- 1Henan Provincial Key Laboratory of Biological Processing and Nutritional Function of Wheat, School of Biological Engineering, Henan University of Technology, Zhengzhou, China
- 2College of Food and Biological Engineering, Henan University of Animal Husbandry and Economy, Zhengzhou, China
- 3School of Food and Pharmacy, Xuchang University, Xuchang, China
- 4State Key Laboratory of Crop Stress Adaptation and Improvement, College of Agriculture, Henan University, Kaifeng, China
- 5Food Laboratory of Zhongyuan, Henan University of Technology, Zhengzhou, China
In this paper, we study the effect of microbial fermentation on the nutrient composition and flavor of sweet potato slurry, different strains of Aspergillus niger, Saccharomyces cerevisiae, Lactobacillus plantarum, Bacillus coagulans, Bacillus subtilis, Lactobacillus acidophilus, and Bifidobacterium brevis were employed to ferment sweet potato slurry. After 48 h of fermentation with different strains (10% inoculation amount), we compared the effects of several strains on the nutritional and functional constituents (protein, soluble dietary fiber, organic acid, soluble sugar, total polyphenol, free amino acid, and sensory characteristics). The results demonstrated that the total sugar level of sweet potato slurry fell significantly after fermentation by various strains, indicating that these strains can utilize the nutritious components of sweet potato slurry for fermentation. The slurry’s total protein and phenol concentrations increased significantly, and many strains demonstrated excellent fermentation performance. The pH of the slurry dropped from 6.78 to 3.28 to 5.95 after fermentation. The fermentation broth contained 17 free amino acids, and the change in free amino acid content is closely correlated with the flavor of the sweet potato fermentation slurry. The gas chromatography-mass spectrometry results reveal that microbial fermentation can effectively increase the kinds and concentration of flavor components in sweet potato slurry, enhancing its flavor and flavor profile. The results demonstrated that Aspergillus niger fermentation of sweet potato slurry might greatly enhance protein and total phenolic content, which is crucial in enhancing nutrition. However, Bacillus coagulans fermentation can enhance the concentration of free amino acids in sweet potato slurry by 64.83%, with a significant rise in fresh and sweet amino acids. After fermentation by Bacillus coagulans, the concentration of lactic acid and volatile flavor substances also achieved its highest level, which can considerably enhance its flavor. The above results showed that Aspergillus niger and Bacillus coagulans could be the ideal strains for sweet potato slurry fermentation.
Introduction
Sweet potato is an extremely popular food item. This tuber plant has a pleasant flavor, and many individuals enjoy it. The production of sweet potatoes in China has surpassed 85% of the globe. The production of sweet potatoes have reached 51 million tonnes in 2022 (1). The cultivation area and output of sweet potato are only lower than those of rice, wheat, and corn among China’s food crops, ranking fourth (2). It is adaptable, resistant to drought, produces a large yield, has a wide range of applications, and is both edible and feed. Sweet potatoes can also be processed into starch and utilized as raw materials in the light industrial sector. It is crucial to boost grain output and improve people’s living standards to raise the production of sweet potatoes according to local requirements (3).
Furthermore, to a small amount of fresh food, certain sweet potatoes are currently utilized for biological fermentation to make fuel ethanol. In contrast, others are used for primary processing, such as sweet potato vermicelli, sweet potato starch, dried sweet potato, sweet potato juice, etc. (4, 5). During primary processing, a significant quantity of nutrients cannot be utilized and are wasted. According to the reports, sweet potatoes are rich in dietary fiber, protein, amino acids, polysaccharides, and phenolic compounds, etc., and their nutritional worth is acknowledged worldwide (6, 7). If sweet potatoes can be treated aggressively to acquire additional nutrients, their use will be substantially enhanced. Microbial fermentation has historically been one of the most prevalent food processing methods (8–10). Under the influence of beneficial microorganisms, carbohydrates, lipids, and proteins in food materials are decomposed and transformed into distinctive flavors and other nutrients. Domestic and international researchers use lactic acid bacteria, bacillus, and edible fungi, etc. to ferment fruit and vegetable juice to increase its nutritional and sensory qualities (11–13). However, the utilization of these microbes in the fermentation of sweet potato slurry has not been reported.
Therefore, this study employs sweet potato slurry as its basic material and ferments it with various microorganisms. To provide data support and a theoretical framework for the preparation of beverages from industrial fermentation slurry, the microorganisms suitable for the fermentation of sweet potato slurry were chosen through a thorough comparison of the functional nutritional components (protein, total dietary fiber, sugar components, organic acid, total phenols, and free amino acids) and sensory characteristics (GC-MS) of the fermented slurry.
Materials and methods
Preparation of sweet potato slurry
Fresh, pest- and disease-free sweet potatoes were peeled (Longshu No.9, purchased from Yonghui Supermarket). Then, they were combined with water in a 1:2 (w/w) ratio in the pulping wall-breaker (SP301S, Zhejiang Supor Co., Ltd., Hangzhou, China). The slurry of sweet potato was kept at 95°C for 2 h after 10 U/g of high-temperature amylase (20,000 U/mL, provided by Shanghai Yuanye Bio-Technology Co., Ltd.) addition and then kept at 40°C for 3 h after 20 U/g amyloglucosidase (100,000 U/mL, provided by Shanghai Yuanye Bio-Technology Co., Ltd.). The sweet potato slurry was then split into 250 mL shake flasks containing 100 mL of liquid. The liquid is then pasteurized and stored at 4°C for future fermentation studies.
