- 1Hangzhou Vocational & Technical College, Hangzhou, China
- 2Key Laboratory of Biology, Genetics and Breeding of Special Economic Animals and Plants, Ministry of Agriculture and Rural Affairs, Tea Research Institute Chinese Academy of Agricultural Sciences, Hangzhou, China
- 3College of Food Science, Southwest University, Chongqing, China
- 4Jingdezhen Jin Gui Yuan Agricultural Development Co Ltd, Jingdezhen, China
- 5College of Biological and Environmental Engneering, Zhejiang Shuren University, Hangzhou, China
- 6College of Food and Health, Zhejiang A&F University, Hangzhou, China
Introduction: In recent years, scented black tea has attracted much attention due to its pleasant floral aroma and mellow flavor, but little research has been carried out on its flavor metabolic profile.
Methods: In this study, the flavor metabolic profiles of unscented, Chloranthus spicatus scented, and Osmanthus fragrans (Thunb.) Lour. scented Congou black teas were investigated using full-spectrum metabolomics analysis method, the first time that the flavor profiles of scented black tea were characterized in detail.
Results and Discussion: The results revealed that a total of 3,128 metabolites were detected in the three teas. Based on the criteria of variable importance in the project >1 and fold change ≥2 or ≤ 0.5, 761 non-volatile metabolites and 509 volatile metabolites were filtered as differential metabolites. Many differential non-volatile metabolites belonged to flavonoids, phenolic acids, and terpenoids. Floral, fruity and herbaceous volatile metabolites were significantly up-regulated in Chloranthus spicatus scented Congou black tea while sweet and fruity volatile metabolites were significantly down-regulated in Osmanthus fragrans (Thunb.) Lour. scented Congou black tea. The results contribute to a better understanding of the scenting techniques on the flavor quality of scented black teas and provide some information on the flavor chemistry theory of scented black teas.
1. Introduction
Black tea, a fully fermented tea, is loved by consumers around the world for its health benefits (1–4) and mellow taste. Black tea is widely produced in countries such as China, India, Sri Lanka, Kenya and Indonesia (5, 6). It is popular and ranks first in the world in terms of production and consumption. The world black tea production accounted for around 65% of total tea production in 2017 (7). The Congou black tea (CK), a unique Chinese black tea variety, are with tightly striped shape, sweet and mellow taste, and floral and fruity aroma (8). The aroma of CK can be classified as sweet, floral or fruity, based on sensory characteristics (9). The general processes of producing CK are divided into two phases. The enzymatic phase includes the withering, rolling and fermentation processes, while the non-enzymatic phase include the drying process (10). The flavour quality is mainly formed in the enzymatic phase by the conversion of non-volatile metabolites, such as flavonoids, amino acids, fatty acids, and volatile metabolites derived from fatty acids, amino acids, terpenoids, carotenoids, and glycosidically bound volatiles (11).
In recent years, scented teas have been gaining attention for their pleasant floral aroma and mellow flavour. As a material with both ornamental and health benefits, such as protecting stomach and liver, dispersing phlegm, and antioxidant activity, Osmanthus fragrans (Thunb.) Lour. is ideal to be the raw material for the production of scented tea (12). Linalool, ionone and ocimene are aroma-contributing substances in it (13). Chloranthus spicatus is well-known for its elegance and is one of the “The Four Famous Chinese Camellias,” along with jasmine, Michelia alba DC. and Citrus aurantium. As one of the traditional Chinese scented teas, Chloranthus spicatus was also ideal for the production of scented tea.
The changes in various volatile metabolites during the scenting process are crucial to the formation of scented tea aroma. The scenting process decreased alcohols but increased ketones and esters in Osmanthus scented black tea (14). Nonyl aldehyde, γ-decalactone, 1-octene-3-ol, trans-2-hexenyl pentanoate, and phenethyl butyrate were key odorants contributing to its Osmanthus aroma. Currently, the changes of volatile metabolites during the scenting process of producing Chloranthus spicatus scented black tea is less studied. The changes of non-volatiles metabolites during the scenting process should have an effect on the formation of scented tea quality, but still largely remain unveiled.
Based on these facts, the present study characterized the flavor metabolites of CK, Osmanthus scented Congou black tea (OF), and Chloranthus spicatus scented Congou black tea (CH) by ultra-performance liquid chromatography tandem mass spectrometry (UPLC-MS/MS) and gas chromatography–mass spectrometry (GC–MS). The results aim to understand the effect of the scenting process on the metabolic profile of black tea and help broaden the ideas for the study of flavor quality of scented black tea.
2. Materials and methods
2.1. Chemicals
Methanol, acetonitrile and hexane (all chromatography grade) for the tests were obtained from Merck (Darmstadt, Germany). Formic acid was purchased from Aladdin (Shanghai, China). Sodium chloride was produced from Sinopharm (Shanghai, China). Authentic standards used in the full-spectrum metabolomics analysis were obtained from MetWare (Wuhan, China).
2.2. Preparation of tea samples
The CK used in the experiment was purchased from Jingdezhen Jin Gui Yuan Agricultural Development Co (Jiangxi, China). The black tea was made according to the traditional Congou black teas process, which consisted mainly of indoor withering, rolling, fermentation and drying. The CH and OF were scented with CK as the raw material, and the specific scenting process was as follows (15):
1. Flower spreading: Pick Chloranthus spicatus and Osmanthus buds, ensure their integrity during the picking process, remove impurities such as flower tips, stalks and leaves and then stall them indoors. Indoor environment: ventilation, humidity of 65–75%, temperature of 20–24°C, thickness of 15 cm, spreading time of 30 min.
2. Mixing and resting of tea flowers: 1/3 of the CK was used to produce CH and the other 1/3 was used to produce OF. For CH, the flowers are mixed with CK in a ratio of 3:100 (flowers: dried tea, w:w) for 100 d. For OF, the flowers are mixed with CK in a ratio of 3:10 (flowers: dried tea, w: w) for 2 days, with 1 renewal, the process lasts about 4 days in total.
3. Drying: The scented teas were baked at 85°C (6CTH-30, Zhejiang Chunjiang Tea Machinery Co., Ltd.) for 412 min, cooled for 40 min, baked at 75°C for 90 min and cooled for 120 min before removing the petals and debris, resulting in scented Congou black teas. Three replicates were set for each treatment. The samples from three treatments were stored in a refrigerator at −80°C until assayed. The three samples were mixed and sampled three times for subsequent testing to ensure homogeneity and traceability.
2.3. UPLC-MS/MS based analysis of non-volatile metabolites
2.3.1. Sample pretreatment
The three samples were vacuum freeze-dried in a lyophilizer (Scientz-100F) before being ground (30 Hz, 1.5 min) into powder by a grinder (MM 400, Retsch). Afterwards, 50 mg of sample powder was accurately weighed and 1.2 mL of 70% methanolic aqueous internal standard extraction solution pre-chilled at −20°C was added. The sample was vortexed once every 30 min for 30 s, for a total of 6 times. Then centrifuged (12,000 rpm, 3 min), the supernatant was aspirated and filtered through 0.22 μm microporous membrane for determination.
