- 1College of Chemistry, Zhengzhou University, Zhengzhou, Henan, China
- 2Food Laboratory of Zhongyuan, Zhengzhou University, Zhengzhou, Henan, China
- 3School of Ecology and Environment, Zhengzhou University, Zhengzhou, Henan, China
- 4Center of Advanced Analysis and Gene Sequencing, Zhengzhou University, Zhengzhou, Henan, China
Food safety is a widespread global concern with the emergence of foodborne diseases. Thus, establishing accurate and sensitive detection methods of harmful contaminants in different food matrices is essential to address and prevent the associated health risks. Among various analytical tools, mass spectrometry (MS) can quantify multiple impurities simultaneously due to high resolution and accuracy and can achieve non-target profiling of unknown pollutants in food. Therefore, MS has been widely used for determination of hazardous contaminants [e.g., mycotoxin, pesticide and veterinary drug residues, polychlorinated biphenyls (PCBs), dioxins, acrylamide, perfluorinated compounds (PFCs) and p-Phenylenediamine compounds (PPDs) in food samples]. This work summarizes MS applications in detecting harmful contaminants in food matrices, discusses advantages of MS for food safety study, and provides a perspective on future directions of MS development in food research. With the persistent occurrence of novel contaminants, MS will play a more and more critical role in food analysis.
Introduction
Over the past few years, food safety has become a growing global concern. The food safety analysis included the daily presence of various contaminants and residues, such as plant protection products, veterinary medicines, mycotoxins, chemical impurities, additives, packaging materials, and impurities added during processing packaging materials (1). PFCs, polybrominated diphenyl ethers (PBDEs), dioxins, mycotoxins, and PCBs, are common food contaminants that pose risks to human health. Therefore, identifying these contaminants is critical. Maximum residue limits (MRLs) have been stipulated in many countries to limit the use of contaminants in food to minimize public health concerns (2). Generally, the quantification of unknown pollutants in food has been determined by conventional methods, such as gas chromatography (GC) and high-performance liquid chromatography (HPLC). However, these approaches have several limitations in determining multiple residues simultaneously. More effective and powerful tools are needed due to the complexity of food samples.
MS has been widely applied in protein identification, biological analysis, and food analysis. It can be used in different scanning modes, including Full Scan, Daughter Ion, Parent Ion, Neutral Loss, and multiple reaction monitoring (MRM). Moreover, volatile and non-volatile components, high and low ionic components, high and low melting point substances, and their combinations without ionizable groups are analyzed by MS-based techniques (2). MS-based detectors offer excellent recovery, selection, sensitivity, reproducibility, and minimum interference. MS is widely used to analyze diverse food residues, such as vegetables (3), fruits (4), meat (5). MS combines powerful chromatographic separation to identify and confirm the presence of target compounds. It is a futuristic approach with a wide range of applications like, identifying separated compounds, elucidating the structure of compounds, and investigating degradation pathways.
Especially high resolution mass spectrometry (HRMS), it offers the advantage of higher sensitivity and full scanning. It provides a better understanding of sample composition than LC–MS/MS. HRMS has been described as a modern tool to test drug residues. Large-scale analytical screening methods have been published for veterinary medicines analysis in different food and animal matrices (6). HRMS is the most promising tool for the development of non-targeted methods. Besides, due to its excellent sensitivity and specificity, GC–MS has evolved as an important technique in recent years for food contaminants and is widely used for low molecular weight compound identification (7). According to the survey, LC–MS/MS is known for its ability to develop a wide range of residues and a large number of methods. It is considered the most popular and well-established analytical technique, but has shortcomings in some aspects such as limited number of analytes, inability to screen for unknowns and time-consuming assay setup.
The purpose of this paper is to [1] highlight the advantages of MS in detecting hazardous contaminants in food, [2] summarize recent advances in MS methods for mycotoxins, pesticide and veterinary drug residues, acrylamide, food additives, PCBs, dioxins, PFCs, and PPDs analysis over the past 10 years, and [3] overview the future applications of MS methods in food research.
Mycotoxins
Mycotoxins, a common food contaminant, are poisonous secondary metabolites produced by fungi, such as Aspergillus, Penicillium, and Fusarium. Their presence in hot processed foods is a worldwide concern. These naturally occurring compounds are very toxic to humans and enter the human body via the food web, causing hepatotoxicity, genotoxicity, immunosuppression, nephrotoxicity, teratogenicity, and carcinogenicity (8). Mycotoxins are found in many agricultural products, such as grains and nuts. Therefore, considerable attention has been paid to mycotoxins analysis. Table 1 summarizes the use of MS in analyzing natural mycotoxin in foods.
For more accurate quantification of mycotoxins, LC–MS is now the most widely used technique for the detection of mycotoxins in food (17), especially for analyzing grain and grain products (10). Similarly, GC–MS also plays a role in mycotoxin screening and quantitative analysis (18). Singh et al. (19) summarized the mycotoxin analysis using traditional (HPLC) and advanced methods (LC–MS and GC–MS) and analyzed mycotoxins characteristics. The result showed that LC–MS and GC–MS have better sensitivity than conventional methods. Another work discussed mycotoxins in cereal products and summarized the most commonly used detection techniques, including LC-electron spray ionization (ESI)-MS. Sample pre-treatment was carried out using the minimal clean-up method, QuEChERS, which is fast, simple, inexpensive, effective, durable and safe. In this work, the ranking of cereal products according to the number of trials was cereals > cornflakes > bread > breakfast > flour > baby products > pasta > other products, suggesting that research on mycotoxins in cereal products had attracted much attention (20).
Studies have reported that 120 food matrices have been analyzed for mycotoxins using LC–MS/MS technique (11). Alsharif et al. (11) used LC–MS/MS to determine mycotoxins in raisins, pistachios, peanuts, wheat flour, spices, and pepper samples simultaneously. Sample pretreatment was performed by the QuEChERS technique, with recoveries ranging from 81.94 to 101.67%. Similarly, Lee et al. (13) used LC–MS/MS and stable isotope dilution to analyze multiple mycotoxins in ready-to-eat foods, yielding good results. Mycotoxins are also present in bee products,which can boost immunity and are important diets for adults and children. However, the health effects of mycotoxins-contaminated bee products in healthy individuals are unknown. Keskin et al. (12) performed HPLC-UV analysis of mycotoxins, followed by LC–MS/MS, to detect the positive samples and improve sensitivity. It was found that mycotoxins consumed from certain amounts of bee products do not pose a health risk. Beans are highly nutritious foods, and their consumption is becoming increasingly popular. However, mycotoxins are found in beans. A work by Acuña-Gutiérrez et al. (21) summarized the mycotoxins detection techniques in beans, including GC–MS/MS and HPLC–MS/MS. Compared to GC–MS/MS and HPLC–MS/MS, HRMS is also used for the detection of mycotoxins with higher sensitivity and efficiency. Rodríguez-Carrasco et al. (15) first used acetonitrile-based extraction and ultra-high-performance liquid chromatography coupled with quadrupole exactive orbitrap high resolution mass spectrometry (UHPLC-Q-Exactive-Orbitrap HRMS) to analyze mycotoxins in soy burgers with recoveries in the range of 78 to 108% and precision less than 12%. The limits of quantitation (LOQs) of all investigated compounds were in the low ng/g range, and the co-existence of mycotoxins was observed in approximately all samples. Similarly, a technique to detect 19 mycotoxins in grain flour matrices using real-time orbitrap mass spectrometry (DART-Orbitrap MS) was developed and validated by Tsagkaris et al. (16), based on a LC method combined with both a hybrid HRMS (q-Orbitrap MS) and a low-level resolution mass spectrometry (triple quadrupole, QqQ) detectors. Compared to the liquid–liquid method, the DART-Orbitrap MS exhibited high throughput, speed and excellent detection capability, monitoring up to 96 samples in a single runtime of approximately 40 s, which is crucial for the regulation of mycotoxins. The emerging DART-Orbitrap MS can improve and accelerate the detection of selected mycotoxins in cereal samples compared to LC–MS, and the superiority of this technique makes it more promising for future applications in the detection of mycotoxins in a wide range of foods. For mycotoxin analysis in fatty matrices such as nuts, Castilla-Fernández et al. (9) isolated mycotoxins from walnuts by solid–liquid extraction (SLE) and then analyzed them using UHPLC–MS/MS. Due to the strong matrix effect in fatty matrices, the dilution-injection method was the newest trend and solved the problem of the matrix effect. This method has the advantages of simplicity, minimal loss of analytes, high sample flow rate, and many analytical classes covered. Six purification columns were used to purify fatty matrices, none of which effectively reduced the strong matrix effects encountered by SLE. Finally, weak or negligible matrix effects were obtained by applying 1:100 dilutions of SLE extracts. LOQs met The European Union (EU) requirements, and satisfactory recovery and precision were obtained. To reduce the effects of matrix effects, Lago et al. (14) first evaluated a liquid chromatography coupled with quadrupole time-of-flight mass spectrometry (LC-QTOF-MS) for concurrently analyzing legal and newly discovered mycotoxins in malt and beer. The method overcame matrix effects and is rapid, requiring only 1.2 min for simultaneous analysis of emerging mycotoxins. The MS was applied to detect mycotoxins using MRM and total mode, with satisfactory linearity and recoveries. In summary, the detection of contaminants in food samples with complex matrices and difficult sample processing has been a challenging task. The development of LC-QTOF-MS with low matrix effects and high speed has to accelerated the study of food contaminants.
Pesticides residues
Pesticides can enter the food chain during their application. Food is common exposure to pesticide at low doses. Surprisingly, some substances become more potent when they accumulate in the food chain. When the concentration reaches a critical level, damage to human health becomes more serious (22). Pesticides mainly have higher risks to the nervous system (23). Also, multiple sclerosis, cancer, and several chronic diseases have been linked to long-term pesticide exposure. Table 2 summarizes how MS analyzes residues in pesticides residues.
