- 1Biology Department, Center for Biodiversity Research and Extension in Mindanao, Central Mindanao University, Musuan, Philippines
- 2School of Biological and Food Engineering, Suzhou University, Suzhou, China
- 3Engineering Research Center for Development and High Value Utilization of Genuine Medicinal Materials in North Anhui Province, Suzhou, China
- 4School of Biology, Food and Environment, Hefei University, Hefei, China
- 5Anhui Promotion Center for Technology Achievements Transfer, Anhui Academy of Science and Technology, Hefei, China
Fruits and vegetables contain numerous nutrients, such as vitamins, minerals, phenolic compounds, and dietary fibers. They reduce the incidence of cardiovascular diseases and the risk of certain chronic diseases, and improve the antioxidant and anti-inflammatory capacity. Moreover, melatonin was found in various fruits and vegetables species. Melatonin acts as a multifunctional compound to participate in various physiological processes. In recent years, many advances have been found that melatonin is also appraised as a key modulator on the fruits and vegetables post-harvest preservation. Fruits and vegetables post-harvest usually elicit reactive oxygen species (ROS) generation and accumulation. Excess ROS stimulate cell damage, protein structure destruction, and tissue aging, and thereby reducing their quality. Numerous studies find that exogenous application of melatonin modulates ROS homeostasis by regulating the antioxidant enzymes and non-enzymatic antioxidants systems. Further evidences reveal that melatonin often interacts with hormones and other signaling molecules, such as ROS, nitric oxide (NO), hydrogen sulfide (H2S), and etc. Among these ‘new’ molecules, crosstalks of melatonin and ROS, especially the H2O2 produced by RBOHs, are provided in fruits and vegetables post-harvest preservation in this review. It will provide reference for complicated integration of both melatonin and ROS as signal molecules in future study.
Introduction
Fruits and vegetables contain numerous nutrients, such as vitamins, minerals, phenolic compounds, and dietary fibers (1–4). They play an essential part of a well-balanced daily food. It is generally recommended to eat more fruits and vegetables to reduce the incidence of cardiovascular diseases and the risk of certain chronic diseases, and improve the antioxidant and anti-inflammatory capacity (3, 5). For example, polyphenols inhibit chronic inflammation through regulating multiple inflammation-associated cell signaling pathways (6). However, fruits and vegetables often generate significant post-harvest losses after harvest (3). They are vulnerable to mechanical damages, water and phytochemicals loss, microbial infections, thus resulting in a considerable concern during long-term storage (7, 8). To reduce post-harvest losses, several appropriate storage technologies are used, including cold chain management, hypobaric storage, modified atmosphere package (MAP), and ultraviolet treatment (9–13). To some extent, natural/synthetic preservative agent can also preserve fruits and vegetables storage, whereas there are some residues of chemical compounds (14). To date, previous studies also indicate that plant natural hormones (melatonin, ethylene (ET), salicylic acid (SA), and methyl jasmonate (MeJA), etc) and signaling molecules (nitric oxide (NO), hydrogen sulfide (H2S), and reactive oxygen species (ROS), etc) can play key roles in regulating the maturation and senescence of fruits and vegetables, delaying postharvest senescence and extending shelf life (15–21).
Acting as a pleiotropic compound, melatonin (N-acetyl-5-methoxytryptamine) has a wide range of cellular and physiological functions in living organisms (22–24). For example, melatonin modulates sleep and circadian rhythms, enhances immunity and anti-inflammatory activities (23, 24). Melatonin improves the anti-inflammatory activity, particularly against the chronic inflammation which induced by many chronic diseases (25). In plants, melatonin was firstly detected in 1995 (26, 27). Since then, it was found in various plant species and their different tissue parts, such as rice, wheat, tomato, apple, strawberry, grape, pepper, cucumber, and solanaceous, etc (28–36). Melatonin acts a key molecule to mediate multiple physiological processes, such as the alleviation of abiotic and biotic stresses, and plant growth and development (37–42). For example, melatonin obviously promoted the lateral root formation in Arabidopsis thaliana (37). Recently, many studies have reported that melatonin plays an vital role in the fruit and vegetable post-harvest preservation (43–46). In general, endogenous melatonin was increased by exogenous application of melatonin in broccoli, pear, and Zizyphus jujuba fruit (43, 44, 46). Then, melatonin observably decreased the accumulation of ROS by enhancing antioxidant capacity and total phenolic and ascorbic acid (AsA) content, and improved the quality of fruits and vegetables (43, 44, 46). Besides, melatonin improved the polyphenol accumulation and antioxidant capacity via ethylene signaling in grape berries (47).
ROS contain a group of molecules, mainly including hydrogen peroxide (H2O2), hydroxyl radical (OH), superoxide anion (O2•–), and singlet oxygen (1O2) (48). ROS can cause the oxidation of lipids, and damages of proteins and many other small molecules structures (48). Accordingly, plants have evolved sophisticated antioxidant strategies to regulate the ROS homeostasis, such as antioxidant enzymes [catalase (CAT), ascorbate peroxidase (APX), superoxide dismutase (SOD), and glutathione peroxidase (GPX)] and non-enzymatic antioxidants (glutathione (GSH), AsA, flavonoids, carotenoids, and alkaloids, etc) (40, 41). Moreover, numerous studies revealed that ROS play key dual roles in the signaling networks in plant stress responses and developmental processes (49, 50). Interestingly, several studies have revealed that the signaling crosstalk between melatonin and ROS was also suggested in red pear and strawberry fruits during post-harvest period (51, 52).
In this review, we mainly discuss exogenous application of melatonin in fruits and vegetable preservation, synthesis of endogenous melatonin, effects of melatonin on the quality of postharvest fruits and vegetable, and the mechanism of melatonin-modulated postharvest protection of fruits and vegetables. We further highlight and discuss the vital role of ROS signaling during the processes, so as to provide reference for future complicated integration of both melatonin and ROS as signal molecules.
The changes of phenomenon and quality of fruits and vegetables during the postharvest period
Fruits and vegetables contain diverse nutrients, such as phenolic compounds, AsA, carotenoids, and mineral content, which beneficial for the anti-nflammation, antioxidation, anti-diabetes, cancer prevention, and cardio-protection in human (1, 2). Many popular kinds of fruits and vegetables, such as tomato, apple, banana, papaya, etc., are consumed worldwide with the rapidly increasing demand and production. However, most of these are highly susceptible to soften rapidly and over-ripen, and often accompanying by the chlorophyll degradation and pathogens (53–59). For example, papaya ripened and softened rapidly, and the fruit peel color gradually turned from green to yellow after harvest (53). Meanwhile, the lightness value declined slightly, the chroma value increased, and the hue angle value gradually dropped during late storage. The most serious damage was disease incidence, and thus decreasing the papaya commodity rate. Similar changes of fruit firmness, hue angle, brightness, and color saturation values were also found in guava during the postharvest period (54). After harvest for 11 days, the anthracnose disease index and disease incidence increased rapidly. In cherry tomato and litchi fruits, the weight loss and fruit firmness were declined, accompanied by fruit decay during storage (57, 59). Furthermore, other fruits and vegetables usually encountered the same cases as well (56, 57, 60). Hence, low-temperature preservation for fruits and vegetables has received increasing research attention (61). Nevertheless, storage for long times may cause chilling injury, such as surface pitting and browning, inability to ripen, watersoaking lesions, and rapid decay (62, 63).
The changes of melatonin content in fruits and vegetables during the postharvest period
Our previous reviews systematically summarized the melatonin biosynthesis and catabolism in plant tolerance to abiotic stresses (38, 40–42). In general, various abiotic stresses, such as salinity, heat, cold, drought, and cadmium metal stresses induce melatonin accumulation by the upregulation of genes which encoding tryptamine 5-hydroxylase (T5H), tryptophan decarboxylase (TDC), N-acetylserotonin methyltransferase (ASMT), serotonin N-acetyltransferase (SNAT), and caffeic acid O-methyltransferase (COMT) (40). Interestingly, the changes of melatonin content have different trends among different kinds of fruits and vegetables, and some findings were listed in Table 1 and (19, 44, 58, 64–71, 73). Wang et al. (19) found that endogenous melatonin was increased at 0 d to 14 d, and decreased at 14 d to 63 d throughout storage period in cherry fruit. Interestingly, it was decreased dramatically from anthesis to maturity period (45). These results suggested that endogenous melatonin accumulation was regulated by growing and picking storage periods in fruits. Similarly, melatonin content of table grape, mango, cassava, and strawberry was in parallel with the change trend of cherry fruit, and manifested a trend of rising first and then falling (64–66, 69). Nevertheless, in “Summer black” grape, the change of melatonin accumulation showed an contrary tendency (67). Besides, it showed an decreasing trend in angeleno plum, pakchoi, and cherry tomato (70, 71, 73). Moreover, expression of the genes TDCs, T5Hs, SNATs, and ASMTs related to melatonin biosynthesis were also differently regulated in table grape, mulberry fruits, cassava, strawberry, and cherry tomato (64–66, 68, 73). Therefore, melatonin accumulation and its biosynthesis genes transcripts are dynamic and highly regulated in various fruits and vegetables during the post-harvest period.
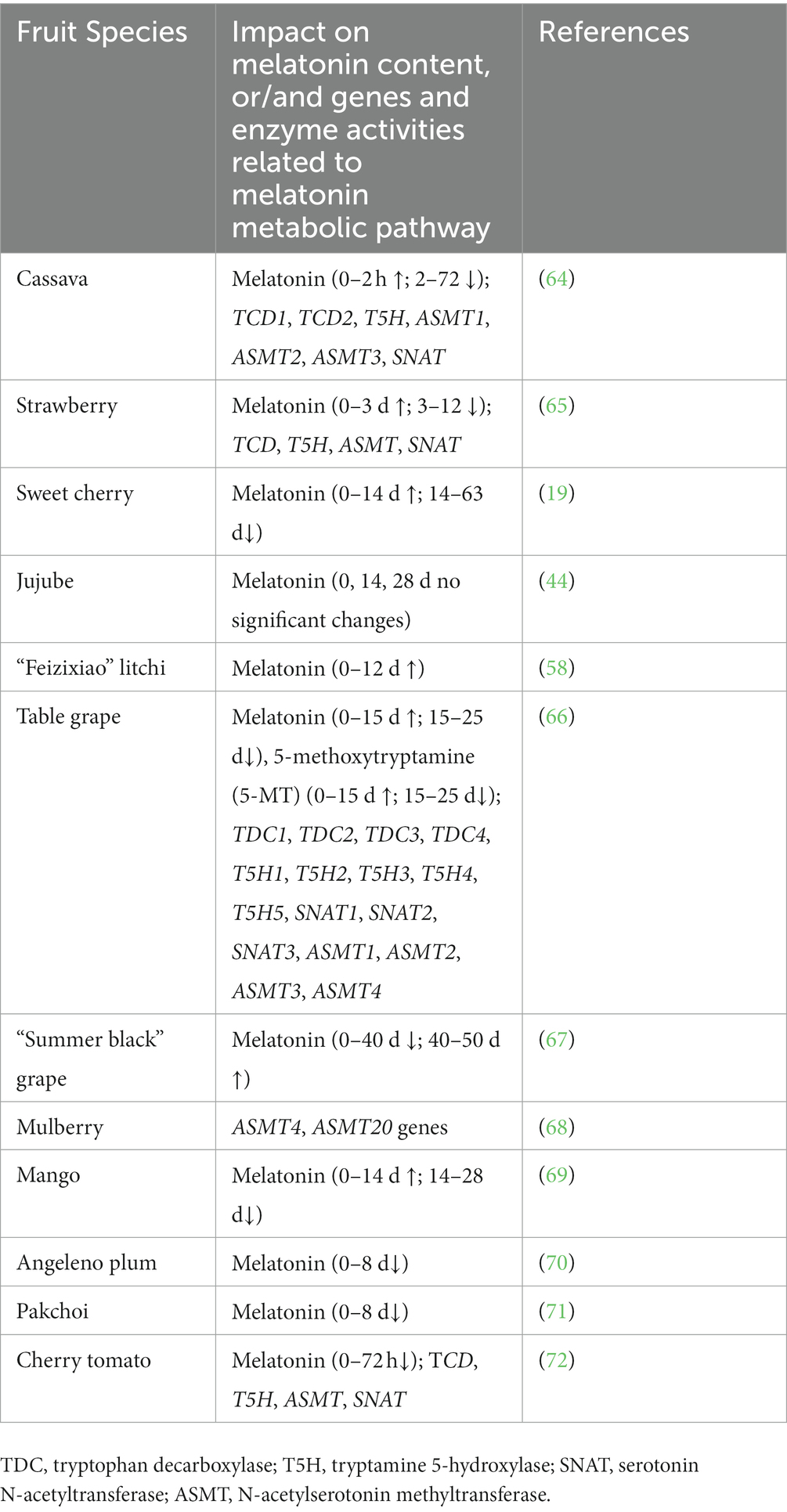
Table 1. Summary table explaining the changes of melatonin content, and genes related to melatonin metabolic pathway in fruits and vegetables during the postharvest period.
