- 1Department of Pediatrics, Yonsei University College of Medicine, Seoul, Republic of Korea
- 2Biostatistics Collaboration Unit, Yonsei University College of Medicine, Seoul, Republic of Korea
- 3Department of Pediatrics, Konyang University College of Medicine, Daejeon, Republic of Korea
Introduction: The parent–child correlation in metabolic syndrome (MetS) and elevated transaminases is sparsely researched. We assessed the correlation of parental MetS and elevated transaminase status with these conditions in their children.
Methods: Data of 4,167 youths aged 10–18 years were analyzed in a population-based survey, and the parental characteristics were stratified by the presence or absence of MetS or alanine aminotransferase (ALT) elevation in their children. The prevalence of these conditions in children was analyzed according to their parents’ status. Logistic regression analyses were performed with MetS and ALT elevation in youth as the dependent variables.
Results: The proportions of MetS and ALT elevation were higher in parents of children with MetS and ALT elevation than in those without, even among youths without obesity. In logistic regression analyses, age, body mass index–standard deviation score (BMI–SDS), and ALT elevation were positively associated with MetS, whereas age, male sex, BMI–SDS, protein intake, and MetS were positively associated with ALT elevation. Higher protein intake was related to ALT elevation, whereas metabolic components and nutritional factors were closely related in parents and their children. Odds ratios (OR) of ALT elevation for MetS was 8.96 even after adjusting nutritional factors in the children. The OR was higher for ALT elevation in the children of parents with MetS and ALT elevation compared to those without. ORs for MetS and ALT elevation in the children of parents with MetS were higher than those of children of parents without MetS, even after adjusting for nutritional intake. ORs for ALT elevation were higher in the children of parents with ALT elevation than those without, even after adjusting for nutritional intake and BMI of parents as well as the nutritional intake, age, sex, and BMI–SDS of the children.
Conclusion: MetS and elevated liver transaminase statuses in children were associated with those of their parents even after adjusting for nutritional factors, and the relationships were more prominent in the youth without obesity.
1. Introduction
Metabolic syndrome (MetS) constitutes a cluster of risk factors for cardiovascular disease and increases the risk of atherosclerotic cardiac disease; moreover, childhood MetS increases the risk of adult MetS (1, 2). A systematic review showed that, among children and adolescents, MetS prevalence ranged from 0.3% (in Columbia) to 26.4% (in Iran) (3). The overall median prevalence was 3.8%, and it was 5 and 5.4% in Saudi Arabia and the United States, respectively. A study in the United States reported a decline in the prevalence of MetS among youth from 7.3% in 1988–1994 to 6.5% in 2003–2006 (4). In contrast, a Korean study showed that the prevalence of MetS increased from 1.5% in 2008 to 3.2% in 2017 among youth (1). In adults, obesity, overnutrition, and low lean mass are risk factors for MetS (5). In a cohort study, MetS in children was correlated with MetS in parents (6).
Nonalcoholic fatty liver disease (NAFLD) is a chronic liver disease characterized by excessive hepatic fat accumulation in a spectrum that ranges from nonalcoholic steatohepatitis, hepatic fibrosis, to idiopathic cirrhosis, without significant alcohol consumption (7–9). Among patients with nonalcoholic steatohepatitis, the annual cumulative incidence of liver cirrhosis is 0.25–3.2%; of this, 0.3–2.6% of cases with cirrhotic nonalcoholic steatohepatitis progress to hepatocellular carcinoma per year (10). In a global study, the prevalence of NAFLD was 30%, whereas in a pediatric study, the prevalence increased from 3.9% in 1988–1994 to 10.7% in 2007–2010 (11, 12).
In the pathogenesis of MetS and NAFLD, insulin resistance plays a key role (13–15). Furthermore, obesity, unhealthy dietary habits, and MetS are risk factors for NAFLD progression in adults (10, 16). A meta-analysis showed an association of NAFLD with a twofold increased risk of MetS in adults (17). Based on the above-described relationships, a novel concept—metabolic-associated fatty liver disease (MAFLD)—has been posited (18). MAFLD is identified by its association with metabolic dysregulation, irrespective of alcohol consumption. Therefore, addressing pediatric MAFLD and NAFLD is important to prevent severe hepatic as well as metabolic disorders in adulthood (18, 19). However, research on the risk factors for pediatric MetS and MAFLD or NAFLD in association with parental MetS and MAFLD or NAFLD remains limited.
In this population-based study, we aimed to investigate the association of pediatric MetS and elevated liver transaminase levels with these conditions in the parents. The study’s objectives were to investigate: (1) the relationship between MetS and elevated liver transaminases in youths; (2) the risk factors of MetS and elevated liver transaminases in youths; and (3) the association of MetS and elevated liver transaminase levels in parents with these conditions in children.
2. Materials and methods
2.1. Study design and participants
This cross-sectional study analyzed data from the Korea National Health and Nutrition Examination Survey (KNHANES), a national population-based survey conducted by the Korea Centers for Disease Control and Prevention based on the National Health Promotion Act, that were obtained between 2007 and 2019. The KNHANES was conducted in 1998, 2001, 2005, and annually since 2007 using a rolling sampling survey method. The cross-sectional survey includes approximately 10,000 participants each year as a cohort and investigates data on health behaviors and clinical examination, including anthropometric and laboratory analysis-based information, socioeconomic status, nutritional status, the presence of chronic disease, and quality of life. The survey involved a two-step stratified sampling method that used sampling units and households as the primary and secondary sampling units, respectively. In total, 200 and 192 primary sampling units (PSU) per year were drawn from approximately 200,000 geographically defined PSU nationwide in KNHANES 2007–2009 and KNHANES 2010–2019, respectively. On average, a PSU comprises 60 households, and 20 final target households were sampled for each PSU using systematic sampling; individuals who were ≥ 1-year-old were targeted from the selected households.
From among children and adolescents as well as their parents who participated in KNHANES 2007–2019, individuals whose anthropometric, blood pressure, fasting glucose and lipid, and alanine aminotransferase (ALT) data were missing were excluded. Finally, data from 4,167 children and adolescents as well as their respective parents were analyzed in this study; details of the participant screening and selection are presented in a flowchart in Figure 1.
