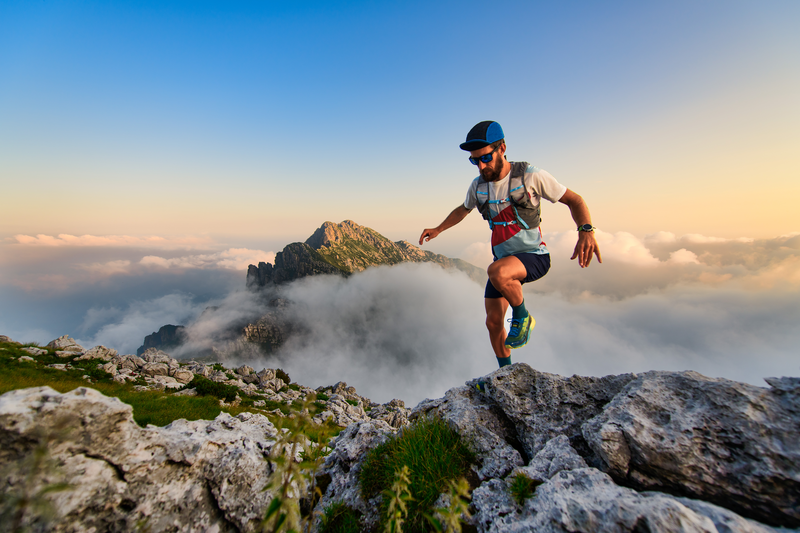
95% of researchers rate our articles as excellent or good
Learn more about the work of our research integrity team to safeguard the quality of each article we publish.
Find out more
ORIGINAL RESEARCH article
Front. Nutr. , 26 July 2023
Sec. Nutrition and Sustainable Diets
Volume 10 - 2023 | https://doi.org/10.3389/fnut.2023.1210934
This article is part of the Research Topic Nutrition and Sustainable Development Goal 15: Life on Land View all 9 articles
Introduction: Brown seaweeds are excellent sources of bioactive molecules with a wide range of pharmacological effects, whose content can vary depending on several factors, including the origin and the environment in which the algae grow.
Methods: This study aimed to estimate 19 compounds regarding primary and secondary metabolites of eight brown macroalgal species from a clean Egyptian Red Sea coast. A proximate analysis, pigment, phenolic compounds, and vitamin contents were determined. In addition, the energy content and antioxidant activity were estimated to explore the potential application of algae as functional foods to encourage the species’ commercialization.
Results: Based on the chemical composition, Polycladia myrica was the most valuable species, with a comparatively high protein content of 22.54%, lipid content of 5.21%, fucoxanthin content of 3.12 μg/g, β-carotene content of 0.55 mg/100 g, and carbohydrate content of 45.2%. This species also acts as a great source of vitamin C, flavonoids, tannins, phenol content and total antioxidant capacity.
Discussion: The antioxidant activity of the selected algae indicated that its phenol, vitamin and pigment contents were powerful antioxidant compounds based on the structure–activity relationships. This result was verified by the strong correlation in statistical analysis at the 95% confidence level. From a worldwide perspective and based on the obtained results, these brown species may be reinforced as an essential line in future foods.
The global variety of all algae (micro and macro) consists of over 164,000 species, with approximately 9,800 of them being seaweeds, 0.17% of which have been domesticated for commercial exploitation (1, 2). In recent years, the consumption of seaweeds has gained popularity in countries where their consumption was not a traditional custom, both as a food ingredient and for making beverages. Many types of seaweeds are edible by humans and are an important source of proteins, polysaccharides, vitamins and minerals (3–5). In general, their nutritional composition can vary significantly, depending on many factors (6).
Among the three groups of macroalgae, brown seaweeds have received considerable attention because of their numerous bioactive compounds and biological properties. Among bioactive properties, brown seaweeds can cause beneficial effects on improving the microbiota, antioxidant and anti-inflammatory properties, dyslipidemia control, reduced obesity, diabetes control, immunomodulatory, dyslipidemia, hypertension control, diabetes, and some types of cancer (7).
Brown seaweeds have a distinctive and abundant composition of pertinent polysaccharides and bioactive substances that can make up as much as 70% of the tissue with several uses in food, nutraceutical, pharmaceutical, cosmetic, and biopolymer applications (8). Brown seaweeds are characterized by the production of several types of carotenoids, such as fucoxanthin and β-carotene, which have anti-obesity properties and antioxidant activity. Moreover, they are a valuable source of lipophilic antioxidants such as vitamins A (retinoic acid), C (ascorbic acid) and E (tocopherol) (9). Algal vitamins are important not only for biochemical functions and antioxidant activity but also for other health benefits, such as lowering blood pressure (9). Vitamin C is a water-soluble vitamin and is considered an essential micronutrient with antioxidant function that can reduce the risk for stomach cancer (8). Additionally, β-carotene, carotenoids, vitamins E and C contribute to preventing cardiovascular disease and lowering cancer risk (8, 9).
The antioxidant substances contained in algae depend on their exposure to external environmental factors such as salinity, nutrient availability, light, depth at which they grow and seasonality. In addition, they also depend on intrinsic factors such as algal species, age, length and tissue type (6). The main groups of antioxidants in seaweeds are phenolics, polysaccharides, vitamins, and pigments (10, 11). Polyphenol compounds (phenolic acid, flavonoids, and tannins) have been classified as strong antioxidant compounds (3). In this work, eight brown seaweeds were selected for valorization regarding nutritional characteristics and bioactive profiles, all of which are widely grown throughout the Red Sea and relatively underexplored. Such seaweeds were tested as sources of bioactive components with antioxidant properties. This trend is aimed at addressing the issues that the world has encountered because of population growth outpacing food resources. The relationship between the chemical structure and antioxidant activity of the selected compounds was also elucidated.