Microorganism
This study employed these strains, Aspergillus niger (A.n), Saccharomyces cerevisiae (S.c), Lactobacillus plantarum (L.p), Bacillus coagulans (B.c), Bacillus subtilis (B.s), Lactobacillus acidophilus (L.a), and Bifidobacterium brevis (B.b). All these strains were preserved in our laboratory at −80°C.
Seed culture of different microorganisms
YPD culture medium was purchased from Shanghai Yuanye Bio-Technology Co., Ltd. (Shanghai, China) and is majorly used for S. cerevisiae seed culture; MRS culture medium, purchased from Solarbio Biotechnology Co., Ltd. (Beijing, China) and it is mostly employed for the seed culture of other microorganisms. All media were autoclaved for 20 min at 115°C.
The seed culture medium was inoculated with different strains after sterilization, and then incubated at 37°C and 150 rpm/min for 24 h in the rotary shaker (HYL-C, Qiangle Experimental Co., Ltd., Taicang, China). Typically, 10% of the seeds were then transferred to a 250 mL shake flask containing 100 mL of sweet potato slurry and incubated for 48 h at 37°C and 150 rpm on a rotating shaker. Also, 10% sterile water was utilized as the inoculant in the control experiment. All fermentations were repeated three times.
Measurement of total sugars and soluble sugars
The 3,5-dinitrosalicylic acid (DNS) colorimetric method was used to determine the total sugar concentration (8). High-Performance Liquid Chromatography (HPLC 1515, Waters, United States) was used to examine soluble carbohydrates (fructose, glucose, and trehalose) (14). Briefly, 1 mL of each sample was transferred to an injection vial and then loaded onto the autosampler of a chromatograph equipped with a sulfonated polystyrene divinylbenzene column (Aminex HPX-87C 300 mm × 7.8 mm, 9 μm; Bio-Rad Chemical Division, California) and a refractive index detector. The mobile phase consisted of acetonitrile: water (70:30) at a 0.6 mL/min flow rate. The injection volume was 20 μL. The column and detector were kept at 50°C.
Measurement of pH and organic acids
The pH was measured using a pH electrode (FE28-Micro, Mettler Toledo, Zurich, Switzerland). According to Li et al., organic acids (acetic acid, citric acid, lactic acid, malic acid, and succinic acid) were analyzed using HPLC (15). Briefly, 1 mL of each sample was injected into the injection vial and placed on the autosampler of the chromatograph with a C18 column (150 × 4.6 mm, 5 μm; Agilent) and an ultraviolet detector. The mobile phase was 40 mM Na2SO4 (adjusted to pH 2.68 using CH3SO3H) at a 0.8 mL/min flow rate and injection volume of 10 μL. The column temperature was maintained at 25°C, and the UV detector’s wavelength was 210 nm.
The fermentation samples were centrifuged, and the supernatant was filtered through a 0.22 μm aqueous membrane. Identification and quantification were performed based on standard solutions retention times and calibration curves.
Measurement of the content of protein and soluble dietary fiber
Using a BCA protein assay kit (Tiangen, Beijing, China) with bovine serum albumin as a reference, the protein concentration of fermentation broth was measured. The absorbance in reaction units was determined using the Synergy H4 Hybrid Microplate Reader (Biotek, Winooski, VT, United States) at room temperature.
Soluble dietary fiber was performed following the AOAC 991.43 method (16). The 50 mL of fermentation broth was centrifuged to extract the supernatant, then 5 mL of protease was added, and the mixture was agitated for 60 min. The reactant was centrifuged at 12,000 × g for 15 min. The supernatant was filtered using a 0.45 μM millipore filter (Jinteng Laboratory Equipment Co., Ltd., Tianjin, China) and precipitated by injecting four volumes of ethanol at 4°C for 24 h. The precipitate was again filtered, dissolved in distilled water, and freeze-dried as SDF.
Measurement of total polyphenols
Total polyphenol content was determined using the technique described (10). One mL of each juice and Folin–Ciocalteu were mixed and reacted for 1 min, then 3 mL of 20% Na2CO3 was added to the mixture. The mixture was then reacted for 30 min at 50°C, and the absorbance was measured at 765 nm. The total polyphenol content was gallic acid equivalent (mg GAE/L).
Measurement of free amino acid concentration
The FAA composition and content of various fermentation samples were analyzed using a fully automated amino acid analyzer L8900 (Hitachi, Japan) following the method of Liao et al. (17). The fermentation broth was centrifuged to extract the supernatant, then 400 μL supernatant was taken, 100 μL of sulfonic acid was added, and the mixture was uniformly mixed and stored at 4°C. After 1 hour, the supernatant was centrifuged at 12000 rpm for 5 min and diluted with PBS to an acceptable onboard concentration (estimated according to the protein content of the sample). The filtrate was collected through a 0.22 μm microporous aqueous membrane and evaluated with an automated amino acid analyzer.
Determination of volatile compounds
The volatile compounds in fermentation samples were analyzed by a Headspace Solid-Phase Microextraction coupled with Gas Chromatography-Mass Spectrometer (HS-SPME-GC/MS) method described by Yang et al. (18) with certain modifications.
