- 1Platform of Clinical Investigation Department, University Hospital Clermont-Ferrand, INSERM CIC, Clermont-Ferrand, France
- 2Department of Pharmacology, University Clermont Auvergne, Inserm, Clermont-Ferrand, France
- 3Sanofi, Gentilly, France
- 4Clinical Research and Innovation Department, University Hospital Clermont-Ferrand, Clermont-Ferrand, France
- 5Université Clermont Auvergne, Clermont Auvergne INP, CNRS, CHU Clermont Ferrand, ICCF, Clermont-Ferrand, France
- 6Université Clermont Auvergne, CHU, CNRS, Clermont Auvergne INP, Institut Pascal, Clermont-Ferrand, France
Introduction: This randomized, controlled, single-blinded trial assessed the effect of magnesium (Mg)-Teadiola (Mg, vitamins B6, B9, B12, Rhodiola, and green tea/L-theanine) versus placebo on the brain response to stressful thermal stimulus in chronically stressed, but otherwise healthy subjects. Impacts on stress-related quality-of-life parameters (depression, anxiety, sleep, and perception of pain) were also explored.
Methods: The study recruited a total of 40 adults (20 per group), suffering from stress for more than 1 month and scaling ≥14 points on the Depression Anxiety Stress Scale (DASS)-42 questionnaire at the time of inclusion. Individuals received oral Mg-Teadiola or placebo for 28 days (D). fMRI analysis was used to visualize the interplay between stress and pain cerebral matrices, using thermal stress model, at baseline (D0) and after D28.
Results: Based on blood-oxygen-level-dependent (BOLD) signal variations during the stress stimulation (before pain perception), a significantly increased activation between D0 and D28 was observed for left and right frontal area (p = 0.001 and p = 0.002, respectively), left and right anterior cingulate cortex (ACC) (p = 0.035 and p = 0.04, respectively), and left and right insula (p = 0.034 and p = 0.0402, respectively) in Mg-Teadiola versus placebo group. During thermal pain stimulation, a significantly diminished activation of the pain matrix was observed between D0 and D28, for left and right prefrontal area (both p = 0.001), left and right insula (p = 0.008 and p = 0.019, respectively), and left and right ventral striatum (both p = 0.001) was observed in Mg-Teadiola versus placebo group. These results reinforce the clinical observations, showing a perceived benefit of Mg-Teadiola on several parameters. After 1 month of treatment, DASS-42 stress score significantly decreased in Mg-Teadiola group [effect size (ES) −0.46 (−0.91; −0.01), p = 0.048]. Similar reductions were observed on D14 (p = 0.011) and D56 (p = 0.008). Sensitivity to cold also improved from D0 to D28 for Mg-Teadiola versus placebo [ES 0.47 (0.02; 0.92) p = 0.042].
Conclusion: Supplementation with Mg-Teadiola reduced stress on D28 in chronically stressed but otherwise healthy individuals and modulated the stress and pain cerebral matrices during stressful thermal stimulus.
1. Introduction
Stress has an increasing prevalence in modern societies (1), reaching 29.6% in the general population (2), and its deleterious health consequences are numerous at individual and societal levels (3–8). Vitamins (e.g., vitamin B6) and herbal extracts such as green tea/L-theanine, Rhodiola, ashwagandha, and saffron extracts (9–11) have shown to possess stress– and anxiety-relieving effects (12–19). Previous clinical studies demonstrated that Mg-Teadiola (a combination of Mg; vitamins B6, B9, and B12; Rhodiola, and green tea extracts) significantly alleviated subjective stress and mood responses (20, 21) and increased spectral theta brain activity, thereby increasing the attentional capacity (22) under acute stress conditions in healthy individuals (20, 21). Further, a recent placebo-controlled, randomized clinical trial showed that Mg-Teadiola significantly decreased stress scores [using the validated Depression Anxiety Stress Scale (DASS)-42 questionnaire] following 14 and 28 days of treatment in stressed but otherwise healthy individuals. Interestingly, a significant reduction in sensitivity to cold pain and a trend for lower sensitivity to warm pain was also reported (23). The way Mg-Teadiola or its components modulate both stress and pain is yet to be explored.
The relationship between stress and perception of pain has been reported earlier (24). Chronic stress and pain share neural circuits that operate in the amygdala, the hippocampus, and the prefrontal cortex (PFC) (25), and cortisol plays an essential role in the PFC-dependent regulation of the hypothalamic–pituitary–adrenal (HPA) axis and in the processing of emotion-related information by the amygdala (26). Usually, the response toward the similar psychosocial stressors or similar pain intensity varies significantly among different individuals based on differences in their executive functional capacities, self-control, and self-regulation. Executive functions that are generated in PFC are functionally connected to the limbic system and are regulated by the hippocampal-PFC pathway. This pathway is also essential for emotional regulation, which is also vulnerable to dysregulation by chronic stress and pain. Though a short-term stress is generally adaptive, a repeated or continued exposure to psychosocial stressors may lead to the impairment of the mechanisms of self-regulation and self-control, resulting in maladaptive behavior (e.g., vulnerability to adverse emotional and stress experience and exaggerated pain perception) (24).
Functional neuroimaging technique [functional magnetic resonance imaging (fMRI)] (27, 28) represents an important tool to investigate the beneficial impact of Mg-Teadiola at the brain level as it is the primary organ involved in the interplay between stress and pain pathways. fMRI is a noninvasive imaging technique that can be used for mapping brain cerebral areas, neural circuits, and networks critical to the stress response and also to pain integration. The fMRI technique allows to study the stress response in human brains (29). Given the importance of executive functioning in the stress–pain interplay, and because it is impaired under stress, the true efficacy of executive functioning should be evaluated under stressful conditions (30). For this, several paradigms have been developed to induce acute stress in fMRI settings (29), and these underline the complexity of activation of brain areas involved in emotion and endocrine response. Anticipation of the fMRI environment itself may serve as a stressful variable experience (31). Concerning the brain signature of pain (32, 33), the context and emotional status of the individual can influence pain perception, and reciprocal neural, endocrine, and immune interactions exist between pain and stress (34).
The primary study demonstrated the beneficial effects of Mg-Teadiola versus placebo on stress and pain perception in chronically stressed, otherwise healthy individuals (23). This ancillary study evaluated—with fMRI—the cerebral effect of Mg-Teadiola versus placebo in stressed but otherwise healthy individuals and further explored their neural activity when exposed to an acute physical stress (thermal stimulus).
2. Materials and methods
2.1. Study setting
This section has been previously described in a recent article (23). This was a 28-day, randomized, single-blinded, placebo-controlled, parallel-group study. The study was conducted at the Platform of Clinical Investigation Center, University Hospital, Clermont-Ferrand, France from July 2020 to July 2021.
The study was organized in five visits (Figures 1A,B): inclusion visit [visit 1, 14 days (D) before visit 2 (D0–14)], randomization visit [D0 (visit 2)], two follow-up visits [D14 (visit 3) and D28 (visit 4)], and end-of-study visit [D56 (visit 5)].
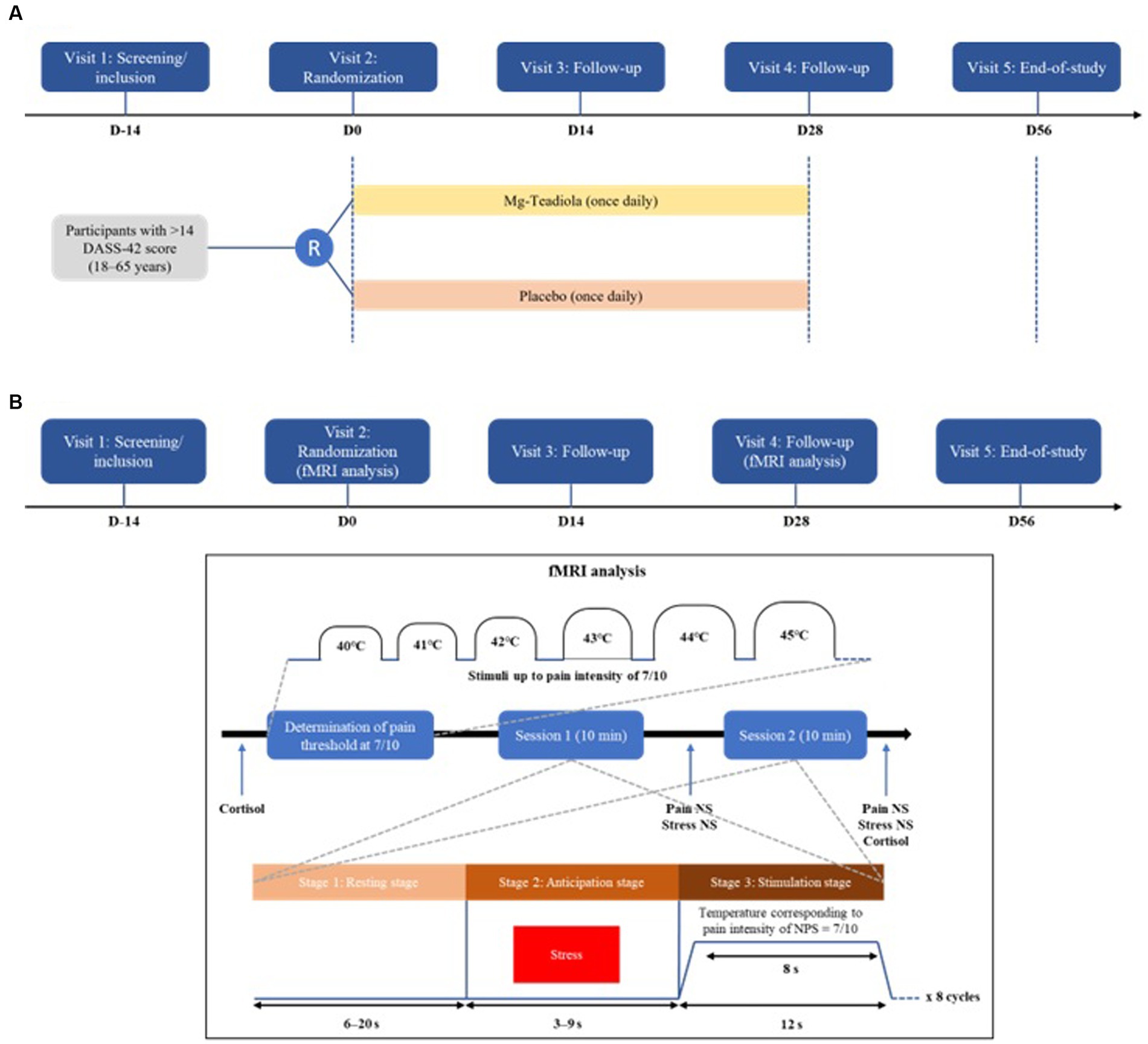
Figure 1. Study design and thermal pain stimulus cycles (A) Study design (B) Thermal pain stimulus cycles Mg-Teadiola, a combination of 150 mg magnesium (Mg), 0.7 mg vitamin B6, 0.1 mg vitamin B9, 1.25 μg vitamin B12, 222 mg of Rhodiola extract, and 125 mg of green tea extract including 50 mg of L-theanine. D, day; DASS-42, Depression Anxiety Stress Scale; Mg, magnesium; NPS, Neuropathic Pain Scale; NS, numerical scale.