Pesticides can protect crops and avoid pests and diseases. However, there are many abuse instances of pesticides due to their widespread use, leading to serious pesticide residues in vegetables, fruits, water (24), and soil (25). Due to their wide variety, analyzing pesticides in food is daunting since the matrix concentration in foodstuffs is higher than that of pesticides. Pesticide contamination also occurs and is increasing in food of livestock origin, bringing comprehensive multi-residual analysis of plant protection products to the global attention forefront. EU has now set MRLs for various pesticides in different foods to monitor risks to people’s health and the natural environment (26). MS is highly sensitive and can unambiguously detect trace amounts of samples, and it is now becoming increasingly common for detecting pesticide residues, particularly in food (27). Different MS-based techniques have been frequently used to detect food contaminants, such as HPLC-MS, GC–MS, ultra performance liquid chromatography–tandem mass spectrometry (UPLC-MS/MS) and GC–MS/MS (28), UHPLC–MS/MS, UHPLC-QTOF-MS, DART-MS (29), Desorption electrospray ionization mass spectrometry (DESI-MS).
QuEChERS is frequently used for sample pretreatment with the advantage of quick, easy, affordable, efficient, reliable, and secure. Liu et al. (30) reported the improved QuEChERS extraction technology for sample preparation. Acetylene was used as the extraction agent, and SAX, XFM42, and C18 as the stationary phase. The proposed HPLC-MS/MS was used to identify and quantify fipronilide in food samples and the LOQs range was 0.5 to 50 μg/kg. Han et al. (31) pretreated food samples using a modified QuEChERS method, followed by UPLC-MS/MS with simultaneous MRM concerning quantification via a standard outer method. The mean recoveries of the developed were 75.6–106.2%, with an RSD of ≤8.8%. Kottadiyil et al. (32) adapted the QuEChERS method to detect and quantify pesticide residues in vegetables and fruits by GC–MS/MS and UHPLC-QTOF-MS and used MRM mode to quantify samples. This model exhibited high specificity, selectivity, and sensitivity in SCAN and SIM (single ion monitoring) modes. Goon et al. (33) employed a new method to screen non-targeted pesticides multi-residues in spice matrices by UHPLC-Q-Orbitrap MS. Samples were extracted using the standard QuEChERS method. Compared with LC–MS/MS, recoveries obtained by the UHPLC-Q-Orbitrap MS method were in the 78–100% range with successive full instrument scans and stable quantitative performance. In addition, HLB purified samples, and the recoveries ranged from 70 to 120% with an accuracy RSD of <20%. Chen et al. (34) used a developed derivatization-based QuEChERS approach for online HPLC–MS/MS detection of fungicide pesticide residues in vegetable-based food products (Figure 1). Good performance can be obtained in the range of 0.001–1 mg/L under the negative ionization scan mode. Dispersive solid-phase extraction (DSPE) is a new sample pre-treatment technique that is easy, fast, efficient, accurate and sensitive. Shin et al. (35) used d-SPE for sample pre-processing and cleaning by octadecylsilane (C18) and primary secondary amine (PSA) as d-SPE absorbents. Multiple agrochemicals in fish and shrimps were analyzed simultaneously by LC-MS/MS with good recoveries (70–125%). Similarly, Prata et al. (36) used QuEChERS extraction and d-SPE purification method to monitor pesticides and aflatoxins in infant food. Quantifying multiple contaminants and aflatoxin levels in infant formula sold in Brazil by UHPLC-Q-Orbitrap MS technique achieved low LODs and LOQs.

Figure 1. Improved derivatization-QuEChERS method for HPLC–MS/MS analysis of fungicide residues in foods of plant origin (34).
Multi-walled carbon nanotubes are often modified as substrates for the extraction and adsorption of contaminants. Synthetic magnetic amino-modified multi-walled carbon nanotubes (m-MWCNTs-NH2) were used for sample purification and enrichment of pesticides in tea samples (37). They used UHPLC–MS/MS and ultra-sound-based magnetic solid-phase extraction (UA-MSPE) to simultaneously determine medomel, diquat, and oxytetracycline in tea samples. UA-MSPE provided a simple, rapid, and efficient analysis for targeted samples with promising LODs and LOQs. In addition, three plant protection products were detected in different tea samples with a recovery higher than 75.1%, suggesting that the method can be used in assessing plant protection products in other matrices. Magnetic multi-walled carbon nanotubes (Fe3O4MWCNTs) were used as sorbents to detect mycotoxins and pesticides in cereals by QuEChERS extraction.
Pesticide residues are also present in Chili peppers and Sichuan peppercorns, which are consumed globally for their unique flavour and high nutritional value. In addition, the converted product is in high demand as an essential spice. However, to get high yields, unscrupulous traders use illegal pesticides. Studies have shown that LC–MS/MS methods can be applied to analyze spices’ pesticide residues; however, only target compounds can be identified. Using these methods for non-targeted screening is impossible (38). Liu et al. (39) established a combined LC-Q-TOF-MS-based technique that analyzed all relevant pesticides in peppers and chilies with LOQ ≤ 5 μg kg-1. Song et al. (38) developed a novel, single-step technique with quick and easy, requiring no further vortexing or washing steps. A small column was placed in an extraction spinner to remove unwanted material. The absorbents were MWCNT and PSA blended with salts. 47 typical pesticides in pepper, chili, and chili sauce were detected by LC–MS/MS and GC–MS/MS, with satisfactory recoveries. GC–MS/MS was used for volatiles and lipophilic compounds, while LC–MS/MS analyzed pesticides containing polar moieties which are not heat resistant and non-volatile. To improve the accuracy of the analysis of various pesticide residues, an analytical approach using both LC–MS/MS and GC–MS/MS was reported by Lee et al. (40) The data indicated that recoveries were between 70 and 120%, and cross-checking was more effective and reliable than a single method in identifying several pesticides. The LODs for LC–MS/MS were 0.03 to 0.5 μg/kg, and LOQs were 0.6 to 1.5 μg/kg. For GC–MS/MS, the LODs and LOQs were 0.9 to 2.0 μg/kg and 3.0 to 5.7 μg/kg, respectively. Harischandra et al. (41) developed the concurrent measurements for 79 different pesticide residues in wood beans using LC–MS/MS and GC–MS/MS with recoveries ranging from 70 to 120%. According to MRLs for wood beans, satisfactory precision (RSD < 20%) was achieved for detecting and quantifying pesticide residues under 10 μg/kg. Similarly, Mozzaquatro et al. (42) determined 80 pesticides, including 5 metabolites in passion fruit by LC–MS/MS and GC–MS/MS in a cross-synchronous analysis, allowing a more comprehensive and detailed sample determination. Therefore, the scattered omnidirectional solvent extraction method was adopted, and the results showed that the sample recovery was more than 70%; RSD ≤ 20%.
Ambient ionisation mass spectrometry (AMS) is a state-of-the-art technique that allows rapid chemical analysis without the need for sample preparation and chromatographic separation. It is now being used to detect food contaminants, particularly in the detection of pesticide residues on fruits and vegetables. Cheng et al. (43) developed a combination of multi-probe samples, TD-ESI, and composite MS/MS analysis methods to determine many trace pollutants in fruits and vegetables. The method involves desorption and ionization of samples collected on the probe in a TD-ESI source, followed by QqQ-MS detection in MRM mode. Samples collected by QuEChERS extraction combined with GC–MS/MS or LC–MS/MS showed similar results to those determined by TD-ESI-MS/MS, which is a simple and time-saving technique that can simultaneously monitor multiple MRM channels. Therefore, QuEChERS coupled with TD-ESI-MS/MS exhibits significant potential to quantify pesticide residues in agricultural products. Pesticide residues in food can cause human health risks, especially for infants and children. An ultrasonic-vortex-assisted liquid–liquid microextraction method coupled with gas chromatography-ion trap mass spectrometry (UVA LLME-GC-IT/MS) was proposed by Notardonato et al. (23). The method was quick, simple, inexpensive, and accurate for 19 organophosphorus pesticides (OPs) determination in baby food. The LODs and LOQs were in the 0.2–1.3 and 0.5–2.9 ng/g range, respectively. Based on the developed LLME extraction with subsequent GC-IT/MS analysis, the recovery was in the 81 to 109% range.
Veterinary drug residues
Veterinary medicines can prevent, diagnose or treat animal diseases. Due to increased human demand for meat products, many people add veterinary drugs to livestock and aquaculture processes to achieve high yields. However, the overuse of veterinary medicines can contaminate the aquatic environment and affect the safety of drinking water. More importantly, some people use illegal veterinary medicines for their own benefit, which can pose a serious threat to human health and cause greater pollution of the environment. Therefore, the detection and quantification of these drugs in animal tissues cannot be ignored in terms of food safety, human health and the environment. Current techniques to detect veterinary drug residues in animal-origin food are GC–MS/MS, capillary electrophoresis-mass spectrometry (CE-MS/MS) (44), LC–MS/MS (45), hydrophilic interaction liquid chromatography–tandem mass spectrometry (HILIC-MS/MS), reversed-phase liquid chromatography coupled with tandem mass spectrometry (RPLC-MS/MS) (46), QTOF-MS (47), Quadrupole Mass Spectrometry (QMS), UHPLC–MS/MS (48) and HPLC-Q-Orbitrap HRMS. In this review, we summarized the application of MS in the analysis of veterinary drug residues, as shown in Table 3.
Beef and dairy products are rich in nutrients and widely consumed by the public, but the residues of veterinary drugs in these should not be ignored. Jung et al. (45) used LC–MS/MS to identify and quantify 115 veterinary drugs and their metabolites in beef. The QuEChERS technique was developed by dispersive solid-phase extraction using EMR as a sorbent. Under the positive ion mode, only three veterinary drugs could not achieve the required recoveries, while the recoveries of the remaining 112 were 70.7 to 117.9%. Melekhin et al. (49) developed an HPLC-MS/MS-based method to determine multiple animal drug residues in milk quantitatively. Magnetic supercross-linked polystyrene (HCP/Fe3O4) was used to develop a MSPE without a deproteinization step. As a new form of SPE, MSPE can eliminate the limitations of traditional SPE, such as backpressure fouling, complex filtration, and solvent waste. In addition, MSPE has simple and rapid sample preparation, solvent savings, low cost, and high recoveries of analytes. This has been reported as the first and most complete MSPE method for multi-residue analysis of animal drugs in milk. Zhao et al. (50) used UHPLC–MS/MS to analyze approximately 150 veterinary drugs in baby formula milk and associated constituents. The method achieved a LOD of 1-10 ng/g in baby food, whole milk powder, and isolated milk proteins.
