- 1The Wiener Cardiovascular Institute/Marie-Josée and Henry R. Kravis Center for Cardiovascular Health at Mount Sinai Heart, New York, NY, United States
- 2Icahn School of Medicine at Mount Sinai, New York, NY, United States
- 3Endocrinology Associates, Inc., Columbus, OH, United States
- 4Medical Education and Research Alliance (Med-ERA, Inc.), New York, NY, United States
- 5Rutgers New Jersey Medical School, Newark, NJ, United States
- 6Novo Nordisk Inc., Plainsboro Township, NJ, United States
- 7Internal Medicine and Medical Nutrition, San Diego, CA, United States
- 8Abbott Nutrition, Columbus, OH, United States
- 9Joslin Diabetes Center, Boston, MA, United States
- 10Harvard Medical School, Boston, MA, United States
The coronavirus disease 2019 (COVID-19) pandemic challenges our collective understanding of transmission, prevention, complications, and clinical management of severe acute respiratory syndrome coronavirus 2 (SARS-CoV-2) infection. Risk factors for severe infection, morbidity, and mortality are associated with age, environment, socioeconomic status, comorbidities, and interventional timing. Clinical investigations report an intriguing association of COVID-19 with diabetes mellitus and malnutrition but incompletely describe the triphasic relationship, its mechanistic pathways, and potential therapeutic approaches to address each malady and their underlying metabolic disorders. This narrative review highlights common chronic disease states that interact epidemiologically and mechanistically with the COVID-19 to create a syndromic phenotype—the COVID-Related Cardiometabolic Syndrome—linking cardiometabolic-based chronic disease drivers with pre-, acute, and chronic/post-COVID-19 disease stages. Since the association of nutritional disorders with COVID-19 and cardiometabolic risk factors is well established, a syndromic triad of COVID-19, type 2 diabetes, and malnutrition is hypothesized that can direct, inform, and optimize care. In this review, each of the three edges of this network is uniquely summarized, nutritional therapies discussed, and a structure for early preventive care proposed. Concerted efforts to identify malnutrition in patients with COVID-19 and elevated metabolic risks are needed and can be followed by improved dietary management while simultaneously addressing dysglycemia-based chronic disease and malnutrition-based chronic disease.
Introduction
The coronavirus disease 2019 (COVID-19) pandemic spread rapidly worldwide in less than a year and activated an unprecedented acceleration of medical research, revealing a new understanding of the relationships among viral infections and chronic metabolic diseases. The juxtaposition of threat and swift knowledge acquisition observed during the COVID-19 pandemic contrasts starkly with the slower rise in prevalence of chronic cardiometabolic diseases and the growing clinical knowledge of residual health risks determined over many decades (1). Of note, cardiometabolic drivers, risk factors, and resulting chronic metabolic states interact with COVID-19 to create a syndromic phenotype of hazards for disease severity, morbidity, and mortality, as well as long-term insults to quality of life, symptom burden, and socioeconomic impact. In a recent narrative review, the COVID-Related Cardiometabolic Syndrome (CIRCS) (2) was introduced based on consistent and compelling evidence linking pre-, acute, and chronic/post-COVID-19 disease stages with cardiometabolic-based chronic disease (CMBCD) (3, 4).
The CMBCD framework is a novel vehicle to expose opportunities for early and sustainable prevention and is comprised of three dimensions: (1) staged progression over time (1- “risk,” 2- “predisease,” 3- “disease,” and 4- “complications”); (2) multiple interacting primary (genetics, environment, and behavior/lifestyle) and secondary/metabolic (abnormal adiposity, dysglycemia, hypertension, dyslipidemia, and nutrition) drivers; and (3) social determinants of health and transcultural factors (SDOH/TF) (Figure 1) (3–5). Many of the conventional terms commonly used to describe cardiometabolic risk factors are now subsumed in driver-based chronic disease models. For instance, in adiposity-based chronic disease (ABCD), overweight is stage 2, obesity is stage 3, and obesity-related complications is stage 4 (3). In dysglycemia-based chronic disease (DBCD), insulin resistance is stage 1, prediabetes is stage 2, type 2 diabetes (T2D) is stage 3, and diabetes complications is stage 4 (3, 6). In malnutrition-based chronic disease (MBCD), which is under development, malnutrition is stage 3, and malnutrition complications is 4. The purpose of incorporating the CMBCD model into this discussion is to provide a template for understanding a specific interaction between CIRCS and nutritional status.
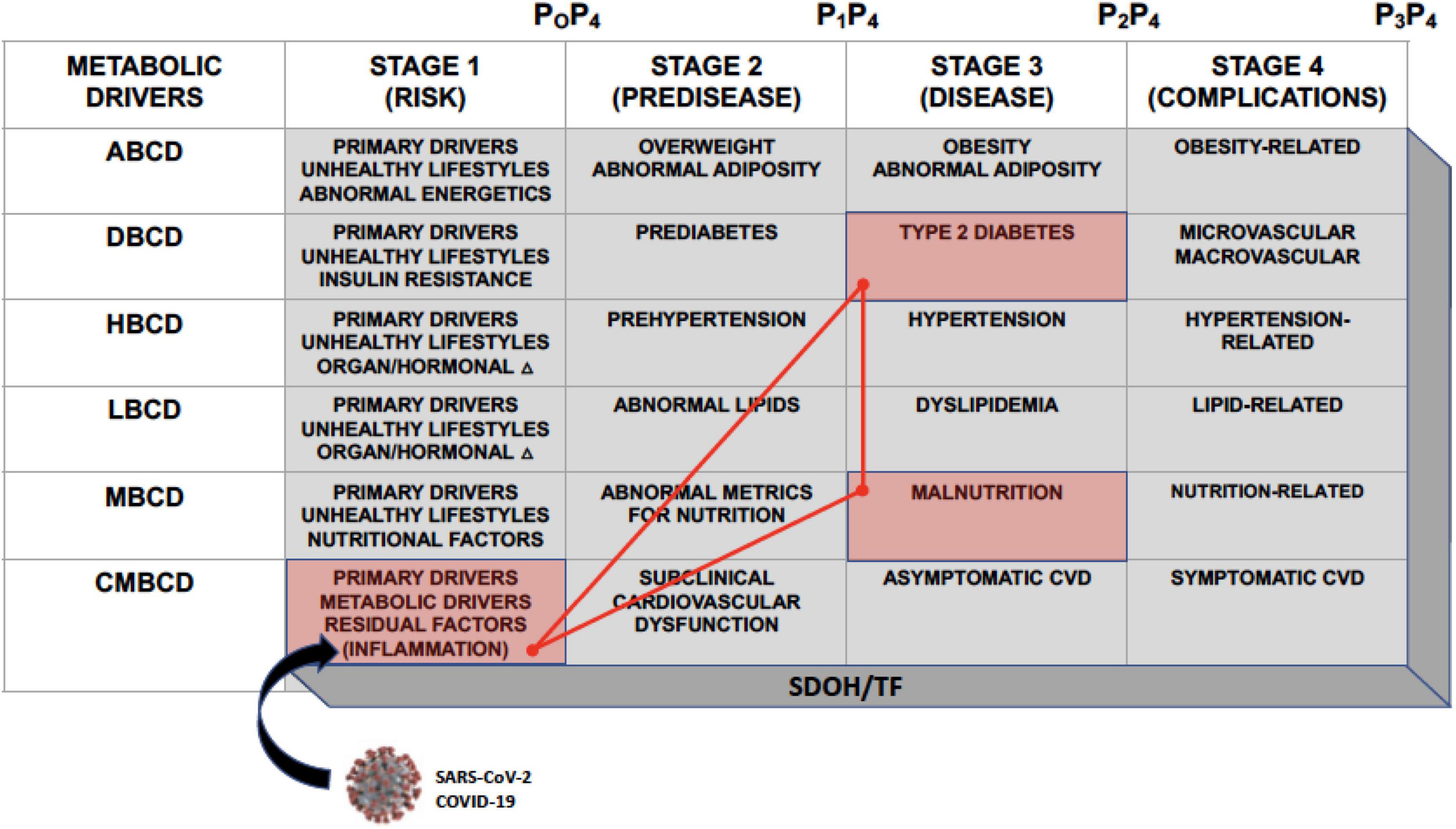
Figure 1. The CMBCD template. The CMBCD model comprises 3 dimensions: (1) Staged progression over time along the top row; (2) interactions among metabolic drivers culminating in CVD in the far-left column; and (3) adapting each cell in the stage x driver matrix to SDOH/TF. Prevention modalities are indicated as P0–P4. Primary drivers are genetics, environment, and behavior/lifestyle. The SARS-CoV-2 virus responsible for COVID-19 intersects with CMBCD at the level of inflammation in stage 1. This CMBCD template provides context for the novel syndromic triad of COVID-19, T2D, and malnutrition, which is depicted by the red cells and bright red triangle network. ABCD, adiposity-based chronic disease; CMBCD, cardiometabolic-based chronic disease; COVID-19, coronavirus disease 2019; CVD, cardiovascular disease; DBCD, dysglycemia-based chronic disease; HBCD, hypertension-based chronic disease; LBCD, lipid-based chronic disease; MBCD, malnutrition-based chronic disease; P0, primordial prevention; P1, primary prevention; P2, secondary prevention; P3, tertiary prevention; P4, quaternary prevention; SDOH, social determinants of health; TF, transcultural factors; SARS-CoV-2, severe acute respiratory syndrome coronavirus 2. Adapted from (4).
In a recent scoping review, various nutritional disorders are linked with pre-, acute, and chronic/post COVID-19 stages (7). A distillation of the complex interactions of CIRCS and nutrition prompts a hypothesized syndromic triad with COVID-19, T2D, and malnutrition as key inter-related disease states (Figure 2). The purpose of constructing this new triad model is to expose early opportunities for better lifestyle, glycemic, and nutritional management of patients with COVID-19. The present narrative review will summarize key epidemiological and mechanistic aspects of these networked relationships, discuss relevant nutritional therapies, and propose testable hypotheses and structure for early preventive care.
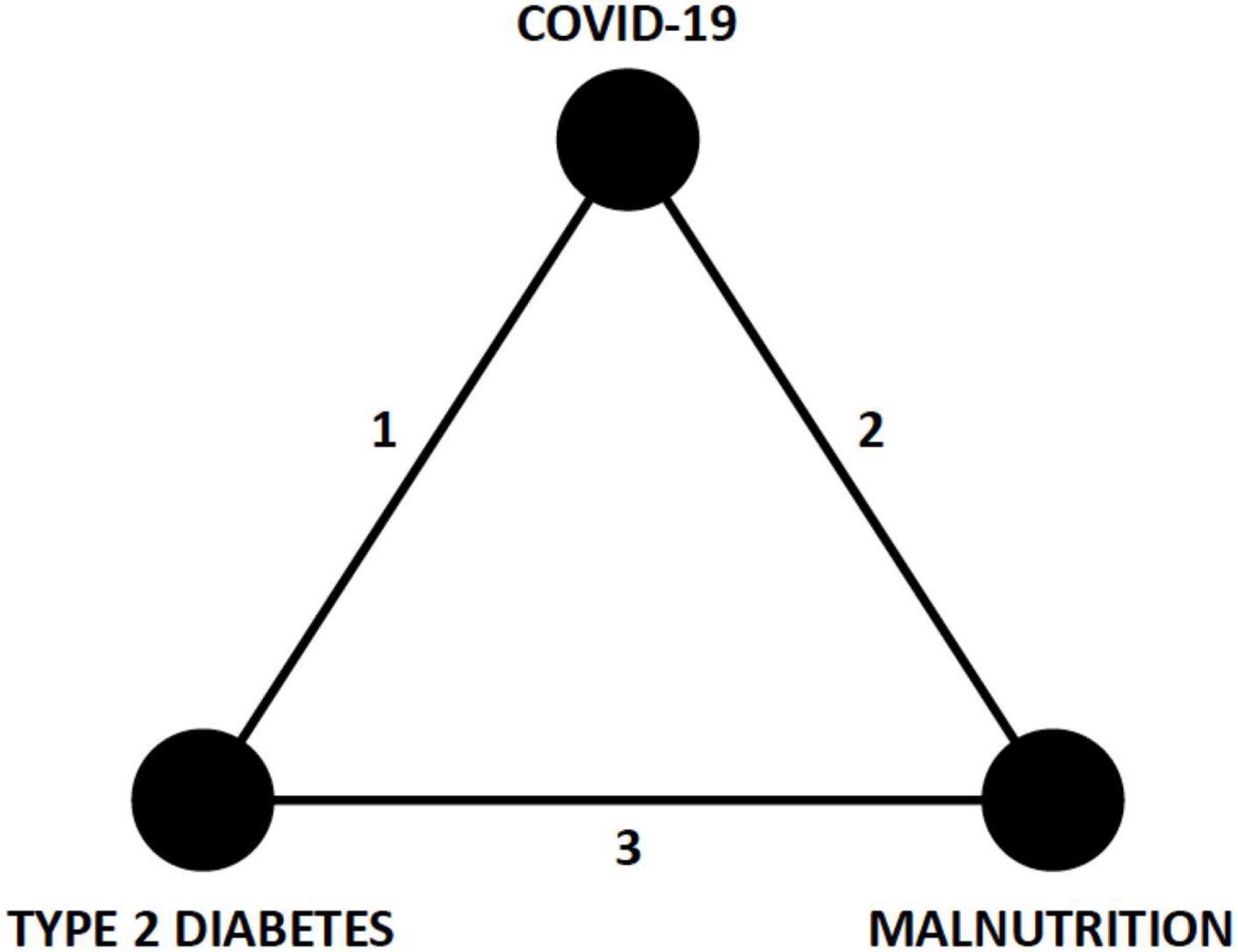
Figure 2. Syndromic triad of COVID-19, T2D, and malnutrition. The three edges (1, 2, and 3) of this triangle network represent epidemiological associations and pathophysiological mechanisms that connect each of the nodes (COVID-19, T2D, and malnutrition) and are discussed in the text. Recognition of this triad should prompt earlier consideration of nutritional and glycemic interventions in patients with COVID-19. COVID-19, coronavirus disease 2019; T2D, type 2 diabetes.
