- 1College of Food Science, Southwest University, Chongqing, China
- 2Chinese-Hungarian Cooperative Research Centre for Food Science, Chongqing, China
- 3Chongqing Key Laboratory of Speciality Food Co-Built by Sichuan and Chongqing, Chongqing, China
- 4College of Food and Pharmaceutical Engineering Institute, Guiyang University, Guiyang, Guizhou, China
To enhance the content of adlay bran soluble dietary fiber (SDF) and improve its functionality, we investigated the influences of steam explosion (SE) on the physicochemical, structural properties, and in vitro hypoglycemic activities of adlay bran SDF. The cellulose, hemicellulose, and lignin contents of adlay bran decreased significantly after SE treatment. When the SE strength was 0.8 MPa for 3 min, the SDF content was 9.37%, which was a significant increase of 27.48% compared to the control. Under these conditions, SDF showed the highest oil-holding capacity (OHC) (2.18 g/g), cholesterol adsorption capacity (CAC) (27.29 mg/g), glucose adsorption capacity (GAC) (15.54 mg/g), glucose dialysis retardation index (GDRI) (36.57%), and α-Amylase activity inhibition ratio (α-AAIR) (74.14%). Compared with SDF from untreated adlay bran, SDF from SE-treated adlay bran showed lower weight molecular. In addition, differential scanning calorimetry (DSC) measurement showed that the peak temperature of SDF from adlay bran treated by SE increased by 4.19°C compared to the untreated SDF sample. The structure of SDF from adlay bran treated by SE showed that the SDF surface was rough and poriferous and the specific surface areas increased. In conclusion, SE pretreatment increases the content of SDF in adlay bran and improves its physicochemical, structural properties, and biological activities, which will be beneficial for the further exploitation of adlay bran.
1. Introduction
Adlay (Coix lachryma-jobi L. var. ma-yuen Stapf) is a functional food in Asia that is also called Job’s tears and which has been proven to exhibit various anti-inflammatory (1), anti-cancer (2), and anti-allergic effects (3). Therefore, adlay is widely utilized and processed into a variety of products, and the processing of these products generates large amounts of adlay bran. Adlay bran, a primary by-product of adlay processing, is rich in a broad spectrum of nutrients, including phenolic, polysaccharides, fatty acids and dietary fiber (DF), all of which exhibit certain bioactivities, including anti-hemolysis, anti-proliferative, and xanthine oxidase inhibitory capacities (4, 5). However, adlay bran is typically used as feed or discarded as waste. This phenomenon results in a huge waste of resources and greatly reduces the overall economic value of adlay. In recent years, DF has received increasing attention in production applications of food industry due to its multiple health-promoting benefits. DF is a carbohydrate-based polymer whose intake is associated with overall metabolic health and which has been proposed to ameliorate a variety of other pathologies, including cardiovascular disease, colon dysfunction, impaired gut motility and colorectal carcinoma risk (6, 7). Not only does DF help regulate intestinal function, but it also increases stool volume and maintains stable postprandial blood glucose (8, 9). Based on its water solubility, DF is usually classified into soluble dietary fiber (SDF) and insoluble dietary fiber (IDF) (10). It is well-documented that SDF is primarily responsible for the bioactivities of DF, based on its physiological functions and physicochemical properties (11). For example, SDF showed stronger anti-hyperlipidemia activity than IDF, and it plays a major role in postprandial glucose and insulin response (12, 13). In addition, SDF has antioxidant activity, oil-holding capacity (OHC) and cholesterol-lowering capacity (14, 15). Adlay bran has the potential to be used as a source of DF; in particular SDF from adlay bran is similar in composition to adlay, endowing the adlay-bran-based products with additional functional properties. However, the low levels of SDF in natural DF and adlay bran limits food applications. Therefore, appropriate methods to increase the SDF content can make it more widely used in the food industry.
Currently, methods of DF modification have been reported, including physical (16), chemical (17), and biological methods (18). Among these, physical methods, which are used most commonly, have the advantages of high efficiency and environmental protection. Steam explosion (SE) is an emerging physical modification technique which mainly used in the treatment of wood fibers. During SE, a sample is rapidly heated by high-pressure water vapor for a given time, after which a rapid release of pressure causes the steam to expand within the sample, resulting in the separation of individual fibers and destruction of the raw material structures (19). Under these circumstances, the effects of acidolysis, mechanical breakage and thermal degradation can degrade and convert cellulose and hemicellulose into SDF. In addition, compared with other pretreatment methods, SE enables faster mass transfer, has a lower energy cost, requires less hazardous process chemicals, and involves simple technical manipulation (20, 21). In recent years, SE has also been used to modify DF, including improving the content of SDF from sweet potato residue (22), wheat bran (23), and okara (24). However, the application of SE in SDF extraction from adlay bran has not yet been reported, and the effects of SE on the physicochemical, structure properties and in vitro hypoglycemic activity of SDF from adlay bran are unclear.
Based on the aforementioned points, the objective of this present study was to investigate the influences of SE modification on the physicochemical, structure properties, and in vitro hypoglycemic activity of adlay bran SDF. To these ends, the adlay bran was treated by SE at different conditions. Then, SDF was extracted from adlay bran by enzymatic-hydrolysis method. Finally, the physicochemical properties [e.g., OHC, cholesterol adsorption capacity (CAC)] and in vitro hypoglycemic activity [e.g., glucose adsorption capacity (GAC), α-Amylase activity inhibition ratio (α-AAIR), glucose dialysis retardation index (GDRI)] of SDF before and after SE-treatment were determined and evaluated. In addition, scanning election microscopy (SEM), differential scanning calorimetry (DSC) and Fourier transform infrared spectroscopy (FT-IR) were also used to evaluate the effects of SE on the structure of SDF.
2. Materials and methods
2.1. Materials and chemicals
Adlay bran was purchased from a local rural supplier (Guizhou, China) and stored at 4°C until use. Cellulose reagent kit, hemicellulose reagent kit and lignin reagent kit were obtained from Suzhou Michy Biomedical Technology Co., Ltd. (Suzhou, China). Thermostable α-amylase (2,600 U/g) was supplied by Shanghai Aladdin Biochemical Technology (Shanghai, China). Alkaline protease (200 U/mg) and cholesterol standard were obtained from Beijing Solarbio Technology (Beijing, China). 3, 5-dinitrosalicylic acid was purchased from Guangzhou Caozhiyuan Biotechnology (Guangzhou, China). Glucose standard was purchased from Tianjin Solomon Biotechnology (Tianjin, China). Other chemical reagents were of analytical grade.
2.2. Steam explosion treatment
Based on preliminary laboratory studies, steam pressures of 0.8, 1.2, and 1.6 MPa were selected, and residence times were set at 1, 2, and 3 min. Approximately 500 g of adlay bran was loaded into the 2 L reactor of a SE system (QB-300, Zhang dao Co., Ltd., Hebi, China), and high-pressure steam was introduced into the reactor chamber. After the steam pressure has been maintained for a given time, and then pressure was released immediately. The adlay bran was collected after SE, dried in a hot blast oven (GZX-9140 MBE, Shanghai, China) at 50°C and crushed through a 60-mesh sieve. Crushed bran was then defatted by Soxhlet extraction using petroleum ether (boiling range 30–60°C) at 70°C for 10 h. Residual solvent was removed by hot air drying at 60°C, after which samples were stored at 4°C.
2.3. Extraction of adlay bran SDF and calculation of yield
Soluble dietary fiber was prepared using the enzymatic-hydrolysis method (21) with slight modifications. The pretreatment bran power was dispersed in 25 times volumes of phosphate buffer solution (pH 6.5), containing 100 μL thermostable α-amylase, and this solution was subjected to further hydrolysis at 98°C for 30 min. The hydrolysate was then cooled to 50°C and adjusted to a pH of 10.0 using 6 M NaOH solution. Subsequently, 80 μL of alkaline protease was added, and samples were subjected to further hydrolysis for 2 h. After enzymatic hydrolysis reaction, the hydrolysate was adjusted to a pH of 4.5 with 6 M HCI solution to inactivate enzymes. The supernatant was obtained by centrifugation at 4,000 × g for 10 min and evaporated to approximately one-tenth volume using rotary evaporation (RE-52, Shanghai, China). Subsequently, the concentrated supernatant was added into 95% (v/v) ethanol solution, incubated at 25°C for 12 h. Finally, the precipitate was obtained by centrifugation and dried at 60°C. The dried precipitate crushed, sifted through a 60-mesh sieve, and then stored at 4°C, the power obtained was SDF. The SDF yield was calculated as follows:
Where W1 is the weight of SDF obtained after drying (g); W is the weight of adlay bran sample (g).
2.4. Chemical composition assays
Levels of cellulose, hemicellulose, and lignin from adlay bran before and after SE pretreatment were assessed by the spectrophotometric method according to kit instructions.
2.5. Physicochemical properties of SDF from adlay bran
2.5.1. Oil-holding capacity (OHC)
The OHC was performed according to the method described by Wang et al. (21). A total of 0.5 g of SDF was mixed with 10 mL olive oil in a centrifugal tube and placed at 4°C for 1 h. Then the mixture was centrifuged at 4,200 × g for 15 min, the supernatant was removed and the residue was weighed. The OHC was calculated as follows:
Where W1 is the weights of SDF sample after oil absorption (g); W is the weight of SDF sample (g).
