- 1Department of Exercise Physiology, Faculty of Sport Sciences, University of Mazandaran, Babolsar, Iran
- 2Department of Health and Human Performance, Marymount University, Arlington, TX, United States
- 3Department of Internal Medicine, School of Medicine, Firoozgar General Hospital, Iran University of Medical Sciences, Tehran, Iran
- 4Department of Exercise Physiology, Faculty of Sport Sciences, University of Mazandaran, Babolsar, Iran
- 5Athletic Performance and Health Research Center, University of Mazandaran, Babolsar, Iran
The purpose of this study was to examine the association between the dietary antioxidant quality score (DAQS) and the severity of Coronavirus disease 2019 (COVID-19). The present case–control study was carried out on 295 patients diagnosed with COVID-19 (≥18 years old), including 104 critical patients (Intensive care unit [ICU] admission) and 191 COVID-19 patients without severe complications (Non-intensive care unit [Non-ICU] patients) as cases. Dietary intake was assessed by a 147-item, semi-quantitative food frequency questionnaire (FFQ). Logistic regression was performed to calculate the odds ratio (OR) and 95% confidence interval (CI) for the considered risk factors. Our outcomes (after multivariate adjustment) suggested that higher adherence to DAQS was significantly associated with a decreased risk of COVID-19 infection severity (OR = 0.12; 95% CI: 0.04–0.29, p < 0.001). Similar results were seen when analyzed by sex [men (OR = 0.02; 95% CI: 0.002–0.15, p < 0.001) and women (OR = 0.21; 95% CI: 0.06–0.68, p = 0.012)]. A significant association between vitamin D3 intake and decreased risk of COVID-19 severity (OR = 0.91; 95% CI: 0.89–0.94, p < 0.001) was also observed. Moreover, multivariate results revealed that there were no significant associations between vitamin C (OR = 1.00; 95% CI: 0.99–1.00, p = 0.067), vitamin E (OR = 0.98; 95% CI: 0.86–1.11, p = 0.798), zinc (OR = 1.02; 95% CI: 0.86–1.20, p = 0.805), and selenium (OR = 0.99; 95% CI: 0.99–1.00, p = 0.077) intakes with the risk of COVID-19 severity. However, subgroup analyses by sex suggested a significant association between vitamin C intake and the risk of COVID-19 infection severity in women (OR = 1.00; 95% CI: 1.00–1.00, p = 0.028). Our findings showed a negative association between DAQS adherence and the risk of COVID-19 infection severity. Our results may be used to develop potential dietary therapies to decrease COVID-19 severity.
Introduction
Severe acute respiratory syndrome coronavirus 2 (SARS-CoV-2) appeared a little over 3 years ago, and since then, it has triggered a worldwide pandemic of acute respiratory disease known as the coronavirus disease of 2019 (COVID-19) (1). COVID-19 induces oxidative stress, which in severe cases can lead to the cytokine storm (2), a life-threatening systemic inflammatory syndrome characterized by an uncontrolled expression of immune cells and circulating cytokines (2, 3). The major risk factors for increased COVID-19 severity include male gender, obesity, smoking, older age, cancer, diabetes, cardiovascular disease (CVD), hypertension, chronic obstructive pulmonary disease (COPD), and acute kidney injury (4), which alone or together can lead to elevated oxidative stress levels and inflammation (3, 5–7). Hence, controlling or reducing oxidative stress and inflammation may be considered as the therapeutic target to reverse cytokine storm-associated outcomes (8).
Antioxidants are compounds with important scavenging power that can stop chain reactions, decomposition of hydrogen peroxide, metal-chelating, and antioxidant enzyme induction and thus can prevent or reduce oxidative damage (9). Indeed, previous studies have found that increases in total oxidative stress occur concurrently with declines in antioxidant enzymes (10, 11). Several studies reported that the new antioxidant discoveries for COVID-19 therapy are useful and highlighted the role of dietary antioxidants in COVID-19 infection severity, such as Aminothiadiazole antioxidants (12), Teriflunomide (13), polyphenolic compounds (14, 15), adenosine analogue cordycepin (16), novel 5-Substituted-2-(3,4,5-trihydroxyphenyl)-1,3,4-oxadiazoles compound (17), and novel 5-Substituted-1,3,4-thiadiazole-2-thiols compound (18). In addition, trace elements such as Zinc (Zn), Selenium (Se), Manganese (Mn), Copper (Cu), and vitamins including vitamin C and E act as cofactors of antioxidant enzymes. This is important to point out as plasma concentrations of vitamin A, vitamin C, vitamin E, Se, Zn, Mg, and Cu have been shown to be lower in COVID-19 patients compared to controls (10, 11). Although a relationship between antioxidant compounds and COVID-19 severity is not completely clear, recent literature suggested that supplementary antioxidant compounds could decrease COVID-19 severity (19). For example, previous studies documented the beneficial effects of Zn supplementation in improving gustatory sensitivity (20) and decreasing the duration of anosmia/hyposmia in COVID-19 patients (21). Moreover, selenium parenteral therapy improved respiratory mechanics in adults with acute respiratory distress syndrome (ARDS) (22). Furthermore, COVID-19 patients that underwent parenteral ascorbic acid therapy had higher survival rates (23), a lower incidence of thrombosis (24), and improvements in oxygenation (25) compared to controls. Interestingly, Bousquet et al. (26) pointed out that besides many factors such as trained immunity of the population and public hygiene, diet had an important role in the extension and severity of COVID-19. In fact, Mohajeri et al. (27) demonstrated that dietary intake of antioxidants such as vitamin D, vitamin C, vitamin E, Zn, Se, and some functional foods such as onion, garlic, and oat was significantly higher in healthy cohorts than in COVID-19 patients. Functional foods maintain gut health via the modulation of the gut microbiota, which has enormous beneficial effects (28), while antioxidant supplements may help to boost the immune system, reduce oxidative stress and inflammation, and prevent the progression of COVID-19 disease severity (27, 29). Ebrahimzade et al. (30) indicated that, compared with unhealthy or traditional dietary patterns, healthy dietary patterns can decrease the C-reactive protein (CRP) and erythrocyte sedimentation rate (ESR) levels, as well as negative COVID-19 outcomes. Prior research has also revealed that adherence to the Mediterranean diet decreases COVID-19 severity indicators such as duration of hospitalization and circulating inflammatory biomarkers (31). Additionally, a healthy plant-based diet was associated with a lower risk of severe COVID-19 (32). Despite these encouraging findings, no prior research has focused on overall dietary antioxidant intake and its possible benefits for COVID-19. Focusing on this topic is a necessary step to expand on current knowledge, as people eat a variety of foods with complex substances that can act as antioxidants (33).
The dietary antioxidant quality score (DAQS), used to calculate antioxidant-nutrient intake (vitamin A, vitamin C, vitamin E, Zn, and Se), is derived from the validated food frequency questionnaire (34) and has previously been recommended for the assessment of the overall effects of antioxidants on health-related outcomes. Indeed, some studies evaluated the association between DAQS and cardiorespiratory fitness (34), the risk of mortality among diabetic adults (35), and metabolic syndrome outcomes (36). However, no current research is available evaluating DAQS in COVID-19 hospitalized patients. Therefore, the purpose of the present cross-sectional study was to examine the association between DAQS and COVID-19 severity.
Materials and methods
Participants
The present case–control study was conducted at Firosgar Hospital, located in Tehran, Iran, from April to August 2022. The sample consisted of 295 patients diagnosed with COVID-19 (≥ 18 years old), including 104 critical patients (Intensive Care Unit [ICU] admission) and 191 COVID-19 patients without severe complications (Non-intensive Care Unit [Non-ICU] patients) as cases. Anthropometric measurements and biochemical parameter evaluations were performed within 24 h after hospital admission. Additionally, information on demographic characteristics, socioeconomic status, physical activity levels, and dietary intake was compiled in the same timeframe using validated questionnaires. All participants signed an informed consent form prior to participating in this research. The original ethical participants’ consent form is provided in Supplementary Material 1. This work was approved by the local ethics committee of Mazandaran University (Ethic ID: IR.UMZ.REC.1401.001) and conducted according to the Declaration of Helsinki. The original ethical approval documents are provided in Supplementary Material 2. The participants were registered based on the following inclusion criteria: adults (≥18 years old), positive SARS-CoV-2 detected using the polymerase chain reaction (PCR) method, no previous history of chronic diseases, no adherence to special diets or consumption of antioxidant supplements in the last 3 months. Participants were excluded if they were less than 18 years of age, pregnant or lactating, smokers, or had recent dietary changes. Moreover, those who did not complete questionnaires, did not respond to more than 35 food items available in the food frequency questionnaire, and those who under- or over-reported (less than 800 and more than 4,200 kcal) total daily energy intake were excluded from the analysis.
Demographics, socioeconomic status and physical activity levels
Demographic parameters, including age, sex, education, smoking, marital status, and patients’ admission information, were gathered via a general questionnaire. Socioeconomic levels were evaluated using the Socioeconomic Survey (SES) questionnaire, consisting of five indexes, namely income, occupation, education, wealth, and place of living. Based on this score, the participants were classified into three levels of SES: low (<12), middle (≥12 to <16), and high (≥16) (37). In addition, physical activity levels were evaluated by the International Physical Activity Questionnaire (IPAQ) short form, which is an instrument designed primarily for population surveillance activity among adults (38). The validity and reliability of this questionnaire have been previously established (39).
Biochemical parameters
Within 24 h after hospital admission, venous blood samples were collected between 8 and 10 A.M. and subsequently analyzed. Biochemical parameter levels were then incorporated into the patients’ medical history. Relevant biochemical parameter levels were extracted from the patients’ medical history, including high-sensitive C-reactive protein (hs-CRP), hydrogen potential (pH), partial carbon dioxide pressure (pCO2), base excess (BE), bicarbonate (HCO3), partial oxygen pressure (pO2), lactic dehydrogenase (LDH), creatine phosphokinase (CPK), and creatine kinase MB (CK-MB).
