- 1Brain Plasticity Group, Swammerdam Institute for Life Sciences, University of Amsterdam, Amsterdam, Netherlands
- 2Emma Children's Hospital, Amsterdam University Medical Centers, University of Amsterdam, Vrije Universiteit, Amsterdam, Netherlands
- 3Amsterdam Reproduction and Development, Amsterdam, Netherlands
- 4Danone Nutricia Research, Utrecht, Netherlands
- 5Department of Epidemiology and Data Science, Amsterdam University Medical Centers, University of Amsterdam, Amsterdam, Netherlands
- 6Amsterdam Public Health Research Institute, Aging and Later Life, Health Behaviors and Chronic Diseases, Amsterdam, Netherlands
Background: Maternal stress in the postpartum period affects not only the mother but also her newborn child, who is at increased risk of developing metabolic and mental disorders later in life. The mechanisms by which stress is transmitted to the infant are not yet fully understood. Human milk (HM) is a potential candidate as maternal stress affects various components of HM, e.g., fat and immunoglobulin concentrations. To date, it is unknown whether maternal stress also affects the amino acids (AAs) in HM, even though this nutrient is of extreme importance to child health and development. This study aimed to investigate whether and how maternal stress is associated with the AA composition of HM.
Methods: In this observational cohort study (Amsterdam, The Netherlands), lactating women were recruited in two study groups: a high-stress (HS) group; women whose child was hospitalized (n = 24), and a control (CTL) group; women who gave birth to a healthy child (n = 73). HM was collected three times a day, on postpartum days 10, 17, and 24. Perceived psychological stress was measured using validated questionnaires, while biological stress measures were based on hair, saliva, and HM cortisol concentrations. HM protein-bound and free AAs were analyzed by liquid chromatography and compared between groups.
Results: Maternal perceived stress scores were higher in the HS group (p < 0.01). The concentrations of protein-bound AAs in HM were higher in the HS group compared to the CTL group (p = 0.028) and were positively associated with HM cortisol concentrations (p = 0.024). The concentrations of free AAs did not differ between study groups and were unrelated to cortisol concentrations.
Conclusion: Findings from this prospective cohort study suggest that maternal stress in the postpartum period is associated with an altered human milk amino acid composition, which could play a role in the transmission of maternal stress effects to her child. The physiological implications of these stress-induced changes for infant development await future research.
1. Introduction
Maternal stress in the postpartum period not only affects the mother but may also have consequences for her newborn child. It has been shown that maternal stress occurring during this sensitive developmental period is associated with the infant's risk of developing a wide range of disorders, including metabolic and mental health disorders (1–3). Because the prevention of stressful maternal circumstances in the early postnatal period is generally difficult, a better understanding of the processes underlying these detrimental consequences for the infant is needed. Several mechanisms by which transmission of maternal stress to her infant occurs have been suggested, one of them being stress-induced changes in human milk (HM) composition (4, 5).
HM is the optimal source of nutrition for newborn infants (6–8). It is a highly complex fluid, consisting of over 100 different components that are influenced by many different factors (9). It has been demonstrated previously that maternal psychopathology and maternal stress in the postpartum period are associated with an altered composition of fatty acids in HM (10–16). However, whether maternal stress is also associated with changes in other HM nutrients, e.g., amino acids (AAs), is so far unknown. As AAs in HM are critical for infant growth and development and are necessary for almost all infant body processes (17), it is important to understand how they are affected by maternal stress. In addition, previous human studies point toward an effect of stress on AAs in the plasma, and previous animal experimental studies even suggest a change in milk composition under the influence of stress.
In both human and animal plasma, AAs decrease as a result of stress (18–20). As maternal plasma is the source from which AAs are transported into milk, lower maternal plasma AA levels, e.g., due to stress, may likely result in lower AA levels in milk (21). Whereas, human studies addressing this aspect are lacking, two studies in mice have shown that while maternal stress resulted in lower concentrations of AA in maternal plasma and reduced growth of the offspring, the concentrations of the AAs asparagine and alanine were increased in milk (22, 23). In another animal study, maternal stress during lactation lowered methionine levels in the offspring brain and plasma and induced cognitive deficits later in life, which, notably, could be partly counteracted by methionine supplementation in the dam's diet (24). While this suggests that maternal stress lowers methionine levels in milk, which could have important programming effects on brain health, the AA concentrations in milk were not determined in this study (24).
This study aimed to investigate whether maternal stress in the first month postpartum is associated with the fraction of protein-bound AA (BAA), which makes up 90–95% of the AAs, and the free AA (FAA), which comprises a relatively small fraction of the AA in HM (17). We further focused on methionine as a key AA that may play a role in the long-term consequences of early life stress and investigated the associations between maternal stress and this specific AA. A better understanding of potential stress-induced changes in HM AA composition will contribute to our knowledge of how maternal stress can be transferred to the infant.
2. Materials and methods
2.1. Research design and study population
We studied a prospective observational cohort of lactating women who were followed over their first month postpartum, and experienced various amounts of stress. Participants were recruited during pregnancy or within the first 10 days after giving birth, via social media, flyers at midwife practices, or at the maternal or neonatal ward of the Amsterdam University Medical Center (Amsterdam, The Netherlands). Mothers were eligible to participate when they were 18 years of age or older, and if they had the intention to breastfeed their infant for at least the first month after birth. Exclusion criteria were as follows: (1) maternal (gestational) diabetes mellitus, as the glucocorticoid system may be regulated differently (25, 26), (2) maternal use of psychopharmaceuticals or glucocorticoid medication, as this may interfere with questionnaire scoring, glucocorticoid system regulation, and maternal cortisol concentrations (27), and (3) major congenital disease of the neonate and/or a life expectancy of the neonate of <1 month (duration of the study).
To ensure the inclusion of a large enough range of stress levels among the included participants, two groups of women who delivered at term were included: a high-stress group (HS group) and a control group (CTL group). Women were included in the CTL group when they gave birth to a healthy infant at term. Women were included in the HS group when they gave birth to an infant at term who was admitted to the hospital for a minimum of 2 days. Hospitalization of the infant was considered a maternal stressor.
Recruitment took place in The Netherlands between November 2017 and December 2019. Written informed consent was obtained from all participants prior to participation. This study was approved by the Ethics Committee of the Amsterdam University Medical Centre, AMC on 2 May 2017 (METC 2017025, NL59994.018.16) and conducted in accordance with the Declaration of Helsinki.
2.2. Data collection and storage
2.2.1. Study timeline
Figure 1 shows the study timeline. Recruitment took place within the first 10 days postpartum. After the participant's recruitment, a strand of hair was collected for glucocorticoid measurements, and the participants completed a questionnaire about their general health, their pregnancy, and their lifetime stress experiences. The study collected data on 3 collection days: at postpartum (P) days 10, 17, and 24. On these collection days, women collected two saliva samples and three HM samples. After each collection day, the participants filled out a 24-h food recall questionnaire. At the end of the study, participants filled out three questionnaires about their stress experiences during the study period. See the items below for further details on sample collection, measurements, and questionnaires.
