- 1Department of Zoology, Wildlife and Fisheries, University of Agriculture, Faisalabad, Pakistan
- 2Department of Physiology, Government College University, Faisalabad, Pakistan
- 3Department of Community Health Sciences, College of Applied Medical Sciences, King Saud University, Riyadh, Saudi Arabia
- 4Institute of Cellular Medicine, Newcastle University Medical School, Newcastle University, Newcastle upon Tyne, United Kingdom
- 5Department of Pharmaceutical Chemistry, College of Pharmacy, King Saud University, Riyadh, Saudi Arabia
Introduction: Cadmium (Cd) is a highly toxic heavy metal that can be found everywhere in the environment and can have harmful effects on both human and animal health. Pinostrobin (PSB) is a bioactive natural flavonoid isolated from Boesenbergia rotunda with several pharmacological properties, such as antiinflammatory, anticancer, antioxidant, and antiviral. This investigation was intended to assess the therapeutic potential of PSB against Cd-induced kidney damage in rats.
Methods: In total, 48 Sprague Dawley rats were divided into four groups: a control, a Cd (5 mg/kg), a Cd + PSB group (5 mg/kg Cd and 10 mg/kg PSB), and a PSB group (10 mg/kg) that received supplementation for 30 days.
Results: Exposure to Cd led to a decrease in the activities of catalase (CAT), glutathione reductase (GSR), superoxide dismutase (SOD), and glutathione peroxidase (GSH-PX), whereas levels of reactive oxygen species (ROS) and malondialdehyde (MDA) increased. Cd exposure also caused a substantial increase in urea, kidney injury molecule-1 (KIM-1), neutrophil gelatinase-associated lipocalin (NGAL), and creatinine levels. Moreover, a noticeable decline was noticed in creatinine clearance. Moreover, Cd exposure considerably increased the levels of inflammatory indices, including interleukin-1b (IL-1b), tumor necrosis factor-a (TNF-a), interleukin-6 (IL-6), nuclear factor kappa-B (NF-kB), inducible nitric oxide synthase (iNOS), and cyclooxygenase-2 (COX-2) activity. Cd treatment decreased the expression of the antiapoptotic markers (Bcl-2) while increasing the expression of apoptotic markers (Bax and Caspase-3). Furthermore, Cd treatment substantially reduced the TCA cycle enzyme activity, such as alpha-ketoglutarate dehydrogenase, succinate dehydrogenase, malate dehydrogenase, and isocitrate dehydrogenase. Moreover, mitochondrial electron transport chain enzymes, succinatedehydrogenase, NADH dehydrogenase, cytochrome c-oxidase, and coenzyme Q-cytochrome reductase activities were also decreased following Cd exposure. PSB administration substantially reduced the mitochondrial membrane potential while inducing significant histological damage. However, PSB treatment significantly reduced Cd-mediated renal damage in rats.
Conclusion: Thus, the present investigation discovered that PSB has ameliorative potential against Cd-induced renal dysfunction in rats.
Introduction
Cadmium (Cd) is a ubiquitous environmental toxin that is extensively used in industrial and agricultural products (1). Cd is one of the major heavy metals that pollute the ecosystem and has damaging effects on different biological processes in both humans and animals (2). Exposure to Cd can occur through various sources, such as plant-based foods, fertilizers, contaminated water, plastic toys, ceramics, batteries, paints, air, soil, and cigarette smoke (3, 4). The accumulation of Cd in plants mainly occurs through anthropogenic activities such as the application of phosphate fertilizers, wastewater, sewage sludge, and manures. Moreover, the high mobility of soils makes the accumulation of Cd easy in plants. Industries involved in the production of batteries, pigments, coatings, electroplating, and plastic stabilizers are often associated with occupational exposure to Cd.
Moreover, these industries are the sources that make the water contaminated. However, the major Cd occupational exposure occurs through fume inhalation in the cadmium-nickel battery industry. Currently, Cd pollution poses a serious environmental problem. Cd accumulation in the body can affect health, and its exposure may cause hazardous effects such as hepatotoxicity, ototoxicity, carcinogenesis, and nephrotoxicity (5). In living organisms, heavy metals, including Cd, can damage cellular organelles along with components such as lysosomes, cell membranes, endoplasmic reticulum, and mitochondria, as well as some enzymes involved in damage repair, metabolism, and detoxification (6).
The kidneys are the primary target site of Cd exposure in the body. Approximately 50% of the Cd accumulates in the kidney, particularly in the proximal convoluted tubules, which leads to renal dysfunction and chronic kidney disorders (7–10). According to previous research, up to 7% of the world's population suffers from chronic kidney disorders due to Cd exposure (11). Cd induces nephrotoxicity via inflammation, apoptosis, and the generation of ROS in renal tissues (12, 13). Cd exposure generates proinflammatory cytokines such as interleukin IL-1 and TNF-α that trigger inflammation by recruiting innate immune cells (14). These proinflammatory cytokines contribute to inflammation by inducing the expression of adhesion molecules on endothelial cells, causing leukocytes in circulation to adhere to the endothelium. Long-term exposure to Cd significantly decreases the glomerular filtration rate, which can lead to renal failure (15), increased creatinine, blood urea nitrogen (BUN), hydropic swelling, and proximal tubular cells' hypertrophy (12). Mitochondria have long been recognized as a major site for the production of reactive nitrogen species and ROS (16). Furthermore, it has been stated that Cd exposure can cause mitochondrial dysfunction, which may lead to renal failure (17).
Pinostrobin (PSB), or 5-hydroxy-7-methoxy flavanone, is a dietary flavonoid isolated from Boesenbergia rotunda. This plant was originally characterized as a medicinal plant due to its potential therapeutical properties such as antiinflammatory (18), antileukemia (19), anticancer (20), antioxidant (21, 22), antimicrobial (23), anti-Alzheimer's (24), and antiviral (25). Cd-induced renal dysfunction occurs due to oxidative stress, inflammation, and apoptosis, as the kidney is the primary target site of Cd-induced toxicity. Therefore, by considering the aforementioned pharmacological activities of PSB, the present investigation was intended to evaluate the potential of PSB to alleviate Cd-induced renal damage in Sprague-Dawley rats.
Materials and methods
Chemicals
Cd and PSB were purchased from Sigma-Aldrich (Germany).
Animals
Mature male Sprague-Dawley rats with an average weight of 185 ± 15 g. The rats were housed at the animal research station of the University of Agriculture, Faisalabad, with a temperature of 25 ± 1°C and a 12-h light/dark cycle maintained throughout the experiments. Furthermore, the rats were given standard feed and water ad libitum throughout the whole trial. The rats were acclimatized to the laboratory environment for 7 days before the start of the trial. All animal procedures were conducted in accordance with the approved protocol of the European Union for Animal Care and Experimentation (CEE Council 86/609).
Experimental layout
A total of 48 male Sprague-Dawley rats were divided into four different groups, 12 in each group, and stored in separate cages. The trial was conducted for 30 days. Group 1 was designated as the control group. Group 2 was given Cd (5 mg/kg) orally. Group 3 was administered Cd (5 mg/kg) and PSB (10 mg/kg) orally until the completion of the experiment (30 days). The rats in group 4 were supplemented with PSB (10 mg/kg). Cd at a dose of 5 mg/kg was given according to a previous investigation [26]. The rats were anesthetized with 60 mg/kg of ketamine and 6 mg/kg of xylazine and dissected after 28 days of treatment. The blood was drawn to analyze the serum profile. Following dissection, the kidneys were removed; one kidney was packaged in a zipper bag and preserved at −80°C for biochemical examination. For histological evaluation, the other kidney was stored in a 10% formalin solution.
Separation of kidney mitochondria
Mingatto et al.'s approach was used to isolate mitochondria from renal cells (26). The renal tissue was mixed with medium-I (70 mM sucrose, 250 mM mannitol, 50 mM Tris-HCl, 10 mM HEPES, 1 mM EDTA, 120 mM KCl, and pH 7.4). Homogenate was centrifuged for 5 min at 755 × g. The pellets were rinsed in medium-II (250 mM mannitol, 50 mM Tris-HCl, 70 mM sucrose, 10 mM HEPES, and pH 7.4) before being rinsed two times with the same buffer and centrifuged at 13,300 × g for 15 min. Mitochondrial pellets were resuspended in the same medium and utilized for additional investigation.
