- 1Hospital of Stomatology, Jilin University, Changchun, China
- 2Jilin Provincial Key Laboratory of Tooth Development and Bone Remodeling, Changchun, China
“Medicine food homology” (MFH) is a term with a lengthy history. It refers to the fact that a lot of traditional natural products have both culinary and therapeutic benefits. The antibacterial, anti-inflammatory and anticancer effects of MFH plants and their secondary metabolites have been confirmed by numerous research. A bacterially generated inflammatory illness with a complicated pathophysiology, periodontitis causes the loss of the teeth’s supporting tissues. Several MFH plants have recently been shown to have the ability to prevent and treat periodontitis, which is exhibited by blocking the disease’s pathogens and the virulence factors that go along with them, lowering the host’s inflammatory reactions and halting the loss of alveolar bone. To give a theoretical foundation for the creation of functional foods, oral care products and adjuvant therapies, this review has especially explored the potential medicinal benefit of MFH plants and their secondary metabolites in the prevention and treatment of periodontitis.
1. Introduction
A multifactorial microbial infectious disease known as periodontitis is characterized by gingival bleeding, swelling, attachment loss and bone absorption, as well as other destructive changes in the periodontium that finally lead to tooth loss, decreased chewing function, changes in food intake or eating habits and a reduction in the quality of the patient’s life (1). Simply put, the intricate interaction between subgingival microorganisms and the host immune system leads to periodontitis. The incidence of periodontitis has increased by 83.4% in the last 10 years, and population growth and aging are raising the disease’s global burden (2). In addition, studies have revealed that periodontitis is linked to several different health conditions throughout the body, including atherosclerosis (3), diabetes (4), cancer (5) and respiratory problems (6).
Traditional Chinese medicine has used the term “MFH” since the beginning of time. Eating when one is hungry is treated as taking medicine and given to the patient as medicine, which is a statement that reflects the concept of “MFH” made in “Huang Di Nei Jing” (7). The term “MFH plants” alludes to the fact that many Chinese medicines double as food and medication. In addition, many traditional Chinese medicines can also be used in functional foods that are suitable for specific groups. Although functional food cannot replace medicine, it can regulate human bodily functions and help maintain and promote human health (8). Furthermore, many secondary metabolites found in MFH plants have been demonstrated to have anti-inflammatory (9), antibacterial (10) and anticancer (11)effects, indicating the potential use of MFH plants in disease prevention and medical care.
The idea of “food therapy” has recently been used in the preservation of periodontal health. Several studies have noted that the body can get anti-inflammatory and antioxidant substances from the diet, like polyphenols and flavonoids, that the body cannot generate on its own (12). Several MFH plants are now useful against periodontitis in a variety of ways, including antibacterial action against periodontal pathogens, decreasing host inflammatory response and improvement of alveolar bone loss (Figure 1). In order to offer a theoretical framework for the use of pharmacological and food homology in periodontitis, the mechanism and research progress of MFH plants and their secondary metabolites against periodontitis were categorized and explained in this paper.
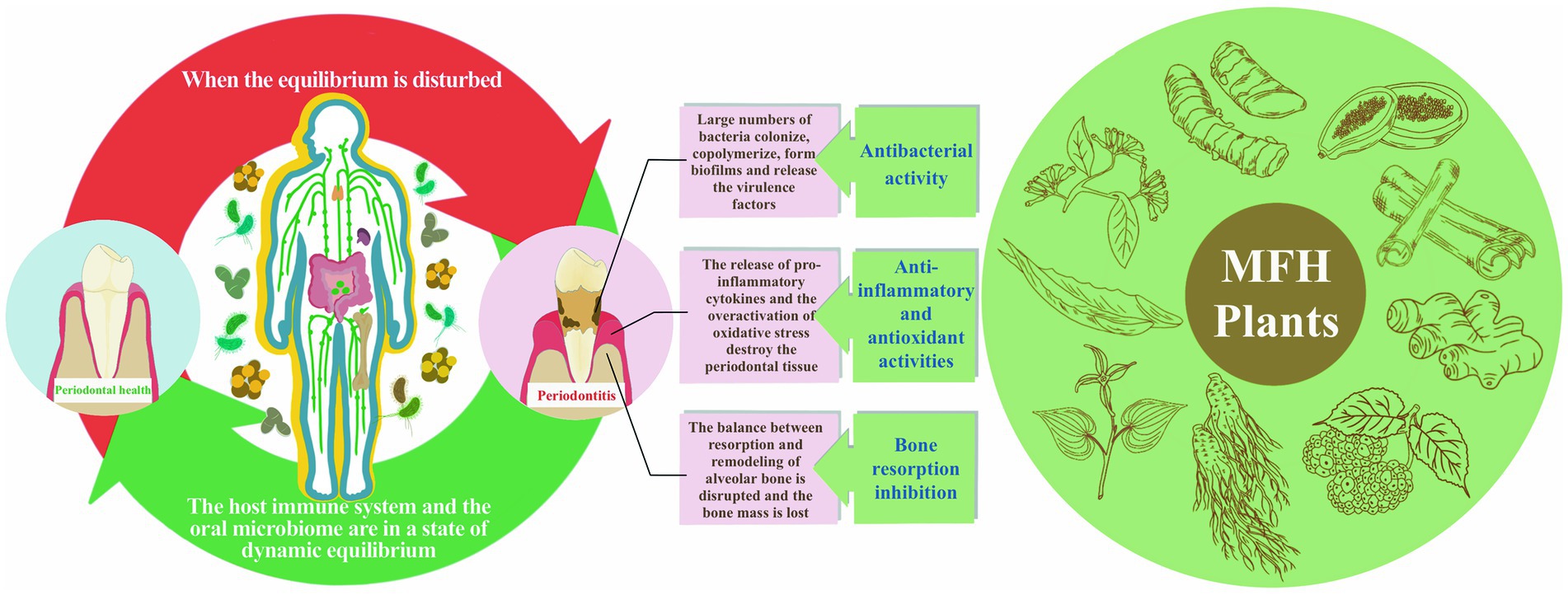
Figure 1. The etiology and pathogenic process of periodontitis as well as MFH plants’ anti-periodontitis effects.
2. Pathogenic mechanism of periodontitis
The most prevalent form of bacteria in the oral microenvironment is plaque biofilm. It is primarily present on the outside of oral soft and hard tissues or prostheses and is made up of a very complex extracellular polymeric matrix community. Subgingival plaque is a crucial contributor to the etiology of periodontitis and is distinguished from supragingival plaque by the gingival margin. The rich substances in the gingival fluid serve as the essential nutrient supply for bacteria, and the gingival sulcus offers a rather stable dwelling environment for bacteria. The amount and makeup of the bacterial population in plaque vary dynamically depending on the host’s state of health or sickness so that the environment in plaque is not constant (13). In contrast to the healthy condition, the subgingival bacterial community will be much more diverse and abundant in diseased periodontal tissue. The proportion of some populations, such as those pathogens linked to periodontitis, will also be relatively higher (14). Studies have shown that Porphyromonas gingivalis (P. gingivalis), Tannerella forsythia, Treponema, Fusobacterium nucleatum (F. nucleatum), Prevotella intermedia (P. intermedia), Actinobacteria, Pseudomonas aeruginosa (P. aeruginosa) and so on (15–17), has been confirmed to have a strong correlation with the onset of periodontitis. P. gingivalis, Tannerella forsythia, and Treponema, also known as the red complex, have the strongest correlation with periodontitis (18). Even at low concentrations, these bacteria can cause inflammatory reactions in the periodontal tissues, yet these reactions are consistent with the tissues’ defensive capabilities, allowing the periodontal tissues to continue to function normally (19). However, when this balance is upset by some factors, such as poor host oral hygiene, bad habits like smoking, or systemic diseases, the host periodontal tissue’s defensive capability is significantly diminished, which promotes the growth of some periodontal pathogens and makes them absolutely dominant in quantity and abundance, as well as has an impact on the host-microbial crosstalk (20). The microbiota is encouraged to change into a more pathogenic state when the host’s periodontal tissues experience an inflammatory reaction (21). Additionally, some bacterial structural elements, biomacromolecules and byproducts are cytotoxic and aid in bacterial colonization, copolymerization, biofilm formation, nutrient uptake, host cell invasion and escape ability, among other processes that are thought to participate in the inflammatory response of periodontitis (22–24). According to certain studies, a healthy, stable biological community is less likely to cause an inflammatory response in periodontal tissue than an ecologically unbalanced population rich in virulence factors (25). Moreover, the etiology of systemic disorders may be influenced by the ectopic colonization or circulation of periodontal bacteria, virulence factors, or specific mediators (26, 27).
Although bacteria are the initial causes of periodontal tissue inflammation and injury, they are not the primary component driving the development of the disease (28). When some cells and receptors in the host periodontal tissue recognize and kill invading pathogens, they also cause damage to the periodontal tissue. The equilibrium between tissue breakdown and regeneration is upset when the immune response is overactive and activates to levels above what is considered normal. This intensifies organizational destruction. Host immunity consists mainly of congenital and acquired immunity (29). Bacterial metabolites stimulate epithelial cells to produce pro-inflammatory cytokines and neurons release neuropeptides to induce vasodilation and promote neutrophil migration to the inflammatory site of the periodontal tissue to resist pathogen invasion. If the infection is not eliminated, it can lead to early lesions, mainly characterized by gingival hyperplasia, bleeding and increased crevicular fluid flow. Innate immunity progressively changes into acquired immunity as the severity of gingivitis rises, and this process is mediated by macrophages, plasma cells and T and B lymphocytes. Blood circulation is hampered and collagen fibers keep dissolving. Mild gingivitis now advances to moderate to severe gingivitis, and it also appears to change color and shape at this stage. Gingivitis develops into periodontitis as the inflammation progresses deeper into the deep tissues, leading to loss of attachment and dental bone resorption (30). In the aforementioned process, cytokine release has the potential to directly destroy periodontal tissues in an irreversible manner as well as indirectly destroy them by altering intracellular signaling and gene expression (31). Aside from killing harmful bacteria, the respiratory burst of neutrophils can result in excessive production of reactive oxygen species (ROS) and matrix metalloproteinases, which can cause an oxidative imbalance in periodontal tissues and permanent tissue deterioration (32). The many immune response mechanisms used by the various cell types in the periodontitis immune response intersect and overlap, eventually generating the immunity/inflammation cascade reaction process, which leads to the complexity and multi-level pathogenesis of periodontitis.
3. Dietary intervention in periodontitis
Many researchers have supported the fact that diet quality can either promote or inhibit periodontal health. A healthy plant-based diet decreased the incidence of periodontitis and was favorably correlated with antibody levels, according to a cross-sectional study (33). Anti-inflammatory diets may help people with periodontitis improve their periodontal condition and reduce tooth loss, according to some research (34, 35). Cross-sectional research of Hispanic or Latino populations between the ages of 18 and 74 years revealed that those who consumed more whole grains, fruits, and vegetables and less red or processed meat had lower incidences of severe periodontitis (36). Sugar, snacks, and fast food, especially those high in processed carbohydrates, may promote the development of periodontitis by affecting the biofilm formation in the mouth (37). The buildup of oral biofilms is not decreased by a healthy diet, which includes fruits, dietary fiber, vegetables, and dairy products, but a good diet can encourage the growth of non-pathogenic microbial communities by controlling oral microbial populations. For instance, in saliva samples from obese people, the Mediterranean diet decreased the relative abundance of P. gingivalis, P. intermedia, and Treponema but did not affect the makeup of the overall salivary microbial community (38). However, there are difficulties in determining a causal link between dietary variables and periodontitis from the above-mentioned cross-sectional investigations. Several theories on the connection between diet and periodontitis have also been advanced by researchers. The Western diet, which predominantly includes processed meat, butter, high-fat dairy products and so on, was only linked to an increased risk of periodontitis among obese individuals, according to a prospective study of 34,940 men (39). Systemic inflammation, insulin resistance, and metabolic disorders associated with obesity may be key factors in the increased risk of periodontitis (40). In addition, obesity is significantly associated with diabetes, and numerous studies have shown that diabetes has a significant bidirectional promoting relationship with periodontitis (41). As everyone knows, dietary intervention is one of the most fundamental forms of treatment for diabetes and obesity. Therefore, we hypothesize that dietary intervention influences periodontal health through many mechanisms and has the ability to prevent periodontal damage brought on by diabetes and obesity. In recent years, significant advancements have been made by MFH plants in the treatment and management of diabetes and its complicating disease (8). MFH plants also have great potential in the prevention and treatment of periodontitis which is one of the complications of diabetes. Its dual use as medicine and food makes it safe, widely available, low cost and remarkable effect, which gives a fresh concept and approach to periodontitis dietaryintervention.
4. Antibacterial effects and the inhibition of bacteria-related virulence factors of MFH plants and their secondary metabolites
Many secondary MFH plant metabolites or extracts have demonstrated direct antibacterial action against periodontal infections. The most popular evaluation index is minimum inhibitory concentration (MIC). The MIC is the minimum concentration of an antibacterial agent required to totally prevent test strains of organisms from growing visibly under tightly controlled in vitro circumstances. It serves as an indicator of how sensitive bacteria are to antibiotics (42). Table 1 displays the findings of the MIC analysis for the MFH plant extract, secondary metabolites and associated products. The main mechanisms by which MFH plants exert their antibacterial effects on bacteria include the breakdown of bacterial cell walls or membranes as well as interference with the synthesis of DNA and other vital biological macromolecules. The key bioactive components of MFH plants’ basic chemical structure are directly related to this inhibitory function.
Additionally, MFH plants and their secondary metabolites can reduce bacterial viability. Instead of immediately killing and suppressing bacteria under the sub-MIC concentration, MFH plants are more likely to influence bacterial copolymerization, adhesion, biofilm formation, signal transmission, and nutrition intake by decreasing virulence factors associated with their survival and invasion. A specific component or metabolite of the bacteria may be the source of the virulence factor they create. It can encourage the inflammatory response of periodontal tissue and ultimately result in tissue loss in addition to aiding bacterial survival (23). Table 2 displays the correlational researches of the MFH plant extracts, secondary metabolites and associated products inhibiting the virulence factors of P. gingivalis. P. gingivalis, a kind of gram-negative anaerobic bacteria, has been linked to the etiology of periodontitis, which is the primary subject of investigations on the virulence factors connected to periodontal infections at the moment. Animal models exposed to P. gingivalis develop inflammatory reactions and lose alveolar bone. The virulence factors present in P. gingivalis include pili, gingipains, outer membrane vesicles (OMVs) and hemagglutinin (56, 57). The fimA gene encodes the protein FimA, which can be polymerized to produce pili and is involved in bacterial invasion of host cells, copolymerization, colonization and biofilm formation (58). By destroying periodontal tissue’s constituents, gingipains, such as the two kinds of arginine-specific gingipains (RgpA, RgpB) and the lysine-specific gingipains (Kgp) encoded by rgpA, rgpB and kgp, can hasten the start and progression of periodontitis (59). The P. gingivalis hemagglutinin may bind heme and hemoglobin, which is helpful for bacterial survival and the spread of infection. The hemagglutinin-encoding genes, hagA and hagB, are also strongly linked to the development of biofilms (60). Furthermore, A variety of virulence factors are enriched in OMVs, which include outer membrane proteins, lipopolysaccharide (LPS), phospholipids, DNA and cytoplasm (61).
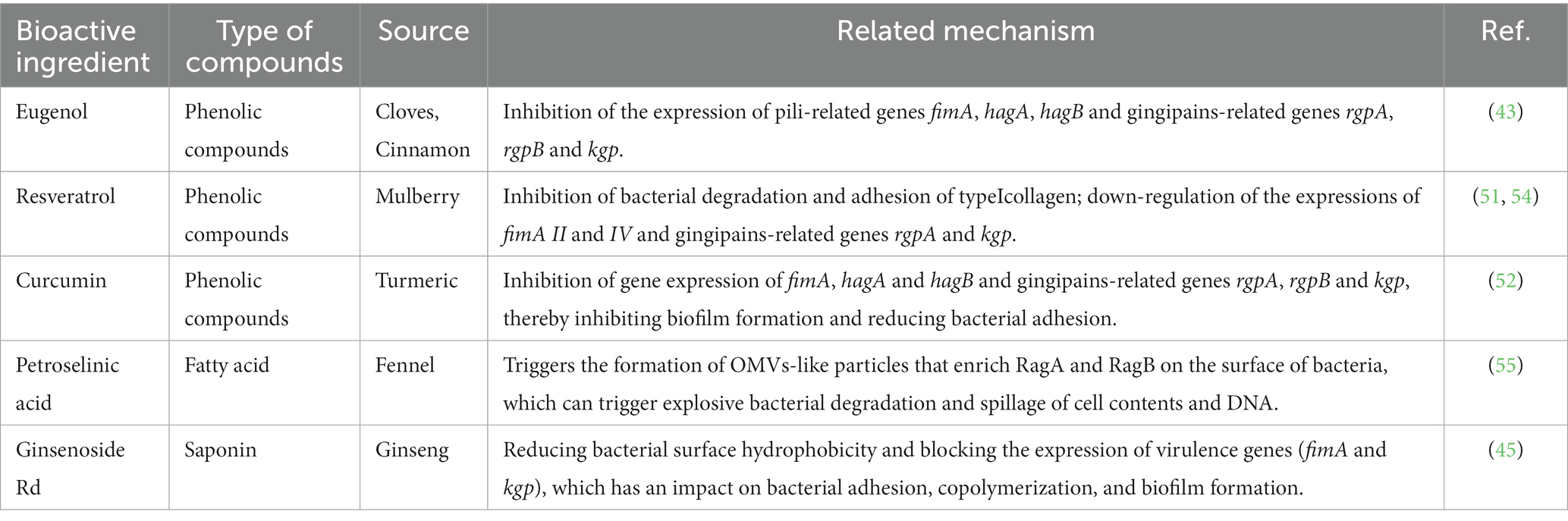
Table 2. The ingredients from MFH plants and their inhibitory mechanism against virulence factors of P.gingivalis.
