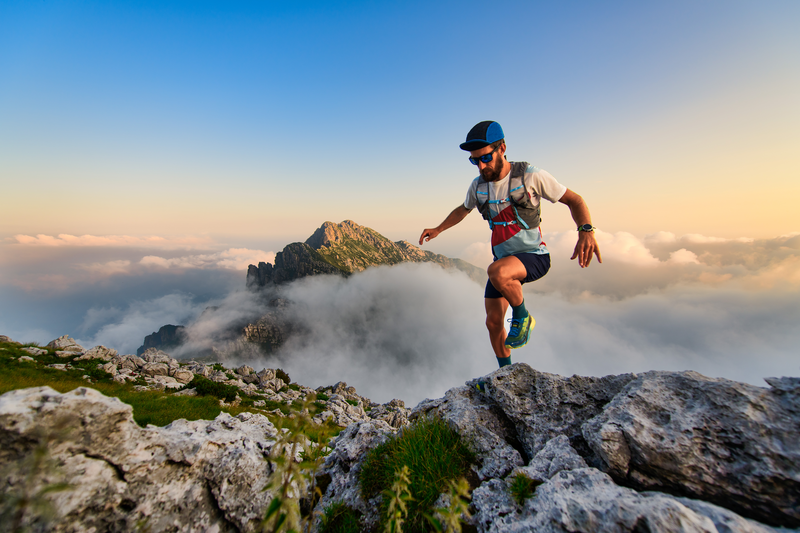
94% of researchers rate our articles as excellent or good
Learn more about the work of our research integrity team to safeguard the quality of each article we publish.
Find out more
REVIEW article
Front. Nutr. , 15 May 2023
Sec. Nutritional Immunology
Volume 10 - 2023 | https://doi.org/10.3389/fnut.2023.1161894
This article is part of the Research Topic Microbiota, Nutrition and Stress: Modulators of Immunity View all 11 articles
Severe Acute Respiratory Syndrome Coronavirus-2 (SARS-COV-2) may cause inflammation and increased cytokine secretion. Dietary factors may play an important role in enhancing the immune responses against infectious diseases such as SARS-COV-2. This narrative review aims to determine the effectiveness of macronutrients and probiotics to improve immunity in SARS-COV-2 patients. Dietary proteins may boost pulmonary function in SARS-COV-2 patients through inhibitory effects on the Angiotensin-converting enzyme (ACE) and reduce Angiotensin (ANG-II). Moreover, omega-3 fatty acids may improve oxygenation, acidosis, and renal function. Dietary fiber may also produce anti-inflammatory effects by reducing the level of high-sensitivity C-Reactive Protein (hs-CRP), Interleukin (IL-6), and Tumor necrosis factor (TNF-α). In addition, some evidence indicates that probiotics significantly improve oxygen saturation which may enhance survival rate. In conclusion, the consumption of a healthy diet including adequate macronutrients and probiotic intake may decrease inflammation and oxidative stress. Following this dietary practice is likely to strengthen the immune system and have beneficial effects against SARS-COV-2.
Severe Acute Respiratory Syndrome Coronavirus 2 (SARS-COV-2) is a viral infectious disease that in the previous 2 years has caused a worldwide pandemic (1). In December 2019 (Wuhan, China) SARS-COV-2 was first reported and characterized as a pandemic in March 2020 by the World Health Organization (WHO) (2). Millions of people worldwide have been infected with SARS-COV-2, while over six million people have died from this disease (3). The symptoms of COVID-19 vary from asymptomatic to mild upper respiratory tract symptoms and can progress to pneumonia. Patients with severe pneumonia rapidly deteriorate over a short period and require advanced medical support (4–6). SARS-COV-2 colonizes the respiratory tract but can also invade the gastrointestinal tract, neurological system, kidneys, and other organs (7, 8). SARS-COV-2 utilizes the host cell membrane receptor ACE2 to enter the virus into lymphocytes, monocytes, pulmonary alveoli, and esophageal epithelial cells (9). The cytokine storm can be induced by increases in the production of pro-inflammatory cytokines, such as IL-6, IL-1, and TNF-α, in which a hyperactive immune response is experienced. The effects of this response include vessel and lung alveoli damage, and systemic organ failure, such as acute respiratory syndrome (ARDS), which has been associated with high rates of mortality (10).
Immunity to viruses can be influenced by several factors such as genetics (11), vaccination history (12), illness, medications, sex (13), stage of life (e.g., pregnancy, infancy, and old age) (14), and diet (15). Specifically, an adequate intake of some dietary components is essential for developing, supplying, and expressing immune responses (16). A balanced diet of macronutrients (i.e., carbohydrates, protein, and fats) has anti-inflammatory effects and can affect different stages of the immune response. Consequently, an unbalanced diet of macronutrients can affect innate and adaptive immunity, hence making people more susceptible to infections and severe clinical conditions (17).
Recent studies reported that critically ill patients with SARS-COV-2 are at high risk for malnutrition (18). In this context, a recent study emphasized the supportive role of dietary supplementation in SARS-COV-2 patients (19). Therefore, following a well-balanced diet in combination with certain dietary supplements may strengthen and optimise the function of the immune system (20). Particular types of supplements shown to have antiviral activities against common respiratory viruses are probiotics. These dietary supplements can regulate cytokine secretion and affect the immune response (20, 21). At present there is limited evidence on macronutrients and probiotics intake in patients with SARS-COV-2. The aim of this narrative review was to examine the effects of macronutrients and probiotics on the immune system in patients with SARS-COV-2.
Proteins are essential to life because they facilitate biochemical reactions and enzyme production. They also act as cellular signals in the form of hormones and cytokines (22). An adequate protein intake is necessary for antibody production in the body. An adult requires 0.8 g of protein per kg of body weight to meet the recommended dietary allowance (RDA) (23). Key amino acids for stimulating the immune system include arginine and glutamine (24). Arginine serves as the vital substrate for synthesizing nitric oxide by macrophages and endows them with pro-inflammatory and microbicidal properties (25). As for glutamine, this amino acid is used as an energy source for the immune cells similar to glucose (26).