2.3.2. UPLC-MS/MS conditions
An UPLC system equipped with a tandem mass spectrometer was used to analyze the non-volatile metabolites in the samples. (1) Liquid phase conditions: An Agilent SB-C18 1.8 μm, 2.1 mm * 100 mm column was equipped with a flow rate of 0.35 mL/min, a column temperature of 40°C, and an injection volume of 2 μL. The mobile phase A was 0.1% formic acid/ultrapure water and mobile phase B was 0.1% formic acid/acetonitrile. The gradient elution method was as follows: mobile phase B was 5% at 0 min; increased linearly to 95% at 9 min, maintained for 1 min; decreased to 5% at 10–11.10 min and equilibrated at that ratio until 14 min. (2) Mass spectrometry conditions: electrospray ionization temperature (ESI) of 500°C, ion spray (IS) voltage of 5,500 V (positive ion mode) and − 4,500 V (negative ion mode); ion source gases I (GSI), gases II (GS II), and curtain gas (CUR) of 50, 60 and 25 psi, respectively; collision-induced ionization parameters of high. The multiple reaction monitoring (MRM) mode was used for triple quadrupole (QQQ) scanning, and the collision gas (nitrogen) was medium. The declustering potential (DP) and collision energy (CE) of individual MRM ion pairs were evaluated by further DP and CE optimization. A specific set of MRM ion pairs was monitored in each period depending on the metabolites eluted during the period (16).
2.3.3. Identification of non-volatile metabolites
Based on the MWDB (metware database), the substance characterization was performed based on the secondary spectrum information of the substance, and the analysis removed the isotopic signals, the duplicate signals containing K+, Na+, NH4+, and the duplicate signals of fragment ions that were themselves other larger molecular weight substances. After obtaining the metabolite spectral analysis data of different samples, the peaks of all substances were integrated by peak area using MultiQuant software, and the mass spectra of the same metabolite in different samples among them were corrected for the integration of the peaks.
2.4. GC–MS based analysis of volatile metabolites
2.4.1. Sample pretreatment
The sample was removed from the refrigerator at −80°C and firstly ground by liquid nitrogen, followed by vortexing to make a homogeneous mixture. The 500 mg of the sample was accurately weighed in a headspace vial, subsequently 2 mL of saturated sodium chloride solution were added, the vial was sealed, and then the sample was extracted by fully automated headspace solid phase microextraction (HS-SPME) for GC–MS analysis.
2.4.2. GC–MS conditions
The headspace vial containing the tea samples was shaken and equilibrated at 60°C for 5 min, followed by insertion of a 120 μm divinylbenzene/carboxen/polydimethylsiloxane (DVB/CWR/PDMS) extraction head into the vial for 15 min (stirring while extracting was used in the extraction process with a stirring rate of 1,000 rpm, thus stability and homogeneity of the extraction process and reducing human error), followed by desorption at the inlet end at 250°C for 5 min for subsequent GC–MS separation and identification. It should be noted that the extraction head should be heated at the inlet end at 250°C for 5 min before sampling. (1) Chromatographic conditions: DB-5MS capillary column (30 m × 0.25 mm × 0.25 μm, Agilent J&W Scientific, Folsom, CA, United States) supplemented with high purity helium (purity not less than 99.999%) as carrier gas. The program temperature was set as follows: 40°C for 3.5 min, then 10°C/min to 100°C, then 7°C/min to 180°C, and finally 25°C/min to 280°C for 5 min. (2) Mass spectrometry conditions: electron bombardment ion source (EI); ion source, quadrupole and mass spectrometry interface temperatures of 230°C, 150°C and 280°C, respectively; electron energy of 70 eV, scanning mode of selected ion detection mode (SIM), qualitative and quantitative ion precision scanning (GB 23200.8–2016).
2.4.3. Identification of volatile metabolites
Based on multi-species, literatures, authentic standards, and retention index (RI), the database was established independently, containing the identified retention time (RT) as well as qualitative and quantitative ions for the selected ion detection mode for precise scanning, with one quantitative ion and two to three qualitative ions selected for each compound, respectively. All ions to be detected in each group were detected separately in the order of of their peaks, in separate time periods. If the retention time of the detected peaks was consistent with the authentic standard and the selected ions all appeared in the mass spectra of the samples after background deduction, the substance would be ascertained. The odor descriptions for the qualitative aroma substances were taken from websites such as http://www.thegoodscentscompany.com, http://perflavory.com/, http://www.odour.org.uk, http://foodflavorlab.cn. The quantitative ions were selected by MassHunter software for the integration and calibration of the peaks in order to enhance the accuracy of quantification.
2.5. Statistical analysis
All samples were set up with three biological replicates and statistical analysis was performed using IBM SPSS statistics (version 25.0, SPSS Inc., Chicago, IL). The principal component analysis (PCA), partial least squares-discriminant analysis (PLS-DA), clustering heat map, K-Means plot, and key metabolite kyoto encyclopedia of genes and genomes (KEGG) enrichment pathway maps were done by R software.1 The identified metabolites were first annotated through the KEGG compound database,2 and subsequently the annotated completed metabolites were mapped to the KEGG pathway database.3 The pathways mapped to metabolites with significant regulation were subsequently sent to metabolite set enrichment analysis (MSEA), whose significance was determined by p-values of hypergeometric tests.
3. Results and discussion
3.1. Overall profile of metabolites in the three black teas
Metabolomics allows the detection and screening of significant differential metabolites from different biological samples, which can be used as a basis to further elucidate the metabolic processes and mechanisms of change in biological samples. In this study, a full-spectrum metabolomics analysis based on dual platforms of UPLC-MS/MS and GC–MS was carried out. A total of 3,128 metabolites, including 2,396 non-volatile metabolites and 732 volatile metabolites, were detected in three black tea samples.
As shown in Figures 1A, a total of 26 categories of metabolites were detected in black teas. Flavonoids, phenolic acids, terpenoids, others, alkaloids, lipids, amino acids and derivatives together occupied 68.76% of all metabolites. Lignans and coumarins (4.03%), organic acids (4.19%), esters (3.8%), heterocyclic compounds (3.64%), nucleotides and derivatives (2.65%), tannins (2.05%), hydrocarbons (1.92%), ketones (1.85%), alcohols (1.76%), aldehydes (1.44%), aromatics (1.37%) were minor metabolites. Acids, quinones, amines, sulfur compounds, nitrogen compounds, phenols, ethers, and halogenated hydrocarbons accounted for less than 1%. A previous study also pointed out that flavonoids played an important role in the quality and flavour of black tea during black tea processing (17), which was similar to the above results.
