- 1China-Malaysia National Joint Laboratory, Biomedical Research Center, Northwest Minzu University, Lanzhou, China
- 2College of Life Sciences and Engineering, Northwest Minzu University, Lanzhou, China
- 3Sino-German Joint Research Institute, Nanchang University, Nanchang, China
- 4Taizishan Ecosystem Observatory of Carbon Neutralization, Northwest Minzu University, Lanzhou, China
Introduction: Arctium lappa L. root has high nutritional and medicinal values and has been identified as a healthy food raw material by the Ministry of Health of the People's Republic of China.
Methods: In the present study, an aqueous two-phase system (ATPS) of polyethylene glycol (PEG)-(NH4)2SO4 was used to extract Arctium lappa L. polysaccharides (ALPs) from the Arctium lappa L. roots, the optimal extraction conditions of crude ALPs were optimized by using the single-factor experiment and response surface methodology. The structure and composition of ALPs were determined by fourier transform infrared spectroscopy (FTIR), scanning electron microscopy (SEM), and high-performance liquid chromatography (HPLC). At the same time, the antioxidant activity of ALPs was investigated by in vitro antioxidant experiment.
Results: The optimized extraction parameters for extraction ALPs were as follows: the PEG relative molecular weight of 6,000, a quality fraction of PEG 25%, a quality fraction of (NH4)2SO4 18%, and an extraction temperature of 80°C. Under these conditions, the extraction rate of ALPs could reach 28.83%. FTIR, SEM and HPLC results showed that ALPs were typical acidic heteropolysaccharides and had uneven particle size distribution, an irregular shape, and a rough surface. The ALPs were chiefly composed of glucose, rhamnose, arabinose, and galactose with a molar ratio of 70.19:10.95:11.16:6.90. In addition, the ALPs had intense antioxidant activity in vitro with IC50 values in the ·OH radical (1.732 mg/ml), DPPH radical (0.29 mg/ml), and superoxide anion (0.15 mg/ml) scavenging abilities.
Discussion: The results showed that ATPS was an efficient method to extract polysaccharides and could be used for the extraction of other polysaccharides. These results indicated that ALPs had great prospects as a functional food and could be exploited in multiple fields.
Introduction
Arctium lappa L., also called “black radish” or “ginseng,”—is a straight-root biennial herb belonging to Asteraceae (Compositae) family (1, 2)—that was cultivated in Europe approximately 3,000 years earlier (3). Warm and humid climates are suitable for Arctium lappa L. It mainly grows in Northeast, North, and Northwest China (4). Arctium lappa L. root is a very popular vegetable in China, which is rich in the soluble dietary fiber found in inulin, polysaccharides, polyphenols, flavonoids, arctiin, and other active ingredients (5, 6), used as a kind of nutritive and functional food in traditional Chinese medicine (TCM), has very high nutritional and medicinal value, such as antioxidant, hypoglycemic, and lipid-lowering effects; immunity enhancement; bacteriostasis; and anti-inflammatory and other effects (7–9).
Polysaccharide is a kind of polymeric polymer compound with more than 10 monosaccharides linked by glycosidic bonds (10, 11). Studies have shown that polysaccharides have a variety of biological activities, including improving immunity, inhibiting the proliferation and diffusion of tumor cells, and regulating blood sugar balance (12–14). As a natural metabolic regulatory factor, polysaccharides have broad development prospects. With the continuous development of the social economy, the enormous potential value of ALPs is gradually recognized by people, and its functional food is increasingly favored by consumers (15). Currently, research on ALPs focuses on processing, physiological functions, and their applications in food. However, most of the ALP extraction methods are traditional and inefficient. Furthermore, the traditional extraction methods of ALPs are time-consuming and inefficient. There have been few studies on the antioxidant activity of ALPs (16, 17). Meanwhile, the biological activity of polysaccharides is related to the composition and structure of polysaccharides; therefore, it is essential to analyze the monosaccharide composition and structure of ALPs (18, 19).
Currently, the hot water extraction method, enzyme-assisted extraction, the lye extraction method, the ultrasonic-assisted method, and the microwave-assisted method are frequently used to extract plant polysaccharides (16, 20–22). Aqueous two-phase system (ATPS) is a new extraction technology developed in recent years, which has the advantages of simple operation, mild conditions, and environmental protection (23). When the nature of the extraction system is different, the substance enters the double aqueous phase system. Due to the surface properties, the charge effect, various forces (water-repellent bonding, hydrogen bonding, ionic bonding, etc.), and the influence of environmental factors, their concentration in the upper and lower phases are different. To improve the extraction rate and efficiency of ALPs, PEG-(NH4)2SO4, the ATPS method was used to extract ALPs from Arctium lappa L. root in the present study, and FTIR, SEM, and HPLC were used to determine the structure and composition of ALPs. In addition, the antioxidant abilities of ALPs were investigated to lay the foundation and provide the basis for further functional food development and application.
Materials and methods
Materials and reagents
The Arctium lappa L. root was purchased from the local market of Lanzhou, Gansu Province, China. Dry Arctium lappa L. root was crushed by a BJ-400 high disintegrator (Yongkang Boou Instrument Co., Ltd., Zhejiang, China) and treated with a sieving of 80 meshes. The screen underflow was degreased with normal hexane (5:1, v/m) for 12 h. The degreased powder was dried using a DHG-9030A oven (Shanghai Grows Instrument Co., Ltd., China).
Monosaccharide standard products, acetonitrile, 1,1-diphenyl-2-trinitrophenylhydrazine (DPPH), and trifluoroacetic acid (TFA) were purchased from Sigma–Aldrich Chemical Co., Ltd (St. Louis, USA). Sulfuric acid and ammonium sulfate were purchased from Sinopharm Chemical Reagent Co., Ltd (Beijing, China), and all other reagents used in this study were of analytical grade.
Extraction of ALPs
Preparation of crude ALPs
An amount of 5 g degreased Arctium lappa L. powder was added into 150 ml distilled water with a ratio of material to liquid at 1:30; then the mixture was incubated at 50°C for 2.0 h, centrifuged (5,000 rpm, 20 min) to obtain the supernatant by Heraeus Multifuge X1R (Thermo Co., America). Sevag reagent (n-butanol: chloroform, 1:5, v/v) was added into the supernatant to remove proteins and the solution was centrifuged (5,000 rpm, 20 min) to obtain the crude ALPs.
Preparation of PEG-(NH4)2SO4 with ATPS
The ATPS studied in this article constituted PEG solution, (NH4)2SO4 solution, crude ALP solution, and deionized water, and the total quality of the ATPS was set as 20 g. A certain amount of PEG, ALP extract, and ammonium sulfate was added to the test tube, mixed and dissolved, and filled up to 20 ml with distilled water. The mixture was shaken for 2 min and extracted by a water bath at a certain temperature for 30 min. The solution was centrifuged at 4,000 rpm for 5 min to form two stable phases. The volume and absorbance of the upper and lower phases were measured, respectively. For details, refer to the study by Zhang et al. (24).
Determination of the extraction rate of ALPs
The content of ALPs was determined by the phenol–sulfuric acid method (25). A volume of 0.1 g/ml glucose standard solution at different concentrations (0.2, 0.4, 0.6, 0.8, and 1.0 ml) was removed with 1.0 ml of 6% phenol and added into different test tubes. Then, 5.0 ml of concentrated sulfuric acid was immediately added, shaken well, and allowed to stand for 30 min. The absorbance of samples was measured at 490 nm by an ultraviolet-visible spectrophotometer (Shanghai Spectrum Instrument Co., Ltd., Shanghai, China). A volume of 2.0 ml of distilled water was used as blank control. Using the different concentrations of the D-glucose (x) as the abscissa and the absorbance value (y) as the ordinate, a standard curve was drawn, and the linear regression equation (y = 103.161x – 2.3722, R2 = 0.9996) was obtained for calculating the sample concentration according to the absorbance value of the sample.