To defeat the barrier and bind the host cells in the alveoli, SARS-COV-2 utilizes ACE2 (membrane-bound enzyme localized on type II pneumocystis) and cellular serine protease transmembrane protease, serine 2 (TMPRSS2) to prime and replicate in infected organisms (27). ACE2 is expressed in endothelial cells including the heart, kidneys, and intestines (28). When the SARS-COV-2 has entered ACE2-positive cells, there is a down-regulation of the enzyme (29). ACE2 usually binds angiotensin II, a molecule recently certified as a critical player in the conventional renin-angiotensin system (RAS) which promotes inflammation, oxidative stress, and apoptosis (23, 30). The pro-inflammatory angiotensin II is converted into the anti-inflammatory angiotensin 1–7 by binding the enzyme to angiotensin receptors, which exerts a pro-inflammatory effect (23). Therefore, higher production of angiotensin 1–7 can protect the endothelial barriers of the lungs, kidneys, heart, and intestines supporting patients with viral respiratory tract infections from harmful inflammation (31). Dietary peptides with inhibitory effects on the angiotensin-converting-I enzyme (ACE-I) can significantly reduce angiotensin (ANG-II). Dietary-derived peptides can contribute to the downstream ANG-II function. The lactoferrin-released tetra-peptide RPYL (62% of concentration 300 μmol/l) has been found to have inhibitory effects on ANG-II vasoconstriction (32). Additionally, lysine has been found to down-regulate the level of ANG-II (33).
Asymptomatic and mild SARS-COV-2 patients treated with lactoferrin (a milk-derived 80-kDa glycoprotein) had faster clinical recovery compared to untreated patients (34). Therefore, lactoferrin (Lf) may be a safe treatment combined with other therapies for COVID-19 patients. A retrospective study focused on the antiviral activity of Lf found that SARS-CoV-2 RNA was negativized faster in Lf treated versus untreated patients (35).
Evidence from previous review studies indicates that a diet that positively impacts immune function contains adequate amounts of protein, particularly glutamine, arginine, and branched-chain amino acids (BCAAs) (36, 37). Dietary intake of anserine, carnosine, and 4-hydroxyproline has been shown to improve the body’s immunological defenses against bacteria, fungi, parasites, and viruses (38). Soy protein enhances cellular immunity through a reduction in TNF-α concentrations (39). However, primary sources of amino acids include fish, meat, and poultry. Therefore ensuring that the intake of peptides is optimised may improve respiratory function, symptoms, and fatigue in SARS-COV-2 patients (40). Support for this recommendation is shown by impaired immunity with protein-deficient diets, which would increase the risk of COVID-19 infection (26) (Figure 1).
Patients with SARS-COV-2 receiving enteral nutrition for >7 days where consistent levels of adequate protein and energy are received have a lower death rate compared to patients with suboptimal protein intake (32). Additionally, patients who died of SARS-COV-2 in the hospital were found to have a lower protein and energy intake compared to patients that survived (41). It could be postulated that the intake of protein and energy in critical status patients with SARS-COV-2 hospitalized in ICU are suboptimal. Therefore, it would be prudent that patients with SARS-COV-2 consumed higher protein and energy intake in the early acute phase increasing their chances of survival and lowering the risk of in-hospital mortality.
Dietary intake of fatty acids positively influences the immune response. The two essential fatty acids include omega-3 and omega-6 fatty acids. Additionally, long-chain polyunsaturated fatty acids (PUFA) need to be obtained from foods in the diet since they cannot be synthesized by the human body (42). The omega-3 fatty acids essentially comprise of α-linolenic acid (ALA) from plant sources and docosahexaenoic acid (DHA) and eicosapentaenoic acid (EPA) from fish and seafood sources (43). Anti-inflammatory responses in the body have been shown with the consumption of omega-3 fatty acids (44). In contrast, omega-6 fats mainly provide energy to the body, but the population should ideally receive more omega-3 fats (45). The ratio of omega-6 and omega-3 fatty acids in diets should be between 1:1–4:1.
EPA and arachidonic acid (ARA) compete with the lipoxygenase and cyclooxygenase enzymes to synthesize eicosanoids [prostaglandins (PG), thromboxane (TXA), leukotrienes (LT), hydroxylated fatty acids, and lipoxins] and lipid mediators. ARA is a precursor for pro-inflammatory eicosanoids that are both immunosuppressive and thrombotic (46). EPA converts eicosanoids to antithrombotics. EPA-derived LTB5 has a less chemotactic effect on neutrophils than ARA-derived LTB4. Therefore, EPA has a lower pro-inflammatory response than ARA. LTB4 is a critical pro-inflammatory mediator in cell proliferation and immune response (16).
Omega-3 fatty acids alter the phospholipid bilayer of the cell membrane, thus preventing viral entry. EPA gets incorporated into the plasma membrane and affects the clumping of toll-like receptors. This results in inhibiting signals that activate NF-kB, producing fewer pro-inflammatory mediators, and finally, reducing complications of SARS-COV-2 infection (33, 38). Another mechanism by which Omega-3 fatty acids reduce inflammation levels is via the inhibition of leukocyte chemotaxis, expression of adhesion molecules, and interaction between leukocytes and endothelial (26, 40). They also have antiviral effects through the inhibition of influenza virus replication. Furthermore, the use of omega-3 fatty acids is shown to improve oxygenation in SARS-COV-2 patients (47). Consistent with this finding is evidence that omega-3 fatty acids via parenteral and enteral means could improve oxygenation in SARS-COV-2 patients, although currently, the evidence is insufficient (48).