Gas chromatography-mass spectrometry analysis
The GC-MS parameters were set as follows: The injector temperature was set at 300°C under the splitless mode. The initial oven temperature was 100°C and held for 1 min, then increased at a rate of 15°C/min to 300°C and held there for 5 min. The total running time was 16 min. Helium (99.999%) was the carrier gas with a 1.0 mL/min flow rate and a 6.0 mL/min blow-down flow rate. The EI source and mass transfer line temperatures were set to 230°C and 280°C, respectively. Selective ion monitoring (SIM) mode was selected with a solvent delay of 4 min. The mass spectrum was scanned by electron ionization (EI) mode at 70 eV to obtain a range of 35–500 m/z. The peak area ratio calculated the substance content (percentage).
Statistical analysis
All studies were carried out in triplicate, and the data are shown as the mean ± standard deviation (mean ± S.D.). T-test used for significance analysis with GraphPad Prism 6.0 to confirmed the validity of the comparison tests; p < 0.05 was considered significant.
Results and discussion
Nutrient composition of sweet potato slurry fermented by different bacterial strains
Rich in nutrients, the sweet potato slurry can serve as a nitrogen-rich source for microbial development. Simultaneously, the microorganism-produced extracellular protease can hydrolyze the protein into small molecular oligopeptides and amino acids, supplying the product with abundant flavoring compounds (19). Compared with the unfermented sweet potato slurry, the soluble protein content was increased to a certain extent after fermentation (except for S. cerevisiae), among which the protein content increased the most significantly after fermentation by B. coagulans (Table 1), reached 52.05%. According to the results, B. coagulans produced a substantial amount of extracellular protein during the fermentation process, enhancing sweet potato slurry’s protein content. This was consistent with prior findings (1).
Dietary fiber is a material that cannot be digested and absorbed by the small intestine of humans (20). Fermented sweet potato slurry contained considerably less soluble dietary fiber (SDF) (Table 1). According to studies, dietary fiber can be fermented and metabolized by microorganisms to generate acids (21, 22).
Changes of total phenol in sweet potato slurry after fermentation
Phenols are bioactive compounds capable of ameliorating hypertension, hyperlipidemia, hyperglycemia, and inflammatory response (23). After fermentation, the total phenol content of sweet potato slurry increased considerably relative to unfermented sweet potato slurry (except for S. cerevisiae and B. coagulans). The total phenol concentration of the sweet potato slurry fermented with L. acidophilus and A. niger was 74.74 mg/L and 63.53 mg/L, respectively, while the unfermented sweet potato slurry had 37.02 mg/L (Figure 1). According to numerous studies, the microbial fermentation of fruit and vegetable juice can significantly enhance the overall phenol content of the fermentation broth (24–26). This may be because microorganisms synthesize carboxylase, tannase, and glycosidase during fermentation to facilitate polyphenols’ degradation and metabolic transformations (27). The data above indicate that S. cerevisiae does not affect the total phenol level of sweet potato slurry fermentations.
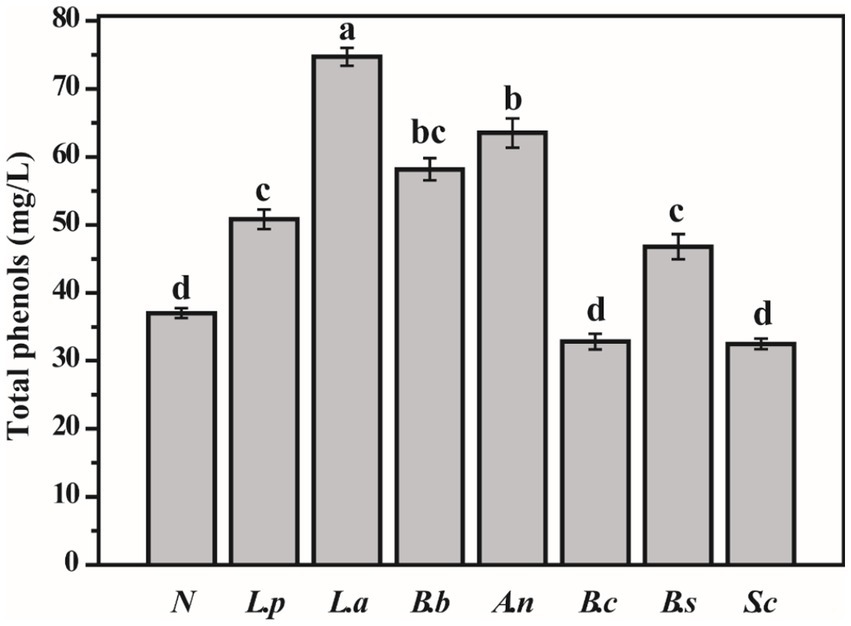
Figure 1. Determination of total phenols in fermented sweet potato slurry (Different letters indicate significant differences at p < 0.05. ND, not detected).
Changes of total sugars and soluble sugars in sweet potato slurry after fermentation
The starch in the sweet potato slurry is degraded into glucose, fructose, and maltose through early enzymatic hydrolysis, thereby supplying energy for the growth and metabolism of microorganisms during the fermentation process. The metabolism and biotransformation of carbohydrates by microorganisms during fermentation considerably decreased the glucose level of fermented sweet potato slurry compared to unfermented sweet potato slurry (p < 0.05) (Table 2). This result is consistent with the changes in sugars during fermentation of most fruits and vegetables (28).
