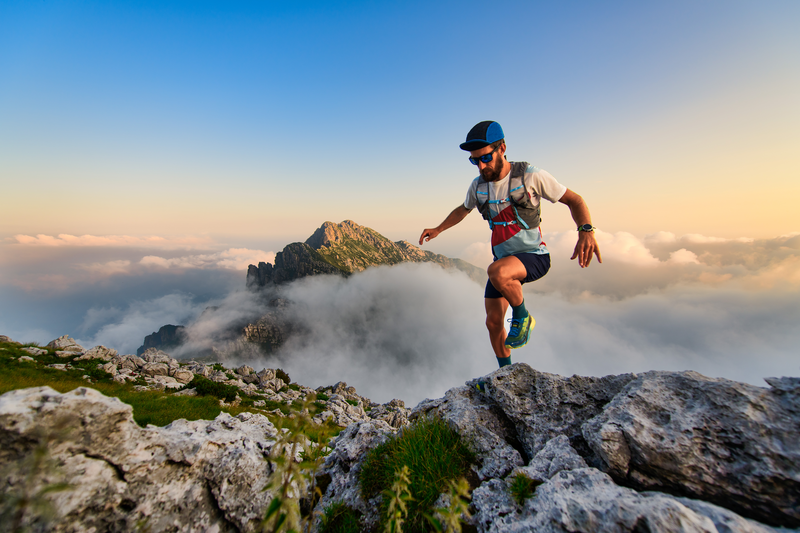
95% of researchers rate our articles as excellent or good
Learn more about the work of our research integrity team to safeguard the quality of each article we publish.
Find out more
SYSTEMATIC REVIEW article
Front. Nutr. , 16 February 2023
Sec. Clinical Nutrition
Volume 10 - 2023 | https://doi.org/10.3389/fnut.2023.1137371
Aim: Chronic obstructive pulmonary disease (COPD) patients vary widely in terms of the prevalence of sarcopenia, which is partially attributed to differences in diagnostic criteria and disease severity. There are several different musculature measurements that are used to quantify sarcopenia. This study included published literature for meta-analysis to assess the sarcopenia prevalence in COPD patients and correlate the disease with the clinical characteristics of such patients.
Methods: A comprehensive review of the English and Chinese literature on sarcopenia prevalence in COPD patients was conducted using electronic databases such as China National Knowledge Infrastructure (CNKI), Web of Science, Cochrane Library, EMBASE, PubMed, and Wanfang. Two researchers analyzed the studies for Newcastle-Ottawa Scale. The software Stata 11.0 was employed for the analysis of the acquired data. The standard mean differences method was utilized for the estimation and quantification of the effect size. Furthermore, a fixed- or random-effects model was employed for conducting a combined analysis.
Results: In total, 56 studies were included as per the specific inclusion criteria. The resulting data of the assessed COPD patients in this research indicated a 27% prevalence of sarcopenia. Further analysis of subgroups was executed per disease severity, ethnicity, diagnostic criteria, gender, and age. Per these findings, increased disease severity elevated the prevalence of sarcopenia. The Latin American and Caucasian populations indicated an increased prevalence of sarcopenia. In addition, the prevalence of sarcopenia was related to diagnostic criteria and definition. Male COPD patients had a higher prevalence of sarcopenia than female COPD patients. COPD patients with an average age greater than 65 had a slightly higher prevalence of sarcopenia. COPD patients with comorbid sarcopenia had poorer pulmonary function, activity tolerance, and clinical symptoms than patients with COPD alone.
Conclusion: Sarcopenia prevalence is high (27%) in COPD patients. In addition, these patients had worse pulmonary function and activity tolerance compared to patients without sarcopenia.
Systematic review registration: https://www.crd.york.ac.uk/prospero/display_record.php?RecordID=367422, identifier CRD42022367422.
Characterized by abnormal extra-pulmonary manifestations and chronic inflammation, chronic obstructive pulmonary disease (COPD), negatively affects the quality of life of patients and physical function (decreased physical activity endurance levels, strength, and mass of muscles) (1, 2). These aforementioned factors are also strongly associated with the onset and progression of sarcopenia. Sarcopenia is defined as a syndrome of progressive age-related decline in the strength and mass of muscles, with or without low physical performance. Notably, sarcopenia-related changes are not restricted to the muscle itself, as neurological and endocrine disorders are also linked to sarcopenia (3). The evaluation of sarcopenia mainly concerns to assess muscle mass, muscle strength, and physical performance, which was measured by dual-energy X-ray absorptiometry (DXA), bioelectrical impedance analysis, handgrip dynamometry, gait speed, etc. (3). Increased falls, hospitalization, and death are all highly correlated with sarcopenia, a primary cause of frailty in the elderly population (4), with a prevalence of approximately 5–10% in people older than 65 years of age (5). Moreover, Asian people appear to have greater sarcopenia prevalence than other regions (6).
Patients with COPD are at a significantly high risk of developing sarcopenia, with prevalence estimates ranging from 15 to 55% (7, 8). Sarcopenia prevalence of COPD is often associated with different musculature measurements, diagnostic criteria, ethnicity and disease severity, etc. According to a cross-sectional investigation of a Southeast Asian population, DXA was used to assess muscle mass, and 24% of individuals with COPD were found to have sarcopenia (6). Limpawattana et al. (6) pointed out that COPD patients often had relative or an absolute increase in fat mass which might lead to systemic inflammation, loss of fat-free mass, and insulin resistance which were significantly related to sarcopenia. Furthermore, increased systemic inflammation, and the possible presence of hypoxia and the more frequent use of systemic corticosteroids also resulted in excessive apoptosis of skeletal muscle (9). Thus, patients with COPD generally have a higher sarcopenia prevalence. In patients with concurrent COPD and sarcopenia, sarcopenia appears to negatively affect functional and health-related clinical indicators, and the increase in sarcopenia prevalence is linked to the severity of COPD (10). Sarcopenia can also lead to an increased risk of non-alcoholic liver disease, metabolic syndrome, and osteoporosis in COPD patients (11–13). Lower muscle strength also predicts an increased risk of acute exacerbations in COPD patients (14). Weakness of the diaphragm and the accessory muscle of inspiration is universally found in patients with long-duration COPD. Weakness of inspiratory muscle results in dyspnea and a decrease in exercise tolerance, as well as hypoxemia, in patients with COPD (15). All of these findings suggest that COPD and sarcopenia may have a mutually reinforcing relationship that leads to a vicious cycle. The actual clinical impact of sarcopenia in COPD remains to be elucidated despite its correlation with the worsening prognosis in COPD patients (16). However, it is challenging to adequately assess this impact due to the broad range of sarcopenia prevalence estimates in COPD.
Based on observational data, the most recent research has demonstrated wide-ranging and inconsistent results. Sepúlveda-Loyola et al. (17) conducted a meta-analysis involving literature published between 2006 and 2018 in 2020 analyzing sarcopenia prevalence in COPD patients and comparing the variances in pulmonary function and activity tolerance between patients with sarcopenia and those without sarcopenia. The main sites included in the previous meta-analyses were developed countries, such as Europe and North America. As China is also one of the countries with a high burden of COPD (18), therefore, the inclusion of studies involving patients from the Chinese population in the meta-analysis is necessary. Different musculature measurements used for the diagnosis of sarcopenia may lead to the different prevalences of sarcopenia in patients with COPD. In addition, several studies on concurrent COPD and sarcopenia have been conducted in recent years, yielding widely varying results. Therefore, this meta-analysis aimed to include the most recent observational studies to determine the differences in sarcopenia prevalence in the different subgroups of COPD patients.