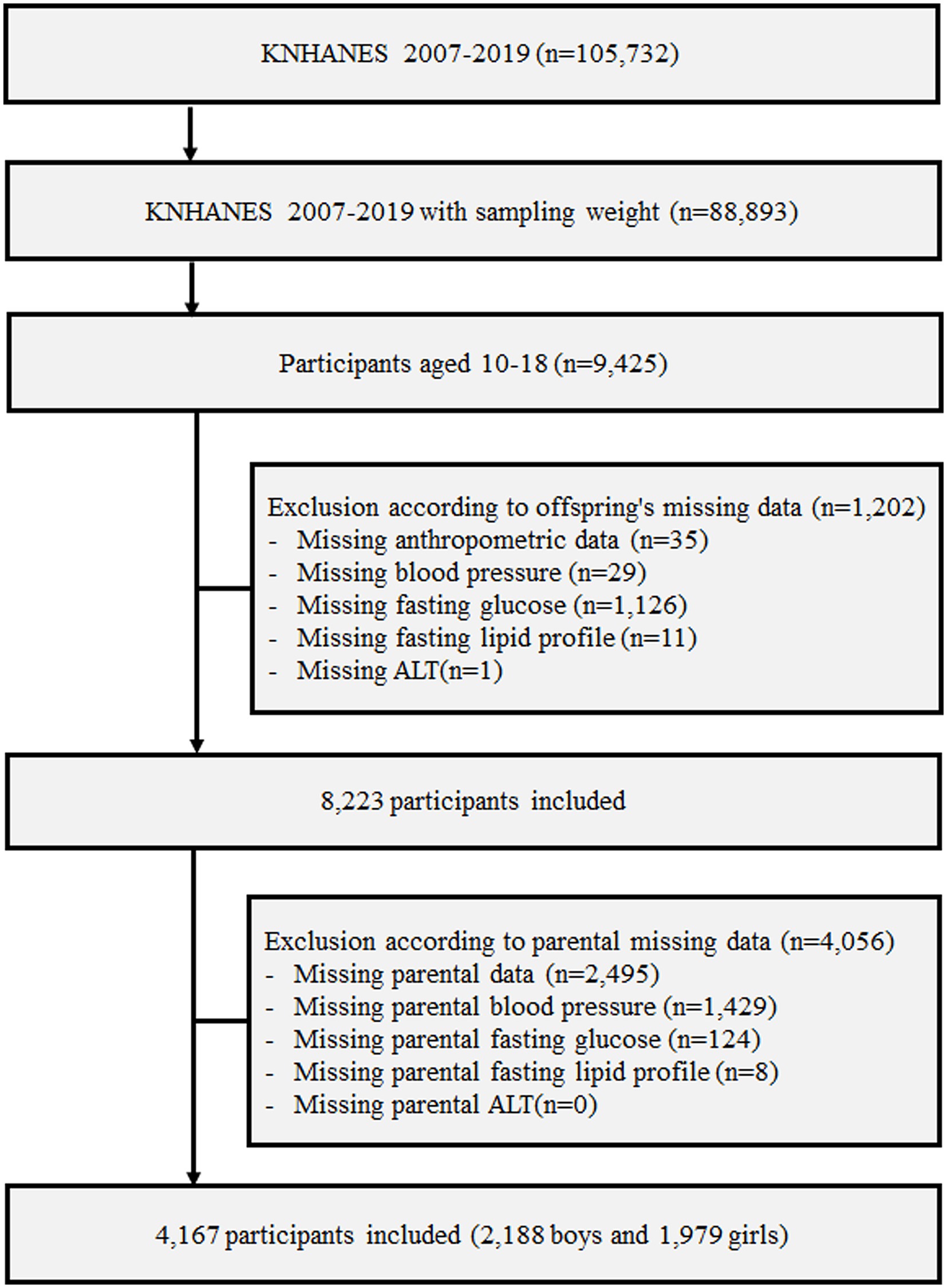
Figure 1. Design and flowchart of study population. KNHANES, Korea National Health and Nutrition Examination Survey; ALT, alanine aminotransferase.
To ensure accurate representation of the Korean population and minimize selection bias, a multistage-stratified systematic sampling design was used wherein the sample weights were constructed for sample participants by accounting for the complex survey design, survey non-response, and post-stratification. The weights based on the inverse of selection probabilities and response rates were modified by adjusting them to the sex- and age-specific Korean populations (20).
2.2. Study variables
Data on sex, age, and anthropometric measurements were recorded. Height was measured using a portable stadiometer (range, 850–2060 mm; Seriter, Holtain Ltd., Crymych, UK), and weight was measured using a calibrated balance beam scale (Giant 150N; HANA, Seoul, Republic of Korea). Body mass index (BMI) was calculated as the weight (kg) divided by the height (in meters) squared (m2). Waist circumference (WC) was measured at the narrowest point between the lower borders of the rib cage and the iliac crest at the end of normal expiration. Height, weight, and BMI were presented as standard deviation scores (SDS) based on the 2017 Korean National Growth Charts (21). Based on their BMI, children were categorized as normal (<85th percentile), overweight (85th to <95th percentile), or obese (≥95th percentile). Central obesity was defined as a WC >90th percentile using Korean waist reference data (22).
Information on food intake was obtained via face-to-face interview (20). The food frequency questionnaire consisted of 63 food items that are important sources of energy and nutrients. The food intake questionnaire was created as an open-ended survey to report different meals and foods, and utilized the 24 h recall method with different measurement tools.
2.3. Laboratory analysis
Blood samples were collected from the antecubital vein after at least 8 h of fasting and were subsequently processed and immediately refrigerated. Serum levels of fasting glucose, total cholesterol, high-density lipoprotein cholesterol (HDL-C), triglycerides, aspartate aminotransferase (AST), and ALT were measured using Labospect 008AS (Hitachi, Tokyo, Japan). Low-density lipoprotein cholesterol (LDL-C) was calculated using the Friedewald formula (LDL-C = total cholesterol − [HDL-C + (triglycerides/5)]). The triglycerides/5 were used for serum samples with triglyceride values ≤400 mg/dL, whereas samples with triglyceride levels >400 mg/dL were considered as missing values in the analysis (23). “ALT elevation” was defined as an increased ALT level (>26, > 22, >30, and > 19 IU/L for boys, girls, men, and women, respectively) without hepatitis B virus infection or significant alcohol consumption, which was defined as the intake of >140 and > 70 g/week alcohol in men and women, respectively (8, 16, 24, 25).