Anthropometrics
The weight of participants was measured and recorded using a Seca scale with an accuracy of 100 grams. The participants’ height was measured using a commercial stadiometer. Body mass index (BMI) was calculated by dividing weight (kilograms) by the square of height (square meters).
Dietary assessment
Dietary intake was evaluated using a valid and reliable (40), 147-item semi-quantitative food frequency questionnaire (FFQ) to determine the usual dietary intake during the year preceding the assessment. Energy and nutrient intake were estimated using the Nutritionist IV software. The DAQS was evaluated based on the daily dietary intake of vitamin E, vitamin A, vitamin C, selenium, and zinc compared to the daily recommended intake (DRI) (41). This comparison was performed for each of the five mentioned nutrients, and the following values were assigned: 0 if the intake was <2/3 of the DRI and 1 if the intake was >2/3 of the DRI. The total DAQS score was calculated by adding the scores of the five antioxidant nutrients, with a range of 0 (very poor quality) to 5 (high quality) (41). To examine the association between DAQS and COVID-19 severity, the DAQS score was divided into three tertiles as follows: Tertile 1: 0 < DAQS score < 3, Tertile 2: DAQS score = 3, and Tertile 3: DAQS score > 3.
Statistical analysis
Baseline characteristics were summarized using mean and standard deviation for continuous variables, and frequencies (%) were utilized for categorical variables. One-way analysis of variance and chi-square tests were used to compare continuous and categorical variables between the groups. Logistic regression was performed to calculate the odds ratio (OR) and 95% confidence interval (CI) for the identified risk factors. Variables with a significance level of p < 0.2 were included in the multivariable model for further analysis. Bivariable regression models, referred to as model 1, were initially presented without adjustment for participant characteristics. This was followed by multivariable regression models, specifically model 2, which accounted for age (years). Additional adjustments were made in model 3, which included age (years), saturated fats (g/d), linoleic fat (g/d), iron (mg/d), biotin (mg/d), dietary fiber (g/d), β-carotene (mg/d), BMI (kg/m2), and magnesium (mg/d). To assess the association between DAQS tertiles, dietary antioxidants, and COVID-19 severity, ORs and 95% CI were estimated. These associations were adjusted for confounding factors. Separate models were developed for sex to examine potential sex-specific effects. Statistical significance was set at p < 0.05. All analyses were performed using STATA Version 16 (Stata Corp).
Results
Participant’s characteristics
Participant’s anthropometrics and biochemical parameters are reported in Table 1. Our results showed that the age (mean ± SD) of ICU patients (62.4 ± 15.3 years, p < 0.001) was significantly higher than non-ICU patients (56.5 ± 14.8 years). Compared to Non-ICU, ICU patients had a higher hs-CRP concentration (16.0 ± 20.1 vs. 9.1 ± 17.8 mg/dL, p < 0.001), D-Dimer (1.39 ± 1.58 vs. 0.98 ± 1.12 mg/L, p = 0.01), LDK (712.6 ± 276.6 vs. 559.0 ± 219.2 U/L, p < 0.001), CPK (213.1 ± 267.1 vs. 104.8 ± 243.7, p < 0.001) and CK-MB (32.8 ± 50.1 vs. 19.5 ± 37.2 U/L, p < 0.001). However, weight (p = 0.415), BMI (p = 0.101), PH (p = 0.402), pCO2 (p = 0.461), BB (p = 0.533), HCO3 (p = 0.176) and pO2 (p = 0.787) did not significantly differ between groups. In addition, non-ICU patients had higher levels of ferritin compared to their ICU counterparts (376.2 ± 166.9 vs. 289.5 ± 163.2 mg/L, p < 0.001).
Participant’s characteristics, physical activity levels and nutrient intake by DAQS tertiles are illustrated in Table 2. Our outcomes indicated that there were fewer critical status patients in highest tertile of DAQS compare to lowest tertile (T3 vs. T1) (p < 0.001). Most dietary intake items differed significantly between DAQS tertiles, such as intake of energy (p < 0.001), protein (p < 0.001), total fat (p < 0.001), saturated fats (p = 0.040), polyunsaturated fats (p < 0.001), linoleic fats (p < 0.001), calcium (p < 0.001), iron (p < 0.001), magnesium (p < 0.001), zinc (p < 0.001), manganese (p < 0.001), fluoride (p = 0.020), vitamin E (p < 0.001), vitamin B1 (p < 0.001), B6 (p < 0.001), vitamin C (p < 0.001), vitamin A (p < 0.001), soluble fiber (p = 0.007), crude fiber (p = 0.001), glucose (p < 0.001), fructose (p < 0.001), lactose (p < 0.001), oleic fat (p < 0.001), sodium (p = 0.08), phosphorus (p < 0.001), copper (p < 0.001), selenium (p = 0.001), B2 (p < 0.001), B6 (p = 0.001), B12 (p < 0.001), biotin (p < 0.001), vitamin D (p < 0.001), dietary fiber (p < 0.001), insoluble fiber (p < 0.001), sugar (p < 0.001), galactose (p = 0.0008), sucrose (p = 0.0012), α-Carotene (p < 0.001) and β-Carotene (p < 0.001). There were no significant differences in other factors, including age (p = 0.647), weight (p = 0.392), BMI (p = 0.143), sex (p = 0.236), education (p = 0.717), socioeconomic status (p = 0.23), physical activity levels (p = 0.441), smoking (p = 0.173), marital status (p = 0.754) as well as EPA-Omega 3 (p = 0.125), sodium (p = 0.084) and maltose (p = 0.440) intakes.
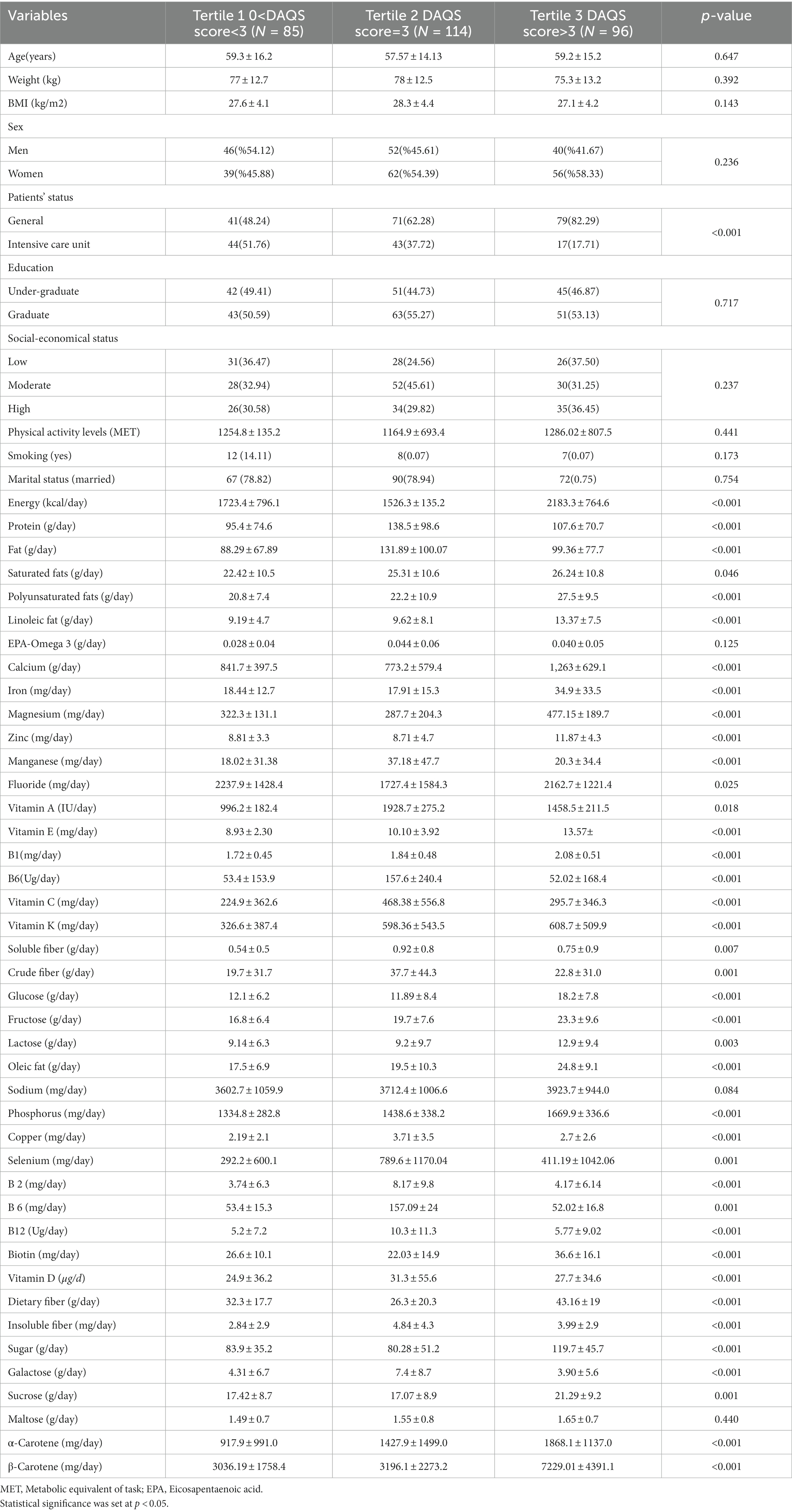
Table 2. Participant’s characteristics, socioeconomic status, physical activity levels, and nutrient intake by dietary antioxidant quality score tertiles.
