- 1Division of Agrotechnology, Council of Scientific and Industrial Research–Institute of Himalayan Bioresource Technology, Palampur, Himachal Pradesh, India
- 2Division of Biotechnology, Council of Scientific and Industrial Research–Institute of Himalayan Bioresource Technology, Palampur, Himachal Pradesh, India
Nowadays, the human population is more concerned about their diet and very specific in choosing their food sources to ensure a healthy lifestyle and avoid diseases. So people are shifting to more smart nutritious food choices other than regular cereals and staple foods they have been eating for a long time. Pseudocereals, especially, amaranth and quinoa, are important alternatives to traditional cereals due to comparatively higher nutrition, essential minerals, amino acids, and zero gluten. Both Amaranchaceae crops are low-input demanding and hardy plants tolerant to stress, drought, and salinity conditions. Thus, these crops may benefit developing countries that follow subsistence agriculture and have limited farming resources. However, these are underutilized orphan crops, and the efforts to improve them by reducing their saponin content remain ignored for a long time. Furthermore, these crops have very rich variability, but the progress of their genetic gain for getting high-yielding genotypes is slow. Realizing problems in traditional cereals and opting for crop diversification to tackle climate change, research should be focused on the genetic improvement for low saponin, nutritionally rich, tolerant to biotic and abiotic stresses, location-specific photoperiod, and high yielding varietal development of amaranth and quinoa to expand their commercial cultivation. The latest technologies that can accelerate the breeding to improve yield and quality in these crops are much behind and slower than the already established major crops of the world. We could learn from past mistakes and utilize the latest trends such as CRISPR/Cas, TILLING, and RNA interference (RNAi) technology to improve these pseudocereals genetically. Hence, the study reviewed important nutrition quality traits, morphological descriptors, their breeding behavior, available genetic resources, and breeding approaches for these crops to shed light on future breeding strategies to develop superior genotypes.
1. Introduction
Crops that store carbohydrates in the perisperm are called Pseudocereals, unlike true cereals, where carbohydrates are stored in the endosperm and both are sources of energy in the human diet (1). These days with the health-conscious human population, people are more interested in healthier diet opportunities and more focused on micronutrient supply to their food other than usual food sources through major cereal crops. With the increasing trend to add more nutrition to the diet, the rise in demand for pseudocereals, grain amaranth, and quinoa's popularity is increasing daily in the market, resulting in their increasing cultivation (2, 3). Therefore, to meet the demand, there is a need for genetic improvement of these crops. Amaranth and quinoa are gaining space in the markets among other grains such as Buckwheat, Chia, and Wattle seeds due to their high nutritional qualities (4).
The grain amaranth (Amaranthus spp.) is valued as leaf vegetables, nutritional grain, and ornamentals by people worldwide. The “Amaranth” is a Greek word called “everlasting” as it is hardy; thus, it is famous for cultivation. It is the main staple food in many parts of the world such as Mexico, and it is consumed as a multipurpose crop in the form of grain and leaves, and in many places as forage for livestock using some amaranths (5). It is also used for natural dyes, as lubricants, and in the pharmaceutical industry. Amaranth flour is gluten-free, so it is a good food source for people with gluten allergies. Amaranth leaves are anti-cancerous, it stops the irregular growth of cancer cells in the breast, colon, and liver, thus are also suitable for cancer patients (6). In addition, many products are famous in the market made from amaranth grains (7). Among dicotyledonous plants, amaranth is one example that uses the C4 pathway for photosynthesis and could be a model plant in genetic regulation studies for photosynthesis (8). Amaranth is resistant to all abiotic and biotic stresses, including stress, drought, and insect-pest attacks, and needs less care, thus easy to cultivate in a wide range of agro-climatic regions (9). It is thought of as a saprophytic halophyte and is tolerant to high salinity; thus, it is suited for subsistence agriculture (9).
Another one, quinoa (Chenopodium quinoa Willd.) is a nutritious grain modern crop and old enough to have originated from the Andean area of South America (10). It was cultivated in earlier times in countries such as the Andean region, Bolivia, Peru, and Chile. About 7,000 years ago, the report of the first domestication was from Lake Titicaca, from where it spread further from South America and to around the world (11, 12). In Chile, cultivation of the quinoa plant began as early as 5,000 BC. Presently, about 250 different Chenopodium species are known all over the world (13). Since then, it has been a major staple grain for the Inca civilization, known in their native Quechua language as “chisiya mama” or “Mother Grain.” It is categorized either on the plant's color, shape, and fruit (14, 15). In the Andean region, quinoa has various names within the same community; it was famous for names such as kinua, quinhua, and jupha in the Aymara language, depending on the diversity in the color of its grains. Quinoa grows well in poor soil of rainfed conditions, has superior adaptability to varied agro-climatic conditions, and has unrealized commercial potential in India (16, 17). Quinoa's exceptional adaptability to various agro-ecological zones can be grown in all regions (2, 18) with water scarcity, from hot to dry deserts and in arid, semi-arid, and even tropical locations with humidity up to 88% and temperatures ranging from 8 to 40°C (19). It can also grow on varied topography, both in plains or high mountainous regions up to sea level (4,000 m), and thus it is a significant crop from an agricultural point of view to grow in a wide range of regions (18). Numerous European nations took part in the 1993-approved initiative titled “Quinoa-A Multipurpose Crop” which was most popular to be used for “Agricultural Diversification” (20). Quinoa is super famous these days in modern supermarkets due to its super nutritional quality of grains and leaves and is available as processed products in fancy packaging to attract customers as an alternate food to regular sources of carbohydrates instead of major cereals in the modern diet.
Food security is a major concern for future generations. These crops can be grown in most countries' environmental conditions and are easily available plant sources of micronutrients to alleviate micronutrient malnutrition (21). There is a need for their genetic improvement and to explore the breeding behavior of these crops, possibilities to create variations in population, and the use of molecular markers to study diversity in amaranth and quinoa (22–24) (Table 3). We have reviewed genetic mapping studies and DNA barcoding to understand the gene sequence of these crops (Table 3). Gene mapping studies could help us target those particular genes to express desirable traits such as high nutritional and low antinutritional factors that can improve the genetics of both crops. Thus, the current review was undertaken to compile all the breeding studies, available germplasm, and other genetic resources to accelerate the process of genetic improvement in these crops to create varieties with low antinutrient factors and tolerance to biotic and abiotic stresses. The genetics of qualitative and quantitative characters would help breeders to adopt better breeding methods (25, 26).
2. Nutritional and nutraceutical qualities
Many reviews about the nutritional benefits of amaranth and quinoa showed higher protein content, higher fiber, low saturated fat, and balanced amino acid composition of their seeds than other major cereals (27, 28) (Tables 1, 2; Figure 1). The grain amaranth has many essential micronutrients such as calcium, magnesium, iron, vitamin C, β carotene, and folic acid (54, 55). The young leaves of amaranth are consumed (56, 57). The amaranth grain has great nutraceutical value and thus is known to be a new-millennium crop (58). Ripen seeds of amaranth are very famous in hills. The grain amaranths seed is high in crude protein (22.5%), dry fiber matter (8%), and high lysine content (0.73–0.84%) more than maize (3–3.5 times) and wheat (2–2.5 times) (36, 59). The amaranth seeds have mainly high methionine and lysine contents and high levels of squalene which play a precursor for all steroids. The most studied nutritional aspect concerning the food value of grain amaranth is the identification of the limiting amino acids of the protein component. Out of 20, 17 amino acids such as isoleucine, leucine, lysine, cysteine, phenylalanine, tyrosine, threonine, methionine, valine, alanine, arginine, glutamic acid, aspartic acid, glycine, histidine, proline, and serine are present in amaranth (28).
The unique gel characteristics of amaranth starch are also the reason for the increasing demand for amaranth (60). The starch granules in grain amaranth are polygonal, having a diameter (1–3 μm) and a very high swelling power (61). Waxy and non-waxy starch granules were present in amaranth (62). Amaranth germ contains 6%−10% of oil (31, 33, 63), mostly unsaturated oil (76%) having high linoleic acid, which is necessary for the human diet. Amaranth is a good source of energy food as it contains high protein and high-fat content, thus is a potential source of high calories. Milled and toasted amaranth products are perfect for digestion and absorption (64). Only high-protein rice is considered to satisfy protein and energy needs than other cereals (28, 65). The main constituent, amarantin substance (C29H31N2O19), is alkaloids-betalains, present in amaranths derived from grain species of amaranth (Amaranthus caudatus L., Amaranthus tricolor L., and Amaranthus cruentus L.) and are also used as medicine (66) and as food colorants (67, 68) having antioxidant properties (69).
Amaranth has many antinutritional factors which make it less popular to be used as major food grains. It has phytates, phenolic compounds, trypsins inhibitors, chymotrypsin inhibitors, and amylase inhibitors as unwanted constituents which need to be reduced by processing or by developing varieties having less amount of these factors (70). The phytates levels (0.52%−0.61%) were higher than in rice but much less than in corn and wheat, and tannins levels (0.043%−0.116% catechin equivalents) were much lesser than in sorghum and millet (71). Tender leaves from young Amaranth plants are consumed in Mexico and Kenya and used as an ingredient in common meals.
Amaranthus dubius, Amaranthus viridis, and A. tricolor leaves are known for their medicinal and nutraceutical properties (72). Amaranth has active ingredients that act as phytochemical compounds from its leaves (73, 74). These active components are tannins, saponins, phenols, flavonoids, cardiac glycosides, steroids, and triterpenoids (75). These chemicals have antipyretic, anti-inflammatory, antihepatotoxic, antiulcer antiallergic, and antiviral activity (75). In traditional medicines, it is used to reduce labor pain (75). In Spain, Amaranthus leaves and root paste is used directly on the skin to cure eczema, psoriasis, gonorrhea, menorrhagia, bruises, burns, and rashes (76). It is also used to cure urinary tract diseases, treat intestinal worms, and gastric ulcers, and as a laxative to improve digestion problems. It is good to improve appetite. It is also good for treating eye infections and breathing-related problems like asthma (75). Amaranth leaves are used as protective food due to their curative properties having strong antioxidant and phytochemical compounds present in them (73, 77, 78). If boiled leaves are given to patients suffering from jaundice, rheumatic pains, and stomach aches, it works wonders to cure them. The paste of the root is also possessing several beneficial effects when used internally and externally. The paste of roots of Amaranth controls vomiting and is thus good for dysentery patients, and the paste with black pepper is given to rabies patients (79). Its seeds can be consumed directly to stop internal bleeding, excessive menstruation, and diarrhea. It also works externally as a poultice for broken bones. The whole plant of Amaranth is also helpful in treating cholera, piles, and snake bites (80).
For centuries, Chenopodium spp. has been cultivated by human populations in a few parts of the world, among them few species were used as leafy vegetables (Chenopodium album) and a few species as grain crops (C. quinoa and C. album). It is good for the human diet due to its high protein content (14.1%) with high lysine (5.1%−6.4%) and methionine (0.4%−1.0%) contents (30, 81). Amaranthus caudatus is also used as animal feed. Quinoa has high fiber (7.0%), vitamins (thiamine and niacin), and minerals such as phosphorus and potassium (82–85). Due to layers of calcium oxalate on leaves, quinoa plants can tolerate droughts. As such grains cannot be consumed due to the unpleasant taste of their grains containing saponin (a glycoside), but nowadays, some types of grains are selected that no longer have that flavor (86).
Quinoa can be used instead of rice, as a hot breakfast cereal or for making baby cereal by boiling it in water. Even popping the seeds like popcorn is an option. Seeds can be sprouted or processed and used as flour. Sprouts must turn green before being put into salads (84). Ancient populations discovered the excellent nutritional content of quinoa, called it “golden grain,” and considered it an auspicious food on good occasions (87). The protein in pseudo cereals, such as quinoa, is mostly located in the endosperm. Albumin and globulins make up around 44%−77% of the protein fraction, whereas prolamins, a group of proteins associated with gluten, make up just a very small percentage of the protein fraction (0.5%−7.0%) or are completely absent in other kinds (88). Quinoa is rich in phosphorus which is five times more than cow milk and rice (89). Value additions in breads and snack food such as oats use quinoa. It may be either consumed as whole flour directly or mixed with other cereals like rice or in other recipes in combinations. Its beer can also be produced by a fermentation process similar to malt (90). Chenopodium quinoa is a rich source of all goodies such as minerals (calcium, magnesium, iron, phosphorus, potassium, manganese, zinc, copper, and sodium), high fiber, vitamins E and C, and vitamin B complex [such as thiamine (B1), riboflavin (B2), niacin (B3), and folic acid (B9)]. Quinoa has more nutrition than other traditional cereals such as barley, maize, rice, and wheat (14, 91–94).
The consumption of quinoa as a grain is less due to the presence of the antinutritional factor saponin in it that is present in the seed coat to protect the plant from the attack of insects and pests, but it needs to be removed before consumption (95, 96). To make quinoa worldwide staple food, we need to work on quinoa processing to reduce the saponin content of the quinoa seeds (97–101). Improved varieties had been developed called “Sweet” varieties with less saponin content but are less protected from insects, pests, and certain herbivores attack (102, 103). Many other factors such as phytic acids, tannins, and protease inhibitors are also present in quinoa. Phytic acids are present in the outer layers and the endosperm of quinoa seeds. Phytic acid binds minerals, thus it reduces the absorption of minerals in our body.
Quinoa has saponin, which acts as anticarcinogenic and hypocholesterolemic and is useful for health (49, 104). Polyphenols are present in quinoa and there are three main polyphenols (flavonoids, phenolic acids, and tannins) which are the reason for bitterness, astringency, color, taste, and oxidative stability (105–107) and also act as an antioxidant thus preventing cardiovascular diseases (39), anti-allergic, anti-inflammatory, antiviral, and anticarcinogenic. In ancient times, black quinoa was mixed with alcohol and applied to the wounded area. Quinoa grains are used by patients suffering from muscle sprains, twists, and muscular strains (108).
The domestication of amaranth declined at the time of Spanish arrival, but the reasons for the decline of this crop are unclear (109). Many myths explained the decrease in grain amaranth cultivation. Maize coevolved with amaranth, but now maize is a major cereal crop and this crop remains underrated despite its more health benefits, and it is a more nutritionally valuable food for the human population. The size of the seeds of amaranth may be the reason for the reduction in cultivation of this crop as compared to maize. Small-seeded crops require more care in handling than larger-seeded crops. The cultivation of amaranth becomes a part of small plots only in Mexico, the Andean region, and a few areas of India, Bhutan, and Nepal (110). Currently, it is cultivated throughout Asian countries such as China, Bhutan, India, Indonesia, Nepal, Malaysia, Philippines, Central America, Mexico, and Southern and Eastern Africa. Another reason might be the beauty of amaranth leaves which prevent this crop from disappearing from the world as it was always part of the garden in rural areas and is fascinating and difficult to ignore. America leads in quinoa production. Due to crop diversification, quinoa production increased by 72% in Peru, Bolivia, and Ecuador (84).
