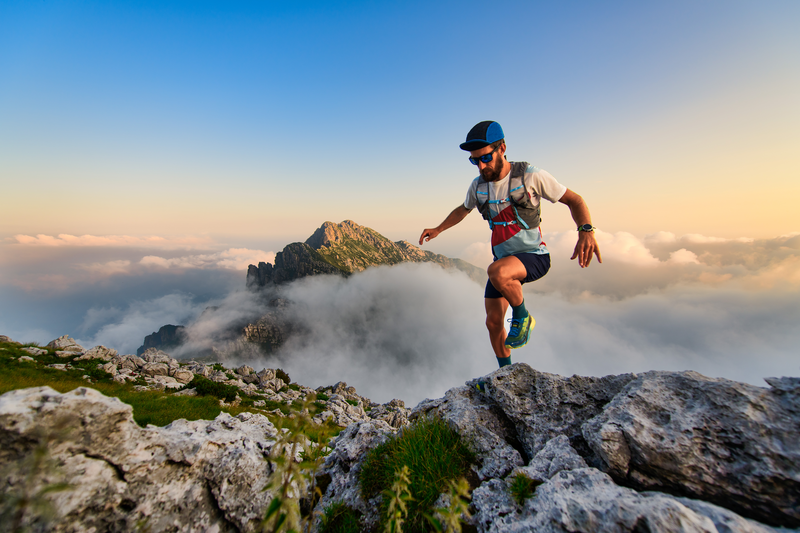
95% of researchers rate our articles as excellent or good
Learn more about the work of our research integrity team to safeguard the quality of each article we publish.
Find out more
CLINICAL TRIAL article
Front. Nutr. , 06 July 2023
Sec. Clinical Nutrition
Volume 10 - 2023 | https://doi.org/10.3389/fnut.2023.1204561
This article is part of the Research Topic Sulforaphane and Isothiocyanates in Health View all 9 articles
Background and aims: As our understanding of platelet activation in response to infections and/or inflammatory conditions is growing, it is becoming clearer that safe, yet efficacious, platelet-targeted phytochemicals could improve public health beyond the field of cardiovascular diseases. The phytonutrient sulforaphane shows promise for clinical use due to its effect on inflammatory pathways, favorable pharmacokinetic profile, and high bioavailability. The potential of sulforaphane to improve platelet functionality in impaired metabolic processes has however hardly been studied in humans. This study investigated the effects of broccoli sprout consumption, as a source of sulforaphane, on urinary 11-dehydro-thromboxane B2 (TXB2), a stable thromboxane metabolite used to monitor eicosanoid biosynthesis and response to antithrombotic therapy, in healthy participants exposed to caloric overload.
Methods: In this double-blind, placebo-controlled, crossover trial 12 healthy participants were administered 16g of broccoli sprouts, or pea sprouts (placebo) followed by the standardized high-caloric drink PhenFlex given to challenge healthy homeostasis. Urine samples were collected during the study visits and analyzed for 11-dehydro-TXB2, sulforaphane and its metabolites. Genotyping was performed using Illumina GSA v3.0 DTCBooster.
Results: Administration of broccoli sprouts before the caloric load reduced urinary 11-dehydro-TXB2 levels by 50% (p = 0.018). The amount of sulforaphane excreted in the urine during the study visits correlated negatively with 11-dehydro-TXB2 (rs = −0.377, p = 0.025). Participants carrying the polymorphic variant NAD(P)H dehydrogenase quinone 1 (NQO1*2) showed decreased excretion of sulforaphane (p = 0.035).
Conclusion: Sulforaphane was shown to be effective in targeting platelet responsiveness after a single intake. Our results indicate an inverse causal relationship between sulforaphane and 11-dehydro-TXB2, which is unaffected by the concomitant intake of the metabolic challenge. 11-Dehydro-TXB2 shows promise as a non-invasive, sensitive, and suitable biomarker to investigate the effects of phytonutrients on platelet aggregation within hours.
Clinical trial registration: [https://clinicaltrials.gov/], identifier [NCT05146804].
Our understanding of platelet functionality has changed dramatically over the past decade. Platelets are no longer viewed simply as hemostasis regulators; they are now recognized as crucial in coordinating inflammatory and immune responses (1, 2). Impaired platelet function has been observed in several chronic inflammatory conditions, including thrombosis and thrombotic disorders (3), asthma (4), myocardial infarction (5), unstable angina pectoris (6), atherosclerosis (7), and type 2 diabetes (8, 9). Interestingly, also postprandial hyperglycemia has been shown to cause platelet activation (10) and promote inflammatory state in healthy individuals (11–13). In the clinical setting, administering caloric loads to humans has been used as a tool to pressurize adaptive mechanisms and trigger inflammation which may also be associated with an increase in platelet reactivity (14).
Thromboxanes are arachidonic acid metabolites with significant biological activity, including regulation of platelet functionality (15–17). Increased production of thromboxanes was recognized to contribute to vasculopathy by adversely affecting endothelial function and promoting vascular inflammation (18). By activating the thromboxane receptor, those metabolites cause significant alterations of platelet shape, inside-out activation of integrins, and degranulation (15–17), which might subsequently lead to increased platelet aggregation and thrombosis. On the other hand, increased production of thromboxane A and activation of platelets are recognized to be crucial modulators of the functionality of multiple inflammatory pathways involved in the progression of the cardiovascular diseases (19). 11-Dehydro-thromboxane B2 (TXB2) is produced from the breakdown of thromboxane A and can be measured in urine as a relevant marker of platelet reactivity, e.g., to monitor the response to aspirin (ASA) therapy when used to prevent heart disease (20, 21).
In fact, targeting thromboxane production via antiplatelet agents has become a cornerstone of cardiovascular disease treatment (1, 22). Antiplatelet drugs (i.e., ASA, clopidogrel, prasugrel and abciximab) typically irreversibly suppress a specific pathway of platelet aggregation (1, 22). Therefore, the main limitations of current antiplatelet agents include the risk of bleeding and prolonged duration of action that cannot be reversed if the need for hemostasis or emergency surgery arises (22, 23), which limits their use in many clinical situations, e.g., prophylaxis of cardiovascular events in high-risk groups (1). Phytonutrients have also been shown to affect platelet functionality with water-soluble tomato concentrate (WSTC) being the first to be recognized by the European Food Safety Agency (EFSA) as functional food that helps to maintain normal platelet aggregation, which contributes to healthy blood flow (24). Unlike drug therapy, the antiplatelet effect of most phytochemicals is reversible, making them potentially safe for use in a variety of clinical scenarios, including primary prevention of cardiovascular diseases (17.9 million deaths annually) in the general population (1, 25, 26). Therefore, it is paramount to search for novel nutritional strategies that support platelet functionality.
Of the various plant-derived bioactive nutrients, sulforaphane holds the most promise for clinical testing due to superior absorption and pharmacokinetic profile (27–46). Glucoraphanin, the biogenic precursor of sulforaphane, is present in large amounts in broccoli and to a lesser extent in other Brassica species (47, 48). Since glucoraphanin is biologically inert, factors controlling the biosynthesis of glucoraphanin and conversion to sulforaphane by the enzyme myrosinase are of great importance to the potential health effects of these vegetables (28, 39, 48). The antithrombotic properties of sulforaphane have been demonstrated in animals and in vitro studies (49, 50). Sulforaphane exerts antiplatelet activity which may initially activate adenylate cyclase/cAMP, followed by reversible inhibition of multiple intracellular signals such as the PI3-kinase/Akt and PLCγ2-PKC-p47 cascades (49, 50). Although promising, demonstrating an antithrombotic effect in vivo after oral administration of the compound has been scarcely explored in clinical trials, hence sulforaphane’s ability to modulate platelet functionality in humans is still poorly studied (51).
These findings motivated us to investigate the effects of broccoli sprout consumption, as a source of sulforaphane, on urinary 11-dehydro-TXB2 in healthy participants exposed to a standardized caloric load and to investigate interindividual genetic variability.
A randomized, placebo-controlled, double-blind study was conducted with a cross-over design. The study protocol (NL77272.068.21) was approved by the Medical Ethics Review Committee of Maastricht University Medical Centre+ (MUMC+) and Maastricht University, Maastricht, the Netherlands, and performed in full accordance with the declaration of Helsinki of 1975 as revised in 2013, Fortaleza, Brazil (52). The trial registration number within ClinicalTrials.gov is NCT05146804. All subjects provided written informed consent to participate.