The ALP contents were calculated from the glucose standard curve and the ALP extraction rate was calculated according to the following equations:
where y is the extraction rate of ALPs of the lower phase (%), K is the distribution coefficient of polysaccharide in the ATPS, R is the volume ratio of the upper and lower phases, Vt is the upper phase volume (ml), Vb is the lower phase volume (ml), Ct is the mass concentration of polysaccharide in the upper phase (mg/ml), and Cb is the mass concentration of polysaccharide in the lower phase (mg/ml).
Experimental design of optimization extraction conditions
In the single-factor experiments, four variables, including the PEG molecular weights (1,000, 2,000, 4,000, 6,000, and 8,000), the quality fractions of PEG (10, 15, 20, 25, and 30%), the quality fractions of (NH4)2SO4 (10, 14, 18, 22, and 26%), and the extraction temperatures (50, 60, 70, 80, and 90°C) were taken into consideration to investigate their effects on the extraction rate of ATPS.
According to the results of single-factor tests, the optimal extraction parameters of ATPs were performed by response surface methodology (RSM) with a Box–Behnken design (BBD) to obtain the maximum extraction rate of ATPs. The quality fraction of PEG (X1, %), the quality fraction of (NH4)2SO4 (X2, %), and extraction temperature (X3, °C) were used as individual variables, and the ALPs extraction rate (Y) was used as the response value. A total of 17 experiments were conducted on a 3-factor, 3-level BBD with 5 replicates of central and 12 of factorial points (−1, 0, and 1). Test factors and horizontal design are shown in Table 1. The experimental data were fitted to a second-order polynomial regression model using the following equation:
where Y is the response variable (ALPs extraction rate, %); B0, Bi, Bii, and Bij are the regression coefficients of variables for the intercept, the linear term, the quadratic term, and the interaction term, respectively; and Xi and Xj are the independent variables (i ≠ j).
FT-IR analysis of ALPs
We mixed and ground 1 mg ALPs with 500 mg KBr, and the mixed samples were pressed for 5 min using an HYP-15 machine (Tianjin Port East Technology Co., Ltd, China). The chemical bonds and functional groups of polysaccharides were analyzed by scanning in the mid-infrared frequency range of 4,000–400 cm−1 using FTIR spectrometer (FTIR-650, Tianjin Guangdong Science and Technology Co., Ltd, China) (26).
SEM analysis of ALPs
The ALPs were fixed on the specimen stage with an electrically conducting adhesive, and the samples were coated with gold sputtering. Then, the surface morphology of the samples was observed using a ZEISS EVO18 scanning electron microscope (Carl Zeiss AG, Bruker Co., Germany) at an accelerating voltage of 10 kV with different magnifications (27).
Monosaccharide composition analysis of ALPs
The monosaccharide components of the ALPs were measured by the method of Chen et al. (28) with slight modifications. Briefly, 10.00 mg of the crude ALP sample was precisely weighed and put into a 10-ml test tube, and 5 ml of the TFA solution (2 mol/L) was added. Then, the sample was hydrolyzed in a water bath (100°C, 5 h). After cooling, the pH value of the mixture solution was adjusted to 7.0 by adding 3 mol/L NaOH and centrifuged (5,000 rpm, 10 min) to obtain the supernatant. Volumes of 0.2 ml PMP methanol solution (0.5 mol/L) and 0.2 ml NaOH (0.3 mol/L) were added into the ALP supernatant after hydrolysis and mixed well. It was incubated in a hot water bath at 70°C for 1 h and then 0.2 ml of 0.3 mol/L HCl solution and 1 ml chloroform were added to the supernatant, and the upper aqueous phase was filtered through a membrane of 0.22 μm to produce the solution to be tested. Rhamnose, glucose, galactose, fructose, and arabinose standards were treated as same as the sample.
A 1260 Infinity HPLC System (Agilent, USA) coupled with a diode array detector (DAD) was used to detect the monosaccharide components of ALPs, HPLC conditions were set as follows: a mobile phase of 0.02 mol/L phosphate buffer (pH 6.8) and acetonitrile in a ratio of 81:19 (v/v), an Agilent ZORBAX Eclipse XDB-C18 column (4.6 mm × 250 mm, 5 μm, Agilent Co., USA), a column temperature of 28°C, a flow rate of 1 ml/min, sample volume of 5 μl, and a detection wavelength of 250 nm.
In vitro antioxidant activity assay
·OH radical scavenging activity
The OH scavenging activity of the ALPs was determined according to the Fenton-type reaction studied by Wang et al. (29). We added 1 ml of ALPs solution at different concentrations (0.2, 0.4, 0.6, 0.8, and 1.0 mg/ml), 1 ml FeSO4 solution (9 mmol/L), 1 ml salicylic acid solution (9 mmol/L), and 1 ml H2O2 solution (8.8 mmol/L) into a 10-ml test tube and reacted in a water bath at 37°C for 30 min. The absorbance values were measured at the wavelength of 510 nm. VC solution with different concentrations (0.2, 0.4, 0.6, 0.8, and 1.0 mg/ml) was used as a positive control. All experiments were carried out in three parallel samples, and the average value was used to calculate the OH scavenging activity of ALPs. The scavenging rate was calculated according to the following equation:
where E is the scavenging activity of the ·OH radical (%); A0 is the absorbance value of blank control; A1 is the absorbance value of the samples; and A2 is the absorbance value of the sample without the ·OH radical.
DPPH radical scavenging activity
The DPPH radical scavenging activity of ALPs was measured by the method of Liu et al. (30). A volume of 2 ml DPPH solution (0.2 mmol/L) was mixed with 2 ml ALPs samples at different concentrations (0.2, 0.4, 0.6, 0.8, and 1.0 mg/ml) and placed in the unlit environment at room temperature for 30 min. We used 2.0 ml of distilled water instead of ALPs as control 1, 2.0 ml of sample solution and 2.0 ml of distilled water as control 2, and 2.0 ml DPPH solution and 2.0 ml VC solutions of 0.2, 0.4, 0.6, 0.8, and 1.0 mg/ml were used as positive control. The absorbance values of each experimental group were measured at 517 nm. The absorbance values were substituted into the following equation to calculate the scavenging percentage of DPPH radical:
where E is DPPH radical scavenging activity (%); Aa is the absorbance values of the samples; and Ab and Ac are the absorbance values of control 1 and control 2, respectively.
· radical scavenging activity
The method of Liu et al. was used to measure the scavenging activity of the ALPs (31). We mixed 1 ml ALPs solution with different concentrations (0.2, 0.4, 0.6, 0.8, and 1.0 mg/ml) and 4.5 ml Tris-HCl solution (1 mol/L, pH 8.2) and reacted at 50°C for 30 min. Then, 1 ml of pyrogallic acid (2 mmol/L) was added and placed in a 20°C water bath for 10 min. Finally, two drops of HCl solution (8 mol/L) were immediately added to terminate the reaction; 0.3 ml distilled water was used to substitute pyrogallol instead of pyrogallic acid; and the VC solution at different concentrations (0.2, 0.4, 0.6, 0.8, and 1.0 mg/ml) was used as positive control instead of the ALP solutions. The absorbance was measured at 320 nm and the scavenging activity was calculated by substituting into the following equation:
where E is the scavenging activity of the · radical (%) and Ai and Aii were the absorbance values of the control and samples, respectively.