2.5.2. Cholesterol adsorption capacity (CAC)
The CAC was performed using the method described by Chen et al. (25) with slight modifications. Fresh egg yolk was whipped with nine volumes of hot distilled water. Accurately weigh 500 mg of SDF into 15 mL of diluted yolk emulsion. After that, the mixture was adjusted to a pH of 7.0 using NaOH solution, incubated at 37°C for 120 min, and then centrifuged. The supernatant was obtained for determination of cholesterol concentration by o-phthalaldehyde method. The CAC was calculated as follows:
Where Ci is the concentration of cholesterol in the diluted yolk emulsion; Cs is the concentration of cholesterol in the diluted yolk emulsion after SDF adsorption (mg/mL); W is the weight of SDF (g), and V is the volume of diluted yolk (mL).
2.6. In vitro hypoglycemic activity of SDF
2.6.1. Glucose adsorption capacity (GAC)
The GAC was determined according to the method by Ma and Mu (26) with slight modifications. Accurately weigh 1 g SDF into 100 mL of glucose solution (100 mmol/L). After incubation for 6 h at 37°C in an incubator, the supernatant was obtained by centrifugation. Finally, the concentration of glucose in the supernatant was measured using 3, 5- Dinitrosalicylic acid method. The GAC was calculated as follows:
Where W and Vi are the weight of SDF (g) and glucose solution volume (mL), respectively; Ci and Cs are the concentration of glucose in the supernatant before and after SDF adsorption (mg/mL), respectively.
2.6.2. α-Amylase activity inhibition ratio (α-AAIR)
The α-AAIR was evaluated according to the method described by Chau et al. (27). Briefly, 4 mg of α-amylase and 1 g of SDF were added into 40 mL of 4% starch solution, and the mixture was homogenized and oscillated at 37°C for 1 h. Then the mixture was centrifuged, the concentration of glucose in the supernatant was measured using 3, 5- Dinitrosalicylic acid method. α-AAIR was calculated as follows:
Where Cc and Cs are the concentration of glucose in the supernatant with and without SDF (mg/mL), respectively.
2.6.3. Glucose dialysis retardation index (GDRI)
The GDRI was determined using the method described by Tang et al. (28). Briefly, 500 mg of SDF was thoroughly hydrated with 15 mL of glucose solution (100 mmol/L), and then the solution was transferred to a 15 cm dialysis bag. The dialysis bag was put in a beaker containing 200 mL distilled water and incubated at 37°C for 2 h. Finally, at 0.5 h intervals the concentration of glucose in the dialysate was measured using 3, 5- Dinitrosalicylic acid method. The GDRI was calculated as follows:
Where Cs and Cc are the concentration of glucose in the dialysates with and without SDF, respectively.
2.7. Structural characteristics
2.7.1. Particle size distribution
The particle size distributions of SDF were measured with a laser particle size instrument (Mastersizer 3000, Malvern Instruments Co., Ltd., UK). Ethanol was used as a dispersant, the appropriate amount of SDF was taken and ultrasonically assisted to disperse it evenly. The particle size determination range was from 0.01 to 2,000 μm.
2.7.2. Scanning election microscopy (SEM)
The microstructure of SDF from adlay bran before and after SE pretreatment was obtained using a SEM (Phenom Pro, Phenom World, Holland). The dried SDF sample was fixed on double-sided conducting adhesive tapes and sputter-coated with gold (29). Subsequently, samples were observed using SEM at 10 kV.
2.7.3. Fourier transform infrared spectroscopy (FT-IR)
Each SDF sample was ground into power and analyzed by spectrum100 FTIR spectrometer (Spectrum 100,9PerkinElmore, USA). IR spectra of SDF samples were recorded from 4,000 to 600 cm–1 with a resolution of 4 cm–1.
2.7.4. Molecular weight distribution
The molecular weight of SDF was analyzed by gel permeation chromatography (GPC). Samples (10 mg) of SDF from untreated and SE-treated adlay bran were dissolved in 1 mL ultrapure water. Then, the mixture was filtered by 0.45 μm membranes. The chromatographic conditions were as follows: gel chromatography column was TSKgel GMPWXL (TOSOH CORP., Japan), mobile phase was 0.1 mol/L NaCO3 at a flow rate of 0.6 mL/min, and injection volume was 100 μL.
2.7.5. Monosaccharide composition
Soluble dietary fiber sample and 2 ml/L trifluoroacetic acid (3 mL) were added to 10 mL ampoules, hydrolyzed at 120°C for 4 h. After removing the trifluoroacetic acid with methanol, the residue was dissolved in 3 mL of water to obtain the polysaccharide hydrolysate. Then, 250 μL of hydrolysate was derivatized with 250 μL 0.6 mol/L NaOH solution and 500 μL 0.4 mol/L PMP-methanol solution for 1 h at 70°C. After cooling to room temperature, 500 μL of 0.3 mol/L HCl was added to neutralize the reaction products. The mixture was centrifuged for 10 min to obtain the supernatant. Then, 1 mL of chloroform was added to the supernatant and the extraction was repeated three times. The analysis conditions were as follows: chromatographic column: Xtimate (C18) column (250 mm × 4.6 mm, 5 μm), column temperature: 30°C, flow rate: 1.0 mL/min and injection volume was 20 μL.
2.7.6. Thermal stability analysis
Thermal analysis of SDF samples from untreated and SE-pretreated adlay bran were analyzed with a differential scanning calorimeter (DSC25, TA Instruments Co., Ltd., USA). Approximately 15 mg of SDF sample was put in a crucible and an empty crucible was used as a control. The crucibles were heated from 30 to 300°C at 50°C/min with a nitrogen atmosphere flow of 50 mL/min.
2.8. Statistical analysis
Each experiment was repeated at least in triplicate. The data was subjected to analysis of variance testing using SPSS 26.0 software. The data were expressed as mean ± standard deviation (X ± SD). Differences were considered statistically significant when p < 0.05 according to Duncan’s multiple range tests.
3. Results and discussion
3.1. Effects of steam pressure and residence time on SDF content
The influence of different steam pressure and residence time on the content of SDF from adlay bran was presented in Figure 1. SE treatment (except 1.6 MPa, 1 min) significantly improved (p < 0.05) the content of SDF by contrast with the untreated control. Moreover, the SDF content generally increased with steam pressure decreased and residence time increased. The highest yield was 9.37% at 0.8 MPa for 3 min. This was significantly increased by 27.48% compared to the control (7.35%). This increase in SDF content is due to the fact that, during SE, material is rapidly swollen by superheated liquid for a given time, the steam into the tissue, after which the pressure is instantly released such that the steam volume abruptly expands (19). Mechanical action causes some fibers breakage and disrupts cell wall structures, which may facilitate dissolution of inclusive SDF and also increase SDF content.
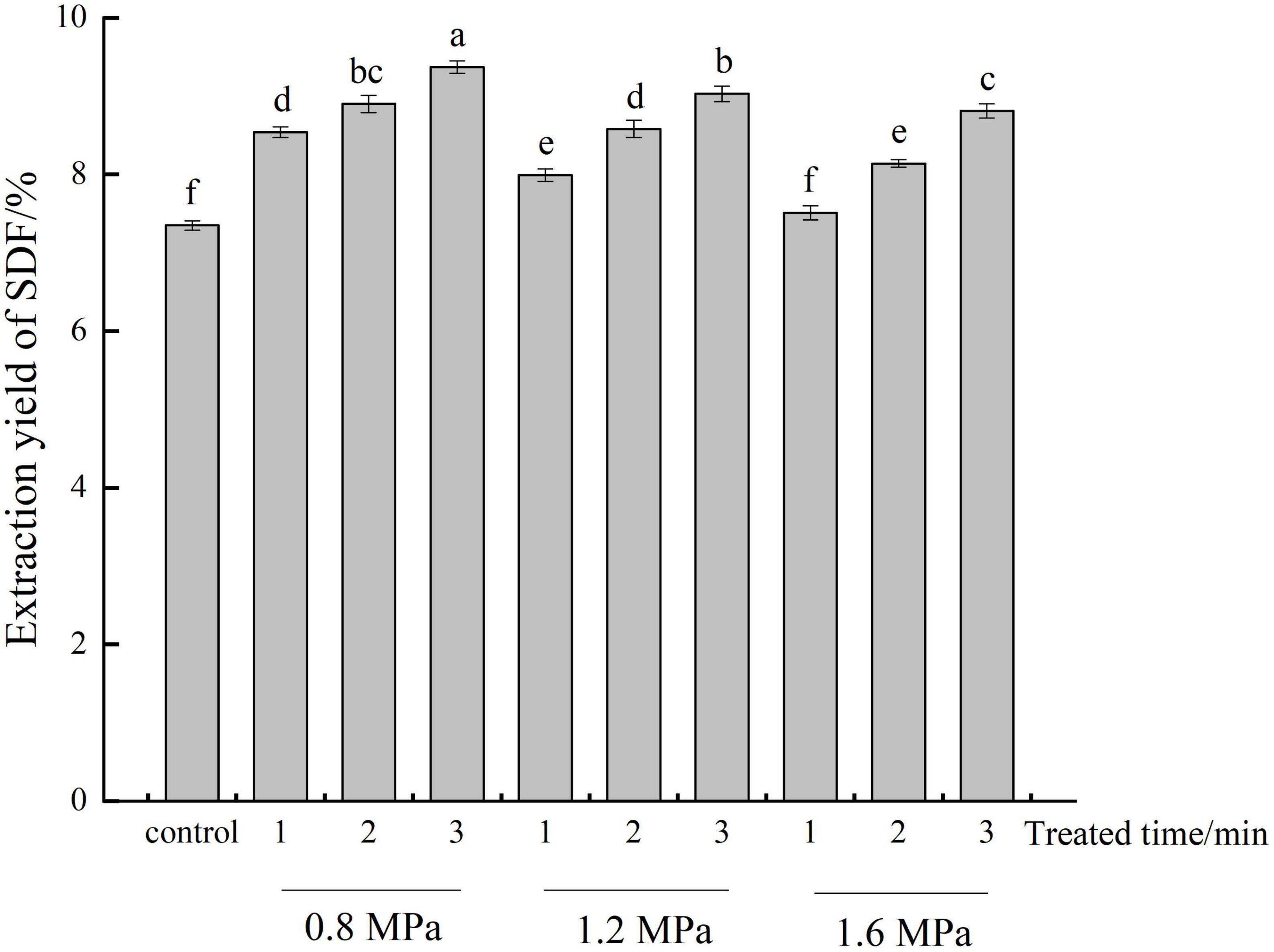
Figure 1. Extraction yields of soluble dietary fiber (SDF) at different steam explosion (SE) treatment conditions. Control: untreated adlay bran; adlay bran treated by SE at different steam pressures (0.8, 1.2, and 1.6 MPa) and different residence times (1, 2, and 3 min). Different lowercase letters over column indicate significant differences (p < 0.05).