3. Plant descriptors
3.1. Amaranth
Amaranth is a non-grass annual plant, having an herbaceous stem with height varying from 30 to 210 cm, a solid stem of varying colors, petioled morphology, ovate in shape, hairy or non-hairy with wavy margins and alternate pattern of length (7.5–15 cm), and different colored leaves. The size of the flower varies from 1 to 2 cm in different colors from red to maroon. The seeds are oval in shape with colors, white, red, and black, that germinate at high humidity (111). Amaranth seeds have folded flange, reticulation cartridges over spermoderm, and the presence of verucate processes, and fruits have dehiscent pyxis (112). It is having taproot system which is long, fleshy red or pink, deep, and developed, thus it stands in water stress conditions. Amaranth is monoecious, with few dioecious species. Pollination occurs by wind or by insects. The color of amaranth leaves varies from bright red to violet, and its maroon color is due to battalions pigments (6). Its inflorescences are 0.50–0.90 m long and glomerulated (Figure 2), erect or inclined, and yellowish, reddish, or purple in color.
The phyllotaxy and vascular supply study was carried out in A. caudatus (113), Amaranthus graecizans L., and Amaranthus hybridus L. (114), and in other common species by Costea and Demason (115). Cultivated grain amaranth (A. caudatus, A. cruentus, and Amaranthus hypochondriacus) from their wild ancestors were studied for segregation within the “hybridus complex” with characters related to morphology, phyllotaxy of leaves, epidermal characters of leaves, vascular supply studies, and secondary growth in plants.
Amaranthus's pollen grains are pantoporate with more than 18 sunken pores and tectate with granules and spinules (116, 117). Similar type pollens were present in the other members of Amaranthaceae (118) and several other Centrospermous families (119). Amaranth has different ploidy levels and interspecific hybrids determined by applying pollen grain features. In dioecious species, pollen grains have multiple apertures on the surface (120). The size of pollen grains is dependent on the ploidy level of the plant. The lower ploidy level has a smaller size, size increases with an increase in the ploidy level. In polyploids, the exine shows more patterns than in diploids (121).
3.2. Quinoa
Quinoa is a dicotyledonous annual plant belonging to the Amaranthaceae family. The plant grows well in India, with several varieties having a height of 1.5 m, with a number of branches, and a large leaf size (122). There is a well-developed ramified tap root system protecting against drought conditions (123). The seeds are round and flat, have a seed diameter of 1.5–4.0 mm, and take a variety of forms from white to gray and black, with shades of yellow, rose, red, purple, and violet. Unisexual female flowers are a significant characteristic of quinoa (124, 125). Quinoa has three panical shapes: amarantiform, intermediate, and glomerulate; amarantiform ones have elongated glomeruli growing from the secondary axis, while the others have spherical glomeruli growing from the tertiary axis and sometimes a plant will display both traits, producing an intermediate inflorescence (126). Gandarillas (127) also noted that a dominant allele influences the glomerulated panicle feature. The ovary is superior having two or three stigmas, five perianth lobes, and five anthers. Some cultivars exhibit partial or complete male sterility in the female flowers. According to Risi and Galwey (128), the fruit (achene type) can be conical, cylindrical, or ellipsoidal with three layers present: perigonium, pericarp, and episperm, with saponins present in the pericarp. Seeds vary in size and color, with black dominance over both colors and red dominance over white (128). Quinoa has shown good tolerance to varied temperatures in both temperate and tropical regions. Prego et al. (129) found that quinoa seeds' perisperm, embryo, and endosperm contain nutritional reserves. In some places, such as the Andean highlands, planting season lasts from December to January; in others, it starts in August and lasts until December. Where mechanized agriculture techniques are utilized, quinoa is planted in row spacing (40–80 cm) (84).
4. Genetic resources
4.1. Amaranth
Amaranth has a basic chromosome number (x = 8 or 9) (130) and is allotetraploid with chromosome number n = 16 or 17. The first domesticated species (A. cruentus) originated from A. hybridus in Central America; then, A. hypochondriacus by recurrent crossing between A. cruentus and A. powellii in Mexico and the domestication of A. caudatus by crossing between A. cruentus and Amaranthus quitensis. These three grain Amaranthus species along with A. hybridus formed a complex or aggregate (“hybridus complex”) structure.
Amaranth is an ancient crop belonging to the Amaranthaceae family, Caryophyllales order, and Amaranthoideae subfamily. The genus Amaranthus has ~87 species and out of them 17 are from Europe, 14 are from Australia, and 56 are from America (87, 131, 132). Moreover, out of 87 identified species of amaranth, 17 species are for vegetative purposes, and mainly three for grain purposes, namely, A. caudatus, A. cruentus, and A. hypochondriacus (133), and the others are widely dispersed weeds. All these grain amaranths are famous for their magnificent appearance and are popularly known by a few names, such as A. hypochondriacus, famous as prince's feather, A. cruentus as purple amaranth, A. caudatus as love-lies-bleeding, grown more as an ornamental, and A. tricolor as tampala, grown mostly for the leaves. Among them, A. caudatus is mainly a tropical plant species. Other vegetable species are A. dubius, Amaranthus blitum, and A. cruentus; weed species are Amaranthus retroflexus (as redroot pigweed), Amaranthus albus (and also tumbleweed), and Amaranthus spinosus (due to spiny leaves as spiny amaranth). Amaranth species within themselves have fewer genetic differences, thus different species can do easy crossing over and even wild types will cross with domesticated varieties if not timely rogued from the field (83).
Amaranthus cruentus accessions (“African” grain type) have seeds of dark colors which branched heavenly belonging to West Africa and have inflorescence which matures early. The other accession, A. hybridus L. (“Prima” grain type), is also a dark-seeded, highly branched, short plant stature belonging to Asia. Few barriers were reported to prevent inter-specific hybridization between crosses of A. cruentus L., A. hypochondriacus L., and A. hybridus L. Like, whenever hybridization of A. caudatus L. and with any grain type was carried out, this often results in non-viable seed formation (134). Adaptations and migration patterns were predicted for all amaranth species and showed potential for adaptation to diverse climate regions because it showed a wider adaptation rate than other wild and cultivated species (135). About 121 different crops and wild individuals of amaranth were studied through a relative genetic map and analyzed that grain amaranth was domesticated from a single wild ancestor (136).
The germplasm of Amaranth is widely spread across the globe including the USA, India, and Peru. In the USA, they are grown for healthy diet food while in countries such as Peru, Bolivia, India, and Mexico, it is a traditional food that lost its identity after the introduction of new world cereals such as wheat and rice (132). The USA germplasm collection has a conservatory of 3,300 accessions of A. hypochondriacus from 40 different countries (137). In India at the National Bureau of Plant Genetic Resources (NBPGR), there is a collection of 3,081 accessions (138) and the National Botanical Research Institute (NBRI) has 2,500 accessions (139) of A. hypochondriacus. In Peru, the Univ. Nacional San AntonioAbad del Cusco (UNSAAC/CICA) has 740 accessions of A. caudatus (140). Analysis of 20 accessions of amaranth species (Amaranthus L.) provides evidence that wild accessions from Central Malawi have more nutrients, minerals, and vitamins than those domesticated from other agro-ecological regions (141). Amaranth species accessions were assessed by using simple sequence repeat markers and genetic variability was also present (142), thus it shows the scope of variations for future breeding programs for developing a rich germplasm pool of amaranth crop. Genotypes were grouped into 10 clusters which can be selected for the hybridization program as parents, as those analyzed accessions showed variability for improvement in crop (143). Ninety-eight genotypes were analyzed for 14 characters of grain amaranth (A. hypochondriacus L.) for studying the relationship between genetic divergence and eco-geographical region. However, no significant results were obtained among clustering patterns (144). These clusters could be used in a hybridization program to select good parents having maximum variability and select transgressive segregants from the population developed to increase the yield. Phenotypic characterization of amaranth genotypes based on biomass yield and related traits laid the future for trait-focused breeding programs (145). Many species of amaranth were studied for the complete chloroplast genome sequences using simple sequence repeats by Chaney et al. (146). SNPs and indels proved to be great genetic resources for studying phylogeny from diverse genetic diversity. From three grain species of amaranth, 37 accessions from Nigeria were studied, and after evaluation, clusters were made (147) and concluded a great scope of genetic diversity to improve yield parameters through breeding. From 20 Amaranthus species, 229 genotypes were evaluated for diversity among genotypes for the improvement of high-yielding cultivars according to the origin and the preferred area of production (148). Thirty-two Amaranthus species were evaluated for 16 traits for the morphological characterization of genetic resources for breeding purposes (149); 13 genotypes of different species of A. hypochondriacus and A. tricolor were grouped into two major clusters, to differentiate between ornamental and edible (150). Two selected mutant lines developed through gamma radiation treatment of A. cruentus L. were evaluated (151), and treated plants showed significantly higher seed yield and seed weight than non-treated plants.
4.2. Quinoa
There are many ex situ conservatories for quinoa in gene banks that use seed properties to conserve germplasm. All over the world, 30 countries conserve quinoa in 59 gene banks. The ex situ conservatories of Chenopodium have 16,263 accessions preserved in the world, originated and thus maintained in the Andean Region (mainly in Bolivia and Peru) (152). The different countries that contribute to quinoa germplasm conservation are America having 10 countries associated with it, Europe having 11 countries, Africa having five countries, and Asia having three countries. Bolivia and Peru have the largest diverse regions of all. In Bolivia, there are 6,721 quinoa accessions, Peru has 6,302 accessions, Argentina has 492 accessions, Ecuador has 673 accessions, Chile has 286 accessions, and Colombia has 28 accessions. These conservatories are run by different institutes all over the world, such as INIAF (Instituto Nacional de Innovación Agropecuaria y Forestal—National Institute of Agricultural and Forestry Innovation), UMSA (Universidad Mayor de San Andrés—Major University of San Andrés), UTO (Universidad Técnica de Oruro—Oruro Technical University), UCB (Universidad Católica Boliviana—Bolivian Catholic University), UPEA, and in the Centro de Investigación y Promoción Comunal (Municipal Research and Promotion Center—CIPROCOM) (153). Seed collections conservatories are also in South America at Universidad Nacional del Altiplano (UNAP, Peru), the National Institute of Agricultural Research (INIA, Peru), the Research Center for Andean Studies (CICA, Peru), the National Seed Bank of Chile, Royal Botanical Gardens Kew (UK), the USDA-ARS (USA), the National Bureau of Plant Genetic Resources (India), and IPK-Gatersleben (Germany) (154). Wild Chenopodium species have 357 accessions from USDA-ARS and the Royal Botanical Gardens Kew (132). Quinoa has two distinct germplasm pools: Andean highland quinoa, which is the primary germplasm, and central and southern Chilean quinoa, the second germplasm center (155), and Argentina of C. hircinum, the third germplasm pool, which are major areas from South America (156). The classification is done based on different agro-morphological variables such as growth habits (four growth habits), plant color (panicle emergence or start of flowering), panicle shape and density (amarantiform or glomerulate or intermediate), grain color and shape (white, cream, yellow, orange, pink, red, purple, light coffee, greenish coffee, or black), grain size (ranges from 1.36 to 2.66 mm), crop cycle (physiological maturity within 119–220 days), and grain yield (13).
5. Breeding behavior and approaches
5.1. Genetic improvement of amaranth
Amaranth is a self-pollinating crop, with few percentages of cross-pollination (157). Amaranth is monoecious in nature. Homozygous, true-to-type lines were maintained by repeated self-pollination and within selection for 6–8 generations and further used for hybrid production. For hybridization, male and female parents are planted at a proper isolation distance. Emasculation procedures and controlled pollination were performed and developed (158, 159). Chromosomal studies were carried out (160, 161) and polyploids were studied (162). Indian germplasm showed a lot of diversity as documented by researchers (163). The mode of inheritance of traits was studied in amaranth crop and inheritance was studied for different characters (164, 165), including yield parameters (166). Parameters such as harvest index and grain yield were studied (167).
Inheritance studies were important for improved hybrid production for selecting important characters which are significant to be carried out further. Gene markers are the easiest approach to studying the inheritance of desirable traits for developing good varieties. Markers for a few parameters such as plant growth, plant morphology, seed characteristics, and flowering behavior were identified in the past, and their inheritance was studied thoroughly (168–170). Inheritance of nutritional facts, including starch characteristics and perisperm layer of grain, was studied in Japan (62, 171, 172). Studies were also carried out in India about seed protein content and their inheritance (173). Inheritance of male sterility was investigated and used for hybridization (174, 175).
Good hybrids are produced by selecting germplasm which is rich in desirable characteristics. Hybrids' performance depends only on carefully selecting good recombinants from the present gene pool and combining them intelligently with each other. Selection is made according to environmental adaptations and beautiful vigorous vegetative features with respect to growth and yield characteristics. The environment affects the expression of many traits such as plant height, days to maturity, and plant habit. Interspecific hybridization is carried out successfully between a few species, and crosses between the species A. cruetus L., A. hypochondriacus L., and A. hybridus L. produce viable offspring (134). Few interspecific hybrids were successful on a commercial scale also. In the United States, a large area was under the cross of A. hypochondriacus and A. hybridus; the amaranth grain production guide was developed to study agronomical methods to grow these hybrids (176). Genetic stability has been achieved for the development of improved lines by interspecific hybridization. Amaranth plants have undergone improvements for many decades using hybridization, selection, and mutagenesis methods.
Genetic diversity research is essential for utilizing plant genetic resources for amaranth crops (8, 177). Amaranth genotypes also show evolution by the influence of the environment of specific agro-ecological regions (178). Introgression and hybridization between species showed great variability and phenotypic plasticity (179). Mass selection and pure selection methods were used to improve the amaranth germplasm by self-pollination and cross-pollination methods (169, 180, 181). Amaranthus hypochondriacus, A. caudatus, and A. cruentus species were cream-colored seeds generally used for grain purposes. These present-day species were domesticated from the wild black-seeded A. hybridus (132, 182). Studies had been conducted to study genetic diversity among Indian populations of amaranth (163). Different breeding strategies were used to study different parameters in amaranth (164–166).