Table 3 shows the results of the multivariable model for the association between dietary antioxidants and risk of COVID-19 severity. After multivariate adjustment, we observed a significant association between vitamin D intake and decreased risk of severity of COVID-19 infection (OR = 0.91; 95% CI: 0.89–0.94, p < 0.001). Sex subgroup analyses showed similar results among men (OR = 0.87; 95% CI: 0.82–0.92, p < 0.001) and women (OR = 0.94; 95% CI: 0.91–0.97, p < 0.001). Moreover, multivariate results revealed that there was no significant association between vitamin C (OR = 1.00; 95% CI: 0.99–1.00, p = 0.067), vitamin E (OR = 0.98; 95% CI: 0.86–1.11, p = 0.798), Zn (OR = 1.02; 95% CI: 0.86–1.20, p = 805), and Se (OR = 0.99; 95% CI: 0.99–1.00, p = 0.077) intake and risk of COVID-19 infection severity. However, sex subgroup analyses suggested a significant positive association between vitamin C intake and enhanced risk of COVID-19 infection severity in women (OR = 1.00; 95% CI: 1.00–1.00, p = 0.028).
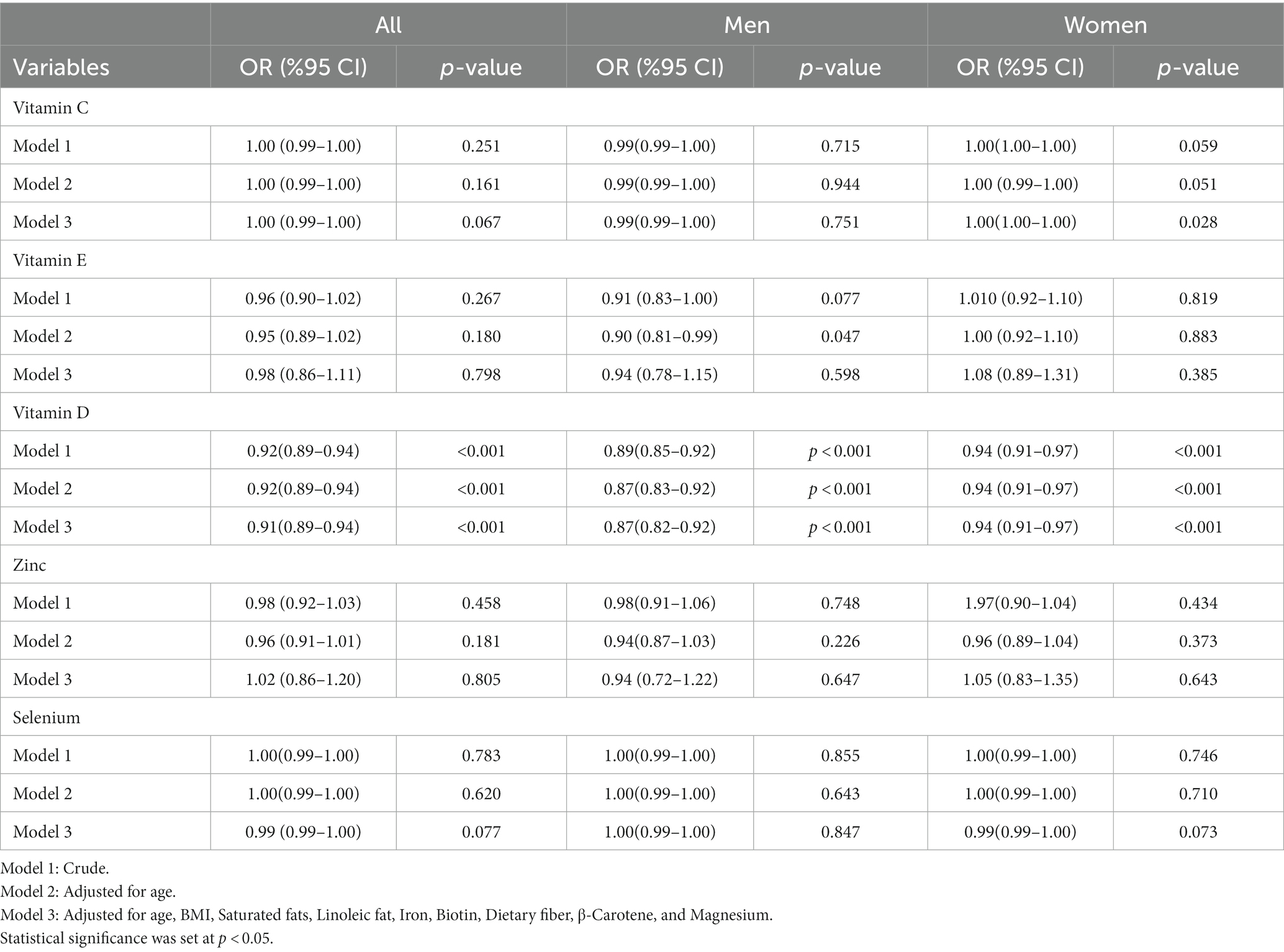
Table 3. Odds ratios (ORs) and 95% confidence intervals (CIs) for the association between dietary antioxidants and risk of COVID-19 severity multivariable model.
Outcomes from the logistic regression univariable model for the factors associated with COVID-19 severity are reported in Table 4. Our results suggested that age (as a factor) is positively associated with severity of COVID-19 infection (OR = 1.02; 95% CI: 1.01–1.04, p = 0.002). Moreover, vitamin D (OR = 0.92; 95% CI: 0.89–0.94, p < 0.001) and dietary fiber (OR = 0.98; 95% CI: 0.97–0.99, p = 0.034) intakes are associated with COVID-19 infection severity. The associations are not significant for other potential factors (p > 0.05).
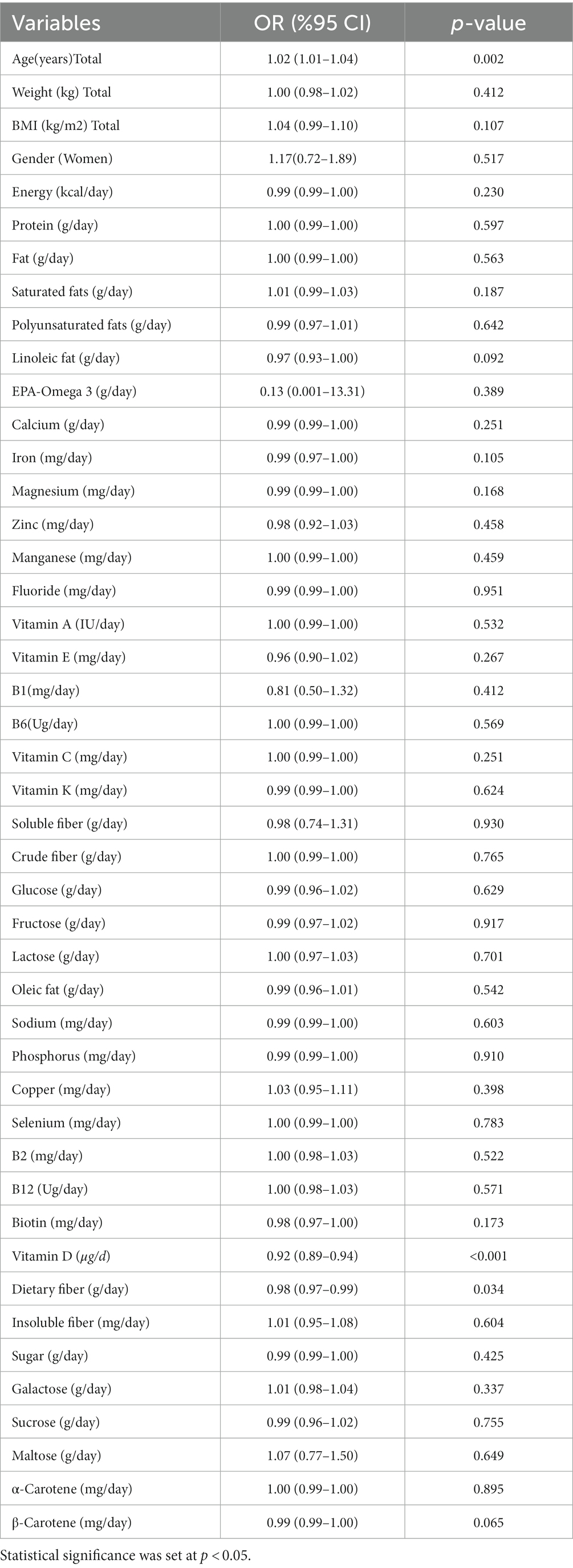
Table 4. Odds ratios (ORs) and 95% confidence intervals (CIs) for the factors associated with severity of COVID-19: results from logistic regression univariable model.
Results of the logistic regression multivariable model (T1 as reference group) analyses evaluating the association between DAQS and severity of COVID-19 infection are reported in Table 5. Our crude model outcomes suggest that increases in DAQS as a continuous variable are related to a significant decrease in the risk of COVID-19 infection severity (OR = 0.20; 95% CI: 0.10–0.38, p < 0.001). Sex subgroup analyses show consistent results in men (OR = 0.12; 95% CI: 0.03–0.36, p < 0.001) and women (OR = 0.28; 95% CI: 0.11–0.70, p = 0.005). In addition, the relationship remained significant even after multivariate adjustment (OR = 0.12; 95% CI: 0.04–0.29, p < 0.001). Moreover, the same results are observed in men (OR = 0.02; 95% CI: 0.002–0.15, p < 0.001) and women (OR = 0.21; 95% CI: 0.06–0.68, p = 0.012). Supplementary Material 3 provides detailed statistical calculations for all the reported results. Additionally, Supplementary Material 4 includes the original statistical analytical documents supporting the findings of this study.