2.2. Individuals
All individuals were screened from the volunteer database of the Platform of Clinical Investigation Center, University Hospital, Inserm 1,405 in Clermont-Ferrand, France. This is an ancillary study of a primary study by Noah et al. (23) wherein 40 volunteers participated in the fMRI study (23).
The individuals gave their informed consent before participation in the study. Individuals were men or women between 18 and 65 years of age. At the time of inclusion, individuals reporting stress for at least 1 month, scaling greater than or equal to 14 on the DASS-42 stress questionnaire, and not ready for introducing any new treatment, diet, or use of any new analgesic and anti-inflammatory drugs, were included in the study. Individuals who were contraindicated to performing MRI (i.e., having claustrophobia, hearing aid, pacemaker, or brain clip), contraindicated in taking Mg (a plasma Mg >1.07 mmol/L), with moderate or severe kidney failure, with an inability to distinguish colors during the MRI and an inability to distinguish sensation resulting from nociceptive stimuli during psychometric tests, were excluded from the study. All the inclusion and exclusion criteria are detailed in the Supplementary Information 1.
2.3. Randomization, masking, and treatment
Details of this section have been reported earlier (23). Briefly, each participant received orally either Mg-Teadiola or placebo treatment, once a day in the morning for 28 days. The attribution of treatment between the two arms was equal (1:1). Mg-Teadiola tablet is composed of 150 mg of elemental Mg, 0.7 mg of vitamin B6, 0.1 mg of vitamin B9, 1.25 μg of vitamin B12, 222 mg of Rhodiola (Rhodiola rosea L.) extract, and 125 mg of green tea (Camellia sinensis [L.] Kuntze) extract (containing 50 mg of L-theanine) (35). This product was provided by Sanofi-Aventis Group. Placebo was a tablet composed of only excipients.
The allocation of treatments followed a predefined randomization plan and was performed by an individual who did not contribute to the study protocol. To avoid bias, a randomization list was placed in a sealed envelope and was blinded from the individuals, investigator, and other personnel involved in this study. Although the placebo and drug were distinguishable by appearance, the identity of placebo and drug was not revealed to the patients. The randomization code was computer-generated through randomly permuted blocks. Within each block, the number of participants allocated to each of the two treatment arms was equal (23).
2.3.1. Study objectives
The primary objective of the parent study was to demonstrate the efficacy of Mg-Teadiola in managing stress in healthy individuals (23). This ancillary study evaluated the effect of Mg-Teadiola on brain areas activation related to stress (thermal stimulus) in healthy volunteers after 28 days of use. Other objectives were the evaluation of the impact of supplementation on stress, anxiety, depression, and sleep and its effects on biological parameters such as the levels of salivary cortisol and Mg levels in plasma, erythrocyte, and urine in the volunteers selected for the study.
2.4. Endpoints
The details of the primary endpoint (change in the DASS-42 stress scores from baseline to D28) and secondary endpoints (change in the stress, anxiety, and depression scores from baseline to D14 and/or D56 using DASS-42), of the parent study have been reported earlier (23). In the present ancillary study, main endpoint was the changes in cerebral areas (stress matrix, pain matrix) activation related to stress during the fMRI between baseline (D0) and D28 with Mg-Teadiola as compared with placebo.
Other endpoints included (i) the change in the DASS-42 stress subscale scores from D0 to D14, D28, and D56; (ii) the change in the DASS-42 anxiety subscale scores from D0 to D14, D28, and D56; (iii) the change in the DASS-42 depression subscale scores from D0 to D14, D28, and D56; (iv) the change in the Pain Catastrophizing Scale (PCS) scores from D0 to D14, D28, and D56; (v) the change in the Pittsburg Sleep Quality Index (PSQI) scores from D0 to D14, D28, and D56; (vi) the change in the quantitative somatosensory thermotest (QST) temperature from D0 to D28; (vii) the change in levels of salivary cortisol from D0 to D28; (viii) the change in levels of Mg in blood and urine from baseline to D14, D28, and D56; and (ix) the frequency of adverse events (AEs).
2.5. Study measurements
2.5.1. Functional magnetic resonance imaging
Experiments were conducted on a Siemens Magnetom Vida 3.0 T using a 64-channel head coil, with individuals lying supine. Initial localizer images were acquired in three planes as a reference for slice positioning for subsequent fMRI studies. A standard whole-brain gradient echo planar imaging sequence was used for the functional scans (repetition time = 2,000 ms; echo time = 30 ms; 300 volumes field of view = 192 × 192 mm2, matrix = 128 × 128, voxel size = 2.0 × 2.0 × 2.0 mm3, simultaneous multislice (SMS) accelerator factor 2, acquisition time = 10 min). Two functional runs were acquired during thermal stimulation. A high-resolution volumetric three-dimensional (3D) T1-weighted acquisition was performed—for anatomical overlay of activation—in the same session as the functional scans (magnetization-prepared rapid acquisition gradient echo [MPRAGE] sequence with repetition time = 1,800 ms, echo time = 2.46 ms, inversion time = 900 ms, field of view = 240 × 240 mm2, matrix = 512 × 512, voxel size = 0.4 × 0.4 × 0.9 mm3, acquisition time = 3 min 38 s). To achieve synchronization, the trigger output of the scanner was used to initialize the fMRI paradigm and triggers from contact heat-evoked potential stimulation and the scanner were recorded together. fMRI sequences were assessed in the following order: anatomical scout, 3D brain volume, fMRI blood-oxygen-level-dependent (BOLD) sequences echo planar imaging (thermal stimulation).
2.5.2. Evoked thermal pain stimulation
During fMRI sessions, volunteers were comfortably lying on the examination table of the MRI scanner with the thermode strapped on the dominant hand for thermal tests. First, the pain threshold—defined as the temperature where the stimulation becomes painful—was determined after an increase of temperature until pain reached 7 out of 10 on the numerical scale (Figures 1A,B). The stimulation paradigm consisted of eight cycles followed by 6–20 s rest followed by an anticipation visual stress challenge before pain stimuli. During stress challenge (3–9 s), the temperature of the thermode was 32°C and a red color was presented to individuals followed immediately by pain stimuli. Stimuli at the noxious stimulation temperature lasted 8 s. The cerebral “stress” matrix and “pain” matrix were identified using the images acquired during these two respective sequences (i.e., stress challenge, then pain stimulus). fMRI BOLD acquisitions were repeated twice for a global duration of 20 min.
2.5.3. The Depression Anxiety Stress Scale-42 questionnaire
This questionnaire is an auto-evaluation of 42 items assessing the negative emotional states over the last week. It allows the evaluation of the mental health owing to 3 scales composed of 14 items: depression, anxiety, and stress (36, 37). For each question, individuals were graded between 0 “not present” and 3 “very frequently present”. Stress and pain scores were categorized as 0–14 “normal”, 15–18 “mild”, 19–25 “moderate”, 26–33 “severe”, or 34+ “extremely severe”.
2.5.4. Quantitative somatosensory thermotest
Quantitative somatosensory thermotest (38) with an advanced thermal stimulator thermode connected to a Medoc PATHWAY (Medoc Ltd., Ramat Yishay, Israel) was used to apply thermal stimuli. The stimuli were delivered to the thenar eminence of the dominant hand from a D0 value of 32°C, and the chosen paradigm was applied. QST testing included thermal detection and thermal pain thresholds: cold detection and warm detection thresholds and cold pain and warm pain thresholds. From the D0 value of 32°C, the Medoc PATHWAY delivered an adjustable temperature peak (in cold and heat, depending upon a regular slope of 1°C) and was controlled by rapid feedback. This device was used to evaluate the thermal detection (when the individual began to feel the thermal change) and pain threshold (when the individual began to feel pain) to heat and cold by calculating the mean of three measures.
2.5.5. Pain catastrophizing scale
The PCS is an evaluation of 13 items in which the individuals describe how they feel during a pain experience (39). It allows to assess the type of thought and emotion related to pain. For each question, individuals were graded between 0 “not at all” and 4 “all the time”. The final score was a sum of the scores for each question. A high value represented a higher catastrophism.
2.5.6. The Pittsburg Sleep Quality Index
The PSQI is an evaluation of 19 items measuring sleep quality during the last month (40). It allows to assess seven parameters: subjective sleep quality, sleep latency, sleep duration, usual sleep efficiency, sleep disorders, use of a sleep medication, and poor daytime fitness. The global score was a sum of these domains and varied from 0–21. A high score represented a higher alteration of the quality of sleep.
2.5.7. Salivary collection
Salivary collection was done before D0 and after fMRI (D28) to assess free cortisol, and the change in the salivary cortisol concentration from D0 to D28 was determined using a radioimmunoassay (Gamma Coat, DiaSorin, Stillwater, MN, United States) with a modified procedure after dichloromethane extraction as previously described (41). The limit of quantification was 2 nmol/L. The intra-assay coefficients of variation (CVs) were 7.1 and 3.0% at 10.1 and 22.6 nmol/L, respectively. The inter-assay CVs were 12.7 and 10.2% at 8.7 and 48.0 nmol/L, respectively.
2.5.8. Magnesium assays
Magnesium assays were assessed by taking a total of 40 mL of venous blood from each patient and collected into heparin tube with gel (for serum Mg) or a heparin tube without gel (for erythrocyte Mg). Urinary Mg levels were measured and collected for 24 h, a day before visits 2, 3, 4, and 5. The tubes were sent to the biochemistry department of the University Hospital of Clermont-Ferrand for assay. Urinary and serum Mg levels were analyzed using the Dimension Vista® System Flex® Mg reagent cartridge (MAGNETOM Vida from Siemens). This is a modified version of the methyl thymol blue complexometric procedure. Methyl thymol blue forms a blue complex in the presence of Mg, and the amount of complex formed can be measured using a bichromatic endpoint method to determine the concentration of Mg. Erythrocyte Mg concentration was measured using a colorimetric method based on the formation of a colored complex of Mg with Xylidyl blue reagent in alkaline solution (Eurofins Biomnis, Lyon, France) (42).
2.5.9. Safety
All AEs were reported and analyzed for each visit according to the intensity, duration, and evolution to determine if the AEs were due to the study combination.
3. Statistical analysis
3.1. Sample size calculation
A sample size estimation has been reported in the primary study (23). As this was an ancillary study, no sample estimation was assessed. However, according to (i) previous results reported in the literature; (ii) CONSORT 2010 statement, extension to randomized pilot and feasibility trials; and (iii) Cohen’s recommendations, which define effect-size bounds as small (effect size [ES]: 0.2), medium (ES: 0.5), and large (ES: 0.8, “grossly perceptible and therefore large”), we estimated that 20 individuals per arm would allow to fulfill the primary objective of this study with a satisfactory statistical power as sample sizes of 40 are generally able to detect regions with high ESs (43).
