- 1Department of Physiology, Anatomy and Cellular Biology, University of Pablo de Olavide, Seville, Spain
- 2Department of Physical Education and Sports, University of Seville, Seville, Spain
- 3Department of Didactics of Languages, Arts and Sport, University of Malaga, Andalucía-Tech, Instituto de Investigación Biomédica de Málaga (IBIMA), Malaga, Spain
Purpose: Numerous articles have recently studied the involvement of the gut microbiota in neurological diseases. Aging is associated with changes in the microbiome, which implies a reduction in microbial biodiversity among other changes. Considering that the consumption of a fermented-food diet improves intestinal permeability and barrier function, it seems of interest to study its participation in the prevention of neurodegenerative diseases. This article reviews existing studies to establish whether the consumption of fermented foods and fermented beverages prevents or ameliorates neurodegenerative decline in old age.
Methods: The protocol used was performed according to the Preferred Reporting Items for Systematic Reviews and Meta-Analyses (PRISMA) guidelines. Details of the protocol for this systematic review are registered on PROSPERO (CRD42021250921).
Results: Out of 465 articles identified in the Pubmed, Scopus, and Cochrane Library databases, a total of 29 that examined the relationship of the consumption of fermented products with cognitive impairment in old people were selected (22 cohort, 4 case-control, and 3 cross-sectional studies). The results suggest that low-to-moderate alcohol consumption and daily intake of coffee, soy products, and fermented-food diets in general are associated with a lower risk of dementia and Alzheimer's disease.
Conclusion: Daily consumption of fermented foods and beverages, either alone or as part of a diet, has neuroprotective effects and slows cognitive decline in old people.
Systematic review registration: https://www.crd.york.ac.uk/prospero/display_record.php?RecordID=250921, identifier: CRD42021250921.
1. Introduction
A healthy gut microbiota is responsible for synthesizing vitamins and essential amino acids and has an important role in the structural integrity of the intestinal mucosa producing neuromodulators, including bacteria-derived choline, tryptophan, intestinal-released hormones–such as ghrelin or leptin–and short-chain fatty acids (SCFA)–such as butyrate, acetate, and propionate. These metabolites are an energy and trophic-factors source for the intestinal epithelial cells, and thus strengthen the mucosal barrier (1–4), but are also crucial in the regulation of regulatory T-cell (Treg) colonies, supporting the hypothesis of their action in the brain (2, 5, 6).
Recently, the role of the gut microbiota in the central nervous system, through the microbiota gut-brain axis, has been revealed (2, 3, 5–21). Neurons in the enteric nervous system interact directly with neurochemicals produced by the gut microbiota, thus influencing signaling to the central nervous system (22).
Dysbiosis and some microbial metabolites are involved in a variety of diseases, such as inflammatory bowel disease, type 1 and type 2 diabetes mellitus, fatty liver, metabolic syndrome, obesity, cardiovascular disease, colorectal and breast cancer, hepatocellular carcinoma, asthma, osteoporosis, sarcopenia, atherosclerotic stroke, and nervous inflammatory disorders, and in neurodegenerative diseases, such as Alzheimer's disease, Parkinson's disease, vascular dementia, fibromyalgia, autism spectrum disorder, and depression (2, 4, 5, 11, 12, 14–16, 23, 24). Conditions such as age, chemicals (antibiotics, tobacco, etc.), stress, and eating habits, among others, alter the composition of the gut microbiota, producing changes at the level of the immune system. These changes are caused by increased permeability of the gut and the blood-brain barrier, leading to a chronic inflammatory response (3–6, 8, 9, 12, 15, 25–27).
It is proposed that systematic inflammatory processes may effect inflammation in the central nervous system by microglial activation, cytokines, astrocytes, neurotransmitters (serotonin, dopamine, noradrenaline, and Y-aminobutyric acid), and altered short-chain fatty acids (SCFA) secreted by bacteria, or changes in enteric neuron activity detected by the vagus nerve (3–6, 8, 12, 15, 22, 28).
Lipid accumulation in glia is a pathological characteristic of Alzheimer's disease (29). The APOE gene encodes a lipid transporter protein that serves as a ligand for membrane receptors that mediate lipoprotein uptake (30–32). The human gene encoding APOE has three isoforms that differ by two amino acids: ε3 (APOE3), ε4 (APOE4) and ε2 (APOE2) (33). The most-validated risk factor for developing the late form of Alzheimer's disease is the presence of the E4 allele of the APOE gene (APOE4) (30–32, 34). In APOE4 carriers, plaque formation is increased due to oxidation of apolipoprotein E and binding to beta-amyloid (34). APOE4 decreases the age of onset and increases the risk of developing the disease by modulating several pathways that contribute to the development of this pathogenesis, including lipid metabolism and transport (32). The existence has been shown of metabolic changes in dementia and the protective role of specific food metabolites in cognitive aging.
Considering the role of microbiome balance in neurological diseases and that numerous pathological processes require much time of performance before cognitive decline appears, it seems essential to protect its stability. There are several strategies to counteract gut dysbiosis, such as fecal microbiota transplant (35). However, diet management is an even simpler way to deal with an imbalance in the microbiota, and the consumption of fermented foods and beverages produces significant improvements in gut permeability and the barrier function (2, 4, 16, 36–38).
Fermentation is traditionally used as a biological method of food preservation. Fermented foods and beverages are defined as those prepared using microorganisms—bacteria, yeasts, and fungi- and enzymatic action to transform their components into various fermentation end-products. The type of fermentation depends on the final product. Due to their health benefits, fermented foods are considered functional foods. The process of microbial fermentation converts food substrates into nutritionally and functionally richer products, resulting in functional micro-organisms (probiotics), substrates that enhance the growth of beneficial bacteria in the gut (prebiotics) and bioactive components (biogenics). These substances act in the gastrointestinal tract by modifying the microbiota, influencing exogenous endotoxin translocation and subsequent immune activation, and promoting host nutrition (39).
When administered in adequate amounts, probiotics can exert a health benefit to the host by restoring the microbiota and maintaining immune homeostasis (40). Some probiotics, known as brain probiotics or psychobiotics, regulate neurotransmitters such as serotonin, gamma-aminobutyric acid (GABA), glutamate and brain-derived neurotrophic factor (BDNF) in learning, memory, mood, and other cognitive processes (41–43). The most common probiotic bacteria currently used are representatives of Lactobacilli, Enterococci, Bifidobacteria, yeasts and bacterial mixtures (44).
Prebiotics are non-digestible substrates that host microorganisms use to provide a health benefit. The three most important prebiotic compounds are the polysaccharide inulin, fructooligosaccharides (FOS) derived from various crops or sucrose, and galactooligosaccharides (GOS). These substrates are present in various fermented foods, making them symbiotic foods (with probiotic and prebiotic effect), such as cheddar, gouda and parmesan cheeses, sauerkraut (fermented cabbage), kimchi (Korean pickle made from radish and cabbage), kefir, yogurt, kombucha, tempeh (made from fermented soybeans), miso (fermented soybean paste), soy sauce and apple cider vinegar (45).
Because of the many food-microbe combinations (Acetobacter, Leuconostoc, Lactobacillus, Streptococcus, etc.), we find various types of fermented foods and beverages (16). In this review, we summarize the results obtained for cognitive performance in elderly individuals after the consumption of fermented products.
2. Materials and methods
This review was performed according to the Preferred Reporting Items for Systematic Reviews and Meta-Analyses (PRISMA) guidelines (46). Details of the protocol used are registered on PROSPERO (CRD42021250921) and can be accessed at: https://www.crd.york.ac.uk/prospero/display_record.php?RecordID=250921 (47).
2.1. Literature search
The articles included in this review were selected from PUBMED, SCOPUS, and Cochrane Library databases, limited to Spanish and English languages and published from 16 March 1991 to 16 June 2022. Several searches were performed in March, May, and June 2021, and in June 2022 (just before the final analysis of the results, to include possible new articles). We also include articles from manual searches.
We used Medical Subject Headings (Mesh) terms for the PUBMED search and Boolean operators for all the databases. The search followed the PICO strategy: population (Aged); intervention (Beer OR Cheese OR Koumiss OR Buttermilk OR Kefir OR Yogurt OR “Cultured milk products” OR “Soy foods” OR “Wine” OR “Fermented foods and beverages” OR “Kombucha tea” OR “Fermented beverages” OR “Fermented foods” OR “Cultured food” OR “Fermented dairy products”); and outcome (“Memory Disorders” OR “Alzheimer's disease” OR Dementia OR “Neurocognitive disorders” OR “Cognitive dysfunction” OR “Cognitive aging” OR “Mental status and dementia tests” OR “Intellectual disability” OR “Learning disabilities”).
2.2. Inclusion and exclusion criteria
The studies included in this review were cross-sectional, cohort, and case-control studies, which passed the Newcastle-Ottawa Scale (NOS) (48, 49).
Inclusion criteria were available full-text articles, conducted in elderly individuals (65 years or older) with preserved cognition at the initial cognitive evaluation, that investigated the relationship between consumption of fermented foods or beverages (including alcohol (wine and beer); fermented dairy products; Kombucha, fermented or semi-fermented tea; soy-based foods; and coffee and cocoa, but not supplements) and cognitive dysfunction (dementia or Alzheimer's disease) as first outcome.
Exclusion criteria applied to works done in animals or in people who were cognitively impaired at the start of the study and under 65 years old. We also excluded articles in languages other than English and Spanish, those published before 16 March 1991, and those that did not achieve 7 points or more on the Newcastle-Ottawa Scale (NOS) (48, 49).
2.3. Study selection and data extraction
The authors independently selected and removed the duplicate studies, using Mendeley as database. The titles and the abstracts (in a first step) and the full text (in a second) of the remaining studies were screened according to the inclusion criteria. The authors extracted data from the included studies independently. The number of included and excluded studies and the selection process are illustrated in Figure 1.
From the included documents, the authors extracted the number, sex, and age of participants, the characteristics and the duration of the intervention, the outcome measures, and the results obtained.
2.4. Risk of bias
The authors independently carried out the selection of the papers, the extraction of the data, and their analysis. We used the Newcastle-Ottawa Scale (48) to assess the quality of the cohort and case-control studies and an adapted form of the Newcastle-Ottawa Scale for cross-sectional studies (49), checking characteristics such as randomization and blinding.
3. Results
3.1. Study selection
The articles included in this review were collected from PUBMED (24), SCOPUS (383), and Cochrane Library (44) databases. We enclosed 12 more studies from a manual review of the bibliography of the included articles. From these 465 articles, duplicates (6) and some papers after reading the title and abstract (395) were removed. Of 64 articles selected from the main text, 35 were rejected in accord with the exclusion criteria. Finally, we selected 29 articles for inclusion in this systematic review (Figure 1).
The studies included analyzed the association between cognitive impairment and consumption of alcohol (36, 50–77), coffee (36, 50, 52, 57, 58, 68, 73, 76, 77), cocoa (36, 52, 58) and soy-based foods (72, 73, 76, 77). Some of these articles studied the effects on cognition of fermented foods and beverages as part of a diet (36, 72–76) and will be discussed in a separate section.
