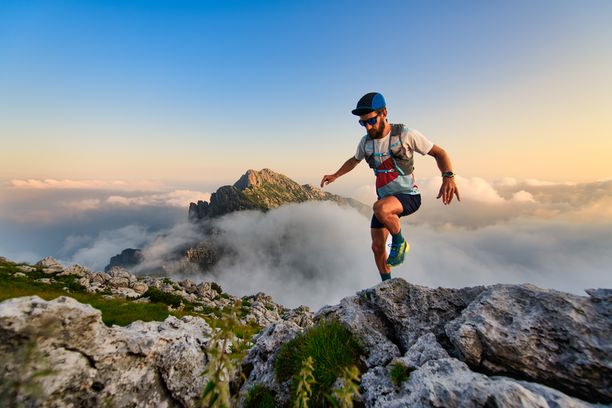
94% of researchers rate our articles as excellent or good
Learn more about the work of our research integrity team to safeguard the quality of each article we publish.
Find out more
ORIGINAL RESEARCH article
Front. Nutr., 24 August 2023
Sec. Food Chemistry
Volume 10 - 2023 | https://doi.org/10.3389/fnut.2023.1205200
This article is part of the Research TopicNanotechnology in NutraceuticalsView all 4 articles
In this study, the oil-in-water nanoemulsion (NE) was prepared and loaded with vitamin D3 in food-grade (edible) canola oil and stabilized by Tween 80 and Span 80 by using a water titration technique with droplet sizes of 20 to 200 nm. A phase diagram was established for the influence of water, oil, and S-Mix concentration. The outcomes revealed that the particle size of blank canola oil nanoemulsion (NE) ranged from 60.12 to 62.27 (d.nm) and vitamin D3 NE ranged from 93.92 to 185.5 (d.nm). Droplet size and polydispersity index (PDI) of both blank and vitamin D3-loaded NE results were less than 1, and zeta potential results for blank and vitamin D3 loaded NE ranged from −9.71 to −15.32 mV and −7.29 to −13.56 mV, respectively. Furthermore, the pH and electrical conductivity of blank NE were 6.0 to 6.2 and 20 to 100 (μs/cm), respectively, whereas vitamin D3-loaded NE results were 6.0 to 6.2 and 30 to 100 (μs/cm), respectively. The viscosity results of blank NE ranged from 0.544 to 0.789 (mPa.s), while that of vitamin D3-loaded NE ranged from 0.613 to 0.793 (mPa.s). In this study, the long-term stability (3 months) of canola oil NE containing vitamin D3 at room temperature (25 C) and high temperature (40 C) was observed.
Due to their effectiveness and efficacy, nanoemulsion has an eminent place among the many drug delivery strategies. During their formulation, lipophilic active compounds including nutraceuticals, drugs, and flavors are incorporated into the aqueous medium of pharmaceuticals and food products. The emulsion is defined as the merging of two immiscible liquids together in the presence of an emulsifying agent, in which one liquid is dispersed into another, referred to as the dispersed phase and the continuous phase (1, 2). Moreover, colloidal suspensions with very minute droplets, generally 20–200 nm in diameter, distributed in a liquid medium are known as nanoemulsions (NEs) (3). Due to the changes in physiochemical properties and biological performances related to the alteration in particle size, nanoemulsions are extensively utilized in the food industry. These nanodroplets in emulsion-based delivery systems are beneficial in the field of the food industry due to their higher optical clarity, increased oral bioavailability, and greater stability for droplet aggregation separation (4, 5). For the administration of probiotics, nutraceuticals, flavors, and colors, nanoemulsions hold interesting potential and have been shown to be one of the most effective methods for protecting sensitive compounds' characteristics (6, 7). These are kinetically stable and thermodynamically unstable; however, their physical stability without coalescence makes them distinctive conventional emulsions (8). The majority of nutrients are passed through the digestive process in an oil-in-water emulsion, which can be categorized as oil-in-water in which oil droplets are dragged in a continuous water phase or water-in-oil in which water droplets are submerged in a continuous oil phase (9). The size of all the particles may tend toward nano to mixed micelle array before the time of absorption. However, the majority of people are deficient in vitamin D, which may lead to rickets, osteomalacia, and bone disease. Due to its lower stability in the food chain, the beverage industry's fortification of food products with fat-soluble vitamins has proved problematic (10, 11). For oral consumption, emulsion-based delivery of fat-soluble vitamin D3 can be a suitable method (12). The previous studies demonstrated the formation of nano-phytosomes using lipid- and solvent-based derivative methods (13), micro/nanoemulsion formulations (14), encapsulation of vitamin D3, loaded with cyclodextrin (15), zein nanodroplets enveloped with carboxymethyl chitosan (12), phase inversion temperature