This review’s protocol was registered in PROSPERO (CRD42022367422).1 From their establishment to October 2022, the following free-text and subject heading terms were utilized to search these six electronic databases [i.e., EMBASE, PubMed, Cochrane library, Web of Science, Wanfang, and China National Knowledge Infrastructure (CNKI)]: “COPD,” “chronic obstructive pulmonary disease,” “chronic obstructive lung disease,” “COAD,” “chronic obstructive airway disease,” and “sarcopenia.” To find more potential research, reference lists from included papers were manually searched. This research included papers based on whether they ultimately involved a sarcopenia diagnosis (defined per any criteria given that it was described in the methodology) and whether the COPD participants were adults regardless of the severity of the disease. Disease severity was assessed via the Global Initiative for Chronic Obstructive Lung Disease (GOLD) staging system (19). The nature of the study’s research question allowed for the inclusion of cross-sectional studies and clinical trials (whether randomized or not) and observational (e.g., cohort) studies. It was not possible to include an abstract or works published in languages other than English, Spanish, or Portuguese. The Preferred Reporting Items for Systematic Reviews and Meta-Analyses (PRISMA) flow diagram below (Figure 1) summarizes the results of the article selection processes.
Figure 1. Preferred reporting item for meta-analysis (PRISMA) flow diagram for the study selection process.
This review’s key results were (i) the criteria utilized for defining sarcopenia and its prevalence, (ii) clinical indicators from studies that provided collected data between COPD patients who did and did not have sarcopenia. The clinical indicators were pulmonary function, modified Medical Research Council (mMRC) Dyspnea Scale score, BMI, obstruction, dyspnea, exercise capacity (BODE) index, and walking distance on the 6-min Walk Test (6MWT).
The bibliographical reference manager software (EndNote 20, Thomson Reuters, Toronto, ON, Canada) was utilized to assemble the data resulting from examining the databases and the duplicates were eliminated. Two reviewers who worked independently analyzed the citations as per their abstract and title and determined their eligibility. They categorized these citations as either “include,” “exclude,” or “maybe” whose full text was further reviewed in order to reach a final yield, with an independent third reviewer resolving any conflict. This procedure was summarized per preferred reporting item for systematic reviews and meta-analysis recommendations. Using standardized templates suited to the objectives of the study, two team members extracted the data.
Using the Newcastle-Ottawa Scale (NOS) (20), which is commonly applied to examine case-control and cross-sectional studies, two researchers independently scored each study’s quality. NOS included study population selection (4 items), comparability (1 item), and exposure or outcome (3 items); scores for 0–3, 4–6, and 7–9 were thought to indicate low, medium, and high-quality studies, respectively. Higher scores indicate better methodological quality. Additionally, discussions between the two researchers helped in resolving their disputes.
The proportion of sarcopenia-associated COPD patients in each separate research (each research correlating to one estimate) was combined in a meta-analysis to determine the overall estimate of sarcopenia prevalence. An aggravated value, if it could be quantified, or the most “conventional” type was utilized in the case of the depiction of various sarcopenia types in a study (such as severe sarcopenia, sarcopenic obesity, and sarcopenia with normal body mass index). In the case of the use of more than one criterion for diagnosis (comparisons of different cut-off values with a single cohort) in a study, the estimates were summed up utilizing their stated main method or the one closely resembling the current the European Working Group on Sarcopenia in Older People (EWGSOP) recommendation (21) to avoid double counting. Subgroups were separately comparatively analyzed, where possible, through the χ2 test concerning the prevalence effect estimates between different elements such as disease severity (GOLD stages I–II vs. III–IV), gender (male vs. female), age, sarcopenia definitions (1 vs. >1 diagnostic criteria), and ethnicity (Caucasian, Latin American, and Asian). The Stata 11.0 (Stata Corporation, College Station, TX, USA) software via the function “metaprop” executed the meta-analysis with 95% confidence intervals (CIs) quantified through the score (Wilson) method as well as to account for the sarcopenia’s definitional variability across studies, a random-effects model (Dersimonian and Laird method).
Comparative analyses of COPD patients with and without sarcopenia were examined in terms of their resulting clinical outcomes, which were subjected to meta-analysis via Stata 11.0. Data in the form of weighted mean differences (WMD) and 95% CI were summarized. The primary technique of analysis was a random-effects model, with Deeks et al.’s interpretation of I2 statistic employed to characterize statistical heterogeneity (with values <25, 50–75, and >75% regarded as low, moderate, and high, respectively).
The literature was searched systematically and thoroughly (Figure 1), and the databases provided 356 unique records, which were reduced to 56 articles (7, 8, 11, 16, 22–73) after the final review. Among these, 1 was a clinical non-randomized trial, 7 were observational cohort studies, and 48 had a cross-sectional design. In total, 28 reports provided comparative information on COPD patients with and without sarcopenia. The literature quality scores were summarized in Supplementary Table 1. The selected research’s characteristics were summarized in Table 1. This research covered various populations, such as Latinos, Asians, and Caucasians with 11, 24, and 21 studies from each, respectively.
Table 1. Characteristics of the included studies regarding the prevalence of sarcopenia in subjects with COPD.
The selected research was assessed through the specific diagnostic criteria utilized for sarcopenia (Table 1). The diagnosis was based upon the measurement of low muscle mass (LMM) and low muscle strength (LMS) with the former solely used in 36 studies, and in 20 studies in combination with lower physical performance (LPP) and/or LMS. LMM, LMS, and LPP were identified through various methods and cut-off points in those studies. DXA, bioelectrical impedance analysis, and calf circumference were utilized to quantify muscle mass. Muscle strength was measured via handgrip dynamometry. Physical performance was measured via 6MWT. The various cut-off values utilized for defining each test’s “positive” results are depicted (Supplementary Table 2). Per the Asian group of sarcopenias (74) and EWGSOP (75) recommended threshold muscle mass, strength, as well as physical performance were mostly examined. The sarcopenia detection guidelines for COPD patients were comparatively analyzed (Supplementary Table 3).
Concerning sarcopenia, the COPD patients’ overall pooled prevalence was estimated at 27% (95% CI = 0.23–0.31; Figure 2). This analysis depicted considerable statistical heterogeneity (I2 = 99.1%) implying that the individual study weighting was uniform (range 1.45–1.94%).
Research that utilized only one criterion depicted considerably increased effect estimates [LMM; 28%, (95% CI = 0.22–0.34)] as compared to those that employed more than one criterion [LMM + LMS and/or LPP; 24% (95% CI = 0.20–0.29] (Figure 2 and Table 2).
A higher sarcopenia prevalence was indicated in male [35% (95% CI = 0.28–0.41)] than in female [28% (95% CI = 0.22–0.34)] in studies concerning gender (Table 2).
In some studies that provided data specific to disease severity, sarcopenia was found to be remarkably higher in patients with more severe disease [GOLD stages III–IV; 39% (95% CI = 0.32–0.46)] than those with less severe disease [GOLD stages I–II; 28% (95% CI = 0.20–0.37)] (Table 2).
Subgroup analysis was performed according to ethnicity. The combined prevalence values of concurrent COPD and sarcopenia were 28% (95% CI = 0.19–0.37), 25% (95% CI = 0.20–0.29), and 29% (95% CI = 0.20–0.37) among Caucasians, Asians, and Latinos, respectively (Table 2).
