- 1The First Clinical School of Guangzhou University of Chinese Medicine, Guangzhou, China
- 2Hospital of Chengdu University of Traditional Chinese Medicine, Chengdu University of Traditional Chinese Medicine, Chengdu, China
- 3Department of Gynecology, Zhengzhou Second Hospital, Zhengzhou, China
- 4Guangzhou First People’s Hospital, Guangzhou, China
- 5Department of Laboratory Medicine, The First Affiliated Hospital of Guangzhou University of Chinese Medicine, Guangzhou, China
- 6Department of Ultrasound, The First Affiliated Hospital of Guangzhou University of Chinese Medicine, Guangzhou, China
Background: In recent years, there has been considerable growth in abnormal inflammatory reactions and immune system dysfunction, which are implicated in chronic inflammatory illnesses and a variety of other conditions. Dietary fibers have emerged as potential regulators of the human immune and inflammatory response. Therefore, this study aims to investigate the associations between dietary fibers intake and systemic immune and inflammatory biomarkers.
Methods: This cross-sectional study used data from the National Health and Nutrition Examination Survey (2015–2020). Dietary fibers intake was defined as the mean of two 24-h dietary recall interviews. The systemic immune-inflammation index (SII), systemic inflammation response index (SIRI), neutrophil-to-lymphocyte ratio (NLR), platelet-lymphocyte ratio (PLR), red blood cell distribution width-to-albumin ratio (RA), ferritin, high-sensitivity C-reactive protein (hs-CRP), and white blood cell (WBC) count were measured to evaluate systemic immune and inflammatory states of the body. The statistical software packages R and EmpowerStats were used to examine the associations between dietary fibers intake and systemic immune and inflammatory biomarkers.
Results: Overall, 14,392 participants were included in this study. After adjusting for age, gender, race, family monthly poverty level index, alcohol consumption, smoking status, vigorous recreational activity, body mass index, hyperlipidemia, hypertension, diabetes, and dietary inflammatory index, dietary fibers intake was inversely associated with SII (β = −2.19885, 95% CI: −3.21476 to −1.18294, p = 0.000248), SIRI (β = −0.00642, 95% CI: −0.01021 to −0.00263, p = 0.001738), NLR (β = −0.00803, 95% CI: −0.01179 to −0.00427, p = 0.000284), RA (β = −0.00266, 95% CI: −0.00401 to −0.00131, p = 0.000644), ferritin (β = −0.73086, 95% CI: −1.31385 to −0.14787, p = 0.020716), hs-CRP (β = −0.04629, 95% CI: −0.0743 to −0.01829, p = 0.002119), WBC (β = −0.01624, 95% CI: −0.02685 to −0.00563, p = 0.004066), neutrophils (β = −0.01346, 95% CI: −0.01929 to −0.00764, p = 0.000064). An inverse association between dietary fibers and PLR was observed in the middle (β = −3.11979, 95% CI: −5.74119 to −0.4984, p = 0.028014) and the highest tertile (β = −4.48801, 95% CI: −7.92369 to −1.05234, p = 0.016881) and the trend test (βtrend = −2.2626, 95% CI: −3.9648 to −0.5604, Ptrend = 0.0150). The observed associations between dietary fibers intake and SII, SIRI, NLR, RA, ferritin, hs-CRP, WBC, and neutrophils remained robust and consistent in the sensitivity analysis. No significant interaction by race was found.
Conclusion: Dietary fibers intake is associated with the improvement of the parameters of the immune response and inflammatory biomarkers, supporting recommendations to increase dietary fibers intake for enhanced immune health.
Introduction
In recent decades, there has been a significant increase in abnormal inflammatory responses and immune system dysfunction, contributing to the development of chronic inflammatory disorders, as well as conditions such as cancer and diabetes (1–3). Therefore, the identification of potential regulators of inflammation and the immune system holds great significance in preventing and treating these diseases. It is well-established that changes in dietary factors play a crucial role in regulating immune function and inflammatory biomarkers (4). Both preclinical and clinical studies provide compelling evidence that a dietary shift from traditional diets abundant in plant-based foods to ultra-processed foods renders individuals susceptible to various chronic and debilitating inflammatory diseases (5, 6). Consequently, the influence of dietary nutrients on immune and inflammatory responses has emerged as an attractive and vital area of research. This study will specifically focus on one such dietary component: dietary fibers.
Dietary fibers are complex dietary components found mainly in grains, vegetables, and fruits that consist of three or more monomeric units (7, 8). These fibers are indigestible in the intestinal tract, but they play a unique and important role in the human body. Higher dietary fibers intake has been reported to improve immune responses and certain inflammatory disorders (8). In vitro and in vivo experiments have identified that dietary fibers impact immune cells through gut microbiota and may help prevent inflammatory conditions (9). More specifically, clinical studies suggest that dietary fibers act as protective factors against asthma (10), metabolic syndrome (11, 12), and radiation-induced gastrointestinal toxicity (13). Beyond diseases, a variety of immune and inflammatory biomarkers such as C-reactive protein, fibrinogen (14), tumor necrosis factor-α, and interleukin-10 (15) are associated with dietary fibers intake.
The systemic immune-inflammation index (SII) was first proposed by Hu et al. (16) as a prognostic predictor for hepatocellular carcinoma patients (16). However, the clinical interest in SII has grown significantly due to its ability to reflect systemic inflammation and immunity. Previous studies have established strong associations between SII and various diseases, including cancer (17), diabetes (18), hepatic steatosis (19), kidney injury (20), and cardiovascular risk (21). Similarly, the systemic inflammation response index (SIRI) was initially developed to predict the prognosis of pancreatic cancer, with higher levels of SIRI being linked to unfavorable prognostic outcomes (22). The neutrophil-to-lymphocyte ratio (NLR) and platelet-lymphocyte ratio (PLR) are calculated based on blood cell count and have been widely recognized as potential indicators for early diagnosis and prognosis monitoring in inflammatory diseases and cancers (23). Additionally, Red blood cell distribution width-to-albumin ratio (RA) has emerged as a novel inflammatory biomarker, showing associations with conditions such as stroke (24), diabetic ketoacidosis (25), acute respiratory distress syndrome (26), and all-cause mortality in cancer patients (27). Ferritin and high-sensitivity C-reactive protein (hs-CRP) are classical inflammatory biomarkers extensively used in routine clinical practice and inflammatory research.
Consequently, it has been established with certainty that these biomarkers can serve as reliable indicators of the immune and inflammatory condition of the human body, and they are correlated with various diseases that pose a threat to health. However, few studies have delved into whether these biomarkers can be modulated by dietary fibers. This study aimed to analyze the association between dietary fibers intake and systemic immunity and inflammation using data from the National Health and Nutrition Examination Survey (NHANES) survey conducted from 2015–2020.
Materials and methods
Study population
The NHANES is an epidemiological program developed to assess the health and nutritional conditions of adults and children in the United States. Conducted by the National Center for Health Statistics, a subdivision of the Centers for Disease Control and Prevention, NHANES combines interviews on demographic, socioeconomic, dietary, and health-related queries, physical examinations incorporating medical, dental, physiological measurements, and laboratory tests by highly qualified medical personnel. NHANES sample constitutes a representation of the noninstitutionalized civilian population in the United States, comprising the 50 states and the District of Columbia. From 1999 onwards, the sample design has employed a multi-year, stratified, clustered four-stage sampling approach, with data release in 2-year cycles.
This study included NHANES data from 2015–2020. A total of 20,520 participants remained after excluding those younger than 20. We further excluded those lacking systemic immune-inflammation index (SII) or dietary fibers intake data, leaving 14,392 participants for the association analysis. In order to perform a sensitivity analysis with complete cases, 6,526 participants with incomplete data in any kind of variable were excluded. A flowchart presents the process of selecting participants (Figure 1).