2.2.2. Hair sample collection and storage
A strand of hair (~100 hairs, 3 mm diameter) was cut by the researcher as close to the scalp as possible at the posterior vertex position. The hair was stored in the dark at room temperature until analysis. A short questionnaire was filled out by the participants in order to correct for factors influencing hair glucocorticoid concentrations. Hair samples were analyzed for cortisol and cortisone as a baseline biological stress measurement reflecting the last trimester of pregnancy (28, 29).
2.2.3. Saliva sample collection and storage
Saliva was collected two times in the morning on every collection day to measure the cortisol awakening response. Participants were instructed to chew on a swab (Salivette, Sarstedt, Nümbrecht, Germany) for 1 min. The first sample (S1) was obtained within 0–10 min after waking, and the second sample (S2) was obtained 30–45 min after waking (30). Participants were requested to write down their wake-up time and the time of saliva collection. After collection, saliva samples were sent to the study site, where they were centrifuged, aliquoted, and stored at −20°C until analysis.
2.2.4. Milk sample collection and storage
On every collection day (P10, P17, and P24), participants collected three HM samples in which concentrations of AAs and cortisol/cortisone were measured. HM cortisol/cortisone was measured to be able to directly correlate this with the HM AAs. To take into consideration, the circadian rhythm of HM cortisol and to make sure that circadian variation in HM AAs was represented in the samples (31, 32), participants were instructed to collect one HM sample in the morning, one in the afternoon, and one in the evening on each day that they collected milk. To make sure the HM sample would contain a mixture of both foremilk and hindmilk, participants were requested to fully empty one breast before feeding their infant, mix the milk, and thereafter put 5 ml of HM in a sterile polypropylene container (Sarstedt, Germany) for analysis. Participants were free to choose from which breast the milk was collected. Participants were asked to record the date and time of milk collection, the pumping method used (i.e., manually or with an electric pump), and the total amount of milk collected. Participants stored the milk samples in their freezer (−20°C) up until collection by the researcher. At the study site, HM samples were stored at −20°C until analysis.
2.3. Questionnaires
The questionnaires used in the study are described below. For the analyses, the total questionnaire scores and their ranges were used. To establish the participant's lifetime stress exposure, the participants filled out two questionnaires at the start of the study.
2.3.1. The life stressor checklist-revised
The Dutch version of the LSC-r questionnaire. This checklist is a 26-item scale to identify exposure to traumatic events or other stressful life events (33). Each item questions whether a certain event happened in the participant's life (34).
2.3.2. The youth trauma checklist
The Dutch version of the JTV questionnaire (25 items) is a self-reported inventory that provides a brief and relatively non-invasive retrospective assessment of early life traumatic experiences (35). The JTV discriminates against five domains of abuse/neglect (physical, sexual and emotional abuse, and physical and emotional neglect).
2.3.3. Twenty-four-hour food recall questionnaire
After each collection day, participants received a 24 h-recall using the digital program Compl-eat™ developed by the Department of Human Nutrition at Wageningen University (the Netherlands) (36). This questionnaire assesses the exact food intake (in grams per day) on the collection day. The Compl-eat™ web-based module was specifically designed for the Dutch population and guides participants to accurately report all foods and drinks consumed during the previous 24 h. Information on AA supplement intake was taken into account in the 24 h-recall. The mean intake for protein and all AAs of the three collection days was calculated and used as a measure of maternal intake over the study period.
A measure of psychological stress levels during the study period was obtained by three questionnaires that the participants filled out at the end of the study, concerning the levels of stress they experienced during the past month.
2.3.4. Perceived stress scale
The perceived stress scale is a validated 14-item questionnaire. The questionnaire determines the degree to which certain situations are experienced as stressful (37, 38). Each question is scored on a 5-point Likert scale.
2.3.5. Edinburgh postnatal depression scale
The Dutch version of the well-validated EPDS is a 10-item self-inventory to assess symptoms of depression and/or anxiety in women who recently gave birth (39).
2.3.6. State-trait anxiety inventory
The Dutch version of the STAI is a well-established measure of trait and state anxiety and consists of two parts. The first part of this inventory, the STAI-state (STAI-s), contains 20 items to assess anxiety at this moment, rated on a 4-point intensity scale. The second part, the STAI-trait (STAI-t), contains 20 items and assesses anxiety, in general, rated on a 4-point intensity scale (40).
2.4. Laboratory analysis
2.4.1. Hair cortisol/cortisone
For analyses, the proximal 3-cm hair segment was used. Wash and steroid extraction procedures were performed as described by Stalder et al., with some changes being made to allow analysis by liquid chromatography-tandem mass spectrometry (LC-MS/MS) (41). The lower limits of quantification were below 0.1 pg/mg for cortisol and cortisone. The inter- and intra-assay coefficients of variance were between 3.7 and 8.8%.
2.4.2. Saliva cortisol/cortisone
Cortisol and cortisone in saliva were determined using supported liquid extraction (SLE+) followed by LC-MS/MS detection. Quantification was performed using an isotope dilution, with the limit of quantification being 0.3 nmol/L. The mean intra-assay variation was 6 and 7% for cortisol and cortisone, respectively.
2.4.3. HM cortisol/cortisone
For each HM sample, cortisol and cortisone were determined using liquid extraction followed by SLE+ and LC-MS/MS detective as described earlier by van der Voorn et al. (42). Quantification was performed using an isotope dilution.
2.4.4. Protein-bound AA in HM
For the determination of BAA in HM samples, the three milk samples from 1 collection day were mixed to have a good representation of BAA concentrations during the whole day. Thereafter, 0.20 ml of diluted hydrochloric acid containing 0.5% 2-mercaptoethenol was added to the HM sample and mixed. The present oxygen was removed by flushing the headspace of the tube with nitrogen for 60 s. The protein was hydrolyzed by heating the mixture for 20–22 h. When the mixture was cooled down, 0.20 ml of sodium hydroxide solution, 2.0 ml of demi water, and 0.2 ml of internal standard (Norvaline 20 μg) were added and mixed. The mixture was centrifuged, and a small part of the liquid was filtered over a 0.45 mm polyvinylidene difluoride filter. The peak area of each AA was calculated using the LabSolutions software from Shimadzu and compared to the peak area of the internal standard (Sigma).
2.4.5. Free AA in HM
The mixed HM samples were also used to determine the free AA in HM. An ultra-fast liquid chromatography (UFLC)-based protocol was used. Each 50 μl of milk sample was mixed with 1.0 ml of internal standard solution (2.5 mg/ml of L-norvaline). This mixture was centrifuged, and 25 μl of supernatant was transferred into a sample vial. A pre-column derivatization process was carried out by adding 30 μl of o-phthalaldehyde (OPA) reagent to the vial and mixing three times with a mixing volume of 45 μl. One microliter of this OPA-derivatized sample was injected and analyzed in a UFLC system with fluorimetry to detect the signal.
Standard AA solution Sigma AA-S-18 was used for calibration. To prepare the calibration AA solution, asparagine, and tryptophan were added in to Sigma AA-S-18 stock solution to reach a concentration of 2.5 μM/ml of each AA. Next, 0.50, 1.0, 2.0, and 5.0 ml of this solution were mixed with 1.6 ml of perchloric acid and further diluted to 50 ml. The calibration AA solution was prepared to OPA derivate as described previously and measured in an UFLC system. The calibration curve was constructed from peak areas and AA concentrations. Response factors for each AA were obtained by an extra analysis of a standard AA solution containing internal standards.