4.1. Phenolic compound
Phenolic compounds are a typical class of active ingredient found in a variety of natural plants. They have at least one aromatic ring structure linking one hydroxyl group. Three categories are frequently used to categorize phenolic compounds: lignans and tannins, which are polyphenols; low phenolic oligophenols including flavonoids, stilbenes, and coumarins; and monophenols (62, 63). Numerous studies have established that phenolic compounds have an antibacterial impact, and some researchers have outlined the antibacterial mechanisms in detail. The current generally held beliefs can be summed up as follows: 1. The phenolic compounds alter cell permeability and causes leakage of cellular contents by interacting with bacterial cell walls or membranes, as well as surface proteins; 2. Reactive oxygen species (ROS) are produced by phenolic chemicals, which enhances oxidative stress in bacterial cells; 3. Phenolic compounds prevent the synthesis of bacterial-related biological macromolecules, which inhibits the bacteria’s development and metabolism; 4. Phenolic substances prevent DNA and ATP from being synthesized; 5. The production of proteins or virulence factors necessary for bacterial survival and biofilm formation are weakened by phenolic chemicals (64). The positions and amounts of the hydroxyl and methoxy groups (65–67), as well as the amounts and locations of hydrophobic substituents such as isopentenyl, alkylamino, and alkyl chains (68, 69), are all intimately related to the aforementioned activities. Figure 2 depicts the chemical composition of phenolic compounds found in MFH plants that have antibacterial properties.
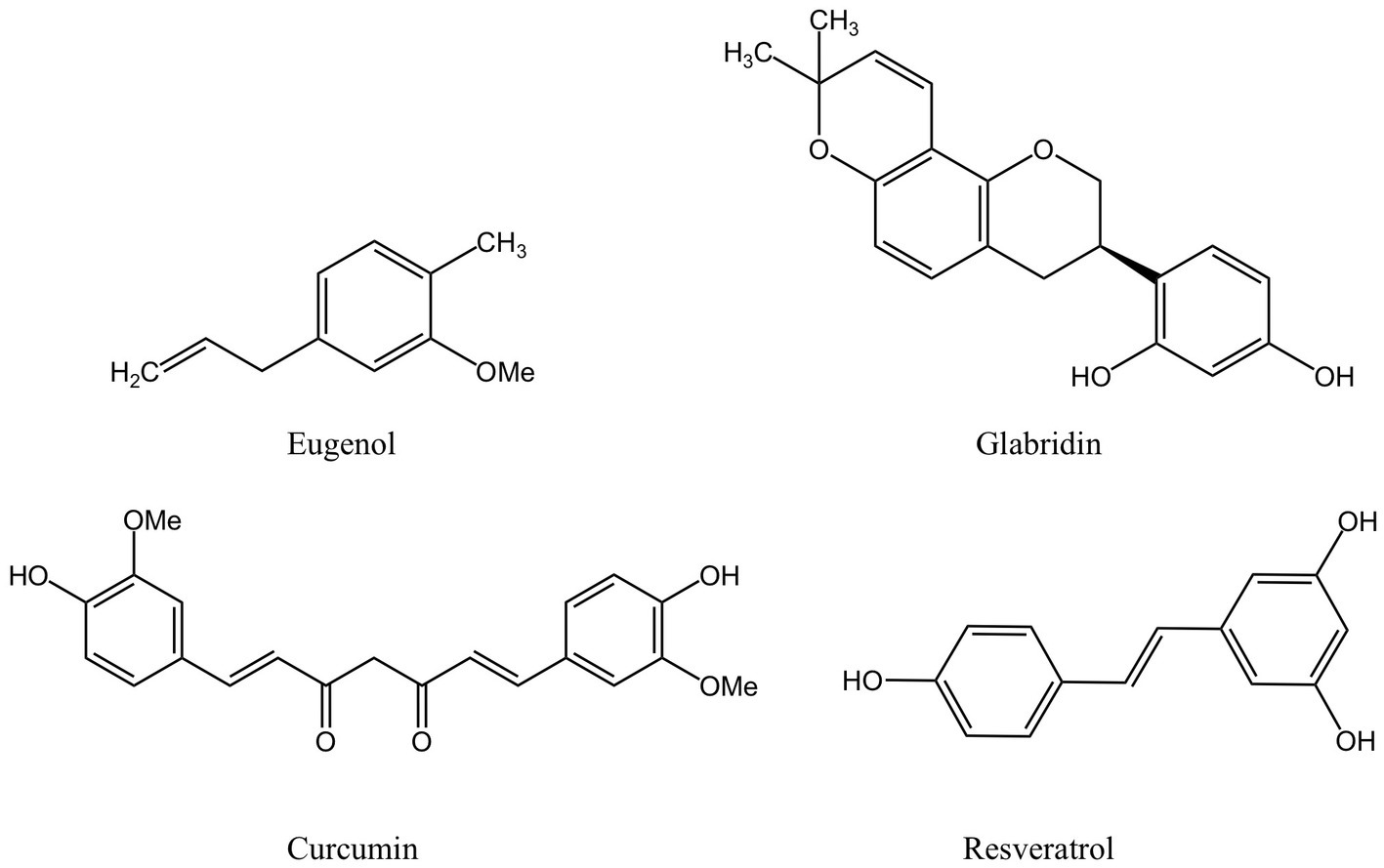
Figure 2. Phenolic compounds derived from MFH plants with antibacterial properties (created with KingDraw chemical structure editor).
The primary active component of the essential oils of several MFH plants, including Syringa aromaticum (cloves) and Cinnamomum cassia (cinnamon), is eugenol, a widely available monophenol molecule (70, 71). At MIC concentrations, eugenol can reduce the planktonic activity of P. gingivalis in a time-dependent manner. After being exposed to eugenol, P.gingivalis cells exhibited atrophic changes and severe cell membrane destruction, as revealed by a scanning electron microscope(SEM). In addition, leakage of nucleic acids and proteins within cells can be observed, indicating that eugenol interferes with cell membrane permeability and integrity. The hydrophobicity of eugenol and the impact of hydroxyl groups are closely related to the aforementioned actions (43). The extremely reactive hydroxyl groups in eugenol can create hydrogen bonds with a specific target enzyme of bacteria, which can disrupt the activity of the enzyme and result in a malfunction of the cell membrane system (72). Besides that, eugenol can indirectly inhibit the production of bacterial virulence factors by P. gingivalis by down-regulating the expression of the fimA genes, the hemagglutinin encoding genes hagA and hagB and the gingipain genes rgpA, rgpB and kgp, thereby affecting bacterial colonization, copolymerization, biofilm formation and nutrient uptake (43).
As a traditional Chinese medicine, Glycyrrhiza glabra (licorice) has high medicinal value in its dry roots and rhizomes and can also be used in the daily diet (73). The research demonstrated that the licorice extract’s flavonoid known as glabridin had a potent antibacterial effect against P. gingivalis. Furthermore, glabridin and the antibacterial peptide -poly L-lysine work together to kill bacteria (47). Glabridin has a high affinity for DNA gyrase and dihydrofolate reductase (DHFR) and can be utilized as inhibitors of two enzymes, even though the antibacterial mechanism is still unknown. Glabridin’s phenolic hydroxyl generates two hydrogen bonds with certain amino acids at DNA gyrase’s ATP binding site, preventing the synthesis of nucleic acids, and the affinity is higher than ciprofloxacin. Glabridin’s affinity for DHFR is primarily mediated via a hydrogen bond and other factors and is slightly weaker than trimethoprim’s (74).
Fructus mori (mulberry), the fruit clusters of the mulberry tree, are juicy and delicious fruits rich in the polyphenolic compound resveratrol (75). Resveratrol is a common stilbene compound, which can kill P.gingivalis and can be found that the size and shape of bacteria are damaged to varying degrees through SEM (51). Despite the fact that there is currently no agreement on resveratrol’s antibacterial capabilities (76), the double bond between the two benzene rings and the location and number of hydroxyl groups in the resveratrol structure, according to some researchers, may be important components in resveratrol’s ability to inhibit bacteria (77, 78). Other studies have demonstrated that following resveratrol therapy, the expression of the P. gingivalis pili-related genes fimA II and IV decreased by more than 50%, and the expression of the gingipains-related genes rgpA and kgp also decreased (51). Resveratrol can impair P.gingivalis’ adhesion to the basement membrane model and prevent P.gingivalis from degrading type I collagen in a dose/time-dependent manner (54).
Curcuma longa (turmeric) is an ancient Chinese medicinal plant of the ginger family, whose dried roots can be used in everyday cooking as well as for medicinal purposes. Curcumin is a phenolic compound extracted from turmeric (79). At concentrations below the MIC, curcumin can considerably limit the activity of six different strains of P. gingivalis and reduce its adhesion ability (52). Similarly, the researchers stressed the significance of aromatic hydroxyl groups in curcumin’s antibacterial effect (80, 81). Studies have shown that curcumin can downregulate the gene expressions of fimA, hagA, hagB, rgpA, rgpB, and kgp, inhibit the formation of P. gingivalis biofilm and reduce bacterial adherence (52).
4.2. Saponin
Saponins are composed of oligosaccharides partially linked with triterpenoids or steroidal aglycones, which can be divided into Triterpenoid saponin and steroidal saponin (82). They are prevalently present in plants as active compounds and have important pharmacological activities. Similar to surfactants, saponins include both hydrophilic and lipophilic groups. By lowering surface tension in aqueous solutions and producing micelles at crucial locations, these chemicals can alter the structure of biological macromolecules (44). Figure 3 displays the saponins from MFH plant sources that can be employed as antibacterial agents.
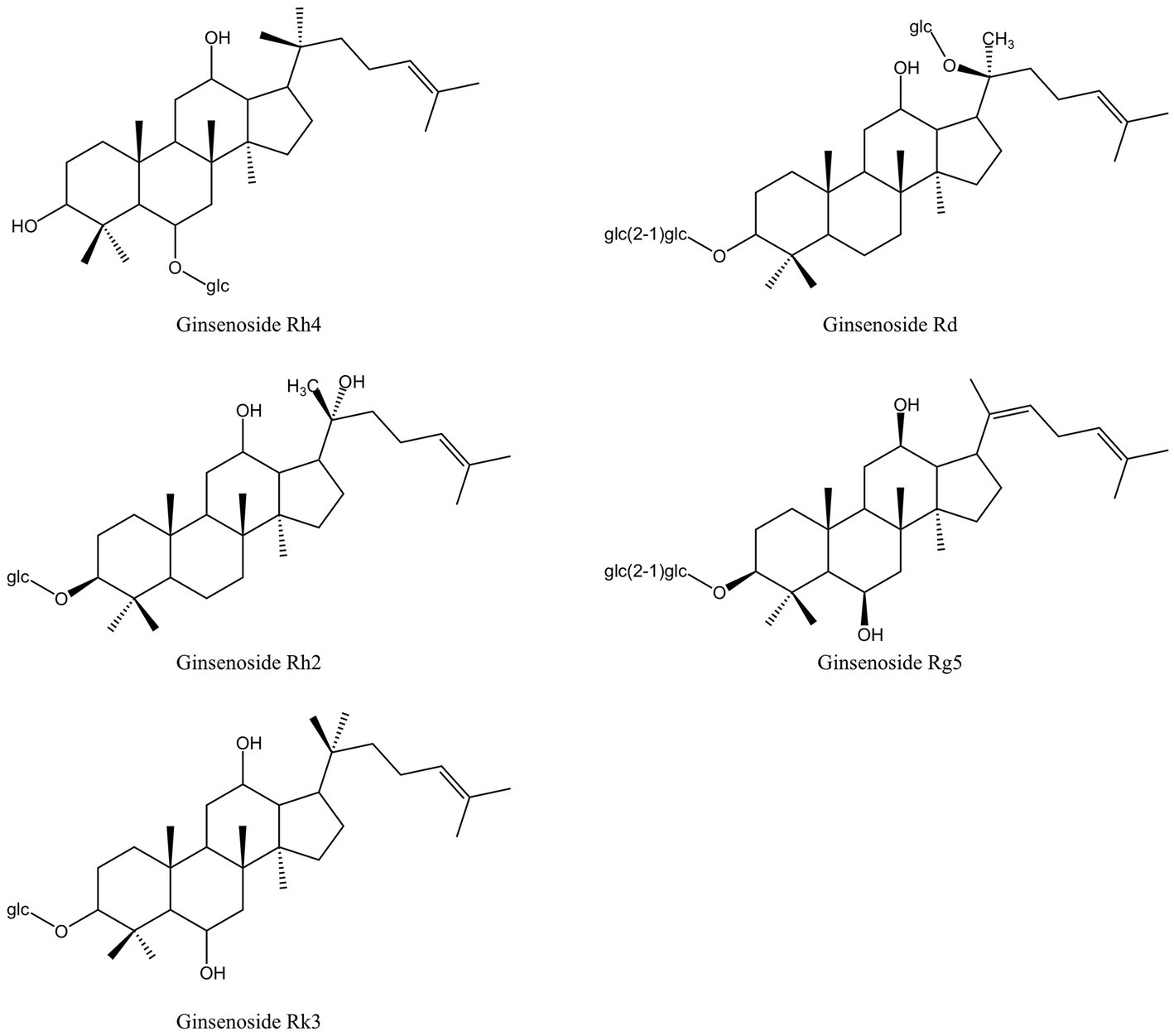
Figure 3. Saponins derived from MFH plants with antibacterial properties (created with KingDraw chemical structure editor).
In China, Panax ginseng Meyer (ginseng) has an extended tradition of use in both food and medicinal. Ginsenosides are triterpenoid saponins isolated from ginseng (83). According to research, ginsenosides’ primary antibacterial targets are bacterial cell membranes, and their amphiphilic characteristics are essential for membrane binding and permeability (84). P. gingivalis treated with ginsenosides can show the damage of cell membrane system and the outflow of cell contents, and the membrane binding characteristics of ginsenosides also depend on the polarity of ginsenosides, that is, the number of polar sugar groups in the structure (85). High-polarity ginsenosides can be decalcified and dehydrated employing high temperature or biotransformation to reduce polarity and enhance affinity with bacterial cell membranes (44, 86, 87). According to studies, less polar ginsenosides, which contain just one glycosyl group, such as Rk3, Rh2, Rh4 and Rg5, exhibit a better bactericidal action than highly polar ginsenosides, which contain three glycosyl groups and include Rg1, Re, Rb1, Rb2, Rc and Rd. (MIC >500 g/mL). According to TEM images of F. nucleatum treated with ginsenoside Rh2, the cell membrane had been destroyed and the contents of the cell had leaked (44). Through experimentation, some researchers have demonstrated that high polar ginsenoside Rd can likewise have a lethal effect on P. gingivalis (MIC = 400 μM), but this concentration is quite considerable. Intriguingly, P. gingivalis might be limited or even destroyed after treatment with modest concentrations of ginsenoside Rd (100 and 200 μM). Low concentrations of ginsenoside Rd can also drastically reduce bacterial surface hydrophobicity and block the expression of fimA and kgp, which has an impact on bacterial adhesion, copolymerization, and biofilm formation (45).
4.3. Aldehyde
The dried bark of the cinnamon tree, known as cinnamon, is a common element in Chinese cuisine and traditional herbal remedies. Cinnamaldehyde is an organic compound of olefine aldehydes extracted from cinnamon essential oil (Figure 4) (88). According to several researchers, the main cause of cinnamaldehyde’s antibacterial effects is the destruction of bacterial cell walls and membranes, which causes the contents of the cells to leak out. The interaction of hydrocarbons with hydrophobic bacterial cell membranes is thought to be the cause of this effect, but the antibacterial mechanism is not just restricted to this. It may also be connected to how cinnamaldehyde permeates bacteria and how it affects genetic material (89). In comparison to other chemicals lacking an aldehyde functional group, cinnamaldehyde had the strongest effect on removing Escherichia coli biofilm, leading Kot et al. to conclude that the antibacterial action of cinnamaldehyde may be derived from the aldehyde functional group it carries (90). The major component of cinnamon bark essential oil (CBEO), cinnamaldehyde, has been shown in experiments to have a substantial antibacterial impact on the cell wall and membrane of P. gingivalis. When P. gingivalis was exposed to CBEO and cinnamaldehyde, it developed uneven surfaces, folds, or damage that allowed intracellular proteins, DNA and RNA to leak out. Cinnamaldehyde at the sub-MIC level can inhibit the formation of 67.3% biofilm of P. gingivalis (46).