Changes of pH and organic acids in sweet potato slurry after fermentation
After fermentation by several bacterial strains, the pH value of sweet potato slurry was reduced to a certain level, with the greatest fall occurring in B. coagulans fermentation slurry (6.78 to 3.28) (Table 3). This is mostly because the amylase and lipase produced by the metabolism of B. coagulans during fermentation can convert sugars, lipids, and other compounds into organic acids and fatty acids, resulting in a considerable fall in pH (29). Simultaneously, after fermentation by several bacterial strains, the concentrations of lactic acid, acetic acid, citric acid, and succinic acid in the slurry increased significantly (p < 0.05). However, malic acid concentrations exhibited a decreasing trend, which was in consistent with Liang et al. (9), it means that malic acid can be used by these strains to produce other metabolites. The highest content of lactic acid was fermented by B. coagulans (2325.47 mg/L). The acetic acid was undetectable in the unfermented sweet potato slurry but reached 400.05 mg/L following B. brevis fermentation. A. niger produced the greatest concentration of citric acid (418.22 mg/L), indicating that A. niger has an abundant enzyme system that can convert glucose to make citric acid (30). The succinic acid was only detected after the fermentation of A. niger, B. coagulans, and S. cerevisiae. These organic acids can impart distinctive fermentation flavors to foods that have been fermented. The distinct sour flavor of lactic acid can improve food flavor, maintain the stability and safety of the product’s microorganisms, and make the taste gentle. Acetic acid can be absorbed by the blood, enter the liver for metabolism, and produce lipids and cholesterol; Citric acid functions as a flavoring agent and possesses antioxidant characteristics that increase the shelf life of food (31). Succinic acid, when absorbed by the human body, has a protective impact on organs. It can strengthen the body and enhance immune function (32, 33).
Types and contents of free amino acids in sweet potato slurry after fermentation
Amino acids are one of the active macromolecules used in building biological organisms that can supply energy for life processes. Some microbes can create proteases and degrade macromolecular proteins into peptides and amino acids during fermentation. Therefore, the quantities of total amino acids and various amino acids in sweet potato slurry have altered significantly after fermentation. Compared with the unfermented sweet potato slurry, the total FAAs reached to 1819.74 mg/L with the fermentation of B. coagulans, which was 64.83% increased (Table 4).
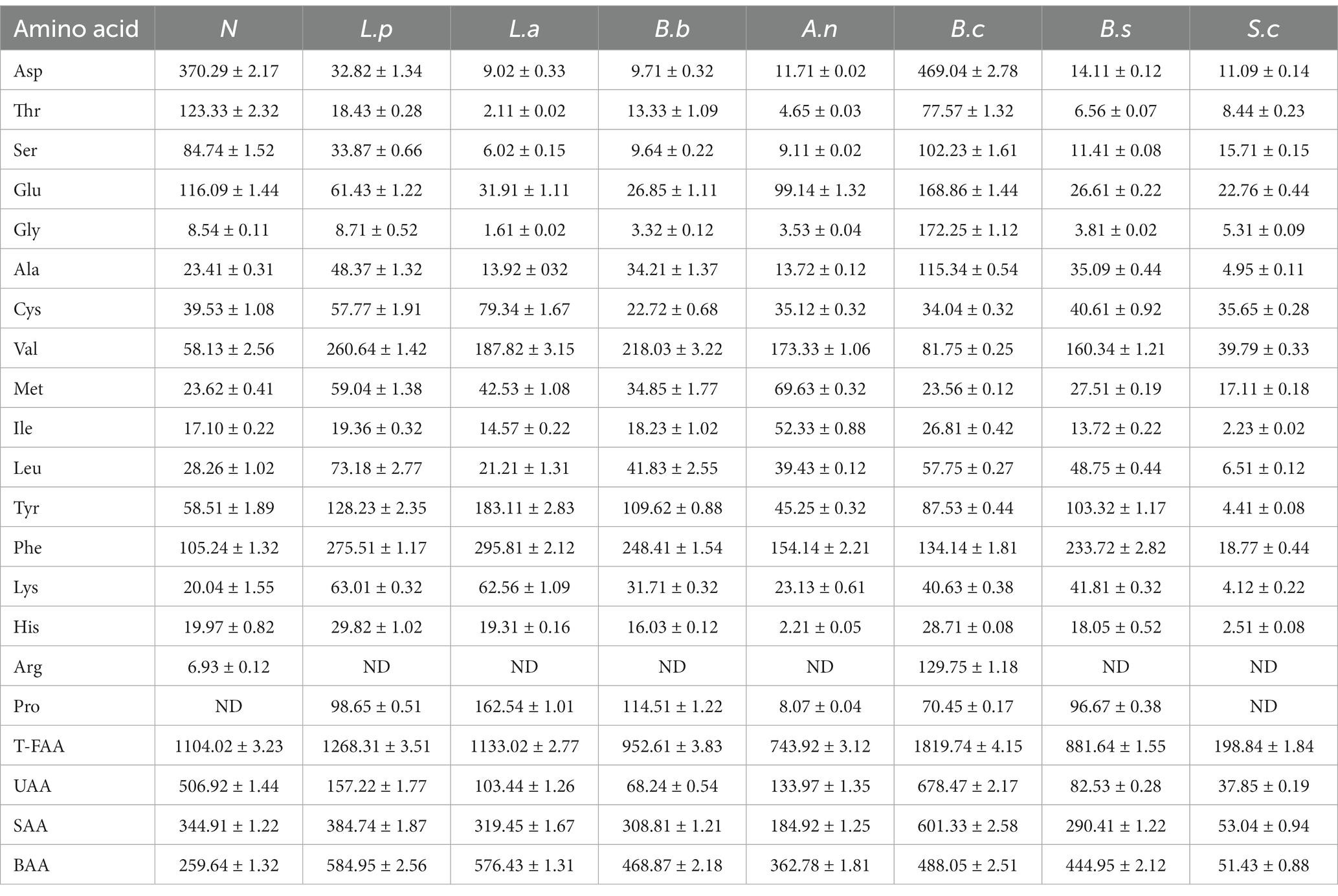
Table 4. Content of FAAs in sweet potato slurry with the fermentation of different microorganisms (mg/L).
