- 1Tecnología Alimentaria, Centro de Investigación y Asistencia en Tecnología y Diseño del Estado de Jalisco (CIATEJ), Zapopan, Mexico
- 2Tecnologico de Monterrey, The Institute for Obesity Research, Monterrey, Mexico
The milpa system is a biocultural polyculture technique. Heritage of Mesoamerican civilizations that offers a wide variety of plants for food purposes. Corn, common beans, and pumpkins are the main crops in this agroecosystem, which are important for people’s nutritional and food security. Moreover, milpa system seeds have great potential for preventing and ameliorating noncommunicable diseases, such as obesity, dyslipidemia, type 2 diabetes, among others. This work reviews and analyzes the nutritional and health benefits of milpa system seeds assessed by recent preclinical and clinical trials. Milpa seeds protein quality, vitamins and minerals, and phytochemical composition are also reviewed. Evidence suggests that regular consumption of milpa seeds combination could exert complementing effect to control nutritional deficiencies. Moreover, the combination of phytochemicals and nutritional components of the milpa seed could potentialize their individual health benefits. Milpa system seeds could be considered functional foods to fight nutritional deficiencies and prevent and control noncommunicable diseases.
1. Introduction
Milpa is an agro-productive system used in Mesoamerica, especially in Mexico, since pre-Hispanic times; its etymological origin comes from the Nahuatl language. The words (“milli” means “planted plot” and “pan” means “above”) (1). The milpa system (MS) is a traditional polyculture method, which constitutes a dynamic space for genetic resources. Corn (Zea mays L.) is the main species in the MS and is accompanied by other edible species, such as common beans (Phaseolus vulgaris L.), pumpkin (Cucurbita pepo L.), chili (Capsicum annum L.), and tomato (Solanum lycopersicum L.) (1–3). The MS is considered the first organized agricultural system in the Americas and was the base of the feeding of the Mesoamerican civilizations. However, the MS has lost popularity and has been replaced by monoculture practices (2–4).
The combination of corn, common beans, and pumpkin is known as “the Mesoamerican triad” or “milpa triad.” The MS is more efficient in using natural sources (such as lower water demand, soil, space, and light) compared to traditional monocultures (2–5). Moreover, the MS offers various food products compared to conventional agriculture (5, 6). In the same way, MS polyculture helps the crops’ resilience to the adverse effects of climate change (7). In an eco-friendly future scenario, the MS offers one of the most sustainable alternatives to producing food with low environmental impact (6, 8–11).
The milpa system-derived food products represent an important source of nutritional components (protein, complex carbohydrates, fiber, lipids, vitamins and minerals) and phytochemical compounds (phenolics, saponins, phytosterols, carotenoids, polyunsaturated fatty acids). Several investigations highlight milpa seeds’ nutritional and health benefits due to their chemical composition (12–17). Regular consumption of corn, common beans, and pumpkin seeds have been related to preventing and mitigating noncommunicable diseases, such as cancer, type 2 diabetes, hypertension, obesity, among others (12–17).
Nutritional and pharmacological evaluations of the milpa seeds have been performed on seeds cultivated in monoculture. Besides, most of these studies were made on the seeds individually. The evaluation of the combined milpa seed’s nutritional and biological potential has not been explored (4). This work reviews and analyzes the nutritional and health benefits of milpa system seeds assessed by recent preclinical and clinical trials. A comprehensive evaluation of the potential nutritional and health benefits of the milpa triad seeds diet incorporation was performed.
2. Materials and methods
2.1. Bibliographic review
The bibliographic search was carried out using the following keywords: “milpa system,” “Phaseolus vulgaris,” “Zea mays,” and “Cucurbita pepo”; using Scopus,1 PubMed,2 Elsevier,3 Google Scholar,4 ResearchGate,5 Web of Science,6 and ScienceDirect7 as the main database; those articles not older than 5 years were selected.
2.1.1. Nutritional quality estimation
The combination of milpa seed proteins in the diet could be a strategy to reach the recommended daily dose for adequate nutrition (FAO/WHO/UNU) (18, 19). Corn, common bean, or pumpkin seed proteins show a deficiency in one or more essential amino acids. To estimate the value of the proteins from the milpa seeds, the following protein quality parameters were calculated:
• Amino acid score (AAS) of seed proteins was calculated using the FAO/WHO/UNU (19) reference pattern and using the following equation:
• Essential amino acid index (EAAI) was estimated using the following equation comparing the amino acid composition of the whole egg protein as standard (20):
• where “a” represents the amino acid content specified in the formula in seed or grain protein samples and “b” is the content of the same amino acids in standard egg protein (%), respectively.
• Predicted biological value (BV) was calculated using the following equation (21):
• Protein efficiency ratios (PER) were calculated from the amino acid composition of grains samples based on the following five equations (21):
3. Main findings
3.1. Milpa system
The milpa system is a polyculture-diversified food production and is considered by the Food and Agriculture Organization (FAO) as an “important system of world agricultural heritage.” This system guaranteed the perpetuation of the culture and covers the basic food needs of peasant families. The MS favors the production of foods due to the morphological differences between the roots of common beans, corn, and pumpkin, which promote the absorption of nutrients. The entanglement of the common bean plant in the corn canes favors sheltering beneficial insects. It decreases the development of weeds and generates a microenvironment that conserves humidity in times of drought. Moreover, the common beans promote the fixation of atmospheric nitrogen. Besides, the pumpkin covers the soil, reducing the appearance of pests and weeds, and reducing weeding, thus increasing the fertility of the land for prolonged periods (Figure 1) (22–24).
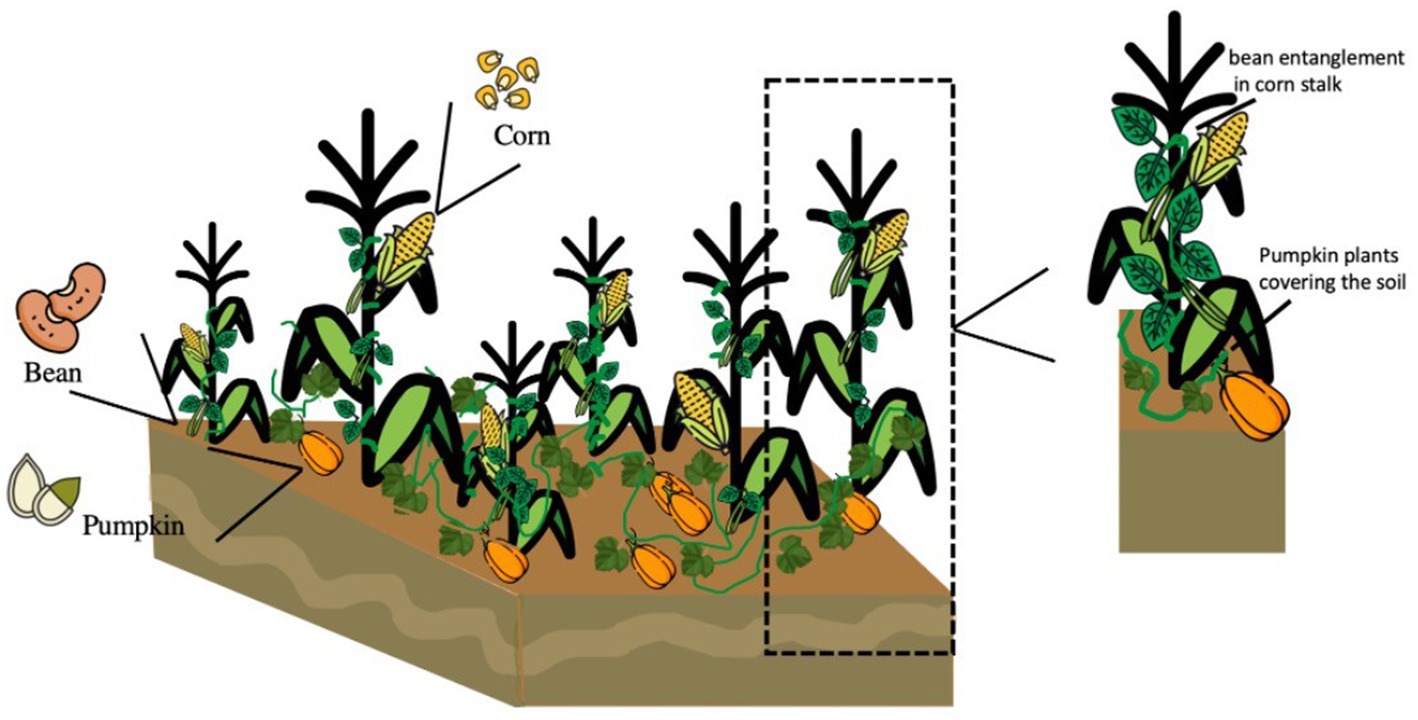
Figure 1. Interaction of the plants of the main milpa system components (corn, common beans, pumpkin).
This type of agricultural food production system in Mexico has decreased over time due to the introduction of monocultures with high economic impact and the loss of farmers’ financial support. Another important factor is the change in traditional diets due to industrialization in the food sector and changes in lifestyles that have promoted the introduction of highly processed foods with high calories and poor nutritional value, resulting in the appearance of diet-related diseases (25).
3.2. Nutritional composition
Milpa system plants are considered crops of great importance worldwide. Their nutritional importance is related to their carbohydrate, lipid, and protein content, as well as vitamins and minerals (Table 1) (12, 15, 26–38).
4. Macronutrients
The major macronutrient of cereals, pulses, and pumpkin seeds is carbohydrates (54–80%), which consist of structure and reserve materials, such as fiber and starch, respectively (39). The carbohydrate content in corn ranges between 67.8–74.9%, mostly composed of starch and dietary fiber (2–11.2%), such as hemicellulose and pectin (26–30). In common beans, carbohydrates range between 43 and 65%, mainly composed of starch, resistant starch, and dietary fiber (30–33). In pumpkin seeds, carbohydrates range between 10.6–25%, mainly composed of starch, and dietary fiber (3–6.5%), such as hemicellulose and lignin; the content of carbohydrates and fiber varies with the consumption of whole seed or only the kernel seed (12, 34–37).
Lipids in corn and common beans present a proportion not higher than 5%, but their chemical composition is especially rich in mono- and polyunsaturated fatty acids (38). The lipid content in corn varies between 2.7–4.4% and is composed mainly of unsaturated (oleic acid), polyunsaturated (linoleic acid), and saturated (palmitic acid) fatty acids (26–29). In common beans, lipids vary between 0.3–2.5%, composed mainly of polyunsaturated fatty acids, such as linoleic and linolenic acids (30–34). In pumpkin seeds, lipids are about 41.4–50.5%, showing a profile of polyunsaturated fatty acids (mainly linoleic acid) (12, 34–37).
Protein content is an important criterion for determining the quality of seeds. Corn has a protein content of 9.3–15.8%, mainly composed of zeins and globulins (26–29). Common beans present a protein content of 19.1–28.3%, including albumins, phaseolins, and glutelins (29–33). Pumpkin seeds are rich in protein with 21.3–37.7%, mainly constituted of globulins, glutelins, and albumins (12, 34–37).
4.1. Nutritional properties of protein in milpa system seeds
4.1.1. Amino acid score
The amino acid score (AAS) is based on the calculation of limiting amino acids (Table 2). The AAS is a complement for calculating protein digestibility and should be corrected for incomplete protein digestibility and the unavailability of individual amino acids (40). The AAS of corn, common bean, and pumpkin seeds ranged from 90 to 170.9%, 107.9–173.1%, and 32.1–160.1%, respectively. The three seeds showed maximum values above 100%, which means that the consumption of certain varieties of these seeds could be above the amino acid intake recommended by the FAO/WHO (40). Ranges in AAS could be associated with phenotypic characteristics derived from environmental factors influencing the genotype of the crops evaluated. Several varieties of milpa seeds are grown under different latitudes, which could influence the chemical composition of these seeds (26–33, 39). However, according to the amino acid profile of common bean seeds showed the best AAS among the MS triad grains compared to egg protein reference (20).
4.1.2. Essential amino acid index
The essential amino acid index (EAAI) is the geometric mean of the ratios of the essential amino acids in the food protein relative to their content in a highly nutritious reference protein vs. whole egg (Table 2) (41). The EAAI estimated was 61.8–123.4% for corn, 81.5–151.4% for common beans, and 2.2–46.4% for pumpkin seeds. Values of EAAI over 90% are assumed as good nutritional quality (20). Corn and common beans could be considered that meet this qualification. Common beans presented the highest EAAI values due to their high protein content and the relatively high proportion of essential amino acids, compared with cereals (i.e., oat flour and oat protein concentrate present 44.55 and 45.92%, respectively) and non-legume seeds (i.e., canola meal show 80% and 32.8–32.9% for amaranth flours) (42, 43).
4.1.3. Biological value
The EAAI not only integrates all essential amino acids into the calculation but also allows estimating the effect of amino acid or protein supplementation on biological value (BV, nitrogen with potential to be absorbed from food for tissue formation) (Table 2) (41). As EAAI, values of BV over 70% may be considered good nutritional quality products (20). The BV of corn, common bean, and pumpkin seeds were estimated at 55.7–122.8%, 77.1–153.3%, and 9.3–38.9%, respectively. Similarly, the highest BV values were found for common beans.