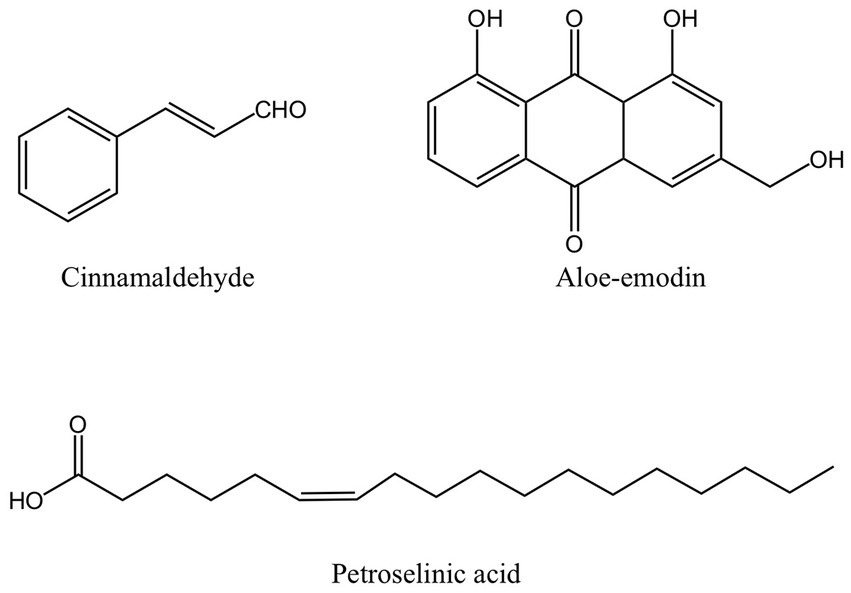
Figure 4. The structure of other antibacterial active ingredients from MFH plants including cinnamaldehyde, aloe-emodin and petroselinic acid (created with KingDraw chemical structure editor).
4.4. Anthraquinone
Aloe vera (aloe) is an edible herb of the lily family. Studies have shown that the gel prepared from mature fresh aloe leaf has an inhibitory effect on pathogens extracted from patients with subgingival stones, periapical abscesses, and periodontal abscesses, but only at a higher concentration. A low concentration of aloe gel does not show an antibacterial effect (91). In other studies, fresh aloe gel has an inhibitory effect on P. gingivalis, A. actinomycetemcomitans, and other periodontal pathogens, but the effect is not significant compared with S. mutans (49). However, both studies lack an analysis of the main active ingredients. When researchers used dried aloe powder extract, the outcomes were very different from those of fresh aloe gel and did not demonstrate any antibacterial activity against P. gingivalis. The reason might be that the dried aloe powder contains so little of the anthraquinone component aloe-emodin (Figure 4) (50). Anthracene and two carbonyl groups make form the chemical molecule known as anthraquinone. It is a typical chemical found in numerous plants. Its antibacterial activity primarily consists of impeding DNA synthesis and replication, compromising the integrity of cell membranes, preventing bacterial virulence factors, and preventing the development of biofilm (92–94). However, some academics have also asserted that Aloe-emodin is more likely to attach with peptidoglycan to destroy bacterial membranes and damage cell membrane permeability. Aloe-emodin consequently has more antibacterial effect against gram-positive bacteria than against gram-negative bacteria (95). This may also be one of the reasons why the outcomes of some studies on the bacteriostasis of aloe-emodin on P. gingivalis are not ideal. Aloe-emodin’s chemical composition also contributes to its antibacterial properties. According to several researchers, the antibacterial impact is directly tied to the aromatic hydroxyl groups on the anthracene core, and the antibacterial activity is further increased by the hydroxyl methyl groups the compound carries (96).
4.5. Fatty acid
Foeniculum vulgare (fennel) is a traditional medicinal and culinary plant. Petroselinic acid extracted from fennel is an unsaturated fatty acid (Figure 4). Petroselinic acid induced the formation of moniliform OMVs-like particles on the surface of P. gingivalis, which, unlike naturally occurring OMVs, induced explosive degradation of the bacteria and leakage of cell contents and DNA. The accumulation of RagA and RagB in OMVs-like particles can lead to the reduction of RagA and RagB in P. gingivalis (55). RagA and RagB proteins are believed to have a part in the uptake of macromolecules like polysaccharides or glycoproteins (97, 98). In addition, fennel extract and petroselinic acid also showed inhibitory effects on gingipains (55). However, the relationship between the anti-P. gingivalis and its virulence factor effect of petroselinic acid and its molecular structure has not been elaborated. Some scholars have found that the antibacterial activity of unsaturated fatty acids can be affected by the length of the carbon chain, the degree of unsaturation, and the position and configuration of the double bond. Moreover, the virulence factor and its gene expression of Staphylococcus aureus were significantly inhibited by petroselinic acid, suggesting that there may be targets between petroselinic acid and these virulence factors, but this part has not been confirmed by molecular docking research (99).
4.6. Others
In addition, some MFH plants have antibacterial activity. Some researchers have proposed that ethanol extract of Hibiscus sabdariffa L. (roselle calyx) has inhibitory effects on a variety of periodontal pathogens (48), but this study did not discuss the key active elements or the precise processes of roselle calyx. However, other researchers have hypothesized that protocatechuic acid, which, by generating ROS, damages DNA and causes lipid peroxidation in bacteria, changing the redox equilibrium (100), may be in charge of the roselle calyx’s antibacterial properties (101). More research is necessary to determine whether proto-catechuic acid has the same antibacterial impact against periodontal infections. Ethanol extract of Morus alba leaves can reduce the growth of periodontal pathogens in a dose-dependent manner (102). Similarly, diosgenin extracted from Trigonella foenum-graecum L. (fenugreek) also showed dose-dependent inhibition of P. gingivalis and P. intermedia (103). Yet neither study provided information on effective doses.
In conclusion, periodontitis-related bacteria have been found to be significantly inhibited by MFH plants and their active ingredients. In contrast to the direct killing of bacteria, the majority of MFH plants and their active ingredients appear to be more effective at preserving the stability and balance of the periodontal microenvironment by inhibiting bacterial accumulation, copolymerization, biofilm establishment, and the production and activity of bacterial virulence factors. Based on the aforementioned studies, several academics stress the significance of these active components’ chemical structures for their antibacterial activity. Each active ingredient’s antibacterial effect can be attributed to a unique bond or functional group in its chemical structure that can interact and bind with a particular bacterial structure or enzyme, disrupt the physiological function of bacteria result in abnormal bacterial activity or metabolism, and ultimately produce the desired antibacterial effect.
The aforementioned research still has some incomplete aspects in several areas, though. First off, rather than being primarily linked to the pathogenic properties of a specific bacterium, periodontitis tends to be more susceptible to abnormalities in the oral microbial ecosystem. The mouth cavity contains about 1,000 bacteria. Other microbes outside bacteria exist as well, including fungi, viruses, and archaea (104). Distinct strains of microbes can produce distinct active components due to differences in their surface properties and functional groups. Future research should take into account the variations between the harmful bacteria because the aforementioned tests mostly consider one or two of them. In addition, the aforementioned studies did not address whether the active components of some MFH plants can achieve the ideal antibacterial effect in vivo, whether the bactericidal effect is particular, or whether it can affect the stability of the overall microenvironment of the oral flora. Therefore, more research is required.
5. Medicine food homology plants inhibit host inflammatory response and bone resorption
Although the development of early periodontal tissue lesions requires the presence of bacteria, their significance should not be overstated. As was previously stated, bacteria alone cannot predict how periodontitis will develop. Recent studies have also highlighted the importance of the role of host inflammation and the immune system as opposed to particular bacteria in the etiology of periodontitis. To take part in the inflammatory response, immune cells react to bacterial stimuli by triggering a number of signaling pathways and producing a range of inflammatory cytokines.
The oxidative stress in periodontal tissue and the absorption of alveolar bone may be directly related to the inflammatory response of periodontal tissue. The development of oxidative stress is attributed to an imbalance between the immune system’s capacity to eradicate ROS produced by tissues and the ROS’s actual creation. Some researchers have hypothesized that inflammation and oxidative stress are interconnected and can be mutually causative, causing and boosting one another in terms of their relationship (105). The oxidative stress environment in periodontal tissue, which is created by neutrophil hyperactivity and excessive ROS generation, is critical in causing periodontitis to develop, as was previously discussed. Additionally, the high production and ongoing stimulation of pro-inflammatory cytokines appear to be intimately linked to this excessive activation (106). Therefore, the development of oxidative stress can heighten the periodontal tissue’s inflammatory response, causing substantial harm to the tissue. Similar to this, because of the intricate relationships and dynamic interplay between inflammatory cells and bone cells, inflammatory reactions can significantly alter bone homeostasis and bone remodeling (107). Firstly, cytokines activated during the inflammatory response process, such as IL-1β, IL-6, and TNF- α, and signal pathways, especially nuclear factor kappa B (NF- κβ), have been shown to be closely related to the differentiation and activity of osteoclast, and can promote the process of bone absorption by promoting the differentiation of osteoclast; Secondly, numerous inflammatory mediators can further inhibit the differentiation of osteoblasts and affect bone remodeling. The above two points are important mechanisms for bone mass reduction caused by inflammation (108, 109). In other words, an inflammatory response can undermine the stability of the bone microenvironment, upset the delicate balance between bone creation and absorption, and is inextricably linked to periodontitis’ symptoms of bone absorption. Numerous studies have demonstrated that MFH plant extracts and secondary metabolites can inhibit the production of proinflammatory cytokines, decrease the inflammatory response and oxidative stress of periodontal tissue, and affect the process of osteoclast formation, thereby inhibiting alveolar bone absorption by regulating the aforementioned signal pathways or by altering the transcription and translation of specific regulators (Table 3).
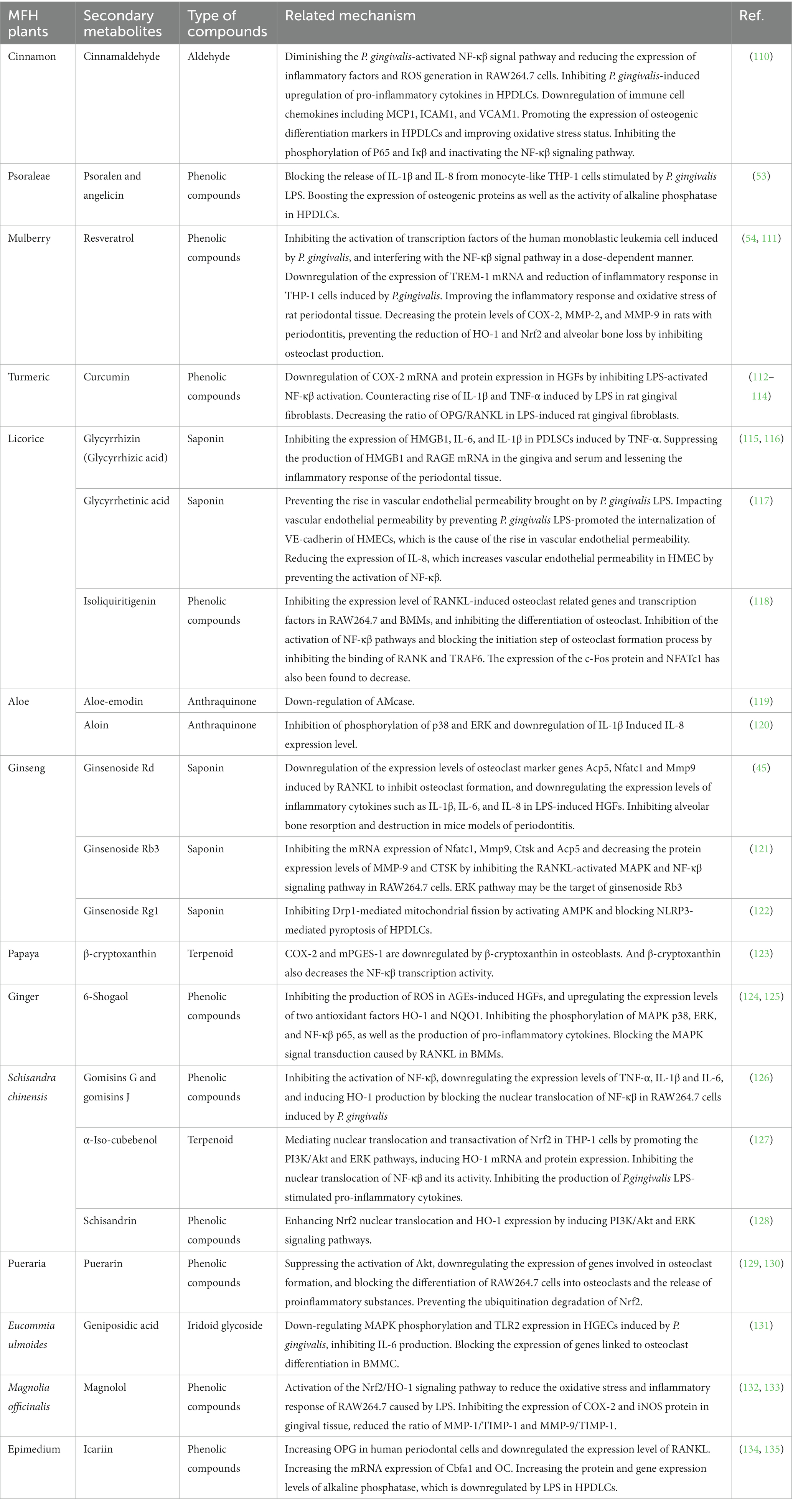
Table 3. Inhibitory effects of secondary metabolites derived from the plant MFH on the inflammatory response and bone resorption.
5.1. Aldehyde
It has been demonstrated that cinnamaldehyde is a very effective anti-inflammatory medication (136). Cinnamaldehyde was found to diminish the P. gingivalis-activated NF-κβ signal pathway, which in turn reduced the expression of inflammatory factors and ROS generation in RAW264.7 cells. The same anti-inflammatory function has also been confirmed in Human periodontal ligament cells (HPDLCs). Cinnamaldehyde inhibits P. gingivalis-induced upregulation of pro-inflammatory cytokines in HPDLCs, including IL-6, IL-8, TNF-α and IL-1β. Downregulation of immune cell chemokines including monocyte chemoattractant protein 1 (MCP1), intercellular adhesion molecule 1 (ICAM1) and vascular cell adhesion molecule 1 (VCAM1) is also observed. Meanwhile, cinnamaldehyde also promotes the expression of osteogenic differentiation markers in HPDLCs and improves oxidative stress status. Cinnamaldehyde also inhibits the phosphorylation of P65 and Iκβ and inactivates the NF-κβ signaling pathway (110). According to academics, the impacts of cinnamaldehyde can be summed up as follows: 1. Cinnamaldehyde can chelate metal ions and alter several signaling pathways; 2. The aldehyde functional groups carried by cinnamaldehyde can give hydrogen ions to neutralize free radicals, lowering oxidative stress and inflammatory reactions in tissues (137). Cinnamaldehyde can be given orally to mice with ligation-induced periodontitis to slow the rate of alveolar bone loss and the levels of inflammatory cells and oxidative stress in periodontal tissue (110).
5.2. Phenolic compound
Phenolic compounds are electron or hydrogen atom donors with aromatic rings and multiple hydroxyl groups, which can eliminate free radicals through hydrogen ion or proton transfer, inhibit lipid peroxidation, and inhibit the activation of pro-inflammatory cytokine-mediated inflammatory signaling pathways, reducing oxidative stress and inflammatory response (138, 139). They have developed into one of the areas of research that has received a lot of attention lately. MFH plant-derived phenolic compounds with anti-inflammatory, antioxidant, and bone resorption inhibition properties are shown in Figure 5.