Estimation of biochemical markers
CAT activity was evaluated by following the methodology of Aebi (27). The activity of SOD was estimated via the method popularized by Sun et al. (29). Carlberg and Mannervik's (28) technique was used to measure GSR content. GSH-PX activity was estimated by following the method of Lawrence and Burk (29). The MDA level was determined in accordance with the method of Ohkawa et al. (30). ROS level was measured by ELISA kits (Shanghai Enzyme-Linked Biotechnology Co. Ltd., Shanghai, China) as per the manufacturer's instructions.
Estimation of kidney function markers
Urea, creatinine, and creatinine clearance were determined using standard diagnostic kits (MediScreen kit, France). Urinary KIM-1 and NGAL levels were measured using the KIM-1 Quantikine ELISA Kit and the NGAL Quantikine ELISA Kit, respectively, following the manufacturer's guidelines.
Estimation of inflammatory indices
By using a tissue lyser device (Tissue Lyser II, Oiagen), renal tissues were homogenized in a cold phosphate buffer (pH 7.4) (31). The commercial kits were used for the measurement of inflammatory indices, i.e., interleukin-6 (IL-6), tumor necrosis factor-α (TNF-α), inducible nitric oxide synthase (iNOS), interleukin-1β (IL-1β), nuclear factor kappa B (NFκB), and cyclooxygenase-2 (COX-2) in renal tissues.
Estimation of apoptosis markers
RT-qPCR was used to evaluate Bax, Bcl-2, and Caspase-3 expressions. Total RNA isolation was performed using the TRIzol reagent, which was then reverse-transcribed into complementary DNA. Variations in apoptotic markers' expression were observed by 2−ΔΔCT using β-actin as the internal control (32). Sequences (primers) of β-actin and target genes are displayed in Table 1, as reported previously (33).
Assessment of TCA cycle enzymes
Isocitrate dehydrogenase (ICdH) activity was evaluated according to the practice of Bernt and Bergmeyer (34). Succinate dehydrogenase (SDH) activity was determined according to the protocol of Slater and Borner (35). Malate dehydrogenase (MDH) activity was assessed by following the technique of Mehler et al. (36). α-KGDH activity was determined in accordance with the procedure of Reed and Mukherjee (37).
Analysis of the activity of respiratory chain complexes in the renal mitochondria
Mitochondrial respiratory-chain complex test kits (Suzhou-Comin Biotechnology Ltd., China) were used to evaluate the activity of respiratory chain complexes in renal mitochondria.
Assessment of mitochondrial membrane potential
Mitochondrial membrane potential (MMP) was determined by measuring the absorption of a cationic fluorescent dye by the mitochondria (38). To incubate the mitochondrial suspension (0.5 mg protein ml-1) with Rh 123 (1.5 M), the tubes were gently shaken for 10 min at 37°C. The Elmer LS-50B luminescence fluorescence spectrophotometer was used to determine fluorescence at emission (490 nm) and excitation (535 nm) wavelengths (38).
Tissue histology
The renal tissues were fixed in a 10% formalin solution for 48 h. The samples were then desiccated in increasing degrees of alcohol before being embedded in paraffin wax. A rotatory microtome was used to cut 4–5 μm-thick slices, which were then stained with the hematoxylin-eosin stain. In the end, the slides were examined using a light microscope (Nikon, 187842, Japan) at 400X. Microphotography was performed with a Leica LB microscope linked to Olympus Optical Co., Ltd., Japan.
Statistical analysis
Data were displayed as mean ± SEM. The interpretation of the data was conducted using Minitab software. The data were analyzed using a one-way ANOVA followed by Tukey's test. The significance level was set at a P-value of < 0.05.
Results
Effect of Cd and PSB on antioxidant enzymes and oxidative markers
Cd exposure considerably (P < 0.05) decreased the enzymatic activities of SOD, GSR, and CAT, as well as GSH-PX while markedly (P < 0.05) increasing the levels of MDA and ROS compared to the control group. Co-administration of PSB with Cd resulted in a noticeable (P < 0.05) increase in the activities of SOD, GSR, and CAT enzymes, as well as GSH-PX while significantly (P < 0.05) decreasing MDA and ROS levels compared to the Cd-treated group. The PSB-treated group demonstrated antioxidant enzyme activities, ROS, and MDA levels that were similar to those of the control rats (Table 2).
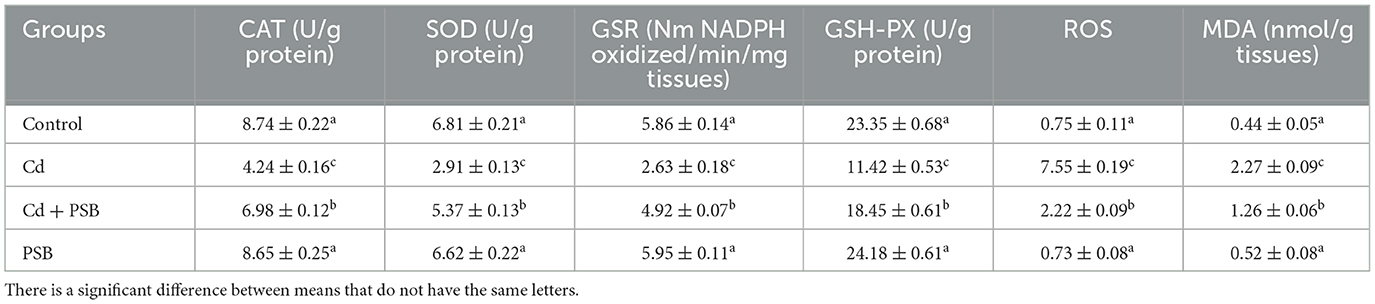
Table 2. Mean ± SEM of biochemical markers in the kidney of control, cadmium, cadmium + pinostrobin, and pinostrobin-supplemented rats.
Effect of Cd and PSB on kidney function markers
Cd treatment showed remarkable (P < 0.05) escalation in urea, KIM-1, and NGAL, as well as creatinine levels, and a noticeable (P < 0.05) downregulation in creatinine clearance compared to the control rats. The co-administration of Cd with PSB showed a considerable (P < 0.05) reduction in urea, KIM-1, NGAL, and creatinine levels, whereas a substantial increase was noticed in the creatinine clearance when compared to the CD-administrated group. Only the administration of PSB showed levels of renal function markers that were similar to those of the control rats (Table 3).
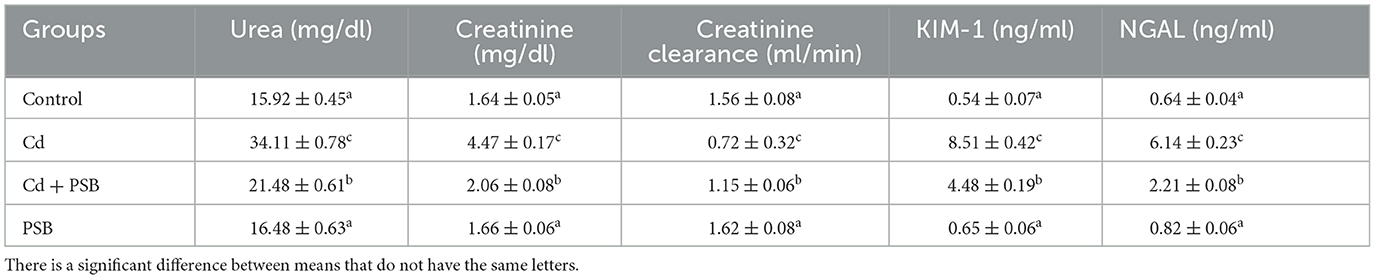
Table 3. Mean ± SEM of renal function markers in control, cadmium-administrated, co-administrated, and pinostrobin-supplemented rats.
Effect of Cd and PSB on inflammatory cytokines
Cd exposure resulted in a significant (P < 0.05) increase in the levels of IL-1β, NF-κB, IL-6, TNF-α, and the activity of iNOS and COX-2 compared to the control group. The co-administration of Cd with PSB led to a noticeable (P < 0.05) decline in the levels of these indices compared to the Cd-exposed rats. PSB administration alone demonstrated the same levels of these markers as those observed in the control rats (Table 4).