2.4. Statistical analysis
All statistical analyses were performed using SAS (version 9.4; SAS Institute, Cary, NC, USA) for the complex sampling design, with clustering, stratification, and unequal weighting of the KNHANES sample and considering sampling weights to present data on the representative Korean youth population and to overcome limitations conferred by the sampling survey design. All categorical variables are presented as weighted percentages with standard errors, whereas continuous variables are presented as weighted means with standard errors. Participants were stratified into subgroups based on the presence of MetS and ALT elevation, and the proportion of participants with MetS or ALT elevation and the characteristics of their parents were analyzed. Moreover, the prevalence of MetS and ALT elevation was analyzed in subgroups stratified by BMI and the presence of parental MetS and ALT elevation. Intergroup differences were ascertained using the Student’s t-test for continuous variables and the Rao–Scott chi-square test for categorical variables. To clarify the risk factors for pediatric MetS and ALT elevation, logistic regression analyses were performed with MetS and ALT elevation in youth as the dependent variables. Furthermore, to clarify the association of MetS and ALT elevation between children and their parents, logistic regression analyses were performed with the MetS and ALT elevation of the children and parents as dependent and independent variables, respectively. Linear regression analyses were performed with each component of MetS, liver enzymes, and nutritional intake of parents and children as independent and dependent variables, respectively. A p-value < 0.05 was considered statistically significant.
3. Results
3.1. Association of MetS with elevated liver transaminases among youths
Table 1 shows the baseline characteristics of participants according to the presence of MetS and ALT elevation. Compared to the youth without MetS, the youth with MetS had higher values for age, BMI SDS, AST, ALT, and the proportion of obesity and ALT elevation. Each component of MetS, including WC, blood pressure, triglycerides, and glucose, as well as the proportion of central obesity and MetS, were higher in the youth with ALT elevation than in those without ALT elevation. Furthermore, compared to the youth without ALT elevation, age, BMI SDS, intake of energy and protein, and the proportion of male sex and obesity were higher in the youths with ALT elevation.
In univariable logistic regression analyses, the odds ratio (OR; 95% confidence interval [CI]) for MetS in age, BMI SDS, and ALT elevation were 1.13 (1.02–1.25), 1.52 (0.90–2.55), 4.01 (3.31–4.85), and 8.91 (5.16–15.38), respectively (Supplementary Table 1). The OR (95% CI) for ALT elevation in age, male sex, BMI SDS, protein intake, and MetS were 1.08 (1.03–1.14), 2.73 (2.10–3.54), 2.35 (2.11–2.61), 1.05 (1.02–1.08), and 8.91 (5.16–15.38). After adjusting nutrition factors, including carbohydrate, protein, and fat, the OR (95% CI) of MetS for ALT elevation was 8.98 (5.22–15.46) and that of ALT elevation for MetS was 8.96 (5.20–15.44). However, these relationships were not significant after adjusting for age, sex, BMI–SDS, and nutritional factors.
3.2. Parental characteristics in subgroups stratified by the children’s MetS and elevated liver transaminase status
Table 2 shows the parental characteristics according to the MetS and ALT elevation status of the children. Compared to the fathers and mothers of the youth without MetS, the parents of the youth with MetS had a higher BMI, WC, and proportion of MetS. Similarly, parents of youth with ALT elevation had higher values of age, BMI, WC, AST, ALT, and the proportion of obesity and ALT elevation compared to the parents of youth without ALT elevation. Moreover, fathers of youth with ALT elevation had higher systolic and diastolic blood pressures and glucose levels compared to fathers of youth without ALT elevation.
3.3. Characteristics of youth according to parental MetS and elevated liver transaminase status
Table 3 shows the characteristics of the youth according to the parents’ MetS and ALT elevation status. The progeny of parents with MetS had higher BMI SDS, WC, systolic and diastolic blood pressures, glucose levels, and proportions of obesity, central obesity, and MetS compared to the progeny of parents without MetS. Moreover, AST, ALT, and fat intake levels were higher in the progeny of parents with MetS than in those of parents without MetS. The children of parents with ALT elevation had higher WC, ALT level, and proportions of obesity and ALT elevation compared to those of parents without ALT elevation.
Among youth with normal BMI or overweight, the proportion of children with MetS was higher in the progeny of parents with MetS than in those without (p = 0.033 for normal BMI and p = 0.017 for overweight; Figure 2A), whereas the proportion of offspring with ALT elevation was higher in the offspring of parents with ALT elevation than in those without (p = 0.021 for normal BMI and p = 0.003 for overweight; Figure 2B). However, these relationships were not significant among the youth with obesity.