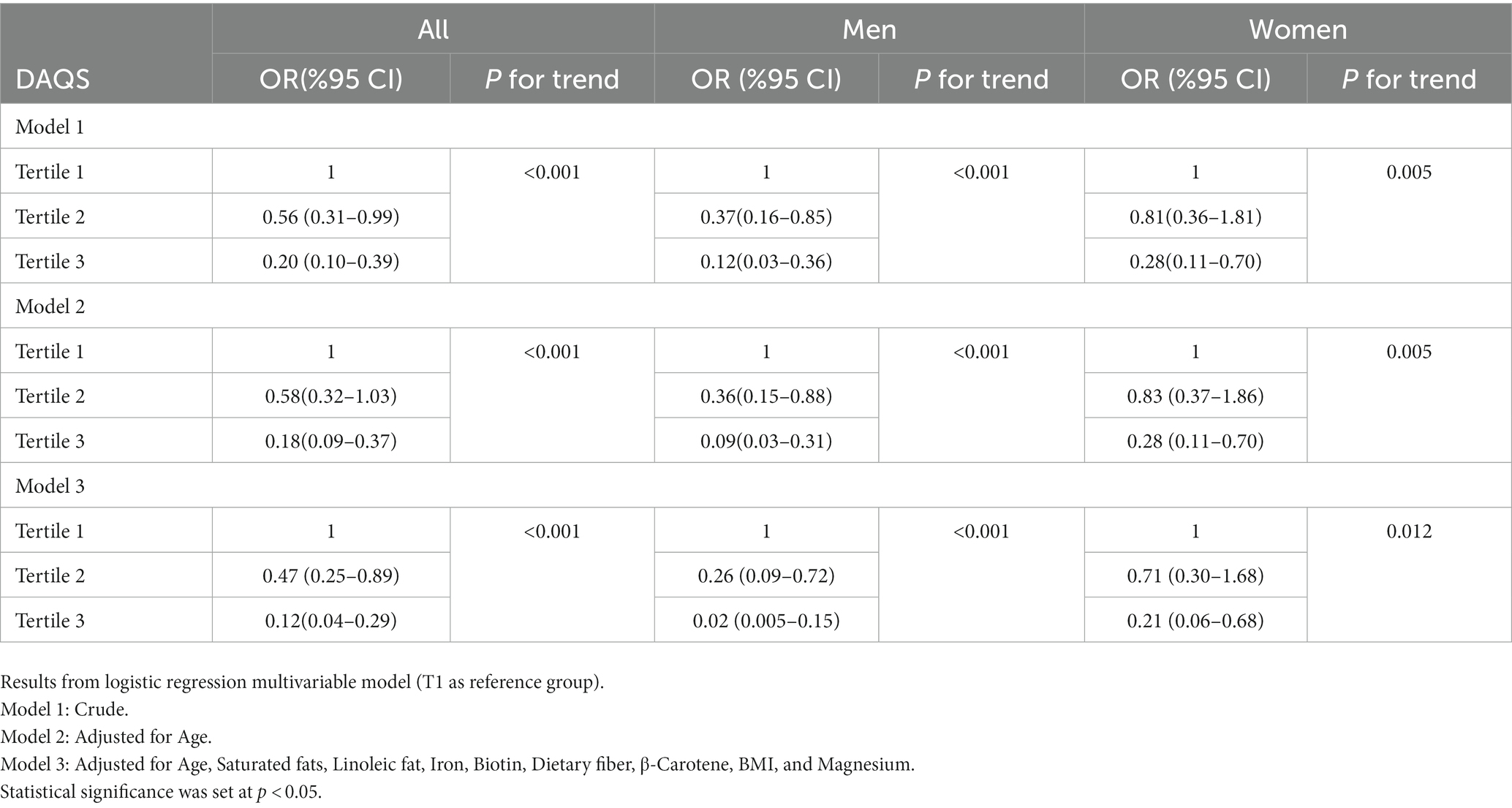
Table 5. Odds ratios (ORs) and 95% confidence intervals (CIs) for the association between Dietary Antioxidant Quality Score tertiles and COVID-19 severity.
Discussion
The aim of the present case–control study was to examine the association between DAQS and COVID-19 infection severity. Our results indicate a negative relationship between DAQS and the risk of COVID-19 infection severity. This relationship remained significant after adjusting for possible confounders, as well as in the subgroups of men and women. To our knowledge, this is the first study to examine the association between DAQS and COVID-19 outcomes.
Several investigations have reported an association between selected dietary antioxidants and COVID-19-related outcomes (29, 42–46). Vitamins A, B group, C, and D, as well as trace elements such as Zn, Se, Cu, and Iron, are listed by the European Food Safety Authority as micronutrients that can maintain immune system function (47). Furthermore, previous studies have highlighted the role of vitamin E, vitamin K, and Mg in the management of infectious respiratory disease (48–52). COVID-19, like other viral infections, can induce oxidative stress by enhancing reactive oxygen species (ROS) production and weakening defense mechanisms. Neutrophils and mononuclear phagocytic cells are also responsible for the widespread release of ROS into lung tissue (53). Additionally, the massive release of tumor necrosis factor-alpha (TNF-α) during the cytokine storm could exacerbate ROS production and, consequently, the severity of the COVID-19 infection (54, 55). The excessive formation of ROS could be controlled by appropriate antioxidant intake (43) as these play the role of free radical scavengers that can lower or terminate chain reactions (56). Therefore, due to the bold role of antioxidants in the pathogenesis of COVID-19, these compounds have been proposed as an adjuvant therapy in the management of this condition (43).
Our results revealed that vitamin D intake was negatively associated with the severity of COVID-19 infection. Similar outcomes were found in the analyses of the male and female subgroups. In agreement with our findings, a previous study in Iranian patients showed that vitamin D intake lower than 25 ng/ml was associated with COVID-19 infection severity (57). Dror et al. (58), in a case–control study, reported that levels of vitamin D in severe or critical disease were lower than those in patients with mild or moderate disease. Additionally, Sulli et al. (59) reported that the risk of death and severe COVID-19 infection was higher among elderly patients with insufficient 25-OH-vitamin D serum levels compared to patients with sufficient levels. While the previously mentioned studies were performed in adult cohorts, the association between serum vitamin D3 levels and clinical COVID-19 outcomes in children has been investigated in several studies (60, 61). For instance, Alpcan et al. (60) reported that there was a significant relationship between vitamin D levels and COVID-19 infection severity in children. Similarly, Yilmaz et al. (61) suggested that pediatric patients with COVID-19 had significantly lower vitamin D levels than control groups, along with a negative association between symptomatology and vitamin D serum levels. Vitamin D supplementation also appears to benefit COVID-19 outcomes in hospitalized patients. For example, Annweiler et al. (62) found that early administration of a single oral high dose of vitamin D3 (400,000 IU) versus the standard dose (50,000 IU) can improve overall mortality rates among at-risk older patients with COVID-19. Furthermore, Zurita-Cruz et al. (63) reported that vitamin D3 supplementation in COVID-19 pediatric hospitalized patients decreased disease severity and death. The inverse association between vitamin D and COVID-19 infection severity highlighted in this study, among many others, may be related to its role as a major immunologic mediator. Indeed, uncontrolled inflammation is the main factor in the severity of clinical COVID-19 outcomes such as ARDS, myocarditis, microvascular thrombosis, and/or the cytokine storm (64). Vitamin D supplementation can increase T regulatory lymphocyte (Tregs) levels, the principal defense mechanism against uncontrolled inflammation (64, 65). Additionally, vitamin D3 can control the cytokine storm by downregulating the release of inflammatory cytokines, such as TNF-alpha and Interleukin 6 (66). Some studies showed that higher levels of vitamin D were associated with lower levels of D-dimer and thrombotic complications (67, 68). Hence, vitamin D may regulate thrombotic pathways (69).
Another important outcome of this research is that low vitamin C intake was associated with an increased risk of COVID-19 infection severity in women, but no significant association was found in males. Previous studies have investigated the relationship between vitamin C and COVID-19 infection severity, but research findings are contradictory and controversial (70–72). Tomasa-Irriguible et al. (71) indicated that up to 82% of ICU patients had low plasma vitamin C values. Similarly, Chiscano-Camon et al. (70) reported that the plasma vitamin C levels in individuals with acute respiratory distress syndrome were very low. Additionally, Shahbaz et al. (72) reported that high doses of intravenous ascorbic acid may be beneficial for critically ill and older COVID-19-infected patients, by reducing inflammation and improving oxygenation status, leading to a decrease in mortality. However, a recent randomized controlled trial showed that treatment of severe COVID-19 with high-dose intravenous vitamin C did not improve outcomes in infected individuals compared to controls (73). The discrepant results on the effectiveness of ascorbic acid for the treatment of COVID-19 severity may be related to several factors such as dosage utilized, age, genetic background, and characteristics of infected participants. Nevertheless, vitamin C is well-known to play a major role in immune functions (72). It is a cofactor of enzymes such as dioxygenases and ketoglutarate, which can neutralize ROS and regenerate vitamin E. Moreover, vitamin C can deplete or block Neutrophil Extracellular Trap (NETosis) formation and control the cytokine storm in the alveolar region, which is relevant in COVID-19 (72). Additionally, vitamin C can block Interleukin-1 and TNF-mediated suppression of kappaBalpha (NFκB) activation. NFκB is a nuclear transcription factor and plays a central role in altered gene expression during inflammation (74) (Figure 1).
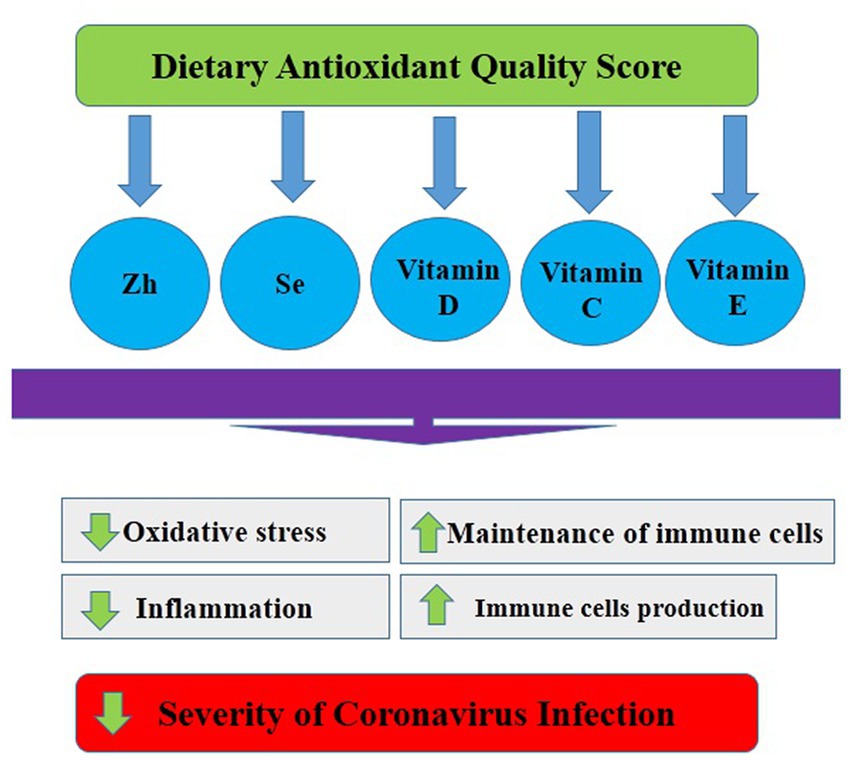
Figure 1. Possible mechanisms of the relationship between dietary antioxidant quality score and Covid-19.