Protective effects of exogenous melatonin on qualities of fruits and vegetables during the postharvest period
Previous studies have shown that hormones, such as ET, SA, gibberellins [GAs, including gibberellin 1 (GA1), gibberellin 3 (GA3), gibberellin 4 (GA4), and gibberellin 7 (GA7)], MeJA, and abscisic acid (ABA), modulate the postharvest preservation of fruits and vegetables (70, 74–77). Over the past several years, numerous reports have proposed that melatonin acts as an important role on qualities of fruits and vegetables during the postharvest period (53, 54, 56–60, 67, 76). For example, exogenous melatonin treatments delayed fruit firmness decrease, maintained higher hue of the peel fruit, and retained greater lightness of papayas than the control group during the later storage period (53, 54). Similarly, it observably alleviated the decrease of fifirmness and the weight loss in cherry tomato (59). Fruit colour index (a*/b*) was also obviously increased by melatonin treatment in both sweet cherry and guava fruits (78). In pepper, broccoli, and Chinese flowering cabbage vegetables, exogenous melatonin application inhibited the degradation of chlorophyll during the postharvest period (43, 56, 79). In addition to the above phenotypic changes, melatonin also reduced the decay and disease index in fruits (41, 53, 54, 80). Moreover, exogenous melatonin also bought about significant increases in total soluble solids, sugar, protein, AsA, carotenoids, and total flavonoid and phenols contents, which were important substances of fruits and vegetables (43, 56, 81–83). Besides, melatonin mediated the aroma volatiles (propyl acetate and hexyl acetate) of postharvest pear fruit (84, 85).
Effects of exogenous melatonin on the redox homeostasis of fruits and vegetables during the postharvest period
In general, ROS (mainly MDA, H2O2, and O2•–) are largely caused during fruit ripening period, and induce oxidizing proteins and membrane lipids formation (53). For example, O2•– produce by the oxygen reduction by the electron transport chain (ETC) (53, 54). They also generate by photorespiration pathway and fatty acid-oxidation reaction (59). Then, H2O2 produces from O2•– by the activity of SOD and/or glycolate oxidases. Moreover, NADPH oxidases, polyamine oxidases (PAO), and cell wall bound peroxidases (POX) induce the ROS generation in cell membrane, cell wall, and apoplast, respectively (7, 57, 58). As toxic byproducts, ROS could cause serious damages to proteins and quality of fruits and vegetables. Combined with the antioxidant capacity of melatonin, these led to study the role of melatonin in the postharvest preservation of fruits and vegetables, especially in recent years (86–110). In this review, the protective impacts of melatonin on the antioxidant capacity of fruits and vegetables during the postharvest period have been summarized in Table 2. In fact, ROS were largely stimulated in fruits and vegetables, including papaya, cherry tomato, pepper, wax apple, Chinese flowering cabbage, pear, peach, litch, pomegranate, sweet cherry, sapota, apple, blueberry, longan, zucchini, guava, rambutan, water bamboo shoot, mango, tomato, eggplant, rosa roxburghii fruit, cucumber, jujube, sweetpotato, avocado, persimmons, and table grape during the postharvest period (stored at room temperature and/or low temperature; Table 2). Then, the ROS accumulation were significantly decreased by exogenous application of melatonin. Two main pathways might be involved in melatonin-inhibited ROS acumulation. Exogenous application of melatonin improved the antioxidant contents, such as GSH, AsA, proline, flavonoids, carotenoids, anthocyanins, and dehydroascorbate (DHA) through inducing the expression of GSH, GR1, GR2, GMDH, GME, GGGT, GPP, GDH, and GLDH genes (Table 2) and (88, 92, 95, 101). In most of the above fruits and vegetables, the antioxidant enzymes act as key roles in melatonin-downregulated ROS overproduction, such as CAT, SOD, APX, GR, GPX, DHAR, and MDHAR (Table 2). Besides, exogenous application of melatonin enhanced the total antioxidant capacity (T-AOC), cupric-reducing antioxidant power (CUPRAC), ferric-reducing antioxidant power (FRAP), trolox equivalent antioxidant capacity (TEAC), ferric reducing antioxidant power (FRAP), and 1,1-diphenyl-2-trinitrophenylhydrazine (DPPH) and 2,2′-azino-bis (3-ethylbenzothiazoline-6-sulfonic acid) (ABTS) radical scavenging capacity. For example, exogenously melatonin obviously induced the expression of PpAPXs, PpSODs, and PpCATs, and thereby activating the antioxidant system in peach fruit during storage for 14 d (88). Furthermore, the expression of AsA biosynthetic genes (including GMDH, GME, GGGT, GPP, GDH, and GLDH) were also stimulated, which increase the content of AsA to inhibit the ROS accumulation (88). In addition, exogenous melatonin interacted with ROS by regulating the expression of genes involved in AsA-GSH cycle, such as DHA, DHAR, MDHAR, GSH, GSSG, and GR in sweet cherry (101). Among the above fruits, blueberry contains high level of bioactive compounds, flavonoids and anthocyanins. These were also increased by exogenous melatonin to improve the nutraceutical traits of blueberry fruit during storage time (98).
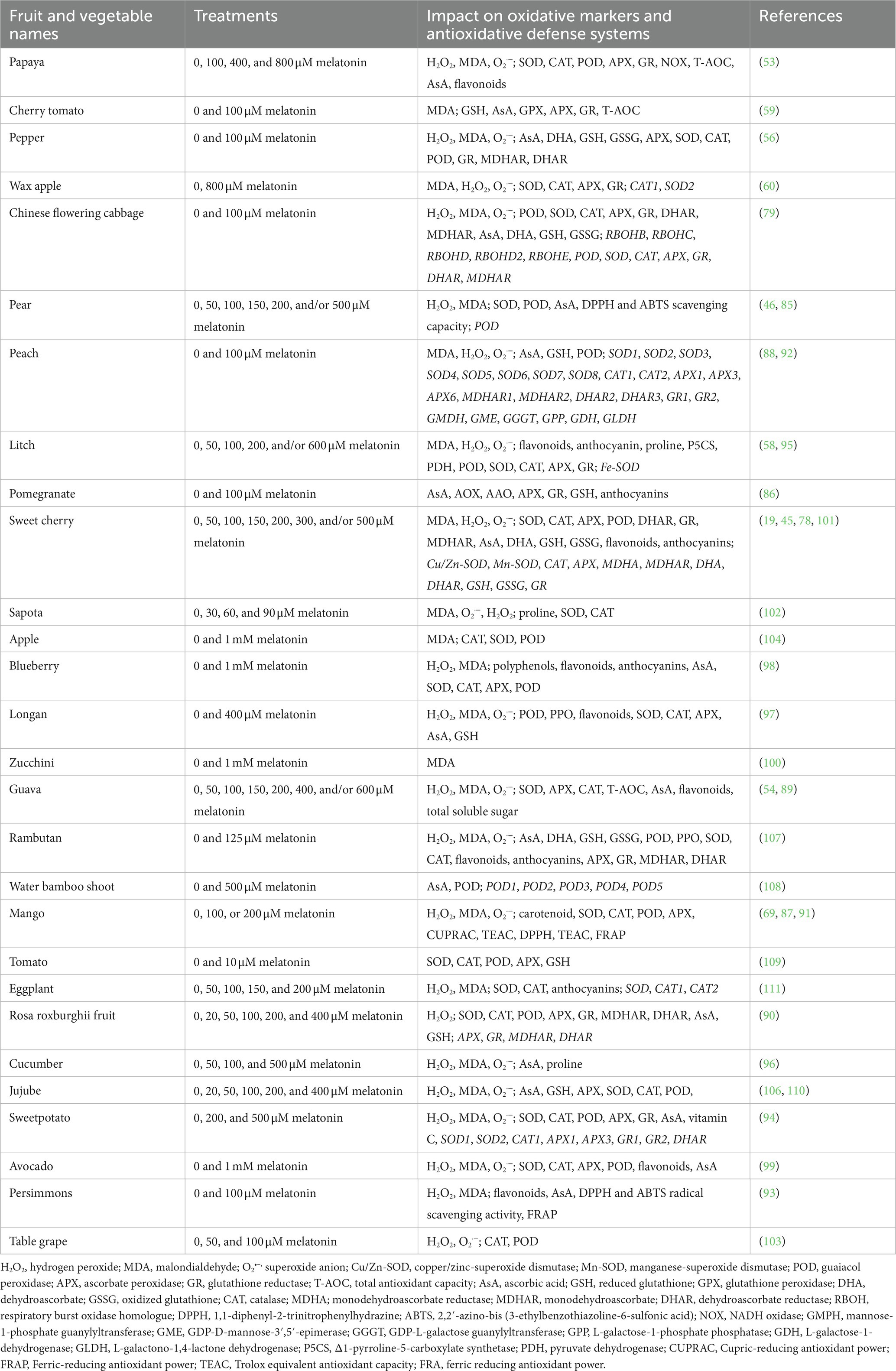
Table 2. Summary table explaining the impacts of exogenous melatonin on the antioxidative defense systems of fruits and vegetables during the postharvest period.
The roles of hormones in melatonin-modulated postharvest protection of fruits and vegetables during storage period
In recent years, hormones have been described to regulate fruits and vegetables postharvest performance (112). For example, ET and ABA played central roles in modulating senescence that strongly influence fruits and vegetables shelf-life (21, 113, 114). Ethylene is synthesized from S-adenosylmethionine to 1-aminocyclopropane-1-carboxylate (ACC) by ACC synthase (ACS), and then ACC is oxidazed by ACC oxidase (ACO) (21). Thus, ACS and ACO are the rate-limiting enzymes involved in this biosynthetic pathway. To reduce the ethylene accumulation through regulating the expression of genes encoding ACS and ACO enzymes might contribute to delay fruits and vegetables senescence (21, 114). Exogenous application of ABA induced flavanols and anthocyanin accumulation to promote the fruit coloration in fruits, including apple, grape, tomato, and litchi (115–118). Meanwhile, JA and SA have been suggested to be involved in the disease resistance during postharvest period (15, 16, 119, 120). MeJA induced the expression of JA synthesis genes, increased the allene oxide cyclase (AOC) activity, and thereby resulting in high endogenous JA generation (119). Nevertheless, DIECA treatment reduced the endogenous levels of JA, and AOC and 12-oxo-phytodienoic acid reductase activities. Then, a significant correlation between JA and chlorophyll content was observed in broccoli flowers, and that was the important reason for broccoli postharvest yellowing (119). Besides, SA-mediated defense response was involved in litchi downy blight possibly via modulating fruit senescence (120). Other hormones, such as auxins, cytokinins (CK) or GAs, are usually at very low contents and attributed to the anti-senescence properties as well (74, 121, 122).