3.2. Statistical methods
3.2.1. Functional magnetic resonance imaging data analysis
The fMRI data were preprocessed and analyzed using the Statistical Parametric Mapping software (44) on MATLAB R2018b (Mathworks Inc., Natick, MA, United States). The anatomical scan was spatially normalized to the avg152 T1-weighted brain template defined by the Montreal Neurological Institute using the default parameters (nonlinear transformation). Functional volumes were temporally and spatially realigned and normalized (using the combination of deformation field, co-registered structural and sliced functional images) and smoothed with an isotropic Gaussian kernel (full-width at half-maximum = 6 mm).
For each participant and each time point (D0 and D28), first-level statistical parametric maps (SPMs) were first generated using the general linear model to describe the variability of the data on a voxel-by-voxel basis. The model consisted of a boxcar function, using the stress-related and rest-related blocks for stress stimulation and the pain-related and rest-related blocks for pain stimulation as regressors of interest, convolved with the canonical SPM hemodynamic response function. The six motion regressors (translation and rotation) were also included in the model. Functional data were filtered with a 128-s high-pass filter. The contrasts between stimulations and rest were then generated.
Statistical parametric maps for each experimental factor and each participant calculated at the first level were then entered in a second-level two-factor analysis of variance (ANOVA) (group factor and time-point factor with repeated measure). Then, BOLD response amplitude for stress and pain stimulation were assessed for a significant decrease between D28 and D0 sessions and between the two groups—placebo and Mg-Teadiola. All statistical comparisons were performed with a voxel wise threshold of p < 0.05 and a cluster extent threshold of five voxels.
For a given zone of interest and based on previous ANOVA statistical results, we extracted each participant’s percent signal changes with the contrast stimulation-rest at D0 higher than stimulation-rest at D28 for the two groups using MarsBaR software (45). This beta analysis allowed to test for the first time a correlation between the BOLD signal variation between D28 and D0 (D0 > D28) and the difference in the DASS-42 stress subscale scores between D28 and D0 for both Mg-Teadiola and placebo groups.
3.2.2. Clinical data analysis
Continuous data were expressed as mean and standard deviation (SD). The Shapiro–Wilk test was used to analyze the assumption of the Gaussian distribution. The comparisons between Mg-Teadiola and placebo groups at D28 were performed using the analysis of covariance adjusted on D0 value of the dependent endpoint. The results were expressed using ES and 95% confidence interval (CI), and the clinically relevant difference was defined based on judgment of expert clinician. When appropriate, a logarithmic transformation of the dependent variables was proposed. Similarly, the comparisons between randomization groups at D14 and D56 were carried out. No adjustments were made for multiple comparisons. Because of the potential for type I error due to multiple comparisons, the findings for analyses of secondary analyses were interpreted as exploratory with no adjustment made for multiple comparisons. Statistical analysis was performed with Stata software (version 15, Stata Corp., College Station, TX, United States).
4. Results
4.1. Individuals and baseline characteristics
Out of 53 individuals screened, 40 were randomized (Mg-Teadiola: n = 20; placebo: n = 20, Figure 2), and all individuals completed the study. Overall, baseline characteristics (Table 1) were comparable between the two groups. Median age was 22.9 ± 4.4 years in the Mg-Teadiola group and 23.7 ± 6.5 years in the placebo group. Mean body mass indices (Mg-Teadiola: 22.5 ± 4.3; placebo: 22.9 ± 3.8) as well as psychological profiles were comparable in both groups. No relevant psychological disturbances were observed.
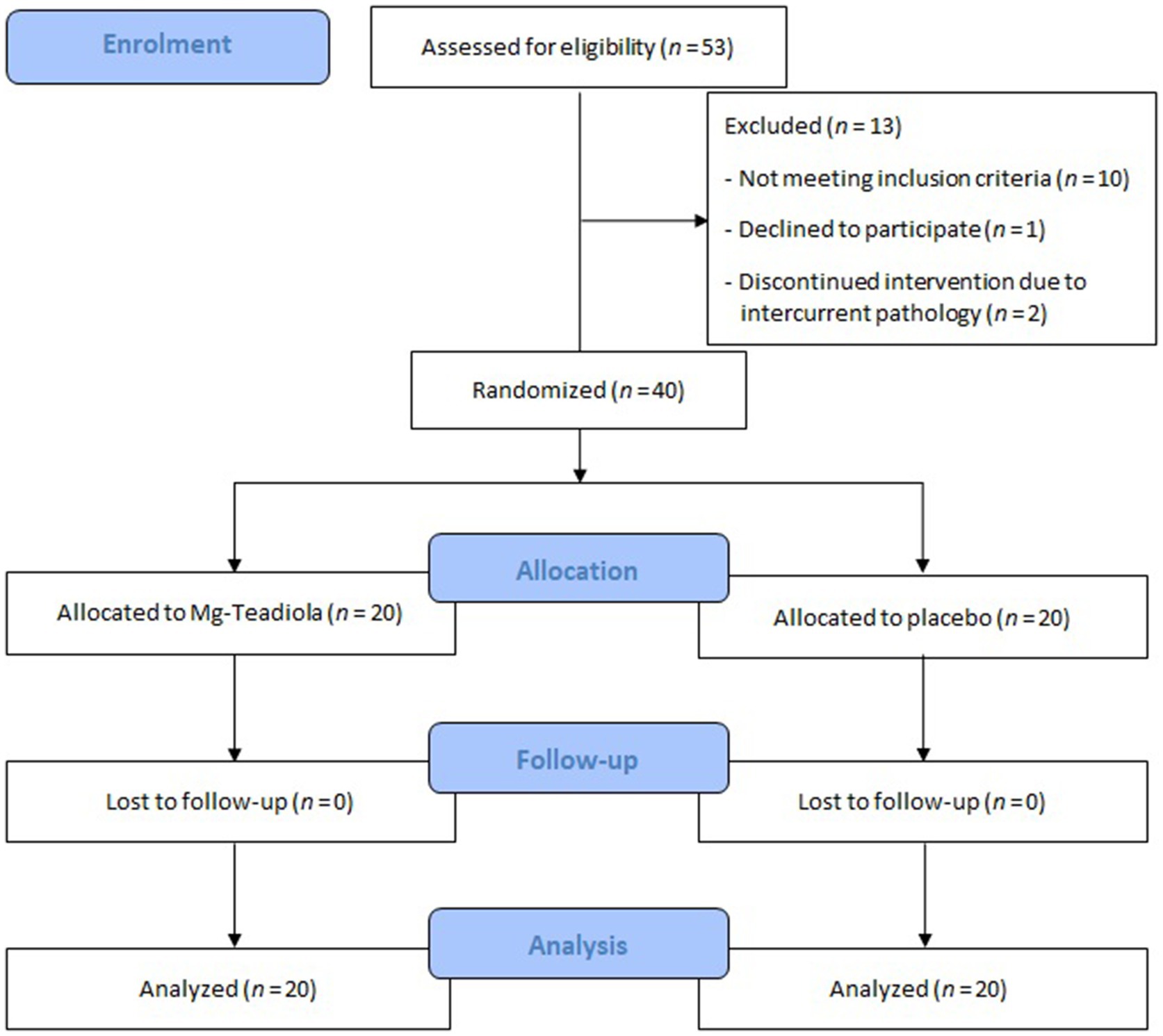
Figure 2. Patient disposition Mg-Teadiola, combination of 150 mg Mg, 0.7 mg vitamin B6, 0.1 mg vitamin B9, 1.25 μg vitamin B12, 222 mg of Rhodiola extract, and 125 mg of green tea extract including 50 mg of L-theanine. Mg, magnesium.
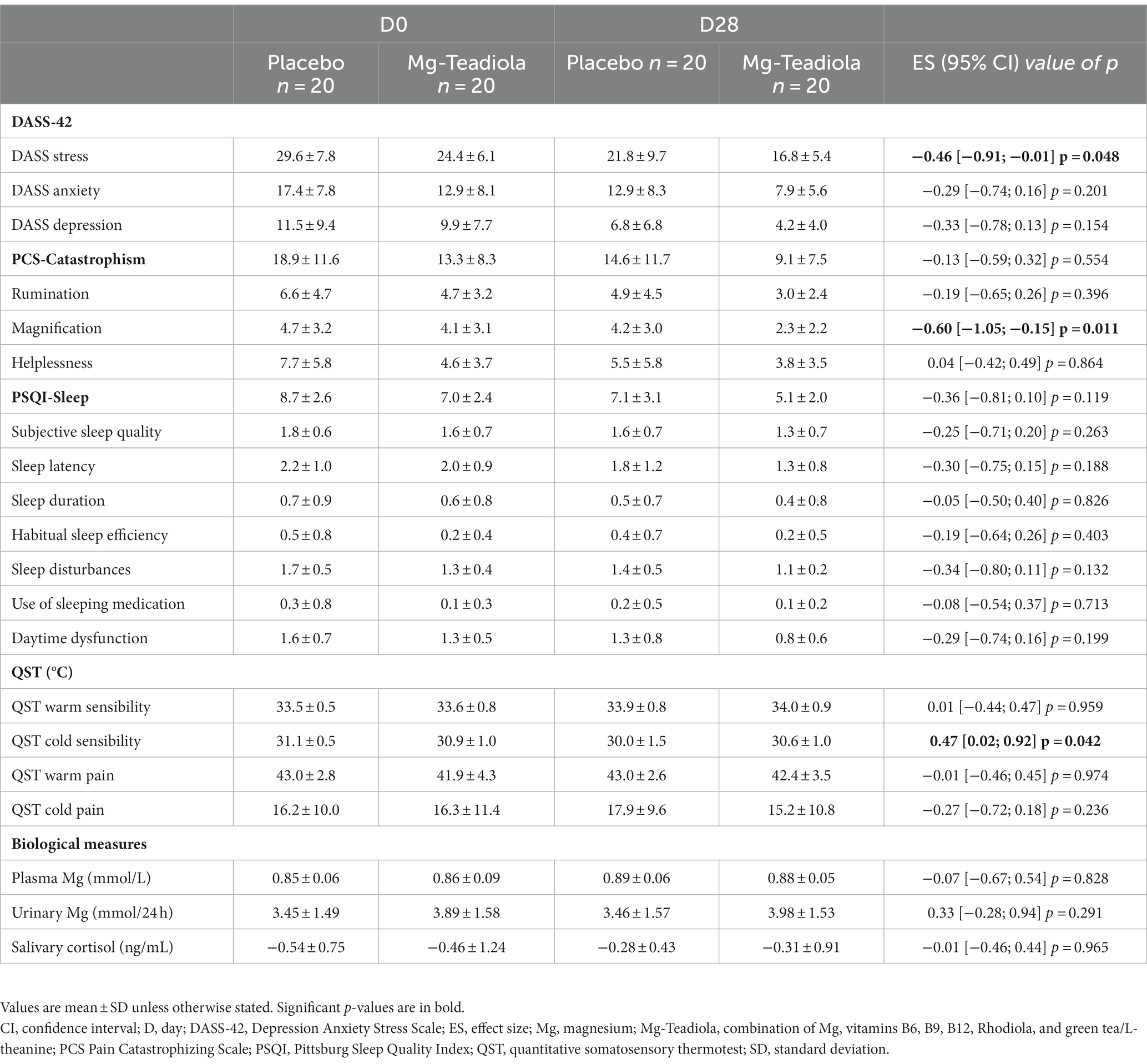
Table 1. Depression Anxiety Stress Scale (DASS)-42, quantitative somatosensory thermotest (QST), and biological measures following supplementation with Mg-Teadiola versus placebo at visit 2 (baseline, D0) and visit 4 (D28).