method (16) and preparation, modeling characterization, and release profile of vitamin D3 nanoemulsion (17). Therefore, using nanotechnology to encapsulate vitamin D into nano-delivery systems and then using them for additional fortification is an efficient way to increase solubility, bioavailability, distribution, and protection against oxidation, UV light, and manufacturing conditions (16). Canola oil has health-supporting nutritional properties due to its good source of oleic acid (50–60% portion), α-linolenic acid (6–14% portion), and some unsaturated fatty acids (18). Moreover, canola oil exhibited strong anti-oxidant properties due to the presence of phenolic compounds, primarily sinapic acid and its derivatives (19). In many studies, these two surfactants (Tween 80 and Span 80) show high compatibility to build a stable interfacial film and give better performance during emulsification process studies (20–22). Canola oil, distilled water, the surfactant Tween 80, and the co-surfactant Span 80 were specifically generated in this study's canola oil-enhanced vitamin D3 oil-in-water nanoemulsion formulations utilizing the water titration technique. The physicochemical characteristics of nanoemulsions were examined by a pseudo-ternary phase diagram, electrical conductivity, pH, viscosity, droplet size distribution, thermal stability, and shelf life study.
Pure canola oil was purchased from the local market in Faisalabad (Pakistan). Span 80 and Tween 80 were purchased from Daejung. Distilled water was used in emulsions and solutions. Vitamin D3 (cholecalciferol) was kindly gifted by Textile Testing Laboratories in Lahore. All chemical reagents were used for analytical and food grades.
Canola oil, surfactant (Tween 80), and co-surfactant (Span 80) (S/Co-S) were used for nanoemulsion formulation. For the production of the pseudo-ternary phase diagram, the surfactant/co-surfactant (S/Co-s) ratio of 1:1 was adopted. The water titration technique was used to create a pseudo-ternary phase structure to locate the nanoemulsion zone and determine the concentration ratio of the constituent ingredients (23). During the experimental phase, in different falcon tubes, oil was thoroughly mixed with surfactant/co-surfactant (S/Co-s) by using a 2-min vortex mixer (PCSIR, Pakistan) at different weight ratios of 0.95:0.05, 0.9:0.1, 0.85:0.15, 0.8: 0.20, 0.75:0.25, 0.70:0.3, 0.65:0.35, 0.6:0.4, 0.55:0.45, 0.5:0.5, 0.4:0.6, 0.3:0.7, 0.2:0.8, 0.1:0.9, and 0.05:0.95 (w/w). The aqueous phase was then added with an increment of 50 μl into each tube (at 26 ± 2°C) followed by 2 min of mixing. After homogenization, samples were visually observed by enlightening the sample with white light to confirm the nature of the sample. The resultant mixture is described as transparent, turbid, gel-like, or milky in physical appearance. The milky emulsion was declared a nanoemulsion (24). Chemix (cxse 700) was utilized to construct the ternary diagram.
The pseudo-ternary phase diagram's appropriate weight proportions for S/Co-S and oil were chosen, and several equations were created based on the diagram's nanoemulsion (NE) area. Due to the much smaller oil-to-water weight ratio, nanoemulsions were anticipated to be made with these weight proportions. In these NE systems, the physical condition and milkiness were seen.
The nanoemulsions were prepared after considering the daily suggested intake of vitamin D (600 IUs). Vitamin D3 (Cholecalciferol) was added to the oil, and after 2 min of vigorous vortex mixing, a 1:1 combination of Tween 80 and Span 80 was added, and further mixing was performed continuously. Finally, to create a homogenous nanoemulsion combination, a specific weight of liquid phases was incorporated drop by drop (25).
Dynamic light scattering was used to measure the droplet size and polydispersity index of a vitamin D3 nanoemulsion at a temperature of 25°C (Malvern Zeta Sizer Ver. 7.11, Malvern Instruments, UK). The word “droplet size” refers to nm. The outcomes of each measurement were determined using the mean and standard error. After the proper dilution, test samples were placed into sample cells. To ensure that the nanoemulsions' aggregate diameter was uniform, polydispersity index values were applied (26).