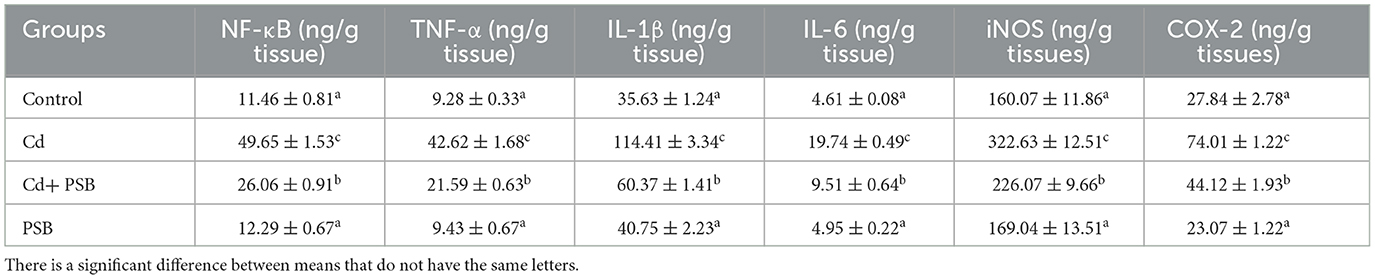
Table 4. Mean ± SEM of inflammatory indices in the renal tissues of control, cadmium-administrated, co-administrated, and pinostrobin-supplemented rats.
Effect of Cd and PSB on apoptotic markers
Cd exposure led to a significant (P < 0.05) decrease in the expression of the antiapoptotic marker (Bcl-2) while the expression of the apoptotic marker (caspase-3 & Bax) was upregulated in PSB-administrated rats when compared to the control group. However, co-treatment with Cd and PSB resulted in a significant (P < 0.05) reversal of these antiapoptotic and apoptotic marker expressions compared to the Cd-treated group. Administration of PSB alone demonstrated normal expressions of these markers that were comparable to those observed in the control rats (see Figure 1).
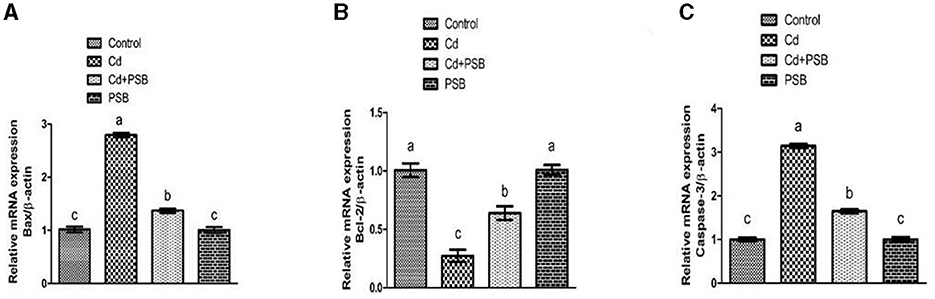
Figure 1. Effect of Cd and PSB on the renal (A, B) pro (Bax) and anti-apoptotic (Bcl-2) markers and (C) Caspease-3. First bar represents control; Second bar represents Cd treated group; Third bar represents Cd+PSB treated group; Fourth bar represents PSB treated group. Values are expressed as Mean ± SEM (12 rats per group). Values having different superscripts are significantly p < 0.05 different from each other.
Effect of Cd and PSB on TCA cycle enzymes
Cd intoxication markedly (P < 0.05) lowered the TCA cycle enzymes (MDH, ICdH, α-KGDH, and SDH) activities in comparison to the control group. However, co-administration of PSB and Cd led to a significant increase in TCA cycle enzyme activities compared with Cd-administrated rats. The PSB-administrated group showed TCA cycle enzyme activities that were similar to those of the control rats (Table 5).
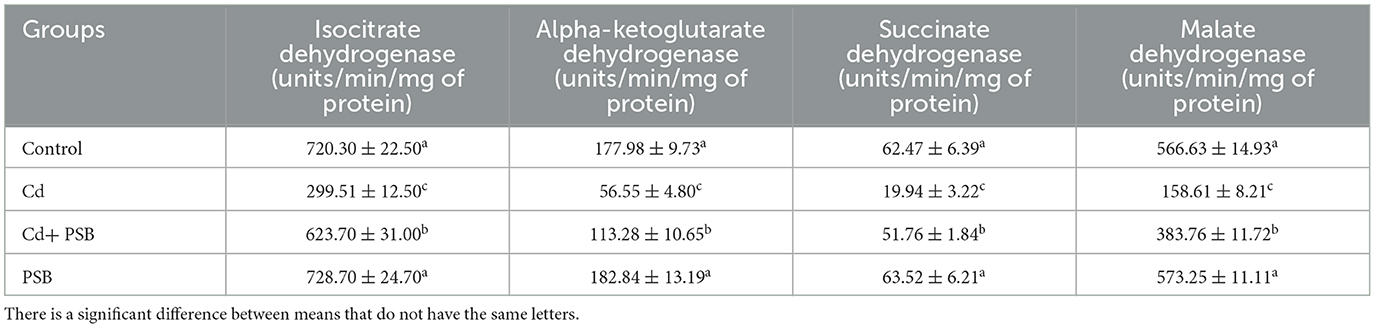
Table 5. Mean ± SEM of tricarboxylic acid cycle enzymes in the renal tissues of control, cadmium-administrated, co-administrated, and pinostrobin-supplemented rats.
Effect of Cd and PSB on mitochondrial respiratory chain complexes
The co-administration of PSB and Cd substantially restored the activities of mitochondrial respiratory chain complexes when compared to the Cd-treated group. PSB-administrated rats exhibited activities in these complexes that were similar to those of the control rats (Table 6).
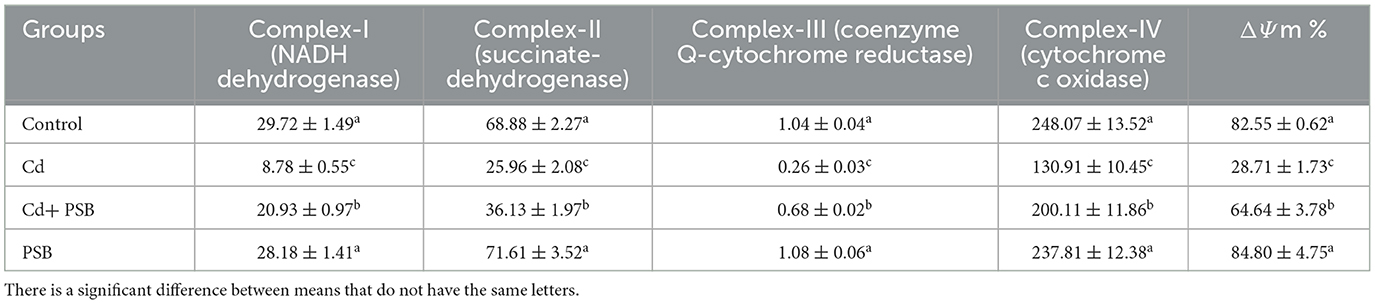
Table 6. Mean ± SEM of renal mitochondrial respiratory chain complexes along with ΔΨm in control, cadmium-administrated, co-administrated, and pinostrobin-supplemented rats.
Effect of Cd and PSB on mitochondrial membrane potential
The rats exposed to Cd presented a noticeable (P < 0.05) depolarization in mitochondrial membrane potential (ΔΨm) when compared to the control rats. However, the co-administration of PSB and Cd partially mitigated the loss of ΔΨm when compared to the Cd-administrated group. Only PSB-administrated rats exhibited mitochondrial membrane potential, which was similar to that observed in the control rats (Table 6).
Effect of Cd and PSB on histopathology
The kidneys of the rats in the control group showed normal renal tubules and glomeruli. Most of the renal glomeruli appeared regular, without evidence of mesangial cell proliferation or vascular congestion. The shape of the renal tubules ranged from round to oval ducts lined with cuboidal epithelial or polygonal cells. However, kidneys in the Cd-exposed group exhibited a distorted structure, with atrophied glomerular tufts, disruption of Bowman's capsule, and vacuolation in the epithelium of renal tubules. However, the co-treated group displayed mild to moderate vacuolation in the epithelium of the tubules, while the glomeruli were normal in size with a mild level of tuft and distortion in the capillaries. Only administration of PSB showed normal renal tubules and a regular histological profile similar to that observed in the control group (Figure 2 and Table 7).