2.5. Statistical analysis
Sample characteristics were described as mean with standard deviation (SD), median with 25th and 75th percentiles (Q1–Q3), or frequencies. To test differences in maternal and infant characteristics (including maternal BMI and infant sex), dietary AA intake over the study period, and stress measurements (questionnaires and cortisol) between both study groups, unpaired Student's t-tests (for continuous normally distributed data), chi-square tests (for binary categorical data), Mann–Whitney U-tests (for continuous not normally distributed data), or linear mixed models (for data that were measured at multiple time points) were used. The mean of the three 24-h recalls was used to compare the dietary intake of AA between groups, as this reflects the overall intake of the participants during the study period.
The HM cortisol area under the curve (AUC) was calculated to provide a value that better reflects HM cortisol throughout the day, which is known to follow a circadian rhythm (31). Therefore, all HM cortisol values were standardized to 7:00 a.m., 14:00, and 22:00 by regressing the time of collection to cortisol values. For each participant, we then calculated the estimated cortisol value at 07:00, 14:00, and 22:00. To do this, the following formula was used: HM cortisol in mmol/L ± unstandardized regression coefficient of all HM cortisol values * [new (standardized)] time point – real-time point) (43). Subsequently, the HM cortisol AUC for each collection day was calculated as described by Pruessner et al. (44). The cortisol value at 7:00 a.m. was considered the HM cortisol morning peak (31). The highest cortisol value of the two morning saliva samples was considered the saliva cortisol morning peak. When the time of S1 collection was >30 min after waking up, saliva values were excluded.
Analyses were performed separately for BAA and FAA. Before analysis, the HM AAs were categorized into different outcome variables: total AA, essential AA, and non-essential AA. Because AA from one precursor family can be converted into other members of this family, AAs were also grouped into precursor groups: the glutamate precursor group (glutamic acid, glutamine, and arginine), the aspartate precursor group (aspartic acid, methionine, isoleucine, threonine, and lysine), the serine precursor group (serine, glycine), the pyruvate precursor group (valine, leucine, and alanine), the aromatic precursor group (phenylalanine, tyrosine, and tryptophan), and the histidine precursor group (histidine). Methionine was analyzed separately (24).
All outcome variables were checked to see whether they were normally distributed. When variables were not distributed normally, a log transformation was performed. When participants completed <1 full day of sample collection, they were excluded from the final analysis.
To answer the research question whether and how maternal stress affects the BAA and FAA concentrations in HM, the AA outcomes as described above were compared between the HS and CTL groups. As all study time points were taken into account in the comparison, linear mixed models were used to analyze the group differences to control for within-person repeated measures. The analysis was corrected for factors differing between study groups. In addition, we tested whether maternal dietary AA intake during the study period differed between study groups. If the maternal dietary intake of a specific AA statistically differed between groups, the comparison of that specific HM AA was corrected for the maternal intake. As HM AA concentrations differ between the different weeks and stages of lactation, the interaction between the study time point and study group was investigated and reported.
To answer the research question of whether HM cortisol concentrations (cortisol AUCs) are related to HM AA concentrations, we performed a secondary analysis. The relationship between HM AA and HM cortisol AUCs was investigated independently of the study group. This relationship was only investigated for total AA, essential AA, non-essential AA, and methionine in HM. As all study time points were taken along in this secondary analysis, linear mixed models were used to control for within-person repeated measures. The analysis was corrected for potential confounding factors that have been shown to influence the AA composition of HM in the previous literature: AA dietary intake and maternal BMI (17, 45–47). Due to the explorative nature of the study, the statistical analyses were not corrected for multiple testing. To reduce the number of statistical tests and the likelihood of a type 1 error, most of the separate AAs were categorized into their precursor families and analyzed as such. Statistical analyses were two-sided. A p-value of <0.05 was considered statistically significant. Statistical analyses were performed using IBM SPSS Statistics for Windows, version 27. GraphPad Prism 9 for Windows was used to display the results.
3. Results
3.1. Maternal characteristics, food intake, and stress measures
In total, 86 lactating women were included in the CTL group and 30 in the HS group. Nineteen women stopped participating before the end of the study due to various reasons (see Figure 2 for drop-out reasons), 13 women (15%) in the CTL group, and six women (20%) in the HS group (Figure 2). Characteristics and dietary protein and AA intake of the participants are shown in Tables 1, 2, respectively. Maternal baseline characteristics, including maternal age, BMI, ethnicity, education level, alcohol consumption, smoking, dietary habits, and mode of delivery, did not differ between the study groups. Maternal protein/AA intake during the study period was also similar between both study groups. In addition, the HM pumping method used and the storage time of the samples did not differ between study groups. The only difference between study groups was that mothers in the HS group gave birth to a male infant more often than mothers in the CTL group, 75 and 49%, respectively (p = 0.048); the birthweights did not differ between study groups. In the HS group, hospitalization duration ranged between 2 and 12 days, with a median of 7 days. The duration of hospitalization in this group was not associated with maternal stress scores (p = 0.282 for PSS score).
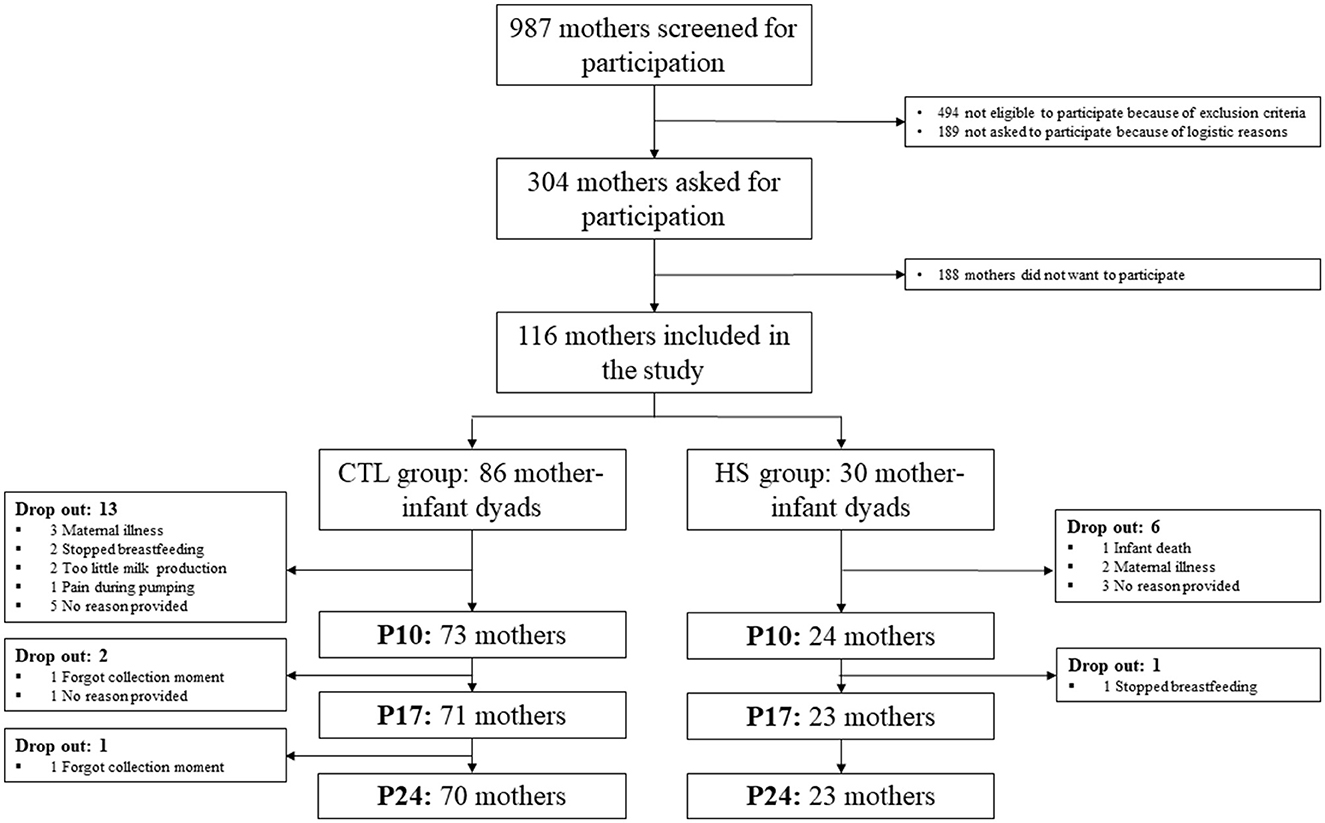
Figure 2. Flowchart of the study population. CTL group, control group; HS group, high-stress group; p, postpartum day.