4.1.4. Protein efficiency ratios
The protein efficiency ratio (PER) is an index of nutritional quality represented by values ranging from 0 to up to 2 (from low to high protein quality) (21). According to the amino acid profile and PER criteria for estimating MS seeds, there is a wide range of PER values, especially in pumpkin seeds (Table 2). These disparities could be related to the amino acid content variation among the seeds. For example, the limiting amino acids in corn are Lys and Trp, sulfur amino acids Cis and Met in common beans, and Lys and Thr in pumpkin seeds. However, consuming these seeds in combination could complement the amino acid deficiencies of individual seed proteins (44, 45).
5. Micronutrients
Micronutrients are small amounts of vitamins and minerals required by the body for most cellular functions. Novel research highlights the relevance of the consumption of seeds from the MS due to their high content and diversity of micronutrients (46).
5.1. Vitamins
Numerous studies have demonstrated that milpa crops present a wide diversity of vitamins (Table 3). Vitamin content and diversity depend strongly on the growth conditions, and it varies among the milpa seed species, i.e., corn: B4 > C > B3 > B5 > B6 > B1 > E > B2 > B9 > A > K; common beans: B4 > E > B3 > B1 > B5 > B6 > B2 > C > K > B9; and pumpkin seeds: B4 > B3 > C > E > B5 > B1 > B2 > B6 > B9 > K > A (16, 17, 47, 48).
Vitamin A or retinol is present in corn (9 μg/100 g), and pumpkin seeds (16 μg/100 g), reaching 1 and 1.8% of the retinol recommended dietary allowance (RDA), respectively. This vitamin is important for development and growth, as well as good vision and immune system maintenance (49). Vitamin A deficiency or hypovitaminosis A is related to growth and development disorders and increased susceptibility to severe infections, common in children and women of reproductive age from developing countries (50).
The vitamin B complex is a group of 8 vitamins; 6 are found in MS seeds, and all are related to cellular metabolism (51). Vitamin B1 or thiamine is reported in corn, common beans, and pumpkin seeds at 0.2–0.4, 0.9, and 0.3 mg/100 g, respectively, equivalent to 13.3–32.4% of RDA in corn, 73.3% in common bean and 22.5% in pumpkin. Thiamine (Vitamin B1) is an essential micro-nutriment. Its deficiency is related to the development of beriberi and Wernicke-Korsakoff syndromes, as well as behavioral circumstances in the nervous system (such as irritability, depression, poor memory, and ability to concentrate, lack of mental dexterity, palpitations at the cardiovascular level), and heart hypertrophy (52). Vitamin B2 or riboflavin was quantified in 0.01–0.02, 0.2–0.5, and 0.15–0.2 mg/100 g, respectively, for corn, common bean, and pumpkin seeds, representing an RDA for corn of 0.4–0.8%, 7.6–20% for common beans and 6–8% for pumpkin seeds. Riboflavin is necessary for the metabolism of lipids, carbohydrates, and proteins. Additionally, this vitamin participates in energy metabolism to maintain the integrity of the skin and mucous membranes (including the cornea) (53). Vitamin B3 or niacin has been reported in 1.8–3.6, 2.1–2.5, and 4.4–5.0 mg/100 g in corn, common beans, and pumpkin seeds, respectively. Niacin’s RDA for corn is 5.1–10.4%, 6–7.1% in common beans, and 12.6–14.3% in pumpkin seeds. Niacin contents in corn, common beans and pumpkin seeds represent 5.1–10.4%, 6–7.1% and 12.6–14.3% of the RDA, respectively. Niacin is an essential nutrient that acts as a coenzyme, forming nicotinamide adenine dinucleotide (NAD) and nicotinamide adenine dinucleotide phosphate (NADP), playing a key role in energy transfer reactions in the metabolism of glucose, fat, and alcohol (54). Vitamin B5, or pantothenic acid, is related to the oxidation of carbohydrates and fatty acids, synthesizing amino acids, fatty acids, ketone bodies, phospholipids, acetylcholine, and other neurotransmitters, steroid hormones, antibodies, and cholesterol (55, 56). Vitamin B6 or pyridoxine has been reported in corn, common beans, and pumpkin seeds in amounts of 0.09–0.6, 0.5, and 0.1–0.14 mg/100 g, respectively. Pyridoxine RDA is about 1.3 mg, corn provides 6.4–47.7%, while common beans give 37.7–38.5%, and pumpkin seeds offer 7.7–10.8%. This vitamin synthesizes neurotransmitters as a cofactor in enzymes related to amino acid synthesis (57). Vitamin B9 or folate in corn, common beans, and pumpkin seeds is quantified in 42, 4.1–4.6, and 57–58 μg/100 g, respectively, representing 10.5%, 1.04–1.16% and 14.3–14.5% of the RDA for this vitamin. Folate is a coenzyme in the synthesis of purine and pyrimidine nucleotides, and it is also involved in erythropoiesis (58).
Vitamin B4 or choline is estimated at 23 mg/100 g in corn, 99.4 mg/100 g in common beans, and 15.9 mg/100 g in pumpkin seeds. This vitamin is the most abundant of the three MS seeds. Its RDA is also high (200 mg), corn contributes 11.5%, common beans 49.7%, and pumpkin seeds 7.9%. Choline is an essential micro-nutrient in producing neurotransmitters, such as acetylcholine, and methyl donors, such as S-adenosylmethionine. Its deficiency is rare in humans but may produce muscle damage and non-alcoholic fatty liver disease (59).
Vitamin C or ascorbic acid is quantified in corn, common beans, and pumpkin seeds in 6.8, 0.01, and 0.3–1.9 mg/100 g, respectively, contributing to the RDA of vitamin C with the 9.1, 0.01%, and 0.4–1.9%. Ascorbic acid is a potent antioxidant and an essential micro-nutrient in humans. Play a key role in growth and development, such as in synthesizing collagen and carnitine. It also maintains endothelial integrity and lipoprotein metabolism. Its deficiency is related to scurvy (60).
Vitamin E or tocopherol has been reported in corn, common beans, and pumpkin seeds in amounts of 0.7, 2.5, and 1 mg/100 g, respectively, which represents the 0.5, 16.7, and 6.9%, respectively, of the tocopherol RDA. Tocopherol (α- and γ-tocopherol forms) is an essential micro-nutriment related to beneficial effects on the immune system: It also minimizes and delays the aging process, and benefits endothelial integrity, lipoprotein metabolism and protects against the development of cancer, dementia, and cardiovascular diseases. Its deficiencies cause neurological disorders due to poor conduction of nerve impulses, and also some individuals may have problems absorbing fats in the gastrointestinal tract (61).
Finally, vitamin K or phytonadione abundance in corn, common beans, and pumpkin seeds has been registered at 0.3, 10, and 17.7 μg/100 g, respectively. Its RDA is about 200 μg. Corn represents 0.3%, common beans contribute 8.2%, and pumpkin seeds 14.5%. Vitamin K acts as a cofactor for activating proteins related to liver coagulation factors, prothrombin, and factor X, among others. Its deficiency is associated with coagulation problems, calcium fixation difficulties, and arteriosclerosis (62).
5.2. Minerals
The ash of corn, common bean, and pumpkin seeds is constituted by various minerals (Table 3). The mineral content depends on several factors, such as genotype and environmental conditions during cultivation. The mineral profile is variable among the milpa triad (corn: K > P > Mg > Ca > Fe > Zn > Mn > Cu; common beans: Fe > Zn > K > P > Mg > Cu > Mn > Ca; pumpkin seeds: P > K > Mg > Ca > Zn > Fe > Mn > Cu) (11, 17, 47, 48, 63–68).
Potassium (K) is the most abundant mineral in corn (77.2–327.3 mg/100 g), the second more abundant in common beans (73.6–249 mg/100 g), and the third in pumpkin seeds (579–788 mg/100 g). Potassium main role in the body is to help maintain normal fluid levels inside the cells (69). Its RDA is 2,000 mg. Moderate consumption of MS seeds is enough to reach this amount (Table 3).
Phosphorous (P) is the most frequent mineral in pumpkin seeds (332–1,570 mg/100 g), while in corn (25–165.5 mg/100 g) is the second most important. Phosphorous has a key role in forming bones and teeth, in the metabolism of carbohydrates and fats, and in making processes of proteins for the growth, maintenance, and repair of cells and tissues (69). Its RDA is about 700 mg, meaning less than 50 g of pumpkin seed reaches the RDA of P.
Calcium (Ca) is present in lower amounts than K and P in the three seeds (corn: 3.2–64.7 mg/100 g; common beans: 0.1–99.8 mg/100 g; 15–52 mg/100 g). The consumption of moderated Ca is associated with keeping healthy bones and teeth, muscle contraction, nervous functions, and regulating normal heart rhythms and blood clotting (69). Calcium RDA is 800 mg, and its content in MS seeds will not exceed its permitted levels in a moderated consumption.
Magnesium (Mg) is a major element in pumpkin seeds with a content of 156–569 mg/100 g, while its content in corn is 20–141.3 mg/100 g and 10–74.6 mg/100 g in common beans. Magnesium (Mg) is an important element for regulating muscle and nerve functions, blood sugar levels, and blood pressure, as well as making proteins, bones, and DNA, assisting more than 300 enzyme reactions (69). Mg′s RDA is about 375 mg, which means that a moderated consumption of these seeds may provide the daily dose recommendation for adults.
Iron (Fe) is the major mineral in common beans, ranging from 183 to 1,207 mg/100 g, and 0.4–4.2 and 2.3–10.6 mg/100 g for corn and pumpkin seeds, respectively. The body needs Fe for hemoglobin and some hormone-making. Iron is also part of myoglobin and is important for healthy brain development and children’s growth (69). Moderate intake of common beans may provide more than the Fe′s RDA (14 mg), but corn and pumpkin seeds present a lower content.
Zinc (Zn) is an important element in common beans with a content of 41–624 mg/100 g, also in pumpkin seeds with 2.2–11.3 mg/100 g, but only contains 0.4–0.9 mg/100 g in corn. Zn is an important trace mineral necessary for almost 100 enzymes. It also participates in the DNA and protein synthesis, growth of cells, healing of damaged tissue, and supporting the proper functionating of the immune system (69). A portion of 100 g of corn does not exceed the RDA of Zn (10 mg). On the other hand, 100 g of common beans or pumpkin seeds exceed the RDA of this mineral.
Manganese (Mn) is a minor mineral in corn and pumpkin seeds, with 0.3–0.8 and 1.3–4.9 mg/100 g, respectively, but this element ranges from 2.1 to 263 mg/100 g in common beans. Mn is important for multiple body functions, such as carbohydrate metabolism, Ca absorption, regulating brain and nerve functions, forming connective tissue, sex hormones, bones, and blood clotting factors (69). Manganese RDA (2 mg) could be exceeded with common beans and pumpkin seeds.
Copper (Cu) is reported in common beans in a range of 4–140 mg/100 g, while in corn and pumpkin seeds, this represents a content of 0.1–0.2 and 0.4–1.5 mg/100 g, respectively. In nutritional terms, Cu is considered an essential trace mineral for its key role in assisting several enzymes and enzymatic systems related to the chemical energy production in the body and the breaking down and absorption of Fe. Also, Cu is involved in the red blood cells, collagen, connective tissue, and brain neurotransmitter building (69). The RDA for Cu is about 1 mg, which means that consumption of a few amounts of common bean easily meets the demand for this trace mineral.
Despite the diversity and content of minerals in corn, common beans and pumpkin seeds. To reach the RDA for those minerals, it is necessary to consider their chemical properties, bioaccessibility, and bioavailability to fulfill their bioactive and nutritional potential.
The MS has a great diversity of high-quality macro- and micro-nutrients. Together, the cultivation and consumption of these three seeds could complement the nutritional limitations in amino acid, vitamin, and mineral profiles. They provide, in combination, a good balance of protein, fat, carbohydrates, vitamins, and minerals in quantities that contribute to meeting the daily RDAs (2, 3).
5.3. Bioactive compounds
The three seeds part of the Mesoamerican triad are also known to contain a wide diversity of bioactive components, such as polyphenols, phytosterols, saponins, fiber, bioactive peptides, and carotenoids (Table 4) (70). Many of these components are synthesized by plants mainly as defense and adaptation mechanisms against environmental conditions. Also, form part of macronutrients, including complex carbohydrates (resistant starch, cellulose, fiber, etc.) and protein-derived peptides (from dipeptides to polypeptides) (71). On the other hand, there are anti-nutritional components that could exert health benefits. In adequate concentrations and suitable conditions of bioaccessibility and bioavailability, the anti-nutritional components found in MS seeds could be considered bioactive compounds with beneficial health effects galactooligosaccharides, phytic acid, tannins, and enzyme inhibitors, among others (71, 72).