4.2. Functional magnetic resonance imaging outcomes
At D0, during acute stress stimulation for all individuals, robust BOLD signals were seen in cortical regions, mainly the left and right occipital cortices. In addition, during acute pain stimulation, robust BOLD signals were seen in cortical regions typically involved in pain processing. These areas included the prefrontal cortices, anterior cingulate cortex (ACC) (anterior, medium, and dorsal), and insula (Figures 3, 4).
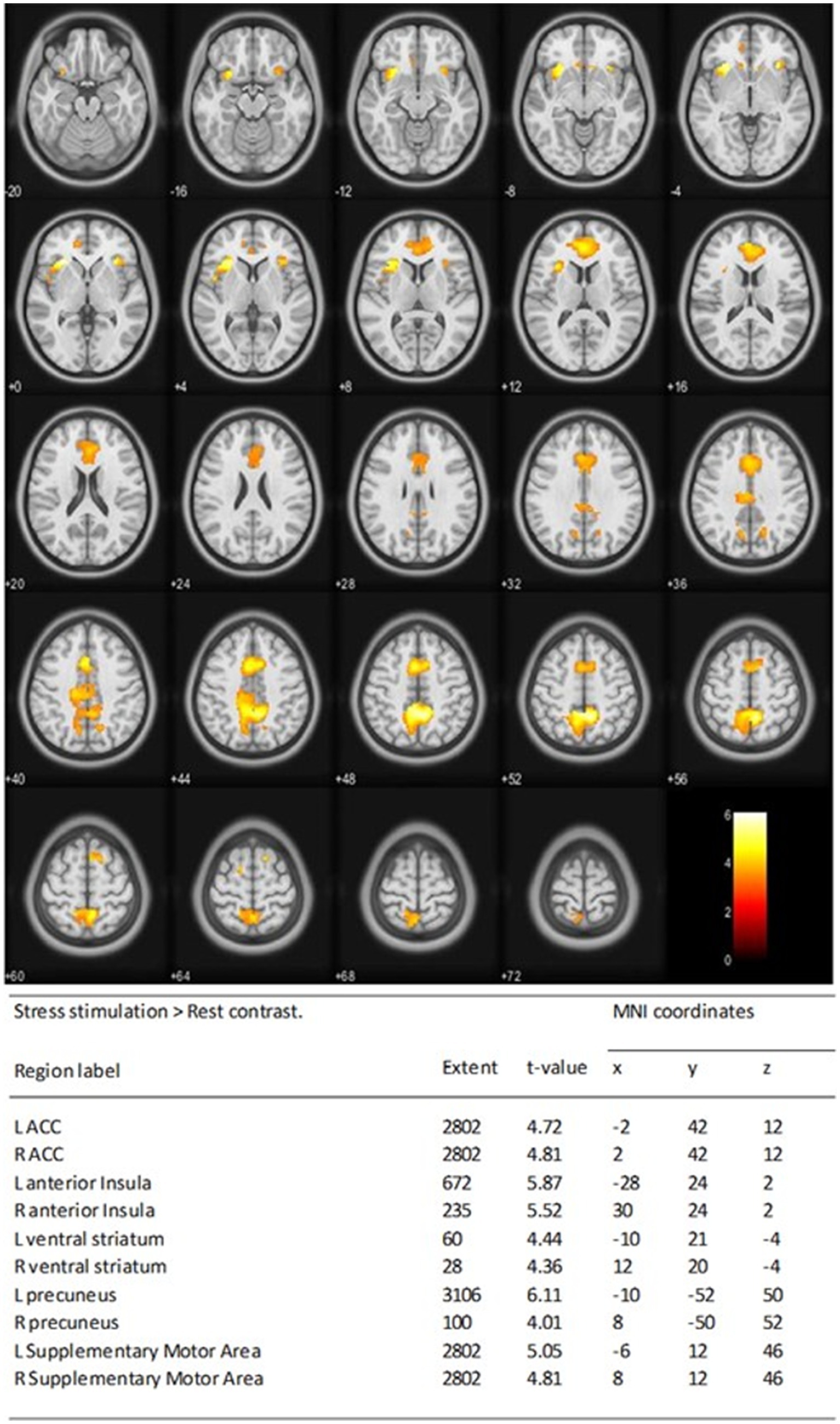
Figure 3. Stress matrix at D0 showing activation of the left (L) and right (R) of anterior cingulate cortex (ACC), the L and R of anterior insula (AI), the L and R of ventral striatum, the L and R of precuneus, and supplementary motor cortices One-sample t-test statistical results of the whole-brain analysis of the BOLD responses of stress stimulation > rest contrast at D0 for all individuals was performed. Statistical t maps were overlaid on MNI slices using a voxel-wise threshold of p < 0.001 and an extent threshold of 20 voxels. Regions were automatically labeled using the Anatomy Toolbox atlas of SPM and were presented in the table below. x, y, and z were MNI coordinates in the L-R, anterior–posterior, and inferior–superior dimensions, respectively. All peaks were significant at a voxel-wise threshold of p < 0.001 (extend threshold = 20 voxels). ACC, anterior cingulate cortex; AI, anterior insula; BOLD, blood oxygen level dependent; L, left; R, right; MNI, Montreal Neurological Institute; SPM, Statistical Parametric Mapping.
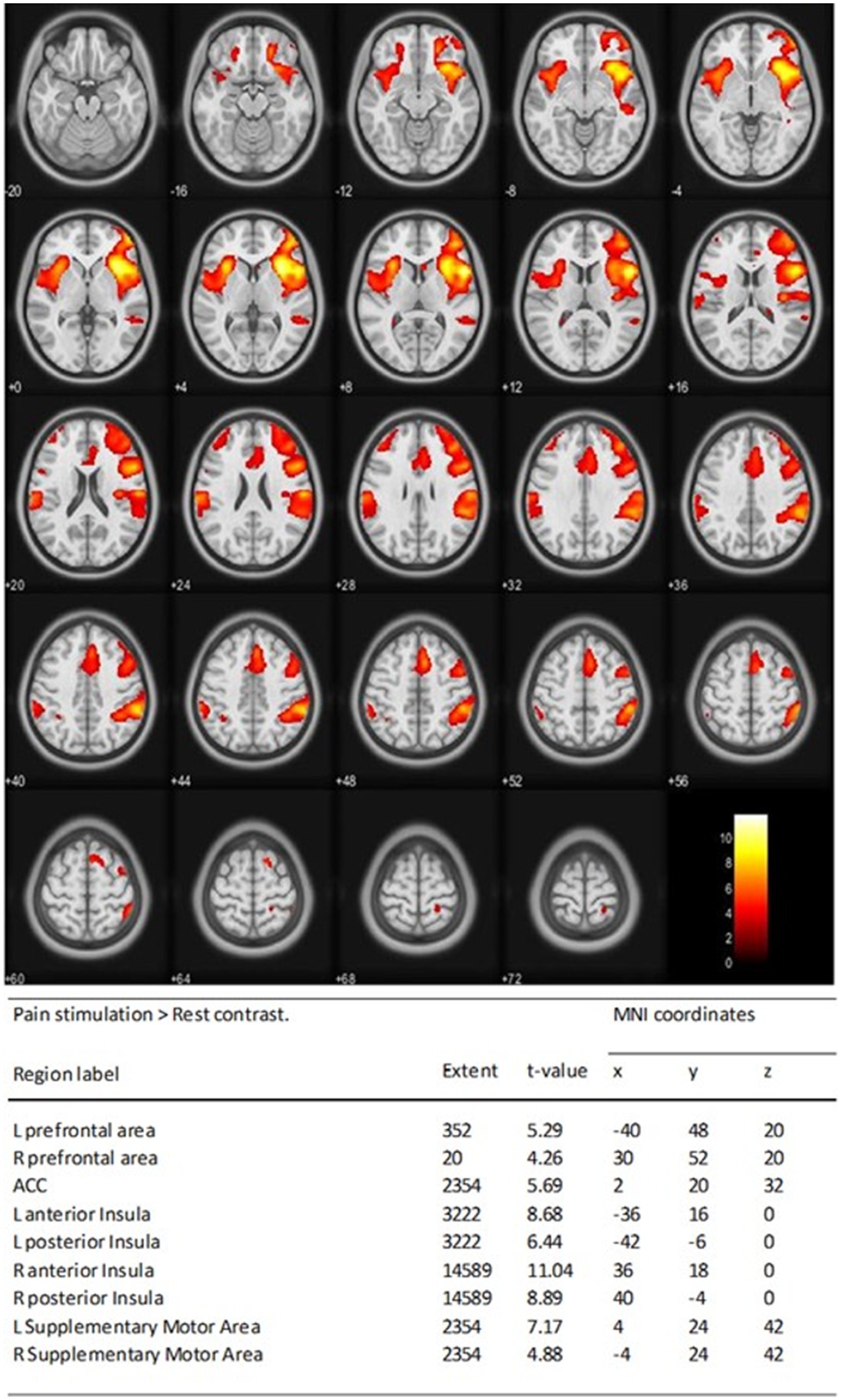
Figure 4. Pain matrix at D0 showing activation of the L and R of prefrontal cortices, ACC, anterior and posterior, the L and R of insula, and supplementary motor cortices One-sample t-test statistical results of the whole-brain analysis of the BOLD responses of pain stimulation > rest contrast at D0 for all individuals was performed. Statistical t maps are overlaid on MNI slices using a voxel-wise threshold of p < 0.001 and an extent threshold of 20 voxels. Regions were automatically labeled using the Anatomy Toolbox atlas of SPM and were presented in the table below. x, y, and z were MNI coordinates in the L-R, anterior–posterior, and inferior–superior dimensions, respectively. All peaks were significant at a voxel-wise threshold of p < 0.001 (extend threshold = 20 voxels). ACC, anterior cingulate cortex; BOLD, blood oxygen level dependent; L, left; R, right; MNI, Montreal Neurological Institute; SPM, Statistical Parametric Mapping.
Comparison of BOLD signal variations between D0 and D28 (D0 > D28) during stress stimulation between Mg-Teadiola versus placebo groups showed significantly increased activations in the left and right frontal area (p = 0.001 and p = 0.002, respectively), the left and right ACC (p = 0.035 and p = 0.04, respectively), and the left and right insula (p = 0.034 and p = 0.0402, respectively) (Figure 5).