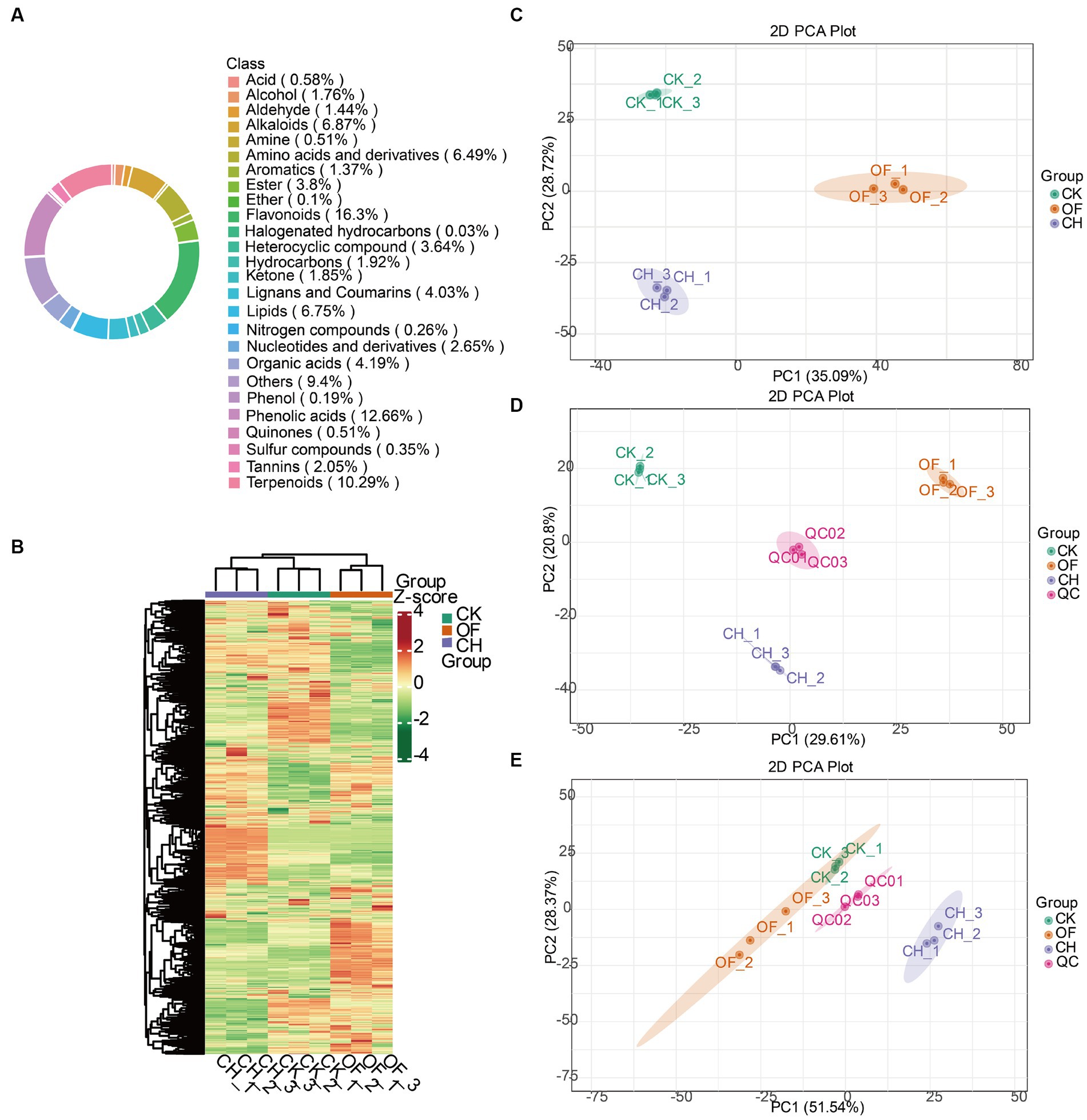
Figure 1. Analysis of all metabolites in three types of black tea (CK, CH and OF). (A) Ring diagram of total metabolite class composition; (B) Heat map of total metabolite content clustering (processed by Z-score); (C) PCA analysis of total metabolites; (D) PCA analysis of non-volatile metabolites; (E) PCA analysis of volatile metabolites. CK, Congou black tea; OF, Osmanthus black tea; CH, Chloranthus spicatus black tea. Same below.
The heatmap distribution in Figure 1B indicated that the metabolite contents in the three black tea samples were significantly different. To further validate the above results, PCA, as an unsupervised pattern recognition method for multidimensional data statistical analysis, was introduced to analyze the differences of overall metabolites (Figure 1C), non-volatile metabolites (Figure 1D), and volatile metabolites (Figure 1E) among the three black tea samples. The results revealed that the three samples achieved good separation in each PCA model, and the sum of the first and second principal components of the above three data sets explained more than 50% of the whole data set, reaching 63.81, 50.41 and 79.91%, respectively, indicating that the three black tea metabolites reached significant differences among them. The results provided some data support for further screening the important differential metabolites.
3.2. Important differential metabolites among the three black teas
1,270 differential metabolites were initially identified based on the screening criteria of variable importance in the project (VIP)>1 and fold change (FC) ≥2 or ≤ 0.5, among which 761 non-volatile metabolites (Supplementary Table S1) and 509 volatile metabolites (Supplementary Table S2) were identified.
The Venn plot indicated the relationships of differential metabolites between different black tea samples. The results showed that there were 59 differential metabolites between the three comparison groups (CK vs. OF, CK vs. CH, OF vs. CH) and 82, 50, 96 differential metabolites were unique to each group (Figure 2A). To further verify whether the differential metabolites among the three black tea samples reached significant differences, an unsupervised model statistical method, i.e., PCA (Figure 2B), and a supervised model statistical method, i.e., PLS-DA (Figure 2C), were used simultaneously to screen for differential metabolites. It was found that both models could achieve good separation. In 200 permutations of validation of the PLS-DA model (Figure 2D), R2X = 0.733, R2Y = 1 (p < 0.005), Q2 = 0.979 (p < 0.005), indicating a good model fit.
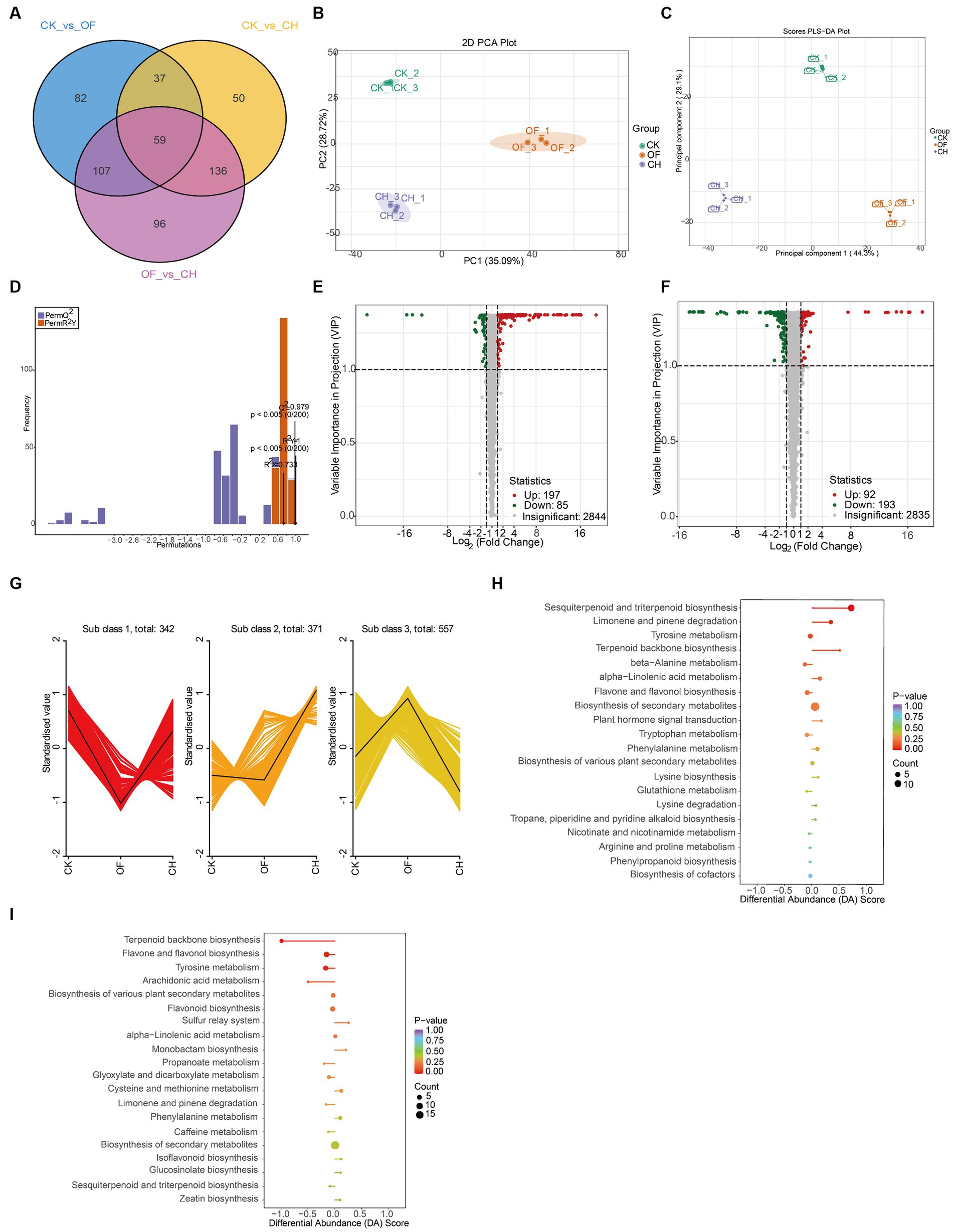
Figure 2. Analysis of important differential metabolites in three types of black tea (CK, CH and OF). (A) Venn plot; (B) PCA plot; (C) PLS-DA plot; (D) PLS-DA 200-permutation plot; (E,F) Volcano plot (E. CK vs. CH; F. CK vs. OF); (G) K-means plot; (H,I) KEGG differential abundance score plot (H. CK vs. CH; I. CK vs. OF; where the color and size of the dots indicated the size of the p-values and number of metabolites, respectively; DA score indicated the overall change of all metabolites in the metabolic pathway, 1: up-regulation, −1: down-regulation, and the length of the line segment indicated the DA score).