3.2. Study characteristics
Of the 29 selected studies, 22 were cohort studies (52–56, 58–62, 64–66, 68–75), 4 case-control (36, 57, 63, 67) and 3 (51, 76, 77) cross-sectional studies. All the articles were published in English.
The possibility of doing a meta-analysis was rejected because of the high heterogeneity of the data. We found many differences in the included articles in clinical aspects (participants: number, sex, age; intervention: consumption of wine, beer, or alcohol in general, individual food or integrated in a diet, etc.); methodology (design of the studies and the risk of bias); and statistical processes (different statistical analysis).
To evaluate the quality of these articles, we used the Newcastle-Ottawa Scale (48, 49). All articles included in this review scored at least 7 points on the Newcastle-Ottawa Scale.
Information about the design, quality, and intervention of the included studies is shown in Table 1.
To determine the cognitive performance, the outcome measures were examined neuropsychologically and clinically, including brain MRI scans (51, 52)—taking into account anatomical aspects such as total brain volume size, white matter preservation or cerebral blood flow-and ordinary validated cognitive scales for assessing different domains of cognition (general cognitive ability, episodic memory, semantic memory, working memory, verbal memory, etc.), such as a mini-mental state examination (MMSE), a structured interview for the diagnosis of dementia of the Alzheimer's disease type (SIDAM), the diagnostic and statistical manual of mental disorders (DSM) and variations, the short portable mental status questionnaire (SPMSQ), National Institute of Neurological and Communicative Disorders and Stroke (NINCDS), clinical dementia rating scale (CDR), and the Consortium to Establish a Registry for Alzheimer's Disease (CERAD) tests, etc. (36, 53–77).
3.3. Fermented products consumption and cognitive function
The effect of the consumption of fermented products either as individual items (36, 50–71, 77) or integrated into a diet (72–76) was analyzed in the articles included. To avoid possible confusion due to food interactions, the studies in the latter category have been excluded from the overall analysis and will be discussed in a separate section.
3.3.1. Alcohol consumption
The consumption of alcohol–particularly wine and beer–was examined by most of the articles included in this review (50–71, 77). The main results obtained, and their statistical values are shown in Table 2.
To facilitate the comparison between the different results, we have converted the alcohol intake quantities to grams of ethanol. In those articles where the ethanol intake was not specified in g, the conversion was made so that 1 drink (5 ounces of wine, 12 ounces of beer, or 1, 5 ounces of liquors) is equivalent to 14 g of alcohol (74).
The reviewed articles have found some beneficial cognitive effect after low-moderate alcohol consumption (1 drink/month-4 drinks/day) (50–58, 60, 61, 63–71, 77). Only in one case was no cognitive benefit observed, but a worse visual reproduction was reported following the ingestion of 8.43 g of alcohol/day (<1 drink/day) (59). This beneficial effect disappeared in carriers of the APOE4 allele (50, 53, 67) although an increase in the size of the total brain volume was observed in them (51). Heavy alcohol or binge consumption was associated with an increased risk of cognitive impairment, especially in women (50, 62, 68).
When stratifying by gender, some controversies were revealed. While some found a protective effect of alcohol in general (65, 66, 68), and for wine (55, 68) and beer (68) in women, others associated wine consumption in women with an increased risk of Alzheimer's disease or memory decline (50) or, in the case of beer, with functional impairment (68), so further studies are needed.
Specific analysis of the effects of wine consumption has shown that moderate consumption (from 1 drink per day up to 4) may reduce the risk of dementia and/or Alzheimer's disease and enhance cognitive functions (50, 53, 54, 56, 63, 68, 71) improve memory capabilities in women and verbal ability in men and women (55), and result in a larger total brain volume (≤2 drinks/day in men and ≤1 drink/day in women) (51). However, one study showed an unfavorable cognitive outcome, but better white-matter preservation and improved cerebral blood flow (52).
In relation to white wine, a higher intake (≥1 drink/day) was significantly associated with a higher incidence of Alzheimer's disease in APOE4 allele carriers (50).
Although some results are not statistically significant, moderate beer consumption (1–3 drinks/day) was related with a larger brain volume (51) and a trend toward a neuroprotective effect for dementia (53, 54, 63, 68), but it was also associated with poorer verbal ability in men (55). Regarding protection against Alzheimer's disease, some consider beer to have protective effects (54) and others do not (53).
Many of the articles reviewed also found cognitive benefits following consumption of low-moderate amounts of liquors (51, 55, 63, 68), such as memory performance in men (55), improvement in cognitive impairment (63, 68) and a tendency to increased total brain volume (51). These data are not shown in Table 2 because they do not specify the type of liquor, so we cannot include them as fermented beverages.
3.3.2. Intake of coffee and cocoa
The effects of the intake of coffee (50, 52, 57, 58, 68, 77) and chocolate (52, 58) were also examined (Table 3). Tea consumption was excluded when the study was with non-fermented tea, the type used was not specified, or the tea consumption was integrated into a diet (73, 76).
Where data allowed, and to facilitate comparison between the different results, we estimated caffeine content (milligrams/standard unit) as 115 mg for regular coffee and 6 mg for chocolate (58).
All the results analyzed agree that the daily consumption of at least one cup of coffee was correlated with better cognitive performance (50, 52, 57, 58, 68, 77). Better preservation of bilateral deep white matter (p < 0, 05) and cerebral blood flow in cognitively stable elderly subjects (p < 0, 05) was also observed on MRI, with increasing consumption up to 5–6 cups (52). However, the neuroprotective role of chocolate is less clear in the reviewed articles (52, 58).
3.3.3. Consumption of other fermented foods and beverages
Daily consumption of soy-based foods was inversely associated with cognitive impairment [OR (95% CI) = 0.45 (0.25–0.81); p < 0.01] (77). The same beneficial results were obtained when soya products were integrated into a diet (72, 73, 76) (see Section 3.3.4).
On the other hand, higher intakes of dairy desserts and ice cream were associated with higher odds of cognitive impairment [OR (95% CI) = 1.33 (1,07-1,65); p = 0.01] (68).
3.3.4. Fermented products integrated into a diet
When fermented foods and beverages were integrated into a Mediterranean-type (74) or into a MIND diet—Intervention for neurodegenerative delay integrated for Mediterranean and DASH (dietary approach to systolic hypertension) diet (75)—rates of cognitive impairment decreased [(74): β = 0.014, SEE = 0.0004, p = 0.0004; (75): β = 0.0092; p < 0.0001].
Other dietary patterns including fermented foods and beverages—such as soy products, alcoholic beverages, coffee, etc.—were significantly associated with a better cognitive performance [βadjusted = 0.41 (95% CI):0, 17-0, 65; p < 0.001 (76)] and against decline of logical memory-recall [β = 0.18 (95% CI):0, 02-0, 33; p = 0.03 (73)]. Logical memory-recall decline was also improved in APOE4-carriers [OR (95% CI) = 0.71 (0.41–1.24); p = 0.23] and non-carriers [β (95% CI) = 0.18 (0.02–0.33); p = 0.03] with a dietary pattern rich in fermented foods (73).
Research on optimal polyphenol intake to reduce the risk of dementia and Alzheimer's disease by 50% proposes a dietary pattern (including for APOE4 carriers) that includes various fermented products [dementia: HR (95% CI) = 0.57 (0.37–0.86); p = 0.016; AD: HR (95% CI) = 0.54 (0.32–0.93); p = 0.045] (72).
Moreover, studying the relationship of diet-related metabolites with cognitive impairment showed two of the three biomarkers of coffee intake were inversely associated (atractyligenin glucuronide [OR = 0.72] and cyclo (leucyl-prolyl) [OR = 0.68]), but not that of the caffeine biomarker [OR = 1.75]. Cyclo (prolyl-valyl)—a metabolite found in chocolate and other fermented foods, e.g., beer, bread, cheddar cheese, cocoa, coffee, wine, Greek yogurt (78)—and an unidentified ion highly correlated with red wine and alcohol intake [OR = 0.69] were also inversely associated with cognitive impairment (36).
4. Discussion
Diet is increasingly used as a method of preventing disease and delaying aging. The use of fermented foods dates to prehistoric times, but findings on the benefits of this diet on the gut microbiota are relatively recent. The relationship between gut microbiota and the immune and nervous systems has been widely studied, and the implications of fermented food for mental processes performance, such as in dementia or Alzheimer's disease, are starting to be analyzed. For this reason, and considering that these are foods of daily consumption, it seems necessary to compile and analyze all the existing information on the elderly.
4.1. Strengths and weaknesses of this review
The main limitation encountered in conducting this review was the difference in information provided by the articles. It was difficult to compare results obtained in different populations, by different covariates, and using different methodologies. An attempt was made to solve this problem by converting fermented beverage intakes (amount in the form of drinks, cups, ounces, shots, or frequency of intake) to a standard measure (mg/day or g/day). Furthermore, the covariates for the different elements are diverse and may become very significant; for example, in the case of tobacco, which is associated not only with altered gut microbiota but also with gut inflammation (14). The variables in the papers were adjusted to these models to solve this problem.
The definition used of an elderly subject was a person aged 65 years or older (79–81), but perhaps the age range should have been extended to those under this age, since, as we have seen, the preventive effect of fermented products would occur in the long term.
In relation to the risk of bias, this review followed the preferred reporting items for systematic reviews and meta-analyses (PRISMA) guidelines (46) and the authors independently performed article selection, data extraction, analysis and assessed the quality of the included articles using the Newcastle-Ottawa scale (33, 34). All selected papers achieved the minimum quality criterion–i.e., 7 points on the NOS scale.
This work comprises a comprehensive review of current knowledge on the neuroprotective use of fermented foods and beverages. An important strength is that the protocol used to carry it out was registered and approved by the International Prospective Register of Systematic Reviews (PROSPERO) as CRD42021250921 (47).
4.2. Importance of a healthy gut microbiota
The gut microbiota is essential to maintain the structural integrity of the intestinal mucosa and the immune system (see Section 1), but some diseases can disrupt the microbiota composition (1–3, 6, 12, 82). The balance in the gut microbiota can also be altered by diet, stress, antibiotic treatment, aging, and tobacco, among other factors (Figure 2). Pathogenic bacteria and their products such as lipopolysaccharides (LPS) may disrupt intestinal mucosal barriers, increasing the permeability of the blood-brain barrier. The increased permeability of the intestinal epithelial barrier results in an invasion of different bacteria, viruses, and their neuroactive products that support neuroinflammatory response in the brain (11). Alterations in the composition of the microbiota affect brain health due to the existence of the gut-brain axis, a complex bidirectional system involving the endocrine, immune, and nervous systems (3, 5, 6, 11, 12, 83).