Many studies have confirmed the role of melatonin in modulating hormone levels during fruits and vegetables postharvest period (Figure 1) and (123). Melatonin can significantly delay fruit and vegetables senescence through inhibiting ET and ABA accumulation. For example, exogenous application of melatonin inhibited the expression of ACSs and ACOs genes, and reduced ethylene production to delay the banana and tomato fruits color through (124, 125). It significantly down-regulated the expression of ET synthetase genes (PcACS and PcACO), reduced ethylene production and rates of respiration, then thereby delaying senescence in pear fruit (126). Correspondingly, melatonin also down-regulated the expression of ET transcription factors (AdERF4, AdERF74, and AdERF75), and inhibited the ET release in kiwifruit during the storage period (127). Interestingly, research studies have showed that exogenous application of melatonin repressed the expression of BrABF1, BrABF4, BrABI5 (128). They binded to the promoters of ABA biosynthetic genes (BrNCED, BrABA2, and BrAAO) and chlorophyll catabolic genes, and regulated the expression levels of above genes, thus resulting in a low endogenous ABA level (128). Therefore, melatonin regulated the inhibition of Chinese flowering cabbage senescence by the suppression of ABFs-modulated ABA synthesis and chlorophyll degradation (128). Furthermore, exogenous application of melatonin reduced both ET and ABA contents to modulate the softening through inhibiting the activities of ACS, ACO, and 9-cis-epoxycarotenoid dioxygenase (NCED) in “Guifei” mango fruit (73). Additionally, exogenous application of melatonin induced the expression of JA synthesis genes (VaLOX, VaAOS, and VaAOC), and promoted JA accumulation (129). Hence, melatonin modulated the jasmonic acid signaling pathway to enhance the postharvest disease resistance of blueberries fruit (129). Similarly, generation of SA was also promoted by exogenous application of melatonin in tomato. Afterwards, the increase of activities of chitinase (CHI) and β-1,3-glucanase (GLU) inhibited tomato gray mold development, which caused by B. cinerea (130). Besides, after melatonin treatment for 4 days, GA1 had a sharp increase, and no differences were observed in the content of GA3, GA4, and GA7 in Angeleno plums during postharvest decay (70). Furthermore, it was also suggested that WRKY, MYB, ERF, ARF and bHLH3 transcription factors were mainly involved in auxin and ethylene signalings in postharvest banana fruit peel (131, 132). These transcription factors were also beneficial to maintain redox homeostasis (133). Some others, such as auxin and mitogen-activated protein kinase (MAPK) signaling pathway, might be involved in melatonin-regulated fruits and vegetables postharvest preservation and/or disease resistance during the storage period. In summary, an appropriate amount of melatonin can prolong fruits and vegetables senescence shelf life by regulating the release of ET, ABA, SA, and etc. Additionally, more genetic evidence needs to be explored in future study.
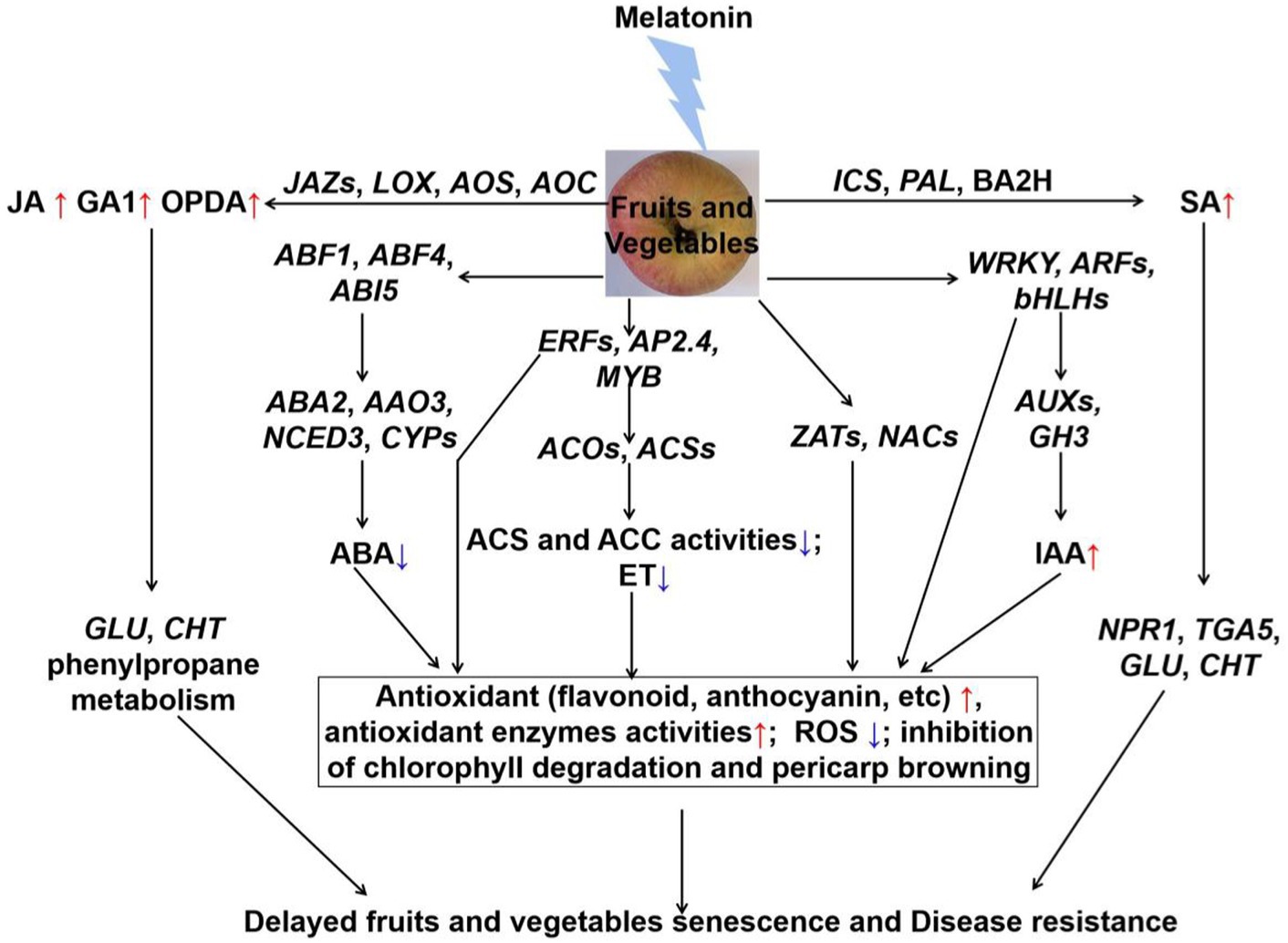
Figure 1. The crosstalk between melatonin and hormones of fruits and vegetables during the postharvest period. Exogenous application of melatonin reduced the ABA and ET content, and increased IAA content through regulating the related transcription factors and synthetic genes. Then, the significant antioxidants and antioxidant enzymes activities were induced to reduce the ROS accumulation, and chlorophyll degradation and pericarp browning were also inhibited. Besides, exogenous application of melatonin induced the JA and SA signaling pathways, and activated the proteins in the defense system to enhance the disease resistance in fruits and vegetables during storage. Jasmonic acid (JA), gibberellin 1 (GA1), 12-oxo-phytodienoic acid (OPDA), jasmonate ZIM-domain (JAZ), phenylalanine ammonia lyase (PAL), benzoic acid 2-hydroxylase (BA2H), lipoxygenase (LOX), allene oxide synthase (AOS), allene oxide cyclase (AOC), isochorismate synthase (ICS), salicylate (SA), non-expresser of pathogenesis-related genes 1 (NPR1), basic/leucine zipper-type transcription factor (TAG5), β − 1,3-glucanase (GLU), chitinase (CHT), abscisic acid (ABA), ABRE-binding factor (ABF), ABA-insensitive (ABI); 9-cis-epoxycarotenoid dioxygenase (NCED), aldehyde oxidase (AAO), ethylene-response factor (ERF), adipocyte protein (AP), v-myb avian myeloblastosis viral oncogene homolog (MYB), zinc finger protein (ZAT), NAM/ATAF/CUC (NAC), basic/helix–loop–helix (bHLH), 1-aminocyclopropane-1-carboxylic acid (ACC), ACC oxidase (ACO), ACC synthase (ACS), ethylene (ET), auxin response factor (ARF), indole-3-acetic acid-amido synthetase (GH3), indole-3-acetic acid (IAA).
The crosstalk between melatonin and signal molecules (NO, H2S, and ROS) in the postharvest protection of fruits and vegetables during storage period
Numerous studies showed that signal molecules, such as ROS, NO, and H2S, play key roles in resistances to biotic and abiotic damages in plants (134–139). Recent studies have shown that there are interactions between melatonin (applied exogenously) and the signalig molecules (37, 40–42, 126, 130, 140). For example, our previous studies revealed that H2O2 signaling was required for melatonin-promoted root growth and melatonin-improved salinity tolerance in alfalfa and Arabidopsis, respectively (37, 38). NO signaling was also involved in melatonin-regulated salinity tolerance in Brassica napus L. and sunflower seedlings (140, 141). Furtherly, melatonin induced H2S generation through increasing L-/D-cysteine desulfhydrase (LCD/DCD) activity. Similarly, it also stimulated NO generation. However, the H2S and NO induced by melatonin were inhibited by H2S scavenger (hypotaurine, HT) and NO scavenger (2-(4-carboxyphenyl)-4,4,5,5-tetramethylimidazoline-1-oxyl-3-oxide, cPTIO), respectively. Therefore, the H2S and NO jointly were participated in the melatonin-enhanced salinity tolerance in cucumber (34). In fact, the complex regulatory function of melatonin and its crosstalk with H2O2, NO and H2S is existed in many cases.
Interestingly, these signal molecules were also involved in exogenous melatonin-modulated fruits and vegetables postharvest protection, and thus improving their quality and yield (Figure 2) and (72, 142, 143). For instance, exogenous melatonin treatment rapidly elicited ROS burst. These ROS acted as signaling molecules to enhance SA accumulation and improve the expression of related defense genes in cherry tomato fruit during the storage (94). In litchi fruit, exogenous application of melatonin activated the NR and NOS activities and triggered NO accumulation (142). Endogenous NO mediated the melatonin-enhanced cold tolerance via regulation of redox status (142). Similarly, exogenous melatonin increased NOS activity, and induced endogenous NO production to maintain normal mitochondrial function in lotus seeds (144). Besides, it also induced NOS gene expression and enzyme activity to keep safe membrane integrity in tomato fruit (145). Furthermore, H2S has been reported to regulate the process by delaying senescence (146). However, more studies should be investigated on the crosstalks among melatonin, NO, and H2S in the postharvest preservation of fruits and vegetables using pharmacological, genetic, and proteomic approaches.