The Volcano plot was mainly used to show the relative abundances of metabolites between two samples. Each dot in the plot indicated a differential metabolite. Green dots indicated down-regulated substances, red dots indicated up-regulated substances, and gray indicated substances with insignificant differences. The results showed that compared to CK, 197 substances were up-regulated and 85 substances were down-regulated in CH (Figure 2E), while 92 substances were up-regulated and 193 substances were down-regulated in OF (Figure 2F), which might be related to the different flowers used in the scenting process.
The K-means plot focused on classifying the trends of relative abundances of important differential metabolites under different comparison groups to facilitate subsequent targeted analysis. According to Figure 2G, three different trends in the levels of these important differential metabolites were detected in CK, CH and OF. Among them, 342 metabolites were lower in OF than in CK and 371 metabolites were higher in CH than in CK. It was obvious that more substances were up-regulated in CH and down-regulated in OF after CK was scented, suggesting that the type of flower had a great influence on the metabolites of CK.
Subsequently, the top 20 KEGG metabolic pathways in the three samples were screened based on differential abundance (DA) scores and p-values sizes (Figures 2H,I). The sesquiterpenoid and triterpenoid biosynthesis, limonene and pinene degradation, and tyrosine metabolism reached significant levels in the metabolite expression of CH (compared to CK), with the first two pathways being significantly up-regulated and the last one remaining basically stable. Sesquiterpenoids and triterpenoids were generally biosynthesized via the cytoplasmic mevalonate (MVA) pathway in plants (18–20). Triterpenoids usually had a variety of stereoisomers and positional isomers, but the main compounds were the madecassic acid isomer and asiatic acid isomer (21). In OF, the expression of metabolic pathways such as terpenoid backbone biosynthesis, flavone and flavonol biosynthesis, and tyrosine metabolism were significantly down-regulated overall. Terpenoids were derived from the same C5 isoprene backbone produced from isopentenyl pyrophosphates (IPP) and dimethyl allyl pyrophosphates (DMAPP) and were used in the synthesis of terpene backbones by rearrangement and cyclisation reactions (22), where linalool, nerol and alpha-terpineol could be produced by further reactions in the biosynthesis of terpene backbones (23). The results revealed differences in flavor quality between different scented black teas (CH and OF) from a metabolomics perspective. However, the most critical contributors to this difference were still unknown, both in terms of non-volatile and volatile substances.
3.3. Analysis of key differential non-volatile metabolites among the three black teas
3.3.1. Analysis of key differential categories
Based on the percentage of metabolite categories, all non-volatile metabolites were further screened and a total of 173 metabolites were selected (Table 1). In terms of categories, the metabolites in the three black teas mainly consisted of amino acids and derivatives, phenolic acids, flavonoids, lipids, accounting for 7.69, 20.12, 52.66 and 19.53%, respectively. In terms of peak area, flavonoids accounted for a relatively large proportion of all key metabolites, reaching 96.30% in CK, and compared with CK, the percentage of peak area of flavonoids increased by 0.39 and 6.72% in CH and OF, respectively. The variation of flavonoids was determined by the thermal stability during the drying stage (17). Compared with CK, the peak area of lipids in CH was reduced by 0.43%. The lipids were important precursors of volatile metabolites in tea leaves (24). It was reported that lipids were degraded by enzymatic hydrolysis or oxidation of glycolipids and phospholipids to produce flavor volatiles during black tea processing (25), which was in accordance with our experimental results. The total peak area of amino acids and derivatives in OF was reduced by 5.18% compared to CK (Supplementary Table S1), which indicated that the Maillard reaction, deamidation polymerization and caramelization might occur during the scenting process (26).
Overall, there was a downward trend in the non-volatile metabolites of black tea after scenting. Compared to CK, phenolic acids were significantly up-regulated in CH (Log2FC = 31.66) whilst flavonoids were significantly down-regulated (Log2FC = −39.00). Amino acids and derivatives were significantly up-regulated in OF (Log2FC = 17.60) whilst flavonoids were significantly down-regulated (Log2FC = −30.43).
3.3.2. Analysis of key differential compounds
Based on the values of magnitude of the difference multiplier (Log2FC) in each comparison group (CK vs. CH, CK vs. OF), the substances ranked the top 20 of the key non-volatile differential metabolites were prioritized for further discussion. As illustrated in Figures 3A,B, the Log2FC of the selected metabolites ranged from −22.58 to 18.83 in CK vs. CH and − 14.62 to 18.03 in CK vs. OF. Based on |Log2FC| > 15, 7 and 4 substances were screened as key differential compounds in CH (Figure 3C) and OF (Figure 3D), respectively.