Statistical analysis
All experimental data were analyzed by the ORIGIN 2018 Graphing and Analysis and SPSS 25.0 software (IBM, SPSS, Chicago, USA), and the data results were expressed as mean value ± standard deviation (SD). Response surface experimental data were statistically analyzed using the Stat-Ease 360 version 8.0.6 software (Stat-Ease, Inc., Minneapolis, USA). Significance among different groups was analyzed by a one-way analysis of variance (ANOVA), followed by Duncan's test, and all experiments or analyses were performed in triplicate.
Results and discussion
Effects of PEG molecular weight, PEG quality fraction, (NH4)2SO4 quality fraction, and extraction temperature on the extraction rate of ALPs
Due to its good solubility in water and many organic solvents and its non-toxicity, PEG is considered a kind of non-ionic polymer. PEG has been widely used to extract polysaccharides from a variety of plants (32). As shown in Figure 1A, when the PEG molecular weight was between 2,000 and 6,000, the extraction rate of ALPs increased significantly. When the PEG molecular weight was 6,000, the extraction rate of ALPs reached a maximum of 23.47 ± 0.08%. However, when the PEG molecular weight exceeded 6,000, the extraction rate of ALPs decreased gradually. The molecular weight and hydrophobicity of PEG affected the extraction capacity of the two-phase aqueous extraction system (33). As the molecular weight of PEG increased, the molecular chain became longer, the relative fraction of hydroxyl decreased, and the higher molecular weight grades were less hydrophilic and had stronger hydrophobicity (34, 35). Therefore, to accumulate the extracted substance as much as possible in the upper phase, PEG of lower molecular weight was selected and vice versa. Therefore, the optimum PEG molecular weight was 6,000. At the same time, PEG-6000 was also selected as the extraction solvent for the extraction of aloe polysaccharide by Xing et al. (36).
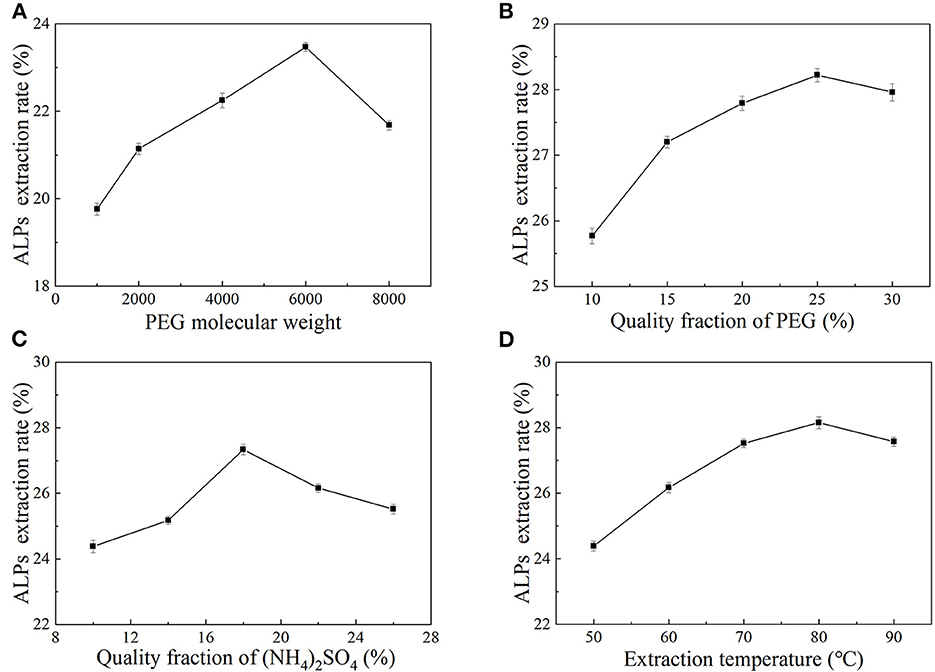
Figure 1. The effects of PEG molecular weight (A), PEG quality fraction (B), (NH4)2SO4 quality fraction (C), and extraction temperature (D) on the extraction rate of ALPs.
Figure 1B shows the influence of PEG quality fraction on the extraction rate of ALPs. In the range of 10%−30%, the extraction rate of ALPs increased first and then decreased with the increase of PEG quality fraction, and the ALP extraction rate reached the maximum value (28.22 ± 0.09%) when a quality fraction of PEG was 25%. The main reason for the increase in the extraction rate of ALPs may be that, when the quality fraction of PEG increased, water molecules in the system may have gathered in the lower phase, and the volume of the lower phase increased, dissolving more ALPs (37). The high PEG quality fraction leads to a high viscosity solution, which hinders the transfer of polysaccharide molecules and causes the ALPs in the upper phase to remain between the two phases (38). Considering all aspects, the quality fraction of PEG of 25% was selected as the optimal PEG quality fraction, and it was chosen as the central point of RSM.
Inorganic salts can ionize positive or negative ions in solution, and the tendency of liquid-liquid phase separation is related to the interaction between inorganic salt ions and water molecules, which affects the distribution of extracted substances (39–41). The influence of (NH4)2SO4 quality fraction on the extraction rate of ALPs is shown in Figure 1C. With the increase of an (NH4)2SO4 quality fraction, the extraction rate of ALPs increased significantly as the (NH4)2SO4 quality fraction increased from 10% to 18% and then decreased when the (NH4)2SO4 quality fraction increased from 18% to 26%. When the (NH4)2SO4 quality fraction reached 18%, the extraction rate of ALPs reached the maximum value at 27.34 ± 0.12%. The main reason for this phenomenon may be due to the increase of an (NH4)2SO4 quality fraction, the improvement in the separation performance of the two aqueous phases and the increase in the volume of the lower phase of the system, which is beneficial to the aggregation of polysaccharides in the lower phase. However, when the (NH4)2SO4 quality fraction was too high, the “salting out” effect was enhanced in the presence of (NH4)2SO4, and the solubility of polysaccharides was reduced (42), which is the main reason for the decrease in the extraction rate of ALPs. Therefore, the (NH4)2SO4 quality fraction of 18% as the center point of RSM and the optimal (NH4)2SO4 quality fraction were selected.
According to the graph of temperature vs. ALPs extraction rate (Figure 1D), it was observed that the extraction rate of ALPs increased significantly when the extraction temperature was in the range of 50°C−80°C and rose to the maximum value (25.75 ± 0.10%) at 80°C. However, when the temperature exceeded 80°C, the extraction rate of ALPs decreased. This may be because that higher temperature could enhance the solubility and diffusion coefficient of ALPs in the extracting solvent, which could improve the extraction rate of ALPs. However, when the extraction temperature was too high, the volume of the upper and lower phases changed, the energy consumption increased, and the amount of polysaccharide dissolution increased, leading to a decrease in the ALP extraction rate. Therefore, the extraction temperature should not be too high in work. With careful consideration, the extraction temperature of 80°C was selected as the center point of RSM.
Analysis of the response surface
Statistical analysis and the model fitting
The results of the three-factor three-level experimental data are shown in Table 2. The Y (ALP extraction rate) range was 23.55%−28.96%. A total of 17 group experiments were designed by the Design-Expert 8.0.6 software; after multiple regression fitting, the second-order polynomial equation of the response regression model was obtained, as shown in the following equation:
where Y is the ALPs rate (%) and X1, X2, and X3 are the coded values of the PEG quality fraction (%), (NH4)2SO4 quality fraction (%), and extraction temperature (°C), respectively.
An analysis of variance (ANOVA) was performed on the experimental data to assess the significance and applicability of the quadratic model. The significance of each coefficient was estimated by comparing the magnitude of the p-value. The smaller the p-value, the more significant the coefficient, and vice versa (43). The results of ANOVA analysis are presented in Table 3. It was found that the p-value of the response regression model had a very high significance (p < 0.0001), indicating that the model had good applicability to this experiment. The lack-of-fit F-value of 1.46 and the lack-of-fit p-value of 0.3525 implied that the lack of fit was not significant relative to the pure error. The determination coefficient (R2) of the model was 0.9951, which indicated that this predicted model can explain 99.79% of the results, and only 0.49% of the total variance was not explained by the model. The adjustment determination coefficient () was 0.9951, indicating there was a good agreement between the experimental values and predicted values.