We also found that the age of ICU patients was significantly higher than that of non-ICU patients, and age (as a factor) was positively associated with the severity of COVID-19 infection. In line with our results, previous studies found that the risk of severe COVID-19 infection was higher in elderly patients compared to young and middle-aged cohorts (75–78). These studies documented that DNA damage, telomere dysfunction, epigenetic disruption, mitogenic signaling, and oxidative stress increase with aging (75–78). Furthermore, inflammation, immunosenescence, cellular senescence, and immune cell dysfunction contribute to chronic inflammation with age, providing another possible link between aging and the severity of COVID-19 infection (78). Furthermore, declines in sex steroids and growth hormone levels during the aging process may impact immune regulation and, consequently, disease severity (77). Moreover, comorbidities such as hypertension, diabetes, coronary artery disease, chronic kidney disease, and hyperlipidemia are common in the elderly, and previous studies have shown that these comorbidities were predictors of the severity of COVID-19 infection (75). Thus, advanced age may be considered an important risk factor for the severity of COVID-19 infection.
Another outcome of the current research is that dietary fiber intake was inversely associated with the severity of COVID-19 infection. The dietary fiber is divided into two groups: water-soluble fiber and non-water-soluble fiber (79). Water-soluble fibers can be fermented by intestinal microorganisms and modulate intestinal microbiota (79). Moreover, many soluble fibers are prebiotics, and they can feed the bacteria that are beneficial for the digestive system (80). Prior findings by Shahramian et al. (81) supported the use of galactan and fructan as prebiotics in COVID-19 infant formula, as these reduced upper-respiratory infection. Additionally, Olaimat et al. (82) reported that COVID-19 patients have an imbalanced gut microbiota and that the non-digestible fibers in vegetables have an anti-inflammatory and antioxidant effect that can support microbiota-mediated antiviral immunity (83). Importantly, dietary fibers have a prebiotic effect on immunity by the growth and function of probiotics. For instance, fermentable probiotics including fructan, glucan, and arabinoxylan produce short-chain fatty acids (SCFAs). These metabolites can increase host immunity through improvement in specific receptor intensity, such as G protein-coupled receptor activation (84). Also, metabolites such as butyric acid and propionic acid have beneficial effects on T cells, macrophages, and dendritic cell function (85). In this study, levels of biomarkers such as hs-CRP, D-Dimer, LDH, CPK, and CKMB were higher in the ICU compared to non-ICU patients. In line with our results, Xu et al. (86) reported that D-Dimer levels in COVID-19 patients were associated with inflammatory factors and organ function. A body of knowledge also reported that CRP concentration (87), CK-MB (88), CK (89), and LDH (90) levels were higher in COVID-19 patients who died compared to survivors and that these biomarkers were predictors of COVID-19 infection severity.
The present study showed no significant association between Zn and Se intake and the severity of COVID-19 infection. Zinc, an essential trace metal, plays a crucial role in the development and maintenance of immune cells. Insufficient zinc levels may lead to impaired humoral function and cell-mediated immunity (91). Several studies have reported that zinc status can impact the outcome of COVID-19 infection, including disease duration and the risk of persistent respiratory infections with severe symptoms (92–94). Selenium, another vital trace element, is essential for mammalian redox biology (91). In the absence of adequate dietary selenium and in the presence of increased oxidative stress in the host, a viral genome can transform from a mildly pathogenic virus into a highly virulent agent. This phenomenon was observed with the Coxsackie 3B virus in Keshan disease in individuals deficient in selenium (91, 95). Moreover, it has been suggested that selenium deficiency may substantially contribute to the genesis of SARS-CoV (91, 96). Given the roles and importance of zinc and selenium in immune system function, their maximum impact is likely observed in patients with deficiencies in these minerals. Considering the lack of association between these elements and the severity of COVID-19 infection, the most plausible explanation could be that the patients had sufficient levels of these elements in their bodies.
Our findings also revealed no significant association between vitamin E intake and the severity of COVID-19 infection. However, there is limited information regarding the effects of vitamin E or selenium supplementation in humans with COVID-19 infection. Previous studies have suggested that vitamin E may impact respiratory tract infection severities through several possible mechanisms (97–99). Vitamin E acts through antioxidant mechanisms to initiate T-cell activation signals, increase the count of T cells, enhance mitogenic lymphocyte activities, enhance IL-2 cytokine secretion, enhance NK cell responses, and reduce the risk of infection (100). Despite these beneficial roles in immunity, a prior clinical trial reported that supplementation with 200 mg of vitamin E did not have a favorable effect on the incidence and severity of acute respiratory tract infections in well-nourished noninstitutionalized elderly individuals (97). Another study conducted by Meydani et al. (101) revealed that supplementation with 200 IU per day of vitamin E did not have a statistically significant effect on lower respiratory tract infections in elderly nursing home residents. Similarly, Hakamifard et al. (102) demonstrated that the combination of oral vitamins C (1,000 mg daily) and E (400 IU daily) supplementation had no beneficial effect in COVID-19 patients. However, it is worth noting that while vitamin E supplementation did not exhibit sharp efficacy in COVID-19 infection management, it was reported that administering 60 or 200 mg of vitamin E daily resulted in a higher humoral immune response to poliovirus and fewer mutant viral strains (101). Therefore, it is suggested that vitamin E supplementation may improve the efficacy of the COVID-19 vaccination (103), although it does not directly impact infection management.
Strengths and limitations
Our study has some strengths. To our knowledge, this was the first study to investigate the association between DAQS and COVID-19 severity. Indeed, this was also the first study in this research area assessing numerous factors and biomarkers. Nevertheless, this work is not without limitations. First, even though valid and widely used self-reported questionnaires were utilized for the assessment of dietary intake (40), this method might lead to the misclassification of food intake. Second, other confounding factors such as race/ethnicity or genetic background were not evaluated in this study. Lastly, we did not evaluate any mechanisms that may explain the relationship between DAQS and the severity of COVID-19. Therefore, to explain the possible mechanisms, future studies must assess biological biomarkers and factors associated with the severity of COVID-19.
Implications for practice and research
The outcomes of this cross-sectional study have important implications for both practice and future research. The study demonstrated a significant and negative relationship between DAQS and the risk of COVID-19 infection severity among adults. However, to establish a causal relationship between dietary DAQS score and COVID-19 infection severity, further investigation is required. Additionally, the use of antioxidant therapy shows promising potential as an adjuvant therapy in the prevention and management of infection severity in adults with COVID-19. However, more research is needed to validate these findings and determine the specific antioxidants that are most effective in this context. Antioxidants such as vitamin E, vitamin A, vitamin C, selenium, and zinc should be specifically investigated in future longitudinal studies and/or clinical trials to better understand their relationship with the risk of COVID-19 severity associated with DAQS score.
Conclusion
In summary, this study suggests that a lower age and higher intake of DAQS, vitamin D, and dietary fibers are associated with a lower risk of COVID-19 severity. Although we found no relationship between vitamin C intake and the risk of severe COVID-19 infection, subgroup analysis by sex showed a negative association in women. Future gender-specific studies should be conducted to provide detailed recommendations for each gender. Furthermore, clinical trial studies with larger sample sizes are needed to support the veracity of our findings.
Data availability statement
The original contributions presented in the study are included in the article/Supplementary material, further inquiries can be directed to the corresponding author.
Ethics statement
All participants signed an informed consent prior to partaking in this research. This work was approved by the local ethics committee of Mazandaran University (Ethic ID: IR.UMZ.REC.1401.001) and performed according to the Declaration of Helsinki. The patients/participants provided their written informed consent to participate in this study.
Author contributions
MoA and SH: concept and design, acquisition, analysis, interpretation of data, and statistical analysis. MoA, AW, MeA, and SH: drafting of the manuscript. SH: supervision. All authors contributed to critical revision of the manuscript for important intellectual content, and read and approved the final manuscript.
Conflict of interest
The authors declare that the research was conducted in the absence of any commercial or financial relationships that could be construed as a potential conflict of interest.
Publisher’s note
All claims expressed in this article are solely those of the authors and do not necessarily represent those of their affiliated organizations, or those of the publisher, the editors and the reviewers. Any product that may be evaluated in this article, or claim that may be made by its manufacturer, is not guaranteed or endorsed by the publisher.