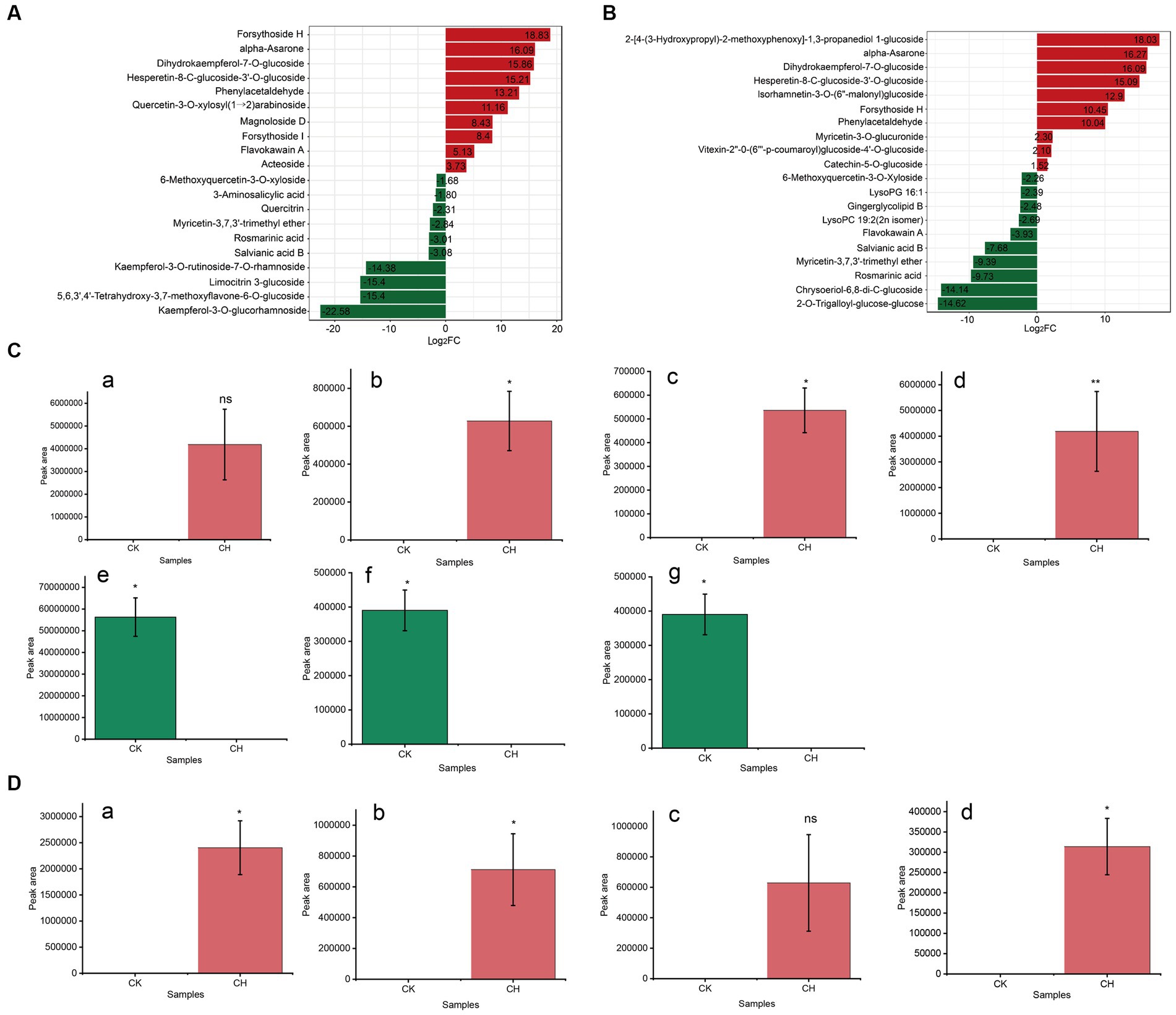
Figure 3. Analysis of key differential non-volatile metabolites in three types of black tea (CK, CH and OF). (A,B) Bar charts of the multiplicity of differences between different comparison groups (A. CK vs. CH, B. CK vs. OF); (C,D) Abundance histograms of key metabolites (|Log2FC| > 15, C. CK vs. CH; D. CK vs. OF). C-a, Forsythoside H; C-b, alpha-Asarone; C-c, Dihydrokaempferol-7-O-glucoside; C-d, Hes-peretin-8-C-glucoside-3’-O-glucoside; C-e, Kaempferol-3-O-glucorhamnoside; C-f, 5,6,3′,4’-Tetrahydroxy-3,7-dimethoxyflavone-6-O-glucoside; C-g, Limocitrin 3-Glucoside; D-a, 2-[4-(3-Hydroxypropyl)-2-methoxyphenoxy]-1,3-propanediol 1-glucoside; D-b, alpha-Asarone; D-c, Dihydrokaempferol-7-O-glucoside; D-d, Hesperetin-8-C-glucoside-3’-O-glucoside. * Indicated a significant difference between treated samples at the 0.05 level; ** indicated a significant difference at the 0.01 level and ‘ns’ indicated a non-significant difference.
The results revealed that the 11 substances were divided into two main groups, which were flavonoids and phenolic acids. Combined with the relevant information in Table 1, it was concluded that compared to CK, forsythoside H, alpha-asarone, dihydrokaempferol-7-O-glucoside, and hesperetin-8-C-glucoside-3’-O-glucoside in CH were increased, with FC levels of 465047.40, 69747.14, 59587.87, and 37838.48, respectively. Forsythoside H, a caffeoyl phenylethanoid glycoside (CPG) isolated from Forsythia suspense (Thunb.) Vahl, was a type of forsythoside and might possess anti-inflammatory activity. Alpha-asarone was a polyphenol with anti-bacterial and anti-inflammatory activities. It was worth noting that the level of dihydrokaempferol-7-O-glucoside was reported to reduce during the withering process of CK (17), which was inconsistent with our results. It suggested that reactions during different stages of black tea processing were not the same. On the contrary, the relative abundances of three substances, including kaempferol-3-O-glucorhamnoside, 5,6,3′,4′-tetrahydroxy-3,7-dimethoxyflavone-6-O-glucoside, and limocitrin 3-glucoside, decreased significantly and the metabolites were significantly down-regulated compared to CK. Limocitrin 3-glucoside, as a flavonoid, which was previously detected in hickory seeds (27). In OF, the relative abundances of 2-[4-(3-Hydroxypropyl)-2-methoxyphenoxy]-1,3-propanediol 1-glucoside, alpha-asarone, dihydrokaempferol-7-O-glucoside, and hesperetin-8-C-glucoside-3’-O-glucoside were significantly increased compared to CK and the FC values ranged from 34878.58 to 267109.16. Among them, the latter three substances were common to both scented teas.
In summary, flavonoids and phenolic acids were key differential non-volatile metabolites between unscented and scented black teas. The relative abundances of dihydrokaempferol-7-O-glucoside, hesperetin-8-C-glucoside-3’-O-glucoside, and alpha-asarone significantly increased in scented teas (CH & OF). There was a significant decrease in kaempferol-3-O-glucorhamnoside, 5,6,3′,4′-tetrahydroxy-3,7-dimethoxyflavone-6-O-glucoside, and limocitrin 3-glucoside in CH, while the relative abundance of 2-[4-(3-Hydroxypropyl)-2-methoxyphenoxy]-1,3-propanediol 1-glucoside was significantly increased in OF. This result was in general concordance with the results of KEGG metabolic pathways analysis (Figures 2H,I).
3.4. Analysis of key differential volatile metabolites among the three black teas
3.4.1. Analysis of key differential categories
Based on the percentage of metabolite categories, all volatile metabolites were further screened and 110 metabolites with aroma contribution were selected, including 11 alcohols, 9 ketones, 29 esters, 31 terpenoids, 14 heterocyclic compounds, 4 aldehydes, 5 aromatics, 3 acids, 3 nitrogen compounds, and 1 phenol (Table 2). It was consistent with a previous study which revealed that terpenoids were the most important aroma components in CK (28). The sum of relative abundances of esters, terpenoids, heterocyclic compounds, alcohols, ketones and aromatics was 97.08% in CK. Compared to CK, the relative abundances of heterocyclic compounds and alcohols in CH decreased by 19.62 and 11.49%, respectively, while aromatics increased by 21.23%. In OF, the relative abundances of heterocyclic compounds and esters increased by 17.21 and 13.59%, respectively, while the relative abundances of terpenoids and ketones showed a decreasing trend, reaching 14.87 and 10.31%, respectively (Supplementary Table S2). A previous study found a significant increase in both ketones and esters during the scenting of Osmanthus black tea (14), which was not consistent with the results of this study and may be influenced by the variety of tea leaves, tea processing parameters and scenting process.
Overall, compared to CK, the sum of key volatile metabolites was significantly up-regulated (Log2FC = 187.05) in CH and down-regulated (Log2FC = −77.28) in OF. In particular, esters, terpenoids, heterocyclic compounds, aldehydes, acids, nitrogen compounds were down-regulated, while alcohols, ketones, aromatics and phenols were up-regulated.
3.4.2. Analysis of key differential compounds
Based on the magnitude of Log2FC in each comparison group (CK vs. CH, CK vs. OF), the top 20 substances in the key volatile differential metabolites were selected preferentially and their FCs were shown in Figures 4A,B. It was found that the selected metabolites were significantly up-regulated in CH compared to CK, while those in OF were significantly down-regulated. Based on |Log2FC| > 6, six and five substances were screened as key differential compounds in CH (Figure 4C) and OF (Figure 4D), respectively.
