- 1Department of Gastroenterology and Hepatology, The Third Affiliated Hospital, Southern Medical University, Guangzhou, China
- 2The Third School of Clinical Medicine, Southern Medical University, Guangzhou, China
- 3Department of Orthopedics, The Third Affiliated Hospital, Southern Medical University, Guangzhou, China
A high-fat diet plays a key role in the pathogenesis of colorectal cancer, and this effect on the gut can also occur in the offspring of mothers with a high-fat diet. In this review, we discuss the role of a high-fat diet in the pathogenesis of colorectal cancer and summarize the effects of a maternal high-fat diet on the activation of inflammation and development of colorectal cancer in offspring. Studies have found that a maternal high-fat diet primarily induces an inflammatory response in the colorectal tissue of both the mother herself and the offspring during pregnancy. This leads to the accumulation of inflammatory cells in the colorectal tissue and the release of inflammatory cytokines, which further activate the NF-κb and related inflammatory signaling pathways. Research suggests that high levels of lipids and inflammatory factors from mothers with a high-fat diet are passed to the offspring through the transplacental route, which induces colorectal inflammation, impairs the intestinal microecological structure and the intestinal barrier, and interferes with intestinal development in the offspring. This in turn activates the NF-κb and related signaling pathways, which further aggravates intestinal inflammation. This process of continuous inflammatory stimulation and repair may promote the uncontrolled proliferation of colorectal mucosal cells in the offspring, thus increasing their susceptibility to colorectal cancer.
1. Introduction
Colorectal cancer (CRC) is a common malignant tumor of the digestive tract. According to the global cancer statistics published by the World Health Organization (WHO) in 2020, CRC was the third most commonly diagnosed cancer (incidence: 10.0%) and the second most deadly cancer (mortality: 9.4%) (1). It is estimated that the incidence of CRC will increase by 60% by 2030, affecting ~2.2 million people (2). CRC can be divided into two main types based on the carcinogenesis process: one derived from adenomas and the other associated with inflammation (3). Notably, in both adenoma-derived CRC and inflammation-associated CRC, the continuous stimulation of inflammation and cell proliferation persists throughout the process of CRC development (4). However, the pathogenesis of CRC is not yet clear, and any external risk factors that can cause colorectal inflammation may lead to the onset and development of CRC.
Obesity is a common public health issue worldwide and is recognized as a major risk factor for cancer (5). In the United States, 5% of male cancer cases and 10% of female cancer cases among patients aged over 30 were associated with obesity from 2011 to 2015 (6). Multiple studies have found that obesity increases the risk of CRC (7–9). Obesity is mostly caused by the excessive intake of dietary fat, accompanied by impaired lipid metabolism, and often causes systemic inflammation, with the process of cell repair following inflammation being accompanied by continuous cell proliferation. This high-fat-diet-induced inflammation is suggested to be closely associated with the onset and development of CRC. Furthermore, a maternal high-fat diet has been shown to increase the incidence of malignant tumors in the offspring (Table 1). In previous studies, increased risks of breast cancer were found in the offspring of mothers who consumed a high-fat diet, and an increased risk of tumor recurrence was found in the offspring of breast cancer patients with a high-fat diet (10, 11). Studies have also shown a maternal high-fat diet to be a risk factor for pancreatic cancer and primary liver cancer (12–14) as well as colorectal inflammation and CRC (15) in offspring. This finding has great significance for in-depth investigation of the pathogenesis and treatment of CRC.
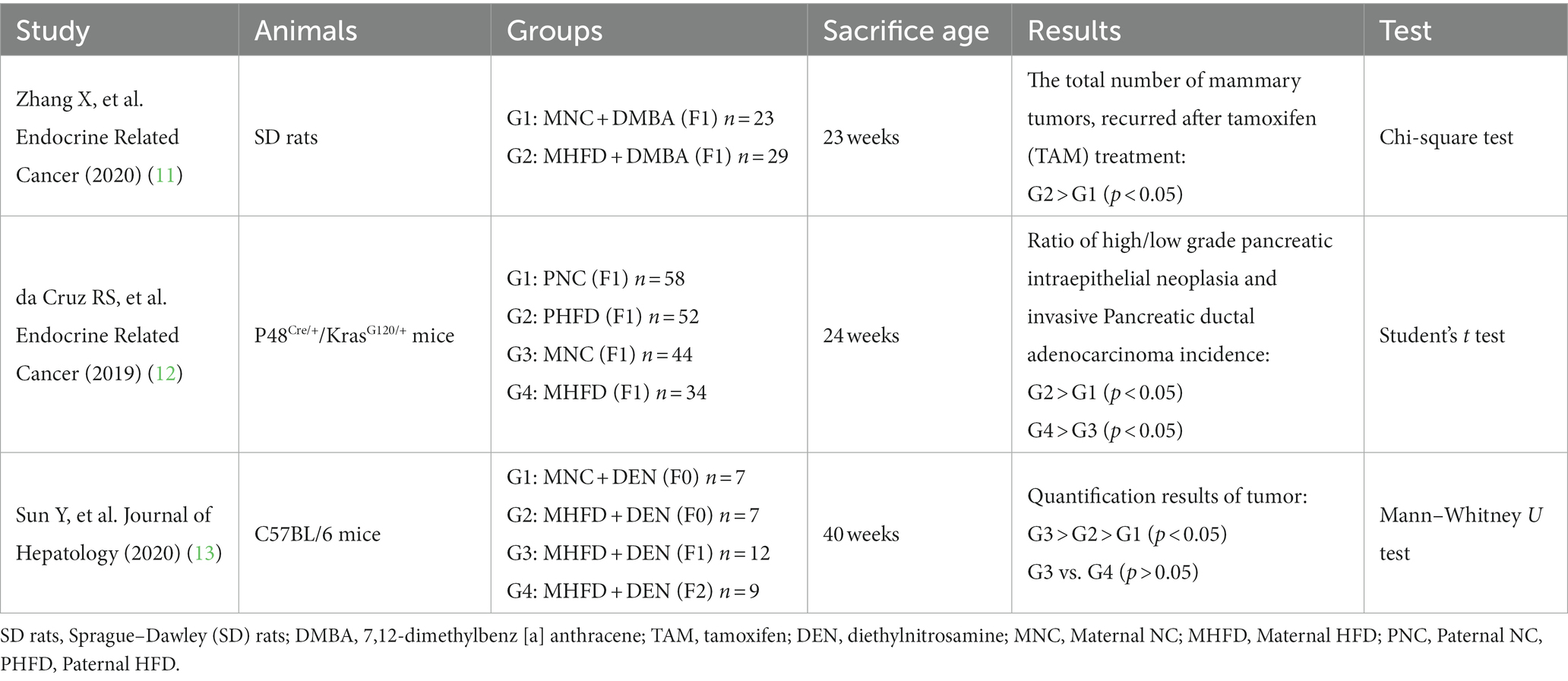
Table 1. Study on the relationship between high-fat diet of parents and malignant tumor in offspring.