Healthy men and women were recruited by local and social media advertisements. Inclusion criteria were that participants were between 18 and 50 years old, had a body mass index (BMI) between 18.5 and 30 kg/m2, with a stable weight (<5% body weight change) and constant eating habits over the past 3 months. Exclusion criteria were the previous diagnosis of an inflammatory condition or disease or a history of hypothyroidism, chronic kidney or/and liver disorders, coronary artery disease, malignant hypertension, seizures, involved in intensive sports activities more than four times a week or at top sport level, regular intake of medication that may affect inflammatory response including NSAIDs, psychotic, addictive, or other mental disorders, aversion, intolerance or allergy to cruciferous vegetables and/or palm olein, dextrose, protein supplement, vanilla aroma, the use of dietary supplements with potential effects on antioxidant or inflammatory status and/or viral or bacterial infections requiring the use of antibiotics, laxatives and anti-diarrheal drugs 4 weeks prior to inclusion, excessive alcohol consumption (≥28 consumptions, approx. 250 g alcohol per week), pregnancy and/or breastfeeding, reported slimming or medically prescribed diet, as well as adhering to a vegetarian or vegan lifestyle.
Commercially available broccoli sprouts BroccoCress®, a rich source of sulforaphane, were used as the experimental product. In total, 16 g of sprouts were used per serving. Sulforaphane (BroccoCress®) and placebo (Affilla Cress®) were administered to each participant in the randomized fashion on different testing days. The period between two visits was 7 ± 3 days. Information about demographics, alcohol consumption, and anthropometric data were assessed on the first visit. BMI, total body fat and visceral fat were measured using the Omron BF511R® monitor. The same testing scheme was applied during two visits (Figure 1), i.e., each participant received a single serving of intervention/placebo, which after 90 min was followed by oral administration of the PhenFlex challenge. Urine samples were collected throughout the day of the visit, preferably before the intervention/placebo, between intervention/placebo and the PhenFlex challenge, and after the PhenFlex administration. All participants were instructed to come fasted to each visit, to avoid consumption of broccoli or other cruciferous vegetables 2 days before each visit and to refrain from intense physical activity on the day of the visit. During the visit, participants remained in the testing location and were allowed to drink water ad libitum. No food intake was permitted during the visit.
Figure 1. Schematic presentation of a study visit. Administration of intervention (sulforaphane/placebo) was followed in 90 min by administration of standardized caloric challenge PhenFlex. Urine samples were classified into three groups: (A) (baseline, green line), (B) (after intervention or placebo, blue lines), and (C) (after PhenFlex challenge, red lines). In addition, samples were also divided into 5 timepoints: 0 (baseline), 1 (<60 min after intervention or placebo), 2 (>60 min after intervention or placebo), 3 (<60 min after PhenFlex challenge), and 4 (>60 min after PhenFlex challenge).
Shortly (maximum of 3 min) before administration, the sprouts were cut approximately 1 cm below the leaves, weighed, and mashed with a small amount of tap water (approximately 13°C) in a kitchen blender for 30s at room temperature (Premium Impuls Blender Smoothiemaker; Impuls; 180 W). Subsequently, tap water (approximately 13°C) was added to a total amount of 250 mL and participants were instructed to drink the entire mixture. Commercially available pea sprouts (Affilla Cress®) were used as placebo in this study since pea sprouts do not contain glucoraphanin/sulforaphane. Affilla Cress (16 g) was prepared and administered in a similar fashion. Blinding of participants was ensured by the even appearance of both drinks and the use of nasal plugs during consumption of the investigational products. The placebo or intervention product was prepared by a researcher who was not involved in any other study procedures and data analysis. Ninety minutes after administration of the investigational products, participants were asked to drink a high-fat, high-glucose, high-caloric product (PhenFlex) (53). For the preparation of the PhenFlex (400 mL, 950 kcal) 60 g palm olein, 75 g dextrose, 20 g protein, 0.5 g artificial vanilla aroma and 320 mL tap water were used (53). In all cases, PhenFlex mixtures were freshly prepared, and the participants were instructed to consume the drink within 5 min.
Urine samples were collected throughout the days of the visits in pre-labeled containers. The total volume and time of collection of each sample was recorded. Samples were aliquoted and stored at ≤ −80°C until the day of analysis. Urine samples were analyzed for 11-dehydro-TXB2 using an enzyme-linked immunoassay kit (UTxB2: assay #519510, Cayman Chemical, Ann Arbor, MI) following the manufacturer’s instructions. The manufacturer recommended standardizing urinary 11-Dehydro-TXB2 values for creatinine levels using a colorimetric assay kit [Creatinine (urinary) Colorimetric Assay Kit: assay #500701, Cayman Chemical, Ann Arbor, MI]. 11-dehydro-TXB2 concentrations were normalized to urinary creatinine concentrations expressed as μg/mg Cr to account for inter-individual variability in urine dilution. Urine samples were classified into three groups for analysis: A (baseline), B (after intervention or placebo) and C (after PhenFlex challenge). In addition, samples were also divided into five timepoints: 0 (baseline), 1 (<60 min after intervention or placebo), 2 (>60 min after intervention or placebo), 3 (<60 min after PhenFlex challenge) and 4 (>60 min after PhenFlex challenge).
The determination of sulforaphane (SFN) and sulforaphane-glutathione (SFN-GSH), sulforaphane-cysteine (SFN-Cys), sulforaphane-cysteine-glycine (SFN-CG) and sulforaphane-N-acetylcysteine (SFN-NAC) in human urine was based on an HPLC–MS/MS method from Egner et al. (54).
Trc Canada deuterated stable Isotope solutions of sulforaphane-d8 and sulforaphane-d8-N-acetyl-L-cysteine were purchased from LGC standards (Wesel, Germany). J.T. Baker ethanol and Biosolve acetonitrile were purchased from Boom (Meppel, the Netherlands). Formic acid (FA) was purchased from VWR (Amsterdam, the Netherlands). Fresh water was obtained from an inhouse MilliQ Advantage A10 system containing a LC–MS filter pack (Millipore, Burlington, MA). All chemicals were of analytical quality or higher.
The stock solutions of SFN-d8-NAC and SFN-d8 were diluted with ethanol containing 0.2% FA by a factor of 10 and 100, respectively. From both dilutions 40 μL was taken and combined in a new tube. The mixture was further diluted with ethanol containing 0.2% FA to give final concentrations of 2 μg/mL for SFN-d8-NAC and 0.2 μg/mL for SFN-d8 (IS mix). Stability of IS mix was verified by repeated analysis over a time period of several days.
Urine samples were taken from the −80°C storage and thawed on ice. The samples were vortexed briefly and centrifuged at 21000 g and 4°C for 5 min. From the supernatant 20 μL was transferred to a HPLC vial with a 200 μL insert. IS mix (20 μL) was added and mixed with the urine sample. Thereafter, the samples (5 μL) were directly injected into the analytical system.
For chromatographic separation an ExionLC UHPLC system (AB Sciex Framingham, MA) equipped with a binary pump, a thermostated autosampler and a column oven was used to maintain a constant temperature (40°C) during the analytical run. For detection a X500R qToF MS system (AB Sciex Framingham, MA) was used. Data acquisition was performed using SciexOS V2.1.6.59781 (AB Sciex). Data processing and identification of sulforaphane and its metabolites was performed by MS-DIAL (version 4.92).
The participants were requested to take a DNA sample using cheek swabs. Subsequently, all samples were processed at the Human Genomics Facility (HuGe-F) of the Genetic Laboratory of the Department of Internal Medicine at Erasmus MC using the Illumina GSA v3.0 DTC array consisting of 703,320 unique Single Nucleotide Polymorphisms (SNPs). The HuGe-F applied genotyping using the GenomeStudio v2 software with in-house cluster files for reference genome GRCh37. The resulting genotype files are subjected to in-house quality control pipelines which includes correcting for missing data and removing SNPs violating Hardy–Weinberg Equilibrium (55). Finally, imputation is applied to the corrected and filtered SNPs with Beagle software (56) using a reference panel from the 1.000 Genomes project consisting of 2,141 samples (57). Seven gene regions were selected for the analysis based on literature review (36, 58–64). For these genes, their genomic location according to the GRCh37 reference genome was determined from the NCBI Gene database and can be viewed in Table 1 (72). The preprocessed SNPs were filtered on overlap with these gene regions. The resulting subset of potentially interesting SNPs was annotated with information from the NCBI dbSNP database (72).
All normally distributed data are presented as mean ± standard deviation (SD). The non-normally distributed data are shown as median (interquartile range). For categorical variables, frequency and/or percentages are presented. Differences between the groups were assessed by repeated measures ANOVA for normally distributed parameters, or Skillings-Mack tests for the data that was not normally distributed. In addition, paired sample t-tests and Wilcoxon rank-sum tests were performed as post-hoc tests for the normally distributed samples and the non-normally distributed samples, respectively. Mann–Whitney U tests were performed to compare the change in urinary 11-dehydro-TXB2 within sample groups between subjects. To study the association between 11-dehydro-TXB2 and sulforaphane (metabolites), Spearman correlation was performed. The tool Stargazer (73) was used for phenotyping the metabolizer type of the participants for the NAT1 and NAT2 pharmacogenes. Participants were subdivided into low versus high excretion of sulforaphane. An adaptation of Fisher’s Exact test within the plink genomics software was applied on the groups to test for significantly different genotypes (74). Additionally, adaptive permutation testing within plink was executed to test the validity of the resulting p-values. All analyses were performed two-tailed with p ≤ 0.05 considered statistically significant.