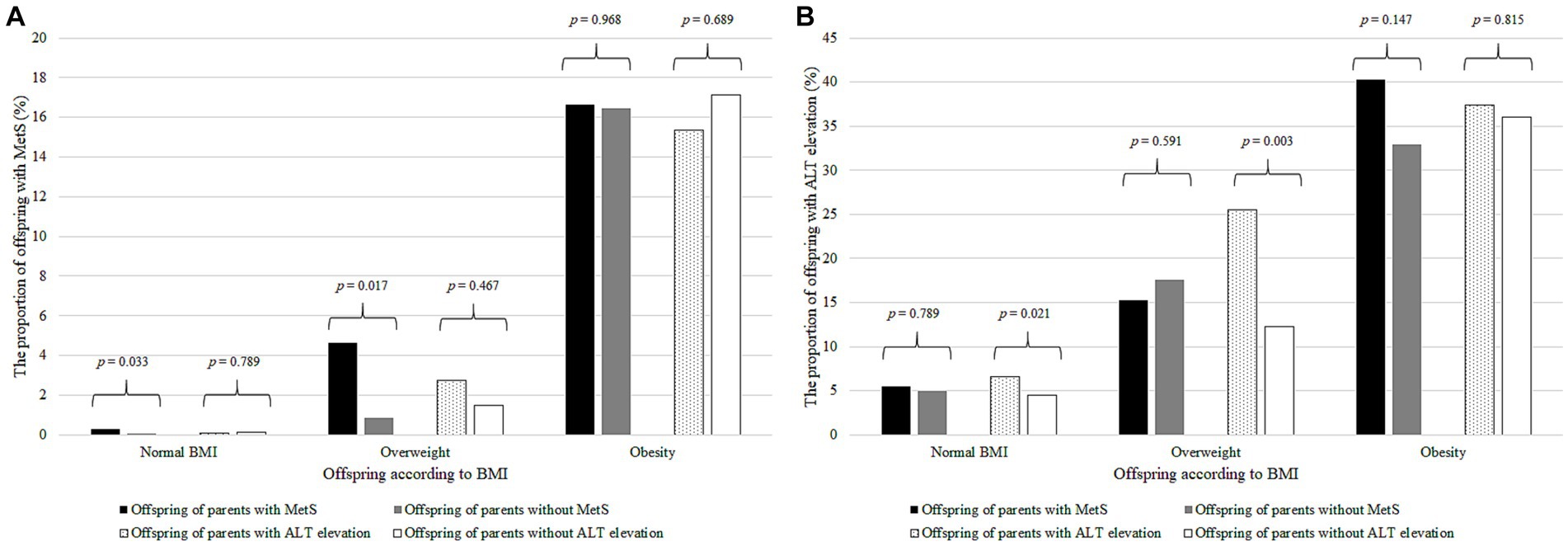
Figure 2. The proportion of offspring with MetS or ALT elevation according to BMI and parental MetS and ALT elevation. (A) The proportion of offspring with MetS according to BMI and parental MetS and ALT elevation. The black bar represents the offspring with MetS of the parents with MetS; gray bar, parents without MetS; dotted bar, parents with ALT elevation; and the white bar, parents without ALT elevation. The number on the bar is the p–value of Student’s t–test. (B) Proportion of offspring with ALT elevation according to BMI and parental MetS and ALT elevation. The black bar represents the offspring with ALT elevation of the parents with MetS; gray bar, those of the parents without MetS; dotted bar, those of the parents with ALT elevation; and the white bar, those of the parents without ALT elevation. The number on the bar is the p–value of Student’s t–test. MetS, metabolic syndrome; ALT, alanine aminotransferase; BMI, body mass index.
Supplementary Table 2 shows the characteristics of the children of parents without MetS or ALT elevation. The children of parents with MetS had higher BMI SDS, WC, systolic and diastolic blood pressures, glucose, triglycerides, ALT, and proportions of obesity, central obesity, and ALT elevation compared to those without MetS. Compared to the children of parents without ALT elevation, those whose parents had ALT elevation had higher BMI SDS, WC, systolic and diastolic blood pressures, AST, ALT, protein intake, and proportions of obesity, central obesity, and MetS.
3.4. Linear regression of children’s and parents’ metabolic components and nutrition
Linear regression analyses showed that BMI, each component of MetS, AST, ALT, and nutritional factors of the father and mother were postively associted with those of the children, except for the glucose level between the mother and child (Supplementary Table 3). Among the factors, the association with WC was the strongest.
3.5. OR of parental and children’s MetS and elevated liver transaminase levels
The children of fathers and mothers with MetS had a higher OR (2.26, 95% CI 1.27–4.04 and 3.88, 95% CI 1.99–7.56, respectively) for MetS compared to the children of parents without MetS; the OR was highest in the children whose both parents had MetS (OR 4.97, 95% CI 1.97–12.53) (Table 4). Additionally, a relationship between the parental MetS and the child’s MetS and ALT elevation status was identified even after adjusting for the parent’s and progeny’s nutritional intake, including carbohydrate, protein, and fat. Moreover, compared to the children whose parents did not have ALT elevation, the OR for ALT elevation was higher in the youths whose father, mother, or both parents had ALT elevation, and this effect persisted even after adjusting for the children’s age, sex, BMI–SDS, nutritional intake, and parental BMI and nutritional intake status.
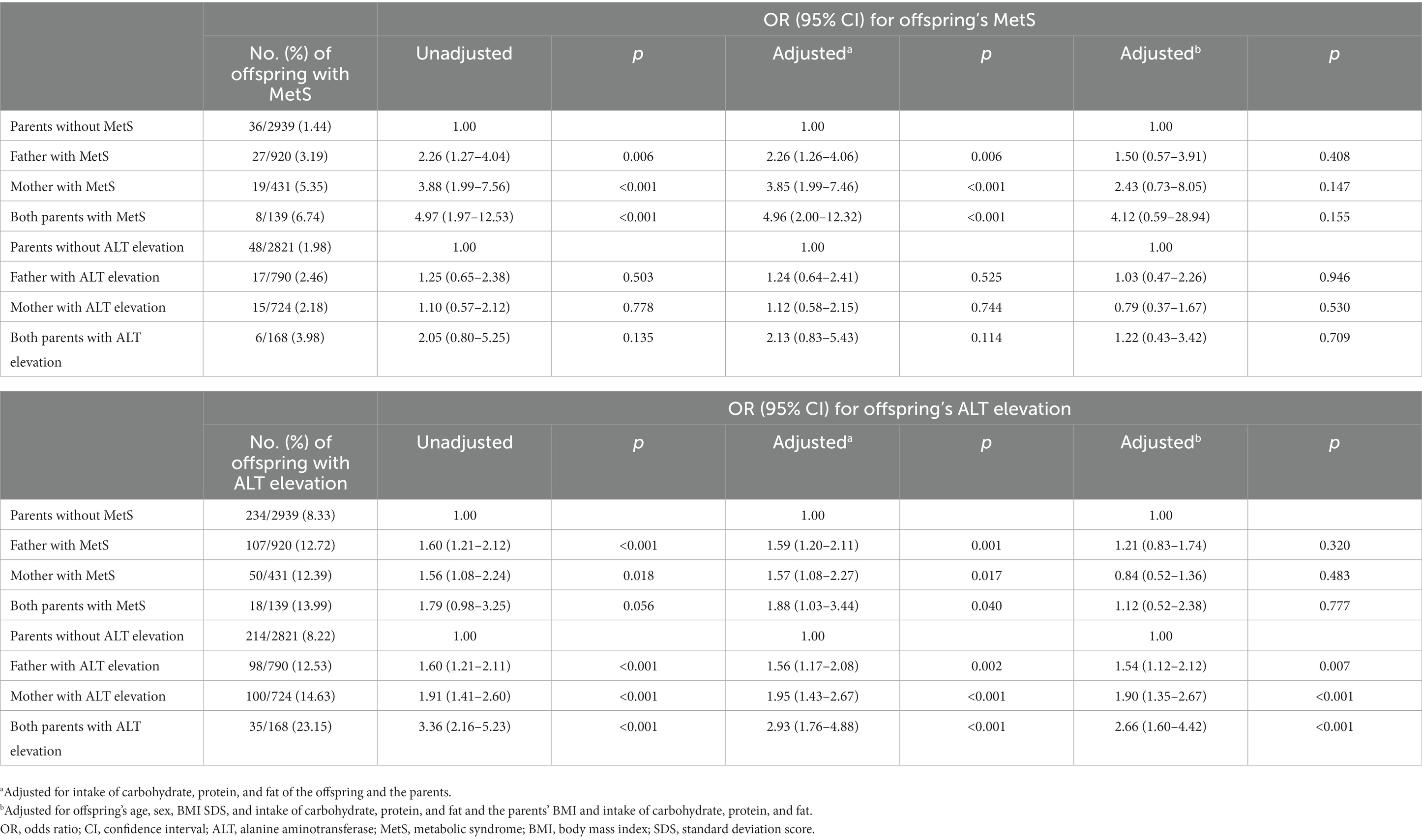
Table 4. ORs and 95% CIs for MetS and ALT elevation in offspring by parents’ MetS and ALT elevation.