Lifetime psychological (JTV and LSC-r questionnaire) and biological stress (hair cortisol) measurements were the same between study groups (Table 3). Perceived stress during the study period was higher in the HS group, and women in the HS groups scored higher on the PSS, EPDS, STAI-s, and STAI-t (p < 0.01) (Table 3). There were no differences in the HM cortisol AUCs or the HM/saliva cortisol morning peak concentrations between study groups.
3.2. BAA concentrations in HM are higher in the HS group
Table 4 shows the concentrations of BAAs in HM per study group on all collection days, and Figures 3A, B depicts the BAA dynamics over the study period per study group. Total concentrations of BAAs were higher in the milk of women in the HS group compared to the CTL group [819 (92.4, 1,547); p = 0.028]. This was also the case for essential BAAs [476 (55.8, 896); p = 0.027] and non-essential BAAs [363 (55.9, 669); p = 0.021] and for the concentrations of the BAA precursor groups (p < 0.035), except for the glutamate precursor group, which showed the same concentrations in both study groups. There were no interactions between the study group and study time point (Table 3; Figure 3). All statistical analyses were corrected for infant sex.
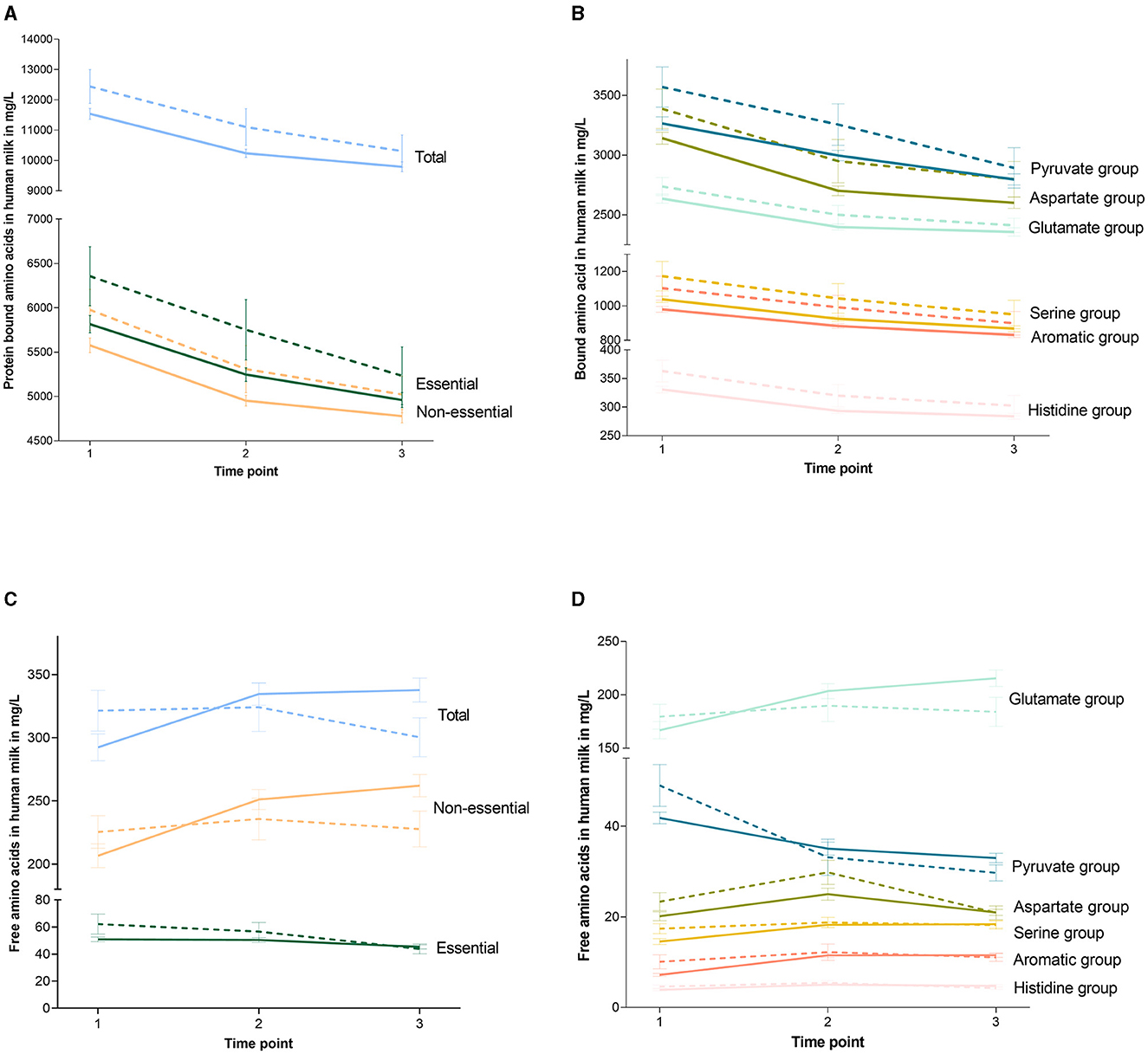
Figure 3. Dynamics of amino acids over the study period per study group. Graphs show the concentrations of the protein-bound amino acids (A, B) and the free amino acids (C, D) at the different collection moments. The continued line indicates the control group and the dotted line indicates the high-stress group. Error bars indicate the standard error of the mean. Glutamate family members contain glutamine, arginine, and proline. Aspartate family members contain asparagine, methionine, threonine, isoleucine, and lysine. Serine family members contain serine, glycine, and cysteine. Pyruvate family members contain valine, alanine, and leucine. Aromatic family members contain phenylalanine and tyrosine. Histidine family members contain histidine.