Supplementing with omega-3 fatty acids might be beneficial in managing inflammation-mediated clinical symptoms in SARS-COV-2 patients (49). Omega-3 fatty acids are precursors of specialized pro-resolution mediators (SPMs) that present long-lasting analgesic and anti-inflammatory effects (50). As such, omega-3 fatty acids are used for the clinical management of patients with SARS-COV-2. Resolvins, protecting, and maresins of omega-3 fatty acids can inactivate polymorphonuclear leukocytes and stimulate the movement of non-inflammation leukocytes, thereby eliminating programmed cell death (32). Omega-3 supplementation was found to have promising effects on acidosis and renal function, possibly improving clinical outcomes in SARS-COV-2 patients (51). a systematic review and meta-analysis of randomized controlled trials on the effect of Omega-3 fatty acids supplementation in patients with SARS-COV-2 have reported that Omega-3 supplementations have been associated with alleviating the inflammatory response by the CRP level reduction (52).
A study found that omega-3 fatty acids from marine food were correlated with lower SARS-COV-2 mortality rates. Omega-3 fatty acids could reduce SARS-COV-2 medical complications by limiting the entry of the virus into human cells via a mechanism that involves fatty acids binding to viral spike proteins (53). Hathaway et al. proposed that omega-3 fatty acids supplementation might inhibit entry of the virus through changes to the lipid composition in a cell’s bilipid membrane. Moreover, the omega-3 fatty acids role is critical in mediating inflammatory processes and producing a modulation effect on innate and acquired immune responses (54) (Figure 2).
Caution has been warranted with the use of omega-3 fatty acids in SARS-COV-2 patients. This is due to evidence of a counter-intuitive increase in oxidative stress and inflammation because of a higher susceptibility to damage to cell membranes (55). However, the correlation between omega-3 fatty acids and recovery in SARS-COV-2 patients is still controversial.
Carbohydrates are mostly found in plants and used to support parts (fiber) of the plant, for plant growth, while the remaining part is for food storage (i.e., starch or sugar). Rich food sources of carbohydrates include bread, grains, vegetables, fruits, and legumes (56, 57). Carbohydrates intake should comprise 45 to 65% of total daily calories (58). Furthermore, the recommended daily fiber intake for males and females is 25 g and 38 g, respectively. A recent review study reported that the consumption of dietary fiber has anti-inflammatory effects by producing short-chain fatty acids, mucosa protection, and lower glycemic index (36). Diets with a high glycemic index produce inflammatory cytokines, TNF-α, IL-6, and C-reactive protein. Dietary fibers reduce inflammation, hs-CRP, IL-6, and TNF-α. Production of short-chain fatty acids occurs through the fermentation of carbohydrates in the gut and causes anti-inflammatory effects with up-regulated IL-10 production in monocytes (36, 57) (Figure 3). The effect of dietary fiber and prebiotic oligosaccharides is greater and typically promotes the growth of lactobacilli and bifidobacteria which benefits immunity (57). There is no evidence suggesting that carbohydrates influence SARS-COV-2 prevention and improvement (59).
The human body hosts many bacteria and organisms that colonize the skin, mouth, and gut. When there is a community of organisms within a particular location is referred to as the microbiota. The colon is the site of the most significant number and variety of bacterial species (60, 61). The usual diet strongly affects the intestinal microbiota. Probiotics including lactobacillus and bifidobacterium are live microorganisms with long-term effects as part of a typical diet through fermented foods. These foods include traditionally cultured dairy products, some fermented products (dairy and non-dairy), or supplements for their beneficial effects on gut microorganisms (62). Furthermore, both aging and health significantly affect the microbiota composition. An abnormal intestine microbiota, called dysbiosis, is commonly seen in obesity and adults with various human diseases (63). Probiotics have been investigated to prevent and treat various diseases (64). It has been suggested that probiotics are beneficial for human health through their effects on strengthening the immune system (40).
Beneficial effects from probiotics are likely the result of immune regulation, and controlling proinflammatory and anti-inflammatory cytokines (65). Studies have reported that microbiota improves resistance to viruses or pathogenic attacks of respiratory mucosa (66, 67). The results of the network and meta-analysis study showed that probiotics have therapeutic effects through many procedures such as: limiting virus entry via ACE2 receptor, mitigating the adverse effects of dysregulated RAS system, improving the systemic immune response, mediating Toll-like receptors (TLRs) innate immune response, improving cardiovascular complications, and reducing nitric oxide (NO) production, hypertension, and oxidative stress (68). Furthermore, another meta-analysis reported a positive correlation between probiotics intake and a reduction in COVID symptoms, especially cough, headaches, and diarrhea (69). Some specific mechanisms that explain the health benefits of probiotics include the activation of the immune reaction by interleukins and natural killer cells. Additionally, probiotics with macrophages lead to the formation of IL-12 which activates the generation of interferon-γ, considered a principal antiviral cytokine (70).
Probiotics enhance mucosal protection via increasing IgA production and the differentiation of CD8+ T cells into cytotoxic T-cells as well as CD4+ T cells into Th1 and Th2 cells (71). Different trials have demonstrated that probiotics exert positive effects on the gut and lungs through increases of regulatory T cells, improving anti-viral defense, and reducing pro-inflammatory cytokines during systemic and respiratory infections. Immunomodulatory benefits are crucial for SARS-CoV-2 patients or people at risk of contracting SARS-CoV-2 (72). This effect is likely the result of the gut-lung axis, which can form immune responses and disrupt respiratory diseases (73). The probiotics also affect the junction’s integrity and maintenance of the respiratory and gastrointestinal tract epithelium enterocytes, hence limiting the risk of SARS-CoV-2 entry (74). Furthermore, several probiotics especially lactic acid bacteria (LAB) produce peptides with an ACE inhibitory effect, which binds to the site of SARS-CoV-2.
A cross-sectional study on COVID-19 patients, influenza patients, and healthy controls (HC) examining gut microbiota reported that COVID-19 patients had lower gut microbiota diversity compared to HC, which was related to respiratory viral infectious diseases. Moreover, disease-specific shifts in microbiota composition were found between COVID-19 patients and HC (75). The studies on SARS-CoV-2 found significantly decreased gut bacterial diversity compared to HC, while it was increased in numerous bacterial species, such as Collinsella aerofaciens, Morganella morganii, and Streptococcus infantis (76, 77).