Methodology
To guide this initiative, a virtual meeting of coauthors was held in December 2021 to establish investigative questions, objectives, and methods to support the syndromic triad concept and to plan reporting if findings were thought worthy of publication. Study populations of interest include adults who contracted COVID-19 infections that were complicated by either pre-existing or newly developed T2D and malnutrition to determine if this triad of illnesses exists and has a noticeable impact on clinical outcomes. World literature was searched for relevant articles involving the stated population using several tradition engines (PubMed, Google, Cochrane, Embase, and Science Direct) without language or geographic restrictions. The following terms, alone and in combinations, directed the searches: COVID-19, coronavirus; type 2 diabetes, malnutrition, epidemiology, mechanisms, adiposity-based chronic disease, cardiometabolic-based chronic disease, dysglycemia-base chronic disease, hypertension-based chronic disease, lipid-based chronic disease, metabolic based chronic disease, therapy, treatment, nutrition, and outcomes. Meaningful publications (181 references) among the hundreds that were identified in multiple literature searches report data regarding epidemiologies and disease mechanisms that link triad components as well as clinical information related to outcomes for studied populations. Retrieved information was assessed to confirm or deny the existence of the triad and to propose clinical care to address each component and its drivers, which was the aim of the initiative.
Edge 1: COVID-19 and type 2 diabetes
Epidemiology
Adults with COVID-19 are more likely to develop T2D than those with other acute upper respiratory infections (8). In an analysis of retrospective data from the US Veterans Administration (VA), there are increased risks of incident T2D and additional disease burden among patients with COVID-19 (n = 181,281) vs. both contemporary control patients (n = 4,118,911) and also historical controls (n = 4,286,911) without infection (9). In a study of hospitalized adults, the prevalence of diabetes is higher among those with a positive vs. negative COVID-19 test result 30 days after testing (10). The Centers for Disease Control and Prevention (CDC) reports an observed increased risk for T2D in patients <18 years of age who had COVID-19, compared to those without COVID-19 and those with pre-pandemic acute respiratory infection (11). Moreover, incident cases of pediatric T2D and severity of illness as reflected by the degree of diabetic ketoacidosis at presentation are greater during, compared with before, the pandemic (12); but also worth noting, some increase could have been associated with other issues such as delayed healthcare or supply shortages rather than infection. Pooled data from four observational studies show that SARS-CoV-2-infected patients compared with healthy controls carried a 59% higher risk of developing incident diabetes in the post-acute phase (13). However, in this study, a high degree of heterogeneity and a short follow-up period in the contributing studies (4 months) are limitations of the meta-analysis expressed by investigators.
In a single large retrospective cohort study of VA in- and outpatient men without preexisting diabetes, SARS-CoV-2 infection is associated with a higher risk of incident (120 days, OR 2.56 [95% CI 2.32–2.83]) and all time (237 days, OR 1.95 [1.80–2.12]) diabetes (14). In contrast, among women, who comprised 14% of the total VA study population, an association is not definitively established (120 days, 1.21 [0.88–1.68]; all time, 1.04 [0.82–1.31]; p-values were both <0.1) (14).
Many observational studies highlight compelling relationships among cardiometabolic conditions, COVID-19 infection, and severity of illness, with up to 94% of hospitalized patients presenting with at least one significant comorbidity (15–18). In a large cohort (n = 5,700) of hospitalized patients (17), diabetes, hypertension, and obesity are among the top comorbidities associated with COVID-19 infection, with similar patterns replicated globally in other analyses (19). Data also demonstrate a disproportionately high number of COVID-19 deaths in people with diabetes (20, 21). Likewise, obesity, with characteristic insulin resistance (22), also carries a higher risk for COVID-19 death (21).
Hyperglycemia is indicated by elevated fasting plasma glucose, post-challenge plasma glucose, and hemoglobin A1c (A1C) levels, and arises from a pancreatic β-cell defect following chronic exposure to insulin resistance. Hyperglycemia is associated with inflammation, coagulation disorders, low oxygenation, and higher risk of mortality in patients with COVID-19, compared to those without COVID-19 (6, 23). In the intensive care unit (ICU), poorly controlled diabetes with moderate-severe obesity greatly increases the risk of COVID-19-related mortality (24, 25).
Type 2 diabetes and obesity are two principal risk factors for the development of severe COVID-19 symptoms, and individuals with these comorbidities constitute a specific risk group (26). Of related interest, patients with type 1 diabetes (T1D), among other observed factors, require intensive care for COVID-19 twice as often as controls and are more likely to die (HR 2.90, 95% CI 1.66–5.47) of their COVID-19 infection (27).
Abnormal adiposity (i.e., elevated waist circumference and/or body mass index [BMI]), dysglycemia (i.e., insulin resistance or hyperglycemia [prediabetes or T2D]), elevated blood pressure (i.e., hypertension), dyslipidemia (i.e., hypertriglyceridemia and low concentration of high-density lipoprotein cholesterol), and residual risks (e.g., microalbuminuria and other features of insulin resistance) often cluster together as metabolic syndrome, which exhibits a higher odds for intensive care unit (ICU) requirement, invasive ventilation, ARDS (acute respiratory distress syndrome), and mortality compared to individual cardiometabolic risk factors (28, 29). Metabolic syndrome differs from CMBCD by only considering specific features for each metabolic driver at a particular timepoint, not as a staged progression over time based on pathophysiology, and not incorporating SDOH/TF. Aggregate cardiometabolic risk, individual risk factors, and vulnerability to severe COVID-19 each increase with age (30). Thus, cardiometabolic risk factors can be considered discretely as modifiable COVID-19 risk factors, which can be addressed with preventative approaches (i.e., “primordial” to prevent risk; “primary” to prevent disease; “secondary” to prevent disease progression; “tertiary” to prevent suffering and mortality in advanced disease; and “quaternary” to prevent overmedicalization at each disease stage).
Mechanisms
Infection with SARS-CoV-2 appears to alter pancreatic β-cell function and consequently reduce insulin secretion, while the accompanying hypercytokinemia promotes insulin resistance (30, 31). This combination of decreased insulin secretion and sensitivity induces and then aggravates hyperglycemia. Evidence from animal models have demonstrated markers of diminished immune function in hyperglycemic states (32). In human studies, phagocytosis function is restored in subjects with T2D following an intensive glycemic control intervention combining medication, insulin, and dietary modifications provided in a clinical trial (33). In effect, a vicious cycle is created by the bidirectional relationship wherein T2D worsens COVID-19 severity and COVID-19 worsens dysglycemia.
Relative hyperglycemia for an individual at a certain time, as opposed to absolute hyperglycemia, is defined as a blood glucose concentration at a particular timepoint divided by the estimated average glucose based on a current A1C level (34). This measure, also referred to as “stress hyperglycemia ratio” (SHR) is typically measured at hospital or ICU admission to predict clinical outcomes. The SHR controls for background glycemia in patient evaluations and is also a superior marker of critical illness compared to absolute measurements. The SHR is associated with adverse outcomes for patients with moderate-to-severe COVID-19 (35), including elevated in-hospital morbidity and mortality (34).
An early investigation of patients with COVID-19 (n = 99; 67% men, 33% women) in Wuhan, China reports arterial and/or endocrine comorbidities in 53%, and hyperglycemia in 52% of those studied (36). Subsequently, diabetes, predominantly T2D, emerges as one of the most common and consequential comorbidities to worsen outcomes for those infected with the SARS-CoV-2 virus (37). New-onset hyperglycemia, with and without T2D, is also commonly observed with COVID-19 (38) and may result from inflammation, metabolic stress, and/or steroid therapy.
Regardless of underlying diabetes, stress-induced hyperglycemia is a well-documented occurrence during acute infections and has been observed even in mild cases of COVID-19 (35). The purported mechanisms causing hyperglycemia rely on the imbalance between insulin action and insulin secretion, and are primarily instigated by inflammation, cytokine action, neuroendocrine mechanisms, and counter-regulatory hormones (3). For a patient with T1D, the degree of hyperglycemia can be severe due to the presence of absolute insulin deficiency. The acute rise of blood glucose levels and catabolic processes can lead to diabetic ketoacidosis, a life-threatening event. In a patient with T2D, the severity of the hyperglycemia may not be a medical emergency, but the prolonged nature is associated with increased risk of cardiovascular morbidity and mortality (39). Hyperglycemia can also result in increased morbidity and mortality due to decompensation of the immune system in the face of glucotoxicity (40).
The mechanisms of increased morbidity and mortality associated with acute or chronic hyperglycemia in diabetes are multifocal. The increased cytokines of an acute inflammatory response are known to globally blunt insulin receptor responsiveness (41). Poor insulin receptor function disproportionally allows degradation of the visceral fat compartment releasing a repertoire of proinflammatory adipokines such as leptin and adiponectin (42). The blunting of glucose uptake and insulin action by adipokines further aggravates hyperglycemia. In addition, increased inflammatory cytokines combined with proinflammatory adipokines promote the glycation of proteins, rendering them pro-adherent and prothrombotic (43). The net effect of an overabundance of these reactive glycated proteins promotes endothelial dysfunction, thrombosis, hypertension, compromised cellular function, and organ dysfunction (44).
Of particular interest in patients with SARS-CoV-2 infection is the exploitation of the angiotensin-converting enzyme (ACE)-2 receptor as an entry point into cells and initiation of infection. Patients with T2D have an overactive renin–angiotensin–aldosterone system (RAAS), with ACE-2 as a principal factor (45, 46). Upregulation of ACE-2 expression in cardiomyocytes increases susceptibility to COVID-19 in patients with T2D by facilitating SARS-CoV2 cellular entry (45, 46). Abnormal adiposity is a necessary feature of ABCD and includes unusual quantity (eutopic [including visceral fat] and ectopic [e.g., intrahepatic and peri/epicardial fat]), distribution (primary related to visceral and ectopic fat), and function (i.e., adipocyte secretome; adipokine secretion). Abnormal adiposity not only leads to inflammation, insulin resistance, DBCD, and CMBCD, but also contributes to increased RAAS activation (47). These mechanisms involve cytokine activation of multiple elements of the RAAS cascade, such as angiotensinogen and ACE, resulting in inflammatory adipokine release from fatty tissue (48). This imbalance of RAAS function can increase susceptibility to COVID-19 in patients with T2D (49).
The immunopathogenesis of COVID-19 also involves an excessive inflammatory response that can intensify into a cytokine storm in extreme cases (50, 51). Numerous inflammatory pathways are activated in this process, including facilitation of immune cell (e.g., monocytes, macrophages, neutrophils, natural killer cells, and T cells) as well as stimulation and secretion of proinflammatory cytokines (e.g., interferons, interleukins, tumor necrosis factors, and chemokines [e.g., C-base sequence chemokine ligands]) (51). In turn, a proinflammatory response recruits and activates more innate and adaptive immune cells that overstimulate the immune system, leading to massive inflammation (51). This detrimental inflammatory process can incite and exacerbate acute respiratory distress syndrome (ARDS), the leading cause of COVID-19 related mortality.