3.3. FAA concentrations in HM did not differ between study groups
Table 5 shows the concentrations of FAA in HM per study group on all collection days, and Figures 3C, D depicts the FAA dynamics over the study period per study group. Total concentrations of FAA over the entire study group did not differ between the HS and CTL groups, nor did concentrations of essential FAA, non-essential FAA, and the concentrations of the FAA precursor family groups. All statistical analyses were corrected for infant sex.
3.4. Bound methionine does not differ between study groups, free methionine is higher in the HS group
Despite higher concentrations of BAA of the aspartate precursor family in the HS group [255 (46.0, 465); p = 0.017], to which protein-bound methionine belongs, protein-bound methionine concentrations did not differ between the HS and CTL groups. In contrast, free methionine concentrations were higher in the HS group compared to the control group [0.49 (0.21, 0.76); p = 0.001]. All statistical analyses were corrected for infant sex.
3.5. Dynamics of FAAs in HM over the first month of lactation differ between study groups
In general, the BAA concentrations in HM decreased over the study period (p < 0.001), while the FAA concentrations increased (p = 0.019). As indicated in Table 5, for total FAA, there was an interaction between the study group and study time point. This was the same for essential and non-essential FAAs, as well as glutamate, pyruvate, and aromatic precursor groups. The different dynamics over the study period between the HS and CTL groups are depicted in Figures 3C, D. All statistical analyses were corrected for infant sex.
3.6. HM BAAs are positively associated with HM cortisol levels
Total BAA concentrations, as well as essential BAAs and non-essential BAAs, were positively associated with the HM cortisol AUC [5.54 (0.73, 10.35); p = 0.024, 2.66 (0.01, 5.30); p = 0.049 and 3.07 (0.85, 5.29); p = 0.007, respectively]. Protein-bound methionine was not associated with the HM cortisol AUC. Total FAA, essential FAA, non-essential FAA, and free methionine were also not associated with the HM cortisol AUC, at the separate study time points.
4. Discussion
In this study, we explored associations between maternal stress and the AA composition of HM. We demonstrated that perceived and biological maternal stresses in the first month postpartum were positively associated with the concentrations of BAAs in HM. In particular, BAA concentrations were increased in the HM of mothers with high-stress levels, with the exception of bound methionine. Methionine was, contrary to our hypothesis, not associated with maternal stress. However, while the overall concentrations of FAAs in HM did not differ between study groups, free methionine was higher in the HM of mothers with high levels of perceived stress.
Our results are in line with previous animal experimental studies (22, 23). In stressed dams, an increase in some of the milk BAAs was observed, while milk FAAs were overall not affected by stress exposure. To date, human studies on the effect of maternal stress on the AA composition of HM are scarce. One study investigated the influence of maternal postpartum stress on the metabolome of HM, including three AAs, and found that high-stress levels were positively associated with the concentrations of tryptophan and tyrosine. However, after correction for multiple testing, these associations disappeared (16). Another study by Ziomkiewicz et al. found that proteins in HM were not associated with maternal psychological or biological stress (15). This difference may be attributed to the fact that Ziomkiewicz et al. measured whole proteins instead of specific AAs.
The biological mechanism behind the observed associations between maternal stress and HM AA composition is not yet clear. In fact, the process behind the transport of AAs from the maternal bloodstream into the mammary gland and into the HM is complex and not yet fully understood (21). Both BAAs and FAAs in HM can be transported from the maternal circulation, and BAAs can be synthesized out of FAAs by the mammary gland itself (21). It has further been demonstrated, in contrast to our current results in HM, that almost all AAs in the maternal plasma are decreased as a result of maternal stress (18–20). The origin of such a reduction is not fully elucidated. It might be due to the influence of catecholamines produced during stress, which exert an anti-insulin effect on the metabolism of AAs (18–20). As we observed higher concentrations of HM BAAs in the HS group but no association between stress and FAAs in HM, this may indicate an increased active transport of AAs from the maternal circulation into HM and/or an increased synthesis of BAAs in the mammary gland.
Exactly how and to what extent stress influences transport processes also remains to be elucidated. As previous research showed associations between glucocorticoid levels and the expression of certain AA transporters in the mammary gland, e.g., the system L and the system y+ amino acid transporters, one could hypothesize that cortisol is involved in the mechanisms leading to these stress-induced changes in HM (21, 48). Indeed, we observed a positive association between cortisol levels and BAA levels in HM, which was also found in previous animal experimental research (22). A possible explanation for the fact that cortisol values were not elevated in the HS group but were positively associated with HM BAAs might be that the sample size of the HS group was not sufficient to detect a statistically significant difference. In fact, there was a “trend” toward a higher HM cortisol AUC in the HS group (p = 0.074).
We further focused specifically on methionine, as in mice, maternal stress has led to reduced methionine in the brain and plasma of the offspring, which was associated with later-life cognitive deficits (24). Contrary to our hypothesis, bound methionine levels were not decreased in the HM samples in our HS group, and free methionine levels were even higher in mothers with high perceived stress levels. This was an unexpected result, but it can be speculated that the absence of an increase in protein-bound methionine is the result of stress-induced breakdown into free methionine, which would result in an increase in the free form of this essential AA. In addition, another possible explanation for the absence of an increase in protein-bound methionine is that while some transporters of AAs in the mammary gland seem to be upregulated under the influence of cortisol, one of the three transporters that facilitate the transport of methionine into HM is rather downregulated by glucocorticoids (21, 48). Unfortunately, the influence of cortisol on the other two methionine transporters remains so far unknown (21).
Increased concentrations of BAA in the HM of mothers in the HS group may be due to the fact that mothers with high levels of stress produce less milk, in general, compared to mothers with lower levels of stress (49–51). When AA transport into milk is maintained at the same level, the concentrations of BAA in HM will then increase. This might lead to the appropriate transmission of these important nutrients to the infant. On the other hand, this would also mean that the infant might be at risk of receiving inappropriate amounts of methionine, which was not increased in HM under stressful circumstances, as was shown in our results. As we did not measure the total milk volume production during the day or AA concentrations in the infant, this remains speculation and awaits future research.
During lactation, milk-specific proteases break down HM proteins into FAAs. HM further contains protease activators and protease inhibitors (52). Over the first month of lactation, protease inhibitors decrease, which leads to an increase in total FAAs, but subsequently, this also contributes to a decrease in HM BAAs over time (52). Indeed, a decrease in BAA and an increase in total FAA over time were observed for the mothers in our study, except for the concentrations of HM FAAs of the mothers in the HS group, where the total FAA levels decreased over time. The different dynamics of FAA observed in the HS group might be due to a different regulation or production of proteases under the influence of stress. Another explanation for the decrease in total HM FAA under stressful circumstances is that FAAs are more often bound in response to stress, resulting in the increase in HM BAA observed in the HS group.