Several randomized controlled trials are underway investigating the effect of probiotic strains on SARS-CoV-2 severity, which may directly affect patients (78, 79). A study comparing probiotic-enriched formulas with placebo formula in adult symptomatic Covid19 outpatients reported that the probiotic-enriched formulas reduced symptom duration, viral load, and lung infiltrates while increasing SARS-CoV2-specific IgM and IgG in Covid19 outpatients (80). In a randomized trial, adults with symptomatic SARS-CoV-2 outpatients’ consumed probiotic formula (strains Lactiplantibacillus Plantarum KABP022, KABP023, and KAPB033, plus strain Pediococcus acidilactici KABP021, totaling 2 × 109 colony-forming units (CFU)) or placebo for 30 days. The results showed a reduction in symptom duration, viral load, and lung infiltrate while increasing SARS-CoV-2 specific IgM and IgG (81).
A randomized controlled clinical trial was conducted with eighty SARS-CoV-2 patients in stages III. The control group received a hospital diet and medical treatment. The intervention group received a hospital diet, medical treatment, and the NSS (vitamins, minerals, fiber, omega-3, amino acids, B-complex, and probiotics). In the intervention group, a significant increase in survival and a decrease in mortality were observed compared to the control group (82) (Figure 4).
The authors of this study assumed that this probiotic might primarily help the gut-lung axis (GLA) by interfering with the host’s immune system. However, with the lack of evidence that probiotics affect fecal microbiota, it seems that this hypothesis cannot be confirmed. More studies are needed to confirm this hypothesis.
The adequacy of globally considered macronutrients and microbiota is always fundamental for health in general and the ability of the immune system to respond adequately, not only to SARS-CoV-2, but to any other aggression by microorganisms. This article highlights the influential role of macronutrients and probiotics against SARS-CoV-2. Boosted immunity properties of macronutrients have opened a new window for their potential use for SARS-CoV-2 treatment and prognosis. Immunomodulatory agents, including macronutrients and probiotics, are among the current therapies applied in clinical settings for SARS-CoV-2. There is evidence that a diet high in protein and amino acids facilitates antibody production (83). Furthermore, a healthy balanced diet enriched in proteins, dietary fiber, and omega-3 fatty acids is highly recommended during the pandemic. Malnutrition, such as macronutrient deficiencies, is common in vulnerable groups, especially in elderly patients with multiple comorbidities and also in those groups of subjects who voluntarily submit to specific nutritional indications (for example vegan diets, ketogenic diets, vegetarian diets, food exclusion diets, etc…), or in patients affected by particular pathologies (anorexia, celiac disease, bulimia, etc…). As a result of the comorbidities and associated drugs, this may lead to tissues depleted of valuable nutrients, increase urinary excretion, or reduce nutrient absorption (37). These conditions conflict with an upcoming cytokine storm. Currently, no definitive recommendations for macronutrient and micronutrient supplementation in patients with SARS-CoV-2 exist. However, it is advised that SARS-CoV-2 patients be monitored to assess risk factors for nutrient depletion, i.e., inadequate intake and nutrient-depleting drugs. Education would also be imperative concerning depletion symptoms for laboratory monitoring of nutrients.
With numerous research groups currently involved in developing vaccines, and novel therapeutics against SARS-CoV-2, a healthy diet, balanced macronutrient status, and probiotics that reduce inflammation and oxidative stress may be an important strategy for managing SARS-CoV-2. This review highlights the role of macronutrients and probiotics in reducing inflammation, cytokine storm, and strengthening the immune system in SARS-CoV-2 patients (Supplementary Table S1).
MZ, SD, AH, and AK: writing original draft. FP, AM, MZ, and SD: writing—review. NA, and DH: review and editing. All authors contributed to the article and approved the submitted version.
The authors declare that the research was conducted in the absence of any commercial or financial relationships that could be construed as a potential conflict of interest.
All claims expressed in this article are solely those of the authors and do not necessarily represent those of their affiliated organizations, or those of the publisher, the editors and the reviewers. Any product that may be evaluated in this article, or claim that may be made by its manufacturer, is not guaranteed or endorsed by the publisher.
The Supplementary material for this article can be found online at: https://www.frontiersin.org/articles/10.3389/fnut.2023.1161894/full#supplementary-material
1. Ji, W, Wang, W, Zhao, X, Zai, J, and Li, X. Homologous recombination within the spike glycoprotein of the newly identified coronavirus 2019-nCoV may boost cross-species transmission from snake to human. J Med Virol. (2020) 92:433–40. doi: 10.1002/jmv.25682
2. Wu, D, Wu, T, Liu, Q, and Yang, Z. The SARS-CoV-2 outbreak: what we know. Int J Infect Dis. (2020) 94:44–8. doi: 10.1016/j.ijid.2020.03.004
3. COVID W. Coronavirus Pandemic; (2020). Available at: https://www.worldometers.info/coronavirus/. (Accessed December 12, 2020).