Supplementary material
The Supplementary material for this article can be found online at: https://www.frontiersin.org/articles/10.3389/fnut.2023.1174113/full#supplementary-material
References
1. Fogleman, C, Cohen, D, Mercier, A, Farrell, D, Rutz, J, Bresz, K, et al. A pilot of a randomized control trial of melatonin and vitamin C for mild-to-moderate COVID-19. J Am Board Family Med. (2022) 35:695–707. doi: 10.3122/jabfm.2022.04.210529
2. Fajgenbaum, DC, and June, CH. Cytokine storm. N Engl J Med. (2020) 383:2255–73. doi: 10.1056/NEJMra2026131
3. Chernyak, BV, Popova, EN, Prikhodko, AS, Grebenchikov, OA, Zinovkina, LA, and Zinovkin, RA. COVID-19 and oxidative stress. Biochemistry (Mosc). (2020) 85:1543–53. doi: 10.1134/S0006297920120068
4. Dessie, ZG, and Zewotir, T. Mortality-related risk factors of COVID-19: a systematic review and meta-analysis of 42 studies and 423,117 patients. BMC Infect Dis. (2021) 21:1–28. doi: 10.1186/s12879-021-06536-3
5. Liguori, I, Russo, G, Curcio, F, Bulli, G, Aran, L, Della-Morte, D, et al. Oxidative stress, aging, and diseases. Clin Interv Aging. (2018) 13:757–72. doi: 10.2147/CIA.S158513
6. Rahman, I. The role of oxidative stress in the pathogenesis of COPD: implications for therapy. Treat Respir Med. (2005) 4:175–200. doi: 10.2165/00151829-200504030-00003
7. Saha, SK, Lee, SB, Won, J, Choi, HY, Kim, K, Yang, GM, et al. Correlation between oxidative stress, nutrition, and cancer initiation. Int J Mol Sci. (2017) 18. doi: 10.3390/ijms18071544
8. Huang, Q, Wu, X, Zheng, X, Luo, S, Xu, S, and Weng, J. Targeting inflammation and cytokine storm in COVID-19. Pharmacol Res. (2020) 159:105051. doi: 10.1016/j.phrs.2020.105051
9. Ntyonga-Pono, M-P. COVID-19 infection and oxidative stress: an under-explored approach for prevention and treatment? Pan Afr Med J. (2020) 35. doi: 10.11604/pamj.supp.2020.35.2.22877
10. Muhammad, Y, Kani, YA, Iliya, S, Muhammad, JB, Binji, A, El-Fulaty Ahmad, A, et al. Deficiency of antioxidants and increased oxidative stress in COVID-19 patients: a cross-sectional comparative study in Jigawa, Northwestern Nigeria. SAGE Open Med. (2021) 9. doi: 10.1177/2050312121991246
11. Singh, B, Singh, S, Bhatia, J, Kapoor, R, and Bhatia, K. Role of matrix degradation, oxidative stress, inflammation & trace elements in COVID-19 patients: a multivariate study from India. Indian J Clin Biochem. (2022) 38:193–203. doi: 10.1007/s12291-022-01059-3
12. Rabie, AM, and Eltayb, WA. Strong dual antipolymerase/antiexonuclease actions of some aminothiadiazole antioxidants: a promising in-silico/in-vitro repurposing research study against the COVID-19 omicron virus (B. 1.1. 529.3 lineage). Adv Redox Res. (2023):100064. doi: 10.1016/j.arres.2023.100064
13. Rabie, AM. Teriflunomide: a possible effective drug for the comprehensive treatment of COVID-19. Curr Res Pharmacol Drug Discov. (2021) 2:100055. doi: 10.1016/j.crphar.2021.100055
14. Rabie, AM. Two antioxidant 2, 5-disubstituted-1, 3, 4-oxadiazoles (CoViTris2020 and ChloViD2020): successful repurposing against COVID-19 as the first potent multitarget anti-SARS-CoV-2 drugs. New J Chem. (2021) 45:761–71. doi: 10.1039/D0NJ03708G
15. Rabie, AM. Potent toxic effects of Taroxaz-104 on the replication of SARS-CoV-2 particles. Chem Biol Interact. (2021) 343:109480. doi: 10.1016/j.cbi.2021.109480
16. Rabie, AM. Potent inhibitory activities of the adenosine analogue cordycepin on SARS-CoV-2 replication. ACS Omega. (2022) 7:2960–9. doi: 10.1021/acsomega.1c05998
17. Rabie, AM, Tantawy, AS, and Badr, SM. Design, synthesis, and biological evaluation of novel 5-substituted-2-(3, 4, 5-trihydroxyphenyl)-1, 3, 4-oxadiazoles as potent antioxidants. Am J Org Chem. (2016) 6:54–80. doi: 10.5923/j.ajoc.20160602.02
18. Rabie, A, Tantawy, A, and Badr, S. Design, synthesis, and biological evaluation of new 5-substituted-1, 3, 4-thiadiazole-2-thiols as potent antioxidants. Eur Res. (2018) 10:21–43.
19. Delgado-Roche, L, and Mesta, F. Oxidative stress as key player in severe acute respiratory syndrome coronavirus (SARS-CoV) infection. Arch Med Res. (2020) 51:384–7. doi: 10.1016/j.arcmed.2020.04.019
20. Santos, HO. Therapeutic supplementation with zinc in the management of COVID-19–related diarrhea and ageusia/dysgeusia: mechanisms and clues for a personalized dosage regimen. Nutr Rev. (2022) 80:1086–93. doi: 10.1093/nutrit/nuab054
21. da Costa, BT, Araújo, GRL, da Silva Júnior, RT, de Sá Santos, LK, de Souza Gonçalves, VL, Lima, DBA, et al. Effects of nutrients on immunomodulation in patients with severe COVID-19: current knowledge. World J Crit Care Med. (2022) 11:201–18. doi: 10.5492/wjccm.v11.i4.201
22. Martinez, SS, Huang, Y, Acuna, L, Laverde, E, Trujillo, D, Barbieri, MA, et al. Role of selenium in viral infections with a major focus on SARS-CoV-2. Int J Mol Sci. (2021) 23:280. doi: 10.3390/ijms23010280
23. Majidi, N, Rabbani, F, Gholami, S, Gholamalizadeh, M, BourBour, F, Rastgoo, S, et al. The effect of Vitamin C on pathological parameters and survival duration of critically ill coronavirus disease 2019 patients: a randomized clinical trial. Front Immunol. 12:717816. doi: 10.3389/fimmu.2021.717816
24. Al Sulaiman, K, Aljuhani, O, Saleh, KB, Badreldin, HA, Al Harthi, A, Alenazi, M, et al. Ascorbic acid as an adjunctive therapy in critically ill patients with COVID-19: a propensity score matched study. Sci Rep. (2021) 11:1–8.
25. Zhang, J, Rao, X, Li, Y, Zhu, Y, Liu, F, Guo, G, et al. Pilot trial of high-dose vitamin C in critically ill COVID-19 patients. Ann Intensive Care. (2021) 11:5–12. doi: 10.1186/s13613-020-00792-3
26. Bousquet, J, Anto, JM, Iaccarino, G, Czarlewski, W, Haahtela, T, Anto, A, et al. Is diet partly responsible for differences in COVID-19 death rates between and within countries? Clin Transl Allergy. (2020) 10:16. doi: 10.1186/s13601-020-00323-0
27. Mohajeri, M, Mohajery, R, Nemati, A, and Pourfarzi, F. The difference in the dietary inflammatory index, functional food, and antioxidants intake between COVID-19 patients and healthy persons. Mediterr J Nutr Metab. (2022) 15:219–27.
28. Ashaolu, TJ. Immune boosting functional foods and their mechanisms: a critical evaluation of probiotics and prebiotics. Biomed Pharmacother. (2020) 130:110625. doi: 10.1016/j.biopha.2020.110625
29. Mrityunjaya, M, Pavithra, V, Neelam, R, Janhavi, P, Halami, P, and Ravindra, P. Immune-boosting, antioxidant and anti-inflammatory food supplements targeting pathogenesis of COVID-19. Front Immunol. (2020) 11:570122. doi: 10.3389/fimmu.2020.570122
30. Ebrahimzadeh, A, Taghizadeh, M, and Milajerdi, A. Major dietary patterns in relation to disease severity, symptoms, and inflammatory markers in patients recovered from COVID-19. Front Nutr. (2022) 9:9. doi: 10.3389/fnut.2022.929384
31. Zargarzadeh, N, Vajargah, KT, Ebrahimzadeh, A, Mousavi, SM, Khodaveisi, H, Akhgarjand, C, et al. Higher adherence to the Mediterranean dietary pattern is inversely associated with severity of COVID-19 and related symptoms: a Cross-Sectional Study. Front Med. (2022) 9:9. doi: 10.3389/fmed.2022.911273
32. Merino, J, Joshi, AD, Nguyen, LH, Leeming, ER, Mazidi, M, Drew, DA, et al. Diet quality and risk and severity of COVID-19: a prospective cohort study. Gut. (2021) 70:2096–104. doi: 10.1136/gutjnl-2021-325353
33. El Ansari, W, Adetunji, H, and Oskrochi, R. Food and mental health: relationship between food and perceived stress and depressive symptoms among university students in the United Kingdom. Cent Eur J Public Health. (2014) 22:90–7. doi: 10.21101/cejph.a3941
34. Shahinfar, H, Shahavandi, M, Jibril, AT, Djafarian, K, Clark, CC, and Shab-Bidar, S. The association between dietary antioxidant quality score and cardiorespiratory fitness in Iranian adults: a cross-sectional study. Clin Nutr Res. (2020) 9:171–81. doi: 10.7762/cnr.2020.9.3.171
35. Wang, W, Wang, X, Cao, S, Duan, Y, Xu, C, Gan, D, et al. Dietary antioxidant indices in relation to all-cause and cause-specific mortality among adults with diabetes: a prospective cohort study. Front Nutr. (2022) 9:849727. doi: 10.3389/fnut.2022.849727
36. Shahavandi, M, Shahinfar, H, Payande, N, Sheikhhossein, F, Djafarian, K, and Shab-Bidar, S. The association between dietary antioxidant quality score with metabolic syndrome and its components in Iranian adults: a cross-sectional study. Food Sci Nutr. (2021) 9:994–1002. doi: 10.1002/fsn3.2067
37. Islami, A, Mahmoud, A, Khabiri, M, and Najafian, R. The role of socio-economic (SES) in motivating citizen participation in sport recreational. J Appl Res Sport Manag. (2013) 2:104–89.