The strengths of our current study are its longitudinal design and the timing and frequency of HM sample collection. The first month postpartum is a sensitive time window that has been frequently missed in earlier HM research, but since breastfed infants depend on HM during this period as their only source of nutrition these first weeks after birth represent a very important period. Furthermore, the fact that mothers were exposed to a stressor (i.e., infant hospitalization) ensured that the study population contained participants with established high levels of perceived stress. Additionally, extensive information on both maternal psychological and biological stress was collected, and the HS and CTL groups were similar at baseline with regard to lifetime stress levels or other important characteristics that could have influenced the relationship between stress and HM AA levels. The limitations of this study are, first, the relatively small sample size, especially in the HS group. Second, current perceived stress scores were only measured once, i.e., at the end of the study. Therefore, we were not able to investigate whether the different dynamics of FAA in the HS group were related to changes in the amount of stress experienced at that exact moment. Moreover, because of the exploratory nature of this study, we decided not to correct for multiple testing, even though we did perform a relatively large number of statistical tests, which increases the likelihood of a type 1 error. Therefore, our results should be interpreted with caution, and future studies should demonstrate whether these findings can be replicated. In addition, no reliable information was collected about infant feeding modes and additional feeding practices. The relatively high loss to follow-up and the fact that this was higher in the HS group compared to the CTL group (19 vs. 23%, respectively) may have caused selection bias. Dropout of women who may have been more stressed, especially in the HS group, may have led to an underestimation of associations between maternal stress and AA. Finally, selection bias might have contributed to the fact that our cohort mostly consisted of healthy and highly educated women. Furthermore, the majority of our participants were of Western ethnic background. This may limit the generalizability of the results.
The results of our study suggest that mothers with high-stress levels have increased concentrations of BAA in their milk. The increased concentrations of BAA that we found may partially compensate for the higher nutritional requirements of infants under stressful circumstances. However, as impaired growth and development have been observed in children of mothers with high amounts of stress in the postnatal period, this increase in AA in the HM might not be sufficient to maintain optimal growth and development. Moreover, the increase was not observed for protein-bound methionine or total HM FAA.
In conclusion, findings from this unique prospective cohort study suggest that there is a relationship between maternal stress in the first month postpartum and the AA concentrations of HM during this time. Our findings emphasize the importance of the maternal psychological state during lactation. In the last few years, attention to the prevention of the detrimental consequences of stressful experiences in early life has increased. Stress reduction programs for children and parents have been developed, such as family-integrated care and relaxation therapy using relaxing music (53–59), with one study demonstrating effects on milk composition (59). Future research should replicate our findings, investigate the entire period of lactation, and focus on to what extent these stress-induced changes in human milk composition are of clinical importance for short- and long-term infant development and health.
Data availability statement
The raw data supporting the conclusions of this article will be made available by the authors, without undue reservation.
Ethics statement
The studies involving human participants were reviewed and approved by Ethics Committee of the Amsterdam University Medical Centre, AMC. The patients/participants provided their written informed consent to participate in this study.
Author contributions
EN, JG, SR, LS, and AK designed the research. HJ and EN conducted the research. LS, JG, and AK provided essential materials. HJ, JH, and SR analyzed the data or performed statistical analysis. JH, HJ, BK, SR, and AK accessed, verified, and interpreted the data. HJ, SR, and AK wrote the first version of the manuscript. AK had primary responsibility for the final content. All authors critically read and contributed to finalizing the manuscript, had full access to all the data in the study, and accept responsibility to submit it for publication.
Funding
This research was funded by the NWO Food, Cognition, and Behavior program (057-14-003) and by the Amsterdam Brain and Cognition. PL was supported by the Center for Urban Mental Health.
Acknowledgments
The authors gratefully acknowledge the assistance of Gerrit Witte for the HM amino acid analysis performed on the samples at Danone Nutricia Research in Utrecht and Bernd Stahl for his advice on the study design and analyses. The authors would like to thank the Dutch Human Milk Bank for helping with the transport of study samples and all of the parents who participated in the Amsterdam Mother's Milk study.
Conflict of interest
JG is the founder and director of the Dutch National Human Milk Bank and a member of the National Health Council. JG has been a member of the National Breastfeeding Council from March 2010 to March 2020. LS was employed by Danone Nutricia Research.
The remaining authors declare that the research was conducted in the absence of any commercial or financial relationships that could be construed as a potential conflict of interest.
Publisher's note
All claims expressed in this article are solely those of the authors and do not necessarily represent those of their affiliated organizations, or those of the publisher, the editors and the reviewers. Any product that may be evaluated in this article, or claim that may be made by its manufacturer, is not guaranteed or endorsed by the publisher.
References
1. Chan JC, Nugent BM, Bale TL. Parental advisory: maternal and paternal stress can impact offspring neurodevelopment. Biol Psychiatry. (2018) 83:886–94. doi: 10.1016/j.biopsych.2017.10.005
2. Oyetunji A, Chandra P. Postpartum stress and infant outcome: a review of current literature. Psychiatry Res. (2020) 284:112769. doi: 10.1016/j.psychres.2020.112769
3. Simons SSH, Zijlmans MAC, Cillessen AHN, de Weerth C. Maternal prenatal and early postnatal distress and child stress responses at age 6. Stress. (2019) 22:654–63. doi: 10.1080/10253890.2019.1608945
4. Di Benedetto MG, Bottanelli C, Cattaneo A, Pariante CM, Borsini A. Nutritional and immunological factors in breast milk: a role in the intergenerational transmission from maternal psychopathology to child development. Brain Behav Immun. (2020) 85:57–68. doi: 10.1016/j.bbi.2019.05.032
5. Reemst K, Ruigrok SR, Bleker L, Naninck EFG, Ernst T, Kotah JM, et al. Sex-dependence and comorbidities of the early-life adversity induced mental and metabolic disease risks: Where are we at? Neurosci Biobehav Rev. (2022) 138:104627. doi: 10.1016/j.neubiorev.2022.104627
6. WHO. Essential Nutrition Actions: Improving Maternal, Newborn, Infant and Young Child Health and Nutrition. WHO Guidelines Approved by the Guidelines Review Committee. Geneva (2013).