Eight species of brown algae were handpicked collected during the winter months of 2020 in the vicinity of the National Institute of Oceanography and Fisheries, between latitude 27°17′13” N and longitude 33°46′21″ E, located in Hurghada city, Red Sea, Egypt (Figure 1). The collected samples were thoroughly washed with distilled water and, subsequently, cleaned with a soft brush to remove deposits and epiphytes. A portion of the fresh algae was then processed as herbarium specimens. Other fresh samples were preserved in fresh seawater spiked with 5% formalin for taxonomic classification. The other portions were dried at room temperature in a place protected from sunlight, ground to a fine powder, and stored at −20°C until use. The taxonomy of the algal samples was explored using their morphological characteristics (12, 13), and species names were verified according to the Algae Base website (14) (Figure 2). Species collected from the studied sites were identified as Dictyota spiralis Montagne, Hormophysa cuneiformis (J.F. Gmelin) P.C. Silva, Polycladia myrica (S.G. Gmelin) C. Agardh, Sirophysalis trinodis (Forsskal) Kützing, Sargassum cinerum (J. Agardh), Sargassum euryphyllum (Grunow) Tseng & Lu Baoren, Sargassum latifolium (Turner) C. Agardh and Turbinaria decurrens (Bory de Saint-Vincent).
The carbohydrate content was determined using the method described by Dubois et al. (15). The total protein content was quantified in accordance with the method described by Lowry et al. (16). The lipids were determined by Soxhlet extraction on the basis of the Association of Official Analytical Chemists (AOAC) assay (17). These parameters were expressed as ratios (%). The ash content of the algae were determined by muffle incineration at 550°C in accordance with the AOAC method (17). Crude fiber was estimated by acid and alkaline digestion according to AOAC (17) method and expressed as percentage loss in weight on ignition.
where W1 is the weight (g) of the digested sample before incineration, W2 is the weight (g) of the digested sample after incineration, and W is the weight (g) of the algal sample taken.
The caloric contents were determined based on the following formula:
Chlorophyll contents “a, c, and d” and carotenoids in the algal acetone extracts (90%) were detected using the technique described by Connan (18). They are estimated using the following formula and expressed as μg/g fresh weight.
For the determination of fucoxanthin content “Fuc,” the fresh algal species was extracted in a mixture of dimethyl sulfoxide: water (4,1, v/v). The concentration of the extracted pigments was calculated using the following equation (19).
β-carotene and lycopene were determined in an acetone–hexane mixture according to Nagata and Yamashita (20), using the following formulas:
The vitamin C content of the tested algae was determined following the method of Pantelidis et al. (21). The results were expressed as mg ascorbic acid (AsA) per 100 g fresh weight (FW).
Vitamin E content was estimated in accordance with the method of Prieto et al. (22) and expressed as mg α-tocopherol equivalents per g of extract.
Total phenolic contents (TPCs) were measured using a colorimetric approach with the Folin–Ciocalteu technique (23). The absorbance of the reaction mixture was measured at 750 nm. The findings were presented in milligrams (+) gallic acid equivalents per g of seaweed dry weight (mg GAE/g DW). Total flavonoids were determined using a colorimetric method with aluminum chloride (24). The absorbance of the reaction mixture was estimated at 415 nm. The results were represented as mg (+) catechin equivalents (CE) per g of seaweed dry weight (mg CE/g DW). The tannin content was measured using a Folin–Ciocalteu reagent at 760 nm (25) and expressed as mg GAE/g DW.
Three methods were used to evaluate the antioxidant capacity of the methanolic extracts of brown species (100 μg/mL), including the total antioxidant capacity (TAC), 2,2-diphenyl-1-picrylhydrazyl (DPPH) free radical scavenging activity, and hydrogen peroxide assay.
The TAC of the methanolic algal extracts was determined in accordance with the method of Prieto et al. (22). Ascorbic acid was used as the standard. The TAC was expressed as ascorbic acid equivalents (AAE)/g.
The DPPH radical scavenging activity was determined according to the Yepez et al. (26) method. The DPPH free radical scavenging activity was calculated using the following equation:
The hydrogen peroxide activity was estimated using the spectrophotometric method of Gülçin (27). AsA was used as a standard. The percentage of algal sample scavenging activity was calculated using the formula:
Each experiment was carried out in triplicate, and the results are presented as the means and standard deviation. The statistically significant difference between the studied seaweed parameters was detected using one-way ANOVA. The correlation matrix (r) was performed using IBM-SPSS version 20 at a significance level of p ≤ 0.05 to determine the relationship among different estimated parameters.
The cell walls of brown algae are characterized by a fibrillar compartment consisting mainly of cellulose microfibrils embedded in an amorphous matrix of acidic polysaccharides bound together by proteins (28). Carbohydrates are the main components that influence different physiological responses in genes regulated during photosynthesis, metabolism, and self-protective replications. As illustrated in Figure 3 and Table 1, carbohydrate content is the main compound compared with protein and lipid contents.
The results showed that the carbohydrate content of the collected species ranged from 29.22% DW in S. cinerum to 57.32% DW in S. latifolium. These results are similar to those of brown species collected from Hurghada City during spring reported by Fouda et al. (29) (24.79–41.66% DW) and Farghl et al. (30) (27.5–38.62% DW). The variation in algal carbohydrate content may be due to algal species, growth stage, habitat, metabolic preferences, and photosynthetic activity (31). In the present work, S. cinerum achieved the maximum protein content with 25.13% DW, and the minimum content was observed in S. euryphyllum with 12.34% DW. The protein content in all studied seaweeds (12.34–25.13% DW) was greater than that in traditional protein sources, such as milk (3.4%) (32) or eggs (12.1%) (33). Protein data exhibited a higher ratio than brown algae collected from the Red Sea, as estimated by Farghl et al. (30) (5–17% DW) and Fouda et al. (29) (2.81–5.31% DW). Brown seaweeds commonly have protein contents between 3 and 15% DW. However, some species contain up to 24% protein (34). Therefore, brown seaweed-derived protein can be a source of protein that is sustainable and highly nutritious and does not require land for cultivation or fresh water for irrigation (6). The differences in the protein content of seaweeds are due to species, different habitats, time of the year, and levels of maturity (31). At present, there are few products on the functional food market that utilize seaweed proteins, most of which are used in human foods and animal feeds because of their antioxidant properties (35).
The total lipid content of brown species varied between 1.67% DW in S. cinerum and 5.21% DW in P. myrica (Figure 3). However, these values were higher than previous results of brown algae collected from the Red Sea (29) (0.11–0.27% DW) or (1.12–3.04% DW) (30). The differences in lipid contents were assigned to either environmental parameters or species types or both (31).