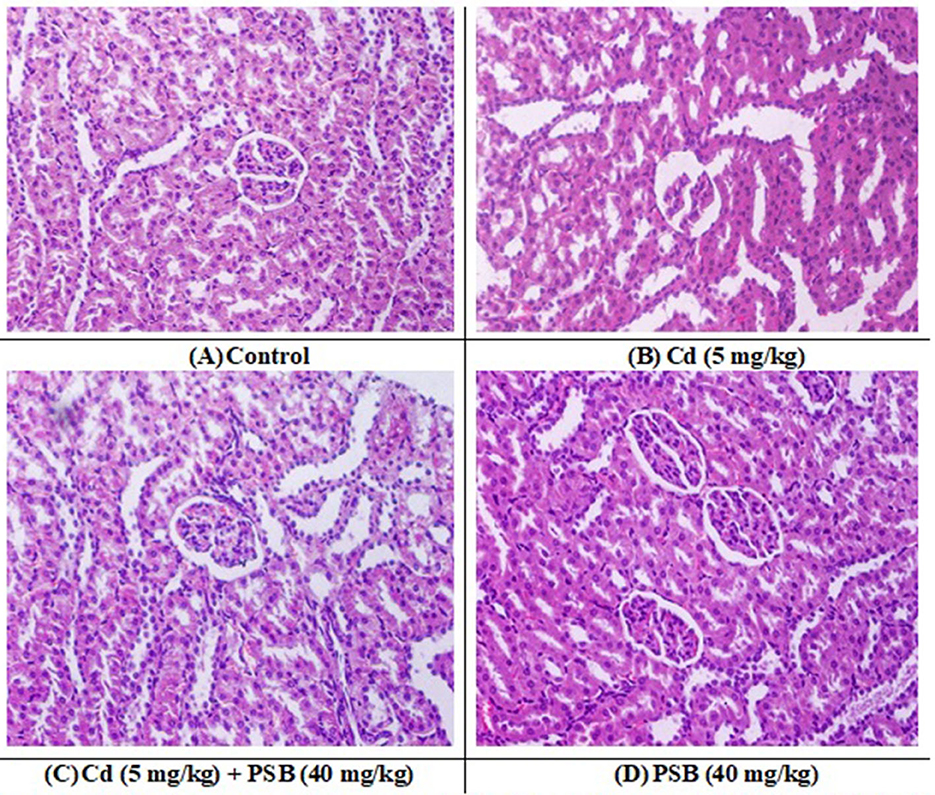
Figure 2. (A) control group; displaying normal histological structure (H and E, 400X). (B) Cd treated group; showing dilation, vacuolation, degeneration, and widened Bowman's capsule necrosis in the kidney tissues. (C) Cd + PSB treated group showing improved histoarchitecture with reduce degenerative architecture in renal epithelium and renal tubules. (D) PSB treated group showing normal renal histoarchitecture. Cd, Cadmium; PSB, Pinostrobin.
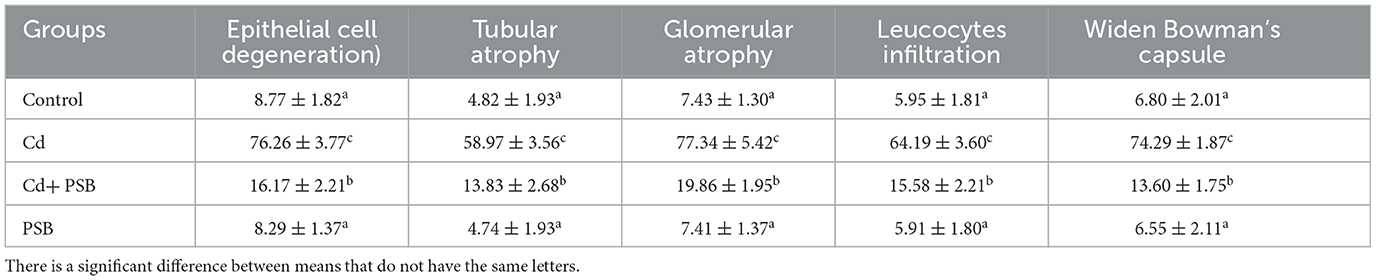
Table 7. Mean ± SEM of renal histology in control, cadmium-administrated, co-administrated, and pinostrobin-supplemented rats.
Discussion
In underdeveloped nations, Cd is believed to be the most prevalent environmental and accidental contaminant that frequently poses a major threat to both humans and animals (39). Cd is a widely present hazardous industrial pollutant that can accumulate in tissues such as the testis, hepatic tissue, lungs, bones, and renal tissue and cause acute injuries (40). One can become exposed to Cd through various sources, such as plant-based food, fertilizers, contaminated water, ceramics, batteries, and cigarette smoke (3, 4). Cd accumulates in the body and can have various detrimental effects, such as hepatotoxicity, ototoxicity, carcinogenesis, and nephrotoxicity (5). When Cd is released into the cytoplasm, it can induce the production of reactive oxygen species (ROS), lipid peroxidation, depletion of glutathione (GH), cross-linking of proteins, and inflammation. As a result, proinflammatory cytokines accumulate, and kidney cells die, resulting in kidney damage (13, 41). ROS production is the major mechanism of Cd-induced kidney toxicity as it may change cell redox balance (42). However, PSB is well-recognized for its wide variety of pharmaceutical potentials, including analgesic, antioxidant, and antiinflammatory properties (43). PSB has benzo-γ-pyrone in its structure, possibly imparting ROS scavenging potential to PSB and reducing oxidative stress and inflammation (44). Keeping this evidence under consideration, we aimed to assess the therapeutic role of PSB against Cd-induced kidney damage.
Cd exposure led to a significant decrease in the activities of antioxidant enzymes and an increase in the levels of MDA and ROS. This imbalance between ROS and antioxidant enzymes resulted in oxidative stress (45). GPx, CAT, and SOD are critical enzymes involved in the removal of ROS (46). SOD is the primary antioxidant enzyme that converts O−2 into hydrogen peroxide (45). Both CAT and GPx then convert hydrogen peroxide into oxygen and H2O (47). Previous studies have shown that Cd induces damage in renal cells by producing ROS (48). In earlier investigations, it has been stated that Cd exposure can increase ROS levels, which, as a result, can decrease antioxidant enzyme activities and increase LPO (49). However, the administration of PSB has the potential to reverse these Cd-induced effects on antioxidant enzymes by reducing ROS production. The ability of PSB to protect against oxidative stress may be due to the presence of benzo-γ-pyrone in its structure, which may impart antioxidant potential to PSB (50).
The removal of creatinine and urea from the body is based on the glomerular filtration rate (51). Increased levels of urea can lead to kidney dysfunction, abnormal excretion, and tissue damage (52). A substantial increase in creatinine concentration can be due to the loss of glomerular function and tubular damage in renal tissues (53). KIM-1 and NGAL are promising markers for the assessment of acute renal failure (54). KIM-1 is a type 1 membrane glycoprotein that is highly expressed in proximal tubule cells after exposure to nephrotoxic agents (54). Furthermore, NGAL is a cytoplasmic protein that can be detected in the blood, urine, renal, and proximal-distal tubules following renal damage (55). Therefore, elevated levels of these markers indicate kidney damage. These findings are further supported by the study conducted by Kamel et al. (56), who reported that Cd administration increased the levels of KIM-1, NGAL, creatinine, and urea in rat kidneys. However, PSB treatment normalized these markers and restored KIM-1, NGAL, creatinine, urea, and creatinine clearance. These findings demonstrate the nephroprotective properties of PSB.