Edge 2: COVID-19 and malnutrition
Epidemiology
Malnutrition is the necessary and central driver of MBCD, which in turn is one of the secondary/metabolic drivers in CMBCD. The American Society for Parenteral and Enteral Nutrition (ASPEN) defines malnutrition as insufficient energy intake leading to loss of weight, muscle, and subcutaneous fat; regional or widespread fluid accumulation; and decreased strength (52). Malnutrition is typically interpreted along these somewhat narrow lines that relate to undernutrition, particularly in COVID-19 discussions, but technically the broader definition includes any abnormal interaction between dietary factors and metabolism. For instance, abnormal adiposity is a form of malnutrition (i.e., imbalance of too much dietary energy for an individual’s metabolic needs—overnutrition) and is briefly considered above in the discussions on inflammation, insulin resistance, and T2D with COVID-19. However, for the purposes of presenting the syndromic triad of COVID-19, T2D, and malnutrition, the term “malnutrition” will be based on the ASPEN definition (53). In the MBCD model, stage 1 arises through complex interactions of primary drivers (genetics, environment, and behavior/lifestyle) and defines a state of nutritional risk; MBCD stage 2 arises from progression of nutritional risks to create a phenotype characterized by abnormal metrics of nutritional status, but not yet satisfying current diagnostic criteria for malnutrition (i.e., in terms of undernutrition) (54–57) or other abnormal nutritional states, and defines a state of “pre-malnutrition;” MBCD stage 3 meets established definitions for the disease state referred to as malnutrition (54–57); and stage 4 is malnutrition-related complications (generally in terms of organ dysfunction, behaviors, and other pathophysiological abnormalities).
Population-level malnutrition is associated with increased rates of fatal COVID-19 in areas where undernutrition is commonplace (58). Moreover, nutritional status is adversely affected by acute and chronic infections, which serve as negative prognosticators, especially in institutional settings (59–61). Patients with COVID-19 are especially vulnerable to the metabolic derangements associated with malnutrition, particularly in light of the significant inflammatory response that accompanies both conditions (62–64). A high prevalence of malnutrition in a general cohort of patients with COVID-19 has been reported in prospective studies (65). For example, among elderly patients with COVID-19, the prevalence of malnutrition reaches 52.7% (66). Poor nutritional status is associated with in-hospital death among 295 patients with COVID-19, including 66 with severe illness and 41 with critical illness (67). In this study, the mortality rate is 8.47% for the total study population and 37.88% for the critically ill subgroup (67). Furthermore, despite significantly different nutritional parameters and inflammatory markers across all subgroups, patients with higher Controlling Nutritional Status (CONUT) scores and lower Geriatric Nutrition Risk and Prognostic Nutritional Indices (GNRI and PNI) have a higher risk of in-hospital mortality (67).
Coronavirus disease 2019 symptoms (e.g., anorexia, nausea, vomiting, dysphagia, bloating, abdominal pain, and diarrhea) can disrupt eating and diminish adequate food consumption. In various studies, approximately 50% of patients with COVID-19 report olfactory and gustatory dysfunction, which may contribute to loss of appetite and a subsequent reduction of nutrient intake (65, 68–70). Although malnutrition associated with COVID-19 can be overlooked during the management of critical medical issues, nutrition support for patients with COVID-19 is an essential component of care, though timing and other specifics require further empirical study.
Mechanisms
The intersection of nutritional and cardiometabolic risk in patients with COVID-19 occurs at the level of inflammation and insulin resistance (2, 6, 7). The CMBCD model represents a range of patients who may be more susceptible to infections, including COVID-19, and could benefit from nutritional interventions to mitigate DBCD, MBCD, and CMBCD progression (53, 71–73).
Various micronutrients are known to affect host immunity and the natural history of COVID-19. Some vitamins (e.g., A and D) are direct regulators of immune-cell gene expression, while others (e.g., C and E) promote a pro-oxidant milieu to improve immunity (74). Trace elements, such as zinc, copper, and iron, can modulate susceptibility to respiratory infections (74). Also, phytonutrients (e.g., berberine, curcumin, epigallocatechin gallate, genistein, resveratrol, and sulforaphane) can activate nuclear factor (erythroid-derived 2)–like 2 antioxidant transcription factor, thought to be an important mechanism in COVID-19 pathogenesis (75). Dietary fiber, a critically important component of healthy diets, is fermented into short-chain fatty acids in the intestine and can also mount significant anti-inflammatory effects (76). The net message is that all populations require a healthy eating pattern to control weight and ABCD, prevent DBCD/MBCD/CMBCD progression, and optimize immunity before, during, and after COVID-19 (2, 6, 7, 77).
The co-existence of undernutrition with micronutrient deficiencies is associated with COVID-19 and its sequelae. The effects are compounded by a disrupted sense of smell and taste, food insecurity, and social distancing that disrupts normal lifestyle behavior and leads to unhealthy eating patterns, physical inactivity, and routine change that can affect micronutrient intake (78–81). In some patients, COVID-19 also involves the gastrointestinal tract causing nausea, vomiting, and diarrhea, which further contributes to MBCD staged progression (82). In general, patients with cough, pneumonia, respiratory failure, and immune-neuroendocrine axis activation via a stress response to acute or chronic illness have an impaired ability to maintain adequate nourishment (83). Put another way, MBCD and other CMBCD drivers (especially ABCD and DBCD) can sufficiently alter the immune response so that prevention and treatment are compromised, and the progression of COVID-19 results in more severe disease.
Patients hospitalized with COVID-19 are at higher nutrition risk (84). Nutritional status becomes worse in patients with COVID-19 who are admitted to the ICU or require artificial ventilation (84). Immobility in the hospital bed is also associated with sarcopenia, which may affect whole-body functioning in patients with COVID-19 (85). In the short-term, these body composition changes can impact susceptibility and immunological responses to SARS-CoV-2, subsequent inflammatory response, and resulting metabolic and respiratory distress. In the long-term, these body composition changes can modulate the time required for recovery, risk of ICU-acquired weakness and long-term disabilities, and mortality risk (84). Importantly, malnutrition has been shown to persist 30 days post-COVID-19 discharge (86). As such, patients with COVID-19, especially those with diabetes, may require tailored medical nutrition therapy to improve short- and long-term COVID-19 outcomes (71, 72, 87, 88).
Edge 3: Malnutrition and type 2 diabetes
Epidemiology
Although abnormal adiposity (overnutrition) is one of the most common comorbidities of T2D, undernutrition is also commonplace, with a frequency of one in seven patients with a high BMI, based on an outpatient diabetes cohort (89). A 21.2% malnutrition rate has been observed among elderly patients with diabetes, regardless of BMI (90). Additionally, many other studies have been conducted to determine the frequency of coexisting T2D and malnutrition. Among hospitalized patients in Spain, the risk of malnutrition (52) is higher with T2D (90). The risk of malnutrition and the actual malnutrition rate were 31 and 13%, respectively, among patients with diabetes assessed in a Turkish outpatient clinic; whereas, a similar assessment among hospitalized patients revealed even higher numbers: 39% risk vs. 25% prevalence (91, 92). Taken together, these associations suggest that when T2D is complicated by malnutrition (i.e., when diet is insufficient to meet age-related requirements), clinical challenges worsen and warrant a diligent approach to nutrition support and prudent supplementation with micro- and macronutrients (93, 94).
Patients with T2D can also exhibit sarcopenia (95, 96), a degenerative condition characterized by decreased skeletal muscle mass and weakness, typically observed in elderly populations and commonly associated with neurodegeneration, inflammation, and/or malnutrition (97–102). The association between T2D and sarcopenia had been shown in community-dwelling elderly adults (OR = 1.40, 95% CI: 1.18–1.66) (103). Older adults with either diagnosed or undiagnosed T2D showed excessive loss of skeletal muscle mass compared with those without T2D (103). While those without T2D lose an average of 198 ± 10 g of their total lean mass per year, patients with T2D lose about 222 ± 29 g/year and patients with undiagnosed T2D lose around 340 ± 37 g/year (104). Generalized loss of muscle mass is observed after age 40 and estimated to be 8% per decade up to age 70 years, and 15–25% every decade afterward (105). Additionally, in patients with T2D, plus or minus sarcopenia, omega-3 fatty acid intake is reduced (2.6 vs. 3.0 g/day, respectively) (106). Sarcopenia also compromises glycemic control and contributes to lower energy expenditure and generalized weakness as patients age, amplifying nutritional imperatives (101).
While sarcopenia is commonly conceptualized as weight loss and weakness related to diminished muscle mass, obesity may also accompany the disorder (107, 108). Thus, patients with T2D may present with both a low BMI, characteristic of sarcopenia, and high body fat content, characteristic of adiposity, leading to the descriptive terminology—“sarcopenic obesity.” Diagnostic criteria often combine single or multiple assessments of sarcopenia with the quantification of systemic and central adiposity. Depending on definition and population, the prevalence of sarcopenic obesity ranges from 0 to 20% (with average prevalence rates between 5 and 10%) in numerous international studies of older adults (108, 109). Prevalence calculations are lower (3–8%) if the height-adjusted appendicular lean mass (ALM) index is used to define sarcopenia (110) rather than weight- or BMI-adjusted ALM indices (6–10%) (111). Moreover, prevalence rates of sarcopenic obesity are significantly higher (16–25%) among people 80 years of age and older or when lower quintiles of muscle mass or higher quintiles of body fat are factored into the assessments (112).
Among both inpatients and outpatients with diabetes, malnutrition is associated with a dysregulated immune system, higher risk for acute and chronic diseases, and protracted illness (113, 114). Such patients, particularly those with low lean body mass and high adiposity, consistently experience poorer outcomes in many different diseases (115). Manifestations of compromised immunity in patients with COVID-19 include lymphopenia upon admission and thrombocytopenia with leukopenia as infections worsen (116). Likewise, elevated levels of C-reactive protein and proinflammatory cytokines have been associated with increasing severity of illness and attendant nutritional risk (116, 117).
Mechanisms
In general, patients with T2D and sarcopenia exhibit specific underlying pathophysiological mechanisms that have implications for nutritional care and lifestyle modifications. Among them are the consequences of aging, including altered physical activity and dietary patterns, as well as hormonal deficiencies, low-grade systemic inflammation, loss of protein homeostasis in muscle, mitochondrial dysfunction, and reduced quantity and function of small mononuclear satellite cells that abut muscle fibers (118–121). Hormonal deficiencies related to sarcopenia include growth hormone, testosterone, thyroid hormone, and insulin-like growth factor, all of which contribute to loss of muscle mass and subsequent physical weakness starting in midlife (122, 123). As anabolic hormonal signals decrease, catabolic signals increase via pro-inflammatory cytokines (tumor necrosis factor alpha [TNF-α] and possibly interleukin-6 [IL-6]), homocysteine and high-sensitive C-reactive protein levels rise, and muscle wasting accelerates (96, 122). Muscle loss, in turn, exacerbates insulin resistance, hyperglycemia and DBCD progression (102).
Changes in muscle metabolism and the diminished capability to synthesize sufficient protein to maintain muscle mass contribute to wasting syndromes (124). Over prolonged time, oxidized proteins accumulate in skeletal muscle, and accrued lipofuscin and cross-linked protein deposits are retained (119). Non-contractile dysfunctional protein replaces normal tissue and leads to the loss of muscle function and the diminished strength that characterize sarcopenia (125). Moreover, motor nerve cells that carry impulses from brain to muscle diminish with age, and movement is compromised by insufficient neurotransmission. Supportive satellite cells, normally responsive to injury or activity, fail to undergo functional differentiation and fusion with myocytes, leading to loss of contractile function (119, 122). These pathophysiological mechanisms can affect diaphragmatic muscles (126), which has significant implications for patients suffering from the syndromic triad of COVID-19, T2D, and malnutrition.
Nutritional therapy in patients with acute COVID-19, T2D, and malnutrition
Although no unified therapeutic regimen exists for the comprehensive management of patients with the syndromic triad, physical activity and therapeutic nutrition represent two approaches that have proven merit across the triad spectrum. Persistent daily activity and dedicated exercise programs can improve glycemic regulation and decrease muscle degradation, while diets rich in protein or amino acids are helpful for patients with T2D and malnutrition (127). Diets that accentuate protein and antioxidants may combat sarcopenia by increasing muscle mass and strength via improved protein homeostasis and autophagy (the orderly degradation and recycling of cellular components) as well as reduced oxidative stress (120). Likewise, branched-chain amino acids, polyunsaturated fatty acids, selenium, vitamin D, and zinc can reduce oxidative stress, support mitochondrial homeostasis, and mitigate low-grade inflammation, thus suggesting their potential roles in the treatment of sarcopenia (128). To the contrary, however, a Mendelian randomization analysis shows little effect from these nutrients with the exception of a genetically high concentration of serum iron, which increased sarcopenia risk (129).