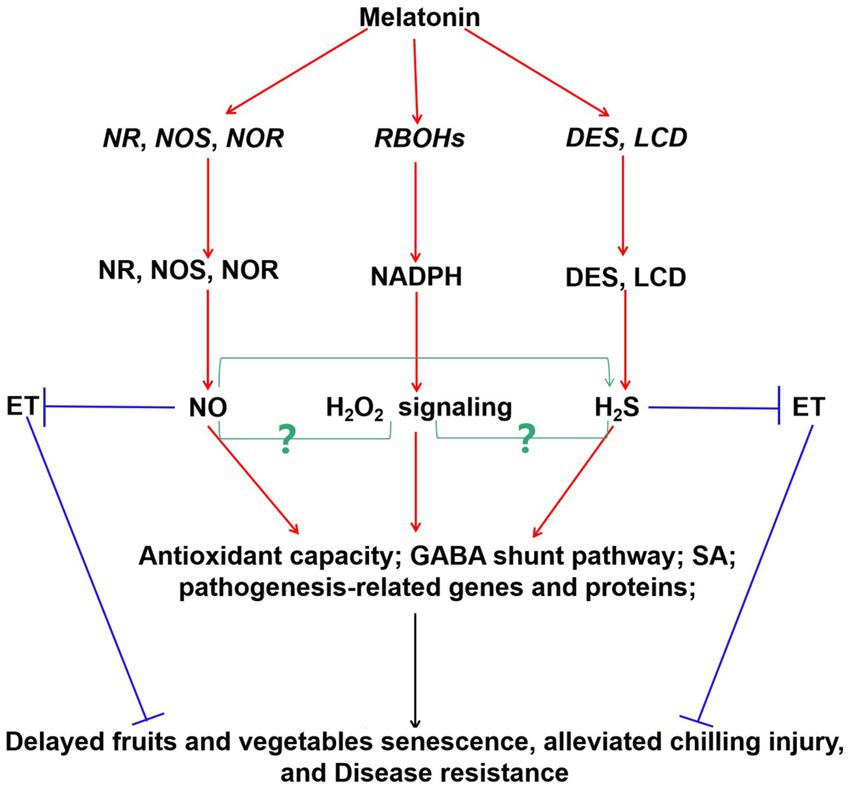
Figure 2. Probable integrative model of melatonin and signaling molecules (H2O2, NO, and H2S) in postharvest protection of fruits and vegetables during storage period. Increasing evidences showed that melatonin enhanced the expression of RBOHs, NR, NOS, NOR, DES, and LCD genes, and induced H2O2, NO, and H2S generation, thereby activating the signaling pathways in fruits and vegetables. Besides, hormones were also involved in these pathways to regulate fruits and vegetables quality. Interaction between NO and H2S was also suggested. The relationships between the H2O2 signaling and NO/H2S in postharvest protection of fruits and vegetables are still largely unknown (green arrow, yet largely unknown). Red arrow, induced; blue arrow, inhibited. Respiratory burst oxidase homologs (RBOHs), nitrate reductase (NR), nitric oxide synthase (NOS), desulfhydrase, (DES), L-cysteine desulfhydrase (LCD), nitric oxide (NO;), hydrogen peroxide (H2O2), hydrogen sulfide (H2S), ethylene (ET), salicylic acid (SA), γ-aminobutyric acid (GABA).
Crosstalk between the RBOH-regulated ROS signaling and melatonin in the postharvest protection of fruits and vegetables during storage period
Previous studies suggested that melatonin is a potent free radical scavenger, and reacts with ROS via the addition of a hydroxyl group (-OH) in position 2, 4, or 6 to form a family of molecules (147). Among the hydroxymelatonin metabolites, 2-hydroxymelatonin (2-OHMel) and 4-hydroxymelatonin (4-OHMel) were found in 24 plant species and predicted to have the antioxidant protection (147–149). For example, 4-OHMel reacted with ROO• about 200 times faster than trolox. Furthermore, ROS act as key signaling molecules at low concentrations in regulating plant biotic and abiotic stress (150, 151). Recent studies have shed new light on the interactions of melatonin and ROS in higher plants development and growth (37, 38, 41). For example, Bian et al. (111) identified that melatonin acted as upstream signaling of ROS to facilitate lateral root development. Besides, the phytomelatonin receptor (PMTR) sensed and binded with melatonin to release G-protein α (Gα), and activated Ca2+ signaling. Afterwards, the Ca2+ signaling activated H2O2 production, while H2O2 worked with Ca2+ signaling to induce the expression of cell cycle regulatory genes, and thereby promoting the lateral root development.
Previous reviews summarized the pathways of ROS generation in plant organs, including cell membrane, peroxisome, mitochondria, chloroplast, apoplast, and etc (150, 151). Among these, respiratory burst oxidase homolog (RBOH) proteins localize on plasma membrane, and encode the NADPH oxidases, which associate with the signal transduction (152). There are several RBOHs genes encoding NADPH oxidase in various plants (150, 151). Recently, many studies have revealed the vital roles of RBOH-regulated ROS signaling in melatonin-enhanced plant abiotic stress tolerance (41). Furthermore, it is necessary to balance intracellular ROS homeostasis to maintain to the quality of postharvest fruits and vegetables. Recently, the functions of H2O2 signaling in melatonin-mediated fruits and vegetables postharvest protection were also preliminarily studied (Figure 2) and (72, 130, 132, 153, 154). For example, O2·− and H2O2 generation of cherry tomato fruit increased to a maximum by exogenous melatonin treatment at 12 h and 36 h, respectively, and then decreased during the storage period (130). Exogenous melatonin treatment significantly up-regulated the expression of respiratory burst oxidase homolog protein B (RbohB) gene, which accelerated the response signaling in banana peel in banana during postharvest storage period (132). Similarly, melatonin treatment also up-regulated the RBOH1 expression in tomato, however, it was significantly attenuated by treatments of diphenyleneiodonium (DPI, an NADPH oxidase inhibitor) and dimethylthiourea (DMTU, a ROS scavenger) (153). Exogenous melatonin elevated O2·− and H2O2 accumulation by upregulating the SlNOX expression and NOX activity for the first 36 h in cherry tomato fruit during storage (94). These results were further confirmed by the transcriptome analysis in cherry tomato fruit (155). Besides, the positive crosstalks between melatonin and H2O2 have also been observed in apple and strawberry fruits against Diplocarpon mali infection and decay, respectively (51, 156). Moreover, SA signaling acted as the downstream pathway of the crosstalk between melatonin and H2O2 signaling to modulate the postharvest protection of fruits and vegetables during storage period (94, 156). Therefore, ROS generation-induced transiently by melatonin serve as the key signal in fruits and vegetables, especially in resistance to various diseases. However, it is important to further clarify the roles of this crosstalk on the quality and extending storage times in diverse fruits and vegetables species.
Conclusion and perspectives
Melatonin is ubiquitous in fruits and vegetables. This reviews describes the changes of melatonin content and synthesis sites in fruits and vegetables during the postharvest period. Exogenous melatonin can increase endogenous melatonin accumulation, alleviate the weight loss, fruit firmness decrease and discoloration, reduce the decay incidence, decay and disease index, and improve the quality of fruits and vegetables. In addition, it increases GSH, AsA, DHA, anthocyanins, carotenoids, and total flavonoid and phenols contents, and decreases MDA, H2O2, and O2•– contents. It has also been noted that melatonin enhances the CAT, SOD, APX, GR, GPX, DHAR, and MDHAR activities to improve the antioxidant capacity. Application of exogenous melatonin increases proline content and decreases the membrane lipid peroxidation to protect cell membrane integrity in fruits and vegetables during the cold storage. Furtherly, exogenous melatonin regulates hormones, such as ethylene, salicylic acid, and abscisic acid, to delay postharvest senescence and protect fruits and vegetables aganist bacterial invasion. However, the effective concentrations of melatonin are different for postharvest protection of different fruits and vegetables species. Therefore, it is important to use the appropriate melatonin concentrations to prolong fruits and vegetables postharvest shelf life.
ROS signaling during fruit and vegetable ripening has been extensively studied (147). Recently, several studies revealled that ROS signaling is involved in melatonin-modulated fruits and vegetables post-harvest preservation. In particular, the vital role of RBOHs-regulated H2O2 generation during these processes are shown. However, there are still many questions that should be characterized to understand the crosstalk of melatonin and ROS. For example, it is necessary to focus more attention on the signaling role of ROS produced by PAO in melatonin-modulated fruits and vegetables post-harvest preservation in future studies. Since the transmembrane receptor of melatonin (PMTR1/CAND2) were found in plants, researches focus on the mechanisms that the interaction between PMTR1/CAND2 and Gα subunits acts on the expression of the RBOHs in plant responses to abiotic stress (56, 71, 157). In this review, it is urgent to deeply study whether or how Gα directly regulates the crosstalk between melatonin and reactive oxygen species in fruits and vegetables post-harvest preservation.
Author contributions
NL, KZ, QY, and QG: writing—original draft preparation. XZ: writing—provided deeply discussion and sorted out the references. MM and ZC: writing—review and editing. All authors have read and agreed to the published version of the manuscript.
Funding
This research was mainly supported by the Natural Science Foundation of Anhui Province (No. 2008085MC101), Anhui Province Innovation Team of Authentic Medicinal Materials Development and High Value Utilization (No. 2022AH010080), Talent Research Fund Project of Hefei University (No. 18-19RC13), Anhui Provincial First-class Professional Construction (Biotechnology and Food Quality and Safety), and the Natural Science Foundation of Anhui Educational Committee (No. KJ2017ZD36).
Conflict of interest
The authors declare that the research was conducted in the absence of any commercial or financial relationships that could be construed as a potential conflict of interest.
Publisher’s note
All claims expressed in this article are solely those of the authors and do not necessarily represent those of their affiliated organizations, or those of the publisher, the editors and the reviewers. Any product that may be evaluated in this article, or claim that may be made by its manufacturer, is not guaranteed or endorsed by the publisher.