Next, we performed a subgroup analysis based on age. COPD patients with an average age greater than 65 had a slightly higher prevalence of sarcopenia [Mean age group ≥65 vs. Mean age <65 group: 28% (95% CI = 0.22–0.34) vs. 26% (95% CI = 0.18–0.36)] (Table 2).
A combined analysis of the prevalence of sarcopenia in all COPD patients showed a high degree of heterogeneity (I2 = 99.1%, P < 0.001). Therefore, a meta-regression analysis was executed to determine the source of the high heterogeneity. Meta-regression analysis recorded P-values of 0.401, 0.176, 0.368, 0.442, and 0.598 for race, gender, disease severity, age, and diagnostic criteria, respectively, indicating no significant effect of these factors on heterogeneity. In the sensitivity analysis, each of the 56 studies was excluded in turn. Subsequently, a meta-analysis of the remaining studies was executed, and the results were compared with the results before the previous exclusion, indicating that the aforementioned exclusion of each study had no significant effect on the combined results (Figure 3).
Figure 3. Sensitivity analysis of studies on the prevalence of sarcopenia in COPD patients in meta-analysis.
A meta-analysis of pulmonary function data from 24 reports depicted that COPD patients with sarcopenia had poorer values on average concerning Forced Expiratory Volume in 1 s / Forced Vital Capacity (FEV1/FVC), predicted FEV1%, and predicted FVC% than those without sarcopenia (Table 3). The result of FEV1/FVC between the COPD group and the COPD + sarcopenia group was reported in Figure 4. The result of predicted FEV1% between the COPD group and the COPD + sarcopenia group was shown in Supplementary Figure 1. The result of predicted FVC% between the COPD group and the COPD + sarcopenia group was reported in Supplementary Figure 2.
Table 3. Meta-analysis, differences regarding lung function, 6MWT, mMRC, and BODE index between patients with and without sarcopenia.
Figure 4. WMD forest plot and its 95% CI for FEV1/FVC in COPD group and sarcopenia + COPD group. FEV1, forced expiratory volume in 1 s; FVC, forced vital capacity; WMD, weighted mean differences.
Data from 11 studies were available for outcomes related to 6MWT wherein a worse performance was linked to the sarcopenia-afflicted individuals in comparison with those lacking sarcopenia [COPD vs. COPD + sarcopenia: weighted mean difference = 72.17 (95% CI = 45.11–99.23), P < 0.001; I2 = 83.3%; Figure 5 and Table 3].
Figure 5. WMD forest plot and its 95% CI for the 6-min walk test in COPD group and sarcopenia + COPD group. WMD, weighted mean differences.
Seven studies involving reported data on mMRC scores were involved in the meta-analysis. The presence of sarcopenia was linked to mMRC scores [COPD vs. COPD + sarcopenia: weighted mean difference = −0.47 (95% CI = −0.81 to −0.13), P < 0.001; I2 = 87.6%; Supplementary Figure 3 and Table 3].
Four studies provided data on the BODE index in COPD patients without sarcopenia vs. those with sarcopenia, and the findings depicted that COPD patients had a lower BODE index than those in the sarcopenia group [COPD vs. COPD + sarcopenia: weighted mean difference: −1.49 (95% CI = −2.12 to −0.86), P < 0.001; I2 = 59.2%; Supplementary Figure 4 and Table 3].
These systematic evaluations and meta-analyses provide unique insights into sarcopenia’s clinical relevance in patients with COPD. This study depicts the prevalence of sarcopenia in patients with COPD and the impact of gender, age, and different diagnostic criteria on its prevalence. Additionally, the present study compared the indicators reflecting pulmonary function and activity tolerance between the COPD group and the COPD + sarcopenia group.
Female COPD patients are slightly less likely to have comorbid sarcopenia than male COPD patients, which may be attributed to the higher proportion of men who smoke. Free radicals in cigarette smoke can lead to oxidative stress in skeletal muscle (76) and chronic smoke exposure can degenerate neuromuscular junctions (77). Regarding the different prevalences of sarcopenia between male and female in this meta-analysis, we believed smoking status might be one of the interfering factors. Unfortunately, we were not able to perform subgroup analysis by smoking status as included studies did not provide concrete data about sex differences in smoking.
In addition, the prevalence of concurrent COPD and sarcopenia varies between races and may be attributed to the large differences in race, body size, lifestyle habits, and muscle mass as well as strength measurements in different geographical populations. Moreover, we found that COPD patients with an average age greater than 65 had a slightly higher prevalence of sarcopenia. Sarcopenia is a common disease in the elderly population (78). Sarcopenia is a condition where muscle fiber cross-sectional area is reduced due to muscle atrophy and protein degradation (79). The occurrence of sarcopenia results from the combined action of internal and external factors. Age is considered an important contributor to sarcopenia, the skeletal muscle mass, myofiber size, and muscle strength endurance which reduce significantly with age (80). In addition, organismal inflammation increases during aging, which may excessively activate the ubiquitin-proteasome system (UPS). Protein degradation in skeletal muscle primarily depends on the activation of the two major proteolytic pathways, the UPS and the autophagy-lysosome system (81). Furthermore, aging perturbs organismal homeostasis and leads to multi-organ dysfunction and frailty, especially mitochondrial dysfunction, which has a central role in the occurrence of sarcopenia (82, 83). In this meta-analysis, the majority of included patients were older than 60 years, and several included studies did not provide information about the precise age. Thus, the difference in sarcopenia prevalence between the two groups (Mean age ≥65 group vs. Mean age <65 group) was not obvious. Therefore, more research into the non-elderly COPD group are required in the future.
Concerning sarcopenia diagnosis, two primary methods are currently used in patients with COPD: LMM-based independent assessment (50) and LMM-based assessment combined with LMS or LPP (21). The prevalence of sarcopenia in COPD patients diagnosed with LMM alone was 28%, while the value was 24% when diagnosed with LMM in combination with LMS and/or physiological function. Differences in prevalence due to different diagnostic criteria have been documented previously in older community-dwelling adults (84). Therefore, the different prevalences of concurrent COPD and sarcopenia reported in different studies may be partly due to the different diagnostic criteria for sarcopenia. This is not surprising, as increasing the number of restrictive conditions in the definition of sarcopenia will certainly decrease the incidence of a “positive” diagnosis. However, it may improve diagnostic accuracy. This is congruent with the current international diagnostic criteria, an important premise of which is the definition of sarcopenia as a geriatric syndrome indicated by LMM and LPP rather than by LMM alone. Only 20 of the included studies applied a sarcopenia definition that met these new criteria. According to the results of this study, increasing the severity of COPD elevated the probability of developing sarcopenia. Therefore, it seems sensible to stratify patients according to disease severity to guarantee as precise conclusions as possible from the current study. Combining the results of these studies may improve the understanding of the interactions between COPD and sarcopenia. International recommendations suggest DXA and bioelectrical impedance analysis as the preferred methods for assessing LMM at sites including the lower extremities and chest wall muscles (85). These testing methods are common in the literature included in this meta-analysis.
