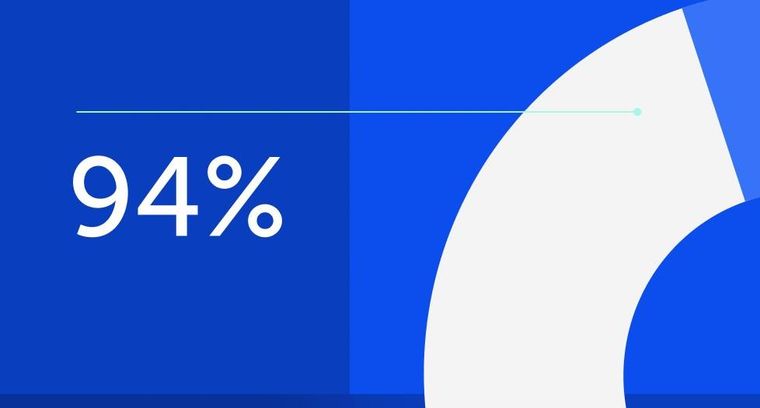
94% of researchers rate our articles as excellent or good
Learn more about the work of our research integrity team to safeguard the quality of each article we publish.
Find out more
ORIGINAL RESEARCH article
Front. Nutr., 29 June 2023
Sec. Sport and Exercise Nutrition
Volume 10 - 2023 | https://doi.org/10.3389/fnut.2023.1205310
This article is part of the Research TopicNew Insights and Advances in Body RecompositionView all 5 articles
Background: The effects of a high protein diet in combination with chronic resistance training (RT) on skeletal muscle adaptation responses in untrained older ex-military men is unknown. Therefore, we compared the effects of 8 weeks of RT in combination with either a high (1.6 g/kg/d) or low protein diet (0.8 g/kg/d) on body composition [skeletal muscle mass (SMM) and body fat percentage (BFP)], muscular strength, power, and endurance (upper and lower body), markers of liver [alanine transaminase (ALT), aspartate aminotransferase (AST), and gamma-glutamyl transferase (GGT)] and kidney (creatinine and urea) function, and lipid profile low-density lipoprotein (LDL), high-density lipoprotein (HDL), and cholesterol levels in a cohort of healthy, untrained older ex-military males.
Methods: Forty healthy untrained older ex-military males (age: 61 ± 2 yr, body mass index: 23.2 ± 1.3 kg.m−2) performed 8 weeks (three sessions·w−1) of RT with either 1.6 g/kg/d (RHP; n = 20) or 0.8 g/kg/d of protein (RLP; n = 20). Body composition (assessed by Inbody 720), muscular strength (1-RM for chest and leg press), power (Wingate test), endurance (75% 1-RM for chest and leg press), and markers of liver and kidney function (biochemical kits) were assessed pre and post-intervention.
Results: SMM and muscular strength (upper and lower body) increased post-intervention in both groups and were significantly greater in RHP compared to RLP, while muscular power increased to the same extent in both groups (p < 0.05) with no between-group differences (p > 0.05). In contrast, there were no post-intervention changes in muscular endurance, HDL, and BFP remained in either group (p > 0.05). ALT and creatinine significantly increased in RHP compared to RLP while GGT, AST, and urea only increased in the RLP group (p < 0.05). LDL and cholesterol significantly decreased in both groups (p < 0.05).
Conclusion: A daily intake of 1.6 g/kg/d protein was superior to 0.8 g/kg/d (current recommended daily intake) for promoting greater improvements in SMM and muscle strength and thus may be a more suitable level of intake for promoting such adaptive responses. Notwithstanding observed between-group differences in ALT and creatinine and the fact that levels remained within normal ranges, it is feasible to conclude that this daily protein intake is efficacious and well tolerated by healthy, untrained older ex-military males.
Quality and quantity of skeletal muscle and physical function are of importance with advancing age (1). Indeed, skeletal muscle mass (SMM) and strength/power decline at rates of 0.8–1% and 2–3% each year, respectively (2). While it is difficult to precisely pinpoint the prevalence of sarcopenia in the community, current statistics indicate that 2.5 to 30% of older persons (≥ 60 years) are classified as having low SMM (3, 4). The delicate balance between muscle protein synthesis and muscle protein breakdown determines SMM (5). The existence of anabolic resistance reduces the sensitivity of older adults to anabolic stimuli and dietary protein intake, irrespective of the fact that evidence suggests that skeletal muscle is typically well-maintained in young, healthy people who do not engage in scheduled physical activity (6). Preserving SMM in the older population is thus complicated. Consequently, the selection and implementation of effective physical activity and dietary approaches to combat the reduced anabolism in the skeletal muscle of older persons may assist to enhance muscular hypertrophy or at the very least aid in the maintenance of SMM (7), particularly in retired military personnel.
Resistance training (RT) serves as the most efficient non-pharmaceutical stimulant for increasing SMM, strength, and physical function, and is consequently the most effective intervention for age-related sarcopenia (8–11). Although muscle protein synthesis is significantly enhanced after RT is executed in a post-adaptive state (i.e., when fasting), muscle protein breakdown is also elevated, and the muscle net protein balance consequently maintains negative (12). Protein intake, especially essential amino acids in somewhat close temporal approximation, such as <3–4 h after RT, increases muscle protein synthesis while decreasing muscle protein breakdown, which supports a favorable muscle net protein balance (13). Regarding total daily protein intake, the current recommended daily allowance has remained stable over many decades at 0.8 g/kg/d (14). Due to the prevalence of anabolic resistance in older adults, certain expert groups (15, 16) have advised protein needs between 1 and 1.5 g/kg/d (16). In support, higher daily protein intakes (>78.5 g/d) have been linked to increased SMM retention in older males (17). In addition, a large number of research on older persons indicate that increased protein intake is favorably linked with step count and adversely associated with inactive time in older adults (18). Additionally, older persons who consumed over 1 g/kg/d protein had a 22% lower risk of functional impairment as measured by 19 functional tests (19) while a six-year study in persons aged 60 and older observed a correlation between protein intake and grip strength maintenance (20). While these findings collectively suggest that older persons who desire to retain SMM and function may get substantial advantages from a protein intake in excess of the recommended daily allowance, several lines of evidence suggest a higher protein diet is hazardous to renal and bone health (21–24). However, these perceptions remain unsupported (14, 25–29). In the absence of renal disease, greater protein intake is linked with normal kidney function (28) and is associated with an enhanced glomerular filtration rate (25). Furthermore, protein intake over the recommended daily allowance may be advantageous for bone health (14) and may assist to lower the risk of hip fracture and bone mineral density loss (26, 29). Therefore, despite the fact that the daily protein intake of the majority of older individuals is insufficient and worsened with age, it is safe for older adults to consume protein over the recommended daily allowance (~1.6 g/kg/d), and this seems to be advantageous for combating sarcopenia.