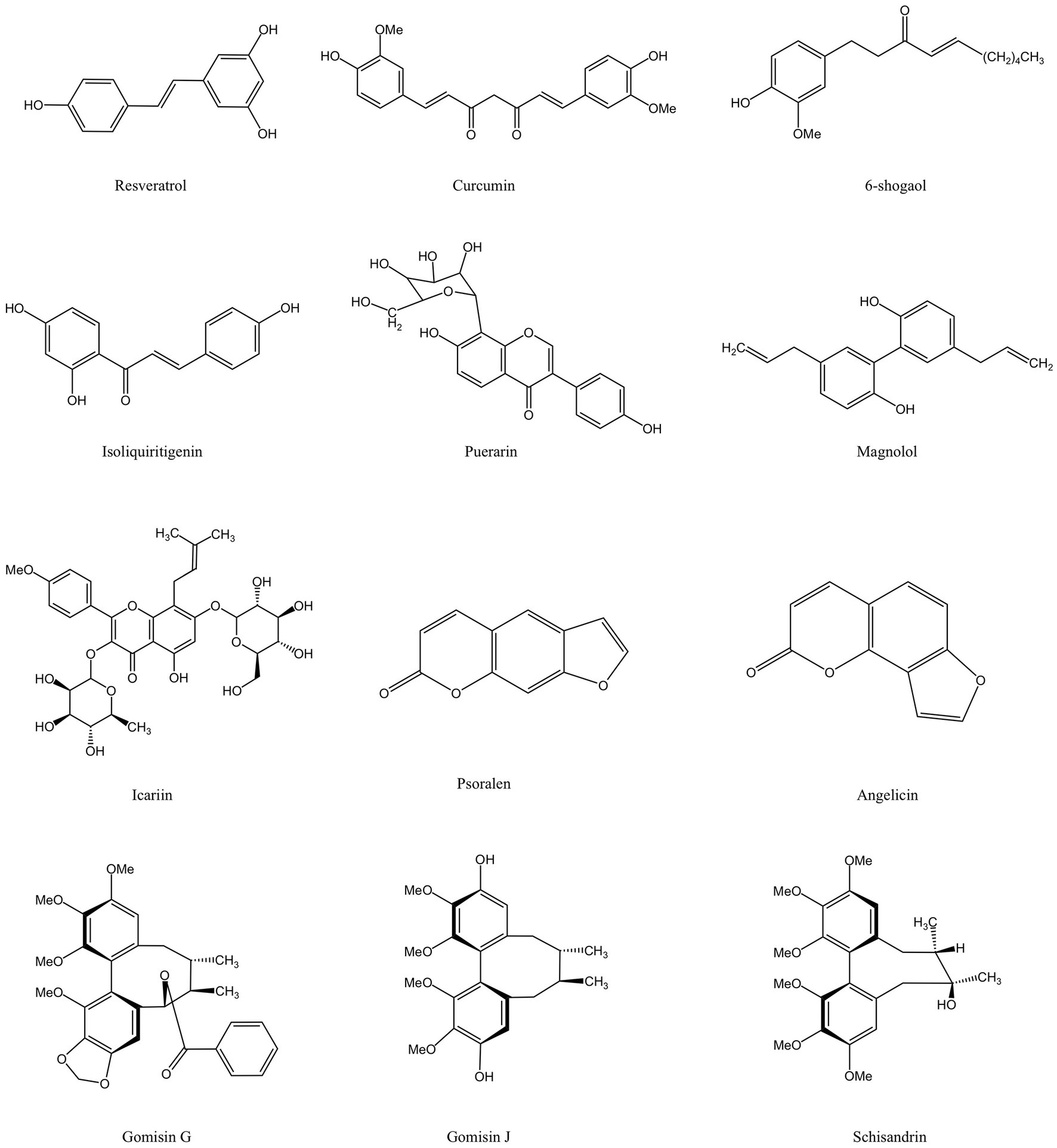
Figure 5. Phenolic compounds derived from MFH plants with anti-inflammatory effects (created with KingDraw chemical structure editor).
In-vitro studies have demonstrated that resveratrol blocks the activation of human monoblastic leukemia cell transcription factors induced by P. gingivalis, and interfere with the NF-κβ signal pathway in a dose-dependent manner (54). Triggering receptor expressed on myeloid cells-1 (TREM-1) is closely associated with the production of IL-1β and TNF-α that contribute to the persistence of inflammatory pathways (140). Resveratrol significantly down-regulates the expression of TREM-1 mRNA and reduces the inflammatory response in THP-1 cells induced by P. gingivalis (54). Toll-like receptor-4 (TLR-4) is an LPS-specific cell sensor that inhibits LPS-induced osteoblast differentiation and bone remodeling (141). Resveratrol down-regulates LPS-stimulated elevated levels of TLR-4 in human gingival fibroblasts (HGFs), thereby reducing the inflammatory response (111). Matrix metalloproteinase (MMP) is a family of zinc-dependent endopeptidases whose main function is to cleave extracellular matrix proteins, reshape tissues and degrade extracellular matrix under physiological and pathological conditions, and is considered to be an important substance involved in periodontal tissue collapse and inflammatory response during the development of periodontitis (142). Cyclooxygenase-2 (COX-2) promotes the disease process of periodontitis by mediating the inflammatory process in periodontal tissues (143). Nuclear factor erythroid 2-related factor 2 (Nrf2) and its downstream anti-invertases, such as heme oxygenase-1 (HO-1), are essential in resisting cellular oxidative stress and down-regulating the inflammatory response (144). Resveratrol has been shown to help reduce the inflammatory response and oxidative stress of rat periodontal tissue in vivo studies, decrease the protein levels of COX-2, MMP-2, and MMP-9 in rats with periodontitis, prevent the reduction of HO-1 and Nrf2, and effectively prevent alveolar bone loss by inhibiting osteoclast production (111).
Similarly, curcumin has shown superior anti-inflammation and antioxidant potential in vitro and in vivo. Curcumin downregulates COX-2 mRNA and protein expression in HGFs by inhibiting LPS-activated NF-κβ activation (112). Based on this, additional researchers have discovered that LPS can greatly upregulate IL-1β and TNF-α in rat gingival fibroblasts, and that curcumin can counteract this rise. In addition, the ratio of osteoprotegerin (OPG)/nuclear factor B receptor activator ligand (RANKL) increased in LPS-induced rat gingival fibroblasts treated with curcumin, and this bone protection feature was also demonstrated in rats with ligation-induced periodontitis (113). To treat rats with LPS-induced periodontitis, some researchers gave them an oral dose of the corn oil carrier that diluted curcumin each day. In periodontal tissue, downregulation of the expression of the IL-6, TNF-α, and prostaglandin E2 (PGE2) synthase genes was seen after 15 days. But curcumin can only block the pathway at a dose of 30 mg/kg (114). Additionally, more investigators discovered that the curcumin gel shell can minimize inflammatory infiltration and restrict bone absorption similarly to tetracyclines when used as an antibacterial supplementary treatment for scaling and root planning (SRP). Contrary to tetracyclines, interestingly, curcumin-treated rats showed iron deposits in their bone trabeculae, suggesting that curcumin may have the ability to encourage bone growth (145).
Zingiber officinale Roscoe (ginger) is a kind of herb of the ginger genus. Its rhizome is used as an essential cooking spice in the home and has a high medicinal value. Volatile phenolic compounds 6-Gingerol are the main irritating compounds present in ginger rhizomes. After heat treatment, they are prone to dehydration reactions to form 6-shogaol, which is also the source of the spicy flavor of ginger (146). Hyperglycemia in diabetes patients can induce protein glycosylation, and produce the advanced glycation end products (AGEs) that can damage periodontal tissue by increasing the oxidative stress of periodontal histiocytes and the expression of inflammatory-related factors (147). In vitro experiments showed that 6-shogaol can inhibit the generation of ROS in AGE-induced HGFs and upregulate the expression levels of two antioxidant factors, HO-1 and NAD(P)H quinone dehydrogenase 1 (NQO1). In addition, 6-shogaol can also inhibit the phosphorylation of mitogen-activated protein kinases (MAPK) p38, ERK, and NF-κβ p65, as well as the production of pro-inflammatory cytokines (124). Other studies discovered that 6-shogaol blocked the MAPK signal transduction caused by RANKL in mice bone marrow macrophages (BMMs), which prevented the development of mice osteoclasts in a dose-dependent manner. Additionally, this research showed through in vivo tests that 6-shogaol can successfully stop bone loss and inflammation in the periodontal tissue of periodontally ligated mice (125).
Licorice isoliquiritigenin is a flavonoid compound. Some studies have suggested that isoliquiritigenin may reduce the levels of expression of RANKL-induced osteoclast-related genes and transcription factors in RAW264.7 and BMMs, and inhibit the differentiation of osteoclasts. And isoliquitigenin inhibits the activation of NF-κβ pathways and blocks the initiation step of the osteoclast formation process by inhibiting the binding of RANKL-stimulated receptor RANK and signal adapter molecule TRAF6. The expression of the c-Fos protein and nuclear factor of activated T cells c1 (NFATc1) has also been found to decrease. Animal studies have also supported isoliquiritigenin’s inhibitory effect on bone resorption. When isoliquiritigenin was administered intraperitoneally to mice, their bone loss was significantly reduced (118).
The dried rhizome of Pueraria lobata is a kind of traditional medicinal and edible plant. An isoflavone substance called puerarin is derived from P. lobata. Puerarin has been shown to suppress the activation of Akt, downregulate the expression of genes involved in osteoclast formation, and block the differentiation of RAW264.7 cells into osteoclasts caused by LPS and the release of proinflammatory substances. Puerarin can greatly reduce LPS-induced bone loss and the number of osteoclasts in the mice skull in later animal trials (129). According to an intriguing study, signaling pathways connected to Nrf2 may be able to shield periodontal tissue from oxidative stress in hypoxic conditions, preventing damage to the tissue. In typical conditions, Nrf2 can be weakened by Kelch ECH-associating protein 1(Keap1). Nevertheless, p62 and Nrf2 are regulated by a positive feedback loop. By strengthening its association with Keap1, p62 can prevent the degradation of Nrf2 by that protein, and the Akt/ mammalian target of the rapamycin(mTOR)signaling pathway influences the production of p62. Puerarin can indirectly prevent the ubiquitination degradation of Nrf2 mediated by Keap1 and enhance the nuclear translocation of Nrf2 in HPDLCs. It can also upregulate the production of p62 via stimulating the Akt/mTOR signaling pathway. Puerarin has additionally demonstrated in vivo studies that it can successfully stop alveolar bone loss in animals with periodontitis (130).
Magnolia officinalis is a member of the magnolia family whose dried stem bark, root bark and branch bark used in ancient Chinese medicine and can also be incorporated into the diet. Magnolia officinalis is the source of the plant polyphenol known as magnolol. According to studies, magnolol can trigger the Nrf2/HO-1 signaling pathway to reduce the oxidative stress and inflammatory response of RAW264.7 caused by LPS (132). In addition, magnolol significantly reduced the inflammatory response of periodontal tissue and alveolar bone loss and decreased the number of osteoclasts and the expression level of RANKL in experimental periodontitis rats. Magnolol also inhibited the expression of COX-2 and iNOS protein in gingival tissue, reduced the ratio of MMP-1/tissue inhibitor of metalloproteinases-1 (TIMP-1) and MMP-9/TIMP-1, and inhibited the destruction of periodontal tissue (133).
Epimedium brevicornu Maxim. (epimedium) is a Ranunculus Berberis plant whose dried leaves are used both medicinally and for food. Icariin is a flavonoid compound that is the main active ingredient in epimedium. 0.01 μg/mL icariin increased OPG in human periodontal cells and downregulated the expression level of RANKL, leading to a decrease in the RANKL/OPG ratio. In addition, icariin also increased the mRNA expression of core binding factor alpha1 (Cbfa1) and osteocalcin (OC) in a dose-dependent manner, and the icariin group at 0.01 μg/mL showed the highest expression, which had a bone-promoting effect; however, when the icariin concentration was greater than 0.1 μg/mL, the expression of Cbfa1 and OC was decreased (134). Icariin can also increase the protein and gene expression levels of alkaline phosphatase, which is downregulated by LPS in HPDLCs (135). Local injection of icariin can attenuate the inflammatory process in minipig periodontitis models, and levels of the pro-inflammatory cytokines IL-1β and IFN-γ were lower in the icariin group than in the control group. In addition, icariin can effectively promote the regeneration of periodontal fibers and bone tissue (148).
Psoralea corylifolia (psoraleae) is a traditional plant whose fruits can be eaten and used medicinally. Psoralen and angelicin, which are active components of psoraleae and are coumarin compounds, can, in a dose-dependent manner, block the release of IL-1β and IL-8 from monocyte-like THP-1 cells stimulated by P.gingivalis LPS. Additionally, psoralen and angelica can boost the expression of osteogenic proteins as well as the activity of alkaline phosphatase in HPDLCs. The osteoprotective activities of angelicin on the alveolar bone of periodontitis-affected mice were further supported by this work, although the in vivo effects of psoralen remain unaccounted for (53).
Schisandra chinensis (Turcz.) Baill is a traditional Chinese medicine in the magnolia family. Its fruit can be used as a medicine or as a daily food. The active ingredient of Schisandra chinensis is lignans, including gomisins and schisandrin (149). Gomisins G and J inhibit the activation of NF-κβ, downregulate the expression levels of TNF-α, IL-1β and IL-6, and induce HO-1 production by blocking the nuclear translocation of NF-κβ in RAW264.7 cells induced by P. gingivalis (126). HO-1 expression is mainly regulated by the phosphoinositide 3-kinase (PI3K)/Akt pathway. In RAW264.7 cells induced by LPS of P. gingivalis, schisandrin can enhance Nrf2 nuclear translocation and HO-1 expression by inducing PI3K/Akt and ERK signaling pathways (128). However, there have been no reports on the in vivo anti-inflammatory effects of the active ingredients of Schisandra chinensis mentioned above.
5.3. Terpenoid
The class of active compounds known as terpenoids, which are abundantly present in nature, is made up of isoprene structural units. They can be categorized as monoterpenes, sesquiterpenes, diterpenes, triterpenes, tetraterpenes, and polyterpenes based on how many isoprene units they contain. Terpenoids have been proven to have significant anti-inflammatory benefits (150). Terpenoids can suppress the development of osteoclasts and the oxidative stress on periodontal tissue as well as operate on a variety of targets in the signal pathway and diminish the generation of inflammatory cytokines. Figure 6 displays the MFH plant terpenoids having anti-inflammatory properties reported in this paper.
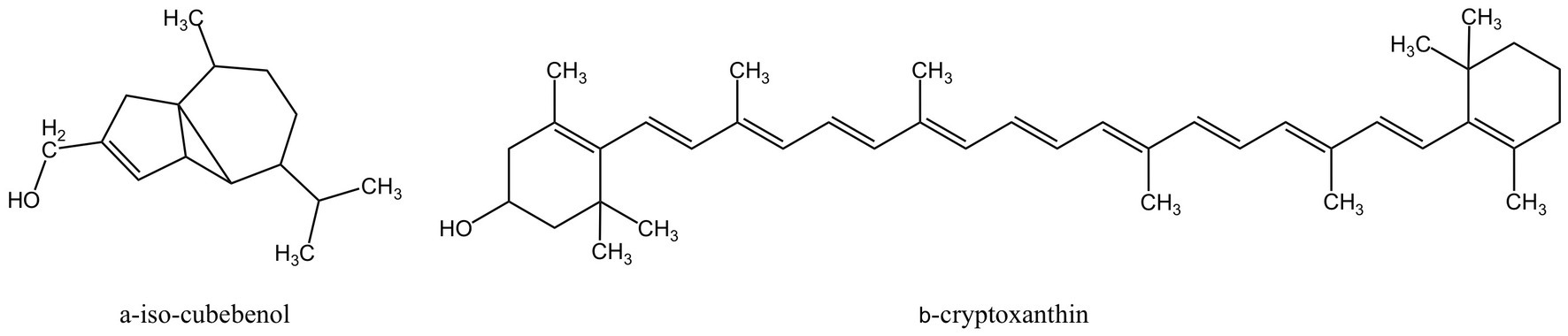
Figure 6. Terpenoids derived from MFH plants with anti-inflammatory effects (created with KingDraw chemical structure editor).
α-Iso-cubebenol is a cubebene sesquiterpene compound extracted from Schisandra chinensis (151). α-Iso-cubebenol mediated nuclear translocation and transactivation of Nrf2 in human macrophage THP-1 cells by promoting the PI3K/Akt and ERK pathways, induced HO-1 mRNA and protein expression in a time- and dose-dependent manner, and the anti-inflammatory effect of α-Iso-cubebenol can be reversed by the selective inhibitor tin-protoporphyrin of HO-1. In addition, α-Iso-cubebenol can also inhibit the nuclear translocation of NF-κβ and its activity, as well as inhibit the production of P.gingivalis LPS-stimulated pro-inflammatory cytokines (127). However, in-vivo research has not been done to further support the effects of α-Iso-cubebenol mentioned above.
Chaenomeles sinensis (Papaya) is a plant of the genus Papaya in the family of rosaceae. Its fruits are very common in daily life and can be eaten raw or used as a cooking ingredient and, after drying, can also be used as a medicine. β-cryptoxanthin is a carotenoid extracted from Papaya. In LPS-induced mice, β-cryptoxanthin can considerably reduce the amount of cranial bone resorption. Additionally, COX-2 and membrane-bound PGE synthase-1 (mPGES-1), two related enzymes that induce to production of PGE2 in the body, are downregulated by β-cryptoxanthin in osteoblasts. And β-cryptoxanthin also decreases the NF-κβ transcription activity. Molecular docking showed that β-cryptoxanthin competitively binds to the ATP-binding domain of inhibitor of NF-κβ kinase (IKKβ), inhibiting its activity and attenuating the activity of the LPS-induced NF-κβ pathway. In addition, β-cryptoxanthin inhibited RANKL-induced osteoclast differentiation and significantly reduced cathepsin K (CTSK) expression levels in RAW264.7 cells (123).But the effects of β-cryptoxanthin also lack proofs of in-vivo experiments.
5.4. Iridoid glycoside
Iridoid is a natural compound that often combines with sugars in plants to form iridoid glycoside. Eucommia ulmoides Oliv. is a plant whose bark is used in traditional medicine and food. Geniposidic acid (Figure 7), an iridoid glycoside extracted from Eucommia ulmoides, down-regulates MAPK phosphorylation and TLR2 expression in human gingival epithelial cells (HGECs) induced by P. gingivalis, inhibits IL-6 production. Additionally, Geniposidic acid can block the expression of genes linked to osteoclast differentiation in Bone marrow mononuclear cells (BMMC) in a concentration-dependent way. However, Geniposidic acid has no impact on IL-6 levels in BMMC, even though IL-6 is also linked to osteoclast differentiation. Geniposidic acid taken orally can reduce the inflammation of periodontal tissues and alveolar bone absorption in the mice of periodontitis caused by P.gingivalis (131).