Regardless, normality or abnormality was assigned to test results per the observed various cut-off points. Advocated by the EWGSOP, the criteria of Baumgartner et al. (86) and Newman et al. (87) were the predominantly used criteria. Chen et al. (74) and Cruz-Jentoft et al. (75) did not support van de Bool et al. (54) measurement of muscle mass using calf circumference as a sarcopenia screening method, despite its simplicity. Such guidance is also present for measuring physical performance (gait speed) and muscular strength (handgrip force), although discrepancies were once again noticeable. A total of 4 m gait speed (51) and 6MWT (63) were employed for assessing the gait speed. Although both tests employed the same threshold for sarcopenia diagnosis (<0.8 m/s), they are both extremely different tests. A distance of 4 m is usually traversed at a normal walking speed in the 4 m gait speed (however, variances at various walk speeds and track lengths exist), while a walking track of 30 m is traversed in the 6MWT with the exercise tolerance of participants assessed by encouraging them to walk as far as they can [oftentimes faster than normal speed (88)]. There is a considerable risk that a measure of walking speed estimated from the 6MWT may be inaccurately interpreted. For instance, it was unable to differentiate between individuals moving slowly and those moving quickly but pausing to rest during the test. Therefore, it is possible that the prevalence of sarcopenia was underestimated in the research that adopted this strategy. Therefore, it is essential that future studies adopt standardized cut-off values to enable precise test interpretation in addition to using consistent diagnostic procedures to detect sarcopenia.
Sarcopenia has a persistent negative impact on a range of clinical features associated with COPD, such as balance, exercise capacity, grip strength, gait speed, and physical activity levels (53). It is also linked to an elevated symptom burden and a worse quality of life. It is noteworthy that the two studies that quantified dyspnea (Medical Research Council scale) (8, 60) classified sarcopenia solely per physical function, which is important since it suggests that functional impairment may be more strongly associated with dyspnea than LMM. Additionally, this brings up some difficult issues regarding the strategies employed for clinical management. Despite the correlation between COPD and sarcopenia, a causal relationship between the two diseases cannot be inferred. This research suggested that sarcopenia should be emphasized as an important “treatable trait” in adult respiratory medicine. Improvement in sarcopenia should serve as an important indicator of the efficacy of COPD rehabilitation. Jones et al. (8) indicated that an exercise-based, comprehensive intervention, pulmonary rehabilitation, was a multi-component technique that benefited a wide variety of clinical outcomes and decreased sarcopenia incidence in COPD patients. However, research is required to authenticate the findings of Jones et al. further, such as the use of nutritional supplementation and muscle strength training as recommended adjunctive therapies.
The actual mortality rate in patients with concurrent COPD and sarcopenia was not accurately quantified due to the lack of relevant literature. Nevertheless, it is speculated that sarcopenia may be associated with elevated mortality in patients with COPD due to the higher prevalence of sarcopenia in patients with GOLD stages III–IV. Leivseth et al. (89) reported that over 12 years of follow-up women depicted more than a sixfold increase in mortality risk while men depicted more than double the increase in risk in individuals with GOLD stages III and IV disease severity. Elevated fatality risk was indicated in COPD patients assessed via BODE, which is a widely used, valid tool for predicting the risk of death in COPD. In individuals with GOLD stage III and IV, Costa et al. (53) documented a rise in sarcopenia prevalence, with these quartiles linked to lower 4-year survival. A poorer physical and pulmonary function and quality of life were indicative of sarcopenia and these factors are known to be linked to an increased risk of death in patients with COPD. Multiple studies part of this meta-analysis reported data on pulmonary function, 6MWT, mMRC scale scores, and BODE scores comparing COPD patients with or without sarcopenia. The combined results revealed that COPD patients with comorbid sarcopenia had considerably lower projected FEV1 values than those without comorbid sarcopenia. In addition, the 6-min walk distance was notably lower in patients with comorbid sarcopenia than in patients without comorbid sarcopenia, and the mMRC score and BODE index was increased in patients with comorbid sarcopenia than in patients without comorbid sarcopenia. Considering that COPD is both an airway and systemic inflammatory disease, a high concentration of inflammatory factors could affect muscle atrophy and decreased muscle function (90, 91). A study involving 164 older adults suggested that interleukin-6 levels were negatively associated with skeletal muscle mass index and grip strength, whereas tumor necrosis factor-α and C-reactive protein levels were negatively associated with muscle mass and strength (92). In addition, mechanisms such as hypoxia and oxidative stress, myocyte mitochondrial dysfunction, and muscle metabolism disorders in COPD (93–96) can all produce pathological changes in skeletal muscle in terms of muscle volume and fiber type, ultimately leading to a significant decrease in muscle function. A definite relationship between sarcopenia and inflammatory biomarkers was not depicted because relevant data could not be extracted and obtained. Moreover, patients with acute exacerbations of COPD and severe COPD receive systemic applications of glucocorticoids that lead to negative nitrogen balance in the body and affect muscle mass and strength (97). Therefore, clinicians should be alert to the occurrence of sarcopenia and enhance screening for sarcopenia in patients with COPD to improve patients’ quality of survival and slow down the progression of the disease. In addition, patients with COPD should actively participate in endurance exercise training, pulmonary rehabilitation, and nutritional support to prevent the occurrence of sarcopenia.
This literature review has several limitations. First, factors such as different definitions of sarcopenia in different studies, subject characteristics, and diagnostic thresholds can lead to heterogeneity. Therefore, the interpretation of the results obtained from meta-analysis is challenging. Second, this review did not elucidate the direct relationship between sarcopenia and mortality due to the lack of data. Therefore, a long-term follow-up is needed to observe the survival time in the patient cohort. Nevertheless, the association observed between sarcopenia and mortality risk (assessed by the BODE index) is noteworthy. Third, this study lacks direct evidence highlighting the clinical impact of sarcopenia on healthcare costs, which is an important area to be addressed in future studies. Fourth, the different subtypes of sarcopenia (e.g., sarcopenic obesity and severe sarcopenia) may lead to some limitations in the clinical application of the results of this study. In addition, most of the included studies were cross-sectional studies. Consequently, a causal relationship between sarcopenia and COPD could not be determined.
Sarcopenia prevalence is high (27%) in COPD patients. The prevalence of sarcopenia differs in COPD patients according to musculature measurements, gender, disease severity, ethnicity, and age. In addition, the pulmonary function and physical performance of COPD patients with sarcopenia were significantly worse than that of COPD patients without sarcopenia. In further clinical work, attention should be paid to screening for sarcopenia in patients with COPD.
The original contributions presented in this study are included in this article/Supplementary material, further inquiries can be directed to the corresponding author.
JH and HL developed and refined the research idea, performed the data collection and data analysis, prepared the first manuscript draft, and edited the manuscript. YW and JY validated the data collection, developed the research idea, and proofread the manuscript. All authors had made a substantial, direct, and intellectual contribution to the work and approved it for publication.
This work was supported by the Sichuan Medical Research Project Foundation (S21054).
The authors declare that the research was conducted in the absence of any commercial or financial relationships that could be construed as a potential conflict of interest.
All claims expressed in this article are solely those of the authors and do not necessarily represent those of their affiliated organizations, or those of the publisher, the editors and the reviewers. Any product that may be evaluated in this article, or claim that may be made by its manufacturer, is not guaranteed or endorsed by the publisher.
The Supplementary Material for this article can be found online at: https://www.frontiersin.org/articles/10.3389/fnut.2023.1137371/full#supplementary-material
Supplementary Figure 1 | WMD forest plot and its 95% CI for FEV1% predicted in the COPD group and sarcopenia + COPD group. WMD, weighted mean differences; FEV1, forced expiratory volume in 1 s.
Supplementary Figure 2 | WMD forest plot and its 95% CI for FVC% predicted in the COPD group and sarcopenia + COPD group. WMD, weighted mean differences; FVC, forced vital capacity.