5.3.1. Phenolic compounds
Phenolic compounds or polyphenols are organic compounds whose molecular structures contain at least one aromatic ring attached to a hydroxyl group (phenol) group (73). They are the result of the secondary metabolism of plants in response to environmental stimuli (73). It is known that these types of compounds are present in corn, common beans, and pumpkin seeds (74–76). The main phenolics in these seeds are hydroxybenzoic acids: gallic, vanillic, and protocatechuic acids; hydroxycinnamic acids: coumaric, caffeic, and ferulic acids anthocyanins: glycosylated and acylated forms of delphinidin, petunidin, cyanidin, malvidin, pelargonidin, and peonidin; flavonols: quercetin, myricetin, naringenin, catechin, hesperidin, and kaempferol; and isoflavonoids: daidzein and genistein (74–76).
Free and bounded derivatives from cinnamic and benzoic acids are easily found in milpa seeds. Bounded phenolic compounds can be released by acid, alkali, and enzyme hydrolysis from seed bran, endosperm, and coat (77). Phenolic compounds are the main bioactive compounds in corn. Their content varies between 1,377–1,421 mg GAE/100 g, including gallic acid, coumarin, quercetin, catechin, kaempferol, and anthocyanins, mainly in colored varieties. While in common beans, phenolic compounds concentrations range from 600 to 2,624 mg GAE/100 g, conformed by gallic acid, coumarin, quercetin, catechin, kaempferol, and many others. Besides, in pumpkin seeds, phenolic compounds concentration ranged from 31.9–224.6 mg GAE/100 g (Table 4) (12, 16, 30, 37, 78–87).
The role of polyphenols from Mesoamerican triad seeds on human health has been thoroughly investigated individually. Research has shown that the polyphenols in these seeds can scavenge free radicals found in cells and tissues by donating electrons or a single proton in oxidation–reduction reactions. Still, they can chelate ionic metals that could harm cell structures and other biological molecules (73, 88). Additionally, phenols can inhibit the lipid peroxidation of cell membranes from converting them into stable compounds. Moreover, polyphenols could modulate enzymes involved in the antioxidant process, i.e., by induction of the expression of Nfr2 to produce antioxidant molecules such as superoxide dismutase (SOD), Catalase (CAT), glutathione peroxidase (GPx) and glutathione reductase (GR) (84). In this way, polyphenols could, directly and indirectly, neutralize oxidizing agents in the human body. Phenolic compounds have also been shown to participate actively in other physiological processes. These molecules can decrease the oxidative state by reducing proinflammatory molecules, and controlling signaling pathways, including inflammatory cytokines such as interleukins 1, 6, 8, growth factors such as TNF-α and Interferon-γ, the latter indicating that the consumption of these compounds has an antiinflammatory potential (89).
5.3.2. Carotenoids
Carotenoids are organic pigments responsible for providing orange and red coloration to vegetables. The general structure of the carotenoid is a polyene chain consisting of 9–11 double bonds (possibly ending in rings). This conjugated double-bond structure leads to a high reducing potential, or the ability to transfer electrons through the molecule (90). Carotenoids such as lutein, zeaxanthin, astaxanthin, or lycopene could be found in the milpa triad seeds (91, 92). Carotenoids found in corn are present in concentrations of 9.2–19.8 mg/100 g, mainly zeaxanthin, α-cryptoxanthin, lutein, and β-carotene. Common beans carotenoids concentration ranged from 0.2 to 9.2 mg/100 g, primarily β-carotene. At the same time, pumpkin seeds carotenoids are found at a concentration of 7–35.2 mg/100 g, mainly β-carotene and cryptoxanthin (Table 4) (12, 16, 27, 31, 78–83, 85–87, 93, 94).
Similar to polyphenols, carotenoids function as electron donors to reactive species due to their structure and the delocalization of electrons along their chain, presenting high antioxidant potential. The hypocholesterolemic potential of carotenoids has been related to their ability to inhibit the oxidation of LDL cholesterol and the blockage of the enzyme HMG-CoA reductase, which is involved in cholesterol synthesis (Table 4) (95).
5.3.3. Saponins
Saponins are glycosides of steroids or triterpenoids, made up of a lipid-soluble element (the steroid or triterpenoid) and a water-soluble component (sugar) (96). Saponins are found in plants; their name is due to their ability to form foams. It is known that corn, common bean, and pumpkin seeds contain triterpene-type saponins derived from mevalonic, betulinic, and olean acids, which can form various glycosides depending on the union with sugars. The saponins are reported in amounts of 0.9–6.2, 44–148, and 5–7.4 mg/100 g in corn, common beans, and pumpkin seeds, respectively (Table 4) (27, 31, 37, 78–83, 85, 86).
Saponins have multiple benefits, including inhibiting lipases, which prevent the absorption of lipids in the intestinal lumen. This function has been related to the decrease in cholesterol and triglyceride levels. Besides, saponins have been associated with reducing adipogenesis by regulating the AMPK pathway, increasing AMPK phosphorylation, and activating peroxisome proliferator-activated receptors (PPARs) and sterol regulatory element-binding proteins (SREBP). Resulting in decreasing the accumulation of triglycerides and preventing the growth of preadipocytes (Table 5) (97–101).
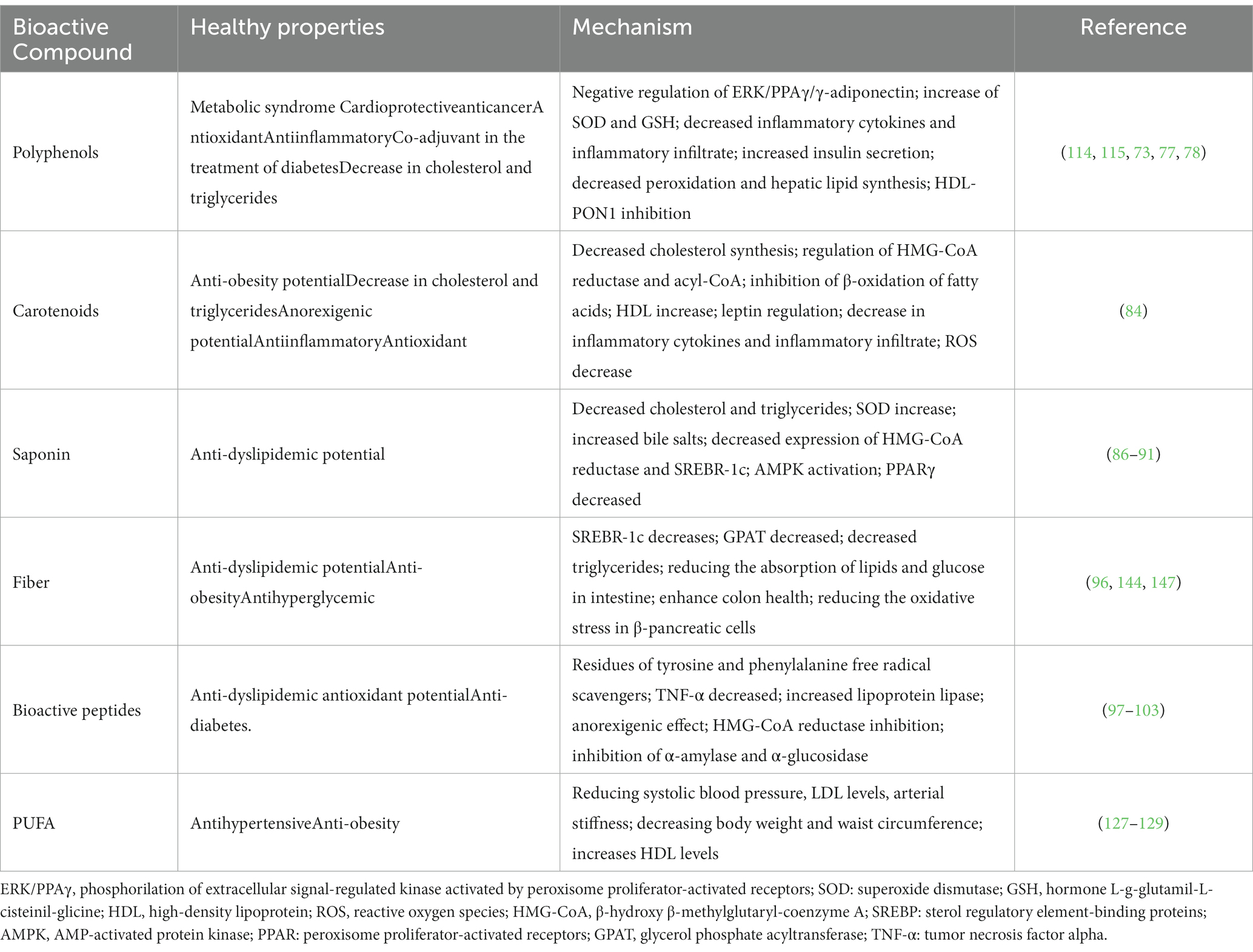
Table 5. In vivo and clinical healthy properties of some bioactive compounds present in the seeds of the milpa triad system.
5.3.4. Phytosterols
Phytosterols are natural sterols present in fruits, seeds, and leaves. Structurally, they share characteristics with cholesterol. Phytosterols’ structure is a fused polycyclic molecule composed of high variable carbon side chains and/or the presence or absence of a saturation (double bond) (102). These compounds have been reported in some oilseeds, cereals, and legumes, such as soybean, beans, and corn, as well as in Cucurbitacea seeds (16, 103). The total phytosterol content in corn, common beans, and pumpkin seeds have been estimated at 112.4–362.1, 242–350, and 782.1–805.2 mg/100 g, respectively (Table 4) (16, 27, 31, 37, 78–83, 85–87, 93).
Principal phytosterols are α-sitosterol, campesterol, and stigmasterol. These represent approximately 98% of these molecules. The consumption of phytosterols has been related to reducing cholesterol in the blood. This effect can be connected to the similarity between both molecules. The reduction is due to the competition for the absorption of dietary cholesterol in the intestinal lumen, reducing transport to the liver and preventing its metabolism (104).
5.3.5. Fiber
Dietary fiber is the edible part of plants or analogous fibers resistant to digestion and absorption in the small intestine, with partial or complete fermentation in the large intestine. Dietary fiber includes polysaccharides, oligosaccharides, and lignin (105, 106). It is known that corn contains high amounts of insoluble fiber, mainly due to the presence of cellulose and hemicellulose, starch, and low soluble fiber content. On the other hand, common beans and pumpkin seeds present high soluble and insoluble fiber contents (13, 15, 17).
Dietary fiber has been related to cardioprotection due to its ability to reduce the absorption of lipids in the gastrointestinal tract, reduce appetite and promote satiety in individuals. Moreover, the intestinal microbiota uses polysaccharides as a substrate, generating short-chain fatty acids (propionate, butyrate, acetate) as metabolites. These short-chain fatty acids are absorbed in the colon and metabolized in the liver, preventing hepatic cholesterol synthesis and lowering blood cholesterol levels (Table 5) (106).
5.3.6. Bioactive peptides
Bioactive peptides are obtained from dietary proteins or derivatives of precursor proteins. Several peptides with biological potential related to the modulation of markers of diseases such as type 2 diabetes, hypertension, oxidative stress, and inflammation have been studied in common beans. Also, bioactive corn peptides exert antiinflammatory, antioxidant, hepatoprotective, antihypertensive, and antimicrobial potential (107).
Moreover, the health benefits of bioactive peptides from the milpa triad seeds are associated with multiple mechanisms of action. These mechanisms include the modulation of inflammatory cytokines, enzymatic inhibitions such as HMG-CoA reductase, and increased expression of proteins, such as lipoprotein lipase, as well as increased bile salt excretion (Table 5) (108–110).
Díaz-Gómez et al. (111) reported that zein-derived peptides from corn (≤5 kDa) demonstrated antioxidant (against HepG2 and Caco2 cells exposed to high oxidative stress), antihypertensive (in spontaneously hypertensive rats), hepatoprotective (in rats with liver damage induced by exposure to lipopolysaccharides from Bacillus Calmette-Guérin), anticancer (on HepG2 cells and H22-tumor bearing mice model) and antimicrobial potential (on Caenorhabditis elegans in an in vivo study). On the other hand, common bean bioactive peptides (LVTTTVDL, QTSTPLFS, VELVGPK, and TRGVLG) biological potential was related to reducing the inflammatory process, inhibition of dipeptidyl peptidase-4 (DPP-IV) and angiotensin-converting-enzyme inhibitor (ACE), and promoting glucose uptake (112). In a recent report of several edible Cucurbitaceae species, it was reported the in vitro antioxidant, antiinflammatory, α-amylase inhibitory potential of some bioactive peptides (<15 kDa) (113). However, more studies on pumpkin protein hydrolyzates are needed to investigate their biological potential.
5.3.7. Fatty acids
A fatty acid is a carboxylic acid joined to an aliphatic chain, which could be saturated or unsaturated. Fatty acids are the basic units of fat in the human body and foods. Milpa seeds have a diverse profile of saturated and unsaturated fatty acids. The lipid profile of corn, common beans, and pumpkin seeds are composed of at least 16, 21, and 9 different fatty acids, respectively (Table 6) (114–124).