4. Bedford, J, Enria, D, Giesecke, J, Heymann, DL, Ihekweazu, C, Kobinger, G, et al. COVID-19: towards controlling of a pandemic. Lancet. (2020) 395:1015–8. doi: 10.1016/S0140-6736(20)30673-5
5. Xu, X-W, Wu, X-X, Jiang, X-G, Xu, K-J, Ying, L-J, Ma, C-L, et al. Clinical findings in a group of patients infected with the 2019 novel coronavirus (SARS-Cov-2) outside of Wuhan, China: retrospective case series. BMJ. (2020) 368:m606. doi: 10.1136/bmj.m606
6. Wang, D, Hu, B, Hu, C, Zhu, F, Liu, X, Zhang, J, et al. Clinical characteristics of 138 hospitalized patients with 2019 novel coronavirus–infected pneumonia in Wuhan, China. JAMA. (2020) 323:1061–9. doi: 10.1001/jama.2020.1585
7. Redd, WD, Zhou, JC, Hathorn, KE, McCarty, TR, Bazarbashi, AN, Thompson, CC, et al. Prevalence and characteristics of gastrointestinal symptoms in patients with severe acute respiratory syndrome coronavirus 2 infection in the United States: a multicenter cohort study. Gastroenterology. (2020) 159:765–767.e2. doi: 10.1053/j.gastro.2020.04.045
8. Gu, J, Han, B, and Wang, J. COVID-19: gastrointestinal manifestations and potential fecal–oral transmission. Gastroenterology. (2020) 158:1518–9. doi: 10.1053/j.gastro.2020.02.054
9. Zhang, H, Kang, Z, Gong, H, Xu, D, Wang, J, Li, Z, et al. Digestive system is a potential route of COVID-19: an analysis of single-cell coexpression pattern of key proteins in viral entry process. Gut. (2020) 69:1010–8. doi: 10.1136/gutjnl-2020-320953
10. Huang, C, Wang, Y, Li, X, Ren, L, Zhao, J, Hu, Y, et al. Clinical features of patients infected with 2019 novel coronavirus in Wuhan, China. Lancet. (2020) 395:497–506. doi: 10.1016/S0140-6736(20)30183-5
11. Ovsyannikova, IG, Haralambieva, IH, Crooke, SN, Poland, GA, and Kennedy, RB. The role of host genetics in the immune response to SARS-CoV-2 and COVID-19 susceptibility and severity. Immunol Rev. (2020) 296:205–19. doi: 10.1111/imr.12897
12. de Laval, B, Maurizio, J, Kandalla, PK, Brisou, G, Simonnet, L, Huber, C, et al. C/EBPβ-dependent epigenetic memory induces trained immunity in hematopoietic stem cells. Cell Stem Cell. (2020) 26:657–674.e8. doi: 10.1016/j.stem.2020.01.017
13. Huang, Z, Chen, B, Liu, X, Li, H, Xie, L, Gao, Y, et al. Effects of sex and aging on the immune cell landscape as assessed by single-cell transcriptomic analysis. Proc Natl Acad Sci. (2021) 118:e2023216118. doi: 10.1073/pnas.2023216118
14. Maggini, S, Pierre, A, and Calder, PC. Immune function and micronutrient requirements change over the life course. Nutrients. (2018) 10:1531. doi: 10.3390/nu10101531
15. Calder, PC. Nutrition, immunity and COVID-19. BMJ Nutr Prev Health. (2020) 3:74–92. doi: 10.1136/bmjnph-2020-000085
16. Shakoor, H, Feehan, J, Al Dhaheri, AS, Ali, HI, Platat, C, Ismail, LC, et al. Immune-boosting role of vitamins D, C, E, zinc, selenium and omega-3 fatty acids: could they help against COVID-19? Maturitas. (2021) 143:1–9. doi: 10.1016/j.maturitas.2020.08.003
17. Pedrosa, LF, Barros, AN, and Leite-Lais, L. Nutritional risk of vitamin D, vitamin C, zinc, and selenium deficiency on risk and clinical outcomes of COVID-19: A narrative review. Clin Nutr ESPEN. (2022) 47:9–27. doi: 10.1016/j.clnesp.2021.11.003
18. Ligthart-Melis, GC, Luiking, YC, Kakourou, A, Cederholm, T, Maier, AB, and Schueren, MA. Frailty, sarcopenia, and malnutrition frequently (co-) occur in hospitalized older adults: a systematic review and meta-analysis. J Am Med Dir Assoc. (2020) 21:1216–28. doi: 10.1016/j.jamda.2020.03.006
19. Moscatelli, F, Sessa, F, Valenzano, A, Polito, R, Monda, V, Cibelli, G, et al. COVID-19: role of nutrition and supplementation. Nutrients. (2021) 13:976. doi: 10.3390/nu13030976
20. Gombart, AF, Pierre, A, and Maggini, S. A review of micronutrients and the immune system–working in harmony to reduce the risk of infection. Nutrients. (2020) 12:236. doi: 10.3390/nu12010236
21. Synodinou, KD, Nikolaki, MD, Triantafyllou, K, and Kasti, AN. Immunomodulatory effects of probiotics on COVID-19 infection by targeting the gut–lung Axis microbial cross-talk. Microorganisms. (2022) 10:1764. doi: 10.3390/microorganisms10091764
22. Childs, CE, Calder, PC, and Miles, EA. Diet and immune function. MDPI. (2019) 11:1933. doi: 10.3390/nu11081933
23. Peters, EM, Schedlowski, M, Watzl, C, and Gimsa, U. To stress or not to stress: brain-behavior-immune interaction may weaken or promote the immune response to SARS-CoV-2. Neurobiol Stress. (2021) 14:100296. doi: 10.1016/j.ynstr.2021.100296
24. Zeng, Q, Dong, S-Y, Sun, X-N, Xie, J, and Cui, Y. Percent body fat is a better predictor of cardiovascular risk factors than body mass index. Braz J Med Biol Res. (2012) 45:591–600. doi: 10.1590/S0100-879X2012007500059
