, et al. High-dose squalene ingestion increases type I procollagen and decreases ultraviolet-induced DNA damage in human skin in vivo but is associated with transient adverse effects. Clin Exp Dermatol. (2009) 34:500–8. doi: 10.1111/j.1365-2230.2008.03133.x
30. Khan AA, Alanazi AM, Jabeen M, Chauhan A, Abdelhameed AS. Design, synthesis and in vitro anticancer evaluation of a stearic acid-based ester conjugate. Anticancer Res. (2013) 33:2517–24.
31. Varsha KK, Devendra L, Shilpa G, Priya S, Pandey A, Nampoothiri KM. 2, 4.–Di–tert–butyl phenol as the antifungal, antioxidant bioactive purified from a newly isolated Lactococcus sp. Int. J. Food Microbiol. (2015) 211:44–50. doi: 10.1016/j.ijfoodmicro.2015.06.025
32. Imran M, Ghorat F, Ul-Haq I, Ur-Rehman H, Aslam F, Heydari M, et al. Lycopene as a natural antioxidant used to prevent human health disorders. Antioxidants. (2020) 9:706. doi: 10.3390/antiox9080706
33. Sanclemente T, Marques-Lopes I, Fajo-Pascual M, Cofan M, Jarauta E, Ros E, et al. 2012. Naturally-occurring phytosterols in the usual diet influence cholesterol metabolism in healthy subjects. Nutr Metab Cardiovasc Dis. (2011) 22:849–55. doi: 10.1016/j.numecd.2011.01.010
34. Smith GI, Atherton P, Reeds DN, Mohammed BS, Rankin D, Rennie MJ, et al. Dietary omega-3 fatty acid supplementation increases the rate of muscle protein synthesis in older adults: a randomized controlled trial. Am J Clin Nutr. (2011) 93:402–12. doi: 10.3945/ajcn.110.005611
35. Ramakrishnan U, Stein AD, Parra-Cabrera S, Wang M, Imhoff-Kunsch B, Juarez-Marquez S, et al. Effects of docosahexaenoic acid supplementation during pregnancy on gestational age and size at birth: randomized, double-blind, placebo-controlled trial in Mexico. Food Nutr Bull. (2010) 31:108–16. doi: 10.1177/15648265100312S203
36. Abdelsalam A, Mahran E, Chowdhury K, Boroujerdi A, El-Bakry A. NMR-based metabolomic analysis of wild, greenhouse, and in vitro regenerated shoots of Cymbopogon schoenanthus subsp. proximus with GC–MS assessment of proximadiol. Physiol Mol Biol Plants. (2017) 23:369–83. doi: 10.1007/s12298-017-0432-0
37. Ikegami T, Matsuzaki Y. Ursodeoxycholic acid: mechanism of action and novel clinical applications. Hepatology Research. (2008) 38:123–31.
38. Okahara F, Suzuki J, Hashizume K, Osaki N, Shimotoyodome A. Triterpene alcohols and sterols from rice bran reduce postprandial hyperglycemia in rodents and humans. Mol Nutr Food Res. (2016) 60:1521–31. doi: 10.1002/mnfr.201500897
39. Zhou J, Chan L, Zhou S. Trigonelline: a plant alkaloid with therapeutic potential for diabetes and central nervous system disease. Curr Med Chem. (2012) 19:3523–31. doi: 10.1007/978-3-642-28917-0
40. Kim M, Sowndhararajan K, Choi HJ, Park SJ, Kim S. Olfactory stimulation effect of aldehydes, nonanal, and decanal on the human electroencephalographic activity, according to nostril variation. Biomedicines. (2019) 7:57. doi: 10.3390/biomedicines7030057
41. Zhong Z, Wheeler MD, Li X, Froh M, Schemmer P, Yin M, et al. L-Glycine: a novel antiinflammatory, immunomodulatory, and cytoprotective agent. Curr Opin Clin Nutr Metab Care. (2003) 6:229–40. doi: 10.1097/00075197-200303000-00013
42. Roopashree PG, Shetty SS, Kumari NS. Effect of medium chain fatty acid in human health and disease. J Funct Foods. (2021) 87:104724. doi: 10.1016/j.jff.2021.104724
43. Wang W, Li Y, Dang P, Zhao S, Lai D, Zhou L, et al. Rice secondary metabolites: structures, roles, biosynthesis, and metabolic regulation. Molecules. (2018) 23:98. doi: 10.3390/molecules23123098