5.5. Anthraquinone
Both the periodontitis inflammation response and bone resorption are improved by aloe-emodin and aloin derived from aloe (Figure 8). In rat models of periodontitis, aloe emodin has been suggested to lessen alveolar bone loss and periodontal inflammation. The mechanism may be the downregulation of aloe-emodin on acidic mammalian chitinase (AMcase) (119). AMcase is a hydrolase that cleaves glycoside saccharide bonds in chitin. Although humans cannot synthesize chitin, the expression level of AMcase is higher in inflammatory periodontal tissues than in normal periodontal tissues and is associated with the immune response to various inflammatory diseases (152). Aloin is also an anthraquinone compound extracted from aloe, composed of two types of diastereomers (153). Research has shown that aloin down-regulates IL-1β-induced IL-8 expression by inhibiting the phosphorylation of p38 and ERK in KB cells (120), but the anti-inflammatory mechanism in vivo has not been elucidated.
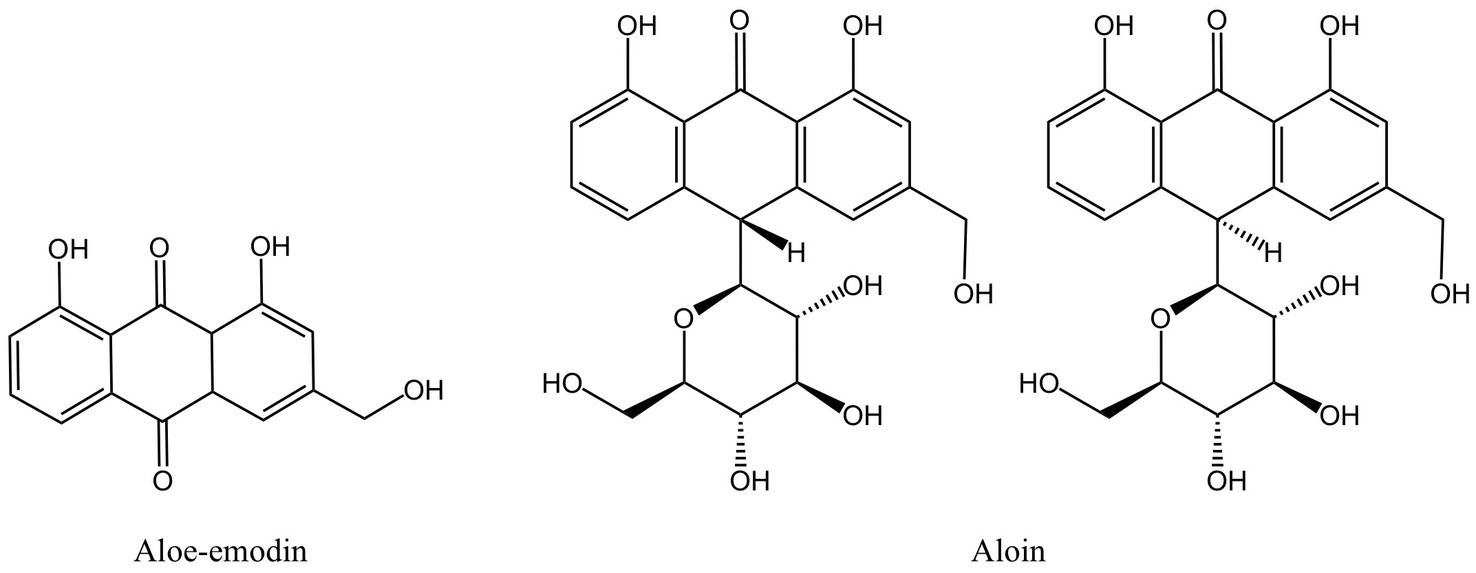
Figure 8. Anthraquinones derived from MFH plants with anti-inflammatory effects (created with KingDraw chemical structure editor).
5.6. Saponin
The saponin components derived from MFH plants also have anti-inflammatory effects (Figure 9). Ginsenoside Rd can downregulate the expression levels of osteoclast marker genes Acp5, Nfatc1 and Mmp9 induced by RANKL to inhibit osteoclast formation in vitro and downregulate the expression levels of inflammatory cytokines such as IL-1β, IL-6, and IL-8 in LPS-induced HGFs. Furthermore, in vivo experiments have shown that ginsenoside Rd can significantly inhibit alveolar bone resorption and destruction in mice models of periodontitis (45). Ginsenoside Rb3 inhibited the mRNA expression of Nfatc1, Mmp9, Ctsk and Acp5 and decreased the protein expression levels of MMP-9 and CTSK by inhibiting the RANKL-activated MAPK and NF-κβ signaling pathway in RAW264.7 cells. Besides that, ginsenoside Rb3 has the most significant inhibitory effect on the MARK/ERK pathway, suggesting that the ERK pathway may be the target of ginsenoside Rb3 (121). In experimental periodontitis rats stimulated by P. gingivalis LPS, Ginsenoside Rb3 inhibits alveolar bone loss and osteoclast differentiation as well (154). Activation of NLRP3 inflammasomes plays an important role in the production of pro-inflammatory cytokines and pyroptosis. Mitochondria are the key regulators of NLRP3 inflammasome activation. Mitochondrial homeostasis is closely linked to AMP-activated protein kinase (AMPK) and dynamin-related protein 1 (Drp1). Ginsenoside Rg1 can inhibit Drp1-mediated mitochondrial fission by activating AMPK, as well as block NLRP3-mediated pyroptosis of HPDLCs in a variety of ways (122). However, the in-vivo activity of ginsenoside Rg1 still needs further investigation.
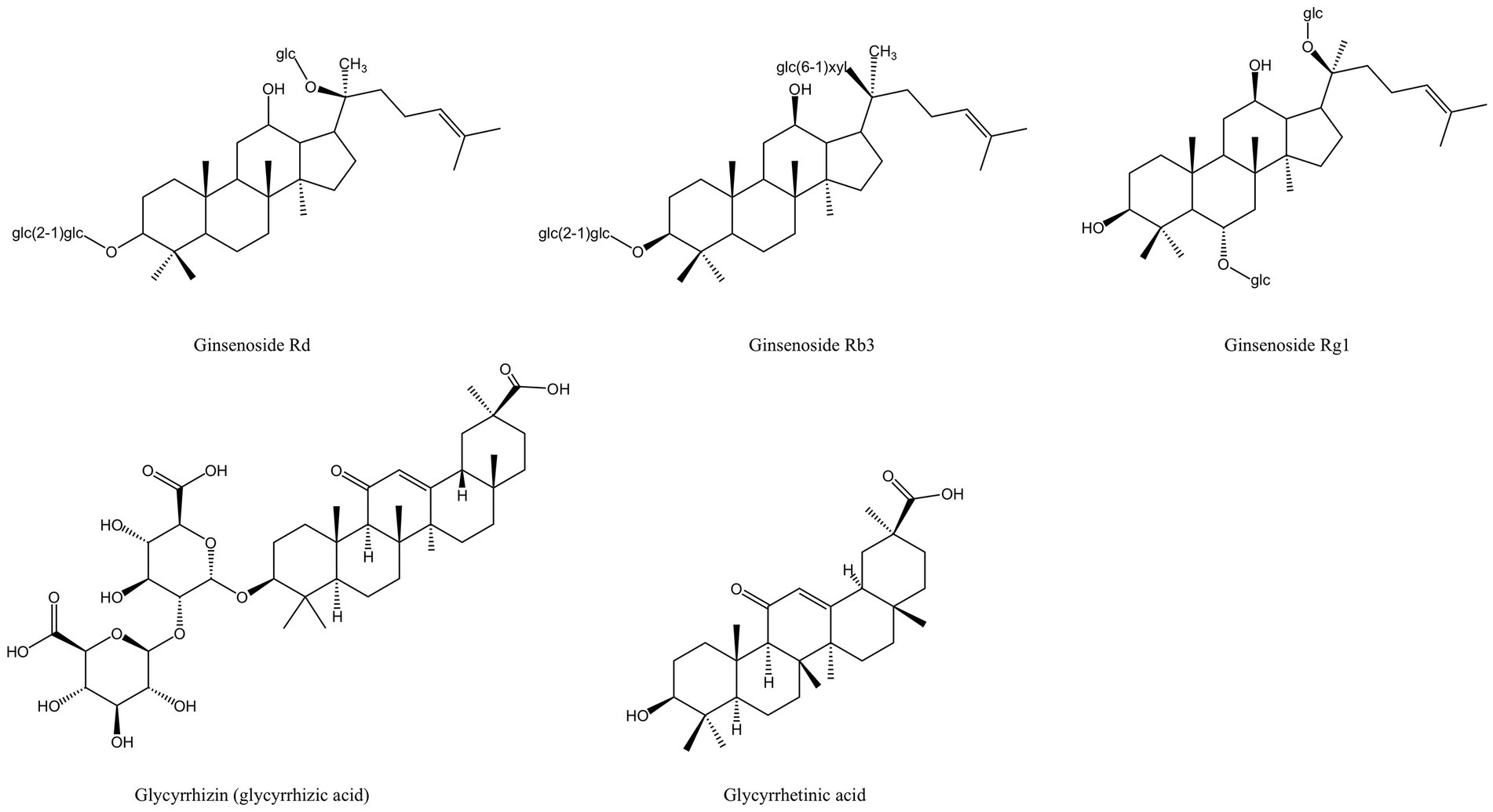
Figure 9. Saponin derived from MFH plants with anti-inflammatory effects (created with KingDraw chemical structure editor).
High mobility group protein 1(HMGB1) is a group of non-histone chromosomal proteins that can be released from necrotic or damaged cells. It is highly expressed in periodontitis and can mediate bone resorption by promoting the differentiation of osteoclasts (155, 156). Glycyrrhizin, also known as glycyrrhizic acid, is a triterpenoid saponin isolated from the root of licorice (157). Glycyrrhizin can inhibit the expression of HMGB1, IL-6, and IL-1β in human periodontal ligament stem cells (PDLSCs) induced by TNF-α, according to in vitro investigations. In vivo test using a rat periodontitis model treated with glycyrrhizin revealed the downregulation of HMGB1, IL-6, and IL-1β in the gingival crevicular fluid and periodontal tissue (115). AGEs receptors (RAGE) in tissues and plasma of diabetic patients bound to HMGB1 are key in the association between periodontitis and diabetes (158). In diabetic mice with periodontitis, glycyrrhizic acid can suppress the production of HMGB1 and RAGE mRNA in the gingiva and serum and lessen the inflammatory response of the periodontal tissue (116). Glycyrrhetinic acid is the main metabolite of glycyrrhizin with two residues of glucose acid (157). According to the in-vivo Miles vascular permeability assay, glycyrrhetinic acid can prevent the rise in vascular endothelial permeability brought on by P. gingivalis LPS. Studies conducted in vitro have demonstrated that glycyrrhetinic acid impacts vascular endothelial permeability by preventing P. gingivalis LPS-promoted internalization of VE-cadherin of human microvascular endothelial cells (HMECs), which is the cause of the rise in vascular endothelial permeability. Additionally, by preventing the activation of NF-κβ, glycyrrhetinic acid can reduce the expression of IL-8, which increases vascular endothelial permeability in HMEC (117). Some researchers applied glycyrrhetinic acid locally to the gingival sulcus of the rat periodontitis model and found that glycyrrhetinic acid could effectively inhibit the formation of LPS-stimulated immune complex and inflammatory cell infiltration, and no loss of attachment was observed (159).
5.7. Others
In addition to the secondary metabolites from MFH plants outlined above, several MFH plant extracts also have notable inhibiting impacts on periodontal tissue inflammation and bone resorption, such as Angelica dahurica, Ginkgo biloba, Houttuynia cordata, Chrysanthemum morifolium Ramat. (chrysanthemum), and Evodia rutaecarpa (160–164), although the active biological components they contain have not yet been identified. Table 4 lists the detailed effects of these extracts of MFH plants.
In conclusion, there is considerable biological activity and minimal toxicity in the extracts and secondary metabolites of MFH plants against periodontal tissue inflammation. Although there has been a substantial amount of in vitro and animal experimentation demonstrating the majority of the anti-inflammatory and anti-bone resorption benefits of MFH plants, clinical research is still in its infancy. Additionally, it is a difficult and time-consuming operation to identify and analyze the active components in some research that focus on extracts of MFH plants. There are still a lot of undiscovered linkages that need to be investigated, but some researches have established a causal link between the chemical makeup of some active chemicals and their anti-inflammatory and bone resorption action. However, the number of such studies is too limited. Furthermore, some researches indicate that the dosage or concentration of MFH plants is strongly related to their biological action, and high or low doses may significantly decrease or boost the biological activity of the active ingredient, such as large quantities of phenolic compounds that can cause oxidation (138). And different active component dosages may have various modes of action. For instance, various dosages of curcumin can influence various signal pathways and prevent the creation of certain pro-inflammatory substances (114). Therefore, dose control still requires special care from us.
6. Evidence from clinical studies of MFH plants
The number of clinical investigations is still extremely small, although some MFH plants have demonstrated considerable anti-periodontitis benefits in vitro and animal tests. The ability of MFH plants to enhance periodontal health in individuals with periodontitis has been validated by certain researchers, however, the majority of the studies have been modest in scope and substance. The present research and development philosophy for MFH plant products is centered on clinical adjuvant therapies, medicines and functional foods.
6.1. Medicine food homology plants related periodontal adjuvant therapy
Plant-based MFH products are frequently used in conjunction with periodontal therapy to help keep the therapeutic impact going strong. Babaei et al. (165) assigned 40 patients with chronic periodontitis to intervention and control groups and administered 1 g of Cichorium intybus L. (chicory) leaf methanol extract capsules and placebo capsules, respectively, twice daily for consecutive 8 weeks. Before the study, all subjects underwent non-surgical periodontal therapy. According to the findings, chicory leaf extract was able to significantly improve the depth of the periodontal pocket, increase total antioxidant capacity, uric acid and high-density lipoprotein cholesterol and decrease malondialdehyde, triglycerides, low-density lipoprotein cholesterol and total cholesterol levels in the intervention group compared to the control group and baseline (p < 0.05).
6.2. Medicine food homology plants related functional foods and oral care products
Twenty young adults with healthy periodontal conditions were recruited by Gao et al. (166) and divided into an intervention group and a control group before being given either Phyllanthus emblica L. gum or a placebo gum. It turns out that in the group of P. emblica gum, the generation of volatile sulfide was inhibited, and the overall bacterial count, S. mutans, and P. gingivalis counts were significantly reduced (p < 0.05). Assiry et al. (167)recruited 50 subjects with moderate gingival scores and fair plaque scores without systemic diseases and randomly assigned them to the intervention and control groups. The intervention group received Illicium verum (star anise) mouthwash, whereas the control group received colored placebos, twice daily. Results indicate that subjects in the I. verum intervention group significantly improved in mean gingival index, papillary bleeding index and microbial count (P 0.05) when compared to the control group and baseline (p < 0.05).
In summary, MFH plant-based functional foods and care items such as chewing gum, chewing tablets, mouthwash, toothpaste, and so on need to be enhanced in terms of flavor and taste while ensuring effectiveness, as adding MFH plant-based ingredients may result in an unpleasant odor and taste. How to strike the right balance between the degree of delicacy and the optimal clinical effect is one of the issues that need to be resolved. Future research should also take into account the low solubility issue with some active compounds generated from MFH plants. This issue can be resolved by developing a scientifically sound drug delivery system that will prolong the action duration inside the periodontal tissue and decrease drug loss.
7. Conclusion
The following might be used to summarize how MFH plants work to treat and prevent periodontitis: First, bacteria are the primary causes of inflammation. Bacterial cell membranes are susceptible to the effects of MFH plants and their secondary metabolites, which can cause bacterial cell disruption and expulsion of cell contents. They may also hinder the synthesis of biological macromolecules vital to bacterial survival at the same time, impairing bacterial growth and reproduction; Second, by preventing the action of bacterial virulence factors and the expression of associated genes, MFH plants and their secondary metabolites can also affect bacterial invasion, colonization, copolymerization, biofilm formation, and periodontal tissue damage; Additionally, MFH plants can lessen bone absorption by limiting the development of osteoclasts and can lower the level of inflammatory cytokines in periodontal tissue by controlling the synthesis of a range of signal pathways and regulatory variables; Last but not least, the modulation of antioxidant macromolecules, the downregulation of the oxidative stress response in periodontal tissue, and the lessening of damage to periodontal tissue are all possible functions of MFH plants. Significant progress has been made in the study of the use of MFH plants in treating periodontitis, which is encouraging for the creation of new MFH plant-related functional meals, oral care products, or additional methods of treating periodontitis.
