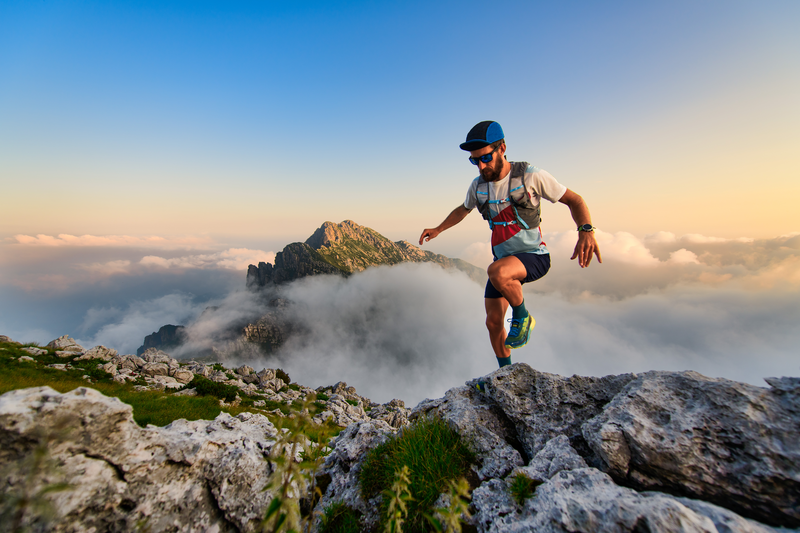
94% of researchers rate our articles as excellent or good
Learn more about the work of our research integrity team to safeguard the quality of each article we publish.
Find out more
ORIGINAL RESEARCH article
Front. Nutr. , 13 February 2023
Sec. Nutrition and Metabolism
Volume 10 - 2023 | https://doi.org/10.3389/fnut.2023.1113789
This article is part of the Research Topic Food-Derived Polyphenols: Functional Regulation in Chronic Diseases View all 7 articles
Background: Metabolic associated fatty liver disease (MAFLD) has become the most common liver disease globally, yet no new drugs have been approved for clinical treatment. Therefore, we investigated the relationship between dietary intake of soy-derived daidzein and MAFLD, to find potentially effective treatments.
Methods: We conducted a cross-sectional study using data from 1,476 participants in National Health and Nutrition Examination Survey (NHANES) from 2017 to 2018 and their associated daidzein intake from the flavonoid database in the USDA Food and Nutrient Database for Dietary Studies (FNDDS). We investigated the relationship between MAFLD status, controlled attenuation parameter (CAP), AST/Platelet Ratio Index (APRI), Fibrosis-4 Index (FIB-4), liver stiffness measurement (LSM), nonalcoholic fatty liver disease (NAFLD) fibrosis score (NFS), hepatic steatosis index (HSI), fatty liver index (FLI), and daidzein intake by adjusting for confounding variables using binary logistic regression models and linear regression models.
Results: In the multivariable-adjusted model II, there was a negative association between daidzein intake and the incidence of MAFLD (OR for Q4 versus Q1 was 0.65, 95% confidence interval [CI] = 0.46–0.91, p = 0.0114, p for trend was 0.0190). CAP was also negatively associated with daidzein intake, β = −0.37, 95% CI: −0.63 to −0.12, p = 0.0046 in model II after adjusting for age, sex, race, marital status, education level, family income-to-poverty ratio (PIR), smoking, and alcohol consumption. Stratified by quartiles of daidzein intake, trend analysis of the relationship between daidzein intake and CAP remained significant (p for trend = 0.0054). In addition, we also found that HSI, FLI, and NFS were negatively correlated with daidzein intake. LSM was negatively related to daidzein intake but had no statistical significance. The correlation between APRI, FIB-4, and daidzein intake was not strong (although p < 0.05, β values were all 0).
Conclusion: We found that MAFLD prevalence, CAP, HSI, and FLI, all decreased with increased daidzein intake, suggesting that daidzein intake may improve hepatic steatosis. Therefore, dietary patterns of soy food or supplement consumption may be a valuable strategy to reduce the disease burden and the prevalence of MAFLD.