By applying an electric field of one volt, the Malvern Zetasizer Nano ZS (Malvern Instruments, Ltd., UK) Ver. 7.11 with electrophoretic light scattering and a particulate size detector was used to evaluate the zeta potential of NE formulas. The samples were adequately diluted with pre-filtered double-distilled water for the zeta potential measurement, and measurements were made in triplicate (27).
pH and conductivities of formulated nanoemulsion were determined by using a portable pH/EC temperature meter (HANNA Instruments HI9811-5, Romania) as per the guidelines of (28).
Viscosity was determined by using a viscometer (BROOKFIELD Instruments, DV2TRVTJO, USA). To assure accuracy, measurements were performed three times, and average results were noted (29). Using a viscometer, a two-number spindle was coated with nanoemulsion and revolved at speeds of 5, 10, 20, and 50 rpm at 25°C (30) and a shear rate range of 3 and 218/s with some modifications. Readings on the viscometer were recorded for each speed. Mean values were computed when the samples were repeated in triplicate. The dispersion medium was purified water (20 ml), and the nanoemulsion (2 g) was weighed. For pH meter calibration, buffer solutions with pH values of 4, 7, and 9 were utilized. Mean values were calculated after the samples were repeated in triplicate (31).
Stability analyses of nanoemulsions containing vitamin D3 were evaluated. Each formulation of 5 ml was added into glass vials, sealed, and kept at room temperature (25 ± 0.5 C) and 40 C for 3 months. Then, samples were visually analyzed for milkiness, flocculation, phase separation, zeta potential, droplet size, PDI viscosity, pH, and conductivity. All studies were conducted in triplicate (32).
For data analysis, SPSS version 20 was utilized, and all findings were presented as mean and standard deviation (SD).
The isolation of the nanoemulsion zone devoid of vitamin D3 and the optimization of concentration for oil, surfactant, and co-surfactant were both designed using a pseudo-ternary phase diagram. For the ternary diagram's creation, a ratio of 1:1 was used. The colored region in Figure 1 denotes the nanoemulsion zone.
Figure 1. Pseudo-ternary phase diagram consisting of water, canola oil, and S/Co-s (Tween 80 and Span 80).
Even though several formulations could be chosen from the secured nanoemulsion region but only four formulae were selected to develop nanoemulsion, as shown in Table 1. It is documentary supported that the composition of a nanoemulsion may affect many characteristics of nanoemulsion; so, the oil surfactant and co-surfactant (S-Mix) in different concentrations were taken at 5% intervals.
All the formulations (blank and vitamin D3-loaded) were physically fairly stable and did not indicate precipitation, phase separation, and color change.
Nanoemulsion size decreased to < 200 nm (33) as a result of vitamin D3-loaded formulations showing that the droplet size was reduced by increasing the S-Mix ratio (34). The small particle size was found to prevent flocculation, facilitating the system to remain dispersed with no phase separation. These findings imply that monounsaturated-rich oils, such as maize oil, are superior to polyunsaturated-rich oils, such as flaxseed or fish oil, at encapsulation and delivering the D3 vitamin. The conclusions revealed here may help in the development of more effective plant-derived vitamin-fortified meals and drinks reported by Schoener et al. (35). Similar results were shown by Maurya and Aggarwal (36), and the chosen nanodroplet size, zeta potential, and the durability of vitamin D3 storage under various environmental stress conditions were also examined. (i) moisture and the temperature: among them are (a) the accelerated condition (45 ± 2°C and RH 75 ± 5%), (b) the ambient condition (25 ± 3°C and RH 65 ± 5%), and (c) the chilled condition (six two °C and RH 55 ± 5%); (ii) pH (3–7) in a chilled environment; and (iii) ionic strength: NaCl concentration (0, 250, 500, and 750 mM) in a chilled environment. The values of droplet size of blank nanoemulsion formulations were found to range from 60.12 to 66.48 nm, and nanoemulsions with vitamin D3-loaded ranged from 93.92 to 185.5 nm as shown in Table 2. The PDI results show that the droplets are distributed uniformly throughout the compositions. The PDI value for each formulation is < 1.0. The value of PDI of blank formulations was found to range from 0.36 to 0.50, and PDI values of vitamin D3-loaded formulations were found to range from 0.29 to 0.93. All values of the nanoemulsion formulations were observed to be < 1 in this investigation.