References
1. Alegbeleye, O, Odeyemi, OA, Strateva, M, and Stratev, D. Microbial spoilage of vegetables, fruits and cereals. Appl Food Res. (2022) 2:100122. doi: 10.1016/j.afres.2022.100122
2. Cömert, ED, Mogol, BA, and Gökmen, V. Relationship between color and antioxidant capacity of fruits and vegetables. Curr Res Food Sci. (2019) 2:1–10. doi: 10.1016/j.crfs.2019.11.001
3. Jiang, Q, Zhang, M, and Xu, B. Application of ultrasonic technology in postharvested fruits and vegetables storage: a review. Ultrason Sonochem. (2020) 69:105261. doi: 10.1016/j.ultsonch.2020.105261
4. Maheshwari, S, Kumar, V, Bhadauria, G, and Mishra, A. Immunomodulatory potential of phytochemicals and other bioactive compounds of fruits: a review. Food Front. (2022) 3:221–38. doi: 10.1002/fft2.129
5. Boeing, H, Bechthold, A, Bub, A, Ellinger, S, Haller, D, Kroke, A, et al. Critical review: vegetables and fruit in the prevention of chronic diseases. Eur J Nutr. (2012) 51:637–63. doi: 10.1007/s00394-012-0380-y
6. Jantan, I, Haque, MA, Arshad, L, Harikrishnan, H, Septama, AW, and Mohamed-Hussein, ZA. Dietary polyphenols suppress chronic inflammation by modulation of multiple inflammation-associated cell signaling pathways. J Nutr Biochem. (2021) 93:108634. doi: 10.1016/j.jnutbio.2021.108634
7. Barbhuiya, RI, Tinoco, NN, Ramalingam, S, Elsayed, A, Subramanian, J, Routray, W, et al. A review of nanoparticle synthesis and application in the suppression of diseases in fruits and vegetables. Crit Rev Food Sci. (2022) 23:2511. doi: 10.1080/10408398.2022.2142511
8. Salehi, F. Recent applications and potential of infrared dryer systems for drying various agricultural products: a review. Int J Fruit Sci. (2020) 20:586–602. doi: 10.1080/15538362.2019.1616243
9. Oliveira, M, Abadias, M, Usall, J, Torres, R, Teixidó, N, and Viñas, I. Application of modified atmosphere packaging as a safety approach to fresh-cut fruits and vegetables–a review. Trends Food Sci Tech. (2015) 46:13–26. doi: 10.1016/j.tifs.2015.07.017
10. Prajapati, U, Asrey, R, Varghese, E, Singh, AK, and Singh, MP. Effects of postharvest ultraviolet-C treatment on shelf-life and quality of bitter gourd fruit during storage. Food Packaging Shelf. (2021) 28:100665. doi: 10.1016/j.fpsl.2021.100665
11. Qi, T, Ji, J, Zhang, X, Liu, L, Xu, X, Ma, K, et al. Research progress of cold chain transport technology for storage fruits and vegetables. J Energy Storage. (2022) 56:105958. doi: 10.1016/j.est.2022.105958
12. Tarangini, K, Kavi, P, and Rao, KJ. Application of sericin-based edible coating material for postharvest shelf-life extension and preservation of tomatoes. eFood. (2022) 3:e36. doi: 10.1002/efd2.36
13. Wu, X, Wu, H, Yu, M, Ma, R, and Yu, Z. Effect of combined hypobaric and cold storage on defense-related enzymes in postharvest peach fruit during ripening. Acta Physiol Plant. (2022) 44:1–8. doi: 10.1007/s11738-022-03430-6
14. Valencia-Chamorro, SA, Palou, L, Del Río, MA, and Pérez-Gago, MB. Antimicrobial edible films and coatings for fresh and minimally processed fruits and vegetables: a review. Crit Rev Food Sci Nutr. (2011) 51:872–900. doi: 10.1080/10408398.2010.485705
15. Chen, M, Guo, H, Chen, S, Li, T, Li, M, Rashid, A, et al. Methyl jasmonate promotes phospholipid remodeling and jasmonic acid signaling to alleviate chilling injury in peach fruit. J Agric Food Chem. (2019) 67:9958–66. doi: 10.1021/acs.jafc.9b03853
16. Kong, J, Zhang, Y, Ju, J, Xie, Y, Guo, Y, Cheng, Y, et al. Antifungal effects of thymol and salicylic acid on cell membrane and mitochondria of Rhizopus stolonifer and their application in postharvest preservation of tomatoes. Food Chem. (2019) 285:380–8. doi: 10.1016/j.foodchem.2019.01.099
17. Madebo, MP, Ayalew, Y, Zheng, Y, and Jin, P. Nitric oxide and its donor sodium-nitroprusside regulation of the postharvest quality and oxidative stress on fruits: a systematic review and meta-analysis. Food Rev Int. (2022) 29:2995. doi: 10.1080/87559129.2022.2122995
18. Meitha, K, Pramesti, Y, and Suhandono, S. Reactive oxygen species and antioxidants in postharvest vegetables and fruits. Int J Food Sci. (2020) 2020:7778. doi: 10.1155/2020/8817778
19. Wang, F, Zhang, X, Yang, Q, and Zhao, Q. Exogenous melatonin delays postharvest fruit senescence and maintains the quality of sweet cherries. Food Chem. (2019) 301:125311. doi: 10.1016/j.foodchem.2019.125311
20. Wang, W, Ni, ZJ, Thakur, K, Cao, SQ, and Wei, ZJ. Recent update on the mechanism of hydrogen sulfide improving the preservation of postharvest fruits and vegetables. Curr Opin Food Sci. (2022c) 47:100906. doi: 10.1016/j.cofs.2022.100906
21. Wei, H, Seidi, F, Zhang, T, Jin, Y, and Xiao, H. Ethylene scavengers for the preservation of fruits and vegetables: a review. Food Chem. (2021) 337:127750. doi: 10.1016/j.foodchem.2020.127750
22. Cajochen, C, Kräuchi, K, and Wirz-Justice, A. Role of melatonin in the regulation of human circadian rhythms and sleep. J Neuroendocrinol. (2003) 15:432–7. doi: 10.1046/j.1365-2826.2003.00989.x
23. Hardeland, R. Aging, melatonin, and the pro- and anti-inflammatory networks. Int J Mol Sci. (2019) 20:1223. doi: 10.3390/ijms20051223
24. Zhao, D, Yu, Y, Shen, Y, Liu, Q, Zhao, Z, Sharma, R, et al. Melatonin synthesis and function: evolutionary history in animals and plants. Front Endocrinol. (2019) 10:249. doi: 10.3389/fendo.2019.00249
25. Nabavi, SM, Nabavi, SF, Sureda, A, Xiao, J, Dehpour, AR, Shirooie, S, et al. Anti-inflammatory effects of melatonin: a mechanistic review. Crit Rev Food Sci Nutr. (2019) 59:S4–S16. doi: 10.1080/10408398.2018.1487927
26. Dubbels, R, Reiter, RJ, Klenke, E, Goebel, A, Schnakenberg, E, Ehlers, C, et al. Melatonin in edible plants identified by radioimmunoassay and by high performance liquid chromatography-mass spectrometry. J Pineal Res. (1995) 18:28–31. doi: 10.1111/j.1600-079X.1995.tb00136.x
27. Hattori, A, Migitaka, H, Iigo, M, Itoh, M, Yamamoto, K, Ohtani-Kaneko, R, et al. Identification of melatonin in plants and its effects on plasma melatonin levels and binding to melatonin receptors in vertebrates. Biochem Mol Biol Int. (1995) 35:627–34.
28. Altaf, MA, Sharma, N, Singh, J, Samota, MK, Sankhyan, P, Singh, B, et al. Mechanistic insights on melatonin-mediated plant growth regulation and hormonal cross-talk process in solanaceous vegetables. Sci Hortic. (2023) 308:111570. doi: 10.1016/j.scienta.2022.111570
29. Byeon, Y, Lee, HY, and Back, K. Cloning and characterization of the serotoninn-acetyltransferase-2 gene (SNAT2) in rice (Oryza sativa). J Pineal Res. (2016) 61:198–207. doi: 10.1111/jpi.12339
30. Iqbal, N, Fatma, M, Gautam, H, Umar, S, and Khan, NA. The crosstalk of melatonin and hydrogen sulfide determines photosynthetic performance by regulation of carbohydrate metabolism in wheat under heat stress. Plan Theory. (2021) 10:1778. doi: 10.3390/plants10091778
31. Kaya, C, Ugurlar, F, Ashraf, M, Alyemeni, MN, Bajguz, A, and Ahmad, P. The involvement of hydrogen sulfide in melatonin-induced tolerance to arsenic toxicity in pepper (Capsicum annuum L.) plants by regulating sequestration and subcellular distribution of arsenic, and antioxidant defense system. Chemosphere. (2022) 309:136678. doi: 10.1016/j.chemosphere.2022.136678
32. Li, MQ, Hasan, MK, Li, CX, Ahammed, GJ, Xia, XJ, Shi, K, et al. Melatonin mediates selenium-induced tolerance to cadmium stress in tomato plants. J Pineal Res. (2016b) 61:291–302. doi: 10.1111/jpi.12346
33. Meng, JF, Xu, TF, Wang, ZZ, Fang, YL, Xi, ZM, and Zhang, ZW. The ameliorative effects of exogenous melatonin on grape cuttings under water-deficient stress: antioxidant metabolites, leaf anatomy, and chloroplast morphology. J Pineal Res. (2014) 57:200–12. doi: 10.1111/jpi.12159
34. Sun, Y, Ma, C, Kang, X, Zhang, L, Wang, J, Zheng, S, et al. Hydrogen sulfide and nitric oxide are involved in melatonin-induced salt tolerance in cucumber. Plant Physiol Biochem. (2021c) 167:101–12. doi: 10.1016/j.plaphy.2021.07.023
35. Tan, K, Zheng, J, Liu, C, Liu, X, Liu, X, Gao, T, et al. Heterologous expression of the melatonin-related gene HIOMT improves salt tolerance in Malus domestica. Int J Mol Sci. (2021a) 22:12425. doi: 10.3390/ijms222212425
36. Wu, S, Wang, Y, Zhang, J, Gong, X, and Wang, Y. Exogenous melatonin improves physiological characteristics and promotes growth of strawberry seedlings under cadmium stress. Hortic Plant J. (2021) 7:13–22. doi: 10.1016/j.hpj.2020.06.002
37. Chen, Z, Gu, Q, Yu, X, Huang, L, Xu, S, Wang, R, et al. Hydrogen peroxide acts downstream of melatonin to induce lateral root formation. Ann Bot. (2018) 121:1127–36. doi: 10.1093/aob/mcx207
38. Chen, Z, Xie, Y, Gu, Q, Zhao, G, Zhang, Y, Cui, W, et al. The AtrbohF-dependent regulation of ROS signaling is required for melatonin-induced salinity tolerance in Arabidopsis. Free Radic Biol Med. (2017) 108:465–77. doi: 10.1016/j.freeradbiomed.2017.04.009
39. Gu, Q, Chen, Z, Yu, X, Cui, W, Pan, J, Zhao, G, et al. Melatonin confers plant tolerance against cadmium stress via the decrease of cadmium accumulation and reestablishment of microRNA-mediated redox homeostasis. Plant Sci. (2017) 261:28–37. doi: 10.1016/j.plantsci.2017.05.001
40. Gu, Q, Wang, C, Xiao, Q, Chen, Z, and Han, Y. Melatonin confers plant cadmium tolerance: An update. Int J Mol Sci. (2021) 22:11704. doi: 10.3390/ijms222111704
41. Gu, Q, Xiao, Q, Chen, Z, and Han, Y. Crosstalk between melatonin and reactive oxygen species in plant abiotic stress responses: An update. Int J Mol Sci. (2022) 23:5666. doi: 10.3390/ijms23105666
42. Su, J, Yang, X, Shao, Y, Chen, Z, and Shen, W. Molecular hydrogen-induced salinity tolerance requires melatonin signaling in Arabidopsis thaliana. Plant Cell Environ. (2021) 44:476–90. doi: 10.1111/pce.13926