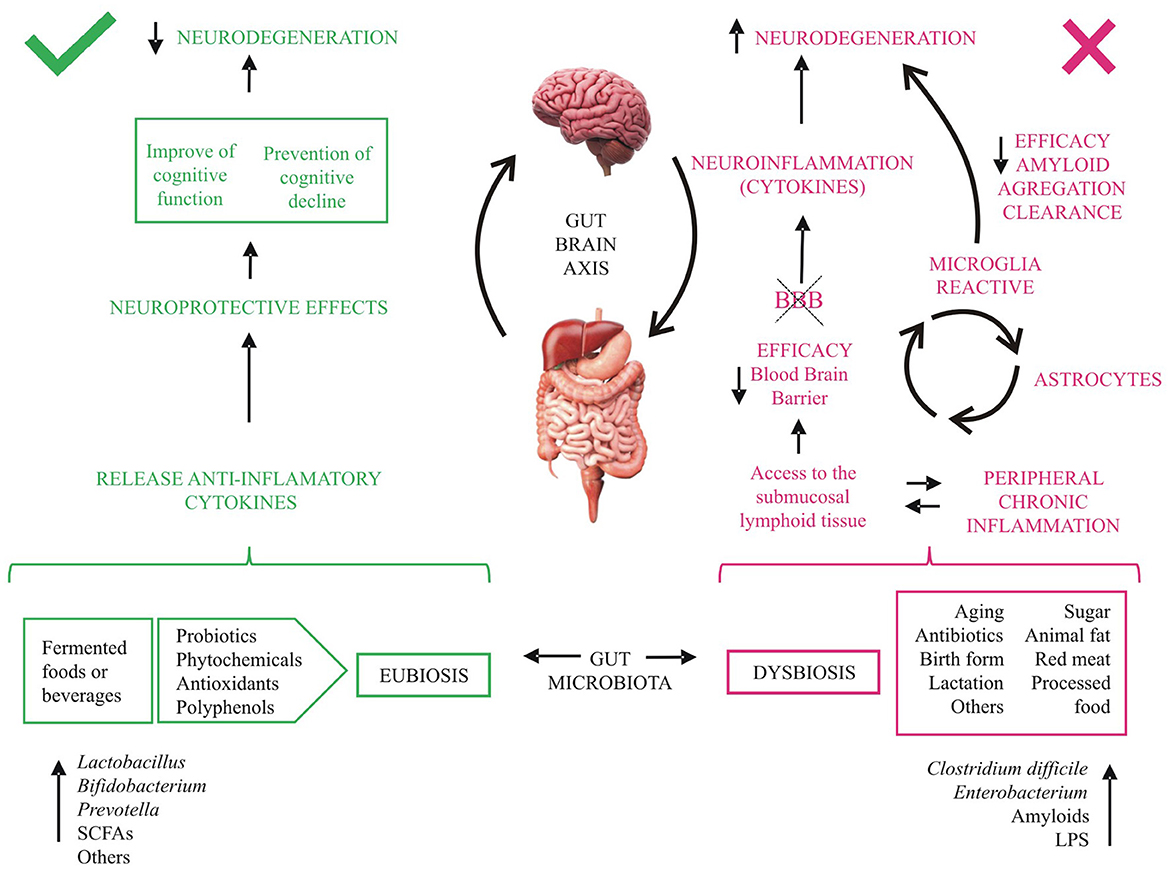
Figure 2. Effects of diet on cognitive decline. aa, amino acids; BBB, blood brain barrier; LPS, lipopolysaccharides; SCFAs, short- chain fatty acids. Fermented-food diet, ingestible microorganisms and others metabolites (green) act beneficially on intestinal health and inflammation and release anti-inflammatory cytokines, which promote neuroprotective effects. Several external factors, bacteria and components secreted by them (red) reduce over time the tightness of the intestinal barrier allowing access to the submucosal lymphoid tissue, favoring neuroinflammation and neurodegeneration.
4.2.1. Implications of aging on gut microbiota
The changes in microbiome during aging involve a reduction in microbial biodiversity and metabolites, leading to a chronic inflammatory reaction and changes in the immune system (Figure 2). These processes generate a permanent activation of microglia that produces chronic inflammation and leads to damage and neuronal death, probably mediated by cytokines (2, 4–6, 12, 15, 28). Furthermore, during aging, the microglia activate astrocytes, worsening the neural inflammation and the blood-brain barrier dysfunction, reducing its efficiency in preventing the entrance of microorganism and other cells and metabolites into the central nervous system (4, 13–15). Moreover, gut microbes have been found to influence the maturation and function of microglia (11).
4.2.2. The gut-brain axis in neurodegenerative diseases
A correlation has been found between an unhealthy gut microbiota and some nervous inflammatory and neurodegenerative diseases, such as some types of dementias and Alzheimer's disease (see Section 1).
Lately, the inflammatory hypothesis of Alzheimer's disease etiology is receiving attention (13, 14, 84–89). This involves the dysbiosis of the gut, mouth, and nose microbiota during aging, producing a systematic inflammatory response and the activation of the microglia to reduce it. Components secreted by bacteria reduce over time the impermeability of the intestinal barrier, enabling access to the submucosal lymphoid tissue. This phenomenon, together with deposits of amyloid-beta (Aβ) protein and hyperphosphorylated tau proteins, produces an inflammatory reaction, which impairs the blood-brain barrier, promoting neuroinflammation and neurodegeneration (13, 14, 43, 84). Accordingly, dysbiosis in the gut microbiota may provoke or enhance Alzheimer's disease, and it is possible that the structure of the gut microbiota is altered in Alzheimer's disease (13, 90).
Liu et al. identified the microbiome in patients with Alzheimer's disease and found its diversity was decreased. Moreover, this difference (and its importance) depended on the severity of the neurodegeneration. In individuals with Alzheimer's disease, the proportion of Firmicutes was significantly reduced, whereas that of Proteobacteria, Gammaproteobacteria, Enterobacteriales, and Enterobacteriaceae was increased, showing a progressively enriched prevalence from healthy individuals to mildly cognitive-impaired ones and patients with Alzheimer's disease (88).
The group of Harach generated germ-free mice using APPPS1 mice as an animal model of Alzheimer's disease (a double transgenic mice expressing mutated forms of the genes for human amyloid protein (APP) and presenilin 1) and found a drastic reduction of cerebral amyloid pathology when compared with control APP mice with intestinal microbiota. Furthermore, these amyloid deposits increased when germline mice were colonized with microbiota from APP transgenic mice more than when they were colonized with microbiota from wild-type mice (89).
In another mouse model of Alzheimer's disease, a dysregulated gut microbiota associated with the onset and progression of Alzheimer's disease was also observed, indicating that dysbiosis may occur before significant clinical signs appear, as evidenced by early alterations in short-chain fatty acids, compatible with intestinal inflammation (87).
The inefficiency of the microglia in reducing the amyloid deposits could explain the relationship between Alzheimer's disease and neuroinflammation (14, 15). Furthermore, pathogenic bacteria that are present in the gut microbiota due to dysbiosis can release amyloids, forming amyloid deposits in the brain. These amyloid deposits activate microglia, which fail to remove them, and participate in the production of proinflammatory cytokines, thus worsening neuroinflammation and initiating neurodegeneration (13, 15).
Choline supplementation has recently been found to restore a healthy cellular lipid status by promoting phospholipid synthesis in APOE4 cells in vitro and even in human APOE4 astrocytes (32). Moreover, women with APOE4 genotype have a higher risk of Alzheimer's disease (91, 92) and they tend to develop choline deficiency (93). The supplementation with citicoline improved cognitive performance, cerebral blood perfusion, and the brain bioelectrical activity pattern in Alzheimer's disease patients (94); fermented products contain choline, such as germs, soy, and milk (93).
In addition, several studies associate the dysregulation of serotonin and kynurenine route of tryptophan pathways, due to an alteration in the microbiota, with some neurodegenerative diseases, including Alzheimer's disease (15). Zhu proposes that tryptophan intake through the diet reduces central nervous system inflammation by decreasing astrocyte and microglial pathogenic activity (3, 95) and experiments in mice with multiple sclerosis showed dietary tryptophan to be neuroprotective (95). In this regard, tryptophan, and other derivatives in the kynurenine pathway (i.e., kynurenine, kynurenic acid, niacin, and nicotinamide) have been found in fermented food products: bread, beer, red wine, white cheese, yogurt, kefir, and cocoa (96).
4.3. Fermented diet as a possible preventive treatment in aging and in neurodegenerative diseases
Aging appears to be responsible for increasing the production of free radicals—enhancing brain oxidative stress—and for decreasing the activity of protective antioxidant enzymes. Thus, oxidative stress and vascular factors may also be involved in the development of dementia (56). In this regard, diets rich in antioxidant vitamins have shown cognitive improvements (97–99). Moreover, considering that nutrients—and their metabolites—can change the composition of the gut microbiota, a therapy focused on a diet recovering gut microbiota- such as the fermented diet- could have benefits in cognitive processes (4, 13, 15, 16, 100).
Fermented products are rich in probiotics and prebiotics. Probiotics are live microorganisms found naturally in some fermented foods. By consuming these foods, probiotics reach the gut, helping to balance the intestinal flora and improve digestion. Prebiotics are a type of food that is not fully digested by our bodies but can be fermented by beneficial bacteria in the gut, which contributes to their growth and development. This activity in the gut can affect the brain through two-way communication via the vagus nerve (90, 101).
The group of Hayden showed the diets with higher pro-inflammatory capacity were associated with a higher risk of mild cognitive impairment or dementia (102). Therefore, ingestible microorganisms present in fermented food act beneficially on intestinal health and inflammation, due to their positive effect on existing flora, as well as the release of anti-inflammatory cytokines (103, 104). Fermented-food diets have a very interesting nutritional content due to the biosynthesis of B vitamins, essential fatty acids and amino acids, proteins, and to the fact that they reduce anti-nutritional and toxic components and increase digestibility–as in the case of dairy products (reducing lactose content) and in legumes (reducing flatulence) (105–108).
Fermentation processes also enrich the bioactive peptides and create phytochemicals that can enhance the neuroprotective effects. Components modified through fermentation may improve bioavailability at the level of intestinal absorption and utilization of ingested nutrients and modulate the release of neurotransmitters such as brain-derived neurotrophic factor (BDNF), gamma-aminobutyric acid (GABA), and serotonin, which are involved in learning and memory processes (109). The study with FOS and GOS prebiotics by Savignac's group attributes its neuroprotective effects to increased BDNF levels in the dentate gyrus of the hippocampus in rats (42).
Moreover, fermented products such as tea, coffee, red wine, and cocoa products are rich in polyphenols—flavonoids, non-flavonoids (resveratrol), and phenolic acids. These bioactive compounds are being analyzed for their antioxidant properties and their possible role in intestinal permeability (2, 4, 16, 17, 36–38, 110) and their neuroprotective effects (2, 7, 8, 11, 26, 27, 111–114), even specifically in dementia and Alzheimer's disease (7, 8, 26, 27, 112, 113, 115). These effects could be due to their antioxidant, anti-inflammatory properties, and their ability to enhance the action of neurotrophic factors –among others, by increasing their concentration and/or the expression of tropomyosin receptor kinase (Trk) receptors (116, 117) or extracellular signal-regulated kinase and CREB pathways (117). Recent studies support the neuroprotective effect of resveratrol (13, 26), while others associate flavonoids with a lower risk of cognitive impairment (102, 118, 119). These authors argue that the mechanism by which flavonoids are neuroprotective appears to be the modification of the gut microbiota, specifically by increasing the proportion of Bifidobacterium, Prevotella, and Lactobacillus (17, 119, 120).