In this review, we used the keywords “high fat diet” or “obesity,” “offspring,” and “colorectal” to summarize the literature on the Pubmed website1 from 2000 to 2023. We summarize the role of a maternal high-fat diet in colorectal inflammation activation and carcinogenesis in offspring and speculate the possible mediators and signal transduction pathways involved in CRC development in offspring. This review highlights that a maternal high-fat-diet-induced CRC onset in offspring is possibly mediated by the activation of intestinal inflammation. This review explores the role of a high-fat diet in colorectal health and disease and its mechanism of action in CRC development and progression. Improving the maternal dietary structure and avoiding the long-term consumption of a high-fat diet may be necessary measures to prevent CRC in offspring.
2. High-fat diet promotes maternal CRC by activating intestinal inflammation
A high-fat diet has been linked to an increased incidence of CRC (16, 17). A meta-analysis of studies on CRC risk factors that followed participants for up to 10 years showed that participants with abnormally high lipid levels had a significantly increased risk of CRC compared with participants with normal lipid levels (18). Early studies have found that a high saturated fatty acid intake increases the risk of CRC or precancerous lesions and that animal fat intake is positively correlated with the risk of CRC (19, 20). Liu et al. also confirmed this finding in a retrospective cohort study of CRC patients (21). Another study showed that under the same exposure conditions to carcinogenic agents, the colorectal cells of high-fat diet-fed mice proliferated actively and the levels of the intestinal crypt cell-related proliferation factor Ki67 increased compared with the colorectal cells of normal diet-fed mice (22). In some studies, colorectal tissue exposed to a high-fat diet showed elevated expression levels of stem cell markers and a significant tendency to become cancerous (23, 24).
The susceptibility to colorectal carcinogenesis caused by a high-fat diet may be associated with inflammation caused by increased serum levels of inflammatory cytokines (25). Chronic inflammation has been shown to promote cell proliferation and repair, possibly inducing cell overproliferation and malignant transformation (26). A study found that, under the treatment of dextran sodium sulfate (DSS), the levels of the inflammatory cytokines interleukin (IL)-1β, IL-6 and tumor necrosis factor (TNF) were significantly increased in the colorectal tissue of high-fat diet mice compared with mice on the normal diet (27). Notably, the levels of colorectal inflammatory cytokines were also significantly increased in mice fed a high-fat diet without DSS compared with mice on a normal diet (28). IL-1β, IL-6 and TNF are proinflammatory cytokines secreted by macrophages. Our previous study found that mice fed a high-fat diet that were not treated with carcinogenic or pro-inflammatory drugs demonstrated visceral lipid accumulation, reduced colorectal length and significantly increased levels of macrophages and the tumor stem cell markers CD133 and CD44 in the colorectal tissue (29). This result confirmed that a high-fat diet promotes colorectal inflammation and potentially induces CRC development.
According to the above reports, intestinal inflammation is an important step in the CRC induction mechanism of a high-fat diet. Macrophages, as important immune cells, secrete the inflammatory cytokines IL-1β, IL-6 and TNF, which are involved in the NF-κb pathway, an important signaling pathway involved in the pathogenesis of CRC (30). In addition, a high-fat diet often causes intestinal dysbacteriosis, which damages the intestinal mucosal barrier. The resulting inflammatory response upregulates the levels of circulating lipopolysaccharide and TNF-α, both of which further activate the NF-κb pathway (31). The NF-κb pathway regulates inflammatory responses, and the activation of this signaling pathway may be a mechanism by which high-fat diet-related inflammatory responses promote the onset and development of CRC. Thus, the inflammation caused by a high-fat diet activates the relevant signaling pathways and further destroys the intestinal barrier. The process of continuous proliferation and repair in the intestinal mucosa may cause the uncontrolled proliferation of colorectal mucosal cells, which may induce the proliferation of tumor stem cells, eventually leading to CRC. You may insert up to 5 heading levels into your manuscript as can be seen in “Styles” tab of this template. These formatting styles are meant as a guide, as long as the heading levels are clear, Frontiers style will be applied during typesetting.
3. Maternal high-fat diet causes lipid metabolism disorder in offspring
A high-fat diet not only increases blood lipid levels and causes visceral fat accumulation in parents but also affects lipid metabolism in offspring during pregnancy. Epidemiological studies have found that maternal obesity and weight gain during pregnancy increase the risk of adverse outcomes, such as obesity, in infants (32–35), and children with obesity are more likely to have lifelong obesity, which compromises their health and life expectancy (36). Obesity and metabolic abnormalities have a genetic predisposition, and most studies have confirmed that babies born to mothers who consumed a high-fat diet during pregnancy were more likely to develop metabolic syndrome and exhibit weight gain and elevated lipid levels that may continue into adulthood (36–39). Similar findings have been found in animal studies wherein the offspring of mice fed a high-fat diet were more likely to develop neonatal obesity than the offspring of mice fed a normal diet. When mice were fed a high-fat diet during pregnancy, their offspring showed significant metabolic abnormalities such as visceral fat accumulation and elevated blood lipids, even after the offspring were fed a normal diet. Similar effects were also observed in their grandpups under normal diet. Preperitoneal fat deposition and systemic inflammation were also found in the offspring mice (25). Other studies have found that a maternal high-fat diet is a risk factor for systemic inflammation and hepatic steatosis in offspring mice (40). Wang et al. found that these offspring mice had not only impaired lipid metabolism but also fatty degeneration of the liver and intestinal flora disorder (41). All of the above studies indicate that a maternal high-fat diet has a significant effect on lipid metabolism in the offspring, which can lead to elevated lipid levels, fat accumulation in multiple organs and systemic inflammation.
The intestinal flora also plays an important role in lipid metabolism disorder. A high-fat diet strongly affects the intestinal flora, and the intestinal flora further has a feedback effect on the process of lipid metabolism. Intestinal microbes can regulate the function of fat cells and affect the nutritional intake and metabolism of the host to regulate the energy balance in the body (42). A high-fat diet often causes intestinal dysbacteriosis (43, 44), which can in turn disrupt the host energy balance by dysregulating fat cells and further promote lipid metabolism disorders. In multiple studies, the gut microbial composition of offspring and grandpups in the maternal high-fat diet group was different from that in the control group (25, 27, 45). The changes in the intestinal flora of the offspring of mice fed a high-fat diet were mainly manifested as an increase in the abundance of Firmicutes (mainly Lactococcus), a decrease in the abundance of γ-Proteobacteria (mainly Escherichia) and a partial increase in the abundance of Betaproteobacteria (mainly Comamonas), which are associated with a high-fat environment in the intestine (46). Furthermore, the offspring of mice treated with probiotics showed improved composition of the intestinal flora, improved intestinal microecological function and significantly decreased body weight and lipid levels compared with the offspring of mice fed a high-fat diet (47). It can be concluded that the changes in the intestinal flora of offspring caused by a maternal high-fat diet play an important role in promoting lipid metabolism disorder in the offspring.