The study of genetic control of trait(s) is a basic requirement for the purposeful management of genetic variability. Both additive and non-additive gene effects are recorded for different parameters in grain amaranth (167, 183). Multiplicative characters such as yield or panicles plant−1 are controlled either predominantly by non-additive or larger non-additive than additive components (167, 183). Research on heritability and genetic advances are reported to be mostly moderate to low for yield plant−1, panicles plant−1, panicle length, seed weight panicle−1, and test weight (184). Plant growth, grain harvest index, days to 50% flowering, and days to maturity were observed to exhibit largely moderate-to-high heritability and genetic advance. The maximum genetic gain would be difficult to realize by exercising selection on seed yield or panicle alone. Selection based on yield components with more weightage on panicles per plant and grain weight per panicle would be more beneficial (184). A recurrent selection program for the exploitation of both of these gene actions would be useful in grain amaranth as both additive and non-additive gene effects controlled agronomical traits. Additive gene variance and enhanced genetic recombination were used for the diallel selective mating system. Considerable heterosis has been recorded in grain amaranth. Pandey (167) reported heterosis over better parents to 71.36% for grain yield per plant. The manifestation of heterosis for grain yield per plant seemed to be primarily due to the heterotic response of panicle per plant. Sterile lines can be developed for the production of hybrids seed, but till now, no male sterile line is identified for the commercial scale for seed productions.
To reconstitute the plant for creating genetic variation, improving adaptability, imparting resistance, alleviating nutritional status, and developing ideal plant types, several attempts are being made taking the recourse of polyploidy and hybridization at the interspecific level. Autotetraploids are developed in several species/varieties of grain amaranth (134, 185). Pal and Khoshoo (134) raised autotetraploid in A. hypochondriacus var. AG-21 and with normal growth that produced bold seeds but suffered with the reduction of grain yield plant-1 perhaps due to the reduction in the number of seeds glomerule. Pal and Pandey (186) again induced autotetraploidy in several grain amaranth species but observed a similar trend. This indicates that polyploidy does not hold well in this crop but such variation can be used for further breeding programs. Scientists are trying to raise allopolyploids from species hybrids between the wild/weedy and cultivated types as well as at the intraspecific level (162, 186). Pal and Khoshoo (134), Khoshoo and Pal (162), Pal (187), Pal et al. (188), and Pal and Pandey (186) produced both wild and cultivated species of grain amaranth. These interspecific hybrids usually showed high variation in pollen and seed fertility. Some interspecific combinations suffered from sterility. But, in general, the hybrids were fertile and there was copious seed formation. Thus, the desirable features of wild/weedy species can be introduced into the edible types of grain amaranth. Future research can be focused on the development of embryo culture, anther culture, and gene transfer.
In recent years, the plant's chemical composition and agronomic properties have improved using biotechnological methods. Genetic engineering methods make it possible to improve not only the useful properties of a plant but also to provide additional useful characteristics during plant transformation. Different technologies such as Agrobacterium transformation and direct gene insertion by biolistic gene gun transfer methods insert genes with the desired gene. Genomic-assisted breeding (GAB) is very helpful to select potent parents with novel characters for breeding using hybridization and selection in future. Studies such as chloroplast gene sequencing, genetic mapping, and the use of biomarkers are useful to make easy the task of choice combinations to carry out breeding approaches. Chloroplast genome assembly of A. hypochondriacus (Amaranthaceae) was developed (146) to reconstruct the chloroplast genomes between two related grain species (A. cruentus and A. caudatus) and their putative progenitor (A. hybridus). Lightfoot et al. (189) identified a major QTL for flower color in A. hypochondriacus using linkage mapping. The physical map of the A. hypochondriacus genome has 16 chromosome-scale major scaffolds with an N50 of 24.4 Mb (189). Germplasm from A. hypochondriacus species (22) and SNP alleles were generated and a genome-wide association study (GWAS) was carried out for the qualitative traits between specific phenotypes and genetic variants within the genome and identified marker-trait associations (MTAs) on 16 amaranthus species. SNP markers were used to produce genetic resources for the phenotyping and development of cultivars. Genetic similarities were also important for the effective use of available germplasm by using phenotypic and molecular markers. Amaranth accessions were assessed for variations using random amplified polymorphic DNA (RAPD) primers (24) for getting information on genetic diversity for reliable gene recombination. For carrying out a successful hybrid program, analysis of phenotypic diversity and traits is very valuable.
Taxonomic confusion exists among A. hybridus species complex, among all species (A. cruentus, A. caudatus, A. hypochondriacus, A. hybridus, A. quitensis, and Amaranthus powellii) (190). DNA markers were used to examine the taxonomic and phylogenetic relationships of grain amaranth and their wild relatives and to study phylogeny.
5.2. Genetic improvement of quinoa
Quinoa is a self-pollinated crop with few crossing chances (17.36%) and purity can be maintained by enforced selfing by covering the inflorescence stalk with a paper bag (Figure 3) (191). Cleistogamy leads to autogamy in quinoa; however, obligatory out-crossing due to self-incompatibility and male sterility have been reported (192). The breeding objectives for quinoa were the short stature of plants with fewer branches, growth, short cycle, earliness, low saponin content abiotic and biotic stress, and higher grain yields for commercial varieties by mass selection and hybridization for direct future breeding strategies. The diversity present in the primary gene pool help to characterize Quinoa (128). Due to Quinoa's extensive genetic variety, wide agronomic adaptability, tolerance to many soil types, notably salty soils and situations with highly varying humidity, elevations, and temperatures, and good nutritional health benefits, breeding studies for Quinoa are important (193). To fulfill farmers' demands in various varied environments and agronomic systems, global collaborative work is needed for quinoa which might serve as a basis for improved plant breeding initiatives for developed or developing nations.
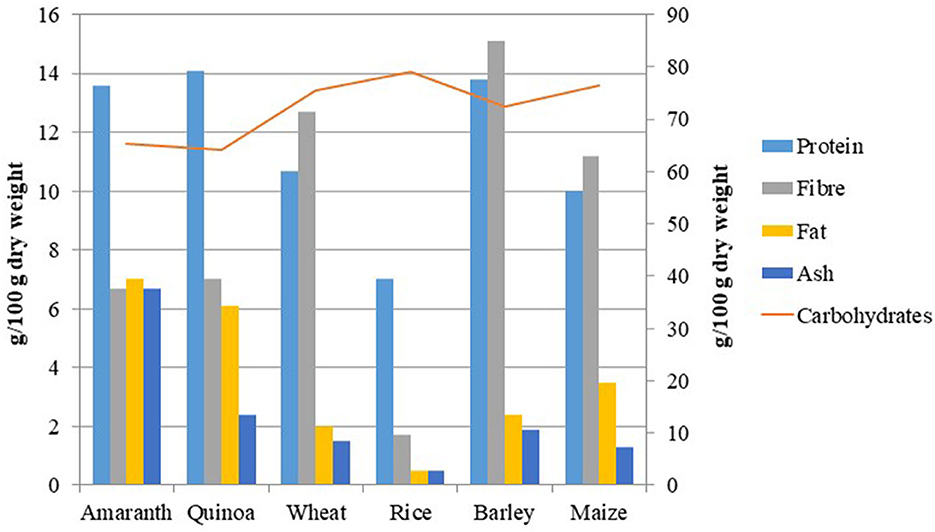
Figure 3. Comparison of the general nutritional profile of amaranth and quinoa with major cereals (28).
DNA markers and linkage maps are important tools for germplasm conservation and crop improvement programs. The first molecular studies were using allozyme markers to establish genetic variability in domesticated quinoa and wild species (C. hircinum and wild quinoa ajara) (194, 195). The distribution of quinoa is high in the Andes in the context of climate changes affected by drought and frost and managed based on the latest technologies and the ecosystem (196). Quinoa has two distinctive groups: a coastal type from southwestern Chile and an Andean type from northwestern Argentina to southern Colombia and their co-evolutionary relationship between domesticated and wild populations is based on molecular studies (195). Quinoa is characterized based on seed storage proteins for the identification of cultivars and breeding purpose for improved protein quantity and quality (197). Morphological variability and various selection parameters were assessed in 44 germplasm lines of Chenopodium spp. (198). Taxonomical and biochemical studies and cross-ability relationships were reported on these species in quinoa (199).
Genetic variability is important for rich germplasm and their conservation for genetic improvement in quinoa. Furthermore, molecular markers are very beneficial in allowing the development of better breeding programs. Genetic resources are very helpful in quinoa cultivars improvement using marker-assisted selection and marker-aided backcross breeding.
As DNA markers, random amplified polymorphic DNA (RAPD) was used first time in quinoa by Fairbanks et al. (200). These markers detect genetic variations within the existing germplasm collection (201, 202). Genetic linkage maps could be generated from intergeneric crosses for hybrid production by using DNA markers (203). Different types of markers such as RAPD and ISSR were used for genetic variation by molecular analysis in quinoa. These markers play a very significant role in distinguishing different genotypes. Simple sequence repeat (SSR) markers were also helpful due to their co-dominant effect and their capability to detect polymorphism in quinoa (204). Various researchers have also studied the effect of SSR markers in different crops and also in quinoa. Jarvis et al. (203) developed 216 new polymorphic SSR (simple sequence repeats) markers from libraries enriched for GA, CAA, and AAT repeats, as well as six SSR markers from bacterial artificial chromosome-end sequences (BES-SSRs) in quinoa. Maughan et al. (205) also reported that the primer sequences and map locations for 19 SSR markers are valuable tools for quinoa genome analysis. This map also indicates starting point for genetic dissection of quinoa, grain yield, seed saponin, maturity, and resistance to diseases, frost, and rot. Fluorescence-tagged markers are used to characterize genetic diversity within quinoa germplasm. Microsatellite markers were used for the characterization of C. quinoa (206). These markers showed genetic diversity between quinoa and other Chenopodium species (207). Microsatellite markers confirmed high polymorphism between two and three nucleotide motifs and the differences in having repeats of motifs (208). Single nucleotide polymorphism (SNPs) was identified by floral expressed sequenced tag (EST) libraries in the development of immature seeds in quinoa (209). This helps to understand the gene associated with its expression and regulation for seed development and gene mapping for breeding development and their evolutionary studies within the genus. The new polymorphic simple sequence repeats (SSR) markers were developed from repeats in gene libraries and few markers were created from bacterial artificial chromosome-end sequences (BES-SSRs) (203). For several marker loci, segregation distortion was observed with markers having an easily transferable nature and showed chromosomal regions linked with selection or gametophytic lethality.
The SNP assays were detected using the Fluidigm dynamic array based on KASPar genotyping (23). The SNPs are important genomic tools for molecular analysis for the improvement of desirable parameters in quinoa. Genome characterization and evaluation are important for selection and cultivar improvement (210). DNA fingerprinting is one of the best tools to assess the chromosomal studies of quinoa genotypes. Biochemical and genetic studies play a more significant role in the development of core collections than the old breeding techniques. The Flowering Locus T-Like genes were used as markers to characterize the genome of diploid species of quinoa (211). Gene editing is useful for the evolution of quinoa germplasm by inserting mitochondrial DNA in C. quinoa. Phylogenetic trees could also be constructed to study unknown ancestry, their relationships with each other, and the role of parents in the origin of present quinoa genotypes. Quinoa is a potential pseudocereal crop thus the study of its ancestry is important to understand sources of heat and biotic tolerance genotypes. Polymorphism was studied in quinoa accessions by using EST-SSR markers for molecular analysis (212).
Due to cross pollination, existing of heterozygosity, and allotetraploidy with genome complexity the molecular analysis of quinoa is restricted (213). To avoid such barriers, inbred lines were developed. The mechanisms involving allotetraploidy were studied for genetic evolution in quinoa. Molecular real-time sequencing with optical, chromosome-contact, and mapping studies was conducted to have a thorough view of the chromosome-scale reference genome sequence for quinoa breeding (96). The sequencing helped us to study the ancestral gene pools which allow for the characterization of sub-genomes in quinoa. The genome sequence was helpful to study the protein synthesis process for the production of anti-nutritional factors (saponins) found in quinoa and to recognize mutations affecting translation in sweet quinoa strains. Thus, quinoa is a model plant for studying polyploidy, genome evolution, and the science behind abiotic stress tolerance, especially salinity tolerance. A complete genome sequencing showed a high number of polymorphic single nucleotide polymorphism (SNP) loci which have been utilized with repeated multiple-year phenotypic data to identify QTLs (quantitative trait loci), controlling all important yield and quality parameters (214).
To expose the phylogeny, the complete chloroplast (cp) DNA was analyzed by next-generation sequencing (complete plastid genome sequences) and molecular markers (215). With sequencing, the genetic diversity of the Chenopodium genus was studied thoroughly. The coding and non-coding regions were analyzed. Inter-simple sequence repeat (ISSR) markers are easier to use than simple sequence repeat (SSR) markers, which are frequently used for genotyping quinoa. ISSR markers are cheap, scoring can be done manually, and a sequence of flanking sites is not necessary, but this is not the situation in SSR markers (216). Whole-genome re-sequencing, using InDel (insertion/deletion), would significantly provide information on population diversity and their role in creating future desirable genotypes in quinoa (217) and can be used to re-sequenced quinoa accessions. These markers clearly depict the difference between the Andean highland type and the Chilean coastal type quinoa.
The molecular markers were used in China to investigate variations in their varieties (218). The ISSR markers were used to recognize polymorphism and identify unique markers for genotypes. ISSR markers characterized five quinoa genotypes and they showed polymorphism (219). ISSR markers are helpful in DNA fingerprinting, and bands formed by these markers are useful to go through the phylogenetic relationship among quinoa present to cultivate wild species, thus this study guides us to identify desirable genes and their sources to plan future breeding programs. This practice also helped us to understand polymorphism in the quinoa genotypes to distinguish them from each other. Quinoa improvement could be possible by germplasm preservation, and conservation is important for developing better-performing hybrids. Molecular characterization was done by different molecular markers, i.e., ISSR, SCoT, and DNA chloroplast markers (rbcL and rpoC1), to study genetic polymorphism and to develop unique markers for each of the seven quinoa genotypes different in seed color and origin (220). These sequences provide good genetic information to the gene bank for the conservation of germplasm.
Accessions of C. quinoa and C. album were evaluated (221) and SDS–PAGE protein profiling of soluble proteins seed in the quinoa was done. Using microsatellite markers with available genome sequences, a thorough analysis of quinoa's genome and its relatives was carried out in five Amaranthaceae species (222). The results demonstrated that high proportions of nucleotide characterized the microsatellite repeats with A/T rich motifs and showed the conservation of genomes and composition of microsatellites in the Amaranthaceae family. The microsatellite markers (SSRs) were used for DNA analysis of nine loci located in 26 varieties of quinoa (223) for the genetic characterization of those varieties.