Supplementary Figure 3 | WMD forest plot and its 95% CI for mMRC scores in the COPD group and sarcopenia + COPD group. WMD, weighted mean differences; mMRC, modified Medical Research Council.
Supplementary Figure 4 | WMD forest plot and its 95% CI for BODE index in the COPD group and sarcopenia + COPD group. WMD, weighted mean differences.
1. Pérez-Peiró M, Alvarado M, Martín-Ontiyuelo C, Duran X, Rodríguez-Chiaradía D, Barreiro E. Iron depletion in systemic and muscle compartments defines a specific phenotype of severe COPD in female and male patients: implications in exercise tolerance. Nutrients. (2022) 14:3929. doi: 10.3390/nu14193929
2. de Lima F, Camillo C, Grigoletto I, Uzeloto J, Vanderlei F, Ramos D, et al. Effects of combining functional exercises with exercise training on daily physical activities and functionality in patients with COPD: a protocol for a randomized clinical trial. Trials. (2019) 20:680. doi: 10.1186/s13063-019-3780-y
3. Fry C, Rasmussen B. Skeletal muscle protein balance and metabolism in the elderly. Curr Aging Sci. (2011) 4:260–8. doi: 10.2174/1874609811104030260
4. Wiśniowska-Szurlej A, Ćwirlej-Sozańska A, Wołoszyn N, Sozański B, Wilmowska-Pietruszyńska A. Association between handgrip strength, mobility, leg strength, flexibility, and postural balance in older adults under long-term care facilities. Biomed Res Int. (2019) 2019:1042834. doi: 10.1155/2019/1042834
5. Park H, Jun D, Park H, Park K, Kim M, Hwang H. A critical appraisal of the definition of sarcopenia in patients with non-alcoholic fatty liver disease: pitfall of adjusted muscle mass by body weight. Life (Basel). (2020) 10:218. doi: 10.3390/life10100218
6. Limpawattana P, Inthasuwan P, Putraveephong S, Boonsawat W, Theerakulpisut D, Sawanyawisuth K. Sarcopenia in chronic obstructive pulmonary disease: a study of prevalence and associated factors in the Southeast Asian population. Chron Respir Dis. (2018) 15:250–7. doi: 10.1177/1479972317743759
7. Cebron Lipovec N, Schols A, van den Borst B, Beijers R, Kosten T, Omersa D, et al. Sarcopenia in advanced COPD affects cardiometabolic risk reduction by short-term high-intensity pulmonary rehabilitation. J Am Med Dir Assoc. (2016) 17:814–20. doi: 10.1016/j.jamda.2016.05.002
8. Jones S, Maddocks M, Kon S, Canavan J, Nolan C, Clark A, et al. Sarcopenia in COPD: prevalence, clinical correlates and response to pulmonary rehabilitation. Thorax. (2015) 70:213–8. doi: 10.1136/thoraxjnl-2014-206440
9. Dao T, Green A, Kim Y, Bae S, Ha K, Gariani K, et al. Sarcopenia and muscle aging: a brief overview. Endocrinol Metab (Seoul). (2020) 35:716–32. doi: 10.3803/EnM.2020.405
10. Huang H, Chou P, Joa W, Chen L, Sheng T, Lin H, et al. Pulmonary rehabilitation coupled with negative pressure ventilation decreases decline in lung function, hospitalizations, and medical cost in COPD: a 5-year study. Medicine (Baltimore). (2016) 95:e5119. doi: 10.1097/md.0000000000005119
11. Chung J, Hwang H, Han C, Son B, Kim D, Park M. Association between sarcopenia and metabolic syndrome in chronic obstructive pulmonary disease: the Korea national health and nutrition examination survey (KNHANES) from 2008 to 2011. COPD. (2015) 12:82–9. doi: 10.3109/15412555.2014.908835
12. Lee D, Choi E. Sarcopenia as an independent risk factor for decreased BMD in COPD patients: Korean national health and nutrition examination surveys IV and V (2008-2011). PLoS One. (2016) 11:e0164303. doi: 10.1371/journal.pone.0164303
13. Hong K, Kim M, Ahn J. Sarcopenia is an independent risk factor for NAFLD in COPD: a nationwide survey (KNHANES 2008-2011). Int J Chron Obstruct Pulmon Dis. (2020) 15:1005–14. doi: 10.2147/copd.S249534
14. Lee C, Wang P. Handgrip strength during admission for COPD exacerbation: impact on further exacerbation risk. BMC Pulm Med. (2021) 21:245. doi: 10.1186/s12890-021-01610-7
15. Wu W, Zhang X, Lin L, Ou Y, Li X, Guan L, et al. Transdiaphragmatic pressure and neural respiratory drive measured during inspiratory muscle training in stable patients with chronic obstructive pulmonary disease. Int J Chron Obstruct Pulmon Dis. (2017) 12:773–81. doi: 10.2147/copd.S126354
16. Kaluźniak-Szymanowska A, Krzymińska-Siemaszko R, Deskur-Śmielecka E, Lewandowicz M, Kaczmarek B, Wieczorowska-Tobis K. Malnutrition, sarcopenia, and malnutrition-sarcopenia syndrome in older adults with COPD. Nutrients. (2021) 14:44. doi: 10.3390/nu14010044
17. Sepúlveda-Loyola W, Osadnik C, Phu S, Morita A, Duque G, Probst V. Diagnosis, prevalence, and clinical impact of sarcopenia in COPD: a systematic review and meta-analysis. J Cachexia Sarcopenia Muscle. (2020) 11:1164–76. doi: 10.1002/jcsm.12600
18. Wang Y, Liao J, Zhong Y, Zhang C, Li X, Wang G. Predictive value of combining inflammatory biomarkers and rapid decline of FEV(1) for COPD in Chinese population: a prospective cohort study. Int J Chron Obstruct Pulmon Dis. (2019) 14:2825–33. doi: 10.2147/copd.S223869
19. Jang J, Lee K, Chung J, Shin K, Choi E, Jin H, et al. Assessment of inhaler satisfaction and determinants of high satisfaction among Korean COPD patients. J Korean Med Sci. (2022) 37:e327. doi: 10.3346/jkms.2022.37.e327
20. Stang A. Critical evaluation of the Newcastle-Ottawa scale for the assessment of the quality of nonrandomized studies in meta-analyses. Eur J Epidemiol. (2010) 25:603–5. doi: 10.1007/s10654-010-9491-z
21. Cruz-Jentoft A, Bahat G, Bauer J, Boirie Y, Bruyère O, Cederholm T, et al. Sarcopenia: revised European consensus on definition and diagnosis. Age Ageing. (2019) 48:601. doi: 10.1093/ageing/afz046
22. Trajanoska K, Schoufour J, Darweesh S, Benz E, Medina-Gomez C, Alferink L, et al. Sarcopenia and its clinical correlates in the general population: the Rotterdam study. J Bone Miner Res. (2018) 33:1209–18. doi: 10.1002/jbmr.3416
23. Kovelis D, Gomes A, Mazzarin C, de Miranda A, Valderramas S. Association between the results of the timed up-and-go test adjusted for disease severity and sarcopenia in patients with chronic obstructive pulmonary disease: a pilot study. Clinics (Sao Paulo). (2019) 74:e930. doi: 10.6061/clinics/2019/e930
24. Machado F, Schneider L, Fonseca J, Belo L, Bonomo C, Morita A, et al. Clinical impact of body composition phenotypes in patients with COPD: a retrospective analysis. Eur J Clin Nutr. (2019) 73:1512–9. doi: 10.1038/s41430-019-0390-4
25. Chua J, Tee M. Association of sarcopenia with osteoporosis in patients with chronic obstructive pulmonary disease. Osteoporos Sarcopenia. (2020) 6:129–32. doi: 10.1016/j.afos.2020.07.004