The exact mechanism of lipid metabolism disorder in offspring caused by a maternal high-fat diet is not clear. Research indicates that some of the energy in the pregnant woman is converted to and stored as fat in the fetus. Mothers with pre-existing metabolic disorders or metabolic disorders developed during pregnancy have elevated blood lipid levels. This metabolic imbalance leads to excessive energy storage in the fetus during pregnancy. The insulin resistance caused by metabolic imbalance during pregnancy further elevates maternal blood glucose and blood lipid levels, resulting in excessive transplacental transfer of glucose, triglycerides and fatty acids to the fetus (48). The placenta is the key organ for nutrition and waste exchange between mother and fetus, and thus, maternal nutrition and placental status play a crucial role in regulating the growth and development of the fetus (49, 50). Fatty acids in the maternal circulation can pass to the fetal circulation through transplacental transfer (51). Zhu et al. found that the fetuses of obese ewes had significantly increased levels of circulating free fatty acids, cholesterol and triglycerides compared with the fetuses of normal ewes (52). Impaired maternal lipid metabolism may affect fetal energy metabolism through the transplacental route and cause lipid metabolism disorders in offspring. In addition, maternal obesity causes increased levels of glucose and amino acid transporter transcription in the placenta as a result of impaired placental function (53, 54). Impaired placental function may also cause metabolic abnormalities in offspring. A maternal high-fat diet indices a series of inflammatory reactions, such as oxidative stress, in the placenta (55). Macrophages activated in the placenta dysregulate the blood vessels of the placenta, resulting in decreased vascular maturity and decreased blood flow in the placenta (52, 53); this further aggravates hypoxia and inflammatory reactions in the placenta, leading to maladaptation of the placenta and impaired placental function (31). A study that isolated umbilical cord-derived mesenchymal stem cells found that infants with inherited obesity had impaired fatty acid metabolism in the stem cells (56), which may be a manifestation of impaired placental function.
The above studies suggest that a maternal high-fat diet disrupts lipid metabolism in the offspring via the transplacental transport of high levels of lipid into fetal circulation. In addition, metabolic disorders in the offspring may be related to placental function. The disrupted maternal lipid metabolism activates inflammation in the placenta, which damages placental function and in turn impairs offspring lipid metabolism. In addition, intestinal dysbacteriosis caused by maternal lipid metabolism disorder is also reflected in the offspring intestinal microecology, which further affects lipid metabolism in the offspring. However, the specific mechanism of lipid metabolism disorder in offspring caused by a maternal high-fat diet still needs to be clarified.
4. Maternal high-fat diet causes intestinal inflammation and damages the epithelial barrier function of the intestinal mucosa in offspring
A maternal high-fat diet can disrupt lipid metabolism in offspring, increasing their susceptibility to intestinal inflammation, and has been shown to promote the early onset of intestinal inflammation in offspring (57). For example, Xie et al. demonstrated that the offspring of mice fed a high-fat diet were more susceptible to DSS-induced colitis in adulthood than those of mice fed a normal diet despite being fed a normal diet since birth (27). Furthermore, the gut of these offspring of mice fed a high-fat diet showed severe intestinal ulcers, together with increased mRNA expression of the pro-inflammatory cytokines TNF-α, interferon (IFN)-γ, IL-1β, IL-12, IL-6, IL-17 and IL-22 and decreased mRNA expression of the anti-inflammatory cytokine IL-10. Compared with the offspring of mice fed a normal diet, these offspring had greater weight loss, a higher disease activity index, a significantly shorter colorectum, greater intestinal permeability and inflammation and a significantly lower survival rate (58, 59). In sheep, in addition to elevated levels of proinflammatory cytokines and chemokines in the circulation of parents fed a high-fat diet, the colorectal tissue of their fetuses showed increased expression levels of genes encoding inflammatory signaling proteins and, consequently, increased intestinal permeability after birth. These offspring also had decreased expression levels of intestinal compact junction protein, decreased goblet cell density and a decreased ileum villi–crypt ratio (60). Xue et al. studied the 16-week-old offspring of mice fed a high-fat diet and found that, compared with mice on the normal diet, the serum and intestinal levels of inflammatory factors were significantly increased, while the level of tight junction protein in the intestinal barrier epithelium was decreased. Morphologically, the number of cup cells in the ileum and the height of the intestinal epithelial villi were decreased, while the depth of the intestinal crypt was increased. The decreased villus–crypt ratio suggested intestinal mucosal injury (61). Xie et al. studied the 3-week-old offspring of high-fat diet-fed mice and found that the expression of tight junction protein in the intestinal tissue was inhibited and the membrane localization of the protein was also changed. In addition, the IgA expression cells and SIgA levels in the gut were significantly decreased, while the levels of the inflammatory cytokines IL-6 and TNF-α were significantly increased. (27) This series of studies confirmed that a maternal high-fat diet induces intestinal inflammation and damages the intestinal mucosal barrier in offspring.
A maternal high-fat diet may promote the progression of intestinal inflammation and induce intestinal carcinogenesis in the offspring through vertically transmitted disturbance of the intestinal flora. The establishment of the intestinal flora begins in the fetus. Studies have found that the intestinal microecological state can be passed directly from mother to offspring, and this intestinal colonization often occurs during fetal intrauterine development, delivery and lactation (62, 63). The transfer of intestinal flora disturbances from parents fed a high-fat diet to their offspring may be related to inherited high lipid levels in the offspring (64, 65). Gohir et al. found that abnormal intestinal development in the offspring of parents fed a high-fat diet can also induce intestinal microecological disorder (31). These findings are consistent with the phenomenon of “hereditary intestinal flora disorder” proposed by Barbosa et al., which may also be one of the reasons for the increased susceptibility to intestinal inflammation observed among the offspring of parents fed a high-fat diet. As an integral part of the intestinal environment, the intestinal flora is closely involved in the regulation of the intestinal barrier and lipid metabolism. A high-fat diet causes intestinal dysbacteriosis, which in turn promotes colorectal inflammation and even carcinogenesis (66, 67). These offspring exhibit a damaged intestinal barrier caused by the metabolites of disordered intestinal flora, which also increases susceptibility to intestinal inflammation and carcinogenesis.