Table 3 shows the analysis of the significance detection results of each factor. It was clear from the table that the p-value of the first terms X1 (p < 0.0001), X2 (p < 0.0001), and X3 (p = 0.0041) were lower than 0.01, which indicated that the PEG quality fraction (X1), (NH4)2SO4 quality fraction (X2), and extraction temperature (X3) showed a significant impact on the extraction rate of ALPs. It was easy to determine that the PEG quality fraction and the (NH4)2SO4 quality fraction had a more significant impact on the extraction rate of ALPs than the extraction temperature. The second terms (p < 0.0001), (p < 0.0001), and (p < 0.0001) were also highly significant at the 1% level. The p-values of interaction items X1X2 (p = 0.0038) and X2X3 (p = 0.0047) were lower than 0.01, which was highly significant at the 1% level, and the interaction item X1X3 (p = 0.0527 > 0.05) was not significant. These p-values of interaction item indicated intense interaction between a PEG quality fraction and the (NH4)2SO4 quality fraction, an (NH4)2SO4 quality fraction and extraction temperature, and the interaction between a PEG quality fraction and extraction temperature were weaker.
Response surface plot and contour plot analyses
Studies have shown that the shape of the contour and response surface can reflect the strength of the interaction between the factors. The closer the contour was to an ellipse, the more vital the interaction between these two factors. If the interaction between the two factors was weak, the contour line was closer to a circle (31, 44). There was strong interaction among the factors, and if the surface diagram was steeper, the influence was more significant (43). The surface diagram of ALPs extraction results between any two factors was analyzed systematically to determine the optimal factor level and evaluate the effect on the extraction rate of ALPs. According to the regression equation, the 2D contour plots and 3D response surfaces generated are displayed in Figure 2. Their significance is consistent with the results of ANOVA.
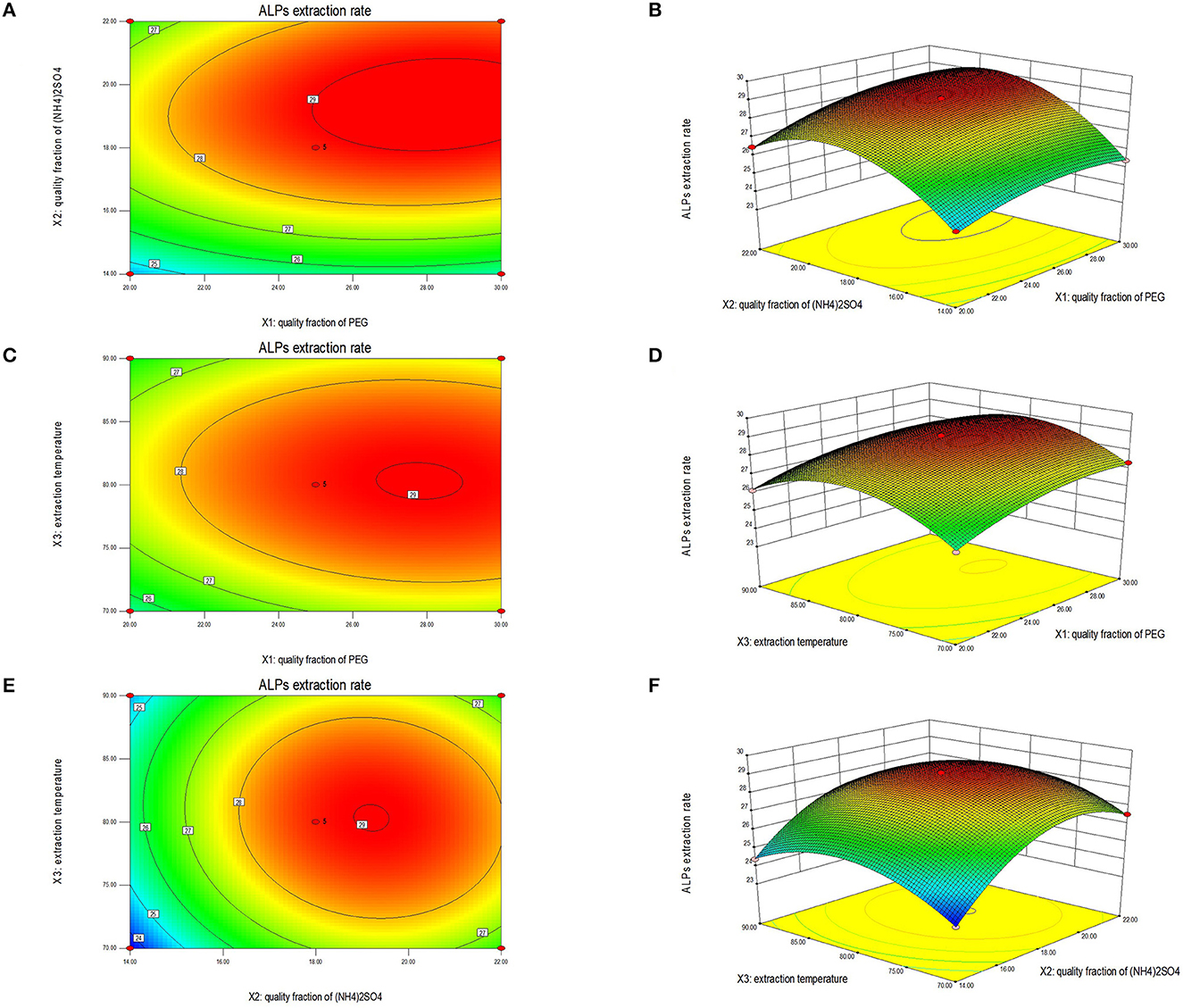
Figure 2. The 2D contour plot and 3D response surface showing the effect of PEG quality fraction and (NH4)2SO4 quality fraction (A, B), PEG quality fraction and extraction temperature (C, D), (NH4)2SO4 quality fraction and extraction temperature (E, F) on the extraction rate of ALPs.
Figures 2A, B show the 2D contour plot and 3D response surface of the interaction effect of the PEG quality fraction and the (NH4)2SO4 quality fraction on the extraction rate of ALPs. If the contour line (Figure 2A) of the PEG quality fraction and the (NH4)2SO4 quality fraction was an ellipse, and the response surface (Figure 2B) was steep, the quality fraction of PEG and the (NH4)2SO4 quality fraction had a significant interaction on the extraction rate of ALPs. Moreover, when the ratio of the PEG quality fraction was 25%, and the (NH4)2SO4 quality fraction was 18%, the extraction rate of ALPs could reach the maximum value. Figures 2C, D show the interaction effects of a PEG quality fraction and extraction temperature on the extraction rate of ALPs. According to the contour line shown in Figure 2C, the ellipticity was insignificant; therefore, the interaction was considered weaker. It can be observed from Figure 2D that the 3D graphics were relatively flat, indicating that the interaction between the PEG quality fraction and extraction temperature was not significant. When the ratio of the PEG quality fraction was 25%, and the extraction temperature was 80°C, the extraction rate of ALPs could reach the maximum value. Figures 2E, F show the contour plots and response surfaces of (NH4)2SO4 quality fraction and PEG quality fraction on the extraction rate of ALPs. While the contour shape was elliptical as shown in Figure 2E, the 3D graphics is steep as shown in Figure 2F. It was clear from this result that there was a strong interaction between an (NH4)2SO4 quality fraction and extraction temperature. When the (NH4)2SO4 quality fraction was 18%, and the extraction temperature was 80°C, the extraction rate of ALPs reached maximum value.