Furthermore, being healthy nutrients, amino acids play a crucial function in flavor. Microorganisms can convert amino acids into fragrances peculiar to fruits and vegetables, such as fruit scent and fat aroma, through fermentation (34). According to different flavor approaches, free amino acids are classified as umami amino acids (UAA: including Glu, Asp., and Lys), sweet amino acids (SAA: including Thr, Ser, Gly, Ala, and Phe), and bitter amino acids (BAA: containing Tyr, Ile, Leu, Phe, Arg, His, and Met) (35). After fermentation by B. coagulans, the concentrations of UAA and SAA in unfermented sweet potato slurry increased to 678.47 mg/L and 601.33 mg/L, respectively. BAA level rises during fermentation (except in the case of S. cerevisiae), which may be related to the production of taste compounds during microbial metabolism (36). The modifications of these flavor amino acids can serve as a benchmark for the future regulation of flavor compounds in the food processing industry.
Analysis of volatile components in sweet potato slurry fermented by different strains
Flavor substances are the primary sensory component of all foods, imparting the most intuitive feeling to consumers. Using HS-SPME-GC-MS, 49 volatile chemicals were identified in various fermentation samples. There are 17 alcohols, 12 aldehydes, 5 acids, 6 esters, 6 ketones, and 3 indoles. As depicted in Figure 2, the components of volatile taste compounds in sweet potato slurry have experienced substantial modifications during bacterial fermentation. The percentage of flavor substances was the highest after fermentation by B. coagulans, which reached 86.55%.
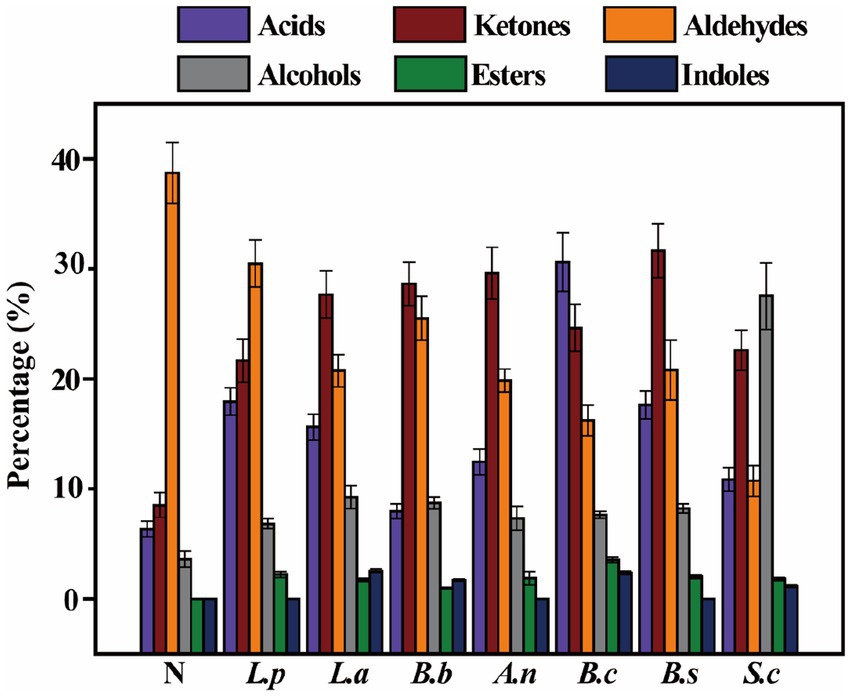
Figure 2. Changes of volatile compounds in sweet potato starch slurry fermented by seven different strains.
The concentration of aldehydes was highest in unfermented slurry, reaching 38.72%, although it fell significantly after fermentation by various strains. This may be due to aldehyde molecules’ instability, which is reduced to alcohol or oxidized to acids when bacteria are present (37). Isobutyraldehyde, hydroxyacetaldehyde, and 3-furfural are primarily found in unfermented sweet potato slurry and are undetectable after fermentation. 5-hydroxymethylfurfural is the predominant aldehyde found in sweet potato slurry that has been fermented. Esters were not detected in unfermented sweet potato slurry, although their concentration increased to variable degrees after various microorganism fermentation. Esters are important components of flavor compounds, and increasing their content can impart a pleasant odor in fermented sweet potato slurry (38). The main esters after fermentation are glycidyl acrylate and diethyl phthalate. The level of alcohol in unfermented sweet potato slurry was rather low, and it was only greatly raised by S. cerevisiae-fermented sweet potato slurry, which reached 27.53%. This means that S. cerevisiae could convert sugars in sweet potato slurry to alcohols, mainly were ethanol and methanol (39). After fermentation, the content of ketones was significantly increased after the fermentation. Sweet potato slurry. The main ketones are 1,3-dihydroxyacetone and methyl-4 (H)-pyran-ketone. Except for B.brevis, the acid components in sweet potato slurry increased significantly after fermentation. After B. coagulans fermentation, the acid concentration was the highest among these strains, reaching 30.61%. This result was consistent with the trend of pH change after fermentation, showing that a high quantity of acid flavor substances was formed after the fermentation of B. coagulans, thereby significantly enhancing the flavor components of sweet potato slurry after fermentation (40).