At the onset of the pandemic, limited therapeutic options existed to combat the specific problems eventually seen with COVID-19, especially infection complicated by T2D and malnutrition. Consequently, several expert groups in clinical nutrition adapted standard critical care guidelines centered on nutrition for COVID-19 (130). A comparison of approaches by ASPEN and the European Society for Parenteral and Enteral Nutrition (ESPEN) is given in Table 1 (131, 132). A brief summary of nutritional recommendations for patients with COVID-19 and critical illness includes: a blood glucose target of 6–8 mmol/L (106–145 mg/dL), nutrition assessments with malnutrition considerations, high-protein enteral and parenteral formulas, and up to a 50:50 ratio of fat-to-carbohydrate in patients receiving ventilatory support (132).
Individualized medical nutrition therapy can include diabetes-specific nutritional formulas (DSNFs) that are commercial products designed to improve glycemic status. The DSNFs are supported by extensive clinical research using oral enteral access routes for better glycemic control in the ICU setting (131–133). Additionally, specific benefits for DSNFs are observed in a randomized clinical trial where 73% of patients are ventilated and 51% of these have diabetes upon admission. Those who receive DSNF vs. a standard enteral formula require significantly less insulin to maintain lower glycemic variability through 48 h of care (134). Likewise, a study of patients with critical illness and hyperglycemia on mechanical ventilation reports lower insulin requirements and diminished glycemic variability using a DSNF compared to a high-protein control formula (135). Patients using a DSNF also experience a lower incidence of ventilator complications (135). Interpretation and application of these findings are important for patients with COVID-19 and T2D, as hyperglycemia and glycemic variability are each associated with worse clinical outcomes (136, 137).
Chronic/post-COVID-19
Recovery from COVID-19 also presents unique nutritional challenges related to both hospital/ICU duration and disease severity. Despite usual recommendations for increased protein intake for patients with critical illness (>1.3–1.5 g/kg/day) (132, 138), muscle loss and potential sarcopenia are still anticipated due, in part, to inactivity coupled with an inflamed hypermetabolic state (139). One Brazilian study reports a 30% decrease in rectus femoris cross-sectional area in patients with COVID-19 after just 10 days in the ICU (140). These patients may also experience post-ICU syndrome and/or dysphagia, which may adversely affect nutritional status (141–143). Special consideration for lingering COVID-19 symptoms is often necessary as 57% of COVID-19 survivors report ongoing problems through 6 months of recovery (144). In such circumstances, individualized rehabilitation efforts and conscientious diets are required to address malnutrition, sarcopenia, and/or dysphagia (145).
For patients with DBCD, particularly stage 3 T2D or stage 4 T2D with complications during prolonged recovery and rehabilitation, DSNF supplementation may be advisable as well. Research pre-dating the COVID-19 pandemic demonstrate that lower A1C values and increased body weight along with improvements in nutritional status and quality of life at 6 and 12 weeks are attainable with 2 servings/day of a high-protein DSNF in compromised older subjects (n = 402) with T2D and malnutrition (146). However, in a small study of enterally fed patients with T2D and unintentional weight loss, subsequent increases in weight are primarily attributed to body fat (147). Therefore, to improve body composition, rehabilitation efforts that include physical therapy or progressive resistance training should be part of multimodality care to increase muscle protein synthesis and enhance functional, metabolic, and psychological status (148, 149).
A significant knowledge gap surrounding specific micronutrient or anti-inflammatory supplementation still exists for patients with COVID-19 (7). Until more specific clinical evidence is available, expert opinions should prevail for implementing standard supplementation practices in patients with critical illness associated with COVID-19 (131). Emerging evidence suggests using vitamins, minerals, or other supportive micronutrients and standard nutrition formulas as tolerated by select patient groups.
For example, clinical practice guidelines propose administration of vitamins A, B complex, D, C, as well as selenium, zinc, and iron (132). Due to their anti-inflammatory qualities, omega-3 fatty acids are also studied in patients with critical illness and included in evidence-based guidelines (132, 150). In one study of critical illness and COVID-19, improved respiratory and renal function, along with higher 1-month survival, is noted in patients who received omega-3 fatty acid (400 mg EPA and 200 mg DHA) supplementation for 2 weeks, compared to patients receiving a standard enteral formula (151). Moreover, vitamin D and zinc gained attention for prophylaxis at the start of the pandemic (152).
In a cohort where over 50% of the sample have T2D, hospitalized patients with mild-to-moderate COVID-19 experience a faster recovery time for cough (∼3 days) and altered taste (∼5 days) when supplemented daily with 5,000 IU compared to 1,000 IU of vitamin D (153). Another study notes attenuated muscle catabolism with post-COVID-19 vitamin D supplementation of 200 IU/day for 6 weeks (154). However, the muscle retention is not reflected by improvements in physical function, which questions the adequacy of vitamin D metabolism to active 1,25-dihydroxyvitamin D in patients with critical illness and COVID-19, which could limit therapeutic potential (154, 155).
Other immunonutrients, including the amino acids glycine, arginine, and glutamine, may mitigate inflammation, protect lung and intestinal integrity during acute illness, and support muscle renewal during recovery (156). Unfortunately, the volatile status of the pandemic continues to limit clinical trials on COVID-19-specific nutrition recommendations, and some reports indicate suboptimal institutional adherence to existing guidelines (157–159). The completion of well-designed clinical trials and then creation and adoption of subsequent evidence-based guidelines is critically important for lowering mortality and shortening hospital stays (158).
Hypotheses and structure for early preventive care: The critical role of lifestyle medicine
Patient surveys conducted during the initial 2020 quarantines and social-distancing mandates disclose disruptive changes in lifestyle and personal routines with the COVID-19 pandemic (81). In particular, routine change negatively affects diabetes self-management, delays required healthcare, and accentuates individual pandemic-associated stress (81, 160–162). The effects of widespread systemic disruptions, such as lulls in screening practices and routine medical oversight, are now more clear and prompting greater attention by healthcare professionals (163–167). Reports and qualitative assessments pointing to patient-perceived practice gaps in usual diabetes support are collectively underscoring the need for countermeasures to reverse these disruptions and restore healthy lifestyles (168). This is particularly true in contemporary multimorbidity care models that seek to manage multiple chronic disease states (e.g., chronic/post-COVID-19 + T2D + malnutrition) concurrently (168, 169). In effect, the COVID-19 pandemic draws much needed attention to comprehensive chronic disease management, creating opportunities to advance diabetes and nutrition care.
Encouragement for lifestyle modification has the potential to minimize infection risk during the COVID-19 era. For example, one prospective cohort study observes higher risk (3.5%) for COVID-19 infection and severe COVID-19 illness in participants in the lowest vs. highest quartile of diet quality (170). Specifically, crude incidence rates are 3.5% higher for COVID-19 infection in the lowest diet quality quartile compared to the highest (170). Using very low-calorie diets, which often utilize meal replacement products, and incorporating DNSFs as part of lifestyle change, support weight loss and adequate glycemic control (171, 172). Awareness of the connection between COVID-19 risk and cardiometabolic impairment presents a unique opportunity to emphasize preventive and complementary initiatives to promote better health, reduce CMBCD risk, and mitigate DBCD progression with comprehensive interventions that incorporate lifestyle modifications.
Although access to health resources is challenged during the pandemic, telehealth offers a solution with a 154% increase in usage at the beginning of the pandemic (173). Telehealth may be especially applicable to diabetes with one study reporting that 95% of diabetes-related visits are virtual during the first year of the pandemic (174), and its use in the diabetes space is associated with improved patient outcomes (175–180). As an example, a recent meta-analysis reports increased time in range by 70.74 min and a slight decrease in A1C (−0.17%) among people using continuous glucose monitors (CGMs) compared to usual care (175). This effect could stem from healthful behavior modification associated with CGM use (technological nudges and motivation) (176). The integration of telehealth stands to diminish pre-pandemic barriers to healthcare, but it is important to consider stakeholder acceptance and inclusion of vulnerable populations (181).
The creation of a new construct—the syndromic triad of COVID-19, T2D, and malnutrition—not only allows the derivation of hypotheses relating early detection and management of malnutrition with mitigation of ABCD, DBCD, MBCD, and even CMBCD progression, but also prompts clinical decision-making now centered on early implementation of healthy lifestyle change. The pragmatic value of this new triad framework is supported by the coalescing of multiple clinical imperatives (i.e., COVID-19, dysglycemia, and nutrition) into a focused comprehensive approach. Core recommendations, which will require clinical validation, include:
1. Conduct aggressive case-finding protocols for malnutrition in all patients with COVID-19 at any DBCD stage
2. Implement current standards of care to optimize nutrition in all patients with COVID-19 at any DBCD stage who have malnutrition or are at-risk for malnutrition
3. Clarify and manage specific DBCD stages in all patients with COVID-19 at any MBCD stage; and
4. Assign a higher risk classification to patients newly diagnosed with COVID-19 when any DBCD or MBCD stage is also present.
Conclusion and future directions
There is an inherent association of COVID-19, T2D, and malnutrition supported by theoretical modeling, epidemiological data, and mechanistic relationships. Metabolic changes incurred by COVID-associated systemic inflammation increase the risk of dysglycemia, muscle protein catabolism, and nutritional deficiencies. Moreover, both T2D and malnutrition are risk factors of severe COVID-19. Awareness of these associations should encourage early diagnosis, prevention, and management of dysglycemia and malnutrition especially in vulnerable populations. Nutritional and lifestyle interventions aiming at optimizing glycemic control and improving nutritional status, as well as muscle health, could potentially decrease risk of COVID-19 complications. An individualized T2D-specific lifestyle and nutritional approach, and a close monitoring and management of glycemic status by experienced healthcare professionals, are essential to improve clinical outcomes for people with COVID-19.
Author contributions
All authors listed have made a substantial, direct, and intellectual contribution to the work, and approved it for publication.
Funding
This study received funding from Abbott Nutrition. The funder was not involved in the study design, collection, analysis, interpretation of data, and the writing of this article or the decision to submit it for publication.
Conflict of interest
EA was employed by Endocrinology Associates, Inc. AM was employed by Medical Education and Research Alliance (Med-ERA, Inc). KH was employed by Abbott Nutrition during the creation of the present manuscript. The manuscript was funded by Abbott Nutrition and completed independently from KH’s role at Novo Nordisk, Inc., at the time of submission. Novo Nordisk, Inc., was not involved in the creation, funding, review, or submission of the completed manuscript. RH was employed by Abbott Nutrition.
The remaining authors declare that the research was conducted in the absence of any commercial or financial relationships that could be construed as a potential conflict of interest.
Publisher’s note
All claims expressed in this article are solely those of the authors and do not necessarily represent those of their affiliated organizations, or those of the publisher, the editors and the reviewers. Any product that may be evaluated in this article, or claim that may be made by its manufacturer, is not guaranteed or endorsed by the publisher.
Abbreviations
ABCD, adiposity-based chronic disease; ARDS, acute respiratory distress syndrome; ASPEN, American Society for Parenteral and Enteral Nutrition; ALM, appendicular lean mass; CDC, centers for disease control; CGM, continuous glucose monitor; CIRCS, COVID-related cardiometabolic syndrome; CMBCD, cardiometabolic-based chronic disease; CONUT, controlling nutritional status; COVID-19, coronavirus disease 2019; DBCD, dysglycemia-base chronic disease; DSNF, diabetes-specific nutrition formula; EN, enteral nutrition; ESPEN, European Society for Parenteral and Enteral Nutrition; GNRI-geriatric nutrition risk index; HbA1c, hemoglobin A1C; HBCD, hypertension-based chronic disease; ICU, intensive care unit; IL-6, interleukin-6; LBCD, lipid-based chronic disease; MBCD, metabolic based chronic disease; PNI, prognostic nutritional index; PPE, personal protective equipment; RAAS, renin-angiotensin-aldosterone system; SARS-CoV-2, severe acute respiratory syndrome coronavirus 2; SCCM, society of critical care medicine; SDOH/TF, social determinants of health/transcultural factors; T1D, type 1 diabetes; T2D, type 2 diabetes; TFN, tumor necrosis factor.
References
1. Liu J, Ren Z, Qiang H, Wu J, Shen M, Zhang L, et al. Trends in the incidence of diabetes mellitus: results from the global burden of disease study 2017 and implications for diabetes mellitus prevention. BMC Public Health. (2020) 20:1415. doi: 10.1186/s12889-020-09502-x
2. Mechanick J, Rosenson R, Pinney S, Mancini D, Narula J, Fuster V. Coronavirus and cardiometabolic syndrome: JACC focus seminar. J Am Coll Cardiol. (2020) 76:2024–35. doi: 10.1016/j.jacc.2020.07.069
3. Mechanick J, Farkouh M, Newman J, Garvey W. Cardiometabolic-based chronic disease, adiposity and dysglycemia drivers: JACC state-of-the-art review. J Am Coll Cardiol. (2020) 75:525–38. doi: 10.1016/j.jacc.2019.11.044
4. Mechanick J, Farkouh M, Newman J, Garvey W. Cardiometabolic-based chronic disease, addressing knowledge and clinical practice gaps: JACC state-of-the-art review. J Am Coll Cardiol. (2020) 75:539–55. doi: 10.1016/j.jacc.2019.11.046
5. de Oliveira Correia E, Mechanick J, Dos Santos Barbetta L, Jorge A, Mesquita E. Cardiometabolic-based chronic disease: adiposity and dysglycemia drivers of heart failure. Heart Fail Rev. (2022). doi: 10.1007/s10741-022-10233-x [Epub ahead of print].