43. Cano, A, Giraldo-Acosta, M, García-Sánchez, S, Hernández-Ruiz, J, and Arnao, M. Effect of melatonin in broccoli postharvest and possible melatonin ingestion level. Plan Theory. (2022) 11:2000. doi: 10.3390/plants11152000
44. Wang, L, Luo, Z, Ban, Z, Jiang, N, Yang, M, and Li, L. Role of exogenous melatonin involved in phenolic metabolism of Zizyphus jujuba fruit. Food Chem. (2021c) 341:128268. doi: 10.1016/j.foodchem.2020.128268
45. Xia, H, Shen, Y, Shen, T, Wang, X, Zhang, X, Hu, P, et al. Melatonin accumulation in sweet cherry and its influence on fruit quality and antioxidant properties. Molecules. (2020) 25:753. doi: 10.3390/molecules25030753
46. Zheng, H, Liu, W, Liu, S, Liu, C, and Zheng, L. Effects of melatonin treatment on the enzymatic browning and nutritional quality of fresh-cut pear fruit. Food Chem. (2019) 299:125116. doi: 10.1016/j.foodchem.2019.125116
47. Xu, L, Yue, Q, Bian, F, Sun, H, Zhai, H, and Yao, Y. Melatonin enhances phenolics accumulation partially via ethylene signaling and resulted in high antioxidant capacity in hrape berries. Front Plant Sci. (2017) 8:1426. doi: 10.3389/fpls.2017.01426
48. Mittler, R, Zandalinas, SI, Fichman, Y, and Van Breusegem, F. Reactive oxygen species signaling in plant stress responses. Nat Rev Mol Cell Bio. (2022) 23:663–79. doi: 10.1038/s41580-022-00499-2
49. Choudhury, FK, Rivero, RM, Blumwald, E, and Mittler, R. Reactive oxygen species, abiotic stress and stress combination. Plant J. (2017) 90:856–67. doi: 10.1111/tpj.13299
51. Aghdam, MS, and Fard, JR. Melatonin treatment attenuates postharvest decay and maintains nutritional quality of strawberry fruits (Fragaria×anannasa cv. Selva) by enhancing GABA shunt activity. Food Chem. (2017) 221:1650–7. doi: 10.1016/j.foodchem.2016.10.123
52. Sun, H, Cao, X, Wang, X, Zhang, W, Li, W, Wang, X, et al. RBOH-dependent hydrogen peroxide signaling mediates melatonin-induced anthocyanin biosynthesis in red pear fruit. Plant Sci. (2021b) 313:111093. doi: 10.1016/j.plantsci.2021.111093
53. Fan, S, Li, Q, Feng, S, Lei, Q, Abbas, F, Yao, Y, et al. Melatonin maintains fruit quality and reduces anthracnose in postharvest papaya via enhancement of antioxidants and inhibition of pathogen development. Antioxidants. (2022a) 11:804. doi: 10.3390/antiox11050804
54. Fan, S, Xiong, T, Lei, Q, Tan, Q, Cai, J, Song, Z, et al. Melatonin treatment improves postharvest preservation and resistance of guava fruit (Psidium guajava L.). Foods. (2022b) 11:262. doi: 10.3390/foods11030262
55. Thole, V, Vain, P, Yang, RY, da Silva, J, Enfissi, EMA, Nogueira, M, et al. Analysis of tomato post-garvest properties: fruit color, shelf life, and fungal susceptibility. Curr Protoc Plant Biol. (2020) 5:e20108. doi: 10.1002/cppb.20108
56. Wang, L, Shen, X, Chen, X, Ouyang, Q, Tan, X, and Tao, N. Exogenous application of melatonin to green horn pepper fruit reduces chilling injury during postharvest cold storage by regulating enzymatic activities in the antioxidant system. Plan Theory. (2022a) 11:2367. doi: 10.3390/plants11182367
57. Wang, Z, Zhang, L, Duan, W, Li, W, Wang, Q, Li, J, et al. Melatonin maintained higher contents of unsaturated fatty acid and cell membrane structure integrity in banana peel and alleviated postharvest chilling injury. Food Chem. (2022e) 397:133836. doi: 10.1016/j.foodchem.2022.133836
58. Xie, J, Qin, Z, Pan, J, Li, J, Li, X, Khoo, HE, et al. Melatonin treatment improves postharvest quality and regulates reactive oxygen species metabolism in "Feizixiao" litchi based on principal component analysis. Front Plant Sci. (2022) 13:965345. doi: 10.3389/fpls.2022.965345
59. Yan, R, Li, S, Cheng, Y, Kebbeh, M, Huan, C, and Zheng, X. Melatonin treatment maintains the quality of cherry tomato by regulating endogenous melatonin and ascorbate-glutathione cycle during room temperature. J Food Biochem. (2022a) 46:e14285. doi: 10.1111/jfbc.14285
60. Chen, Y, Zhang, Y, Nawaz, G, Zhao, C, Li, Y, Dong, T, et al. Exogenous melatonin attenuates post-harvest decay by increasing antioxidant activity in wax apple (Syzygium samarangense). Front Plant Sci. (2020) 11:569779. doi: 10.3389/fpls.2020.569779
61. Liu, DK, Xu, CC, Guo, CX, and Zhang, XX. Sub-zero temperature preservation of fruits and vegetables: a review. J Food Eng. (2020a) 275:109881. doi: 10.1016/j.jfoodeng.2019.109881
62. Murmu, SB, and Mishra, HN. Selection of the best active modified atmosphere packaging with ethylene and moisture scavengers to maintain quality of guava during low-temperature storage. Food Chem. (2018) 253:55–62. doi: 10.1016/j.foodchem.2018.01.134
63. Singh, SP, and Pal, RK. Controlled atmosphere storage of guava (Psidium guajava L.) fruit. Postharvest Biol. Tec. (2008) 47:296–306. doi: 10.1016/j.postharvbio.2007.08.009
64. Hu, W, Tie, W, Ou, W, Yan, Y, Kong, H, Zuo, J, et al. Crosstalk between calcium and melatonin affects postharvest physiological deterioration and quality loss in cassava. Postharvest Biol. Tec. (2018) 140:42–9. doi: 10.1016/j.postharvbio.2018.02.007
65. Liu, C, Zheng, H, Sheng, K, Liu, W, and Zheng, L. Effects of melatonin treatment on the postharvest quality of strawberry fruit. Postharvest Biol. Tec. (2018) 139:47–55. doi: 10.1016/j.postharvbio.2018.01.016
66. Wang, L, Luo, Z, Yang, M, Li, D, Qi, M, Xu, Y, et al. Role of exogenous melatonin in table grapes: first evidence on contribution to the phenolics-oriented response. Food Chem. (2020) 329:127155. doi: 10.1016/j.foodchem.2020.127155
67. Xia, H, Shen, Y, Deng, H, Wang, J, Lin, L, Deng, Q, et al. Melatonin application improves berry coloration, sucrose synthesis, and nutrient absorption in “summer black” grape. Food Chem. (2021) 356:129713. doi: 10.1016/j.foodchem.2021.129713
68. Zheng, S, Zhu, Y, Liu, C, Zhang, S, Yu, M, Xiang, Z, et al. Molecular mechanisms underlying the biosynthesis of melatonin and its isomer in mulberry. Front Plant Sci. (2021) 12:708752. doi: 10.3389/fpls.2021.708752
69. Bhardwaj, R, Pareek, S, Domínguez-Avila, JA, Gonzalez-Aguilar, GA, Valero, D, and Serrano, M. An exogenous pre-storage melatonin alleviates chilling injury in some mango fruit cultivars, by acting on the enzymatic and non-enzymatic antioxidant system. Antioxidants. (2022b) 11:384. doi: 10.3390/antiox11020384
70. Arabia, A, Munné-Bosch, S, and Muñoz, P. Melatonin triggers tissue-specific changes in anthocyanin and hormonal contents during postharvest decay of Angeleno plums. Plant Sci. (2022) 320:111287. doi: 10.1016/j.plantsci.2022.111287
71. Wang, N, Fang, H, Yang, Q, Liu, Z, Feng, H, and Ji, S. Exogenous melatonin alleviated leaf yellowing via inhibiting respiration and ethylene biosynthesis during shelf life in pakchoi. Plan Theory. (2022b) 11:2102. doi: 10.3390/plants11162102
72. Li, S, Huan, C, Liu, Y, Zheng, X, and Bi, Y. Melatonin induces improved protection against Botrytis cinerea in cherry tomato fruit by activating salicylic acid signaling pathway. Sci Hortic. (2022b) 304:111299. doi: 10.1016/j.scienta.2022.111299
73. Liu, S, Huang, H, Huber, DJ, Pan, Y, Shi, X, and Zhang, Z. Delay of ripening and softening in ‘Guifei’ mango fruit by postharvest application of melatonin. Postharvest Biol. Tec. (2022b) 163:111136. doi: 10.1016/j.postharvbio.2020.111136
74. do Amarante, CV, Silveira, JPG, Steffens, CA, de Freitas, ST, Mitcham, EJ, and Miqueloto, A. Post-bloom and preharvest treatment of ‘Braeburn’ apple trees with prohexadione-calcium and GA4+7 affects vegetative growth and postharvest incidence of calcium-related physiological disorders and decay in the fruit. Sci Hortic. (2020) 261:108919. doi: 10.1016/j.scienta.2019.108919
75. Lindo-García, V, Muñoz, P, Larrigaudière, C, Munné-Bosch, S, and Giné-Bordonaba, J. Interplay between hormones and assimilates during pear development and ripening and its relationship with the fruit postharvest behavior. Plant Sci. (2020) 291:110339. doi: 10.1016/j.plantsci.2019.110339
76. Sun, C, Liu, L, Wang, L, Li, B, Jin, C, and Lin, X. Melatonin: a master regulator of plant development and stress responses. J Integr Plant Biol. (2021a) 63:126–45. doi: 10.1111/jipb.12993
77. Wang, SY, Shi, XC, Liu, FQ, and Laborda, P. Effects of exogenous methyl jasmonate on quality and preservation of postharvest fruits: a review. Food Chem. (2021d) 353:129482. doi: 10.1016/j.foodchem.2021.129482
78. Carrión-Antolí, A, Martínez-Romero, D, Guillén, F, Zapata, PJ, Serrano, M, and Valero, D. Melatonin pre-harvest treatments leads to maintenance of sweet cherry quality during storage by increasing antioxidant systems. Front Plant Sci. (2022) 13:863467. doi: 10.3389/fpls.2022.863467
79. Tan, XL, Zhao, YT, Shan, W, Kuang, JF, Lu, WJ, Su, XG, et al. Melatonin delays leaf senescence of postharvest Chinese flowering cabbage through ROS homeostasis. Food Res Int. (2020) 138:109790. doi: 10.1016/j.foodres.2020.109790
80. Zhang, Z, Wang, T, Liu, G, Hu, M, Yun, Z, Duan, X, et al. Inhibition of downy blight and enhancement of resistance in litchi fruit by postharvest application of melatonin. Food Chem. (2021b) 347:129009. doi: 10.1016/j.foodchem.2021.129009
81. Fan, Y, Li, C, Li, Y, Huang, R, Guo, M, Liu, J, et al. Postharvest melatonin dipping maintains quality of apples by mediating sucrose metabolism. Plant Physiol Biochem. (2022c) 174:43–50. doi: 10.1016/j.plaphy.2022.01.034
82. Tan, XL, Fan, ZQ, Zeng, ZX, Shan, W, Kuang, JF, Lu, WJ, et al. Exogenous melatonin maintains leaf quality of postharvest Chinese flowering cabbage by modulating respiratory metabolism and energy status. Postharvest Biol Tec. (2021b) 177:111524. doi: 10.1016/j.postharvbio.2021.111524
83. Verde, A, Míguez, JM, and Gallardo, M. Role of melatonin in apple fruit during growth and ripening: possible interaction with ethylene. Plan Theory. (2022) 11:688. doi: 10.3390/plants11050688