HRMS is now widely used in the field of detecting drug residues in food. LC-HRMS was used to extend the detection of veterinary drug residues in foodstuffs. The LC-HRMS-based technique applies to screening raw materials of animal origin (53). TOF/QTOF and Orbitrap are often combined with HRMS, TOF analyzers are particularly popular in analyzing drug residues. HPLC-QTOF-MS exhibits high resolution and mass accuracy, and its full scan acquisition offers large amounts of target and non-target identification data. Hou et al. (52) developed an HPLC-QTOF-MS multi-class screening approach for measuring veterinary medicines and pesticides in eggs. A one-time injection procedure was developed for the qualitative analysis. Similarly, veterinary drug and pharmaceuticals residue analysis in fish tissues and milk using UHPLC-QTOF-MS was reported by Dasenaki et al. (47) Detecting veterinary medicinal residues in beef and chicken samples is complicated due to their complexity. Wang et al. (56). used QuEChERS pretreatment and HPLC-Q-Orbitrap HRMS for quantitative screening. The obtained recoveries were 52.1 to 138.2%, and the RSDs were 0.4 to 17.7%. In addition, the LODs were 0.15–3.03 g/kg, and LOQs were 0.5–10 g/kg. The method improved the accuracy and range of screening in full mode. With the demand for faster and more accurate detection of food contaminants, this will also drive the development of HRMS in combination with more chromatographic techniques. However, scanning rates need to be improved and user-friendly data-processing software needs to be developed as the most promising analytical technique.
A high throughput SPE method was developed based on Oasis PRiME HLB. Wang et al. (51) used UHPLC-QTRAP for simple and efficient quantitative analysis of 155 veterinary drugs in cattle and chicken feeds in MRM-IDA-EPI positive and negative scan modes. The obtained LOQs were in the range of 0.5 μg/kg and 5 μg/kg, and LODs were between 2 μg/kg and 20 μg/kg. In addition, satisfactory recoveries were obtained for more than 17 of 20 analytes. Zhao et al. (54) used a UPLC-Q-Exactive-Orbitrap MS system to screen and analyze multiple foodstuff veterinary residues. The method was rapid, simple, and sensitive and consisted of two steps; extraction with 0.2% formic acid-acetonitrile water and purification with PRiME HLB SPE columns. The analyses were performed in positive and negative MS1/MS2 full scan modes, with targeted identification by full scan MS. Obtained detection limits were 0.1 to 10 μg/kg, and recovery was 79.2 and 118.5% in all matrices.
A 15-level multi-source method to screen hydrophilic and hydrophobic veterinary drugs in milk, eggs, and meat was developed by Chung et al. (46) Using HILIC-MS and HILIC/RPLC. The method typically achieved 70 to 120% recoveries with an RSD accuracy of <20%. Due to the limited screening capacity of current veterinary drugs, trace analysis is always time-consuming and expensive. Mehl et al. (55) first developed an automated HTpSPE-UV/vis/FLD-HPLC-HRMS/MS to screen 81 veterinary drug residues in honey, pig muscle, milk, and eggs (Figure 2). The developed method is green, fast, and solvent-saving compared to conventional methods. Based on automatic elution using an auto TLC-LC–MS interface, online HPLC separation, and detection by Orbitrap HRMS, most veterinary drugs were measured in honey at a 25 μg/kg concentration. The other three samples were found at a 5 μg/kg concentration.
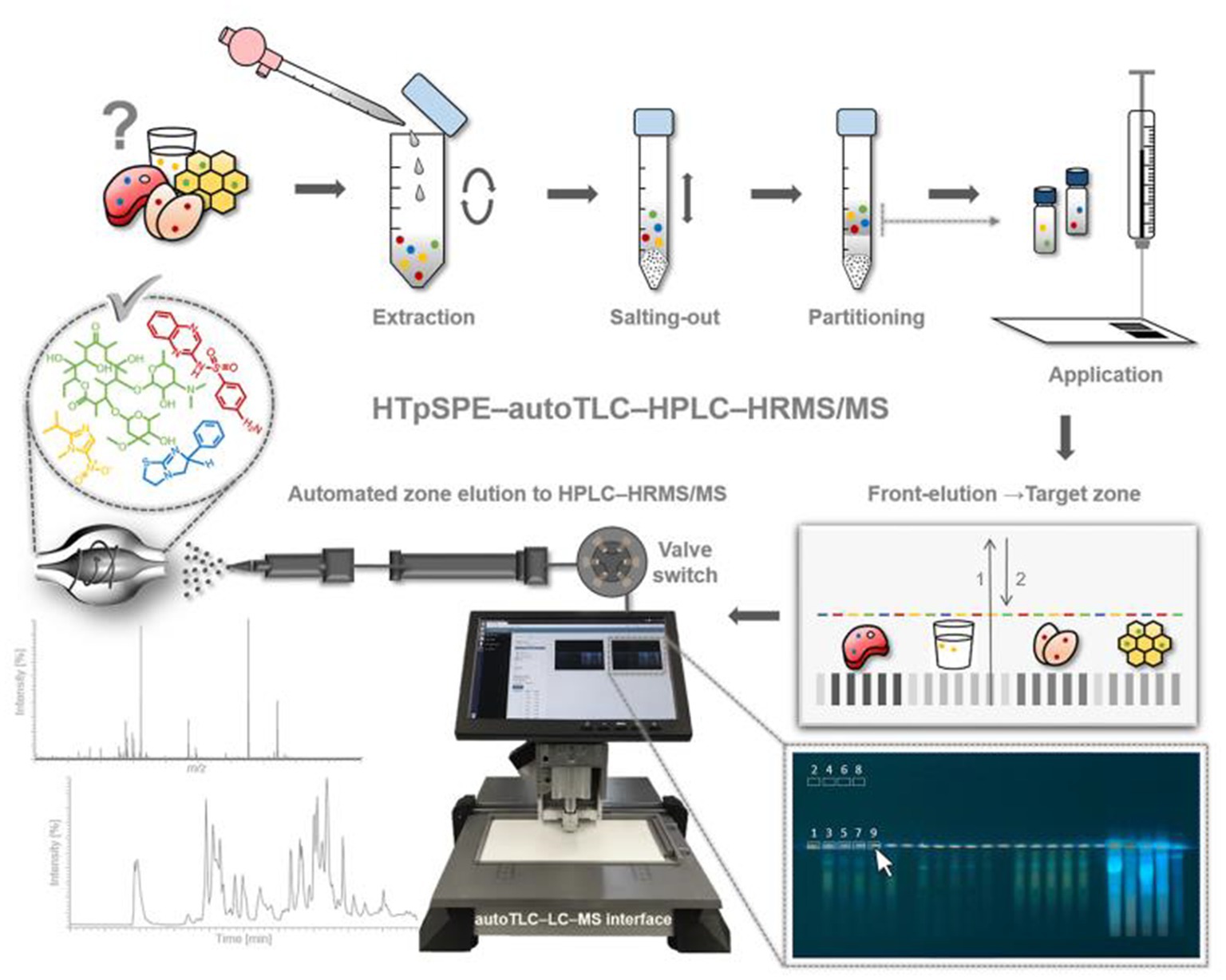
Figure 2. Workflow of the developed HTpSPE-UV/vis/FLD-autoTLC-LC-HRMS/MS method (55).
Antibiotics are commonly used veterinary drugs. Currently, residues in meat products are one of the most severe food contamination problems, presenting a health risk to the public. Chloramphenicol (CAP), as an antibiotic, is used to treat diseases in honey bee larvae. However, CAP has high toxicity and less dose dependence in sensitive individuals. Therefore, it is banned in edible animals and honey bees. Kikuchi et al. (57) used LC–MS/MS to determine CAP and CAPG residues in animal products, fish and crustaceans, honey, and royal jelly. They used mixed hydrophilic–lipophilic copolymer for methanol extraction, beta-glucuronidase hydrolysis, and solvent exchange column purification. The method was simple and accurate, depicting excellent CAP and CAPG recoveries (79–109%). Recently, Zhou et al. (58) used LC–MS/MS to determine milk antibiotics (vancomycin and desmethyl vancomycin). They developed a surface molecularly imprinted solid phase extraction (SMISPE) based on a highly selective method. Sample handling and material effects were greatly reduced for targeting analytes, with recoveries in the range of 83.3–92.1%. Aminoglycosides (AGs) are antibiotics commonly found in milk and eggs, posing health risks to humans, including long-term exposure causing multiple allergic reactions. CE-ESI-MS/MS method was adopted by Yue et al. (44) to identify 4 AGs in milk simultaneously. CE-ESI-MS/MS combined with SPE and t-ITP showed recoveries above 76.20% for all AGs. Separation conditions were simpler than LC–MS, without ion-pairing reagents in the mobile phase.
In summary, LC–MS is a satisfactory technique for detecting pesticide and veterinary residues in food. Compared with other MS methods, LC–MS has the advantages of high sensitivity and selectivity, wide linear range and the ability to simultaneously analyze multiple pesticide and veterinary drug residues in food. This makes LC–MS become an important analytical tool in food safety monitoring and quality control. However, the high cost of LC–MS, insufficient resolving power, strong matrix effects and sometimes false-positive results pose challenges for the detection of pesticide and veterinary drug residues in food. Therefore, development of more superior MS detection methods is still needed.
Acrylamide
Acrylamide is a regulated compound. It is used in food contact materials, plastics, paper, printing inks, etc. The human body can be exposed through a variety of routes, including the digestive tract, the respiratory tract and the mucous membranes of the skin. Food is the main source of acrylamide in humans. Acrylamide is produced during high-temperature processing, especially in high-protein and carbohydrate-containing foods, mostly found in processed food such as chips, crisps, bread, biscuits, crackers, and breakfast muesli. They are ingested with food and dispersed in the human body. Human exposure to acrylamide may result in toxicological effects (neurotoxicity, genotoxicity, carcinogenicity, reproductive toxicity and so on). Therefore, there is an urgent need to detect and quantify acrylamide in processed foods. Table 4 summarizes the use of MS for the analysis of acrylamide.