5. Maternal high-fat diet increases the susceptibility of offspring to CRC
The offspring of mothers fed a high-fat diet show an increased risk of cancer such as breast cancer, pancreatic cancer and CRC compared with the offspring of mothers fed a normal diet (10–14). A large prospective cohort study from the United States found that maternal obesity and gestational weight gain are important risk factors for CRC in offspring (15). Many animal experiments have found that a maternal high-fat diet causes visceral fat accumulation in offspring (68, 69). This visceral fat accumulation, in turn, significantly increases the risks of CRC and cancer-related death (70). The specific mechanism by which a maternal high-fat diet causes CRC in offspring remains unclear, but the abovementioned studies confirm that a maternal high-fat diet can cause lipid metabolism disorders and intestinal inflammation in offspring. Thus, the increased susceptibility to intestinal cancer in offspring may be attributable to impaired lipid metabolism.
The intestinal growth and development of the fetus begins during pregnancy. A maternal high-fat diet may affect the intestinal growth and development of offspring through inflammation. In rats, it was found that a maternal high-fat diet not only increased the levels of intestinal inflammatory factors but also significantly changed the intestinal tissue morphology in the offspring (71). Studies have shown that placental oxidative stress caused by a high-fat diet not only damages placental blood vessels and induces placental hypoxia but also impairs placental function, thus affecting the intestinal growth and development of the fetus (31). Xie et al. found that the villus length and crypt depth of 3-week-old offspring of mice fed a high-fat diet were significantly smaller than those of mice fed a normal diet (27). Intestinal pluripotent stem cells present in the intestinal crypts can differentiate into various types of mature intestinal cells. The shallow crypt depth of 3-week-old mice might be due to restricted intestinal development in the fetal stage. However, lactation promotes the proliferation of intestinal stem cells, which further differentiate rapidly under the stress of weaning, eventually showing increased intestinal crypt depth and decreased intestinal villus height. (72) Xue et al. found that the height of intestinal epithelial villi was decreased and the depth of intestinal crypts was increased in 16-week-old offspring of mice fed a high-fat diet (27). These findings suggest that, without the influence of maternal factors such as the placenta and lactation, the intestinal growth and development of offspring after weaning are still continuously regulated. The process of cell proliferation and differentiation is accelerated due to a high-fat diet, which increases the proportion of immature intestinal epithelial cells and goblet cells, thus increasing intestinal permeability and resulting in impaired intestinal barrier function. The above studies indicate that a maternal high-fat diet not only affects the intestinal growth and development of the fetus during pregnancy, but also has a continuous effect on the intestinal growth and development of the offspring even after birth and weaning, which may be a manifestation of persistent intestinal inflammation. This developmental abnormality is manifested by accelerated cell proliferation and differentiation, which may increase the susceptibility of offspring to intestinal malignancies.
The molecular mechanism of action of a maternal high-fat diet on the offspring’s gut is not completely clear. Studies have demonstrated that a maternal high-fat diet activates the NF-κb pathway in the offspring’s nervous system, leading to nervous system inflammation (73). Similar, NF-κb pathway activation has also been found in the gut of the offspring of parents fed a high-fat diet, which is often accompanied by significant intestinal inflammation (31). As a classic inflammatory pathway, the NF-κb pathway is closely involved in the inflammatory response caused by impaired lipid metabolism. Thus, NF-κb pathway activation has been suggested as a mechanism by which high-fat-diet-induced inflammation promotes CRC development. Inflammatory factors in the maternal circulation and in the placenta can be transmitted through the placenta to the fetal circulation (51, 74), where these may activate the NF-κb pathway in the offspring (59). In addition, lipid metabolism disorder is a known risk factor for CRC. The high level of lipid and impaired lipid metabolism in the offspring of parents fed a high-fat diet may also increase the risk of CRC via colorectal inflammation. It was found that the increase in free fatty acid concentration in the fetal circulation can enhance the NF-κb signal. (52, 75) The resulting NF-κb pathway activation may further promote colorectal inflammation and carcinogenesis in the offspring.
Taken together, these studies suggest that carcinogenesis in the colorectal tissue of the offspring of parents fed a high-fat diet may be due to mucosal barrier destruction, intestinal dysbacteriosis, and abnormal intestinal growth and development caused by high-fat-diet-induced intestinal inflammation. The activation of the NF-κb pathway may play an important role in this process. This persistent and progressive inflammatory response may be an important factor in the increased susceptibility to CRC in the offspring of parents fed a high-fat diet (Table 2).
6. Conclusion
This review discusses the association of a high-fat diet with CRC development and summarizes the role of a maternal high-fat diet in colorectal inflammation and CRC development in offspring. Our review reveals that a high-fat diet increases visceral fat accumulation in the host, which induces inflammatory cell aggregation and inflammatory cytokine release. This further activates the NF-κb and related signaling pathways, thereby increasing susceptibility to CRC. A maternal high-fat diet also elevates blood lipids and visceral fat accumulation in the offspring via the transplacental transfer of high levels of lipid from the maternal serum, and may impair placental function, causing metabolic disorders in the offspring. These changes also lead to intestinal dysbacteriosis in the offspring, which further aggravates lipid metabolism disorder. The impaired lipid metabolism and maternal and placental inflammatory factors in offspring circulation cause colorectal and systemic inflammation in the offspring. Colorectal inflammation impairs the intestinal barrier, hinders the normal growth and development of the intestine, accelerates the proliferation and differentiation of intestinal pluripotent stem cells and further aggravates intestinal dysbacteriosis. This process of continuous inflammatory stimulation and repair leads to the activation of the NF-κb signaling pathway, the uncontrolled proliferation of colorectal mucosal cells and, eventually, CRC onset (Figure 1).