Between November 2021 and January 2022, a total of 12 subjects were found to be eligible to participate in the present study and were randomly allocated to either initial administration of sulforaphane or placebo. Baseline characteristics of the study population (n = 12) are summarized in Table 2. All participants completed the study and were included in the data analysis for biochemical testing (Figure 2). A total of 54 urine samples were collected and assessed. Urine samples were classified into three groups: A (baseline), B (after intervention or placebo) and C (after PhenFlex challenge). In addition, samples were also divided into five timepoints: 0 (baseline), 1 (<60 min after intervention or placebo), 2 (>60 min after intervention or placebo), 3 (<60 min after PhenFlex challenge) and 4 (>60 min after PhenFlex challenge). Given the crossover design of the study, a pre-test was first performed to verify whether the time of the washout period was sufficient and to exclude any carry-over effects. The pre-test (Wilcoxon rank sum test) revealed no differences between treatment allocations for 11-dehydro-TXB2 (z < −4.47, p > 0.655). A significant difference between the time of sampling in each sample group was checked by Kruskal-Wallis test H (5) = 63.647, p < 0.001.
Differences between groups were assessed for uncorrected and creatinine-corrected 11-dehydro-TXB2. For the uncorrected data, a significant difference was observed in urinary 11-dehydro-TXB2 concentrations between all groups in the sulforaphane group (X2(2) = 11.60, p = 0.003) and in the placebo group (X2(2) = 7.58, p = 0.023). Post hoc analysis showed significant differences for the sulforaphane group between samples A and B, and between samples A and C (p = 0.028 and p = 0.018), and between samples A and B for placebo (p = 0.028; Figure 3). Analysis of the corrected data showed a significant difference in urinary 11-dehydro-TXB2 concentration between all groups in the sulforaphane group (X2(2) = 9.27, p = 0.010), no significant differences were observed in the placebo group (X2(2) = 3.53, p = 0.171). Post hoc analysis showed a significant difference in the sulforaphane group between samples A and C for corrected data (p = 0.018; Figure 4). Analysis between treatment allocations within samples showed no significant results (Table 3).
Figure 3. Changes in urinary 11-dehydro-TXB2 concentration in sulforaphane and placebo groups. Uncorrected data are presented as boxplots [median, interquartile range, outliers (circles) and far outliers (squares)]. Urine samples were clustered into three timepoints: (A) (baseline), (B) (after intervention or placebo), and (C) (after PhenFlex challenge). *p < 0.05.
Figure 4. Changes in urinary 11-dehydro-TXB2 concentration in sulforaphane and placebo groups corrected for creatinine. Data are presented as boxplots [median, interquartile range, outliers (circles) and far outliers (squares)]. Urine samples were clustered into three timepoints: (A) (baseline), (B) (after intervention or placebo), and (C) (after PhenFlex challenge). *p < 0.05.
Table 3. Uncorrected and corrected urinary 11-dehydro-TXB2 concentrations (mean ± SD) per timepoint per treatment allocation.
The presence of sulforaphane after consuming fresh broccoli sprouts, and absence after placebo, was determined in urine samples collected during the study visits, indicating an adequate period of abstinence (mean total amount of sulforaphane and metabolites: 8.2 vs. 0.4 μmol, p < 0.001). Sulforaphane, SFN-N-acetylcysteine (SFN-NAC), SFN-cysteine, and total amount of metabolites were determined in all urine samples. No detectable levels of SFN-glutathione and SFN-cysteine-glycine were quantified. In the intervention group, the amount of sulforaphane excreted in urine significantly increased 120 min after its administration (p = 0.028) and decreased during the subsequent hour (p = 0.043; Figure 5A). The amount of SFN-NAC excreted in urine significantly increased 120 min after the intervention (p = 0.028), and from the first 45 min to 1.5 h afterwards (p = 0.028) decreased during the subsequent hour (p = 0.043; Figure 5B). The amount of SFN-cysteine excreted in urine significantly increased 2 h after the intervention (p = 0.028; Figure 5C). The total amount of metabolites excreted in urine significantly increased 120 min after the intervention (p = 0.028) and from the first 45 min to an hour and a half afterwards (p = 0.046) and decreased during the subsequent hour (p = 0.043) (Figure 5D). In addition, the amount of sulforaphane excreted in the urine during the study visits correlated negatively with 11-dehydro-TXB2 (rs = −0.377, p = 0.025). Moderate strength, inverse correlations between other metabolites SFN-NAC (rs = −0.210, p = 0.225), SFN-Cys (rs = −0.131, p = 0.452) and total metabolites (rs = −0.196, p = 0.258) were observed.
Figure 5. Urinary sulforaphane (SFN), sulforaphane-cysteine (SFN-Cys), sulforaphane-N-acetylcysteine (SFN-NAC), and total metabolites excretion after participants consumed a single intake (16 g) of broccoli sprouts. Data are presented as boxplots [median, interquartile range, and outliers (circles)]. The dashed lines indicate when the PhenFlex challenge was administered. Urine samples were clustered into five timepoints: 0 (baseline), 1 (<60 min after intervention), 2 (>60 min after intervention), 3 (<60 min after PhenFlex challenge), and 4 (>60 min after PhenFlex challenge). The amount of SFN (A) excreted in the urine correlated negatively with 11-dehydro-TXB2 (rs = −0.377, p = 0.025). Moderate strength, inverse correlations between other metabolites were observed, i.e., SFN-NAC (B) (rs = −0.210, p = 0.225), SFN-Cys (C) (rs = −0.131, p = 0.452) and total metabolites (D) (rs = −0.196, p = 0.258). *p < 0.05.
The 703,320 SNPs were subjected to the quality control pipeline, after which 624,240 SNPs were left. The subsequent imputation with Beagle resulted in 24,232,949 SNPs available for downstream analysis. Seven genes were selected for further analysis based on previous literature. Filtering the imputed SNPs using the gene regions as defined in Table 1 resulted in 77 SNPs that were further annotated and tested. Based on dbSNP annotations these included seven missense, 63 intronic, three synonymous, three 3′ untranslated regions (UTR), and one 5′ prime UTR variants. Testing the 77 relevant SNPs using Plink’s adoption of Fisher’s Exact test resulted in six SNPs associated with sulforaphane excretion (Table 4). All SNPs were found in the NQO1 gene and all, but one were intronic variants, while the other SNP was a missense variant causing a change from proline to serine. Participants carrying this polymorphic variant NQO1*2, which is characterized by a C609T (rs1800566, Pro187Ser) polymorphism of the NQO1 gene, showed decreased excretion of sulforaphane during the study visits [6.2 (1.0) vs. 9.2 (1.6) μmol total metabolites, p = 0.035]. No influence of these SNPs on 11-dehydro-TXB2 levels was observed. No influence of SNPs in GSTM1, GSTP1, GSTT1, CYP1A2, UGT1A1 and NAT2 genes, or type of NAT1 and NAT2 metabolizer on sulforaphane excretion and TXB2 levels was observed (Supplementary Tables 1, 2).
The aim of this work was to investigate the effects of sulforaphane on the arachidonic acid metabolite, as well as the relationship between 11-dehydro-TXB2 and sulforaphane and its mercapturic conjugates in urine. Therefore, we assessed the effect of a single intake of fresh broccoli sprouts on urinary 11-dehydro-TXB2 in healthy participants exposed to a standardized caloric load.
This study showed that consumption of 16 g of broccoli sprouts before a caloric overload reduced the amount of urinary 11-dehydro-TXB2 in healthy participants by 50%. These results are consistent with previous research examining the effects of sulforaphane on urinary biomarkers associated with inflammation. Medina et al. (51) showed that higher doses (30 g and 60 g) of broccoli sprouts significantly reduced urinary 11-dehydro-TXB2 levels by 91 and 94%, respectively, within 12 h in healthy subjects. The higher reduction found by Medina et al. (51) is probably due to the combination of the higher amount of broccoli sprouts given, the lack of a caloric challenge, and the longer time of observation. These results, additionally, indicate that urinary 11-dehydro-TXB2 shows promise as a sensitive and suitable biomarker to investigate the effects of phytonutrients on platelet aggregation, at least in young healthy participants who are metabolically challenged in a short period of time. For example, in this study, the amount of urinary inflammatory biomarker high-sensitivity C-reactive protein (hs-CRP) remained unchanged during the study visits and was only detectable in 33/54 samples (data not shown).