LC–MS/MS and GC–MS/MS are the most commonly used methods to analyse acrylamide in food. Hasan et al. (63) used GC–MS/MS to determine acrylamide content in 180 hot-processed carbohydrate-rich foods. According to this analysis, the highest levels of acrylamide were found in crisps. Acrylamide has the characteristics of high polarity, low volatility and low molecular weight. Therefore, derivatisation is required for the determination of acrylamide by GC–MS/MS. LC–MS/MS is relatively easy to perform and is now the gold standard for accurate quantification of acrylamide in food (65). Mousavi Khaneghah et al. (66) investigated acrylamide concentrations in different kinds of food. They summarized the acrylamide concentrations in relevant foods in daily life, such as potato-based foods > fried foods > breakfast cereals > coffee > chocolate > baby food > bread > cookies > desserts > cakes > cereals > nuts. Similarly, Andačić et al. (59) reported that bakery products contributed approximately 37.2% to overall acrylamide exposure in Croatia. QuEChERS extraction of acrylamide, purification with PSA, and quantification with LC-ESI-MS/MS are widely used.
UPLC is becoming increasingly popular due to its high sensitivity, high selectivity and lack of need for derivatisation. Sun et al. (60) used an isotopic displacement method coupled with UPLC–MS/MS in ESI positive ion mode and MRM mode to quantitatively analyze common contaminants, such as AA, HMF, and PhIP in fried meatballs. The method was rapid and sensitive, achieving high recovery and linearity (R2 > 0.9998) of all three analytes within 4.5 min. Edible insects are becoming increasingly popular in diet as they contain many micronutrients and high protein levels. However, acrylamide is generally produced in food processing. Therefore, its determination in insect food cannot be ignored. Simultaneous determination of furans and acrylamide in insect food by HPLC-QqQ-MS/MS was reported by González-Gómez et al. (61). This technique used acidified water for SLE followed by SPE using functionalized mesoporous structured silica as a solid-phase extraction sorbent. The recoveries of furan compounds and acrylamides were 70–101%, respectively, with an adequate precision (RSD < 9%) and good linearity (R2 ≥ 0.995). It can be seen that LC–MS plays an important role in the detection acrylamide in thermally processed foods, which is an important tool for food safety monitoring.
HRMS is particularly suited for the detection of low molecular weight amides, with simple extraction, no clean-up step and shorter chromatographic times than other MS techniques. Fernandes et al. (62) used LC-ESI-Orbitrap HRMS to identify and quantify acrylamide in specific food substrates of biscuits. The HRMS method is reliable, can accurately analyze acrylamide, and has low dependence on matrix composition. The method had good reproducibility, with a LOD of 3.55 μg/kg and a LOQ of 11.8 μg/kg. Troise et al. (64) used UHPLC-Orbitrap HRMS to determine acrylamide in biscuits, French fries, ground coffee and brewed coffee. The results were in perfect agreement with those obtained by the LC-MS/MS method. LOD was 2.65 ppb. LOQ was 5 ppb. UHPLC-Orbitrap HRMS is going to be a promising technique for determining acrylamide in food.
Food additives
Food additives are added to preserve food’s flavor or improve its taste, appearance, and other qualities (67). Typical food additives include coloring, sweetening, preserving, antioxidant, and flavoring agents. Artificial foods have slowly taken over natural foods since they are cheaper. However, the widespread use of synthetic food additives can cause many problems related to misusing food additives, overdosing, and even toxicity. In addition, they pose potential risks to human health. Studies reveal that these artificial colors can cause allergy, asthma, damage DNA, cause hyperactivity, cancer, and human mutations. Therefore, screening food additives and quantifying their content is important since many unscrupulous companies use illegal additives for their benefit. MS is widely used in food analysis due to their multiple benefits. Table 5 summarizes the use of MS to analyze food additives.
Rhodamine B (RhB) is a prohibited food color additive. Many unscrupulous traders add RhB illegally to food products, especially spices. Numerous studies showed that RhB is carcinogenic, a neurotoxin, and a chronic toxicant for humans and animals. Wang et al. (69) used a sensitive UPLC-MS/MS method to monitor RhB in 292 different spices and reported that all samples were contaminated with RhB, suggesting that its exposure has a potential risk to consumers. Therefore, determining these illegal additives in food cannot be neglected. A synthetic color in food, AO is a non-approved food additive, but AO is still used for the color development of sour sprouts and chicken. Therefore, detecting this compound is vital, and a study was carried out to monitor the presence of AO in 211 samples of foods and spices using a UPLC-MS/MS method (70).
Improving in people’s living standards makes them more health-conscious, resulting in a growing demand for functional foods. Therefore, some people illegally add drugs to functional foods. When healthy people unknowingly consume these products, they suffer from side effects of decreased immunity, nutritional deficiencies, sickness, nausea, diarrhea, emesis, and kidney and liver damage. Wang et al. (71) proposed a new method for rapidly screening 42 widely used illegal additives in 6 functional food groups, based on an Atmospheric Solids Analytical Probe coupled with MS (ASAP-MS), without LC separation. The proposed method was used to analyze liquid or gaseous samples without pre-treatment. In addition, using a homemade library allowed rapid identification of suspect additives. The method was highly sensitive for samples in complex matrices, such as coffee samples. The ASAP-MS method was accurate, with the benefits of mobility, low cost, ease of operation, and automatic adjustment; thus, it can be widely used for rapid on-site detection of analytes in public security bureaus, especially grassroots police stations without corresponding equipment. Kim et al. (72) used LC-ESI-MS/MS to detect 7 food additives in kimchi with LODs of 0.00004–0.24 lg/mL, LOQs in the range of 0.00012–0.8 lg/mL, and the recoveries were 85.65–120.82%. Himmelreich et al. (68) determined preservative pseudomycin in cheese using MALDI-MS imaging (MALDI-MSI). MALDI-MSI can be used to detect a range of highly polar, non-volatile and thermally unstable samples. It is particularly promising for the detection of contaminants in food samples due to the reduced sample handling requirements and the ability to directly analyze untreated samples.
PCBs and Dioxins
PCBs and Dioxins are toxic chemicals present in the environment and accumulate through the food chain, and both are highly toxic due to chlorine atoms (Figure 3). Table 6 summarizes the MS methods used to analyze PCBs, dioxins and contaminants in food.
PCBs are a mixture of 209 different compounds. Due to their extensive use, six kinds of NDL PCBs are regarded as proxies for PCBs in food and are highly dispersed in the surrounding environment (80). PCBs affect people through several routes, and food is the main source, including eggs, seafood (74), poultry, meat (81), milk, and dairy products. PCBs are neurotoxic, carcinogenic, reprotoxic, immunotoxic, hepatotoxic, and cardiovascular toxic to humans. Therefore, it is essential to determine the PCBs’ occurrence in foodstuffs. Liu et al. (82) reviewed the progress of PCB determination by GC–MS, GC–HRMS, and HPLC-MS/MS. Arshad et al. (75) monitored mean levels and toxic equivalents (TEQ) of PCBs by GC–MS in vegetables, pulses, and cereals in Khanewal and Multan, Pakistan. Although the mean concentrations and TEQs were safe, some vegetables and cereals still posed a moderate risk to human health due to their high consumption rates. Shahsavari et al. (73) used a modified QuEChERS extraction and GC-QqQ-MS/MS method to monitor the levels of the 6 NDL-PCBs in cream and ice cream, and the recoveries were 95.54–107.18%. Among these, the cream exhibited higher levels of NDL-PCBs than ice cream. Lu et al. (79) used d-SPE with GC–MS/MS to determine PCBs in milk and obtained LODs were < 0.6 pg./g; the recovery range was 82.8 to 106%. PCBs are present in food at extremely low levels and the diversity and complexity of food matrices make the determination of PCBs in food difficult. Therefore, it is important to develop more efficient, sensitive and accurate methods to detect and quantify PCBs. Dioxins are highly toxic and difficult to break down. They are usually present in food at ultra-trace levels. Consuming vegetables grown in PCDD/Fs contaminated soil can cause transient liver damage, peripheral nerve damage, and cancer. Therefore, their detection is is crucial to preventing health risks (83). Polybrominated dibenzo-p-dioxins and dibenzofurans (PBDD/Fs) are produced during combustion and are considered environmental contaminants as by-products of industrial chemicals. However, their presence in food samples has only been reported recently. The primary route of exposure is dietary intake, producing toxic reactions. In addition, PBDF was found in higher concentrations than PBDD (84). Currently, detection is usually by GC–MS, with HRGC–HRMS being the most accurate but time-consuming method. Li et al. (76) used GC-(APCI) MS/MS to determine PCBs (one to ten) and PCDD/Fs in the food web of Chinese hairy crabs. Results showed that GC-(APCI) MS/MS detection was related to HRGC–HRMS, and the PCB concentration was correlated. Major PCB congeners in the aquatic food web were dichlorobenzene and 3, 3-dichlorobiphenyl; however, other congeners, such as MoCB and DiCB, were also identified in large quantities. Malavia et al. (78) analyzed PCDDs, PCDFs, and dl-PCBs in food using GC-ITMS/MS and obtained consistent results between GC-ITMS/MS and GC-HRMS. Moreover, the GC-ITMS/MS method was more accurate and faster. Therefore, it was recommended to determine PCDDs/PCDFs and dl-PCBs in foodstuffs and animal feed samples. A combination of HRMS was used to accurately quantify PCDD/Fs, PCBs, PBBFs, and PBDEs in eggs, milk, fish, shellfish, pork, beef, and poultry (77). Analyses were accomplished using HRGC-HRMS with a Trace Series 1,310 GC with DFS. The sample contaminants (except for PCDD/Fs) were sprayed in undivided mode, and the injector operated in undivided surge mode. All four pollutants were present in the sample, with PCBs being the most prominent.