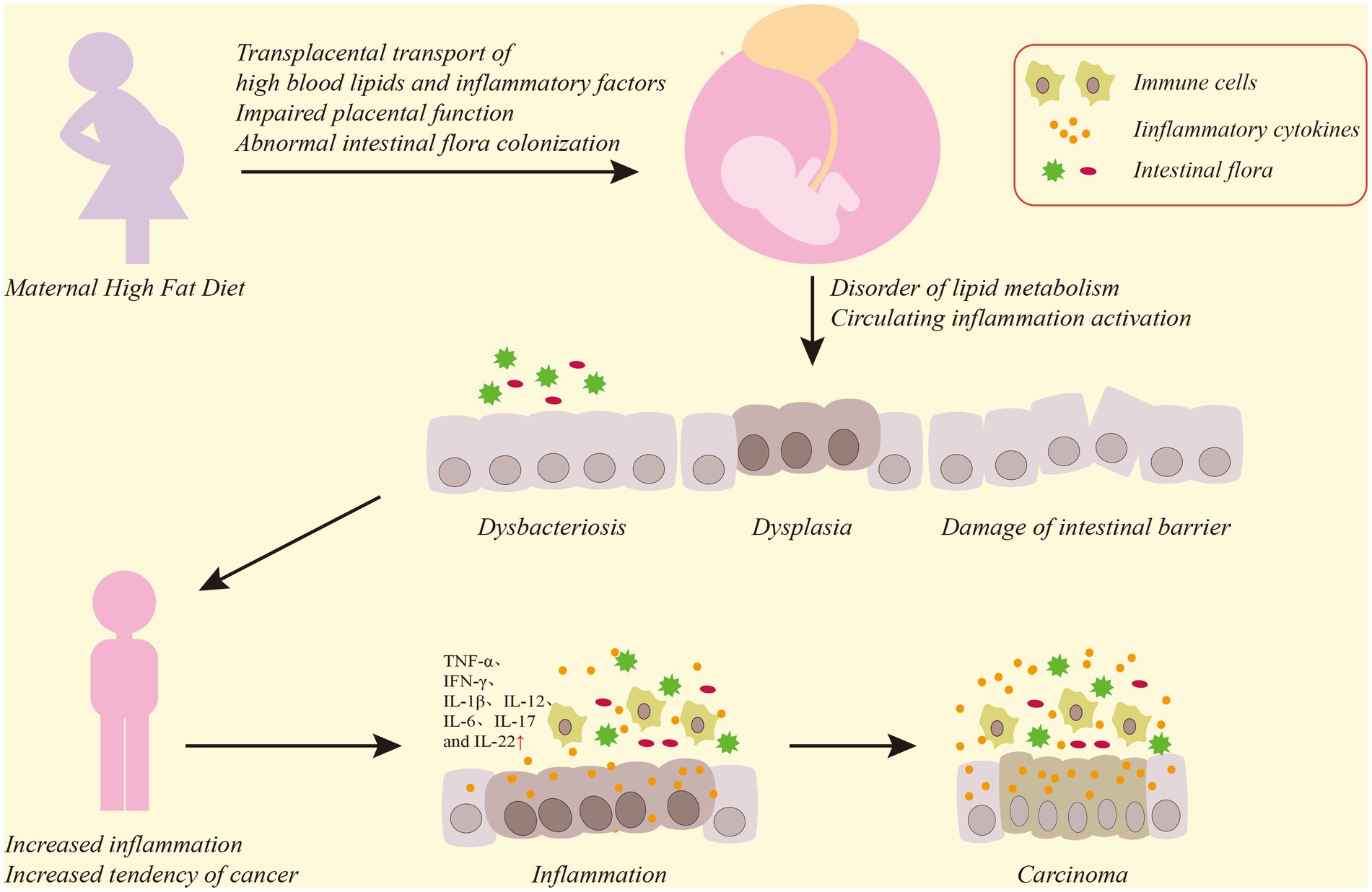
Figure 1. Diagram of the effect of a maternal high-fat diet on the offspring CRC. Maternal high-fat diet causes high levels of serum lipids and inflammatory factors to transfer through the placenta to offspring, damaging placental function, and phenomena such as maternal intestinal flora disorder will also be transmitted to offspring, which will lead to dysbacteriosis and functional damage to the colorectal mucosal barrier in the offspring’s colon, affect the normal growth and development of the colon, and induce intestinal inflammatory reactions. Persistent destruction of colorectal inflammation and uncontrolled proliferation of mucosal cells will ultimately lead to CRC.
At present, the age of diagnosis of CRC in the world is gradually getting younger, and the therapeutic effect of CRC is not ideal. This may be closely related to the diverse pathogenesis of CRC. Few studies have focused on the effect of maternal high-fat diet on offspring colorectal. A series of changes caused by high fat diet in parents may increase colorectal carcinogenesis in offspring, but the related mechanism is not clear at present. Further research to understand the cellular and molecular mechanisms of action of a high-fat diet in CRC development and the role of a maternal high-fat diet in colorectal inflammation and increased CRC susceptibility in offspring is warranted. These studies contribute to the discovery of potential therapeutic targets for CRC and the development of new therapeutic strategies for CRC based on these targets, which may be helpful to improve the efficacy of CRC. Such studies could contribute to the discovery and development of new therapeutic strategies for CRC.
Author contributions
SZ, HY, and LL contributed to conception and design of the study. SZ and JY collect and organize the literature. SZ wrote the first draft of the manuscript. JY, HY, and LL wrote sections of the manuscript. All authors contributed to the article and approved the submitted version.
Acknowledgments
We thank Professional English language editing support provided by AsiaEdit (asiaedit.com).
Conflict of interest
The authors declare that the research was conducted in the absence of any commercial or financial relationships that could be construed as a potential conflict of interest.
Publisher’s note
All claims expressed in this article are solely those of the authors and do not necessarily represent those of their affiliated organizations, or those of the publisher, the editors and the reviewers. Any product that may be evaluated in this article, or claim that may be made by its manufacturer, is not guaranteed or endorsed by the publisher.