NF-κB is a key regulator of the immune response and various inflammatory ailments. It also plays a major role in the activation of inflammatory cytokines such as COX-2, IL-6, TNF-α, IL-1β, and iNOS (57). Previous investigations have proven that heavy metals can directly increase the production of proinflammatory cytokines (58). Moreover, COX-2 and iNOS enzymes modulate the inflammatory response by producing prostaglandin E2 and nitric oxide, respectively (59). Nitric oxide can make the cell more vulnerable to ROS by reducing intracellular glutathione content (60). Several previous studies have indicated that Cd exposure in kidney tissues can activate NF- κB, which increases IL-1, IL-6, and TNF-α while decreasing IL-10 (61–63). Conversely, PSB treatment downregulated NF-κB expression and significantly reduced the levels of IL-6, TNF-α, and IL-1β. PSB significantly suppressed iNOS and COX-2 activities, which might be attributed to its ring structure, as confirmed by the earlier study that reported the antiinflammatory potential of flavonoids to be attributed to the non-methoxylation of the 3′-hydroxyl groups on the B-ring or methoxylation of the 5- or 7-hydroxyl groups on the A-ring (64).
Apoptosis occurs due to an imbalance in apoptotic and antiapoptotic proteins through mitochondrial-independent and dependent pathways (65). Downregulation of Bcl-2 and upregulation of Bax severely change the stability of the mitochondrial membrane (66). Bax and Bcl-2 facilitate the discharge of cytochrome c from mitochondria, which initiates the basic apoptotic pathway (67, 68). Caspase-3 is identified as a crucial apoptosis mediator, as it starts the apoptotic mechanism by stimulating other caspase enzymes (69). A previous investigation supports our results that Cd exposure significantly increased the expression of Caspase-3 and Bax while decreasing the level of Bcl-2 in the renal tissues of rats (70, 71). Cd treatment led to a substantial increase in Bax and Caspase-3 expression while reducing the levels of Bcl-2. However, PSB treatment prevented Cd-induced renal apoptosis by suppressing the activation of Caspase-3 and downregulating Bax, leading to an upregulation of Bcl-2. The outcomes of our investigation indicate that PSB possesses antiapoptotic potential against Cd exposure.
Mitochondria are known as the powerhouse of the cell due to their ability to produce ATP through the process of oxidative phosphorylation (OP) (72). Mitochondria are the most critical organelles and play a central role in maintaining cellular homeostasis. Therefore, mitochondrial dysfunction may lead to tissue or cellular damage (73). Through the TCA cycle, mitochondrial enzymes catalyze the oxidation of various substrates, reducing their equivalents. These electrons are transferred across the respiratory chain to generate ATP through OP (74, 75). The study conducted by Ijaz et al. (17) demonstrated that Cd exposure decreased TCA cycle enzyme activities, which eventually caused mitochondrial impairment in the kidneys of rats. Conversely, co-administration of PSB with Cd reversed TCA cycle enzyme activities, possibly by downregulating OS.
In mitochondria, the electron transport chain (ETC) is responsible for oxidative phosphorylation, which uses fatty acids and pyruvate to produce ATP. Under physiological conditions, ETC is supposed to be the primary cause of ROS generation (76). According to earlier studies, the accumulation of intracellular ROS is a major cause of mitochondrial ETC damage (77, 78). Mitochondrial ETC can be damaged, which is usually indicated by OS and reduced ATP generation (79). When the mitochondrial electron transport chain's transmission is interrupted, ATP production is affected, which results in mitochondrial damage (80). In an earlier study, Belyaeva et al. (81) stated that both membrane permeability and mitochondrial ETC are the major targets of Cd-induced mitochondrial damage. However, PSB treatment probably diminished mitochondrial dysfunction by increasing complex (I-V) activities to their normal ranges due to its mitigative effects.
Cd exposure resulted in mitochondrial membrane potential collapse (ΔΨm). ΔΨm produced via mitochondrial complexes during oxidative phosphorylation is important in energy storage. ΔΨm also plays a critical role in maintaining mitochondrial homeostasis (82). Overproduction of ROS can interrupt multiple mitochondrial processes, i.e., mitochondrial swelling, MMP collapse, and reduced dehydrogenase activity (83).
The maintenance of ΔΨm is also essential for the movement of mitochondria (84). According to a previous study, the anterograde mobility of mitochondria is crucial for their proper functioning (85). According to previous research, the downregulation of ETC reduces proton efflux through the inner membrane of mitochondria, which disrupts the depolarization of ΔΨm (86). Our findings suggested that PSB has the potential to restore Cd-induced ΔΨm loss by upregulating the activities of ETC complexes. Moreover, PSB administration significantly restored Cd-mediated ΔΨm. This normalization is possibly due to an increase in the activity of the ETC complex.
Cd exposure resulted in degeneration of tubular cells and epithelial cells, glomerular atrophy, leucocyte infiltration, and disruption of Bowman's capsule in the proximal renal tubules. These histomorphological changes could reflect direct impairment in renal tissues. Our findings are further supported by the investigation of Kamel et al. (56), who reported that Cd administration led to histomorphological changes in renal tissues, such as tubular and glomerular atrophy. It is speculated that histomorphological changes may be due to the excessive production of ROS caused by Cd exposure (87), which resulted in oxidative impairment (88) and morphological changes in renal tissue. However, the PSB-co-treated group displayed mild to moderate vacuolation in the epithelium of tubules. Furthermore, Cd co-administration with PSB significantly mitigated the above-mentioned histopathological damage. This may be attributed to the antioxidant capability of PSB, which significantly decreased oxidative stress, leading to a decrease in pathological alterations.
Conclusion
In conclusion, our research demonstrated that PSB supplementation potentially attenuated the Cd-induced hazardous effects on respiratory chain complexes, urea, creatinine, antioxidant enzymes, creatinine clearance, TCA cycle enzymes, and ΔΨm. Furthermore, PSB administration considerably reduced Cd-induced renal dysfunction by mitigating renal oxidative stress, apoptosis, and inflammatory reactions. PSB regulates renal functions by restoring TCA cycle enzyme activities and ETC complexes. These nephroprotective effects of PSB against Cd-mediated nephrotoxicity may be attributed to its antiinflammatory, antiapoptotic, and antioxidant nature. Taken together, it can be concluded that PSB may have some clinical applications in the future to cure Cd-induced renal dysfunctions in humans.
Limitation
The limitation of the study is that it was conducted on animal models, and it is necessary to conduct clinical trials in the future to determine the effectiveness and safety of PSB in humans.
Data availability statement
The original contributions presented in the study are included in the article/supplementary material, further inquiries can be directed to the corresponding author.
Ethics statement
This animal study was authorized by the Institutional Biosafety/Bioethics Committee (IBC) of the University of Agriculture, Faisalabad, according to the (CEE Council 86/609) protocol.
Author contributions
MI, TA, SR, and SS perceived the idea and planned the investigation. AH and RA conducted the experiments. HA assisted in the statistical analysis. MI, AN, MA-O, SR, TA, MB, HS, SS, and AH wrote the manuscript. All authors have read and authorized the final version of the manuscript.
Acknowledgments
The authors are grateful to the Researchers Supporting Project Number (RSPD2023R740), King Saud University, Riyadh, Saudi Arabia, for funding this project.
Conflict of interest
The authors declare that the research was conducted in the absence of any commercial or financial relationships that could be construed as a potential conflict of interest.
Publisher's note
All claims expressed in this article are solely those of the authors and do not necessarily represent those of their affiliated organizations, or those of the publisher, the editors and the reviewers. Any product that may be evaluated in this article, or claim that may be made by its manufacturer, is not guaranteed or endorsed by the publisher.