Conclusion
In this investigation, seven strains were applied to the fermentation of sweet potato slurry. The effects of several fermentation strains on the nutritional, functional components, and volatile taste compounds of sweet potato slurry was systematically investigated. The findings demonstrated that different fermentation strains could alter the pH, total sugar, total protein, total phenols, SDF, free amino acids, organic acid, and volatile taste compounds after fermentation compared to unfermented sweet potato starch processing slurry. The results demonstrated that the fermentation of sweet potato slurry by A. niger and B. coagulans could greatly increase protein, total phenolic content and volatile flavor, which is crucial for enhancing nutrient absorption and flavor considerably. These two strains have the potential to serve as ideal strains for enhancing the nutritional value of sweet potato fermentation slurry and enhancing its flavor and taste, hence enhancing their application value. Therefore, this study can provide ideas and theoretical support for developing a sweet potato slurry with several uses.
Data availability statement
The raw data supporting the conclusions of this article will be made available by the authors, without undue reservation.
Author contributions
LP: writing-original draft, data curation, investigation, methodology, formal analysis, resources, software, and visualization. C-JZ, ZB, W-ZT, and YZha: data curation, investigation, and methodology. Y-YL, YZho, and Y-YZ: formal analysis, resources, software, and visualization. A-ML, Y-CH, and G-HY: writing-review and editing, and supervision. MH: supervision. J-HH: writing-review and editing, resources, supervision, and project administration. All authors contributed to the article and approved the submitted version.
Funding
This work was supported by High-Level Talents Research Fund of HAUT (Grant No. 2020BS064), Henan Province Science and Technology Research and Development Plan Joint Fund Project (222103810060), Henan Province Postdoctoral Research Project Funding (202103120), Henan Province Youth Science Fund Project (232300421266). This work was also financially supported by, Major Science and Technology Projects for Public Welfare of Henan Province in China (Grant No. 201300110300), Zhongyuan Scholars of Henan Province in China (192101510004), Zhongyuan Scholar Workstation Funded Project (ZYGZZ2021056, 224400510026), The Open competition Research Projects of Xuchang University (2022JBGS11), and Central Government Guides the Local Science and Technology Development Special Fund (Z20221341069).
Conflict of interest
The authors declare that the research was conducted in the absence of any commercial or financial relationships that could be construed as a potential conflict of interest.
Publisher’s note
All claims expressed in this article are solely those of the authors and do not necessarily represent those of their affiliated organizations, or those of the publisher, the editors and the reviewers. Any product that may be evaluated in this article, or claim that may be made by its manufacturer, is not guaranteed or endorsed by the publisher.
References
1. Food and Agriculture Organization of the United Nations Database [DB/OL]. Available at: http://www.fao.org/faostat/zh (Accessed May 20, 2023).
2. Cao, JJ, Mu, TH, and Ma, MM. Effect of fermentation with different lactic acid Bacteria starter cultures on nutritional and functional components and sensory characteristics of sweet potato slurry for starch production. Food Sci. (2022) 43:134–42. doi: 10.7506/spkx1002-6630-20211025-263
3. Liang, Z, Qian, C, Jin, Y, Xue, H, Guan, J, Wang, Z, et al. Energy-saving direct ethanol production from viscosity reduction mash of sweet potato at very high gravity (VHG). Fuel Process Technol. (2010) 91:1845–50. doi: 10.1016/j.fuproc.2010.08.009
4. Zhang, L, Zhao, H, Gan, M, Jin, Y, Gao, X, Chen, Q, et al. Application of simultaneous saccharification and fermentation (SSF) from viscosity reducing of raw sweet potato for bioethanol production at laboratory, pilot and industrial scales. Bioresour Technol. (2011) 102:4573–9. doi: 10.1016/j.biortech.2010.12.115
5. Guo, L, Tao, H, Cui, B, and Janaswamy, S. The effects of sequential enzyme modifications on structural and physicochemical properties of sweet potato starch granules. Food Chem. (2018) 277:504–14. doi: 10.1016/j.foodchem.2018.11.014
6. Deng, FM, Mu, TH, Zhang, M, and Abegunde, OK. Composition, structure, and physicochemical properties of sweet potato starches isolated by sour liquid processing and centrifugation. Starch – Strke. (2013) 65:162–71. doi: 10.1002/star.201200106
7. Na, JH, Kim, HR, Kim, Y, Lee, JS, and Lee, CJ. Structural characteristics of low-digestible sweet potato starch prepared by heat-moisture treatment. Int J Biol Macromol. (2019) 151:1049–57. doi: 10.1016/j.ijbiomac.2019.10.146