Periodontitis, as a worldwide public health problem, mainly manifests as gingival bleeding and retraction, alveolar bone loss, bad breath, and so on. In extreme circumstances, it can lead to tooth loss and affect the patient’s quality of life. Periodontitis is currently mostly treated with clinically necessary care and antibacterial adjuvant therapy. However, the development of multidrug-resistant bacteria brought on by antibiotics has long been a challenge that many academics are working to overcome. Therefore, one of the major research hotspots is the hunt for plant-based medicines that can replace antibiotics. The fact that variables other than oral ones can promote inflammation in periodontal tissue adds to the complexity of the etiology of periodontitis. It is undeniable that some systemic conditions, including diabetes and obesity, have strong associations with periodontitis. Dietary intervention is one of the most crucial treatment modalities for chronic disorders. The response to the question of whether a balanced diet may help with the prevention and treatment of periodontitis brought on by diabetes or obesity has been raised by a number of academics, and it is clear that it can. Consuming wholesome, anti-inflammatory foods and changing one’s dietary habits have been demonstrated to help treat periodontitis in numerous clinical investigations. In keeping with the new idea of contemporary illness prevention, MFH plants have emerged as the ideal option for disease prevention and a balanced diet due to their dual usage as food and medicine. In conclusion, MFH plants are rich resources that should be further explored to support the clinical conversion of MFH plant-related products. For the future prevention and treatment of periodontitis, they are extremely promising natural products.
Author contributions
SQ: conceptualization, visualization, and writing–original draft preparation. SY: conceptualization and writing–review and editing. XM: writing–original draft preparation. RW: supervision, validation, and writing–review and editing. All authors have read and agreed to the published version of the manuscript.
Funding
This research was funded by “Jilin Health Science and Technology Capacity Improvement Project, 2021JC029” and “Jilin Health Research Project, 2021JK02.”
Conflict of interest
The authors declare that the research was conducted in the absence of any commercial or financial relationships that could be construed as a potential conflict of interest.
Publisher’s note
All claims expressed in this article are solely those of the authors and do not necessarily represent those of their affiliated organizations, or those of the publisher, the editors and the reviewers. Any product that may be evaluated in this article, or claim that may be made by its manufacturer, is not guaranteed or endorsed by the publisher.
References
1. Uy, S, Deng, K, Fok, CTC, Fok, MR, Pelekos, G, and Tonetti, MS. Food intake, masticatory function, tooth mobility, loss of posterior support, and diminished quality of life are associated with more advanced periodontitis stage diagnosis. J Clin Periodontol. (2022) 49:240–50. doi: 10.1111/jcpe.13588
2. Chen, MX, Zhong, YJ, Dong, QQ, Wong, HM, and Wen, YF. Global, regional, and national burden of severe periodontitis, 1990-2019: an analysis of the global burden of disease study 2019. J Clin Periodontol. (2021) 48:1165–88. doi: 10.1111/jcpe.13506
3. Schenkein, HA, Papapanou, PN, Genco, R, and Sanz, M. Mechanisms underlying the association between periodontitis and atherosclerotic disease. Periodontol 2000. (2020) 83:90–106. doi: 10.1111/prd.12304
4. Graziani, F, Gennai, S, Solini, A, and Petrini, M. A systematic review and Meta-analysis of epidemiologic observational evidence on the effect of periodontitis on diabetes an update of the Efp-Aap review. J Clin Periodontol. (2018) 45:167–87. doi: 10.1111/jcpe.12837
5. Ma, HZ, Zheng, JM, and Li, XL. Potential risk of certain cancers among patients with periodontitis: a supplementary meta-analysis of a large-scale population. Int J Med Sci. (2020) 17:2531–43. doi: 10.7150/ijms.46812
6. Gomes-Filho, IS, Cruz, SSD, Trindade, SC, Passos-Soares, JS, Carvalho-Filho, PC, Figueiredo, A, et al. Periodontitis and respiratory diseases: a systematic review with meta-analysis. Oral Dis. (2020) 26:439–46. doi: 10.1111/odi.13228
7. Shan, F, Huang, LQ, Guo, J, and Chen, M. History and development of “one root of medicine and food”. Chin Bull Life Sci. (2015) 27:1061–9. doi: 10.13376/j.cbls/2015146
8. Gong, X, Ji, M, Xu, J, Zhang, C, and Li, M. Hypoglycemic effects of bioactive ingredients from medicine food homology and medicinal health food species used in China. Crit Rev Food Sci. (2020) 60:2303–26. doi: 10.1080/10408398.2019.1634517
9. Lu, Q, Li, R, Yang, Y, Zhang, Y, Zhao, Q, and Li, J. Ingredients with anti-inflammatory effect from medicine food homology plants. Food Chem. (2022) 368:130610. doi: 10.1016/j.foodchem.2021.130610
10. Batiha, GE, Beshbishy, AM, El-Mleeh, A, Abdel-Daim, MM, and Devkota, HP. Traditional uses, bioactive chemical constituents, and pharmacological and toxicological activities of Glycyrrhiza glabra L (Fabaceae). Biomol Ther. (2020) 10:352. doi: 10.3390/biom10030352
11. Luo, H, Vong, CT, Chen, H, Gao, Y, Lyu, P, Qiu, L, et al. Naturally occurring anti-Cancer compounds: shining from Chinese herbal medicine. Chin Med. (2019) 14:48. doi: 10.1186/s13020-019-0270-9
12. Kaur, G, Kathariya, R, Bansal, S, Singh, A, and Shahakar, D. Dietary antioxidants and their indispensable role in periodontal health. J Food Drug Anal. (2016) 24:239–46. doi: 10.1016/j.jfda.2015.11.003
13. Abusleme, L, Hoare, A, Hong, BY, and Diaz, PI. Microbial signatures of health, gingivitis, and periodontitis. Periodontol. (2021) 86:57–78. doi: 10.1111/prd.12362
14. Abusleme, L, Dupuy, AK, Dutzan, N, Silva, N, Burleson, JA, Strausbaugh, LD, et al. The subgingival microbiome in health and periodontitis and its relationship with community biomass and inflammation. ISME J. (2013) 7:1016–25. doi: 10.1038/ismej.2012.174
15. Gao, H, Sun, T, Yang, F, Yuan, J, Yang, M, Kang, W, et al. The pathogenic effects of Fusobacterium nucleatum on the proliferation, osteogenic differentiation, and transcriptome of osteoblasts. Front Cell Dev Biol. (2020) 8:807. doi: 10.3389/fcell.2020.00807
16. Baek, K, Ji, S, and Choi, Y. Complex intratissue microbiota forms biofilms in periodontal lesions. J Dent Res. (2018) 97:192–200. doi: 10.1177/0022034517732754
17. Colombo, AV, Barbosa, GM, Higashi, D, di Micheli, G, Rodrigues, PH, and Simionato, MRL. Quantitative detection of Staphylococcus aureus, Enterococcus faecalis and Pseudomonas aeruginosa in human oral epithelial cells from subjects with periodontitis and periodontal health. J Med Microbiol. (2013) 62:1592–600. doi: 10.1099/jmm.0.055830-0
18. Socransky, SS, Haffajee, AD, Cugini, MA, Smith, C, and Kent, RL. Microbial complexes in subgingival plaque. J Clin Periodontol. (1998) 25:134–44. doi: 10.1111/j.1600-051x.1998.tb02419.x
19. Joseph, S, and Curtis, MA. Microbial transitions from health to disease. Periodontol 2000. (2021) 86:201–9. doi: 10.1111/prd.12377
20. Hajishengallis, G, and Lamont, RJ. Beyond the red complex and into more complexity: the polymicrobial synergy and dysbiosis (Psd) model of periodontal disease etiology. Mol Oral Microbiol. (2012) 27:409–19. doi: 10.1111/j.2041-1014.2012.00663.x
21. Bartold, PM, and Van Dyke, TE. An appraisal of the role of specific bacteria in the initial pathogenesis of periodontitis. J Clin Periodontol. (2019) 46:6–11. doi: 10.1111/jcpe.13046
22. Dahlen, G, Basic, A, and Bylund, J. Importance of virulence factors for the persistence of oral bacteria in the inflamed gingival crevice and in the pathogenesis of periodontal disease. J Clin Med. (2019) 8:1339. doi: 10.3390/jcm8091339
23. How, KY, Song, KP, and Chan, KG. Porphyromonas Gingivalis: an overview of periodontopathic pathogen below the gum line. Front Microbiol. (2016) 7:53. doi: 10.3389/fmicb.2016.00053
24. Sharma, G, Garg, N, Hasan, S, and Shirodkar, S. Prevotella: an insight into its characteristics and associated virulence factors. Microb. Pathog. (2022):105673:169. doi: 10.1016/j.micpath.2022.105673
25. Herrero, ER, Fernandes, S, Verspecht, T, Ugarte-Berzal, E, Boon, N, Proost, P, et al. Dysbiotic biofilms deregulate the periodontal inflammatory response. J Dent Res. (2018) 97:547–55. doi: 10.1177/0022034517752675
26. Kononen, E, Gursoy, M, and Gursoy, UK. Periodontitis: a multifaceted disease of tooth-supporting tissues. J Clin Med. (2019) 8:1135. doi: 10.3390/jcm8081135
27. Hajishengallis, G. Interconnection of periodontal disease and comorbidities: evidence, mechanisms, and implications. Periodontol. (2022) 89:9–18. doi: 10.1111/prd.12430
28. Bartold, PM, and Van Dyke, TE. Periodontitis: a host-mediated disruption of microbial homeostasis. unlearning learned concepts. Periodontol. (2013) 2000:203–17. doi: 10.1111/j.1600-0757.2012.00450.x
29. Silva, N, Abusleme, L, Bravo, D, Dutzan, N, Garcia-Sesnich, J, Vernal, R, et al. Host response mechanisms in periodontal diseases. J Appl Oral Sci. (2015) 23:329–55. doi: 10.1590/1678-775720140259
30. Cekici, A, Kantarci, A, Hasturk, H, and Van Dyke, TE. Inflammatory and immune pathways in the pathogenesis of periodontal disease. Periodontol. (2014) 64:57–80. doi: 10.1111/prd.12002
31. Wang, C, Wang, LL, Wang, XX, and Cao, ZG. Beneficial effects of melatonin on periodontitis management: far more than oral cavity. Int J Mol Sci. (2022) 23:14541. doi: 10.3390/ijms232314541
32. Chakravarti, A, Raquil, MA, Tessier, P, and Poubelle, PE. Surface Rankl of toll-like receptor 4-stimulated human neutrophils activates osteoclastic bone resorption. Blood. (2009) 114:1633–44. doi: 10.1182/blood-2008-09-178301
33. Li, A, Qiu, B, Goettsch, M, Chen, Y, Ge, S, Xu, S, et al. Association between the quality of plant-based diets and periodontitis in the us general population. J Clin Periodontol. (2023) 50:591–603. doi: 10.1111/jcpe.13785
34. Machado, V, Botelho, J, Viana, J, Pereira, P, Lopes, LB, Proenca, L, et al. Association between dietary inflammatory index and periodontitis: a cross-sectional and mediation analysis. Nutrients. (2021) 13:1194. doi: 10.3390/nu13041194
35. Kotsakis, GA, Chrepa, V, Shivappa, N, Wirth, M, Hébert, J, Koyanagi, A, et al. Diet-borne systemic inflammation is associated with prevalent tooth loss. Clin Nutr. (2018) 37:1306–12. doi: 10.1016/j.clnu.2017.06.001
36. Salazar, CR, Laniado, N, Mossavar-Rahmani, Y, Borrell, LN, Qi, Q, Sotres-Alvarez, D, et al. Better-quality diet is associated with lower odds of severe periodontitis in us Hispanics/Latinos. J Clin Periodontol. (2018) 45:780–90. doi: 10.1111/jcpe.12926
37. Costa, SA, Nascimento, GG, Colins, PMG, Alves, CMC, Thomaz, E, Carvalho Souza, SF, et al. Investigating oral and systemic pathways between unhealthy and healthy dietary patterns to periodontitis in adolescents: a population-based study. J Clin Periodontol. (2022) 49:580–90. doi: 10.1111/jcpe.13625
38. Laiola, M, De Filippis, F, Vitaglione, P, and Ercolini, D. A Mediterranean diet intervention reduces the levels of salivary periodontopathogenic bacteria in overweight and obese subjects. Appl Environ Microbiol. (2020) 86:e00777. doi: 10.1128/aem.00777-20
39. Alhassani, AA, Hu, FB, Li, Y, Rosner, BA, Willett, WC, and Joshipura, KJ. The associations between major dietary patterns and risk of periodontitis. J Clin Periodontol. (2021) 48:2–13. doi: 10.1111/jcpe.13380
40. Li, W, He, Y, Zheng, Q, and Deng, X. The causal effect of life course adiposity on periodontitis: a Mendelian randomization study. J Periodontol. (2023) 94:256–62. doi: 10.1002/jper.21-0632
41. Preshaw, PM, Alba, AL, Herrera, D, Jepsen, S, Konstantinidis, A, Makrilakis, K, et al. Periodontitis and diabetes: a two-way relationship. Diabetologia. (2012) 55:21–31. doi: 10.1007/s00125-011-2342-y
42. Kowalska-Krochmal, B, and Dudek-Wicher, R. The minimum inhibitory concentration of antibiotics: methods, interpretation, clinical relevance. Pathogens. (2021) 10:165. doi: 10.3390/pathogens10020165
43. Zhang, Y, Wang, Y, Zhu, X, Cao, P, Wei, S, and Lu, Y. Antibacterial and Antibiofilm activities of eugenol from essential oil of Syzygium aromaticum (L.) Merr. & L. M. Perry (clove) leaf against periodontal pathogen Porphyromonas gingivalis. Microb Pathog. (2017) 113:396–402. doi: 10.1016/j.micpath.2017.10.054
44. Xue, P, Yang, XS, Zhao, L, Hou, ZH, Zhang, RY, Zhang, FX, et al. Relationship between antimicrobial activity and amphipathic structure of ginsenosides. Ind Crop Prod. (2020):111929:143. doi: 10.1016/j.indcrop.2019.111929
45. Zhou, SH, Ji, YT, Yao, HT, Guo, HY, Zhang, ZC, Wang, ZJ, et al. Application of Ginsenoside Rd in periodontitis with inhibitory effects on pathogenicity, inflammation, and bone resorption. Front Cell Infect Mi. (2022) 12:813953. doi: 10.3389/fcimb.2022.813953
46. Wang, Y, Zhang, Y, Shi, YQ, Pan, XH, Lu, YH, and Cao, P. Antibacterial effects of cinnamon (Cinnamomum zeylanicum) bark essential oil on Porphyromonas gingivalis. Microb Pathog. (2018) 116:26–32. doi: 10.1016/j.micpath.2018.01.009
47. Tsukatani, T, Kuroda, R, and Kawaguchi, T. Screening biofilm eradication activity of ethanol extracts from foodstuffs: potent biofilm eradication activity of glabridin, a major flavonoid from licorice (Glycyrrhiza glabra), alone and in combination with varepsilon-poly-L-lysine. World J Microbiol Biotechnol. (2022) 38:24. doi: 10.1007/s11274-021-03206-z
48. Sulistyani, H, Fujita, M, Miyakawa, H, and Nakazawa, F. Effect of roselle calyx extract on in vitro viability and biofilm formation ability of oral pathogenic bacteria. Asian Pac J Trop Med. (2016) 9:115–20. doi: 10.1016/j.apjtm.2016.01.020
49. Fani, M, and Kohanteb, J. Inhibitory activity of Aloe vera gel on some clinically isolated cariogenic and periodontopathic bacteria. J Oral Sci. (2012) 54:15–21. doi: 10.2334/josnusd.54.15
50. Mueller-Heupt, LK, Wiesmann, N, Schroeder, S, Korkmaz, Y, Vierengel, N, Gross, J, et al. Extracts of Rheum palmatum and Aloe vera show beneficial properties for the synergistic improvement of oral wound healing. Pharmaceutics. (2022) 14:2060. doi: 10.3390/pharmaceutics14102060
51. Kugaji, MS, Kumbar, VM, Peram, MR, Patil, S, Bhat, KG, and Diwan, PV. Effect of resveratrol on biofilm formation and virulence factor gene expression of Porphyromonas gingivalis in periodontal disease. APMIS. (2019) 127:187–95. doi: 10.1111/apm.12930