Chronic liver disease is the leading cause of both morbidity and mortality worldwide, with 2 million deaths from the liver disease each year (1, 2). With the improvement of people’s living conditions, dietary patterns, and lifestyle changes, the incidence of non-alcoholic fatty liver disease (NAFLD) has been on the rise year by year and is now the fastest-growing cause of cirrhosis and liver cancer incidence and its associated mortality, resulting in a serious clinical and socio-economic burden (3–5). NAFLD has become the most prevalent chronic liver disease worldwide in the last two decades, and affects about a quarter of the world’s population (6). In addition to liver involvement, NAFLD is associated with an increased risk of diabetes, cardiovascular disease, cerebrovascular disease, chronic kidney disease, and extrahepatic tumors (7). In 2020, the international consensus proposed a new concept: metabolic associated fatty liver disease (MAFLD), which emphasized the role of metabolic disorders without excluding patients with an intake of alcohol or other chronic liver diseases and differed significantly from the diagnostic criteria for NAFLD (8, 9). In the real world, MAFLD diagnostic criteria are more practical than NAFLD diagnostic criteria for recognizing patients with a fatty liver at a high risk of progressive disease (10). The use of MAFLD criteria is more helpful in identifying and treating fatty liver patients at risk of hepatic fibrosis through non-invasive tests (11, 12). However, the treatment of NAFLD/MAFLD is limited to lifestyle changes, and there is a lack of clear and effective drugs (13).
Current studies have confirmed that the macronutrient and micronutrient composition of food can promote or prevent MAFLD (14–16). MAFLD is considered to be the liver manifestation of metabolic syndrome, and its occurrence is usually associated with chronic exposure to a nutrient-deficient diet (16). Wang et al. (17) showed that flavonoids can block oxidative stress by inhibiting CYP2E1 activity, thus improving insulin resistance, lipid peroxidation, and endoplasmic reticulum stress to prevent and treat NAFLD. Flavonoids are natural polyphenolic compounds that are widely found in plants and can be classified into several subtypes based on the degree of oxidation, mainly including isoflavones, flavonoids, flavanones, flavanols, flavonols, and flavan-3-ols (18). Among them, isoflavone compounds belong to phytoestrogens, mainly including daidzein, glycitein, genistein, biochanin A and formononetin (19). Equol is not a phytoestrogen, but as a metabolite of daidzein, it is sometimes included in the isoflavone class (20). The main dietary sources of human isoflavones are soybeans and soy products, containing daidzein and genistein (21). The chemical structure of daidzein is similar to that of mammalian estrogens and can act by replacing or blocking this hormone and its corresponding receptors, thus making daidzein a drug candidate with dual use (22). This makes daidzein a potential therapeutic option in estrogen-dependent diseases like prostate cancer, breast cancer, diabetes, and cardiovascular disease (23–25). Yamagata et al. (26) showed that daidzein had the potential to prevent metabolic syndrome by affecting hypertension, hyperglycemia, dyslipidemia, and atherosclerosis in patients. Another research surveyed the relationship between dietary isoflavone intake and risk of metabolic disorders in 6786 Chinese adults, which showed that total isoflavones, genistein, and daidzein intake were all negatively associated with NAFLD, hyperlipidemia, and hypertension (27). However, it is necessary for us to study the role of daidzein in MAFLD of different ethnicities. In this study, we prospectively surveyed the relationship between dietary daidzein intake and the incidence of MAFLD, hepatic steatosis, and hepatic fibrosis in participants in the National Health and Nutrition Examination Survey (NHANES) to find a potentially effective treatment for MAFLD.
The National Health and Nutrition Examination Survey (NHANES) is a continuous cross-sectional sample survey conducted since 1999 by the National Center for Health Statistics of the U.S. Centers for Disease Control and Prevention, every 2 years representing a survey cycle. The project is a complex, nationally representative, stratified, multi-stage probabilistic health survey of the non-institutionalized civilian population of the United States. The specific data collection procedures and detailed methodology for NHANES have been described by the National Center for Health Statistics (28). NHANES data are obtained from interviews, laboratory tests, and physical examinations conducted by trained staff. In our study, we specifically used data from one cycle of NHANES 2017–2018, which evaluated parameters of hepatic steatosis and fibrosis in patients using vibration-controlled transient elastography (VCTE) (29). Flavonoid intake data for patients in this study are derived from the 2017–2018 Food and Beverage Survey flavonoid values in the NHANES-associated USDA Food and Nutrient Database for Dietary Studies (FNDDS). There are more than 7,000 foods/beverages with flavonoid values in FNDDS 2017–2018, which can calculate the estimated value of flavonoid intake representing the United States population of all ages. Linking these estimates to laboratory data, physical examination, and interview data in NHANES can better investigate the relationship between flavonoid intake and human health (30). The data and documents used in this study are unidentified data frames that are publicly available on the National Center for Health Statistics (31) and Agricultural Research Service (30) websites. Ethics approval has been granted by the NCHS Ethics Review Committee, and protocol descriptions are available at.1 Written informed consent is required for participants 12 and over, and parental consent is also required for participants under 18 years old.