4. Discussion
Our results demonstrate an association between parental MetS and elevated liver transaminases and offspring’s MetS and elevated liver transaminases. Moreover, parental MetS was associated with ALT elevation as well as MetS in their children. The relationship between mothers and descendant was stronger than that between fathers and their children. In addition, nutritional factors were closely related between parents and descendant. Among the nutritional factors, higher protein intake was related to ALT elevation. Additionally, older age, male sex, and higher BMI were associated with MetS and ALT elevation. The association of parental MetS and ALT elevation with descendant was significant, even after adjusting for nutritional factors. Moreover, parental ALT elevation was positively associated with descendant, even after adjusting for age and BMI. In addition, the relationship between parental MetS and ALT elevation with their children was more prominent in youths with normal BMI or overweight compared than in those with obesity. These findings suggest a genetic link between parents and descendant. Beyond genetics, epigenetic modifications can play a pivotal role by altering gene expression without changing the DNA sequence itself, potentially passing on metabolic risks to the offspring (26). Additionally, the transmission or shared characteristics of the gut microbiome between parents and their children might also influence metabolic health, considering its profound role in nutrient processing, inflammation, and other metabolic processes (27).
Elevated liver transaminases was closely related to MetS as well as each metabolic component among the youth in our study. MetS and NAFLD share insulin resistance as an important factor in pathogenesis (18). Insulin resistance promotes hepatic de novo lipogenesis by activating sterol regulatory element–binding protein 1 and lipid accumulation by inactivating Forkhead box protein A2, a transcription factor that promotes fatty acid oxidation in the liver (28, 29). Meanwhile, the secretion of hepatokine and metabolites related to lipid metabolism and insulin sensitivity is altered in NAFLD, which induces insulin resistance and MetS (30). A cross–sectional study reported that WC, blood pressure, and triglycerides increased, and HDL–C decreased with an increase in NAFLD grade among youth (31). A retrospective study reported that the odds ratio of NAFLD for MetS was 2.65 among children with obesity (32).
In our study, older age, male sex, BMI SDS, and obesity were found to be positively associated with MetS and elevated liver transaminases. Moreover, the association of elevated liver transaminases with age, male sex, and BMI SDS and that of MetS with BMI SDS were significant even after adjusting for nutritional factors. In a population–based study, the prevalence of NAFLD was 1.5–fold higher in participants aged 16–18 years than in those aged 10–12 years, while obesity was associated with a five–fold risk of NAFLD (7). In addition, the prevalence of NAFLD was two–fold higher in boys than in girls. In a systematic review, the prevalence of MetS was 29.2% in children with obesity and 3.3% in the whole population, while the corresponding value was 5.6% among older age groups and 2.9% among younger age groups (33).
Among nutritional factors, protein intake was associated with elevated liver transaminases in our study. Diets rich in proteins can induce production of harmful intestinal metabolites by gut microbiome, which may exacerbate MetS and NAFLD (34). A population–based study reported that protein intake in men with NAFLD was higher than that in men without, and this was thought to be related to meat intake and intestinal metabolites that are harmful for metabolic health due to intestinal protein fermentation (35, 36). However, another study reported that NAFLD development was not significantly related to protein intake (37). Meanwhile, the association of protein intake, age, and BMI SDS with ALT elevation was demonstrated in the youth of parents without MetS or ALT elevation in our study. Therefore, attention to liver enzymes and dietary intake is required in older boys with obesity, even though their parents do not have NAFLD or MetS, although more studies are required to clarify the association between nutritional intake and pediatric NAFLD.
The association of nutritional intake between parents and descendant was strong in our study. Thus, sharing meals and nutrition habits might affect the relationship between MetS and NAFLD of children and their parents. A meta–analysis reported that parental food preferences were significantly correlated with children’s preferences (38). In another meta–analysis, family based interventions improved the BMI of obese children (39). A population–based study reported that parents’ restriction on unhealthy food was negatively associated with the intake of unhealthy food in children (40). Parental nutrition can affect the descendant even during the prenatal period. Brion et al. (41) reported that the association between the descendant’s dietary habits and maternal dietary habits was stronger prenatally than postnatally. Moreover, a systematic review reported that the periconceptional nutritional status of parents could increase the risk of descendant MetS through epigenetic imprinting (42). Therefore, the assessment of familial nutritional status is required for the risk management of MetS and NAFLD in children, especially those with parents with MetS and NAFLD.
In our study, the association between parental MetS and elevated liver transaminases and those of their children was significant even after adjusting for nutritional intake. Moreover, parental elevated liver transaminases was associated with those of their even after adjusting for age, sex, BMI, and nutritional factors. These findings support the role of genetic links between MetS and elevated liver transaminases in parents and descendant. Several genes, including adiponutrin/patatin–like phospholipase domain–containing 3 and glucokinase regulatory protein, are known to be associated with both MetS and NAFLD (43). Adiponutrin/patatin–like phospholipase domain–containing 3 encodes adiponectin, which is expressed in both the liver and adipose tissue and has lipogenesis and lipolytic activity. Thus, limited triglyceride hydrolysis by the adiponutrin/patatin–like phospholipase domain–containing 3 rs738409 variant increases lipogenic activity. In a cohort study, glucokinase regulatory protein, a gene related to the regulation of glucokinase, was associated with triglyceride elevation and NAFLD among youths with obesity in a cohort study (44).