There are two ways to generate crops that are resistant to salt and drought stress. First, by adding genes from tolerant plants, main crops may be enhanced. Mostly, all the important crops have wild species which has more potential to resist drought and salinity than the cultivated genotypes. We need to understand the mechanism of the fundamental resistance processes going on within plants, then crops might be genetically modified to have higher tolerance. Second, certain marginally grown minor (orphan) crops are already salt and drought resistant. Gene editing about tolerance in crops and improving these crops' agronomic performance may be a useful strategy for boosting crop and food variety. Developing a nutrient-dense, high-performing crop that can fulfill upcoming food demand in a changing environment by selectively altering a few of these genes through induced mutagenesis appears promising. DNA manipulation will provide great tools to study key genes' role in stress tolerance regulation (87, 224, 225). There is a need to study transformation systems in quinoa to conduct gene editing research. Two transformation systems using hairy root and leaf agroinfiltration were developed for quinoa to study the genomics of quinoa, and translation in plants in transgenic quinoa roots was obtained successfully by in vivo method, but having low efficiency. QTL mapping of agronomic parameters can be useful for photoperiod and involved in flowering time by transcriptome analysis to identify genes differentially responding; and by contributing to the development of an Agrobacterium-mediated transformation protocol in quinoa can contribute to improving the quality of crop (226). In vitro studies are very important for supporting genetic engineering studies. Hypocotyl tissue proved to be a suitable explant for the micropropagation of quinoa through the induction of indirect organogenesis (227).
Traditional breeding methods take so much time (nearly 10–12 years) to develop good varieties. To accelerate the genetic improvement of these pseudocereals, we can also go for speed breeding like other major crops to reduce the time period of good quality germplasm development. In this, plants are grown in controlled conditions with controlled photoperiods, humidity, and temperature conditions. This speeds up the crop cycles of these crops so that we can get 5–6 crops in 1 year (136). The tissue culture studies allow us to grow plants throughout the year; thus, we are not dependent on the season to conduct our experiments. The studies on transformation and tissue culture can help us to conduct genetic engineering technologies to improve the germplasm of these pseudocereals (Table 3) (225, 226, 255, 258). A list of available advanced molecular and biotechnological tools and techniques is given in Table 3.
The new breeding technologies such as CRISPR/Cas [clustered regularly interspaced palindromic repeats (CRISPR)/CRISPR-associated protein]-based systems are very precise genome editing tools that allow editing of several genes or alleles simultaneously, thus proved to be a promising platform to insert desirable gene for nutrition enhancement, to lower saponin content and abiotic stress tolerance (262–270). Earlier work is done in potatoes to use endogenous promoters with CRISPR genome editing (271, 272). This technique can also be used to modify the advanced germplasm lines in Amaranth and Quinoa. In the genetic engineering of wheat, there is a need to optimize the codon of the Cas9 sequence with the CRISPR/Cas9 system and further use of a promoter from maize for expression (269). Similarly, as in wheat and maize, efficient genome editing methods can be used in Amaranth and Quinoa, and in future, there may be a need to use different promoters, terminators, or other elements from other sources of common plasmids from different crops. The molecular and molecular approaches discussed earlier and studies on the expression of different genes in this plant may be helpful to select suitable DNA fragments for generating improved Amaranth and Quinoa-optimized vectors in future research.
The latest technologies like RNA interference (RNAi) technology or Post-Transcriptional Gene Silencing (PTGS) can also be used to understand the genomes of Amaranthus and Quinoa, where reverse genetics is followed. By using this gene silencing technique, gene functional studies are carried out to understand functional genomics (273). This study can be used to understand the genomics of anti-nutritional factors present in both Amaranth and Quinoa.
Another new genome editing method is TILLING (Targeting Induced Local Lesions in Genomes), which proved to be the most effective and fast technology for identifying induced mutations in any gene (274). At present, large TILLING libraries typically contain up to 3,000 highly mutagenized individuals of quinoa (275). Advanced genetic screens enable the establishment and screening of much larger libraries derived from mutagenized populations (276). These big libraries help us to identify the genes for desirable characters to obtain good germplasm, reducing the load to identify individual mutations from such a large population. These are collections of all genes and provide instant access to numerous alternative functional alleles for every gene.
6. Conclusion
Amaranth and quinoa are superfoods, particularly for people with allergies to wheat or gluten. It can become part of the diet in developing countries like Africa, where people suffer from malnutrition. These crops can perform well in stress conditions with minimal use of resources. However, the anti-nutritional factors present in these crops like saponin in quinoa need to be tackled under focused breeding programs. The enormous variability in genetic resources available for these crops should be further utilized under selection for targeted traits. These crops have so far attained their full potential as major crops, but the breeding programs to enhance these crops are not up to the mark to achieve the place these crops should be given in the food industry. The cost of sophisticated technology and infrastructure is difficult to be available and so genetic advances under classical and molecular breeding methods are expected to modify this scenario by developing a nutrient-dense, high-performing crop that can fulfill upcoming food demand in a changing environment. Therefore, the region-specific varieties and agrotechnologies would be the key factors for their promotion and large-scale cultivation.
Author contributions
A and MK: writing. GZ: editing nutritional traits. RC and AK: editing plant descriptors and discussion. SanS: editing genetic resources. SatS: conceptualization, review, and editing breeding approaches. All authors contributed to the article and approved the submitted version.
Acknowledgments
The authors are very thankful and acknowledge the encouragement and support received from the Director, CSIR-IHBT, Palampur. The IHBT publication number is 5240.
Conflict of interest
The authors declare that the research was conducted in the absence of any commercial or financial relationships that could be construed as a potential conflict of interest.
Publisher's note
All claims expressed in this article are solely those of the authors and do not necessarily represent those of their affiliated organizations, or those of the publisher, the editors and the reviewers. Any product that may be evaluated in this article, or claim that may be made by its manufacturer, is not guaranteed or endorsed by the publisher.
References
1. Pickergill B. Domestication of plants in the Americas: insights from mendelian and molecular genetics. Ann Bot. (2007) 100:925–40. doi: 10.1093/aob/mcm193
2. Alandia G, Odone A, Rodriguez JP, Bazile D, Condori B. Quinoa—evolution and future perspectives. In:Schmöckel SM, , editor. The Quinoa Genome: Compendium of Plant Genomes. Cham: Springer (2021), 179–95. doi: 10.1007/978-3-030-65237-1_11
3. Boukid F, Folloni S, Sforza S, Vittadini E, Prandi B. Current trends in ancientgrains based foodstuffs: insights into nutritional aspects and technological applications. Compr Rev Food Sci Food Saf. (2018) 17:123–36. doi: 10.1111/1541-4337.12315
4. Fletcher RJ. Pseudocereals: overview. In:Wrigley C, Corke H, Seetharaman K, Faubion J, , editors. Encyclopedia of Food Grains, 2nd ed. Kidlington: Elsevier (2016), 274–9. doi: 10.1016/B978-0-12-394437-5.00039-5
5. Hart TGB, Vorster HJ. Indigenous Knowledge on the South African Landscape: Potentials for Agricultural Development. Urban, Rural and Economic Development Programme, Occasional Paper no. 1. Cape Town: HSRC Press (2006).
6. Hongyan L, Deng Z, Liu R, Zhu H, Draves J, Marcone M, et al. Characterization of phenolics, betacyanins and antioxidant activities of the seed, leaf, sprout, flower and stalk extracts of three Amaranthus species. J Food Compos Anal. (2015) 37:75–81. doi: 10.1016/j.jfca.2014.09.003
7. Mlakar SG, Turinek M, Jakop M, Bavec M, Bavec F. Nutrition value and use of grain amaranth: potential future application in bread making. Agricultura. (2009) 6:45–53.
8. Joshi DC, Sood S, Hosahatti R, Kant L, Pattanayak A, Kumar A, et al. From zero to hero: the past, present, and future of grain amaranth breeding. Theor Appl Genet. (2018) 131:1807–23. doi: 10.1007/s00122-018-3138-y
9. Barba de la Rosa AP, Fomsgaard IS, Laursen B, Mortensen AG, Martinez LO, Silva-Sanchez C, et al. Amaranth (Amaranthushypochondriacus) as an alternative crop for sustainable foodproduction: phenolic acids and flavonoids with potential impacton its nutraceutical quality. J Cereal Sci. (2009) 49:117–21. doi: 10.1016/j.jcs.2008.07.012
10. Adolf VI, Shabala S, Andersen MN, Razzaghi F, Jacobsen SE. Varietal differences of quinoa's tolerance to saline conditions. Plant Soil. (2012) 357:117–29. doi: 10.1007/s11104-012-1133-7
11. Bruno MC. Waranq Waranqa: Ethnobotanial Perspectives on Agricultural Intensification in the Lake Titicaca Basin (Taraco Peninsula, Bolivia) [Ph.D. dissertation]. St. Louis, MO: Department of Anthropology, Washington University in St. Louis (2008).
12. Pearsall DM. Plant domestication and the shift to agriculture in the Andes. In: Silverman H, Isbell WH, ediotrs. The Handbook of South American Archaeology. New York, NY: Springer New York (2008), p. 105–20. doi: 10.1007/978-0-387-74907-5_7
13. Rojas W, Pinto M, Alanoca C, Gomez PL, Leon-Lobos P, Alercia A, et al. Quinoa geneticresources and ex situ conservation. In:Bazile D, Bertero HD, Nieto C, , editors. State of the Art Report on Quinoa Around the World in 2013. Rome: FAO and CIRAD (2015), p. 56–82.
14. Vega-Galvez AV, Miranda M, Vergara J, Uribe E, Puente L, Martinez EA. Nutrition facts and functional potential of quinoa (Chenopodium quinoa Willd.), an ancient Andean grain: a review. J Sci Food Agric. (2010) 90:2541–7. doi: 10.1002/jsfa.4158
15. Jancurová M, Minarovicova L, Dandar A. Quinoa - a review. Czech J Food Sci. (2009) 27:71–9. doi: 10.17221/32/2008-CJFS
16. Bhargava A, Shukla S, Ohri D. Genome size variation in some cultivated and wild species of Chenopodium (Chenopodiaceae). Caryologia. (2007) 60:245–50. doi: 10.1080/00087114.2007.10797943
17. Jacobsen SE. The worldwide potential for Quinoa (Chenopodium quinoa Willd.). Food Rev Int. (2003) 19:167–77. doi: 10.1081/FRI-120018883
18. Risi J, Galwey NW. Chenopodium grains of the Andes: a crop for the temperate latitudes. In:Wickens GE, Haq N, Day P., , editors. New Crops for Food and Industry. New York, NY: Chapman and Hall (1989).
19. Galwey NW. The potential of quinoa as a multipurpose crop for agricultural diversification: a review. Ind Crops Prod. (1992) 1:101–6. doi: 10.1016/0926-6690(92)90006-H
20. Mujica A, Jacobsen SE, Ezquierdo J, Marathee JP. Resultados de la Prueba Amricana y Europes de la Quinoa. Rome: FAO, UNS-Puno, CIP (2001), p. 51.
21. Smith FI, Eyzaguirre P. African leafy vegetables: their role in the World Health Organization's global fruit and vegetables initiative. In:Oniang'o R, Grum M, Obel-Lawson E, , editors. Developing African Leafy Vegetables for Improved Nutrition, Regional Workshop. Nairobi: Rural Outreach Program (2007), p. 1–8. doi: 10.18697/ajfand.14.IPGRI1-1
22. Jamalluddin N, Massawe FJ, Mayes S, Ho WK, Symonds RC. Genetic diversity analysis and marker-trait associations in Amaranthus species. PLoS ONE. (2022) 17:0267752. doi: 10.1371/journal.pone.0267752
23. Maughan PJ, Smith SM, Rojas BJA, Elzinga D, Raney JA, Jellen EN, et al. Single nucleotide polymorphism identification, characterization, and linkage mapping in quinoa. Plant Genome. (2012) 5:114–25. doi: 10.3835/plantgenome2012.06.0011
24. Oduwaye OA, Ayo-Vaughan MA, Porbeni JBO, Oyelakin OO. Genetic diversity in Amaranth (Amaranthus spp.) based on phenotypic and RAPD markers. Nig J Biotech. (2019) 36:62–8. doi: 10.4314/njb.v36i1.9
25. Bonifacio A. Caracteres Hereditarios y Ligamiento Factorial en la Quinua (Chenopodium quinoa Willd.) Ing. Agr [Thesis]. Cochabamba: Bolivia UniversidadMayor de San Simón (1990), p. 189.
27. Diwan IS, Shukla N, Sharma D. Assessment of genetic variability among genotypes of Amaranthus tricolor. Plant Arch. (2017) 17:129−31.
28. Schmdit D, Verruma-Bernardi MR, Forti VA, Borges MTMR. Quinioa and Amaranth as a fuctional food: a review. Food Rev Int. (2021) 39:2277–96. doi: 10.1080/87559129.2021.1950175
29. Gebhardt S, Lemar L, Haytowitz D, Pehrsson P, Nickle M, Showell B, et al. USDA National Nutrient Database for Standard Reference, Release 21. Washington, DC: United States Department of Agriculture. Agricultural Research Service (2008).
30. Prakash D, Pal M. Chenopodium: seed protein, fractionation and amino acid composition. Int J Food Sci Nutr. (1998) 49:271–5. doi: 10.3109/09637489809089398
31. Betschart AA, Irving DW, Shepherd AD, Saunders RM. Amaranthus cruentus: milling characteristics, distribution of nutrients within seed components, and the effects of temperature on nutritional quality. J Food Sci. (1981) 46:1181–7. doi: 10.1111/j.1365-2621.1981.tb03019.x
32. Dhellot JR, Matouba E, Maloumbi MG, Nzikou JM, Ngoma DS, Linder M, et al. Extraction, chemical composition and nutrional characterization of vegetable oils: Case of Amaranthus hybridus (var 1 and 2) of Congo Brazzaville. Afr J Biotechnol. (2006) 5:1095–101.
34. Berghofer E, Schoenlechner R. Grain amaranth. In:Belton P, Taylor J, , editors. Pseudocereals and Less Common Cereals, Grain Properties and Utilization Potential (Berlin: Springer Verlag (2002), p. 219–60. doi: 10.1007/978-3-662-09544-7_7
35. Yanez E, Zacarias I, Granger D, Vasquez M, Estevez AM. Chemical and nutritional characterization of amaranthus (Amaranthus cruentus). Arch Latinoam Nutr. (1994) 44:57–62.