Validation of the predictive model
The predicted extraction rate of ALPs was obtained under the following conditions: the quality fraction of PEG-6000 27.65%, the quality fraction of (NH4)2SO4 19.18%, and the extraction temperature of 88.09°C. For operational convenience, the actual operating process conditions differed from the predicted optimal process conditions: the quality fraction of PEG-6000 was modified to 25%, the quality fraction of (NH4)2SO4 was 18%, and the extraction temperature was 80°C. The actual extraction rate of ALPs was 28.83%. The extraction rate was higher than the one discussed in the previous report by Guo et al. who reported that ALPs were extracted by ultrasound-assisted extraction method and the extraction rate was reached at 14.22% under solid–liquid radio of 1:20, with an extraction temperature of 55°C, extraction time of 55 min, and power of 175 W (45). Li et al. used a water extraction method to extract ALP, and the extraction rate was only 4.69% (16). It was indicated that the ATPS method had a better effect in extracting ALPs than the water extraction method and the ultrasonic-assisted method.
FTIR spectrum of ALPs
From the FTIR spectra of ALPs (Figure 3), we observed that the broad absorption peak at 3,500–3,200 cm−1 was due to the O–H stretching vibration and the peak at 2,950–2,900 cm−1 was due to C–H stretching vibration. The characteristic absorption peak of the acetyl group in the amide group at 1,620.5 cm−1 indicated the presence of aminosaccharide in ALPs, and the peak width of this characteristic peak indicated the low content of aminosaccharide. The peak at 1,348.1 cm−1 was a characteristic peak of the O–H bending vibration and at 1,283.7 cm−1 was a characteristic peak of the C–H bending vibration. The absorption peaks at 1,100–900 cm−1 indicated that ALPs had pyranose rings. The absorption peaks caused by the furan ring's C–H bending vibration and the pyran ring's C–O–C vibration were at 802.5 and 750.2 cm−1, respectively. The results indicate that ALPs are typical acidic polysaccharides.
Microstructure of ALPs
Figures 4A, B show the images of ALPs at 1,000× and 5,000× magnifications under scanning electron microscopy, respectively. In addition, ALPs appear as clumps, with an uneven surface and an irregular shape at magnification of 1,000×. Moreover, ALPs consist of many small particles of irregular shape and size. The aggregation and adhesion of many molecules or molecular groups may cause it. When magnified to 5,000×, ALPs had a wooly, coarse, and fine surface. The results indicate that the attraction between polysaccharide molecules exists, and the repulsive force between molecules is relatively weak.
Monosaccharide composition of ALPs
The monosaccharide composition of ALPs was determined by HPLC and the results are shown in Figure 5. It was found that ALPs mainly consisted of glucose, rhamnose, arabinose, and galactose with a molar ratio of 70.19:10.95:11.16:6.90. Previous research reported by Juliane et al. found that the polysaccharide RF30 of a crude polysaccharide fraction [Soluble Arctium lappa L. polysaccharide (SAA)] from Arctium lappa L. leaves contained galacturonic acid, galactose, arabinose, rhamnose, and glucose (17). The results of Li et al. also proved that ALPs mainly contain arabinose and galactose (16). The research of Yu et al. found that the acid hydrolyzed fragment (ALP2-A) of pectin from Arctium lappa L. was composed of rhamnose and galacturonic acid, and enzymatically hydrolyzed fragment (ALP2-E) contained rhamnose, glucuronic acid, galacturonic acid, galactose, and arabinose (46).
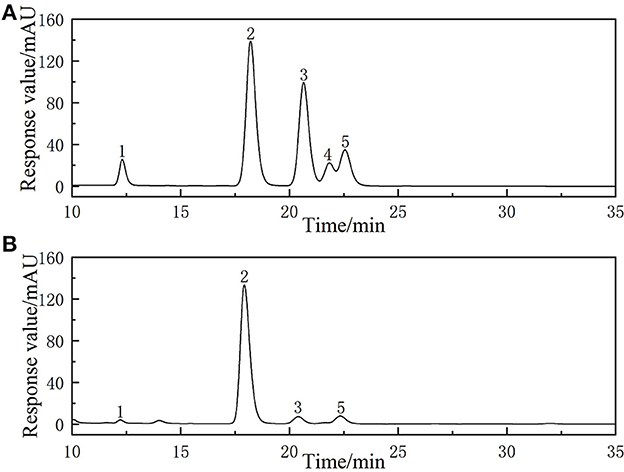
Figure 5. The HPLC chromatogram of (A) reference monosaccharides and (B) monosaccharides composition of ALPs.
Antioxidant activity analysis
·OH radical scavenging
It can be easily observed from Figure 6A that the OH radical scavenging capacity of ALPs is directly proportional to the concentration of ALPs. The scavenging ability of ALPs on the ·OH radical increased with increasing concentration of ALPs. It kept increasing until the OH radical scavenging rate was maximum (43.1%) at 1.0 mg/ml. The OH free radical can cause oxidative stress, triggering chronic diseases such as cancer and coronary heart disease (47). Furthermore, ALPs, which act as antioxidants, can regulate oxidative stress, reducing the potential harm of free radical oxidation (48). Therefore, hydroxyl radical scavenging ability is often used as one of the indicators to test antioxidant activity in vitro (49). The results indicated that ALPs had a certain degree of scavenging ability of the ·OH radical. In addition, the half-maximal inhibitory concentration (IC50) value of ALPs was 1.732 mg/ml, and the IC50 value of VC was 0.385 mg/ml. The study by Liu et al. reported the OH radical scavenging capacity of Arctium lappa L. polysaccharides (ALP1) was only 4.20% at 0.3125 mg/ml, and the OH radical scavenging rate of ALP1 reached 99.19% at 2.5 mg/ml (7). It indicated that ALPs had a powerful ability to scavenge the ·OH radicals and can regulate oxidative stress, reducing the potential harm of free radical oxidation.
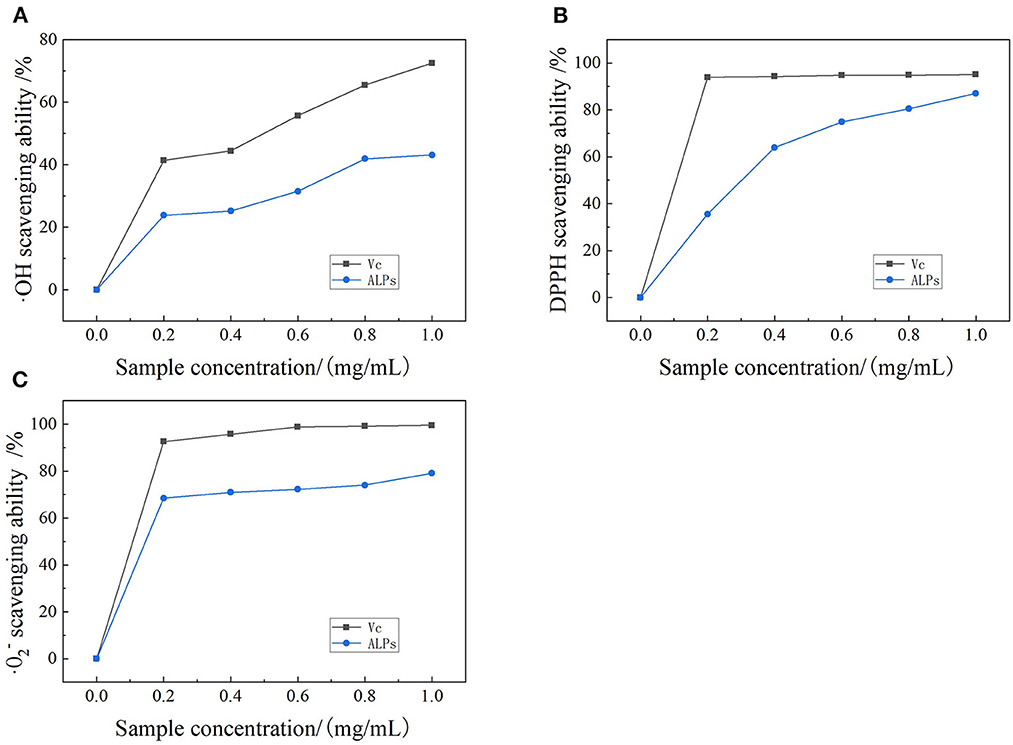
Figure 6. Scavenging effect of ALPs on (A) the ·OH radicals compared with VC, (B) the DPPH radicals compared with VC, and (C) the · radicals compared with VC.