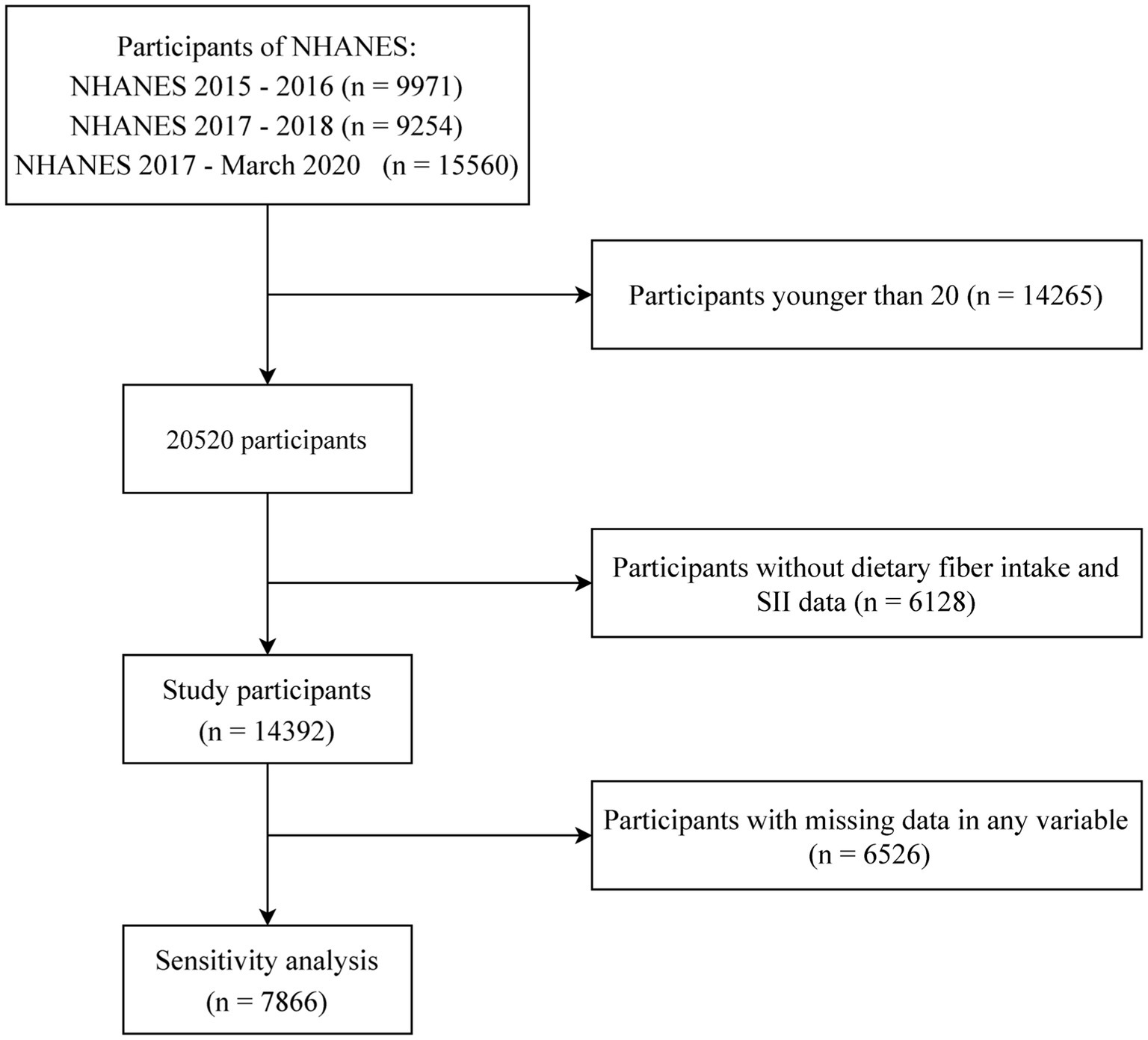
Figure 1. Participant screening flowchart based on age, dietary fibers intake, systemic immune/inflammatory biomarkers, and sensitivity analyses.
Measurement of dietary fibers intake
Dietary intake data was collected through two 24-h dietary recalls conducted 3–10 days apart during the Mobile Examination Center component of NHANES. The recalls were jointly processed by NHANES, the United States Department of Agriculture, and the United States Department of Health and Human Services. Average daily dietary fibers intake was calculated using the two 24-h of intake data. Full documentation of the dietary assessment methods is available in the NHANES dietary interviewer procedures manuals (28, 29).
Measurement of primary and secondary outcomes
The primary outcome was the SII, calculated as: platelet counts × neutrophil count/lymphocyte count (16). SIRI, NLR, PLR, RA, ferritin, hs-CRP, and six kinds of white blood cell (WBC) count are the secondary outcomes of this study. The formulas for SIRI, NLR, PLR and RA are presented as follows: SIRI = neutrophil count × monocyte/lymphocyte count. (22), NLR = neutrophil counts/lymphocyte counts, PLR = platelet counts/lymphocyte counts, and RA = red blood cell distribution width (%)/albumin (mg/dl) (30). Ferritin and hs-CRP are well-acknowledged acute inflammation indicators obtained using blood specimen tests. NHANES provides standardized protocols for measuring these biomarkers, available on the NHANES website1 (31).
Selection of covariates
Sociodemographic characteristics included age, gender (male and female), race (Mexican American, other Hispanic, non-Hispanic white, non-Hispanic black, and other), and family monthly poverty level index (≤1.3, 1.5–1.85, >1.85) were collected. Lifestyle characteristics included alcohol consumption (never, mild, moderate, and heavy), smoking status (never, former, and current), and vigorous recreational activity (Yes and No) were obtained. Never drinkers were ascertained by the questionnaire: “Ever had a drink of any kind of alcohol?” Furthermore, participants who had >4 drinks per day, 3–4 drinks per day, and up to 2 drinks per day were classified as heavy, moderate, and mild drinkers, respectively. Participants who smoked less than 100 cigarettes in life were considered as never smoking and the others were divided into former and current smokers according to the question “Do you now smoke cigarettes?” Metabolic characteristics included body mass index (BMI), hyperlipidemia, hypertension, and diabetes. An adult with a BMI below 18.5 kg/m2 is considered underweight, 18.5 to 24.9 is considered normal weight, 25 to 29.9 is considered overweight, and 30 or above is considered obesity. Hyperlipidemia was defined by high-density lipoprotein cholesterol <1.0 mmoL/L in men, < 1.3 mmoL/L in women, or triglycerides ≥1.8 mmoL/L regardless of gender. Hypertension was defined as systolic blood pressure ≥ 130 mmHg and/or diastolic blood pressure ≥ 80 mmHg on ≥3 occasions. Moreover, participants who take an anti-hypertensive agent or who answered “yes” to the questions: “Are you now taking prescribed medicine for high blood pressure?” and “Ever told you had high blood pressure?” were also defined as having hypertension. Diabetes was defined as a positive response to the question “Doctor told you have diabetes?.” Additionally, participants who achieved one or more of the following conditions were diagnosed with diabetes: glycohemoglobin ≥6.5%, fasting glucose ≥7 mmol/L, two-hour glucose of oral glucose tolerance test, or serum glucose ≥11.1 mmol/L. The dietary inflammatory index (DII) is a scoring algorithm developed through comprehensive analysis of scientific literature on the inflammatory properties of dietary components. The DII was used to categorize participants’ dietary patterns as either pro-inflammatory or anti-inflammatory (32).
Statistical analyses
Dummy variables were used to denote missing covariate values. Continuous variables were presented as survey-weighted mean (95% confidence interval (CI)) and categorical variables were expressed as survey-weighted percentage (95% CI). The weighted χ2 test (categorical variable) and weighted linear regression model (continuous variable) compared tertiles of dietary fibers intake. A univariate and multivariate weighted linear regression model and/or weighted binary logistic regression model were used to examine the associations between dietary fibers intake and SII, as well as other outcomes. A total of three statistical models were constructed in each regression analysis. Model I was the non-adjusted model with no covariates adjusted. Model II was the minimally adjusted model with age and gender adjusted. Model III was a fully adjusted with age, gender, race, family monthly poverty level index, alcohol consumption, smoking status, vigorous recreational activities, BMI, hyperlipidemia, hypertension, diabetes and DII adjusted. The fully adjusted model took into account demographic factors, lifestyle factors, dietary factors, and metabolic factors. Covariates were selected by referring to cross-sectional studies related to our prespecified outcome indicators (33–36). Sensitivity analysis was conducted by excluding the participants with incomplete data in covariates. Taking the biochemical markers between human races into consideration (37), a subgroup analyses between races were performed using a stratified logistic regression model and a interaction test for effect modification for different races were followed by the likelihood-ratio test. Data analysis was performed with the statistical software packages R2 and EmpowerStats (http://www.empowerstats.com, X&Y Solutions, Inc., Boston, MA). All statistical tests were two-sided, and a p value <0.05 was considered statistically significant.
Results
Baseline characteristics
Table 1 shows baseline of the 14,392 participants by dietary fiber intake tertiles (low: 0–11.65 g/d, n = 4,790; middle: 11.7–18.45 g/d, n = 4,801; high: 18.5–89.55 g/d, n = 4,801). Participants with higher dietary fibers intake had lower levels of SII, SIRI, NLR, RA, hs-CRP, WBC, neutrophils and basophils. Furthermore, these individuals also exhibited exhibited a higher proportion of male and Mexican American participants, greater affluence, lower prevalence of obesity, and healthier lifestyle reflected by increased engagement in rigorous recreational activities, decreased usage of cigarettes and alcohol, and a higher percentage of adherence to an anti-inflammatory diet.