In any case, not all fermented foods contain polyphenols, such as dairy products. The concentration of polyphenols and their gastrointestinal absorption vary depending on the fermentation process; they are not useful if the intestinal microbiota is not healthy and is not able to break them down properly; and not all of them affect the colonic microflora and its fermentative capacity (121).
4.3.1. Potential benefits of fermented alcohol beverages in delaying cognitive decline
Most of the reviewed articles found cognitive benefits in old adults following low-moderate alcohol consumption (36, 50–58, 60, 61, 63–71, 77). However, most of these benefits did not appear with compulsive intakes or in carriers of the APOE4 allele (50, 53, 62, 67, 68). Although some neuroprotective effects appear after moderate intake of beer (51, 53–55, 63, 68), the results obtained with wine (50–56, 63, 68, 71), especially in men and after red wine intake (50), are particularly promising.
Some authors claim vascular benefits after moderate alcohol consumption because it increases high-density lipoprotein (HDL) and decreases triglycerides and low- and very low-density lipoprotein (LDL, VLDL) levels, thus reducing the risk of vascular lesions (56, 61). That this occurs, not only with wine, but also with beer and liquors, could suggest a protective effect of alcohol itself on vascular factors (56).
This hypothesis is supported by the results of the articles included in this review that analyzed moderate consumption of liquor (not shown), which indicated a positive effect on memory performance in men (55) and on cognitive function (63, 68) and a tendency to increase total brain volume (51). Only in one case was it associated with a higher risk of dementia and Alzheimer's disease, though the results were not statistically significant (53).
On the other hand, the health benefits of alcohol consumption may be due not only to the fermentation process itself, but also to the food matrix, fermentation process and microorganisms, and to the content of polyphenols (resveratrol, anthocyanins, flavonoids, and catechins), vitamins, and other metabolites (see Section 4.3). That this occurs especially in the case of red wine could be explained by the fact that red wine is richer in resveratrol than white wine due to the different production processes, although the concentration varies according to climate, soil, and fermentation time of the wine (114, 122). This also happens with beer (for the flavonoid xanthohumol and its metabolites), but not with liquors, which—with some exceptions—have a lower concentration of polyphenols and lower antioxidant activity (114, 122).
Fischer found differences in the effect of wine consumption in women and men, probably due to gender variations in alcohol metabolism. Women are more susceptible to alcohol toxicity, perhaps due to their smaller volume of distribution for ethanol, decreased first-pass metabolism or more rapid absorption, and more rapid metabolism of ethanol (114, 123). Furthermore, there are also inter-individual differences, as each subject can metabolize the alcohol differently (114).
Fischer also reported a higher incidence of Alzheimer's disease in APOE4 carriers with white wine consumption (50). These findings contradict those obtained in a recent in vitro study, which showed that natural compounds, including resveratrol, could modify the structure of APOE4 forms and thus ameliorate the pathogenic effects associated with Alzheimer's disease (115). Fischer's results could therefore be due to the low concentration of resveratrol in white wine.
4.3.2. Neuroprotective effects of coffee and chocolate
We analyzed the cognitive effects of caffeine intake in the form of coffee (36, 50, 52, 57, 58, 77) or chocolate (36, 52, 58).
The articles included in this review showed a neuroprotective effect of coffee in the elderly from the consumption of one cup per day (36, 52, 57, 58, 77), but not of chocolate (36, 52, 58). As some authors advocate a protective effect of caffeine (36, 124), it should be considered that the caffeine content of chocolate is much lower than that of coffee and that the concentration of cocoa in chocolate may differ. Moreover, these neuroprotective effects may not be due only to caffeine (125); coffee and cocoa are rich in phytochemicals (caffeine, chlorogenic acid, flavonoids, non-flavonoids and catechins) that confer on them antioxidant, prebiotic, anti-inflammatory, antihypertensive, hypoglycemic, vasculoprotective, neurostimulating, and neuroprotective properties (124, 126). Flavonoids and their metabolites can cross the blood-brain barrier and have been localized in brain areas related to learning and memory, such as the hippocampus, cerebral cortex, cerebellum, and striatum, enhancing or even being responsible for their possible cognitive effects (111, 125, 127–130). In animal models of Alzheimer's disease, flavonoids reduce amyloid-beta protein oligomerization and modulate the brain-derived neurotrophic factor (BDNF) signaling pathway (131, 132). They also interact at the cellular level with signaling cascades involving protein and lipid kinases that lead to inhibition of apoptosis induced by neurotoxic agents, such as oxygen radicals, and promote neuronal survival and synaptic plasticity, but also improve blood-flow and angiogenesis in the brain (133, 134). However, co-morbidities and personal genetics may influence the potential benefits and risks of coffee and cocoa (135).
4.3.3. Other fermented products
The results analyzed showed that daily consumption of soy-based foods was inversely associated with cognitive impairment (77). These results were like those from other articles in younger populations not included in this review (136), in mice (137, 138) and those obtained when soya products were integrated into a diet (72, 73, 76).
Soy is rich in phytoestrogens and isoflavones (daidzein and genistein), which exert an anti-inflammatory and antioxidant effect and inhibit the effects of mitochondrial apoptosis (139–141), elevating existing neuronal function, and boost neuronal regeneration (142, 143). In addition, because of their structural similarity, isoflavones can bind to estrogen receptors β (ERβ), which are abundant in the central nervous system, thus affecting brain activity (139). Soy phytoestrogens can modify the gut microbiota, thereby influencing the gut-brain axis. Isoflavone supplementation has also been shown to improve cognitive function (139, 144). The content of isoflavones has been found to be different in different types of soy product, and their bioavailability is higher in fermented products (141).
On the other hand, sweet dairy products were associated with higher odds of cognitive impairment (68) in line with another study performed in 55–75-year-old adults (145). This could be due to their high fat and sugar content, but this later study associated less cognitive impairment with consumption of whole-fat milk and dairy products (145). These results are in contradiction with other findings that advocate the neuroprotective effects of milk and dairy consumption on the risk of dementia (136, 146, 147), so more studies are needed.
4.3.4. Fermented-food diets
Dietary patterns including fermented foods and beverages—such as soy products, alcoholic beverages, coffee, etc.—showed some benefits regarding cognitive function (21, 36, 72–75), also in APOE4-carriers (72, 73).
The neuroprotective effects after consumption of fermented products are mainly due to a decrease in inflammatory processes (103, 104) and an increased release of brain-derived neurotrophic factors (42, 109), which enhance neuronal survival and differentiation (43). In animal models of Alzheimer's disease, probiotics modified the expression of GABA receptors in some brain regions related to learning and memory -such as the hippocampus, amygdala, and prefrontal cortex- and has been observed an improvement in hippocampal functions (43). As well as a decrease in pro-inflammatory cytokines levels -IL-1α, IL-1β, IL-2, IL-12, interferon-γ and TNF- α- leading to a decrease in accumulation of Aβ protein, neuroinflammation and neurodegeneration processes (90, 137, 148, 149). Probiotics also improved learning and memory processes (43, 149–151) regulated by long-term potentiation (LTP) possibly due to an increase in presynaptic neurotransmitter release (151) and excitatory postsynaptic potential in hippocampus (149). It should be considered that the beneficial effects of probiotics and fermented diets may depend on several factors, such as strain, dose, duration, age, host physiology, etc. (43).
These benefits could be due not only to the fermentation process itself, but also to a synergistic effect of the joint consumption of fermented products and to their concentrations of polyphenol compounds and vitamins (114). All these reasons encourage the possibility of a preventive treatment of cognitive decline through this type of diet, considering that a high consumption of some of these products (wine, coffee, etc.) may have undesirable effects, especially in vulnerable population.
4.3.5. Current implications and outlook
Due to the aging of the population, dementias and cognitive impairment are becoming an increasingly important public health problem. This carries a significant economic and psychological cost for which there is no etiological treatment, so the most effective approach is an early detection and prevention.
Eurostat predicts the population of the European Union in 2040 will be 524 million, of which the estimate of patients with Alzheimer's disease will be 13.1 million. By 2080, this figure is calculated to increase to 13.7 million individuals with Alzheimer's disease out of a total population of 520 million persons (152).
Of the total global burden of diseases, 23% is attributed to disorders in people aged 60 years and over. Of this expenditure, neurological and mental disorders in the elderly accounted for 6.6% in 2010 (153). Primary prevention in adults below this age would dramatically improve morbidity, mortality, and expenditure data related to these chronic diseases.
We have already mentioned the importance of a healthy gut flora in inflammatory reactions and to the immune system (see Sections 1 and 4.2). The involvement of the microbiota in pathologies, including neurodegenerative diseases, is increasingly accepted. Therefore, one strategy to prevent cognitive decline could be to take care of the composition of this microbiota through diet.
In accordance, studies have shown that fermented foods and beverages produce significant improvements in gut permeability and the barrier function (2, 16, 36–38). In this sense, the Mediterranean-type diet has proved to be a balanced and healthy diet, compared with others (2, 4, 83), due to its monounsaturated and polyunsaturated fatty acids, fiber and low-glycemic carbohydrates, and polyphenols and other antioxidants. This could be because it includes a wide variety of fermented foods and beverages, such as coffee, wine, dairy products, etc., as opposed to the higher consumption of processed foods, sweets, and red meat in other diets. Studies associate this fermented diet and its beneficial effects with an increase in Lactobacillus and Bifidobacterium, and a decrease in Clostridium and Enterobacterium, among others, in the gut microbiota, providing anti-inflammatory effects and cardiovascular and brain protection (2–4, 25, 83) (see Figure 2).
In terms of digestibility, fermented foods are often easier to digest than non-fermented ones (154) because the microorganisms included provide certain enzymes, such as cellulases, which cannot be synthesized by humans (155). For example, microbial cellulases hydrolyze cellulose into sugars, which are easily digestible by humans, and pectinases soften the texture of food and release sugars for digestion. In addition, fermentation is a very productive and energy-efficient preservation process. It requires minimal cooking times and reduces the need for refrigeration or other forms of food preservation technology (155). Besides, during the fermentation process, toxins and anti-nutritional compounds commonly found in fruits and vegetables can be removed or detoxified by the action of microorganisms (155). Lactic acid bacteria produce lactate and acetate, which reduce the pH of food and inhibit other pathogenic organisms. It can also produce ethanol, hydrogen peroxide, and bacteriocins, which kill or suppress the growth of food-borne pathogenic bacteria and enhance food preservation and safety (106).
The use of microorganisms to meet the world's growing demand for food has enormous scope and potential. Fermentation is a very sustainable method as it can rescue waste that would otherwise be unusable as food by changing the consistency of the product and making it digestible. This increases the range of raw materials available as nutrients through the efficient utilization of natural foods and available feedstocks (155). Microorganisms in fermented foods are potential sources of useful components, such as organoleptic properties, textures, and colors that offer a wide range of foods to the consumer. Moreover, fermentation can improve the taste and appearance of foods. The strong flavors of fermented products can enhance the taste of a monotonous and boring diet (105–108).
Altough more studies in humans are necessary, this dietary pattern gives hope for the prevention of neurodegenerative diseases, above and beyond the public health and economic savings it would bring and the impact it could have on the food market.