52. Kumbar, VM, Peram, MR, Kugaji, MS, Shah, T, Patil, SP, Muddapur, UM, et al. Effect of curcumin on growth, biofilm formation and virulence factor gene expression of Porphyromonas gingivalis. Odontology. (2021) 109:18–28. doi: 10.1007/s10266-020-00514-y
53. Li, XT, Yu, CB, Hu, Y, Xia, XY, Liao, Y, Zhang, J, et al. New application of psoralen and Angelicin on periodontitis with anti-bacterial, anti-inflammatory, and osteogenesis effects. Front Cell Infect Microbiol. (2018):8:178. doi: 10.3389/fcimb.2018.00178
54. Ben Lagha, A, Andrian, E, and Grenier, D. Resveratrol attenuates the pathogenic and inflammatory properties of Porphyromonas gingivalis. Mol Oral Microbiol. (2019) 34:118–30. doi: 10.1111/omi.12260
55. Yoshino, N, Ikeda, T, and Nakao, R. Dual inhibitory activity of petroselinic acid enriched in fennel against Porphyromonas gingivalis. Front Microbiol. (2022):13. doi: 10.3389/fmicb.2022.816047
56. Xu, W, Zhou, W, Wang, H, and Liang, S. Roles of Porphyromonas gingivalis and its virulence factors in periodontitis. Adv Protein Chem Struct Biol. (2020) 120:45–84. doi: 10.1016/bs.apcsb.2019.12.001
57. Kobayashi, T, Kaneko, S, Tahara, T, Hayakawa, M, Abiko, Y, and Yoshie, H. Antibody responses to Porphyromonas gingivalis hemagglutinin a and outer membrane protein in chronic periodontitis. J Periodontol. (2006) 77:364–9. doi: 10.1902/jop.2006.050138
58. Lunar Silva, I, and Cascales, E. Molecular strategies underlying Porphyromonas gingivalis virulence. J Mol Biol. (2021) 433:166836. doi: 10.1016/j.jmb.2021.166836
59. Qiu, Y, Tan, X, Lei, Z, Chen, X, Chen, J, Gong, T, et al. A Gntr family transcription factor in Porphyromonas gingivalis regulates bacterial growth, acylpeptidyl oligopeptidase, and gingipains activity. Mol Oral Microbiol. (2023) 38:48–57. doi: 10.1111/omi.12400
60. Attallah, NGM, Negm, WA, Elekhnawy, E, Altwaijry, N, Elmongy, EI, El-Masry, TA, et al. Antibacterial activity of Boswellia sacra Flueck. Oleoresin extract against Porphyromonas gingivalis periodontal pathogen. Antibiotics (Basel). (2021) 10:859. doi: 10.3390/antibiotics10070859
61. Zhang, Z, Liu, D, Liu, S, Zhang, S, and Pan, Y. The role of Porphyromonas gingivalis outer membrane vesicles in periodontal disease and related systemic diseases. Front Cell Infect Microbiol. (2020) 10:585917. doi: 10.3389/fcimb.2020.585917
62. Durazzo, A, Lucarini, M, Souto, EB, Cicala, C, Caiazzo, E, Izzo, AA, et al. Polyphenols: a concise overview on the chemistry, occurrence, and human health. Phytother Res. (2019) 33:2221–43. doi: 10.1002/ptr.6419
63. Marchiosi, R, dos Santos, WD, Constantin, RP, de Lima, RB, Soares, AR, Finger-Teixeira, A, et al. Biosynthesis and metabolic actions of simple phenolic acids in plants. Phytochem Rev. (2020) 19:865–906. doi: 10.1007/s11101-020-09689-2
64. Efenberger-Szmechtyk, M, Nowak, A, and Czyzowska, A. Plant extracts rich in polyphenols: antibacterial agents and natural preservatives for meat and meat products. Crit Rev Food Sci. (2021) 61:149–78. doi: 10.1080/10408398.2020.1722060
65. Wu, T, He, M, Zang, X, Zhou, Y, Qiu, T, Pan, S, et al. A structure-activity relationship study of flavonoids as inhibitors of E. coli by membrane interaction effect. Biochim Biophys Acta. (2013) 1828:2751–6. doi: 10.1016/j.bbamem.2013.07.029
66. Wu, T, Zang, X, He, M, Pan, S, and Xu, X. Structure-activity relationship of flavonoids on their anti-Escherichia coli activity and inhibition of DNA gyrase. J Agric Food Chem. (2013) 61:8185–90. doi: 10.1021/jf402222v
67. Yang, W-Y, Kim, C-K, Ahn, C-H, Kim, H, Shin, J, and Oh, K-B. Flavonoid glycosides inhibit Sortase a and Sortase a-mediated aggregation of Streptococcus mutans, an oral bacterium responsible for human dental caries. J Microbiol Biotechnol. (2016) 26:1566–9. doi: 10.4014/jmb.1605.05005
68. Xie, Y, Yang, W, Tang, F, Chen, X, and Ren, L. Antibacterial activities of flavonoids: structure-activity relationship and mechanism. Curr Med Chem. (2015) 22:132–49. doi: 10.2174/0929867321666140916113443
69. Kalli, S, Araya-Cloutier, C, Hageman, J, and Vincken, J-P. Insights into the molecular properties underlying antibacterial activity of prenylated (Iso)flavonoids against Mrsa. Sci Rep. (2021) 11:14180. doi: 10.1038/s41598-021-92964-9
70. Li, J, Li, CZ, Shi, C, Aliakbarlu, J, Cui, HY, and Lin, L. Antibacterial mechanisms of clove essential oil against Staphylococcus aureus and its application in pork. Int J Food Microbiol. (2022):380. doi: 10.1016/j.ijfoodmicro.2022.109864
71. Nabavi, SF, Di Lorenzo, A, Izadi, M, Sobarzo-Sanchez, E, Daglia, M, and Nabavi, SM. Antibacterial effects of cinnamon: from farm to food cosmetic and pharmaceutical industries. Nutrients. (2015) 7:7729–48. doi: 10.3390/nu7095359
72. Guimaraes, AC, Meireles, LM, Lemos, MF, Guimaraes, MCC, Endringer, DC, Fronza, M, et al. Antibacterial activity of terpenes and terpenoids present in essential oils. Molecules. (2019) 24:2471. doi: 10.3390/molecules24132471
73. Yang, R, Yuan, BC, Ma, YS, Zhou, S, and Liu, Y. The anti-inflammatory activity of licorice, a widely used Chinese herb. Pharm Biol. (2017) 55:5–18. doi: 10.1080/13880209.2016.1225775
74. Lin, HY, Hu, JB, Mei, F, Zhang, YH, Ma, YD, Chen, QQ, et al. Anti-microbial efficacy, mechanisms and druggability evaluation of the natural flavonoids. J Appl Microbiol. (2022) 133:1975–88. doi: 10.1111/jam.15705
75. Malaguarnera, L. Influence of resveratrol on the immune response. Nutrients. (2019) 11:946. doi: 10.3390/nu11050946
76. Vestergaard, M, and Ingmer, H. Antibacterial and antifungal properties of resveratrol. Int J Antimicrob Agents. (2019) 53:716–23. doi: 10.1016/j.ijantimicag.2019.02.015
77. Sheng, J-Y, Chen, T-T, Tan, X-J, Chen, T, and Jia, A-Q. The quorum-sensing inhibiting effects of stilbenoids and their potential structure-activity relationship. Bioorg Med Chem Lett. (2015) 25:5217–20. doi: 10.1016/j.bmcl.2015.09.064
78. Luo, HZ, Guan, Y, Yang, R, Qian, GL, Ya, XH, Wang, JS, et al. Growth inhibition and metabolomic analysis of Xanthomonas oryzae Pv. oryzae treated with resveratrol. BMC Microbiol. (2020) 20:117. doi: 10.1186/s12866-020-01803-w
79. Tagde, P, Tagde, P, Islam, F, Tagde, S, Shah, M, Hussain, ZD, et al. The multifaceted role of curcumin in advanced nanocurcumin form in the treatment and management of chronic disorders. Molecules. (2021) 26:7109. doi: 10.3390/molecules26237109
80. Kim, MK, Park, JC, and Chong, Y. Aromatic hydroxyl group plays a critical role in antibacterial activity of the curcumin analogues. Nat Prod Commun. (2012) 7:57–8. doi: 10.1177/1934578X1200700120
81. Sekiya, M, Chiba, E, Satoh, M, Yamakoshi, H, Iwabuchi, Y, Futai, M, et al. Strong inhibitory effects of curcumin and its demethoxy analog on Escherichia coli Atp synthase F-1 sector. Int J Biol Macromol. (2014) 70:241–5. doi: 10.1016/j.ijbiomac.2014.06.055
82. Cui, X, Ma, X, Li, C, Meng, H, and Han, C. A review: structure-activity relationship between saponins and cellular immunity. Mol Biol Rep. (2022) 50:2779–93. doi: 10.1007/s11033-022-08233-z
83. Xue, Q, He, N, Wang, Z, Fu, X, Aung, LHH, Liu, Y, et al. Functional roles and mechanisms of Ginsenosides from Panax ginseng in atherosclerosis. J Ginseng Res. (2021) 45:22–31. doi: 10.1016/j.jgr.2020.07.002
84. Verstraeten, SL, Lorent, JH, and Mingeot-Leclercq, M-P. Lipid membranes as key targets for the pharmacological actions of ginsenosides. Front Pharmacol. (2020):11. doi: 10.3389/fphar.2020.576887
85. Xue, P, Yao, Y, Yang, XS, Feng, J, and Ren, GX. Improved antimicrobial effect of ginseng extract by heat transformation. J Ginseng Res. (2017) 41:180–7. doi: 10.1016/j.jgr.2016.03.002
86. Chang, KH, Jo, MN, Kim, KT, and Paik, HD. Evaluation of glucosidases of Aspergillus niger strain comparing with other glucosidases in transformation of ginsenoside Rb1 to ginsenosides Rg3. J Ginseng Res. (2014) 38:47–51. doi: 10.1016/j.jgr.2013.11.008
87. Li, X, Yao, F, Fan, H, Li, K, Sun, LW, and Liu, YJ. Intraconversion of polar ginsenosides, their transformation into less-polar ginsenosides, and ginsenoside acetylation in ginseng flowers upon baking and steaming. Molecules. (2018) 23:759. doi: 10.3390/molecules23040759
88. Nakhaee, S, Kooshki, A, Hormozi, A, Akbari, A, Mehrpour, O, and Farrokhfall, K. Cinnamon and cognitive function: a systematic review of preclinical and clinical studies. Nutr Neurosci. (2023) 2023:1–15. doi: 10.1080/1028415X.2023.2166436
89. Shen, S, Zhang, T, Yuan, Y, Lin, S, Xu, J, and Ye, H. Effects of cinnamaldehyde on Escherichia coli and Staphylococcus aureus membrane. Food Control. (2015) 47:196–202. doi: 10.1016/j.foodcont.2014.07.003
90. Kot, B, Wicha, J, Piechota, M, Wolska, K, and Gruzewska, A. Antibiofilm activity of trans-cinnamaldehyde, P-coumaric, and ferulic acids on uropathogenic Escherichia coli. Turk J Med Sci. (2015) 45:919–24. doi: 10.3906/sag-1406-112
91. Jain, S, Rathod, N, Nagi, R, Sur, J, Laheji, A, Gupta, N, et al. Antibacterial effect of Aloe vera gel against oral pathogens: an in-vitro study. J Clin Diagn Res. (2016) 10:ZC41–4. doi: 10.7860/JCDR/2016/21450.8890
92. Raghuveer, D, Pai, VV, Murali, TS, and Nayak, R. Exploring anthraquinones as antibacterial and antifungal agents. Chemistryselect. (2023) 8:e202204537. doi: 10.1002/slct.202204537
93. Liang, XY, Battini, N, Sui, YF, Ansari, MF, Gan, LL, and Zhou, CH. Aloe-Emodin derived azoles as a new structural type of potential antibacterial agents: design, synthesis, and evaluation of the action on membrane, DNA, and Mrsa DNA isomerase. Rsc Med Chem. (2021) 12:602–8. doi: 10.1039/d0md00429d
94. Deng, Z, Bheemanaboina, RRY, Luo, Y, and Zhou, C-H. Aloe emodin-conjugated sulfonyl hydrazones as novel type of antibacterial modulators against S. aureus 25923 through multifaceted synergistic effects. Bioorg Chem. (2022) 127:106035. doi: 10.1016/j.bioorg.2022.106035
95. Li, T, Lu, Y, Zhang, H, Wang, L, Beier, RC, Jin, Y, et al. Antibacterial activity and membrane-targeting mechanism of aloe-emodin against Staphylococcus epidermidis. Front Microbiol. (2021) 12:621866. doi: 10.3389/fmicb.2021.621866
96. Wang, J, Zhao, H, Kong, W, Jin, C, Zhao, Y, Qu, Y, et al. Microcalorimetric assay on the antimicrobial property of five hydroxyanthraquinone derivatives in rhubarb (Rheum palmatum L.) to Bifidobacterium adolescentis. Phytomedicine. (2010) 17:684–9. doi: 10.1016/j.phymed.2009.10.009
97. Nagano, K, Murakami, Y, Nishikawa, K, Sakakibara, J, Shimozato, K, and Yoshimura, F. Characterization of raga and Ragb in Porphyromonas gingivalis: study using gene-deletion mutants. J Med Microbiol. (2007) 56:1536–48. doi: 10.1099/jmm.0.47289-0
98. Potempa, J, Madej, M, and Scott, DA. The Raga and Ragb proteins of Porphyromonas gingivalis. Mol Oral Microbiol. (2021) 36:225–32. doi: 10.1111/omi.12345
99. Lee, JH, Kim, YG, and Lee, J. Inhibition of Staphylococcus aureus biofilm formation and virulence factor production by petroselinic acid and other unsaturated C18 fatty acids. Microbiol Spectrum. (2022) 10:e0133022. doi: 10.1128/spectrum.01330-22
100. Ajiboye, TO, Habibu, RS, Saidu, K, Haliru, FZ, Ajiboye, HO, Aliyu, NO, et al. Involvement of oxidative stress in protocatechuic acid-mediated bacterial lethality. Microbiology. (2017) 6:e00472. doi: 10.1002/mbo3.472
101. Da-Costa-Rocha, I, Bonnlaender, B, Sievers, H, Pischel, I, Hibiscus, HM, and Sabdariffa, L. A phytochemical and pharmacological review. Food Chem. (2014) 165:424–43. doi: 10.1016/j.foodchem.2014.05.002
102. Kim, D, and Kang, KH. Anti-inflammatory and anti-bacterial potential of mulberry leaf extract on oral microorganisms. Int J Env Res Pub He. (2022) 19:4984. doi: 10.3390/ijerph19094984
103. Cong, S, Tong, Q, Peng, Q, Shen, T, Zhu, X, Xu, Y, et al. In vitro anti-bacterial activity of diosgenin on Porphyromonas gingivalis and Prevotella intermedia. Mol Med Rep. (2020) 22:5392–8. doi: 10.3892/mmr.2020.11620
104. Radaic, A, and Kapila, YL. The oralome and its dysbiosis: new insights into oral microbiome-host interactions. Comput Struct Biotechnol J. (2021) 19:1335–60. doi: 10.1016/j.csbj.2021.02.010
105. Biswas, SK. Does the interdependence between oxidative stress and inflammation explain the antioxidant paradox? Oxidative Med Cell Longev. (2016) 2016:5698931. doi: 10.1155/2016/5698931
106. Carneiro Sczepanik, FS, Grossi, ML, Casati, M, Goldberg, M, Glogauer, M, Fine, N, et al. Periodontitis is an inflammatory disease of oxidative stress: we should treat it that way. Periodontol 2000. (2020) 84:45–68. doi: 10.1111/prd.12342
107. Loi, F, Cordova, LA, Pajarinen, J, Lin, T-h, Yao, Z, and Goodman, SB. Inflammation, fracture and bone repair. Bone. (2016) 86:119–30. doi: 10.1016/j.bone.2016.02.020
108. Terkawi, MA, Matsumae, G, Shimizu, T, Takahashi, D, Kadoya, K, and Iwasaki, N. Interplay between inflammation and pathological bone resorption: insights into recent mechanisms and pathways in related diseases for future perspectives. Int J Mol Sci. (2022) 23:1786. doi: 10.3390/ijms23031786
109. Yu, B, and Wang, C-Y. Osteoporosis and periodontal diseases - an update on their association and mechanistic links. Periodontol. (2022) 89:99–113. doi: 10.1111/prd.12422
110. Ou, YJ, Yan, MD, Gao, GL, Wang, WJ, Lu, QQ, and Chen, J. Cinnamaldehyde protects against ligature-induced periodontitis through the inhibition of microbial accumulation and inflammatory responses of host immune cells. Food Funct. (2022) 13:8091–106. doi: 10.1039/d2fo00963c
111. Bhattarai, G, Poudel, SB, Kook, SH, and Lee, JC. Resveratrol prevents alveolar bone loss in an experimental rat model of periodontitis. Acta Biomater. (2016) 29:398–408. doi: 10.1016/j.actbio.2015.10.031
112. Hu, P, Huang, P, and Chen, MW. Curcumin attenuates cyclooxygenase-2 expression via inhibition of the Nf-B pathway in lipopolysaccharide-stimulated human gingival fibroblasts. Cell Biol Int. (2013) 37:443–8. doi: 10.1002/cbin.10050
113. Xiao, CJ, Yu, XJ, Xie, JL, Liu, S, and Li, S. Protective effect and related mechanisms of curcumin in rat experimental periodontitis. Head Face Med. (2018) 14:12. doi: 10.1186/s13005-018-0169-1
114. Guimaraes, MR, de Aquino, SG, Coimbra, LS, Spolidorio, LC, Kirkwood, KL, and Rossa, C. Curcumin modulates the immune response associated with Lps-induced periodontal disease in rats. Innate Immun. (2012) 18:155–63. doi: 10.1177/1753425910392935