In general, seaweeds are characterized by their high fiber content (36). Most of the dietary fiber provided by seaweed cannot be absorbed by the human body, which causes the caloric intake of seaweed to be low. In addition, the soluble fiber contained in algae, once it reaches the human intestine, forms a viscous mass that traps digestive enzymes and some other nutrients, which slows down the digestibility of food and decreases the absorption of nutrients in the intestine (37). In the present work, the fiber content ranged from 11.01% DW in H. cuneiformis to 37.53% DW in P. myrica (Figure 1). These results are consistent with a previous work (30), who detected that brown seaweed fiber content varied between 11.63% DW and 35.65% DW. Moreover, a recent work reported that P. myrica has a higher content of dietary fiber (32.39 mg/g) than other seaweeds from the Southern Red Sea, Eritrea (38).
Seaweeds contain considerably higher concentrations of all necessary minerals than any other land vegetation, which is represented by their ash content (38). The highest ash content of the seaweed species tested was found in S. euryphyllum (45.91% DW), whereas H. cuneiformis showed the lowest ash content (26.57% DW) (Figure 3). Ash content varies with environmental, geographical and physiological factors (31). Ash contents were compatible with previous works found in seaweeds collected from the Red Sea (19.6–45.48% DW) (29) and from the Southern Red Sea (30.30–50.91%) (38). Based on the biochemical results of the selected species, P. myrica can be labeled as an alternative protein, and a high content of lipids (5.21% DW) and fiber (37.53% DW) could contribute to a sustainable and nutritious diet.
The estimated calorie value of the tested seaweeds was low, ranging between 2.32 and 3.52 kcal/100 g. A similar finding was obtained by El Zokm et al. (39), but these values were lower than those previously estimated (137.47–167.51 kcal/100 g) (40). However, low calorie values may lead to high quantities of carbohydrate and protein and low lipid contents in algal species. Consequently, these algae can be used as supplements and alternative sources to lower the risk of obesity.
The specific photosynthetic pigments and their concentrations in brown seaweeds vary depending on the environmental conditions, including season, light intensity, growth depth, and extracted solvent (19, 41). Additionally, the species and morphological structure could affect pigment concentrations (42). Information on the pigment composition and quantity of brown seaweeds may promote the selection of pigment sources for future use. The chlorophyll a and c contents of the selected species had the following ranges: 201.1–432.60 μg/g FW, 106.29–344.80 μg/g FW, and 5.36–25.87 μg/g FW. The total chlorophyll content fluctuated from 353.67 to 689.37 μg/g FW in S. cinerum and S. euryphyllum, respectively. These results indicated that S. euryphyllum is a potential source of natural pigments in brown seaweed. In accordance with our results, Garcia-Perez et al. (41) reported that the total chlorophyll content of brown seaweeds varied between 415.3 μg/g and 1555.2 μg/g FW. In addition, Ismail et al. (3) reported an average total chlorophyll content of 522 μg/g in brown seaweed.
Chlorophyll c in brown seaweed plays an important role in light harvesting antennae. In the seaweed species investigated, the chlorophyll c content in S. trinodis (25.87 μg/g FW) was higher than that in the other tested seaweeds. Osório et al. (19) reported 15.98 ± 1.6 and 17.9 ± 0.7 μg/g chlorophyll c content in the brown algae Undaria pinnatifida and Laminaria ochroleuca, respectively, which supports the obtained results. Therefore, conducting considerable research on pigment contents in algae is necessary in the future because of the significant demand for natural pigments in food products, particularly dairy, and beverages as food additives, and in cosmetics.
Carotene is a primary source of vitamin A, which can affect many different tissue types. It is also essential for retinal function (43). Carotenoids have 750 known natural sources, approximately 200 of which are collected from algal sources, and many of these are only found in a limited number of species (44). Carotenoids may participate in antioxidant activities via the transfer of the excess energy of singlet oxygen (O) in the long central allenic chain (44).
Carotenoids can scavenge oxidizing free radicals via three primary reactions: (i) electron transfer (ET) between the free radical (R·) and Crt, resulting in the formation of a Crt radical cation (Crt·+) [Equation (1)] or Crt radical anion (Crt·−) [Equation (2)]; (II) they can transfer the electrons forming a radical cation (RCrt·+) [Equation (3)]; (III) hydrogen atom transfer leading to a neutral Crt radical (Crt·) [Equation (4)] (45).
In the present research, the carotenoid content ranged from 24.2 μg/g in P. myrica to 60.44 μg/g in S. cinerum. The range of the estimated carotenoids was higher than that recorded in the brown algae S. polycystum (45.28 ± 1.77 μg/100 g DW) collected from the coast of Peninsular Malaysia by Fiedor (45), but it was lower than that estimated by Heriyanto et al. (42) (0.55 to 4.06 mg/g DW).
β-Carotene, fucoxanthin, and lycopene are obtained from the main carotenoids present in algae. β-Carotene is a liposoluble pigment that has pro-vitamin A activity with a long chain of conjugated double bonds produced from an acyclic structure. In the present investigation, the average content of β-carotene (0.52 ± 0.08 mg/100 g DW) is in accordance with that reported by De Sousa (46), with an average content of 0.4185 ± 0.1559 mg/100 g DW for brown algae Lobophora collected from Brazil. This result was lower than that recorded from the Red Sea (0.47 mg/g DW) (29) or 0.55 to 4.06 mg/g DW obtained by another work (42).
Fucoxanthin is a distinctive pigment present in brown algae, and it is a major accessory pigment found in chloroplasts, together with chlorophyll a and c and β-carotene. It masks the other pigments, like chlorophyll a, c, β carotenes, and other xanthophylls (5). In addition, it is an abundant carotenoid in nature, and it constitutes 10% of total carotenoid production (19). Fucoxanthin has large application potential as an antioxidant, anticancer, antidiabetic, antiobesity, and anti-inflammatory agent in vitro and in vivo (5, 47). Fucoxanthin exerts its antioxidant action by donating an electron to the reactive oxygen species (ROS) instead of a proton (hydrogen), as do most antioxidants, such as AsA or β-carotene. Fucoxanthin can also act on the ROS under physiological conditions of hypoxia compared to most antioxidants present in foods (24).The unique chemical structure of fucoxanthin has an allenic bond and an acetyl functional group, which are responsible for its antioxidant properties (48). Using chemiluminescence detection, Nishida et al. (49) reported that fucoxanthin had higher singlet oxygen-buffering activity than vitamin C, vitamin E, and lycopene. The fucoxanthin content ranged between 0.96 and 3.55 μg/g FW in T. decurrens and S. cinerum, respectively (Table 1). Sargassum species had the highest fucoxanthin content compared with other species. In general, Sargassaceae species had higher abundances than species from other groups (42).