36. Svirskis A. Investigation of amaranth cultivation and utilization in Lithuania. Agronomy Res. (2003) 1:253–64.
37. Gorinstein S, Moshe R. Evaluation of four amaranthus species through protein electrophoretic patterns and their amino acid composition. J Agric Food Chem. (1991) 39:851–4. doi: 10.1021/jf00005a006
38. Schoenlechner R, Siebenhandl S, Berghofer E. Pseudocereals. In:Arendt EK, Dal Bello F, , editors. Gluten-free Cereal Products and Beverages. London: Academic Press (2008), p. 149–76. doi: 10.1016/B978-012373739-7.50009-5
39. Repo-Carrasco-Valencia R, Hellstroem JK, Pihlava JM, Mattila PH. Flavonoids and other phenolic compounds in andean indigenous grains: quinoa (Chenopodium quinoa), kafiiwa (Chenopodium pallidicaule) and kiwicha (Amaranthus caudatus). Food Chem. (2010) 120:128–33. doi: 10.1016/j.foodchem.2009.09.087
40. Ramos D, Martin J. University of Helsinki, Department of Food and Environmental Sciences. EKT Series 1522 Jose Martin Ramos Diaz Helsinki 2011. (2011), p. 1−86.
41. Villa DYG, Russo L, Kerbab K, Landi M, Rastrelli L. Chemical and nutritional characterization of Chenopodium pallidicaule (cañihua) and Chenopodium quinoa (quinoa) seeds. Emirates J Food Agri. (2014) 26:609–15. doi: 10.9755/ejfa.v26i7.18187
42. Kozioł M. Chemical composition and nutritional evaluation of Quinoa. (Chenopodium quinoa Willd). J Food Comp Anal. (1992) 5:35–68. doi: 10.1016/0889-1575(92)90006-6
43. Wright KH, Pike OA, Fairbanks DJ, Huber SC. Composition of Atriplex hortensis, sweet and bitter Chenopodium quinoa seeds. Food Chem Toxicol. (2002) 67:1383–5. doi: 10.1111/j.1365-2621.2002.tb10294.x
44. De Bruin AA. Investigation of the food value of quinoa and Cañihua Seed. J Food Sci. (1963) 29:872–6. doi: 10.1111/j.1365-2621.1964.tb00464.x
45. Dini A, Rastrelli L, Saturnino P, Schettino O. A compositional study of Chenopodium quinoa seeds. Nahrung. (1992) 36:400–4. doi: 10.1002/food.19920360412
46. Repo-Carrasco R, Espinoza C, Jacobsen SE. Nutritional value and use of the andean crops quinoa, and kaiwa. Food Rev Int. (2003) 19:179–89. doi: 10.1081/FRI-120018884
47. González JA, Roldán A, Gallardo M, Escudero T, Prado FE. Quantitative determinations of chemical compounds with nutritional value from inca crops: Chenopodium quinoa (‘quinoa'). Plant Foods Hum Nutr. (1989) 39:331–7. doi: 10.1007/BF01092070
48. Konishi Y, Hirano S, Tsuboi H, Wada M. Distribution of minerals in quinoa (Chenopodium quinoa Willd.) seeds. Biosci Biotechnol Biochem. (2004) 68:231–4. doi: 10.1271/bbb.68.231
49. Alvarez-Jubete L, Arendt EK, Gallagher E. Nutritive value of pseudocereals and their increasing use as functional gluten-free ingredients. Trends Food Sci Technol. (2010) 21:106–13. doi: 10.1016/j.tifs.2009.10.014
50. Miranda M, Vega AA, Martínez E, López J, Rodríguez M, Henriquez K, et al. Genetic diversity and comparison of physicochemical and nutritional characteristics of six quinoa (Chenopodium quinoa wild) genotypes cultived in Chile. Cienc Tecnol Aliment. (2012) 32:1–9. doi: 10.1590/S0101-20612012005000114
51. Ruales J, Nair BM. Properties of starch and dietary fibre in raw and processed quinoa (Chenopodium quinoa. Willd) seeds. Plant Foods Hum Nutr. (1994) 45:223–46. doi: 10.1007/BF01094092
52. Bhargava A, Shukla S, Ohri D. Chenopodium quinoa: an Indian perspective. Ind Crops Prod. (2006) 23:73–87. doi: 10.1016/j.indcrop.2005.04.002
53. Sanders M. Estudio del secado industrial de la quinoa (Chenopodium quinoa Willd.) cultivada en Chile: Efecto de la temperatura sobre su composicion. Tesis de Pregrado. Chile: Dep. Food Engineering, Universidad de Las Serena (2009).
54. Gupta S, Lakshmia AJ, Manjunath MN, Prakash J. Analysis of nutrient and anti-nutrient content of underutilized green leafy vegetables. LWT Food Sci Technol. (2005) 38:339–45. doi: 10.1016/j.lwt.2004.06.012
55. Priya VP, Celine VA, Gokulapalan C, Rajamony L. Screening of amaranth genotypes (Amaranthus spp.) for yield and resistance to leaf blight caused by Rhizoctoniasolani. Kuhn Plant Genet Res News. (2007) 147:1–4.
56. Tang Y, Li X, Chen PX, Zhang B, Liu R, Hernandez M, Tsao R. Assessing the fatty acid, carotenoid, and tocopherol compositions of amaranth and quinoa seeds grown in Ontario and their overall contribution to nutritional quality. J Agric Food Chem. (2016) 64:1103–10. doi: 10.1021/acs.jafc.5b05414
57. Sreelathakumary I, Peter KV. Amaranth – Amaranthus spp. In:Kalloo G, Bergh BO, , editors. Genetic Improvement of Vegetable Crops. Oxford: Pergamon (1993), p. 315–23. doi: 10.1016/B978-0-08-040826-2.50026-6
58. Rastogi A, Shukla S. Amaranth: a new millennium crop of nutraceutical values. Crit Rev Food Sci Nutr. (2013) 53:109–25. doi: 10.1080/10408398.2010.517876
59. Bressani R, Sánchez-Marroquín A, Morales E. Chemical composition of grain amaranth cultivars and effects of processing ontheir nutritional quality. Food Rev Int. (1992) 8:23–49. doi: 10.1080/87559129209540928
60. Yanez GA, Messinger JK, Walker CE, Rupnow JH. Amaranthus hypochondriacus: starch isolation and partial characterization. Cereal Chem. (1986) 63:273–6.
61. Stone LA, Lorenz K. The starch of Amaranthus: physics-chemical properties and functional characteristics (Amaranthus cruentus, Amaranthus hypochondriacus). Starke. (1984) 36:232–7. doi: 10.1002/star.19840360704
62. Konishi Y, Nojima H, Okuno K, Asaoka M, Fuwa H. Characterization of starch granules from waxy, nonwaxy, and hybrid seeds of Amaranthus hypochondriacus L. Agric Biol Chem. (1985) 49:1965–71. doi: 10.1080/00021369.1985.10867018
63. Garcia LA, Alfaro MA, Bressani R. Digestibility and protein-quality of raw and heat-processed defatted and nondefatted flours prepared with 3 amaranth species. J Agric Food Chem. (1987) 35:604–7. doi: 10.1021/jf00076a039
64. Ferreira TAPC, Matias ACG, Arêas JAG. Nutritional and functional characteristics of amaranth (Amaranthus Spp.). Nutrire. (2007) 32:91–116.
65. Morales E, Lembcke J, Graham GG. Nutritional value for young children of grain amaranth and maize-amaranth mixtures: effect of processing. J Nutr. (1988) 118:78–85. doi: 10.1093/jn/118.1.78
66. Yaacob JS, Hwei LC, Taha RM, Mat Nor NA, Aziz N. Pigment analysis and tissue culture of Amaranthus cruentus L. Acta Hortic. (2012) 958:171–8. doi: 10.17660/ActaHortic.2012.958.20
67. Sarker U, Lin Y, Oba S, Yoshioka Y, Hoshikawa K. Prospects and potentials of underutilized leafy amaranths as vegetable use for health-promotion. Plant Phys Biochem. (2022) 182:104–23. doi: 10.1016/j.plaphy.2022.04.011
68. Teutonico RA, Knorr D. Amaranth: composition, properties, and applications of a rediscovered food crop. Food Technol. (1985) 6:145–50.
69. Burd NB. Pharmacognostic Study of Some Species of Amaranth Genus Plants. Manuscript/Thesis for the candidate of pharmaceutical science degree in speciality 15.00.02 – Pharmaceutical Chemistry and Pharmacognosy. Kharkov: National University of Pharmacy (2006).
70. Gamel T, Linssen Jozef H, Mesallam P, Damir AS, Shekib Lila AA. Seed treatments affect functional and antinutritional properties of amaranth flours. J Sci Food Agric. (2006) 86:1095–102. doi: 10.1002/jsfa.2463
71. Lorenz K, Wright B. Phytate and Tannin content of amaranth. Food Chem. (1984) 14:27–34. doi: 10.1016/0308-8146(84)90015-3
72. Girish S, Dhan P, Charu G. Phytochemicals of nutraceutical importance: do they defend against diseases? In: Karwande V, Borade R, ediors. Phytochemicals of Nutraceutical Importance. Wallingford: CAB International (2014), 1–19. doi: 10.1079/9781780643632.0001
73. Maseko I, Mabhaudhi T, Tesfay S, Araya H, Fezzehazion M, Plooy C, et al. African leafy vegetables: a review of status, production and utilization in South Africa. Sustainability. (2017) 10:16. doi: 10.3390/su10010016
74. Qumbisa ND, Ngobese N, Kolanisi U. Potential of using amaranthus leaves to fortify instant noodles in the South African context: a review. Afr J Food Agric Nutr Dev. (2020) 20:16099–111. doi: 10.18697/ajfand.92.18690
75. Reyad-ul-Ferdous M, Shahjahan DMS, Tanvir S, Mukti M. Present biological status of potential medicinal plant of amaranthus viridis: a comprehensive review. Am J Clin Exp Med. (2015) 3:12. doi: 10.11648/j.ajcem.s.2015030501.13
76. Khongsai M, Saikia SP, Kayang H. Ethnomedicinal plants used by different tribes of Arunachal Pradesh. Indian J Tradit Know. (2011) 10:541–6.
77. Njonje WA. Nutrients, Anti-Nutrients and Phytochemical Evaluation of Ten Amaranth Varieties at Two Growth Stages. Nairobi: Jomo Kenyatta University of Agriculture and Technology (2015).
78. Achigan-Dako EG, Sogbohossou OED, Maundu P. Current knowledge on Amaranthus spp.: research avenues for improved nutritional value and yield in leafy amaranths in sub-Saharan Africa. Euphytica. (2014) 197:303–17. doi: 10.1007/s10681-014-1081-9
79. Nawaz AM, Hossain M, Karim M, Khan M, Jahan R, Rahmatullah M, et al. An ethnobotanical survey of Rajshahi district in Rajshahi division, Bangladesh. Am Eurasian J Sustain Agric. (2009) 3:143–50.
80. Sebasttian MK, Bhandari MM. Medicoethno botany of Mount Abu, Rajasthan, Indian. J Pharmacol. (1984) 12:223–30. doi: 10.1016/0378-8741(84)90050-3
81. Bhargava A, Shukla S, Ohri D. Genetic variability and heritability of selected traits during different cuttings of vegetable Chenopodium. Ind J Genet Plant Breed. (2003) 63:359–60.
82. Spehar CR. Adaptation of Quinoa (Chenopodium quinoa Willd) to increase the agricultural and alimentary diversity in Brazil. Cadernos de Ciencia and Tecnologia. (2006) 23:41–62.
84. Valencia-Chamorro SA. Quinoa. In:Caballero B, , editor. Encyclopedia of Food Science and Nutrition, Vol. 8. Amsterdam: Academic Press (2003), p. 4895–902. doi: 10.1016/B0-12-227055-X/00995-0
85. Angeli V, Silva PM, Massuela DC, Khan MW, Khajehei HA, Graeff-Hönninger F, et al. Quinoa, C. quinoa (Chenopodium quinoa Willd): an overview of the potentials of the “golden grain” and socioeconomic and environmental aspects of its cultivation and marketization. Foods. (2020) 9:1–31. doi: 10.3390/foods9020216
86. Spehar CR, Santos RLB. Quinoa BRS piabiru: alternative for diversification of cropping systems. Pesq Agropec Bras. (2002) 37:889–93. doi: 10.1590/S0100-204X2002000600020
87. Jacobsen SE, Mujica A, Jensen CR. The resistance of quinoa (Chenopodium quinoa Willd.) to adverse abiotic factors. Food Rev Int. (2003) 19:99–109. doi: 10.1081/FRI-120018872
88. Fuentes F, Paredes-Gonzalez X. Nutraceutical perspectives of quinoa: biological properties and functional applications. In:Bazile D, Bertero D, Nieto C, , editors. State of the Art Report of Quinoa in the World in 2013. Food and Agriculture Organization of the United Nations, Agricultural Research Centre for International Development. Rome: FAO (2015), p. 286–299.
89. Bazile D, Bertero D, Nieto C. Estado del Arte de la Quinua en el Mundo en 2013: FAO (Santiago de Chile) y CIRAD (Montpellier, Francia). Perspectivas Nutracéuticas de la Quinua: Propiedades Biológicas y Aplicaciones Funcionales. Rome: FAO (2014), p. 341–57.
91. Chauhan GS, Eskin NAM, Tkachuk R. Nutrients and antinutrients in quinoa seed. Cereal Chem. (1992) 69:85–8.
92. Ogungbenle NH. Nutritional evaluation and functional properties of quinoa (Chenopodium quinoa) flour. Int J Food Sci Nutr. (2003) 54:153–8. doi: 10.1080/0963748031000084106
93. Spehar CR. Quinoa: Alternativa Para a Diversificação Agricola e Alimentar (Planaltina DF editor). Brasil: Tecnico, Embrapa Cerrados (2007), p. 103.