Military recruit training programs are intended to transform untrained civilians into trained soldiers; consequently, physical training is necessarily rigorous, consisting of a combination of endurance training, strength and conditioning, obstacle courses, swimming, circuit training, and loaded marching (30). Although numerous studies (both original and review) to date have been published in older adults following RT and protein (dietary or supplemental form) intake indicating improved body composition and muscular performance (8, 31, 32), research on retired military older adults is lacking. Therefore, we compared the effects of 8 weeks of RT in the combination of 1.6 g/kg/d of protein vs. 0.8 g/kg/d on body composition, muscular performance (strength, power, and endurance), and biochemical markers of liver and kidney function in a cohort of healthy, untrained older ex-military males.
The current research included 40 healthy, untrained ex-military older males (>55 years). At the gym, interested participants were educated about the research and testing procedures in person. Participants were required to self-report a health and fitness history questionnaire, confirming that they had not exercised in the previous 6 months, sleeping for at least seven to 8 h per day, not taking any steroids or illegal agents known to increase SMM in the previous year, less than 0.8 g/kg/d of protein ingestion, and being free of musculoskeletal disorders. Participants who were deemed eligible based on the aforementioned criteria supplied written and verbal agreement to participate. In addition, while agreeing, a medical history questionnaire was gathered, and individuals were requested to return to complete the research procedures. Participants were ex-military for five to 10 years since they retired and were in the military for 30 to 35 years. The protocol was reviewed by the Institutional Human Subject Committee and the Ethics Committee of Baqiyatallah University of Medical Sciences (IR.BMSU.BAQ.REC.1401.132) and carried out in accordance with the Declaration of Helsinki. This study has been registered with the Iranian Registry of Clinical Trials (IRCT20191204045612N3).
Figure 1 depicts an overview of the study protocol. Following baseline measures (detailed below), participants were acquainted with the study tests and procedures before being randomly assigned (using a digital tool)1 to one of two groups for an 8-week intervention involving either RT + 1.6 g/kg/d of protein (RHP; n = 20) or RT + 0.8 g/kg/d of protein (RLP; n = 20). Measurements were taken at the same time of day at baseline and week 9 (the week after the training protocols were finished). Participants first completed four preliminary testing days: questionnaires were assessed on the first visit; blood sampling and body composition were performed on the second visit; muscular strength and endurance (75% 1-RM) were performed on the third visit; and a muscular power test was performed on the fourth visit. Following the completion of these tests, participants met with the study nutritionist for an initial session to establish dietary preferences as well as target protein and energy intakes before beginning training procedures. The Pittsburgh Sleep Quality Index (PSQI) and the General Health Questionnaire-28 (GHQ-28) were used to measure sleep quality and health status, respectively. All tests were carried out in the precise sequence specified for all time measurements.
Participants were instructed to arrive at the lab hydrated following an overnight fast, and a 24-h food recall was gathered before testing. To avoid hydration status mistakes, participants were urged to urinate entirely within 30 min of the test and to abstain from caffeinated drinks, alcohol, and other diuretics 12 h before measurements. Body mass and height were measured using a digital scale (Lumbar, China) to the closest 0.1 kg and a stadiometer (Race industrialization, China), respectively. As previously described, a multi-frequency bioelectrical impedance device (Inbody 720, South Korea) was used to measure SMM, body fat percentage (BFP), and body mass index (BMI) (9). Before the measurement, the participant’s palms and soles were cleaned with an electrolyte tissue. Subjects then stood on the InBody 720, placing the soles of their feet on the electrodes. The instrument derived the participant’s body mass, and their age and sex were manually entered into the display by a researcher. Subjects then grasped the handles of the unit ensuring that the palm and fingers of each hand made direct contact with the electrodes, with arms fully extended and abducted approximately 20o. Analysis of body composition was determined by the unit with participants remaining as motionless as possible (33). The bioelectrical impedance approach has good test–retest reliability (R = 0.97 to 0.99).
Maximal strength was evaluated using 1-RM for the leg press and chest press, and these measures were also used to estimate training intensity for RT regimens. Before commencing the test, the researchers described the goal, associated risks, discomforts, participant obligations, benefits, questions, and permission. Before the testing session, participants were told to abstain from consuming alcohol for 48 h, caffeinated beverages for 12 h, and meals for 2 h. Water consumption was permitted. Before the test, participants completed a general 10-min warm-up (5 min of slow jogging on a treadmill; 3–5 km/h or elliptical; with 5–10 level) and specialized warm-up exercises (two to three sets of light RT). Participants then completed two attempts, noting their heaviest weight lifted and the number of repetitions. The number of repetitions required to reach fatigue did not surpass ten. The participants were given 3–5 min of rest between trials, and there was no stimulating input throughout testing. Using the formula 1-RM = weight/ (1.0278–0.0278 reps) (34), the maximum strength of participants was estimated after the testing session.
Upper- and lower-body anaerobic power was assessed via Monark Wingate cycle ergometry (Monark model 894e, Vansbro, Sweden) as previously described (35, 36). Briefly, participants were acquainted with the test and instructed to stay seated in the saddle for the test duration. Participants cycled or cranked against a pre-determined resistance (7.5% of body mass for the lower body test and 5.5% for the upper body test) as fast as possible for 30 s. Participants were verbally encouraged to pedal as hard and fast as possible throughout the whole 30 s test. Peak power output was documented in real-time during the test using Monark Anaerobic test software (3.3.0.0).
After completing the 1-RM and power testing in the morning, participants were instructed to perform leg-and chest press exercises at 75% of the 1-RM to test muscular endurance, denoted as the number of successful repetitions completed before technical failure (i.e., point where participants fail to achieve another repetition in good technical form) in the evening (37).
Fasting blood samples (5 mL) were taken from the cubital vein using standard procedures following an 8-h overnight fast at the same time of day in pre-and post-testing. Liver enzymes [alanine transaminase (ALT; intra-assay CV: 1.81%; inter-assay CV: 2%)], aspartate aminotransferase (AST; intra-assay CV: 2.01%; inter-assay CV: 2.54%), and gamma-glutamyl transferase (GGT; intra-assay CV: 1.56%; inter-assay CV: 0.92%), creatinine (intra-assay CV: 1.60%; inter-assay CV: 2.24%), and Urea Nitrogen (BUN) (intra-assay CV: 2.20%; inter-assay CV: 3.36%), were measured in serum. Liver and kidney function markers and lipid profiles [low-density lipoprotein (LDL; intra-assay CV: 0.64%; inter-assay CV: 1.37%), high-density lipoprotein (HDL; intra-assay CV: 0.77%; inter-assay CV: 1.80%), cholesterol (intra-assay CV: 1.11%; inter-assay CV: 1.18%)].
were measured in duplicate using Pars Azmoon kits and the spectrophotometric method (DiaSys Diagnostic Systems GmbH, Germany). These were performed after 48 h of rest.