The decrease in extraction yield at pressures beyond 0.8 MPa may be due to high pressure causes cellulose and lignin in the raw material to degrade into smaller molecules which are not precipitated with alcohol, resulting in a lower extraction rate of SDF (21). Shen et al. (30) reported that high SE intensity can cause cellulose, hemicellulose, and other substances to degrade into monosaccharides or oligosaccharides with smaller molecular weights, leading to decreased SDF content in soybean hull.
The SE reaction process includes two parts: pressurized high-temperature cooking and instantaneous release of high pressure. An increase in the residence time essentially extends the pressurized high-temperature cooking time; because to a certain extent, IDF are degraded and converted into SDF with the increase of residence time, the content of SDF keeps increasing (19). It was conclude that the SDF content from adlay bran can be increased by SE pretreatment, and optimized SDF extraction rates can be achieved using a suitable range of steam pressures and residence times.
3.2. Chemical compositions
Adlay bran contains a lot of cellulose, hemicellulose, and lignin. These components are interconnected by chemical bonds and hydrogen bonds, making them difficult to separate (31). The instantaneous mechanical action of SE, high pressure and the rapid expansion of water vapor can break the connections between cellulose, hemicellulose, and lignin, and promoting the breakage of glycosidic bonds in large molecules, thus increasing SDF content (32). The cellulose, hemicellulose, and lignin contents of adlay bran pretreated under different steam explosion intensity treatments were determined as shown in Table 1. By contrast with the untreated control, the content of cellulose, hemicellulose and lignin in adlay bran decreased significantly after SE treatment. To be precise, the contents of the three compounds in the adlay bran sample after SE pretreatment at 1.6 MPa for 3 min were reduced by 40.44, 9.65, and 34.95%, respectively, in comparison with the control. This is consistent with other the findings of reduced cellulose, hemicellulose and lignin contents in steam-exploded materials (31). Tanpicha et al. (32) concluded that the SE technique can partially eliminate hemicellulose and lignin from pineapple leaves. Nie et al. (33) concluded that hemicellulose in corn stover was partially degraded during SE treatment. Zhao et al. (34) found that the cellulose and hemicellulose contents of sorghum decreased after SE treatment.
The degradation of cellulose and hemicellulose may be due to the fact that glycosidic and hydrogen bonds in the fibers are broken by SE treatment, causing them to form small molecules of reducing sugars to be leached (35). After SE treatment, cellulose, hemicellulose, and lignin are partially degraded into small molecules, thus increasing the SDF content.
3.3. Physicochemical properties of SDF
3.3.1. OHC
The OHC of SDF is an important indicator to maintain food flavor (36). As shown in Figure 2A, SE pretreatment effectively increased (p < 0.05) the OHC of SDF. Moreover, increasing residence time generally led to SDF with stronger OHC. The OHC of SDF treated by SE was increased from 1.48 g/g to 2.18 g/g. The maximum OHC of SDF was obtained at 0.8 MPa, 3 min, which was 1.47-fold greater than that of the control. This increase in OHC was probably caused by the increased in short-chain DF content after SE, which increased oil absorption (22). Kong et al. (37) reported that the increase in OHC for wheat bran power might be attributed to the SE-assisted superfine grinding process changing the spatial structure of the bran, with its porosity and looseness allowing the bran power to hold more oil molecules. In addition, Wang et al. (22) also reported that the spatial structure of SDF is altered by SE, with looseness and swelling properties allowing SDF to accommodate more oil molecules. Since SDF absorbs fat, increases satiety, and increases bowel motility, high OHC can help with the control of weight (38).
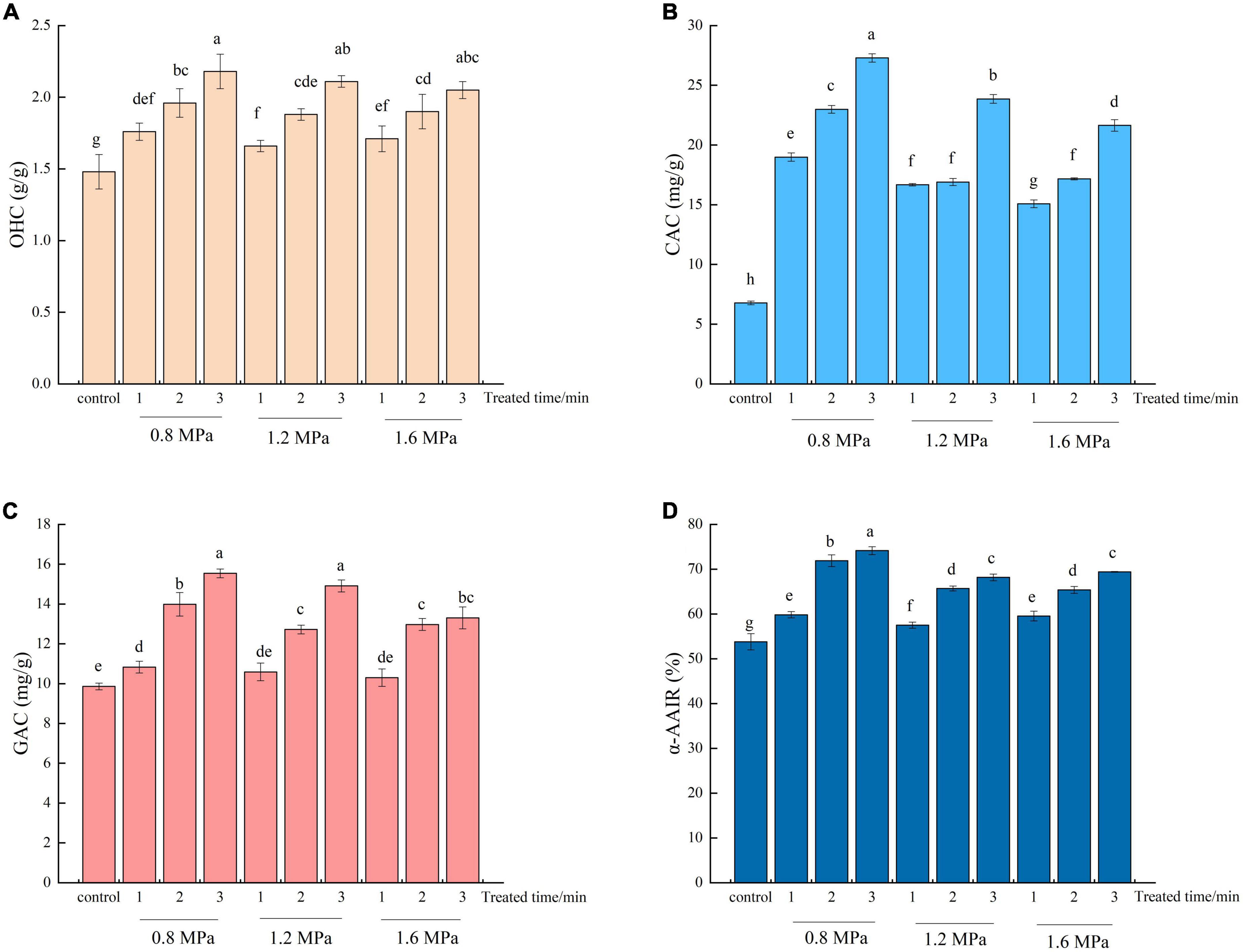
Figure 2. The oil holding capacity (OHC) (A), cholesterol adsorption capacity (CAC) (B), glucose adsorption capacity (GAC) (C) and α-Amylase activity inhibition ratio (α-AAIR) (D) of adlay bran soluble dietary fiber before and after steam explosion (SE). Different lowercase letters over column indicate significant differences (p < 0.05).