PFCs and PPDs
Controlling the risks associated with changing mixtures of contaminants is one of the major challenges facing food safety today. Among the most prominent emerging food contaminants are PFCs and PPDs, which are of particular concern. PFCs are used in many applications, such as food packaging, nonstick pans, electronics, carpeting, fabrics, paints, adhesives, personal care products, and fire-fighting foam. The use and disposal of PFCs has resulted in the widespread distribution of these chemicals in the environment and their widespread presence in humans and wildlife. Bioaccumulation in fish has been shown to be a major source of PFCs in the diet. They are carcinogenic and are associated with hormonal disorders. In addition, they can accumulate and biomagnify through the food chain. PPDs is a synthetic compound and the newest pollutant, widely used to manufacture tires, belts, hoses, and cables. Due to the extensive use of these products in daily life, large quantities of PPDs and its breakdown products are released into the environment. Humans are exposed to PPDs and PPD-Q by inhalation, drinking water, eating, and skin contact. PPDs poses a potential risk to human health and causes angioneurotic edema, methemoglobinemia, acute tubular necrosis, and hepatotoxicity. Therefore, the determination of PFC and PPDs levels in food is essential for food safety. Table 7 summarises the MS methods used to analyse contaminated PFCS and PPD in food.
PFCs are a large group of man-made organic chemicals. The two most hotly debated PFCs are perfluorooctane sulfonic acid (PFOS) and perfluorooctanoic acid (PFOA). The PFOA and PFOS structures are shown in Figure 4. They have thermal and chemical inertness, high surface activity, and relatively low surface energy. Therefore, they are widely used in public and industry. Several PFOAs have been identified as persistent, bioaccumulating, and toxic. Therefore, it is important to determine the PFOA and PFOS levels in food. Their highest levels are known to occur in the Arctic. Kantiani et al. (93) reviewed industrial organic contaminants PFCs in food. They presented the major techniques used to detect and quantify them in food. Marine bioaccumulation is a major dietary source of PFCs. A recent review described several new PFAS methods published in the past 2 years, using ion mobility spectrometry (IMS) and MS (94).
Surma et al. (87) applied d-SPE and micro-UHPLC–MS/MS to determine PFOA and PFOS in honey samples, using ENV as sorbent and acetonitrile as an extraction solvent. Obtained results showed that the best recoveries were 84 and 87% for PFOA and PFOS, respectively. Ren et al. (85) recently used a novel MIP-PR-dispersion filter extraction (DFE) coupled with LC–MS/MS to determine PFOA and PFOS in milk. Compared with previously established methods, the proposed MIP-PR-DFE method combined the strengths of d-SPE and SPE with good cleaning performance and recovery regarding speed, selectivity, and cost-effectiveness. DFE was applied for the first time with LC–MS/MS to analyze PFOA and PFOS in milk, providing a new approach for efficient pollutant detection in food chemistry. The analysis results showed excellent recoveries (94.7–109%) and precision (RSD ≤9.5%). Sungur et al. (88) used LC–MS/MS to monitor PFOA and PFOS in 123 foods and beverages, such as fish, meat, offal, eggs, biscuits, French fries, cakes, chocolate, vegetables, milk, and fruit juices and described that fish was the main source of PFOS intake, while meat and offal were main sources of PFOA intake. Later, Tahziz et al. (86) determined PFOS and PFOA in egg yolk samples using LC–MS/MS. PFOS was quantified with concentrations ranging between 0.5 and 1.01 ng/g. The developed technique was economical, labor-saving, and sensitive. In conclusion, LC–MS is a good tool for the detection of PFOA and PFOS in food.
In China, the annual production of 6PPD is the highest of the PPD antioxidants. The rubber antioxidant (6PPD) and its ozonation product (6PPD-Q) are widely found in air (95), dust (96), and water (97, 98). Tian et al. (99) reported that 6PPD-Q causes acute pre-spawning mortality of silver salmon in freshwater streams of the Pacific Northwest, a phenomenon known as ‘urban runoff mortality syndrome’. This groundbreaking study generated great interest and concern. 6PPD-Q is a greater toxicant than its parent compound 6PPD, and contact with tire rubber-derived contaminants 6PPD-Q can induce in vitro mitochondrial dysfunction (90). The 6PPD and 6PPD-Q structures are shown in Figure 5. These compounds are widely found in vegetables, animal foods, or seafood.
The effects of 6PPD-Q on 4 commercially, culturally, and ecologically important fish species, including rainbow trout, brook trout, Arctic charr, and white sturgeon, were investigated by Brinkmann et al. (89) using UHPLC-Q-Exactive HF-Q-Orbitrap HRMS. This work demonstrated the acute toxicity of 6PPD-Q at environmentally relevant concentrations to other commercially, culturally, and ecologically important fish species. Salmonids are taxa of great cultural, ecological, and market value worldwide. Hiki et al. (100) conducted a 6PPD-Q 96-h acute toxicity test using LC–MS/MS on three salmonids, showing lethal toxicity to white-spotted salmon. Ji et al. (91) developed a modified QuEChERS method combined with HPLC–MS/MS to study the 6PPD and 6PPD-Q levels in fish and honey. Both these compounds were detected in blackfish, sea bass, and horse mackerel, while none were in honey samples. The linearity, recovery, and matrix effects were satisfactory, ranging from 70.4 to 95.6%, with excellent reproducibility (RSD < 8.4%). This work demonstrated the presence of 6PPD and 6PPD-Q in fishery samples for the first time, suggesting that they could enter the human body by eating polluted fish. To source and quantify the TWPs-derived compounds in edible plants. Castan et al. (92) first incubated lettuce plants in a TWPs-derived hydroponic solution. Their leaves, roots, and nutrients were collected for processing, and QMS determined concentrations. In addition, the compounds in plant leaves were identified by high-resolution Orbitrap MS. This work indicated that TWP could be a persistent source of TWP-derived compounds in edible plants, with TWP metabolites accumulating in lettuce leaves. In addition, 6PPD was readily absorbed by lettuce. 6PPD has been detected in environmental matrices, including water, soil, and particulates, so lettuce safety should also be a cause of concern. In summary, MS is an useful tool for the determination of new contaminants and LC–MS is very beneficial for the screening of unknown pollutants in food.
Conclusions and future perspectives
This work completely reviewed MS methods in analysis of harmful contaminants in food. The combination of MS with modern chromatographic and other separation tools offers higher resolution and precision than conventional MS, enabling isomeric and conformational analogs’ identifications. Compared to conventional chromatographic approaches, LC–MS and GC–MS allow more rapid and sensitive detection of toxic and hazardous substances in food, which can effectively solve food safety emergencies. Moreover, MS can identify unknown compounds from complex background matrices and consequently has been fully implemented in routine analytical and research laboratories. The capabilities of MS-based proteomics are expanding with recent advances in MS technologies. HRMS methods provide rapid and comprehensive information on food contamination via targeted and non-targeted analysis, which is a breakthrough. However, the standardization of untargeted analysis still needs much work. Furthermore, there are emerging food safety issues, like the misuse of antibiotics, the application of toxic nanomaterials (e.g., food packaging materials) and the occurrence of unreported contaminants. Hence, development of more rapid, sensitive and accurate MS approaches in food analysis is still needed.
Author contributions
QS wrote the original draft of the manuscript. QS, YD, XW, XZ, and SH conducted the searching processes. WZ and DY reviewed, edited, and supervised the manuscript and done the funding acquisition. All authors contributed to the article and approved the submitted version.
Funding
This work was supported by the National Natural Science Foundation of China (22206172, 22076174).
Conflict of interest
The authors declare that the research was conducted in the absence of any commercial or financial relationships that could be construed as a potential conflict of interest.
Publisher’s note
All claims expressed in this article are solely those of the authors and do not necessarily represent those of their affiliated organizations, or those of the publisher, the editors and the reviewers. Any product that may be evaluated in this article, or claim that may be made by its manufacturer, is not guaranteed or endorsed by the publisher.