Hippalgaonkar et al. (37) stated that the zeta potential is the potential difference between the stationary layer and the dispersion medium of a fluid. Zeta potential is an electro-kinetic potential, and nanoemulsion is electrostatically stable as the zeta potential is below −30 mV and above +30 mV. The results of the zeta potential of blank and vitamin D3-loaded nanoemulsion are mentioned in Table 3. The values of zeta potential were found to be negative, ranging from −9.71 to −15.32 mV and −7.29 to −13.56 mV for blank and vitamin D3-loaded nanoemulsion, respectively. All the results of the zeta potential fall below ± 30 mV.
The results of the pH and conductivity of the nanoemulsion are presented in Table 4. The pH of blank formulations was observed to range from 6.0 to 6.2, and the pH of vitamin D3-loaded formulations was found to range from 6.1 to 6.2, with slight variation in all formulations, which is considered a good candidate for oral administration (38). CA-3 treatment showed the best pH value of 6.2 for the oral administration of nanoemulsion. To determine the type of nanoemulsion, an electrical conductivity test was applied. Conductivity measurements of oil-in-water nanoemulsion have higher electrical conductivity values (10–100 μs/cm) (39). The electrical conductivity value before loading vitamin D3 ranged from 20 to 80 μsec/cm, and the vitamin D3-loaded nanoemulsion had an electrical conductivity ranging between 30 and 100 μsec/cm. Electrical conductivity values were found to decrease with the decrease in water content in the emulsions.
Viscosity is another crucial consideration for the stability of nanoemulsions. The viscosity of nanoemulsion is a function of water, surfactant and co-surfactant concentration, and oil concentration. The results of viscosity are presented in Table 5. Viscosities of stable samples were found in the range of 0.54 mPa.s to 0.78 mPa.s of blank samples, and viscosities of vitamin D3-loaded nanoemulsions were observed to range from 0.61 mPa.s to 0.79 mPa.s. An increase in the viscosity of nanoemulsions might be due to an increase in oil content. The higher the oil content in the nanoemulsion, the higher will be the viscosity, and vice versa (40). As the oil content increased, it was discovered that CA-1 had a lower viscosity than CA-4 and that the viscosity of nanoemulsion was also rising. Because the blank sample had more oil than the vitamin D3-loaded formulation, the viscosities of the two were found to be different. By using dynamic light scattering (DLS) analysis, the nanoemulsions' particle size, polydispersity, and stability over time were examined. The polysorbate 20 and lecithin-based nanoemulsions that were investigated created systems with EVOO droplets that were stable for 37 days and measured 285 ± 5 nm in diameter and 0.202 ± 0.01 PdI. Calcium citrate and vitamin D3 were added to these durable nanoemulsions to create formulations that include both water- and oil-soluble micronutrients, as reported by Demisli et al. (41).
Based on visual observation, droplet size, zeta potential, pH, conductivity, and viscosity results, nanoemulsion formulation (CA-3) was selected for stability testing. There is no flocculation, and phase separations have appeared. All formulations were stable for more than 3 months as shown in Tables 9–11. The parameters including droplet size, polydispersity index, and zeta potential of formulation CA-3 (both blank and vitamin D3-loaded) were monitored at time zero; thereafter, 1, 2, and 3 months of storing at 25 ± 2 C/55% RH and 40 C/75% RH are shown in Tables 6–8. There is no difference in zeta potential, droplet size, or viscosity between ambient and accelerated storage conditions. Even after 3 months, there is no phase separation at room temperature or 40 C in nanoemulsion formulations. Morais et al. (42) conducted a related investigation as well. The purpose of this study was to assess the physicochemical properties of canola oil–water nanoemulsions, including zeta potential and droplet size values before and after stability testing. Owing to vitamin D's limited water solubility and degradation from exposure to the sun and high temperatures, fortifying foods and drinks with it are difficult, according to Mehmood and Ahmed (43). The results obtained imply that the nanoemulsion produced by the EPI and necessary HLB procedures is highly stable. The stated nanometer-sized droplets and strong zeta potential values can be supported by this shown in Tables 9–11. The tested parameters showed no discernible change, even though the emulsions were exposed to a variety of temperatures. Our study showed a slight decrease in viscosity with the elevation of temperature to 40 C, which was similar to the study described by Cheng et al. (44), whereas conductivity has not shown any changes during stability studies and PDI was also measured as < 1 (34). Similar results were reported by Zhang et al. (45). Vitamin D3 NEs showed greater stability under gastric digestion and prolonged release under gastrointestinal digestion as contrasted with unencapsulated (bare) vitamin D3. After 5 h of intestinal digestion, the total release of vitamin D3 reached 69%. Additionally, at 40–60°C, vitamin D3 NEs showed greater thermal stability than pure vitamin D3, and first-order kinetics was a good fit to characterize its thermal deterioration. Our research offers a fresh perspective on the use of vitamin D3 NEs stabilized by Span 80 and Tween 80 in the food sector. In systems stabilized by polysorbates, partial vitamin embedding that made the interface more fluid was also demonstrated. The vitamin-enriched nanoemulsions were then added to whole-fat milk, which remained resistant to particle development and gravitational dispersion for at least 10 days. Employing an EPR method, the antiradical capabilities of encapsulated cholecalciferol were examined and reported by Golfomitsou et al. (46).