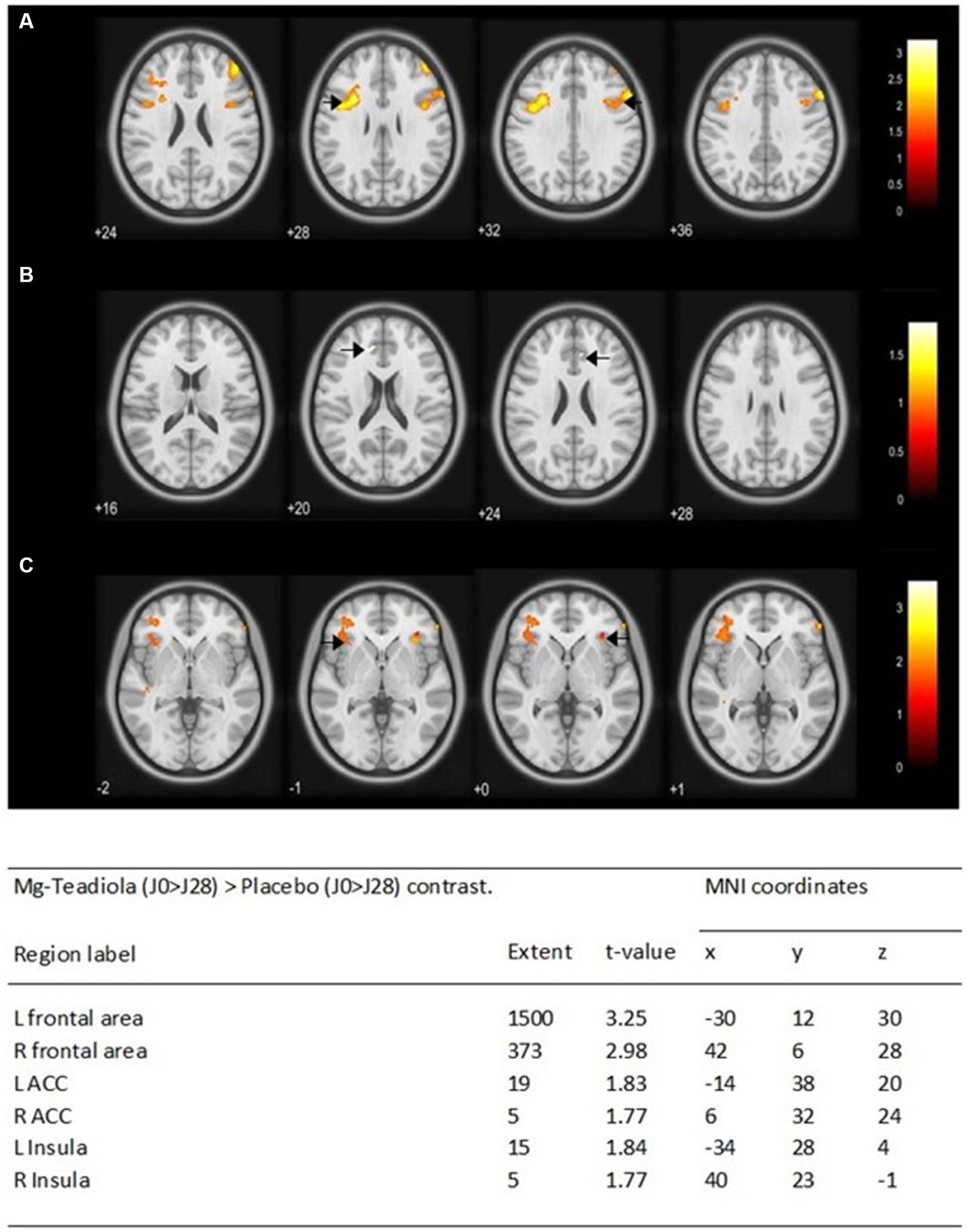
Figure 5. Whole-brain analysis of the blood-oxygen-level-dependent (BOLD) responses of the Mg-Teadiola (D0 > D28) > Placebo (D0 > D28) contrast during stress stimulation Statistical t maps were overlaid on MNI slices using a voxel-wise threshold of p < 0.05 and an extent threshold of five voxels. They were covering frontal area (A), ACC (B), and insula (C). Black arrow points each region of interest at L and R. Regions were automatically labeled using the Anatomy Toolbox atlas of SPM and were presented in the table below. x, y, and z were MNI coordinates in the L-R, anterior–posterior, and inferior–superior dimensions, respectively. All peaks were significant at a voxel-wise threshold of p < 0.05 (extend threshold = 5 voxels). ACC, anterior cingulate cortex; L, left; R, right; MNI, Montreal Neurological Institute; SPM, Statistical Parametric Mapping.
Comparison of BOLD signal variations between D0 and D28 (D0 > D28) during thermal pain stimulation between Mg-Teadiola and placebo groups showed significant diminished activations, mainly in the left and right prefrontal area (p = 0.001 and p = 0.001, respectively), the left and right insula (p = 0.008 and p = 0.019, respectively), and the left and right ventral striatum (p = 0.001 and p = 0.001, respectively; Figure 6).
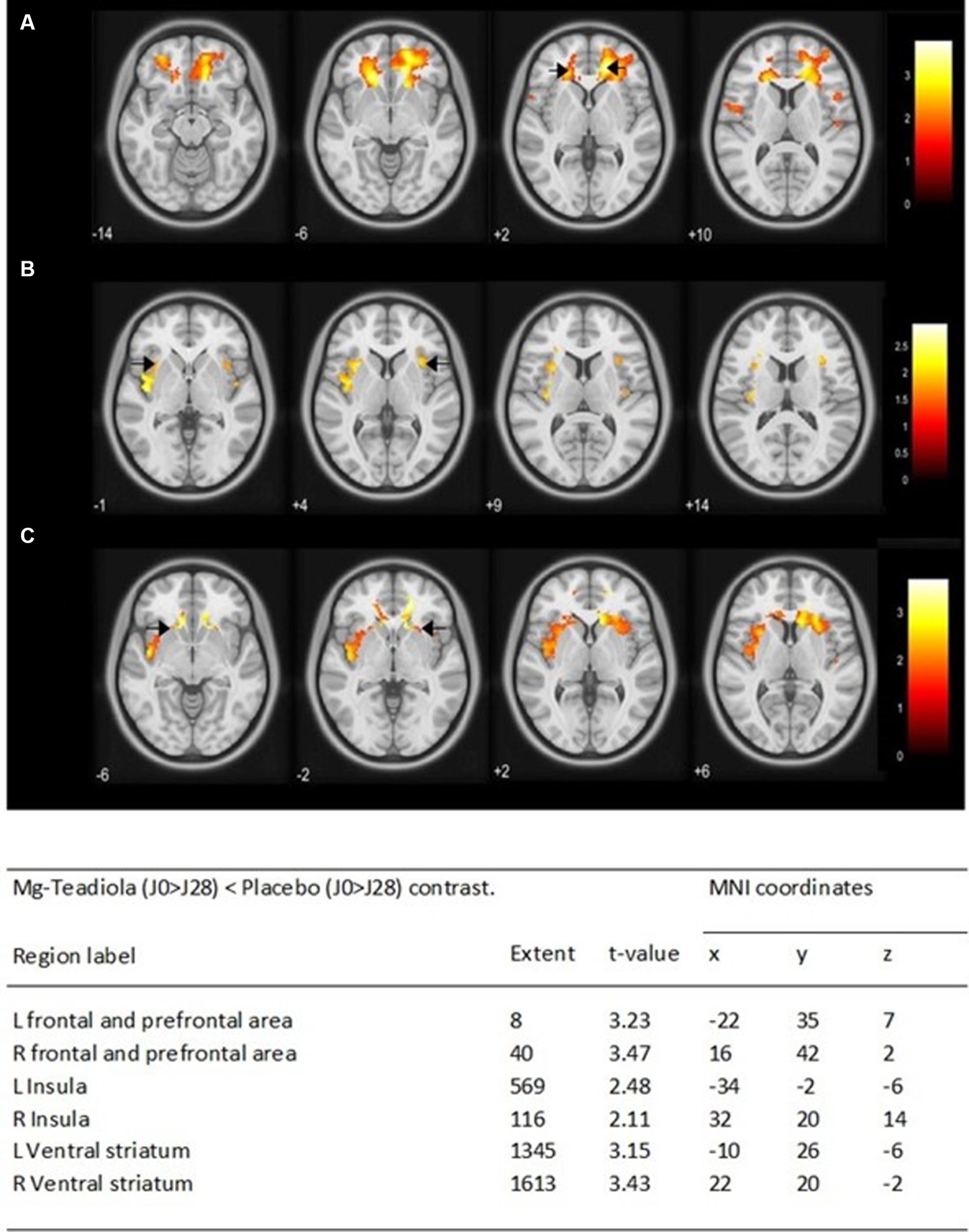
Figure 6. Whole-brain analysis of the BOLD responses of the Mg-Teadiola (D0 > D28) < Placebo (D0 > D28) contrast during pain stimulation Statistical t maps are overlaid on MNI slices using a voxel-wise threshold of p < 0.05 and an extent threshold of five voxels. They are covering frontal and prefrontal area (A), insula (B), and ventral striatum (C). Black arrow points each region of interest at L and R. Regions are automatically labeled using the Anatomy Toolbox atlas of SPM and are presented in the table below. x, y, and z are MNI coordinates in the L-R, anterior–posterior, and inferior–superior dimensions, respectively. All peaks are significant at a voxel-wise threshold of p < 0.05 (extend threshold = 5 voxels). L, left; R, right; MNI, Montreal Neurological Institute; SPM, Statistical Parametric Mapping.
4.3. Clinical outcomes
DASS-42 stress scores significantly decreased from D0 to D28 in both groups (Table 1) with a difference of five points between placebo versus Mg-Teadiola and a significant diminution was observed for Mg-Teadiola versus placebo at D14 (ES −0.60; p = 0.011) (Supplementary Table S1). One month after the end of treatment (D56), scores and difference between the groups remained stable (ES −0.62; p = 0.008) (Supplementary Table S1). A trend to diminution was also noted between D0 and D28 for anxiety and depression (Table 1). Concerning QST, sensitivity to cold improved from D0 to D28 for Mg-Teadiola versus placebo (ES 0.47; p = 0.042). No difference was observed for warm sensibility, warm pain, and cold pain (Table 1).
At D0, the plasma concentration of Mg was 0.85 ± 0.06 mmol/L for placebo and 0.86 ± 0.09 mmol/L for Mg-Teadiola. These measures were stable from D0 to D14 and up to D28. Urinary Mg concentration was 3.45 ± 1.49 mmol/24 h for placebo and 3.89 ± 1.58 mmol/24 h for Mg-Teadiola at D0 and remained stable on D14, D28, and D56. Cortisol was not significantly different between the groups (Table 1 and Supplementary Table S1).
Intake of Mg-Teadiola did not improve the PCS catastrophism global score from D0 to D28. However, magnification sub-scale score was significantly diminished for Mg-Teadiola at D28 (ES −0.60; p = 0.011) (Table 1). The same trend was observed at D14 and D56 but results did not reach statistical significance (Supplementary Table S1).
No significant difference was observed between the Mg-Teadiola and placebo groups for PSQI score between D0 and D28 (Table 1). However, subjective sleep quality (component 1) and daytime dysfunction (component 7) significantly improved for Mg-Teadiola at D14 (ES −0.47; p = 0.042) and D56 (ES −0.76; p = 0.002), respectively (Supplementary Table S1).