References
1. Dong, H, Xu, Y, Ye, H, Huang, M, Hu, J, Xian, Y, et al. Advances in analysis of contaminants in foodstuffs on the basis of orbitrap mass spectrometry: a review. Food Anal Methods. (2022) 15:803–19. doi: 10.1007/s12161-021-02168-0
2. Kotretsou, S, and Koutsodimou, A. Overview of the applications of tandem mass spectrometry (MS/MS) in food analysis of nutritionally harmful compounds. Food Rev Int. (2006) 22:125–72. doi: 10.1080/87559120600574543
3. Yang, B, Ma, W, Wang, S, Shi, L, Li, X, Ma, Z, et al. Determination of eight neonicotinoid insecticides in chinese cabbage using a modified QuEChERS method combined with ultra performance liquid chromatography-tandem mass spectrometry. Food Chem. (2022) 387:132935. doi: 10.1016/j.foodchem.2022.132935
4. Yuan, X, Kim, C, Lee, R, Kim, M, Shin, H, Kim, L, et al. Validation of a multi-residue analysis method for 287 pesticides in citrus fruits mandarin orange and grapefruit using liquid chromatography-tandem mass spectrometry. Foods. (2022) 11:3522. doi: 10.3390/foods11213522
5. Lee, S, Kwak, S, Sarker, A, Moon, J, and Kim, J. Optimization of a multi-residue analytical method during determination of pesticides in meat products by GC-MS/MS. Foods. (2022) 11:2930. doi: 10.3390/foods11192930
6. Jongedijk, E, Fifeik, M, Arrizabalaga-Larrañaga, A, Polzer, J, Blokland, M, and Sterk, S. Use of high-resolution mass spectrometry for veterinary drug multi-residue analysis. Food Cont. (2023) 145:109488. doi: 10.1016/j.foodcont.2022.109488
7. Xu, M, Gao, Y, Wang, X, Han, X, and Zhao, B. Comprehensive strategy for sample preparation for the analysis of food contaminants and residues by GC-MS/MS: a review of recent research trends. Foods. (2021) 10:2473. doi: 10.3390/foods10102473
8. Alassane-Kpembi, I, Schatzmayr, G, Taranu, I, Marin, D, Puel, O, and Oswald, I. Mycotoxins co-contamination: methodological aspects and biological relevance of combined toxicity studies. Crit Rev Food Sci Nutr. (2017) 57:3489–507. doi: 10.1080/10408398.2016.1140632
9. Castilla-Fernández, D, Rocío-Bautista, P, Moreno-González, D, García-Reyes, J, and Molina-Díaz, A. Dilute-and-shoot versus clean-up approaches: a comprehensive evaluation for the determination of mycotoxins in nuts by UHPLC-MS/MS. LWT. (2022) 169:113976. doi: 10.1016/j.lwt.2022.113976
10. Malachová, A, Stránská, M, Václavíková, M, Elliott, C, Black, C, Meneely, J, et al. Advanced LC-MS-based methods to study the co-occurrence and metabolization of multiple mycotoxins in cereals and cereal-based food. Anal Bioanal Chem. (2018) 410:801–25. doi: 10.1007/s00216-017-0750-7
11. Alsharif, A, Choo, Y, and Tan, G. Detection of five mycotoxins in different food matrices in the malaysian market by using validated liquid chromatography electrospray ionization triple quadrupole mass spectrometry. Toxins. (2019) 11:196. doi: 10.3390/toxins11040196
12. Keskin, E, and Eyupoglu, O. Determination of mycotoxins by HPLC, LC-MS/MS and health risk assessment of the mycotoxins in bee products of Turkey. Food Chem. (2023) 400:134086. doi: 10.1016/j.foodchem.2022.134086
13. Lee, Y, Kim, J, You, D, Kim, C, Lee, J, and Park, H. Analytical method of multi-mycotoxins in table-ready foods for a total diet study using stable isotope dilution liquid chromatography-tandem mass spectrometry. J AOAC Int. (2019) 102:1657–65. doi: 10.5740/jaoacint.19-0031
14. Lago, L, Nievierowski, T, Mallmann, L, Rodrigues, E, and Welke, J. QuEChERS-LC-QTOFMS for the simultaneous determination of legislated and emerging mycotoxins in malted barley and beer using matrix-matched calibration as a solution to the commercial unavailability of internal standards for some mycotoxins. Food Chem. (2021) 345:128744. doi: 10.1016/j.foodchem.2020.128744
15. Rodríguez-Carrasco, Y, Castaldo, L, Gaspari, A, Graziani, G, and Ritieni, A. Development of an UHPLC-Q-Orbitrap HRMS method for simultaneous determination of mycotoxins and isoflavones in soy-based burgers. LWT. (2019) 99:34–42. doi: 10.1016/j.lwt.2018.09.046
16. Tsagkaris, A, Hrbek, V, Dzuman, Z, and Hajslova, J. Critical comparison of direct analysis in real time orbitrap mass spectrometry (DART-Orbitrap MS) towards liquid chromatography mass spectrometry (LC-MS) for mycotoxin detection in cereal matrices. Food Cont. (2022) 132:108548. doi: 10.1016/j.foodcont.2021.108548
17. Iqbal, S. Mycotoxins in food, recent development in food analysis and future challenges; a review. Curr Opin Food Sci. (2021) 42:237–47. doi: 10.1016/j.cofs.2021.07.003
18. Vargas Medina, D, Bassolli Borsatto, J, Maciel, E, and Lanças, F. Current role of modern chromatography and mass spectrometry in the analysis of mycotoxins in food. TrAC Trends Anal Chem. (2021) 135:116156. doi: 10.1016/j.trac.2020.116156
19. Singh, J, and Mehta, A. Rapid and sensitive detection of mycotoxins by advanced and emerging analytical methods: a review. Food Sci Nutr. (2020) 8:2183–204. doi: 10.1002/fsn3.1474
20. Mousavi Khaneghah, A, Fakhri, Y, Gahruie, H, Niakousari, M, and Sant’Ana, A. Mycotoxins in cereal-based products during 24 years (1983–2017): a global systematic review. Trends Food Scie Tech. (2019) 91:95–105. doi: 10.1016/j.tifs.2019.06.007
21. Acuña-Gutiérrez, C, Jiménez, V, and Müller, J. Occurrence of mycotoxins in pulses. Compr Rev Food Sci Food Saf. (2022) 21:4002–17. doi: 10.1111/1541-4337.13008
22. Masiá, A, Suarez-Varela, M, Llopis-Gonzalez, A, and Picó, Y. Determination of pesticides and veterinary drug residues in food by liquid chromatography-mass spectrometry: a review. Anal Chim Acta. (2016) 936:40–61. doi: 10.1016/j.aca.2016.07.023
23. Notardonato, I, Russo, M, Vitali, M, Protano, C, and Avino, P. Analytical method validation for determining organophosphorus pesticides in baby foods by a modified liquid-liquid microextraction method and gas chromatography-ion trap/mass spectrometry analysis. Food Anal Methods. (2019) 12:41–50. doi: 10.1007/s12161-018-1335-6
24. Zhang, Y, Zhang, H, Wang, J, Yu, Z, Li, H, and Yang, M. Suspect and target screening of emerging pesticides and their transformation products in an urban river using LC-QTOF-MS. Sci Total Environ. (2021) 790:147978. doi: 10.1016/j.scitotenv.2021.147978
25. Bhandari, G, Atreya, K, Vašíčková, J, Yang, X, and Geissen, V. Ecological risk assessment of pesticide residues in soils from vegetable production areas: a case study in S-Nepal. Sci Total Environ. (2021) 788:147921. doi: 10.1016/j.scitotenv.2021.147921
26. Cutillas, V, Murcia-Morales, M, Gómez-Ramos, M, Taha, S, and Fernández-Alba, A. Supercritical fluid chromatography coupled to tandem mass spectrometry for the analysis of pesticide residues in dried spices. benefits and drawbacks. Anal Chim Acta. (2019) 1059:124–35. doi: 10.1016/j.aca.2019.01.010
27. Pérez-Fernández, V, Mainero Rocca, L, Tomai, P, Fanali, S, and Gentili, A. Recent advancements and future trends in environmental analysis: sample preparation, liquid chromatography and mass spectrometry. Anal Chim Acta. (2017) 983:9–41. doi: 10.1016/j.aca.2017.06.029
28. Tran-Lam, T, Bui, M, Nguyen, H, Dao, Y, and Le, G. A combination of chromatography with tandem mass spectrometry systems (UPLC-MS/MS and GC-MS/MS), modified QuEChERS extraction and mixed-mode SPE clean-up method for the analysis of 656 pesticide residues in rice. Foods. (2021) 10:2455. doi: 10.3390/foods10102455
29. Xu, L, Abd El-Aty, A, Eun, J, Shim, J, Zhao, J, Lei, X, et al. Recent advances in rapid detection techniques for pesticide residue: a review. J Agric Food Chem. (2022) 70:13093–117. doi: 10.1021/acs.jafc.2c05284
30. Liu, W, Su, Y, Liu, J, Zhang, K, Wang, X, Chen, Y, et al. Determination of cyflufenamid residues in 12 foodstuffs by QuEChERS-HPLC-MS/MS. Food Chem. (2021) 362:130148. doi: 10.1016/j.foodchem.2021.130148
31. Han, C, Hu, B, Chen, S, Wang, N, Hou, J, Jin, N, et al. Determination of Xinjunan pesticide residue in foodstuffs of plant origin by a modified QuEChERS method and ultra performance liquid chromatography-tandem mass spectrometry. LWT. (2021) 151:112101. doi: 10.1016/j.lwt.2021.112101
32. Kottadiyil, D, Mehta, T, and Thasale, R. Determination and dietary risk assessment of 52 pesticide residues in vegetable and fruit samples by GC-MS/MS and UHPLC-QTOF/MS from Gujarat. India J Food Compos Anal. (2023) 115:104957. doi: 10.1016/j.jfca.2022.104957
33. Goon, A, Khan, Z, Oulkar, D, Shinde, R, Gaikwad, S, and Banerjee, K. A simultaneous screening and quantitative method for the multiresidue analysis of pesticides in spices using ultra-high performance liquid chromatography-high resolution (Orbitrap) mass spectrometry. J Chromatogr A. (2018) 1532:105–11. doi: 10.1016/j.chroma.2017.11.066
34. Chen, Z, Sun, X, Chen, X, Wang, D, Yu, X, and Jiang, W. HPLC-MS/MS analysis of zinc-thiazole residues in foods of plant origin by a modified derivatization-QueChERS method. Food Chem. (2022) 386:132752. doi: 10.1016/j.foodchem.2022.132752
35. Shin, D, Kim, J, and Kang, H. Simultaneous determination of multi-pesticide residues in fish and shrimp using dispersive-solid phase extraction with liquid chromatography-tandem mass spectrometry. Food Cont. (2021) 120:107552. doi: 10.1016/j.foodcont.2020.107552