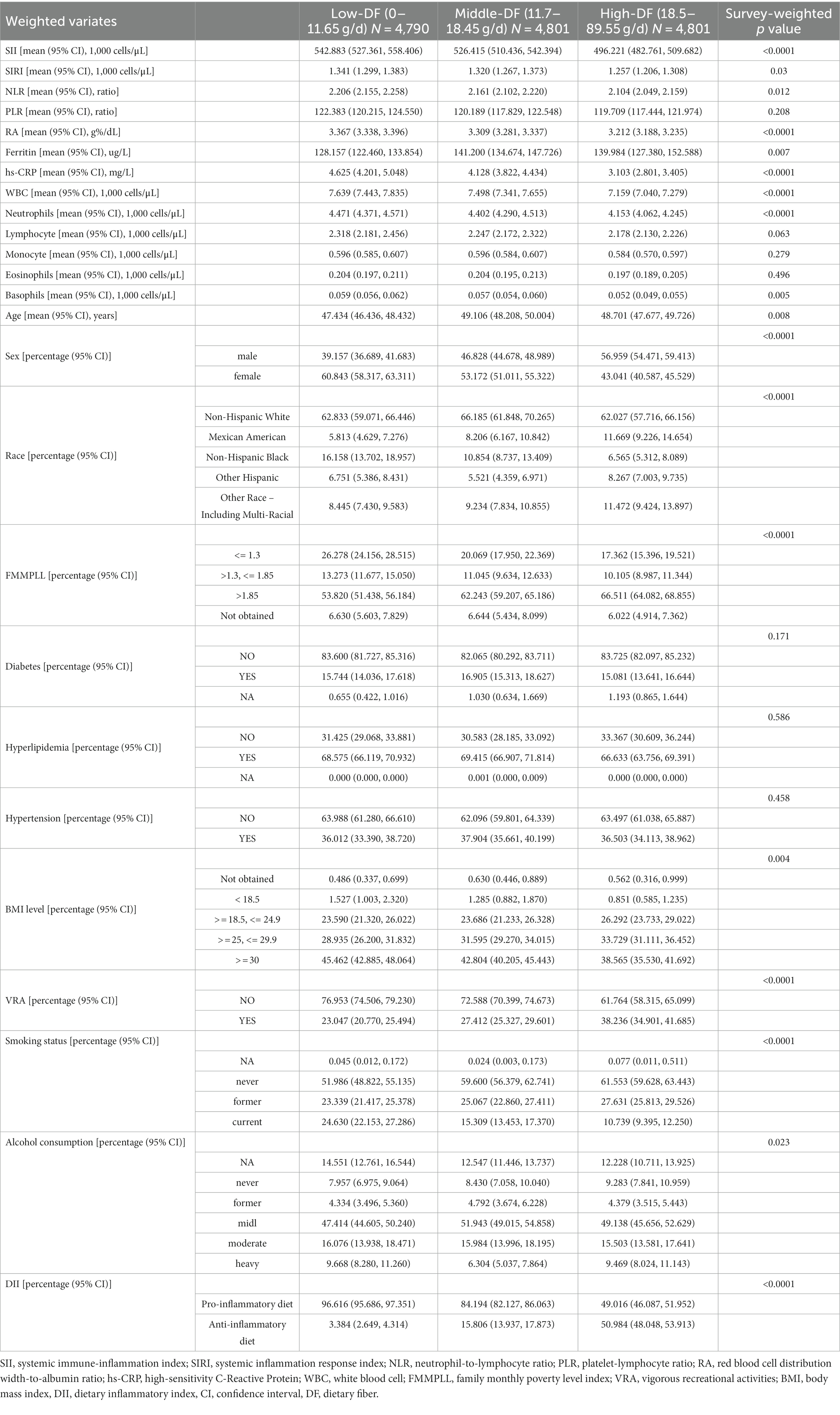
Table 1. Survey-weighted baseline characteristics by dietary fibers intake level in the study population.
Associations between dietary fibers intake and SII, SIRI, NLR, and PLR
Dietary fibers intake shows significant inverse associations with SII, SIRI, NLR in all 3 models (Table 2). The effect size (β) and 95% confidence interval (CI) for SII in the fully-adjusted model are −2.19885 (−3.21476, −1.18294) and the highest tertile significantly associated with decreased SII (β = −43.29833, 95% CI: −67.46845 to −19.12821, p = 0.001073). The p for trend across dietary fibers intake categories reaches statistical significance (βtrend = −21.5411, 95% CI: −33.0049 to −10.0772, Ptrend = 0.0011).
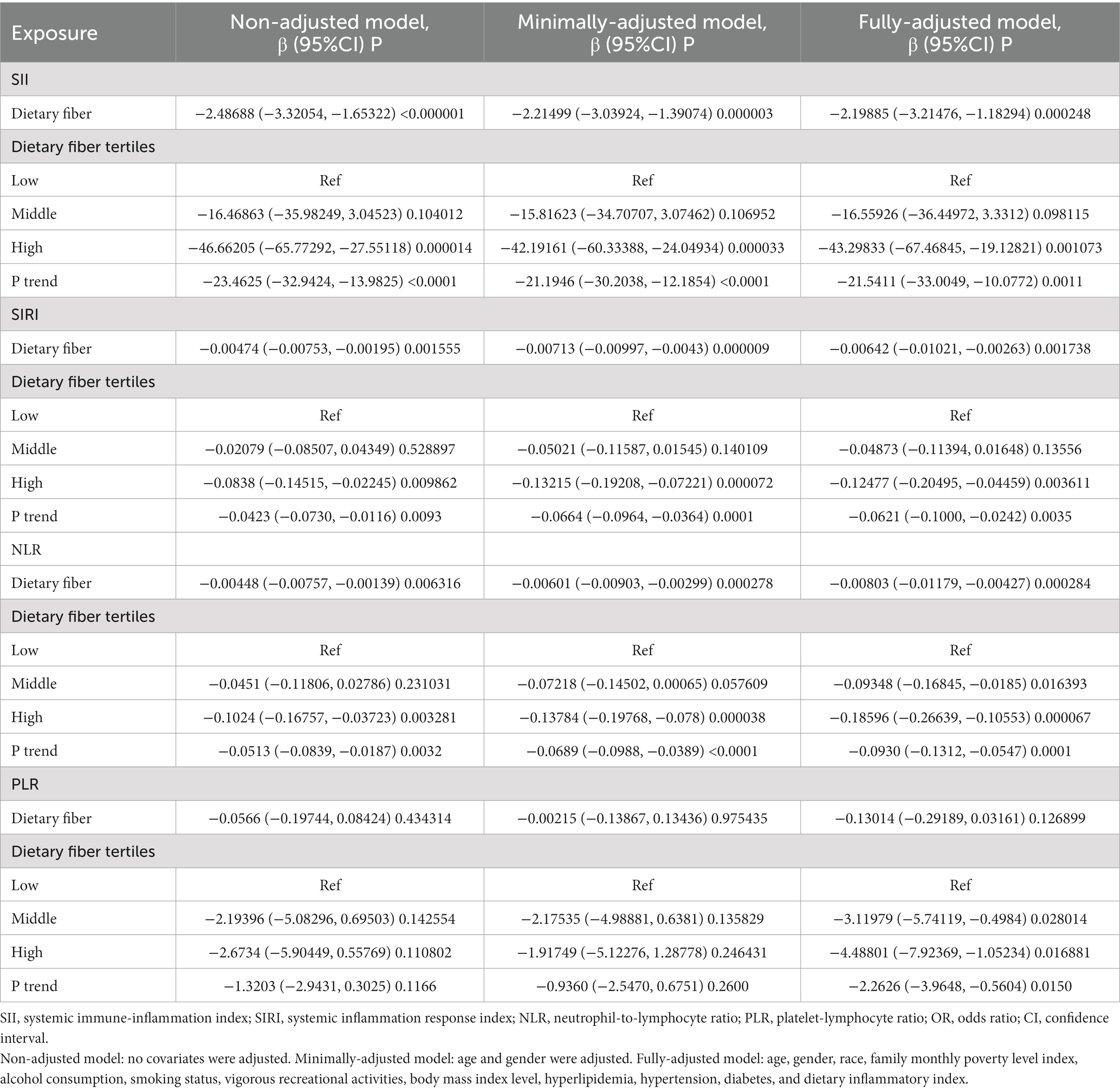
Table 2. Survey-weighted univariate and multivariate regression analyses of associations between dietary fibers intake and SII, SIRI, NLR, and PLR.
The β and 95% CI for SIRI in the fully-adjusted model are −0.00642 (−0.01021, −0.00263) and the highest tertile significantly associated with decreased SIRI (β = −0.12477, 95% CI: −0.20495 to −0.04459, p = 0.003611). The p for trend across dietary fibers intake categories reaches statistical significance (βtrend = −0.0621, 95% CI: −0.1000 to −0.0242, Ptrend = 0.0035).
The β and 95% CI for NLR in the fully-adjusted model are −0.00803 (−0.01179, −0.00427) and the highest tertile significantly associated with decreased NLR (β = −0.18596, 95% CI: −0.26639 to −0.10553, p = 0.000067). The p for trend across dietary fibers intake categories reaches statistical significance (βtrend = −0.0930, 95% CI: −0.1312 to −0.0547, Ptrend = 0.0001).
The β and 95% CI for PLR in the fully-adjusted model are −0.13014 (−0.29189, 0.03161) and the highest tertile significantly associated with decreased PLR (β = −4.48801, 95% CI: −7.92369 to −1.05234, p = 0.016881). The P for trend across dietary fibers intake categories reaches statistical significance (βtrend = −2.2626, 95% CI: −3.9648 to −0.5604, Ptrend = 0.0150).
Associations between dietary fibers intake and RA
Dietary fibers intake presents significant inverse associations with RA (β = −0.00266, 95% CI: −0.00401 to −0.00131, p = 0.000644). The β and 95% CI for the highest tertile is −0.07064 (−0.010227, −0.03901) in the fully-adjusted model. A significant negative trend is observed across dietary fiber intake categories (βtrend = −0.0351, 95% CI: −0.0503 to −0.0199, Ptrend = 0.0001). The β and corresponding 95% CI for all the statistical models are presented in Table 3.