6. Mechanick J, Garber A, Grunberger G, Handelsman Y, Garvey W. Dysglycemia-based chronic disease: an American association of clinical endocrinologists position statement. Endocr Pract. (2018) 24:995–1011. doi: 10.4158/PS-2018-0139
7. Mechanick J, Carbone S, Dickerson R, Hernandez B, Hurt R, Irving S, et al. Clinical nutrition research and the COVID-19 pandemic: a scoping review of the ASPEN COVID-19 task force on nutrition research. JPEN J Parenter Enteral Nutr. (2021) 45:13–31. doi: 10.1002/jpen.2036
8. Rathmann W, Kuss O, Kostev K. Incidence of newly diagnosed diabetes after Covid-19. Diabetologia. (2022) 65:949–54. doi: 10.1007/s00125-022-05670-0
9. Xie Y, Al-Aly Z. Risks and burdens of incident diabetes in long COVID: a cohort study. Lancet Diabetes Endocrinol. (2022) 10:311–21. doi: 10.1016/S2213-8587(22)00044-4
10. Hernandez-Romieu A, Carton T, Saydah S, Azziz-Baumgartner E, Boehmer T, Garret N, et al. Prevalence of select new symptoms and conditions among persons aged younger than 20 years and 20 years or older at 31 to 150 days after testing positive or negative for SARS-CoV-2. JAMA Netw Open. (2022) 5:e2147053. doi: 10.1001/jamanetworkopen.2021.47053
11. Barrett C, Koyama A, Alvarez P, Chow W, Lundeen E, Perrine C, et al. Risk for newly diagnosed diabetes >30 days after SARS-CoV-2 infection among persons aged <18 years - united states, march 1, 2020-June 28, 2021. MMWR Morb Mortal Wkly Rep. (2022) 71:59–65. doi: 10.15585/mmwr.mm7102e2
12. Modarelli R, Sarah S, Ramaker M, Bolobiongo M, Benjamin R, Gumus Balikcioglu P. Pediatric diabetes on the rise: trends in incident diabetes during the COVID-19 pandemic. J Endocr Soc. (2022) 6:bvac024. doi: 10.1210/jendso/bvac024
13. Banerjee M, Pal R, Dutta S. Risk of incident diabetes post-COVID-19: a systematic review and meta-analysis. Prim Care Diabetes. (2022) 16:591–3. doi: 10.1016/j.pcd.2022.05.009
14. Wander P, Lowy E, Beste L, Tulloch-Palomino L, Korpak A, Peterson A, et al. The incidence of diabetes among 2,777,768 veterans with and without recent SARS-CoV-2 infection. Diabetes Care. (2022) 45:782–8. doi: 10.2337/dc21-1686
15. Docherty A, Harrison E, Green C, Hardwick H, Pius R, Norman L, et al. Features of 20 133 UK patients in hospital with covid-19 using the ISARIC WHO clinical characterisation protocol: prospective observational cohort study. BMJ. (2020) 369:m1985. doi: 10.1136/bmj.m1985
16. Garg M, Kim L, Whitaker M, O’Halloran A, Kambhampati A, Chai S, et al. Hospitalization rates and characteristics of children aged <18 years hospitalized with laboratory-confirmed COVID-19 - COVID-NET, 14 states, march 1-July 25, 2020. MMWR Morb Mortal Wkly Rep. (2020) 69:1081–8. doi: 10.15585/mmwr.mm6932e3
17. Richardson S, Hirsch J, Narasimhan M, Crawford J, McGinn T, Davidson K, et al. Presenting characteristics, comorbidities, and outcomes among 5700 patients hospitalized with COVID-19 in the New York city area. JAMA. (2020) 323:2052–9. doi: 10.1001/jama.2020.6775
18. Suleyman G, Fadel R, Malette K, Hammond C, Abdulla H, Entz A, et al. Clinical characteristics and morbidity associated with coronavirus disease 2019 in a series of patients in metropolitan Detroit. JAMA Netw Open. (2020) 3:e2012270. doi: 10.1001/jamanetworkopen.2020.12270
19. Thakur B, Dubey P, Benitez J, Torres J, Reddy S, Shokar N, et al. A systematic review and meta-analysis of geographic differences in comorbidities and associated severity and mortality among individuals with COVID-19. Sci Rep. (2021) 11:8562. doi: 10.1038/s41598-021-88130-w
20. Wortham J, Lee J, Althomsons S, Latash J, Davidson A, Guerra K, et al. Characteristics of persons who died with COVID-19 - united states, february 12-May 18, 2020. MMWR Morb Mortal Wkly Rep. (2020) 69:923–9. doi: 10.15585/mmwr.mm6928e1
21. Bailly L, Fabre R, Courjon J, Carles M, Dellamonica J, Pradier C. Obesity, diabetes, hypertension and severe outcomes among inpatients with coronavirus disease 2019: a nationwide study. Clin Microbiol Infect. (2022) 28:114–23. doi: 10.1016/j.cmi.2021.09.010
22. Hoddy K, Axelrod C, Mey J, Hari A, Beyl R, Blair J, et al. Insulin resistance persists despite a metabolically healthy obesity phenotype. Obesity. (2022) 30:39–44.
23. Unluguzel Ustun G, Keskin A, Aci R, Arslanbek Erdem M, Ari M. Association between Hb A1c and severity of COVID-19 patients. Hemoglobin. (2021) 45:124–8. doi: 10.1080/03630269.2021.1926278
24. Roncon L, Zuin M, Rigatelli G, Zuliani G. Diabetic patients with COVID-19 infection are at higher risk of ICU admission and poor short-term outcome. J Clin Virol. (2020) 127:104354. doi: 10.1016/j.jcv.2020.104354
25. Corona G, Pizzocaro A, Vena W, Rastrelli G, Semeraro F, Isidori A, et al. Diabetes is most important cause for mortality in COVID-19 hospitalized patients: systematic review and meta-analysis. Rev Endocr Metab Disord. (2021) 22:275–96. doi: 10.1007/s11154-021-09630-8
26. Alberti A, Schuelter-Trevisol F, Iser B, Traebert E, Freiberger V, Ventura L, et al. Obesity in people with diabetes in COVID-19 times: important considerations and precautions to be taken. World J Clin Cases. (2021) 9:5358–71. doi: 10.12998/wjcc.v9.i20.5358
27. Rawshani A, Kjölhede E, Rawshani A, Sattar N, Eeg-Olofsson K, Adiels M, et al. Severe COVID-19 in people with type 1 and type 2 diabetes in Sweden: a nationwide retrospective cohort study. Lancet Reg Health Eur. (2021) 4:100105. doi: 10.1016/j.lanepe.2021.100105
28. Xie J, Zu Y, Alkhatib A, Pham T, Gill F, Jang A, et al. Metabolic syndrome and COVID-19 mortality among adult black patients in New Orleans. Diabetes Care. (2020) 44:188–93. doi: 10.2337/dc20-1714
29. Sperling L, Mechanick J, Neeland I, Herrick C, Després J, Ndumele C, et al. The cardiometabolic health alliance: working toward a new care model for the metabolic syndrome. J Am Coll Cardiol. (2015) 66:1050–67. doi: 10.1016/j.jacc.2015.06.1328
30. Mason K, Maudsley G, McHale P, Pennington A, Day J, Barr B. Age-adjusted associations between comorbidity and outcomes of COVID-19: a review of the evidence from the early stages of the pandemic. Front Public Health. (2021) 9:584182. doi: 10.3389/fpubh.2021.584182
31. Pal R, Bhadada S. COVID-19 and diabetes mellitus: an unholy interaction of two pandemics. Diabetes Metab Syndr. (2020) 14:513–7. doi: 10.1016/j.dsx.2020.04.049
32. Berbudi A, Rahmadika N, Tjahjadi A, Ruslami R. Type 2 diabetes and its impact on the immune system. Curr Diabetes Rev. (2020) 16:442–9. doi: 10.2174/1573399815666191024085838
33. Singh V. Can vitamins, as epigenetic modifiers, enhance immunity in COVID-19 patients with non-communicable disease? Curr Nutr Rep. (2020) 9:202–9. doi: 10.1007/s13668-020-00330-4
34. Roberts G, Quinn S, Valentine N, Alhawassi T, O’Dea H, Stranks S, et al. Relative hyperglycemia, a marker of critical illness: introducing the stress hyperglycemia ratio. J Clin Endocrinol Metab. (2015) 100:4490–7. doi: 10.1210/jc.2015-2660
35. Mondal S, DasGupta R, Lodh M, Garai R, Choudhury B, Hazra A, et al. Stress hyperglycemia ratio, rather than admission blood glucose, predicts in-hospital mortality and adverse outcomes in moderate-to severe COVID-19 patients, irrespective of pre-existing glycemic status. Diabetes Res Clin Pract. (2022) 190:109974. doi: 10.1016/j.diabres.2022.109974
36. Chen N, Zhou M, Dong X, Qu J, Gong F, Han Y, et al. Epidemiological and clinical characteristics of 99 cases of 2019 novel coronavirus pneumonia in Wuhan, China: a descriptive study. Lancet. (2020) 395:507–13. doi: 10.1016/S0140-6736(20)30211-7
37. Caballero A, Ceriello A, Misra A, Aschner P, McDonnell M, Hassanein M, et al. COVID-19 in people living with diabetes: an international consensus. J Diabetes Complications. (2020) 34:107671. doi: 10.1016/j.jdiacomp.2020.107671
38. Singh A, Singh R. Hyperglycemia without diabetes and new-onset diabetes are both associated with poorer outcomes in COVID-19. Diabetes Res Clin Pract. (2020) 167:108382. doi: 10.1016/j.diabres.2020.108382
39. Hasheminasabgorji E, Jha J. Dyslipidemia, diabetes and atherosclerosis: role of inflammation and ros-redox-sensitive factors. Biomedicines. (2021) 9:1602. doi: 10.3390/biomedicines9111602
40. Pezhman L, Tahrani A, Chimen M. Dysregulation of leukocyte trafficking in type 2 diabetes: mechanisms and potential therapeutic avenues. Front Cell Dev Biol. (2021) 9:624184. doi: 10.3389/fcell.2021.624184
41. Scheen M, Giraud R, Bendjelid K. Stress hyperglycemia, cardiac glucotoxicity, and critically ill patient outcomes current clinical and pathophysiological evidence. Physiol Rep. (2021) 9:e14713. doi: 10.14814/phy2.14713
42. Zatterale F, Longo M, Naderi J, Raciti G, Desiderio A, Miele C, et al. Chronic adipose tissue inflammation linking obesity to insulin resistance and type 2 diabetes. Front Physiol. (2020) 10:1607. doi: 10.3389/fphys.2019.01607
43. Rojas A, Lindner C, Gonzàlez I, Morales M. Advanced-glycation end-products axis: a contributor to the risk of severe illness from COVID-19 in diabetes patients. World J Diabetes. (2021) 12:590–602. doi: 10.4239/wjd.v12.i5.590
44. Landecho M, Marin-Oto M, Recalde-Zamacona B, Bilbao I, Frühbeck G. Obesity as an adipose tissue dysfunction disease and a risk factor for infections - Covid-19 as a case study. Eur J Intern Med. (2021) 91:3–9. doi: 10.1016/j.ejim.2021.03.031
45. D’Onofrio N, Scisciola L, Sardu C, Trotta M, De Feo M, Maiello C, et al. Glycated ACE2 receptor in diabetes: open door for SARS-COV-2 entry in cardiomyocyte. Cardiovasc Diabetol. (2021) 20:99. doi: 10.1186/s12933-021-01286-7
46. Norouzi M, Norouzi S, Ruggiero A, Khan M, Myers S, Kavanagh K, et al. Type-2 diabetes as a risk factor for severe COVID-19 infection. Microorganisms. (2021) 9:1211. doi: 10.3390/microorganisms9061211
47. Miricescu D, Balan D, Tulin A, Stiru O, Vacaroiu I, Mihai D, et al. Impact of adipose tissue in chronic kidney disease development (review). Exp Ther Med. (2021) 21:539. doi: 10.3892/etm.2021.9969
48. Satou R, Penrose H, Navar L. Inflammation as a regulator of the renin-angiotensin system and blood pressure. Curr Hypertens Rep. (2018) 20:100. doi: 10.1007/s11906-018-0900-0
49. Steenblock C, Richter S, Berger I, Barovic M, Schmid J, Schubert U, et al. Viral infiltration of pancreatic islets in patients with COVID-19. Nat Commun. (2021) 12:3534. doi: 10.1038/s41467-021-23886-3
50. Ye Q, Wang B, Mao J. The pathogenesis and treatment of the ‘cytokine storm’ in COVID-19. J Infect. (2020) 80:607–13. doi: 10.1016/j.jinf.2020.03.037
51. Jiang Y, Zhao T, Zhou X, Xiang Y, Gutierrez-Castrellon P, Ma X. Inflammatory pathways in COVID-19: mechanism and therapeutic interventions. MedComm (2020). (2022) 3:e154. doi: 10.1002/mco2.154
52. White J, Guenter P, Jensen G, Malone A, Schofield M, Academy Malnutrition Work Group, et al. Consensus statement: academy of nutrition and dietetics and American society for parenteral and enteral nutrition: characteristics recommended for the identification and documentation of adult malnutrition (undernutrition). JPEN J Parenter Enteral Nutr. (2012) 36:275–83. doi: 10.1177/0148607112440285