In our study, the association of children with mothers was stronger than that of fathers, and the ORs of children with both parents with MetS or elevated liver transaminases for children with MetS and elevated liver transaminases were higher than those with one parent with MetS and elevated liver transaminases. In a cohort study, the odds ratio of hepatic steatosis in at least one parent with hepatic steatosis was 2.0, whereas that of both parents with hepatic steatosis was 6.4 (8). In a meta–analysis study, the association of pediatric MetS with maternal MetS was stronger than that of paternal MetS, and that with both parents was strongest (45). The stronger lifestyle associations of children with mothers than those with fathers might be related to this difference. A cohort study reported that the association of children’s dietary intake with maternal dietary intake was stronger than that of paternal dietary intake (41). A Korean population–based study reported that adolescent physical activity is significantly associated with maternal physical activity (46).
The stronger association of offspring’s MetS and elevated liver transaminases with their parent in participants with normal BMI compared to those with obesity in our study is thought to be due to the strong association between obesity and MetS and NAFLD (7, 12, 33). In other words, the proportion of genetic effects on MetS and NAFLD may be higher in children without obesity. In an adult cohort study, parental hepatic steatosis is associated with hepatic steatosis in the descendant, even after adjusting for BMI, age, sex, alcohol, insulin resistance, and lipid–lowering agents (8). Therefore, attention to MetS and NAFLD is required, even in normal–weight children, if their parents have MetS and/or NAFLD.
This study has some limitations. First, this was a cross–sectional study limited to Koreans. Second, confounding factors such as physical activity, puberty, gut microbiome, and epigenetic modification were not considered. Third, liver biopsy and imaging studies were not performed for diagnosis of NAFLD or MAFLD because information on liver biopsy or imaging studies is not provided in the KNHANES. Fourth, genetic studies were not performed because the KNHANES does not include information on genetic evaluation. Fifth, KNHANES is not a complete enumeration, thus we analyzed the data with complex sampling design considering sampling weight to overcome selection bias.
5. Conclusion
This study showed a significant correlation between the MetS and elevated liver transaminase statuses of parents and their children, even after adjusting for nutritional factors, and a correlation between MetS and elevated liver transaminases in youths. In children, protein intake was associated with elevated liver transaminases, whereas the parental nutritional intake was associated with the child’s nutritional intake. The correlation between parental MetS and elevated liver transaminase status with that of children was more apparent in youths with a normal BMI or overweight than in those with obesity. These findings emphasize the role of genetic links and lifestyle in pediatric MetS, MAFLD, and NAFLD. Therefore, close monitoring of metabolic risk factors, including liver enzymes and dietary assessment, is required among the children of parents with MetS and/or NAFLD, even among those without obesity. Further studies to investigate the risk of pediatric MetS, NAFLD, and MAFLD by integrating genetic background and lifestyle factors are required to guide the management of future risks of cardiovascular disease and liver disease.
Data availability statement
The raw data supporting the conclusions of this article will be made available by the authors, without undue reservation.
Ethics statement
The studies involving humans were approved by the Institutional Review Board of Yonsei University Gangnam Severance Hospital (3-2022-0242). The studies were conducted in accordance with the local legislation and institutional requirements. Written informed consent for participation in this study was provided by the participants’ legal guardians/next of kin.
Author contributions
KS and HWC had the idea and designed the study, had full access to all the data in the study, take responsibility for the integrity of the data and the accuracy of the data analysis, critically revised the manuscript for important intellectual content, and gave final approval for the version to be published. KS, JY, and HSL drafted the manuscript and did the analysis. JO, SK, ML, JS, AK, and H-SK take the responsibility for double check of the data analysis. All authors agree to be accountable for all aspects of the work in ensuring that questions related to the accuracy or integrity of any part of the work are appropriately resolved.
Funding
This study was supported by a faculty research grant from Yonsei University College of Medicine (6-2021-0150).
Conflict of interest
The authors declare that the research was conducted in the absence of any commercial or financial relationships that could be construed as a potential conflict of interest.
Publisher’s note
All claims expressed in this article are solely those of the authors and do not necessarily represent those of their affiliated organizations, or those of the publisher, the editors and the reviewers. Any product that may be evaluated in this article, or claim that may be made by its manufacturer, is not guaranteed or endorsed by the publisher.