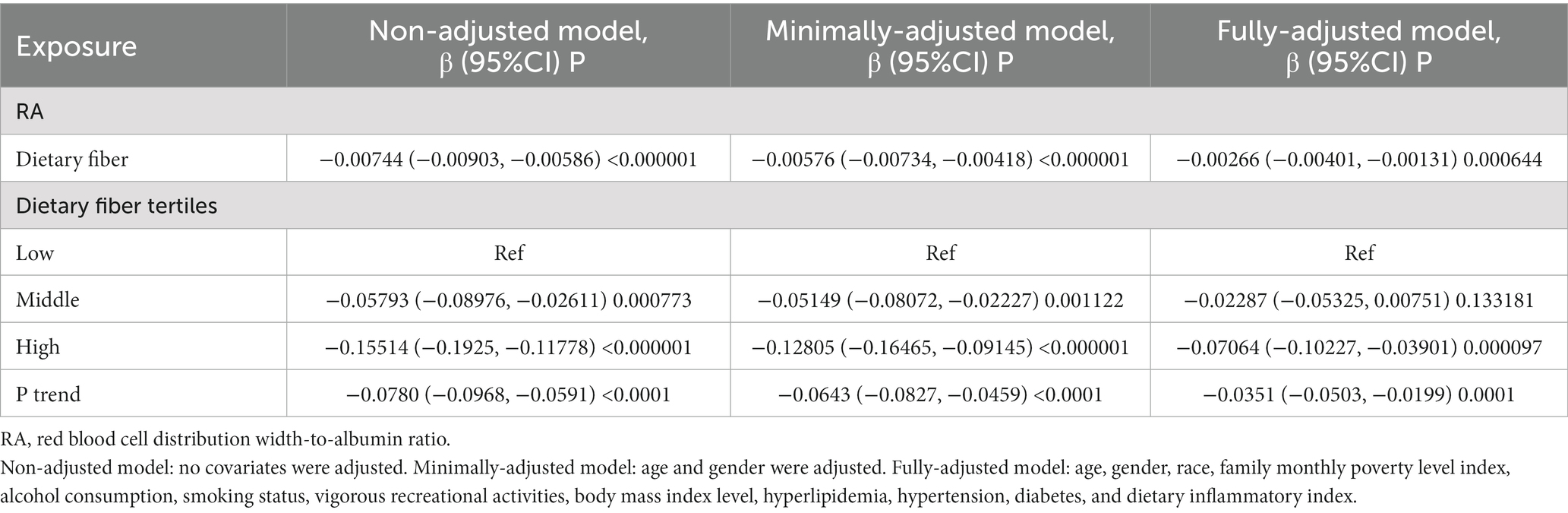
Table 3. Survey-weighted univariate and multivariate regression analyses of the association between dietary fibers intake and RA.
Associations between dietary fibers intake and ferritin and hs-CRP
An inverse association is observed between dietary fibers intake and ferritin (β = −0.73086, 95% CI: −1.31385 to −0.14787, p = 0.020716). However, when we stratified dietary fibers into tertiles, statistical significance was not attained in any tertile or across tertiles (Table 4). Dietary fibers intake also shows an inverse correlation with hs-CRP (β = −0.04629, 95% CI: −0.0743 to −0.01829, p = 0.002119), with the highest tertile significantly associated with decreased hs-CRP (β = −0.8598, 95% CI: −1.49918 to −0.22043, p = 0.010218) and the P for trend across dietary fibers intake categories reaches statistical significance (βtrend = −0.4261, 95% CI: −0.7299 to −0.1224, Ptrend = 0.0105).
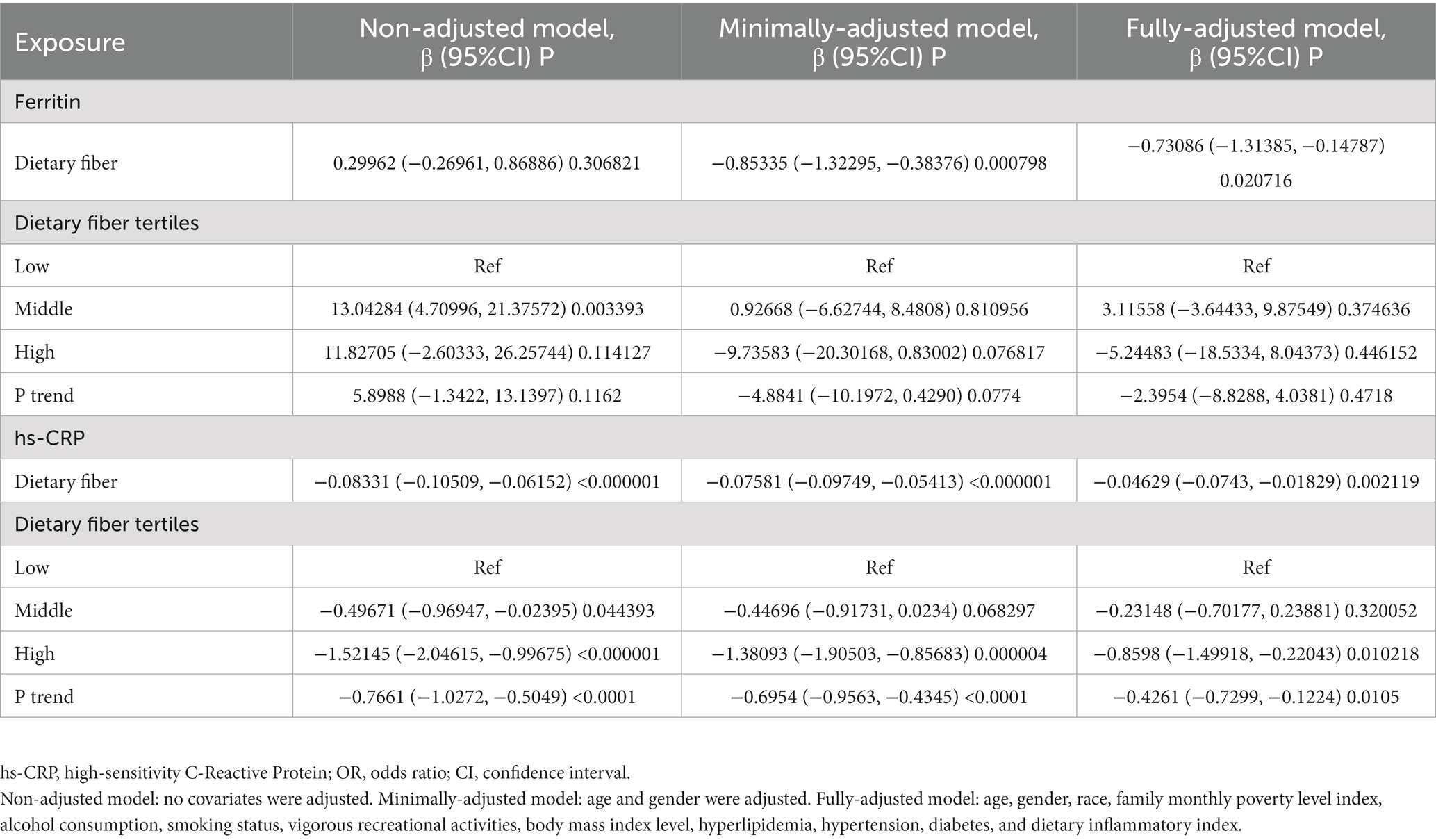
Table 4. Survey-weighted univariate and multivariate regression analyses of the associations between dietary fibers intake and ferritin and hs-CRP.
Associations between dietary fibers intake and white blood cell
Significant inverse correlations are observed between dietary fibers intake and WBC (β = −0.01624, 95% CI: −0.02685 to −0.00563, p = 0.004066; βtrend = −0.1268, 95% CI: −0.2277 to −0.0258, Ptrend = 0.0209), and neutrophils (β = −0.01346, 95% CI: −0.01929 to −0.00764, p < 0.000064; βtrend = −0.1047, 95% CI: −0.1641 to −0.0453, Ptrend = 0.0019) (Table 5). However, no significant associations are seen with lymphocytes, monocytes, eosinophils or basophils.
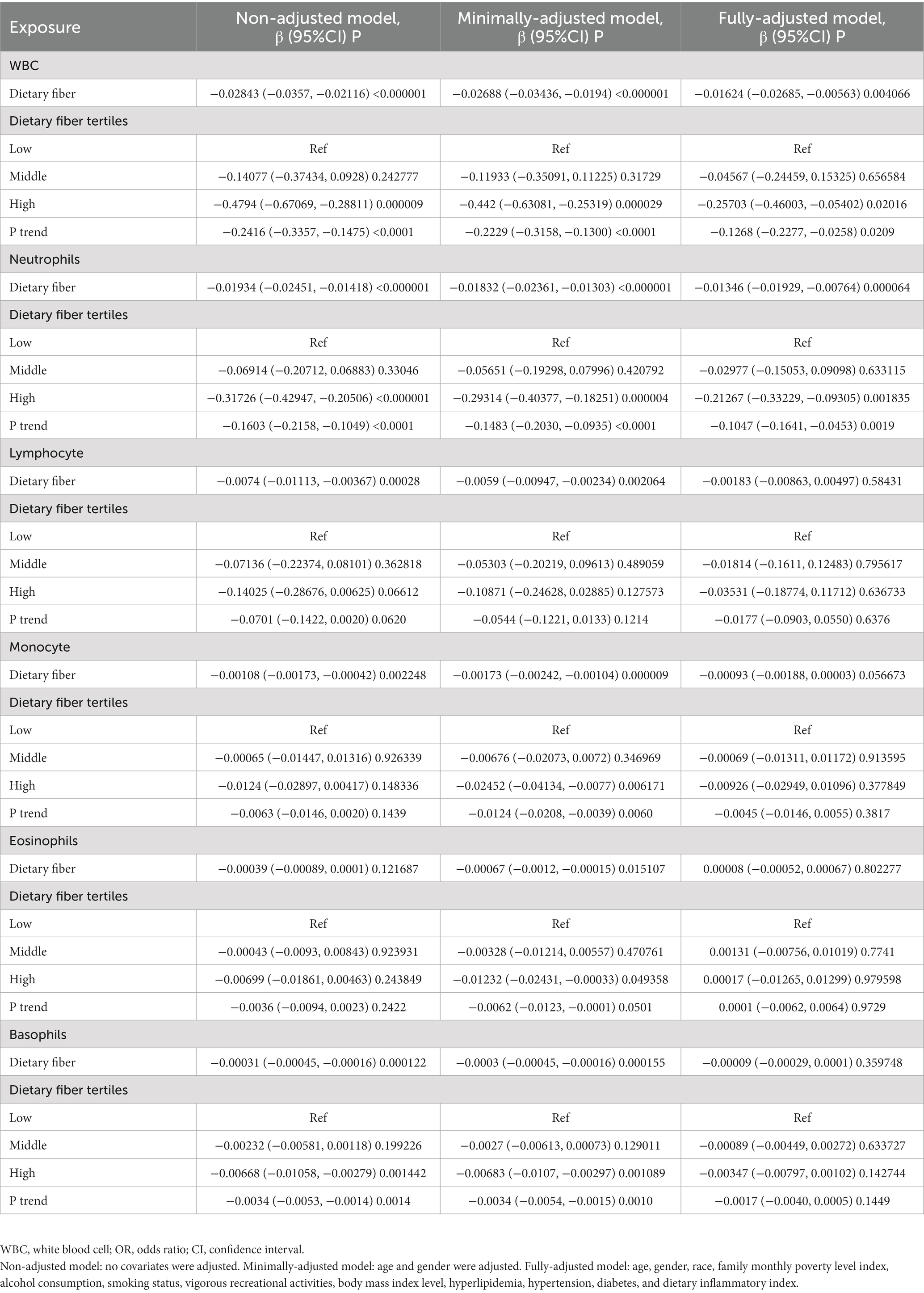
Table 5. Survey-weighted univariate and multivariate regression analyses of the association between dietary fibers intake and WBC.