3.3.2. CAC
Dietary fibers with high cholesterol adsorption capacities can exhibit hypolipidemic effects in vivo (39). CAC is an essential indicator for the evaluation the hypolipidemic performance of DFs (40). According to the results shown in Figure 2B, SE pretreatment significantly enhances cholesterol absorption capacity. The CAC of SDF increased with increasing residence time at a certain pressure. After SE processing, the CAC value significantly increased, especially for samples treated by SE at 0.8 MPa, 3 min, which reached values of up to 27.29 mg/g. Zhai et al. (41) reported that the surface of SE-SDF exhibits more binding sites, promoting adsorption. Moreover, SE disrupted the internal disordered structure of SDF, increasing the exposure of polar groups on the surface, which was more favorable to its adsorption of cholesterol (42). Kong et al. (37) also found that SE-treated wheat bran powder exhibited superior cholesterol adsorption. The high CAC of SDF from adlay bran treated by SE may indicate a high potential hypocholesterolemic effect and represent a valuable dietary resource for cholesterol-lowering foods.
3.4. Hypoglycemic capacity of SDF
3.4.1. GAC
Glucose adsorption capacity is a significant indicator for accessing the ability of SDF to adsorb glucose (36). The GAC of SDF from adlay bran was presented in Figure 2C. SE increased significantly the GAC of SDF (except 1.2 MPa, 1 min and 1.6 MPa, 2 min). The GAC of SDF increased from 9.85 to 15.54 mg/g after SE. The GAC of SDF pretreated with SE at 0.8 MPa, 3 min was the highest, representing a significant increase of 57.77% compared to the untreated adlay bran. After SE, the specific surface area of Rosa roxburghii pomace SDF was increased, as were the numbers of pores and folds, which was beneficial to the adsorption of glucose molecules (41). In addition, the modified SDF surface exposed more polar and non-polar groups, which further enhanced the interaction between DF and glucose molecules (43). The results above show that SE pretreatment can enhance the GAC of adlay bran SDF, delay glucose diffusion and reduce the effective concentration of glucose, which may be helpful to inhibit the increase postprandial blood glucose (28).
3.4.2. GDRI
The GDRI of SDF from adlay bran was presented in Table 2. SE significantly improved (p < 0.05) the GDRI of SDF from adlay bran compared to untreated control. The GDRI of SDF pretreated at 0.8 MPa for 3 min was the highest, with values of 36.57% at 30 min, 22.46% at 60 min, 23.84% at 90 min, and 18.30% at 120 min. Moreover, this decrease in GDRI values over time. The GDRI of DFs was related to microstructure and GAC (44). The enhanced GDRI of SDF following SE pretreatment might be attributed to the more porous surface structure associated with physical effects, potentially trapping glucose in the fiber network and thereby delaying the diffusion of glucose (18). In addition, SE increases the GAC of SDF, resulting in a higher GDRI. The results showed that SE can increased the GDRI of adlay bran SDF, delay glucose diffusion, which may be beneficial in delaying the absorption of glucose in the gastrointestinal tract (39).
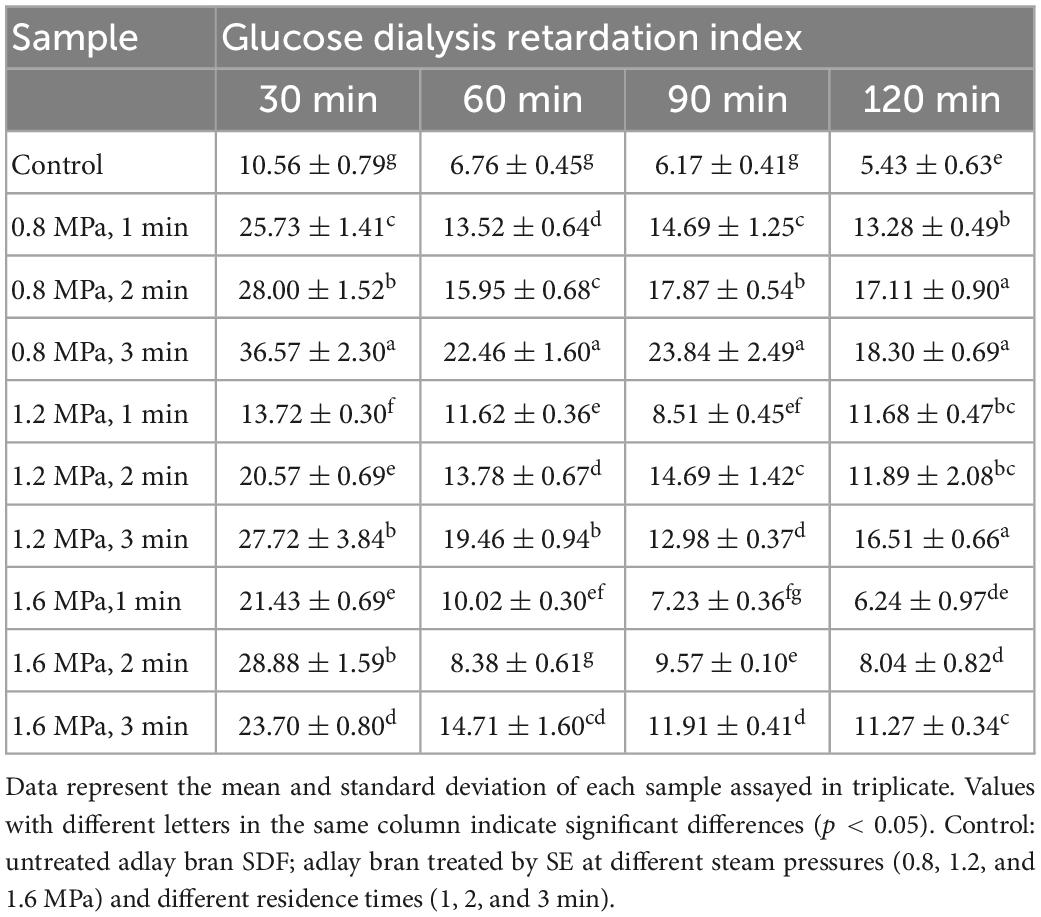
Table 2. Effect of steam explosion (SE) pretreatment on glucose dialysis retardation index (GDRI) of adlay bran soluble dietary fiber (SDF).
3.4.3. α-AAIR
The effects of SE pretreatment on the α-AAIR of SDF samples were presented in Figure 2D. Compared with untreated SDF, SE pretreatment increased significantly the α-AAIR of SDF, reaching a maximum value of 74.14% after 0.8 MPa for 3 min, which was 37.80% higher than the untreated control. DFs with high GAC can significantly reduce the contact between starch andα-amylase by adsorbing starch granules, thereby reducing α-amylase activity (26). Furthermore, a high GDRI indicates that SDF can effectively delay the diffusion of produced glucose, resulting in reduced α-amylase activity (45). Liu et al. (42) concluded that SE-R. roxburghii pomace SDF had a higher α-AAIR due to the fact that it adsorbs and immobilizes more enzymes after SE, resulting in more α-amylase inhibition. The a-AAIR results suggest that the enhanced abilities of the SE-SDF from adlay bran to reduce amylase activity are indicative of its potential to reduce glucose levels in serum.
3.5. Particle size properties
The particle size distributions of SDF from untreated adlay bran and SE-treated (0.8 MPa, 3 min) adlay bran were investigated and are presented in Table 3. The D50 value of SDF from untreated adlay bran was 72.80 μm, while that of SDF with SE treatment was 51.07 μm (p < 0.05). After SE treatment, D (4, 3) and D (3, 2) significantly decreased from 92.07 to 69.63 μm and from 32.30 to 24.60 μm, respectively, whereas the specific surface area of the SE-treated sample increased by 1.31-fold in comparison with the untreated control. These results showed that SE loosens the surface structure of SDF and decreases the particle size, resulting in increased surface area, which corroborates reported results for wheat bran powders and dietary fiber from R. roxburghii pomace (37, 41). The particle size distribution affects the functionality of fiber (46). The reduction in particle size may lead to changes in physicochemical properties and biological activities of SDF (26). Dubey et al. (47) reported that plant cellulose with small particle size exhibited enhanced capacities to hold water and bind sugars. SDF from adlay bran treated with SE had a larger specific surface area, facilitates physiological functions related to substance adsorption.