Table 6. Stability data of droplet size at room temperature (RT) and 40°C for the nanoemulsion CA-3 formulation (both blank and vitamin D3-loaded). Mean ± SD, N = 3.
Table 7. Stability data of PDI at room temperature (RT) and 40°C for the nanoemulsion CA-3 formulation (both blank and vitamin D3-loaded).
Table 8. Stability data of zeta potential at room temperature (RT) and 40°C for the nanoemulsion CA-3 formulation (both blank and vitamin D3-loaded).
Table 9. Stability data of pH at room temperature (RT) and 40 C for the nanoemulsion CA-3 formulation (both blank and vitamin D3-loaded).
Table 10. Stability data of conductivity at room temperature (RT) and 40°C for the nanoemulsion CA-3 formulation (both blank and vitamin D3-loaded).
Table 11. Stability data of viscosity at room temperature (RT) and 40 C for the nanoemulsion CA-3 formulation (both blank and vitamin D3-loaded).
It was also observed that the primary size of nanoemulsion droplets depended on the type of S-Mix, S-Mix- level, and stirring conditions. It contains a droplet size of < 200 nm and could be developed by using a non-ionic surfactant and emulsifier (Tween 80 and Span 80) at a ratio of 1:1 with constant stirring during the titration process. By varying the composition of oil (5–20%), S-Mix (30–40%), and water (40–65%), 15 formulations were prepared. The outcomes delineated that the canola oil-based nanoemulsions proved efficient for the entrapment of vitamin D3, which can be used for the fortification of different food products to promote the delivery of this deficient micronutrient. Four nanoemulsion formulation combinations were finalized for the encapsulation of vitamin D3 through a phase diagram. Higher zeta potential, droplet size within an acceptable range, standard pH and conductivity, and stable viscosity were achieved in the CA-3 vitamin D3-encapsulated nanoemulsion. Thermal stability studies indicated that nanoemulsions stayed physically stable for 3 months at room temperature and also at an elevated temperature of 40 C. However, structural elucidation analysis such as FTIR should be performed in future studies to indicate bond formation.
The original contributions presented in the study are included in the article/supplementary material, further inquiries can be directed to the corresponding authors.
AK: prepared original article. MA: supervision equal. AI: methodology equal. SH: methodology. MS: conceptulization equal. All authors contributed to the article and approved the submitted version.
The authors are thankful to Government College University for providing literature collection facilities, and they are also thankful to Mohd Asif Shah for their help in publication.
The authors declare that the research was conducted in the absence of any commercial or financial relationships that could be construed as a potential conflict of interest.
All claims expressed in this article are solely those of the authors and do not necessarily represent those of their affiliated organizations, or those of the publisher, the editors and the reviewers. Any product that may be evaluated in this article, or claim that may be made by its manufacturer, is not guaranteed or endorsed by the publisher.