Sensitivity analysis of complete cases for SII
Sensitivity analysis continues to show an inverse association between dietary fibers intake and SII (Table 6). The β and 95% CI are −2.0099 (−3.08293, −0.93687) for the non-adjusted model, −1.7928 (−2.85182, −0.73377) for the minimally-adjusted model, and − 1.59067 (−3.09644, −0.08491) for fully-adjusted model. The highest tertile (β = −34.10908, 95% CI: −65.05815 to −3.16001, p = 0.03861) is significantly associated with decreased SII in fully-adjusted model. The P for trend across dietary fibers intake categories reaches statistical significance (βtrend = −17.2185, 95% CI: −32.7649 to −1.6721, Ptrend = 0.0375). Additionally, robust inverse associations are also observed between dietary fibers intake and SIRI, NLR, RA, ferritin, hs-CRP, WBC, and neutrophils (Supplementary Table S1).
Interaction effect of race on the associations between dietary fibers intake and outcomes
Interaction tests showed no significant difference in the associations between dietary fibers intake and systemic immune and inflammatory biomarkers by race (Table 7). The Pinteraction for race and SII, SIRI, NLR, PLR, RA, ferritin, hs-CRP, and six kinds of WBC count were 0.9941, 0.9085, 0.9054, 0.0495, 0.6856, 0.476, 0.1873, 0.3227, 0.1548, 0.2794, 0.2081, 0.659 and 0.6209.
Discussion
This study conducted a comprehensive cross-sectional investigation using data from the NHANES 2015–2020 survey, which represents the U.S. population, to explore the association between dietary fibers intake and systemic immune and inflammatory biomarkers. The results of our study indicate that dietary fibers intake is inversely associated with SII, SIRI, NLR, RA, hs-CRP, WBC, and neutrophils. Furthermore, the sensitivity analysis confirmed the robustness of these findings. To the best of our knowledge, this is the initial investigation to evaluate such associations within a nationally representative sample.
SII, SIRI, NLR, and RA are potent biomarkers of the body’s immune and inflammatory state and have demonstrated predictive value for a wide range of diseases. The role of dietary factors as potential regulators of these biomarkers is evident in the literature review. In a case–control study involving 527 participants, dietary inflammation levels in women with polycystic ovary syndrome showed a positive correlation with SII, NLR, and PLR (38). Similarly, in a cross-sectional study with 1,050 participant, dietary inflammation level was positively associated with SIRI in individuals with mild cognitive impairment (39). Another study revealed a negative correlation between dietary antioxidant capacity and NLR in cancer patients (40). Additionally, a retrospective study found that dietary omega- 6 to omega- 3 fatty acids was associated with reduced PLR level in men with chronic coronary syndrome. (41). Our study unveiled an inverse association between dietary fibers and SII, SIRI, NLR, and RA, suggesting that a high-fiber diet may help regulate these biomarkers and potentially benefit the immune system.
Ferritin, initially identified as a reactant of acute inflammation caused by infectious agents, has subsequently been linked to acute and chronic inflammatory conditions precipitated by non-infectious sources. Moreover, it has been been demonstrated to play a pivotal role in the pathogenesis of various inflammatory and autoimmune diseases (42, 43). The rapid elevation in serum ferritin levels at the onset of viral or bacterial infections renders it a sensitive biomarker with clinical utility (44). However, it takes up to 5 weeks for ferritin levels to decrease (45). Elevated ferritin levels have been shown to be associated with autoimmune diseases such as rheumatoid arthritis, systemic lupus erythematosus, and multiple sclerosis, in which ferritin predicts disease severity or contributes to disease development (46–48). In vitro experiments have shown that Low Phytate Peas containing dietary fibers can affect hepatic ferritin concentrations (49). However, the relationship between dietary fibers intake and ferritin is controversial in clinical and cross-sectional studies. A prospective, randomized, placebo-controlled clinical trial conducted in China on end-stage renal disease patients treated with dietary fibers (composed of galactomannan, resistant dextrin, fructooligosaccharide, and starch) or potato starch for 8 weeks showed that the patients in the dietary fibers group had higher serum ferritin levels (50). Another randomized, double-blind, placebo-controlled with 32 female athletes demonstrated that daily synbiotic supplement along with Fe supplementation increased serum ferritin levels (51). However, some studies have come to the opposite conclusion. In a crossover-design clinical trial, healthy participants who took a high-fiber snack for 6 weeks and maintained it with a low-fiber snack for 6 weeks had lower ferritin levels compared to the control group (52). A French epidemiological survey study that included 4,358 subjects also found a negative association between dietary fibers intake and serum ferritin levels (53). In addition, vegans with high-fiber diets have been found to have low ferritin levels in several dietary investigations (54). Although the fully adjusted model indicated that dietary fibers intake was negatively associated with ferritin levels, there was no statistical difference in the analysis of the trend test, making the relationship between dietary fibers intake and ferritin unstable in our study. Hs-CRP is a biomarker of systemic inflammation in the body, in addition to being regarded as an indicator of acute inflammation, it is associated with many chronic diseases, including coronary heart disease (55), metabolic syndrome (56), diabetes mellitus (57), and cancer (58). Evidence of an inverse correlation between the dietary fibers intake and hs-CRP concentrations has emerged from multiple cohort studies conducted on the American population. Two cross-sectional analyses of NHANES data from 1999–2000 included 3,920 and 4,900 participants, respectively (59, 60). Concurrently, a longitudinal cohort study involving 524 healthy adults (61) and a small clinical trial have been conducted (62). A parallel dietary intervention trial has demonstrated that incorporating high-fiber wholegrain rye foods with added fermented rye bran led to a reduction in hs-CRP levels among Chinese adults (63). However, no association between dietary fibers intake and hs-CRP was seen among postmenopausal women in a cross-sectional study of 1958 participants (64). Our current cross-sectional study, which boasts the largest sample size to date, aligns with prior research findings.
Dietary fibers constitute essential components of human nutrition. The Institute of Medicine stipulates a daily recommended intake of 30.8 g for males aged 31–50 and 25 g for females aged 31–50 (65). However, the European Food Safety Authority advocates for a higher intake range of 25–38 g/day to mitigate risks associated with type 2 diabetes, cardiovascular disease, colorectal cancer, overweight, and obesity (66). It is evident that a significant portion of participants in this study fail to meet the recommended dietary fibers intake.
Dietary fibers have demonstrated both direct or indirect protective effects on the immune system in in vivo, in vitro and population-based study. Despite their lack of digestion or absorption in the intestinal tract, dietary fibers are regarded as vital fuel sources for gut microbiota (67). The gut microbiota, such as Clostridium, Bacteroides, Bifidobacterium, Prevotella, and Ruminococcus (3), can ferment dietary fibers and produce a variety of metabolites associated with immune system and inflammation, the most pivotal of which are short-chain fatty acids (SCFAs) (68). Cellular experiments have illustrated that SCFAs can function as inhibitors of histone deacetylases and as ligands for G-protein-coupled receptors and aryl hydrocarbon receptors, impacting various physiological processes including immunophysiology (69–72). Previous studies have demonstrated the ability of SCFAs to affect immune niches in the lungs, intestines, and other organs of the host. Lung dendritic cells in propionate-treated mice displayed high phagocytic capacity but impaired promotion of T helper type 2 cell effector function, owing to SCFA-induced alterations in bone marrow hematopoiesis leading to increased macrophage and dendritic cell precursors (72). Lung Type 2 innate lymphoid cells (ILC2s)-driven airway hyperreactivity and inflammation were ameliorated by systemic or intranasal SCFA butyrate administration in mice, likely through histone deacetylase inhibition suppressing ILC2 proliferation, GATA3 expression, and cytokine production; similar SCFA butyrate effects were confirmed in human ILC2s (73). For intestinal immunity, in vitro SCFA treatment of human intestinal epithelial cells enhances the epithelial barrier and dampens immune responses via increased IL-10RA (74), while SCFA binding to GPR43 on colonocytes stimulates potassium (K+) efflux and hyperpolarization, activating the NLRP3 inflammasome and protecting intestinal epithelial integrity (75). Beyond SCFAs production, recent studies suggests that dietary fibers have direct effect on the epithelial cells and immune cells in the gastrointestinal tract. In vitro studies show dietary fibers can directly attenuate inflammatory cytokine production from dendritic cells co-cultured with intestinal epithelial supernatants, dependent on fiber interactions with Toll-like receptors. Specific fibers differentially modulate T cell responses and regulatory T cell cytokines. β-Glucan protects intestinal epithelial barrier integrity during Salmonella infection by preserving tight junctions and limiting invasion. Additionally, some fibers elicit cytokine secretion from intestinal epithelial cells through MyD88/TLR4 signaling (76–78). These findings demonstrate dietary fiber interactions with intestinal immune and epithelial cells regulate inflammatory responses and barrier function via pattern recognition receptor pathways. In addition to in vivo and in vitro evidence, prospective cohort studies have indicated that the early consumption of dietary fibers may assist in decreasing the chances of allergies and asthma in adulthood (79). Likewise, high fiber maternal diets during pregnancy are linked to lower risk of allergic diseases like rhinitis and eczema in offspring (80). A cross-section study based on NHANES data conducted in adults indicates that high-fiber diet may reduce the serum CRP level and decrease odds of having asthma (10).