36. Prata, R, López-Ruiz, R, Petrarca, M, Teixeira Godoy, H, Garrido Frenich, A, and Romero-González, R. Targeted and non-targeted analysis of pesticides and aflatoxins in baby foods by liquid chromatography coupled to quadrupole Orbitrap mass spectrometry. Food Cont. (2022) 139:109072. doi: 10.1016/j.foodcont.2022.109072
37. Liu, C, Ji, Y, Jiang, X, Yuan, X, Zhang, X, and Zhao, L. The determination of pesticides in tea samples followed by magnetic multiwalled carbon nanotube-based magnetic solid-phase extraction and ultra-high performance liquid chromatography-tandem mass spectrometry. New J Chem. (2019) 43:5395–403. doi: 10.1039/C8NJ06536E
38. Song, L, Han, Y, Yang, J, Qin, Y, Zeng, W, Xu, S, et al. Rapid single-step cleanup method for analyzing 47 pesticide residues in pepper, chili peppers and its sauce product by high performance liquid and gas chromatography-tandem mass spectrometry. Food Chem. (2019) 279:237–45. doi: 10.1016/j.foodchem.2018.12.017
39. Liu, X, Liu, Z, Bian, L, Ping, Y, Li, S, Zhang, J, et al. Determination of pesticide residues in chilli and Sichuan pepper by high performance liquid chromatography quadrupole time-of-flight mass spectrometry. Food Chem. (2022) 387:132915. doi: 10.1016/j.foodchem.2022.132915
40. Lee, J, Jang, S, Hur, S, Bang, H, Bae, I, and Kim, H. LC-MS/MS and GC-MS/MS cross-checking analysis method for 247 pesticide residues in sweet pepper (Capsicum annuum). Int J Food Prop. (2021) 24:1758–76. doi: 10.1080/10942912.2021.1993251
41. Harischandra, N, Pallavi, M, Bheemanna, M, PavanKumar, K, Chandra Sekhara Reddy, V, Udaykumar, N, et al. Simultaneous determination of 79 pesticides in pigeonpea grains using GC-MS/MS and LC-MS/MS. Food Chem. (2021) 347:128986. doi: 10.1016/j.foodchem.2020.128986
42. Mozzaquatro, J, César, I, Pinheiro, A, and Caldas, E. Pesticide residues analysis in passion fruit and its processed products by LC-MS/MS and GC-MS/MS: method validation, processing factors and dietary risk assessment. Food Chem. (2022) 375:131643. doi: 10.1016/j.foodchem.2021.131643
43. Cheng, S, Lee, R, Jeng, J, Lee, C, and Shiea, J. Fast screening of trace multiresidue pesticides on fruit and vegetable surfaces using ambient ionization tandem mass spectrometry. Anal Chim Acta. (2020) 1102:63–71. doi: 10.1016/j.aca.2019.12.038
44. Yu, Y, Liu, Y, Wang, W, Jia, Y, Zhao, G, Zhang, X, et al. Highly sensitive determination of aminoglycoside residues in food by sheathless CE-ESI-MS/MS. Anal Meth. (2019) 11:5064–9. doi: 10.1039/C9AY01728C
45. Jung, Y, Kim, D, Nam, T, Seo, D, and Yoo, M. Identification and quantification of multi-class veterinary drugs and their metabolites in beef using LC-MS/MS. Food Chem. (2022) 382:132313. doi: 10.1016/j.foodchem.2022.132313
46. Chung, S, and Lam, C. Development of a 15-class multiresidue method for analyzing 78 hydrophilic and hydrophobic veterinary drugs in milk, egg and meat by liquid chromatography-tandem mass spectrometry. Anal Meth. (2015) 7:6764–76. doi: 10.1039/C5AY01317H
47. Dasenaki, M, Bletsou, A, Koulis, G, and Thomaidis, N. Qualitative multiresidue screening method for 143 veterinary drugs and pharmaceuticals in milk and fish tissue using liquid chromatography quadrupole-time-of-flight mass spectrometry. J Agric Food Chem. (2015) 63:4493–508. doi: 10.1021/acs.jafc.5b00962
48. Li, J, Ren, X, Diao, Y, Chen, Y, Wang, Q, Jin, W, et al. Multiclass analysis of 25 veterinary drugs in milk by ultra-high performance liquid chromatography-tandem mass spectrometry. Food Chem. (2018) 257:259–64. doi: 10.1016/j.foodchem.2018.02.144
49. Melekhin, A, Tolmacheva, V, Goncharov, N, Apyari, V, Dmitrienko, S, Shubina, E, et al. Multi-class, multi-residue determination of 132 veterinary drugs in milk by magnetic solid-phase extraction based on magnetic hypercrosslinked polystyrene prior to their determination by high-performance liquid chromatography-tandem mass spectrometry. Food Chem. (2022) 387:132866. doi: 10.1016/j.foodchem.2022.132866
50. Zhao, H, Zulkoski, J, and Mastovska, K. Development and validation of a multiclass, multiresidue method for veterinary drug analysis in infant formula and related ingredients using UHPLC-MS/MS. J Agric Food Chem. (2017) 65:7268–87. doi: 10.1021/acs.jafc.7b00271
51. Wang, J, Zhao, W, Guo, W, Li, Y, Jiang, R, Li, H, et al. Simultaneous screening and analysis of 155 veterinary drugs in livestock foods using ultra-high performance liquid chromatography tandem quadrupole linear-ion-trap mass spectrometry. Food Chem. (2022) 393:133260. doi: 10.1016/j.foodchem.2022.133260
52. Hou, X, Xu, X, Xu, X, Han, M, and Qiu, S. Application of a multiclass screening method for veterinary drugs and pesticides using HPLC-QTOF-MS in egg samples. Food Chem. (2020) 309:125746. doi: 10.1016/j.foodchem.2019.125746
53. Desmarchelier, A, Savoy, M, Delatour, T, and Mottier, P. Extended coverage of veterinary drug residues in food by LC-HRMS to ensure food compliance and prevent the spread of antimicrobial resistance. Microchem J. (2022) 183:108057. doi: 10.1016/j.microc.2022.108057
54. Zhao, W, Jiang, R, Guo, W, Guo, C, Li, S, Wang, J, et al. Screening and analysis of multiclass veterinary drug residues in animal source foods using UPLC-Q-Exactive Orbitrap/MS. B Environ Contam Tox. (2021) 107:228–38. doi: 10.1007/s00128-021-03273-w
55. Mehl, A, Hudel, L, Bücker, M, and Morlock, G. Validated screening method for 81 multiclass veterinary drug residues in food via online-coupling high-throughput planar solid-phase extraction to high-performance liquid chromatography-orbitrap tandem mass spectrometry. J Agric Food Chem. (2022) 70:10886–98. doi: 10.1021/acs.jafc.2c03925
56. Wang, H, Tian, H, Ai, L, and Liang, S. Screening and quantification of 146 veterinary drug residues in beef and chicken using QuEChERS combined with high performance liquid chromatography-quadrupole orbitrap mass spectrometry. Food Chem. (2023) 408:135207. doi: 10.1016/j.foodchem.2022.135207
57. Kikuchi, H, Sakai, T, Teshima, R, Nemoto, S, and Akiyama, H. Total determination of chloramphenicol residues in foods by liquid chromatography-tandem mass spectrometry. Food Chem. (2017) 230:589–93. doi: 10.1016/j.foodchem.2017.03.071
58. Zhou, H, Liu, R, Chen, Q, Zheng, X, Qiu, J, Ding, T, et al. Surface molecularly imprinted solid-phase extraction for the determination of vancomycin and norvancomycin in milk by liquid chromatography coupled to tandem mass spectrometry. Food Chem. (2022) 369:130886. doi: 10.1016/j.foodchem.2021.130886
59. Andačić, I, Tot, A, Ivešić, M, Krivohlavek, A, Thirumdas, R, Barba, F, et al. Exposure of the croatian adult population to acrylamide through bread and bakery products. Food Chem. (2020) 322:126771. doi: 10.1016/j.foodchem.2020.126771
60. Sun, G, Wang, P, Chen, W, Hu, X, Chen, F, and Zhu, Y. Simultaneous quantitation of acrylamide, 5-hydroxymethylfurfural, and 2-amino-1-methyl-6-phenylimidazo [4, 5-b]pyridine using UPLC-MS/MS. Food Chem. (2022) 375:131726. doi: 10.1016/j.foodchem.2021.131726
61. González-Gómez, L, Morante-Zarcero, S, Pérez-Quintanilla, D, and Sierra, I. Simultaneous determination of furanic compounds and acrylamide in insect-based foods by HPLC-QqQ-MS/MS employing a functionalized mesostructured silica as sorbent in solid-phase extraction. Foods. (2021) 10:1577. doi: 10.3390/foods10071557
62. Fernandes, C, Carvalho, D, and Guido, L. Determination of acrylamide in biscuits by high-resolution orbitrap mass spectrometry: a novel application. Foods. (2019) 8:597. doi: 10.3390/foods8120597
63. Hasan, G, Das, A, and Satter, M. Detection of acrylamide traces in some commonly consumed heat-treated carbohydrate-rich foods by GC-MS/MS in Bangladesh. Heliyon. (2022) 8:e11092. doi: 10.1016/j.heliyon.2022.e11092
64. Troise, A, Fiore, A, and Fogliano, V. Quantitation of acrylamide in foods by high-resolution mass spectrometry. J Agric Food Chem. (2014) 62:74–9. doi: 10.1021/jf404205b
65. Delatour, T, Desmarchelier, A, and Stadler, R. Challenges in the measurement of acrylamide in food by confirmatory methods. Curr Opin Food Sci. (2022) 48:100951. doi: 10.1016/j.cofs.2022.100951
66. Mousavi Khaneghah, A, Fakhri, Y, Nematollahi, A, Seilani, F, and Vasseghian, Y. The concentration of acrylamide in different food products: a global systematic review, meta-analysis, and meta-regression. Food Rev Int. (2022) 38:1286–304. doi: 10.1080/87559129.2020.1791175
67. Wu, L, Zhang, C, Long, Y, Chen, Q, Zhang, W, and Liu, G. Food additives: from functions to analytical methods. Crit Rev Food Sci Nutr. (2022) 62:8497–517. doi: 10.1080/10408398.2021.1929823
68. Kokesch-Himmelreich, J, Wittek, O, Race, A, Rakete, S, Schlicht, C, Busch, U, et al. MALDI mass spectrometry imaging: from constituents in fresh food to ingredients, contaminants and additives in processed food. Food Chem. (2022) 385:132529. doi: 10.1016/j.foodchem.2022.132529
69. Wang, M, Nie, X, Tian, L, Hu, J, Yin, D, Qiao, H, et al. Rhodamine B in spices determined by a sensitive UPLC-MS/MS method. Food Addit Contam B. (2019) 12:59–64. doi: 10.1080/19393210.2018.1548504