84. Liu, J, Liu, H, Wu, T, Zhai, R, Yang, C, Wang, Z, et al. Effects of melatonin treatment of postharvest pear fruit on aromatic volatile biosynthesis. Molecules. (2019a) 24:4233. doi: 10.3390/molecules24234233
85. Wei, S, Jiao, H, Wang, H, Ran, K, Dong, R, Dong, X, et al. The mechanism analysis of exogenous melatonin in limiting pear fruit aroma decrease under low temperature storage. PeerJ. (2022b) 10:e14166. doi: 10.7717/peerj.14166
86. Aghdam, MS, Luo, Z, Li, L, Jannatizadeh, A, Fard, JR, and Pirzad, F. Melatonin treatment maintains nutraceutical properties of pomegranate fruits during cold storage. Food Chem. (2020) 303:125385. doi: 10.1016/j.foodchem.2019.125385
87. Bhardwaj, R, Aghdam, MS, Arnao, MB, Brecht, JK, Fawole, OA, and Pareek, S. Melatonin alleviates chilling injury symptom development in mango fruit by maintaining intracellular energy and cell wall and membrane stability. Front Nutr. (2022a) 9:936932. doi: 10.3389/fnut.2022.936932
88. Cao, S, Shao, J, Shi, L, Xu, L, Shen, Z, Chen, W, et al. Melatonin increases chilling tolerance in postharvest peach fruit by alleviating oxidative damage. Sci Rep. (2018) 8:806. doi: 10.1038/s41598-018-19363-5
89. Chen, H, Lin, H, Jiang, X, Lin, M, and Fan, Z. Amelioration of chilling injury and enhancement of quality maintenance in cold-stored guava fruit by melatonin treatment. Food Chem. (2022) 14:100297. doi: 10.1016/j.fochx.2022.100297
90. Dong, B, Yao, Q, Zhu, D, Han, H, Tang, H, and Ding, X. Exogenous melatonin maintains quality of postharvest Rosa roxburghii fruit by modulating reactive oxygen species metabolism and energy status. Sci Hortic. (2022) 304:111346. doi: 10.1016/j.scienta.2022.111346
91. Dong, J, Kebbeh, M, Yan, R, Huan, C, Jiang, T, and Zheng, X. Melatonin treatment delays ripening in mangoes associated with maintaining the membrane integrity of fruit exocarp during postharvest. Plant Physiol Biochem. (2021) 169:22–8. doi: 10.1016/j.plaphy.2021.10.038
92. Gao, H, Lu, Z, Yang, Y, Wang, D, Yang, T, Cao, M, et al. Melatonin treatment reduces chilling injury in peach fruit through its regulation of membrane fatty acid contents and phenolic metabolism. Food Chem. (2018) 245:659–66. doi: 10.1016/j.foodchem.2017.10.008
93. Jiao, X, Deng, B, Zhang, L, Gao, Z, Feng, Z, and Wang, R. Melatonin and 1-methylcyclopropene improve the postharvest quality and antioxidant capacity of ‘Youhou’ sweet persimmons during cold storage. Int. J. Fruit Sci. (2022) 22:809–25. doi: 10.1080/15538362.2022.2134959
94. Li, Y, Zhang, L, Zhang, L, Nawaz, G, Zhao, C, Zhang, J, et al. Exogenous melatonin alleviates browning of fresh-cut sweetpotato by enhancing anti-oxidative process. Sci Hortic. (2022d) 297:110937. doi: 10.1016/j.scienta.2022.110937
95. Liu, G, Zhang, Y, Yun, Z, Hu, M, Liu, J, Jiang, Y, et al. Melatonin enhances cold tolerance by regulating energy and proline metabolism in litchi fruit. Foods. (2020b) 9:454. doi: 10.3390/foods9040454
96. Liu, Q, Xin, D, Xi, L, Gu, T, Jia, Z, Zhang, B, et al. Novel applications of exogenous melatonin on cold stress mitigation in postharvest cucumbers. J Agr Food Res. (2022a) 10:459. doi: 10.1016/j.jafr.2022.100459
97. Luo, T, Yin, F, Liao, L, Liu, Y, Guan, B, Wang, M, et al. Postharvest melatonin treatment inhibited longan (Dimocarpuslongan Lour.) pericarp browning by increasing ROS scavenging ability and protecting cytomembrane integrity. Food Sci Nutr. (2021) 9:4963–73. doi: 10.1002/fsn3.2448
98. Magri, A, and Petriccione, M. Melatonin treatment reduces qualitative decay and improves antioxidant system in high bush blueberry fruit during cold storage. J Sci Food Agric. (2022) 102:4229–37. doi: 10.1002/jsfa.11774
99. Magri, A, Cice, D, Capriolo, G, and Petriccione, M. Effects of ascorbic acid and melatonin treatments on antioxidant system in fresh-cut avocado fruits during cold storage. Food Bioprocess Tech. (2022) 15:2468–82. doi: 10.1007/s11947-022-02892-3
100. Medina-Santamarina, J, Serrano, M, Ruiz-Aracil, MC, Ilea, MIM, Martínez-Romero, D, and Guillén, F. A synergistic effect based on the combination of melatonin with 1-Methylcyclopropene as a new strategy to increase chilling tolerance and general quality in zucchini fruit. Foods. (2022) 11:2784. doi: 10.3390/foods11182784
101. Miranda, S, Vilches, P, Suazo, M, Pavez, L, García, K, Méndez, MA, et al. Melatonin triggers metabolic and gene expression changes leading to improved quality traits of two sweet cherry cultivars during cold storage. Food Chem. (2020) 319:126360. doi: 10.1016/j.foodchem.2020.126360
102. Mirshekari, A, Madani, B, Yahia, EM, Golding, JB, and Vand, SH. Postharvest melatonin treatment reduces chilling injury in sapota fruit. J Sci Food Agric. (2020) 100:1897–903. doi: 10.1002/jsfa.10198
103. Nasser, MA, El-Mogy, MM, Samaan, MS, Hassan, KM, El-Sayed, SM, Alsubeie, MS, et al. Postharvest exogenous melatonin treatment of table grape berry enhances quality and maintains bioactive compounds during refrigerated storage. Horticulturae. (2022) 8:860. doi: 10.3390/horticulturae8100860
104. Onik, JC, Wai, SC, Li, A, Lin, Q, Sun, Q, Wang, Z, et al. Melatonin treatment reduces ethylene production and maintains fruit quality in apple during postharvest storage. Food Chem. (2021) 337:127753. doi: 10.1016/j.foodchem.2020.127753
105. Song, L, Zhang, W, Li, Q, Jiang, Z, Wang, Y, Xuan, S, et al. Melatonin alleviates chilling injury and maintains postharvest quality by enhancing antioxidant capacity and inhibiting cell wall degradation in cold-stored eggplant fruit. Postharvest Biol. Tec. (2022) 194:112092. doi: 10.1016/j.postharvbio.2022.112092
106. Wang, Y, Zhang, J, Ma, Q, Zhang, X, Luo, X, and Deng, Q. Exogenous melatonin treatment on post-harvest jujube fruits maintains physicochemical qualities during extended cold storage. PeerJ. (2022d) 10:e14155. doi: 10.7717/peerj.14155
107. Wei, D, Yang, J, Xiang, Y, Meng, L, Pan, Y, and Zhang, Z. Attenuation of postharvest browning in rambutan fruit by melatonin is associated with inhibition of phenolics oxidation and reinforcement of antioxidative process. Front Nutr. (2022a) 9:905006. doi: 10.3389/fnut.2022.905006
108. Yang, B, Han, Y, Wu, W, Fang, X, Chen, H, and Gao, H. Impact of melatonin application on lignification in water bamboo shoot during storage. Food Chem X. (2022) 13:100254. doi: 10.1016/j.fochx.2022.100254
109. Zang, H, Ma, J, Wu, Z, Yuan, L, Lin, ZQ, Zhu, R, et al. Synergistic effect of melatonin and selenium improves resistance to postharvest gray mold disease of tomato fruit. Front Plant Sci. (2022) 13:903936. doi: 10.3389/fpls.2022.903936
110. Zhang, L, Yu, Y, Chang, L, Wang, X, and Zhang, S. Melatonin enhanced the disease resistance by regulating reactive oxygen species metabolism in postharvest jujube fruit. J Food Process Pres. (2022b) 46:e16363. doi: 10.1111/jfpp.16363
111. Bian, L, Wang, Y, Bai, H, Li, H, Zhang, C, Chen, J, et al. Melatonin-ROS signal module regulates plant lateral root development. Plant Signal Behav. (2021) 16:1901447. doi: 10.1080/15592324.2021.1901447
112. Xiang, W, Wang, HW, and Sun, DW. Phytohormones in postharvest storage of fruit and vegetables: mechanisms and applications. Crit Rev Food Sci Nutr. (2021) 61:2969–83. doi: 10.1080/10408398.2020.1864280
113. Yan, Y, Zhao, S, Ye, X, Tian, L, Shang, S, Tie, W, et al. Abscisic acid signaling in the regulation of postharvest physiological deterioration of sliced cassava tuberous roots. J Agric Food Chem. (2022b) 70:12830–40. doi: 10.1021/acs.jafc.2c05483
114. Zhang, H, Han, M, Xie, Y, Wang, M, and Cao, C. Application of ethylene-regulating packaging in post-harvest fruits and vegetables storage: a review. Packag Technol Sci. (2022a) 35:461–71. doi: 10.1002/pts.2644
115. An, JP, Yao, JF, Xu, RR, You, CX, Wang, XF, and Hao, YJ. Apple bZIP transcription factor MdbZIP44 regulates abscisic acid-promoted anthocyanin accumulation. Plant Cell Environ. (2018) 41:2678–92. doi: 10.1111/pce.13393
116. Hu, B, Li, J, Wang, D, Wang, H, Qin, Y, Hu, G, et al. Transcriptome profiling of Litchi chinensis pericarp in response to exogenous cytokinins and abscisic acid. Plant Growth Regul. (2017a) 84:437–50. doi: 10.1007/s10725-017-0351-7
117. Mou, W, Li, D, Luo, Z, Mao, L, and Ying, T. Transcriptomic analysis reveals possible inflfluences of ABA on secondary metabolism of pigments, flavonoids and antioxidants in tomato fruit during ripening. PLoS One. (2015) 10:e0129598. doi: 10.1371/journal.pone.0129598
118. Villalobos-Gonzalez, L, Pena-Neira, A, Ibanez, F, and Pastenes, C. Long-term effects of abscisic acid (ABA) on the grape berry phenylpropanoid pathway: gene expression and metabolite content. Plant Physiol Biochem. (2016) 105:213–23. doi: 10.1016/j.plaphy.2016.04.012
119. Fang, H, Luo, F, Li, P, Zhou, Q, Zhou, X, Wei, B, et al. Potential of jasmonic acid (JA) in accelerating postharvest yellowing of broccoli by promoting its chlorophyll degradation. Food Chem. (2020) 309:125737. doi: 10.1016/j.foodchem.2019.125737
120. Yin, C, Xie, L, Wu, Y, Qu, H, Yang, B, Gong, L, et al. Involvement of miRNAs-mediated senescence and salicylic acid defense in postharvest litchi downy blight. Food Chem. (2023) 404:134662. doi: 10.1016/j.foodchem.2022.134662
121. Figueroa, CR, Opazo, MC, Vera, P, Arriagada, O, Díaz, M, and Moya-León, MA. Effect of postharvest treatment of calcium and auxin on cell wall composition and expression of cell wall-modifying genes in the Chilean strawberry (Fragaria chiloensis) fruit. Food Chem. (2012) 132:2014–22. doi: 10.1016/j.foodchem.2011.12.041
122. Li, L, Li, D, Luo, Z, Huang, X, and Li, X. Proteomic response and quality maintenance in postharvest fruit of strawberry (Fragaria × ananassa) to exogenous cytokinin. Sci Rep. (2016a) 6:27094. doi: 10.1038/srep27094
123. Sati, H, Khandelwal, A, and Pareek, S. Effect of exogenous melatonin in fruit postharvest, crosstalk with hormones, and defense mechanism for oxidative stress management. Food Front. (2022) 22:180. doi: 10.1002/fft2.180