5. Conclusions
In this systematic review, we have presented an array of published articles investigating the effects on cognitive status due to the consumption of fermented foods and beverages in the elderly. We can draw some conclusions from the articles studied:
1. Beneficial cognitive effects have been found following a low-moderate alcohol consumption, except in carriers of the APOE4 allele.
2. Heavy alcohol or binge consumption is associated with an increased risk of cognitive impairment, especially in women.
3. Moderate wine consumption appears to reduce the risk of dementia and/or Alzheimer's disease, to improve cognitive function, cerebral blood flow, and white-matter preservation, and to increase total brain volume.
4. Consumption of at least one cup of coffee per day is associated with better cognitive performance. Higher intakes (2-6 cups per day) are related to better preservation of bilateral deep white matter and cerebral blood flow.
5. An improvement in cognitive function following the daily intake of soy products has been observed.
6. Dietary patterns that include fermented foods and beverages have been shown to decrease the rates of cognitive decline, even in carriers of the APOE4 allele.
7. Due to the fermentation process itself and to the polyphenolic compounds and other antioxidants and vitamins in fermented products, the fermented-food diet could become an effective, safe, and inexpensive preventive or cognitive enhancement strategy.
Data availability statement
The original contributions presented in the study are included in the article/supplementary material, further inquiries can be directed to the corresponding author.
Author contributions
EP-G: literature search and data analysis and writing. IF-E: literature search and data analysis. JG-G and JF-G: data analysis and writing—review and editing. All authors contributed to the study conception and design.
Funding
This study was supported by Plan Andaluz de Investigación - CTS-563.
Acknowledgments
We thank Cristina Aguilera Xiol, José Mª Delgado-García, and Roger Churchill for providing writing assistance and language help.
Conflict of interest
The authors declare that the research was conducted in the absence of any commercial or financial relationships that could be construed as a potential conflict of interest.
Publisher's note
All claims expressed in this article are solely those of the authors and do not necessarily represent those of their affiliated organizations, or those of the publisher, the editors and the reviewers. Any product that may be evaluated in this article, or claim that may be made by its manufacturer, is not guaranteed or endorsed by the publisher.
Abbreviations
APOE4, E4 allele of the Apolipoprotein E gene; AD, Alzheimer's disease; ASL, arterial spin labeling; B, regression coefficient; β, regression beta coefficient; CI, confidence interval; HR, Hazard ratio; MRI, magnetic resonance imaging; OR, Odds ratio; SEE, standard error of estimate.
References
1. Bäckhed F, Ley RE, Sonnenburg JL, Peterson DA, Gordon JI. Host-bacterial mutualism in the human intestine. Science. (2005) 307:1915–20. doi: 10.1126/science.1104816
2. Singh RK, Chang HW, Yan D, Lee KM, Ucmak D, Wong K, et al. Influence of diet on the gut microbiome and implications for human health. J Transl Med. (2017) 15:1–17. doi: 10.1186/s12967-017-1175-y
3. Zhu S, Jiang Y, Xu K, Cui M, Ye W, Zhao G, et al. The progress of gut microbiome research related to brain disorders. J Neuroinflammation. (2020) 17:1–20. doi: 10.1186/s12974-020-1705-z
4. Bernardi S, del Bo' C, Marino M, Gargari G, Cherubini A, Andrés-Lacueva C, et al. Polyphenols and intestinal permeability: rationale and future perspectives. J Agric Food Chem. (2020) 68:1816–29. doi: 10.1021/acs.jafc.9b02283
5. Fung TC, Olson CA, Hsiao EY. Interactions between the microbiota, immune and nervous systems in health and disease. Interactions. (2017) 20:145–55. doi: 10.1038/nn.4476
6. Silva YP, Bernardi A, Frozza RL. The role of short-chain fatty acids from gut microbiota in gut-brain communication. Front Endocrinol. (2020) 11:1–14. doi: 10.3389/fendo.2020.00025
7. Wang D, Ho L, Faith J, Ono K, Janle EM, Lachcik PJ, et al. Role of intestinal microbiota in the generation of polyphenol-derived phenolic acid mediated attenuation of Alzheimer's disease β-amyloid oligomerization. Mol Nutr Food Res. (2015) 59:1025–40. doi: 10.1002/mnfr.201400544
8. Leblhuber F, Steiner K, Schuetz B, Fuchs D, Gostner JM. Probiotic supplementation in patients with Alzheimer's dementia—An explorative intervention study. Curr Alzheimer Res. (2018) 15:1106–13. doi: 10.2174/1389200219666180813144834
9. Vogt NM, Kerby RL, Dill-McFarland KA, Harding SJ, Merluzzi AP, Johnson SC, et al. Gut microbiome alterations in Alzheimer's disease. Sci Rep. (2017) 7:1–11. doi: 10.1038/s41598-017-13601-y
10. Power SE, O'Toole PW, Stanton C, Ross RP, Fitzgerald GF. Intestinal microbiota, diet and health. Br J Nutri. (2014) 111:387–402. doi: 10.1017/S0007114513002560
11. Shimizu Y. Gut microbiota in common elderly diseases affecting activities of daily living. World J Gastroenterol. (2018) 24:4750–8. doi: 10.3748/wjg.v24.i42.4750
12. Mirzaei R, Bouzari B, Hosseini-Fard SR, Mazaheri M, Ahmadyousefi Y, Abdi M, et al. Role of microbiota-derived short-chain fatty acids in nervous system disorders. Biomed Pharmacotherapy. (2021) 139:111661. doi: 10.1016/j.biopha.2021.111661
13. Pistollato F, Sumalla Cano S, Elio I, Masias Vergara M, Giampieri F, Battino M. Role of gut microbiota and nutrients in amyloid formation and pathogenesis of Alzheimer's disease. Nutr Rev. (2016) 74:624–34. doi: 10.1093/nutrit/nuw023
14. Quigley EMM. Microbiota-brain-gut axis and neurodegenerative diseases. Curr Neurol Neurosci Rep. (2017) 17:6. doi: 10.1007/s11910-017-0802-6
15. Sochocka M, Donskow-Łysoniewska K, Diniz BS, Kurpas D, Brzozowska E, Leszek J. The gut microbiome alterations and inflammation-driven pathogenesis of Alzheimer's disease—A critical review. Mol Neurobiol. (2019) 56:1841–51. doi: 10.1007/s12035-018-1188-4
16. Marco ML, Heeney D, Binda S, Cifelli CJ, Cotter PD, Foligné B, et al. Health benefits of fermented foods: microbiota and beyond. Curr Opin Biotechnol. (2017) 44:94–102. doi: 10.1016/j.copbio.2016.11.010
17. Zhang Y, Cheng L, Liu Y, Zhan S, Wu Z, Luo S, et al. Dietary flavonoids: a novel strategy for the amelioration of cognitive impairment through intestinal microbiota. J Sci Food Agric. (2023) 103:488–95. doi: 10.1002/jsfa.12151
18. Chandra S, Sisodia SS, Vassar RJ. The gut microbiome in Alzheimer's disease: what we know and what remains to be explored. Mol Neurodegener. (2023) 18:7. doi: 10.1186/s13024-023-00595-7
19. Koblinsky ND, Power KA, Middleton L, Ferland G, Anderson ND. The role of the gut microbiome in diet and exercise effects on cognition: a review of the intervention literature. J Gerontol Series A. (2022) 3:166. doi: 10.1093/gerona/glac166
20. Strasser B, Ticinesi A. Intestinal microbiome in normal ageing, frailty and cognition decline. Curr Opin Clin Nutr Metab Care. (2023) 26:8–16. doi: 10.1097/MCO.0000000000000878
21. Dahiya D, Nigam PS. Antibiotic-Therapy-Induced Gut Dysbiosis Affecting Gut Microbiota-Brain Axis and Cognition: Restoration by Intake of Probiotics and Synbiotics. Int J Mol Sci. (2023) 24:74. doi: 10.3390/ijms24043074
22. Sharma R, Gupta D, Mehrotra R, Mago P. Psychobiotics: the next-generation probiotics for the brain. Curr Microbiol. (2021) 78:449–63. doi: 10.1007/s00284-020-02289-5
23. Minerbi A, Gonzalez E, Brereton NJB, Anjarkouchian A, Dewar K, Fitzcharles MA, et al. Altered microbiome composition in individuals with fibromyalgia. Pain. (2019) 160:2589–602. doi: 10.1097/j.pain.0000000000001640
24. Clos-Garcia M, Andrés-Marin N, Fernández-Eulate G, Abecia L, Lavín JL, van Liempd S, et al. Gut microbiome and serum metabolome analyses identify molecular biomarkers and altered glutamate metabolism in fibromyalgia. EBioMedicine. (2019) 46:499–511. doi: 10.1016/j.ebiom.2019.07.031
25. Tomova A, Bukovsky I, Rembert E, Yonas W, Alwarith J, Barnard ND, Kahleova H. The effects of vegetarian and vegan diets on gut microbiota. Front Nutr. (2019) 6:47. doi: 10.3389/fnut.2019.00047
26. Köbe T, Witte AV, Schnelle A, Tesky VA, Pantel J, Schuchardt J-P, et al. Impact of resveratrol on glucose control, hippocampal structure and connectivity, and memory performance in patients with mild cognitive impairment. Front Neurosci. (2017) 11:1–11. doi: 10.3389/fnins.2017.00105
27. Witte A V, Kerti L, Margulies DS, Flöel A. Effects of resveratrol on memory performance, hippocampal functional connectivity, and glucose metabolism in healthy older adults. J Neurosci. (2014) 34:7862–70. doi: 10.1523/JNEUROSCI.0385-14.2014
28. Rogers GB, Keating DJ, Young RL, Wong ML, Licinio J, Wesselingh S. From gut dysbiosis to altered brain function and mental illness: Mechanisms and pathways. Mol Psychiatry. (2016) 21:738–48. doi: 10.1038/mp.2016.50
29. Stelzmann RA, Norman Schnitzlein H, Reed Murtagh F. An english translation of alzheimer's 1907 paper,??ber eine eigenartige erkankung der hirnrinde? Clinical Anatomy. (1995) 8:429–31. doi: 10.1002/ca.980080612
30. Innerarity TL, Mahley RW. Enhanced binding by cultured human fibroblasts of apo-E-containing lipoproteins as compared with low density lipoproteins. Biochemistry. (1978) 17:1440–7. doi: 10.1021/bi00601a013
31. Mahley R, Bersot T, LeQuire V, Levy R, Windmueller H, Brown W. Identity of very low density lipoprotein apoproteins of plasma and liver Golgi apparatus. Science. (1970) 68:380–2. doi: 10.1126/science.168.3929.380
32. Sienski G, Narayan P, Bonner JM, Kory N, Boland S, Arczewska AA, et al. APOE4 disrupts intracellular lipid homeostasis in human iPSC-derived glia. Sci Transl Med. (2021) 13:4564. doi: 10.1126/scitranslmed.aaz4564
33. McIntosh AM, Bennett C, Dickson D, Anestis SF, Watts DP, Webster TH, et al. The Apolipoprotein E. (APOE) gene appears functionally monomorphic in Chimpanzees (Pan troglodytes) PLoS One. (2012) 7:e47760. doi: 10.1371/journal.pone.0047760
34. Strittmatter WJ, Weisgraber KH, Huang DY, Dong LM, Salvesen GS, Pericak-Vance M, et al. Binding of human apolipoprotein E to synthetic amyloid beta peptide: isoform-specific effects and implications for late-onset Alzheimer's disease. Proc Nat Acad Sci. (1993) 90:8098–102. doi: 10.1073/pnas.90.17.8098