Before the beginning of the study, all participants performed 1 week of RT, consisting of three exercise sessions, for familiarization before the main training intervention. This phase allowed instruction relating to correct lifting technique, and familiarization with all exercises and equipment, and ensured that the participants initiated the study with a comparable training base (38). Following the preparatory phase, RT began at 50% of 1-RM at the beginning of the training intervention and progressed to 80% of 1-RM in the last week of training (Table 1). Repetitions varied from 6 to 12 and exercises (in order) included leg extension, leg curl, leg press, calf raises, chest press, lat pulldown, lateral raise, biceps curl, triceps extension, abdominal crunch, and back extension. Participant supervision was performed using one personal trainer for five participants (eight personal trainers in total). Maximum strength testing (1-RM) was been performed every 2 weeks to subsequently adjust training intensity. The periodized RT protocols were adapted from previous literature on older adults (9, 39–42). RT volume was calculated using the following formula in each session and was reported weekly (43):
Estimated training volume = [repetitions (n) × sets (n) × load or selected weight (kg)].
Participants completed six 24 h dietary logs (4 non-consecutive weekdays and 2 non-consecutive weekend days) to determine habitual protein intakes. To assist in achieving their targeted protein intake (i.e., 0.8 or 1.6 g/kg/d), participants consumed 0.4 g/kg of dietary protein after each session and other meals based on the recommendations for older adults to maximally stimulate muscle protein synthesis (7). Participants attended consultations with an accredited practicing dietitian every week, where they were provided guidelines to reach protein and energy needs. Protein quantities were consumed via foods, and habitual dietary protein intake remained stable throughout the intervention for both groups. Carbohydrate and fat intake were suggested to be within the Acceptable Macronutrient Distribution Range for these macronutrients (45–65% and 20–35% TEI for carbohydrate and fat, respectively). Participants were asked to remain in a positive energy balance to alleviate any potential of energetic stress-related interferences to anabolic adaptations (44, 45). Food records were kept daily by participants throughout the study using “My Fitness Pal” mobile phone application (MyFitnessPal Inc., United States). All dietary intake data were analyzed using (Diet Analysis Plus, version 10; Cengage) to ensure the same food database was used for all analyses.
A priori sample size calculation was conducted using G-power 3.1.9.2 software. The rationale for the sample size was based on our previous work which documented significant improvements in lean mass after 8 weeks of high protein diet in older males (8). By utilizing the equation for effect size (ES) [(mean before-mean after the high protein diet)/the pooled standard deviation], this study revealed an ES of 0.34 [(43.9–45.2)/3.78]. In the present study and based on α = 0.05, a power (1-β) of 0.80, and an ES = 0.34 (highest approximate effect size), a total sample size of at least 34 participants (n = 17 per group) was needed for sufficient power to detect significant changes in the primary outcome of lean mass. We recruited 20% more participants due to potential dropouts. The normality of the distribution of all variables was evaluated before performing statistical analyzes using the Shapiro–Wilk test; there were no missing values at any time point. Baseline characteristics (at PRE) between groups were reported using mean (SD). The student’s t-test was used for group comparisons at baseline. Effects of training and nutritional interventions on dependent variables were analyzed using analysis of covariance (ANCOVA) to determine the differences between the groups over time. Training volume was analyzed using repeated measures of ANOVA. Pearson’s simple linear regressions were performed with a 95% confidence interval (CI). Values between 0 and 0.3 (0 and-0.3) indicate a weak positive (negative) linear relationship through a shaky linear rule. Values between 0.3 and 0.7 (−0.3 and − 0.7) indicate a moderate positive (negative). Values between 0.7 and 1.0 (−0.7 and − 1.0) indicate a strong positive (negative) (46). All analyses were performed using SPSS 26, and figure production was performed using GraphPad Prism (version 8.4.3) and adobe illustrator artwork (version 25).
Sixty participants were assessed for eligibility and twenty did not meet the inclusion criteria (Figure 2). Three participants from each group (personal issues and COVID-19) withdrew from the study. There were no significant between-group differences in all baseline characteristics (Table 2). There were no differences between groups for PSQI (p = 0.556) and GHQ-28 (p = 0.095).
Values are presented as mean ± standard deviation. Abbreviations: PSQI, Pittsburgh Sleep Quality Index; GHQ-28, General Health Questionnaire; BMI, body mass index; SMM, skeletal muscle mass; BFP, body fat percentage; GGT, gamma-glutamyl transferase; AST, aspartate aminotransferase; ALT, alanine aminotransferase; HDL, high-density lipoprotein; LDL, low-density lipoprotein; y, year; cm, centimeter; kg, kilogram; kg.m−2, kilogram.meter−2; g, gram; %, percentage; r, repetition; w, watt; pg./ml, picograms/milliliter; ng/ml, nanogram/milliliter; u/L, unit/liter; mg/dL, milligrams/deciliter; RHP, RT + 1.6 g/kg/d; RLP, RT + 0.8 g/kg/d.
Changes in body composition throughout the intervention are shown in Figure 3. Body mass [RHP = 1.7 kg (95% CI = 1.3 to 2.2, p < 0.001) and RLP = 0.9 kg (95% CI = 0.62 to 1.1, p < 0.001)], SMM [RHP = 1.3 kg (95% CI = 0.95 to 1.6, p < 0.001) and RLP = 0.7 kg (95% CI = 0.5 to 1, p < 0.001), Figure 3A] all significantly increased from baseline to post-intervention in both groups. However, BFP remained unchanged (Figure 3B) in both groups over time (p > 0.05). ANCOVA results indicated significant between-group differences for body mass (p = 0.002) and SMM (p = 0.008) with greater changes in the RHP group over time.
Figure 3. Effects of resistance training in combination with high and low protein diet on body composition and muscular performance. (A) Skeletal muscle mass (SMM; kg), (B) body fat percentage (BFP; %), (C) upper body strength (UB strength; kg), (D) lower body strength (LB strength; kg), (E) upper body power (UB power; w), (F) lower body power (LB power; w), (G) upper body endurance (UB endurance; R), (H) lower body endurance (LB endurance; R). n = 17 per group, error bars represent 95% confidence interval (CI), p-values above pre to post indicate paired sample t-test results, and p-values above groups indicate between-group differences.
Changes in muscular performance throughout the intervention are shown in Figure 3. Chest press [RHP = 8.2 kg (95% CI = 6.1 to 10.3, p < 0.001) and RLP = 4.1 kg (95% CI = 2.7 to 5.5, p < 0.001), Figure 3C], leg press strength [RHP = 11.5 kg (95% CI = 9.1 to 13.9, p < 0.001) and RLP = 6.1 kg (95% CI = 4.4 to 7.7, p < 0.001), Figure 3D] all significantly increased from baseline to post-intervention in both groups. Upper body [RHP = 23.6 w (95% CI = 14.8 to 32.4, p < 0.001) and RLP = 19.9 w (95% CI = 8.7 to 31.1, p = 0.002), Figure 3E] and lower body power [RHP = 19.6 w (95% CI = 9.2 to 30, p = 0.001) and RLP = 17.2 w (95% CI = 12.2 to 22.3, p < 0.001), Figure 3F] significantly increased from baseline to post-intervention in both groups. However, upper and lower body endurance remained unchanged (Figures 3G,H, respectively) over time (p > 0.05). ANCOVA results showed that the gains in the upper (p = 0.005) and lower body strength (p < 0.001) were significantly greater in RHP over time. However, there were no between-group changes in upper and lower body power (p > 0.05).