This study had several advantages. It pioneers the identification of the association between dietary fiber intake and systemic immune and inflammatory states, with the SII serving as the primary indicator. Leveraging a substantial and representative sample from NHANES, the study employs a comprehensive array of indicators to gauge systemic immune and inflammatory status. Nevertheless, the study had some limitations. A cross-sectional study design is incapable of determining the causality and is unable to remove the insidious residual confusing results from unmeasured or unidentified confounding factors. Despite our adjustments to DII, the confounding effects of the anti-inflammatory component of the diet such as vitamins, flavonoids, and other substances could not be completely eliminated. The study’s reliance on dietary fiber intake data from just two 24-h dietary reviews introduces a potential limitation, as dietary preferences naturally fluctuate from day to day, potentially impacting the precision of the assessment. Furthermore, short-term dietary assessments are not considered to be an accurate representation of a participant’s true dietary intake and the recall bias in dietary questionnaire was difficult to evaluate.
The current study has the following implications for future research. Our findings provide evidence for a negative correlation between dietary fibers intake and systemic immunity and inflammation biomarkers, which highlights the potential therapeutic role of dietary fibers in immune and inflammatory diseases. Therefore, well-designed randomized controlled trials or prospective cohort studies with long-term follow-up are warranted to further evaluate dietary fiber intake as an intervention or exposure, respectively. The relationship between ferritin and dietary fibers remains a matter of debate, and further exploration of their association in populations with varying disease states is essential to elucidate the nature of their relationship.
Conclusion
Dietary fibers intake is inversely associated with systemic immune and inflammatory biomarkers in the human body. The associations persisted in the sensitivity analysis. Thus, dietary fibers should be recommended to promote immune health.
Data availability statement
The original contributions presented in the study are included in the article/Supplementary material, further inquiries can be directed to the corresponding author.
Ethics statement
The studies involving humans were approved by National Health and Nutrition Examination Survey. The studies were conducted in accordance with the local legislation and institutional requirements. The participants provided their written informed consent to participate in this study.
Author contributions
XQ designed the study. XQ, CF, YL, and YJ collected the data. XQ, YL, MC, XC, and JJ analyzed the data and drafted the manuscript. JJ revised and approved the final version of the manuscript. All authors contributed to the article and approved the submitted version.
Acknowledgments
The authors would like to express their appreciation to Chi Chen for his guidance on statistics.
Conflict of interest
The authors declare that the research was conducted in the absence of any commercial or financial relationships that could be construed as a potential conflict of interest.
Publisher’s note
All claims expressed in this article are solely those of the authors and do not necessarily represent those of their affiliated organizations, or those of the publisher, the editors and the reviewers. Any product that may be evaluated in this article, or claim that may be made by its manufacturer, is not guaranteed or endorsed by the publisher.
Supplementary material
The Supplementary material for this article can be found online at: https://www.frontiersin.org/articles/10.3389/fnut.2023.1242115/full#supplementary-material
Footnotes
References
1. Zhao, H, Wu, L, Yan, G, Chen, Y, Zhou, M, Wu, Y, et al. Inflammation and tumor progression: signaling pathways and targeted intervention. Signal Transduct Target Ther. (2021) 6:263. doi: 10.1038/s41392-021-00658-5
2. Leuti, A, Fazio, D, Fava, M, Piccoli, A, Oddi, S, and Maccarrone, M. Bioactive lipids, inflammation and chronic diseases. Adv Drug Deliv Rev. (2020) 159:133–69. doi: 10.1016/j.addr.2020.06.028
3. Venter, C, Meyer, RW, Greenhawt, M, Pali-Schöll, I, Nwaru, B, Roduit, C, et al. Role of dietary fiber in promoting immune health-an EAACI position paper. Allergy. (2022) 77:3185–98. doi: 10.1111/all.15430
4. Sánchez-Rosales, AI, Guadarrama-López, AL, Gaona-Valle, LS, Martínez-Carrillo, BE, and Valdés-Ramos, R. The effect of dietary patterns on inflammatory biomarkers in adults with type 2 diabetes mellitus: a systematic review and meta-analysis of randomized controlled trials. Nutrients. (2022) 14:4577. doi: 10.3390/nu14214577
5. Srour, B, Kordahi, MC, Bonazzi, E, Deschasaux-Tanguy, M, Touvier, M, and Chassaing, B. Ultra-processed foods and human health: from epidemiological evidence to mechanistic insights. Lancet Gastroenterol Hepatol. (2022) 7:1128–40. doi: 10.1016/S2468-1253(22)00169-8
6. Elliott, PS, Kharaty, SS, and Phillips, CM. Plant-based diets and lipid, lipoprotein, and inflammatory biomarkers of cardiovascular disease: a review of observational and interventional studies. Nutrients. (2022) 14:5371. doi: 10.3390/nu14245371
7. Jones, JM. CODEX-aligned dietary fiber definitions help to bridge the 'fiber gap'. Nutr J. (2014) 13:34. doi: 10.1186/1475-2891-13-34
8. Islam, MA, Khandker, SS, Kotyla, PJ, and Hassan, R. Immunomodulatory effects of diet and nutrients in systemic lupus erythematosus (SLE): a systematic review. Front Immunol. (2020) 11:1477. doi: 10.3389/fimmu.2020.01477
9. Beukema, M, Faas, MM, and de Vos, P. The effects of different dietary fiber pectin structures on the gastrointestinal immune barrier: impact via gut microbiota and direct effects on immune cells. Exp Mol Med. (2020) 52:1364–76. doi: 10.1038/s12276-020-0449-2
10. Saeed, MA, Gribben, KC, Alam, M, Lyden, ER, Hanson, CK, and LeVan, TD. Association of Dietary Fiber on asthma, respiratory symptoms, and inflammation in the adult National Health and nutrition examination survey population. Ann Am Thorac Soc. (2020) 17:1062–8. doi: 10.1513/AnnalsATS.201910-776OC
11. Grooms, KN, Ommerborn, MJ, Pham, DQ, Djoussé, L, and Clark, CR. Dietary fiber intake and cardiometabolic risks among US adults, NHANES 1999-2010. Am J Med. (2013) 126:1059–1067.e1051-1054, 1067.e4. doi: 10.1016/j.amjmed.2013.07.023
12. Reverri, EJ, Randolph, JM, Steinberg, FM, Kappagoda, CT, Edirisinghe, I, and Burton-Freeman, BM. Black beans, Fiber, and antioxidant capacity pilot study: examination of whole foods vs. Functional components on postprandial metabolic, oxidative stress, and inflammation in adults with metabolic syndrome. Nutrients. (2015) 7:6139–54. doi: 10.3390/nu7085273
13. Wedlake, L, Shaw, C, McNair, H, Lalji, A, Mohammed, K, Klopper, T, et al. Randomized controlled trial of dietary fiber for the prevention of radiation-induced gastrointestinal toxicity during pelvic radiotherapy. Am J Clin Nutr. (2017) 106:849–57. doi: 10.3945/ajcn.116.150565
14. Johansson-Persson, A, Ulmius, M, Cloetens, L, Karhu, T, Herzig, KH, and Onning, G. A high intake of dietary fiber influences C-reactive protein and fibrinogen, but not glucose and lipid metabolism, in mildly hypercholesterolemic subjects. Eur J Nutr. (2014) 53:39–48. doi: 10.1007/s00394-013-0496-8
15. Vitaglione, P, Mennella, I, Ferracane, R, Rivellese, AA, Giacco, R, Ercolini, D, et al. Whole-grain wheat consumption reduces inflammation in a randomized controlled trial on overweight and obese subjects with unhealthy dietary and lifestyle behaviors: role of polyphenols bound to cereal dietary fiber. Am J Clin Nutr. (2015) 101:251–61. doi: 10.3945/ajcn.114.088120
16. Hu, B, Yang, XR, Xu, Y, Sun, YF, Sun, C, Guo, W, et al. Systemic immune-inflammation index predicts prognosis of patients after curative resection for hepatocellular carcinoma. Clin Cancer Res. (2014) 20:6212–22. doi: 10.1158/1078-0432.CCR-14-0442