Footnotes
References
1. Sung, H, Ferlay, J, Siegel, RL, Laversanne, M, Soerjomataram, I, Jemal, A, et al. Global cancer statistics 2020: GLOBOCAN estimates of incidence and mortality worldwide for 36 cancers in 185 countries. CA Cancer J Clin. (2021) 71:209–49. doi: 10.3322/caac.21660
2. Arnold, M, Sierra, MS, Laversanne, M, Soerjomataram, I, Jemal, A, and Bray, F. Global patterns and trends in colorectal cancer incidence and mortality. Gut. (2017) 66:683–91. doi: 10.1136/gutjnl-2015-310912
3. Jia, W, Rajani, C, Xu, H, and Zheng, X. Gut microbiota alterations are distinct for primary colorectal cancer and hepatocellular carcinoma. Protein Cell. (2021) 12:374–93. doi: 10.1007/s13238-020-00748-0
4. Karki, R, Man, SM, and Kanneganti, TD. Inflammasomes and cancer. Cancer Immunol Res. (2017) 5:94–9. doi: 10.1158/2326-6066.Cir-16-0269
5. Lee, H. Obesity-associated cancers: evidence from studies in mouse models. Cells. (2022) 11:1472. doi: 10.3390/cells11091472
6. Islami, F, Goding Sauer, A, Gapstur, SM, and Jemal, A. Proportion of cancer cases attributable to excess body weight by US state, 2011–2015. JAMA Oncol. (2019) 5:384–92. doi: 10.1001/jamaoncol.2018.5639
7. Chen, K, Guo, J, Zhang, T, Gu, J, Li, H, and Wang, J. The role of dyslipidemia in colitis-associated colorectal Cancer. J Oncol. (2021) 2021:6640384–13. doi: 10.1155/2021/6640384
8. Di Franco, S, Bianca, P, Sardina, DS, Turdo, A, Gaggianesi, M, Veschi, V, et al. Adipose stem cell niche reprograms the colorectal cancer stem cell metastatic machinery. Nat Commun. (2021) 12:5006. doi: 10.1038/s41467-021-25333-9
9. Pourvali, K, and Monji, H. Obesity and intestinal stem cell susceptibility to carcinogenesis. Nutr Metab. (2021) 18:37. doi: 10.1186/s12986-021-00567-y
10. Moley, KH, and Colditz, GA. Effects of obesity on hormonally driven cancer in women. Sci Transl Med. (2016) 8:323ps3. doi: 10.1126/scitranslmed.aad8842
11. Zhang, X, de Oliveira, AF, Zhang, H, Cruz, I, Clarke, R, Gaur, P, et al. Maternal obesity increases offspring's mammary cancer recurrence and impairs tumor immune response. Endocr Relat Cancer. (2020) 27:469–82. doi: 10.1530/erc-20-0065
12. da Cruz, RS, Clarke, J, Curi, ACP, Al-Yawar, A, Jin, L, Baird, A, et al. Parental obesity programs pancreatic cancer development in offspring. Endocr Relat Cancer. (2019) 26:511–23. doi: 10.1530/erc-19-0016
13. Sun, Y, Wang, Q, Zhang, Y, Geng, M, Wei, Y, Liu, Y, et al. Multigenerational maternal obesity increases the incidence of HCC in offspring via miR-27a-3p. J Hepatol. (2020) 73:603–15. doi: 10.1016/j.jhep.2020.03.050
14. Moeckli, B, Lacotte, S, and Toso, C. The role of Acsl1 and Aldh2 in the increased risk for liver cancer in offspring of obese mothers. Front Med. (2022) 9:907028. doi: 10.3389/fmed.2022.907028
15. Murphy, CC, Cirillo, PM, Krigbaum, NY, Singal, AG, Lee, M, Zaki, T, et al. Maternal obesity, pregnancy weight gain, and birth weight and risk of colorectal cancer. Gut. (2022) 71:1332–9. doi: 10.1136/gutjnl-2021-325001
16. Keum, N, and Giovannucci, E. Global burden of colorectal cancer: emerging trends, risk factors and prevention strategies. Nat Rev Gastroenterol Hepatol. (2019) 16:713–32. doi: 10.1038/s41575-019-0189-8
17. Maomao, C, He, L, Dianqin, S, Siyi, H, Xinxin, Y, Fan, Y, et al. Current cancer burden in China: epidemiology, etiology, and prevention. Cancer Biol Med. (2022) 19:1121–38. doi: 10.20892/j.issn.2095-3941.2022.0231
18. Zhang, C, Cheng, Y, Luo, D, Wang, J, Liu, J, Luo, Y, et al. Association between cardiovascular risk factors and colorectal cancer: a systematic review and meta-analysis of prospective cohort studies. EClinicalMedicine. (2021) 34:100794. doi: 10.1016/j.eclinm.2021.100794
19. Giovannucci, E, Stampfer, MJ, Colditz, G, Rimm, EB, and Willett, WC. Relationship of diet to risk of colorectal adenoma in men. J Natl Cancer Inst. (1992) 84:91–8. doi: 10.1093/jnci/84.2.91
20. Willett, WC, Stampfer, MJ, Colditz, GA, Rosner, BA, and Speizer, FE. Relation of meat, fat, and fiber intake to the risk of colon cancer in a prospective study among women. N Engl J Med. (1990) 323:1664–72. doi: 10.1056/nejm199012133232404
21. Liu, T, Guo, Z, Song, X, Liu, L, Dong, W, Wang, S, et al. High-fat diet-induced dysbiosis mediates MCP-1/CCR2 axis-dependent M2 macrophage polarization and promotes intestinal adenoma-adenocarcinoma sequence. J Cell Mol Med. (2020) 24:2648–62. doi: 10.1111/jcmm.14984
22. Zhu, QC, Gao, RY, Wu, W, Guo, BM, Peng, JY, and Qin, HL. Effect of a high-fat diet in development of colonic adenoma in an animal model. World J Gastroenterol. (2014) 20:8119–29. doi: 10.3748/wjg.v20.i25.8119
23. Mana, MD, Hussey, AM, Tzouanas, CN, Imada, S, Barrera Millan, Y, Bahceci, D, et al. High-fat diet-activated fatty acid oxidation mediates intestinal stemness and tumorigenicity. Cell Rep. (2021) 35:109212. doi: 10.1016/j.celrep.2021.109212
24. van Driel, MS, van Neerven, SM, and Vermeulen, L. High-fat diet impacts on tumor development in the gut. Trends Cancer. (2021) 7:664–5. doi: 10.1016/j.trecan.2021.06.005
25. Barbosa, CM, Figueiredo, VP, Barbosa, MA, Cardoso, LM, and Alzamora, AC. Maternal high-fat diet triggers metabolic syndrome disorders that are transferred to first and second offspring generations. Br J Nutr. (2020) 123:59–71. doi: 10.1017/s0007114519002708
26. Landskron, G, De la Fuente, M, Thuwajit, P, Thuwajit, C, and Hermoso, MA. Chronic inflammation and cytokines in the tumor microenvironment. J Immunol Res. (2014) 2014:149185:1–19. doi: 10.1155/2014/149185
27. Xie, R, Sun, Y, Wu, J, Huang, S, Jin, G, Guo, Z, et al. Maternal high fat diet alters gut microbiota of offspring and exacerbates DSS-induced colitis in adulthood. Front Immunol. (2018) 9:2608. doi: 10.3389/fimmu.2018.02608