1. McClements DJ, Decker EA, Park Y, Weiss J. Structural design principles for delivery of bioactive components in nutraceuticals and functional foods. Crit Rev Food Sci Nutri. (2009) 49:577–606. doi: 10.1080/10408390902841529
2. Sonneville-Aubrun O, Simonnet J, L'Alloret F. Nanoemulsions: a new vehicle for skincare products. Adv. Col. Interface Sci. (2004) 108:145–9. doi: 10.1016/j.cis.2003.10.026
3. McClements DJ. Edible nanoemulsions: Fabrication, properties, and functional performance. Soft Matter. (2011) 7:2297–316. doi: 10.1039/C0SM00549E
4. McClements DJ. Advances in fabrication of emulsions with enhanced functionality using structural design principles. Curr Opin Col and Inter Sci. (2012) 17:235–45. doi: 10.1016/j.cocis.2012.06.002
5. McClements DJ. Edible lipid nanoparticles: Digestion, absorption, and potential toxicity. Progr Lipid Res. (2013) 52:409–23. doi: 10.1016/j.plipres.2013.04.008
6. Nejatian M, Darabzadeh N, Bodbodak S, Saberian H, Rafiee Z, Kharazmi MS, et al. Practical application of nanoencapsulated nutraceuticals in real food products; a systematic review. Adv Colloid Interface Sci. (2022) 305:102690. doi: 10.1016/j.cis.2022.102690
7. Islam F, Saeed F, Afzaal M, Hussain M, Ikram A, Khalid MA, et al. Food grade nanoemulsions: promising delivery systems for functional ingredients. J Food Sci Technol. (2022) 1–11. doi: 10.1007/s13197-022-05387-3
8. Forgiarini A, Esquena J, Gonza ' lez C, Solans C, Langmuir. Formation of nano-emulsions by low-energy emulsification methods at constant temperature. (2001) 17:2076–83. doi: 10.1021/la001362n
9. Abraham A. Multiple Emulsions Technology Applications. John Wiley Sons, Inc. (2007). p. 1-28. Available online at: https://scholar.google.com/scholar?hl=en&as_sdt=0%2C5&q=+Abraham+A.+Multiple+Emulsions+Technology+and+Applications.+John+Wiley+and+Sons%2C+Inc.+%282007%29.+p.+1-28.&btnG=
10. Haham M, Ish-Shalom S, Nodelman M, Duek I, Segal E, Kustanovich M, et al. Stability and bioavailability of vitamin D nanoencapsulated in casein micelles. Food Funct. (2012) 3:737–44. doi: 10.1039/c2fo10249h
11. Tsiaras WG, Weinstock MA. Factors influencing vitamin D status. Acta Dermato Venereologica. (2011) 91:115. doi: 10.2340/00015555-0980
12. Luo Y, Teng Z, Wang Q. Development of zein nanoparticles coated with carboxymethyl chitosan for encapsulation and controlled release of vitamin D3. J Agric Food Chem. (2012) 60:836–43. doi: 10.1021/jf204194z
13. Molaveisi M, Shahidi-Noghabi M, Naji-Tabasi S. (2020). Vitamin D3-loaded nanophytosomes for enrichment purposes: formulation, structure optimization, and controlled release. J Food Process Engin. 43:13560. doi: 10.1111/jfpe.13560
14. Ozturk B, Argin S, Ozilgen M, McClements DJ. Nanoemulsion delivery systems for oil-soluble vitamins: Influence of carrier oil type on lipid digestion and vitamin D3 bioaccessibility. Food Chem. (2015) 187:499–506. doi: 10.1016/j.foodchem.2015.04.065
15. Delaurent C, Siouffi AM, Pepe G. Cyclodextrin inclusion complexes with vitamin D3: Investigations of the solid complex characterization. Chem Analityczna. (1998) 43:601–16.