To investigate any potential relationships between training-induced changes in SMM (Δ SMM) and changes in muscular performance (Δ performance variable, independently of RHP or RLP group), a correlation matrix was generated (Figure 4A). Lower body strength (Figure 4C), upper body power (Figure 4D), and lower body endurance (Figure 4G) showed moderate positive relationships with Δ SMM, while upper body strength (Figure 4B) and upper body endurance (Figure 4F) showed a weak positive relationship. However, lower body power (Figure 4E) showed a weak negative relationship. For linear regression of individual Δ (performance variable) as a function of Δ SMM, data were examined by the extra sum-of-squares F test to first consider if pooled data could be considered as a single model. All data except for Δ upper and lower body strength were considered a single group. All data showed a non-significant relationship with changes in SMM.
Figure 4. (A) Correlation matrix of Δ SMM and performance variables, r values as shown. The key indicates the magnitude of r (grey = −1 or 1, white = 0). (B–G) linear regression (Pearson’s) of Δ (performance) as a function of Δ SMM (kg). Linear regression is indicated by a solid red line, 95% confidence intervals are indicated by cloud and grey zones.
Changes in biochemical markers throughout the intervention are shown in Figure 5.
Figure 5. Effects of resistance training in combination with high and low protein diet on biochemical markers. (A) gamma-glutamyl transferase (GGT [(u/L)]), (B) Aspartate transaminase (AST [(u/L)]), (C) Alanine transaminase (ALT [(u/L)]), (D) Creatinine (mg/dL), (E) Urea (mg/dL), (F) low-density lipoprotein (LDL [mg/dL]), (G) Cholesterol (mg/dL), (H) High-density lipoprotein (HDL [mg/dL]). n = 17 per group, error bars represent 95% confidence interval (CI), p-values above pre to post indicate paired sample t-test results, and p-values above groups indicate between-group differences.
GGT [RHP = 1.8 (u/L) (95% CI = 1.1 to 2.5, p < 0.001), Figure 5A], AST [RHP = 1.7 (u/L) (95% CI = 0.4 to 2.9, p = 0.012), Figure 5B], and ALT [RHP = 3.6 (u/L) (95% CI = 1.3 to 5.9, p = 0.004), Figure 5C] significantly increased from baseline to post-intervention in RHP group. ANCOVA results indicated that there was only a significant between-group difference for ALT being greater in RHP compared to RLP (p < 0.001).
Creatinine [RHP = 0.05 mg/dL (95% CI = 0.01 to 0.08, p = 0.009), Figure 5D] and urea [RHP = 1.2 mg/dL (95% CI = 0.2 to 2.2, p = 0.016), Figure 5E] significantly increased from baseline to post-intervention in RHP group. ANCOVA results indicated that the increase in creatinine in RHP was significantly greater than in RLP (p = 0.002).
LDL [RHP = −5.5 mg/dL (95% CI = −8.7 to −2.4, p = 0.002) and RLP = −4.5 mg/dL (95% CI = −5.9 to −3, p < 0.001), Figure 5F] and cholesterol [RHP = −4.7 mg/dL (95% CI = −8.5 to −0.8, p = 0.021) and RLP = −6.1 mg/dL (95% CI = −10.7 to −1.6, p = 0.011), Figure 5G] significantly decreased from baseline to post-intervention in both groups. However, HDL remained unchanged (Figure 5H) over time (p > 0.05). ANCOVA indicated no significant between-group difference for any marker (p > 0.05).
No adverse events were reported from both groups. Average dietary intakes at baseline and throughout the intervention are presented in Table 3. There was no significant difference between groups at baseline for any average daily nutrient and energy intake (p > 0.05). Protein intake [RHP = 0.8 g/kg/d (95% CI = 1 to 0.6, p < 0.001)] significantly increased in RHP from pre-to post-intervention. However, no changes in carbohydrates, fat, and energy intakes were observed (p > 0.05).
Table 3. Average relative dietary intake at baseline and throughout the 8-week training intervention.
Changes in training volume throughout the intervention are shown in Figure 6. There was a significant main effect of time for training volume (p < 0.001). Training volume [RHP = 34 kg.kg BM−1 (95% CI = 38.8 to 29.2, p < 0.001) and RLP = −29.9 kg.kg BM−1 (95% CI = 35.6 to 24.1, p < 0.001), Figure 6] significantly increased from pre to post in both groups. However, no significant interaction or group effect was noted (p > 0.05).
Figure 6. Effects of resistance training in combination with high and low protein diet on relative estimated training volume. n = 17 per group, and error bars represent 95% confidence interval (CI).
The objective of the present study was to compare the effects of 8 weeks of RT in the combination of 1.6 g/kg/d of protein vs. 0.8 g/kg/d on body composition, muscular performance (strength, power, and endurance), and biochemical markers of liver and kidney function in a cohort of healthy, untrained older ex-military males. Our results demonstrate greater gains in SMM and muscular strength (both upper and lower body) with a daily protein intake of 1.6 g/kg/d (RHP group) compared to participants ingesting 0.8 g/kg/d (RLP group) in ex-military older adults concomitant with 8-weeks of RT. These findings are of clinical importance since such improvements in SMM and muscular strength are important to reducing the adverse effects of sarcopenia on musculoskeletal function and health.
Beginning at 50 years of age, the steady reduction in strength and SMM becomes noticeable (47), with SMM and strength/power declining at rates of 0.8–1% and 2–3% each year, respectively (2). Maintenance of skeletal muscle strength is an important factor to preserve functional capacity and independent living with advancing age (47). Nevertheless, even in very physically active older adults (e.g., training four to five or more sessions per week), there have been noted deteriorations in leg strength of 3–5% per annum (48). The greater increase in SMM and strength from a high-protein diet in our current work supports previous findings in older males. For instance, 12 weeks of supervised (3 sessions/wk) RT in combination with a high protein diet (1.8 g/kg/d) in older (~63 years) males (n = 6) and females (n = 3) already consuming 1.2 g/kg/d resulted in a 0.6% increase in fat-free mass (FFM), absolute and relative (53–78%) maximal 1-RM strength (leg press, chest press and lat pulldown) compared to baseline. However, the gains in fat-free mass and absolute 1-RM strength were not statistically significant compared to the control group (1.4 g/kg/d). In regards to power, no change was noted in the countermovement jump. Overall, these results suggest that the intake of a high-protein dairy milk beverage, in combination with RT, elicits greater effects on skeletal muscle strength, but not muscle hypertrophy or power outcome, than consuming the dairy milk beverage or RT in isolation (49). When compared to the results noted in the present study, it seems that the main influencer of the lack of difference in fat-free mass between a high protein diet in combination with RT in comparison to RT in isolation and control is the dosage of protein ingestion per day, which was not statistically different. However, in the present study, the dose of protein in the high-protein diet group (1.6 g/kg/d) was two times higher than the low-protein diet (0.8 g/kg/d). Previous studies have shown that older adults’ maximum 1-RM leg strength improves by >25% following 12 weeks of RT (50, 51) which is consistent with our findings. In line with our results, a recent meta-analysis demonstrated that protein supplementation (20 ± 18 g/d) increases leg press 1-RM (33%) in older adults (mean age: 62 ± 6 y) (52), which challenges earlier studies with exercise interventions that have not witnessed protein supplementation to further gains in maximal leg press 1-RM during RT in healthy community-dwelling and active older adults in comparison to placebo or training in isolation group (50, 51). The difference in the average age between the participants in the present investigation (61 ± 2 years) and those in the prior literature (≥70 years) is one probable explanation for the favorable outcomes in the present. Second, the quantity of daily protein consumed by the supplementation groups may not have been adequate to produce a meaningful difference in strength across groups.