Lycopene is an acyclic form of β-carotene without provitamin. It is a potent antioxidant agent, showing twice the activity of β-carotene in quenching singlet oxygen and with ten times more antioxidant activity than α-tocopherol in vitro (47). Its antioxidant effect is enhanced by the fact that it is unstable and highly reactive to oxygen and free radicals. The main difference between β-carotene and lycopene is that β-carotene is the main precursor of dietary vitamin A, whereas lycopene has no pro-vitamin A activity. Lycopene acts mainly as an antioxidant agent by activating the Nrf2/ARE transcription pathway to alleviate oxidative stress (47). Based on several clinical and epidemiological studies conducted in many populations, natural carotenoids (lutein, β-carotene, and lycopene) are beneficial to human and animal health (50). For the first time, lycopene was estimated in Egyptian seaweeds, ranging from 0.02 to 1.61 mg/100 g with an average value of 0.55 mg/100 g. Similarly, Garcia-Perez et al. (41) detected the lycopene content of different macroalgae ranging from 0.32 to 11.2 mg/100 g DW. To our knowledge, no research has indicated the existence of lycopene in the selected algal species.
The vitamin profile of seaweeds is influenced by a number of factors, including seaweed species, developmental stage, geographical location, salinity, seasons, light availability, and seawater temperature (51). Vitamins with a strong antioxidant capacity can be used as the first-line of therapeutic defense against cancer before pharmacological cancer treatment (11, 52).
Vitamin C is an essential nutrient required to maintain normal physiological functions in animal cells. Some animals cannot synthesize it, including invertebrates and fishes, because of the lack of L-gulonolactone oxidase, which catalyzes the terminal step in the conversion of glucose to AsA (53). In the current work, a higher value of vitamin C was found in P. myrica (1.27 mg AA/g DW) than in the other seaweeds investigated. A lower value (0.261 mg AA/g DW) was obtained for H. cuneiformis (Figure 4). These values are higher than those of other reported brown seaweeds from the Red Sea, such as Colpomenia sinuosa (0.0145 μg/g DW) (30). AsA has shown cancer preventive activity by neutralizing free radicals before they can damage DNA and thus initiate tumor cell growth. In addition, it can also act as a prooxidant agent, helping the body’s own free radicals to destroy tumors in their early stages (52).
Figure 4. Variation in algal vitamins (C & E) contents of the tested species. Values are means ± SD of three replicates. Similar superscript letters indicate insignificant difference between species at p ≤ 0.05 level of significance.
Vitamin E is an important fat-soluble antioxidant that prevents oxidation of polyunsaturated fatty acids absorbed from the diet. The α-tocopherols block the production of ROS formed during oxidation and inhibit the oxidation of low-density lipoproteins (11). In addition, α-tocopherol donates its phenolic hydrogen atom to a peroxyl radical and converts it to a hydroperoxide. The tocopheroyl radical formed is sufficiently stable and cannot continue the chain. It is therefore removed from the cycle by reacting with another peroxyl radical to form an inactive, non-radical product. The estimated vitamin E ranged from 1.87 mg/g DW in T. decurrens to 7.38 mg/g DW in D. spiualis (Figure 4). The average values of vitamin E in the selected samples were similar to those of Macrocystis pyrifera, which was approximately 1.33 mg/g DW (54). However, this value was higher than that estimated for the brown algae Ascophyllum and Fucus sp., which contained 0.2 and 0.6 mg/g DW of vitamin E, respectively (35).
Polyphenols contained in seaweeds represent a vast number of secondary metabolites, including approximately 8.000 natural compounds that possess vital biological functions, including antioxidant and free radical scavenging capacity (3, 9, 10). The content of phenolic and flavonoid compounds is high in algae grown under extreme climatic conditions, indicating their key role in chelating metal ions, decreasing radical generation, and strengthening the internal antioxidant system. These functions contribute to the prevention of diseases caused by the ROS. In addition, some of the polyphenols contained in marine algae are metabolites not found in terrestrial plants, which makes their contribution through algae complementary to that of terrestrial vegetables (55).
Brown seaweeds are characterized by the production of phenols and flavonoids (56). In this study, three phenolic compounds were evaluated, including phenol, flavonoids, and tannins (Figure 5). D. spiralis contained the highest total phenolic content (TPC) (52.56 mg GAE/g DW). However, S. trinodis had the lowest TPC (33.54 mg GAE/g DW). Several studies have reported comparable phenol levels (41.82 ± 0.91 mg GAE/g DW) in brown algae compared with our findings (2). The phenolic concentration in the tested algal methanolic extracts exceeded that reported by Elkhateeb et al. (57) in the ethanolic extract of S. subrepandum collected from Hurghada (2.88 ± 0.22 mg GAE/g DW); however, the estimated TPC was lower than that (29.26 ± 0.01 mg GAE/g DW) recorded in Colpmenia sinus by Shobier et al. (58) and by Farghl et al. (30) (14.14 ± 0.1 mg GAE/g DW) in T. ornate. This variation in total phenol content might be related to the types of algal species and the solvent used for extraction. The DPPH activity was evidenced in the present work by an equation that seems to depend mainly on the phenol content.
Figure 5. Phytochemical contents of phenols, flavonoids, and tannins in the tested seaweeds. Values are means ± SD of three replicates. Similar superscript letters indicate insignificant difference between species at p ≤ 0.05 level of significance.
Flavonoids consist of a large group of polyphenolic compounds with a benzo-𝛾-pyrone structure and antioxidant activity, which depends on the number and position of the free OH groups (59, 60). Flavonoids stabilize the ROS by reacting with reactive radical compounds. The hydroxyl group of flavonoids had the high reactivity to convert free radicals to inactive form as shown in the following equation (55):
where R • is a free radical and O • is an oxygen free radical.