16. Maurya VK, Aggarwal M. A phase inversion based nanoemulsion fabrication process to encapsulate vitamin D3 for food applications. J Steroid Biochem Mol Biol. (2019) 190:88–98. doi: 10.1016/j.jsbmb.2019.03.021
17. Jan Y, Ahmed Al-Keridis L, Malik M, Haq A, Ahmad S, Kaur J, et al. Preparation, modelling, characterization and release profile of vitamin D3 nanoemulsion. Foof Sci Technol. (2022) 169. doi: 10.1016/j.lwt.2022.113980
18. Ghazani SM, García-Llatas G, Marangoni AG. Micronutrient content of cold-pressed, hot-pressed, solvent extracted and RBD canola oil: Implications for nutrition and quality. Euro J Lipid Sci Tech. (2014) 116:380–7. doi: 10.1002/ejlt.201300288
19. Zacchi P, Eggers R. High-temperature pre-conditioning of rapeseed: a polyphenol-enriched oil and the effect of refining. Eur J Lipid Sci Technol. (2008) 110:111–9. doi: 10.1002/ejlt.200700135
20. Hou W, Papadopoulos KD. W/O/W and O/W/O globules stabilized with Span 80 and Tween 80, Colloids and Surfaces A: Physicochemical and Engineering Aspects. (1997) 125:181–7. doi: 10.1016/S0927-7757(96)03861-7
21. Porras M, Solans C, Gonz'alez C, Mart'inez A, Guinart A, Guti' errez JM. “Studies of formation of W/O nano-emulsions,”Colloids and Surfaces. Physicochem Engin Aspects. (2004) 249:115–8. doi: 10.1016/j.colsurfa.2004.08.060
22. Bouchemal K, Brianc S, Perrier E, Fessi H. Nanoemulsion formulation using spontaneous emulsification: solvent, oil and surfactant optimization. Int J Pharm. (2004) 280:241–51. doi: 10.1016/j.ijpharm.2004.05.016
23. Elmataeeshy ME, Sokar MS, Bahey-El-Din M, et al. Enhanced transdermal permeability of Terbinafine through novel nanoemulgel formulation; development, in vitro and in vivo characterization. Futur J Pharm Sci. (2018) 4:18–28. doi: 10.1016/j.fjps.2017.07.003
24. Jadhav C, Kate V, Payghan SA. Investigation of effect of non-ionic surfactant on preparation of griseofulvin non-aqueous nanoemulsion. J Nanostruct Chem. (2015) 5:107–13. doi: 10.1007/s40097-014-0141-y
25. Hollis BW. Circulating 25-hydroxyvitamin D levels indicative of vitamin D sufficiency: implications for establishing a new effective dietary intake recommendation for vitamin D. J Nutr. (2005) 135:317–22. doi: 10.1093/jn/135.2.317
26. Algahtani MS, Ahmad MZ, Ahmad J. Investigation of factors influencing formation of nanoemulsion by spontaneous emulsification: impact on droplet size, polydispersity index, and stability. Bioengineering. (2022) 9:384. doi: 10.3390/bioengineering9080384
27. Sharifi F, Jahangiri M, Nazir I, Asim MH, Ebrahimnejad P, Hupfauf A, et al. Zeta potential changing nanoemulsions based on a simple zwitterion. J Colloid Interface Sci. (2021) 585:126–37. doi: 10.1016/j.jcis.2020.11.054
28. Ghosh V, Mukherjee A, Chandrasekaran N. Formulation and characterization of plant essential oil based nanoemulsion: evaluation of its larvicidal activity against Aedes aegypti. Asian J Chemistry. (2013) 25:S321.
29. Chudasama A, Patel V, Nivsarkar M, Vasu K, Shishoo C. Investigation of microemulsion system for transdermal delivery of itraconazole. J Adv Pharm Technol Res. (2011) 2:30–8. doi: 10.4103/2231-4040.79802
30. Garala K, Patel J. Design and development of a self nanoemulsifying drug delivery system for telmisartan for oral drug delivery. J Pharm Invest. (2011) 1:112–8. doi: 10.4103/2230-973X.82431
31. Suppaibulsuk B, Prasassarakich P, Rempel GL. Factorial design for nanosized polyisoprene synthesis via differential microemulsion polymerization. Polym Adv Technol. (2010) 21:467–75. doi: 10.1002/pat.1450
32. Ahmed MZ, Gupta A, Warsi MH, Ali AMA, Hasan N, Ahmad FJ, et al. Nano matrix soft confectionary for oral supplementation of vitamin D: stability and sensory analysis. Gels. (2022) 8:250. doi: 10.3390/gels8050250
33. Liang R, Xu S, Shoemaker CF, Li Y, Zhong F, Huang Q, et al. Physical and antimicrobial properties of peppermint oil nanoemulsions. J Agri and Food Chem. (2012) 60:7548–55. doi: 10.1021/jf301129k
34. Mowafaq M, Ghareeb, Abulfadhel J, Neamah. Formulation and characterization of nimodipine nanoemulsion as ampoule for oral route. Int. J. Pharma Sci. Res. (2017) 8:591–602.