35. Baldi S, Mundula T, Nannini G, Amedei A. Microbiota shaping — the effects of probiotics, prebiotics, and fecal microbiota transplant on cognitive functions: a systematic review. World J Gastroenterol. (2021) 27:6715–32. doi: 10.3748/wjg.v27.i39.6715
36. Low DY, Lefèvre-Arbogast S, González-Domínguez R, Urpi-Sarda M, Micheau P, Petera M, et al. Diet-related metabolites associated with cognitive decline revealed by untargeted metabolomics in a prospective cohort. Mol Nutr Food Res. (2019) 63:1–10. doi: 10.1002/mnfr.201900177
37. Bell V, Ferrão J, Pimentel L, Pintado M, Fernandes T. One health, fermented foods, and gut microbiota. Foods. (2018) 7:195. doi: 10.3390/foods7120195
38. Dimidi E, Cox S, Rossi M, Whelan K. Fermented foods : definitions and characteristics, gastrointestinal health and disease. Nutrients. (2019) 11:1–26. doi: 10.3390/nu11081806
39. Aslam H, Green J, Jacka FN, Collier F, Berk M, Pasco J, et al. Fermented foods, the gut and mental health: a mechanistic overview with implications for depression and anxiety. Nutr Neurosci. (2020) 23:659–71. doi: 10.1080/1028415X.2018.1544332
40. Reid G, Younes JA, Van der Mei HC, Gloor GB, Knight R, Busscher HJ. Microbiota restoration: natural and supplemented recovery of human microbial communities. Nat Rev Microbiol. (2011) 9:27–38. doi: 10.1038/nrmicro2473
41. Perez-Pardo P, Kliest T, Dodiya HB, Broersen LM, Garssen J, Keshavarzian A, et al. The gut-brain axis in Parkinson's disease: possibilities for food-based therapies. Eur J Pharmacol. (2017) 817:86–95. doi: 10.1016/j.ejphar.2017.05.042
42. Savignac HM, Corona G, Mills H, Chen L, Spencer JPE, Tzortzis G, et al. Prebiotic feeding elevates central brain derived neurotrophic factor, N-methyl-d-aspartate receptor subunits and d-serine. Neurochem Int. (2013) 63:756–64. doi: 10.1016/j.neuint.2013.10.006
43. Thangaleela S, Sivamaruthi BS, Kesika P, Chaiyasut C. Role of probiotics and diet in the management of neurological diseases and mood states: a review. Microorganisms. (2022) 10:2268. doi: 10.3390/microorganisms10112268
44. Varankovich NV, Nickerson MT, Korber DR. Probiotic-based strategies for therapeutic and prophylactic use against multiple gastrointestinal diseases. Front Microbiol. (2015) 6:685. doi: 10.3389/fmicb.2015.00685
46. Moher D, Shamseer L, Clarke M, Ghersi D, Liberati A, Petticrew M, et al. Preferred reporting items for systematic review and meta-analysis protocols. (PRISMA-P) 2015 statement. Syst Rev. (2015) 4:1. doi: 10.1186/2046-4053-4-1
47. PROSPERO. (International prospective register of systematic reviews)- CRD42021250921. Available online at: https://www.crd.york.ac.uk/prospero/display_record.php?RecordID=250921 (accessed June 5, 2023).
48. Wells GA, Shea, B, O'Connell, D, Peterson, J, Welch, V, Losos, M, Tugwell, P,. The Newcastle-Ottawa Scale. (NOS) for Assessing the Quality of Nonrandomised Studies in Meta-Analyses. (2015). Available online at: http://www.ohri.ca/programs/clinical_epidemiology/oxford.asp (accessed June 5, 2023).
49. Herzog R, Álvarez-Pasquin MJ, Díaz C. del Barrio JL, Estrada JM, Gil Á. Are healthcare workers' intentions to vaccinate related to their knowledge, beliefs and attitudes? A systematic review. BMC Public Health. (2013) 13:154. doi: 10.1186/1471-2458-13-154
50. Fischer K, Melo van Lent D, Wolfsgruber S, Weinhold L, Kleineidam L, Bickel H, et al. Prospective associations between single foods, alzheimer's dementia and memory decline in the elderly. Nutrients. (2018) 10:852. doi: 10.3390/nu10070852
51. Gu Y, Scarmeas N, Short EE, Luchsinger JA, DeCarli C, Stern Y, et al. Alcohol intake and brain structure in a multiethnic elderly cohort. Clin Nutr. (2014) 33:662–7. doi: 10.1016/j.clnu.2013.08.004
52. Haller S, Montandon ML, Rodriguez C, Herrmann FR, Giannakopoulos P. Impact of coffee, wine, and chocolate consumption on cognitive outcome and MRI parameters in old age. Nutrients. (2018) 10:1–13. doi: 10.3390/nu10101391
53. Luchsinger JA, Tang M-X, Siddiqui M, Shea S, Mayeux R. Alcohol intake and risk of dementia. J Am Geriatr Soc. (2004) 52:540–6. doi: 10.1111/j.1532-5415.2004.52159.x
54. Weyerer S, Schäufele M, Wiese B, Maier W, Tebarth F, van den bussche H, et al. Current alcohol consumption and its relationship to incident dementia: Results from a 3-year follow-up study among primary care attenders aged 75 years and older age. Ageing. (2011) 40:456–63. doi: 10.1093/ageing/afr007
55. Corley J, Jia X, Brett CE, Gow AJ, Starr JM, Kyle JAM, et al. Alcohol intake and cognitive abilities in old age: the Lothian Birth Cohort 1936 study. Neuropsychology. (2011) 25:166–75. doi: 10.1037/a0021571
56. Larrieu S, Letenneur L, Helmer C, Dartigues JF, Barberger-Gateau P. Nutritional factors and risk of incident dementia in the PAQUID longitudinal cohort. J Nutr Health Aging. (2004) 8:150–4. Available online at: http://www.ncbi.nlm.nih.gov/pubmed/15129300 (accessed July 19, 2022).
57. Lindsay J, Laurin D, Verreault R, Hébert R, Helliwell B, Hill GB, et al. Risk factors for Alzheimer's disease: a prospective analysis from the Canadian study of health and aging. Am J Epidemiol. (2002) 156:445–53. doi: 10.1093/aje/kwf074
58. Paganini-Hill A, Kawas CH, Corrada MM. Lifestyle factors and dementia in the oldest-old: the 90 + study. Alzheimer Dis Assoc Disord. (2016) 30:21–6. doi: 10.1097/WAD.0000000000000087
59. Broe GA, Creasey H, Jorm AF, Bennett HP, Casey B, Waite LM, et al. Health habits and risk of cognitive impairment and dementia in old age: a prospective study on the effects of exercise, smoking and alcohol consumption. Aust N Z J Public Health. (1998) 22:621–3. doi: 10.1111/j.1467-842X.1998.tb01449.x
60. Huang W, Qiu C, Winblad B, Fratiglioni L. Alcohol consumption and incidence of dementia in a community sample aged 75 years and older. J Clin Epidemiol. (2002) 55:959–64. doi: 10.1016/S0895-4356(02)00462-6
61. Ogunniyi A, Hall KS, Gureje O, Baiyewu O, Gao S, Unverzagt FW, et al. Risk factors for incident Alzheimer's disease in African Americans and Yoruba. Metab Brain Dis. (2006) 21:235–40. doi: 10.1007/s11011-006-9017-2
62. Järvenpää T, Rinne JO, Koskenvuo M, Räihä I, Kaprio J. Binge drinking in midlife and dementia risk. Epidemiology. (2005) 16:766–71. doi: 10.1097/01.ede.0000181307.30826.6c
63. Hébert R, Lindsay J, Verreault R, Rockwood K, Hill G, Dubois MF. Vascular dementia. Stroke. (2000) 31:1487–93. doi: 10.1161/01.STR.31.7.1487
64. Cervilla JA. Smoking, drinking, and incident cognitive impairment: a cohort community based study included in the Gospel Oak project. J Neurol Neurosurg Psychiatry. (2000) 68:622–6. doi: 10.1136/jnnp.68.5.622
65. Stampfer MJ, Kang JH, Chen J, Cherry R, Grodstein F. Effects of moderate alcohol consumption on cognitive function in women. New England Journal of Medicine. (2005) 352:245–53. doi: 10.1056/NEJMoa041152
66. Espeland MA, Gu L, Masaki KH, Langer RD, Coker LH, Stefanick ML, et al. Association between reported alcohol intake and cognition: results from the women's health initiative memory study. Am J Epidemiol. (2005) 161:228–38. doi: 10.1093/aje/kwi043
67. Anttila T, Helkala E-L, Viitanen M, Kåreholt I, Fratiglioni L, Winblad B, et al. Alcohol drinking in middle age and subsequent risk of mild cognitive impairment and dementia in old age: a prospective population based study. BMJ. (2004) 329:539. doi: 10.1136/bmj.38181.418958.BE
68. Vercambre MN, Boutron-Ruault MC, Ritchie K, Clavel-Chapelon F, Berr C. Long-term association of food and nutrient intakes with cognitive and functional decline: a 13-year follow-up study of elderly French women. Br J Nutri. (2009) 102:419–27. doi: 10.1017/S0007114508201959
69. Ganguli M, Vander-Bilt J, Saxton JA, Shen C, Dodge HH. Alcohol consumption and cognitive function in late life: a longitudinal community study. Neurology. (2005) 65:1210–7. doi: 10.1212/01.wnl.0000180520.35181.24
70. Launer LJ, Feskens EJM, Kalmijn S, Kromhout D. Smoking, drinking, and thinking: the Zutphen elderly study. Am J Epidemiol. (1996) 143:219–27. doi: 10.1093/oxfordjournals.aje.a008732
71. Lemeshow S, Letenneur L, Dartigues JF, Lafont S, Orgogozo JM, Commenges D. Illustration of analysis taking into account complex survey considerations: the association between wine consumption and dementia in the PAQUID study. Personnes Ages Quid Am J Epidemiol. (1998) 148:298–306. doi: 10.1093/oxfordjournals.aje.a009639
72. Lefèvre-Arbogast S, Gaudout D, Bensalem J, Letenneur L, Dartigues JF, Hejblum BP, et al. Pattern of polyphenol intake and the long-term risk of dementia in older persons. Neurology. (2018) 90:e1979–88. doi: 10.1212/WNL.0000000000005607
73. Chen YC, Jung CC, Chen JH, Chiou JM, Chen TF, Chen YF, et al. Association of dietary patterns with global and domain-specific cognitive decline in Chinese elderly. J Am Geriatr Soc. (2017) 65:1159–67. doi: 10.1111/jgs.14741
74. Tangney CC, Kwasny MJ Li H, Wilson RS, Evans DA, Morris MC. Adherence to a Mediterranean-type dietary pattern and cognitive decline in a community population. Am J Clin Nutr. (2011) 93:601–7. doi: 10.3945/ajcn.110.007369
75. Morris MC, Tangney CC, Wang Y, Sacks FM, Barnes LL, Bennett DA, et al. diet slows cognitive decline with aging. Alzheimer's and Dementia. (2015) 11:1015–22. doi: 10.1016/j.jalz.2015.04.011
76. Okubo H, Inagaki H, Gondo Y, Kamide K, Ikebe K, Masui Y, et al. Association between dietary patterns and cognitive function among 70-year-old Japanese elderly: a cross-sectional analysis of the SONIC study. Nutr J. (2017) 16:2. doi: 10.1186/s12937-017-0273-2
77. Lin H-C, Peng C-H, Huang C-N, Chiou J-Y. Soy-based foods are negatively associated with cognitive decline in Taiwan's elderly. J Nutr Sci Vitaminol. (2018) 64:335–9. doi: 10.3177/jnsv.64.335
78. Borthwick AD, da Costa NC. 2,5-diketopiperazines in food and beverages: taste and bioactivity. Crit Rev Food Sci Nutr. (2017) 57:718–42. doi: 10.1080/10408398.2014.911142
79. Lohr KN. Medicare: a strategy for quality assurance. J Healthcare Qual. (1991) 13:10–3. doi: 10.1111/j.1945-1474.1991.tb00115.x
81. Aged- MeSH,. NCBI. (1996). Available online at: https://www.ncbi.nlm.nih.gov/mesh/68000368 (accessed January 17, 2022).