DPPH radical scavenging
Observing the DPPH radical scavenging capacity curve of ALPs (Figure 6B), it was found that the scavenging capacity of ALPs increased continuously in the range of 0.0–1.0 mg/ml, and the scavenging capacity of ALPs increased to the maximum value (87%). When the concentration of ALPs was 1.0 mg/ml, the DPPH radical scavenging capacity of ALPs was close to that of VC. DPPH radical is a stable free radical with a single electron, and its alcohol solution is purple (50, 51). Furthermore, ALPs, which act as antioxidants, can make the single electron pair and DPPH radical alcohol solution fade (52, 53). Hence, the strength of the antioxidant can be determined according to the absorption value of the solution (54). It can be observed that ALPs have concentration-dependent scavenging activity and scavenging solid ability on DPPH free radicals. Moreover, the IC50 of ALPs was 0.290 mg/ml, while the IC50 value of VC was 0.11 mg/ml. A previous study reported that the mean IC50 value of DPPH radicals scavenging capacity of A. lappa root was 29.65 ± 4.03 μg/ml (55). Also, ALPs extracted in this study had stronger DPPH clearing ability.
Scavenging superoxide anion radicals
Superoxide anion (·) free radical is one of the free radicals with high activity and toxicity, leading to the formation of other toxic reactive oxygen species (ROS), which cause tissue damage and various diseases (56, 57). The scavenging ability of ALPs increased continuously within the test range from 0 mg/ml to 1.0 mg/ml (Figure 6C). The · scavenging ability of ALPs was increased rapidly and then slowly decreased to the range of 0.0–1.0 mg/ml. The scavenging rate of ALPs reached the maximum of 79.1% at 1.0 mg/ml. These results indicate that ALPs had a strong scavenging ability on the · free radical. Meanwhile, the IC50 value of ALPs and VC were 0.15 and 0.10 mg/ml. When compared with the result of Skowrońska et al. who reported that the mean IC50 value of the · radical scavenging capacity of A. lappa root was 27.50 ± 8.20 μg/ml (55), ALPs had a more vital scavenging ability against the · radicals.
Conclusion
Arctium lappa L. root is rich in polysaccharide, which has antioxidant, antitumor, and immune regulation effects, and has broad development prospects. In the present study, the ALPs were extracted by the ATPS method. The effects of PEG molecular weight, a quality fraction of PEG, a quality fraction of (NH4)2SO4, and the extraction temperature on the extraction rate of ALPs were studied, and RSM optimized the extraction process conditions of ALPs. The optimal extraction conditions were obtained as follows: the quality fraction of PEG-6000 was 25%, the quality fraction of (NH4)2SO4 was 18%, and the extraction temperature was 80°C. The extraction rate of ALPs could reach the maximum value of 28.83%. Both FTIR and SEM analyses of ALPs showed that ALPs were specific polysaccharides composed of irregular shapes and sizes of particles. The surface was uneven, and there was an attraction between the polysaccharide molecules. The results of HPLC showed that the ALPs were mainly composed of glucose, rhamnose, arabinose, and galactose with a molar ratio was 70.19:10.95:11.16:6.90. Finally, the result of the in vitro antioxidant experiment of ALPs showed that ALPs had a certain degree of scavenging ability on ·OH radical, DPPH radical, and · radical; their scavenging activities increased with the increase in polysaccharide concentration, and their IC50 values of ALPs were 1.732, 0.290, and 0.15 mg/ml, respectively. These results suggest that Arctium lappa L is a promising functional food.
Data availability statement
The original contributions presented in the study are included in the article/supplementary material, further inquiries can be directed to the corresponding author.
Author contributions
JY and DG took the initiative for this work and designed the experiments. DG, HL, and SM prepared experimental design of antioxidant. XY, XG, and HC prepared ALPs. YZ and JM prepared the determination of purified ALPs' structural characterization. DG and HC wrote the manuscript. All authors have read and approved the final manuscript.
Funding
This study was supported by the National Natural Science Foundation of China (No. 31960461), the Fundamental Research Funds for the Central Universities of Northwest Minzu University (Nos. 31920220045, 31920220024, and BELTY201901), and the Science and Technology Funding of Chengguan District, Lanzhou (No. 2022JSCX0011).
Conflict of interest
The authors declare that the research was conducted in the absence of any commercial or financial relationships that could be construed as a potential conflict of interest.
Publisher's note
All claims expressed in this article are solely those of the authors and do not necessarily represent those of their affiliated organizations, or those of the publisher, the editors and the reviewers. Any product that may be evaluated in this article, or claim that may be made by its manufacturer, is not guaranteed or endorsed by the publisher.
References
1. Adham NA, Hegazy MEF, Naqishbandi AM, Efferth T. Induction of apoptosis, autophagy, and ferroptosis by Thymus vulgaris and Arctium lappa extract in leukemia and multiple myeloma cell lines. Molecules. (2020) 25:5016. doi: 10.3390/molecules25215016
2. Cai E, Han J, Yang L, Zhang W, Zhao Y, Chen Q, et al. Novel method of preparation and activity research on arctigenin from Fructus arctii. Pharmacogn Mag. (2018) 14:87–94. doi: 10.4103/pm.pm_514_16
3. Romualdo GR, Silva EDA, Da Silva TC, Aloia TPA, Nogueira MS, De Castro IA, et al. Burdock (Arctium lappa L.) root attenuates preneoplastic lesion development in a diet and thioacetamide-induced model of steatohepatitis-associated hepatocarcinogenesis. Environ Toxicol. (2020) 35:518–27. doi: 10.1002/tox.22887
4. Liu YG, Hao C, Shi S, Dang K, Huang X, Zhao Z, et al. Transcriptome analysis of the immunomodulation by Arctium lappa L. polysaccharides in the Chinese mitten crab Eriocheir sinensis against Aeromonas hydrophila. Aquaculture. (2021) 534:736255. doi: 10.1016/j.aquaculture.2020.736255
5. Li J, Deng C, Zhao P, Wang N, Wang L. Kinetics on the leaching of polyphenol from Arctium lappal root. Adv Mat Res. (2012) 586:235–8. doi: 10.4028/www.scientific.net/AMR.586.235
6. Zhang X, Herrera-Balandrano DD, Huang W, Chai Z, Beta T, Wang J, et al. Comparison of nutritional and nutraceutical properties of burdock roots cultivated in Fengxian and Peixian of China. Foods. (2021) 10:2095. doi: 10.3390/foods10092095