94. Abugoch James LE. Quinoa (Chenopodium quinoa Willd.): composition. chemistry, nutritional and functional properties. Adv Food Nutr Res. (2009) 58:1–31. doi: 10.1016/S1043-4526(09)58001-1
95. Filho AM, Pirozi MR, Borges JT, Pinheiro Sant'Ana HM, Chaves JB, Coimbra JS. Quinoa: nutritional, functional, and antinutritional aspects. Crit Rev Food Sci. (2017) 57:1618–30. doi: 10.1080/10408398.2014.1001811
96. Jarvis D, Ho Y, Lightfoot D. The genome of Chenopodium quinoa. Nature. (2017) 542:307–12. doi: 10.1038/nature21370
97. Rao NK, Shahid M. Quinoa—a promising new crop for the Arabian peninsula. J Agric Environ Sci. (2012) 12:1350–5. doi: 10.5829/idosi.aejaes.2012.12.10.1823
98. Choukr-Allah R, Rao NK, Hirich A, Shahid M, Alshankiti A, Toderich K, et al. Quinoa for marginal environments: toward future food and nutritional security in MENA and Central Asia regions. Front Plant Sci. (2016) 7:346. doi: 10.3389/fpls.2016.00346
99. Ruiz KB, Khakimov B, Engelsen SB, Bak S, Biondi S, Jacobsen SE, et al. Quinoa seed coats as an expanding and sustainable source of bioactive compounds: an investigation of genotypic diversity in saponin profiles. Ind Crops Prod. (2017) 104:156–63. doi: 10.1016/j.indcrop.2017.04.007
100. Gamboa C, Van den BG, Maertens M. Smallholders' preferences for improved quinoa varieties in the Peruvian Andes. Sustainability. (2018) 10:3735. doi: 10.3390/su10103735
101. Präger A, Munz S, Nkebiwe PM, Mast B, Graeff-Hönninger S. Yield and quality characteristics of different quinoa (Chenopodium quinoa willd.) cultivars grown under field conditions in Southwestern Germany. Agronomy. (2018) 8:197. doi: 10.3390/agronomy8100197
102. Singh B, Kaur A. Control of insect pests in crop plants and stored food grains using plant saponins: a review. LWT – Food Sci Technol. (2018) 87:93–101. doi: 10.1016/j.lwt.2017.08.077
103. McCartney NB, Ahumada MI, Muñoz MP, Rosales IM, Fierro AM, Chorbadjian RA, et al. Effects of saponin-rich quinoa (Chenopodium quinoa Willd.) bran and bran extract in diets of adapted and non-adapted quinoa pests in laboratory bioassays. Cienc Investig Agrar. (2019) 46:125–36. doi: 10.7764/rcia.v46i2.2159
104. Kuljanabhagavad T, Thongphasuk P, Chamulitrat W, Wink M. Triterpene saponins from Chenopodium quinoa Willd. Phytochemistry. (2008) 69:1919–26. doi: 10.1016/j.phytochem.2008.03.001
105. Han X, Shen T, Lou H. Dietary polyphenols and their biological significance. Int J Mol Sci. (2007) 8:950–88. doi: 10.3390/i8090950
106. Scalbert A, Manach C, Morand C, Rémésy C. Dietary polyphenols and the prevention of diseases. Crit Rev Food Sci Nutr. (2005) 45:287–306. doi: 10.1080/1040869059096
108. Mujica A. Andean grains and legumes. In:Hernando Bermujo JE, Leon J, , editors. Neglected Crops: 1492 from a Different Perspective, Vol. 26. Rome: FAO (1994), p. 131–48.
109. Sauer JD. The grain amaranths: a survey of their history and classification. Ann Missouri Bot Garden. (1950) 37:561–632. doi: 10.2307/2394403
110. Kauffman CS, Weber LE. Grain amaranth. In:Janick J, Simon JE, , editors. Advances in New Crops. Portland, OR: Timber Press (1990), p. 127–39.
111. Akubugwo IE, Obasi NA, Chinyere GC, Ugbogu AE. Nutritional and chemical value of Amaranthus hybridus L. leaves from Afikpo, Nigeria. Afr J Biotechnol. (2007) 6:2833–9. doi: 10.5897/AJB2007.000-2452
112. Spehar CR. Morphological differences between Amaranthus cruentus, Cv. BRS Alegria, and the weed species A hybridus, A retroflexus, A viridis E A spinosus. Planta Daninha. (2003) 21:481–5. doi: 10.1590/S0100-83582003000300017
113. Gravis A, Constantinesco C. Contribution to the anatomy of Amaranthaceae. Archives of the Botanical Institute of the University of Liege. (1907). p. 1–65.
114. Wilson CL. Medullary bundle in relation to primary vascular system in Chenopodiaceae and Amaranthaceae. Bot Gaz. (1924) 78:175–99. doi: 10.1086/333371
115. Costea M, Demason DA. Stem morphology and anatomy in Amaranthus L. (Amaranthaceae) – taxonomic significance. J Torrey Bot Soc. (2001) 128:254–81. doi: 10.2307/3088717
116. Eliasson U. Floral morphology and taxonomic relation among genera of Amaranthaceae in the new world and the Hawaii islands. J Linn Soc Bot. (1988) 96:235–83. doi: 10.1111/j.1095-8339.1988.tb00683.x
117. Costea M. Amaranthus L. subgenus Albersia (KUNTH) GREN and GODR. Romania Rev Roum Biol. (1998) 43:95–112.
118. Erdtman G. Pollen morphology and plant taxonomy, Angiosperms. Stockholm: Almquist and Wiksell (1966).
119. Nowicke JW. Pollen morphology and exine ultrastructure in Caryophyllales: – in: nutritional value from inca crops: Chenopodium quinoa (‘quinoa'). Plant Foods Hum Nutr. (1993) 39:331–7.
120. Franssen AS, Skinner DZ, Al-Khatib K, Horak MJ. Pollen morphological differences in Amaranthus species and interspecific hybrids. Weed Sci. (2001) 49:732–7. doi: 10.1614/0043-1745(2001)049[0732:PMDIAS]2.0.CO;2
121. Chaturvedi M, Dutta K, Pal M. Cytopalynology of Amaranthus L. Chenopodium L – the two pantoporate taxa. Feddes Rep. (1997) 108:325–33. doi: 10.1002/fedr.19971080506
122. Tapia, M. E. (2000). Cultivos Andinos Subexplotados y Su Aporte a la Alimentación, 2nd ed. Santiago, CA: FAO.
123. Gandarillas H. Mejoramiento genético. In:Tapia M, Gandarillas H, Alandia S, Cardozo A, Mujica A, Ortiz R, et al., , editors. Quinua y Kanihua, Cultivos Andinos. Bogotá Colombia: IICA (1979), 65–82.
124. Hunziker AT. Los especies alimenticias de Amaranthus y Chenopodium cultivadas por los Indios de America. Rev Argen Agron. (1943) 30:297–353.
125. Simmonds NW. The grain chenopods of the tropical American highlands. Econ Bot. (1965) 19:223–35. doi: 10.1007/BF02914309
126. Rojas W, La Diversidad PM. Genética de Quinua de Bolívia. In:Vargas M, , editor. Congreso Científico de la Quinua (Memorias). La Paz: IICA (2013), p. 77–92.
127. Gandarillas H. Observaciones Sobre La Biología Reproductiva de la Quinua. Sayaña. (1967) 5:26–9.
128. Risi J, Galwey NW. The Chenopodium grains of the andes: inca crops for modern agriculture. Adv Appl Biol. (1984) 10:145–216.
129. Prego I, Maldonado S, Otegu M. Seed structure and localization of reserves in Chenopodium quinoa. In: Monitoring and Bioremediation of Mine and Industrial Environment Proc Natl Sem, North Orissa University, Orissa. Ann Bot. (1998) 82:481–8. doi: 10.1006/anbo.1998.0704
130. Turner BL. Chromosome numbers and their phyletic interpretation. In:HD Behenke, TJ Mabry, , editors. Caryophyllales Evolution and Systematic. New York, NY: Springer (1954), p. 27–43. doi: 10.1007/978-3-642-78220-6_3
131. Mujica A, Jacobsen SE. The geneticresources of Andean grain amaranths (Amaranthus caudatus L A cruentus L, A hypochondriacus L) in America Plant. Genet Resour Newsl. (2003) 133:41–4.
132. Brenner DM, Baltensperger DD, Kulakow PA, Lehmann JW, Myers RL, Slabbert MM, et al. Genetic resources and breeding of Amaranthus. Plant Breed Rev. (2013) 19:227–85. doi: 10.1002/9780470650172.ch7
133. Ebert AW, Wu TH, Yang RY. Amaranth sprouts and microgreens–a homestead vegetable production option to enhance food and nutrition security in the rural-urban continuum. In: Proceedings of the Regional Symposium on Sustaining Small-Scale Vegetable Production and Marketing Systems for Food and Nutrition Security (SEAVEG). Bangkok (2014), p. 25–27.
134. Pal M, Khoshoo TN. Evolution and improvement of cultivated amaranths. V. Inability, weakness, and sterility in hybrids. J Hered. (1972) 63:78–82. doi: 10.1093/oxfordjournals.jhered.a108234
135. Escobedo-López D, Núñez-Colín CA, Espitia-Rangel E. Adaptation of cultivated amaranth (Amaranthus spp.) and their wild relatives in Mexico. J Crop Improv. (2014) 28:203–13. doi: 10.1080/15427528.2013.869518
136. Stetter MG, Vidal-Villarejo M, Schmid KJ. Parallel seed color adaptation during multiple domestication attempts of an ancient new world grain. Mol Biol E. (2020) 37:1407–19. doi: 10.1093/molbev/msz304
137. Trucco F, Hager AG, Tranel PG. Acetolactate synthase mutation conferring imidazolinone-specific herbicide resistance in Amaranthus hybridus. J Plant Physiol. (2011) 163:475–9. doi: 10.1016/j.jplph.2005.06.015
138. Das S. Amaranthus: A Promising Crop of Future. Springer: Singapore (2016). doi: 10.1007/978-981-10-1469-7
140. Kalinowski LS, Navarro JP, Concha AIR, Hermoza GC, Pacheco RA, Choquevilca YC, et al. Grain amaranth research in Peru. Food Rev Int. (1992) 8:87–124. doi: 10.1080/87559129209540931
141. Kachiguma NA, Mwase W, Maliro M, Damaliphetsa A. Chemical and mineral composition of Amaranth (Amaranthus L.) species collected from central Malawi. J Food Res. (2015) 4:92–102. doi: 10.5539/jfr.v4n4p92
142. Suresh S, Chung JW, Cho GT, Sung JS, Sung JH, Park JG, et al. Analysis of molecular genetic diversity and population structure in Amaranthus germplasm using SSR markers, Plant biosystems - an international journal dealing with all aspects of plant. Biology. (2014) 148:635–44. doi: 10.1080/11263504.2013.788095
143. Prashantha GS, Nagaraja TE. Variability and genetic diversity studies in grain Amaranth (Amaranthus spp.). Agric Res Technol. (2011) 36:063–6.
144. Pandey RM, Singh R. Genetic divergence in grain amaranth (Amaranthus hypochondriacus L.). Genetika. (2011) 43:41–9. doi: 10.2298/GENSR1101041P
145. Baturaygil A, Stetter MG, Schmid K. Breeding amaranthus for biomass: evaluating dry matter content and biomass potential in early and late maturing genotypes. Agronomy. (2021) 11:970. doi: 10.3390/agronomy11050970
146. Chaney L, Mangelson R, Ramaraj T, Jellen EN, Maughan P. The complete chloroplast genome sequences for four Amaranthus species (Amaranthaceae). Appl Plant Sci. (2016) 4:1600063. doi: 10.3732/apps.1600063
147. Olusanya AC. A multi-species assessment of genetic variability in Nigerian Amaranthus accessions: potential for improving intra- and interspecies hybridization breeding. Arch Agron Soil Sci. (2018) 64:612–25. doi: 10.1080/03650340.2017.1384817
148. Wu H, Sun M, Yue S, Sun H, Cai Y, Huang R, et al. Field evaluation of an Amaranthus genetic resource collection in China. Genet Resour Crop Evol. (2000) 47:43–53. doi: 10.1023/A:1008771103826
149. Gerrano AS, Jansen van Rensburg WS, Mavengahama S, Bairu M, Venter S, Adebola PO. Qualitative morphological diversity of Amaranthus species. J Trop Agric. (2017) 55:12–20.
150. Erum S, Naeemullah M, Masood S, Qayyum A, Rabbani MA. Genetic divergence in amaranthus collected from Pakistan. J Anim Plant Sci. (2012) 22:653–8.
151. Hricova A, Fejer J, Libiakova G, Szabova M, Gazo J, Gajdosova A, et al. Characterization of phenotypic and nutritional properties of valuable Amaranthus cruentus L. mutants. Turk J Agric For. (2016) 40:761–71, 1511–31. doi: 10.3906/tar-1511-31
152. Food and Agriculture Organization of the United Nations. The Second Report on the State of the World's Plantgenetic Resources for Food and Agriculture. Rome: Food and Agriculture Organization of the United Nations (2010). p. 370.
153. FAO-WIEWS. Sistema Mundial de Información y Alerta sobre los Recursos Fitogenéticos para la Agricultura y la Alimentación. (2013). Available online at: https://www.fao.org/wiews (accessed December 15, 2022).
154. Fuentes F, Maughan P, Jellen E. Diversidad genética y recursos genéticos para el mejoramiento de la Quínoa (Chenopodium quinoa Willd). Rev Geogr Valpso. (2009) 42:20–33.
155. Jellen EN, Kolano BA, Sederberg MC, Bonifacio A, Maughan PJ. Chenopodium. In:Kole C, , editor. Wild Crop Relatives: Genomic and Breeding Resources. Berlin: Springer (2011). p. 35–61.
156. Wilson HD. Quinua and relatives (Chenopodium sect. Chenopodium subsect. Cellulata). Econ Bot. (1990) 44:92–110. doi: 10.1007/BF02860478
157. Hauptli H, Jain S. Genetic variation in outcrossing rate and correlated floral traits in a population of grain amaranth (Amaranthus cruetus L.). Genetica. (1985) 66:21–7. doi: 10.1007/BF00123602
158. Murray MJ. Interspecific and Intergenetic Crosses in the Family Amaranthaceae. [Ph.D. Thesis]. Ithaca, NY: Cornell University (1938).
159. Kauffman CS. Grain Amaranth Varietal Improvement: Breeding Program. Emmaus, PA: Rodale Press (1981).
160. Grant WF. Cytogenetic studies in Amaranthus. III Chromosome numbers and phytogenetic aspects. Can J Genet Cytol. (1959) 1:313–28. doi: 10.1139/g59-031
161. Pal M. Chromosome numbers in some Indian agiosperms. Proc Indian Acad Sci LX. (1964) 5:347–50. doi: 10.1007/BF03053892
163. Vaidya KR. Genetic variation in land race populations of Indian amaranths. Diss Abstr Int B. (1984) 44:3625.
164. Ayiecho PO. Quantitative studies in two grain amaranth populations using two selection methods. Diss Abstr Int B. (1986) 46:2189B.