25. Líndez AA, MI, and Reith, W. Arginine-dependent immune responses. Cell Mol Life Sci. (2021) 78:5303–24. doi: 10.1007/s00018-021-03828-4
26. Brahmi, F, Vejux, A, Ghzaiel, I, Ksila, M, Zarrouk, A, Ghrairi, T, et al. Role of diet and nutrients in SARS-CoV-2 infection: incidence on oxidative stress, inflammatory status and viral production. Nutrients. (2022) 14:2194. doi: 10.3390/nu14112194
27. Hoffmann, M, Kleine-Weber, H, Schroeder, S, Krüger, N, Herrler, T, Erichsen, S, et al. SARS-CoV-2 cell entry depends on ACE2 and TMPRSS2 and is blocked by a clinically proven protease inhibitor. Cells. (2020) 181:271–280.e8. e8. doi: 10.1016/j.cell.2020.02.052
28. South, AM, Diz, DI, and Chappell, MC. COVID-19, ACE2, and the cardiovascular consequences. American journal of physiology-heart and circulatory. Physiology. (2020) 318:H1084–90. doi: 10.1152/ajpheart.00217.2020
29. Bourgonje, AR, Abdulle, AE, Timens, W, Hillebrands, JL, Navis, GJ, Gordijn, SJ, et al. Angiotensin-converting enzyme 2 (ACE2), SARS-CoV-2 and the pathophysiology of coronavirus disease 2019 (COVID-19). J Pathol. (2020) 251:228–48. doi: 10.1002/path.5471
30. Valenzuela, R, Rodriguez-Perez, AI, Costa-Besada, MA, Rivas-Santisteban, R, Garrido-Gil, P, Lopez-Lopez, A, et al. An ACE2/mas-related receptor Mrg E axis in dopaminergic neuron mitochondria. Redox Biol. (2021) 46:102078. doi: 10.1016/j.redox.2021.102078
31. Goudarzi, M, Garavand, F, Madadlou, A, and Fogliano, V. Food protein-derived antihypertensive peptides in the COVID-19 pandemic: friends of foes? J Hypertens. (2020) 38:1614–6. doi: 10.1097/HJH.0000000000002534
32. Motti, ML, Tafuri, D, Donini, L, Masucci, MT, De Falco, V, and Mazzeo, F. The role of nutrients in prevention, treatment and post-coronavirus Disease-2019 (COVID-19). Nutrients. (2022) 14:1000. doi: 10.3390/nu14051000
33. Wu, G. Important roles of dietary taurine, creatine, carnosine, anserine and 4-hydroxyproline in human nutrition and health. Amino Acids. (2020) 52:329–60. doi: 10.1007/s00726-020-02823-6
34. Hajimohammadebrahim-Ketabforoush, M, Vahdat-Shariatpanahi, Z, Vahdat-Shariatpanahi, M, Shahbazi, E, and Shahbazi, S. Protein and energy intake assessment and their association with in-hospital mortality in critically ill COVID-19 patients: a prospective cohort study. Frontiers. Nutrition. (2021) 8:708271. doi: 10.3389/fnut.2021.708271
35. Rosa, L, Tripepi, G, Naldi, E, Aimati, M, Santangeli, S, Venditto, F, et al. Ambulatory COVID-19 patients treated with lactoferrin as a supplementary antiviral agent: a preliminary study. J Clin Med. (2021) 10:4276. doi: 10.3390/jcm10184276
36. Iddir, M, Brito, A, Dingeo, G, Fernandez Del Campo, SS, Samouda, H, La Frano, MR, et al. Strengthening the immune system and reducing inflammation and oxidative stress through diet and nutrition: considerations during the COVID-19 crisis. Nutrients. (2020) 12:1562. doi: 10.3390/nu12061562
37. Jayawardena, R, and Misra, A. Balanced diet is a major casualty in COVID-19. Diabetes Metab Syndr. (2020) 14:1085–6. doi: 10.1016/j.dsx.2020.07.001
38. Al-khatib, A. Antiviral functional foods and exercise lifestyle prevention of coronavirus. Nutrients. (2020) 12:2633. doi: 10.3390/nu12092633
39. Kikut, J, Konecka, N, Ziętek, M, Kulpa, D, and Szczuko, M. Diet supporting therapy for inflammatory bowel diseases. Eur J Nutr. (2021) 60:2275–91. doi: 10.1007/s00394-021-02489-0
40. Calcuttawala, F. Nutrition as a key to boost immunity against COVID-19. Clin Nutr ESPEN. (2022) 49:17–23. doi: 10.1016/j.clnesp.2022.04.007
41. de Alencar, ES, dos Santos Muniz, LS, Holanda, JLG, Oliveira, BDD, de Carvalho, MCF, Leitão, AMM, et al. Enteral nutritional support for patients hospitalized with COVID-19: results from the first wave in a public hospital. Nutrition. (2022) 94:111512. doi: 10.1016/j.nut.2021.111512
42. Tazakkori, Z, Zare, M, Nemadi Vosoughi, M, and Tarighat-Esfanjani, A. Nutrition and die Therapy in Nursing. Tehran: Sociologist Publishing (2020).
43. Takic, M, Pokimica, B, Petrovic-Oggiano, G, and Popovic, T. Effects of dietary α-linolenic acid treatment and the efficiency of its conversion to Eicosapentaenoic and docosahexaenoic acids in obesity and related diseases. Molecules. (2022) 27:4471. doi: 10.3390/molecules27144471
44. Radzikowska, U, Rinaldi, AO, Çelebi Sözener, Z, Karaguzel, D, Wojcik, M, Cypryk, K, et al. The influence of dietary fatty acids on immune responses. Nutrients. (2019) 11:2990. doi: 10.3390/nu11122990
45. Torrinhas, RS, Calder, PC, Lemos, GO, and Waitzberg, DL. Parenteral fish oil: an adjuvant pharmacotherapy for coronavirus disease 2019? Nutrition. (2021) 81:110900. doi: 10.1016/j.nut.2020.110900