53. ASPEN. ASPEN Definitions. (2022). Available online at: https://www.nutritioncare.org/ (accessed January 10, 2022).
54. Cederholm T, Barazzoni R, Austin P, Ballmer P, Biolo G, Bischoff S, et al. ESPEN guidelines on definitions and terminology of clinical nutrition. Clin Nutr. (2017) 36:49–64. doi: 10.1016/j.clnu.2016.09.004
55. Cederholm T, Bosaeus I, Barazzoni R, Bauer J, Van Gossum A, Klek S, et al. Diagnostic criteria for malnutrition–an ESPEN consensus statement. Clin Nutr. (2015) 34:335–40. doi: 10.1016/j.clnu.2015.03.001
56. Mogensen K, Malone A, Becker P, Cutrell S, Frank L, Gonzales K, et al. Academy of nutrition and dietetics/American society for parenteral and enteral nutrition consensus malnutrition characteristics: usability and association with outcomes. Nutr Clin Pract. (2019) 34:657–65. doi: 10.1002/ncp.10310
57. Rojer A, Kruizenga H, Trappenburg M, Reijnierse E, Sipilä S, Narici M, et al. The prevalence of malnutrition according to the new ESPEN definition in four diverse populations. Clin Nutr. (2016) 35:758–62. doi: 10.1016/j.clnu.2015.06.005
58. Mertens E, Peñalvo J. The burden of malnutrition and fatal COVID-19: a global burden of disease analysis. Front Nutr. (2021) 7:619850. doi: 10.3389/fnut.2020.619850
59. Burgos R, García-Almeida J, Matía-Martín P, Palma S, Sanz-Paris A, Zugasti A, et al. Malnutrition management of hospitalized patients with diabetes/hyperglycemia and COVID-19 infection. Rev Endocr Metab Disord. (2022) 23:205–13. doi: 10.1007/s11154-022-09714-z
60. Tappenden K, Quatrara B, Parkhurst M, Malone A, Fanjiang G, Ziegler T. Critical role of nutrition in improving quality of care: an interdisciplinary call to action to address adult hospital malnutrition. JPEN J Parenter Enteral Nutr. (2013) 37:482–97. doi: 10.1177/0148607113484066
61. Burgos R, Joaquín C, Blay C, Vaqué C. Disease-related malnutrition in hospitalized chronic patients with complex needs. Clin Nutr. (2020) 39:1447–53. doi: 10.1016/j.clnu.2019.06.006
62. Bedock D, Bel Lassen P, Mathian A, Moreau P, Couffignal J, Ciangura C, et al. Prevalence and severity of malnutrition in hospitalized COVID-19 patients. Clin Nutr ESPEN. (2020) 40:214–9. doi: 10.1016/j.clnesp.2020.09.018
63. Laviano A, Koverech A, Mari A. Cachexia: clinical features when inflammation drives malnutrition. Proc Nutr Soc. (2015) 74:348–54. doi: 10.1017/S0029665115000117
64. Wang J, Chen L, Huang Z, Lu J, Yang Y, Zhao X, et al. A synergistic association between inflammation, malnutrition, and mortality in patients with diabetics. Front Nutr. (2022) 9:872512. doi: 10.3389/fnut.2022.872512
65. Rouget A, Vardon-Bounes F, Lorber P, Vavasseur A, Marion O, Marcheix B, et al. Prevalence of malnutrition in coronavirus disease 19: the NUTRICOV study. Br J Nutr. (2021) 126:1296–303. doi: 10.1017/S0007114520005127
66. Li T, Zhang Y, Gong C, Wang J, Liu B, Shi L, et al. Prevalence of malnutrition and analysis of related factors in elderly patients with COVID-19 in Wuhan, China. Eur J Clin Nutr. (2020) 74:871–5. doi: 10.1038/s41430-020-0642-3
67. Song F, Ma H, Wang S, Qin T, Xu Q, Yuan H, et al. Nutritional screening based on objective indices at admission predicts in-hospital mortality in patients with COVID-19. Nutr J. (2021) 20:46. doi: 10.1186/s12937-021-00702-8
68. Bénézit F, Le Turnier P, Declerck C, Paillé C, Revest M, Dubée V, et al. Utility of hyposmia and hypogeusia for the diagnosis of COVID-19. Lancet Infect Dis. (2020) 20:1014–5. doi: 10.1016/S1473-3099(20)30297-8
69. Giacomelli A, Pezzati L, Conti F, Bernacchia D, Siano M, Oreni L, et al. Self-reported olfactory and taste disorders in patients with severe acute respiratory coronavirus 2 infection: a cross-sectional study. Clin Infect Dis. (2020) 71:889–90. doi: 10.1093/cid/ciaa330
70. Mao L, Jin H, Wang M, Hu Y, Chen S, He Q, et al. Neurologic manifestations of hospitalized patients with coronavirus disease 2019 in Wuhan, China. JAMA Neurol. (2020) 77:683–90. doi: 10.1001/jamaneurol.2020.1127
71. Antwi J, Appiah B, Oluwakuse B, Abu B. The nutrition-COVID-19 interplay: a review. Curr Nutr Rep. (2021) 10:364–74. doi: 10.1007/s13668-021-00380-2
72. James P, Ali Z, Armitage A, Bonell A, Cerami C, Drakesmith H, et al. The role of nutrition in COVID-19 susceptibility and severity of disease: a systematic review. J Nutr. (2021) 151:1854–78. doi: 10.1093/jn/nxab059
73. Lecube A, Pachón G, Petriz J, Hernández C, Simó R. Phagocytic activity is impaired in type 2 diabetes mellitus and increases after metabolic improvement. PLoS One. (2011) 6:e23366. doi: 10.1371/journal.pone.0023366
74. Calder P. Nutrition, immunity and COVID-19. BMJ Nutr Prev Health. (2020) 3:74–92. doi: 10.1136/bmjnph-2020-000085
75. Bousquet J, Cristol J, Czarlewski W, Anto J, Martineau A, Haahtela T, et al. Nrf2-interacting nutrients and COVID-19: time for research to develop adaptation strategies. Clin Transl Allergy. (2020) 10:58. doi: 10.1186/s13601-020-00362-7
76. Iddir M, Brito A, Dingeo G, Fernandez Del Campo S, Samouda H, La Frano M, et al. Strengthening the immune system and reducing inflammation and oxidative stress through diet and nutrition: considerations during the COVID-19 crisis. Nutrients. (2020) 12:1562. doi: 10.3390/nu12061562
77. Butler M, Barrientos R. The impact of nutrition on COVID-19 susceptibility and long-term consequences. Brain Behav Immun. (2020) 87:53–4. doi: 10.1016/j.bbi.2020.04.040
78. Boscolo-Rizzo P, Borsetto D, Spinato G, Fabbris C, Menegaldo A, Gaudioso P, et al. New onset of loss of smell or taste in household contacts of home-isolated SARS-CoV-2-positive subjects. Eur Arch Otorhinolaryngol. (2020) 277:2637–40. doi: 10.1007/s00405-020-06066-9
79. Ammar A, Brach M, Trabelsi K, Chtourou H, Boukhris O, Masmoudi L, et al. Effects of COVID-19 home confinement on eating behaviour and physical activity: results of the ECLB-COVID19 international online survey. Nutrients. (2020) 12:1583. doi: 10.3390/nu12061583
80. Bhutani S, vanDellen M, Cooper J. Longitudinal weight gain and related risk behaviors during the covid-19 pandemic in adults in the us. Nutrients. (2021) 13:671. doi: 10.3390/nu13020671
81. Ray JL, Srinath R, Mechanick JI. The negative impact of routine, dietary pattern, and physical activity on obesity and dysglycemia during the COVID-19 pandemic. Am J Lifestyle Med. (2022) 21:15598276221084923. doi: 10.1177/15598276221084923
82. Marasco G, Cremon C, Barbaro M, Salvi D, Cacciari G, Kagramanova A, et al. Prevalence of gastrointestinal symptoms in severe acute respiratory syndrome coronavirus 2 infection: results of the prospective controlled multinational GI-COVID-19 Study. Am J Gastroenterol. (2022) 117:147–57. doi: 10.14309/ajg.0000000000001541
83. Schulman R, Mechanick J. Metabolic and nutrition support in the chronic critical illness syndrome. Respir Care. (2012) 57:958–77; discussion977–8. doi: 10.4187/respcare.01620
84. Bear D, Merriweather J. Nutrition in postacute rehabilitation of COVID-19 survivors. Curr Opin Clin Nutr Metab Care. (2022) 25:154–8. doi: 10.1097/MCO.0000000000000819
85. Cava E, Carbone S. Coronavirus disease 2019 pandemic and alterations of body composition. Curr Opin Clin Nutr Metab Care. (2021) 24:229–35. doi: 10.1097/MCO.0000000000000740
86. Quilliot D, Gérard M, Bonsack O, Malgras A, Vaillant M, Di Patrizio P, et al. Impact of severe SARS-CoV-2 infection on nutritional status and subjective functional loss in a prospective cohort of COVID-19 survivors. BMJ Open. (2021) 11:e048948. doi: 10.1136/bmjopen-2021-048948
87. Galmés S, Serra F, Palou A. Current state of evidence: influence of nutritional and nutrigenetic factors on immunity in the COVID-19 pandemic framework. Nutrients. (2020) 12:2738. doi: 10.3390/nu12092738
88. Nieto-Martínez R, González-Rivas J, Mechanick J. Cardiometabolic risk: new chronic care models. JPEN J Parenter Enteral Nutr. (2021) 45:85–92. doi: 10.1002/jpen.2264
89. Vural Keskinler M, FeyÝzoglu G, Yildiz K, Oguz A. The frequency of malnutrition in patients with type 2 diabetes. Medeni Med J. (2021) 36:117–22. doi: 10.5222/MMJ.2021.44270
90. Sanz París A, García J, Gómez-Candela C, Burgos R, Martín Á, Matía P, et al. Malnutrition prevalence in hospitalized elderly diabetic patients. Nutr Hosp. (2013) 28:592–9. doi: 10.3305/nh.2013.28.3.6472
91. Kuyumcu ME, Yeşil Y, Oztürk ZA, Halil M, Ulger Z, Yavuz BB, et al. Challenges in nutritional evaluation of hospitalized elderly; always with mini-nutritional assessment? Eur Geriatr Med. (2013) 4:231–6.