17. Chen, JH, Zhai, ET, Yuan, YJ, Wu, KM, Xu, JB, Peng, JJ, et al. Systemic immune-inflammation index for predicting prognosis of colorectal cancer. World J Gastroenterol. (2017) 23:6261–72. doi: 10.3748/wjg.v23.i34.6261
18. Guo, W, Song, Y, Sun, Y, Du, H, Cai, Y, You, Q, et al. Systemic immune-inflammation index is associated with diabetic kidney disease in type 2 diabetes mellitus patients: evidence from NHANES 2011-2018. Front Endocrinol (Lausanne). (2022) 13:1071465. doi: 10.3389/fendo.2022.1071465
19. Xie, R, Xiao, M, Li, L, Ma, N, Liu, M, Huang, X, et al. Association between SII and hepatic steatosis and liver fibrosis: a population-based study. Front Immunol. (2022) 13:925690. doi: 10.3389/fimmu.2022.925690
20. Lu, L, Feng, Y, Liu, YH, Tan, HY, Dai, GH, Liu, SQ, et al. The systemic immune-inflammation index may be a novel and strong marker for the accurate early prediction of acute kidney injury in severe acute pancreatitis patients. J Investig Surg. (2022) 35:962–6. doi: 10.1080/08941939.2021.1970864
21. Kearney, N, McCourt, C, Hughes, R, Alsharqi, A, O'Kane, D, and Kirby, B. Systemic immune inflammation index is a marker of cardiovascular risk and not just disease severity in hidradenitis suppurativa. J Eur Acad Dermatol Venereol. (2022) 36:e928–9. doi: 10.1111/jdv.18322
22. Qi, Q, Zhuang, L, Shen, Y, Geng, Y, Yu, S, Chen, H, et al. A novel systemic inflammation response index (SIRI) for predicting the survival of patients with pancreatic cancer after chemotherapy. Cancer. (2016) 122:2158–67. doi: 10.1002/cncr.30057
23. Ma, SJ, Yu, H, Khan, M, Gill, J, Santhosh, S, Chatterjee, U, et al. Evaluation of optimal threshold of neutrophil-lymphocyte ratio and its association with survival outcomes among patients with head and neck cancer. JAMA Netw Open. (2022) 5:e227567. doi: 10.1001/jamanetworkopen.2022.7567
24. Liu, Y, Yang, X, Kadasah, S, and Peng, C. Clinical value of the prognostic nutrition index in the assessment of prognosis in critically ill patients with stroke: a retrospective analysis. Comput Math Methods Med. (2022) 2022:1–7. doi: 10.1155/2022/4889920
25. Zhou, D, Wang, J, and Li, X. The red blood cell distribution width-albumin ratio was a potential prognostic biomarker for diabetic ketoacidosis. Int J Gen Med. (2021) 14:5375–80. doi: 10.2147/IJGM.S327733
26. Yang, L, Gao, C, Li, F, Yang, L, Chen, J, Guo, S, et al. Monocyte-to-lymphocyte ratio is associated with 28-day mortality in patients with acute respiratory distress syndrome: a retrospective study. J Intensive Care. (2021) 9:49. doi: 10.1186/s40560-021-00564-6
27. Lu, C, Long, J, Liu, H, Xie, X, Xu, D, Fang, X, et al. Red blood cell distribution width-to-albumin ratio is associated with all-cause mortality in cancer patients. J Clin Lab Anal. (2022) 36:e24423. doi: 10.1002/jcla.24423
28. MEC In-Person Dietary Interviewers Procedures Manual (2016). Available at: [https://wwwn.cdc.gov/nchs/data/nhanes/2015-2016/manuals/2016_MEC_In-Person_Dietary_Interviewers_Procedures_Manual.pdf]
29. (NHANES) and Nutrition ES: Phone Follow-Up Dietary Interviewer Procedures Manual. (2016). Available at: https://wwwn.cdc.gov/nchs/data/nhanes/2015-2016/manuals/2016_Phone_Follow-Up_Dietary_Interviewer_Procedures_Manual.pdf
30. Xu, W, Huo, J, Chen, G, Yang, K, Huang, Z, Peng, L, et al. Association between red blood cell distribution width to albumin ratio and prognosis of patients with sepsis: a retrospective cohort study. Front Nutr. (2022) 9:1019502. doi: 10.3389/fnut.2022.1019502
31. MEC Laboratory Procedures Manual (2016). Available at: [https://wwwn.cdc.gov/nchs/data/nhanes/2015-2016/manuals/2016_MEC_Laboratory_Procedures_Manual.pdf]
32. Shivappa, N, Steck, SE, Hurley, TG, Hussey, JR, and Hébert, JR. Designing and developing a literature-derived, population-based dietary inflammatory index. Public Health Nutr. (2014) 17:1689–96. doi: 10.1017/S1368980013002115
33. Li, Y, Liu, M, Cui, Y, Zhu, Z, Chen, J, Zeng, F, et al. Increased risk of testosterone deficiency is associated with the systemic immune-inflammation index: a population-based cohort study. Front Endocrinol (Lausanne). (2022) 13:974773. doi: 10.3389/fendo.2022.974773
34. Li, H, Wu, X, Bai, Y, Wei, W, Li, G, Fu, M, et al. Physical activity attenuates the associations of systemic immune-inflammation index with total and cause-specific mortality among middle-aged and older populations. Sci Rep. (2021) 11:12532. doi: 10.1038/s41598-021-91324-x
35. Wu, D, Gao, X, Shi, Y, Wang, H, Wang, W, Li, Y, et al. Association between handgrip strength and the systemic immune-inflammation index: a Nationwide study, NHANES 2011-2014. Int J Environ Res Public Health. (2022) 19:13616. doi: 10.3390/ijerph192013616
36. Yoo, JW, Ju, S, Lee, SJ, Cho, YJ, Lee, JD, and Kim, HC. Red cell distribution width/albumin ratio is associated with 60-day mortality in patients with acute respiratory distress syndrome. Infect Dis (Lond). (2020) 52:266–70. doi: 10.1080/23744235.2020.1717599
37. Calixte, R, Ye, Z, Haq, R, Aladhamy, S, and Camacho-Rivera, M. Demographic and social patterns of the mean values of inflammatory markers in U.S. adults: a 2009-2016 NHANES analysis. Diseases. (2023) 11:11. doi: 10.3390/diseases11010014
38. Wang, Q, Sun, Y, Xu, Q, Liu, W, Wang, P, Yao, J, et al. Higher dietary inflammation potential and certain dietary patterns are associated with polycystic ovary syndrome risk in China: a case-control study. Nutr Res. (2022) 100:1–18. doi: 10.1016/j.nutres.2021.12.006
39. Wang, X, Li, T, Li, H, Li, D, Wang, X, Zhao, A, et al. Association of Dietary Inflammatory Potential with blood inflammation: the prospective markers on mild cognitive impairment. Nutrients. (2022) 14:2417. doi: 10.3390/nu14122417
40. Alkan, ŞB, Artaç, M, and Rakıcıoğlu, N. Dietary antioxidant capacity and serum inflammatory biomarkers levels in cancer survivors. Nutr Cancer. (2022) 74:1243–51. doi: 10.1080/01635581.2021.1952443
41. Sut, A, Chiżyński, K, Różalski, M, and Golański, J. Dietary intake of omega fatty acids and polyphenols and its relationship with the levels of inflammatory markers in men with chronic coronary syndrome after percutaneous coronary intervention. Kardiol Pol. (2020) 78:117–23. doi: 10.33963/KP.15078
42. Recalcati, S, Invernizzi, P, Arosio, P, and Cairo, G. New functions for an iron storage protein: the role of ferritin in immunity and autoimmunity. J Autoimmun. (2008) 30:84–9. doi: 10.1016/j.jaut.2007.11.003
43. Sharif, K, Vieira Borba, V, Zandman-Goddard, G, and Shoenfeld, Y. Eppur Si Muove: ferritin is essential in modulating inflammation. Clin Exp Immunol. (2018) 191:149–50. doi: 10.1111/cei.13069
44. Birgegård, G, Hällgren, R, Killander, A, Strömberg, A, Venge, P, and Wide, L. Serum ferritin during infection. A longitudinal study Scand J Haematol. (1978) 21:333–40. doi: 10.1111/j.1600-0609.1978.tb00374.x
45. Hulthén, L, Lindstedt, G, Lundberg, PA, and Hallberg, L. Effect of a mild infection on serum ferritin concentration--clinical and epidemiological implications. Eur J Clin Nutr. (1998) 52:376–9. doi: 10.1038/sj.ejcn.1600573
46. Pelkonen, P, Swanljung, K, and Siimes, MA. Ferritinemia as an indicator of systemic disease activity in children with systemic juvenile rheumatoid arthritis. Acta Paediatr Scand. (1986) 75:64–8. doi: 10.1111/j.1651-2227.1986.tb10158.x
47. Vanarsa, K, Ye, Y, Han, J, Xie, C, Mohan, C, and Wu, T. Inflammation associated anemia and ferritin as disease markers in SLE. Arthritis Res Ther. (2012) 14:R182. doi: 10.1186/ar4012
48. Hulet, SW, Powers, S, and Connor, JR. Distribution of transferrin and ferritin binding in normal and multiple sclerotic human brains. J Neurol Sci. (1999) 165:48–55. doi: 10.1016/S0022-510X(99)00077-5
49. Warkentin, T, Kolba, N, and Tako, E. Low Phytate peas (Pisum sativum L.) improve iron status, gut microbiome, and brush border membrane functionality in vivo (Gallus gallus). Nutrients. (2020) 12:12. doi: 10.3390/nu12092563