For instance, cohorts receiving additional milk servings were ingesting 1.3–1.4 g/kg/d of protein at pre-intervention (50, 51). Even though this is greater than the recommendations for older adults (≥1.2 g/kg/d) to combat sarcopenia, it is lower (1.6 g/kg/d) than the threshold suggested to support significant changes in muscle hypertrophy and strength during prolonged RT in healthy active adults (7, 53–55). Muscle hypertrophy and strength adaptations may necessitate a higher protein intake (e.g., ≥ 1.6 g/kg/d) than what is presently suggested for active older individuals (≥1.2 g/kg/d) in light of the findings of this study. Due to the importance of dietary distribution during the day in older adults (7), particularly as research suggests that the distribution of protein is often inadequate at breakfast, lunch, and post-exercise in older adults (56), we instructed our participants to consume 0.4 g/kg at each meal to maximally stimulate muscle protein synthesis (7). This may be another important factor in increases of SMM and strength.
In contrast to changes in SMM and strength, no between-group differences were observed in the magnitude of increases in muscular power (both upper and lower body), which is in agreement with a recent study in which countermovement jump was measured as muscular power (49). Also, in the present study, there was no relationship between SMM and muscular strength with power outcomes. These results indicate that the gains in muscular power are independent of protein ingestion or SMM values and may be more dependent on exercise training mode. In support, previous work suggests high-velocity/power RT, defined by completing the concentric phase of each movement as quickly as feasible while the eccentric phase is executed slowly and under control (57), significantly moderated the influences of RT on muscle power compared to conventional RT, indicating that high-velocity RT is superior to traditional RT for muscular power gains in older adults (58, 59). As muscular power is the product of contraction force and movement velocity, it is conceivable, biomechanically, that fast concentric contractions would improve muscle power more than traditional RT. Since we used traditional RT in the present study, the lack of difference between groups is perhaps unsurprising. Thus, while a high-protein diet may not be optimal for maximizing muscle power adaptations in older individuals, performing high-velocity/power RT in such cohorts should be considered with caution as such movements may increase the likelihood of losing balance or muscle tears due to the rapid nature of muscle contraction in such movements.
High protein diets have been linked to possible negative effects on renal function, namely glomerular filtration rate (22–24). Specifically, it has been proposed that high and persistent consumption of dietary protein may eventually lead to glomerular damage, renal impairment, and kidney failure (21). Although such associations may be more probable in those with impaired renal function (such as chronic kidney disease) (60), the relationship between increased dietary protein availability and impaired kidney and liver function seems to be significantly less pronounced in individuals with healthy kidney function (61). Considering the protein component of our high dietary protein intervention group, which was two-fold higher than the most current national recommended daily allowance for daily protein intake, we evaluated several biochemical markers of lipid, kidney, and liver function. Our results indicated within-group increases in GGT, AST, ALT, creatinine, and urea while only ALT and creatinine values were greater in RHP compared to the RLP group. Compared to referenced ‘normal’ ranges, post-intervention levels of GGT, ALT, AST, urea and creatinine were all within normal reference ranges in the RHP group (62–65). It cannot be discounted that increased levels of these markers outside ‘normal’ healthy ranges may occur over longer periods (i.e., months to years) with a continued high protein diet and possible deterioration in kidney function with advancing age. Nonetheless, our data show that older adults with healthy kidney function can consume a high protein diet (i.e., 1.6 g/kg/d) over an 8-week period without an adverse effect on liver or kidney function. Finally, both groups similarly decreased LDL and cholesterol with no changes in HDL, indicating the role of RT independent of protein ingestion on blood lipid health.
There were several limitations of our present work. Firstly, bioelectrical impedance was used to assess body composition which is not as accurate as dual-energy x-ray absorptiometry (a more suitable method for body composition measurements) although is still a reliable method (66, 67). Additionally, we lacked control over age-matched individuals that were not previously in the military. Regarding strengths of our study design, we investigated a cohort of participants that are under-represented in the skeletal muscle and nutrition research field. Moreover, we used nutrition software to track macronutrient intake which is rarely used in the literature, in older adults.
In conclusion, a daily intake of 1.6 g/kg/d protein was superior to 0.8 g/kg/d (current recommended daily intake) for promoting greater improvements in SMM and muscle strength and thus may be a more suitable level of intake for promoting such adaptive responses. Notwithstanding observed between-group differences in ALT and creatinine and the fact that levels remained within normal ranges, it is feasible to conclude that this daily protein intake is efficacious and well tolerated by healthy, untrained older ex-military males. Such knowledge is of critical importance for older populations since gains in SMM and strength can reduce the many deleterious effects of sarcopenia, reduce the risk of falls, and improve the quality of independent living (7, 68). Therefore, older adults could use the intake of 1.6 g/kg/d of protein to improve muscular (e.g., muscle strength) and body composition (e.g., SMM).
The raw data supporting the conclusions of this article will be made available by the authors, without undue reservation.
The studies involving human participants were reviewed and approved by the Baqiyatallah University of Medical Sciences. The patients/participants provided their written informed consent to participate in this study.
RB and AS: conceptualization and project administration. RB: methodology, writing—original draft preparation, formal analysis, and investigation. RB and EN: software. RB and VS: validation. AS: resources, supervision, and funding acquisition. RB, HG, and HF: data curation. DC, RB, and FD: writing—review and editing. All authors have read and agreed to the published version of the manuscript.
This research was supported by the Baqiyatallah University of Medical Sciences, Tehran, Iran.
We would like to express our gratitude to Amir Roohbakhsh for his support with body composition assessment.
The authors declare that the research was conducted in the absence of any commercial or financial relationships that could be construed as a potential conflict of interest.
All claims expressed in this article are solely those of the authors and do not necessarily represent those of their affiliated organizations, or those of the publisher, the editors and the reviewers. Any product that may be evaluated in this article, or claim that may be made by its manufacturer, is not guaranteed or endorsed by the publisher.
1. ^Available at https://www.randomizer.org/.