7. Liu W, Wang J, Zhang Z, Xu J, Xie Z, Slavin M, et al. In vitro and in vivo antioxidant activity of a fructan from the roots of Arctium lappa L. Int J Biol Macromol. (2014) 65:446–53. doi: 10.1016/j.ijbiomac.2014.01.062
8. Zhou M, Li G, Zhu L, Zhou H, Lu L. Arctiin attenuates high glucose-induced human retinal capillary endothelial cell proliferation by regulating ROCK1/PTEN/PI3K/Akt/VEGF pathway in vitro. J Cell Mol Med. (2020) 24:5695–706. doi: 10.1111/jcmm.15232
9. Li J, Pang JH, Deng C, Zhao P, Hou SZ. Kinetics on the leaching of polysaccharide from Arctium lappa L. root. Adv Mat Res. (2012) 531:334–7. doi: 10.4028/www.scientific.net/AMR.531.334
10. Rosemary T, Arulkumar A, Paramasivam S, Mondragon-Portocarrero A, Miranda J. Biochemical, micronutrient and physicochemical properties of the dried red seaweeds Gracilaria edulis and Gracilaria corticata. Molecules. (2019) 24:2225. doi: 10.3390/molecules24122225
11. Yu M, Chen Y, Liu Y, Yu M, Xu Y, Wang B. Efficient polysaccharides from Crinum asiaticum L's structural characterization and anti-tumor effect. Saudi J Biol Sci. (2019) 26:2085–90. doi: 10.1016/j.sjbs.2019.09.017
12. Wang J, Huang L, Ren Q, Wang Y, Zhou L, Fu Y, et al. Polysaccharides of Scrophularia ningpoensis Hemsl: extraction, antioxidant, and anti-inflammatory evaluation. Evid Based Complement Alternat Med. (2020) 2020:8899762. doi: 10.1155/2020/8899762
13. Zou YF, Zhang YY, Fu YP, Inngjerdingen KT, Paulsen BS, Feng B, et al. A Polysaccharide isolated from Codonopsis pilosula with immunomodulation effects both in vitro and in vivo. Molecules. (2019) 24:3632. doi: 10.3390/molecules24203632
14. Li B, Dong M, De J, Ye L, Chen D, Lu Y. Structural characterization and anti-proliferation activities against tumor cells of an arabinogalactan from Juniperus convallium. Molecules. (2019) 24:1850. doi: 10.3390/molecules24101850
15. Jiang YY Yu J, Li YB, Wang L, Hu L, Zhang L, et al. Extraction and antioxidant activities of polysaccharides from roots of Arctium lappa L. Int J Biol Macromol. (2019) 123:531–8. doi: 10.1016/j.ijbiomac.2018.11.087
16. Li L, Qiu Z, Dong H, Ma C, Qiao Y, Zheng Z. Structural characterization and antioxidant activities of one neutral polysaccharide and three acid polysaccharides from the roots of Arctium lappa L.: a comparison. Int J Biol Macromol. (2021) 182:187–96. doi: 10.1016/j.ijbiomac.2021.03.177
17. Carlotto J, de Souza LM, Baggio CH, Werner MF, Maria-Ferreira D, Sassaki GL, et al. Polysaccharides from Arctium lappa L.: chemical structure and biological activity. Int J Biol Macromol. (2016) 91:954–60. doi: 10.1016/j.ijbiomac.2016.06.033
18. Wang M, Zhao Z, Zhou X, Hu J, Xue J, Liu X, et al. Simultaneous use of stimulatory agents to enhance the production and hypoglycaemic activity of polysaccharides from Inonotus obliquus by submerged fermentation. Molecules. (2019) 24:4400. doi: 10.3390/molecules24234400
19. Xing S, Zhang X, Ke H, Lin J, Huang Y, Wei G. Physicochemical properties of polysaccharides from Dendrobium officinale by fractional precipitation and their preliminary antioxidant and anti-HepG2 cells activities in vitro. Chem Cent J. (2018) 12:103. doi: 10.1186/s13065-018-0468-4
20. Yoo SH, Chang YH. Effects of extraction methods on in vitro biological capacities and rheological properties of polysaccharides from red pepper stems. Prev Nutr Food Sci. (2017) 22:223–30. doi: 10.3746/pnf.2017.22.3.223
21. Neelam K, Dey S, Sim R, Lee J, Au Eong KG. Fructus lycii: a natural dietary supplement for amelioration of retinal diseases. Nutrients. (2021) 13:246. doi: 10.3390/nu13010246
22. Chen M, Xu J, Wang Y, Wang Z, Guo L, Li X, et al. Arctium lappa L. polysaccharide can regulate lipid metabolism in type 2 diabetic rats through the SREBP-1/SCD-1 axis. Carbohydr Res. (2020) 494:108055. doi: 10.1016/j.carres.2020.108055
23. Chen L, Pang Y, Luo Y, Cheng X, Lv B, Li C. Separation and purification of plant terpenoids from biotransformation. Eng Life Sci. (2021) 21:724–38. doi: 10.1002/elsc.202100014
24. Zhang X, Teng G, Zhang J. Ethanol/salt aqueous two-phase system based ultrasonically assisted extraction of polysaccharides from Lilium davidiivar. unicolor Salisb: physicochemical characterization and antiglycation properties. J Mol Liquids. (2018) 256:497–506. doi: 10.1016/j.molliq.2018.02.059
25. Si L, Lin R, Jia Y, Jian W, Yu Q, Wang M, et al. Lactobacillus bulgaricus improves antioxidant capacity of black garlic in the prevention of gestational diabetes mellitus: a randomized control trial. Biosci Rep. (2019) 39:BSR20182254. doi: 10.1042/BSR20182254
26. Yeh SH, Hsu WK, Chang ZQ, Wang SH, Hsieh CW, Liou GG, et al. Purification and characterization of fractions containing polysaccharides from Talinum triangulare and their immunomodulatory effects. Processes. (2021) 9:709. doi: 10.3390/pr9040709
27. Zhang F, Zhang N, Xu Q, Zhang L, Zhang C, Liu H, et al. Decellularized nerve extracellular matrix/chitosan crosslinked by genipin to prepare a moldable nerve repair material. Cell Tissue Bank. (2021) 22:419–30. doi: 10.1007/s10561-020-09889-2
28. Ma XL, Song FF, Zhang H, Huan X, Li SY. Compositional monosaccharide analysis of Morus nigra Linn by HPLC and HPCE quantitative determination and comparison of polysaccharide from Morus nigra Linn by HPCE and HPLC. Curr Pharm Anal. (2017) 13:433–7. doi: 10.2174/1573412913666170330150807
29. Wang L, Wang L, Wang X, Lu B, Zhang J. Preparation of blueberry anthocyanin liposomes and changes of vesicle properties, physicochemical properties, in vitro release, and antioxidant activity before and after chitosan modification. Food Sci Nutr. (2022) 10:75–87. doi: 10.1002/fsn3.2649
30. Liu H, Li F, Luo P. Effect of carboxymethylation and phosphorylation on the properties of polysaccharides from Sepia esculenta Ink: antioxidation and anticoagulation in vitro. Mar Drugs. (2019) 17:626. doi: 10.3390/md17110626
31. Liu J, Li C, Ding G, Quan W. Artificial intelligence assisted ultrasonic extraction of total flavonoids from Rosa sterilis. Molecules. (2021) 26:3835. doi: 10.3390/molecules26133835
32. Ren Y, Wang X, Liang H, He W, Zhao X. Mechanism of miR-30b-5p-Loaded PEG-PLGA nanoparticles for targeted treatment of heart failure. Front Pharmacol. (2021) 12:745429. doi: 10.3389/fphar.2021.745429
33. Gharat NN, Rathod VK. Response surface methodology for the extraction of wedelolactone from Eclipta alba using aqueous two-phase extraction. Prep Biochem Biotechnol. (2020) 50:827–33. doi: 10.1080/10826068.2020.1753071
34. Huang J, Zhao D, Dangaria SJ, Luan X, Diekwisch TG, Jiang G, et al. Combinatorial design of hydrolytically degradable, bone-like biocomposites based on PHEMA and hydroxyapatite. Polymer (Guildf). (2013) 54:909–19. doi: 10.1016/j.polymer.2012.12.017