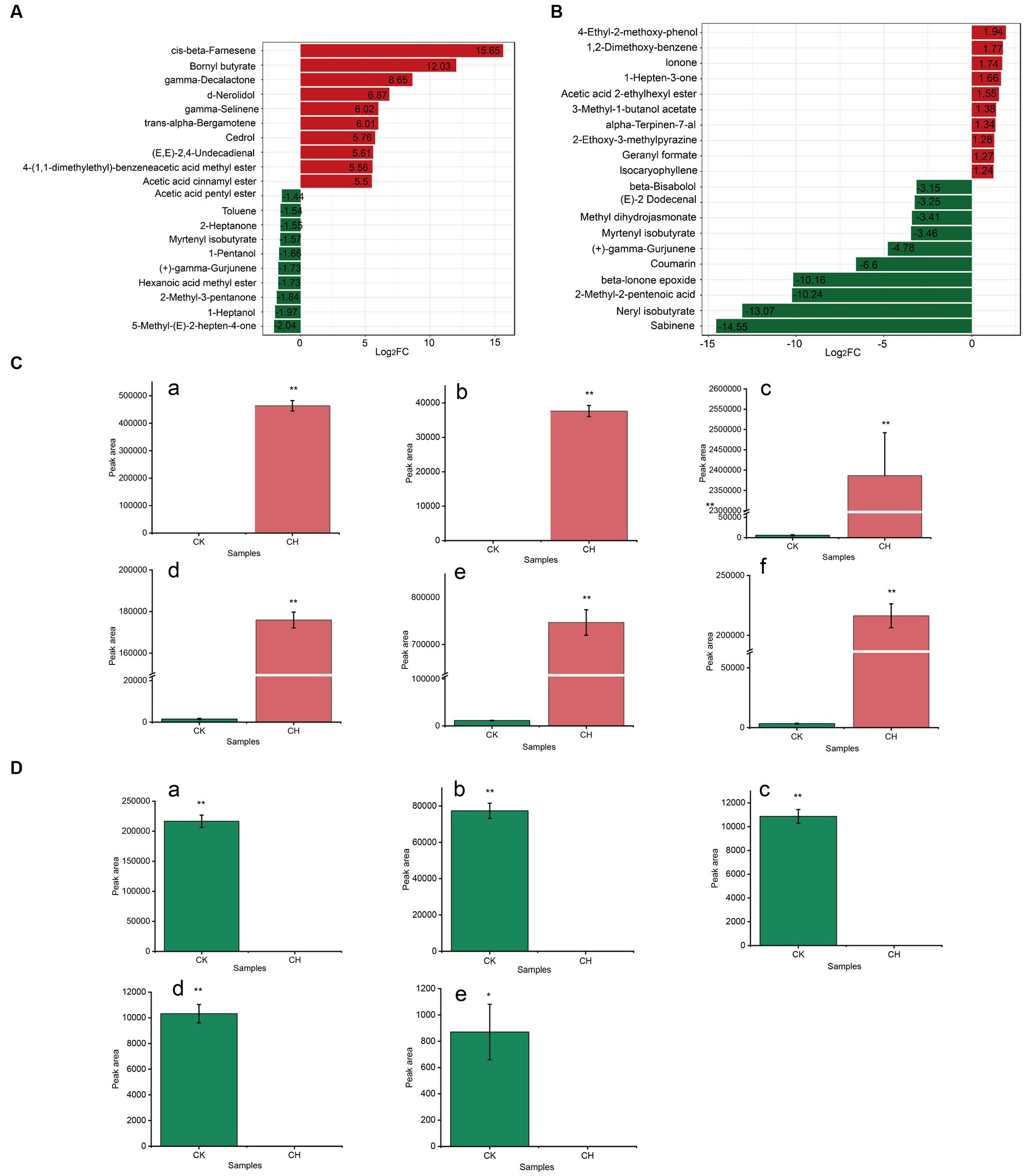
Figure 4. Analysis of key differential volatile metabolites in three types of black tea (CK, CH and OF). (A,B) Bar charts of the multiplicity of differences between different comparison groups (A. CK vs. CH, B. CK vs. OF); (C,D) Abundance histograms of key metabolites (|Log2FC| > 6, C. CK vs. CH; D. CK vs. OF). C-a, cis-beta-Farnesene; C-b, Bornyl butyrate; C-c, gamma-Decalactone; C-d, d-Nerolidol; C-e, gamma-Selinene; C-f, trans-alpha-Bergamotene; D-a, Sabinene; D-b, Neryl isobutyrate; D-c, 2-Methyl-2-pentenoic acid; D-d, beta-Ionone epoxide; D-e, Coumarin. * Indicated a significant difference between treated samples at the 0.05 level; ** indicated a significant difference at the 0.01 level.
Among them, the terpenoids and ester accounted for a greater number, with six and three varieties, respectively. Acids and heterocyclic compounds each accounted for one. Combined with the relevant information in Table 2, the relative abundances of cis-beta-farnesene, bornyl butyrate, gamma-decalactone, d-nerolidol, gamma-selinene, and trans-alpha-bergamotene were significantly increased in CH (compared to CK) and FC values reached 51533.83, 4183.31, 400.92, 116.77, 64.68, 64.57, respectively. The cis-beta-farnesene and trans-alpha-bergamotene were significantly associated with floral aroma (28, 29). Trans-alpha-bergamotene was a citrus odorant which was considered to be the most important sesquiterpene in the characteristic odour of Polygonum minus (30). Bornyl butyrate, which presented an herbaceous aroma (31), usually presented in the Aloysia triphylla and Aloysia citrodora.4 Gamma-decalactone, which delivered a pleasant peachy aroma, was regarded as one of the characteristic components contributing to the Osmanthus aroma of Osmanthus black tea (14). Nerolidol was considered to be one of the skeleton aroma components of CK (32). The decrease of nerolidol after scenting might be related to the drying process, as it was previously reported that there was a significant positive correlation between the content of nerolidol and the degree of roasting (33). Gamma-selinene, as a sesquiterpene with floral aroma, was detected in the essential oils from Chloranthus spicatus flowers (34).
Nevertheless, in OF, sabinene, neryl isobutyrate, 2-methyl-2-pentenoic acid, beta-ionone epoxide, and coumarin were significantly down-regulated (FC ≤ 0.01). Sabinene, a representative of the bicyclic monoterpenes, conveyed a typical citrus note (35). Beta-ionone epoxide (sweet berry aroma), an apo carotenoid monoterpenoid, was found decreased in summer green tea (Camellia sinensis L.) after yellowing (36) and steamed green tea after baking (37), which was consistent with the results of this study. Neryl isobutyrate rendered a sweet rose scent and 2-methyl-2-pentenoic acid offered a fruity note (31). Chen (38) and Su et al. (32) both revealed that coumarin was one of the key substances in the formation of the typical aroma of special grade Keemun black tea. The coumarin was described as a sweet grass and cherry blossom-like aroma, which was formed by intramolecular esterification of hydroxycinnamic acid following hydrolysis of 2-coumaric acid primeveroside (18). The reduction of coumarin in OF might be related to the drying process (39).
In conclusion, terpenes, including monoterpenes and sesquiterpenes, were the main differential category. The increase of cis-beta-farnesene and bornyl butyrate might contribute to an increased in floral, fruity and herbaceous aromas in CH. The decrease of sabinene and neryl isobutyrate might be related to the insignificant sweet fruity aroma in OF. The result was generally consistent with the results of KEGG metabolic pathways analysis (Figures 2H,I). As such, potential differential metabolites between the CK vs. CH and CK vs. OF comparator groups were characterized in scented teas with CK as the tea raw material.