46. Miao, L, Remø, S, Espe, M, Philip, A, Hamre, K, Fjelldal, P, et al. Dietary plant oil supplemented with arachidonic acid and eicosapentaenoic acid affects the fatty acid composition and eicosanoid metabolism of Atlantic salmon (Salmo salar L.) during smoltification. Fish Shellfish Immunol. (2022) 123:194–206. doi: 10.1016/j.fsi.2022.02.049
47. Doaei, S, Gholami, S, Rastgoo, S, Gholamalizadeh, M, Bourbour, F, Bagheri, SE, et al. The effect of omega-3 fatty acid supplementation on clinical and biochemical parameters of critically ill patients with COVID-19: a randomized clinical trial. J Transl Med. (2021) 19:1–9. doi: 10.1186/s12967-021-02795-5
48. Vivar-Sierra, A, Araiza-Macías, MJ, Hernández-Contreras, JP, Vergara-Castañeda, A, Ramírez-Vélez, G, Pinto-Almazán, R, et al. In silico study of polyunsaturated fatty acids as potential SARS-CoV-2 spike protein closed conformation stabilizers: epidemiological and computational approaches. Molecules. (2021) 26:711. doi: 10.3390/molecules26030711
49. Barazzoni, R, Bischoff, SC, Breda, J, Wickramasinghe, K, Krznaric, Z, Nitzan, D, et al. ESPEN expert statements and practical guidance for nutritional management of individuals with SARS-CoV-2 infection. Clin Nutr. (2020) 39:1631–8. doi: 10.1016/j.clnu.2020.03.022
50. Fattori, V, Zaninelli, TH, Rasquel-Oliveira, FS, Casagrande, R, and Verri, WA Jr. Specialized pro-resolving lipid mediators: A new class of non-immunosuppressive and non-opioid analgesic drugs. Pharmacol Res. (2020) 151:104549. doi: 10.1016/j.phrs.2019.104549
51. Sedighiyan, M, Abdollahi, H, Karimi, E, Badeli, M, Erfanian, R, Raeesi, S, et al. Omega-3 polyunsaturated fatty acids supplementation improve clinical symptoms in patients with Covid-19: A randomised clinical trial. Int J Clin Pract. (2021) 75:e14854. doi: 10.1111/ijcp.14854
52. Taha, AM, Shaarawy, AS, Omar, MM, Abouelmagd, K, Shalma, NM, Alhashemi, M, et al. Effect of Omega-3 fatty acids supplementation on serum level of C-reactive protein in patients with COVID-19: a systematic review and meta-analysis of randomized controlled trials. J Transl Med. (2022) 20:1–9. doi: 10.1186/s12967-022-03604-3
53. Hathaway, D III, Pandav, K, Patel, M, Riva-Moscoso, A, Singh, BM, Patel, A, et al. Omega 3 fatty acids and COVID-19: a comprehensive review. Infect Chemother. (2020) 52:478–95. doi: 10.3947/ic.2020.52.4.478
54. Rogero, MM, Leao, MC, Santana, TM, Pimentel, MV, Carlini, GC, da Silveira, TF, et al. Potential benefits and risks of omega-3 fatty acids supplementation to patients with COVID-19. Free Radic Biol Med. (2020) 156:190–9. doi: 10.1016/j.freeradbiomed.2020.07.005
55. Whitney, EN, and Rolfes, SR. Understanding Nutrition. United States of America: Cengage Learning Inc. (2015) 128–168.
56. Yahia, EM, García-Solís, P, and Celis, MEM. “Contribution of fruits and vegetables to human nutrition and health,” In:. Postharvest physiology and biochemistry of fruits and vegetables. eds. EM Yahia and A Carrillo-Lopez. India: Woodhead Publishing; Elsevier Inc. (2019). 19–45.
57. Slavin, J. Fiber and prebiotics: mechanisms and health benefits. Nutrients. (2013) 5:1417–35. doi: 10.3390/nu5041417
58. Raithel, M, Weidenhiller, M, Hagel, AF-K, Hetterich, U, Neurath, MF, and Konturek, PC. The malabsorption of commonly occurring mono and disaccharides: levels of investigation and differential diagnoses. Dtsch Ärztebl Int. (2013) 110:775. doi: 10.3238/arztebl.2013.0775
59. Cobre, AF, Surek, M, Vilhena, RO, Böger, B, Fachi, MM, Momade, DR, et al. Influence of foods and nutrients on COVID-19 recovery: A multivariate analysis of data from 170 countries using a generalized linear model. Clin Nutr. (2022) 41:3077–84. doi: 10.1016/j.clnu.2021.03.018
60. Sender, R, Fuchs, S, and Milo, R. Revised estimates for the number of human and bacteria cells in the body. PLoS Biol. (2016) 14:e1002533. doi: 10.1371/journal.pbio.1002533
61. Khaled, JM. Probiotics, prebiotics, and COVID-19 infection: A review article. Saudi J Biol Sci. (2021) 28:865–9. doi: 10.1016/j.sjbs.2020.11.025
62. Zmora, N, Suez, J, and Elinav, E. You are what you eat: diet, health and the gut microbiota. Nat Rev Gastroenterol Hepatol. (2019) 16:35–56. doi: 10.1038/s41575-018-0061-2
63. Valdes, AM, Walter, J, Segal, E, and Spector, TD. Role of the gut microbiota in nutrition and health. BMJ. (2018) 361:k2179. doi: 10.1136/bmj.k2179
64. Tazakori, Z, Zare, M, and Jafarabadi, MA. Probiotic yogurt effect on macronutrients ingredients, blood glucose and lipid profile in type 2 diabetes. J Pak Med Assoc. (2017) 67:1123.