124. Hu, W, Yang, H, Tie, WW, Yan, Y, Ding, ZH, Liu, Y, et al. Natural variation in banana varieties highlights the role of melatonin in postharvest ripening and quality. J Agr Food Chem. (2017b) 65:9987–94. doi: 10.1021/acs.jafc.7b03354
125. Sun, Q, Liu, L, Zhang, L, Lv, H, He, Q, Guo, L, et al. Melatonin promotes carotenoid biosynthesis in an ethylene-dependent manner in tomato fruits. Plant Sci. (2020) 298:110580. doi: 10.1016/j.plantsci.2020.110580
126. Liu, J, Yang, J, Zhang, H, Cong, L, Zhai, R, Yang, C, et al. Melatonin inhibits ethylene synthesis via nitric oxide regulation to delay postharvest senescence in pears. J Agric Food Chem. (2019b) 67:2279–88. doi: 10.1021/acs.jafc.8b06580
127. Cheng, J, Zheng, A, Li, H, Huan, C, Jiang, T, Shen, S, et al. Effects of melatonin treatment on ethanol fermenation and ERF expression in kiwifruit cv Bruno during postharvest. Sci Hortic. (2022) 293:110696. doi: 10.1016/j.scienta.2021.110696
128. Tan, XL, Fan, ZQ, Kuang, JF, Lu, WJ, Reiter, RJ, Lakshmanan, P, et al. Melatonin delays leaf senescence of Chinese flowering cabbage by suppressing ABFs-mediated abscisic acid biosynthesis and chlorophyll degradation. J Pineal Res. (2019) 67:e12570. doi: 10.1111/jpi.12570
129. Qu, G, Wu, W, Ba, L, Ma, C, Ji, N, and Cao, S. Melatonin enhances the postharvest disease resistance of blueberries fruit by modulating the jasmonic acid signaling pathway and phenylpropanoid petabolites. Front Chem. (2022) 10:57581. doi: 10.3389/fchem.2022.957581
130. Li, S, Xu, Y, Bi, Y, Zhang, B, Shen, S, Jiang, T, et al. Melatonin treatment inhibits gray mold and induces disease resistance in cherry tomato fruit during postharvest. Postharvest Biol. Tec. (2019a) 157:110962. doi: 10.1016/j.postharvbio.2019.110962
131. Aghdam, MS, Mukherjee, S, Flores, FB, Arnao, MB, Luo, Z, and Corpas, FJ. Functions of melatonin during postharvest of horticultural crops. Plant Cell Physiol. (2023) 63:1764–86. doi: 10.1093/pcp/pcab175
132. Li, T, Wu, Q, Zhu, H, Zhou, Y, Jiang, Y, Gao, H, et al. Comparative transcriptomic and metabolic analysis reveals the effect of melatonin on delaying anthracnose incidence upon postharvest banana fruit peel. BMC Plant Biol. (2019b) 19:289. doi: 10.1186/s12870-019-1855-2
133. Zhang, Z, Liu, J, Huber, DJ, Qu, H, Yun, Z, Li, T, et al. Transcriptome, degradome and physiological analysis provide new insights into the mechanism of inhibition of litchi fruit senescence by melatonin. Plant Sci. (2021a) 308:110926. doi: 10.1016/j.plantsci.2021.110926
134. de Bont, L, Mu, X, Wei, B, and Han, Y. Abiotic stress-triggered oxidative challenges: where does H2S act? J Genet Genomics. (2022) 49:748–55. doi: 10.1016/j.jgg.2022.02.019
135. Gao, X, Wu, W, Chen, H, Niu, B, Han, Y, Fang, X, et al. Nitric oxide treatment delays quality deterioration and enzymatic browning of Agaricus bisporus via reactive oxygen metabolism regulation. Food Front. (2023) 12:212. doi: 10.1002/fft2.212
136. Han, Y, Chaouch, S, Mhamdi, A, Queval, G, Zechmann, B, and Noctor, G. Functional analysis of Arabidopsis mutants points to novel roles for glutathione in coupling H2O2 to activation of salicylic acid accumulation and signaling. Antioxid Redox Signal. (2013a) 18:2106–21. doi: 10.1089/ars.2012.5052
137. Han, Y, Mhamdi, A, Chaouch, S, and Noctor, G. Regulation of basal and oxidative stress-triggered jasmonic acid-related gene expression by glutathione. Plant Cell Environ. (2013b) 36:1135–46. doi: 10.1111/pce.12048
138. Yao, GF, Li, C, Sun, KK, Tang, J, Huang, ZQ, Yang, F, et al. Hydrogen sulfide maintained the good appearance and nutrition in post-harvest tomato fruits by antagonizing the effect of ethylene. Front Plant Sci. (2020) 11:584. doi: 10.3389/fpls.2020.00584
139. Yao, GF, Wei, ZZ, Li, TT, Tang, J, Huang, ZQ, Yang, F, et al. Modulation of enhanced aantioxidant activity by hydrogen sulfide antagonization of ethylene in tomato fruit ripening. J Agric Food Chem. (2018) 66:10380–7. doi: 10.1021/acs.jafc.8b03951
140. Zhao, G, Zhao, Y, Yu, X, Kiprotich, F, Han, H, Guan, R, et al. Nitric oxide is required for melatonin-enhanced tolerance against salinity stress in rapeseed (Brassica napus L.) seedlings. Int J Mol Sci. (2018) 19:1912. doi: 10.3390/ijms19071912
141. Arora, D, and Bhatla, SC. Melatonin and nitric oxide regulate sunflower seedling growth under salt stress accompanying differential expression of cu/ZnSOD and MnSOD. Free Radic Biol Med. (2017) 106:315–28. doi: 10.1016/j.freeradbiomed.2017.02.042
142. Liu, J, Zhang, W, Hu, M, Pan, Y, Jiang, Y, Zhang, Z, et al. Nitric oxide is involved in melatonin-induced cold tolerance in postharvest litchi fruit. Postharvest Biol. Tec. (2023) 196:112157. doi: 10.1016/j.postharvbio.2022.112157
143. Zhang, W, Cao, J, Fan, X, and Jiang, W. Applications of nitric oxide and melatonin in improving postharvest fruit quality and the separate and crosstalk biochemical mechanisms. Trends Food Sci Tec. (2020) 99:531–41. doi: 10.1016/j.tifs.2020.03.024
144. Sun, L, Luo, S, Huali, H, Zhou, H, Zhang, Y, An, R, et al. Melatonin promotes the normal cellular mitochondrial function of lotus seeds through stimulating nitric oxide production. Postharvest Biol. Tec. (2022) 185:111814. doi: 10.1016/j.postharvbio.2021.111814
145. Aghdam, MS, Luo, Z, Jannatizadeh, A, Sheikh-Assadi, M, Sharafi, Y, Farmani, B, et al. Employing exogenous melatonin applying confers chilling tolerance in tomato fruits by upregulating ZAT2/6/12 giving rise to promoting endogenous polyamines, proline, and nitric oxide accumulation by triggering arginine pathway activity. Food Chem. (2019) 275:549–56. doi: 10.1016/j.foodchem.2018.09.157
146. Li, TT, Li, ZR, Hu, KD, Hu, LY, Chen, XY, Li, YH, et al. Hydrogen sulfide alleviates kiwifruit ripening and senescence by antagonizing effect of ethylene. Hortic Sci. (2017) 52:1556–62. doi: 10.21273/HORTSCI12261-17
147. Corpas, FJ, Rodríguez-Ruiz, M, Muñoz-Varga, MA, González-Gordo, S, Reiter, RJ, and Palma, JM. Interactions of melatonin, reactive oxygen species, and nitric oxide during fruit ripening: an update and prospective view. J Exp Bot. (2022) 73:5947–60. doi: 10.1093/jxb/erac128
148. Byeon, Y, Tan, DX, Reiter, RJ, and Back, K. Predominance of 2-hydroxymelatonin over melatonin in plants. J Pineal Res. (2015) 59:448–54. doi: 10.1111/jpi.12274
149. Tan, DX, and Reiter, RJ. An evolutionary view of melatonin synthesis and metabolism related to its biological functions in plants. J Exp Bot. (2020) 71:4677–89. doi: 10.1093/jxb/eraa235
150. Marino, D, Dunand, C, Puppo, A, and Pauly, N. A burst of plant NADPH oxidases. Trends Plant Sci. (2012) 17:9–15. doi: 10.1016/j.tplants.2011.10.001
151. Sagi, M, and Fluhr, R. Production of reactive oxygen species by plant NADPH oxidases. Plant Physiol. (2006) 141:336–40. doi: 10.1104/pp.106.078089
152. Suzuki, N, Miller, G, Morales, J, Shulaev, V, Torres, MA, and Mittler, R. Respiratory burst oxidases: the engines of ROS signaling. Curr Opin Plant Biol. (2011) 14:691–9. doi: 10.1016/j.pbi.2011.07.014
153. Peng, X, Wang, N, Sun, S, Geng, L, Guo, N, Liu, A, et al. Reactive oxygen species signaling is involved in melatonin-induced reduction of chlorothalonil residue in tomato leaves. J Hazard Mater. (2023) 443:130212. doi: 10.1016/j.jhazmat.2022.130212
154. Ze, Y, Gao, H, Li, T, Yang, B, and Jiang, Y. Insights into the roles of melatonin in maintaining quality and extending shelf life of postharvest fruits. Trends Food Sci. Tec. (2021) 109:569–78. doi: 10.1016/j.tifs.2021.01.051
155. Li, S, Cheng, Y, Yan, R, Liu, Y, Huan, C, and Zheng, X. Preharvest spray with melatonin improves postharvest disease resistance in cherry tomato fruit. Postharvest Biol. Tec. (2022a) 193:112055. doi: 10.1016/j.postharvbio.2022.112055
156. Yin, LH, Wang, P, Li, MJ, Ke, XW, Li, CY, Liang, D, et al. Exogenous melatonin improves Malus resistance to Marssonina apple blotch. J Pineal Res. (2013) 54:426–34. doi: 10.1111/jpi.12038
157. Wei, J, Li, DX, Zhang, JR, Shan, C, Rengel, Z, Song, ZB, et al. Phytomelatonin receptor PMTR1-mediated signaling regulates stomatal closure in Arabidopsis thaliana. J Pineal Res. (2018) 65:e12500. doi: 10.1111/jpi.12500
158. Wang, LF, Li, TT, Zhang, Y, Guo, JX, Lu, KK, and Liu, WC. CAND2/PMTR1 is required for melatonin-conferred osmotic stress tolerance in Arabidopsis. Int J Mol Sci. (2021a) 22:4014. doi: 10.3390/ijms22084014
Keywords: fruit, melatonin, post-harvest preservation, reactive oxygen species, signaling networks, vegetable
Citation: Li N, Zhai K, Yin Q, Gu Q, Zhang X, Melencion MG and Chen Z (2023) Crosstalk between melatonin and reactive oxygen species in fruits and vegetables post-harvest preservation: An update. Front. Nutr. 10:1143511. doi: 10.3389/fnut.2023.1143511
Edited by:
Jiaojiao Zhang, Zhejiang Agriculture and Forestry University, ChinaReviewed by:
Laifeng Lu, Tianjin University of Science and Technology, ChinaGuiguang Cheng, Kunming University of Science and Technology, China
Chikere Nkwonta, University of Leeds, United Kingdom
Copyright © 2023 Li, Zhai, Yin, Gu, Zhang, Melencion and Chen. This is an open-access article distributed under the terms of the Creative Commons Attribution License (CC BY). The use, distribution or reproduction in other forums is permitted, provided the original author(s) and the copyright owner(s) are credited and that the original publication in this journal is cited, in accordance with accepted academic practice. No use, distribution or reproduction is permitted which does not comply with these terms.
*Correspondence: Merced G. Melencion, Zi5tZXJjZWQubWVsZW5jaW9uQGNtdS5lZHUucGg=; Ziping Chen, enBjaGVuQGFoYXUuZWR1LmNu
†These authors have contributed equally to this work