28. Wu, X, Pfalzer, AC, Koh, GY, Tang, S, Crott, JW, Thomas, MJ, et al. Curcumin and salsalate suppresses colonic inflammation and procarcinogenic signaling in high-fat-fed, azoxymethane-treated mice. J Agric Food Chem. (2017) 65:7200–9. doi: 10.1021/acs.jafc.7b02648
29. Zheng, SM, Chen, H, Sha, WH, Chen, XF, Yin, JB, Zhu, XB, et al. Oxidized low-density lipoprotein stimulates CD206 positive macrophages upregulating CD44 and CD133 expression in colorectal cancer with high-fat diet. World J Gastroenterol. (2022) 28:4993–5006. doi: 10.3748/wjg.v28.i34.4993
30. De Simone, V, Franzè, E, Ronchetti, G, Colantoni, A, Fantini, MC, Di Fusco, D, et al. Th17-type cytokines, IL-6 and TNF-α synergistically activate STAT3 and NF-kB to promote colorectal cancer cell growth. Oncogene. (2015) 34:3493–503. doi: 10.1038/onc.2014.286
31. Gohir, W, Kennedy, KM, Wallace, JG, Saoi, M, Bellissimo, CJ, Britz-McKibbin, P, et al. High-fat diet intake modulates maternal intestinal adaptations to pregnancy and results in placental hypoxia, as well as altered fetal gut barrier proteins and immune markers. J Physiol. (2019) 597:3029–51. doi: 10.1113/jp277353
32. Weng, SF, Redsell, SA, Nathan, D, Swift, JA, Yang, M, and Glazebrook, C. Estimating overweight risk in childhood from predictors during infancy. Pediatrics. (2013) 132:e414–21. doi: 10.1542/peds.2012-3858
33. Voerman, E, Santos, S, Inskip, H, Amiano, P, Barros, H, Charles, MA, et al. Association of gestational weight gain with adverse maternal and infant outcomes. JAMA. (2019) 321:1702–15. doi: 10.1001/jama.2019.3820
34. Mamun, AA, Mannan, M, and Doi, SA. Gestational weight gain in relation to offspring obesity over the life course: a systematic review and bias-adjusted meta-analysis. Obes Rev. (2014) 15:338–47. doi: 10.1111/obr.12132
35. Quecke, B, Graf, Y, Epure, AM, Santschi, V, Chiolero, A, Carmeli, C, et al. Caesarean section and obesity in young adult offspring: update of a systematic review with meta-analysis. Obes Rev. (2022) 23:e13368. doi: 10.1111/obr.13368
36. Gonzalez-Bulnes, A, Ovilo, C, and Astiz, S. Transgenerational inheritance in the offspring of pregnant women with metabolic syndrome. Curr Pharm Biotechnol. (2014) 15:13–23. doi: 10.2174/1389201015666140330192009
37. Flanagan, EW, Most, J, Altazan, AD, Boyle, KE, and Redman, LM. A role for the early pregnancy maternal milieu in the intergenerational transmission of obesity. Obesity. (2021) 29:1780–6. doi: 10.1002/oby.23283
38. Godfrey, KM, Reynolds, RM, Prescott, SL, Nyirenda, M, Jaddoe, VW, Eriksson, JG, et al. Influence of maternal obesity on the long-term health of offspring. Lancet Diabetes Endocrinol. (2017) 5:53–64. doi: 10.1016/s2213-8587(16)30107-3
39. Denizli, M, Capitano, ML, and Kua, KL. Maternal obesity and the impact of associated early-life inflammation on long-term health of offspring. Front Cell Infect Microbiol. (2022) 12:940937. doi: 10.3389/fcimb.2022.940937
40. Wankhade, UD, Zhong, Y, Kang, P, Alfaro, M, Chintapalli, SV, Piccolo, BD, et al. Maternal high-fat diet programs offspring liver steatosis in a sexually dimorphic manner in association with changes in gut microbial ecology in mice. Sci Rep. (2018) 8:16502. doi: 10.1038/s41598-018-34453-0
41. Wang, D, Yan, J, Teng, M, Yan, S, Zhou, Z, and Zhu, W. In utero and lactational exposure to BDE-47 promotes obesity development in mouse offspring fed a high-fat diet: impaired lipid metabolism and intestinal dysbiosis. Arch Toxicol. (2018) 92:1847–60. doi: 10.1007/s00204-018-2177-0
42. Greathouse, KL, Faucher, MA, and Hastings-Tolsma, M. The gut microbiome, obesity, and weight control in women's reproductive health. West J Nurs Res. (2017) 39:1094–119. doi: 10.1177/0193945917697223
43. Malesza, IJ, Malesza, M, Walkowiak, J, Mussin, N, Walkowiak, D, Aringazina, R, et al. High-fat, western-style diet, systemic inflammation, and gut microbiota: a narrative review. Cells. (2021) 10:3164. doi: 10.3390/cells10113164
44. Ley, RE, Bäckhed, F, Turnbaugh, P, Lozupone, CA, Knight, RD, and Gordon, JI. Obesity alters gut microbial ecology. Proc Natl Acad Sci U S A. (2005) 102:11070–5. doi: 10.1073/pnas.0504978102
45. Dreisbach, C, Morgan, H, Cochran, C, Gyamfi, A, Henderson, WA, and Prescott, S. Metabolic and microbial changes associated with diet and obesity during pregnancy: what can we learn from animal studies? Front Cell Infect Microbiol. (2021) 11:795924. doi: 10.3389/fcimb.2021.795924
46. Babu, ST, Niu, X, Raetz, M, Savani, RC, Hooper, LV, and Mirpuri, J. Maternal high-fat diet results in microbiota-dependent expansion of ILC3s in mice offspring. JCI Insight. (2018) 3:e99223. doi: 10.1172/jci.insight.99223
47. Guo, Y, Wang, Z, Chen, L, Tang, L, Wen, S, Liu, Y, et al. Diet induced maternal obesity affects offspring gut microbiota and persists into young adulthood. Food Funct. (2018) 9:4317–27. doi: 10.1039/c8fo00444g
48. Barbour, LA, and Hernandez, TL. Maternal lipids and fetal overgrowth: making fat from fat. Clin Ther. (2018) 40:1638–47. doi: 10.1016/j.clinthera.2018.08.007
49. Howell, KR, and Powell, TL. Effects of maternal obesity on placental function and fetal development. Reproduction. (2017) 153:R97–R108. doi: 10.1530/rep-16-0495
50. Most, J, Dervis, S, Haman, F, Adamo, KB, and Redman, LM. Energy intake requirements in pregnancy. Nutrients. (2019) 11:8. doi: 10.3390/nu11081812
51. Kirwan, JP, Hauguel-De Mouzon, S, Lepercq, J, Challier, JC, Huston-Presley, L, Friedman, JE, et al. TNF-alpha is a predictor of insulin resistance in human pregnancy. Diabetes. (2002) 51:2207–13. doi: 10.2337/diabetes.51.7.2207
52. Zhu, MJ, Du, M, Nathanielsz, PW, and Ford, SP. Maternal obesity up-regulates inflammatory signaling pathways and enhances cytokine expression in the mid-gestation sheep placenta. Placenta. (2010) 31:387–91. doi: 10.1016/j.placenta.2010.02.002
53. Wallace, JG, Bellissimo, CJ, Yeo, E, Fei Xia, Y, Petrik, JJ, Surette, MG, et al. Obesity during pregnancy results in maternal intestinal inflammation, placental hypoxia, and alters fetal glucose metabolism at mid-gestation. Sci Rep. (2019) 9:17621. doi: 10.1038/s41598-019-54098-x