8. Pattarapisitporn, A, Thiangthong, N, Inthajak, P, Jaichakan, P, and Klangpetch, W. Production of xyloligosaccharides from rice straw by microwave-assisted enzymatic hydrolysis and evaluation of their prebiotic properties. Chiang Mai Univ J Nat Sci. (2021) 20:e2021037. doi: 10.12982/CMUJNS.2021.037
9. Liang, JR, Deng, H, Hu, CY, Zhao, PT, and Meng, YH. Vitality, fermentation, aroma profile, and digestive tolerance of the newly selected Lactiplantibacillus plantarum and Lacticaseibacillus paracasei in fermented apple juice. Front Nutr. (2022) 9:9. doi: 10.3389/fnut.2022.1045347
10. Wu, C, Lin, T, Qi, J, Jiang, T, Xu, H, and Lei, H. Effects of lactic acid fermentation-based biotransformation on phenolic profiles, antioxidant capacity and flavor volatiles of apple juice. LWT-Food Sci Technol. (2020):122. doi: 10.1016/j.lwt.2020.109064
11. Ma, J, Wang, Y, Zhao, MY, Tong, PY, Lv, LQ, Gao, ZP, et al. High hydrostatic pressure treatments improved properties of fermentation of apple juice accompanied by higher reserved Lactobacillus Plantarum. Foods. (2023) 12:441. doi: 10.3390/foods12030441
12. Garcia, C, Guerin, M, Souidi, K, and Remize, F. Lactic fermented fruit or vegetable juices: past, present and future. Beverages. (2020) 6:8. doi: 10.3390/beverages6010008
13. Fonseca, HC, Melo, DD, Ramos, CL, Menezes, AGT, Dias, DR, and Schwan, RF. Sensory and flavor-aroma profiles of passion fruit juice fermented by potentially probiotic Lactiplantibacillus plantarum CCMA 0743 strain. Food Res Int. (2022) 152:152. doi: 10.1016/j.foodres.2021.110710
14. Pan, L, Fang, YK, Zhou, P, Jin, KQ, Wang, G, and Liu, YP. Strategy of oxygen transfer coefficient control on the L-Erythrulose fermentation by newly isolated Gluconobacter Kondonii. Electron J Biotechnol. (2016) 24:26–31. doi: 10.1016/j.ejbt.2016.08.006
15. Li, H, Liang, J, Han, M, Wang, X, Ren, Y, Wang, Y, et al. Sequentially fermented dealcoholized apple juice intervenes fatty liver induced by high-fat diets via modulation of intestinal flora and gene pathways. Food Res Int. (2022) 156:111180. doi: 10.1016/j.foodres.2022.111180
16. Cheng, L, Zhang, X, Hong, Y, Li, Z, Li, C, and Gu, Z. Characterisation of physicochemical and functional properties of soluble dietary fibre from potato pulp obtained by enzyme-assisted extraction. Int J Biol Macromol. (2017) 101:1004–11. doi: 10.1016/j.ijbiomac.2017.03.156
17. Liao, AM, Li, XX, Gu, ZS, He, J, Hou, Y, Pan, L, et al. Preparation and identification of an antioxidant peptide from wheat embryo albumin and characterization of its Maillard reaction products. J Food Sci. (2022) 87:2549–62. doi: 10.1111/1750-3841.16191
18. Yang, Y, Zhao, P, Wang, X, Cui, G, and Guo, Y. Using a red-fleshed and 6 varieties of thinned young apple to make juice and their phytochemicals characterization. J Food Process Preserv. (2021) 45:e15361. doi: 10.1111/jfpp.15361
19. Alavi, F, Jamshidian, M, and Rezaei, K. Applying native proteases from melon to hydrolyze kilka fish proteins (Clupeonella Cultriventris Caspia) compared to commercial enzyme Alcalase. Food Chem. (2019) 277:314. doi: 10.1016/j.foodchem.2018.10.122
20. Zhu, L, Gao, M, Li, H, Deng, ZY, and Fan, Y. Effects of soluble dietary fiber from sweet potato dregs on the structures of intestinal flora in mice. Food Biosci. (2021) 40:100880. doi: 10.1016/j.fbio.2021.100880
21. Takamine, K, Hotta, H, Degawa, Y, Morimura, S, and Kida, K. Effects of dietary fiber prepared from sweet potato pulp on cecal fermentation products and microflora in rats. J Appl Glycosci. (2005) 52:1–5. doi: 10.5458/jag.52.1
22. Xu, T, Wu, X, Liu, J, Sun, J, and Zhang, Y. The regulatory roles of dietary fibers on host health via gut microbiota-derived short chain fatty acids. Curr Opin Pharmacol. (2022) 62:36–42. doi: 10.1016/J.COPH.2021.11.001
23. Zhang, S, Hu, C, Guo, Y, Wang, X, and Meng, Y. Polyphenols in fermented apple juice: beneficial effects on human health. J Funct Foods. (2021) 76:104294. doi: 10.1016/j.jff.2020.104294
24. Wang, Y, Tao, Y, Zhang, X, Shao, S, Han, Y, Chu, DT, et al. Metabolic profile of ginkgo kernel juice fermented with lactic aicd bacteria: a potential way to degrade ginkgolic acids and enrich terpene lactones and phenolics. Process Biochem. (2019) 76:25–33. doi: 10.1016/j.procbio.2018.11.006
25. Xu, XX, Luo, DS, Bao, YJ, Liao, XJ, and Wu, J. Characterization of diversity and probiotic efficiency of the autochthonous lactic acid bacteria in the fermentation of selected raw fruit and vegetable juices. Front Microbiol. (2018) 9:2539. doi: 10.3389/fmicb.2018.02539