35. Schoener AL, Zhang R, Lv S, Weiss J, McClements DJ. Fabrication of plant-based vitamin D 3-fortified nanoemulsions: Influence of carrier oil type on vitamin bioaccessibility. Food and function. (2019) 10:1826–35. doi: 10.1039/C9FO00116F
36. Maurya VK, Aggarwal M. Fabrication of nano-structured lipid carrier for encapsulation of vitamin D3 for fortification of ‘Lassi'; a milk based beverage. J Steroid Biochem Mol Biol. (2019) 193:105429. doi: 10.1016/j.jsbmb.2019.105429
37. Hippalgaonkar K, Majumdar S, Kansara V. Injectable lipid emulsions-Advancements, opportunities and challenges. AAPS PharmSciTech. (2010) 11:1526–40. doi: 10.1208/s12249-010-9526-5
38. Schalbart P, Kawaji M, Fumoto K. Formation of tetradecane nanoemulsion by low-energy emulsification methods. Int J refrigeration. (2010) 33:1612–24. doi: 10.1016/j.ijrefrig.2010.09.002
39. Razzaq FA, Asif M, Asghar S, Iqbal MS, et al. Glimperide-Loaded nanoemulgel; development, In-vitro characterization, ex vivo permeation and in vivo antidiabetic evaluation. Cells. (2021) 10:2404. doi: 10.3390/cells10092404
40. Walia N, Dasgupta N, Ranjan S, Chen L, Ramalingam C. Fish oil based vitamin D nanoencapsulation by ultrasonication and bioaccessibility analysis in simulated gastro-intestinal tract. Elsevier, Ultasonics- Sonochem. (2017) 39:623–35. doi: 10.1016/j.ultsonch.2017.05.021
41. Demisli S, Theochari I, Christodoulou P, Zervou M, Xenakis A, Papadimitriou V, et al. Structure, activity and dynamics of extra virgin olive oil-in-water nanoemulsions loaded with vitamin D3 and calcium citrate. J Mol Liq. (2020) 306:112908. doi: 10.1016/j.molliq.2020.112908
42. De Moreira, Morais J, David Henrique dos Santos O, Delicato T, Azzini Gonçalves R, Alves da Rocha-Filho P. Physicochemical characterization of canola oil/water nano-emulsions obtained by determination of required HLB number and emulsion phase inversion methods. J Disp Sci Technol. (2006) 27, 109–115. doi: 10.1081/DIS-200066829
43. Mehmood T, Ahmed A. Tween 80 and soya-lecithin-based food-grade nanoemulsions for the effective delivery of vitamin D. Langmuir. (2020) 36:2886–92. doi: 10.1021/acs.langmuir.9b03944
44. Cheng LN, Mahiran B, Minaketan T, Roghayeh AK, Emilia AM. Physicochemical characterization and thermodynamic studies of nanoemulsion-based transdermal delivery system for fullerene. Sci World J. (2014) 12. doi: 10.1155/2014/219035
45. Zhang X, Song R, Liu X, Xu Y, Wei R. Fabrication of vitamin D3 nanoemulsions stabilized by Tween 80 and Span 80 as a composite surface-active surfactant: characterization and stability. Colloids Surfaces Physicochem Engin Aspects. (2022) 645:128873. doi: 10.1016/j.colsurfa.2022.128873
Keywords: nanoemulsion, canola oil, water titration techniques, vitamin D fortification, bioavailability
Citation: Khalid A, Arshad MU, Imran A, Haroon Khalid S and Shah MA (2023) Development, stabilization, and characterization of nanoemulsion of vitamin D3-enriched canola oil. Front. Nutr. 10:1205200. doi: 10.3389/fnut.2023.1205200
Received: 13 April 2023; Accepted: 17 July 2023;
Published: 24 August 2023.
Edited by:
Shakeel Ahmed, Government Degree College Mendhar, IndiaReviewed by:
Amita Shakya, Amity University, Chhattisgarh, IndiaCopyright © 2023 Khalid, Arshad, Imran, Haroon Khalid and Shah. This is an open-access article distributed under the terms of the Creative Commons Attribution License (CC BY). The use, distribution or reproduction in other forums is permitted, provided the original author(s) and the copyright owner(s) are credited and that the original publication in this journal is cited, in accordance with accepted academic practice. No use, distribution or reproduction is permitted which does not comply with these terms.
*Correspondence: Muhammad Umair Arshad, dW1haXJmb29kMUBnbWFpbC5jb20=; Mohd Asif Shah, ZHJtb2hkYXNpZnNoYWhAa2R1LmVkdS5ldA==
Disclaimer: All claims expressed in this article are solely those of the authors and do not necessarily represent those of their affiliated organizations, or those of the publisher, the editors and the reviewers. Any product that may be evaluated in this article or claim that may be made by its manufacturer is not guaranteed or endorsed by the publisher.
Research integrity at Frontiers
Learn more about the work of our research integrity team to safeguard the quality of each article we publish.