35. Hsu HY, Harris MT, Toth S, Simpson GJ. Drop printing of pharmaceuticals: effect of molecular weight on PEG coated-naproxen/PEG 3350 solid dispersions. AIChE J. (2015) 61:4502–8. doi: 10.1002/aic.14979
36. Xing JM, Li FF. Purification of aloe polysaccharides by using aqueous two-phase extraction with desalination. Nat Prod Res. (2009) 23:1424–30. doi: 10.1080/14786410902800657
37. Flieger J, Feder-Kubis J, Tatarczak-Michalewska M. Chiral ionic liquids: structural diversity, properties and applications in selected separation techniques. Int J Mol Sci. (2020) 21:4253. doi: 10.3390/ijms21124253
38. Tong S, Ito Y, Ma Y. Enantioseparation of (DL)-tryptophan by spiral tube assembly counter-current chromatography and evaluation of mass transfer rate for enantiomers. J Chromatogr A. (2014) 1374:77–84. doi: 10.1016/j.chroma.2014.11.013
39. Neves C, Shahriari S, Lemus J, Pereira JFB, Freire MG, Coutinho JAP. Aqueous biphasic systems composed of ionic liquids and polypropylene glycol: insights into their liquid-liquid demixing mechanisms. Phys Chem Chem Phys. (2016) 18:20571–82. doi: 10.1039/c6cp04023c
40. Lu X, Zheng Z, Miao S, Li H, Guo Z, Zhang Y, et al. Separation of oligosaccharides from lotus seeds via medium-pressure liquid chromatography coupled with ELSD and DAD. Sci Rep. (2017) 7:44174. doi: 10.1038/srep44174
41. Fierascu RC, Fierascu I, Ortan A, Georgiev MI, Sieniawska E. Innovative approaches for recovery of phytoconstituents from medicinal/aromatic plants and biotechnological production. Molecules. (2020) 25:309. doi: 10.3390/molecules25020309
42. Gray Be A, Upshur MA, Liu P, Martin ST, Geiger FM, Thomson RJ. Cloud activation potentials for atmospheric alpha-pinene and beta-caryophyllene ozonolysis products. ACS Cent Sci. (2017) 3:715–25. doi: 10.1021/acscentsci.7b00112
43. Savikin K, Zivkovic J, Jankovic T, Cujic-Nikolic N, Zdunic G, Menkovic N, et al. Optimization of ultrasound-assisted extraction of phenolics from Sideritis raeseri using response surface methodology. Molecules. (2021) 26:3949. doi: 10.3390/molecules26133949
44. Yuan E, Nie S, Liu L, Ren J. Study on the interaction of Hericium erinaceus mycelium polysaccharides and its degradation products with food additive silica nanoparticles. Food Chem X. (2021) 12:100172. doi: 10.1016/j.fochx.2021.100172
45. Guo T, Wei JQ, Wang Y, Su D, Zhang Z, Ma JP, et al. Artificial neural network modeling of ultrasound-assisted polysaccharides extraction from Potentilla anserina and anti-platelet aggregation activity. Appl Mech Mater. (2014) 618:367–75. doi: 10.4028/www.scientific.net/AMM.618.367
46. Yu J, Ye M, Li K, Wang F, Shi X, Pan C, et al. Fragments of a pectin from Arctium lappa L.: molecular properties and intestinal regulation activity. J Funct Foods. (2022) 88:104900. doi: 10.1016/j.jff.2021.104900
47. Kurnia D, Ajiati D, Heliawati L, Sumiarsa D. Antioxidant properties and structure-antioxidant activity relationship of allium species leaves. Molecules. (2021) 26:7175. doi: 10.3390/molecules26237175
48. Naomi R, Ratanavaraporn J, Fauzi MB. Comprehensive review of hybrid collagen and silk fibroin for cutaneous wound healing. Materials. (2020) 13:3097. doi: 10.3390/ma13143097
49. Kim YK, Koppula S, Shim DW, In EJ, Kwak SB, Kim MK, et al. Inhibitory effect and mechanism of Arctium lappa extract on NLRP3 inflammasome activation. Evid Based Complement Alternat Med. (2018) 2018:6346734. doi: 10.1155/2018/6346734
50. Tenuta MC, Deguin B, Loizzo MR, Dugay A, Acquaviva R, Malfa GA, et al. Contribution of flavonoids and iridoids to the hypoglycaemic, antioxidant, and nitric oxide (NO) inhibitory activities of Arbutus unedo L. Antioxidants (Basel). (2020) 9:184. doi: 10.3390/antiox9020184
51. Tang Z, Wang Y, Yang J, Xiao Y, Cai Y, Wan Y, et al. Isolation and identification of flavonoid-producing endophytic fungi from medicinal plant Conyza blinii HLev that exhibit higher antioxidant and antibacterial activities. PeerJ. (2020) 8:e8978. doi: 10.7717/peerj.8978
52. Hachkova H, Nagalievska M, Soliljak Z, Kanyuka O, Kucharska AZ, Sokol-Letowska A, et al. Medicinal plants Galega officinalis L. and yacon leaves as potential sources of antidiabetic drugs. Antioxidants (Basel). (2021) 10:1362. doi: 10.3390/antiox10091362
53. Termer M, Carola C, Salazar A, Keck CM, von Hagen J. Methoxy-monobenzoylmethane protects human skin against UV-induced damage by conversion to avobenzone and radical scavenging. Molecules. (2021) 26:6141. doi: 10.3390/molecules26206141
54. Burgos C, Munoz-Mingarro D, Navarro I, Martin-Cordero C, Acero N. Neuroprotective potential of verbascoside isolated from Acanthus mollis L. leaves through its enzymatic inhibition and free radical scavenging ability. Antioxidants (Basel). (2020) 9:1207. doi: 10.3390/antiox9121207
55. Skowronska W, Granica S, Dziedzic M, Kurkowiak J, Ziaja M, Bazylko A. Arctium lappa and Arctium tomentosum, Sources of Arctii radix: comparison of anti-lipoxygenase and antioxidant activity as well as the chemical composition of extracts from aerial parts and from roots. Plants (Basel). (2021) 10:78. doi: 10.3390/plants10010078
56. Oniszczuk A, Oniszczuk T, Gancarz M, Szymanska J. Role of gut microbiota, probiotics and prebiotics in the cardiovascular diseases. Molecules. (2021) 26:1172. doi: 10.3390/molecules26041172
Keywords: Arctium lappa L., polysaccharides, aqueous two-phase system, response surface methodology, antioxidant activity
Citation: Gao D, Chen H, Li H, Yang X, Guo X, Zhang Y, Ma J, Yang J and Ma S (2023) Extraction, structural characterization, and antioxidant activity of polysaccharides derived from Arctium lappa L. Front. Nutr. 10:1149137. doi: 10.3389/fnut.2023.1149137
Received: 21 January 2023; Accepted: 21 February 2023;
Published: 21 March 2023.
Edited by:
Yanjun Zhang, Chinese Academy of Tropical Agricultural Sciences, ChinaReviewed by:
Jianhua Liu, Zhejiang University of Technology, ChinaJunjie Wang, North Minzu University, China
Ping Li, Zhejiang Gongshang University, China
Copyright © 2023 Gao, Chen, Li, Yang, Guo, Zhang, Ma, Yang and Ma. This is an open-access article distributed under the terms of the Creative Commons Attribution License (CC BY). The use, distribution or reproduction in other forums is permitted, provided the original author(s) and the copyright owner(s) are credited and that the original publication in this journal is cited, in accordance with accepted academic practice. No use, distribution or reproduction is permitted which does not comply with these terms.
*Correspondence: Jutian Yang, anV0aWFueWFuZzk4OCYjeDAwMDQwOzE2My5jb20=
†These authors have contributed equally to this work