Linoleic (C18:2, ω-6), a polyunsaturated omega-6 fatty acid, Oleic (C18:1) and linolenic acid (C18:3) are poly unsaturated fatty acids (PUFA) in corn, common beans, and pumpkin seeds with proportions of 54.5–59.41%, 24.1–67.0%, and 43.68–52.61%, respectively (114–124). Oleic acid (C18:1), another PUFA, was the second fatty acid more abundant in corn and pumpkin seeds with values of 23.74–30.83% and 18.14–42.07%, respectively, while linolenic acid (C18:3), another PUFA, has a content of 6.24–46.0% in common bean. Palmitic acid (C:16), a saturated fatty acid (SFA), was reported as the third most abundant in the three seeds, with contents of 10.32–12.0%, 5.0–13.9%, and 10.21–16.01%for corn, common bean, and pumpkin seed, respectively. In total, more than 70% of the lipid profile of the three seeds is made up of PUFA.
Fatty acids, especially PUFA, positively impact human health and development (125). The evidence shows a strong role in preventing and treating some chronic degenerative diseases. PUFA present in corn and pumpkin seed oils have demonstrated antihypertensive effect in clinical trials (126, 127). At the same time, the fatty acids from pumpkin seeds also have shown antiobesity effects in human trials (128).
Milpa triad seeds bioactive compounds (polyphenols, carotenoids, saponins, phytosterols, or proteins/peptides) have demonstrated individually high potential to modulate molecular markers related to noncommunicable diseases (16, 129, 130). However, there are still research opportunities in order to understand the biological potential of the milpa seeds. The opportunities include the validation of the mechanisms of action observed in biochemical and in vitro assays compared to preclinical and clinical trials and the evaluation of the potential synergistic effect of milpa seeds on health when grown and consumed together. However, preclinical and clinical evaluations using extracts, purified and isolated compounds, or groups of compounds from these seeds provide important information related to the potential of milpa seeds as functional ingredients in the treatments or control of noncommunicable diseases.
5.4. Milpa system seeds health properties in preclinical and clinical trials
Growing evidence highlights the role of a balanced diet in reducing risk factors for developing noncommunicable diseases such as cardiovascular diseases, type 2 diabetes, obesity, cancer, and inflammatory conditions (131). Recent studies have reported the health benefits of MS seeds consumption (Table 7; Figure 2).
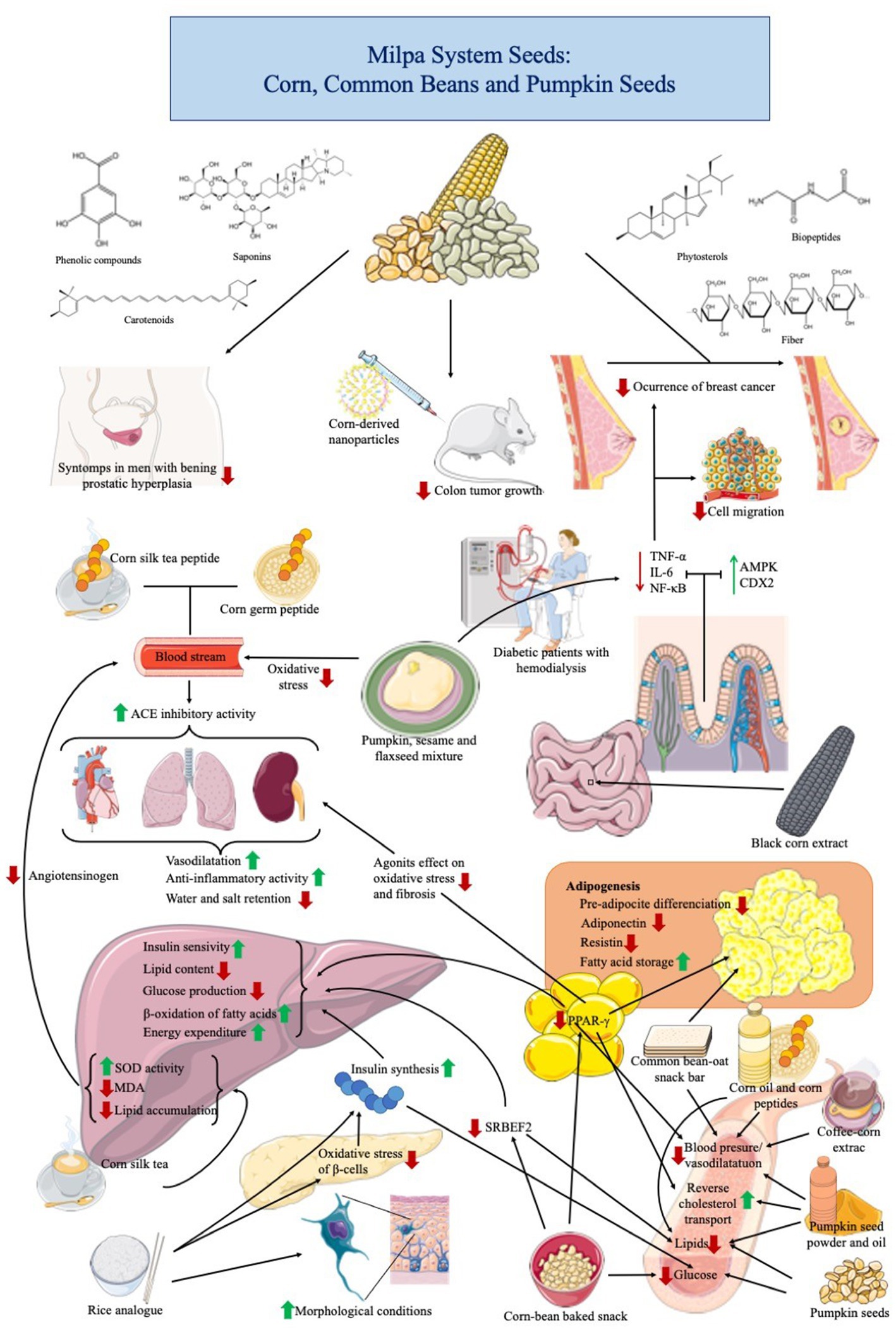
Figure 2. Health properties of milpa system grains in preclinical and clinical trials on chronic-degenerative diseases.
5.4.1. Antihypertensive properties
Cardiovascular diseases are the lead cause of death globally, with >17.9 million per year, an estimated 32% of all deaths worldwide (132). These are a group of heart and vascular tissue disorders and are strongly related to behavioral risk factors such as physical inactivity, tobacco, and alcohol use, as well as an unhealthy diet (132). Antihypertensive potential of milpa seeds has been evaluated by several authors through in vivo and clinical trials. In a preclinical trial, Sugiyanta et al. (133) observed a reduction in the diastolic blood pressure (79.87 mmHg) in induced hypertensive mice (by deoxycorticosterone acetate) supplemented with an aqueous extract of coffee:corn (50:50). The antihypertensive effect of the mix was compared through the concentration of F2-isoprostane, a stable end product of lipid peroxidation present in tissues and biological fluids, whose levels were negatively correlated to the blood pressure reduction (414.33 pg./mL). Authors attribute this effect to ferulic acid, a potent antioxidant compound commonly found in coffee and corn, and as a degradation product of other phenolic compounds such as flavonoids and tannins (134).
In a study selected from the Brisighella Heart Study (a population-based longitudinal epidemiological investigation initiated in 1972 in Bisighella, Italy, still active) cohort subjects who were not treated with antihypertensive drugs and reported with certainty their daily mean intake of dietary fats in cooking and seasoning, the patients who used corn oil as the main source of dietary oil presented low blood pressure (systolic 134.8 mmHg, 72.5 mmHg), arterial stiffness (94.8 mmHg), and cholesterolemia (total cholesterol 212.4 mg/dL, LDL-C 138.6 mg/dL, HDL-C 50.1 mg/dL, triglycerides 110.9 mg/dL). All these values were lower when compared to several vegetable and animal oil sources, except extra-virgin olive oil (126). The high content of monounsaturated and PUFA in corn oil are the responsible compounds for these effects.
In an in vivo experiment, Guo et al. (135) found that a high-dose corn germ peptide (1,000 mg/kg body weight) significantly reduced the systolic blood pressure by acute oral (10.12%) and long-term intragastric (15.89%) administration tests. In a consecutive study, the corn germ peptide also inhibited the ACE activity in the kidney (22.28%), lung (24.53%), and heart (12.93%) of rats. It regulated the level of endothelium-derived vasoconstrictor and relaxing factors in serum (136). A biopeptide identified by bioinformatic tools (SKFDNLYGCR, 1,258 Da), found in corn silk tea, reduced systolic blood pressure levels (36.78 mmHg) in spontaneously hypertensive rats and inhibited the ACE activity (IC50 44.11 μM). Molecular docking analysis showed that this peptide directly interacted with ACE residues (Asn277, Gln281, Thr282, Thr302, His353, Asn374, His513, Ser516, Ser517, and Tyr523) (137). However, further studies are required to validate these in silico outcomes.
Ribeiro et al. (138) described a dose-dependent antihypertensive effect in spontaneously hypertensive Wistar rats treated with common bean protein extract (<3 kDa). Which was related to the presence of short peptide sequences related to cardioprotective activities (i.e., VSE, EV, AVT, DF, ELL, SF, AF, VLL, PLL, and GF). The main activity of these peptides was directly related to vasorelaxation and reducing arterial pressure and resistance in aortic vascular beds. In another study, a fraction of 3–10 kDa of Alcalase common bean hydrolysate showed in vivo antihypertensive potential in naturally hypertensive rats after 2 h of 4 mg/kg intraperitoneal administration (139).
Moreover, a common bean-baked snack consumed by people with overweight or abnormal blood lipid levels reduced apolipoprotein B-100 levels (56.6 mg/dL) (140). The consumption of the snack did not affect other blood indicators, such as lipids or glucose (p > 0.05). The authors did not attribute this effect to any particular compound.
In a study by Majid et al. (127), the effect of pumpkin seed oil on total cholesterol was evaluated. 1,000 mg per day during 3 months showed a significant reduction in the final values of LDL and diastolic blood pressure along with an increase in HDL. These results suggest a hypolipidemic and antihypertensive potential of pumpkin seeds due to the content of unsaturated fatty acids and plant sterols.
The antihypertensive effect of milpa seeds is related to different compounds (mainly phenolic compounds, bioactive peptides, and fatty acids). Vasomodulatory effect could be one of the principal pathways of the antihypertensive potential of the MS seed bioactive components. Principally related to the potential of these molecules to block oxidative systems and modulate enzymes related to vasoconstriction and vasorelaxation. These preclinical and clinical assays show evidence of the potential of the milpa seeds for cardioprotective benefits.
5.4.2. Antihyperglycemic and antiobesity properties
Diabetes is a chronic-metabolic disease characterized by elevated glucose levels in the blood (141). Over 420 million people globally live with diabetes and about 1.5 million deaths are attributed to this disease each year (142). Type 2 diabetes (T2D), the most common, is characterized by insulin resistance and low or null production of insulin (141). Obesity is characterized by a body mass index above 30 kg/m2 in adults (142). A healthy lifestyle and a balanced diet are effective strategies to prevent and delay the development of obesity and T2D.
Milpa seeds have the potential to modulate biological markers related to obesity and T2D. In a 14-day in vivo assay, type 2 diabetes-induced male and female rats were fed with rice analog (formulated with mocaf, corn, pigeon pea, and seaweed, 71:21:7:1, w/w/w/w) and showed better morphological characteristics in islets of Langerhans and an increase in insulin production, since rice analog presented a low glycemic index (47.36) compared with the control diet, based in commercial Broiler-1 product (66.35) (143). Additionally, serum glucose levels decreased in male rats up to 60.79 mg/dL, which was lower compared to female rats (88.98 mg/dL). Authors found that resistant starch and fiber contained in rice analogs might release bioactive compounds during digestion, which could reduce oxidative stress in β-pancreatic cells of diabetic rats (143).
Corn silk extract showed antidiabetic potential in a high-fat diet/streptozotocin-induced rat model (144). After 4-weeks, the treatment effectively prevented the weight loss of diabetic mice and showed a significant reduction in fasting blood glucose and enhanced glucose tolerance. Also, hyperlipidemia was alleviated since total cholesterol, triglycerides, and low-density lipoprotein-C (LDL-C) values decreased and, at the same time, HDL-C increased (high-density lipoprotein). Moreover, the oxidative stress was reduced by decreased malondialdehyde and elevated SOD activity. Besides, hepatic lipid accumulation decreased and prevented liver tissue morphological change (144).
Domínguez-Uscanga et al. (145) reported the beneficial effect of baked snack with 70% corn and 30% of common beans in the reduction of lipid serum by the inhibition of PPAR-γ and SREBP2 in a high-fat diet murine model. The study suggests the reduction of obesity, dyslipidemia, and non-alcoholic fatty liver. In another preclinical study, Gomes et al. (146) showed that the administration of common bean flour at a dose of 346 g per day improves colonic health and the microbiome compared to the high-fat diet-fed group. Results suggest that a diet high in fiber and bioactive compounds from MS seeds could be a strategy to improve consumers’ health.