26. Demircioǧlu H, Cihan F, Kutlu R, Yosunkaya Ş, Zamani A. Frequency of sarcopenia and associated outcomes in patients with chronic obstructive pulmonary disease. Turk J Med Sci. (2020) 50:1270–9. doi: 10.3906/sag-1909-36
27. Perrot L, Greil A, Boirie Y, Farigon N, Mulliez A, Costes F, et al. Prevalence of sarcopenia and malnutrition during acute exacerbation of COPD and after 6 months recovery. Eur J Clin Nutr. (2020) 74:1556–64. doi: 10.1038/s41430-020-0623-6
28. Tsekoura M, Tsepis E, Billis E, Gliatis J. Sarcopenia in patients with chronic obstructive pulmonary disease: a study of prevalence and associated factors in Western Greek population. Lung India. (2020) 37:479–84. doi: 10.4103/lungindia.lungindia_143_20
29. Attaway A, Welch N, Hatipoǧlu U, Zein J, Dasarathy S. Muscle loss contributes to higher morbidity and mortality in COPD: an analysis of national trends. Respirology. (2021) 26:62–71. doi: 10.1111/resp.13877
30. Espíndola de Araújo B, Teixeira P, Valduga K, da Silva Fink J, Silva F. Prevalence, associated factors, and prognostic value of sarcopenia in patients with acute exacerbated chronic obstructive pulmonary disease: a cohort study. Clin Nutr ESPEN. (2021) 42:188–94. doi: 10.1016/j.clnesp.2021.01.042
31. Hirai K, Tanaka A, Homma T, Goto Y, Akimoto K, Uno T, et al. Serum creatinine/cystatin C ratio as a surrogate marker for sarcopenia in patients with chronic obstructive pulmonary disease. Clin Nutr. (2021) 40:1274–80. doi: 10.1016/j.clnu.2020.08.010
32. Kanezaki M, Terada K, Tanabe N, Shima H, Hamakawa Y, Sato S. Effects of sarcopenia on ventilatory behavior and the multidimensional nature of dyspnea in patients with chronic obstructive pulmonary disease. J Am Med Dir Assoc. (2021) 22:827–33. doi: 10.1016/j.jamda.2021.01.081
33. Lin B, Bai L, Wang S, Lin H. The association of systemic interleukin 6 and interleukin 10 levels with sarcopenia in elderly patients with chronic obstructive pulmonary disease. Int J Gen Med. (2021) 14:5893–902. doi: 10.2147/ijgm.S321229
34. Sarwar M, McDonald V, Abramson M, Paul E, George J. Treatable traits in an English cohort: prevalence and predictors of future decline in lung function and quality of life in COPD. ERJ Open Res. (2021) 7:00934–2020. doi: 10.1183/23120541.00934-2020
35. Schneider L, Sartori L, Machado F, Dala Pola D, Rugila D, Hirata R, et al. Physical activity and inactivity among different body composition phenotypes in individuals with moderate to very severe chronic obstructive pulmonary disease. Braz J Phys Ther. (2021) 25:296–302. doi: 10.1016/j.bjpt.2020.07.005
36. Sepúlveda-Loyola W, de Castro L, Matsumoto A, Camillo C, Barbosa D, Galvan C, et al. NOVEL antioxidant and oxidant biomarkers related to sarcopenia in COPD. Heart Lung. (2021) 50:184–91. doi: 10.1016/j.hrtlng.2020.06.001
37. van Beers M, Gosker H, Janssen D, Cleutjens F, Franssen F, van Boxtel M, et al. Cognitive performance in relation to metabolic disturbances in patients with COPD. Clin Nutr. (2021) 40:2061–7. doi: 10.1016/j.clnu.2020.09.029
38. Warnken-Miralles M, López-García F, Zamora-Molina L, Soler-Sempere M, Padilla-Navas I, García-Pachón E. Sarcopenia index in hospitalized patients with chronic obstructive pulmonary disease exacerbation. Medicina. (2021) 81:323–8.
39. Lage V, de Paula F, Lima L, Santos J, Dos Santos J, Viegas ÂA, et al. Plasma levels of myokines and inflammatory markers are related with functional and respiratory performance in older adults with COPD and sarcopenia. Exp Gerontol. (2022) 164:111834. doi: 10.1016/j.exger.2022.111834
40. Benz E, Wijnant S, Trajanoska K, Arinze J, de Roos E, de Ridder M, et al. Sarcopenia, systemic immune-inflammation index and all-cause mortality in middle-aged and older people with COPD and asthma: a population-based study. ERJ Open Res. (2022) 8:00628–2021. doi: 10.1183/23120541.00628-2021
41. Cao J, Zuo D, Han T, Liu H, Liu W, Zhang J, et al. Correlation between bioelectrical impedance analysis and chest CT-measured erector spinae muscle area: a cross-sectional study. Front Endocrinol (Lausanne). (2022) 13:923200. doi: 10.3389/fendo.2022.923200
42. Deng M, Bian Y, Zhang Q, Zhou X, Hou G. Growth differentiation factor-15 as a biomarker for sarcopenia in patients with chronic obstructive pulmonary disease. Front Nutr. (2022) 9:897097. doi: 10.3389/fnut.2022.897097
43. Erbas Sacar D, Kılıc C, Oren M, Erdogan T, Ozkok S, Ozer Aydın C, et al. Probable sarcopenia: associations with common geriatric syndromes and comorbidities in Turkish geriatric patients from a university hospital. Eur Geriatr Med. (2022) 13:1299–308. doi: 10.1007/s41999-022-00691-9
44. Gao J, Deng M, Li Y, Yin Y, Zhou X, Zhang Q, et al. Resistin as a systemic inflammation-related biomarker for sarcopenia in patients with chronic obstructive pulmonary disease. Front Nutr. (2022) 9:921399. doi: 10.3389/fnut.2022.921399
45. Lage V, Silva G, Lacerda A, Paula F, Lima L, Santos J, et al. Functional tests associated with sarcopenia in moderate chronic obstructive pulmonary disease. Expert Rev Respir Med. (2021) 15:569–76. doi: 10.1080/17476348.2021.1850276
46. Leem A, Kim Y, Chung K, Park M, Kang Y, Park Y, et al. Sarcopenia is associated with cardiovascular risk in men with COPD, independent of adiposity. Respir Res. (2022) 23:185. doi: 10.1186/s12931-022-02109-3
47. Martínez-Luna N, Orea-Tejeda A, González-Islas D, Flores-Cisneros L, Keirns-Davis C, Sánchez-Santillán R, et al. Association between body composition, sarcopenia and pulmonary function in chronic obstructive pulmonary disease. BMC Pulm Med. (2022) 22:106. doi: 10.1186/s12890-022-01907-1
48. Sugiya R, Higashimoto Y, Shiraishi M, Tamura T, Kimura T, Chiba Y, et al. Decreased tongue strength is related to skeletal muscle mass in COPD patients. Dysphagia. (2022) 37:636–43. doi: 10.1007/s00455-021-10314-3
49. Wang P, Gow C, Chiu Y, Li T. Determination of low muscle mass by muscle surface index of the first lumbar vertebra using low-dose computed tomography. J Clin Med. (2022) 11:2429. doi: 10.3390/jcm11092429
50. Sergi G, Coin A, Marin S, Vianello A, Manzan A, Peruzza S, et al. Body composition and resting energy expenditure in elderly male patients with chronic obstructive pulmonary disease. Respir Med. (2006) 100:1918–24. doi: 10.1016/j.rmed.2006.03.008
51. Gologanu D, Ionita D, Gartonea T, Stanescu C, Bogdan M. Body composition in patients with chronic obstructive pulmonary disease. Maedica (Bucur). (2014) 9:25–32.