115. Sun, YR, Zhao, BH, Li, ZB, and Wei, JM. Beneficial effects of glycyrrhizin in chronic periodontitis through the inhibition of inflammatory response. Dose-Response. (2020) 18:52660. doi: 10.1177/1559325820952660
116. Akutagawa, K, Fujita, T, Ouhara, K, Takemura, T, Tari, M, Kajiya, M, et al. Glycyrrhizic acid suppresses inflammation and reduces the increased glucose levels induced by the combination of Porphyromonas gulae and ligature placement in diabetic model mice. Int Immunopharmacol. (2019) 68:30–8. doi: 10.1016/j.intimp.2018.12.045
117. Kim, SR, Jeon, HJ, Park, HJ, Kim, MK, Choi, WS, Jang, HO, et al. Glycyrrhetinic acid inhibits Porphyromonas gingivalis lipopolysaccharide-induced vascular permeability via the suppression of interleukin-8. Inflamm Res. (2013) 62:145–54. doi: 10.1007/s00011-012-0560-5
118. Zhu, L, Wei, H, Wu, Y, Yang, S, Xiao, L, Zhang, J, et al. Licorice isoliquiritigenin suppresses rankl-induced osteoclastogenesis in vitro and prevents inflammatory bone loss in vivo. Int J Biochem Cell Biol. (2012) 44:1139–52. doi: 10.1016/j.biocel.2012.04.003
119. Yang, M, Shrestha, SK, Soh, Y, and Heo, SM. Effects of aloe-emodin on alveolar bone in Porphyromonas gingivalis-induced periodontitis rat model: a pilot study. J Periodontal Implant Sci. (2022) 52:383–93. doi: 10.5051/jpis.2104060203
120. Na, HS, Song, YR, Kim, S, Heo, JY, Chung, HY, and Chung, J. Aloin inhibits interleukin (Il)-1β-stimulated Il-8 production in kb cells. J Periodontol. (2016) 87:e108–15. doi: 10.1902/jop.2016.150447
121. Sun, M, Ji, Y, Zhou, S, Chen, R, Yao, H, and Du, M. Ginsenoside Rb3 inhibits osteoclastogenesis via Erk/Nf-kappa B signaling pathway in vitro and in vivo. Oral Dis. (2022) 2022:14352. doi: 10.1111/odi.14352
122. Chu, K, Zhang, Z, Chu, Y, Xu, Y, Yang, W, and Guo, L. Ginsenoside Rg1 alleviates lipopolysaccharide-induced pyroptosis in human periodontal ligament cells via inhibiting Drp1-mediated mitochondrial fission. Arch Oral Biol. (2023) 147:105632. doi: 10.1016/j.archoralbio.2023.105632
123. Hirata, N, Ichimaru, R, Tominari, T, Matsumoto, C, Watanabe, K, Taniguchi, K, et al. Beta-cryptoxanthin inhibits lipopolysaccharide-induced osteoclast differentiation and bone resorption via the suppression of inhibitor of Nf-B kinase activity. Nutrients. (2019) 11:368. doi: 10.3390/nu11020368
124. Nonaka, K, Bando, M, Sakamoto, E, Inagaki, Y, Naruishi, K, Yumoto, H, et al. 6-Shogaol inhibits advanced glycation end-products-induced Il-6 and Icam-1 expression by regulating oxidative responses in human gingival fibroblasts. Molecules. (2019) 24:3705. doi: 10.3390/molecules24203705
125. Kim, YG, Kim, MO, Kim, SH, Kim, HJ, Pokhrel, NK, Lee, JH, et al. 6-Shogaol, an active ingredient of ginger, inhibits osteoclastogenesis and alveolar bone resorption in ligature-induced periodontitis in mice. J Periodontol. (2020) 91:809–18. doi: 10.1002/JPER.19-0228
126. Ryu, EY, Park, SY, Kim, SG, Park, DJ, Kang, JS, Kim, YH, et al. Anti-inflammatory effect of heme oxygenase-1 toward Porphyromonas gingivalis lipopolysaccharide in macrophages exposed to gomisins a, G, and. J Med Food. (2011) 14:1519–26. doi: 10.1089/jmf.2011.1656
127. Park, SY, Park, DJ, Kim, YH, Kim, Y, Choi, Y-W, and Lee, S-J. Schisandra chinensis alpha-iso-cubebenol induces heme oxygenase-1 expression through Pi3k/Akt and Nrf2 signaling and has anti-inflammatory activity in Porphyromonas gingivalis lipopolysaccharide-stimulated macrophages. Int Immunopharmacol. (2011) 11:1907–15. doi: 10.1016/j.intimp.2011.07.023
128. Park, SY, Park, DJ, Kim, YH, Kim, Y, Kim, SG, Shon, KJ, et al. Upregulation of heme oxygenase-1 via Pi3k/Akt and Nrf-2 signaling pathways mediates the anti-inflammatory activity of schisandrin in Porphyromonas gingivalis Lps-stimulated macrophages. Immunol Lett. (2011) 139:93–101. doi: 10.1016/j.imlet.2011.05.007
129. Zhang, Y, Yan, M, Yu, QF, Yang, PF, Zhang, HD, Sun, YH, et al. Puerarin prevents Lps-induced osteoclast formation and bone loss via inhibition of Akt activation. Biol Pharm Bull. (2016) 39:2028–35. doi: 10.1248/bpb.b16-00522
130. Gou, H, Chen, X, Zhu, X, Li, L, Hou, L, Zhou, Y, et al. Sequestered Sqstm1/P62 crosstalk with Keap1/Nrf2 Axis in Hpdlcs promotes oxidative stress injury induced by periodontitis. Free Radic Biol Med. (2022) 190:62–74. doi: 10.1016/j.freeradbiomed.2022.08.001
131. Tamura, T, Zhai, R, Takemura, T, Ouhara, K, Taniguchi, Y, Hamamoto, Y, et al. Anti-inflammatory effects of geniposidic acid on Porphyromonas gingivalis-induced periodontitis in mice. Biomedicine. (2022) 10:3096. doi: 10.3390/biomedicines10123096
132. Lu, SH, Hsu, WL, Chen, TH, and Chou, TC. Activation of Nrf2/ho-1signaling pathway involves the anti-inflammatory activity of Magnolol in Porphyromonas gingivalis lipopolysaccharide-stimulated mouse raw 264.7 macrophages. Int Immunopharmacol. (2015) 29:770–8. doi: 10.1016/j.intimp.2015.08.042
133. Lu, SH, Huang, RY, and Chou, TC. Magnolol ameliorates ligature-induced periodontitis in rats and osteoclastogenesis: in vivo and in vitro study. Evid Based Complement Alternat. (2013) 2013:634095. doi: 10.1155/2013/634095
134. Pei, ZH, Zhang, FQ, Niu, ZY, and Shi, SG. Effect of icariin on cell proliferation and the expression of bone resorption/formation-related markers in human periodontal ligament cells. Mol Med Rep. (2013) 8:1499–504. doi: 10.3892/mmr.2013.1696
135. Lv, XC, Bi, LJ, Jiang, Y, and Wang, X. Effects of icariin on the alkline phosphatase activity of human periodontal ligament cells inhibited by lipopolysaccharide. Mol Med Rep. (2013) 8:1411–5. doi: 10.3892/mmr.2013.1677
136. Sharifi-Rad, J, Dey, A, Koirala, N, Shaheen, S, El Omari, N, Salehi, B, et al. Cinnamomum species: bridging phytochemistry knowledge, pharmacological properties and toxicological safety for health benefits. Front Pharmacol. (2021) 12:600139. doi: 10.3389/fphar.2021.600139
137. Singh, N, Rao, AS, Nandal, A, Kumar, S, Yadava, SS, Ganaie, SA, et al. Phytochemical and pharmacological review of Cinnamomum verum J. Presl-a versatile spice used in food and nutrition. Food Chem. (2021) 338:127773. doi: 10.1016/j.foodchem.2020.127773
138. Zhang, H, and Tsao, R. Dietary polyphenols, oxidative stress and antioxidant and anti-inflammatory effects. Curr Opin Food Sci. (2016) 8:33–42. doi: 10.1016/j.cofs.2016.02.002
139. Działo, M, Mierziak, J, Korzun, U, Preisner, M, Szopa, J, and Kulma, A. The potential of plant phenolics in prevention and therapy of skin disorders. Int J Mol Sci. (2016) 17:160. doi: 10.3390/ijms17020160
140. Wu, XJ, Cai, B, Lu, W, Fu, Y, Wei, B, Niu, Q, et al. Hbv upregulated triggering receptor expressed on myeloid Cells-1 (Trem-1) expression on monocytes participated in disease progression through Nf-kb pathway. Clin Immunol. (2021):223. doi: 10.1016/j.clim.2020.108650
141. Zhu, LF, Li, L, Wang, XQ, Pan, L, Mei, YM, Fu, YW, et al. M1 macrophages regulate Tlr4/Ap1 via paracrine to promote alveolar bone destruction in periodontitis. Oral Dis. (2019) 25:1972–82. doi: 10.1111/odi.13167
142. Bassiouni, W, Ali, MAM, and Schulz, R. Multifunctional intracellular matrix metalloproteinases: implications in disease. FEBS J. (2021) 288:7162–82. doi: 10.1111/febs.15701
143. Li, X, Liang, X, Li, S, Qi, X, Du, N, and Yang, D. Effect of environmental tobacco smoke on cox-2 and Shp-2 expression in a periodontitis rat model. Oral Dis. (2021) 27:338–47. doi: 10.1111/odi.13538
144. Cheng, S, Chen, C, and Wang, L. Gelsemine exerts neuroprotective effects on neonatal mice with hypoxic-ischemic brain injury by suppressing inflammation and oxidative stress via Nrf2/ho-1 pathway. Neurochem Res. (2022) 48:1305–19. doi: 10.1007/s11064-022-03815-6
145. Mohammad, CA, Ali, KM, Al-Rawi, RA, and Gul, SS. Effects of curcumin and tetracycline gel on experimental induced periodontitis as an anti-inflammatory, osteogenesis promoter and enhanced bone density through altered Iron levels: histopathological study. Antibiotics. (2022) 11:521. doi: 10.3390/antibiotics11040521
146. Semwal, RB, Semwal, DK, Combrinck, S, and Viljoen, AM. Gingerols and shogaols: important nutraceutical principles from ginger. Phytochemistry. (2015) 117:554–68. doi: 10.1016/j.phytochem.2015.07.012
147. Nonaka, K, Kajiura, Y, Bando, M, Sakamoto, E, Inagaki, Y, Lew, JH, et al. Advanced glycation end-products increase Il-6 and Icam-1 expression via Rage, Mapk and Nf-kappa B pathways in human gingival fibroblasts. J Periodontal Res. (2018) 53:334–44. doi: 10.1111/jre.12518
148. Zhang, XL, Han, NN, Li, GQ, Yang, HQ, Cao, YY, Fan, ZP, et al. Local icariin application enhanced periodontal tissue regeneration and relieved local inflammation in a minipig model of periodontitis. Int. J Oral Sci. (2018):10. doi: 10.1038/s41368-018-0020-3
149. Park, WS, Koo, KA, Bae, J-Y, Kim, H-J, Kang, D-M, Kwon, J-M, et al. Dibenzocyclooctadiene lignans in plant parts and fermented beverages of Schisandra chinensis. Plants-Basel. (2021) 10:361. doi: 10.3390/plants10020361
150. Yang, W, Chen, X, Li, Y, Guo, S, Wang, Z, and Yu, X. Advances in pharmacological activities of terpenoids. Nat Prod Commun. (2020) 15:3555. doi: 10.1177/1934578x20903555
151. Song, SH, Choi, SM, Kim, JE, Sung, JE, Lee, HA, Choi, YH, et al. Alpha-isocubebenol alleviates scopolamine-induced cognitive impairment by repressing acetylcholinesterase activity. Neurosci Lett. (2017) 638:121–8. doi: 10.1016/j.neulet.2016.12.012
152. Yang, M, Soh, Y, and Heo, SM. Characterization of acidic mammalian chitinase as a novel biomarker for severe periodontitis (stage iii/iv): a pilot study. Int J Environ Res Public Health. (2022) 19:4113. doi: 10.3390/ijerph19074113
153. Xiao, J, Chen, S, Chen, Y, and Su, J. The potential health benefits of aloin from genus Aloe. Phytother Res. (2022) 36:873–90. doi: 10.1002/ptr.7371
154. Sun, M, Ji, Y, Li, Z, Chen, R, Zhou, S, Liu, C, et al. Ginsenoside Rb3 inhibits pro-inflammatory cytokines via Mapk/Akt/Nf-kappa B pathways and attenuates rat alveolar bone resorption in response to Porphyromonas gingivalis Lps. Molecules. (2020) 25:4815. doi: 10.3390/molecules25204815
155. Oyama, M, Ukai, T, Yamashita, Y, and Yoshimura, A. High-mobility group box 1 released by traumatic occlusion accelerates bone resorption in the root furcation area in mice. J Periodontal Res. (2021) 56:186–94. doi: 10.1111/jre.12813
156. Yamamoto, T, Ono, T, Ito, T, Yamanoi, A, Maruyama, I, and Tanaka, T. Hemoperfusion with a high-mobility group box 1 adsorption column can prevent the occurrence of hepatic ischemia-reperfusion injury in rats. Crit Care Med. (2010) 38:879–85. doi: 10.1097/CCM.0b013e3181c58951
157. Bailly, C, and Vergoten, G. Glycyrrhizin: an alternative drug for the treatment of Covid-19 infection and the associated respiratory syndrome? Pharmacol Ther. (2020):214. doi: 10.1016/j.pharmthera.2020.107618
158. Morimoto-Yamashita, Y, Ito, T, Kawahara, K-i, Kikuchi, K, Tatsuyama-Nagayama, S, Kawakami-Morizono, Y, et al. Periodontal disease and type 2 diabetes mellitus: is the Hmgb1-rage axis the missing link? Med Hypotheses. (2012) 79:452–5. doi: 10.1016/j.mehy.2012.06.020
159. Takamori, A, Yoshinaga, Y, Ukai, T, Nakamura, H, Takamori, Y, Izumi, S, et al. Topical application of glycyrrhetinic acid in the gingival sulcus inhibits attachment loss in lipopolysaccharide-induced experimental periodontitis in rats. J Periodontal Res. (2018) 53:422–9. doi: 10.1111/jre.12529
160. Lee, HJ, Lee, H, Kim, MH, Choi, YY, Ahn, KS, Um, JY, et al. Angelica dahurica ameliorates the inflammation of gingival tissue via regulation of pro-inflammatory mediators in experimental model for periodontitis. J Ethnopharmacol. (2017) 205:16–21. doi: 10.1016/j.jep.2017.04.018
161. Ryu, EY, Park, AJ, Park, SY, Park, SH, Eom, HW, Kim, YH, et al. Inhibitory effects of Ginkgo biloba extract on inflammatory mediator production by Porphyromonas gingivalis lipopolysaccharide in murine macrophages via Nrf-2 mediated heme oxygenase-1 signaling pathways. Inflammation. (2012) 35:1477–86. doi: 10.1007/s10753-012-9461-6
162. Kabir, MA, Fujita, T, Ouhara, K, Kajiya, M, Matsuda, S, Shiba, H, et al. Houttuynia cordata suppresses the Aggregatibacter Actinomycetemcomitans-induced increase of inflammatory-related genes in cultured human gingival epithelial cells. J Dent Sci. (2015) 10:88–94. doi: 10.1016/j.jds.2014.03.004
163. Jang, HY, Lee, HS, Noh, EM, Kim, JM, You, YO, Lee, G, et al. Aqueous extract of Chrysanthemum morifolium Ramat. Inhibits Rankl-induced osteoclast differentiation by suppressing the C-Fos/Nfatc1 pathway. Arch Oral Biol. (2021) 122:105029. doi: 10.1016/j.archoralbio.2020.105029
164. Song, HK, Noh, EM, Kim, JM, You, YO, Kwon, KB, and Lee, YR. Evodiae fructus extract inhibits interleukin-1 beta-induced Mmp-1, Mmp-3, and inflammatory cytokine expression by suppressing the activation of Mapk and Stat-3 in human gingival fibroblasts in vitro. Evid-Based Compl Alt. (2021) 2021:5858393. doi: 10.1155/2021/5858393
165. Babaei, H, Forouzandeh, F, Maghsoumi-Norouzabad, L, Yousefimanesh, HA, Ravanbakhsh, M, and Zare, JA. Effects of chicory leaf extract on serum oxidative stress markers, lipid profile and periodontal status in patients with chronic periodontitis. J Am Coll Nutr. (2018) 37:479–86. doi: 10.1080/07315724.2018.1437371
166. Gao, Q, Li, X, Huang, H, Guan, Y, Mi, Q, and Yao, J. The efficacy of a chewing gum containing Phyllanthus emblica fruit extract in improving oral health. Curr Microbiol. (2018) 75:604–10. doi: 10.1007/s00284-017-1423-7
Keywords: medicine food homology plants, periodontitis, antibiosis, virulence factor, anti-inflammatory, bone resorption
Citation: Qu S, Yu S, Ma X and Wang R (2023) “Medicine food homology” plants promote periodontal health: antimicrobial, anti-inflammatory, and inhibition of bone resorption. Front. Nutr. 10:1193289. doi: 10.3389/fnut.2023.1193289
Edited by:
Andrea K. Boggild, University of Toronto, CanadaReviewed by:
Chunsheng Hou, Chinese Academy of Agricultural Sciences, ChinaNorsham Juliana, Universiti Sains Islam Malaysia, Malaysia
Copyright © 2023 Qu, Yu, Ma and Wang. This is an open-access article distributed under the terms of the Creative Commons Attribution License (CC BY). The use, distribution or reproduction in other forums is permitted, provided the original author(s) and the copyright owner(s) are credited and that the original publication in this journal is cited, in accordance with accepted academic practice. No use, distribution or reproduction is permitted which does not comply with these terms.
*Correspondence: Rui Wang, d19ydWlAamx1LmVkdS5jbg==