4. Conclusion
The full-spectrum metabolomics (UPLC-MS/MS and GC–MS) adopted in this study permits a more comprehensive and objective characterization of the differences in non-volatile and volatile flavor metabolites among unscented and scented Congou black teas. The results indicated that the scenting process and the types of flowers had a significant effect on the flavor quality of Congou black tea. Flavonoids, phenolic acids and terpenoids were screened as key differential categories by PCA and PLS-DA, with most significant changes in terpenoids in different scented teas. Floral, fruity and herbaceous volatiles were significantly up-regulated in CH, whilst the sweet fruity volatiles were significantly down-regulated. This study is the first to characterize the flavor profiles of CH and OF in some detail, the results enrich the research on flavor metabolisms of scented black tea and has certain implications for the processing and quality control of scented black tea. Future research needs to broaden the range of other types of flowers and to extend this study to the other five major tea categories, so as to find the most suitable flowers for each tea category to better guide the production of scented teas.
Data availability statement
The original contributions presented in the study are included in the article/Supplementary material, further inquiries can be directed to the corresponding authors.
Author contributions
PT, J-QW, YG, and Y-QX: conceptualization. PT and J-QW: data curation and formal analysis and writing—review and editing. PT and Y-QX: funding acquisition. Y-FW, J-CJ, XM, and YZ: investigation. PT, J-QW, YG, and Y-QX: methodology. Y-QX: project administration and validation. YG and Y-QX: supervision. All authors have read and agreed to the published version of the manuscript.
Funding
This work was supported by the Agricultural and Social Development General Program of Hangzhou (20201203B100), the Key Research and Development Program of Zhejiang (2022C02033), the Central Public-interest Scientific Institution Basal Research Fund (Y2023XK11), and the China Agriculture Research System of MOF and MARA (CARS-19).
Conflict of interest
Y-FW was employed by Jingdezhen Jin Gui Yuan Agricultural Development Co Ltd.
The remaining authors declare that the research was conducted in the absence of any commercial or financial relationships that could be construed as a potential conflict of interest.
Publisher’s note
All claims expressed in this article are solely those of the authors and do not necessarily represent those of their affiliated organizations, or those of the publisher, the editors and the reviewers. Any product that may be evaluated in this article, or claim that may be made by its manufacturer, is not guaranteed or endorsed by the publisher.
Supplementary material
The Supplementary material for this article can be found online at: https://www.frontiersin.org/articles/10.3389/fnut.2023.1234807/full#supplementary-material
Abbreviations
CK, Congou black tea; CH, Chloranthus spicatus black tea; OF, Osmanthus black tea; UPLC-MS/MS, ultra-performance liquid chromatography tandem mass spectrometry; GC–MS, gas chromatography–mass spectrometry; ESI, electrospray ionization temperature; IS, ion spray; GSI I (II), ion source gases I (II); CUR, curtain gas; MRM, multiple reaction monitoring; QQQ, triple quadrupole; DP, declustering potential; CE, collision energy; MWDB, metware database; HS-SPME, headspace solid phase microextraction; DVB/CWR/PDMS, divinylbenzene/carboxen/polydimethylsiloxane; EI, electron bombardment ion source; SIM, selected ion detection mode; RI, retention index; RT, retention time; PCA, principal component analysis; PLS-DA, partial least squares-discriminant analysis; KEGG, kyoto encyclopedia of genes and genomes; MSEA, metabolite set enrichment analysis; VIP, variable importance in the project; FC, fold change; DA, differential abundance; MVA, cytoplasmic mevalonate; IPP, isopentenyl pyrophosphates; DMAPP, dimethyl allyl pyrophosphates; CPG, caffeoyl phenylethanoid glycoside.
Footnotes
References
1. Yi, MS, Wu, XT, Zhuang, W, Xia, L, Chen, Y, Zhao, R, et al. Tea consumption and health outcomes: umbrella review of meta-analyses of observational studies in humans. Mol Nutr Food Res. (2019) 63:e1900389. doi: 10.1002/mnfr.201900389
2. Persson, IAL, Josefsson, M, Persson, K, and Andersson, RGG. Tea flavanols inhibit angiotensin-converting enzyme activity and increase nitric oxide production in human endothelial cells. J Pharm Pharmacol. (2010) 58:1139–44. doi: 10.1211/jpp.58.8.0016
3. Hindmarch, I, Quinlan, PT, Moore, KL, and Parkin, C. The effects of black tea and other beverages on aspects of cognition and psychomotor performance. Psychopharmacology. (1998) 139:230–8. doi: 10.1007/s002130050709
4. Duffy, SJ, Keaney Jr, JF, Holbrook, M, Gokce, N, Swerdloff, PL, Frei, B, et al. Short- and long-term black tea consumption reverses endothelial dysfunction in patients with coronary artery disease. Monograph (American Heart Association). (2001) 104:151–6. doi: 10.1161/01.CIR.104.2.151
5. Jiang, H, Xu, WD, and Chen, QS. Evaluating aroma quality of black tea by an olfactory visualization system: selection of feature sensor using particle swarm optimization. Food Res Int. (2019) 126:108605. doi: 10.1016/j.foodres.2019.108605
6. Mao, SH, Lu, CQ, Li, MF, Ye, Y, Wei, X, and Tong, H. Identification of key aromatic compounds in congou black tea by partial least-square regression with variable importance of projection scores and gas chromatography–mass spectrometry/gas chromatography–olfactometry. J Sci Food Agric. (2018) 98:5278–86. doi: 10.1002/jsfa.9066
7. Liu, PP, Zheng, PC, Gong, ZM, Ye, F, Feng, L, Gao, SW, et al. Quality analysis and comprehensive evaluation of Chinese congou black tea. Food Sci. (2021) 42:195–205. doi: 10.7506/spkx1002-6630-20200611-150
8. Liu, Y, Chen, QC, Liu, DC, Yang, L, Hu, W, Kuang, L, et al. Multi-omics and enzyme activity analysis of flavour substances formation: major metabolic pathways alteration during congou black tea processing. Food Chem. (2023) 403:134263. doi: 10.1016/j.foodchem.2022.134263
9. Kang, SY, Yan, H, Zhu, Y, Liu, X, Lv, HP, Zhang, Y, et al. Identification and quantification of key odorants in the world's four most famous black teas. Food Res Int. (2023) 121:73–83. doi: 10.1016/j.foodres.2019.03.009
10. Zhang, L, Ho, CT, Zhou, J, Santos, JS, Armstrong, L, and Granato, D. Chemistry and biological activities of processed Camellia sinensis teas: a comprehensive review. Compr Rev Food Sci Food Saf. (2019) 18:1474–95. doi: 10.1111/1541-4337.12479
11. Dai, WD, Tan, JF, Lu, ML, Zhu, Y, Li, P, Peng, Q, et al. Metabolomics investigation reveals that 8-C N-Ethyl-2-pyrrolidinone-substituted Flavan-3-ols are potential marker compounds of stored white teas. J Agric Food Chem. (2018) 66:7209–18. doi: 10.1021/acs.jafc.8b02038