1. Rodrigues, F , Domingos, C , Monteiro, D , and Morouço, P . A review on aging, sarcopenia, falls, and resistance training in community-dwelling older adults. Int J Environ Res Public Health. (2022) 19:874. doi: 10.3390/ijerph19020874
2. Goodpaster, BH , Park, SW , Harris, TB , Kritchevsky, SB , Nevitt, M , Schwartz, AV, et al. The loss of skeletal muscle strength, mass, and quality in older adults: the health, aging and body composition study. J Gerontol Ser A Biol Med Sci. (2006) 61:1059–64. doi: 10.1093/gerona/61.10.1059
3. Kim, H , Hirano, H , Edahiro, A , Ohara, Y , Watanabe, Y , Kojima, N, et al. Sarcopenia: prevalence and associated factors based on different suggested definitions in community-dwelling older adults. Geriatr Gerontol Int. (2016) 16:110–22. doi: 10.1111/ggi.12723
4. Shafiee, G , Keshtkar, A , Soltani, A , Ahadi, Z , Larijani, B , and Heshmat, R . Prevalence of sarcopenia in the world: a systematic review and meta-analysis of general population studies. J Diabetes Metab Disord. (2017) 16:1–10. doi: 10.1186/s40200-017-0302-x
5. Bagheri, R , Robinson, I , Moradi, S , Purcell, J , Schwab, E , Silva, T, et al. Muscle protein synthesis responses following aerobic-based exercise or high-intensity interval training with or without protein ingestion: a systematic review. Sports Med. (2022) 52:2713–32. doi: 10.1007/s40279-022-01707-x
6. Barclay, RD , Burd, NA , Tyler, C , Tillin, NA , and Mackenzie, RW . The role of the IGF-1 signaling cascade in muscle protein synthesis and anabolic resistance in aging skeletal muscle. Front Nutr. (2019) 6:146. doi: 10.3389/fnut.2019.00146
7. Mckendry, J , Currier, BS , Lim, C , Mcleod, JC , Thomas, AC , and Phillips, SM . Nutritional supplements to support resistance exercise in countering the sarcopenia of aging. Nutrients. (2020) 12:2057. doi: 10.3390/nu12072057
8. Bagheri, R , Moghadam, BH , Candow, DG , Elliott, BT , Wong, A , Ashtary-Larky, D, et al. Effects of Icelandic yogurt consumption and resistance training in healthy untrained older males. Br J Nutr. (2022) 127:1334–42. doi: 10.1017/S0007114521002166
9. Bagheri, R , Rashidlamir, A , Motevalli, MS , Elliott, BT , Mehrabani, J , and Wong, A . Effects of upper-body, lower-body, or combined resistance training on the ratio of follistatin and myostatin in middle-aged men. Eur J Appl Physiol. (2019) 119:1921–31. doi: 10.1007/s00421-019-04180-z
10. Churchward-Venne, TA , Tieland, M , Verdijk, LB , Leenders, M , Dirks, ML , De Groot, LC, et al. There are no nonresponders to resistance-type exercise training in older men and women. J Am Med Dir Assoc. (2015) 16:400–11. doi: 10.1016/j.jamda.2015.01.071
11. Kirk, B , Mooney, K , Amirabdollahian, F , and Khaiyat, O . Exercise and dietary-protein as a countermeasure to skeletal muscle weakness: Liverpool Hope University – sarcopenia aging trial (LHU-SAT). Front Physiol. (2019) 10:445. doi: 10.3389/fphys.2019.00445
12. Phillips, SM , Tipton, KD , Aarsland, A , Wolf, SE , and Wolfe, RR . Mixed muscle protein synthesis and breakdown after resistance exercise in humans. Am J Physiol. (1997) 273:E99–E107. doi: 10.1152/ajpendo.1997.273.1.E99
13. Biolo, G , Tipton, KD , Klein, S , and Wolfe, RR . An abundant supply of amino acids enhances the metabolic effect of exercise on muscle protein. Am J Physiol. (1997) 273:E122–9. doi: 10.1152/ajpendo.1997.273.1.E122
14. Wolfe, RR , Cifelli, AM , Kostas, G , and Kim, I-Y . Optimizing protein intake in adults: interpretation and application of the recommended dietary allowance compared with the acceptable macronutrient distribution range. Adv Nutr. (2017) 8:266–75. doi: 10.3945/an.116.013821
15. Bauer, J , Biolo, G , Cederholm, T , Cesari, M , Cruz-Jentoft, AJ , Morley, JE, et al. Evidence-based recommendations for optimal dietary protein intake in older people: a position paper from the PROT-AGE study group. J Am Med Dir Assoc. (2013) 14:542–59. doi: 10.1016/j.jamda.2013.05.021
16. Deutz, NE , Bauer, JM , Barazzoni, R , Biolo, G , Boirie, Y , Bosy-Westphal, A, et al. Protein intake and exercise for optimal muscle function with aging: recommendations from the ESPEN expert group. Clin Nutr. (2014) 33:929–36. doi: 10.1016/j.clnu.2014.04.007
17. Otsuka, R , Kato, Y , Tange, C , Nishita, Y , Tomida, M , Imai, T, et al. Protein intake per day and at each daily meal and skeletal muscle mass declines among older community dwellers in Japan. Public Health Nutr. (2020) 23:1090–7. doi: 10.1017/S1368980019002921
18. Cardon-Thomas, DK , Riviere, T , Tieges, Z , and Greig, CA . Dietary protein in older adults: adequate daily intake but potential for improved distribution. Nutrients. (2017) 9:184. doi: 10.3390/nu9030184
19. Mcgrath, R , Stastny, S , Casperson, S , Jahns, L , Roemmich, J , and Hackney, KJ . Daily protein intake and distribution of daily protein consumed decreases odds for functional disability in older Americans. J Aging Health. (2020) 32:1075–83. doi: 10.1177/0898264319881864
20. Mclean, RR , Mangano, KM , Hannan, MT , Kiel, DP , and Sahni, S . Dietary protein intake is protective against loss of grip strength among older adults in the Framingham offspring cohort. J Gerontol A Biol Sci Med Sci. (2016) 71:356–61. doi: 10.1093/gerona/glv184
21. Brenner, BM , Meyer, TW , and Hostetter, TH . Dietary protein intake and the progressive nature of kidney disease: the role of hemodynamically mediated glomerular injury in the pathogenesis of progressive glomerular sclerosis in aging, renal ablation, and intrinsic renal disease. N Engl J Med. (1982) 307:652–9. doi: 10.1056/NEJM198209093071104
22. Kalantar-Zadeh, K , Kramer, HM , and Fouque, D . High-protein diet is bad for kidney health: unleashing the taboo. Walton Street, Oxford: Oxford University Press (2020).