4.4. Safety outcomes
No serious AEs were reported during the study for Mg-Teadiola and placebo groups. A total of 49 non-serious AEs in the Mg-Teadiola group and 44 AEs in the placebo group were reported. A total of 10 individuals on 20 per group reported headaches or migraines, making it the highest frequency AEs reported. The second most frequently reported AE was the gastrointestinal disorder, with 7 persons on 20 per group. However, none of the AEs were considered treatment related.
5. Discussion
This ancillary study is the first to investigate the effect of Mg in combination with green tea, Rhodiola, and B vitamins on stress and acute pain at cerebral level, using fMRI, in chronically stressed but otherwise healthy individuals. The fMRI analysis allowed the visualization of interplay between stress and pain cerebral matrices, using thermal stimulation model to stress the system. The fMRI confirmed that supplementation with Mg-Teadiola for 28 days modulates both matrices (when stimulated) versus placebo. These results reinforce the clinical observations, showing a benefit of Mg-Teadiola on stress and pain perception.
In this study, after D28, Mg-Teadiola increased the activation of frontal cortex (FC)/PFC, AI, and ACC brain areas during the stress sequence (anticipation), while reducing the BOLD signal in FC/PFC and AI (and ventral striatum) during the thermal stimulus. Few studies have noted the significant role played by insula in processing noxious and innocuous thermal stimuli in the brain (46) and the ACC, which plays an essential role in integration of neuronal circuitry for affect regulation as it is connected to both the “emotional” limbic system and the “cognitive” PFC (47). The PFC—which has a vital role in executive functions—has connections to other areas of the cerebral neocortex, hippocampus, periaqueductal gray (PAG), thalamus, amygdala, and basal nuclei; thus, it also plays a significant role in pain processing (48). Previous studies have demonstrated the activation of PFC, AI, and ACC during the anticipation of pain using psychophysiological measures (49) and fMRI (50–52). The anticipation of pain may have an important protective function, allowing the avoidance of bodily harm through the initiation of adaptive behavior. The ACC, PFC, and insula with their associated subcortical regions, together contribute to support the mental representation of an impending stimulus (53).
Previous studies have reported that the chronic stress and stress-related conditions alter brain areas. A metanalysis reported that during depression or a post-traumatic stress disorder (PTSD), there is a reduced activation in response to emotional stimuli in the ACC (47). Across a range of psychiatric disorders, an abnormality in the structure and function of insula is observed (54), whereas individuals with a chronic back pain showed a decrease in the neural activity after the painful stimulation (55). Review articles have reported that a chronic exposure to the psychological stress stimulus may cause dysregulation in the neural pathways that are activated by dopamine, adrenaline, and cortisol and downregulation in the glucocorticoid receptor sensitivity of the PFC. Chronic stress causing the hyperactivity in the limbic system also downregulates excitatory synaptic transmission, with diminution of neural firing in the PFC and in between PFC and other cerebral neural networks having impairment of executive functioning. This further results in poor control and poor regulation over the stress responses and pain experiences, respectively (24, 56, 57).
Thus, the increased activity induced by Mg-Teadiola in these three major brain areas most probably contributed to improvement/normalization of function in the stressed population in the anticipation period. Interestingly, Boyle et al. (22) showed the capacity of Mg-Teadiola to activate the frontal theta electroencephalography (EEG) activity during cognitive tasks conducted under stressful situation (22). This observation, suggesting a capacity of the product to improve executive functions in stressed individuals under acute stressful conditions, supports the findings of the present study (22). Furthermore, because neurons in the PFC (24) and insular cortex (58) contribute to the subjective feeling of pain, the fact that Mg-Teadiola downregulated the BOLD signal in these two areas during the thermal stimulus probably helps to take away the unpleasant feeling associated with a painful event (58). This overall beneficial effect observed after acute stimulation are consistent with the favorable effects of Mg-Teadiola observed on chronic stress and sensitivity to pain during the QST, after D28.
Initially adaptive to direct brain systems for effective coping, sustained activation of the HPA axis during chronic stress can lead to a maladaptive response with the onset of chronic pain (59), mood, sleep, and cognitive functions disorders (60) and thus significantly reducing quality of life (QoL). In this study conducted in a subgroup of 40 individuals, Mg-Teadiola confirmed its potential to improve the overall QoL of chronically stressed individuals, resulting in significant decrease in DASS stress score (ES 0.46 [−0.91; −0.01], p = 0.048) from D0 to D28, showed a trend to improve sleep quality (at D14, Supplementary data), and decreased magnification (as tendency to increase perceived threat) in the PCS test. It also showed a reduction in sensibility to the cold pain (ES 0.47 [95% CI: 0.02; 0.92], p = 0.042), confirming the findings of the primary study (on 100 individuals) where a significant reduction in sensitivity to cold pain (p = 0.01) and a trend for lower sensitivity to warm pain (p = 0.06) were observed on D28. Human imaging and animal studies have shown that major brain regions (including hippocampus, amygdala, and ACC) are altered by stress (61), with chronic stress or depression often inducing synaptic plasticity as evidenced by altered glutamatergic (excitatory) and gamma-aminobutyric acid (GABA)-ergic (inhibitory) transmission (62). These two important regulatory systems are also modulated during chronic pain: animal models, such as peripheral inflammation and nerve injury produce short-term and long-term synaptic plasticity on glutamatergic transmission (63, 64), whereas GABAergic transmission is reduced in chronic pain models (65, 66). In chronic neuropathic pain individuals, magnetic resonance spectroscopy showed reduced thalamic GABA content, correlating with the degree of functional connectivity between the thalamus and the cerebral cortex, including the insula, suggesting increased activity of insula (and pain perception) (67). The observed effects of Mg-Teadiola may be explained by modulation of these two pathways by the active ingredients. Previous studies have reported that Mg blocks the N-methyl-D-aspartate (NMDA) receptor directly by inhibiting glutamate or indirectly by its enhanced reuptake into the synaptic vesicles through stimulation of the sodium–potassium P-type adenosine triphosphatase (ATPase). However, the mechanism of Mg by which it exerts a GABA-agonistic activity is not well understood (1). Mg has an important role in preventing central sensitization and attenuation of the pain hypersensitivity through its voltage-gated action at NMDA receptors and has been investigated for its ability to reduce acute or chronic pain in various clinical conditions (68). A recent study also reported a significant (p = 0.003) reduction in mild/moderate stress (DASS-42 stress score) and the intensity of pain (p = 0.029) following supplementation with oral Mg in fibromyalgia individuals (69). The important effects of the botanical extracts contained in Mg-Teadiola on brain functions have been highlighted in the previous study (20). Concerning Rhodiola, clinical data suggest that its beneficial stress-protective activity may be associated with the HPA axis and regulation of key mediators of stress response, including cortisol (70). However, the effects of Mg-Teadiola on chronic stress and related outcomes in the present study were elicited in the absence of any consistent activation of physiological markers of HPA overstimulation (e.g., cortisol). This may be related to the variability of cortisol pulsatility along the day and explain why there is often a weak correspondence between psychophysiological stress and cortisol circulating levels (71). Other hypotheses, based on preclinical studies (72, 73) suggested alterations in serotoninergic and dopaminergic activities. Regarding L-theanine—the main active constituent of green tea—neurochemistry studies have shown potential NMDA receptors modulatory effects, as well as possible increasing effects on brain serotonin, dopamine, and GABA levels (74–77) that may explain its beneficial effects on the observed outcomes. In addition, green tea extracts may contain polyphenolic compounds at significant levels (>30%) (78). Few polyphenols (mainly Epigallocatechin gallate) have been reported to potentially alter cognitive functions and mood (79). There are almost no studies reporting their effects on stress, anxiety, and depression in contrast to L-theanine (78). As the quantity of green tea extract in the product is low and a significant proportion is L-theanine, polyphenols are unlikely to play a major role in the observed results. In this study, the cognitive effect of polyphenols was not assessed separately and a synergistic effect with Mg-Teadiola could not be determined. The results obtained in this subgroup analysis involving 40 individuals confirmed the results obtained in the primary analysis that involved 100 individuals. Our study showed consistency in the fMRI data (acute stimulation) that confirmed the basal observations of improvement in the stress and pain responses. However, the study has limitations. The study observed no variations in the HPA biomarkers. Thus, further studies involving a specific design that results in an improved quality of data by involving dynamic repeated measurements of stress biomarkers upon stimulation are required. Finally, the clinical benefits of the supplementation over the stress were observed after one intake or 2 weeks; however, the fMRI experiment was conducted only after D28 of supplementation. Also, further research is required to explore and evaluate acute effects on brain matrices.
6. Conclusion
This fMRI study demonstrated that Mg-Teadiola, a combination of Mg, B vitamins, Rhodiola, and green tea (L-theanine) was effective in significantly modulating the brain response to stressful (thermal) stimulus in chronically stressed individuals and potentially alter the pain perception after 28 days. This response to acute stimulus was consistent with the perceived clinical long-term benefits of the product on basal stress level, pain sensitivity, and other aspects related to QoL. Data suggest a potential mechanism of action related to improved executive functions—potentially impaired by chronic stress—resulting in better control of stress response and pain experience, culminating in enhanced well-being. Further studies are needed to validate this hypothesis and further explore the mechanism of action of this promising combination of ingredients.
Data availability statement
The raw data supporting the conclusions of this article will be made available by the authors, without undue reservation.
Ethics statement
The study has been approved on 11 March 2020 by the Ethics committee (Sud-Est II, France), registered at the French National Agency for the Safety Medicines and Healthcare Products (ANSM) in March 2020 (2020-A00040-39) and in May 2020 at (http://www.clinicaltrials.gov). The patients/participants provided their written informed consent to participate in this study.
Author contributions
GP and LN: conceptualization, investigation, and supervision. GP, NM, VR, and BP: methodology, resources, and visualization. VR and NM: software. GP, LN, BP, JG, VL, AT, NM, LB, CD, VR, and CC: validation and writing–review and editing. NM, BP, and CC: data curation. LN, GP, and CC: writing–original draft preparation. GP and LN: project administration and funding acquisition. All authors contributed to the article and approved the submitted version.
Funding
This collaboration study with Sanofi was conducted by the University Hospital of Clermont-Ferrand, France. Sanofi provided the treatments and partial financial support to the study.
Acknowledgments
This clinical trial benefited from funding from Auvergne Region Contrat Plan Etat Région and Sanofi. Vijay Rayasam, an employee of Sanofi, provided writing and editorial support.
Conflict of interest
LN was employed by the company Sanofi S.A.
The authors declare that this study received funding from Sanofi. The funder had the following involvement in the study: conceptualization, investigation, supervision and writing the original draft, review and editing.
Publisher’s note
All claims expressed in this article are solely those of the authors and do not necessarily represent those of their affiliated organizations, or those of the publisher, the editors and the reviewers. Any product that may be evaluated in this article, or claim that may be made by its manufacturer, is not guaranteed or endorsed by the publisher.