The present study achieved a total flavonoid content (TFC) of the tested seaweeds ranging from 37.54 to 52.11 mg CAE/g DW, which was higher than that estimated by Elkhateeb et al. (57) (3.54 ± 0.59 mg CAE/g) and Farghl et al. (30) (9.98 ± 0.09 mg CAE/g DW) in different macroalgal species harvested from the Red Sea.
Tannins can chelate metal ions such as Fe(II) and interfere with one of the reaction steps in the Fenton reaction, hydrogen peroxide (H2O2), and ferric ions (Fe2+), thereby hindering oxidation. The inhibition of lipid peroxidation by tannin constituents can act via the inhibition of cyclooxygenase (56). The highest and lowest contents of tannins were found in D. spiralis (15.26 mg GAE/g DW) and S. euryphyllum (9.01 mg GAE/g DW), respectively (Figure 5). The results obtained were smaller than those detected by Shobier et al. (58), who reported that the total tannin of the brown alga C. sinuosa fluctuated from 0.14 to 4.55 mg/g DW, depending on the extracted solvents. Moreover, Ismail et al. (3) stated that the total tannin content in brown algae collected from the Mediterranean Sea ranged from 1.7 to 4.67 mg/g DW.
The antioxidant activity of seaweeds arises from their chemical compositions, including their phenolic compounds, pigments, polysaccharides, vitamins, and precursor contents (such as AsA), micro- and macroelements, and proteins (61). Antioxidants provided by seaweeds could serve as free radical scavengers and mitigate the ROS/free radicals or could contribute to preventing the formation of hydroxyl radicals by deactivating free metal ions through chelation or converting H2O2 to other innocuous compounds (such as water and oxygen) (62). Among the different types of algae, it is a well-known fact that brown algae usually have the highest antioxidant activity, followed by red and green algae (63).
Three simple methods were used to evaluate the antioxidant capacity of the methanolic extract of the brown species, including the DPPH free-radical scavenging activity, total antioxidant capacity and hydrogen peroxide assay (Figure 6; Table 2). The maximum DPPH scavenging activity of 83.97% inhibition was observed in D. spiralis, and the minimum of 65.78% was observed in S. trinodis. These results are better than the AsA standard (62.35%). These findings are consistent with those of Farghl et al. (30) in brown algae harvested from the Red Sea with a maximum inhibition of 72.48%. In contrast, the TAC approach may be broadly classified as ET, which was used to assess and evaluate the antioxidant activity of various seaweed components (electron donors). TAC showed that the scavenging value fluctuated from 6.71 mg AAE/g DW for S. euryphyllum to 31.05 mg AAE/g DW for S. cinerum. The other six species exceeded that reported by Farghl et al. (30), who recorded the maximum TAC of brown seaweeds (17.41 mg AAE/g DW). The highest and lowest H2O2 scavenging activities among the studied seaweeds were observed in D. spiralis (64.10%) and S. euryphyllum (26.53%), respectively. In general, this estimated H2O2 scavenging activity value of the tested algae was significantly higher than that of the positive control “AsA” (21.9 ± 1.12%; Figure 6). Ismail et al. (65) demonstrated that the antioxidant activity of S. aquifolium and S. euryphyllum collected from the Red Sea may be related to their polysaccharide content.
Table 3 shows the statistically significant correlation between the three antioxidant activities of the selected species and 19 estimated parameters. Significant correlations were observed between phenol, DPPH (r = 0.967), and H2O2 activity (r = 0.564), which confirmed that the total phenol content is closely linked to these antioxidant activities. Moreover, vitamin E and β-carotene exhibited a positive relationship with DPPH (r = 0.507; r = 0.67) and H2O2 activity (r = 0.832; r = 0.855), respectively. Furthermore, a significant relationship was found between flavonoids (r = 0.699) and tannins (r = 0.921) and DPPH efficiency. Positive correlations were found between Chl c, TAC (r = 0.675), and DPPH ability (r = 0.767); between lipids and TAC (r = 0.577); and between fiber and H2O2 ability (r = 0.69). This statistical analysis confirmed the vital role of different estimated antioxidants with different antioxidant mechanisms as previously illustrated.
Table 3. Statistically significant correlations between the estimated antioxidants activity and biochemical composition in the eight studied seaweeds at p ≤ 0.05.
Seaweeds contain a variety of bioactive compounds that have nutritional and therapeutic attributes, making them an intriguing potential resource. They contain low-calorie content and a variety of important nutrients, such as proteins, vitamins, and fiber, as well as the presence of bioactive substances with functional qualities that provide further benefits for health, such as phenolic compounds. There is a growing trend to use Phaeophyta in functional food production around the world and holds great promise for the future. Seaweeds are high in a variety of nutritious compounds as well as unique metabolic chemicals (phlorotannins, fucoxanthin, and sulfated polysaccharides) with intriguing bioactivities, making them excellent candidates for nutraceutical applications with higher value-added.
So, this study has an innovative approach to evaluating the value of the studied brown seaweed as a substitute food source. Applying inexpensive and simple laboratory techniques for analyzing seventeen components is very encouraging. Based on energy and calorie contents, integrating a considerable amount of seaweeds into the diet can reduce the appetite for further eating. In particular, the phenolic compounds are effective antioxidants in marine algae. Moreover, algal vitamins can be used as safe nutraceutical substances. The production of seaweed-based functional food at an industrial scale could address the limitations and challenges faced by the manufacturer. Unfortunately, there remain considerable challenges in quantifying these benefits, as well as possible adverse effects. First, there is a limited understanding of the nutritional content of various algal species, geographical regions, and seasons, all of which can substantially affect their dietary value. The second issue is quantifying which fractions of algal foods are bioavailable to humans and which factors influence how food constituents are released, ranging from food preparation through effects in the gut microbiome. The third understands how algal nutritional and functional constituents interact in human metabolism. Recently, research has been done on a wide range of food products with incorporated macroalgae, as well as some marketed macroalgae-based food products commercially available today, like food ingredients, nutraceuticals, food supplements, and food hydrocolloids.
The original contributions presented in the study are included in the article/Supplementary material, further inquiries can be directed to the corresponding author.
MI: conceptualization, collection, identification of seaweed, data processing, writing original draft, and writing review. GE: conceptualization, methodology, data processing, formal analysis, writing original draft, and writing review. JM: formal analysis, reviewing original draft, and formatting. All authors contributed to the article and approved the submitted version.
This research did not receive any specific grant from funding agencies in the public, commercial, or not-for-profit sectors.