38. El Mouzan, MI, Al Mofarreh, MA, Al Sarkhy, AA, Assiri, AM, and Hamed, YM. Pre-illness diet as risk factor in pediatric inflammatory bowel disease in Saudi Arabia. Saudi J Gastroenterol. (2017) 23:287–90. doi: 10.4103/sjg.SJG_619_16
39. Lee, PH, Macfarlane, DJ, Lam, TH, and Stewart, SM. Validity of the international physical activity questionnaire short form (IPAQ-SF): a systematic review. Int J Behav Nutr Phys Act. (2011) 8:1–11. doi: 10.1186/1479-5868-8-115
40. Asghari, G, Rezazadeh, A, Hosseini-Esfahani, F, Mehrabi, Y, Mirmiran, P, and Azizi, F. Reliability, comparative validity and stability of dietary patterns derived from an FFQ in the Tehran Lipid and Glucose Study. Br J Nutr. (2012) 108:1109–17. doi: 10.1017/S0007114511006313
41. Tur, JA, Serra-Majem, L, Romaguera, D, and Pons, A. Does the diet of the Balearic population, a Mediterranean type diet, still provide adequate antioxidant nutrient intakes? Eur J Nutr. (2005) 44:204–13. doi: 10.1007/s00394-004-0512-0
42. Lammi, C, and Arnoldi, A. Food-derived antioxidants and COVID-19. J Food Biochem. (2021) 45:e13557. doi: 10.1111/jfbc.13557
43. de Flora, S, Balansky, R, and La Maestra, S. Antioxidants and COVID-19. J Prev Med Hyg. (2021) 34:13185–93. doi: 10.1096/fj.202001807
44. Siddique, F, Abbas, RZ, Mansoor, MK, Alghamdi, ES, Saeed, M, Ayaz, MM, et al. An insight into COVID-19: a 21st century disaster and its relation to immunocompetence and food antioxidants. Front Vet Sci. (2021) 7:586637. doi: 10.3389/fvets.2020.586637
45. de Faria, C-RC, Corgosinho, FC, Sanches, FLFZ, Prado, CMM, Laviano, A, and Mota, JF. Dietary recommendations during the COVID-19 pandemic. Nutr Rev. (2021) 79:382–93. doi: 10.1093/nutrit/nuaa067
46. Cobre, AF, Surek, M, Vilhena, RO, Böger, B, Fachi, MM, Momade, DR, et al. Influence of foods and nutrients on COVID-19 recovery: a multivariate analysis of data from 170 countries using a generalized linear model. Clin Nutr. (2021) 41:3077–84. doi: 10.1016/j.clnu.2021.03.018
47. Galmés, S, Serra, F, and Palou, A. Current state of evidence: influence of nutritional and nutrigenetic factors on immunity in the COVID-19 pandemic framework. Nutrients. (2020) 12:2738. doi: 10.3390/nu12092738
48. Dharmalingam, K, Birdi, A, Tomo, S, Sreenivasulu, K, Charan, J, Yadav, D, et al. Trace elements as immunoregulators in SARS-CoV-2 and other viral infections. Indian J Clin Biochem. (2021) 36:416–26. doi: 10.1007/s12291-021-00961-6
49. Dofferhoff, AS, Piscaer, I, Schurgers, LJ, Visser, MP, van den Ouweland, JM, de Jong, PA, et al. Reduced vitamin K status as a potentially modifiable risk factor of severe coronavirus disease 2019. Clin Infect Dis. (2021) 73:e4039–46. doi: 10.1093/cid/ciaa1258
50. Jovic, TH, Ali, SR, Ibrahim, N, Jessop, ZM, Tarassoli, SP, Dobbs, TD, et al. Could vitamins help in the fight against COVID-19? Nutrients. (2020) 12:2550. doi: 10.3390/nu12092550
51. Linneberg, A, Kampmann, FB, Israelsen, SB, Andersen, LR, Jørgensen, HL, Sandholt, H, et al. The association of low vitamin K status with mortality in a cohort of 138 hospitalized patients with COVID-19. Nutrients. (2021) 13:1985. doi: 10.3390/nu13061985
52. Pecora, F, Persico, F, Argentiero, A, Neglia, C, and Esposito, S. The role of micronutrients in support of the immune response against viral infections. Nutrients. (2020) 12:3198. doi: 10.3390/nu12103198
53. Schönrich, G, Raftery, MJ, and Samstag, Y. Devilishly radical NETwork in COVID-19: oxidative stress, neutrophil extracellular traps (NETs), and T cell suppression. Adv Biol Regulat. (2020) 77:100741. doi: 10.1016/j.jbior.2020.100741
54. Blaser, H, Dostert, C, Mak, TW, and Brenner, D. TNF and ROS crosstalk in inflammation. Trends Cell Biol. (2016) 26:249–61. doi: 10.1016/j.tcb.2015.12.002
55. PryorWA, PD, and Church, D. Electron-spinresonance study ofmainstream and sidestream cigarette smoke: nature ofthe free radicals in gas-phase smoke and cigarette tar. Environ Health Perspect. (1983) 47:345–55. doi: 10.1289/ehp.8347345
56. Duarte, TL, and Lunec, J. When is an antioxidant not an antioxidant? A review of novel actions and reactions of vitamin C. Free Radic Res. (2005) 39:671–86. doi: 10.1080/10715760500104025
57. Asgari, A, Hazrati, E, Soleiman-Meigooni, S, Rajaeinejad, M, and Nasiri, M. Vitamin D insufficiency in disease severity and prognosis of the patients with SARS Corona Virus-2 infection. Acta Med Iran. (2021) 59:662–8. doi: 10.18502/acta.v59i11.7779
58. Dror, AA, Morozov, N, Daoud, A, Namir, Y, Yakir, O, Shachar, Y, et al. Pre-infection 25-hydroxyvitamin D3 levels and association with severity of COVID-19 illness. PLoS One. (2022) 17:e0263069. doi: 10.1371/journal.pone.0263069
59. Sulli, A, Gotelli, E, Casabella, A, Paolino, S, Pizzorni, C, Alessandri, E, et al. Vitamin D and lung outcomes in elderly COVID-19 patients. Nutrients. (2021) 13. doi: 10.3390/nu13030717
60. Alpcan, A, Tursun, S, and Kandur, Y. Vitamin D levels in children with COVID-19: a report from Turkey. Epidemiol Infect. (2021) 149:e180. doi: 10.1017/S0950268821001825
61. Yılmaz, K, and Şen, V. Is vitamin D deficiency a risk factor for COVID-19 in children? Pediatr Pulmonol. (2020) 55:3595–601. doi: 10.1002/ppul.25106
62. Annweiler, C, Beaudenon, M, Gautier, J, Gonsard, J, Boucher, S, Chapelet, G, et al. High-dose versus standard-dose vitamin D supplementation in older adults with COVID-19 (COVIT-TRIAL): a multicenter, open-label, randomized controlled superiority trial. PLoS Med. (2022) 19:e1003999. doi: 10.1371/journal.pmed.1003999
63. Zurita-Cruz, J, Fonseca-Tenorio, J, Villasís-Keever, M, López-Alarcón, M, Parra-Ortega, I, López-Martínez, B, et al. Efficacy and safety of vitamin D supplementation in hospitalized COVID-19 pediatric patients: a randomized control trial. Frontiers. Pediatrics. (2022) 10:1181. doi: 10.3389/fped.2022.943529
64. Weir, EK, Thenappan, T, Bhargava, M, and Chen, Y. Does vitamin D deficiency increase the severity of COVID-19? Clin Med. (2020) 20:e107:–e108. doi: 10.7861/clinmed.2020-0301
65. Fisher, SA, Rahimzadeh, M, Brierley, C, Gration, B, Doree, C, Kimber, CE, et al. The role of vitamin D in increasing circulating T regulatory cell numbers and modulating T regulatory cell phenotypes in patients with inflammatory disease or in healthy volunteers: a systematic review. PLoS One. (2019) 14:e0222313. doi: 10.1371/journal.pone.0222313
66. Alhassan Mohammed, H, Mirshafiey, A, Vahedi, H, Hemmasi, G, Moussavi Nasl Khameneh, A, Parastouei, K, et al. Immunoregulation of inflammatory and inhibitory cytokines by vitamin D 3 in patients with inflammatory bowel diseases. Scand J Immunol. (2017) 85:386–94. doi: 10.1111/sji.12547
67. Ates, B, Sazak, S, Turkmenoglu, Y, Irdem, A, and Dursun, H. Relationship of serum vitamin D, D-dimer and uric acid levels with attacks in children with familial Mediterranean fever. Egypt Rheumatol. (2022) 44:301–5. doi: 10.1016/j.ejr.2022.03.004
68. Lu, C, Zanker, D, Lock, P, Jiang, X, Deng, J, Duan, M, et al. Memory regulatory T cells home to the lung and control influenza A virus infection. Immunol Cell Biol. (2019) 97:774–86. doi: 10.1111/imcb.12271
69. Mohammad, S, Mishra, A, and Ashraf, MZ. Emerging role of vitamin D and its associated molecules in pathways related to pathogenesis of thrombosis. Biomol Ther. (2019) 9:649. doi: 10.3390/biom9110649
70. Chiscano-Camón, L, Ruiz-Rodriguez, JC, Ruiz-Sanmartin, A, Roca, O, and Ferrer, R. Vitamin C levels in patients with SARS-CoV-2-associated acute respiratory distress syndrome. Crit Care. (2020) 24:1–3. doi: 10.1186/s13054-020-03249-y
71. Tomasa-Irriguible, TM, and Bielsa-Berrocal, L. COVID-19: up to 82% critically ill patients had low vitamin C values. Nutr J. (2021) 20:1–3. doi: 10.1186/s12937-021-00727-z
72. Shahbaz, U, Fatima, N, Basharat, S, Bibi, A, Yu, X, Hussain, MI, et al. Role of vitamin C in preventing of COVID-19 infection, progression and severity. AIMS Microbiol. (2022) 8:108–24. doi: 10.3934/microbiol.2022010
73. JamaliMoghadamSiahkali, S, Zarezade, B, Koolaji, S, SeyedAlinaghi, S, Zendehdel, A, Tabarestani, M, et al. Safety and effectiveness of high-dose vitamin C in patients with COVID-19: a randomized open-label clinical trial. Eur J Med Res. (2021) 26:1–9. doi: 10.1186/s40001-021-00490-1