65. Bottari, B, Castellone, V, and Neviani, E. Probiotics and COVID-19. Int J Food Sci Nutr. (2021) 72:293–9. doi: 10.1080/09637486.2020.1807475
66. Zelaya, H, Alvarez, S, Kitazawa, H, and Villena, J. Respiratory antiviral immunity and immunobiotics: beneficial effects on inflammation-coagulation interaction during influenza virus infection. Front Immunol. (2016) 7:633. doi: 10.3389/fimmu.2016.00633
67. Racedo, S, Villena, J, Salva, S, and Alvarez, S. Influence of yogurt consumption on the respiratory immune response. Food Agric Immunol. (2009) 20:231–44. doi: 10.1080/09540100903061659
68. Patra, S, Saxena, S, Sahu, N, Pradhan, B, and Roychowdhury, A. Systematic network and meta-analysis on the antiviral mechanisms of probiotics: a preventive and treatment strategy to mitigate SARS-CoV-2 infection. Probiotics Antimicrob Proteins. (2021) 13:1138–56. doi: 10.1007/s12602-021-09748-w
69. Neris Almeida Viana, S, do Reis Santos Pereira, T, de Carvalho Alves, J, de Tianeze Castro, C, Santana, C, da Silva, L, et al. Benefits of probiotic use on COVID-19: a systematic review and meta-analysis. Crit Rev Food Sci Nutr. (2022) 62:1–13. doi: 10.1080/10408398.2022.2128713
70. Gouda, AS, Adbelruhman, FG, Alenezi, HS, and Mégarbane, B. Theoretical benefits of yogurt-derived bioactive peptides and probiotics in COVID-19 patients–A narrative review and hypotheses. Saudi J Biol Sci. (2021) 28:5897–905. doi: 10.1016/j.sjbs.2021.06.046
71. Mirzaei, R, Attar, A, Papizadeh, S, Jeda, AS, Hosseini-Fard, SR, Jamasbi, E, et al. The emerging role of probiotics as a mitigation strategy against coronavirus disease 2019 (COVID-19). Arch Virol. (2021) 166:1819–40. doi: 10.1007/s00705-021-05036-8
73. Willers, M, and Viemann, D. Role of the gut microbiota in airway immunity and host defense against respiratory infections. Biol Chem. (2021) 402:1481–91. doi: 10.1515/hsz-2021-0281
74. Baud, D, Dimopoulou Agri, V, Gibson, GR, Reid, G, and Giannoni, E. Using probiotics to flatten the curve of coronavirus disease COVID-2019 pandemic. Front Public Health. (2020) 8:186. doi: 10.3389/fpubh.2020.00186
75. Xu, K, Cai, H, Shen, Y, Ni, Q, Chen, Y, Hu, S, et al. Management of COVID-19: the Zhejiang experience. Zhejiang Da Xue Xue Bao Yi Xue Ban. (2020) 49:147–57. doi: 10.3785/j.issn.1008-9292.2020.02.02
76. Gu, S, Chen, Y, Wu, Z, Chen, Y, Gao, H, Lv, L, et al. Alterations of the gut microbiota in patients with coronavirus disease 2019 or H1N1 influenza. Clin Infect Dis. (2020) 71:2669–78. doi: 10.1093/cid/ciaa709
77. Zuo, T, Liu, Q, Zhang, F, Lui, GC-Y, Tso, EY, Yeoh, YK, et al. Depicting SARS-CoV-2 faecal viral activity in association with gut microbiota composition in patients with COVID-19. Gut. (2021) 70:276–84. doi: 10.1136/gutjnl-2020-322294
78. Tang, H, Bohannon, L, Lew, M, Jensen, D, Jung, S-H, Zhao, A, et al. Randomised, double-blind, placebo-controlled trial of probiotics to eliminate COVID-19 transmission in exposed household contacts (PROTECT-EHC): a clinical trial protocol. BMJ Open. (2021) 11:e047069. doi: 10.1136/bmjopen-2020-047069
79. Rodriguez-Blanque, R, Sánchez-García, JC, Cobos-Vargas, Á, Aguilar Quesada, A, Maldonado-Lobón, JA, Olivares, M, et al. Evaluation of the effect of Loigolactobacillus coryniformis K8 CECT 5711 consumption in health care workers exposed to COVID-19. Front Nutr. (2020) 9:962566. doi: 10.3389/fnut.2022.962566
80. Chourasia, R, Padhi, S, Chiring Phukon, L, Abedin, MM, Singh, SP, and Rai, AK. A potential peptide from soy cheese produced using Lactobacillus delbrueckii WS4 for effective inhibition of SARS-CoV-2 main protease and S1 glycoprotein. Front Mol Biosci. (2020) 7:601753. doi: 10.3389/fmolb.2020.601753
81. Gutiérrez-Castrellón, P, Gandara-Martí, T, Abreu, Y, Abreu, AT, Nieto-Rufino, CD, López-Orduña, E, et al. Probiotic improves symptomatic and viral clearance in Covid 19 outpatients: a randomized, quadruple-blinded, placebo-controlled trial. Gut Microbes. (2022) 14:2018899. doi: 10.1080/19490976.2021.2018899
82. Leal-Martínez, F, Abarca-Bernal, L, García-Pérez, A, González-Tolosa, D, Cruz-Cázares, G, Montell-García, M, et al. Effect of a nutritional support system to increase survival and reduce mortality in patients with COVID-19 in stage III and comorbidities: a blinded randomized controlled clinical trial. Int J Environ Res Public Health. (2022) 19:1172. doi: 10.3390/ijerph19031172
Keywords: protein, lipid, carbohydrate, probiotic, immune system, SARS-CoV-2
Citation: Mardi A, Kamran A, Pourfarzi F, Zare M, Hajipour A, Doaei S, Abediasl N and Hackett D (2023) Potential of macronutrients and probiotics to boost immunity in patients with SARS-COV-2: a narrative review. Front. Nutr. 10:1161894. doi: 10.3389/fnut.2023.1161894
Received: 08 February 2023; Accepted: 26 April 2023;
Published: 15 May 2023.
Edited by:
Isabelle Mack, University of Tübingen, GermanyReviewed by:
Mario Delfino, University of Naples Federico II, ItalyCopyright © 2023 Mardi, Kamran, Pourfarzi, Zare, Hajipour, Doaei, Abediasl and Hackett. This is an open-access article distributed under the terms of the Creative Commons Attribution License (CC BY). The use, distribution or reproduction in other forums is permitted, provided the original author(s) and the copyright owner(s) are credited and that the original publication in this journal is cited, in accordance with accepted academic practice. No use, distribution or reproduction is permitted which does not comply with these terms.
*Correspondence: Maryam Zare, TS5aYXJlQEtoYWx1bXMuYWMuaXI=; Saeid Doaei, c2RvYWVlQHlhaG9vLmNvbQ==
†ORCID: Aziz Kamran https://orcid.org/0000-0001-6065-4397
Farhad Pourfarzi https://orcid.org/0000-0001-6442-6340
Maryam Zare https://orcid.org/0000-0001-6627-8564
Negin Abediasl https://orcid.org/0000-0002-2461-0829
Disclaimer: All claims expressed in this article are solely those of the authors and do not necessarily represent those of their affiliated organizations, or those of the publisher, the editors and the reviewers. Any product that may be evaluated in this article or claim that may be made by its manufacturer is not guaranteed or endorsed by the publisher.
Research integrity at Frontiers
Learn more about the work of our research integrity team to safeguard the quality of each article we publish.