7. Victora CG, Bahl R, Barros AJ, Franca GV, Horton S, Krasevec J, et al. Breastfeeding in the 21st century: epidemiology, mechanisms, and lifelong effect. Lancet. (2016) 387:475–90. doi: 10.1016/S0140-6736(15)01024-7
8. Sankar MJ, Sinha B, Chowdhury R, Bhandari N, Taneja S, Martines J, et al. Optimal breastfeeding practices and infant and child mortality: a systematic review and meta-analysis. Acta Paediatr. (2015) 104:3–13. doi: 10.1111/apa.13147
9. Andreas NJ, Kampmann B, Mehring Le-Doare K. Human breast milk: a review on its composition and bioactivity. Early Hum Dev. (2015) 91:629–35. doi: 10.1016/j.earlhumdev.2015.08.013
10. Urech C, Eussen S, Alder J, Stahl B, Boehm G, Bitzer J, et al. Levels of n-3 and n-6 fatty acids in maternal erythrocytes during pregnancy and in human milk and its association with perinatal mental health. Nutrients. (2020) 12:2773. doi: 10.3390/nu12092773
11. Keim SA, Daniels JL, Siega-Riz AM, Dole N, Herring AH, Scheidt PC. Depressive symptoms during pregnancy and the concentration of fatty acids in breast milk. J Hum Lact. (2012) 28:189–95. doi: 10.1177/0890334411424727
12. Palnizky Soffer G, Siri M, Mangel L, Mandel D, Lubetzky R. Impact of maternal anxiety on human milk macronutrients content: a prospective observational study. Breastfeed Med. (2020) 15:572–5. doi: 10.1089/bfm.2020.0034
13. Linderborg KM, Kortesniemi M, Aatsinki AK, Karlsson L, Karlsson H, Yang B, et al. Interactions between cortisol and lipids in human milk. Int Breastfeed J. (2020) 15:66. doi: 10.1186/s13006-020-00307-7
14. Hollanders JJ, Kouwenhoven SMP, van der Voorn B, van Goudoever JB, Rotteveel J, Finken MJJ. The association between breastmilk glucocorticoid concentrations and macronutrient contents throughout the day. Nutrients. (2019) 11:259. doi: 10.3390/nu11020259
15. Ziomkiewicz A, Babiszewska M, Apanasewicz A, Piosek M, Wychowaniec P, Cierniak A, et al. Psychosocial stress and cortisol stress reactivity predict breast milk composition. Sci Rep. (2021) 11:11576. doi: 10.1038/s41598-021-90980-3
16. Kortesniemi M, Slupsky CM, Aatsinki AK, Sinkkonen J, Karlsson L, Linderborg KM, et al. Human milk metabolome is associated with symptoms of maternal psychological distress and milk cortisol. Food Chem. (2021) 356:129628. doi: 10.1016/j.foodchem.2021.129628
17. van Sadelhoff JHJ, Siziba LP, Buchenauer L, Mank M, Wiertsema SP, Hogenkamp A, et al. Free and total amino acids in human milk in relation to maternal and infant characteristics and infant health outcomes: The Ulm SPATZ Health Study. Nutrients. (2021) 13:2009. doi: 10.3390/nu13062009
18. Shamoon H, Jacob R, Sherwin RS. Epinephrine-induced hypoaminoacidemia in normal and diabetic human subjects: effect of beta blockade. Diabetes. (1980) 29:875–81. doi: 10.2337/diab.29.11.875
19. Taylor J, Weyers P, Harris N, Vogel WH. The plasma catecholamine stress response is characteristic for a given animal over a one-year period. Physiol Behav. (1989) 46:853–6. doi: 10.1016/0031-9384(89)90048-6
20. De Boer SF, Koopmans SJ, Slangen JL, Van der Gugten J. Plasma catecholamine, corticosterone and glucose responses to repeated stress in rats: effect of interstressor interval length. Physiol Behav. (1990) 47:1117–24. doi: 10.1016/0031-9384(90)90361-7
21. Shennan DB, Boyd CA. The functional and molecular entities underlying amino acid and peptide transport by the mammary gland under different physiological and pathological conditions. J Mamm Gland Biol Neoplasia. (2014) 19:19–33. doi: 10.1007/s10911-013-9305-5
22. Nishigawa T, Nagamachi S, Ikeda H, Chowdhury VS, Furuse M. Restraint stress in lactating mice alters the levels of sulfur-containing amino acids in milk. J Vet Med Sci. (2018) 80:503–9. doi: 10.1292/jvms.17-0661
23. Nishigawa T, Nagamachi S, Takakura M, Ikeda H. Maternal stress during the lactation period rather than the gestion period strongly influences the amino acid composition in milk and affects growth and behaviour in offspring. Kyushu Univ Inst Reposit. (2018) 63:61–70. doi: 10.5109/1909903
24. Naninck EF, Oosterink JE, Yam KY, de Vries LP, Schierbeek H, van Goudoever JB, et al. Early micronutrient supplementation protects against early stress-induced cognitive impairments. FASEB J. (2017) 31:505–18. doi: 10.1096/fj.201600834R
25. Sharma VK, Singh TG. Chronic stress and diabetes mellitus: interwoven pathologies. Curr Diabetes Rev. (2020) 16:546–56. doi: 10.2174/1573399815666191111152248
26. Kokkinopoulou I, Diakoumi A, Moutsatsou P. Glucocorticoid receptor signaling in diabetes. Int J Mol Sci. (2021) 22:11173. doi: 10.3390/ijms222011173
27. Feighner JP. Mechanism of action of antidepressant medications. J Clin Psychiat. (1999) 60:4–13.
28. Braig S, Grabher F, Ntomchukwu C, Reister F, Stalder T, Kirschbaum C, et al. The association of hair cortisol with self-reported chronic psychosocial stress and symptoms of anxiety and depression in women shortly after delivery. Paediatr Perinat Ep. (2016) 30:97–104. doi: 10.1111/ppe.12255
29. Braig S, Grabher F, Ntomchukwu C, Reister F, Stalder T, Kirschbaum C, et al. Determinants of maternal hair cortisol concentrations at delivery reflecting the last trimester of pregnancy. Psychoneuroendocrinology. (2015) 52:289–96. doi: 10.1016/j.psyneuen.2014.12.006
30. Stalder T, Kirschbaum C, Kudielka BM, Adam EK, Pruessner JC, Wust S, et al. Assessment of the cortisol awakening response: Expert consensus guidelines. Psychoneuroendocrinology. (2016) 63:414–32. doi: 10.1016/j.psyneuen.2015.10.010
31. van der Voorn B, de Waard M, van Goudoever JB, Rotteveel J, Heijboer AC, Finken MJ. Breast-milk cortisol and cortisone concentrations follow the diurnal rhythm of maternal hypothalamus-pituitary-adrenal axis activity. J Nutr. (2016) 146:2174–9. doi: 10.3945/jn.116.236349
32. Pundir S, Wall CR, Mitchell CJ, Thorstensen EB, Lai CT, Geddes DT, et al. Variation of human milk glucocorticoids over 24 hour period. J Mamm Gland Biol Neoplasia. (2017) 22:85–92. doi: 10.1007/s10911-017-9375-x
33. Wolfe JK, Brown P, Chrestman K, Levin K. The Life Stressor Checklist-Revised (LSC-R). [Measurement Instrument]. National Center for PTSD (1997). Available online at: http://www.ptsd.va.gov
34. Choi KR, Kim D, Jang EY, Bae H, Kim SH. Reliability and validity of the Korean version of the lifetime stressor checklist-revised in psychiatric outpatients with anxiety or depressive disorders. Yonsei Med J. (2017) 58:226–33. doi: 10.3349/ymj.2017.58.1.226
35. Bernstein DP, Stein JA, Newcomb MD, Walker E, Pogge D, Ahluvalia T, et al. Development and validation of a brief screening version of the Childhood Trauma Questionnaire. Child Abuse Negl. (2003) 27:169–90. doi: 10.1016/S0145-2134(02)00541-0