A clinical trial reported by Escobedo et al. (140) shows the reduction of apolipoprotein b-100 blood levels after a daily 32 g consumption of common bean baked snack. This reduction could have a preventive effect on developing dyslipidemia. Similar to previous reports, Ramírez-Jiménez et al. (147) elaborated a snack bar with common beans and oats to reduce hypertriglyceridemia markers in Mexican women. They found a reduction of triglycerides and glucose by inhibiting adipogenesis after consuming the snack bar.
On the other hand, pumpkin seeds showed antihyperglycemic and antihyperlipidemic effects in albino rats (148). A significant decrease in blood glucose level (128.33 mg/dL), total plasma cholesterol (88.43 mg/dL), triglycerides (69.79 mg/dL), and low-density lipoprotein cholesterol (21.45 mg/dL) were found in the rat groups fed with 15 g pumpkin seed powder. Authors conclude that pumpkin seeds have a high potential to be used in the human diet to manage noncommunicable diseases such as diabetes and hypercholesterolemia. In another study, Indian women (aged 30–50 years) diagnosed with metabolic syndrome received 5 g of pumpkin seeds for 60 days (128). Anthropometric measurements (body weight 72.20 kg, waist circumference 90.62 cm), biochemical parameters (HDL cholesterol 42.24 mg/dL, non-HDL cholesterol 142.05 mg/dL, LDL cholesterol 120.22 mg/dL,), and systolic blood pressure (122 mm Hg) showed statistically significant improvement (p < 0.05) compared to control group (patients without pumpkin seed). The authors found some bioactive compounds (fatty acids, propyl piperidine, and benzene derivatives) in pumpkin seeds with antidiabetic and antiobesity potential effects.
Milpa system antidiabetes and antiobesity potentials have been associated with their bioactive components. Several molecular pathways against these diseases indicated that milpa seeds could reduce glucose levels and lipid accumulation. Including milpa seeds in the diet could protect insulin-producing cells from oxidative stress, activate endogenous molecular regulators of lipids in serum, prevent the development of dyslipidemia, and regulate adipogenesis, among other molecular mechanisms of action.
5.4.3. Anticancer properties
Cancer is a group of diseases that can appear in any part of the body (149). This is characterized by the abnormal and rapid growth of altered cells that may adjoin other tissues and organs (metastasis). In 2020, cancer represented >10 million deaths worldwide. Some of its causes are related to tobacco and alcohol consumption, lack of physical activity, and low plant-based food intake (149). Some research related to the anticancer effect of milpa seed products has been conducted. A recent study demonstrated that regular consumption of corn, legumes, and vegetables was negatively related to the occurrence of breast cancer in postmenopausal women from Northern Mexico. In contrast with women of the same region and biological condition, with a regular diet based mainly on red and processed meats and foods high in fats and sugars (150).
In tumor-bearing mice (151), corn-derived nanoparticles (covered with polyethylene glycol) exhibited significantly higher serum concentrations and lower liver accumulation compared to not-covered corn-derived nanoparticles. Corn-derived nanoparticles were accumulated in the colon of mice, delaying tumor growth without hepatoxicity or nephrotoxicity. In a study performed by Leibbrand et al. (152), the reduction of prostatic hyperplasia symptoms in men using non-fat pumpkin seed extract was evaluated. These subjects were administered for 3 months a dose of 500 mg/day, equivalent to 10 g of seed, showing a decrease in symptoms between 8 and 12 weeks after the intervention. Dietary inclusion of milpa seed could be an effective alternative in preventing cancer. Preclinical and clinical evaluations are scarce; however, innovative treatments based on nanomedicine and nanopharmacology, which use isolated compounds from milpa seeds, have shown promising results.
5.4.4. Antiinflammatory properties
The inflammatory response is a mechanism of the body that activates the immunological system to react against external agents or an injury (153). Some disorders in the activation of the inflammatory response are related to the development of chronic diseases (153). In many cases, lifestyle factors, including diet, could promote antiinflammatory effects. A soluble extract from black corn showed antiinflammatory potential in an animal model (154). This effect was determined by down-regulating the gene expression of tumor TNF-α, interleukin-6, and NF-κB. The extract increased the Goblet cell size and number in the intestine villi and Paneth cell number in the crypt. The epithelial physical barrier was strengthened by up-regulating intestinal biomarkers AMP-activated protein kinase (AMPK) and caudal-related homeobox transcriptional factor 2 (CDX2). The authors concluded that this extract promotes intestinal antiinflammatory responses and enhances intestinal barrier function.
A mixture of seeds, including pumpkin, sesame, and flaxseed, was administered in hemodialysis patients to evaluate the antiinflammatory potential and the decrease in markers related to hypertriglyceridemia and diabetes. Results showed a decrease in the levels of TNF-α, interleukin-6, c-reactive protein, triglycerides, glucose, and insulin. These results suggest that the intake of PUFA in these seeds favors the improvement of the oxidant state and a hypotriglyceridemic effect (155). A study showed that pumpkin seed oil-loaded niosomes displayed a decrement in in vivo hair loss of 44.42%, representing 1.4-fold higher than commercial treatments (156). Authors also evaluated the cellular antiinflammatory activity of the experimental material, finding that functionalized niosomes inhibited 5α-reductase activity and hindered IL-6 activity in DU-145 and RAW 264.7 cellular models, respectively (156). Results indicate the great potential of pumpkin seed oil to reduce hair loss induced by inflammation.
Soluble compounds from milpa seeds may modulate the immune response by regulating gene expression and activating endogenous antiinflammatory mechanisms. At the same time, it is possible to identify other effects related to noncommunicable diseases when patients consume milpa seeds. Indicating that the intake of these seeds may exert multi-beneficial effects against chronic diseases.
In vivo, preclinical, and clinical trial studies reveal an increasing interest in milpa seeds as a source of macronutrients, micronutrients, and bioactive components for nutrition and health. Different phytochemical and bioactive components in the MS seeds could exert antihypertensive, antidiabetic, antiobesity, anticancer, and antiinflammatory potential. Evidence shows that milpa seeds can improve health through several metabolic pathways. However, most of the studies have been performed on the seeds separately or in combination with two seeds or with other foods.
Preclinical and clinical trials evaluating the combination of the three seeds to assess the possible synergistic effects on health are needed. Several molecular markers related to noncommunicable diseases have been associated with milpa seed bioactive components. Including reduction of blood pressure/vasoconstriction, reduction of glucose and lipid levels, modulation of the expression of fat storage/metabolism biomarkers, reducing oxidative stress, enhancing the expression of tumor suppressor genes, and downregulating cancer markers, among many others. Nanomedicine, in silico simulations, omics (epigenomic, genomic, transcriptomic, proteomic, etc.), and human trials are little-explored strategies to validate the milpa seeds potential effects to prevent and treat deficiencies in nutrition and chronic-degenerative diseases.
6. Conclusion
The milpa system has socioenvironmental, economic, cultural, nutritional, and healthy implications that must be deeply studied. There are several benefits that the MS presents for small farmers, especially related to the diversity of foods that could be obtained from relatively small spaces. The combined consumption of milpa seeds could help to meet macro- and micro-nutrient requirements and provide important phytochemicals and bioactive components with beneficial effects for human health.
However, there is limited information related to the effect of the combined cultivation of corn, common beans, and pumpkin seeds on their nutritional and phytochemical composition. Moreover, consuming these seeds in combination could exert health benefits beyond the nutritional requirements. The consumption of combined milpa triad system seed could promote a synergistic effect in the prevention or adjuvants in the treatment of noncommunicable diseases. However, comprehensive and well-designed studies are needed to validate the health benefits of the combined consumption of milpa triad seeds as health promoters. Novel and innovative explorations in biomedicine, nanotechnology, nutrigenomics, and other recent omics fields could be used to guide these multidisciplinary investigations and support the biological potential of this ancient agro-productive system.
Author contributions
LM, EV-T, and DL-V: conceptualization. LM, EV-T, and JC: methodology. LM, JC, DL-V, NM-H, EV-T, and JF-M: validation. OS-V: formal analysis. EV-T, LM, and OS-V: writing–original draft. OS-V, DL-V, NM-H, JC, EV-T, JF-M, and LM: writing–review and editing. All authors contributed to the article and approved the submitted version.
Funding
This project was supported by seed funding from “The Institute for Obesity Research, Tecnologico de Monterrey”. CONAHCYT postdoctoral scholarship No. 504305 and doctoral scholarships No. 717366 and No. 898594.
Publisher’s note
All claims expressed in this article are solely those of the authors and do not necessarily represent those of their affiliated organizations, or those of the publisher, the editors and the reviewers. Any product that may be evaluated in this article, or claim that may be made by its manufacturer, is not guaranteed or endorsed by the publisher.
Conflict of interest
The authors declare that the research was conducted in the absence of any commercial or financial relationships that could be construed as a potential conflict of interest.
Footnotes
2. ^https://pubmed.ncbi.nlm.nih.gov/
4. ^https://scholar.google.com/
References
1. Comisión Nacional para el Conocimiento y Uso de la Biodiversidad . (2016). Available at: https://www.biodiversidad.gob.mx/diversidad/sistemas-productivos/milpa. (Accessed November 25, 2022).
2. Sánchez-Morales, P , and Romero-Arenas, O . El Sistema Milpa y la producción de maíz en la agricultura campesina e indígena de Tlaxcala. (2017).
3. Zizumbo-Villarreal, D , and Colunga-GarcíaMarín, P . La milpa del occidente de Mesoamérica: profundidad histórica, dinámica evolutiva y rutas de dispersión a Suramérica. Rev Geogr Agríc. (2017) 58:33–46. doi: 10.5154/r.rga.2017.58.001
4. Almaguer González, JA , García Ramírez, HJ , Vargas Vite, V , and Padilla Mirazo, M . Fortalecimiento de la salud con comida, ejercicios y buen humor: La dieta de la Milpa modelo de alimentación mesoamericana saludable y culturalmente pertinente. In: Fortalecimiento de la salud con comida, ejercicios y buen humor: La dieta de la Milpa modelo de alimentación mesoamericana saludable y culturalmente pertinente. pp. 67–67. (2018).
5. Casares, G , Cantón, R , Duch, C , Antochiw, J , and Zavala, S . Yucatán en el Tiempo. Mérida, Yucatán: Inversiones Cares, pp. 23–35. (1998).
6. Ebel, R , Pozas-Cárdenas, JG , Soria-Miranda, F , and Cruz-González, J . Manejo orgánico de la milpa: rendimiento de maíz, frijol y calabaza en monocultivo y policultivo. Terra Latinoam. (2017) 35:149–60. doi: 10.28940/terra.v35i2.166
7. Gouveia, CS , Freitas, G , Brito, JHD , Slaski, JJ , and Carvalho, MÂ . Nutritional and mineral variability in 52 accessions of common bean varieties (<i>Phaseolus vulgaris</i> L.) from Madeira Island. Agric Sci. (2014) 05:317–29. doi: 10.4236/as.2014.54034
8. Martín-Castillo, M . Milpa y capitalismo: opciones Para los campesinos mayas yucatecos contemporáneos. LiminaR. (2016) 14:101–14. doi: 10.29043/liminar.v14i2.463
10. Avalos-Rangel, MA , Campbell, DE , Reyes-López, D , Rueda-Luna, R , Munguía-Pérez, R , and Huerta-Lara, M . The environmental-economic performance of a poblano family milpa system: an emergy evaluation. Sustainability. (2021) 2021:9425. doi: 10.3390/su13169425
11. Novotny, IP , Tittonell, P , Fuentes-Ponce, MH , López-Ridaura, S , and Rossing, WAH . The importance of the traditional milpa in food security and nutritional self-sufficiency in the highlands of Oaxaca, Mexico. PLoS One. (2021) 16:e0246281. doi: 10.1371/journal.pone.0246281
12. Rodríguez-Salinas, PA , Zavala-García, F , Urías-Orona, V , Muy-Rangel, D , Heredia, JB , and Niño-Medina, G . Chromatic, nutritional and Nutraceutical properties of pigmented native maize (Zea mays L.) genotypes from the northeast of Mexico. Arab J Sci Eng. (2020) 45:95–112. doi: 10.1007/s13369-019-04086-0
13. Urango, L . Revistas UdeA, pp. 185–209. (2018). Available at: https://www.file:///c:/users/edi/downloads/336229-textodelcapítulo-161342-1-10-20181031.pdf.