44. Trimmer C, Arroyave R, Vuilleumier C, Wu L, Dumer A, DeLaura C, et al. Allosteric modulation of a human odorant receptor. Curr Biol. (2023) 33:1523–34. doi: 10.1016/j.cub.2023.03.016
45. Lozano-Baena MD, Tasset I, Obregón-Cano S, de Haro-Bailon A, Muñoz-Serrano A, Alonso-Moraga Á. Antigenotoxicity and tumor growing inhibition by leafy Brassica carinata and sinigrin. Molecules. (2015) 20:15748–65. doi: 10.3390/molecules200915748
46. Dawczynski C, Martin L, Wagner A, Jahreis G. n-3 LC-PUFA-enriched dairy products are able to reduce cardiovascular risk factors: a double-blind, cross-over study. Clin Nutr. (2010) 29:5929. doi: 10.1016/j.clnu.2010.02.008
47. Wu Y, Li R, Hildebrand D. Biosynthesis and metabolic engineering of palmitoleate production, an important contributor to human health and sustainable industry. Prog Lipid Res. (2012) 51:340–9. doi: 10.1016/j.plipres.2012.05.001
48. Wilson TA, Ausman LM, Lawton CW, Hegsted M, Nicolosi RJ. Comparative cholesterol lowering properties of vegetable oils: beyond fatty acids. J Am Coll Nutr. (2000) 19:601–7. doi: 10.1080/07315724.2000.10718957
49. Yoshida H, Tomiyama Y, Mizushina Y. Lipid components, fatty acids and triacylglycerol molecular species of black and red rices. Food Chem. (2010) 123:210–5. doi: 10.1016/j.foodchem.2010.04.010
50. Sulochana S, Meyyappan RM, Singaravadivel K. Phytochemical screening and GC-MS analysis of Garudan Samba traditional rice variety. Int J Environ Agri Res. (2016) 2:44–7.
51. Caceres AI, Liu B, Jabba SV, Achanta S, Morris JB, Jordt SE, et al. Transient receptor potential cation channel subfamily M member 8 channels mediate the anti-inflammatory effects of eucalyptol. Br J Pharmacol. (2017) 174:867–79. doi: 10.1111/bph.13760
52. Lozano-Grande MA, Gorinstein S, Espitia-Rangel E, Davila-Ortiz G, Martinez-Ayala AL. Plant sources, extraction methods, and uses of squalene. Int J Agron. (2018) 18:1–13. doi: 10.1155/2018/1829160
53. Kohno Y, Egawa Y, Itoh S, Nagaoka S, Takahashi M, Mukai K, et al. Kinetic study of quenching reaction of singlet oxygen and scavenging reaction of free radical by squalene in n-butanol. Biochim Biophys Acta. (1995) 1256:52–6. doi: 10.1016/0005-2760(95)00005-W
54. Burton GW, Joyce A, Ingold KU. Is vitamin E the only lipid-soluble, chain-breaking antioxidant in human blood plasma and erythrocyte membranes? Arch. Biochem Biophys. (1983) 221:281–90. doi: 10.1016/0003-9861(83)90145-5
55. Szczeklik A, Gryglewski RJ, Domagala B, Dworski R, Basista M. Dietary supplementation with vitamin E in hyperlipoproteinemias: effects on plasma lipid peroxides, antioxidant activity, prostacyclin generation and platelet aggregability. Thromb Haemost. (1985) 54:425–30. doi: 10.1055/s-0038-1657865
56. Sesso WG, Kurth JC, Belanger T, MacFadyen C, Bubes J, Manson V, et al. Vitamins E and C in the prevention of cardiovascular disease in men, the physicians' health study ii randomized controlled trial. JAMA. (2008) 300:2123–33. doi: 10.1001/jama.2008.600
57. Maire A, Germain P, Bourguet W. Protein-protein interactions in the regulation of RAR–RXR heterodimers transcriptional activity. In: ed Ehmke P, Methods in Enzymology, Academic Press. (2020) 637:175–207. doi: 10.1016/bs.mie.2020.02.007
58. Gonzalez MA. Aromatic abietane diterpenoids: total syntheses and synthetic studies. Tetrahedron. (2015) 71:1883–908. doi: 10.1016/j.tet.2015.01.058
59. Srinivasan S, Torres AG, De Ribas, Pouplana L. Inosine in biology and disease. Genes. (2021) 12:600. doi: 10.3390/genes12040600
60. Dehpour AA, Babakhani B, Khazaei S, Asadi M. Chemical composition of essential oil and antibacterial activity of extracts from flower of Allium atroviolaceum. J Med Plants Res. (2011) 5:3667–72.