References
1. Ma Y, He X, Qi K, Wang T, Qi Y, Cui L, et al. Effects of environmental contaminants on fertility and reproductive health. J Environ Sci. (2019) 77:210–7. doi: 10.1016/j.jes.2018.07.015
2. Unsal V, Dalkiran T, Cicek M. The role of natural antioxidants against reactive oxygen species produced by cadmium toxicity: a review. Adv Pharm Bull. (2020) 10:184–202. doi: 10.34172/apb.2020.023
3. Siu ER, Mruk DD, Porto CS, Cheng CY. Cadmium-induced testicular injury. Toxicol Appl Pharm. (2009) 238:240–9. doi: 10.1016/j.taap.2009.01.028
4. Branca JJ, Pacini A, Gulisano M, Taddei N, Fiorillo C, Becatti M. Cadmium-induced cytotoxicity: effects on mitochondrial electron transport chain. Front Cell Dev Biol. (2020) 8:604377. doi: 10.3389/fcell.2020.604377
5. Seif MM, Madboli AN, Marrez DA, Aboulthana WM. Hepato-renal protective effects of Egyptian purslane extract against experimental cadmium toxicity in rats with special emphasis on the functional and histopathological changes. Toxicol Rep. (2019) 6:625–31. doi: 10.1016/j.toxrep.2019.06.013
6. Rahman Z, Singh VP. The relative impact of toxic heavy metals (THMs) (arsenic (As) cadmium (Cd) chromium (Cr) (VI) mercury (Hg) and lead (Pb)) on the total environment: an overview. Environ Monit Assess. (2019) 191:1–21. doi: 10.1007/s10661-019-7528-7
7. Gobe GC, Johnson DW. Distal tubular epithelial cells of the kidney: potential support for proximal tubular cell survival after renal injury. Int J Biochem Cell Biol. (2007) 39:1551–61. doi: 10.1016/j.biocel.2007.04.025
8. Kar I, Patra AK. Tissue bioaccumulation and toxicopathological effects of cadmium and its dietary amelioration in poultry—A review. Biol Trace Elem Res. (2021) 199:3846–68. doi: 10.1007/s12011-020-02503-2
9. Handan BA, De-Moura CF, Cardoso CM, Santamarina AB, Pisani LP, Ribeiro DA. Protective effect of grape and apple juices against cadmium intoxication in the kidney of rats. Drug Res. (2020) 70:503–11. doi: 10.1055/a-1221-4733
10. Bharathiraja K, Babu LH, Vijayaprakash S, Tamilselvan P, Balasubramanian MP. Fucoxanthin a marine carotenoid protects cadmium-induced oxidative renal dysfunction in rats. Biomed Preventive Nutrit. (2013) 8:201–7. doi: 10.1016/j.bionut.2013.04.005
11. Jarup L, Akesson A. Current status of cadmium as an environmental health problem. Toxicol Appl Pharmacol. (2009) 238:201–8. doi: 10.1016/j.taap.2009.04.020
12. Kim KS, Lim HJ, Lim JS, Son JY, Lee J, Lee BM, et al. Curcumin ameliorates cadmium-induced nephrotoxicity in Sprague-Dawley rats. Food Chem Toxicol. (2018) 114:34–40. doi: 10.1016/j.fct.2018.02.007
13. Ansari MA, Raish M, Ahmad A, Alkharfy KM, Ahmad SF, Attia SM, et al. Sinapic acid ameliorate cadmium-induced nephrotoxicity: In vivo possible involvement of oxidative stress apoptosis and inflammation via NF-κB downregulation. Environ Toxicol Pharmacol. (2917) 51:100–7. doi: 10.1016/j.etap.2017.02.014
14. El-Boshy ME, Risha EF, Abdelhamid FM, Mubarak MS, Hadda TB. Protective effects of selenium against cadmium induced hematological disturbances immunosuppressive oxidative stress and hepatorenal damage in rats. J Trace Elem Med Biol. (2015) 29:104–10. doi: 10.1016/j.jtemb.2014.05.009
15. Dkhil MA, Al-Quraishy S, Diab MM, Othman MS, Aref AM, Moneim AE. The potential protective role of Physalis peruviana L. fruit in cadmium-induced hepatotoxicity and nephrotoxicity. Food Chem Toxicol. (2014) 74:98–106. doi: 10.1016/j.fct.2014.09.013
16. Bhargava P, Schnellmann RG. Mitochondrial energetics in the kidney. Nat Rev Nephrol. (2017) 13:629–46. doi: 10.1038/nrneph.2017.107
17. Ijaz MU, Batool M, Batool A, Al-Ghanimd KA, Zafar S, Ashraf A, et al. Protective effects of vitexin on cadmium-induced renal toxicity in rats. Saudi J Biol Sci. (2021) 28:5860–4. doi: 10.1016/j.sjbs.2021.06.040
18. Patel NK, Bhutani KK. Pinostrobin and Cajanus lactone isolated from Cajanus cajan (L) leaves inhibits TNF-α and IL-1β production: in vitro and in vivo experimentation. Phytomedicine. (2014) 21:946–53. doi: 10.1016/j.phymed.2014.02.011
19. Smolarz HD, Mendyk E, Bogucka-Kocka A, Kockic J. Pinostrobin–an anti-leukemic flavonoid from Polygonum lapathifolium L. ssp nodosum (Pers) Dans Z Naturforsch C. (2006) 61:64–8. doi: 10.1515/znc-2006-1-212
20. Ashidi JS, Houghton PJ, Hylands PJ, Efferth T. Ethnobotanical survey and cytotoxicity testing of plants of South-western Nigeria used to treat cancer with isolation of cytotoxic constituents from Cajanus cajan Millsp. leaves J Ethnopharmacol. (2010) 128:501–12. doi: 10.1016/j.jep.2010.01.009
21. Tewtrakul S, Subhadhirasakul S, Karalai C, Ponglimanont C, Cheenpracha S. Antiinflammatory effects of compounds from Kaempferia parviflora and Boesenbergia pandurata. Food Chem. (2009) 115:534–8. doi: 10.1016/j.foodchem.2008.12.057
22. Shindo K, Kato M, Kinoshita A, Kobayashi A, Koike Y. Analysis of antioxidant activities contained in the Boesenbergia pandurata Schult. Rhizome Biosci Biotechnol Biochem. (2006) 70:2281–4. doi: 10.1271/bbb.60086
23. Patel NK, Jaiswal G, Bhutani KK, A. review on biological sources chemistry and pharmacological activities of pinostrobin. Nat Prod Res. (2016) 30:2017–27. doi: 10.1080/14786419.2015.1107556
24. Xian YF, Ip SP, Lin ZX, Mao QQ, Su ZR, Lai XP. Protective effects of pinostrobin on β-amyloid-induced neurotoxicity in PC12 cells. Cell Mol Neurobiol. (2012) 32:1223–30. doi: 10.1007/s10571-012-9847-x
25. Wu N, Kong Y, Zu Y, Fu Y, Liu Z, Meng R, et al. Activity investigation of pinostrobin towards herpes simplex virus-1 as determined by atomic force microscopy. Phytomedicine. (2011) 18:110–8. doi: 10.1016/j.phymed.2010.07.001
26. Mingatto FE, Santos AC, Uyemura SA, Jordani MC, Curti C. In vitrointeraction of nonsteroidal antiinflammatory drugs on oxidative phosphorylation of rat kidney mitochondria: respiration and ATP synthesis. Arch Biochem Biophys. (1996) 334:303–8. doi: 10.1006/abbi.1996.0459
28. Carlberg I, Mannervik B. Purification and characterization of the flavoenzyme glutathione reductase from rat liver. J Biol Chem. (1975) 250:5475–80. doi: 10.1016/S0021-9258(19)41206-4
29. Lawrence RA, Burk RF. Glutathione peroxidase activity in selenium–deficient rat liver. Biochem Biophys Res Commun. (1976) 71:952–8. doi: 10.1016/0006-291X(76)90747-6
30. Ohkawa H, Ohishi N, Yagi K. Assay for peroxides in animal tissues by thiobarbituric acid reaction. Anal Biochem. (1979) 95:351–8. doi: 10.1016/0003-2697(79)90738-3
31. Kucukler S, Benzer F, Yildirim S, Gur C, Kandemir FM, Bengu AS, et al. Protective effects of chrysin against oxidative stress and inflammation induced by lead acetate in rat kidneys: a biochemical and histopathological approach. BiolTrace Elem Res. (2021) 199:1501–14. doi: 10.1007/s12011-020-02268-8