82. MacDonald TT, Monteleone G. Immunity, inflammation, and allergy in the gut. Science. (2005) 307:1920–5. doi: 10.1126/science.1106442
83. Rinninella C, Raoul L, Scaldaferri P, Miggiano G, Mele. Food components and dietary habits: keys for a healthy gut microbiota composition. Nutrients. (2019) 11:2393. doi: 10.3390/nu11102393
84. Wang WY, Tan MS, Yu JT, Tan L. Role of pro-inflammatory cytokines released from microglia in Alzheimer's disease. Ann Transl Med. (2015) 3:136. doi: 10.3978/j.issn.2305-5839.2015.03.49
85. Cameron B, Landreth GE. Inflammation, microglia, and Alzheimer's disease. Neurobiol Dis. (2010) 37:503–9. doi: 10.1016/j.nbd.2009.10.006
86. Agostinho PA, Cunha R, Oliveira C. Neuroinflammation, oxidative stress and the pathogenesis of Alzheimer's disease. Curr Pharm Des. (2010) 16:2766–78. doi: 10.2174/138161210793176572
87. Favero F, Barberis E, Gagliardi M, Espinoza S, Contu L, Gustincich S, et al. A Metabologenomic approach reveals alterations in the gut microbiota of a mouse model of Alzheimer's disease. PLoS ONE. (2022) 17:e0273036. doi: 10.1371/journal.pone.0273036
88. Liu P, Wu L, Peng G, Han Y, Tang R, Ge J, et al. Altered microbiomes distinguish Alzheimer's disease from amnestic mild cognitive impairment and health in a Chinese cohort. Brain Behav Immun. (2019) 80:633–43. doi: 10.1016/j.bbi.2019.05.008
89. Harach T, Marungruang N, Duthilleul N, Cheatham V, Mc Coy KD, Frisoni G, et al. Reduction of Abeta amyloid pathology in APPPS1 transgenic mice in the absence of gut microbiota. Sci Rep. (2017) 7:1–13. doi: 10.1038/srep41802
90. Kowalski K, Mulak A. Brain-Gut-Microbiota Axis in Alzheimer's Disease. J Neurogastroenterol Motil. (2019) 25:48–60. doi: 10.5056/jnm18087
91. Neu SC, Pa J, Kukull W, Beekly D, Kuzma A, Gangadharan P, et al. Apolipoprotein E genotype and sex risk factors for Alzheimer disease. JAMA Neurol. (2017) 74:1178. doi: 10.1001/jamaneurol.2017.2188
92. Damoiseaux JS, Seeley WW, Zhou J, Shirer WR, Coppola G, Karydas A, et al. Gender modulates the APOE ε4 effect in healthy older adults: convergent evidence from functional brain connectivity and spinal fluid tau levels. J Neurosci. (2012) 32:8254–62. doi: 10.1523/JNEUROSCI.0305-12.2012
93. Zeisel SH, Da Costa KA. Choline: an essential nutrient for public health. Nutr Rev. (2009) 67:615–23. doi: 10.1111/j.1753-4887.2009.00246.x
94. Alvarez XA, Mouzo R, Pichel V, Pérez P, Laredo M, Fernández-Novoa L, et al. Double-blind placebo-controlled study with citicoline in APOE genotyped Alzheimer's disease patients. Effects on cognitive performance, brain bioelectrical activity and cerebral perfusion. Methods Find Exp Clin Pharmacol. (1999) 21:633–44.
95. Rothhammer V, Borucki DM, Tjon EC, Takenaka MC, Chao C-C, Ardura-Fabregat A, et al. Microglial control of astrocytes in response to microbial metabolites. Nature. (2018) 557:724–8. doi: 10.1038/s41586-018-0119-x
96. Yilmaz C, Gökmen V. Determination of tryptophan derivatives in kynurenine pathway in fermented foods using liquid chromatography tandem mass spectrometry. Food Chem. (2018) 243:420–7. doi: 10.1016/j.foodchem.2017.10.004
97. Morris MC. Dietary intake of antioxidant nutrients and the risk of incident alzheimer's disease in a biracial community study. JAMA. (2002) 287:3230. doi: 10.1001/jama.287.24.3230
98. Engelhart MJ. Dietary intake of antioxidants and risk of Alzheimer's disease. JAMA. (2002) 287:3223. doi: 10.1001/jama.287.24.3223
99. Grodstein F, Chen J, Willett WC. High-dose antioxidant supplements and cognitive function in community-dwelling elderly women. Am J Clin Nutr. (2003) 77:975–84. doi: 10.1093/ajcn/77.4.975
100. Tillisch. Consumption of fermented milk product with probiotic modulates brain activity. Gastroenterology. (2013) 144:1–15. doi: 10.1053/j.gastro.2013.02.043.Conflicts
101. Oak SJ, Jha R. The effects of probiotics in lactose intolerance: a systematic review. Crit Rev Food Sci Nutr. (2019) 59:1675–83. doi: 10.1080/10408398.2018.1425977
102. Hayden KM, Beavers DP, Steck SE, Hebert JR, Tabung FK, Shivappa N, et al. The association between an inflammatory diet and global cognitive function and incident dementia in older women: the Women's Health Initiative Memory Study. Alzheimer's and Dementia. (2017) 13:1187–96. doi: 10.1016/j.jalz.2017.04.004
103. Foligné B, Parayre S, Cheddani R, Famelart MH, Madec MN, Plé C, et al. Immunomodulation properties of multi-species fermented milks. Food Microbiol. (2016) 53:60–9. doi: 10.1016/j.fm.2015.04.002
104. Shen J, Zuo ZX, Mao AP. Effect of probiotics on inducing remission and maintaining therapy in ulcerative colitis, Crohn's disease, and pouchitis: Meta-analysis of randomized controlled trials. Inflamm Bowel Dis. (2014) 20:21–35. doi: 10.1097/01.MIB.0000437495.30052.be
105. Cook PE. Fermented foods as biotechnological resources. Food Res Int. (1994) 27:309–16. doi: 10.1016/0963-9969(94)90099-X
106. Campbell-Platt G. Fermented foods—A world perspective. Food Res Int. (1994) 27:253–7. doi: 10.1016/0963-9969(94)90093-0
107. Reddy NR, Pierson MD. Reduction in antinutritional and toxic components in plant foods by fermentation. Food Res Int. (1994) 27:281–90. doi: 10.1016/0963-9969(94)90096-5
108. Steinkraus KH. Nutritional significance of fermented foods. Food Res Int. (1994) 27:259–67. doi: 10.1016/0963-9969(94)90094-9
109. Kim B, Hong VM, Yang J, Hyun H, Im JJ, Hwang J, et al. Review of fermented foods with beneficial effects on brain and cognitive function. Prev Nutr Food Sci. (2016) 21:297–309. doi: 10.3746/pnf.2016.21.4.297
110. Bo' B, Marino P, Tucc, G, Cherubini C, Kirkup K. Systematic review on polyphenol intake and health outcomes: is there sufficient evidence to define a health-promoting polyphenol-rich dietary pattern? Nutrients. (2019) 11:1355. doi: 10.3390/nu11061355
111. Brickman AM, Khan UA, Provenzano FA, Yeung L-K, Suzuki W, Schroeter H, et al. Enhancing dentate gyrus function with dietary flavanols improves cognition in older adults. Nat Neurosci. (2014) 17:1798–803. doi: 10.1038/nn.3850
112. Turner RS, Thomas RG, Craft S, van Dyck CH, Mintzer J, Reynolds BA, et al. randomized, double-blind, placebo-controlled trial of resveratrol for Alzheimer's disease. Neurology. (2015) 85:1383–91. doi: 10.1212/WNL.0000000000002035
113. Yuan T, Ma H, Liu W, Niesen DB, Shah N, Crews R, et al. Pomegranate's neuroprotective effects against Alzheimer's disease are mediated by urolithins, its ellagitannin-gut microbial derived metabolites. ACS Chem Neurosci. (2016) 7:26–33. doi: 10.1021/acschemneuro.5b00260
114. Arranz S, Chiva-Blanch G, Valderas-Martínez P, Medina-Remón A, Lamuela-Raventós RM, Estruch R. Wine, beer, alcohol and polyphenols on cardiovascular disease and cancer. Nutrients. (2012) 4:759–81. doi: 10.3390/nu4070759
115. Mountaki C, Dafnis I, Panagopoulou EA, Vasilakopoulou PB, Karvelas M, Chiou A, et al. Mechanistic insight into the capacity of natural polar phenolic compounds to abolish Alzheimer's disease-associated pathogenic effects of apoE4 forms. Free Radic Biol Med. (2021) 171:284–301. doi: 10.1016/j.freeradbiomed.2021.05.022
116. Carito V, Ceccanti M, Tarani L, Ferraguti GN, Chaldakov G, Fiore M. Neurotrophins'; modulation by olive polyphenols. Curr Med Chem. (2016) 23:3189–97. doi: 10.2174/0929867323666160627104022
117. Moosavi F, Hosseini R, Saso L, Firuzi O. Modulation of neurotrophic signaling pathways by polyphenols. Drug Des Devel Ther. (2015) 23:6936. doi: 10.2147/DDDT.S96936
118. Omar SH. Mediterranean and MIND diets containing olive biophenols reduces the prevalence of Alzheimer's disease. Int J Mol Sci. (2019) 20:2797. doi: 10.3390/ijms20112797
119. Pei R, Liu X, Bolling B. Flavonoids and gut health. Curr Opin Biotechnol. (2020) 61:153–9. doi: 10.1016/j.copbio.2019.12.018
120. Leblhuber F, Ehrlich D, Steiner K, Geisler S, Fuchs D, Lanser L, et al. The immunopathogenesis of Alzheimer's disease is related to the composition of gut microbiota. Nutrients. (2021) 13:1–34. doi: 10.3390/nu13020361
121. Bravo L. Polyphenols: chemistry, dietary sources, metabolism, and nutritional significance. Nutr Rev. (2009) 56:317–33. doi: 10.1111/j.1753-4887.1998.tb01670.x
122. Mrvcic J, Posavec S, Kazazic S, Stanzer D, Peša A, Stehlik-tomas V. Spirit drinks: a source of dietary polyphenols. Croatian J Food Sci Technol. (2012) 4:102–111.