61. Yogeswari S, Ramalakshmi S, Neelavathy R, Muthumary J. Identification and comparative studies of different volatile fractions from monochaetia kansensis by GCMS. Glob J Pharmacol. (2012) 6:65–71.
62. Wang TT, Nestel FP, Bourdeau V, Nagai Y, Wang Q, Liao J, et al. Cutting edge: 1,25-dihydroxyvitamin D3 is a direct inducer of antimicrobial peptide gene expression. J Immunol. (2004) 173:2909–12. doi: 10.4049/jimmunol.173.10.6490-c
63. Gupta R, Sharma AK, Dobhal M, Sharma M, Gupta R. Antidiabetic and antioxidant potential of β-sitosterol in streptozotocin-induced experimental hyperglycemia. J Diabetes. (2011) 3:29–37. doi: 10.1111/j.1753-0407.2010.00107.x
64. Hemamalini S, Umamaheswari DS, Lavanya DR, Umamaheswara RDC. Exploring the therapeutic potential and nutritional properties of ‘Karuppu Kavuni' variety rice of Tamil Nadu. Int J Pharma Bio Sci. (2018) 9:88–96. doi: 10.22376/ijpbs.2018.9.1.p88-96
65. Monlezun DJ, Bittner EA, Christopher KB, Camargo CA, Quraishi SA. Vitamin D status and acute respiratory infection: cross sectional results from the United States national health and nutrition examination survey, 2001–2006. Nutrients. (2015) 7:1933–44. doi: 10.3390/nu7031933
66. Kosciuczuk EM, Lisowski P, Jarczak J, Strzałkowska N, Jozwik A, Horbanczuk J, et al. Cathelicidins: Family of antimicrobial peptides. A review Mol Biol Rep. (2012) 39:10957–70. doi: 10.1007/s11033-012-1997-x
67. Pinzon RT, Angela A, Pradana AW. Vitamin D Deficiency among patients with COVID-19: case series and recent literature review. Res Sq. (2020) 3:277. doi: 10.1186/s41182-020-00277-w
68. Peehl DM, Krishnan AV, Feldman D. Pathways mediating the growth-inhibitory actions of vitamin D in prostate cancer. J Nutr. (2003) 133:2461S–9S. doi: 10.1093/jn/133.7.2461S
69. Heubi JE, Setchell DR, Jha P, Buckley D, Zhang W, Rosenthal P, et al. Treatment of bile acid amidation defects with glycocholic acid. Hepatology. (2015) 61:268–74. doi: 10.1002/hep.27401
70. Melchior CL, Ritzmann RF. Pregnenolone and pregnenolone sulfate alone and with ethanol, in mice on plus-maze. Pharmacol Biochem Behav. (1994) 48:893–7. doi: 10.1016/0091-3057(94)90197-X
71. Zhang L, Li Y, Liang Y, Liang K, Zhang F, Xu T, et al. Determination of phenolic acid profiles by HPLC-MS in vegetables commonly consumed in China. Food Chem. (2019) 276:538–46. doi: 10.1016/j.foodchem.2018.10.074
72. Thomas E, Ghany MG, Liang TJ. The application and mechanism of action of ribavirin in therapy of hepatitis C. Antivir Chem Chemother. (2012) 23:1–12. doi: 10.3851/IMP2125
73. Töpfer N, Kleessen S, Nikoloski Z. Integration of metabolomics data into metabolic networks. Front Plant Sci. (2015) 6:49. doi: 10.3389/fpls.2015.00049
74. Karlund A, Hanhineva K, Lehtonen M, Karjalainen RO, Sandell M. Nontargeted metabolite profiles and sensory properties of strawberry cultivars grown both organically and conventionally. J Agric Food Chem. (2015) 63:1010–9. doi: 10.1021/jf505183j
75. Xiao R, Maa Y, Zhangb D, Qianb L. Discrimination of conventional and organic rice using untargeted LC-MS based metabolomics. J Cereal Sci. (2018) 2:73–81. doi: 10.1016/j.jcs.2018.05.012