3.6. SEM analysis
Steam explosion treatment exposes the samples to high temperature and pressure, which is the most obvious and essential feature of SE technology. The surface morphology of SDF was shown in Figure 3, the structure of the material significantly changed after SE. Specifically, the surface of SDF from untreated adlay bran was dense and intact (Figures 3A–C). After magnification 4,000 times it is clear that the 0-SDF has an overall blocky appearance (Figure 3C). In contrast, after SE pretreatment, SDF showed honeycomb-like porous with rough and wrinkled surfaces (Figures 3D–F). The SE-SDF surface showed a fluffy structure with a significant increase in internal specific surface area and porosity when the magnification was 4,000 times (Figure 3F). This is because, during SE, water vapor penetrates into the cells and tissues of the adlay bran. When the high pressure is momentarily lifted, water within the sample rapidly expands and overflows, generating a huge shear force that destroys the fiber structure (48). Gan et al. (49) found that the degradation of cellulose and hemicellulose may promote the formation of cracked honeycomb structures on the surface. After SE, the cellulose and hemicellulose contents of adlay bran decreased, resulting in a honeycomb structure of SDF.
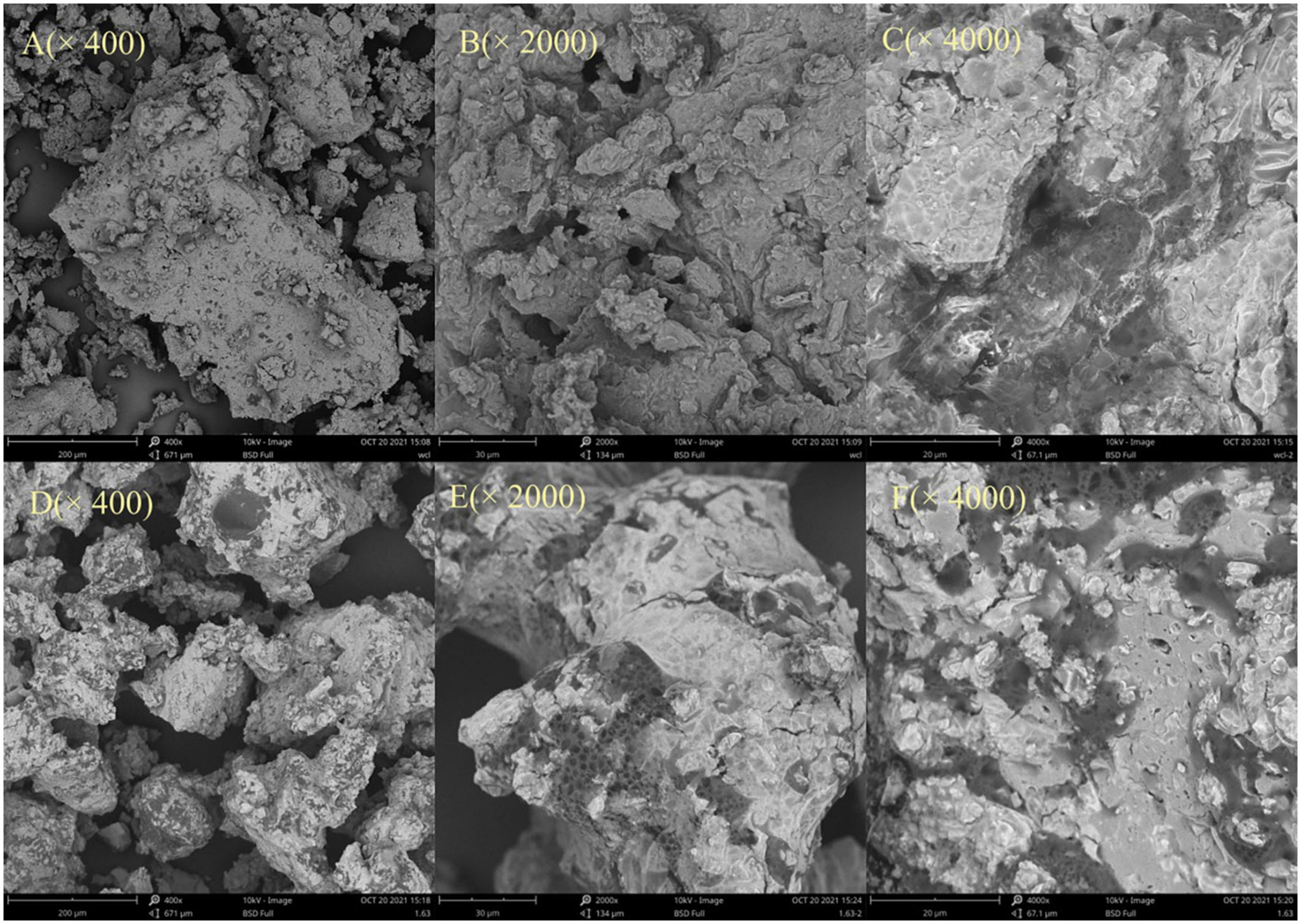
Figure 3. Scanning electronic micrographs of soluble dietary fiber (SDF) from untreated adlay bran (A–C) and SDF from steam explosion (SE)-treated adlay bran (D–F). Magnifications are 400×, 2,000×, and 4,000×.
Porosity, swelling and regional chemistry can explain some of the physiological effects of DF (21). The SEM results indicate that the dense structure of adlay bran is destroyed by SE treatment, and the size of SDF becomes smaller, in combination with the appearance of honeycomb-like porous characteristics, these changes were favorable to its functional performance. Therefore, after SE treatment, adlay bran SDF exhibited higher OHC, CAC, and GAC. Through SEM, Zhao et al. (34) found that more curls and holes appeared in sorghum after SE and making it more conducive to food fermentation. SE alters the spatial structure of okara, resulting in a significant increase in their water solubility (24). The above results show that SDF from adlay bran treated by SE can exhibit superior physicochemical and functional properties.
3.7. FT-IR analysis
Fourier transformed infrared spectra of SDF were presented in Figure 4A. The broad absorption peak at 3,200–3,600 cm–1 was caused by O-H stretch vibrations in cellulose and semi-fiber (50). The absorption peak of SDF from SE-treated adlay bran at 3,200–3,600 cm–1 was broadened, indicating a stronger intermolecular hydrogen bonding force (51). The peak intensity at 3,200–3,600 cm–1 of the SDF after SE was higher than that of the untreated SDF, suggesting that more galacturonic acid may be present in the SDF after SE (52). The absorption peak near 1,640 cm–1 contained carbonyl C = O (21). The small peak at 1,200–1,400 cm–1 was a variable angle vibration of C-H. Moreover, the absorption peak at 1,040 cm–1 was attributed to C-O bond stretching in hemicellulose and lignin, suggesting the presence of sugar-aldehyde groups in SDF from adlay bran (53). The absorption peak near 871 cm–1 indicated a β-glycosidic bond stretching vibration of the polysaccharide molecule. The overall absorption peaks of adlay bran SDF before and after SE had similar infrared spectra; the peak shapes did not change significantly, but the positions and absorption intensities of some absorption peaks were changed.
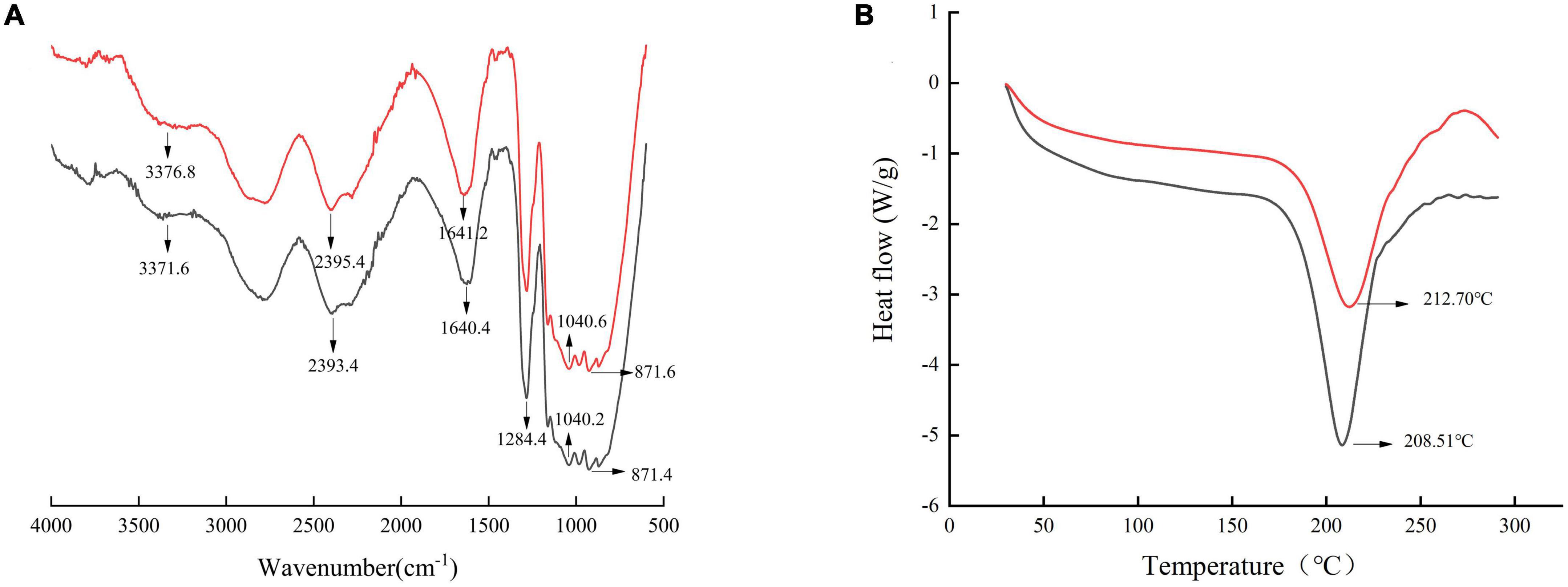
Figure 4. Fourier transform infrared spectroscopy (FT-IR) (A) and differential scanning calorimetry (DSC) thermogram (B) of soluble dietary fiber (SDF) from adlay bran. [Black line: untreated, red line: treated by steam explosion (SE) at 0.8 MPa, 3 min].