14. Mojica, L , and González-De Mejía, E . Optimization of enzymatic produc-tion of anti-diabetic peptides from black bean (Phaseolus vulgaris L.)proteins, their characterization and biological potential. Food Func. (2016) 7:713–27. doi: 10.1039/c5fo01204j
15. Valenciano-Fernández, AF , and Chávez-Sánchez, E . Estudio de las propiedades físico-químicas y calidad nutricional en distintas variedades de frijol consumidas en México. Nova Scientia. (2017) 9:133–48. doi: 10.21640/ns.v9i18.763
16. Dotto, JM , and Chacha, JS . The potential of pumpkin seeds as a functional food ingredient: A review. Sci Afr. (2020) 10:e00575. doi: 10.1016/j.sciaf.2020.e00575
17. Syed, QA . Nutritional and therapeutic importance of the pumpkin seeds. Biomed J Sci Tech Res. (2019) 21:15798–803. doi: 10.26717/bjstr.2019.21.003586
18. Hertzler, SR , Lieblein-Boff, JC , Weiler, M , and Allgeier, C . Plant proteins: assessing their nutritional quality and effects on health and physical function. Nutrients. (2020) 12:3704. doi: 10.3390/nu12123704
19. Food and Agriculture Organization . WHO technical report series 724. In energy and protein requirements. Geneva, Switzerland: World Health Organization. (1985).
20. Amza, T , Balla, A , Tounkara, F , Man, L , and Zhou, HM . Document details - Effect of hydrolysis time on nutritional, functional and antioxidant properties of protein hydrolysates prepared from gingerbread plum (Neocarya macrophylla) seeds. Int Food Res J. (2013) 20:2081–90. doi: 10.1016/j.foodres.2011.06.029
21. Samaei, SP , Ghorbani, M , Tagliazucchi, D , Martini, S , Gotti, R , Themelis, T, et al. Functional, nutritional, antioxidant, sensory properties and comparative peptidomic profile of faba bean (Vicia faba, L.) seed protein hydrolysates and fortified apple juice. Food Chem. (2020) 330:127120. doi: 10.1016/j.foodchem.2020.127120
22. Gómez-Betancur, LM , Márquez-Girón, SM , and Restrepo-Betancur, LF . The milpa as a agricultural conversión alternative of conventional agroecological systems of bean (Phaseolus vulgaris), in the municipality of Carmen viboral, Colombia. Idesia (Arica). (2018) 36:123–31. doi: 10.4067/s0718-34292018000100123
23. Morales, PS . Evaluación de la sustentabilidad del sistema milpa en el estado de Tlaxcala, México. Rev El Colegio San Luis. (2018) 8:107–34.
24. Vásquez González, AY , Chávez Mejía, C , Herrera Tapia, F , and Carreño Meléndez, F . Milpa y seguridad alimentaria: El caso de San Pedro El Alto, México. Rev Cienc Soc. (2018) 24:24–36. doi: 10.31876/rcs.v24i2.24817
25. Razo, A , and Mendoza, D . Más del 50 por ciento del consumo de alimentos en México depende del exterior UNAM Global. (2021). https://unamglobal.unam.mx/mas-del-50-por-ciento-del-consumo-de-alimentos-en-mexico-depende-del-exterior/.
26. Adeniyi, OO , and Ariwoola, OS . Comparative proximate composition of maize (Zea mays L.) varieties grown in south-western Nigeria. Int Ann Sci. (2019) 7:1–5. doi: 10.21467/ias.7.1.1-5
27. Eleazu, CO , Eleazu, KF , Ukamaka, G , Adeolu, T , Ezeorah, V , and Ezeorah, B . Nutrient and antinutrient composition and heavy metal and phenolic profiles of maize (Zea mays) as affected by different processing techniques. ACS Food Sci Technol. (2021) 1:113–23. doi: 10.1021/acsfoodscitech.0c00045
28. Oas, SE , and Adams, KR . The nutritional content of five Southwestern US indigenous maize (Zea mays L.) landraces of varying endosperm type. Am Antiq. (2021) 87:1–19. doi: 10.1017/AAQ.2021.131
29. Ray, K , Banerjee, H , Duttaid, S , Hazra, AK , and Majumdar, K . Macronutrients influence yield and oil quality of hybrid maize (Zea mays L.). PLoS One. (2019) 14:1–23. doi: 10.1371/journal.pone.0216939
30. Celmeli, T , Id, S , Canci, H , Sari, D , Id, AA , and Toker, C . The nutritional content of common bean (Phaseolus vulgaris L.) landraces in comparison to modern varieties. Agronomy. (2018) 8:166. doi: 10.3390/agronomy8090166
31. Los, FGB , Zielinski, AAF , Wojeicchowski, JP , Nogueira, A , and Demiate, IM . Beans (Phaseolus vulgaris L.): whole seeds with complex chemical composition. Curr Opin Food Sci. (2018) 19:63–71. doi: 10.1016/j.cofs.2018.01.010
32. Mecha, E , Figueira, ME , Vaz-Patto, MC , and Do Rosário-Bronze, M . Legume Seed Nutraceutical Research. (2018).
33. Nguyen, AT , Althwab, S , Qiu, H , Zbasnik, R , Urrea, C , Carr, TP, et al. Pinto beans (Phaseolus vulgaris L.) lower non-HDL cholesterol in hamsters fed a diet rich in saturated fat and act on genes involved in cholesterol homeostasis. J Nutr. (2018) 149:996–1003. doi: 10.1093/jn/nxz044
34. Amin, MZ , Islam, T , Uddin, MR , Uddin, MJ , Rahman, MM , and Satter, MA . Comparative study on nutrient contents in the different parts of indigenous and hybrid varieties of pumpkin (Cucurbita maxima Linn.). Heliyon. (2019) 5:e02462. doi: 10.1016/j.heliyon.2019.e02462
35. Kirnak, H , Irik, HA , Sipahioglu, O , and Unlukara, A . Variations in oil, protein, fatty acids and vitamin E contents of pumpkin seeds under deficit irrigation. Grasas Aceites. (2019) 70:692181. doi: 10.3989/gya.0692181
36. Peña-Betancourt, SD , Gutiérrez-Tolentino, R , and Schettino, B . Proximate composition, fatty acid profile and mycotoxin contamination in several varieties of Mexican maize. Food Nutr Sci. (2017) 8:865–72. doi: 10.4236/fns.2017.89062
37. Singh, A , and Kumar, V . Nutritional, phytochemical, and antimicrobial attributes of seeds and kernels of different pumpkin cultivars. Food Front. (2021) 3:182–93. doi: 10.1002/fft2.117
38. Tá Nska, M , Ogrodowska, D , Bartoszewski, G , Korzeniewska, A , and Konopka, I . Seed lipid composition of new hybrids of styrian oil pumpkin grown in Poland. Agronomy. (2020) 10:2–15. doi: 10.3390/agronomy10081104
39. Cochrane, MP . Seed technology and its biological basis. Boca Raton, FL: CRC Press, 85–120. (2000).
40. FAO/WHO . Dietary protein quality evaluation in human Nutri–tion report of an FAO expert consultation. Food and Nutrition Paper no. 92. Rome: Food and Agriculture Organizations and the World Health Organization 2013. Auckland, New Zealand: FAO (2013).
42. Esan, YO , Omoba, OS , and Enujiugha, VN . Biochemical and nutritional compositions of two accessions of Amaranthus cruentus seed flour. Am J Food Sci Tech. (2018) 6:145–50. doi: 10.12691/ajfst-6-4-3
43. Kirimi, JG , Musalia, LM , Magana, A , and Munguti, JM . Protein quality of rations for Nile tilapia (Oreochromis niloticus) containing oilseed meals. J Agric Sci. (2020) 12:82. doi: 10.5539/jas.v12n2p82
44. Kotecka-Majchrzak, K , Sumara, A , Fornal, E , and Montowska, M . Oilseed proteins–properties and application as a food ingredient. Trends Food Sci Technol. (2020) 106:160–70. doi: 10.1016/j.tifs.2020.10.004
45. Mota, C , Santos, M , Mauro, R , Samman, N , Matos, AS , Torres, D, et al. Protein content and amino acids profile of pseudocereals. Food Chem. (2016) 193:55–61. doi: 10.1016/j.foodchem.2014.11.043
46. World Health Organization . Double-duty actions for nutrition: policy brief. Geneva: World Health Organization (2017).
48. Haytowitz, D , Lemar, L , Pehrsson, P , Exler, J , Patterson, K , Thomas, R, et al. US Department of Agriculture. Washington, DC, USA. (2011).
49. Tanumihardjo, SA . Vitamin a: biomarkers of nutrition for development. Am J Clin Nutr. (2011) 94:658S–65S. doi: 10.3945/ajcn.110.005777
50. UNICEF . United Nations international Children’s emergency fund. (2015). Available at: https://data.unicef.org/topic/nutrition/vitamin-a-deficiency/.
51. Stipanuk, MH . Biochemical, physiological, molecular aspects of human nutrition. 2nd ed. St Louis: Saunders Elsevier (2006). 667 p.
52. Jiang, M , Liu, M , Huang, H , and Chen, F . Fully continuous flow synthesis of 5-(Aminomethyl)-2-methylpyrimidin-4-amine: a key intermediate of vitamin B1. Org Process Res Dev. (2021) 25:2331–7. doi: 10.1021/acs.oprd.1c00253
54. Whitney, N , Rolfes, S , Crowe, T , Cameron-Smith, D , and Walsh, A . Understanding nutrition. Melbourne: Cengage Learning (2011).
57. Inazu, M . Functional expression of choline transporters in the blood-brain barrier. Nutrients. (2019) 11:2265. doi: 10.3390/nu11102265
58. ODS, Office of Dietary Supplements . Folate–Fact sheet for health professionals. US National Institutes of Health. (2021).
59. NDA, ESFA Panel on Dietetic Products, Nutrition and Allergies . Dietary reference values for choline. EFSA J. (2016) 14:4484. doi: 10.2903/j.efsa.2016.4484
60. Magiorkinis, E , Beloukas, A , and Diamantis, A . Scurvy: past, present and future. Eur J Intern Med. (2011) 22:147–52. doi: 10.1016/j.ejim.2010.10.006
61. La Fata, G , van Vliet, N , Barnhoorn, S , Brandt, RMC , Etheve, S , Chenal, E, et al. Vitamin E supplementation reduces cellular loss in the brain of a premature aging mouse model. J Prev Alzheimers Dis. (2017) 4:226–35. doi: 10.14283/jpad.2017.30
62. van Ballegooijen, AJ , and Beulens, JW . The role of vitamin K status in cardiovascular health: evidence from observational and clinical studies. Curr Nutr Rep. (2017) 6:197–205. doi: 10.1007/s13668-017-0208-8
63. Sahu, PK , Cervera-Mata, A , Chakradhari, S , Singh Patel, K , Towett, EK , Quesada-Granados, JJ, et al. Seeds as potential sources of phenolic compounds and minerals for the Indian population. Molecules. (2022) 27:3184. doi: 10.3390/molecules27103184
64. Espinoza-García, N , Martínez-Martínez, R , Chávez-Servia, JL , Vera-Guzmán, AM , Carrillo-Rodríguez, JC , Heredia-García, E, et al. Contenido de minerales en semilla de poblaciones nativas de frijol común (Phaseolus vulgaris L.). Rev Fitotec Mex. (2016) 39:215–23. doi: 10.35196/rfm.2016.3.215-223
65. US Department of Agriculture, Agricultural Research Service . USDA food and nutrient database for dietary studies 2019–2020. Food Surveys Research Group Home Page. (2022). Available at: http://www.ars.usda.gov/nea/bhnrc/fsrg.
66. Amin, T , and Thakur, M . Cucurbita mixta (pumpkin) seeds-a general overview on their health benefits. Int J Recent Sci Res. (2013) 4:846–54.
68. FAO . Food and agriculture organization of the United Nations. Rome: FAO. (2018). Available at: http://faostat.fao.org.
69. Plate, HE . The nutrition source. Harvard TH Chan School of public health. (2021). Available at: https://www.Hsph.harvardarvard.Edu/nutritionsource/healthy.
70. Abdel-Aal, ESM . (2016). Available at: http://canadianfoodbusiness.com/2016/06/20/processing-pulses-to-enhance-bioactive and-anti-nutritional-attributes/ (Accessed October 20, 2020).