123. Thomasson HR. “Gender Differences in Alcohol Metabolism.,” Recent Developments in Alcoholism. Boston, MA: Springer US. (2002). p. 163–179 doi: 10.1007/0-306-47138-8_9
124. Ludwig IA, Clifford MN, Lean MEJ, Ashihara H, Crozier A. Coffee: biochemistry and potential impact on health. Food Funct. (2014) 5:1695–717. doi: 10.1039/C4FO00042K
125. Shukitt-Hale B, Miller MG, Chu YF, Lyle BJ, Joseph JA. Coffee, but not caffeine, has positive effects on cognition and psychomotor behavior in aging. Age (Omaha). (2013) 35:2183–92. doi: 10.1007/s11357-012-9509-4
126. Vauzour D. Dietary polyphenols as modulators of brain functions: biological actions and molecular mechanisms underpinning their beneficial effects. Oxid Med Cell Longev. (2012) 2012:1–16. doi: 10.1155/2012/914273
127. Conte A, Pellegrini S, Tagliazucchi D. Synergistic protection of PC12 cells from β-amyloid toxicity by resveratrol and catechin. Brain Res Bull. (2003) 62:29–38. doi: 10.1016/j.brainresbull.2003.08.001
128. Gleason CE, Carlsson CM, Barnet JH, Meade SA, Setchell KDR, Atwood CS, et al. preliminary study of the safety, feasibility and cognitive efficacy of soy isoflavone supplements in older men and women. Age Ageing. (2009) 38:86–93. doi: 10.1093/ageing/afn227
129. Mandel SA, Winreb O, Amit T, Youdim MB. Eve Topf center for neurodegenerative diseases research and department of molecular pharmacology, faculty of medicine, Technion, Haifa, Israel. Front Biosci. (2012) 4:581–98. doi: 10.2741/S286
130. Socci V, Tempesta D, Desideri G, de Gennaro L, Ferrara M. Enhancing human cognition with cocoa flavonoids. Front Nutr. (2017) 4:19. doi: 10.3389/fnut.2017.00019
131. Cimini A, Gentile R, D'Angelo B, Benedetti E, Cristiano L, Avantaggiati ML, et al. Cocoa powder triggers neuroprotective and preventive effects in a human Alzheimer's disease model by modulating BDNF signaling pathway. J Cell Biochem. (2013) 114:2209–20. doi: 10.1002/jcb.24548
132. Wang J, Varghese M, Ono K, Yamada M, Levine S, Tzavaras N, et al. Cocoa extracts reduce oligomerization of amyloid-β: implications for cognitive improvement in Alzheimer's disease. J Alzheimer's Dis. (2014) 41:643–50. doi: 10.3233/JAD-132231
133. Nehlig A. The neuroprotective effects of cocoa flavanol and its influence on cognitive performance. Br J Clin Pharmacol. (2013) 75:716–27. doi: 10.1111/j.1365-2125.2012.04378.x
134. Sokolov AN, Pavlova MA, Klosterhalfen S, Enck P. Chocolate and the brain: Neurobiological impact of cocoa flavanols on cognition and behavior. Neurosci Biobehav Rev. (2013) 37:2445–53. doi: 10.1016/j.neubiorev.2013.06.013
135. Carman AJ, Dacks PA, Lane RF, Shineman DW, Fillit HM. Current evidence for the use of coffee and caffeine to prevent age-related cognitive decline and Alzheimer's disease. J Nutr Health Aging. (2014) 18:383–92. doi: 10.1007/s12603-014-0021-7
136. Ozawa M, Ninomiya T, Ohara T, Doi Y, Uchida K, Shirota T, et al. Dietary patterns and risk of dementia in an elderly Japanese population: the Hisayama study. Am J Clin Nutr. (2013) 97:1076–82. doi: 10.3945/ajcn.112.045575
137. Abdolmaleky HM, Sheng Y, Zhou J-R. Bioactive nutraceuticals oligo-lactic acid and fermented soy extract alleviate cognitive decline in mice in part via anti-neuroinflammation and modulation of gut microbiota. Front Nutr. (2023) 10:6278. doi: 10.3389/fnut.2023.1116278
138. Ko JW, Chung Y-S, Kwak CS, Kwon YH. Doenjang, A Korean traditional fermented soybean paste, ameliorates neuroinflammation and neurodegeneration in mice fed a high-fat diet. Nutrients. (2019) 11:1702. doi: 10.3390/nu11081702
139. Szczerba E, Koch M, Schlesinger S. Soy consumption, cognitive function, and dementia. Curr Opin Lipidol. (2022) 33:68–75. doi: 10.1097/MOL.0000000000000807
140. Rizzo G, Baroni L. Soy, soy foods and their role in vegetarian diets. Nutrients. (2018) 10:43. doi: 10.3390/nu10010043
141. Messina M, Mejia SB, Cassidy A, Duncan A, Kurzer M, Nagato C, et al. Neither soyfoods nor isoflavones warrant classification as endocrine disruptors: a technical review of the observational and clinical data. Crit Rev Food Sci Nutr. (2022) 62:5824–85. doi: 10.1080/10408398.2021.1895054
142. Dias GP, Cavegn N, Nix A, do Nascimento Bevilaqua MC, Stangl D, Zainuddin MSA, et al. The role of dietary polyphenols on adult hippocampal neurogenesis: molecular mechanisms and behavioural effects on depression and anxiety. Oxid Med Cell Longev. (2012) 2012:1–18. doi: 10.1155/2012/541971
143. do Prado FG, Pagnoncelli MGB, de Melo Pereira GV, Karp SG, Soccol CR. Fermented soy products and their potential health benefits: a review. Microorganisms. (2022) 10:1606. doi: 10.3390/microorganisms10081606
144. Cheng PF, Chen JJ, Zhou XY, Ren YF, Huang W, Zhou JJ, et al. Do soy isoflavones improve cognitive function in postmenopausal women? A meta-analysis. Menopause. (2015) 22:198–206. doi: 10.1097/GME.0000000000000290
145. Muñoz-Garach A, Cornejo-Pareja I, Martínez-González MÁ, Bulló M, Corella D, Castañer O, et al. Milk and dairy products intake is related to cognitive impairment at baseline in predimed plus trial. Mol Nutr Food Res. (2021) 65:2000728. doi: 10.1002/mnfr.202000728
146. Ozawa M, Ohara T, Ninomiya T, Hata J, Yoshida D, Mukai N, et al. Milk and dairy consumption and risk of dementia in an elderly Japanese population: the Hisayama study. J Am Geriatr Soc. (2014) 62:1224–30. doi: 10.1111/jgs.12887
147. Ogata S, Tanaka H, Omura K, Honda C, Hayakawa K. Association between intake of dairy products and short-term memory with and without adjustment for genetic and family environmental factors: a twin study. Clin Nutr. (2016) 35:507–13. doi: 10.1016/j.clnu.2015.03.023
148. Bonfili L, Cecarini V, Cuccioloni M, Angeletti M, Berardi S, Scarpona S, et al. SLAB51 PRobiotic formulation activates SIRT1 pathway promoting antioxidant and neuroprotective effects in an AD mouse model. Mol Neurobiol. (2018) 55:7987–8000. doi: 10.1007/s12035-018-0973-4
149. Mehrabadi S, Sadr SS. Assessment of probiotics mixture on memory function, inflammation markers, and oxidative stress in an Alzheimer's disease model of rats. Iran Biomed J. (2020) 24:220–8. doi: 10.29252/ibj.24.4.220
150. Athari Nik Azm S, Djazayeri A, Safa M, Azami K, Ahmadvand B, Sabbaghziarani F, et al. Lactobacilli and bifidobacteria ameliorate memory and learning deficits and oxidative stress in β-amyloid. (1–42) injected rats. Appl Physiol Nutri and Metabol. (2018) 43:718–26. doi: 10.1139/apnm-2017-0648
151. Rezaeiasl Z, Salami M, Sepehri G. The effects of probiotic lactobacillus and bifidobacterium strains on memory and learning behavior, long-term potentiation. (LTP), and some biochemical parameters in β-amyloid-induced rat's model of Alzheimer's disease. Prev Nutr Food Sci. (2019) 24:265–73. doi: 10.3746/pnf.2019.24.3.265
152. Tomaskova H, Kuhnova J, Cimler R, Dolezal O, Kuca K. Prediction of population with Alzheimer's disease in the European Union using a system dynamics model. Neuropsychiatr Dis Treat. (2016) 12:1589–98. doi: 10.2147/NDT.S107969
153. Prince MJ, Wu F, Guo Y, Gutierrez Robledo LM, O'Donnell M, Sullivan R, et al. The burden of disease in older people and implications for health policy and practice. Lancet. (2015) 385:549–62. doi: 10.1016/S0140-6736(14)61347-7
154. Kovač B, Raspor P. The use of the mould Rhizopus oligosporus in food production. Food Technol Biotechnol. (1997) 35:69–73.
155. Battcock M, Azam-Ali S. “Fermented Fruits and Vegetables: A Global Perspective.,” Food & Agriculture Org. (1998). Available online at: https://www.fao.org/3/x0560e/x0560e00.htm (accessed September 12, 2022).
Keywords: fermented-food, fermented beverages, cognitive decline, elderly, Alzheimer's disease, dementia
Citation: Porras-García E, Fernández-Espada Calderón I, Gavala-González J and Fernández-García JC (2023) Potential neuroprotective effects of fermented foods and beverages in old age: a systematic review. Front. Nutr. 10:1170841. doi: 10.3389/fnut.2023.1170841
Received: 21 February 2023; Accepted: 30 May 2023;
Published: 15 June 2023.
Edited by:
Xiaojie Zhang, Central South University, ChinaReviewed by:
Gargi Ghoshal, Panjab University, IndiaYulia Irnidayanti, Jakarta State University, Indonesia
Na Zhang, Harbin University of Commerce, China
Copyright © 2023 Porras-García, Fernández-Espada Calderón, Gavala-González and Fernández-García. This is an open-access article distributed under the terms of the Creative Commons Attribution License (CC BY). The use, distribution or reproduction in other forums is permitted, provided the original author(s) and the copyright owner(s) are credited and that the original publication in this journal is cited, in accordance with accepted academic practice. No use, distribution or reproduction is permitted which does not comply with these terms.
*Correspondence: Juan Gavala-González, amdhdmFsYSYjeDAwMDQwO3VzLmVz