165. Kulakow P, Jain SK. Genetics of grain amaranths. 4 variation and early generation response to selection in Amaranthus cruetus L. Theor Appl Genet. (1987) 74:113–20. doi: 10.1007/BF00290093
166. Vaidya KR, Jain SK. Response to mass selection for plant height and grain yield in amaranth (Amaranthus spp.). Plant Breed. (1987) 98:61–4. doi: 10.1111/j.1439-0523.1987.tb01091.x
167. Pandey RM. Genetic studies of yield contributing traits in Amaranthus. Theor Appl Genet. (1984) 68:121–5. doi: 10.1007/BF00252326
168. Kulakow P, Hauptli H, Jain S. Genetics of grain amaranths I. Mendelian analysis of six color characteristics. J Hered. (1985) 76:27–30.
169. Kulakow PA, Jain SK. The inheritance of flowering in Amaranthus species. J Genet. (1985) 64:85–100. doi: 10.1007/BF02931137
170. Kulakow PA. Genetics of grain amaranths. J Hered. (1987) 78:293–7. doi: 10.1093/oxfordjournals.jhered.a110390
171. Okuno K, Sakoguchi S. Inheritance of starch characteristics in perisperm of Amaranthus hypochondriacus. J Hered. (1982) 73:467. doi: 10.1093/oxfordjournals.jhered.a109700
172. Okuno K. Expression of mutant genes specifying starch synthesis in cereal grains. Gamma Field Symp. (1985) 24:39–62.
174. Gudu S, Gupta VK. Male-sterility in the grain amaranth (Amaranthus hypochondriacus ex-Nepal) variety Jumla. Euphytica. (1988) 37:23–6. doi: 10.1007/BF00037218
175. Peters I, Jain S. Genetics of grain amaranths III. 3. Gene-cytoplasmic male-sterility. J Heredity. (1987) 78:251–6.
176. Weber L, Applegate WW, Baltensperger DD, Irwin MD, Lehmann JW, Putnam DH. Amaranth Grain Production Guide. Emmaus, PA: Rodale Press (1990).
177. Janovska D, Cepkova PH, Dzunkova M. “Characterization of the Amaranthus genetic resourcesin the czech gene bank. In:Caliskan M, , editor. Genetic Diversity of Plants. Washington, DC: In Tech (2012), p. 457–78. doi: 10.5772/34165
178. Makobo ND, Shoko MD. Nutrient content of Amaranth (Amaranthus cruentus L.) under different processing and preservation methods. World J Agric Sci. (2010) 6:639–43.
179. Sauer J. Recent migration and evolution of the dioecious amaranths. Evolution. (1957) 11:11–31. doi: 10.2307/2405808
180. Walton PD. The use of Amaranthus caudatus in stimulating the breeding behaviour of commercial Gossypium Species. Jour Hered. (1968) 59:117–8. doi: 10.1093/oxfordjournals.jhered.a107630
181. Jain SK, Kulakow PA, Peters I. Genetics and breeding of grain amaranth: some research tissues and findings. In: Proc 3rd Amaranth Conf . Emmaus, PA: Rodale Press (1986), p. 174–91.
182. Stetter MG, Müller T, Schmid KJ. Genomic and phenotypic evidence for an incomplete domestication of South American grain amaranth (Amaranthus caudatus). Mol Ecol. (2017) 26:871–86. doi: 10.1111/mec.13974
184. Pandey RM. Heritability and genetic advance in a diallel of grain amaranth. Indian Bot Rep. (1985) 4:183–4.
185. Pandey RM, Pal M. Heterotic effect for protein content in Amaranthus hypochondriacus L. Crop Improv. (1980) 7:71–3.
186. Pal M, Pandey RM. Cytogenetics and evolution of grain amaranths. J Plant Sci. (1989) 11:323–36.
188. Pal M, Pandey RM, Khoshoo TN. Evolution and improvement of cultivated amaranths. IX. Cytogenetic relationship between the two basic chromosome numbers. J Hered. (1982) 73:353–6.
189. Lightfoot DJ, Jarvis DE, Ramaraj T, Lee R, Jellen EN, Maughan PJ, et al. Single-molecule sequencing and Hi-C-based proximityguided assembly of amaranth (Amaranthus hypochondriacus) chromosomes provide insights into genome evolution. BMC Biol. (2017) 15:74. doi: 10.1186/s12915-017-0412-4
190. Xu F, Sun M. Comparative analysis of phylogenetic relationships of grain amaranths and their wild relatives (Amaranthus; Amaranthaceae) using internal transcribed spacer, amplified fragment length polymorphism, and double-primer fluorescent intersimple sequence repeat markers. Mol Phylogenet Evol. (2001) 21:372–87. doi: 10.1006/mpev.2001.1016
191. Silvestri V, Gil F. Alogamia en quinua. Tasa en Mendoza (Argentina) Revista de la facultad de Ciencias Agrarias. Univ Nac Cuyo. (2000) 32:71–6.
192. Murphy KM, Matanguihan JB, Fuentus FF, Gomez-Pando LR, Jellen EN, Maughan PJ, et al. Quinoa breeding and genomics. Plant Breed Rev. (2018) 42:257–320. doi: 10.1002/9781119521358.ch7
193. Murphy KM, Bazile D, Kellogg J, Rahmanian M. Development of a worldwide consortium on evolutionary participatory breeding in quinoa. Front Plant Sci. (2016) 7:608. doi: 10.3389/fpls.2016.00608
194. Wilson HD. Allozyme variation and morphological relationships of Chenopodium hircinum Schrader (s. lat). Syst Bot. (1988) 13:215–28. doi: 10.2307/2419100
195. Wilson HD. Quinua biosystematics I. Domestic populations. Econ Bot. (1988) 42:461–77. doi: 10.1007/BF02862791
196. Bonifacio A. Improvement of quinoa (Chenopodium quinoa willd.) and qañawa (Chenopodium pallidicaule Aellen) in the context of climate change in the high Andes. Cienc Inv Agr. (2019) 46:113–24. doi: 10.7764/rcia.v46i2.2146
197. Fairbanks DJ, Burgener K, Robison LR, Anderson WR, Ballon E. Electrophoretic charactersization of quinoa seed proteins. Plant Breed. (1990) 104:190–5. doi: 10.1111/j.1439-0523.1990.tb00422.x
198. Bhargava A, Shukla S, Ohri D. Genetic association in Chenopodium. Ind J Genet Plant Breed. (2003) 66:283–4.
199. Bhargava A, Rana TS, Shukla S, Ohri D. Seed protein electrophoresis of some cultivated and wild species of Chenopodium (Chenopodiaceae). Biol Plant. (2005) 49:505–11. doi: 10.1007/s10535-005-0042-5
200. Fairbanks D, Waldrigues A, Ruas CF, Maughan PJ, Robison LR, Andersen WR, et al. Efficient characterization of biological diversity using field DNA extraction and random amplified polymorphic DNA markers. Rev Brazil Genet. (1993) 16:11–22.
201. Ruas PM, Bonifacio A, Ruas CF, Fairbanks DJ, Anderson WR. Genetic relationships among 19 accessions of six species of Chenopodium L. by random amplified polymorphic DNA fragments (RAPD). Euphytica. (1999) 105:25–32. doi: 10.1023/A:1003480414735
202. Del Castillo C, Winkel T, Mahy G, Bizoux JP. Genetic structure of quinoa (Chenopodium quinoa Willd) from the Bolivian Altiplano as revealed by RAPD markers. Gen Res Crop E. (2007) 54:897–905. doi: 10.1007/s10722-006-9151-z
203. Jarvis DE, Kopp OR, Jellen EN, Mallory MA, Pattee J, Bonifacio A, et al. Simple sequence repeat marker development and genetic mapping in quinoa (Chenopodium quinoa Willd.). J Genet. (2008) 87:39–51. doi: 10.1007/s12041-008-0006-6
204. Mason SL, Stevens MR, Jellen EN, Bonifacio A, Fairbanks DJ, Coleman CE, et al. Development and use of microsatellite markers for germplasm characterization in quinoa (Chenopodium quinoa Willd.). Crop Sci. (2005) 45:1618–30. doi: 10.2135/cropsci2004.0295
205. Maughan PJ, Bonifacio A, Jellen EN, Stevens MR, Coleman CE, Ricks M, et al. A genetic linkage map of quinoa (Chenopodium quinoa) based on AFLP, RAPD, and SSR markers. Theor Appl Genet. (2004) 109:1188–95. doi: 10.1007/s00122-004-1730-9
206. Christensen SA, Pratt DB, Pratt C, Nelson PT, Stevens MR, Jellen EN, et al. Assessment of genetic diversity in the USDA and CIP-FAO international nursery collections of quinoa (Chenopodium quinoa Willd.) using microsatellite markers. Plant Genet Res. (2007) 5:82–95. doi: 10.1017/S1479262107672293
207. Rana TS, Narzary D, Ohri D. Genetic diversity and relationships among some wild and cultivated species of Chenopodium L. (Amaranthaceae) using RAPD and DAMD methods. Curr Sci. (2010) 98:840–6.
208. Fuentes F, Bazile D, Bhargava A, Martínez EA. Implications of farmers' seed exchanges for on-farm conservation of quinoa, as revealed by its genetic diversity in Chile. J Agric Sci. (2012) 150:702–16. doi: 10.1017/S0021859612000056
209. Coles ND, Coleman CE, Christensen SA, Jellen EN, Stevens MR, Bonifacio A, et al. Development and use of an expressed sequenced tag library in quinoa (Chenopodium quinoa Willd.) for the discovery of single nucleotide polymorphisms. Plant Sci. (2005) 168:439–47. doi: 10.1016/j.plantsci.2004.09.007
210. Zurita-Silva A, Fuentes F, Zamora P, Jacobsen S, Schwember A. Breeding quinoa (Chenopodium quinoa Willd.): potential and perspectives. Mol Breed. (2014) 34:13–30. doi: 10.1007/s11032-014-0023-5
211. Štorchová H, Drabešová J, Cháb D. The introns in FLOWERING LOCUS T-LIKE (FTL) genes are useful markers for tracking paternity in tetraploid Chenopodium quinoa Willd. Genet Resour Crop E. (2015) 62:913–25. doi: 10.1007/s10722-014-0200-8
212. Zhang TF, Qi WC, Gu MF, Zhang X-L, Li T, Zhao H. Exploration and transferability evaluation of EST-SSRs in quinoa. Acta Agron Sin. (2016) 42:492–500. doi: 10.3724/SP.J.1006.2016.00492
213. Yasui Y, Hirakawa H, Oikawa T, Toyoshima M, Matsuzaki C, Ueno M, et al. Draft genome sequence of an inbred line of Chenopodium quinoa, an allotetraploid crop with great environmental adaptability and outstanding nutritional properties. DNA Res. (2016) 23:535–46. doi: 10.1093/dnares/dsw037
214. Patiranage DSR, Rey E, Emrani N, Wellman G, Schmid K, Schmockel SM, et al. Genome-wide association study in Quinoa reveals selection pattern typical for crops with a short breeding history. Genet Genomics. (2022) 11:e66873. doi: 10.7554/eLife.66873
215. Hong SY, Cheon KS, Yoo KO, Lee HO, Cho KS, Suh JT, et al. Complete chloroplast genome sequences and comparative analysis of Chenopodium quinoa and C. album. Front Plant Sci. (2017) 8:1696. doi: 10.3389/fpls.2017.01696
216. Ana-Cruz MC, Helena ME, Yacenia MC. Molecular characterization of Chenopodium quinoa Willd. using inter-simple sequence repeat (ISSR) markers. Afr J Biotechnol. (2017) 16:483–9. doi: 10.5897/AJB2017.15925
217. Zhang T, Gu M, Liu Y, Lv Y, Zhou L, Lu H, et al. Development of novel InDel markers and genetic diversity in Chenopodium quinoa through whole-genome resequencing. BMC Genom. (2017) 18:685–700. doi: 10.1186/s12864-017-4093-8
218. Zou C, Chen A, Xiao L, Muller HM, Ache P, Haberer G, et al. A high-quality genome assembly of quinoa provides insights into the molecular basis of salt bladder-based salinity tolerance and the exceptional nutritional value. Cell Res. (2017) 27:1327–40. doi: 10.1038/cr.2017.124
219. Al-Naggar AMM, Abd El-Salam RM, Badran AEE, El-Moghazi MM. Molecular differentiation of five quinoa (Chenopodium quinoa Willd.) genotypes using inter-simple sequence repeat (ISSR) markers. Adv Trends Biotechnol Genet. (2019) 130:130–41. doi: 10.9734/BJI/2017/37053
220. Ibrahim SD, Abd El-Hakim AF, Ali HE, Abd El-Maksoud RM. Genetic differentiation usingISSR, SCoT and DNA barcoding for Quinoa genotypes. Arab J Biotech. (2019) 22:103–18.
221. Devi RJ, Chrungoo NK. Species relationships in Chenopodium quinoa and Chenopodium album on the basis of morphology and SDS-PAGE profiles of soluble seed proteins. J App Biol Biotech. (2015) 3:29–33. doi: 10.7324/jabb.2015.3605
222. Wang MX, Wu XT, Zou JW, Zhang J, Wang XY, Chang X, et al. Genome wide microsatellite characterisation and marker development in Chenopodium quinoa. Ann Appl Biol. (2019) 175:415–23. doi: 10.1111/aab.12545
223. Romero M, Sanchez AMM, Pineda E, Ccamapaza Y, Zavalla N. Genetic identity based on simple sequence repeat (SSR) markers for Quinoa (Chenopodium quinoa Willd.). Cienc Investig Agra. (2019) 46:166–8. doi: 10.7764/rcia.v46i2.2144
224. Adolf VI, Jacobsen SE, Shabala S. Salt tolerance mechanisms in quinoa (Chenopodium quinoa Willd.). Environ Exp Bot. (2013) 92:43–54. doi: 10.1016/j.envexpbot.2012.07.004
225. Wang Y, Zhang Y, Dai C, Ma J, Zhou Y, Jaikishun S, et al. The Establishment of Two Efficient Transformation Systems in Quinoa. (2021). doi: 10.21203/rs.3.rs-364280/v1
226. Maldonado Taipe NF. Quantitative Trait Loci (QTL) Mapping and Genome-wide Transcriptome Analysis to Identify Agronomically Important Genes in Quinoa (Chenopodium quinoa) [PhD diss]. Faculty of Agricultural and Nutritional Sciences, Christian Albrechts University, Kiel (2022).