71. Muzquiz, M , Varela, A , Burbano, C , Cuadrado, C , Guillamón, E , and Pedrosa, MM . Implications for nutrition and health. Phytochem Rev. (2012) 11:227–44. doi: 10.1007/s11101-012-9233-9
72. Sánchez-Velázquez, OA , Ribéreau, S , Mondor, M , Cuevas-Rodríguez, EO , Arcand, Y , and Hernández-Álvarez, AJ . Impact of processing on the in vitro protein quality, bioactive compounds, and antioxidant potential of 10 selected pulses. Legum Sci. (2021) 3:e88. doi: 10.1002/leg3.88
73. Abbas, M , Saeed, F , Anjum, FM , Afzaal, M , Tufail, T , Bashir, MS, et al. Natural polyphenols: an overview. Int J Food Prop. (2017) 20:1689–99. doi: 10.1080/10942912.2016.1220393
74. Ganesan, K , and Xu, B . Polyphenol-rich dry common beans (Phaseolus vulgaris L.) and their health benefits. Int J Mol Sci. (2017) 18:2331. doi: 10.3390/ijms18112331
75. Singla, RK , Dubey, AK , Garg, A , Sharma, RK , Fiorino, M , Ameen, SM, et al. Natural polyphenols: chemical classification, definition of classes, subcategories, and structures. J AOAC Int. (2019) 102:1397–400. doi: 10.5740/jaoacint.19-0133
76. van Hoed, V , Sampaio, KA , Felkner, B , Bavec, F , Scippo, ML , Brose, F, et al. Tocopherols and polyphenols in pumpkin seed oil are moderately affected by industrially relevant roasting conditions. Eur J Lipid Sci Technol. (2017) 119:1700110. doi: 10.1002/ejlt.201700110
77. Cristianini, M , and Guillén Sánchez, JS . Extraction of bioactive compounds from purple corn using emerging technologies: a review. J Food Sci. (2020) 85:862–9. doi: 10.1111/1750-3841.15074
78. Akin, G , Arslan, FN , Elmas, SNK , and Yilmaz, I . Cold-pressed pumpkin seed (Cucurbita pepo L.) oils from the central Anatolia region of Turkey: Characterization of phytosterols, squalene, tocols, phenolic acids, carotenoids and fatty acid bioactive compounds. Grasas Aceites. (2018) 69:668171. doi: 10.3989/gya.0668171
79. Bello, FA , Akpan, ME , and Sodipo, MA . Physicochemical and sensory properties of cookies produced from wheat, unripe plantain and germinated fluted pumpkin seed. Food Sci Qual Manage. (2020) 34:23–9. doi: 10.7176/fsqm/96-05
80. Cardador-Martínez, A , Martínez-Tequitlalpan, Y , Gallardo-Velazquez, T , Sánchez-Chino, XM , Martínez-Herrera, J , Corzo-Ríos, L, et al. Effect of instant controlled pressure-drop on the non-nutritional compounds of seeds and sprouts of common black bean (Phaseolus vulgaris L.). Molecules. (2020) 25:1464. doi: 10.3390/molecules25061464
81. Chari, KY , Rao-Polu, P , and Shenoy, RR . An appraisal of pumpkin seed extract in 1, 2-dimethylhydrazine induced colon cancer in Wistar rats. J Toxicol. (2018) 2018:6086490. doi: 10.1155/2018/6086490
82. Hussain, A , Kausar, T , Din, A , Murtaza, MA , Jamil, MA , Noreen, S, et al. Determination of total phenolic, flavonoid, carotenoid, and mineral contents in peel, flesh, and seeds of pumpkin (Cucurbita maxima). J Food Proc Preserv. (2021) 45:e15542. doi: 10.1111/jfpp.15542
83. Irondi, EA , Ajani, EO , Aliyu, OM , Olatoye, KK , Abdulameed, HT , and Ogbebor, OF . Bioactive components, enzymes inhibitory and antioxidant activities of biofortified yellow maize (Zea mays L.) and cowpea (Vigna unguiculata L. Walp) composite biscuits. Ann Univ Dunarea Jos Galati Fascicle VI Food Technol. (2021) 45:86–101. doi: 10.35219/FOODTECHNOLOGY.2021.1.06
84. Madrera, RR , Campa-Negrillo, A , Valles, BS , and Ferreira-Fernández, JJ . Phenolic content and antioxidant activity in seeds of common bean (Phaseolus vulgaris L.). Foods. (2021) 10:864. doi: 10.3390/foods10040864
85. Parmar, N , Singh, N , Kaur, A , and Thakur, S . Comparison of color, anti-nutritional factors, minerals, phenolic profile and protein digestibility between hard-to-cook and easy-to-cook grains from different kidney bean (Phaseolus vulgaris) accessions. J Food Sci Technol. (2017) 54:1023–34. doi: 10.1007/s13197-017-2538-3
86. Silva-Fernández, SE , García-Lara, S , and Serna-Saldívar, SO . Physicochemical characterization of the anatomical structures of teosinte (Zea mays subsp. mexicana) covered caryopses. J Cereal Sci. (2022) 103:103353. doi: 10.1016/j.jcs.2021.103353
87. Siyuan, S , Tong, L , and Liu, RH . Corn phytochemicals and their health benefits. Food Sci Hum Wellness. (2018) 7:185–95. doi: 10.1016/j.fshw.2018.09.003
88. Mrduljaš, N , Krešić, G , and Bilušić, T . Functional food–Improve health through adequate food, august. London: IntechOpen (2017).
89. Cory, H , Passarelli, S , Szeto, J , Tamez, M , and Mattei, J . The role of polyphenols in human health and food systems: a Mini-review. Front Nutr. (2018) 5:1–9. doi: 10.3389/fnut.2018.00087
90. Rodriguez-Concepcion, M , Avalos, J , Bonet, ML , Boronat, A , Gomez-Gomez, L , Hornero-Mendez, D, et al. A global perspective on carotenoids: metabolism, biotechnology, and benefits for nutrition and health. Prog Lipid Res. (2018) 70:62–93. doi: 10.1016/j.plipres.2018.04.004
91. Holguín, C , Roberto, J , Álvarez, G , Ricardo, J , Cadillo, C , Guadalupe, M, et al. Propiedades fisicoquímicas y contenido de carotenoides totales de tres variedades de tomate (Lycopersicon esculentum) cultivadas con diferentes niveles de potasio. Investigación y Desarrollo en Ciencia y Tecnología de Alimentos. (2019).
92. Kipping, DR , Laurel, HO , Orozco, AA , and López, HMD . Características físicas y químicas de la semilla de calabaza para mecanización y procesamiento. Nova Sci. (2018) 10:61–77. doi: 10.21640/ns.v10i21.1467
93. Deepak, TS , and Jayadeep, PA . Nutraceutical potential of maize (Zea mays L.) (corn) lab-scale wet milling by- products in terms of β-sitosterol in fiber, gamma-tocopherol in germ, and lutein and zeaxanthin in gluten. Cereal Res Commun. (2022) 2022:914. doi: 10.21203/rs.3.rs-1518914/v1
94. Sipeniece, E , Mišina, I , Qian, Y , Grygier, A , Sobieszczańska, N , Kumar Sahu, P, et al. Fatty acid profile and squalene, tocopherol, carotenoid, sterol content of seven selected consumed legumes. Plant Foods Hum Nutr. (2021) 76:53–9. doi: 10.1007/s11130-020-00875-3/Published
95. Sperkowska, B , Murawska, J , Przybylska, A , Gackowski, M , Kruszewski, S , Durmowicz, M, et al. Cardiovascular effects of chocolate and wine–narrative review. Nutrients. (2021) 13:4269. doi: 10.3390/nu13124269
97. Chen, Z , Lei, Y-L , Wang, W-P , Lei, Y-Y , Liu, Y-H , Hei, J, et al. Effects of saponin from Trigonella foenum-graecum seeds on dyslipidemia. Iran J Med Sci. (2017) 42:577–85.
98. Feriani, A , Tir, M , Hachani, R , Gómez-Caravaca, AM , Del Contreras, MM , Taamalli, A, et al. Zygophyllum album saponins prevent atherogenic effect induced by deltamethrin via attenuating arterial accumulation of native and oxidized LDL in rats. Ecotoxicol Environ Saf. (2020) 193:110318. doi: 10.1016/j.ecoenv.2020.110318
99. Marrelli, M , Conforti, F , Araniti, F , and Statti, GA . Effects of saponins on lipid metabolism: a review of potential health benefits in the treatment of obesity. Molecules. (2016) 21:1404. doi: 10.3390/molecules21101404
100. Shi, Q , Jin, S , Xiang, X , Tian, J , Huang, R , Li, S, et al. The metabolic change in serum lysoglycerophospholipids intervened by triterpenoid saponins from Kuding tea on hyperlipidemic mice. Food Funct. (2019) 10:7782–92. doi: 10.1039/c9fo02142f
101. Wang, Q , Mu, RF , Liu, X , Zhou, HM , Xu, YH , Qin, WY, et al. Steaming changes the composition of saponins of Panax notoginseng (Burk.) F.H. Chen that function in treatment of hyperlipidemia and obesity. J Agric Food Chem. (2020) 68:4865–75. doi: 10.1021/acs.jafc.0c00746
102. Salehi, B , Quispe, C , Sharifi-Rad, J , Cruz-Martins, N , Nigam, M , Mishra, AP, et al. Phytosterols: from preclinical evidence to potential clinical applications. Front Pharmacol. (2021) 11:599959. doi: 10.3389/fphar.2020.599959
103. Bai, G , Ma, C , and Chen, X . Phytosterols in edible oil: distribution, analysis and variation during processing. Grain Oil Sci Technol. (2021) 4:33–44. doi: 10.1016/j.gaost.2020.12.003
104. Cedó, L , Farràs, M , Lee-Rueckert, M , and Escolà-Gil, JC . Molecular insights into the mechanisms underlying the cholesterol- lowering effects of Phytosterols. Curr Med Chem. (2019) 26:6704–23. doi: 10.2174/0929867326666190822154701
105. Vilcanqui-Pérez, F , and Vílchez-Perales, C . Revisión. Archivos Latinoamericanos de Nutrición. (2017). Available at: https://www.alanrevista.org/ediciones/2017/2/art-10/.
106. Nirmala-Prasadi, VP , and Joye, IJ . Dietary fibre from whole grains and their benefits on metabolic health. Nutrients. (2020) 12:1–20. doi: 10.3390/nu12103045
107. Zhu, B , He, H , and Hou, T . A comprehensive review of corn protein-derived bioactive peptides: production, characterization, bioactivities, and transport pathways. Compr Rev Food Sci Food Saf. (2019) 18:329–45. doi: 10.1111/1541-4337.12411
108. Li, G , Liu, W , Wang, Y , Jia, F , Wang, Y , Ma, Y, et al. Functions and applications of bioactive peptides from corn gluten meal. Adv Food Nutr Res. (2019) 87:1–41. doi: 10.1016/bs.afnr.2018.07.001
109. Orona-Tamayo, D , Valverde, ME , and Paredes-López, O . Bioactive peptides from selected latin american food crops–a nutraceutical and molecular approach. Crit Rev Food Sci Nutr. (2019) 59:1949–75. doi: 10.1080/10408398.2018.1434480
110. Pérez-Gregorio, R , Soares, S , Mateus, N , and de Freitas, V . Bioactive peptides and dietary polyphenols: two sides of the same coin. Molecules. (2020) 25:3443. doi: 10.3390/molecules25153443
111. Díaz-Gómez, JL , Castorena-Torres, F , Preciado-Ortiz, RE , and García-Lara, S . Anti-Cancer activity of maize bioactive peptides. Front Chem. (2017) 5:44. doi: 10.3389/fchem.2017.00044
112. Gomes, MJ , Lima, SL , Alves, NE , Assis, A , Moreira, ME , Toledo, RC, et al. Common bean protein hydrolysate modulates lipid metabolism and prevents endothelial dysfunction in BALB/c mice fed an atherogenic diet. Nutr Metab Cardiovasc Dis. (2020) 30:141–50. doi: 10.1016/j.numecd.2019.07.020
113. Ozuna, C , and León-Galván, M . Cucurbitaceae seed protein Hydrolysates as a potential source of bioactive peptides with functional properties. Biomed Res Int. (2017) 2017:1–16. doi: 10.1155/2017/2121878
114. Opapeju, FO , Nyachoti, CM , House, JD , Weiler, H , and Sapirstein, HD . Growth performance and carcass characteristics of pigs fed short-season corn hybrids. J Anim Sci. (2006) 84:2779–86. doi: 10.2527/jas.2005-353
115. Kawakami, Y , Yamanaka-Okumura, H , Naniwa-Kuroki, Y , Sakuma, M , Taketani, Y , and Takeda, E . Flaxseed oil intake reduces serum small dense low-density lipoprotein concentrations in Japanese men: a randomized, double blind, crossover study. Nutr J. (2015) 14:1–9. doi: 10.1186/s12937-015-0023-2
116. Lee, EJ , and Ahn, DU . Production of volatiles from fatty acids and oils by irradiation. J Food Sci. (2003) 68:70–5. doi: 10.1111/j.1365-2621.2003.tb14116.x
117. Zamaninour, N , Ansar, H , Djazayery, A , Pishva, H , Shidfar, F , Fard, RMN, et al. Black grapes can change the physiology of adipose tissue in high-fat diets containing flaxseed oil or corn oil in C57BL/6J mice. Curr Top Nutraceutical Res. (2016) 14:265.
118. Lee, JW , Kil, DY , Keever, BD , Killefer, J , McKeith, FK , Sulabo, RC, et al. Carcass fat quality of pigs is not improved by adding corn germ, beef tallow, palm kernel oil, or glycerol to finishing diets containing distillers dried grains with solubles. J Anim Sci. (2013) 91:2426–37. doi: 10.2527/jas.2012-5328
119. Słowik-Borowiec, M , Zdeb, G , Kuras, W , and Książektrela, P . Influence of fermentation on content of selected macronutrients in seeds and beans. Acta Univ Cibiniensis E Food Technol. (2022) 26:123–38. doi: 10.2478/aucft-2022-0010
120. Bhagya, B , Sridhar, KR , Seena, S , and Bhat, R . Nutritional qualities of ripened beans of mangrove wild legume Canavalia cathartica Thouars. J Agrid Tech. (2007) 3:255–74.