76. Daygon VD, Prakash S, Calingacion M, Riedel A, Ovenden B, Snell P, et al. Understanding the Jasmine phenotype of rice through metabolite profiling and sensory evaluation. Metabolomics. (2016) 12:1–15. doi: 10.1007/s11306-016-0989-6
77. Adom KK, Liu RH. Antioxidant activity of grains. J Agric Food Chem. (2002) 50:6182–7. doi: 10.1021/jf0205099
78. Shen Y, Jin L, Xiao P, Lu Y, Bao JS. Total phenolics, flavonoids, antioxidant capacity in rice grain and their relations to grain color, size and weight. J Cereal Sci. (2009) 49:106–11. doi: 10.1016/j.jcs.2008.07.010
79. Dehpour AA, Yousefian M, Jafary Kelarijani SA, Koshmoo M, Mirzanegad S, Mahdavi V, et al. Antibacterial activity and composition of essential oils of flower Allium rotundum. Adv Environ Biol. (2012) 6:1020.
80. Dharni S, Maurya A, Samad A, Srivastava SK, Sharma A, Patra DD, et al. Purification, characterization, and in vitro activity of 2,4-Di-tert-butylphenol from Pseudomonas monteilii PsF84: conformational and molecular docking studies. J Agric Food Chem. (2014) 62:6138–46. doi: 10.1021/jf5001138
81. Song YW, Lim Y, Cho SK. 2, 4.-Di-tert-butylphenol, a potential HDAC6 inhibitor, induces senescence and mitotic catastrophe in human gastric adenocarcinoma AGS cells. 2018. Biochim Biophysica Acta. (2018) 1865:675–83. doi: 10.1016/j.bbamcr.2018.02.003
Keywords: grain metabolome, traditional rice varieties, gas chromatography-mass spectrometry, univariate and multivariate analysis, hierarchical clustering
Citation: Udhaya Nandhini D, Venkatesan S, Senthilraja K, Janaki P, Prabha B, Sangamithra S, Vaishnavi SJ, Meena S, Balakrishnan N, Raveendran M, Geethalakshmi V and Somasundaram E (2023) Metabolomic analysis for disclosing nutritional and therapeutic prospective of traditional rice cultivars of Cauvery deltaic region, India. Front. Nutr. 10:1254624. doi: 10.3389/fnut.2023.1254624
Received: 07 July 2023; Accepted: 01 September 2023;
Published: 28 September 2023.
Edited by:
Lei Chen, Guangdong Ocean University, ChinaReviewed by:
Somnath Mandal, Uttar Banga Krishi Viswavidyalaya, IndiaCopyright © 2023 Udhaya Nandhini, Venkatesan, Senthilraja, Janaki, Prabha, Sangamithra, Vaishnavi, Meena, Balakrishnan, Raveendran, Geethalakshmi and Somasundaram. This is an open-access article distributed under the terms of the Creative Commons Attribution License (CC BY). The use, distribution or reproduction in other forums is permitted, provided the original author(s) and the copyright owner(s) are credited and that the original publication in this journal is cited, in accordance with accepted academic practice. No use, distribution or reproduction is permitted which does not comply with these terms.
*Correspondence: Eagan Somasundaram, ZWFnYW5zb211QHJlZGlmZm1haWwuY29t; Vellingiri Geethalakshmi, Z2VldGhhQHRuYXUuYWMuaW4=
Disclaimer: All claims expressed in this article are solely those of the authors and do not necessarily represent those of their affiliated organizations, or those of the publisher, the editors and the reviewers. Any product that may be evaluated in this article or claim that may be made by its manufacturer is not guaranteed or endorsed by the publisher.
Research integrity at Frontiers
Learn more about the work of our research integrity team to safeguard the quality of each article we publish.