The authors acknowledge the financial assistance provided by the Universidad de Santiago de Compostela, for recovering the publishing fee. Also, the authors are grateful to Madiha Helmy Mostafa (Master student, geophysics and geology department Faculty of Science, Alexandria University) for her kind help in preparing the maps.
The authors declare that the research was conducted in the absence of any commercial or financial relationships that could be construed as a potential conflict of interest.
All claims expressed in this article are solely those of the authors and do not necessarily represent those of their affiliated organizations, or those of the publisher, the editors and the reviewers. Any product that may be evaluated in this article, or claim that may be made by its manufacturer, is not guaranteed or endorsed by the publisher.
The Supplementary material for this article can be found online at: https://www.frontiersin.org/articles/10.3389/fnut.2023.1210934/full#supplementary-material
1. Duarte, CM, Marbá, N, and Holmer, M. Rapid domestication of marine species. Sci. (2007) 316:382–3. doi: 10.1126/science.1138042
2. Sultana, F, Abdul Wahad, M, Nahiduzzaman, M, Mohiuddin, M, Zafar Iqbal, M, Shakil, A, et al. Seaweed farming for food and nutritional security, climate change mitigation and adaptation, and women empowerment: a review. Aquac Fish. (2023) 8:463–80. doi: 10.1016/j.aaf.2022.09.001
3. Ismail, MM, El Zokm, GM, and El-Sayed, AAM. Variation in biochemical constituents and master elements in common seaweeds from Alexandria coast, Egypt, with special reference to their antioxidant activity and potential food uses: prospective equations. Environ Monit Assess. (2017) 189:648. doi: 10.1007/s10661-017-6366-8
4. Choudhary, B, Chauhan, OP, and Mishra, A. Edible seaweeds: a potential novel source of bioactive metabolites and nutraceuticals with human health benefits. Front Mar Sci. (2021) 8:740054. doi: 10.3389/FMARS.2021.740054
5. Pajot, A, Huynh, GH, Picot, L, Marchal, L, and Nicolau, E. Fucoxanthin from algae to human, an extraordinary bioresource: insights and advances in up and downstream processes. Mar Drugs. (2022) 20:222. doi: 10.3390/md20040222
6. Lopez-Santamarina, A, Miranda, JM, Mondragon, AC, Lamas, A, Cardelle-Cobas, A, Franco, CM, et al. Potential use of marine seaweeds as prebiotics: a review. Molecules. (2020) 25:1004. doi: 10.3390/molecules25041004
7. Tagliaprieta, BL, and Pedrosa, MT. Brown algae and their multiple applications as functional ingredientes in food production. Food Res Int. (2023) 167:112655. doi: 10.1016/j.foodres.2023.112655
8. Shannon, E, and Abu-Ghannam, N. Seaweeds as nutraceuticals for health and nutrition. Phylocologia. (2019) 58:563–77. doi: 10.1080/00318884.2019.1640533
9. Ismail, MM, Gheda, SF, and Pereira, L. Variation in bioactive compounds in some seaweeds from abo Qir bay, Alexandria. Egypt Rend Fis Acc Lincei. (2016) 27:269–79. doi: 10.1007/s12210-015-0472-8
10. Ismail, GA, and Ismail, MM. Variation in oxidative stress indices of two green seaweeds growing under different heavy metal stresses. Environ Monit Assess. (2017) 189:68. doi: 10.1007/s10661-017-5775-z
11. Michalak, I, Tiwari, R, Dhawan, M, Alagawany, M, Farag, MR, Sharun, K, et al. Antioxidant effects of seaweeds and their active compounds on animal health and production–a review. Vet Q. (2002) 42:48–67. doi: 10.1080/01652176.2022.2061744
12. Oza, RM. A revised checklist of Indian marine algae, vol. 111. Bhavnagar: CSMCR Institute (2001). 296 p.
14. Guiry, MD, and Guiry, GM, Algae base. World-wide electronic publication. Galway, Ireland: National University of Ireland (2022).
15. Dubois, M, Gilles, KA, Hamilton, JK, Rebers, PA, and Smith, F. Colorimetric method for determination of sugars and related substances. Anal Chem. (1956) 28:350–6. doi: 10.1021/ac60111a017
16. Lowry, OH, Rosebrough, NJ, Farr, AL, and Randall, RJ. Protein measurement with the Folin phenol reagent. J Biol Chem. (1951) 193:265–75. doi: 10.1016/S0021-9258(19)52451-6
17. Association of Official Analytical Chemists (AOAC). Official methods of analysis. 17th ed. Gaithersburg, MD: The Association of Official Analytical Chemists (2022).
18. Connan, S. Spectrophotometric assays of major compounds extracted from algae. Methods Mol Biol. (2015) 1308:75–101. doi: 10.1007/978-1-4939-2684-8_3
19. Osório, C, Machado, S, Peixoto, J, Bessada, S, Pimentel, FB, Alves, RC, et al. Pigments content (chlorophylls, fucoxanthin and phycobiliproteins) of different commercial dried algae. Separations. (2020) 7:1–14. doi: 10.3390/SEPARATIONS7020033
20. Nagata, M, and Yamashita, I. Simple method for simultaneous determination of chlorophyll and carotenoids in tomato fruit. Nippon Shokuhin Kogyo Gakkaishi. (1992) 39:925–8. doi: 10.3136/nskkk1962.39.925
21. Pantelidis, GE, Vasilakakis, M, Manganaris, GA, and Diamantidis, G. Antioxidant capacity, phenol, anthocyanin and ascorbic acid contents in raspberries, blackberries, red currants, gooseberries and cornelian cherries. Food Chem. (2007) 102:777–83. doi: 10.1016/j.foodchem.2006.06.021
22. Prieto, P, Pineda, M, and Aguilar, M. Spectrophotometric quantitation of antioxidant capacity through the formation of a phosphomolybdenum complex: specific application to the determination of vitamin E. Anal Biochem. (1999) 269:337–41. doi: 10.1006/abio.1999.4019
23. Taga, MS, Miller, EE, and Pratt, DE. Chia seeds as a source of natural lipid antioxidants. J Am Oil Chem Soc. (1984) 61:928–31. doi: 10.1007/BF02542169
24. Yan, X, Chuda, Y, Suzuki, M, and Nagata, T. Fucoxanthin as the major antioxidant in hijikia fusiformis, a common edible seaweed. Biosci Biotechnol Biochem. (1999) 63:605–7. doi: 10.1271/BBB.63.605
25. Tambe, VD, and Bhambar, RS. Estimation of total phenol, tannin, alkaloid and flavonoid in Hibiscus tiliaceus Linn. Wood extracts. J Pharmacogn Phytochem. (2014) 7:1–6. doi: 10.36348/sb.2021.v07i01.001
26. Yepez, B, Espinosa, M, López, S, and Bolaos, G. Producing antioxidant fractions from herbaceous matrices by supercritical fluid extraction. Fluid Phase Equilib. (2022) 194-197:879–84. doi: 10.1016/S0378-3812(01)00707-5
27. Gülçin, I, Sat, IG, Beydemir, S, and Küfrevioglo, ÖI. Evaluation of the in vitro antioxidant properties of broccoli extracts (Brassica oleracea L.). Ital J Food Sci. (2004) 16:17–30.