3.8. Molecular weight distribution
The molecular weight distribution of SDF from untreated adlay bran and SE-treated (0.8 MPa, 3 min) adlay bran were shown in Table 4. The weight-average molecular weight of the SDF from adlay bran after SE was significantly lower than that of the untreated sample. In addition, the polydispersity value of SE-SDF was lower than that of 0-SDF. These results indicated that the molecular weight of SDF from adlay bran after SE was reduced and that the molecular weight distribution was relatively concentrated and uniformly distributed. The decrease in molecular weight may be because SE cause the degradation of macromolecular compounds to micromolecular compounds (43). In addition, SE treatment may break the chemical and hydrogen bonds in macromolecules such as cellulose and break the macromolecular crystal lattice at the molecular level (19). Lower Molecular Weight of SDF usually has better functionality and bioactivity (54). The lower molecular weight indicates better solubility and lower viscosity of SDF (55).
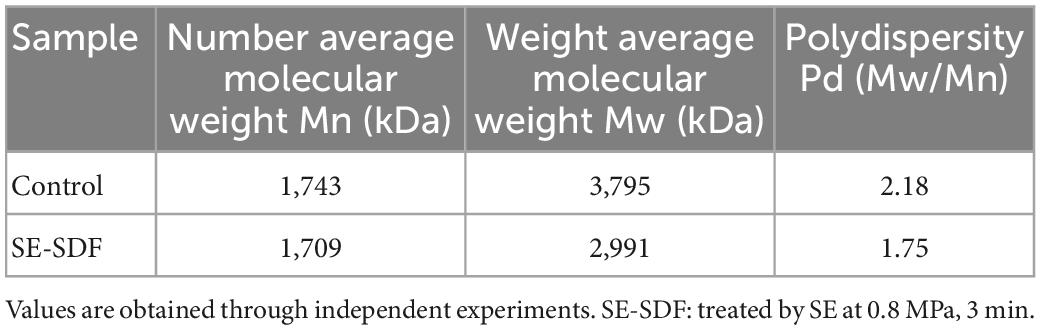
Table 4. Molecular weight of soluble dietary fiber (SDF) from untreated and steam explosion (SE)-treated adlay bran.
3.9. Monosaccharide composition
The monosaccharide composition of SDF from untreated adlay bran and SE-treated (0.8 MPa, 3 min) adlay bran were shown in Table 5. All the SDF samples were composed of seven monosaccharides, including mannose, rhamnose, glucose, galactose, xylose, arabinose, and fucose, and glucose was the major monosaccharide. This indicated that soluble polysaccharides and pectin were the main components of SDF. The monosaccharide species in the SDF remained unchanged after SE treatment, but differed in content. The content of fucose in SDF decreased after SE treatment, which was consistent with that reported by Zhao et al. (43). However, the mannose, glucose, galactose, xylose, and arabinose contents in SDF increased by 29.76, 15.96, 38.65, 173.33, and 86.32% after SE treatment. SE treatment degraded the cellulose, hemicellulose and lignin in adlay bran to small molecular weight oligosaccharides, thereby increasing the mannose, glucose, galactose, xylose, and arabinose content of the modified SDF (56, 57). However, some low molecular weight sugars may be further degraded to smaller molecular products, which cannot be sedimented by alcohol, resulting in a decline in their monosaccharide content. In conclusion, SE treatment changed the content of monosaccharide in SDF which may have resulted in better functional activity of SDF.
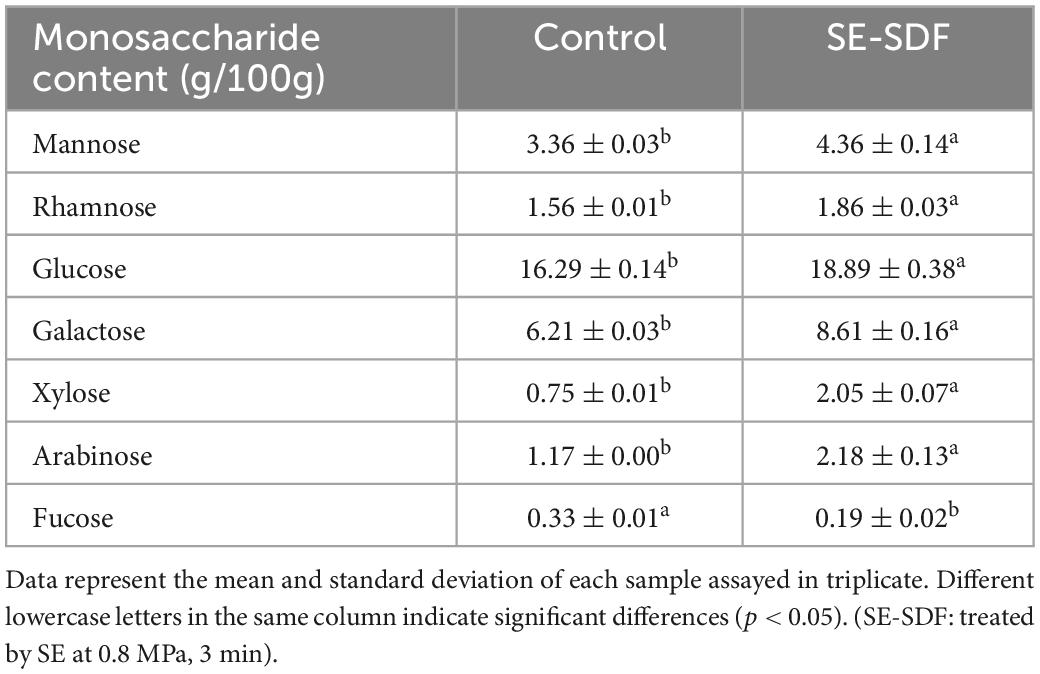
Table 5. Monosaccharide composition of soluble dietary fiber (SDF) from untreated and steam explosion (SE)-treated adlay bran.
3.10. Thermal stability
Differential scanning calorimetry was a thermal analysis method, which can obtain the heat capacity at high temperature in a short time. DSC curves for SDF are showed in Figure 4B. All samples showed typical endothermic peaks, and the peak temperature increased from 208.51 ± 0.15 to 212.70 ± 0.25°C after SE treatment. There was a significant (p < 0.05) upshift in the peak temperature of the treated sample, which could be attributed to the increase in short-chain SDF in comparison with the control, because the short-chain SDF has strong hydrogen bonds within its bonding site that require more energy for structural disruption (58). This conclusion is in strong agreement with previous results (21). SE-SDF from adlay bran has better thermal stability. Ideal thermal properties are crucial to maintain the stability of key nutrients and volatiles in samples (59). Similarly, Zhai et al. and Wang et al. concluded that the thermal stability of SDF from R. roxburghii pomace and orange peel by SE treatment were higher than that of SDF from untreated (29–41). Karakoti et al. also demonstrated that microfiber isolated from Hibiscus sabdariffa var. altissima fiber by SE have better thermal stability (60).
4. Conclusion
Adlay bran is rich in DF but low in SDF, which seriously affects its nutritional value and physiological functions. Appropriate SE treatment can increase significantly the extraction rate of SDF from adlay bran. When steam strength of 0.8 MPa was applied for 3 min, SDF content increased from 7.35 to 9.37%. When the SE intensity was too high, SDF contents decreased. Compared with SDF from untreated adlay bran, the SDF from SE-treated adlay bran had improved OHC and CAC and showed better hypoglycaemic capacity. The average molecular weight of SDF decreased after SE treatment. In addition, the structure of SDF from SE-treated adlay bran was disrupted and exhibited porous, honeycomb-like features, which could contribute to changes in bioactivity and functional properties. This study shows that SE can enhance the SDF content of adlay bran and effectively improve its physicochemical properties and in vitro hypoglycemic activity. At the same time, this study provides new ideas for utilizing SDF from adlay bran and developing new food products.
Data availability statement
The original contributions presented in this study are included in this article/supplementary material, further inquiries can be directed to the corresponding author.
Author contributions
XT: data curation, methodology, formal analysis, writing – original draft, and writing – review and editing. ZW: investigation, data curation, and software. JZ: investigation and data curation. JK: funding acquisition and supervision. GC: funding acquisition and project administration. MD: funding acquisition, supervision, project administration, writing – review and editing, and validation. All authors contributed to the article and approved the submitted version.