Our data on sulforaphane excretion and its relationship to urinary thromboxane metabolites shows a significant inverse correlation between sulforaphane and urinary 11-dehydro-TXB2 (rs = −0.377, p = 0.025) during the metabolic challenge. In contrast to previous studies, this inverse relationship was only significant for sulforaphane and not for other metabolites nor total metabolites, which may have been influenced by the co-ingestion of the caloric load and the shorter duration of urine collection (51). It is striking however, that from these results it can be concluded that the metabolic state apparently has no effect on the effect of sulforaphane on 11-dehydro-TXB2 levels.
Previous research has shown that a single administration of a standardized caloric load, the PhenFlex challenge, impacts relevant metabolic processes involved in maintaining or regaining homeostasis of metabolic health in healthy volunteers (12). Contrary to our expectations, the caloric overload did not increase urinary 11-dehydro-TXB2 measured 2 h after administration of placebo (pea sprouts). Although pea sprouts have a similar nutrient profile to broccoli sprouts, except presence of sulforaphane, the increased amount of retinol and beta-carotene may have counteracted the expected post-challenge increase in 11-dehydro-TXB2. Retinol and beta-carotene are transformed to retinoic acid (RA) by retinaldehyde dehydrogenases via numerous conversions (75). RA is mediated by two nuclear receptor families, the retinoic acid receptors, and the retinoid X receptors (RXR) (76). These receptors interact with retinoic acid response elements on the promoter regions of target genes, resulting in the activation of nuclear transcription factors (77, 78). The main antithrombotic effect of retinol and beta-carotene is based on the interference of RXR with the NF-κB pathway (79, 80). Inhibition of NF-κB disrupts platelet function by reducing its thrombogenic potential and shows promise when compounds that block NF-κB activation, such as sulforaphane and RA, are considered for prophylaxis of various thrombo-inflammatory diseases (80).
Previous reports have shown that genetic predisposition may influence the bioavailability and excretion rate of sulforaphane, however results are incongruent (48, 58, 59, 61, 64, 81–87). Partially consistent with Boddupalli et al. (58), we found lower excretion of sulforaphane in participants carrying the polymorphic variant NQO1*2, but no effect of SNPs in other phase II detoxification enzyme genes.
When cruciferous vegetables are damaged, such as when preparing or chewing, myrosinase is released. This enzyme is normally stored separately from glucosinolates, such as glucoraphanin, in different cells or in different intracellular compartments, depending on the plant species (44). Myrosinase catalyzes the hydrolysis of glucoraphanin to liberate the glucose group, resulting in an unstable aglucone that spontaneously rearranges to give rise to a range of products, of which sulforaphane is the most reactive (Figure 6) (44). Cooking cruciferous vegetables inactivates myrosinase, resulting in less sulforaphane formation (44). Bacterial populations of the gut microbiota are also able to converse glucoraphanin to sulforaphane; however, it is estimated to be approximately six-fold less effective than plant myrosinase (88). After this critical first step, sulforaphane is conjugated with glutathione (GSH) upon entry into the mammalian cell, catalyzed by glutathione S-transferases (GSTs), in the liver, entering the mercapturic acid pathway (89). The glutathione conjugate is subjected to a series of sequential conversions, resulting in the N-acetylcysteine conjugate (mercapturic acid) as the main metabolite, which is excreted in the urine (Figure 6) (44). Consistent with other studies, this study found the N-acetylcysteine conjugate as the primary metabolite in the urine samples (44, 48). However, compared to studies that examined timed urine samples over a longer period of time (8 h and longer), we found lower ratios of SFN-cysteine/total metabolites and no detectable levels of SFN-glutathione and SFN-cysteine-glycine (44, 48). We hypothesize that the longer period of observation and timed collection of urine samples could explain the differences in ratios. The results of Medina et al. (51), which showed that the SFN-cysteine/total metabolites ratio was twice as high in the first 12 h as in the subsequent 12 h, are in line with this hypothesis. Future research into the relationship between different metabolite ratios and time differences could provide an explanation for these inconsistencies.
Figure 6. The enzymatic conversion of glucoraphanin (GRP) to sulforaphane (SFN) by myrosinase (Myr), followed by the absorption, distribution, metabolism, and excretion of SFN via the mercapturic acid pathway. The enzymatic conversion of GRP to SFN is considered to be the rate-limiting step affecting bioavailability within this cascade, as the efficiency of conversion of SFN to metabolites in humans is very high (70–90%) (48). GRP, glucoraphanin; GST, glutathione S-transferase; Myr, myrosinase; γGT, γ-glutamyl transferase; CG, cysteinylglycinase; NAT, N-acetyl transferase; SFN-Cys, sulforaphane-cysteine; SFN-NAC, sulforaphane-N-acetylcysteine; SFN-GSH, sulforaphane glutathione conjugate.
Polymorphisms in phase II enzymes involved in the mercapturic acid pathway may help explain the individual outcomes of sulforaphane interventions (Table 1; Figure 6) (58, 59). In the current study, we found that not the enzymes directly involved, but an antioxidant enzyme, NQO1, indirectly involved in this metabolic route influenced sulforaphane secretion. Previous studies showed that the null NQO1*2 polymorphism results in a lack of functional NQO1 protein due to reduced stability, the ability to bind flavin adenine dinucleotide, and a dramatically reduced half-life due to rapid polyubiquitination and proteasomal degradation (83, 90). The lack of functional NQO1 would then in turn reduce the pool of other enzymes that catalyze detoxification of electrophiles, e.g., GSTs (91, 92). We hypothesize that because sulforaphane is metabolized by GSTs, the reduced glutathione pool will result in longer half-lives of circulating sulforaphane and potentially greater systemic effects of cruciferous vegetables. Paradoxically, the increased activation of Nrf2/ARE-dependent genes by sulforaphane, including GSTs, thioredoxin and heme oxygenase 1, would also result in more non-functional NQO1 in individuals with the NQO1*2 polymorphism (41). This positive feedback loop could explain the lower excretion of sulforaphane in participants carrying the polymorphic variant NQO1*2, while no initial differences in antithrombotic effect were observed after a single administration of broccoli sprouts. Repeated-dose studies could reveal whether individuals with genetic polymorphisms that reduce the activity of detoxification enzymes could benefit even more from consumption of cruciferous vegetables. Further clarification of the interactions between polymorphisms and the downstream effectors of sulforaphane are promising new lines of research to elucidate the relationship between the consumption of cruciferous vegetables and human health and well-being (58).
Overall, only a limited number of food-derived compounds have been clinically investigated for their antiplatelet effects. Some success has been achieved in developing antiplatelet nutraceuticals, with WSTC to date being the only functional food proven to function as a natural cardio-protective functional ingredient, as assessed by the EFSA (25). Therefore, Fruitflow®, the trademarked name of WSTC, is authorized by the European Commission to use the health claim (new function claims, Art. 13.5): “water-soluble tomato concentrate (WSTC) I and II helps maintain normal platelet aggregation, which contributes to healthy blood flow” (93, 94). To provide stakeholders with greater clarity on which effects related to cardiovascular health could be studied to support health claims, in 2018, a guidance was published by EFSA’s Panel on Dietetic Products, Nutrition and Allergies (NDA Panel). This guidance document provides more detailed guidelines for the evaluation of Articles 13.1, 13.5, and 14 health claims in this area (95). According to the Panel, a reduction in platelet aggregation [i.e., the percentage of inhibition of platelet aggregation using light transmission aggregometry (LTA) according to well-accepted and standardized protocols] in subjects with platelet activation during sustained exposure to the food/constituent (at least 4 weeks) is a beneficial physiological effect. Other outcome variables, such as thromboxane A2 (TXA2) or plasma soluble P-selectin, are not considered established markers of platelet aggregation, but can be used as supporting evidence for the scientific substantiation of these claims (95, 96).
Although LTA has been considered the gold standard to assess platelet function for over 40 years, poor standardization and the required manipulation by a skilled technician limit its use to specialized laboratories (20, 97–100). Numerous platelet function tests are currently available; however, their methodologies are diverse, and little is known about the comparability or interchangeability of these tests (20, 97). O’Kennedy et al. examined TXA2 levels (via thromboxane B2, the stable metabolite of TXA2) after a single dose of Fruitflow® (65 mg tomato total active fraction), or 75 mg aspirin (ASA) and found a reduction of approximately 25% at 3 h and 37% at 5 h for WSTC and 66% for ASA after 5 h (26, 94). Our results indicate that sulforaphane seems more effective in reducing overall platelet aggregation at lower doses. In addition, the correlations between sulforaphane and thromboxane demonstrate causation, which is the last criterion in the evaluation of a scientific health claim dossier by EFSA (51, 101, 102). A logical next step for future research would therefore be to investigate the effects of sulforaphane on inhibition of platelet aggregation using LTA in subjects with platelet activation.