23. Ko, G-J , Rhee, CM , Kalantar-Zadeh, K , and Joshi, S . The effects of high-protein diets on kidney health and longevity. J Am Soc Nephrol. (2020) 31:1667–79. doi: 10.1681/ASN.2020010028
24. Schwingshackl, L , and Hoffmann, G . Comparison of high vs. normal/low protein diets on renal function in subjects without chronic kidney disease: a systematic review and meta-analysis. PLoS One. (2014) 9:e97656. doi: 10.1371/journal.pone.0097656
25. Cirillo, M , Zingone, F , Lombardi, C , Cavallo, P , Zanchetti, A , and Bilancio, G . Population-based dose–response curve of glomerular filtration rate to dietary protein intake. Nephrol Dial Transplant. (2015) 30:1156–62. doi: 10.1093/ndt/gfv026
26. Shams-White, MM , Chung, M , Du, M , Fu, Z , Insogna, KL , Karlsen, MC, et al. Dietary protein and bone health: a systematic review and meta-analysis from the National Osteoporosis Foundation. Am J Clin Nutr. (2017) 105:1528–43. doi: 10.3945/ajcn.116.145110
27. Traylor, DA , Gorissen, SH , and Phillips, SM . Perspective: protein requirements and optimal intakes in aging: are we ready to recommend more than the recommended daily allowance? Adv Nutr. (2018) 9:171–82. doi: 10.1093/advances/nmy003
28. Van Elswyk, ME , Weatherford, CA , and Mcneill, SH . A systematic review of renal health in healthy individuals associated with protein intake above the US recommended daily allowance in randomized controlled trials and observational studies. Adv Nutr. (2018) 9:404–18. doi: 10.1093/advances/nmy026
29. Wallace, TC , and Frankenfeld, CL . Dietary protein intake above the current RDA and bone health: a systematic review and meta-analysis. J Am Coll Nutr. (2017) 36:481–96. doi: 10.1080/07315724.2017.1322924
30. Chapman, S , Rawcliffe, AJ , Izard, R , Jacka, K , Tyson, H , Smith, L, et al. Dietary intake and nitrogen balance in British Army infantry recruits undergoing basic training. Nutrients. (2020) 12:2125. doi: 10.3390/nu12072125
31. Hou, L , Lei, Y , Li, X , Huo, C , Jia, X , Yang, J, et al. Effect of protein supplementation combined with resistance training on muscle mass, strength and function in the elderly: a systematic review and meta-analysis. J Nutr Health Aging. (2019) 23:451–8. doi: 10.1007/s12603-019-1181-2
32. Mertz, KH , Reitelseder, S , Bechshoeft, R , Bulow, J , Højfeldt, G , Jensen, M, et al. The effect of daily protein supplementation, with or without resistance training for 1 year, on muscle size, strength, and function in healthy older adults: a randomized controlled trial. Am J Clin Nutr. (2021) 113:790–800. doi: 10.1093/ajcn/nqaa372
33. Schoenfeld, BJ , Nickerson, BS , Wilborn, CD , Urbina, SL , Hayward, SB , Krieger, J, et al. Comparison of multifrequency bioelectrical impedance vs. dual-energy X-ray absorptiometry for assessing body composition changes after participation in a 10-week resistance training program. J Strength Cond Res. (2020) 34:678–88. doi: 10.1519/JSC.0000000000002708
34. Murach, KA , and Bagley, JR . Skeletal muscle hypertrophy with concurrent exercise training: contrary evidence for an interference effect. Sports Med. (2016) 46:1029–39. doi: 10.1007/s40279-016-0496-y
35. Colantonio, E , Barros, RV , and Kiss, MAPDM . Oxygen uptake during Wingate tests for arms and legs in swimmers and water polo players. Rev Bras Med Esporte. (2003) 9:141–4. doi: 10.1590/S1517-86922003000300003
36. Shamim, B , Devlin, BL , Timmins, RG , Tofari, P , Dow, CL , Coffey, VG, et al. Adaptations to concurrent training in combination with high protein availability: a comparative trial in healthy, recreationally active men. Sports Med. (2018) 48:2869–83. doi: 10.1007/s40279-018-0999-9
37. Wilborn, CD , Taylor, LW , Outlaw, J , Williams, L , Campbell, B , Foster, CA, et al. The effects of pre-and post-exercise whey vs. casein protein consumption on body composition and performance measures in collegiate female athletes. J Sports Sci Med. (2013) 12:74–9.
38. Church, DD , Hoffman, JR , Mangine, GT , Jajtner, AR , Townsend, JR , Beyer, KS, et al. Comparison of high-intensity vs. high-volume resistance training on the BDNF response to exercise. J Appl Physiol. (2016) 121:123–8. doi: 10.1152/japplphysiol.00233.2016
39. Bagheri, R , Moghadam, BH , Church, DD , Tinsley, GM , Eskandari, M , Moghadam, BH, et al. The effects of concurrent training order on body composition and serum concentrations of follistatin, myostatin and GDF11 in sarcopenic elderly men. Exp Gerontol. (2020) 133:110869. doi: 10.1016/j.exger.2020.110869
40. Cadore, E , Pinto, R , Lhullier, F , Correa, C , Alberton, C , Pinto, S, et al. Physiological effects of concurrent training in elderly men. Int J Sports Med. (2010) 31:689–97. doi: 10.1055/s-0030-1261895
41. Maltais, ML , Ladouceur, JP , and Dionne, IJ . The effect of resistance training and different sources of postexercise protein supplementation on muscle mass and physical capacity in sarcopenic elderly men. J Strength Cond Res. (2016) 30:1680–7. doi: 10.1519/JSC.0000000000001255
42. Zdzieblik, D , Oesser, S , Baumstark, MW , Gollhofer, A , and König, D . Collagen peptide supplementation in combination with resistance training improves body composition and increases muscle strength in elderly sarcopenic men: a randomised controlled trial. Br J Nutr. (2015) 114:1237–45. doi: 10.1017/S0007114515002810
43. Cunha, PM , Nunes, JP , Tomeleri, CM , Nascimento, MA , Schoenfeld, BJ , Antunes, M, et al. Resistance training performed with single and multiple sets induces similar improvements in muscular strength, muscle mass, muscle quality, and IGF-1 in older women: a randomized controlled trial. J Strength Cond Res. (2020) 34:1008–16. doi: 10.1519/JSC.0000000000002847
44. Hamilton, DL , and Philp, A . Can AMPK mediated suppression of mTORC1 explain the concurrent training effect? Cell Mol Exerc Physiol. (2013) 2:e4. doi: 10.7457/cmep.v2i1.e4
45. Perez-Schindler, J , Hamilton, DL , Moore, DR , Baar, K , and Philp, A . Nutritional strategies to support concurrent training. Eur J Sport Sci. (2015) 15:41–52. doi: 10.1080/17461391.2014.950345
46. Ratner, B . The correlation coefficient: its values range between+ 1/− 1, or do they? J Target Meas Anal Mark. (2009) 17:139–42. doi: 10.1057/jt.2009.5
47. Cruz-Jentoft, AJ , Bahat, G , Bauer, J , Boirie, Y , Bruyère, O , Cederholm, T, et al. Sarcopenia: revised European consensus on definition and diagnosis. Age Ageing. (2019) 48:16–31. doi: 10.1093/ageing/afy169
48. Marcell, TJ , Hawkins, SA , and Wiswell, RA . Leg strength declines with advancing age despite habitual endurance exercise in active older adults. J Strength Cond Res. (2014) 28:504–13. doi: 10.1519/JSC.0b013e3182a952cc