28. Michel, G, Tonon, T, Scornet, D, Cock, JM, and Kloareg, B. Central and storage carbon metabolism of the brown alga Ectocarpus siliculosus: insights into the origin and evolution of storage carbohydrates in eukaryotes. New Phytol. (2010) 188:67–81. doi: 10.1111/j.1469-8137.2010.03345.x
29. Fouda, WA, Ibrahim, WM, Ellamie, AM, and Ramadan, G. Biochemical and mineral compositions of six brown seaweeds collected from Red Sea at Hurghada coast. Indian J Mar Sci. (2019) 48:484–91.
30. Farghl, AAM, Al-Hasawi, ZM, and El-Sheekh, MM. Assessment of antioxidant capacity and phytochemical composition of brown and red seaweeds sampled off red sea coast. Appl Sci. (2021) 11:11079. doi: 10.3390/app112311079
31. Mwalugha, HM, Wakibia, JG, Kenji, GM, and Mwasaru, MA. Chemical composition of common seaweeds from the Kenya coast. J Food Res. (2015) 4:28–38. doi: 10.5539/jfr.v4n6p28
32. Gellrich, K, Meyer, HHD, and Wiedemann, S. Composition of major proteins in cow milk differing in mean protein concentration during the first 155 days of lactation and the influence of season as well as short term restricted feeding in early and mid-lactation. Czech J Anim Sci. (2014) 59:97–106. doi: 10.17221/7289-CJAS
33. Miranda, JM, Anton, X, Redondo-Valbuena, C, Roca-Saavedra, P, Rodriguez, JA, Lamas, A, et al. Egg and egg-derived foods: effects on human health and use as functional foods. Nutrients. (2015) 7:706–29. doi: 10.3390/nu7010706
34. O' Brien, RO’, Hayes, M, Sheldrake, G, Tiwari, B, and Walsh, P. Macroalgal proteins: A review. Foods. (2022) 11:571. doi: 10.3390/foods11040571
35. Peñalver, R, Lorenzo, JM, Ros, G, Amarowicz, R, Pateiro, M, and Nieto, G. Seaweeds as a functional ingredient for a healthy diet. Mar Drugs. (2020) 18:301. doi: 10.3390/md18060301
37. Rajapakse, N, and Kim, SK. Nutritional and digestive health benefits of seaweed. Adv Food Nutr Res. (2011) 64:17–28. doi: 10.1016/B978-0-12-387669-0.00002-8
38. Kasimala, M, Mogos, GG, Negasia, KT, and Bereketa, GA. Biochemical composition of selected seaweeds from intertidal shallow waters of southern Red Sea. Eritrea Indian J Mar Sci. (2020) 49:1153–7.
39. El Zokm, GM, Ismail, MM, and El-Said, GF. Halogen content relative to the chemical and biochemical composition of fifteen marine macro and micro algae: nutritional value, energy supply, antioxidant potency, and health risk assessment. Environ Sci Pollut Res. (2021) 28:14893–908. doi: 10.1007/s11356-020-11596-0
40. Ismail, MM, and El-Sheekh, M. Enhancement of biochemical and nutritional contents of some cultivated seaweeds under laboratory conditions. J Diet Suppl. (2018) 15:318–29. doi: 10.1080/19390211.2017.1345031
41. Garcia-Perez, P, Lourenço-Lopes, C, Silva, A, Pereira, AG, Fraga-Corral, M, Zhao, C, et al. Pigment composition of nine brown algae from the Iberian Northwestern coastline: influence of the extraction solvent. Mar Drugs. (2022) 20:113. doi: 10.3390/md20020113
42. Heriyanto, J, Shioi, Y, Limantara, L, and Brotosudarmo, THP. Analysis of pigment composition of brown seaweeds collected from Panjang Island, Central Java, Indonesia. Philipp J Sci. (2017) 146:323–30.