Supplementary material
The Supplementary material for this article can be found online at: https://www.frontiersin.org/articles/10.3389/fnut.2023.1211321/full#supplementary-material
References
1. Pickering, G, Mazur, A, Trousselard, M, Bienkowski, P, Yaltsewa, N, Amessou, M, et al. Magnesium status and stress: the vicious circle concept revisited. Nutrients. (2020) 12:3672. doi: 10.3390/nu12123672
2. Mahmud, S, Mohsin, M, Dewan, MM, and Muyeed, A. The global prevalence of depression, anxiety, stress, and insomnia among general population during COVID-19 pandemic: a systematic review and meta-analysis. Trends Psychol. (2022) 31:143–70. doi: 10.1007/s43076-021-00116-9
3. Calpe-Lopez, C, Martínez-Caballero, MA, García-Pardo, MP, and Aguilar, MA. Resilience to the effects of social stress on vulnerability to developing drug addiction. World J Psychiatry. (2022) 12:24–58. doi: 10.5498/wjp.v12.i1.24
4. Herselman, MF, Bailey, S, Deo, P, Zhou, X-F, Gunn, KM, and Bobrovskaya, L. The effects of walnuts and academic stress on mental health, general well-being and the gut microbiota in a sample of university students: a randomised clinical trial. Nutrients. (2022) 14:4776. doi: 10.3390/nu14224776
5. Brandl, HB, Pruessner, JC, and Farine, DR. The social transmission of stress in animal collectives. Proc Biol Sci. (2022) 289:20212158. doi: 10.1098/rspb.2021.2158
6. World Health Organisation. Mental disorders (2022). Available at: https://www.who.int/news-room/fact-sheets/detail/mental-disorders. Accessed August 09, 2022.
7. Salari, N, Hosseinian-Far, A, Jalali, R, Vaisi-Raygani, A, Rasoulpoor, S, Mohammadi, M, et al. Prevalence of stress, anxiety, depression among the general population during the COVID-19 pandemic: a systematic review and meta-analysis. Glob Health. (2020) 16:57. doi: 10.1186/s12992-020-00589-w
8. Sara, JDS, Toya, T, Ahmad, A, Clark, MM, Gilliam, WP, Lerman, LO, et al. Mental stress and its effects on vascular health. Mayo Clin Proc. (2022) 97:951–90. doi: 10.1016/j.mayocp.2022.02.004
9. Chandrasekhar, K, Kapoor, J, and Anishetty, S. A prospective, randomized double-blind, placebo-controlled study of safety and efficacy of a high-concentration full-spectrum extract of ashwagandha root in reducing stress and anxiety in adults. Indian J Psychol Med. (2012) 34:255–62. doi: 10.4103/0253-7176.106022
10. Kell, G, Rao, A, Beccaria, G, Clayton, P, Inarejos-García, AM, and Prodanov, M. Affron® a novel saffron extract (Crocus sativus L.) improves mood in healthy adults over 4 weeks in a double-blind, parallel, randomized, placebo-controlled clinical trial. Complement Ther Med. (2017) 33:58–64. doi: 10.1016/j.ctim.2017.06.001
11. Lopresti, AL, Smith, SJ, Malvi, H, and Kodgule, R. An investigation into the stress-relieving and pharmacological actions of an ashwagandha (Withania somnifera) extract: a randomized, double-blind, placebo-controlled study. Medicine (Baltimore). (2019) 98:e17186. doi: 10.1097/MD.0000000000017186
12. Wienecke, E, and Nolden, C. Long-term HRV analysis shows stress reduction by magnesium intake. MMW Fortschr Med. (2016) 158:12–6. doi: 10.1007/s15006-016-9054-7
13. Pouteau, E, Kabir-Ahmadi, M, Noah, L, Mazur, A, Dye, L, Hellhammer, J, et al. Superiority of magnesium and vitamin B6 over magnesium alone on severe stress in healthy adults with low magnesemia: a randomized, single-blind clinical trial. PLoS One. (2018) 13:e0208454. doi: 10.1371/journal.pone.0208454
14. Harris, E, Kirk, J, Rowsell, R, Vitetta, L, Sali, A, Scholey, AB, et al. The effect of multivitamin supplementation on mood and stress in healthy older men. Hum Psychopharmacol. (2011) 26:560–7. doi: 10.1002/hup.1245
15. Mancini, E, Beglinger, C, Drewe, J, Zanchi, D, Lang, UE, and Borgwardt, S. Green tea effects on cognition, mood and human brain function: a systematic review. Phytomedicine. (2017) 34:26–37. doi: 10.1016/j.phymed.2017.07.008
16. Mattioli, L, Funari, C, and Perfumi, M, Effects of Rhodiola rosea L. Extract on behavioural and physiological alterations induced by chronic mild stress in female rats. J Psychopharmacol. (2009) 23:130–42. doi: 10.1177/0269881108089872
17. Noah, L, Dye, L, Bois De Fer, B, Mazur, A, Pickering, G, and Pouteau, E. Effect of magnesium and vitamin B6 supplementation on mental health and quality of life in stressed healthy adults: post-hoc analysis of a randomised controlled trial. Stress Health. (2021) 37:1000–9. doi: 10.1002/smi.3051
18. Noah, L, Pickering, G, Mazur, A, Dubray, C, Hitier, S, Dualé, C, et al. Impact of magnesium supplementation, in combination with vitamin B6, on stress and magnesium status: secondary data from a randomized controlled trial. Magnes Res. (2020) 33:45–57. doi: 10.1684/mrh.2020.0468
19. Zogović, D, Pešić, V, Dmitrašinović, G, Dajak, M, Plećaš, B, Batinić, B, et al. Pituitary-gonadal, pituitary-adrenocortical hormones and IL-6 levels following long-term magnesium supplementation in male students. J Med Biochem. (2014) 33:291–8. doi: 10.2478/jomb-2014-0016
20. Boyle, NB, Billington, J, Lawton, C, Quadt, F, and Dye, L. A combination of green tea, rhodiola, magnesium and B vitamins modulates brain activity and protects against the effects of induced social stress in healthy volunteers. Nutr Neurosci. (2021) 25:1845–59. doi: 10.1080/1028415X.2021.1909204
21. Dye, L, Billington, J, Lawton, C, and Boyle, N. A combination of magnesium, B vitamins, green tea and rhodiola attenuates the negative effects of acute psychosocial stress on subjective state in adults. Curr Dev Nutr. (2020) 4:nzaa067_023. doi: 10.1093/cdn/nzaa067_023
22. Boyle, NB, Dye, L, Lawton, CL, and Billington, J. A combination of green tea, rhodiola, magnesium, and B vitamins increases electroencephalogram theta activity during attentional task performance under conditions of induced social stress. Front Nutr. (2022) 9:935001. doi: 10.3389/fnut.2022.935001
23. Noah, L, Morel, V, Bertin, C, Pouteau, E, Macian, N, Dualé, C, et al. Effect of a combination of magnesium, B vitamins, rhodiola, and green tea (L-theanine) on chronically stressed healthy individuals – a randomized, placebo-controlled study. Nutrients. (2022) 14:1863. doi: 10.3390/nu14091863
24. Feller, L, Feller, G, Ballyram, T, Chandran, R, Lemmer, J, and Khammissa, RAG. Interrelations between pain, stress and executive functioning. Br J Pain. (2020) 14:188–94. doi: 10.1177/2049463719889380
25. Li, X, and Hu, L. The role of stress regulation on neural plasticity in pain chronification. Neural Plast. (2016) 2016:6402942. doi: 10.1155/2016/6402942
26. Veer, IM, Oei, NYL, Spinhoven, P, van Buchem, MA, Elzinga, BM, and Rombouts, SARB. Endogenous cortisol is associated with functional connectivity between the amygdala and medial prefrontal cortex. Psychoneuroendocrinology. (2012) 37:1039–47. doi: 10.1016/j.psyneuen.2011.12.001
27. Dopfel, D, and Zhang, N. Mapping stress networks using functional magnetic resonance imaging in awake animals. Neurobiol Stress. (2018) 9:251–63. doi: 10.1016/j.ynstr.2018.06.002
28. Morton, DL, Sandhu, JS, and Jones, AK. Brain imaging of pain: state of the art. J Pain Res. (2016) 9:613–24. doi: 10.2147/JPR.S60433
29. Berretz, G, Packheiser, J, Kumsta, R, Wolf, OT, and Ocklenburg, S. The brain under stress – a systematic review and activation likelihood estimation meta-analysis of changes in BOLD signal associated with acute stress exposure. Neurosci Biobehav Rev. (2021) 124:89–99. doi: 10.1016/j.neubiorev.2021.01.001
30. Quinn, ME, and Joormann, J. Control when it counts: change in executive control under stress predicts depression symptoms. Emotion. (2015) 15:522–30. doi: 10.1037/emo0000089
31. Weldon, AL, Hagan, M, van Meter, A, Jacobs, RH, Kassel, MT, Hazlett, KE, et al. Stress response to the functional magnetic resonance imaging environment in healthy adults relates to the degree of limbic reactivity during emotion processing. Neuropsychobiology. (2015) 71:85–96. doi: 10.1159/000369027
32. Quesada, C, Kostenko, A, Ho, I, Leone, C, Nochi, Z, Stouffs, A, et al. Human surrogate models of central sensitization: a critical review and practical guide. Eur J Pain. (2021) 25:1389–428. doi: 10.1002/ejp.1768
33. Tracey, I, and Mantyh, PW. The cerebral signature for pain perception and its modulation. Neuron. (2007) 55:377–91. doi: 10.1016/j.neuron.2007.07.012
35. Dietary reference values. European Food Safety Authority [EFSA] (2022); Available at: https://www.efsa.europa.eu/en/topics/topic/dietary-reference-values. Accessed on February 23, 2023.
36. Lovibond, PF, and Lovibond, SH. Depression anxiety and stress scales (DASS-42). Washington, DC: APA PsycTests (1995).
37. Parkitny, L, and McAuley, J. The depression anxiety stress scale (DASS). J Physiother. (2010) 56:204. doi: 10.1016/s1836-9553(10)70030-8
38. Verdugo, R, and Ochoa, JL. Quantitative somatosensory thermotest. A key method for functional evaluation of small calibre afferent channels. Brain. (1992) 115:893–913. doi: 10.1093/brain/115.3.893
39. Sullivan, MJL, Bishop, SR, and Pivik, J. The pain catastrophizing scale: development and validation. Psychol Assess. (1995) 7:524–32. doi: 10.1037/1040-3590.7.4.524
40. Buysse, DJ, Reynolds, CF, Monk, TH, Berman, SR, and Kupfer, DJ. The Pittsburgh sleep quality index: a new instrument for psychiatric practice and research. Psychiatry Res. (1989) 28:193–213. doi: 10.1016/0165-1781(89)90047-4
41. Gatta, B, Hau, DH, Catargi, B, Roger, P, and Tabarin, A. Re-evaluation of the efficacy of the association of cabergoline to somatostatin analogues in acromegalic patients. Clin Endocrinol. (2005) 63:477–8. doi: 10.1111/j.1365-2265.2005.02329.x
42. Gitelman, HJ, Graham, JB, and Welt, LG. A new familial disorder characterized by hypokalemia and hypomagnesemia. Trans Assoc Am Phys. (1966) 79:221–35.