Funding
This work was supported by the Chongqing Natural Science Foundation (grant number cstc2020jcyj-msxmX0392).
Acknowledgments
We thank the participants and professionals involved in this study.
Conflict of interest
The authors declare that the research was conducted in the absence of any commercial or financial relationships that could be construed as a potential conflict of interest.
Publisher’s note
All claims expressed in this article are solely those of the authors and do not necessarily represent those of their affiliated organizations, or those of the publisher, the editors and the reviewers. Any product that may be evaluated in this article, or claim that may be made by its manufacturer, is not guaranteed or endorsed by the publisher.
References
1. Chen H, Chung C, Chiang W, Lin Y. Anti-inflammatory effects and chemical study of a flavonoid-enriched fraction from adlay bran. Food Chem. (2011) 126:1741–8. doi: 10.1016/j.foodchem.2010.12.074
2. Lee M, Lin H, Cheng F, Chiang W, Kuo Y. Isolation and characterization of new lactam compounds that inhibit lung and colon cancer cells from adlay (Coix lachryma-jobi L. Var. ma-yuen stapf) bran. Food Chem Toxicol. (2008) 46:1933–9. doi: 10.1016/j.fct.2008.01.033
3. Chen H, Shih C, Hsu H, Chiang W. Mast cell-dependent allergic responses are inhibited by ethanolic extract of adlay (coix lachryma-jobi l. Var.ma-yuen stapf) testa. J Agr Food Chem. (2010) 58:2596–601. doi: 10.1021/jf904356q
4. Yang Q, Zhao M, Lin L. Adsorption and desorption characteristics of adlay bran free phenolics on macroporous resins. Food Chem. (2016) 194:900–7. doi: 10.1016/j.foodchem.2015.08.070
5. Lin L, Yang Q, Zhao K, Zhao M. Identification of the free phenolic profile of adlay bran by uplc-qtof-ms/ms and inhibitory mechanisms of phenolic acids against xanthine oxidase. Food Chem. (2018) 253:108–18. doi: 10.1016/j.foodchem.2018.01.139
6. Tosh S, Bordenave N. Emerging science on benefits of whole grain oat and barley and their soluble dietary fibers for heart health, glycemic response, and gut microbiota. Nutr Rev. (2020) 78:13–20. doi: 10.1093/nutrit/nuz085
7. Barber T, Kabisch S, Pfeiffer A, Weickert M. The health benefits of dietary fibre. Nutrients. (2020) 12:3209. doi: 10.3390/nu12103209
8. Elleuch M, Bedigian D, Roiseux O, Besbes S, Blecker C, Attia H. Dietary fibre and fibre-rich by-products of food processing: characterisation, technological functionality and commercial applications: a review. Food Chem. (2011) 124:411–21. doi: 10.1016/j.foodchem.2010.06.077
9. Weickert M, Pfeiffer A. Impact of dietary fiber consumption on insulin resistance and the prevention of type 2 diabetes. J. Nutr. (2018) 148:7–12. doi: 10.1093/jn/nxx008
10. Gao A, Yan X, Xu X, Ye R, Chen Y. Physicochemical and bioactive properties of soluble dietary fibers from blasting extrusion processing (bep)-extruded carrot residues. Food Bioprocess Tech. (2015) 8:2036–46. doi: 10.1007/s11947-015-1557-1
11. Qiao H, Shao H, Zheng X, Liu J, Liu J, Huang J, et al. Modification of sweet potato (ipomoea batatas lam.) residues soluble dietary fiber following twin-screw extrusion. Food Chem. (2021) 335:127522. doi: 10.1016/j.foodchem.2020.127522
12. Hu Y, Wang Z, Xu S. Corn bran dietary fibre modified by xylanase improves the mrna expression of genes involved in lipids metabolism in rats. Food Chem. (2008) 109:499–505. doi: 10.1016/j.foodchem.2007.12.045
13. Xie Y, Gou L, Peng M, Zheng J, Chen L. Effects of soluble fiber supplementation on glycemic control in adults with type 2 diabetes mellitus: a systematic review and meta-analysis of randomized controlled trials. Clin Nutr. (2021) 40:1800–10. doi: 10.1016/j.clnu.2020.10.032
14. Ajila C, Leelavathi K, Prasada Rao U. Improvement of dietary fiber content and antioxidant properties in soft dough biscuits with the incorporation of mango peel powder. J Cereal Sci. (2008) 48:319–26. doi: 10.1016/j.jcs.2007.10.001
15. Zhang N, Huang C, Ou S. In vitro binding capacities of three dietary fibers and their mixture for four toxic elements, cholesterol, and bile acid. J Hazard Mater. (2011) 186:236–9. doi: 10.1016/j.jhazmat.2010.10.120
16. Yan J, Wu L, Cai W, Xiao G, Duan Y, Zhang H. Subcritical water extraction-based methods affect the physicochemical and functional properties of soluble dietary fibers from wheat bran. Food Chem. (2019) 298:124987. doi: 10.1016/j.foodchem.2019.124987
17. Nyman E, Svanberg S. Modification of physicochemical properties of dietary fibre in carrots by mono- and divalent cations. Food Chem. (2002) 76:273–80. doi: 10.1016/S0308-8146(01)00271-0
18. Lin D, Long X, Huang Y, Yang Y, Wu Z, Chen H, et al. Effects of microbial fermentation and microwave treatment on the composition, structural characteristics, and functional properties of modified okara dietary fiber. LWT. (2020) 123:109059. doi: 10.1016/j.lwt.2020.109059
19. Singh J, Suhag M, Dhaka A. Augmented digestion of lignocellulose by steam explosion, acid and alkaline pretreatment methods: a review. Carbohyd Polym. (2015) 117:624–31. doi: 10.1016/j.carbpol.2014.10.012
20. Zhang S, Pan Y, Zheng L, Yang Y, Zheng X, Ai B, et al. Application of steam explosion in oil extraction of camellia seed (Camellia oleifera abel.) and evaluation of its physicochemical properties, fatty acid, and antioxidant activities. Food Sci Nutr. (2019) 7:1004–16. doi: 10.1002/fsn3.924
21. Wang L, Xu H, Yuan F, Fan R, Gao Y. Preparation and physicochemical properties of soluble dietary fiber from orange peel assisted by steam explosion and dilute acid soaking. Food Chem. (2015) 185:90–8. doi: 10.1016/j.foodchem.2015.03.112
22. Wang T, Liang X, Ran J, Sun J, Jiao Z, Mo H. Response surface methodology for optimisation of soluble dietary fibre extraction from sweet potato residue modified by steam explosion. Int J Food Sci Technol. (2017) 52:741–7. doi: 10.1111/ijfs.13329
23. Sui W, Xie X, Liu R, Wu T, Zhang M. Effect of wheat bran modification by steam explosion on structural characteristics and rheological properties of wheat flour dough. Food Hydrocolloid. (2018) 84:571–80. doi: 10.1016/j.foodhyd.2018.06.027
24. Li B, Yang W, Nie Y, Kang F, Goff H, Cui S. Effect of steam explosion on dietary fiber, polysaccharide, protein and physicochemical properties of okara. Food Hydrocolloid. (2019) 94:48–56. doi: 10.1016/j.foodhyd.2019.02.042
25. Chen H, Xiong M, Bai T, Chen D, Zhang Q, Lin D, et al. Comparative study on the structure, physicochemical, and functional properties of dietary fiber extracts from quinoa and wheat. LWT. (2021) 149:111816. doi: 10.1016/j.lwt.2021.111816
26. Ma M, Mu T. Effects of extraction methods and particle size distribution on the structural, physicochemical, and functional properties of dietary fiber from deoiled cumin. Food Chem. (2016) 194:237–46. doi: 10.1016/j.foodchem.2015.07.095
27. Chau C, Wang Y, Wen Y. Different micronization methods significantly improve the functionality of carrot insoluble fibre. Food Chem. (2007) 100:1402–8. doi: 10.1016/j.foodchem.2005.11.034
28. Tang C, Wu L, Zhang F, Kan J, Zheng J. Comparison of different extraction methods on the physicochemical, structural properties, and in vitro hypoglycemic activity of bamboo shoot dietary fibers. Food Chem. (2022) 386:132642. doi: 10.1016/j.foodchem.2022.132642
29. Lan G, Chen H, Chen S, Tian J. Chemical composition and physicochemical properties of dietary fiber from polygonatum odoratum as affected by different processing methods. Food Res Int. (2012) 49:406–10. doi: 10.1016/j.foodres.2012.07.047
30. Shen M, Ge Y, Kang Z, Quan Z, Wang J, Xiao J, et al. Yield and physicochemical properties of soluble dietary fiber extracted from untreated and steam explosion-treated black soybean hull. J Chem. (2019) 2019:9736479. doi: 10.1155/2019/9736479
31. Jiang W, Han G, Zhou C, Gao S, Zhang Y, Li M, et al. The degradation of lignin, cellulose, and hemicellulose in kenaf bast under different pressures using steam explosion treatment. J Wood Chem Technol. (2017) 37:359–68. doi: 10.1080/02773813.2017.1303514