12. Li, HL, Chai, Z, Shen, GX, and Li, CY. Polyphenol profiles and antioxidant properties of ethanol extracts from Osmanthus fragrans (Thunb.) Lour Flowers. Pol J Food Nutr Sci. (2017) 67:317–25. doi: 10.1515/pjfns-2017-0008
13. Cai, X, Mai, RZ, Zou, JJ, Zhang, HY, Zeng, XL, Zheng, RR, et al. Analysis of aroma-active compounds in three sweet Osmanthus (Osmanthus fragrans) cultivars by GC-olfactometry and GC-MS. J Zhejiang Univ-Sc B. (2014) 15:638–48. doi: 10.1631/jzus.B1400058
14. Chen, HM, Shi, ZG, Di, TM, Hu, JH, Zhang, ZQ, and Zhang, XF. The scenting technology and aroma analysis by HS-SPME/GC-O-MS for Osmanthus black tea. Modern Food Sci Technol. (2018) 34:243–54. doi: 10.13982/j.mfst.1673-9078.2018.11.036
16. Chen, W, Wang, WS, Peng, M, Gong, L, Gao, Y, Wan, J, et al. Comparative and parallel genome-wide association studies for metabolic and agronomic traits in cereals. Nat Commun. (2016) 7:12767. doi: 10.1038/ncomms12767
17. Liu, F, Wang, Y, Corke, H, and Zhu, H. Dynamic changes in flavonoids content during congou black tea processing. LWT. (2022) 170:114073. doi: 10.1016/j.lwt.2022.114073
18. Yang, ZY, Baldermann, S, and Watanabe, N. Recent studies of the volatile compounds in tea. Food Res Int. (2013) 53:585–99. doi: 10.1016/j.foodres.2013.02.011
19. Liao, P, Hemmerlin, A, Bach, TJ, and Chye, ML. The potential of the mevalonate pathway for enhanced isoprenoid production. Biotechnol Adv. (2016) 34:697–713. doi: 10.1016/j.biotechadv.2016.03.005
20. Hemmerlin, A, Harwood, JL, and Bach, TJ. A raison d'être for two distinct pathways in the early steps of plant isoprenoid biosynthesis? Prog Lipid Res. (2012) 51:95–148. doi: 10.1016/j.plipres.2011.12.001
21. Gao, Y, Wang, JQ, Fu, YQ, Yin, JF, Shi, J, and Xu, YQ. Chemical composition, sensory properties and bioactivities of Castanopsis lamontii buds and mature leaves. Food Chem. (2020) 316:126370. doi: 10.1016/j.foodchem.2020.126370
22. Fei, XT, Qi, YC, Lei, Y, Wang, S, Hu, H, and Wei, A. Transcriptome and metabolome dynamics explain aroma differences between green and red prickly ash fruit. Foods. (2021) 10:391. doi: 10.3390/foods10020391
23. Deng, XJ, Huang, GH, Tu, Q, Zhou, H, Li, Y, Shi, H, et al. Evolution analysis of flavor-active compounds during artificial fermentation of Pu-erh tea. Food Chem. (2021) 357:129783. doi: 10.1016/j.foodchem.2021.129783
24. Zheng, XQ, Li, QS, Xiang, LP, and Liang, YR. Recent advances in volatiles of teas. Molecules. (2016) 21:338. doi: 10.3390/molecules21030338
25. Bhuyan, LP, and Mahanta, PK. Studies on fatty acid composition in tea Camellia sinensis. J Sci of Food Agric. (1989) 46:325–30. doi: 10.1002/jsfa.2740460310
27. Li, Y, Wang, JH, Wang, KT, Lyu, S, Ren, L, Huang, C, et al. Comparison analysis of widely-targeted metabolomics revealed the variation of potential astringent ingredients and their dynamic accumulation in the seed coats of both Carya cathayensis and Carya illinoinensis. Food Chem. (2022) 374:131688. doi: 10.1016/j.foodchem.2021.131688
28. Xue, JJ, Guo, GY, Liu, PP, Chen, L, Wang, W, Zhang, J, et al. Identification of aroma-active compounds responsible for the floral and sweet odors of congou black teas using gas chromatography-mass spectrometry/olfactometry, odor activity value, and chemometrics. J Sci of Food Agric. (2022) 102:5399–410. doi: 10.1002/jsfa.11893
29. Chen, MJ, Guo, L, Zhou, HW, Guo, Y, Zhang, Y, Lin, Z, et al. Absolute quantitative volatile measurement from fresh tea leaves and the derived teas revealed contributions of postharvest synthesis of endogenous volatiles for the aroma quality of made teas. Appl Sci-Basel. (2021) 11:613. doi: 10.3390/app11020613
30. Rusdi, NA, Goh, HH, and Baharum, SN. GC-MS/Olfactometric characterisation and aroma extraction dilution analysis of aroma active compounds in Polygonum minus essential oil. Plant Omics. (2016) 9:289–91. doi: 10.21475/poj.16.09.04.p7901
32. Su, D, He, JJ, Zhou, YZ, Li, YL, and Zhou, HJ. Aroma effects of key volatile compounds in Keemun black tea at different grades: HS-SPME-GC-MS, sensory evaluation, and chemometrics. Food Chem. (2022) 373:131587. doi: 10.1002/ffj.3725
33. Yue, CA, Li, WJ, Li, C, Wang, Z, Peng, H, and Yang, P. Differential characterization of volatile components and aroma sensory properties of different types of Hehong tea (congou black tea). Flavour Frag J. (2023) 38:61–72. doi: 10.1016/j.foodchem.2021.131587
34. Chang-heng, ZHAO, Yong-xiang, WU, Zhi-bing, WAN, Meng-xue, WU, Ya-li, WANG, Tian-zhong, CAO, et al. Effect of different drying methods on composition and biological activities of essential oils from Chloranthus spicatus flowers. Nat Prod Res Dev. (2020) 32:1030–7. doi: 10.16333/j.1001-6880.2020.6.017
36. Wei, YM, Yin, XC, Wu, HT, Zhao, M, Huang, J, Zhang, J, et al. Improving the flavor of summer green tea (Camellia sinensis L.) using the yellowing process. Food Chem. (2022) 388:132982. doi: 10.1016/j.foodchem.2022.132982
37. Wang, JQ, Fu, YQ, Chen, JX, Wang, F, Feng, ZH, Yin, JF, et al. Effects of baking treatment on the sensory quality and physicochemical properties of green tea with different processing methods. Food Chem. (2022) 380:132217. doi: 10.1016/j.foodchem.2022.132217
38. Chen, D. The study of flavor characteristic of premium Keemum black tea. Beijing, China: China Agricultural University (2017). doi: 10.27628/d.cnki.gzndu.2017.000114
39. Ho, CT, Zheng, X, and Li, SM. Tea aroma formation. Food Sci Hum Well. (2015) 4:9–27. doi: 10.1016/j.fshw.2015.04.001
Glossary
Keywords: scented black teas, Osmanthus fragrans (Thunb.) Lour., Chloranthus spicatus , non-volatile metabolites, volatile metabolites
Citation: Tang P, Wang J-Q, Wang Y-F, Jin J-C, Meng X, Zhu Y, Gao Y and Xu Y-Q (2023) Comparison analysis of full-spectrum metabolomics revealed on the variation of potential metabolites of unscented, Chloranthus spicatus scented, and Osmanthus fragrans (Thunb.) Lour. scented Congou black teas. Front. Nutr. 10:1234807. doi: 10.3389/fnut.2023.1234807
Edited by:
Dandan Pu, Beijing Technology and Business University, ChinaReviewed by:
Hasim Kelebek, Adana Science and Technology University, TürkiyeLishuang Lv, Nanjing Normal University, China
Copyright © 2023 Tang, Wang, Wang, Jin, Meng, Zhu, Gao and Xu. This is an open-access article distributed under the terms of the Creative Commons Attribution License (CC BY). The use, distribution or reproduction in other forums is permitted, provided the original author(s) and the copyright owner(s) are credited and that the original publication in this journal is cited, in accordance with accepted academic practice. No use, distribution or reproduction is permitted which does not comply with these terms.
*Correspondence: Ying Gao, eWluZ2dhb0B0cmljYWFzLmNvbQ==; Yong-Quan Xu, eXF4MzNAMTI2LmNvbQ==
†These authors have contributed equally to this work