43. Geuter, S, Qi, G, Welsh, RC, Wager, TD, and Lindquist, MA. Effect size and power in fMRI group analysis. BioRxiv. (2018):295048. doi: 10.1101/295048
44. Neuroimaging TWCfH. SPM12. (2020). UCL Queen Square Institute of Neurology. Available at: https://www.fil.ion.ucl.ac.uk/spm/software/spm12/. Accessed August 09, 2022.
45. MarsBaR region of interest toolbox for SPM. (2022). Available at: http://marsbar.sourceforge.net/. Accessed August 09, 2022.
46. Peltz, E, Seifert, F, DeCol, R, Dörfler, A, Schwab, S, and Maihöfner, C. Functional connectivity of the human insular cortex during noxious and innocuous thermal stimulation. NeuroImage. (2011) 54:1324–35. doi: 10.1016/j.neuroimage.2010.09.012
47. Steven, FL, Hurley, RA, Taber, KH, and Hayman, LA. Anterior cingulate cortex: unique role in cognition and emotion. J Neuropsychiatry Clin Neurosci. (2011) 23:121–5. doi: 10.1176/jnp.23.2.jnp121
48. Ong, WY, Stohler, CS, and Herr, DR. Role of the prefrontal cortex in pain processing. Mol Neurobiol. (2019) 56:1137–66. doi: 10.1007/s12035-018-1130-9
49. Koyama, T, Tanaka, YZ, and Mikami, A. Nociceptive neurons in the macaque anterior cingulate activate during anticipation of pain. Neuroreport. (1998) 9:2663–7. doi: 10.1097/00001756-199808030-00044
50. Porro, CA, Baraldi, P, Pagnoni, G, Serafini, M, Facchin, P, Maieron, M, et al. Does anticipation of pain affect cortical nociceptive systems? J Neurosci. (2002) 22:3206–14. doi: 10.1523/JNEUROSCI.22-08-03206.2002
51. Ploghaus, A, Tracey, I, Gati, JS, Clare, S, Menon, RS, Matthews, PM, et al. Dissociating pain from its anticipation in the human brain. Science. (1999) 284:1979–81. doi: 10.1126/science.284.5422.1979
52. Porro, CA, Cettolo, V, Francescato, MP, and Baraldi, P. Functional activity mapping of the mesial hemispheric wall during anticipation of pain. NeuroImage. (2003) 19:1738–47. doi: 10.1016/S1053-8119(03)00184-8
53. Koyama, T, McHaffie, JG, Laurienti, PJ, and Coghill, RC. The subjective experience of pain: where expectations become reality. Proc Natl Acad Sci U S A. (2005) 102:12950–5. doi: 10.1073/pnas.0408576102
54. Peters, SK, Dunlop, K, and Downar, J. Cortico-striatal-thalamic loop circuits of the salience network: a central pathway in psychiatric disease and treatment. Front Syst Neurosci. (2016) 10:104. doi: 10.3389/fnsys.2016.00104
55. Wippert, PM, and Wiebking, C. Stress and alterations in the pain matrix: a biopsychosocial perspective on back pain and its prevention and treatment. Int J Environ Res Public Health. (2018) 15:785. doi: 10.3390/ijerph15040785
56. Negron-Oyarzo, I, Aboitiz, F, and Fuentealba, P. Impaired functional connectivity in the prefrontal cortex: a mechanism for chronic stress-induced neuropsychiatric disorders. Neural Plast. (2016) 2016:7539065. doi: 10.1155/2016/7539065
57. Woo, E, Sansing, LH, Anrsten, AFT, and Datta, D. Chronic stress weakens connectivity in the prefrontal cortex: architectural and molecular changes. Chronic Stress (Thousand Oaks). (2021) 5:1–22. doi: 10.1177/24705470211029254
58. Berret, E, Kintscher, M, Palchaudhuri, S, Tang, W, Osypenko, D, Kochubey, O, et al. Insular cortex processes aversive somatosensory information and is crucial for threat learning. Science. (2019) 364:eaaw0474. doi: 10.1126/science.aaw0474
59. Herman, JP, McKlveen, JM, Ghosal, S, Kopp, B, Wulsin, A, Makinson, R, et al. Regulation of the hypothalamic-pituitary-adrenocortical stress response. Compr Physiol. (2016) 6:603–21. doi: 10.1002/cphy.c150015
60. Feller, L, Khammissa, RAG, Ballyram, R, Chandran, R, and Lemmer, J. Chronic psychosocial stress in relation to cancer. Middle East J Cancer. (2019) 10:1–8. doi: 10.30476/mejc.2019.44680
61. Knudsen, L, Petersen, GL, Nørskov, KN, Vase, L, Finnerup, N, Jensen, TS, et al. Review of neuroimaging studies related to pain modulation. Scand J Pain. (2018) 2:108–20. doi: 10.1016/j.sjpain.2011.05.005
62. Duman, RS, Sanacora, G, and Krystal, JH. Altered connectivity in depression: GABA and glutamate neurotransmitter deficits and reversal by novel treatments. Neuron. (2019) 102:75–90. doi: 10.1016/j.neuron.2019.03.013
63. Zhuo, M. Cortical excitation and chronic pain. Trends Neurosci. (2008) 31:199–207. doi: 10.1016/j.tins.2008.01.003
64. Zhuo, M. Contribution of synaptic plasticity in the insular cortex to chronic pain. Neuroscience. (2016) 338:220–9. doi: 10.1016/j.neuroscience.2016.08.014
65. Blom, SM, Pfister, J-P, Santello, M, Senn, W, and Nevian, T. Nerve injury-induced neuropathic pain causes disinhibition of the anterior cingulate cortex. J Neurosci. (2014) 34:5754–64. doi: 10.1523/JNEUROSCI.3667-13.2014
66. Koga, K, Shimoyama, S, Yamada, A, Furukawa, T, Nikaido, Y, Furue, H, et al. Chronic inflammatory pain induced GABAergic synaptic plasticity in the adult mouse anterior cingulate cortex. Mol Pain. (2018) 14:174480691878347–14. doi: 10.1177/1744806918783478
67. Henderson, LA, Peck, CC, Petersen, ET, Rae, CD, Youssef, AM, Reeves, JM, et al. Chronic pain: lost inhibition? J Neurosci. (2013) 33:7574–82. doi: 10.1523/JNEUROSCI.0174-13.2013
68. Na, H-S, Ryu, J-H, and Do, S-H. The role of magnesium in pain In: R Vink and M Nechifor, editors. Magnesium in the central nervous system. Australia: University of Adelaide Press (2011). 157–66.
69. Macian, N, Dualé, C, Voute, M, Leray, V, Courrent, M, Bodé, P, et al. Short-term magnesium therapy alleviates moderate stress in patients with fibromyalgia: a randomized double-blind clinical trial. Nutrients. (2022) 14:2088. doi: 10.3390/nu14102088
70. Olsson, EM, von Scheele, B, and Panossian, AG. A randomised, double-blind, placebo-controlled, parallel-group study of the standardised extract shr-5 of the roots of Rhodiola rosea in the treatment of subjects with stress-related fatigue. Planta Med. (2009) 75:105–12. doi: 10.1055/s-0028-1088346
71. Campbell, J, and Ehlert, U. Acute psychosocial stress: does the emotional stress response correspond with physiological responses? Psychoneuroendocrinology. (2012) 37:1111–34. doi: 10.1016/j.psyneuen.2011.12.010
72. Chen, QG, Zeng, YS, Qu, ZQ, Tang, JY, Qin, YJ, Chung, P, et al. The effects of Rhodiola rosea extract on 5-HT level, cell proliferation and quantity of neurons at cerebral hippocampus of depressive rats. Phytomedicine. (2009) 16:830–8. doi: 10.1016/j.phymed.2009.03.011
73. Mattioli, L, Bracci, A, Titomanlio, F, Perfumi, M, and De Feo, V. Effects of Brugmansia arborea extract and its secondary metabolites on morphine tolerance and dependence in mice. Evid Based Complement Alternat Med. (2012) 2012:741925. doi: 10.1155/2012/741925
74. Nathan, PJ, Lu, K, Gray, M, and Oliver, C. The neuropharmacology of L-theanine (N-ethyl-L-glutamine). J Herbal Pharmacother. (2006) 6:21–30. doi: 10.1080/J157v06n02_02
75. Ota, M, Wakabayashi, C, Sato, N, Hori, H, Hattori, K, Teraishi, T, et al. Effect of L-theanine on glutamatergic function in patients with schizophrenia. Acta Neuropsychiatr. (2015) 27:291–6. doi: 10.1017/neu.2015.22
76. Anas Sohail, A, Ortiz, F, Varghese, T, Fabara, SP, Batth, AS, Sandesara, DP, et al. The cognitive-enhancing outcomes of caffeine and L-theanine: a systematic review. Cureus. (2021) 13:e20828. doi: 10.7759/cureus.20828
77. Yuan, S, Brown, JC, Gold, M, Tirrell, E, Jones, RN, and Carpenter, LL. Effects of single-dose L-theanine on motor cortex excitability. Clin Neurophysiol. (2021) 132:2062–4. doi: 10.1016/j.clinph.2021.07.003
78. Tallei, TW, Fatimawali, NNJ, Niode, NJ, Idroes, R, Zidan, BMRM, Mitra, S, et al. A comprehensive review of the potential use of green tea polyphenols in the management of COVID-19. eCAM. (2021) 2021:1–13. doi: 10.1155/2021/7170736
79. Wightman, EL, Haskell, CF, Forster, JS, Veasey, RC, and Kennedy, DO. Epigallocatechin gallate, cerebral blood flow parameters, cognitive performance and mood in healthy humans: a double-blind, placebo-controlled, crossover investigation. Hum Psychopharmacol Clin Exp. (2012) 27:177–86. doi: 10.1002/hup.1263
Keywords: chronic stress, pain, magnesium, vitamins, green tea, L-theanine, Rhodiola, fMRI
Citation: Pickering G, Noah L, Pereira B, Goubayon J, Leray V, Touron A, Macian N, Bernard L, Dualé C, Roux V and Chassain C (2023) Assessing brain function in stressed healthy individuals following the use of a combination of green tea, Rhodiola, magnesium, and B vitamins: an fMRI study. Front. Nutr. 10:1211321. doi: 10.3389/fnut.2023.1211321
Edited by:
Leo Veenman, Consorzio per Valutazioni Biologiche e Farmacologiche, ItalyCopyright © 2023 Pickering, Noah, Pereira, Goubayon, Leray, Touron, Macian, Bernard, Dualé, Roux and Chassain. This is an open-access article distributed under the terms of the Creative Commons Attribution License (CC BY). The use, distribution or reproduction in other forums is permitted, provided the original author(s) and the copyright owner(s) are credited and that the original publication in this journal is cited, in accordance with accepted academic practice. No use, distribution or reproduction is permitted which does not comply with these terms.
*Correspondence: Gisèle Pickering, gisele.pickering@uca.fr