This study is the first to demonstrate that urinary 11-dehydro-TXB2 is a sensitive, easy-to-use, and suitable biomarker to investigate the effects of phytonutrients on platelet aggregation in young healthy participants who are metabolically challenged in a short period of time. This study is not without limitations. First, the glucoraphanin content of the active sprout material and placebo may have differed from product labels and may have been adversely affected by cultivation conditions, batch-to-batch variation, and preparation methods. Second, it is challenging to find an impeccable placebo in nutritional intervention studies, especially for whole foods in a double-blinded setting. In current study, the placebo had to match the broccoli sprouts in terms of nutrient value, except for sulforaphane content. Despite our efforts, the increased amount of β-carotene and some vitamins in the pea sprouts may have counteracted the expected post-challenge increase in 11-dehydro-TXB2 (79, 80). In addition, follow-up studies with a larger sample size will shed more light on the effects of interindividual genetic variability with respect to cruciferous vegetable consumption. Nevertheless, this study has increased our understanding of the antithrombotic effects of sulforaphane and can be used as supporting evidence for the scientific substantiation of claims on the reduction of platelet aggregation (95, 96). When follow-up studies on the effects of sulforaphane using LTA yield positive results, fresh broccoli sprouts or other produce containing enough sulforaphane per serving, could apply for the same authorized claim as WSTC.
This study demonstrated that a single administration of broccoli sprouts reduced urinary 11-dehydro-TXB2 levels by clinically relevant amounts in healthy participants exposed to a standardized caloric load. In addition, the correlations between sulforaphane and thromboxane indicate a causal relationship, which is not influenced by the co-ingestion of the metabolic challenge. 11-Dehydro-TXB2 shows promise as a non-invasive, sensitive, and suitable biomarker to investigate the acute effects of phytonutrients on platelet aggregation within hours. Genetic predisposition may influence the health effects of cruciferous vegetable consumption, but more research is needed to ultimately provide personalized dietary advice for consumers. This study forms the basis for a scientific substantiation of claims on the reduction of platelet aggregation for fresh produce containing sulforaphane in the future.
The data presented in the study are deposited in the European Variation Archive (EVA) at EMBL-EBI repository under accession number PRJEB63376.
The study protocol (NL77272.068.21) was approved by the Medical Ethics Review Committee of Maastricht University Medical Centre+ (MUMC+) and Maastricht University, Maastricht, the Netherlands. The patients/participants provided their written informed consent to participate in this study.
HS: conceptualization, investigation, formal analysis, visualization, and writing—original draft. EW, EK, MB, and JD: investigation and formal analysis. JB: investigation, formal analysis, and writing—review and editing. TL and FT: writing—review and editing. FO: methodology and writing—review and editing. ABa: conceptualization, writing—review and editing, and supervision. KS: conceptualization, data curation, methodology, visualization, and writing—review and editing. ABo: conceptualization, writing—review and editing, funding acquisition, and supervision. All authors contributed to the article and approved the submitted version.
This research was funded by the Dutch Topsector Tuinbouw & Uitgangsmaterialen, grant number TU1118.
The authors thank Diede Nijmeijer and Ardi Cengo for their contributions.
MB and JD were employed by Omnigen B.V.
The remaining authors declare that the research was conducted in the absence of any commercial or financial relationships that could be construed as a potential conflict of interest.
All claims expressed in this article are solely those of the authors and do not necessarily represent those of their affiliated organizations, or those of the publisher, the editors and the reviewers. Any product that may be evaluated in this article, or claim that may be made by its manufacturer, is not guaranteed or endorsed by the publisher.
The Supplementary material for this article can be found online at: https://www.frontiersin.org/articles/10.3389/fnut.2023.1204561/full#supplementary-material
1. O’Kennedy, N , Duss, R , and Duttaroy, AK . Dietary antiplatelets: a new perspective on the health benefits of the water-soluble tomato concentrate Fruitflow(®). Nutrients. (2021) 13:2184. doi: 10.3390/nu13072184
2. von Hundelshausen, P , and Weber, C . Platelets as immune cells: bridging inflammation and cardiovascular disease. Circ Res. (2007) 100:27–40. doi: 10.1161/01.RES.0000252802.25497.b7
3. Saldeen, P , Nilsson, IM , and Saldeen, T . Increased synthesis of thromboxane B2 and 6-keto-PGF1 alpha in hand veins from patients with deep venous thrombosis. Thromb Res. (1983) 32:461–7. doi: 10.1016/0049-3848(83)90256-6
4. Dogné, J-M , de Leval, X , Benoit, P , Delarge, J , and Masereel, B . Thromboxane A2 inhibition: therapeutic potential in bronchial asthma. Am J Respir Med Drugs Devices Other Interv. (2002) 1:11–7. doi: 10.1007/BF03257158
5. Eikelboom, JW , Hirsh, J , Weitz, JI , Johnston, M , Yi, Q , and Yusuf, S . Aspirin-resistant thromboxane biosynthesis and the risk of myocardial infarction, stroke, or cardiovascular death in patients at high risk for cardiovascular events. Circulation. (2002) 105:1650–5. doi: 10.1161/01.cir.0000013777.21160.07
6. Hamm, CW , Lorenz, RL , Bleifeld, W , Kupper, W , Wober, W , and Weber, PC . Biochemical evidence of platelet activation in patients with persistent unstable angina. J Am Coll Cardiol. (1987) 10:998–1004. doi: 10.1016/s0735-1097(87)80336-4
7. Mehta, JL , Lawson, D , Mehta, P , and Saldeen, T . Increased prostacyclin and thromboxane A2 biosynthesis in atherosclerosis. Proc Natl Acad Sci U S A. (1988) 85:4511–5. doi: 10.1073/pnas.85.12.4511
8. Hishinuma, T , Tsukamoto, H , Suzuki, K , and Mizugaki, M . Relationship between thromboxane/prostacyclin ratio and diabetic vascular complications. Prostaglandins Leukot Essent Fatty Acids. (2001) 65:191–6. doi: 10.1054/plef.2001.0310
9. Chen, SY , Yu, BJ , Liang, YQ , and Lin, WD . Platelet aggregation, platelet cAMP levels and thromboxane B2 synthesis in patients with diabetes mellitus. Chin Med J. (1990) 103:312–8.