54. Sureshchandra, S, Marshall, NE, Wilson, RM, Barr, T, Rais, M, Purnell, JQ, et al. Inflammatory determinants of pregravid obesity in placenta and peripheral blood. Front Physiol. (2018) 9:1089. doi: 10.3389/fphys.2018.01089
55. Kelly, AC, Powell, TL, and Jansson, T. Placental function in maternal obesity. Clin Sci. (2020) 134:961–84. doi: 10.1042/cs20190266
56. Boyle, KE, Patinkin, ZW, Shapiro, ALB, Bader, C, Vanderlinden, L, Kechris, K, et al. Maternal obesity alters fatty acid oxidation, AMPK activity, and associated DNA methylation in mesenchymal stem cells from human infants. Mol Metab. (2017) 6:1503–16. doi: 10.1016/j.molmet.2017.08.012
57. Gruber, L, Hemmerling, J, Schüppel, V, Müller, M, Boekschoten, MV, and Haller, D. Maternal high-fat diet accelerates development of Crohn's disease-like ileitis in TNFΔARE/WT offspring. Inflamm Bowel Dis. (2015) 21:2016–25. doi: 10.1097/mib.0000000000000465
58. Al Nabhani, Z, Dulauroy, S, Lécuyer, E, Polomack, B, Campagne, P, Berard, M, et al. Excess calorie intake early in life increases susceptibility to colitis in adulthood. Nat Metab. (2019) 1:1101–9. doi: 10.1038/s42255-019-0129-5
59. Bibi, S, Kang, Y, Du, M, and Zhu, MJ. Maternal high-fat diet consumption enhances offspring susceptibility to DSS-induced colitis in mice. Obesity. (2017) 25:901–8. doi: 10.1002/oby.21816
60. Yan, X, Huang, Y, Wang, H, Du, M, Hess, BW, Ford, SP, et al. Maternal obesity induces sustained inflammation in both fetal and offspring large intestine of sheep. Inflamm Bowel Dis. (2011) 17:1513–22. doi: 10.1002/ibd.21539
61. Xue, Y, Wang, H, Du, M, and Zhu, MJ. Maternal obesity induces gut inflammation and impairs gut epithelial barrier function in nonobese diabetic mice. J Nutr Biochem. (2014) 25:758–64. doi: 10.1016/j.jnutbio.2014.03.009
62. Mueller, NT, Bakacs, E, Combellick, J, Grigoryan, Z, and Dominguez-Bello, MG. The infant microbiome development: mom matters. Trends Mol Med. (2015) 21:109–17. doi: 10.1016/j.molmed.2014.12.002
63. Derrien, M, Alvarez, AS, and de Vos, WM. The gut microbiota in the first decade of life. Trends Microbiol. (2019) 27:997–1010. doi: 10.1016/j.tim.2019.08.001
64. Xue, C, Xie, Q, Zhang, C, Hu, Y, Song, X, Jia, Y, et al. Vertical transmission of the gut microbiota influences glucose metabolism in offspring of mice with hyperglycaemia in pregnancy. Microbiome. (2022) 10:122. doi: 10.1186/s40168-022-01318-8
65. Zhou, L, and Xiao, X. The role of gut microbiota in the effects of maternal obesity during pregnancy on offspring metabolism. Biosci Rep. (2018) 38:BSR20171234. doi: 10.1042/bsr20171234
66. Alberca, GGF, Cardoso, NSS, Solis-Castro, RL, Nakano, V, and Alberca, RW. Intestinal inflammation and the microbiota: beyond diversity. World J Gastroenterol. (2022) 28:3274–8. doi: 10.3748/wjg.v28.i26.3274
67. Yang, J, Wei, H, Zhou, Y, Szeto, CH, Li, C, Lin, Y, et al. High-fat diet promotes colorectal tumorigenesis through modulating gut microbiota and metabolites. Gastroenterology. (2022) 162:135–49.e2. doi: 10.1053/j.gastro.2021.08.041
68. Liang, X, Yang, Q, Fu, X, Rogers, CJ, Wang, B, Pan, H, et al. Maternal obesity epigenetically alters visceral fat progenitor cell properties in male offspring mice. J Physiol. (2016) 594:4453–66. doi: 10.1113/jp272123
69. Saullo, C, Cruz, LLD, Damasceno, DC, Volpato, GT, Sinzato, YK, Karki, B, et al. Effects of a maternal high-fat diet on adipose tissue in murine offspring: a systematic review and meta-analysis. Biochimie. (2022) 201:18–32. doi: 10.1016/j.biochi.2022.06.009
70. Brown, JC, Caan, BJ, Prado, CM, Cespedes Feliciano, EM, Xiao, J, Kroenke, CH, et al. The association of abdominal adiposity with mortality in patients with stage I-III colorectal cancer. J Natl Cancer Inst. (2020) 112:377–83. doi: 10.1093/jnci/djz150
71. Słupecka-Ziemilska, M, Grzesiak, P, Kowalczyk, P, Wychowański, P, and Woliński, J. Maternal high-fat diet exposure during gestation and lactation affects intestinal development in suckling rats. Front Physiol. (2021) 12:693150. doi: 10.3389/fphys.2021.693150
72. Chen, X, Yang, Z, Hu, H, Duan, W, Wang, A, Dong, Y, et al. Differentiation and proliferation of intestinal stem cells and its underlying regulated mechanisms during weaning. Curr Protein Pept Sci. (2019) 20:690–5. doi: 10.2174/1389203720666190125101834
73. Liu, WC, Wu, CW, Fu, MH, Tain, YL, Liang, CK, Hung, CY, et al. Maternal high fructose-induced hippocampal Neuroinflammation in the adult female offspring via PPARγ-NF-κB signaling. J Nutr Biochem. (2020) 81:108378. doi: 10.1016/j.jnutbio.2020.108378
74. Segovia, SA, Vickers, MH, and Reynolds, CM. The impact of maternal obesity on inflammatory processes and consequences for later offspring health outcomes. J Dev Orig Health Dis. (2017) 8:529–40. doi: 10.1017/s2040174417000204
Keywords: maternal, offspring, high-fat diet, colorectal cancer, intestinal inflammation
Citation: Zheng S, Yin J, Yue H and Li L (2023) Maternal high-fat diet increases the susceptibility of offspring to colorectal cancer via the activation of intestinal inflammation. Front. Nutr. 10:1191206. doi: 10.3389/fnut.2023.1191206
Edited by:
Gianvincenzo Zuccotti, University of Milan, ItalyCopyright © 2023 Zheng, Yin, Yue and Li. This is an open-access article distributed under the terms of the Creative Commons Attribution License (CC BY). The use, distribution or reproduction in other forums is permitted, provided the original author(s) and the copyright owner(s) are credited and that the original publication in this journal is cited, in accordance with accepted academic practice. No use, distribution or reproduction is permitted which does not comply with these terms.
*Correspondence: Lifu Li, MzU4MjY4NTUyQHFxLmNvbQ==; Hui Yue, eWgxMjA3MDQzMEB2aXAuc2luYS5jb20=