50. Li, Y, Han, M, Song, J, Liu, S, Wang, Y, Su, X, et al. The prebiotic effects of soluble dietary fiber mixture on renal anemia and the gut microbiota in end-stage renal disease patients on maintenance hemodialysis: a prospective, randomized, placebo-controlled study. J Transl Med. (2022) 20:599. doi: 10.1186/s12967-022-03812-x
51. Sandroni, A, House, E, Howard, L, and DellaValle, DM. Synbiotic supplementation improves response to iron supplementation in female athletes during training. J Diet Suppl. (2022) 19:366–80. doi: 10.1080/19390211.2021.1887423
52. Péneau, S, Dauchet, L, Vergnaud, AC, Estaquio, C, Kesse-Guyot, E, Bertrais, S, et al. Relationship between iron status and dietary fruit and vegetables based on their vitamin C and fiber content. Am J Clin Nutr. (2008) 87:1298–305. doi: 10.1093/ajcn/87.5.1298
53. Bright-See, E, McKeown-Eyssen, G, Jacobson, EA, Newmark, HW, Mathews, R, Morson, L, et al. Dietary fiber and cancer: a supplement for intervention studies. Nutr Cancer. (1985) 7:211–20. doi: 10.1080/01635588509513857
54. Haddad, EH, Berk, LS, Kettering, JD, Hubbard, RW, and Peters, WR. Dietary intake and biochemical, hematologic, and immune status of vegans compared with nonvegetarians. Am J Clin Nutr. (1999) 70:586s–93s. doi: 10.1093/ajcn/70.3.586s
55. Cummings, DM, King, DE, Mainous, AG, and Geesey, ME. Combining serum biomarkers: the association of C-reactive protein, insulin sensitivity, and homocysteine with cardiovascular disease history in the general US population. Eur J Cardiovasc Prev Rehabil. (2006) 13:180–5. doi: 10.1097/01.hjr.0000185973.59512.d3
56. Ford, ES, Ajani, UA, and Mokdad, AH. The metabolic syndrome and concentrations of C-reactive protein among U.S. youth. Diabetes Care. (2005) 28:878–81. doi: 10.2337/diacare.28.4.878
57. Hu, FB, Meigs, JB, Li, TY, Rifai, N, and Manson, JE. Inflammatory markers and risk of developing type 2 diabetes in women. Diabetes. (2004) 53:693–700. doi: 10.2337/diabetes.53.3.693
58. Lawrence, T. Inflammation and cancer: a failure of resolution? Trends Pharmacol Sci. (2007) 28:162–5. doi: 10.1016/j.tips.2007.02.003
59. King, DE, Egan, BM, and Geesey, ME. Relation of dietary fat and fiber to elevation of C-reactive protein. Am J Cardiol. (2003) 92:1335–9. doi: 10.1016/j.amjcard.2003.08.020
60. Ajani, UA, Ford, ES, and Mokdad, AH. Dietary fiber and C-reactive protein: findings from national health and nutrition examination survey data. J Nutr. (2004) 134:1181–5. doi: 10.1093/jn/134.5.1181
61. Ma, Y, Griffith, JA, Chasan-Taber, L, Olendzki, BC, Jackson, E, Stanek, EJ 3rd, et al. Association between dietary fiber and serum C-reactive protein. Am J Clin Nutr. (2006) 83:760–6. doi: 10.1093/ajcn/83.4.760
62. King, DE, Egan, BM, Woolson, RF, Mainous, AG 3rd, Al-Solaiman, Y, and Jesri, A. Effect of a high-fiber diet vs a fiber-supplemented diet on C-reactive protein level. Arch Intern Med. (2007) 167:502–6. doi: 10.1001/archinte.167.5.502
63. Xue, K, Liu, Y, Iversen, KN, Mazidi, M, Qu, Z, Dong, C, et al. Impact of a fermented high-Fiber Rye diet on helicobacter pylori and cardio-metabolic risk factors: a randomized controlled trial among Helicobacter pylori-positive Chinese adults. Front Nutr. (2020) 7:608623. doi: 10.3389/fnut.2020.608623
64. Ma, Y, Hébert, JR, Li, W, Bertone-Johnson, ER, Olendzki, B, Pagoto, SL, et al. Association between dietary fiber and markers of systemic inflammation in the Women's Health Initiative observational study. Nutrition. (2008) 24:941–9. doi: 10.1016/j.nut.2008.04.005
65. You, A. Dietary guidelines for Americans. US Department of Health and Human Services and US Department of Agriculture. (2015) 7:95.
66. EFSA Panel on Dietetic Products N. Allergies: scientific opinion on dietary reference values for carbohydrates and dietary fibre. EFSA J. (2010) 8:1462. doi: 10.2903/j.efsa.2010.1462
67. Sonnenburg, ED, Smits, SA, Tikhonov, M, Higginbottom, SK, Wingreen, NS, and Sonnenburg, JL. Diet-induced extinctions in the gut microbiota compound over generations. Nature. (2016) 529:212–5. doi: 10.1038/nature16504
68. Dang, AT, and Marsland, BJ. Microbes, metabolites, and the gut-lung axis. Mucosal Immunol. (2019) 12:843–50. doi: 10.1038/s41385-019-0160-6
69. Koh, A, De Vadder, F, Kovatcheva-Datchary, P, and Bäckhed, F. From dietary Fiber to host physiology: short-chain fatty acids as key bacterial metabolites. Cells. (2016) 165:1332–45. doi: 10.1016/j.cell.2016.05.041
70. Marinelli, L, Martin-Gallausiaux, C, Bourhis, JM, Béguet-Crespel, F, Blottière, HM, and Lapaque, N. Identification of the novel role of butyrate as AhR ligand in human intestinal epithelial cells. Sci Rep. (2019) 9:643. doi: 10.1038/s41598-018-37019-2
71. Johnstone, RW. Histone-deacetylase inhibitors: novel drugs for the treatment of cancer. Nat Rev Drug Discov. (2002) 1:287–99. doi: 10.1038/nrd772
72. Trompette, A, Gollwitzer, ES, Yadava, K, Sichelstiel, AK, Sprenger, N, Ngom-Bru, C, et al. Gut microbiota metabolism of dietary fiber influences allergic airway disease and hematopoiesis. Nat Med. (2014) 20:159–66. doi: 10.1038/nm.3444
73. Thio, CL, Chi, PY, Lai, AC, and Chang, YJ. Regulation of type 2 innate lymphoid cell-dependent airway hyperreactivity by butyrate. J Allergy Clin Immunol. (2018) 142:1867–1883.e12. doi: 10.1016/j.jaci.2018.02.032
74. Zheng, L, Kelly, CJ, Battista, KD, Schaefer, R, Lanis, JM, Alexeev, EE, et al. Microbial-derived butyrate promotes epithelial barrier function through IL-10 receptor-dependent repression of Claudin-2. J Immunol. (2017) 199:2976–84. doi: 10.4049/jimmunol.1700105
75. Macia, L, Tan, J, Vieira, AT, Leach, K, Stanley, D, Luong, S, et al. Metabolite-sensing receptors GPR43 and GPR109A facilitate dietary fibre-induced gut homeostasis through regulation of the inflammasome. Nat Commun. (2015) 6:6734. doi: 10.1038/ncomms7734
76. Bermudez-Brito, M, Sahasrabudhe, NM, Rösch, C, Schols, HA, Faas, MM, and de Vos, P. The impact of dietary fibers on dendritic cell responses in vitro is dependent on the differential effects of the fibers on intestinal epithelial cells. Mol Nutr Food Res. (2015) 59:698–710. doi: 10.1002/mnfr.201400811
77. Brufau, MT, Campo-Sabariz, J, Bou, R, Carné, S, Brufau, J, Vilà, B, et al. Salmosan, a β-galactomannan-rich product, protects epithelial barrier function in Caco-2 cells infected by Salmonella enterica Serovar Enteritidis. J Nutr. (2016) 146:1492–8. doi: 10.3945/jn.116.232546
78. Ortega-González, M, Ocón, B, Romero-Calvo, I, Anzola, A, Guadix, E, Zarzuelo, A, et al. Sánchez de Medina F, Martínez-Augustin O: nondigestible oligosaccharides exert nonprebiotic effects on intestinal epithelial cells enhancing the immune response via activation of TLR4-NFκB. Mol Nutr Food Res. (2014) 58:384–93. doi: 10.1002/mnfr.201300296
79. Roduit, C, Frei, R, Ferstl, R, Loeliger, S, Westermann, P, Rhyner, C, et al. High levels of butyrate and propionate in early life are associated with protection against atopy. Allergy. (2019) 74:799–809. doi: 10.1111/all.13660
Keywords: dietary fiber, National Health and Nutrition Examination Survey, systemic immune-inflammation index, systemic inflammation response index, red blood cell distribution width-to-albumin ratio
Citation: Qi X, Li Y, Fang C, Jia Y, Chen M, Chen X and Jia J (2023) The associations between dietary fibers intake and systemic immune and inflammatory biomarkers, a multi-cycle study of NHANES 2015–2020. Front. Nutr. 10:1216445. doi: 10.3389/fnut.2023.1242115
Edited by:
Isabelle Wolowczuk, INSERM U1019 Centre d'Infection et Immunité de Lille (CIIL), FranceReviewed by:
Dina Keumala Sari, Universitas Sumatera Utara, IndonesiaMahsa Jalili, University of Copenhagen, Denmark
Copyright © 2023 Qi, Li, Fang, Jia, Chen, Chen and Jia. This is an open-access article distributed under the terms of the Creative Commons Attribution License (CC BY). The use, distribution or reproduction in other forums is permitted, provided the original author(s) and the copyright owner(s) are credited and that the original publication in this journal is cited, in accordance with accepted academic practice. No use, distribution or reproduction is permitted which does not comply with these terms.
*Correspondence: Jie Jia, Jiajie110110@126.com