10. Santilli, F , Formoso, G , Sbraccia, P , Averna, M , Miccoli, R , Di Fulvio, P, et al. Postprandial hyperglycemia is a determinant of platelet activation in early type 2 diabetes mellitus. J Thromb Haemost. (2010) 8:828–37. doi: 10.1111/j.1538-7836.2010.03742.x
11. Stroeve, JHM , van Wietmarschen, H , Kremer, BHA , van Ommen, B , and Wopereis, S . Phenotypic flexibility as a measure of health: the optimal nutritional stress response test. Genes Nutr. (2015) 10:13. doi: 10.1007/s12263-015-0459-1
12. Wopereis, S , Stroeve, JHM , Stafleu, A , Bakker, GCM , Burggraaf, J , van Erk, MJ, et al. Multi-parameter comparison of a standardized mixed meal tolerance test in healthy and type 2 diabetic subjects: the PhenFlex challenge. Genes Nutr. (2017) 12:21. doi: 10.1186/s12263-017-0570-6
13. van den Broek, TJ , Bakker, GCM , Rubingh, CM , Bijlsma, S , Stroeve, JHM , van Ommen, B, et al. Ranges of phenotypic flexibility in healthy subjects. Genes Nutr. (2017) 12:32. doi: 10.1186/s12263-017-0589-8
14. Hoevenaars, F , van der Kamp, J-W , van den Brink, W , and Wopereis, S . Next generation health claims based on resilience: the example of whole-grain wheat. Nutr. (2020) 12:12. doi: 10.3390/nu12102945
15. Ally, AI , and Horrobin, DF . Thromboxane A2 in blood vessel walls and its physiological significance: relevance to thrombosis and hypertension. Prostaglandins Med. (1980) 4:431–8. doi: 10.1016/0161-4630(80)90051-8
16. Ishizuka, T , Taniguchi, O , Yamamoto, M , Kajita, K , Nagashima, T , Takeda, N, et al. Thrombin-induced platelet aggregation, phosphoinositide metabolism and protein phosphorylation in NIDDM patients treated by diet, sulphonylurea or insulin. Diabetologia. (1994) 37:632–8. doi: 10.1007/BF00403384
17. Offermanns, S . Activation of platelet function through G protein–coupled receptors. Circ Res. (2006) 99:1293–304. doi: 10.1161/01.RES.0000251742.71301.16
18. Capra, V , Bäck, M , Angiolillo, DJ , Cattaneo, M , and Sakariassen, KS . Impact of vascular thromboxane prostanoid receptor activation on hemostasis, thrombosis, oxidative stress, and inflammation. J Thromb Haemost. (2014) 12:126–37. doi: 10.1111/jth.12472
19. Lordan, R , Tsoupras, A , and Zabetakis, I . Platelet activation and prothrombotic mediators at the nexus of inflammation and atherosclerosis: potential role of antiplatelet agents. Blood Rev. (2021) 45:100694. doi: 10.1016/j.blre.2020.100694
20. Lordkipanidzé, M , Pharand, C , Schampaert, E , Turgeon, J , Palisaitis, DA , and Diodati, JG . A comparison of six major platelet function tests to determine the prevalence of aspirin resistance in patients with stable coronary artery disease. Eur Heart J. (2007) 28:1702–8. doi: 10.1093/eurheartj/ehm226
21. Catella, F , Healy, D , Lawson, JA , and FitzGerald, GA . 11-Dehydrothromboxane B2: a quantitative index of thromboxane A2 formation in the human circulation. Proc Natl Acad Sci U S A. (1986) 83:5861–5. doi: 10.1073/pnas.83.16.5861
22. Di Minno, MND , Guida, A , Camera, M , Colli, S , Di Minno, G , and Tremoli, E . Overcoming limitations of current antiplatelet drugs: a concerted effort for more profitable strategies of intervention. Ann Med. (2011) 43:531–44. doi: 10.3109/07853890.2011.582137
23. Abbas, H , Kumar Nayudu, S , Ravi, M , Saad, M , Bathini, K , Ravi, P, et al. Does extended use of Clopidogrel-based dual anti-platelet therapy increase the risk of gastrointestinal bleeding? Gastroenterol Res. (2020) 13:146–9. doi: 10.14740/gr1285
24. Cámara, M , Fernández-Ruiz, V , Sánchez-Mata, M-C , Cámara, RM , Domínguez, L , and Sesso, HD . Scientific evidence of the beneficial effects of tomato products on cardiovascular disease and platelet aggregation. Front Nutr. (2022) 9:849841. doi: 10.3389/fnut.2022.849841
25. Tsoupras, A , Lordan, R , and Zabetakis, I . Thrombosis and COVID-19: the potential role of nutrition. Front Nutr. (2020) 7:583080. doi: 10.3389/fnut.2020.583080
26. O’Kennedy, N , Crosbie, L , Song, H-J , Zhang, X , Horgan, G , and Duttaroy, AK . A randomised controlled trial comparing a dietary antiplatelet, the water-soluble tomato extract Fruitflow, with 75 mg aspirin in healthy subjects. Eur J Clin Nutr. (2017) 71:723–30. doi: 10.1038/ejcn.2016.222
27. Mazarakis, N , Snibson, K , Licciardi, PV , and Karagiannis, TC . The potential use of L-sulforaphane for the treatment of chronic inflammatory diseases: a review of the clinical evidence. Clin Nutr. (2020) 39:664–75. doi: 10.1016/j.clnu.2019.03.022
28. Fahey, JW , Zhang, Y , and Talalay, P . Broccoli sprouts: an exceptionally rich source of inducers of enzymes that protect against chemical carcinogens. Proc Natl Acad Sci U S A. (1997) 94:10367–72. doi: 10.1073/pnas.94.19.10367
29. Atwell, LL , Hsu, A , Wong, CP , Stevens, JF , Bella, D , Yu, T-W, et al. Absorption and chemopreventive targets of sulforaphane in humans following consumption of broccoli sprouts or a myrosinase-treated broccoli sprout extract. Mol Nutr Food Res. (2015) 59:424–33. doi: 10.1002/mnfr.201400674
30. Oliviero, T , Verkerk, R , Vermeulen, M , and Dekker, M . In vivo formation and bioavailability of isothiocyanates from glucosinolates in broccoli as affected by processing conditions. Mol Nutr Food Res. (2014) 58:1447–56. doi: 10.1002/mnfr.201300894
31. Saha, S , Hollands, W , Teucher, B , Needs, PW , Narbad, A , Ortori, CA, et al. Isothiocyanate concentrations and interconversion of sulforaphane to erucin in human subjects after consumption of commercial frozen broccoli compared to fresh broccoli. Mol Nutr Food Res. (2012) 56:1906–16. doi: 10.1002/mnfr.201200225
32. Cramer, JM , Teran-Garcia, M , and Jeffery, EH . Enhancing sulforaphane absorption and excretion in healthy men through the combined consumption of fresh broccoli sprouts and a glucoraphanin-rich powder. Br J Nutr. (2012) 107:1333–8. doi: 10.1017/S0007114511004429
33. Hauder, J , Winkler, S , Bub, A , Rüfer, CE , Pignitter, M , and Somoza, V . LC-MS/MS quantification of sulforaphane and indole-3-carbinol metabolites in human plasma and urine after dietary intake of selenium-fortified broccoli. J Agric Food Chem. (2011) 59:8047–57. doi: 10.1021/jf201501x
34. Egner, PA , Chen, JG , Wang, JB , Wu, Y , Sun, Y , Lu, JH, et al. Bioavailability of sulforaphane from two broccoli sprout beverages: results of a short-term, cross-over clinical trial in Qidong, China. Cancer Prev Res. (2011) 4:384–95. doi: 10.1158/1940-6207.CAPR-10-0296
35. Hanlon, N , Coldham, N , Gielbert, A , Sauer, MJ , and Ioannides, C . Repeated intake of broccoli does not lead to higher plasma levels of sulforaphane in human volunteers. Cancer Lett. (2009) 284:15–20. doi: 10.1016/j.canlet.2009.04.004
36. Vermeulen, M , Klöpping-Ketelaars, IWAA , Van Den Berg, R , and Vaes, WHJ . Bioavailability and kinetics of sulforaphane in humans after consumption of cooked versus raw broccoli. J Agric Food Chem. (2008) 56:10505–9. doi: 10.1021/jf801989e
37. Rungapamestry, V , Duncan, AJ , Fuller, Z , and Ratcliffe, B . Effect of meal composition and cooking duration on the fate of sulforaphane following consumption of broccoli by healthy human subjects. Br J Nutr. (2007) 97:644–52. doi: 10.1017/S0007114507381403
38. Conaway, CC , Getahun, SM , Liebes, LL , Pusateri, DJ , Topham, DK , Botero-Omary, M, et al. Disposition of glucosinolates and sulforaphane in humans after ingestion of steamed and fresh broccoli. Nutr Cancer. (2000) 38:168–78. doi: 10.1207/S15327914NC382_5
39. Posner, GH , Cho, CG , Green, JV , Zhang, Y , and Talalay, P . Design and synthesis of bifunctional isothiocyanate analogs of sulforaphane: correlation between structure and potency as inducers of anticarcinogenic detoxication enzymes. J Med Chem. (1994) 37:170–6. doi: 10.1021/jm00027a021
40. Valgimigli, L , and Iori, R . Antioxidant and pro-oxidant capacities of ITCs. Environ Mol Mutagen. (2009) 50:222–37. doi: 10.1002/em.20468
41. Bryan, HK , Olayanju, A , Goldring, CE , and Park, BK . The Nrf2 cell defence pathway: Keap1-dependent and -independent mechanisms of regulation. Biochem Pharmacol. (2013) 85:705–17. doi: 10.1016/j.bcp.2012.11.016