Supplementary material
The Supplementary material for this article can be found online at: https://www.frontiersin.org/articles/10.3389/fnut.2023.1166244/full#supplementary-material
References
1. Park, SI, Suh, J, Lee, HS, Song, K, Choi, Y, Oh, JS, et al. Ten-year trends of metabolic syndrome prevalence and nutrient intake among Korean children and adolescents: a population-based study. Yonsei Med J. (2021) 62:344–51. doi: 10.3349/ymj.2021.62.4.344
2. Gepstein, V, and Weiss, R. Obesity as the main risk factor for metabolic syndrome in children. Front Endocrinol. (2019) 10:568. doi: 10.3389/fendo.2019.00568
3. Reisinger, C, Nkeh-Chungag, BN, Fredriksen, PM, and Goswami, N. The prevalence of pediatric metabolic syndrome-a critical look on the discrepancies between definitions and its clinical importance. Int J Obes. (2021) 45:12–24. doi: 10.1038/s41366-020-00713-1
4. Lim, S, Jang, HC, Park, KS, Cho, SI, Lee, MG, Joung, H, et al. Changes in metabolic syndrome in American and Korean youth, 1997-2008. Pediatrics. (2013) 131:e214–22. doi: 10.1542/peds.2012-0761
5. Grundy, SM. Metabolic syndrome update. Trends Cardiovasc Med. (2016) 26:364–73. doi: 10.1016/j.tcm.2015.10.004
6. Khan, RJ, Gebreab, SY, Riestra, P, Xu, R, and Davis, SK. Parent-offspring association of metabolic syndrome in the Framingham heart study. Diabetol Metab Syndr. (2014) 6:140. doi: 10.1186/1758-5996-6-140
7. Song, K, Park, G, Lee, HS, Lee, M, Lee, HI, Ahn, J, et al. Trends in prediabetes and non-alcoholic fatty liver disease associated with abdominal obesity among Korean children and adolescents: based on the Korea National Health and nutrition examination survey between 2009 and 2018. Biomedicine. (2022) 10:584. doi: 10.3390/biomedicines10030584
8. Long, MT, Gurary, EB, Massaro, JM, Ma, J, Hoffmann, U, Chung, RT, et al. Parental non-alcoholic fatty liver disease increases risk of non-alcoholic fatty liver disease in offspring. Liver Int. (2019) 39:740–7. doi: 10.1111/liv.13956
9. Lee, HW, Kim, M, Youn, J, Singh, S, and Ahn, SH. Liver diseases in South Korea: a pulse check of the public’s knowledge, awareness, and behaviors. Yonsei Med J. (2022) 63:1088–98. doi: 10.3349/ymj.2022.0332
10. D’Avola, D, Labgaa, I, and Villanueva, A. Natural history of nonalcoholic steatohepatitis/nonalcoholic fatty liver disease-hepatocellular carcinoma: magnitude of the problem from a hepatology clinic perspective. Clin Liver Dis. (2016) 8:100–4. doi: 10.1002/cld.579
11. Le, MH, Yeo, YH, Li, X, Li, J, Zou, B, Wu, Y, et al. 2019 global NAFLD prevalence: a systematic review and meta-analysis. Clin Gastroenterol Hepatol. (2021) 20:2809–2817.e28. doi: 10.1016/j.cgh.2021.12.002
12. Welsh, JA, Karpen, S, and Vos, MB. Increasing prevalence of nonalcoholic fatty liver disease among United States adolescents, 1988-1994 to 2007-2010. J Pediatr. (2013) 162:496–500.e1. doi: 10.1016/j.jpeds.2012.08.043
13. Song, K, Park, G, Lee, HS, Lee, M, Lee, HI, Choi, HS, et al. Comparison of the triglyceride glucose index and modified triglyceride glucose indices to predict nonalcoholic fatty liver disease in youths. J Pediatr. (2022) 242:79–85.e1. doi: 10.1016/j.jpeds.2021.11.042
14. Kim, M, and Kim, J. Cardiometabolic risk factors and metabolic syndrome based on severity of obesity in Korean children and adolescents: data from the Korea National Health and nutrition examination survey 2007-2018. Ann Pediatr Endocrinol Metab. (2022) 27:289–99. doi: 10.6065/apem.2142230.115
15. Yoo, SE, Lee, JH, Lee, JW, Park, HS, Lee, HA, and Kim, HS. Increasing prevalence of fasting hyperglycemia in adolescents aged 10-18 years and its relationship with metabolic indicators: the Korea National Health and nutrition examination study (KNHANES), 2007-2018. Ann Pediatr Endocrinol Metab. (2022) 27:60–8. doi: 10.6065/apem.2142068.034
16. Lee, YH, Jung, KS, Kim, SU, Yoon, HJ, Yun, YJ, Lee, BW, et al. Sarcopaenia is associated with NAFLD independently of obesity and insulin resistance: Nationwide surveys (KNHANES 2008-2011). J Hepatol. (2015) 63:486–93. doi: 10.1016/j.jhep.2015.02.051
17. Ballestri, S, Zona, S, Targher, G, Romagnoli, D, Baldelli, E, Nascimbeni, F, et al. Nonalcoholic fatty liver disease is associated with an almost twofold increased risk of incident type 2 diabetes and metabolic syndrome. Evidence from a systematic review and meta-analysis. J Gastroenterol Hepatol. (2016) 31:936–44. doi: 10.1111/jgh.13264
18. Song, K, Kim, H-S, and Chae, HW. Nonalcoholic fatty liver disease and insulin resistance in children. Clin Exp Pediatr. (2023). doi: 10.3345/cep.2022.01312 [Epub ahead of print].
19. Song, K, Yang, J, Lee, HS, Kim, SJ, Lee, M, Suh, J, et al. Changes in the prevalences of obesity, abdominal obesity, and non-alcoholic fatty liver disease among Korean children during the COVID-19 outbreak. Yonsei Med J. (2023) 64:269–77. doi: 10.3349/ymj.2022.0540
20. Kweon, S, Kim, Y, Jang, MJ, Kim, Y, Kim, K, Choi, S, et al. Data resource profile: the Korea National Health and nutrition examination survey (KNHANES). Int J Epidemiol. (2014) 43:69–77. doi: 10.1093/ije/dyt228
21. Kim, JH, Yun, S, Hwang, SS, Shim, JO, Chae, HW, Lee, YJ, et al. The 2017 Korean National Growth Charts for children and adolescents: development, improvement, and prospects. Korean J Pediatr. (2018) 61:135–49. doi: 10.3345/kjp.2018.61.5.135
22. Moon, J. Korean National Growth Charts: review of developmental process and an outlook. Korean J Pediatr. (2007) 51:1. doi: 10.3345/kjp.2008.51.1.1
23. Roberts, WC. The Friedewald-levy-Fredrickson formula for calculating low-density lipoprotein cholesterol, the basis for lipid-lowering therapy. Am J Cardiol. (1988) 62:345–6. doi: 10.1016/0002-9149(88)90248-2
24. Schwimmer, JB, Dunn, W, Norman, GJ, Pardee, PE, Middleton, MS, Kerkar, N, et al. SAFETY study: alanine aminotransferase cutoff values are set too high for reliable detection of pediatric chronic liver disease. Gastroenterology. (2010) 138:64.e1–2. doi: 10.1053/j.gastro.2009.12.052
25. Prati, D, Taioli, E, Zanella, A, Della Torre, E, Butelli, S, Del Vecchio, E, et al. Updated definitions of healthy ranges for serum alanine aminotransferase levels. Ann Intern Med. (2002) 137:1–10. doi: 10.7326/0003-4819-137-1-200207020-00006
26. Wang, J, Wu, Z, Li, D, Li, N, Dindot, SV, Satterfield, MC, et al. Nutrition, epigenetics, and metabolic syndrome. Antioxid Redox Signal. (2012) 17:282–301. doi: 10.1089/ars.2011.4381
27. Vrieze, A, Van Nood, E, Holleman, F, Salojärvi, J, Kootte, RS, Bartelsman, JF, et al. Transfer of intestinal microbiota from lean donors increases insulin sensitivity in individuals with metabolic syndrome. Gastroenterology. (2012) 143:913–6.e7. doi: 10.1053/j.gastro.2012.06.031
28. Samuel, VT, and Shulman, GI. The pathogenesis of insulin resistance: integrating signaling pathways and substrate flux. J Clin Invest. (2016) 126:12–22. doi: 10.1172/jci77812
29. Kohjima, M, Higuchi, N, Kato, M, Kotoh, K, Yoshimoto, T, Fujino, T, et al. SREBP-1c, regulated by the insulin and AMPK signaling pathways, plays a role in nonalcoholic fatty liver disease. Int J Mol Med. (2008) 21:507–11.