26. Terzic-Vidojevic, A, Veljovic, K, Tolinacki, M, Zivkovic, M, Lukic, J, Lozo, J, et al. Diversity of non-starter lactic acid Bacteria in autochthonous dairy products from Western Balkan countries – technological and probiotic properties. Food Res Int. (2020) 136:109494. doi: 10.1016/j.foodres.2020.109494
27. Yang, J, Sun, Y, Gao, T, Wu, Y, and Tao, Y. Fermentation and storage characteristics of "Fuji" apple juice using Lactobacillus Acidophilus, Lactobacillus casei and Lactobacillus Plantarum: microbial growth, metabolism of bioactives and in vitro bioactivities. Front Nutr. (2022) 9:833906. doi: 10.3389/fnut.2022.833906
28. Verón, HE, Cano, PG, Fabersani, E, Sanz, Y, and Torres, S. Cactus pear (Opuntia Ficus-indica) juice fermented with autochthonous Lactobacillus Plantarum S-811. Food Funct. (2019) 10:1085–97. doi: 10.1039/C8FO01591K
29. Majeed, M, Nagabhushanam, K, Arumugam, S, Natarajan, S, Majeed, S, Pande, A, et al. Cranberry seed fibre: a promising prebiotic fibre and its fermentation by the probiotic Bacillus Coagulans Mtcc 5856. Int J Food Sci Technol. (2018) 53:1640–7. doi: 10.1111/ijfs.13747
30. Xu, J, Chen, YQ, Zhang, HJ, Bao, JW, Tang, L, Wang, K, et al. Establishment and assessment of an integrated citric acid-methane production process. Bioresour Technol. (2015) 176:121–8. doi: 10.1016/j.biortech.2014.11.024
31. Gonçalves, P, Araújo, JR, and Santo, JPD. A cross-talk between microbiota-derived short-chain fatty acids and the host mucosal immune system regulates intestinal homeostasis and inflammatory bowel disease. Inflamm Bowel Dis. (2018) 24:558–72. doi: 10.1093/ibd/izx029
32. Jocken, J, Hernández, M, Hoebers, N, Beek, C, and Canfora, EE. Short-chain fatty acids differentially affect intracellular lipolysis in a human white adipocyte model. Front Endocrinol. (2017) 8:372. doi: 10.3389/fendo.2017.00372
33. Heimann, E, Nyman, M, Påbrink, AK, Lindkvist-Petersson, K, and Degerman, E. Branched short-chain fatty acids modulate glucose and lipid metabolism in primary adipocytes. Adipocytes. (2016) 5:359–68. doi: 10.1080/21623945.2016.1252011
34. Yu, HY, Xu, HR, Ying, YB, Xie, LJ, Zhou, Y, and Fu, XP. Prediction of trace metals in Chinese rice wine by Fourier transform near-infrared spectroscopy. Trans ASABE. (2006) 49:1463–7. doi: 10.13031/2013.22028
35. Jiang, WD, Wu, P, Tang, RJ, Liu, Y, Kuang, SY, Jiang, J, et al. Nutritive values, flavor amino acids, healthcare fatty acids and flesh quality improved by manganese referring to up-regulating the antioxidant capacity and signaling molecules TOR and Nrf2 in the muscle of fish. Food Res Int. (2016) 89:670–8. doi: 10.1016/j.foodres.2016.09.020
36. Xia, Y, Wang, G, Tao, L, Yu, J, and Ai, L. Effects of boiling, ultra-high temperature and high hydrostatic pressure on free amino acids, flavor characteristics and sensory profiles in Chinese rice wine. Food Chem. (2019) 275:407–16. doi: 10.1016/j.foodchem.2018.09.128
37. Hamilton, JF. Using comprehensive two-dimensional gas chromatography to study the atmosphere. J Chromatogr Sci. (2010) 48:274–82. doi: 10.1093/chromsci/48.4.274
38. Пономарева, О, Ponomareva, O, Борисова, Е, Borisova, E, and Prokhorchik, I. Lactic acid bacteria of the genus Lactobacillus in the formation of sour ales flavor profile. Food Process Tech Technol. (2019) 48:100–8. doi: 10.21603/2074-9414-2018-2-100-108
39. Campbell, C, Nanjundaswamy, AK, Njiti, V, Xia, Q, and Chukwuma, F. Value-added probiotic development by high-solid fermentation of sweet potato with Saccharomyces boulardii. Food Sci Nutr. (2017) 5:633–8. doi: 10.1002/fsn3.441
Keywords: sweet potato slurry, fermentation, nutritional components, gas chromatography-mass (GC-MS), flavor substances
Citation: Pan L, Zhang C-J, Bai Z, Liu Y-Y, Zhang Y, Tian W-Z, Zhou Y, Zhou Y-Y, Liao A-M, Hou Y-C, Yu G-H, Hui M and Huang J-H (2023) Effects of different strains fermentation on nutritional functional components and flavor compounds of sweet potato slurry. Front. Nutr. 10:1241580. doi: 10.3389/fnut.2023.1241580
Edited by:
Yanhao Zhang, Hong Kong Baptist University, ChinaReviewed by:
Qingtao Liu, Anhui Polytechnic University, ChinaBangrong Cai, Henan University of Chinese Medicine, China
Kolawole Banwo, University of Ibadan, Nigeria
Yushan Jia, Inner Mongolia Agricultural University, China
Copyright © 2023 Pan, Zhang, Bai, Liu, Zhang, Tian, Zhou, Zhou, Liao, Hou, Yu, Hui and Huang. This is an open-access article distributed under the terms of the Creative Commons Attribution License (CC BY). The use, distribution or reproduction in other forums is permitted, provided the original author(s) and the copyright owner(s) are credited and that the original publication in this journal is cited, in accordance with accepted academic practice. No use, distribution or reproduction is permitted which does not comply with these terms.
*Correspondence: Long Pan, panlong@haut.edu.cn; Ji-Hong Huang, huangjihong@haut.edu.cn; huangjih1216@126.com