42. Dong, Z , Shang, H , Chen, YQ , Pan, LL , Bhatia, M , and Sun, J . Sulforaphane protects pancreatic acinar cell injury by modulating Nrf2-mediated oxidative stress and NLRP3 inflammatory pathway. Oxidative Med Cell Longev. (2016) 2016:1–12. doi: 10.1155/2016/7864150
43. Traka, MH , Saha, S , Huseby, S , Kopriva, S , Walley, PG , Barker, GC, et al. Genetic regulation of glucoraphanin accumulation in Beneforté® broccoli. New Phytol. (2013) 198:1085–95. doi: 10.1111/nph.12232
44. Barba, FJ , Nikmaram, N , Roohinejad, S , Khelfa, A , Zhu, Z , and Koubaa, M . Bioavailability of Glucosinolates and their breakdown products: impact of processing. Front Nutr. (2016) 3:24. doi: 10.3389/fnut.2016.00024
45. Okunade, O , Niranjan, K , Ghawi, SK , Kuhnle, G , and Methven, L . Supplementation of the diet by exogenous myrosinase via mustard seeds to increase the bioavailability of sulforaphane in healthy human subjects after the consumption of cooked broccoli. Mol Nutr Food Res. (2018) 62:e1700980. doi: 10.1002/mnfr.201700980
46. Sivapalan, T , Melchini, A , Saha, S , Needs, PW , Traka, MH , Tapp, H, et al. Bioavailability of glucoraphanin and sulforaphane from high-glucoraphanin broccoli. Mol Nutr Food Res. (2018) 62:e1700911. doi: 10.1002/mnfr.201700911
47. Murillo, G , and Mehta, RG . Cruciferous vegetables and cancer prevention. Nutr Cancer. (2001) 41:17–28. doi: 10.1080/01635581.2001.9680607
48. Fahey, JW , Wehage, SL , Holtzclaw, WD , Kensler, TW , Egner, PA , Shapiro, TA, et al. Protection of humans by plant glucosinolates: efficiency of conversion of glucosinolates to isothiocyanates by the gastrointestinal microflora. Cancer Prev Res. (2012) 5:603–11. doi: 10.1158/1940-6207.CAPR-11-0538
49. Chuang, W-Y , Kung, P-H , Kuo, C-Y , and Wu, C-C . Sulforaphane prevents human platelet aggregation through inhibiting the phosphatidylinositol 3-kinase/Akt pathway. Thromb Haemost. (2013) 109:1120–30. doi: 10.1160/TH12-09-0636
50. Jayakumar, T , Chen, W-F , Lu, W-J , Chou, D-S , Hsiao, G , Hsu, C-Y, et al. A novel antithrombotic effect of sulforaphane via activation of platelet adenylate cyclase: ex vivo and in vivo studies. J Nutr Biochem. (2013) 24:1086–95. doi: 10.1016/j.jnutbio.2012.08.007
51. Medina, S , Domínguez-Perles, R , Moreno, DA , García-Viguera, C , Ferreres, F , Gil, JI, et al. The intake of broccoli sprouts modulates the inflammatory and vascular prostanoids but not the oxidative stress-related isoprostanes in healthy humans. Food Chem. (2015) 173:1187–94. doi: 10.1016/j.foodchem.2014.10.152
52. World Medical Association . World Medical Association declaration of Helsinki: ethical principles for medical research involving human subjects. JAMA. (2013) 310:2191–4. doi: 10.1001/jama.2013.281053
53. Hoevenaars, FPM , Esser, D , Schutte, S , Priebe, MG , Vonk, RJ , Van Den Brink, WJ, et al. Whole grain wheat consumption affects postprandial inflammatory response in a randomized controlled trial in overweight and obese adults with mild hypercholesterolemia in the graandioos study. J Nutr. (2019) 149:2133–44. doi: 10.1093/jn/nxz177
54. Egner, PA , Kensler, TW , Chen, J-G , Gange, SJ , Groopman, JD , and Friesen, MD . Quantification of sulforaphane mercapturic acid pathway conjugates in human urine by high-performance liquid chromatography and isotope-dilution tandem mass spectrometry. Chem Res Toxicol. (2008) 21:1991–6. doi: 10.1021/tx800210k
55. Turner, S , Armstrong, LL , Bradford, Y , Carlson, CS , Crawford, DC , Crenshaw, AT, et al. Quality control procedures for genome-wide association studies. Curr Protoc Hum Genet. (2011) Chapter 1:Unit1.19. doi: 10.1002/0471142905.hg0119s68
56. Browning, BL , Zhou, Y , and Browning, SR . A one-penny imputed genome from next-generation reference panels. Am J Hum Genet. (2018) 103:338–48. doi: 10.1016/j.ajhg.2018.07.015
57. Auton, A , Abecasis, GR , Altshuler, DM , Durbin, RM , Abecasis, GR , Bentley, DR, et al. A global reference for human genetic variation. Nature. (2015) 526:68–74. doi: 10.1038/nature15393
58. Boddupalli, S , Mein, JR , Lakkanna, S , and James, DR . Induction of phase 2 antioxidant enzymes by broccoli sulforaphane: perspectives in maintaining the antioxidant activity of vitamins A, C, and E. Front Genet. (2012) 3:7. doi: 10.3389/fgene.2012.00007
59. Lampe, JW , and Peterson, S . Brassica, biotransformation and cancer risk: genetic polymorphisms alter the preventive effects of cruciferous vegetables. J Nutr. (2002) 132:2991–4. doi: 10.1093/jn/131.10.2991
60. Houghton, CA . Sulforaphane: its “coming of age” as a clinically relevant nutraceutical in the prevention and treatment of chronic disease. Oxidative Med Cell Longev. (2019) 2019:1–27. doi: 10.1155/2019/2716870
61. Joseph, MA , Moysich, KB , Freudenheim, JL , Shields, PG , Bowman, ED , Zhang, Y, et al. Cruciferous vegetables, genetic polymorphisms in glutathione S-transferases M1 and T1, and prostate cancer risk. Nutr Cancer. (2004) 50:206–13. doi: 10.1207/s15327914nc5002_11
62. Ambrosone, CB , and Tang, L . Cruciferous vegetable intake and cancer prevention: role of nutrigenetics. Cancer Prev Res. (2009) 2:298–300. doi: 10.1158/1940-6207.CAPR-09-0037
63. Traka, M , Gasper, AV , Melchini, A , Bacon, JR , Needs, PW , Frost, V, et al. Broccoli consumption interacts with GSTM1 to perturb oncogenic signalling pathways in the prostate. PLoS One. (2008) 3:e2568. doi: 10.1371/journal.pone.0002568
64. Gasper, AV , Al-Janobi, A , Smith, JA , Bacon, JR , Fortun, P , Atherton, C, et al. Glutathione S-transferase M1 polymorphism and metabolism of sulforaphane from standard and high-glucosinolate broccoli. Am J Clin Nutr. (2005) 82:1283–91. doi: 10.1093/ajcn/82.6.1283
65. Seow, A , Vainio, H , and Yu, MC . Effect of glutathione-S-transferase polymorphisms on the cancer preventive potential of isothiocyanates: an epidemiological perspective. Mutat. Res. (2005) 592:58–67. doi: 10.1016/j.mrfmmm.2005.06.004
66. Steck, SE , Gammon, MD , Hebert, JR , Wall, DE , and Zeisel, SH . GSTM1, GSTT1, GSTP1, and GSTA1 polymorphisms and urinary isothiocyanate metabolites following broccoli consumption in humans. J. Nutr. (2007) 137:904–9. doi: 10.1093/jn/137.4.904
67. Lin, HJ , Johansson, AS , Stenberg, G , Materi, AM , Park, JM , Dai, A, et al. Naturally occurring Phe151Leu substitution near a conserved folding module lowers stability of glutathione transferase P1-1. Biochim. Biophys. Acta. (2003) 1649:16–23. doi: 10.1016/s1570-9639(03)00149-3
68. Peterson, S , Bigler, J , Horner, NK , Potter, JD , and Lampe, JW . Cruciferae interact with the UGT1A1*28 polymorphism to determine serum bilirubin levels in humans. J. Nutr. (2005) 135:1051–5. doi: 10.1093/jn/135.5.1051
69. Chang, J-L , Bigler, J , Schwarz, Y , Li, SS , Li, L , King, IB, et al. UGT1A1 polymorphism is associated with serum bilirubin concentrations in a randomized, controlled, fruit and vegetable feeding trial. J. Nutr. (2007) 137:890–7. doi: 10.1093/jn/137.4.890
70. Navarro, SL , Peterson, S , Chen, C , Makar, KW , Schwarz, Y , King, IB, et al. Cruciferous vegetable feeding alters UGT1A1 activity: diet- and genotype-dependent changes in serum bilirubin in a controlled feeding trial. Cancer Prev. Res. (2009) 2:345–52. doi: 10.1158/1940-6207.CAPR-08-0178
71. Zhao, H , Lin, J , Grossman, HB , Hernandez, LM , Dinney, CP , and Wu, X . Dietary isothiocyanates, GSTM1, GSTT1, NAT2 polymorphisms and bladder cancer risk. Int. J. cancer. (2007) 120:2208–13. doi: 10.1002/ijc.22549
72. Sayers, EW , Bolton, EE , Brister, JR , Canese, K , Chan, J , Comeau, DC, et al. Database resources of the national center for biotechnology information. Nucleic Acids Res. (2022) 50:D20–6. doi: 10.1093/nar/gkab1112
73. Lee, S , Wheeler, MM , Patterson, K , McGee, S , Dalton, R , Woodahl, EL, et al. Stargazer: a software tool for calling star alleles from next-generation sequencing data using CYP2D6 as a model. Genet Med. (2019) 21:361–72. doi: 10.1038/s41436-018-0054-0