30. Watt, MJ, Miotto, PM, De Nardo, W, and Montgomery, MK. The liver as an endocrine organ-linking NAFLD and insulin resistance. Endocr Rev. (2019) 40:1367–93. doi: 10.1210/er.2019-00034
31. Tominaga, K, Fujimoto, E, Suzuki, K, Hayashi, M, Ichikawa, M, and Inaba, Y. Prevalence of non-alcoholic fatty liver disease in children and relationship to metabolic syndrome, insulin resistance, and waist circumference. Environ Health Prev Med. (2009) 14:142–9. doi: 10.1007/s12199-008-0074-5
32. Gupta, R, Bhangoo, A, Matthews, NA, Anhalt, H, Matta, Y, Lamichhane, B, et al. The prevalence of non-alcoholic fatty liver disease and metabolic syndrome in obese children. J Pediatr Endocrinol Metab. (2011) 24:907–11. doi: 10.1515/jpem.2011.282
33. Friend, A, Craig, L, and Turner, S. The prevalence of metabolic syndrome in children: a systematic review of the literature. Metab Syndr Relat Disord. (2013) 11:71–80. doi: 10.1089/met.2012.0122
34. Croci, S, D’Apolito, LI, Gasperi, V, Catani, MV, and Savini, I. Dietary strategies for management of metabolic syndrome: role of gut microbiota metabolites. Nutrients. (2021) 13:1389. doi: 10.3390/nu13051389
35. Zelber-Sagi, S, Nitzan-Kaluski, D, Goldsmith, R, Webb, M, Blendis, L, Halpern, Z, et al. Long term nutritional intake and the risk for non-alcoholic fatty liver disease (NAFLD): a population based study. J Hepatol. (2007) 47:711–7. doi: 10.1016/j.jhep.2007.06.020
36. Bonsembiante, L, Targher, G, and Maffeis, C. Non-alcoholic fatty liver disease in obese children and adolescents: a role for nutrition? Eur J Clin Nutr. (2022) 76:28–39. doi: 10.1038/s41430-021-00928-z
37. Solga, S, Alkhuraishe, AR, Clark, JM, Torbenson, M, Greenwald, A, Diehl, AM, et al. Dietary composition and nonalcoholic fatty liver disease. Dig Dis Sci. (2004) 49:1578–83. doi: 10.1023/b:ddas.0000043367.69470.b7
38. Borah-Giddens, J, and Falciglia, GA. A meta-analysis of the relationship in food preferences between parents and children. J Nutr Educ. (1993) 25:102–7. doi: 10.1016/S0022-3182(12)80565-6
39. Berge, JM, and Everts, JC. Family-based interventions targeting childhood obesity: a meta-analysis. Child Obes. (2011) 7:110–21. doi: 10.1089/chi.2011.07.02.1004.berge
40. Vaitkevičiūtė, J, and Petrauskienė, A. The associations between body mass index of seven- and eight-year-old children, dietary behaviour and nutrition-related parenting practices. Medicina. (2019) 55:24. doi: 10.3390/medicina55010024
41. Brion, MJ, Ness, AR, Rogers, I, Emmett, P, Cribb, V, Davey Smith, G, et al. Maternal macronutrient and energy intakes in pregnancy and offspring intake at 10 y: exploring parental comparisons and prenatal effects. Am J Clin Nutr. (2010) 91:748–56. doi: 10.3945/ajcn.2009.28623
42. Dunford, AR, and Sangster, JM. Maternal and paternal periconceptional nutrition as an indicator of offspring metabolic syndrome risk in later life through epigenetic imprinting: a systematic review. Diabetes Metab Syndr. (2017) 11:S655–62. doi: 10.1016/j.dsx.2017.04.021
43. D’Adamo, E, Castorani, V, and Nobili, V. The liver in children with metabolic syndrome. Front Endocrinol. (2019) 10:514. doi: 10.3389/fendo.2019.00514
44. Santoro, N, Zhang, CK, Zhao, H, Pakstis, AJ, Kim, G, Kursawe, R, et al. Variant in the glucokinase regulatory protein (GCKR) gene is associated with fatty liver in obese children and adolescents. Hepatology. (2012) 55:781–9. doi: 10.1002/hep.24806
45. Irakoze, L, Manirakiza, A, Zhang, Y, Liu, J, Li, J, Nkengurutse, L, et al. Metabolic syndrome in offspring of parents with metabolic syndrome: a meta-analysis. Obes Facts. (2021) 14:148–62. doi: 10.1159/000513370
Keywords: metabolic syndrome, non-alcoholic fatty liver disease, child, adolescent, parent–child relations
Citation: Song K, Yang J, Lee HS, Oh JS, Kim S, Lee M, Suh J, Kwon A, Kim H-S and Chae HW (2023) Parental metabolic syndrome and elevated liver transaminases are risk factors for offspring, even in children and adolescents with a normal body mass index. Front. Nutr. 10:1166244. doi: 10.3389/fnut.2023.1166244
Edited by:
Pietro Vajro, University of Salerno, ItalyReviewed by:
Beat Moeckli, University of Geneva, SwitzerlandDaniela Maria Tanase, Grigore T. Popa University of Medicine and Pharmacy, Romania
Copyright © 2023 Song, Yang, Lee, Oh, Kim, Lee, Suh, Kwon, Kim and Chae. This is an open-access article distributed under the terms of the Creative Commons Attribution License (CC BY). The use, distribution or reproduction in other forums is permitted, provided the original author(s) and the copyright owner(s) are credited and that the original publication in this journal is cited, in accordance with accepted academic practice. No use, distribution or reproduction is permitted which does not comply with these terms.
*Correspondence: Hyun Wook Chae, aG9wZWNoYWVAeXVocy5hYw==