36. Meijboom S, van Houts-Streppel MT, Perenboom C, Siebelink E, de Wiel AMV, Geelen A, et al. Evaluation of dietary intake assessed by the Dutch self-administered web-based dietary 24-h recall tool (Compl-eatTM) against interviewer-administered telephone-based 24-h recalls. J Nutr Sci. (2017) 6:e49. doi: 10.1017/jns.2017.45
37. Cohen S, Kamarck T, Mermelstein R. A global measure of perceived stress. J Health Soc Behav. (1983) 24:385–96. doi: 10.2307/2136404
38. Yokokura A, Silva A, Fernandes JKB, Del-Ben CM, Figueiredo FP, Barbieri MA, et al. Perceived Stress Scale: confirmatory factor analysis of the PSS14 and PSS10 versions in two samples of pregnant women from the BRISA cohort. Cad Saude Pub. (2017) 33:e00184615. doi: 10.1590/0102-311x00184615
39. Cox JL, Holden JM, Sagovsky R. Detection of postnatal depression. Development of the 10-item Edinburgh Postnatal Depression Scale. Br J Psychiatry. (1987) 150:782–6. doi: 10.1192/bjp.150.6.782
40. Guillen-Riquelme A, Buela-Casal G. Meta-analysis of group comparison and meta-analysis of reliability generalization of the State-Trait Anxiety Inventory Questionnaire (STAI). Rev Esp Salud Pub. (2014) 88:101–12. doi: 10.4321/S1135-57272014000100007
41. Stalder T, Kirschbaum C, Alexander N, Bornstein SR, Gao W, Miller R, et al. Cortisol in hair and the metabolic syndrome. J Clin Endocrinol Metab. (2013) 98:2573–80. doi: 10.1210/jc.2013-1056
42. van der Voorn B, Martens F, Peppelman NS, Rotteveel J, Blankenstein MA, Finken MJ, et al. Determination of cortisol and cortisone in human mother's milk. Clin Chim Acta. (2015) 444:154–5. doi: 10.1016/j.cca.2015.02.015
43. Goedhart G, Vrijkotte TG, Roseboom TJ, van der Wal MF, Cuijpers P, Bonsel GJ. Maternal cortisol and offspring birthweight: results from a large prospective cohort study. Psychoneuroendocrinology. (2010) 35:644–52. doi: 10.1016/j.psyneuen.2009.10.003
44. Pruessner JC, Kirschbaum C, Meinlschmid G, Hellhammer DH. Two formulas for computation of the area under the curve represent measures of total hormone concentration versus time-dependent change. Psychoneuroendocrinology. (2003) 28:916–31. doi: 10.1016/S0306-4530(02)00108-7
45. Saben JL, Sims CR, Pack L, Lan R, Borsheim E, Andres A. Infant intakes of human milk branched chain amino acids are negatively associated with infant growth and influenced by maternal body mass index. Pediatr Obes. (2022) 17:e12876. doi: 10.1111/ijpo.12876
46. Wurtman JJ, Fernstrom JD. Free amino-acid, protein, and fat contents of breast-milk from guatemalan mothers consuming a corn-based diet. Early Hum Dev. (1979) 3:67–77. doi: 10.1016/0378-3782(79)90021-5
47. Motil KJ, Thotathuchery M, Bahar A, Montandon CM. Marginal dietary-protein restriction reduced nonprotein nitrogen, but not protein nitrogen, components of human-milk. J Am Coll Nutr. (1995) 14:184–91. doi: 10.1080/07315724.1995.10718492
48. Shotwell MA, Kilberg MS, Oxender DL. The regulation of neutral amino acid transport in mammalian cells. Biochim Biophys Acta. (1983) 737:267–84. doi: 10.1016/0304-4157(83)90003-5
49. Lau C. Effects of stress on lactation. Pediatr Clin N Am. (2001) 48:221–34. doi: 10.1016/S0031-3955(05)70296-0
50. Dewey KG. Maternal and fetal stress are associated with impaired lactogenesis in humans. J Nutr. (2001) 131:3012S−5S. doi: 10.1093/jn/131.11.3012S
51. Stuebe AM, Grewen K, Meltzer-Brody S. Association between maternal mood and oxytocin response to breastfeeding. J Womens Health. (2013) 22:352–61. doi: 10.1089/jwh.2012.3768
52. Dallas DC, Murray NM, Gan J. Proteolytic systems in milk: perspectives on the evolutionary function within the mammary gland and the infant. J Mamm Gland Biol Neoplasia. (2015) 20:133–47. doi: 10.1007/s10911-015-9334-3
53. Urizar GG Jr, Yim IS, Rodriguez A, Schetter CD. The SMART moms program: a randomized trial of the impact of stress management on perceived stress and cortisol in low-income pregnant women. Psychoneuroendocrinology. (2019) 104:174–84. doi: 10.1016/j.psyneuen.2019.02.022
54. Lenz B, Eichler A, Schwenke E, Buchholz VN, Hartwig C, Moll GH, et al. Mindfulness-based stress reduction in pregnancy: an app-based programme to improve the health of mothers and children (MINDFUL/PMI Study). Geburtshilfe Frauenheilkd. (2018) 78:1283–91. doi: 10.1055/a-0677-2630
55. van Veenendaal NR, van Kempen A, Franck LS, O'Brien K, Limpens J, van der Lee JH, et al. Hospitalising preterm infants in single family rooms versus open bay units: a systematic review and meta-analysis of impact on parents. EClinicalMedicine. (2020) 23:100388. doi: 10.1016/j.eclinm.2020.100388
56. Franck LS, O'Brien K. The evolution of family-centered care: From supporting parent-delivered interventions to a model of family integrated care. Birth Defects Res. (2019) 111:1044–59. doi: 10.1002/bdr2.1521
57. van Veenendaal NR, Heideman WH, Limpens J, van der Lee JH, van Goudoever JB, van Kempen A, et al. Hospitalising preterm infants in single family rooms versus open bay units: a systematic review and meta-analysis. Lancet Child Adolesc Health. (2019) 3:147–57. doi: 10.1016/S2352-4642(18)30375-4
58. Keith DR, Weaver BS, Vogel RL. The effect of music-based listening interventions on the volume, fat content, and caloric content of breast milk-produced by mothers of premature and critically ill infants. Adv Neonatal Care. (2012) 12:112–9. doi: 10.1097/ANC.0b013e31824d9842
Keywords: breast milk, lactation, postpartum stress, amino acid, early life stress
Citation: Juncker HG, Naninck EFG, van Keulen BJ, Harinck JE, Schipper L, Lucassen PJ, van Goudoever JB, de Rooij SR and Korosi A (2023) Maternal stress is associated with higher protein-bound amino acid concentrations in human milk. Front. Nutr. 10:1165764. doi: 10.3389/fnut.2023.1165764
Received: 14 February 2023; Accepted: 10 August 2023;
Published: 07 September 2023.
Edited by:
Julio Villena, CONICET Centro de Referencia para Lactobacilos (CERELA), ArgentinaReviewed by:
Sylvie Vancassel, INRA Centre Bordeaux-Aquitaine, FranceLaura Galante, Swansea University, United Kingdom
Sonia Hernández-Cordero, Ibero American University, Mexico
Copyright © 2023 Juncker, Naninck, van Keulen, Harinck, Schipper, Lucassen, van Goudoever, de Rooij and Korosi. This is an open-access article distributed under the terms of the Creative Commons Attribution License (CC BY). The use, distribution or reproduction in other forums is permitted, provided the original author(s) and the copyright owner(s) are credited and that the original publication in this journal is cited, in accordance with accepted academic practice. No use, distribution or reproduction is permitted which does not comply with these terms.
*Correspondence: Aniko Korosi, a.korosi@uva.nl