32. Tanpichai S, Witayakran S, Boonmahitthisud A. Study on structural and thermal properties of cellulose microfibers isolated from pineapple leaves using steam explosion. J Environ Chem Eng. (2019) 7:102836. doi: 10.1016/j.jece.2018.102836
33. Nie D, Yao L, Xu X, Zhang Z, Li Y. Promoting corn stover degradation via sequential processing of steam explosion and cellulase/lactic acid bacteria-assisted ensilage. Bioresource Technol. (2021) 337:125392. doi: 10.1016/j.biortech.2021.125392
34. Zhao G, Kuang G, Wang Y, Yao Y, Zhang J, Pan Z. Effect of steam explosion on physicochemical properties and fermentation characteristics of sorghum (sorghum bicolor (L.) Moench). LWT. (2020) 129:109579. doi: 10.1016/j.lwt.2020.109579
35. Zhu L, Yu B, Chen H, Yu J, Yan H, Luo Y, et al. Comparisons of the micronization, steam explosion, and gamma irradiation treatment on chemical composition, structure, physicochemical properties, and in vitro digestibility of dietary fiber from soybean hulls. Food Chem. (2022) 366:130618. doi: 10.1016/j.foodchem.2021.130618
36. Qiao C, Zeng F, Wu N, Tan B. Functional, physicochemical and structural properties of soluble dietary fiber from rice bran with extrusion cooking treatment. Food Hydrocolloid. (2021) 121:107057. doi: 10.1016/j.foodhyd.2021.107057
37. Kong F, Wang L, Gao H, Chen H. Process of steam explosion assisted superfine grinding on particle size, chemical composition and physico-chemical properties of wheat bran powder. Powder Technol. (2020) 371:154–60. doi: 10.1016/j.powtec.2020.05.067
38. Chu J, Zhao H, Lu Z, Lu F, Bie X, Zhang C. Improved physicochemical and functional properties of dietary fiber from millet bran fermented by Bacillus natto. Food Chem. (2019) 294:79–86. doi: 10.1016/j.foodchem.2019.05.035
39. Huang Y, Ma Y, Tsai Y, Chang S. In vitro hypoglycemic, cholesterol-lowering and fermentation capacities of fiber-rich orange pomace as affected by extrusion. Int J Biol Macromol. (2019) 124:796–801. doi: 10.1016/j.ijbiomac.2018.11.249
40. Zheng Y, Wang X, Tian H, Li Y, Shi P, Guo W, et al. Effect of four modification methods on adsorption capacities and in vitro hypoglycemic properties of millet bran dietary fibre. Food Res Int. (2021) 147:110565. doi: 10.1016/j.foodres.2021.110565
41. Zhai X, Ao H, Liu W, Zheng J, Li X, Ren D. Physicochemical and structural properties of dietary fiber from rosa roxburghii pomace by steam explosion. J Food Sci Technol. (2022) 59:2381–91. doi: 10.1007/s13197-021-05254-7
42. Liu Y, Ao H, Zheng J, Liang Y, Ren D. Improved functional properties of dietary fiber Fromrosa roxburghii Tratt residue by steam explosion. J Food Process Pres. (2022) 46:e16119. doi: 10.1111/jfpp.16119
43. Zhao Y, Yu K, Tian X, Sui W, Wu T, Wang S, et al. Combined modification of soluble dietary fibers from apple pomace by steam explosion and enzymatic hydrolysis to improve its structural, physicochemical and functional properties. Waste Biomass Valori. (2022) 13:4869–79. doi: 10.1007/s12649-022-01823-9
44. Nsor-Atindana J, Zhong F, Mothibe K. In vitro hypoglycemic and cholesterol lowering effects of dietary fiber prepared from cocoa (Theobroma cacao L.) Shells. Food Funct. (2012) 3:1044–50. doi: 10.1039/c2fo30091e
45. Zheng Y, Xu B, Shi P, Tian H, Li Y, Wang X, et al. The influences of acetylation, hydroxypropylation, enzymatic hydrolysis and crosslinking on improved adsorption capacities and in vitro hypoglycemic properties of millet bran dietary fibre. Food Chem. (2022) 368:130883. doi: 10.1016/j.foodchem.2021.130883
46. Zhang M, Bai X, Zhang Z. Extrusion process improves the functionality of soluble dietary fiber in oat bran. J Cereal Sci. (2011) 54:98–103. doi: 10.1016/j.jcs.2011.04.001
47. Dubey R, Toh Y, Yeh A. Enhancing cellulose functionalities by size reduction using media-mill. Sci Rep. (2018) 8:11343. doi: 10.1038/s41598-018-29777-w
48. Alvira P, Tomás-Pejó E, Ballesteros M, Negro M. Pretreatment technologies for an efficient bioethanol production process based on enzymatic hydrolysis: a review. Bioresource Technol. (2010) 101:4851–61. doi: 10.1016/j.biortech.2009.11.093
49. Gan J, Huang Z, Yu Q, Peng G, Chen Y, Xie J, et al. Microwave assisted extraction with three modifications on structural and functional properties of soluble dietary fibers from grapefruit peel. Food Hydrocolloid. (2020) 101:105549. doi: 10.1016/j.foodhyd.2019.105549
50. Mahloko L, Silungwe H, Mashau M, Kgatla T. Bioactive compounds, antioxidant activity and physical characteristics of wheat-prickly pear and banana biscuits. Heliyon. (2019) 5:e2479. doi: 10.1016/j.heliyon.2019.e02479
51. Chen H, Zhao C, Li J, Hussain S, Yan S, Wang Q. Effects of extrusion on structural and physicochemical properties of soluble dietary fiber from nodes of lotus root. LWT. (2018) 93:204–11. doi: 10.1016/j.lwt.2018.03.004
52. Zhang W, Zeng G, Pan Y, Chen W, Huang W, Chen H, et al. Properties of soluble dietary fiber-polysaccharide from papaya peel obtained through alkaline or ultrasound-assisted alkaline extraction. Carbohyd Polym. (2017) 172:102–12. doi: 10.1016/j.carbpol.2017.05.030
53. Chen H, Fu X, Luo Z. Properties and extraction of pectin-enriched materials from sugar beet pulp by ultrasonic-assisted treatment combined with subcritical water. Food Chem. (2015) 168:302–10. doi: 10.1016/j.foodchem.2014.07.078
54. Shen M, Weihao W, Cao L. Soluble dietary fibers from black soybean hulls: physical and enzymatic modification, structure, physical properties, and cholesterol binding capacity. J Food Sci. (2020) 85:1668–74. doi: 10.1111/1750-3841.15133
55. Liu Y, Fan C, Tian M, Yang Z, Liu F, Pan S. Effect of drying methods on physicochemical properties and in vitro hypoglycemic effects of orange peel dietary fiber. J Food Process Pres. (2017) 41:e13292. doi: 10.1111/jfpp.13292
56. Long D, Ye F, Zhao G. Optimization and characterization of wheat bran modified by in situ enhanced co2 blasting extrusion. LWT Food Sci Technol. (2014) 59:605–11. doi: 10.1016/j.lwt.2014.07.017
57. Yi J, Li X, Wang S, Wu T, Liu P. Steam explosion pretreatment of achyranthis bidentatae radix: modified polysaccharide and its antioxidant activities. Food Chem. (2022) 375:131746. doi: 10.1016/j.foodchem.2021.131746
58. Chen Y, Ye R, Yin L, Zhang N. Novel blasting extrusion processing improved the physicochemical properties of soluble dietary fiber from soybean residue and in vivo evaluation. J Food Eng. (2014) 120:1–8. doi: 10.1016/j.jfoodeng.2013.07.011
59. Xu W, Cui W, Li W, Guo W. Development and characterizations of super-fine wool powder. Powder Technol. (2004) 140:136–40. doi: 10.1016/j.powtec.2003.12.010
Keywords: adlay bran, soluble dietary fiber, steam explosion, physicochemical properties, structural characteristics, in vitro hypoglycemic activity
Citation: Tang X, Wang Z, Zheng J, Kan J, Chen G and Du M (2023) Physicochemical, structure properties and in vitro hypoglycemic activity of soluble dietary fiber from adlay (Coix lachryma-jobi L. var. ma-yuen Stapf) bran treated by steam explosion. Front. Nutr. 10:1124012. doi: 10.3389/fnut.2023.1124012
Received: 14 December 2022; Accepted: 16 January 2023;
Published: 03 February 2023.
Edited by:
Yongsheng Chen, Jinan University, ChinaReviewed by:
Qiang Yu, Nanchang University, ChinaWenjie Sui, Tianjin University of Science and Technology, China
Wenchao Chen, Oil Crops Research Institute (CAAS), China
Aimin Shi, Institute of Food Science and Technology (CAAS), China
Qian Li, Tianjin Agricultural University, China
Copyright © 2023 Tang, Wang, Zheng, Kan, Chen and Du. This is an open-access article distributed under the terms of the Creative Commons Attribution License (CC BY). The use, distribution or reproduction in other forums is permitted, provided the original author(s) and the copyright owner(s) are credited and that the original publication in this journal is cited, in accordance with accepted academic practice. No use, distribution or reproduction is permitted which does not comply with these terms.
*Correspondence: Muying Du, muyingdu_swu@163.com