121. Sutivisedsak, N , Moser, BR , Sharma, BK , Evangelista, RL , Cheng, HN , Lesch, WC, et al. Physical properties and fatty acid profiles of oils from black, kidney, great northern, and pinto beans. J Am Oil Chem Soc. (2011) 88:193–200. doi: 10.1007/s11746-010-1669-8
122. David, I , Orboi, MD , Simandi, MD , Chirilă, CA , Megyesi, CI , Rădulescu, L, et al. Fatty acid profile of Romanian’s common bean (Phaseolus vulgaris L.) lipid fractions and their complexation ability by β-cyclodextrin. PLoS One. (2019) 14:e0225474. doi: 10.1371/journal.pone.0225474
123. Iwuagwu, MO , Solomon, CU , and Amanze, JE . Physicochemical analysis and characterization of edible oil from seeds of orange (Citrus sinensis L.) and pumpkin (Cucurbita pepo L.). Eur J Biotechnol Biosci. (2018) 6:35–40.
124. Vujasinovic, V , Djilas, S , Dimic, E , Romanic, R , and Takaci, A . Shelf life of cold-pressed pumpkin (Cucurbita pepo L.) seed oil obtained with a screw press. J Am Oil Chem Soc. (2010) 87:1497–505. doi: 10.1007/s11746-010-1630-x
125. Öz, M , Ucak, İ , and Nayik, GA . PUFA and MUFA In: J Wu , editor. Nutraceuticals and health care. Cambridge, MA: Academic Press (2022). 199–215.
126. Cicero, AF , Fogacci, F , Grandi, E , Rizzoli, E , Bove, M , D’Addato, S, et al. Prevalent seasoning and cooking fats, arterial stiffness and blood lipid pattern in a rural population sample: data from the Brisighella heart study. Nutrients. (2020) 12:3063. doi: 10.3390/nu12103063
127. Majid, AK , Ahmed, Z , and Khan, R . Effect of pumpkin seed oil on cholesterol fractions and systolic/diastolic blood pressure. Food Sci Technol. (2020) 40:769–77. doi: 10.1590/fst.03720
128. Monica, SJ , John, S , Madhanagopal, R , Sivaraj, C , Khusro, A , Arumugam, P, et al. Chemical composition of pumpkin (Cucurbita maxima) seeds and its supplemental effect on Indian women with metabolic syndrome. Arab J Chem. (2022) 2022, 103985. doi: 10.1016/j.arabjc.2022.103985
129. Gálvez Ranilla, L . The application of metabolomics for the study of cereal corn (Zea mays L.). Meta. (2020) 10:300. doi: 10.3390/metabo10080300
130. Nolan, R , Shannon, OM , Robinson, N , Joel, A , Houghton, D , and Malcomson, FC . It’s no has bean: a review of the effects of white kidney bean extract on body composition and metabolic health. Nutrients. (2020) 12:1398. doi: 10.3390/nu12051398
131. Khan, J , Khan, MZ , Ma, Y , Meng, Y , Mushtaq, A , Shen, Q, et al. Overview of the composition of whole grains’ phenolic acids and dietary fibre and their effect on chronic non-communicable diseases. Int J Environ Res Public Health. (2022) 19:3042. doi: 10.3390/ijerph19053042
132. World Health Organization . Hypertension. (2021). Available at: https://www.who.int/es/news-room/fact-sheets/detail/hypertension. (Accessed January 7, 2023).
133. Sugiyanta, S , Notopuro, H , Nugraha, J , and Handajani, R . F2-Isoprostane levels in Deoxycorticosterone acetate (DOCA)-salt induced hypertensive rats administered with coffee-corn mixture. Res J Pharm Technol. (2021) 14:3353–7. doi: 10.52711/0974-360X.2021.00583
134. Sánchez-Velázquez, OA , Mulero, M , Cuevas-Rodríguez, EO , Mondor, M , Arcand, Y , and Hernández-Álvarez, AJ . In vitro gastrointestinal digestion impact on stability, bioaccessibility and antioxidant activity of polyphenols from wild and commercial blackberries (Rubus spp.). Food Funct. (2021) 12:7358–78. doi: 10.1039/D1FO00986A
135. Guo, Y , Wang, K , Wu, B , Wu, P , Duan, Y , and Ma, H . Production of ACE inhibitory peptides from corn germ meal by an enzymatic membrane reactor with a novel gradient diafiltration feeding working-mode and in vivo evaluation of antihypertensive effect. J Funct Foods. (2020) 64:103584. doi: 10.1016/j.jff.2019.103584
136. Zhu, J , Li, J , Guo, Y , Quaisie, J , Hong, C , and Ma, H . Antihypertensive and immunomodulatory effects of defatted corn germ hydrolysates: an in vivo study. Front Nutr. (2021) 246:679583. doi: 10.3389/fnut.2021.679583
137. Li, CC , Lee, YC , Lo, HY , Huang, YW , Hsiang, CY , and Ho, TY . Antihypertensive effects of corn silk extract and its novel bioactive constituent in spontaneously hypertensive rats: the involvement of angiotensin-converting enzyme inhibition. Molecules. (2019) 24:1886. doi: 10.3390/molecules24101886
138. Ribeiro, JVV , Graziani, D , Carvalho, JHM , Mendonça, MM , Naves, LM , Oliveira, HF, et al. A peptide fraction from hardened common beans (Phaseolus vulgaris) induces endothelium-dependent antihypertensive and renal effects in rats. Curr Res Food Sci. (2023) 6:100410. doi: 10.1016/j.crfs.2022.100410
139. Ariza-Ortega, TDJ , Zenón-Briones, EY , Castrejón-Flores, JL , Yáñez-Fernández, J , Gómez-Gómez, YDLM , and Oliver-Salvador, MDC . Angiotensin-I-converting enzyme inhibitory, antimicrobial, and antioxidant effect of bioactive peptides obtained from different varieties of common beans (Phaseolus vulgaris L.) with in vivo antihypertensive activity in spontaneously hypertensive rats. Eur Food Res Technol. (2014) 239:785–94. doi: 10.1007/s00217-014-2271-3
140. Escobedo, A , Rivera-León, EA , Luévano-Contreras, C , Urías-Silvas, JE , Luna-Vital, DA , Morales-Hernández, N, et al. Common bean baked snack consumption reduces Apolipoprotein B-100 levels: a randomized crossover trial. Nutrients. (2021) 13:3898. doi: 10.3390/nu13113898
141. World Health Organization . Diabetes. (2021). Available at: https://www.who.int/health-topics/diabetes#tab=tab_1. (Accessed January 7, 2023).
142. Lobstein, T , Brinsden, H , and Neveux, M . World obesity atlas 2022. (2022). Available at: https://www.worldobesity.org/resources/resource-library/world-obesity-atlas-2022. (Accessed January 7, 2023).
143. Firdausia, R , Rumiyati Nugroho, AE , Purwestri, YA , and Pranoto, Y . The effect of functional rice analogue diet from mocaf, corn, pigeon pea and seaweed on rats model of type 2 diabetes. Food Res. (2021) 5:238–47. doi: 10.26656/fr.2017.5(4).682
144. Sheng, L , Chen, Q , Di, L , and Li, N . Evaluation of anti-diabetic potential of corn silk in high-fat diet/ Streptozotocin-induced type 2 diabetes mice model. Endocr Metab Immune Disord Drug Targets. (2021) 21:131–8. doi: 10.2174/1871530320666200606224708
145. Domínguez-Uscanga, A , Loarca-Piña, G , and de Mejia, EG . Baked corn (Zea mays L.) and bean (Phaseolus vulgaris L.) snack consumption lowered serum lipids and differentiated liver gene expression in C57BL/6 mice fed a high-fat diet by inhibiting PPARγ and SREBF2. FASEB J. (2017) 31:1–15. doi: 10.1016/j.jnutbio.2017.08.011
146. Gomes, MJC , da Silva, JS , Alves, NEG , de Assis, A , de Mejía, EG , Mantovani, HC, et al. Cooked common bean flour, but not its protein hydrolysate, has the potential to improve gut microbiota composition and function in BALB/c mice fed a high-fat diet added with 6-propyl-2-thiouracil. J Nutr Biochem. (2022) 106:109022. doi: 10.1016/j.jnutbio.2022.109022
147. Ramírez-Jiménez, A , Luzardo, I , Cuellar-Nuñez, L , and Loarca-Pina, G . Common beans and oat snack bars attenuated hypertriglyceridemia markers in a randomized clinical trial of Mexican women. Curr Dev Nutr. (2021) 5:606–6. doi: 10.1093/cdn/nzab044_037
148. Hussain, A , Kausar, T , Jamil, MA , Noreen, S , Iftikhar, K , Rafique, A, et al. In vitro role of pumpkin parts as Pharma-foods: Antihyperglycemic and Antihyperlipidemic activities of pumpkin Peel, flesh, and seed powders, in Alloxan-induced diabetic rats. Int J Food Sci. (2022) 2022:1–10. doi: 10.1155/2022/4804408
149. World Health Organization . Cancer. (2021). Available at: https://www.who.int/news-room/fact-sheets/detail/cancer(Accessed January 7, 2023).
150. Flores-García, MK , Mérida-Ortega, Á , Denova-Gutiérrez, E , Rothenberg, SJ , and López-Carrillo, L . “Western” and “prudent” dietary patterns are associated with breast cancer among Mexican pre- and postmenopausal women. Nutr Res. (2022) 105:138–46. doi: 10.1016/j.nutres.2022.06.007
151. Sasaki, D , Kusamori, K , and Nishikawa, M . Delivery of corn-derived nanoparticles with anticancer activity to tumor tissues by modification with polyethylene glycol for cancer therapy. Pharm Res. (2022) 2022:1–10. doi: 10.1007/s11095-022-03431-7
152. Leibbrand, M , Siefer, S , Schön, C , Perrinjaquet-Moccetti, T , Kompek, A , Csernich, A, et al. Effects of an oil-free hydroethanolic pumpkin seed extract on symptom frequency and severity in men with benign prostatic hyperplasia: a pilot study in humans. J Med Food. (2019) 22:551–9. doi: 10.1089/jmf.2018.0106
153. Chen, L , Deng, H , Cui, H , Fang, J , Zuo, Z , Deng, J, et al. Inflammatory responses and inflammation-associated diseases in organs. Oncotarget. (2018) 9:7204–18. doi: 10.18632/oncotarget.23208
154. Verediano, TA , Martino, HSD , Kolba, N , Fu, Y , Paes, MCD , and Tako, E . Black corn (Zea mays L.) soluble extract showed antiinflammatory effects and improved the intestinal barrier integrity in vivo (Gallus gallus). Food Res Int. (2022) 157:111227. doi: 10.1016/j.foodres.2022.111227
155. Ristic-Medic, D , Perunicic-Pekovic, G , Rasic-Milutinovic, Z , Takic, M , Popovic, T , Arsic, A, et al. Effects of dietary milled seed mixture on fatty acid status and inflammatory markers in patients on hemodialysis. Sci World J. (2014) 2014:1–9. doi: 10.1155/2014/563576
156. Teeranachaideekul, V , Parichatikanond, W , Junyaprasert, VB , and Morakul, B . Pumpkin seed oil-loaded Niosomes for topical application: 5α-Reductase inhibitory, anti-inflammatory, and in vivo anti-hair loss effects. Pharmaceuticals. (2022) 15:930. doi: 10.3390/ph15080930
Glossary
Keywords: milpa system seeds, bioactive components, corn, common beans, health properties, nutritional potential
Citation: Sánchez-Velázquez OA, Luna-Vital DA, Morales-Hernandez N, Contreras J, Villaseñor-Tapia EC, Fragoso-Medina JA and Mojica L (2023) Nutritional, bioactive components and health properties of the milpa triad system seeds (corn, common bean and pumpkin). Front. Nutr. 10:1169675. doi: 10.3389/fnut.2023.1169675
Edited by:
Jayanta Layek, The ICAR Research Complex for North Eastern Hill Region (ICAR RCNEH), IndiaReviewed by:
Seok Shin Tan, Monash University Malaysia, MalaysiaAmit Kumar, Indian Council of Agricultural Research (ICAR), India
Copyright © 2023 Sánchez-Velázquez, Luna-Vital, Morales-Hernandez, Contreras, Villaseñor-Tapia, Fragoso-Medina and Mojica. This is an open-access article distributed under the terms of the Creative Commons Attribution License (CC BY). The use, distribution or reproduction in other forums is permitted, provided the original author(s) and the copyright owner(s) are credited and that the original publication in this journal is cited, in accordance with accepted academic practice. No use, distribution or reproduction is permitted which does not comply with these terms.
*Correspondence: Luis Mojica, bG1vamljYUBjaWF0ZWoubXg=; Diego Armando Luna-Vital, ZGllbHVuYUB0ZWMubXg=