43. Amengual, J, Gouranton, E, van Helden, YGJ, Hessel, S, Ribot, J, Kramer, E, et al. Beta-carotene reduces body adiposity of mice via BCMO1. PLoS One. (2011) 6:e20644. doi: 10.1371/journal.pone.0020644
44. Saini, RK, Prasad, P, Lokesh, V, Shang, X, Shin, J, Keum, YS, et al. Carotenoids: dietary sources, extraction, encapsulation, bioavailability, and health benefits a review of recent advancements. Antioxidants. (2022) 11:795. doi: 10.3390/antiox11040795
45. Fiedor, J, and Burda, K. Potential role of carotenoids as antioxidants in human health and disease. Nutrients. (2014) 6:466–88. doi: 10.3390/nu6020466
46. De Sousa, MB, Dos Santos Pires, KM, De Alencar, DB, Sampaio, AH, and Saker-Sampaio, S. α- and β-carotene, and α-tocopherol in fresh seaweeds. Cienc Tecnol Aliment. (2008) 28:953–8. doi: 10.1590/S0101-20612008000400030
47. Lann, K, Ferret, C, Vanmee, E, Spagnol, C, Lhuillery, M, Payri, C, et al. Total phenolic, size-fractionated phenolics and fucoxanthin content of tropical Sargassaceae (Fucales, Phaeophyceae) from the South Pacific Ocean: spatial and specific variability. Phycol Res. (2012) 60:37–50. doi: 10.1111/j.1440-1835.2011.00634.x
48. Miyashita, K, Beppu, F, Hosokawa, M, Liu, X, and Wang, S. Bioactive significance of fucoxanthin and its effective extraction. Biocatal Agric Biotechnol. (2020) 26:101639. doi: 10.1016/j.bcab.2020.101639
49. Nishida, Y, Yamashita, E, and Miki, W. Quenching activities of common hydrophilic and lipophilic antioxidants against singlet oxygen using chemiluminescence detection system. Carotenoid Sci. (2007) 11:16–20. doi: 10.11501/10996240
50. Mtaki, K, Kyewalyanga, MS, and Mtolera, MSP. Assessment of antioxidant contents and free radical-scavenging capacity of chlorella vulgaris cultivated in low cost media. Appl Sci. (2020) 10:1–11. doi: 10.3390/app10238611
51. Norziah, MH, and Ching, CY. Nutritional composition of edible seaweed Gracilaria changgi. Food Chem. (2000) 68:69–76. doi: 10.1016/S0308-8146(99)00161-2
52. Nielsen, CW, Rustad, T, and Holdt, SL. Vitamin C from seaweed: a review assessing seaweed as contributor to daily intake. Foods. (2021) 10:198. doi: 10.3390/foods10010198
53. Drouin, G, Godin, JR, and Pagé, B. The genetics of vitamin C loss in vertebrates. Curr Genomics. (2011) 12:371–8. doi: 10.2174/138920211796429736
54. Ortiz, J, Uquiche, E, Robert, P, Romero, N, Quitral, V, and Llantén, C. Functional and nutritional value of the Chilean seaweeds Codium fragile, Gracilaria chilensis and Macrocystis pyrifera. Eur J Lipid Sci Technol. (2009) 111:320–7. doi: 10.1002/ejlt.200800140
55. Sugiura, Y, Katsuzaki, H, Imai, K, and Amano, H. The anti-allergic and anti-inflammatory effects of phlorotannins from the edible brown algae, Ecklonia sp. and Eisenia sp. Nat Prod Commun. (2021) 16:1934578X2110609. doi: 10.1177/1934578X211060924
56. Kalasariya, HS, Pereira, L, and Patel, NB. Pioneering role of marine macroalgae in cosmeceuticals. Phycologia. (2022) 2:172–203. doi: 10.3390/phycology2010010
57. Elkhateeb, MI, El-Bitar, AMH, Saleh, SR, and Abdelreheem, AMA. Evaluation of bioactive phytochemical characterization, antioxidant, antimicrobial, and antihemolytic properties of some seaweeds collected from Red Sea coast. Egypt Egypt J Aquat Biol Fish. (2021) 25:417–36. doi: 10.21608/EJABF.2021.190299
58. Shobier, AH, Ismail, MM, and Hassan, SWM. Variation in anti-inflammatory, anti-arthritic, and antimicrobial activities of different extracts of common Egyptian seaweeds with an emphasis on their phytochemical and heavy metal contents. Biol Trace Elem Res. (2022) 201:2071–87. doi: 10.1007/s12011-022-03297-1
59. Guven, H, Arici, A, and Simsek, O. Flavonoids in our foods: a short review. J Basic Clin Appl Heatlh Sci. (2019) 3:96–106. doi: 10.30621/jbachs.2019.555
60. Tsuneda, T. (2020). Fenton reaction mechanism generating no OH radicals in Nafion membrane decomposition. Sci Rep. (2020) 10:1–13. doi: 10.1038/s41598-020-74646-0
61. Begum, R, Howlader, S, Mamun-Or-Rashid, ANM, Rafiquzzaman, SM, Ashraf, GM, Albadrani, GM, et al. Antioxidant and signal-modulating effects of brown seaweed-derived compounds against oxidative stress-associated pathology. Oxidative Med Cell Longev. (2021) 2021:9974890. doi: 10.1155/2021/9974890
62. Rajauria, G, Jaiswal, AK, Abu-Gannam, N, and Gupta, S. Antimicrobial, antioxidant and free radical-scavenging capacity of brown seaweed Himanthalia elongata from western coast of Ireland. J Food Biochem. (2013) 37:322–35. doi: 10.1111/j.1745-4514.2012.00663.x
63. Vladkova, T, Georgieva, N, Staneva, A, and Gospodinova, D. Recent progress in antioxidant active substances from marine biota. Antioxidants. (2022) 11:439. doi: 10.3390/antiox11030439
64. Ismail, MM, Mostafa, MH, and El-Sikaily, A. Phytochemical variation, antioxidant and antidiabetic capacity of extracts of common brown seaweeds from the Red Sea. Egypt Egypt J Aquat Biol Fish. (2022) 26:445–62. doi: 10.21608/ejabf.2022.262605
Keywords: antioxidants, Phaeophyta, phenolic compounds, pigments, vitamins, proximate
Citation: Ismail MM, El Zokm GM and Miranda Lopez JM (2023) Nutritional, bioactive compounds content, and antioxidant activity of brown seaweeds from the Red Sea. Front. Nutr. 10:1210934. doi: 10.3389/fnut.2023.1210934
Received: 23 April 2023; Accepted: 11 July 2023;
Published: 26 July 2023.
Edited by:
Elliot Berry, Hebrew University of Jerusalem, IsraelReviewed by:
Wan Abd Al Qadr Imad Wan-Mohtar, University of Malaya, MalaysiaCopyright © 2023 Ismail, El Zokm and Miranda Lopez. This is an open-access article distributed under the terms of the Creative Commons Attribution License (CC BY). The use, distribution or reproduction in other forums is permitted, provided the original author(s) and the copyright owner(s) are credited and that the original publication in this journal is cited, in accordance with accepted academic practice. No use, distribution or reproduction is permitted which does not comply with these terms.
*Correspondence: Mona M. Ismail, bW0uZXNtYWlsQG5pb2Yuc2NpLmVn; bW9uYV9lczVAeWFob28uY29t
Disclaimer: All claims expressed in this article are solely those of the authors and do not necessarily represent those of their affiliated organizations, or those of the publisher, the editors and the reviewers. Any product that may be evaluated in this article or claim that may be made by its manufacturer is not guaranteed or endorsed by the publisher.
Research integrity at Frontiers
Learn more about the work of our research integrity team to safeguard the quality of each article we publish.