52. Koo H, Park J, Park H, Jung H, Lee S. Conflicting role of sarcopenia and obesity in male patients with chronic obstructive pulmonary disease: Korean national health and nutrition examination survey. PLoS One. (2014) 9:e110448. doi: 10.1371/journal.pone.0110448
53. Costa T, Costa F, Moreira C, Rabelo L, Boguszewski C, Borba V. Sarcopenia in COPD: relationship with COPD severity and prognosis. J Bras Pneumol. (2015) 41:415–21. doi: 10.1590/s1806-37132015000000040
54. van de Bool C, Rutten E, Franssen F, Wouters E, Schols A. Antagonistic implications of sarcopenia and abdominal obesity on physical performance in COPD. Eur Respir J. (2015) 46:336–45. doi: 10.1183/09031936.00197314
55. Borda M, Celis-Preciado C, Pérez-Zepeda M, Ríos-Zuluaga J, Cano-Gutiérrez C. Sarcopenia in the elderly with a history of COPD/asthma: results of the SABE-Bogotá study. Rev Esp Geriatr Gerontol. (2017) 52:313–6. doi: 10.1016/j.regg.2016.07.003
56. Joppa P, Tkacova R, Franssen F, Hanson C, Rennard S, Silverman E, et al. Sarcopenic obesity, functional outcomes, and systemic inflammation in patients with chronic obstructive pulmonary disease. J Am Med Dir Assoc. (2016) 17:712–8. doi: 10.1016/j.jamda.2016.03.020
57. Maddocks M, Kon S, Canavan J, Jones S, Nolan C, Labey A, et al. Physical frailty and pulmonary rehabilitation in COPD: a prospective cohort study. Thorax. (2016) 71:988–95. doi: 10.1136/thoraxjnl-2016-208460
58. Pothirat C, Chaiwong W, Phetsuk N, Liwsrisakun C, Bumroongkit C, Deesomchok A, et al. The relationship between body composition and clinical parameters in chronic obstructive pulmonary disease. J Med Assoc Thail. (2016) 99:386–93.
59. van de Bool C, Gosker H, van den Borst B, Op den Kamp C, Slot I, Schols A. Muscle quality is more impaired in sarcopenic patients with chronic obstructive pulmonary disease. J Am Med Dir Assoc. (2016) 17:415–20. doi: 10.1016/j.jamda.2015.12.094
60. Byun M, Cho E, Chang J, Ahn C, Kim H. Sarcopenia correlates with systemic inflammation in COPD. Int J Chron Obstruct Pulmon Dis. (2017) 12:669–75. doi: 10.2147/copd.S130790
61. Hwang J, Kim Y, Leem A, Park M, Kim S, Chang J, et al. Clinical implications of sarcopenia on decreased bone density in men with COPD. Chest. (2017) 151:1018–27. doi: 10.1016/j.chest.2016.12.006
62. Kneppers A, Langen R, Gosker H, Verdijk L, Cebron Lipovec N, Leermakers P, et al. Increased myogenic and protein turnover signaling in skeletal muscle of chronic obstructive pulmonary disease patients with sarcopenia. J Am Med Dir Assoc. (2017) 18:637.e1–637.e11. doi: 10.1016/j.jamda.2017.04.016
63. Limpawattana P, Putraveephong S, Inthasuwan P, Boonsawat W, Theerakulpisut D, Chindaprasirt J. Frailty syndrome in ambulatory patients with COPD. Int J Chron Obstruct Pulmon Dis. (2017) 12:1193–8. doi: 10.2147/copd.S134233
64. de Blasio F, Di Gregorio A, de Blasio F, Bianco A, Bellofiore B, Scalfi L. Malnutrition and sarcopenia assessment in patients with chronic obstructive pulmonary disease according to international diagnostic criteria, and evaluation of raw BIA variables. Respir Med. (2018) 134:1–5. doi: 10.1016/j.rmed.2017.11.006
65. Munhoz da Rocha Lemos Costa T, Costa F, Jonasson T, Moreira C, Boguszewski C, Borba V. Body composition and sarcopenia in patients with chronic obstructive pulmonary disease. Endocrine. (2018) 60:95–102. doi: 10.1007/s12020-018-1533-4
66. Lee D, Jin H, Shin K, Chung J, Lee H, Lee K. Presence of sarcopenia in asthma-COPD overlap syndrome may be a risk factor for decreased bone-mineral density, unlike asthma: Korean national health and nutrition examination survey (KNHANES) IV and V (2008-2011). Int J Chron Obstruct Pulmon Dis. (2017) 12:2355–62. doi: 10.2147/copd.S138497
67. Lian J. Analysis of risk factors and abnormal bone metabolism in patients with chronic obstructive pulmonary disease complicated with sarcopenia. J Jinzhou Med Univ. (2018) 12:59–73.
68. Chi Y. The influence and correlation analysis of sarcopenia on pulmonary function and blood gas analysis in patients with COPD. J Jilin Univ. (2020) 10:34–58.
69. Shi M, Lv C, Zhao F, Li H, Li Y, Ren X. The effect of stable COPD patients with sarcopenia on their quality of life. Food Nutr China. (2020) 26:69–73. doi: 10.19870/j.cnki.11-3716/ts.20200716.008
70. Xu J, Wu H, Da J, Li F, Ni J. The effect of stable COPD patients with sarcopenia on their quality of life. Chin Prev Med. (2022) 23:146–9. doi: 10.16506/j.1009-6639.2022.02.012
71. Zhang J, Sheng Y, Chang X, Xie C, Zhang R, Li Z, et al. Sarcopenia and its risk factors in elderly patients with chronic obstructive pulmonary disease. Chin J Integr Tradit Western Med. (2021) 41:1042–8.
72. Hu, L, Zhao X, Huang X. Nutritional status of older patients with chronic obstructive pulmonary disaease and the correlation with sarcopenia. Int. J. Geriatr. (2021) 42:31–5.
73. Ju Y, Wang H, Li G, Wang D, Li Y. Analysis of nutritional metabolism phenotype in patients with stable chronic obstructive pulmonary disease. Chin J Clin Healthc. (2021) 24:599–605.