227. Hesami M, Daneshvar MH. Development of a regeneration protocol through indirect organogenesis in Chenopodium quinoa Wild. Indo-Am J Agric Vet Sci. (2016) 4:25–32. doi: 10.1007/ijlbpr_56e24bb54f0a9.pdf
228. Bennici A, Grifoni T, Schiff S, Bovelli R. Studies on callus growth and morphogenesis in several species and lines of Amaranthus. Plant Cell Tissue Organ Cult. (1997) 49:29–33. doi: 10.1023/A:1005882322044
229. Flores HF, Their A, Galston AW. In vitro culture of grain and vegetable amaranths (Amaranthus spp.). Am J Bot. (1982) 69:1049–54. doi: 10.1002/j.1537-2197.1982.tb13350.x
230. Gajdosova A, Libiaková G, Iliev I, Hricová A. Adventitious shoots induction of Amaranthus cruentus L. Propag Ornam Plants. (2013) 13:33–9. Available online at: https://www.researchgate.net/publication/268501670_Adventitious_shoots_induction_of_Amaranthus_cruentus_L_in_vitro
231. Locy RD, Fisher LC. Plant regeneration from tissue cultures of Amaranthus species. In: Henke RR, Hughes KW, Constantin MJ, Hollaender A, Wilson CM, ediors. Tissue Culture in Forestry and Agriculture. Basic Life Sciences, Vol 32. Boston, MA: Springer (1985) 331. doi: 10.1007/978-1-4899-0378-5_42
232. Flores HE, Teutonico RA. Amaranths (Amaranthus spp.): potential grain and vegetable crops. In:Bajaj YPS, , editor. Biotechnology in Agriculture and Forestry, Crops I. Berlin: Springer-Verlag (1986), p. 568–77. doi: 10.1007/978-3-642-61625-9_32
233. Tisserat B, Galletta PD. In vitro flowering in Amaranthus. HortScience. (1988) 23:210–2. doi: 10.21273/HORTSCI.23.1.210
234. Bennici A, Schiff S, Bovelli R. In vitro culture of species and varietes of four Amaranthus L. species. Euphytica. (1992) 62:181–6. doi: 10.1007/BF00041752
235. Arya ID, Chakravarty TN, Sopory SK. Development of secondary inflorescenses and in vitro plantlets from inflorescence cultures of Amaranthus paniculatus. Plant Cell Rep. (1993) 12:286–8. doi: 10.1007/BF00237137
236. Bagga S, Venkateswari K, Sopory SK. In vitro regeneration of plants from hypocotyl segments of Amaranthus paniculatus. Plant Cell Rep. (1987) 6:183–4. doi: 10.1007/BF00268473
237. Jofre-Garfias AE, Sepúlveda V, Cabrera-Ponce JL, Adam e-Alvarez RM, Herrera-Estrella L, Simpson J. Agrobacterium - mediated transformation of Amaranthushypochondriacus: light- and tissue-specific expression of a pea chlorophyll a/b-binding protein promoter. Plant Cell Rep. (1997) 16:847–52. doi: 10.1007/s002990050332
238. Pal A, Swain SS, Das AB, Mukherjee AK, Chand PK. Stable germ line transformation a leafy vegetable crop amaranth (Amaranthus tricolor L.) mediated by Agrobacterium tumefaciens. In Vitro Cell Dev Biol Plant. (2013) 49:114–28. doi: 10.1007/s11627-013-9489-9
239. Antognoni F, Faudale M, Poli F, Biondi S. Methyl jasmonate differentially affects tocopherol content and tyrosine amino transferase activity in cultured cells of Amaranthus caudatus and Chenopodium quinoa. Plant Biol. (2009) 11:161–9. doi: 10.1111/j.1438-8677.2008.00110.x
240. Swain SS, Sahu L, Barik DP, Chand PK. Genetic transformation of Amaranthus tricolor L. using Ri plasmid vectors. In:Bastia AK, Mohapatra UB, , editors. Recent Trends in Monitoring and Bioremediation of Mine and Industrial Environment. Proc Natl Sem. Orissa: North Orissa University (2009), p. 109–16.
241. Dănăilă Guidea S, Băbeanu N, Popa O, Stanciu D, Popa I. Preliminary studies on in vitro behavior of various somatic explants from some cultivated Amaranthus genotypes. Sci Bull Biotechnol. (2012) 16:9–14. doi: 10.1007/BF00234140
242. Amin MAM, Hasbullah NA, Azis NA, Daud NF, Rasad FM, Lassim MM. Morphogenesis studies in Amaranthus gangeticus in vitro. In: Proc. International Conference on Agricultural, Ecological and Medical Science. Phuket (2015).
243. Comia-Yebron R, Aspuria ET, Bernardo EL. Callus induction in Amaranthus tricolor and Amaranthus spinosus. J Int Soc Southeast Asian Agric Sci. (2017) 23:12–23.
244. Gong Y, Guo S, Wu X, Chen S, You C. Direct organogenesis protocol for in vitro propagation of Chenopodium quinoa. Plant Cell Tissue Organ Cult. (2022) 1–15. doi: 10.21203/rs.3.rs-1935859/v1
245. Henarejos-Escudero P, Guadarrama-Flores B, Guerrero-Rubio MA, Gomez-Pando LR, García-Carmona F, Gandía-Herrero F, et al. Developement of betalanin producing callus lines from colored quinoa varieties (Chenopodium quinoa Willd). J Agric Food Chem. (2018) 66:467–74. doi: 10.1021/acs.jafc.7b04642
246. Eisa S, Koyro H, Kogel K. Induction of somatic embryogenesis in cultured cells of Chenopodium quinoa. Plant Cell Tiss Organ Cult. (2005) 81:243–6. doi: 10.1007/s11240-004-4793-z
247. De Cleene M, De Ley J. The host range of crown gall. Bot Rev. (1976) 42:389–466. doi: 10.1007/BF02860827
248. Swain SS, Sahu L, Barik DP, Chand PK. Agrobacterium plant factors influencing transformation of “Joseph's coat” (Amaranthus tricolor L.). Sci Horticult. (2010) 125:461–8. doi: 10.1016/j.scienta.2010.04.034
249. Munusamy U, Abdullah SNA, Aziz MA, Khazaai H. Female reproductive system of Amaranthusas the target for Agrobacterium-mediated transformation. Adv Biosci Biotechnol. (2013) 4:188–92. doi: 10.4236/abb.2013.42027
250. Murugan SB, Sathishkumar R. Establishment of high frequency callus induction and genetic transformation in neglected leafy vegetable Amaranthus trisis. Austin J Biotechnol Bioeng. (2016) 3:1058.
251. Kuluev BR, Mikhaylova EV, Taipova RM, Chemeris AV. Changes in phenotype of transgenic amaranth Amaranthus retroflexus L. overexpressing ARGOS-LIKE gene. Russ J Genet. (2017) 53:67–75. doi: 10.1134/S1022795416120061
252. Taipova RM, Musin KG, Kuluev BR. Agrobacterium- mediated transformation of Amaranthus cruentus L. Epicotils. J Sib Fed. (2019) 13:179–87. doi: 10.17516/1997-1389-0292
253. Yaroshko O, Vasylenko M, Gajdošová A, Morgun B, Khrystan O, Velykozhon L, et al. “Floral-dip” transformation of Amaranthus caudatus L. hybrids A caudatus A paniculatus L. Biologija. (2018) 64:321–30. doi: 10.6001/biologija.v64i4.3904
254. Yaroshko OM, Kuchuk MV. Agrobacterium – caused transformation of cultivars Amaranthus caudates L. hybrids of A caudatusL x A paniculatus L. Int J Second Metab. (2018) 5:312–8. doi: 10.21448/ijsm.478267
255. Yaroshko OM, Morgun BV, Velykozhon LG, Gajdośova A, Andrushenko OL. PCR analyses of first generation plants of Amaranthus caudatus L. after “floral-dip” genetic transformation. Fiziol Rast Genet. (2020) 52:128–39. doi: 10.15407/frg2020.02.128
256. Castellanos-Arévalo A, Estrada-Luna A, Cabrera-Ponce J, Valencia-Lozano E, Herrera-Ubaldo H, de Folter S, et al. Agrobacterium rhizogenes-mediated transformation of grain (Amaranthus hypochondriacus) and leafy (A. hybridus) amaranths. Plant Cell Rep. (2020) 39:1143–60. doi: 10.1007/s00299-020-02553-9
257. Taipova RM, Musin KG, Kuluev BR. Obtaining hairy roots of Amaranthus cruentus L. evaluation of their growth indicator. Ekobioteh. (2019) 2:574–81. doi: 10.31163/2618-964X-2019-2-4-574-581
258. Xiao X, Fanxiao M, Viswanathan S, Xi Y, Lei M. An Agrobacterium-mediated transient expression method contributes to functional analysis of a transcription factor and potential application of gene editing in Chenopodium quinoa. Plant Cell Rep. (2022) 41:1975–85. doi: 10.1007/s00299-022-02902-w
259. Saad-Allah KM, Youssef MS. Phytochemical and genetic characterization of five quinoa (Chenopodium quinoa Willd.) genotypes introduced to Egypt. Physiol Mol Biol Plants. (2018) 24:617–29. doi: 10.1007/s12298-018-0541-4
260. Ma X, Vaistij FE, Li Y, Van Rensburg WSJ, Harvey S, Bairu MW, et al. A chromosome-level Amaranthus cruentus genome assembly highlights gene family evolution and biosynthetic gene clusters that may underpin the nutritional value of this traditional crop. Plant J. (2021) 107:613–28. doi: 10.1111/tpj.15298
261. Bodrug SA, Stralis PN, Buerstmayr H, Dohm JC, Himmelbauer H. Quinoa genome assembly employing genomic variation for guided scaffolding. Theor Appl Genet. (2021) 134:3577–94. doi: 10.1007/s00122-021-03915-x
262. Ma X, Zhang Q, Zhu Q. A robust CRISPR/Cas9 system for convenient, high-efficiency multiplex genome editing in monocot and dicot plants. Mol Plant. (2015) 8:1274–84. doi: 10.1016/j.molp.2015.04.007
263. Lowder LG, Zhang D, Baltes NJ, Paul JW, Tang X, Zheng X, et al. A CRISPR/Cas9 toolbox for multiplexed plant genome editing and transcriptional regulation. Plant Physiol. (2015) 169:971–85. doi: 10.1104/pp.15.00636
264. Qi W, Zhu T, Tian Z, Li C, Zhang W, Song R, et al. High-efficiency CRISPR/Cas9 multiplex gene editing using the glycine tRNA-processing system-based strategy in maize. BMC Biotechnol. (2016) 16:58. doi: 10.1186/s12896-016-0289-2
265. Cermák T, Curtin SJ, Gil-Humanes J. A multipurpose toolkit to enable advanced genome engineering in plants. Plant Cell. (2017) 29:1196–217. doi: 10.1105/tpc.16.00922
266. Gao W, Long L, Tian X, Xu F, Liu J, Singh PK, et al. Genome editing in cotton with the CRISPR/Cas9 system. Front Plant Sci. (2017) 8:1364. doi: 10.3389/fpls.2017.01364
267. Kim H, Kim ST, Ryu J, Kang BC, Kim JS, Kim SG, et al. CRISPR/Cpf1- mediated DNA-free plant genome editing. Nat Commun. (2017) 8:14406. doi: 10.1038/ncomms14406
268. Wang W, Pan Q, He F, Akhunova A, Chao S, Trick H, et al. Transgenerational CRISPR–Cas9 activity facilitates multiplex gene editing in allopolyploid wheat. CRISPR J. (2018) 65–74. doi: 10.1089/crispr.2017.0010
269. Zhang Z, Hua L, Gupta A, Tricoli D, Edwards KJ, Yang B, Li W. Development of an Agrobacterium-delivered CRISPR/Cas9 system for wheat genome editing. Plant Biotechnol J. (2019) 17:1623–35. doi: 10.1111/pbi.13088
270. Zafar SA, Zaidi SS, Gaba Y, Singla-Pareek SL, Dhankher OP, Li X, et al. Engineering abiotic stress tolerance via CRISPR/Cas-mediated genome editing. J Experimental Bot. (2020) 71:470–9. doi: 10.1093/jxb/erz476
271. Liang Z, Chen K, Zhang Y, Liu J, Yin K, Qiu JL, et al. Genome editing of bread wheat using biolistic delivery of CRISPR/Cas9 in vitro transcripts or ribonucleoproteins. Nat Protoc. (2018) 13:413–30. doi: 10.1038/nprot.2017.145
272. Johansen IE, Liu Y, Jørgensen B, Bennett EP, Andreasson E, Nielsen KL, et al. High efficacy full allelic CRISPR/Cas9 gene editing in tetraploid potato. Sci Rep. (2019) 9:17715. doi: 10.1038/s41598-019-54126-w
273. Da Silva LS, Taylor J, Taylor JR. Transgenic sorghum with altered kafirin synthesis: kafirin solubility, polymerization, and protein digestion. J Agric Food Chem. (2011) 59:9265–70. doi: 10.1021/jf201878p
274. Holme IB, Gregersen PL, Brinch-Pedersen H. Induced genetic variation in crop plants by random or targeted mutagenesis: convergence and differences. Front Plant Sci. (2019) 10:1468. doi: 10.3389/fpls.2019.01468
275. Mestanza C, Riegel R, Vásquez SC, Veliz D, Cruz-Rosero N, Canchignia H, et al. Discovery of mutations in Chenopodium quinoa Willd. through EMS mutagenesis and mutation screening using pre-selection phenotypic data and next-generation sequencing. J Agric Sci. (2018) 156:1196–204. doi: 10.1017/S0021859619000182
Keywords: genetic improvement, grain amaranth, quinoa, germplasm, nutrition
Citation: Anuradha, Kumari M, Zinta G, Chauhan R, Kumar A, Singh S and Singh S (2023) Genetic resources and breeding approaches for improvement of amaranth (Amaranthus spp.) and quinoa (Chenopodium quinoa). Front. Nutr. 10:1129723. doi: 10.3389/fnut.2023.1129723
Received: 22 December 2022; Accepted: 03 July 2023;
Published: 24 July 2023.
Edited by:
Natalie Laibach, Spanish National Research Council (CSIC), SpainReviewed by:
Ipsita Samal, Sri Sri University, IndiaDinesh Joshi, ICAR-Vivekananda Institute of Hill Agriculture, India
Didier BAZILE, Centre de Coopération Internationale en Recherche Agronomique pour le Développement (CIRAD), France
Juan Pablo Rodriguez, Julius Kühn-Institut, Germany
Copyright © 2023 Anuradha, Kumari, Zinta, Chauhan, Kumar, Singh and Singh. This is an open-access article distributed under the terms of the Creative Commons Attribution License (CC BY). The use, distribution or reproduction in other forums is permitted, provided the original author(s) and the copyright owner(s) are credited and that the original publication in this journal is cited, in accordance with accepted academic practice. No use, distribution or reproduction is permitted which does not comply with these terms.
*Correspondence: Satbeer Singh, c2F0YmVlciYjeDAwMDQwO2loYnQucmVzLmlu