74. Bowie, AG, and O’Neill, LA. Vitamin C inhibits NF-κB activation by TNF via the activation of p38 mitogen-activated protein kinase. J Immunol. (2000) 165:7180–8. doi: 10.4049/jimmunol.165.12.7180
75. Statsenko, Y, Al Zahmi, F, Habuza, T, Almansoori, TM, Smetanina, D, Simiyu, GL, et al. Impact of age and sex on COVID-19 severity assessed from radiologic and clinical findings. Front Cell Infect Microbiol. (2022) 11:1395. doi: 10.3389/fcimb.2021.777070
76. Neves, MT, de Matos, LV, Vasques, AC, Sousa, IE, Ferreira, I, Peres, S, et al. COVID-19 and aging: identifying measures of severity. SAGE Open Med. (2021) 9:1–6. doi: 10.1177/20503121211027462
77. Farshbafnadi, M, Zonouzi, SK, Sabahi, M, Dolatshahi, M, and Aarabi, MH. Aging & COVID-19 susceptibility, disease severity, and clinical outcomes: the role of entangled risk factors. Exp Gerontol. (2021) 154:111507. doi: 10.1016/j.exger.2021.111507
78. Bartleson, JM, Radenkovic, D, Covarrubias, AJ, Furman, D, Winer, DA, and Verdin, E. SARS-CoV-2, COVID-19 and the aging immune system. Nat Aging. (2021) 1:769–82. doi: 10.1038/s43587-021-00114-7
79. Aleixandre, A, and Miguel, M. Dietary fiber and blood pressure control. Food Funct. (2016) 7:1864–71. doi: 10.1039/C5FO00950B
80. Ghavami, A, Ziaei, R, Talebi, S, Barghchi, H, Nattagh-Eshtivani, E, Moradi, S, et al. Soluble Fiber Supplementation and Serum Lipid Profile: A Systematic Review and Dose-Response Meta-Analysis of Randomized Controlled Trials. Adv Nutr. (2022) 14:465–74. doi: 10.1016/j.advnut.2023.01.005
81. Shahramian, I, Kalvandi, G, Javaherizadeh, H, Khalili, M, Noori, NM, Delaramnasab, M, et al. The effects of prebiotic supplementation on weight gain, diarrhoea, constipation, fever and respiratory tract infections in the first year of life. J Paediatr Child Health. (2018) 54:875–80. doi: 10.1111/jpc.13906
82. Kim, H, Rebholz, CM, Hegde, S, LaFiura, C, Raghavan, M, Lloyd, JF, et al. Plant-based diets, pescatarian diets and COVID-19 severity: a population-based case-control study in six countries. BMJ Nutr Prev Health. (2021) 4:257–66. doi: 10.1136/bmjnph-2021-000272
83. Olaimat, AN, Aolymat, I, Al-Holy, M, Ayyash, M, Abu Ghoush, M, Al-Nabulsi, AA, et al. The potential application of probiotics and prebiotics for the prevention and treatment of COVID-19. NPJ Sci Food. (2020) 4:1–7. doi: 10.1038/s41538-020-00078-9
84. Trompette, A, Gollwitzer, ES, Yadava, K, Sichelstiel, AK, Sprenger, N, Ngom-Bru, C, et al. Gut microbiota metabolism of dietary fiber influences allergic airway disease and hematopoiesis. Nat Med. (2014) 20:159–66. doi: 10.1038/nm.3444
85. Sanchez, HN, Moroney, JB, Gan, H, Shen, T, Im, JL, Li, T, et al. B cell-intrinsic epigenetic modulation of antibody responses by dietary fiber-derived short-chain fatty acids. Nat Commun. (2020) 11:60. doi: 10.1038/s41467-019-13603-6
86. Xu, Y, Qian, Y, Gu, Q, and Tang, J. Relationship between D-dimer concentration and inflammatory factors or organ function in patients with coronavirus disease 2019. Zhonghua Wei Zhong Bing Ji Jiu Yi Xue. (2020) 32:559–63. doi: 10.3760/cma.j.cn121430-20200414-00518
87. Ali, AM, Rostam, HM, Fatah, MH, Noori, CM, Ali, KM, and Tawfeeq, HM. Serum troponin, D-dimer, and CRP level in severe coronavirus (COVID-19) patients. Immun Inflamm Dis. (2022) 10:e582. doi: 10.1002/iid3.582
88. Zinellu, A, Sotgia, S, Fois, AG, and Mangoni, AA. Serum CK-MB, COVID-19 severity and mortality: an updated systematic review and meta-analysis with meta-regression. Adv Med Sci. (2021) 66:304–14. doi: 10.1016/j.advms.2021.07.001
89. Akbar, MR, Pranata, R, Wibowo, A, Lim, MA, Sihite, TA, and Martha, JW. The prognostic value of elevated creatine kinase to predict poor outcome in patients with COVID-19 – a systematic review and meta-analysis. Diabetes Metab Syndr. (2021) 15:529–34. doi: 10.1016/j.dsx.2021.02.012
90. Bartziokas, K, and Kostikas, K. Lactate dehydrogenase, COVID-19 and mortality. Med Clin. (2021) 156:37. doi: 10.1016/j.medcle.2020.07.017
91. Alexander, J, Tinkov, A, Strand, TA, Alehagen, U, Skalny, A, and Aaseth, J. Early nutritional interventions with zinc, selenium and vitamin D for raising anti-viral resistance against progressive COVID-19. Nutrients. (2020) 12:2358. doi: 10.3390/nu12082358
92. Finzi, E. Treatment of SARS-CoV-2 with high dose oral zinc salts: a report on four patients. Int J Infect Dis. (2020) 99:307–9. doi: 10.1016/j.ijid.2020.06.006
93. Sattar, Y, Connerney, M, Rauf, H, Saini, M, Ullah, W, Mamtani, S, et al. Three cases of COVID-19 disease with colonic manifestations. Am J Gastroenterol. (2020) 115:948–50. doi: 10.14309/ajg.0000000000000692
94. Te Velthuis, AJ, van den Worm, SH, Sims, AC, Baric, RS, Snijder, EJ, and van Hemert, MJ. Zn2+ inhibits coronavirus and arterivirus RNA polymerase activity in vitro and zinc ionophores block the replication of these viruses in cell culture. PLoS Pathog. (2010) 6:e1001176. doi: 10.1371/journal.ppat.1001176
95. Guillin, OM, Vindry, C, Ohlmann, T, and Chavatte, L. Selenium, selenoproteins and viral infection. Nutrients. (2019) 11:2101. doi: 10.3390/nu11092101
96. Harthill, M. Micronutrient selenium deficiency influences evolution of some viral infectious diseases. Biol Trace Elem Res. (2011) 143:1325–36. doi: 10.1007/s12011-011-8977-1
97. Graat, JM, Schouten, EG, and Kok, FJ. Effect of daily vitamin E and multivitamin-mineral supplementation on acute respiratory tract infections in elderly persons: a randomized controlled trial. JAMA. (2002) 288:715–21. doi: 10.1001/jama.288.6.715
98. Shakoor, H, Feehan, J, Al Dhaheri, AS, Ali, HI, Platat, C, Ismail, LC, et al. Immune-boosting role of vitamins D, C, E, zinc, selenium and omega-3 fatty acids: could they help against COVID-19? Maturitas. (2021) 143:1–9. doi: 10.1016/j.maturitas.2020.08.003
99. Tavakol, S, and Seifalian, AM. Vitamin E at a high dose as an anti-ferroptosis drug and not just a supplement for COVID-19 treatment. Biotechnol Appl Biochem. (2021) 69:1058–60. doi: 10.1002/bab.2176
100. Iddir, M, Brito, A, Dingeo, G, Fernandez Del Campo, SS, Samouda, H, La Frano, MR, et al. Strengthening the immune system and reducing inflammation and oxidative stress through diet and nutrition: considerations during the COVID-19 crisis. Nutrients. (2020) 12:1562. doi: 10.3390/nu12061562
101. Meydani, SN, Leka, LS, Fine, BC, Dallal, GE, Keusch, GT, Singh, MF, et al. Vitamin E and respiratory tract infections in elderly nursing home residents: a randomized controlled trial. JAMA. (2004) 292:828–36. doi: 10.1001/jama.292.7.828
102. Hakamifard, A, Soltani, R, Maghsoudi, A, Rismanbaf, A, Aalinezhad, M, Tarrahi, MJ, et al. The effect of vitamin E and vitamin C in patients with COVID-19 pneumonia; a randomized controlled clinical trial. Immunopathol Persa. (2021) 8:e8–e.
103. Daei Sorkhabi, A, Sarkesh, A, Daei Sorkhabi, A, Entezari-Maleki, T, Rashedi, J, and Bannazadeh, BH. Vitamin supplementation as a potential adjunctive therapeutic approach for COVID-19: biological and clinical plausibility. J Basic Clin Physiol Pharmacol. (2021) 33:55–77. doi: 10.1515/jbcpp-2021-0111
Keywords: DAQS, COVID-19, antioxidant, diet, ICU
Citation: Aghajani M, Wong A, Azimi M and Harijani SM (2023) Association between dietary antioxidant quality score and severity of coronavirus infection: a case–control study. Front. Nutr. 10:1174113. doi: 10.3389/fnut.2023.1174113
Edited by:
Germano Guerra, University of Molise, ItalyReviewed by:
Emmy Hainida Khairul Ikram, Universiti Teknologi MARA Puncak Alam, MalaysiaAmgad M. Rabie, Ain Shams University, Egypt
Copyright © 2023 Aghajani, Wong, Azimi and Harijani. This is an open-access article distributed under the terms of the Creative Commons Attribution License (CC BY). The use, distribution or reproduction in other forums is permitted, provided the original author(s) and the copyright owner(s) are credited and that the original publication in this journal is cited, in accordance with accepted academic practice. No use, distribution or reproduction is permitted which does not comply with these terms.
*Correspondence: Shadmehr Mirdar Harijani, c2hhZG1laHIubWlyZGFyQGdtYWlsLmNvbQ==