74. Chen L, Liu L, Woo J, Assantachai P, Auyeung T, Bahyah K, et al. Sarcopenia in Asia: consensus report of the Asian working group for sarcopenia. J Am Med Dir Assoc. (2014) 15:95–101. doi: 10.1016/j.jamda.2013.11.025
75. Cruz-Jentoft A, Baeyens J, Bauer J, Boirie Y, Cederholm T, Landi F, et al. Sarcopenia: European consensus on definition and diagnosis: report of the European working group on sarcopenia in older people. Age Ageing. (2010) 39:412–23. doi: 10.1093/ageing/afq034
76. Li S, Chen C, Chen Y, Yen Y, Fang W, Tsai F, et al. Upregulation of CISD2 augments ROS homeostasis and contributes to tumorigenesis and poor prognosis of lung adenocarcinoma. Sci Rep. (2017) 7:11893. doi: 10.1038/s41598-017-12131-x
77. Kapchinsky S, Vuda M, Miguez K, Elkrief D, de Souza A, Baglole C, et al. Smoke-induced neuromuscular junction degeneration precedes the fibre type shift and atrophy in chronic obstructive pulmonary disease. J Physiol. (2018) 596:2865–81. doi: 10.1113/jp275558
78. Choo Y, Chang M. Prevalence of sarcopenia among the elderly in Korea: a meta-analysis. J Prev Med Public Health. (2021) 54:96–102. doi: 10.3961/jpmph.21.046
79. Connor N, Russell J, Wang H, Jackson M, Mann L, Kluender K. Effect of tongue exercise on protrusive force and muscle fiber area in aging rats. J Speech Lang Hear Res. (2009) 52:732–44. doi: 10.1044/1092-4388(2008/08-0105)
80. Iyer S, Hsia R, Folker E, Lovering R. Age-dependent changes in nuclear-cytoplasmic signaling in skeletal muscle. Exp Gerontol. (2021) 150:111338. doi: 10.1016/j.exger.2021.111338
81. Martin-Rincon M, Pérez-López A, Morales-Alamo D, Perez-Suarez I, de Pablos-Velasco P, Perez-Valera M, et al. Exercise mitigates the loss of muscle mass by attenuating the activation of autophagy during severe energy deficit. Nutrients. (2019) 11:2824. doi: 10.3390/nu11112824
82. Rai R, Ghosh A, Eren M, Mackie A, Levine D, Kim S, et al. Downregulation of the apelinergic axis accelerates aging, whereas its systemic restoration improves the mammalian healthspan. Cell Rep. (2017) 21:1471–80. doi: 10.1016/j.celrep.2017.10.057
83. Romanello V. The interplay between mitochondrial morphology and myomitokines in aging sarcopenia. Int J Mol Sci. (2020) 22:91. doi: 10.3390/ijms22010091
84. Mayhew A, Amog K, Phillips S, Parise G, McNicholas P, de Souza R, et al. The prevalence of sarcopenia in community-dwelling older adults, an exploration of differences between studies and within definitions: a systematic review and meta-analyses. Age Ageing. (2019) 48:48–56. doi: 10.1093/ageing/afy106
85. Kim J, Oh S, Park H, Jun J, Kim H. Comparisons of different indices of low muscle mass in relationship with cardiometabolic disorder. Sci Rep. (2019) 9:609. doi: 10.1038/s41598-018-37347-3
86. Baumgartner R, Koehler K, Gallagher D, Romero L, Heymsfield S, Ross R, et al. Epidemiology of sarcopenia among the elderly in New Mexico. Am J Epidemiol. (1998) 147:755–63. doi: 10.1093/oxfordjournals.aje.a009520
87. Newman A, Kupelian V, Visser M, Simonsick E, Goodpaster B, Nevitt M, et al. Sarcopenia: alternative definitions and associations with lower extremity function. J Am Geriatr Soc. (2003) 51:1602–9. doi: 10.1046/j.1532-5415.2003.51534.x
88. Crapo R, Casaburi R, Coates A, Enright P, Hankinson J, Irvin C, et al. Guidelines for methacholine and exercise challenge testing-1999. This official statement of the American thoracic society was adopted by the ATS board of directors, July 1999. Am J Respir Crit Care Med. (2000) 161:309–29. doi: 10.1164/ajrccm.161.1.ats11-99
89. Leivseth L, Brumpton B, Nilsen T, Mai X, Johnsen R, Langhammer A. GOLD classifications and mortality in chronic obstructive pulmonary disease: the HUNT study, Norway. Thorax. (2013) 68:914–21. doi: 10.1136/thoraxjnl-2013-203270
90. Gao Y, Shen L, Zhan R, Wang X, Chen H, Shen X. Association of lung function with functional limitation in older adults: a cross-sectional study. PLoS One. (2021) 16:e0253606. doi: 10.1371/journal.pone.0253606
91. Wang S, Xie H, Gong Y, Kuang J, Yan L, Ruan G, et al. The value of L3 skeletal muscle index in evaluating preoperative nutritional risk and long-term prognosis in colorectal cancer patients. Sci Rep. (2020) 10:8153. doi: 10.1038/s41598-020-65091-0
92. Rong Y, Bian A, Hu H, Ma Y, Zhou X. Study on relationship between elderly sarcopenia and inflammatory cytokine IL-6, anti-inflammatory cytokine IL-10. BMC Geriatr. (2018) 18:308. doi: 10.1186/s12877-018-1007-9
93. Ding W, Chen X, Li W, Fu Z, Shi J. Genistein Protects genioglossus myoblast against hypoxia-induced injury through PI3K-Akt and ERK MAPK pathways. Sci Rep. (2017) 7:5085. doi: 10.1038/s41598-017-03484-4
94. Huang Y, Zhu X, Chen K, Lang H, Zhang Y, Hou P, et al. Resveratrol prevents sarcopenic obesity by reversing mitochondrial dysfunction and oxidative stress via the PKA/LKB1/AMPK pathway. Aging (Albany NY). (2019) 11:2217–40. doi: 10.18632/aging.101910
95. Al-Attar A, Zari T. Influences of crude extract of tea leaves, Camellia sinensis, on streptozotocin diabetic male albino mice. Saudi J Biol Sci. (2010) 17:295–301. doi: 10.1016/j.sjbs.2010.05.007
96. Pan L, Ke J, Zhao C, Huang S, Shen J, Jiang X, et al. Electrical stimulation improves rat muscle dysfunction caused by chronic intermittent hypoxia-hypercapnia via regulation of miRNA-related signaling pathways. PLoS One. (2016) 11:e0152525. doi: 10.1371/journal.pone.0152525
Keywords: sarcopenia, chronic obstructive pulmonary disease, meta-analysis, prevalence, lung function
Citation: He J, Li H, Yao J and Wang Y (2023) Prevalence of sarcopenia in patients with COPD through different musculature measurements: An updated meta-analysis and meta-regression. Front. Nutr. 10:1137371. doi: 10.3389/fnut.2023.1137371
Received: 04 January 2023; Accepted: 31 January 2023;
Published: 16 February 2023.
Edited by:
Tomoko Suzuki, Saitama Medical University, JapanReviewed by:
Corina-Aurelia Zugravu, Carol Davila University of Medicine and Pharmacy, RomaniaCopyright © 2023 He, Li, Yao and Wang. This is an open-access article distributed under the terms of the Creative Commons Attribution License (CC BY). The use, distribution or reproduction in other forums is permitted, provided the original author(s) and the copyright owner(s) are credited and that the original publication in this journal is cited, in accordance with accepted academic practice. No use, distribution or reproduction is permitted which does not comply with these terms.
*Correspondence: Jie He, MTM1NDAyNDY5NzRAMTYzLmNvbQ==
†These authors have contributed equally to this work
Disclaimer: All claims expressed in this article are solely those of the authors and do not necessarily represent those of their affiliated organizations, or those of the publisher, the editors and the reviewers. Any product that may be evaluated in this article or claim that may be made by its manufacturer is not guaranteed or endorsed by the publisher.
Research integrity at Frontiers
Learn more about the work of our research integrity team to safeguard the quality of each article we publish.