32. Livak KJ, Schmittgen TD. Analysis of relative gene expression data using real-time quantitative PCR and the 2–DDCT method. Methods. (2001) 25:402–8. doi: 10.1006/meth.2001.1262
33. Ijaz MU, Tahir A, Samad A, Anwar H. Nobiletin ameliorates nonylphenol-induced testicular damage by improving biochemical steroidogenic hormonal spermatogenic apoptotic and histological profile. Hum Exp Toxicol. (2021) 40:403–16. doi: 10.1177/0960327120950007
34. Bernt E, Bergmeyer HU. Methods of Enzymatic Analysis. New York: Academic Press (1974) 624–627. doi: 10.1016/B978-0-12-091302-2.50023-2
35. Slater EC, Borner WD. The effect of fluoride on the succinic oxidase system. Biochem J. (1952) 52:185–96. doi: 10.1042/bj0520185
36. Mehler AH, Kornberg A, Grisolia S, Ochoa S. The enzymatic mechanism of oxidation-reductions between malate or isocitrate and pyruvate. J Biol Chem. (1948) 174:961–77. doi: 10.1016/S0021-9258(18)57306-3
37. Reed LJ, Mukherjee RB. ά-Ketoglutarate dehydrogenase complex from Escherichia coli. In: Methods in Enzymology (Eds) Colowick SP Kaplon NO Academic press New York. (1969) 13:53–61. doi: 10.1016/0076-6879(69)13016-5
38. Baracca A, Sgarbi G, Solaini G, Lenaz G. Rhodamine 123 as a probe of mitochondrial membrane potential: evaluation of proton flux through F (0) during ATP synthesis. Biochim Biophys Acta. (2003) 1606:137–46. doi: 10.1016/S0005-2728(03)00110-5
39. Renugadevi J, Prabu SM. Quercetin protects against oxidative stress-related renal dysfunction by cadmium in rats. Exp Toxicol Pathol. (2010) 62:471–81. doi: 10.1016/j.etp.2009.06.006
40. Anetor JI. Rising environmental cadmium levels in developing countries: threat to genome stability and health. Niger J Physiol Sci. (2012) 27:103–15. doi: 10.4172/2161-0525.1000140
41. Morales AI, Vicente-Sanchez C, Sandoval JS, Egido J, Mayoral P, Arévalo MA, et al. Protective effect of quercetin on experimental chronic cadmium nephrotoxicity in rats is based on its antioxidant properties. Food Chem Toxicol. (2006) 44:2092–100. doi: 10.1016/j.fct.2006.07.012
42. Lee JC, Son YO, Pratheeshkumar P, Shi X. Oxidative stress and metal carcinogenesis. Free Radic Biol Med. (2012) 53:742–57. doi: 10.1016/j.freeradbiomed.2012.06.002
43. González AS, Tellini VH, Gutiérrez DM. Study of the dermal anti-inflammatory antioxidant and analgesic activity of Pinostrobin. Heliyon. (2022) 8:10413. doi: 10.1016/j.heliyon.2022.e10413
44. Hidajati N, Tukiran T, Setiabudi DA, Wardana AP. Antioxidant activity of palmitic acid and pinostrobin from methanol extract of Syzygium litoralle (Myrtaceae) Int Conf Sci Tech. (2018) 183–187. doi: 10.2991/icst-18.2018.39
45. Birben E, Sahiner UM, Sackesen C, Erzurum S, Kalayci O. Oxidative stress and antioxidant defense. World Allergy Organ J. (2012) 5:9–19. doi: 10.1097/WOX.0b013e3182439613
46. Ma X, Deng D, Chen W. Inhibitors and Activators of SOD GSH-Px and CAT. Enzyme Inhibit Activat. (2017) 29:207. doi: 10.5772/65936
47. Schieber M, Chandel NS, ROS. function in redox signaling and oxidative stress. Curr Biol. (2014) 24:453–62. doi: 10.1016/j.cub.2014.03.034
48. Koobroob A, Chattipakorn N, Wongmekiat O. Caffeic acid phenethyl ester ameliorates cadmium-induced kidney mitochondrial injury. Chem Biol Interact. (2012) 200:21–7. doi: 10.1016/j.cbi.2012.08.026
49. Iserhienrhien LO, Okolie NP. Protective effect of Geophila obvallata (Shumach) Didr leaf extract and its fractions against cadmium-induced nephrotoxicity in male Wistar rats. Toxicol Rep. (2022) 9:87–93. doi: 10.1016/j.toxrep.2021.12.008
50. Kumar S, Pandey AK. Chemistry and biological activities of flavonoids: an overview. Sci World J. (2013) 2013:1–16. doi: 10.1155/2013/162750
51. Higgins C. Urea and Creatinine Concentration the Urea: Creatinine Ratio. Acute Care Testing Handbook. Cambridge: Abcam (2016) 1–8.
52. Yousef MI, Awad TI, Mohamed EH. Deltamethrin-induced oxidative damage and biochemical alterations in rat and its attenuation by Vitamin E. Toxicology. (2006) 227:240–7. doi: 10.1016/j.tox.2006.08.008
53. Mansour SA, Mossa AT. Oxidative damage biochemical and histopathological alterations in rats exposed to chlorpyrifos and the antioxidant role of zinc. Pestic Biochem Physiol. (2010) 96:14–23. doi: 10.1016/j.pestbp.2009.08.008
54. Ichimura T, Asseldonk EJ, Humphreys BD, Gunaratnam L, Duffield JS, Bonventre JV. Kidney injury molecule-1 is a phosphatidylserine receptor that confers a phagocytic phenotype on epithelial cells. J Clin Invest. (2008) 118:1657–68. doi: 10.1172/JCI34487
55. Mori K, Lee HT, Rapoport D, Drexler IR, Foster K, Yang J, et al. Endocytic delivery of lipocalin-siderophore-iron complex rescues the kidney from ischemia-reperfusion injury. J Clin Invest. (2005) 115:610–21. doi: 10.1172/JCI23056
56. Kamel EO, Gad-Elrab WM, Ahmed MA, Mohammedsaleh ZM, Hassanein EH, Ali FE. Candesartan protects against cadmium-induced hepatorenal syndrome by affecting Nrf2 NF-κB Bax/Bcl-2/Cyt-C and Ang II/Ang 1–7 signals. Biol Trace Elem Res. (2022) 3:1–8. doi: 10.1007/s12011-022-03286-4
57. Kandemir FM, Yildirim S, Kucukler S, Caglayan C, Mahamadu A, Dortbudak MB. Therapeutic efficacy of zingerone against vancomycin-induced oxidative stress inflammation apoptosis and aquaporin 1 permeability in rat kidney. Biomed Pharmacother. (2018) 105:981–91. doi: 10.1016/j.biopha.2018.06.048
58. Olszowski T, Gutowska I, Baranowska-Bosiacka I, Piotrowska K, Korbecki J, Kurzawski, et al. The effect of cadmium on COX-1 and COX-2 gene protein expression and enzymatic activity in THP-1 macrophages. Biol Trace Elem Res. (2015) 165:135–44. doi: 10.1007/s12011-015-0234-6
59. Jeong JB, Hong SC, Jeong HJ, Koo JS. Antiinflammatory effect of 2-methoxy-4-vinylphenol via the suppression of NF-κB and MAPK activation and acetylation of histone H3. Arch Pharm Res. (2011) 34:2109–16. doi: 10.1007/s12272-011-1214-9
60. Kandemir FM, Yildirim S, Caglayan C, Kucukler S, Eser G. Protective effects of zingerone on cisplatin-induced nephrotoxicity in female rats. Environ Sci Pollut Res. (2019) 26:22562–74. doi: 10.1007/s11356-019-05505-3
61. Almeer RS, AlBasher GI, Alarifi S, Alkahtani S, Ali D, Abdel Moneim AE. Royal jelly attenuates cadmium-induced nephrotoxicity in male mice. Sci Rep. (2019) 9:1–12. doi: 10.1038/s41598-019-42368-7
62. Das S, Dewanjee S, Dua TK, Joardar S, Chakraborty P, Bhowmick S, et al. Carnosic acid attenuates cadmium induced nephrotoxicity by inhibiting oxidative stress promoting Nrf2/HO-1 signalling and impairing TGF-β1/Smad/collagen IV signalling. Molecules. (2019) 24:4176. doi: 10.3390/molecules24224176
63. Aktoz T„ Kanter M Uz YH, Aktaş C, Erboga M, Atakan IH. Protective effect of quercetin against renal toxicity induced by cadmium in rats. Balk Med J. (2012) 2012:56–61. doi: 10.5152/balkanmedj.2011.014