74. Purcell, S , Neale, B , Todd-Brown, K , Thomas, L , Ferreira, MAR , Bender, D, et al. PLINK: a tool set for whole-genome association and population-based linkage analyses. Am J Hum Genet. (2007) 81:559–75. doi: 10.1086/519795
75. Blomhoff, R , and Blomhoff, HK . Overview of retinoid metabolism and function. J Neurobiol. (2006) 66:606–30. doi: 10.1002/neu.20242
76. Dollé, P , Fraulob, V , Kastner, P , and Chambon, P . Developmental expression of murine retinoid X receptor (RXR) genes. Mech Dev. (1994) 45:91–104. doi: 10.1016/0925-4773(94)90023-x
77. Giguere, V , Ong, ES , Segui, P , and Evans, RM . Identification of a receptor for the morphogen retinoic acid. Nature. (1987) 330:624–9. doi: 10.1038/330624a0
78. Petkovich, M , Brand, NJ , Krust, A , and Chambon, P . A human retinoic acid receptor which belongs to the family of nuclear receptors. Nature. (1987) 330:444–50. doi: 10.1038/330444a0
79. Na, SY , Kang, BY , Chung, SW , Han, SJ , Ma, X , Trinchieri, G, et al. Retinoids inhibit interleukin-12 production in macrophages through physical associations of retinoid X receptor and NFkappaB. J Biol Chem. (1999) 274:7674–80. doi: 10.1074/jbc.274.12.7674
80. Kojok, K , El-Kadiry, AE-H , and Merhi, Y . Role of NF-κB in platelet function. Int J Mol Sci. (2019) 20:4185. doi: 10.3390/ijms20174185
81. Edmands, WMB , Beckonert, OP , Stella, C , Campbell, A , Lake, BG , Lindon, JC, et al. Identification of human urinary biomarkers of cruciferous vegetable consumption by metabonomic profiling. J Proteome Res. (2011) 10:4513–21. doi: 10.1021/pr200326k
82. Clarke, JD , Hsu, A , Riedl, K , Bella, D , Schwartz, SJ , Stevens, JF, et al. Bioavailability and inter-conversion of sulforaphane and erucin in human subjects consuming broccoli sprouts or broccoli supplement in a cross-over study design. Pharmacol Res. (2011) 64:456–63. doi: 10.1016/j.phrs.2011.07.005
83. Ross, D , and Siegel, D . NAD(P)H:quinone oxidoreductase 1 (NQO1, DT-diaphorase), functions and pharmacogenetics. Methods Enzymol. (2004) 382:115–44. doi: 10.1016/S0076-6879(04)82008-1
84. Kiyohara, C , Yoshimasu, K , Takayama, K , and Nakanishi, Y . NQO1, MPO, and the risk of lung cancer: a HuGE review. Genet Med Off J Am Coll Med Genet. (2005) 7:463–78. doi: 10.1097/01.gim.0000177530.55043.c1
85. Fowke, JH , Shu, X-O , Dai, Q , Shintani, A , Conaway, CC , Chung, F-L, et al. Urinary isothiocyanate excretion, brassica consumption, and gene polymorphisms among women living in Shanghai, China. Cancer Epidemiol Biomarkers Prev. (2003) 12:1536–9.
86. Spitz, MR , Duphorne, CM , Detry, MA , Pillow, PC , Amos, CI , Lei, L, et al. Dietary intake of isothiocyanates: evidence of a joint effect with glutathione S-transferase polymorphisms in lung cancer risk. Cancer Epidemiol. Biomarkers Prev. (2000) 9:1017–20.
87. Wang, LI , Giovannucci, EL , Hunter, D , Neuberg, D , Su, L , and Christiani, DC . Dietary intake of cruciferous vegetables, glutathione S-transferase (GST) polymorphisms and lung cancer risk in a Caucasian population. Cancer Causes Control. (2004) 15:977–85. doi: 10.1007/s10552-004-1093-1
88. Shapiro, TA , Fahey, JW , Wade, KL , Stephenson, KK , and Talalay, P . Chemoprotective glucosinolates and isothiocyanates of broccoli sprouts: metabolism and excretion in humans. Cancer Epidemiol Biomark Prev. (2001) 10:501–8.
89. Lampe, JW . Interindividual differences in response to plant-based diets: implications for cancer risk. Am J Clin Nutr. (2009) 89:1553S–7S. doi: 10.3945/ajcn.2009.26736D
90. Ross, D , and Siegel, D . The diverse functionality of NQO1 and its roles in redox control. Redox Biol. (2021) 41:101950. doi: 10.1016/j.redox.2021.101950
91. Dinkova-Kostova, AT , and Talalay, P . NAD(P)H:quinone acceptor oxidoreductase 1 (NQO1), a multifunctional antioxidant enzyme and exceptionally versatile cytoprotector. Arch Biochem Biophys. (2010) 501:116–23. doi: 10.1016/j.abb.2010.03.019
92. Lushchak, VI . Glutathione homeostasis and functions: potential targets for medical interventions. J Amino Acids. (2012) 2012:736837. doi: 10.1155/2012/736837
93. O’Kennedy, N , Raederstorff, D , and Duttaroy, AK . Fruitflow(®): the first European food safety authority-approved natural cardio-protective functional ingredient. Eur J Nutr. (2017) 56:461–82. doi: 10.1007/s00394-016-1265-2
94. European Food Safety Authority . Water-soluble tomato concentrate (WSTC I and II) and platelet aggregation. EFSA J. (2009) 7:1101. doi: 10.2903/j.efsa.2009.1101
95. EFSA Panel on Dietetic Products, Nutrition and Allergies (EFSA NDA Panel)Turck, D , Bresson, J-L , Burlingame, B , Dean, T , Fairweather-Tait, S, et al. Guidance for the scientific requirements for health claims related to antioxidants, oxidative damage and cardiovascular health. EFSA J. (2018) 16:e05136. doi: 10.2903/j.efsa.2018.5136
96. Cattaneo, M , Cerletti, C , Harrison, P , Hayward, CPM , Kenny, D , Nugent, D, et al. Recommendations for the standardization of light transmission aggregometry: a consensus of the working party from the platelet physiology subcommittee of SSC/ISTH. J Thromb Haemost. (2013) 11:1183–9. doi: 10.1111/jth.12231
97. Michelson, AD . Platelet function testing in cardiovascular diseases. Circulation. (2004) 110:e489–93. doi: 10.1161/01.CIR.0000147228.29325.F9
98. Harrison, P . Progress in the assessment of platelet function. Br J Haematol. (2000) 111:733–44. doi: 10.1111/j.1365-2141.2000.02269.x
99. Nicholson, NS , Panzer-Knodle, SG , Haas, NF , Taite, BB , Szalony, JA , Page, JD, et al. Assessment of platelet function assays. Am Heart J. (1998) 135:S170–8. doi: 10.1016/s0002-8703(98)70245-5
100. Gum, PA , Kottke-Marchant, K , Welsh, PA , White, J , and Topol, EJ . A prospective, blinded determination of the natural history of aspirin resistance among stable patients with cardiovascular disease. J Am Coll Cardiol. (2003) 41:961–5. doi: 10.1016/s0735-1097(02)03014-0
101. The European Commission Commission Regulation (EU) No 432/2012. Off J Eur Union. (2012) 13:281–320.
Keywords: immunothrombosis, inflammation, dietary antiplatelets, phytonutrients, thromboxanes, nutrition, non-communicable diseases
Citation: van Steenwijk HP, Winter E, Knaven E, Brouwers JF, van Baardwijk M, van Dalum JB, Luijendijk TJC, van Osch FHM, Troost FJ, Bast A, Semen KO and de Boer A (2023) The beneficial effect of sulforaphane on platelet responsiveness during caloric load: a single-intake, double-blind, placebo-controlled, crossover trial in healthy participants. Front. Nutr. 10:1204561. doi: 10.3389/fnut.2023.1204561
Received: 12 April 2023; Accepted: 15 June 2023;
Published: 06 July 2023.
Edited by:
Vijaya Juturu, Independent Researcher, Flemington, NJ, United StatesReviewed by:
Susan Sergeant, Wake Forest University, United StatesCopyright © 2023 van Steenwijk, Winter, Knaven, Brouwers, van Baardwijk, van Dalum, Luijendijk, van Osch, Troost, Bast, Semen and de Boer. This is an open-access article distributed under the terms of the Creative Commons Attribution License (CC BY). The use, distribution or reproduction in other forums is permitted, provided the original author(s) and the copyright owner(s) are credited and that the original publication in this journal is cited, in accordance with accepted academic practice. No use, distribution or reproduction is permitted which does not comply with these terms.
*Correspondence: Hidde P. van Steenwijk, aC52YW5zdGVlbndpamtAbWFhc3RyaWNodHVuaXZlcnNpdHkubmw=
†ORCID: Hidde P. van Steenwijk https://orcid.org/0000-0001-8409-5753
Disclaimer: All claims expressed in this article are solely those of the authors and do not necessarily represent those of their affiliated organizations, or those of the publisher, the editors and the reviewers. Any product that may be evaluated in this article or claim that may be made by its manufacturer is not guaranteed or endorsed by the publisher.
Research integrity at Frontiers
Learn more about the work of our research integrity team to safeguard the quality of each article we publish.