92. Saka B, Kaya O, Ozturk G, Erten N, Karan M. Malnutrition in the elderly and its relationship with other geriatric syndromes. Clin Nutr. (2010) 29:745–8. doi: 10.1016/j.clnu.2010.04.006
93. Vischer U, Perrenoud L, Genet C, Ardigo S, Registe-Rameau Y, Herrmann F. The high prevalence of malnutrition in elderly diabetic patients: implications for anti-diabetic drug treatments. Diabet Med. (2010) 27:918–24. doi: 10.1111/j.1464-5491.2010.03047.x
94. Ahmed N, Choe Y, Mustad V, Chakraborty S, Goates S, Luo M, et al. Impact of malnutrition on survival and healthcare utilization in Medicare beneficiaries with diabetes: a retrospective cohort analysis. BMJ Open Diabetes Res Care. (2018) 6:e000471. doi: 10.1136/bmjdrc-2017-000471
95. Stangl M, Böcker W, Chubanov V, Ferrari U, Fischereder M, Gudermann T, et al. Sarcopenia–endocrinological and neurological aspects. Exp Clin Endocrinol Diabetes. (2019) 127:8–22. doi: 10.1055/a-0672-1007
96. Mu Z, Fu J, Sun L, Chan P, Xiu S. Associations between homocysteine, inflammatory cytokines and sarcopenia in Chinese older adults with type 2 diabetes. BMC Geriatr. (2021) 21:692. doi: 10.1186/s12877-021-02622-y
97. Liccini A, Malmstrom T. Frailty and sarcopenia as predictors of adverse health outcomes in persons with diabetes mellitus. J Am Med Dir Assoc. (2016) 17:846–51. doi: 10.1016/j.jamda.2016.07.007
98. Venturelli M, Reggiani C, Schena F. Beyond the current knowledge on sarcopenia: new insight on neuromuscular factors. Aging Clin Exp Res. (2022) 34:1183–5. doi: 10.1007/s40520-022-02082-3
99. Mesinovic J, Zengin A, De Courten B, Ebeling P, Scott D. Sarcopenia and type 2 diabetes mellitus: a bidirectional relationship. Diabetes Metab Syndr Obes. (2019) 12:1057–72. doi: 10.2147/DMSO.S186600
100. Qiao Y, Chai Y, Gong H, Zhuldyz Z, Stehouwer C, Zhou J, et al. The association between diabetes mellitus and risk of sarcopenia: accumulated evidences from observational studies. Front Endocrinol (Lausanne). (2021) 12:782391. doi: 10.3389/fendo.2021.782391
101. Izzo A, Massimino E, Riccardi G, Della Pepa G. A narrative review on sarcopenia in type 2 diabetes mellitus: prevalence and associated factors. Nutrients. (2021) 13:183. doi: 10.3390/nu13010183
102. Sousa-Victor P, Muñoz-Cánoves P. Regenerative decline of stem cells in sarcopenia. Mol Aspects Med. (2016) 50:109–17. doi: 10.1016/j.mam.2016.02.002
103. Gao Q, Hu K, Yan C, Zhao B, Mei F, Chen F, et al. Sarcopenia in community-dwelling older adults: a systematic review and meta-analysis. Nutrients. (2021) 13:4291. doi: 10.3390/nu13124291
104. Park S, Goodpaster B, Lee J, Kuller L, Boudreau R, de Rekeneire N, et al. Excessive loss of skeletal muscle mass in older adults with type 2 diabetes. Diabetes Care. (2009) 32:1993–7. doi: 10.2337/dc09-0264
105. Filippin L, Teixeira V, da Silva M, Miraglia F, da Silva F. Sarcopenia: a predictor of mortality and the need for early diagnosis and intervention. Aging Clin Exp Res. (2015) 27:249–54. doi: 10.1007/s40520-014-0281-4
106. Okamura T, Hashimoto Y, Miki A, Kaji A, Sakai R, Iwai K, et al. Reduced dietary omega-3 fatty acids intake is associated with sarcopenia in elderly patients with type 2 diabetes: a cross-sectional study of KAMOGAWA-DM cohort study. J Clin Biochem Nutr. (2020) 66:233–7. doi: 10.3164/jcbn.19-85
107. Xie W, Xiao G, Fan Y, He M, Lv S, Li Y. Sarcopenic obesity: research advances in pathogenesis and diagnostic criteria. Aging Clin Exp Res. (2021) 33:247–52. doi: 10.1007/s40520-019-01435-9
108. Lee D, Shook R, Drenowatz C, Blair S. Physical activity and sarcopenic obesity: definition, assessment, prevalence and mechanism. Future Sci OA. (2016) 2:FSO127. doi: 10.4155/fsoa-2016-0028
109. Meng P, Hu Y, Fan L, Zhang Y, Zhang M, Sun J, et al. Sarcopenia and sarcopenic obesity among men aged 80 years and older in Beijing: prevalence and its association with functional performance. Geriatr Gerontol Int. (2014) 14(Suppl. 1):29–35. doi: 10.1111/ggi.12211
110. Hwang B, Lim J, Lee J, Choi N, Ahn Y, Park B. Prevalence rate and associated factors of sarcopenic obesity in Korean elderly population. J Korean Med Sci. (2012) 27:748–55. doi: 10.3346/jkms.2012.27.7.748
111. Kim Y, Lee Y, Chung Y, Lee D, Joo N, Hong D, et al. Prevalence of sarcopenia and sarcopenic obesity in the Korean population based on the fourth Korean national health and nutritional examination surveys. J Gerontol A Biol Sci Med Sci. (2012) 67:1107–13. doi: 10.1093/gerona/gls071
112. Muñoz-Arribas A, Mata E, Pedrero-Chamizo R, Espino L, Gusi N, Villa G, et al. Obesidad sarcopénica y condición física en octogenarios; proyecto multi-céntrico EXERNET [Sarcopenic obesity and physical fitness in octogenarians: the multi-center EXERNET Project]. Nutr Hosp. (2013) 28:1877–83.
113. Norman K, Haß U, Pirlich M. Malnutrition in older adults – recent advances and remaining challenges. Nutrients. (2021) 13:2764. doi: 10.3390/nu13082764
114. Omura T, Araki A. Skeletal muscle as a treatment target for older adults with diabetes mellitus: the importance of a multimodal intervention based on functional category. Geriatr Gerontol Int. (2022) 22:110–20. doi: 10.1111/ggi.14339
115. Silverio R, Gonçalves D, Andrade M, Seelaender M. Coronavirus disease 2019 (COVID-19) and nutritional status: the missing link? Adv Nutr. (2021) 12:682–92. doi: 10.1093/advances/nmaa125
116. Guan W, Ni Z, Hu Y, Liang W, Ou C, He J, et al. China medical treatment expert group for covid-19. Clinical characteristics of coronavirus disease 2019 in China. N Engl J Med. (2020) 382:1708–20. doi: 10.1056/NEJMoa2002032
117. Zhou F, Yu T, Du R, Fan G, Liu Y, Liu Z, et al. Clinical course and risk factors for mortality of adult inpatients with COVID-19 in Wuhan, China: a retrospective cohort study. Lancet. (2020) 395:1054–62. doi: 10.1016/S0140-6736(20)30566-3
118. Hasegawa Y, Takahashi F, Hashimoto Y, Munekawa C, Hosomi Y, Okamura T, et al. Effect of COVID-19 pandemic on the change in skeletal muscle mass in older patients with type 2 diabetes: a retrospective cohort study. Int J Environ Res Public Health. (2021) 18:4188. doi: 10.3390/ijerph18084188
119. Dhillon R, Hasni S. Pathogenesis and management of sarcopenia. Clin Geriatr Med. (2017) 33:17–26. doi: 10.1016/j.cger.2016.08.002
120. Barbiera A, Pelosi L, Sica G, Scicchitano B. Nutrition and microRNAs: novel insights to fight sarcopenia. Antioxidants (Basel). (2020) 9:951. doi: 10.3390/antiox9100951
121. Sebastián D, Sorianello E, Segalés J, Irazoki A, Ruiz-Bonilla V, Sala D, et al. Mfn2 deficiency links age-related sarcopenia and impaired autophagy to activation of an adaptive mitophagy pathway. EMBO J. (2016) 35:1677–93. doi: 10.15252/embj.201593084
122. Ryall J, Schertzer J, Lynch G. Cellular and molecular mechanisms underlying age-related skeletal muscle wasting and weakness. Biogerontology. (2008) 9:213–28. doi: 10.1007/s10522-008-9131-0
123. Faulkner J, Larkin L, Claflin D, Brooks S. Age-related changes in the structure and function of skeletal muscles. Clin Exp Pharmacol Physiol. (2007) 34:1091–6. doi: 10.1111/j.1440-1681.2007.04752.x
124. Yang Q, Chan P. Skeletal muscle metabolic alternation develops sarcopenia. Aging Dis. (2022) 13:801–14. doi: 10.14336/AD.2021.1107
125. Rong S, Wang L, Peng Z, Liao Y, Li D, Yang X, et al. he mechanisms and treatments for sarcopenia: could exosomes be a perspective research strategy in the future? J Cachexia Sarcopenia Muscle. (2020) 11:348–65. doi: 10.1002/jcsm.12536
126. Elliott J, Greising S, Mantilla C, Sieck G. Functional impact of sarcopenia in respiratory muscles. Respir Physiol Neurobiol. (2016) 226:137–46. doi: 10.1016/j.resp.2015.10.001
127. Argyropoulou D, Geladas N, Nomikos T, Paschalis V. Exercise and nutrition strategies for combating sarcopenia and type 2 diabetes mellitus in older adults. J Funct Morphol Kinesiol. (2022) 7:48. doi: 10.3390/jfmk7020048
128. Romani M, Berger M, D’Amelio P. From the bench to the bedside: branched amino acid and micronutrient strategies to improve mitochondrial dysfunction leading to sarcopenia. Nutrients. (2022) 14:483. doi: 10.3390/nu14030483
129. Sha T, Li W, He H, Wu J, Wang Y, Li H. Causal relationship of genetically predicted serum micronutrients levels with sarcopenia: a Mendelian randomization study. Front Nutr. (2022) 9:913155. doi: 10.3389/fnut.2022.913155
130. Cawood A, Walters E, Smith T, Sipaul R, Stratton RJ. A review of nutrition support guidelines for individuals with or recovering from COVID-19 in the community. Nutrients. (2020) 12:3230. doi: 10.3390/nu12113230
131. Martindale R, Patel J, Taylor B, Arabi Y, Warren M, McClave S. Nutrition therapy in critically ill patients with coronavirus disease 2019. JPEN J Parenter Enteral Nutr. (2020) 44:1174–84. doi: 10.1002/jpen.1930
132. Barazzoni R, Bischoff S, Breda J, Wickramasinghe K, Krznaric Z, Nitzan D, et al. ESPEN expert statements and practical guidance for nutritional management of individuals with SARS-CoV-2 infection. Clin Nutr. (2020) 39:1631–8. doi: 10.1016/j.clnu.2020.03.022
133. Noronha J, Mechanick J. Is there a role for diabetes-specific nutrition formulas as meal replacements in type 2 diabetes? Front Endocrinol (Lausanne). (2022) 13:874968. doi: 10.3389/fendo.2022.874968
134. Doola R, Deane A, Tolcher D, Presneill J, Barrett H, Forbes J, et al. The effect of a low carbohydrate formula on glycaemia in critically ill enterally-fed adult patients with hyperglycaemia: a blinded randomised feasibility trial. Clin Nutr ESPEN. (2019) 31:80–7. doi: 10.1016/j.clnesp.2019.02.013
135. Mesejo A, Montejo-González J, Vaquerizo-Alonso C, Lobo-Tamer G, Zabarte-Martinez M, Herrero-Meseguer J, et al. Diabetes-specific enteral nutrition formula in hyperglycemic, mechanically ventilated, critically ill patients: a prospective, open-label, blind-randomized, multicenter study. Crit Care. (2015) 19:390. doi: 10.1186/s13054-015-1108-1
136. Zhu B, Jin S, Wu L, Hu C, Wang Z, Bu L, et al. J-shaped association between fasting blood glucose levels and COVID-19 severity in patients without diabetes. Diab Res Clin Pract. (2020) 168:108381.
137. Zhu L, She ZG, Cheng X, Qin JJ, Zhang XJ, Cai J, et al. Association of blood glucose control and outcomes in patients with COVID-19 and pre-existing type 2 diabetes. Cell Metab. (2020) 31: 1068–1077.e3.