49. Huschtscha, Z , Parr, A , Porter, J , and Costa, RJ . The effects of a high-protein dairy milk beverage with or without progressive resistance training on fat-free mass, skeletal muscle strength and power, and functional performance in healthy active older adults: a 12-week randomized controlled trial. Front Nutr. (2021) 8:644865. doi: 10.3389/fnut.2021.644865
50. Holwerda, AM , Overkamp, M , Paulussen, KJ , Smeets, JS , Van Kranenburg, J , Backx, EM, et al. Protein supplementation after exercise and before sleep does not further augment muscle mass and strength gains during resistance exercise training in active older men. J Nutr. (2018) 148:1723–32. doi: 10.1093/jn/nxy169
51. Verdijk, LB , Jonkers, RA , Gleeson, BG , Beelen, M , Meijer, K , Savelberg, HH, et al. Protein supplementation before and after exercise does not further augment skeletal muscle hypertrophy after resistance training in elderly men. Am J Clin Nutr. (2009) 89:608–16. doi: 10.3945/ajcn.2008.26626
52. Cermak, NM , Res, PT , De Groot, LC , Saris, WH , and Van Loon, LJ . Protein supplementation augments the adaptive response of skeletal muscle to resistance-type exercise training: a meta-analysis. Am J Clin Nutr. (2012) 96:1454–64. doi: 10.3945/ajcn.112.037556
53. Morton, RW , Murphy, KT , Mckellar, SR , Schoenfeld, BJ , Henselmans, M , Helms, E, et al. A systematic review, meta-analysis and meta-regression of the effect of protein supplementation on resistance training-induced gains in muscle mass and strength in healthy adults. Br J Sports Med. (2018) 52:376–84. doi: 10.1136/bjsports-2017-097608
54. Phillips, SM , and Van Loon, LJ . Dietary protein for athletes: from requirements to optimum adaptation. Food Nutr Sport Perform. (2013) III:37–46. doi: 10.1080/02640414.2011.619204
55. Zempo, H , Isobe, M , and Naito, H . Link between blood flow and muscle protein metabolism in elderly adults. J Phys Fitness Sport Med. (2017) 6:25–31. doi: 10.7600/jpfsm.6.25
56. Paddon-Jones, D , and Rasmussen, BB . Dietary protein recommendations and the prevention of sarcopenia: protein, amino acid metabolism and therapy. Curr Opin Clin Nutr Metab Care. (2009) 12:86–90. doi: 10.1097/MCO.0b013e32831cef8b
57. Hazell, T , Kenno, K , and Jakobi, J . Functional benefit of power training for older adults. J Aging Phys Act. (2007) 15:349–59. doi: 10.1123/japa.15.3.349
58. Steib, S , Schoene, D , and Pfeifer, K . Dose-response relationship of resistance training in older adults: a meta-analysis. Med Sci Sports Exerc. (2010) 42:902–14. doi: 10.1249/MSS.0b013e3181c34465
59. Straight, CR , Lindheimer, JB , Brady, AO , Dishman, RK , and Evans, EM . Effects of resistance training on lower-extremity muscle power in middle-aged and older adults: a systematic review and meta-analysis of randomized controlled trials. Sports Med. (2016) 46:353–64. doi: 10.1007/s40279-015-0418-4
60. Fouque, D , Laville, M , and Boissel, JP . Low protein diets for chronic kidney disease in non diabetic adults. Cochrane Database Syst Rev. (2006). doi: 10.1002/14651858.CD001892.pub2
61. Devries, MC , Sithamparapillai, A , Brimble, KS , Banfield, L , Morton, RW , and Phillips, SM . Changes in kidney function do not differ between healthy adults consuming higher-compared with lower-or normal-protein diets: a systematic review and meta-analysis. J Nutr. (2018) 148:1760–75. doi: 10.1093/jn/nxy197
62. Nicoll, D , and Detmer, W . Current medical diagnosis & treatment. In: Basic principles of diagnostic test use and interpretation. 36th ed. McGraw-Hill. Stamford, CT: Appleton & Lange (1997). 1–16.
63. Shivaraj, G , Prakash, D , Vinayak, H , Avinash, M , Sonal, V , and Shruthi, K . A review on laboratory liver function tests. Pan Afr Med J. (2009) 3
64. Hosten, AO . BUN and creatinine. Clinical methods: the history, physical, and laboratory examinations. 3rd ed. Boston, Butterworth (1990).
65. Shahbaz, H. , and Gupta, M. (2019). Creatinine clearance. Treasure Island (FL): StatPearls Publishing.
66. Jackson, A , Pollock, ML , Graves, JE , and Mahar, M . Reliability and validity of bioelectrical impedance in determining body composition. J Appl Physiol. (1988) 64:529–34. doi: 10.1152/jappl.1988.64.2.529
67. Ling, CH , De Craen, AJ , Slagboom, PE , Gunn, DA , Stokkel, MP , Westendorp, RG, et al. Accuracy of direct segmental multi-frequency bioimpedance analysis in the assessment of total body and segmental body composition in middle-aged adult population. Clin Nutr. (2011) 30:610–5. doi: 10.1016/j.clnu.2011.04.001
68. Talar, K , Hernández-Belmonte, A , Vetrovsky, T , Steffl, M , Kałamacka, E , and Courel-Ibáñez, J . Benefits of resistance training in early and late stages of frailty and sarcopenia: a systematic review and Meta-analysis of randomized controlled studies. J Clin Med. (2021) 10:1630. doi: 10.3390/jcm10081630
Keywords: resistance exercise, skeletal muscle adaptation, nutrition, aging, protein
Citation: Bagheri R, Shakibaee A, Camera DM, Sobhani V, Ghobadi H, Nazar E, Fakhari H and Dutheil F (2023) Effects of 8 weeks of resistance training in combination with a high protein diet on body composition, muscular performance, and markers of liver and kidney function in untrained older ex-military men. Front. Nutr. 10:1205310. doi: 10.3389/fnut.2023.1205310
Received: 13 April 2023; Accepted: 12 June 2023;
Published: 29 June 2023.
Edited by:
Diego A. Bonilla, Dynamical Business & Science Society - DBSS International SAS, ColombiaReviewed by:
Roberto Cannataro, University of Calabria, ItalyCopyright © 2023 Bagheri, Shakibaee, Camera, Sobhani, Ghobadi, Nazar, Fakhari and Dutheil. This is an open-access article distributed under the terms of the Creative Commons Attribution License (CC BY). The use, distribution or reproduction in other forums is permitted, provided the original author(s) and the copyright owner(s) are credited and that the original publication in this journal is cited, in accordance with accepted academic practice. No use, distribution or reproduction is permitted which does not comply with these terms.
*Correspondence: Abolfazl Shakibaee, c2hha2liYWVlYWJvbGZhemwyQGdtYWlsLmNvbQ==
Disclaimer: All claims expressed in this article are solely those of the authors and do not necessarily represent those of their affiliated organizations, or those of the publisher, the editors and the reviewers. Any product that may be evaluated in this article or claim that may be made by its manufacturer is not guaranteed or endorsed by the publisher.
Research integrity at Frontiers
Learn more about the work of our research integrity team to safeguard the quality of each article we publish.