64. During A, Larondelle Y. The O-methylation of chrysin markedly improves its intestinal antiinflammatory properties: structure–activity relationships of flavones. Biochem Pharmacol. (2013) 86:1739–46. doi: 10.1016/j.bcp.2013.10.003
65. Sinha K, Das J, Pal PB, Sil PC. Oxidative stress: the mitochondria-dependent and mitochondria-independent pathways of apoptosis. Arch Toxicol. (2013) 87:1157–80. doi: 10.1007/s00204-013-1034-4
66. Gu YP, Yang XM, Duan ZH, Luo P, Shang JH, Xiao W, et al. Inhibition of chemotherapy-induced apoptosis of testicular cells by squid ink polysaccharide. Exp Ther Med. (2017) 14:5889. doi: 10.3892/etm.2017.5342
67. Caglayan C, Kandemir FM, Yildirim S, Kucukler S, Eser G. Rutin protects mercuric chloride-induced nephrotoxicity via targeting of aquaporin 1 level oxidative stress apoptosis and inflammation in rats. J Trace Elem Med Biol. (2019) 54:69–78. doi: 10.1016/j.jtemb.2019.04.007
68. Kuzu M, Yildirim S, Kandemir FM, Küçükler S, Çaglayan C, Türk E, et al. Protective effect of morin on doxorubicin-induced hepatorenal toxicity in rats. Chem Biol Interact. (2019) 308:89–100. doi: 10.1016/j.cbi.2019.05.017
69. Eldutar E, Kandemir FM, Kucukler S, Caglayan C. Restorative effects of Chrysin pretreatment on oxidant–antioxidant status inflammatory cytokine production and apoptotic and autophagic markers in acute paracetamol-induced hepatotoxicity in rats: an experimental and biochemical study. J Biochem Mol Toxicol. (2017) 31:e21960. doi: 10.1002/jbt.21960
70. Algefare AI. Renoprotective and oxidative stress-modulating effects of taxifolin against cadmium-induced nephrotoxicity in mice. Life. (2022) 12:1150. doi: 10.3390/life12081150
71. Yuan G, Dai S, Yin Z, Lu H, Jia R, Xu J, et al. Sub-chronic lead and cadmium co-induce apoptosis protein expression in liver and kidney of rats. Int J Clin Exp Pathol. (2014) 7:2905–14. doi: 10.1016/j.fct.2013.12.041
72. Bertram R, Pedersen MG, Luciani DS, Sherman A. A simplified model for mitochondrial ATP production. J Theor Biol. (2006) 243:575–86. doi: 10.1016/j.jtbi.2006.07.019
73. Bruno SR, Anathy V. Lung epithelial endoplasmic reticulum and mitochondrial 3D ultrastructure: a new frontier in lung diseases. Histochem Cell Biol. (2021) 155:291–300. doi: 10.1007/s00418-020-01950-1
74. Lodish H, Berk A, Zipursky SL, Matsudaira P, Baltimore D, Darnell J. Electron transport and oxidative phosphorylation. in Molecular Cell Biology. 4th edition. WH Freeman (2000).
75. Chandramohan G, Al-Numair KS, Veeramani C, Alsaif MA, Almajwal AM. Protective effect of kaempferol a flavonoid compound on oxidative mitochondrial damage in streptozotocin-induced diabetic rats. Prog Nutr. (2015) 17:238–44. doi: 10.1179/1351000214Y.000000011
76. Zhao RZ, Jiang S, Zhang L, Yu ZB. Mitochondrial electron transport chain ROS generation and uncoupling. Int J Mol Med. (2019) 44:3–15. doi: 10.3892/ijmm.2019.4188
77. Cheraghi G, Hajiabedi E, Niaghi B, Nazari F, Naserzadeh P, Hosseini MJ. High doses of sodium tungstate can promote mitochondrial dysfunction and oxidative stress in isolated mitochondria. J Biochem Mol Toxicol. (2019) 33:e22266. doi: 10.1002/jbt.22266
78. Druck T, Cheung DG, Park D, Trapasso F, Pichiorri F, Gaspari M, et al. Fhit–Fdxr interaction in the mitochondria: Modulation of reactive oxygen species generation and apoptosis in cancer cells. Cell Death Dis. (2019) 10:1–10. doi: 10.1038/s41419-019-1414-7
79. Fernandez-Mosquera L, Yambire KF, Couto R, Pereyra L, Pabis K, Ponsford AH, et al. Mitochondrial respiratory chain deficiency inhibits lysosomal hydrolysis. Autophagy. (2019) 15:1572–91. doi: 10.1080/15548627.2019.1586256
80. Schwarz K, Siddiqi N, Singh S, Neil CJ, Dawson DK, Frenneaux MP. The breathing heart—mitochondrial respiratory chain dysfunction in cardiac disease. Int J Cardiol. (2014) 171:134–43. doi: 10.1016/j.ijcard.2013.12.014
81. Belyaeva EA, Glazunov VV, Korotkov SM. Cd2C -promoted mitochondrial permeability transition: a comparison with other heavy metals. Acta Biochim Pol. (2004) 51:545–51. doi: 10.18388/abp.2004_3590
82. Zorova LD, Popkov VA, Plotnikov EY, Silachev DN, Pevzner IB, Jankauskas SS, et al. Mitochondrial membrane potential. Anal Biochem. (2018) 552:50–9. doi: 10.1016/j.ab.2017.07.009
83. Georgieva E, Ivanova D, Zhelev Z, Bakalova R, Gulubova M, Aoki I. Mitochondrial dysfunction and redox imbalance as a diagnostic marker of “free radical diseases”. Anticancer Res. (2017) 37:5373–81. doi: 10.21873/anticanres.11963
84. Ly JD, Grubb DR, Lawen A. The mitochondrial membrane potential (Δψm) in apoptosis; an update. Apoptosis. (2003) 8:115–28. doi: 10.1023/A:1022945107762
85. Kalmar B, Innes A, Wanisch K, Kolaszynska AK, Pandraud A, Kelly G, et al. Mitochondrial deficits and abnormal mitochondrial retrograde axonal transport play a role in the pathogenesis of mutant Hsp27- induced Charcot Marie Tooth Disease. Hum Mol Genet. (2017) 26:3313–26. doi: 10.1093/hmg/ddx216
86. Forkink M, Manjeri GR, Liemburg-Apers DC, Nibbeling E, Blanchard M, Wojtala A, et al. Mitochondrial hyperpolarization during chronic complex I inhibition is sustained by low activity of complex II III IV and V. Biochim Biophys Acta. (2014) 1837:1247–56. doi: 10.1016/j.bbabio.2014.04.008
87. Zhong L, Wang L, Xu L, Liu Q, Jiang L, Zhi Y, et al. The role of nitric oxide synthase in an early phase Cd-induced acute cytotoxicity in MCF-7 cells. Biol Trace Elem Res. (2015) 164:130–8. doi: 10.1007/s12011-014-0187-1
Keywords: cadmium, pinostrobin, mitochondria, renal damage, apoptosis
Citation: Ijaz MU, Shahzadi S, Hamza A, Azmat R, Anwar H, Afsar T, Shafique H, Bhat MA, Naglah AM, Al-Omar MA and Razak S (2023) Alleviative effects of pinostrobin against cadmium-induced renal toxicity in rats by reducing oxidative stress, apoptosis, inflammation, and mitochondrial dysfunction. Front. Nutr. 10:1175008. doi: 10.3389/fnut.2023.1175008
Received: 01 March 2023; Accepted: 26 April 2023;
Published: 24 May 2023.
Edited by:
Hui-Xin Liu, China Medical University, ChinaReviewed by:
Sabreen Ezzat Fadl, Matrouh University, EgyptIrmak Ferah Okkay, Atatürk University, Türkiye
Copyright © 2023 Ijaz, Shahzadi, Hamza, Azmat, Anwar, Afsar, Shafique, Bhat, Naglah, Al-Omar and Razak. This is an open-access article distributed under the terms of the Creative Commons Attribution License (CC BY). The use, distribution or reproduction in other forums is permitted, provided the original author(s) and the copyright owner(s) are credited and that the original publication in this journal is cited, in accordance with accepted academic practice. No use, distribution or reproduction is permitted which does not comply with these terms.
*Correspondence: Muhammad Umar Ijaz, dW1hci5pamF6QHVhZi5lZHUucGs=; Suhail Razak, U21hcmF6aUBrc3UuZWR1LnNh