138. Deer R, Volpi E. Protein requirements in critically ill older adults. Nutrients. (2018) 10:378. doi: 10.3390/nu10030378
139. Soares M, Eggelbusch M, Naddaf E, Gerrits K, van der Schaaf M, van den Borst B, et al. Skeletal muscle alterations in patients with acute COVID-19 and post-acute sequelae of Covid-19. J Cachexia Sarcopenia Muscle. (2022) 13:11–22. doi: 10.1002/jcsm.12896
140. de Andrade-Junior M, de Salles I, de Brito C, Pastore-Junior L, Righetti R, Yamaguti W. Skeletal muscle wasting and function impairment in intensive care patients with severe COVID-19. Front Physiol. (2021) 12:640973. doi: 10.3389/fphys.2021.640973
141. Rousseau A, Minguet P, Colson C, Kellens I, Chaabane S, Delanaye P, et al. Post-intensive care syndrome after a critical COVID-19: cohort study from a Belgian follow-up clinic. Ann Intensive Care. (2021) 11:118. doi: 10.1186/s13613-021-00910-9
142. Grilli G, Giancaspro R, Del Colle A, Quarato C, Lacedonia D, Foschino Barbaro M, et al. Dysphagia in non-intubated patients affected by COVID-19 infection. Eur Arch Otorhinolaryngol. (2022) 279:507–13. doi: 10.1007/s00405-021-07062-3
143. Marchese M, Ausili Cefaro C, Mari G, Proietti I, Carfì A, Tosato M, et al. Oropharyngeal dysphagia after hospitalization for COVID-19 disease: our screening results. Dysphagia. (2022) 37:447–53. doi: 10.1007/s00455-021-10325-0
144. Taquet M, Dercon Q, Luciano S, Gettes J, Husain M, Harrison P. Incidence, co-occurrence, and evolution of long-COVID features: a 6-month retrospective cohort study of 273,618 survivors of COVID-19. PLoS Med. (2021) 18:e1003773. doi: 10.1371/journal.pmed.1003773
145. Cereda E, Clavé P, Collins P, Holdoway A, Wischmeyer P. Recovery-focused nutritional therapy across the continuum of care: learning from COVID-19. Nutrients. (2021) 13:3293. doi: 10.3390/nu13093293
146. Matia Martin P, Robles Agudo F, Lopez Medina J, Sanz Paris A, Tarazona Santabalbina F, Domenech Pascual J, et al. Effectiveness of an oral diabetes-specific supplement on nutritional status, metabolic control, quality or life, and functional status in elderly patients. A multicentre study. Clin Nutr. (2019) 38:1253–61. doi: 10.1016/j.clnu.2018.05.007
147. de Luis D, Izaola O, Aller R, Cuellar L, Terroba M, Martin T, et al. A randomized clinical trial with two enteral diabetes-specific supplements in patients with diabetes mellitus type 2: metabolic effects. Eur Rev Med Pharmacol Sci. (2008) 12:261–6.
148. Gentil P, de Lira C, Coswig V, Barroso W, Vitorino P, Ramirez-Campillo R, et al. Practical recommendations relevant to the use of resistance training for COVID-19 survivors. Front Physiol. (2021) 12:637590. doi: 10.3389/fphys.2021.637590
149. Ahmadi Hekmatikar A, Ferreira Júnior J, Shahrbanian S, Suzuki K. Functional and psychological changes after exercise training in post-COVID-19 patients discharged from the hospital: a PRISMA-compliant systematic review. Int J Environ Res Public Health. (2022) 19:2290. doi: 10.3390/ijerph19042290
150. Singer P, Blaser A, Berger M, Alhazzani W, Calder P, Casaer M, et al. ESPEN guideline on clinical nutrition in the intensive care unit. Clin Nutr. (2019) 38:48–79. doi: 10.1016/j.clnu.2018.08.037
151. Doaei S, Gholami S, Rastgoo S, Gholamalizadeh M, Bourbour F, Bagheri S, et al. The effect of omega-3 fatty acid supplementation on clinical and biochemical parameters of critically ill patients with COVID-19: a randomized clinical trial. J Transl Med. (2021) 19:128. doi: 10.1186/s12967-021-02795-5
152. Grant W, Lahore H, McDonnell S, Baggerly C, French C, Aliano J, et al. Evidence that vitamin D supplementation could reduce risk of influenza and COVID-19 infections and deaths. Nutrients. (2020) 12:988. doi: 10.3390/nu12040988
153. Sabico S, Enani M, Sheshah E, Aljohani N, Aldisi D, Alotaibi N, et al. Effects of a 2-week 5000 IU versus 1000 IU vitamin D3 supplementation on recovery of symptoms in patients with mild to moderate COVID-19: a randomized clinical trial. Nutrients. (2021) 13:2170. doi: 10.3390/nu13072170
154. Caballero-García A, Pérez-Valdecantos D, Guallar P, Caballero-Castillo A, Roche E, Noriega D, et al. Effect of vitamin D supplementation on muscle status in old patients recovering from COVID-19 infection. Medicina (Kaunas). (2021) 57:1079. doi: 10.3390/medicina57101079
155. Notz Q, Herrmann J, Schlesinger T, Kranke P, Sitter M, Helmer P, et al. Vitamin D deficiency in critically ill COVID-19 ARDS patients. Clin Nutr. (2021). doi: 10.1016/j.clnu.2021.03.001 [Epub ahead of print].
156. Ferrara F, De Rosa F, Vitiello A. The central role of clinical nutrition in COVID-19 patients during and after hospitalization in intensive care unit. SN Compr Clin Med. (2020) 2:1064–8. doi: 10.1007/s42399-020-00410-0
157. Suliman S, McClave S, Taylor B, Patel J, Omer E, Martindale R. Barriers to nutrition therapy in the critically ill patient with COVID-19. JPEN J Parenter Enteral Nutr. (2022) 46:805–16. doi: 10.1002/jpen.2263
158. Ho D, Nguyen H, Irnandi D, Faradina A, Dang T, Wiratama B, et al. Adherence to COVID-19 nutritional guidelines and their impact on the clinical outcomes of hospitalized COVID-19 patients. Clin Nutr ESPEN. (2021) 46:491–8. doi: 10.1016/j.clnesp.2021.09.003
159. Faradina A, Tseng S, Ho D, Nurwanti E, Hadi H, Purnamasari S, et al. Adherence to COVID-19 nutrition guidelines is associated with better nutritional management behaviors of hospitalized COVID-19 patients. Nutrients. (2021) 13:1918. doi: 10.3390/nu13061918
160. Singhai K, Swami M, Nebhinani N, Rastogi A, Jude E. Psychological adaptive difficulties and their management during COVID-19 pandemic in people with diabetes mellitus. Diabetes Metab Syndr. (2020) 14:1603–5. doi: 10.1016/j.dsx.2020.08.025
161. Grabowski D, Overgaard M, Meldgaard J, Johanson L, Willaing I. Disrupted self-management and adaption to new diabetes routines: a qualitative study of how people with diabetes managed their illness during the COVID-19 lockdown. Diabetol. (2021) 2:1–15.
162. Eberle C, Stichling S. Impact of COVID-19 lockdown on glycemic control in patients with type 1 and type 2 diabetes mellitus: a systematic review. Diabetol Metab Syndr. (2021) 13:95. doi: 10.1186/s13098-021-00705-9
163. Czeisler M, Marynak K, Clarke K, Salah Z, Shakya I, Thierry J, et al. Delay or avoidance of medical care because of COVID-19-related concerns - united states, june 2020. MMWR Morb Mortal Wkly Rep. (2020) 69:1250–7. doi: 10.15585/mmwr.mm6936a4
164. Zhu S, Meehan T, Veerasingham M, Sivanesan K. COVID-19 pandemic gestational diabetes screening guidelines: a retrospective study in Australian women. Diabetes Metab Syndr. (2021) 15:391–5. doi: 10.1016/j.dsx.2021.01.021
165. Holland D, Heald A, Stedman M, Hanna F, Wu P, Duff C, et al. Assessment of the effect of the COVID-19 pandemic on UK HbA1c testing: implications for diabetes management and diagnosis. J Clin Pathol. (2021):jclinpath-2021-207776. doi: 10.1136/jclinpath-2021-207776 [Epub ahead of print].
166. Carr M, Wright A, Leelarathna L, Thabit H, Milne N, Kanumilli N, et al. Impact of COVID-19 restrictions on diabetes health checks and prescribing for people with type 2 diabetes: a UK-wide cohort study involving 618 161 people in primary care. BMJ Qual Saf. (2022) 31:503–14. doi: 10.1136/bmjqs-2021-013613
167. Caruso P, Longo M, Signoriello S, Gicchino M, Maiorino M, Bellastella G, et al. Diabetic foot problems during the COVID-19 pandemic in a tertiary care center: the emergency among the emergencies. Diabetes Care. (2020) 43:e123–4. doi: 10.2337/dc20-1347
168. Evert A, Dennison M, Gardner C, Garvey W, Lau K, MacLeod J, et al. Nutrition therapy for adults with diabetes or prediabetes: a consensus report. Diabetes Care. (2019) 42:731–54. doi: 10.2337/dci19-0014
169. Rodriguez-Blazquez C, João Forjaz M, Gimeno-Miguel A, Bliek-Bueno K, Poblador-Plou B, Pilar Luengo-Broto S, et al. Assessing the pilot implementation of the Integrated multimorbidity care model in five European settings: results from the joint action CHRODIS-PLUS. Int J Environ Res Public Health. (2020) 17:5268. doi: 10.3390/ijerph17155268
170. Merino J, Joshi A, Nguyen L, Leeming E, Mazidi M, Drew D, et al. Diet quality and risk and severity of COVID-19: a prospective cohort study. Gut. (2021) 70:2096–104. doi: 10.1136/gutjnl-2021-325353
171. Churuangsuk C, Hall J, Reynolds A, Griffin S, Combet E, Lean M. Diets for weight management in adults with type 2 diabetes: an umbrella review of published meta-analyses and systematic review of trials of diets for diabetes remission. Diabetologia. (2022) 65:14–36. doi: 10.1007/s00125-021-05577-2
172. Mechanick J, Marchetti A, Hegazi R, Hamdy O. Diabetes-specific nutrition formulas in the management of patients with diabetes and cardiometabolic risk. Nutrients. (2020) 12:3616. doi: 10.3390/nu12123616
173. Koonin L, Hoots B, Tsang C, Leroy Z, Farris K, Jolly T, et al. Trends in the use of telehealth during the emergence of the COVID-19 pandemic - united states, january-march 2020. MMWR Morb Mortal Wkly Rep. (2020) 69:1595–9. doi: 10.15585/mmwr.mm6943a3
174. Pendrith C, Nayyar D, Chu C, O’Brien T, Lyons O, Agarwal P, et al. Outpatient visit trends for internal medicine ambulatory care sensitive conditions after the COVID-19 pandemic: a time-series analysis. BMC Health Serv Res. (2022) 22:198. doi: 10.1186/s12913-022-07566-6
175. Maiorino M, Signoriello S, Maio A, Chiodini P, Bellastella G, Scappaticcio L, et al. Effects of continuous glucose monitoring on metrics of glycemic control in diabetes: a systematic review with meta-analysis of randomized controlled trials. Diabetes Care. (2020) 43:1146–56. doi: 10.2337/dc19-1459
176. Taylor P, Thompson C, Brinkworth G. Effectiveness and acceptability of continuous glucose monitoring for type 2 diabetes management: a narrative review. J Diabetes Investig. (2018) 9:713–25. doi: 10.1111/jdi.12807
177. Huang L, Yan Z, Huang H. The effect of short message service intervention on glycemic control in diabetes: a systematic review and meta-analysis. Postgrad Med. (2019) 131:566–71. doi: 10.1080/00325481.2019.1668723
178. Sahin C, Courtney K, Naylor P, E Rhodes R. Tailored mobile text messaging interventions targeting type 2 diabetes self-management: a systematic review and a meta-analysis. Digit Health. (2019) 5:2055207619845279. doi: 10.1177/2055207619845279
179. Yammine K, Estephan M. Telemedicine and diabetic foot ulcer outcomes. a meta-analysis of controlled trials. Foot (Edinb). (2022) 50:101872. doi: 10.1016/j.foot.2021.101872
180. Knox E, Quirk H, Glazebrook C, Randell T, Blake H. Impact of technology-based interventions for children and young people with type 1 diabetes on key diabetes self-management behaviours and prerequisites: a systematic review. BMC Endocr Disord. (2019) 19:7. doi: 10.1186/s12902-018-0331-6
Keywords: cardiometabolic, cardiometabolic-based chronic disease, coronavirus, COVID-19, COVID-related cardiometabolic syndrome, malnutrition, SARS-CoV-2, type 2 diabetes
Citation: Mechanick JI, Christofides EA, Marchetti AE, Hoddy KK, Joachim J, Hegazi R and Hamdy O (2023) The syndromic triad of COVID-19, type 2 diabetes, and malnutrition. Front. Nutr. 10:1122203. doi: 10.3389/fnut.2023.1122203
Received: 12 December 2022; Accepted: 30 January 2023;
Published: 21 February 2023.
Edited by:
Barbara Troesch, Self Employed, Zurich, SwitzerlandReviewed by:
Sousana Konstantinos Papadopoulou, International Hellenic University, GreeceXiangdong Jian, Qilu Hospital, Shandong University, China
Copyright © 2023 Mechanick, Christofides, Marchetti, Hoddy, Joachim, Hegazi and Hamdy. This is an open-access article distributed under the terms of the Creative Commons Attribution License (CC BY). The use, distribution or reproduction in other forums is permitted, provided the original author(s) and the copyright owner(s) are credited and that the original publication in this journal is cited, in accordance with accepted academic practice. No use, distribution or reproduction is permitted which does not comply with these terms.
*Correspondence: Albert E. Marchetti, albertmarchetti@yahoo.com