70. Tran-Lam, T, Hong, M, Le, G, and Luu, P. Auramine O in foods and spices determined by an UPLC-MS/MS method. Food Addit Contam B. (2020) 13:171–6. doi: 10.1080/19393210.2020.1742208
71. Wang, X, Huang, M, Li, X, Dai, W, and Liang, J. Rapid screening of illegal additives in functional food using atmospheric pressure solids analysis probe coupled to a portable mass spectrometer. J Pharm Biomed Anal. (2022) 214:114722. doi: 10.1016/j.jpba.2022.114722
72. Kim, H, Lee, M, Park, H, Kim, H, Cho, S, and Jeong, M. Simultaneous determination of synthetic food additives in kimchi by liquid chromatography-electrospray tandem mass spectrometry. Food Sci Biotechnol. (2018) 27:877–82. doi: 10.1007/s10068-018-0308-2
73. Shahsavari, S, Shariatifar, N, Arabameri, M, Mahmoudi, R, Moazzen, M, and Ghajarbeygi, P. Analysis of polychlorinated biphenyls in cream and ice cream using modified QuEChERS extraction and GC-QqQ-MS/MS method: a risk assessment study. Int J Dairy Technol. (2022) 75:448–59. doi: 10.1111/1471-0307.12849
74. Chamkasem, N, Lee, S, and Harmon, T. Analysis of 19 PCB congeners in catfish tissue using a modified QuEChERS method with GC-MS/MS. Food Chem. (2016) 192:900–6. doi: 10.1016/j.foodchem.2015.07.088
75. Arshad, M, Asmatullah,, Shafiq Ahmed, M, Shafiq Ahmed, K, Makhdoom Hussain, S, and Hussain, N. Monitoring of level of mean concentration and toxicity equivalence (TEQ) of polychlorinated biphenyls (PCBs) in selected vegetables, beans and grains in Khanewal and Multan, Pakistan. Saudi J Biol Sci. (2022) 29:2787–93. doi: 10.1016/j.sjbs.2022.01.009
76. Li, X, Zhen, Y, Wang, R, Li, T, Dong, S, Zhang, W, et al. Application of gas chromatography coupled to triple quadrupole mass spectrometry (GC-(APCI)MS/MS) in determination of PCBs (mono-to deca-) and PCDD/Fs in hinese mitten crab food webs. Chemosphere. (2021) 265:129055. doi: 10.1016/j.chemosphere.2020.129055
77. Ceci, R, Diletti, G, Bellocci, M, Chiumiento, F, D'Antonio, S, De Benedictis, A, et al. Brominated and chlorinated contaminants in food (PCDD/Fs, PCBs, PBDD/Fs PBDEs): simultaneous determination and occurrence in Italian produce. Chemosphere. (2022) 288:132445. doi: 10.1016/j.chemosphere.2021.132445
78. Malavia, J, Abalos, M, Santos, F, Abad, E, Rivera, J, and Galceran, M. Ion-trap tandem mass spectrometry for the analysis of polychlorinated dibenzo-p-dioxins, dibenzofurans, and dioxin-like polychlorinated biphenyls in food. J Agric Food Chem. (2007) 55:10531–9. doi: 10.1021/jf0719858
79. Lu, Y, Shen, Q, Zhai, C, Yan, H, and Shen, S. Ant nest-like hierarchical porous imprinted resin-dispersive solid-phase extraction for selective extraction and determination of polychlorinated biphenyls in milk. Food Chem. (2023) 406:135076. doi: 10.1016/j.foodchem.2022.135076
80. Saktrakulkla, P, Lan, T, Hua, J, Marek, R, Thorne, P, and Hornbuckle, K. Polychlorinated biphenyls in food. Environ Sci Technol. (2020) 54:11443–52. doi: 10.1021/acs.est.0c03632
81. Barone, G, Storelli, A, Quaglia, N, Dambrosio, A, Garofalo, R, Chiumarulo, R, et al. Dioxin and PCB residues in meats from italy: consumer dietary exposure. Food Chem Toxicol. (2019) 133:110717. doi: 10.1016/j.fct.2019.110717
82. Liu, J, Li, G, Liu, J, Wang, P, Wu, D, Zhang, X, et al. Recent progress on toxicity and detection methods of polychlorinated biphenyls in environment and foodstuffs. Crit Rev Anal Chem. (2022) 1997570:1–26. doi: 10.1016/j.chemosphere.2021.132445
83. Vernez, D, Oltramare, C, Sauvaget, B, Demougeot-Renard, H, Aicher, L, Roth, N, et al. Polychlorinated dibenzo-p-dioxins (PCDDs) and dibenzofurans (PCDFs) soil contamination in Lausanne, Switzerland: combining pollution mapping and human exposure assessment for targeted risk management. Environ Pollut. (2023) 316:120441. doi: 10.1016/j.envpol.2022.120441
84. Fernandes, A, and Falandysz, J. Polybrominated dibenzo-p-dioxins and furans (PBDD/Fs): contamination in food, humans and dietary exposure. Sci Total Environ. (2021) 761:143191. doi: 10.1016/j.scitotenv.2020.143191
85. Ren, J, Lu, Y, Han, Y, Qiao, F, and Yan, H. Novel molecularly imprinted phenolic resin-dispersive filter extraction for rapid determination of perfluorooctanoic acid and perfluorooctane sulfonate in milk. Food Chem. (2023) 400:134062. doi: 10.1016/j.foodchem.2022.134062
86. Tahziz, A, Mohamad Haron, D, and Aziz, M. Liquid chromatographic tandem mass spectrometric (LC-MS/MS) determination of perfluorooctane sulfonate (PFOS) and perfluorooctanoic acid (PFOA) in the yolk of poultry eggs in Malaysia. Molecules. (2020) 25:2335. doi: 10.3390/molecules25102335
87. Surma, M, Wiczkowski, W, Cieślik, E, and Zieliński, H. Method development for the determination of PFOA and PFOS in honey based on the dispersive Solid Phase Extraction (d-SPE) with micro-UHPLC-MS/MS system. Microchem J. (2015) 121:150–6. doi: 10.1016/j.microc.2015.02.008
88. Sungur, Ş, Köroğlu, M, and Turgut, F. Determination of perfluorooctanoic acid (PFOA) and perfluorooctane sulfonic acid (PFOS) in food and beverages. Int J Environ Anal Chem. (2018) 98:360–8. doi: 10.1080/03067319.2018.1468440
89. Brinkmann, M, Montgomery, D, Selinger, S, Miller, J, Stock, E, Alcaraz, A, et al. Acute toxicity of the tire rubber-derived chemical 6PPD-quinone to four fishes of commercial, cultural, and ecological importance. Environ Sci Technol Lett. (2022) 9:333–8. doi: 10.1021/acs.estlett.2c00050
90. Mahoney, H, Da Silva Junior, F, Roberts, C, Schultz, M, Ji, X, Alcaraz, A, et al. Exposure to the tire rubber-derived contaminant 6PPD-Quinone causes mitochondrial dysfunction in vitro. Environ Sci Technol Lett. (2022) 9:765–71. doi: 10.1021/acs.estlett.2c00431
91. Ji, J, Li, C, Zhang, B, Wu, W, Wang, J, Zhu, J, et al. Exploration of emerging environmental pollutants 6PPD and 6PPDQ in honey and fish samples. Food Chem. (2022) 396:133640. doi: 10.1016/j.foodchem.2022.133640
92. Castan, S, Sherman, A, Peng, R, Zumstein, M, Wanek, W, Hüffer, T, et al. Uptake, metabolism, and accumulation of tire wear particle-derived compounds in lettuce. Environ Sci Technol. (2023) 57:168–78. doi: 10.1021/acs.est.2c05660
93. Kantiani, L, Llorca, M, Sanchís, J, Farré, M, and Barceló, D. Emerging food contaminants: a review. Anal Bioanal Chem. (2010) 398:2413–27. doi: 10.1007/s00216-010-3944-9
94. Richardson, S, and Ternes, T. Water analysis: emerging contaminants and current issues. Anal Chem. (2022) 94:382–416. doi: 10.1021/acs.analchem.1c04640
95. Zhang, Y, Xu, C, Zhang, W, Qi, Z, Song, Y, Zhu, L, et al. p-Phenylenediamine antioxidants in PM2.5: the underestimated urban air pollutants. Environ Sci Technol. (2022) 56:6914–21. doi: 10.1021/acs.est.1c04500
96. Hiki, K, and Yamamoto, H. Concentration and leachability of N-(1, 3-dimethylbutyl)-N′-phenyl-p-phenylenediamine (6PPD) and its quinone transformation product (6PPD-Q) in road dust collected in Tokyo. Japan Environ Pollut. (2022) 302:119082. doi: 10.1016/j.envpol.2022.119082
97. Cao, G, Wang, W, Zhang, J, Wu, P, Zhao, X, Yang, Z, et al. New evidence of rubber-derived quinones in water, air, and soil. Environ Sci Technol. (2022) 56:4142–50. doi: 10.1021/acs.est.1c07376
98. Peng, Z, Zhang, W, Yin, D, Zhang, X, Liu, S, Zhao, W, et al. Novel magnetic single-layer nano-MXene as a highly effective adsorbent and new SALDI-TOF-MS matrix for enrichment and rapid determination of p-Phenylenediamine antioxidants in water. Chem Eng J. (2023) 454:139978. doi: 10.1016/j.cej.2022.139978
99. Tian, Z, Zhao, H, Peter, K, Gonzalez, M, Wetzel, J, Wu, C, et al. A ubiquitous tire rubber-derived chemical induces acute mortality in Coho salmon. Science. (2021) 371:185–9. doi: 10.1126/science.abd6951
100. Hiki, K, and Yamamoto, H. The tire-derived chemical 6PPD-quinone is lethally toxic to the white-spotted char salvelinus leucomaenis pluvius but not to two other salmonid species. Environ Sci Technol Lett. (2022) 9:1050–5. doi: 10.1021/acs.estlett.2c00683
Keywords: mass spectrometry, food analysis, mycotoxin, pesticide and veterinary drug residues, acrylamide, food additives, polychlorinated biphenyls and dioxins
Citation: Sun Q, Dong Y, Wen X, Zhang X, Hou S, Zhao W and Yin D (2023) A review on recent advances in mass spectrometry analysis of harmful contaminants in food. Front. Nutr. 10:1244459. doi: 10.3389/fnut.2023.1244459
Edited by:
Chunxue Yang, Sun Yat-sen University, ChinaReviewed by:
Hongzhi Zhao, Nankai University, ChinaHemi Luan, Guangdong University of Technology, China
Emmanouil Tsochatzis, Aarhus University, Denmark
Copyright © 2023 Sun, Dong, Wen, Zhang, Hou, Zhao and Yin. This is an open-access article distributed under the terms of the Creative Commons Attribution License (CC BY). The use, distribution or reproduction in other forums is permitted, provided the original author(s) and the copyright owner(s) are credited and that the original publication in this journal is cited, in accordance with accepted academic practice. No use, distribution or reproduction is permitted which does not comply with these terms.
*Correspondence: Wuduo Zhao, emhhb3d1ZHVvQDE2My5jb20=; Dan Yin, eWluZGFuQHp6dS5lZHUuY24=