A total of 9,524 participants were recruited into the study during the 2017–2018 NHANES cycle. After excluding 2,853 participants who were not assessed for hepatic steatosis and liver fibrosis using VCTE at baseline, 453 participants who could not be diagnosed with MAFLD due to incomplete information, 499 participants with no daidzein value, and 3,973 participants with a daidzein value of 0, a total of 1,476 participants were finally enrolled in the analysis (Figure 1).
Figure 1. Flowchart of the selection process of the participants in NHANES 2017–2018. NHANES, National Health and Nutrition Examination Survey; MAFLD, metabolic associated fatty liver disease.
The flavonoid database in the FNDDS provides the total intake of 29 individual flavonoids, the total of the 6 major flavonoids, and the total intake of all flavonoids consumed by each participant on day 1 and day 2, respectively. Isoflavones are mainly sourced from soy foods/beverages such as soy milk, soy-based proteinaceous powder, and tofu, as well as isoflavone ingredients that are added to foods/beverages as additives to achieve specific functions. The 2017–2018 FNDDS nutrient values were calculated for each food/beverage based on the ingredient data in FoodData Central (32). In this study, we selected the mean of daidzein intake on days 1 and 2 for each participant in the flavonoid database to investigate its association with MAFLD incidence, hepatic steatosis, and hepatic fibrosis.
The diagnosis of MAFLD in this study was by the VCTE measurement of hepatic steatosis and the presence of one of the following three conditions, such as overweight/obesity, diabetes mellitus (DM), or evidence of metabolic abnormalities (9). Evidence of metabolic abnormalities was defined as at least 2 of the following metabolic risk abnormalities such as (1) waist circumference ≥102 and 88 cm in men and women, respectively, (2) blood pressure ≥ 130/85 mm Hg or treated with specific medications, (3) plasma triglycerides (TG) ≥1.70 mmol/l or treated with specific medications, (4) high-density lipoprotein cholesterol (HDL-C) <1.0 mmol/l in men and <1.3 mmol/l in women or treated with specific medications, (5) prodromal diabetes (fasting glucose) level 5.6–6.9 mmol/l or hemoglobin A1c 5.7–6.4%, (6) insulin resistance score ≥2.5 as assessed by the homeostatic model, (7) plasma high-sensitivity C-reactive protein level >2 mg/l.
During the 2017–2018 NHANES cycle, the technicians performed VCTE (Echosens, Paris, France) tests using the FibroScan 502 V2 Touch model after 2 days of professional training and authorization following the liver ultrasound transient elastography operation manual (33). Technicians obtained at least 10 measurements from participants who fasted for at least 3 h, yielding median controlled attenuation parameter (CAP) and liver stiffness measurement (LSM) values and interquartile spacing for each participant. The higher the CAP value measured, the higher the liver fat content (CAP reference range: 100–400 dB/m); similarly, the higher the LSM value, the more severe the hepatic fibrosis (LSM reference range: 1.5–75 kPa). In this study, we defined CAP ≥248 dB/m as hepatic steatosis according to the published data of a large meta-analysis (34) and the findings of a study of hepatic steatosis in an adolescent population (35). Eddowes et al. (36) showed cutoff values of 8.2 kPa, 9.7 kPa, and 13.6 kPa for F > F2, F > F3, and F=F4, respectively; therefore, we defined progressive hepatic fibrosis as LSM ≥ 9.7 KPa.
In the study, we also used serum-based scores for the assessment of non-invasive hepatic steatosis and liver fibrosis to investigate their relationship with daidzein intake. Liver steatosis score includes Fatty liver index (FLI) (FLI is calculated based on TG, gamma glutamyl transferase (GGT), body mass index (BMI), and waist circumference; FLI ≥ 60 is judged as hepatic steatosis) (37) and Hepatic steatosis index (HSI) (calculated based on alanine aminotransferase (ALT), aspartate aminotransferase (AST), BMI, diabetes mellitus (DM), gender; HSI > 36 is judged as NAFLD) (38). Hepatic fibrosis scores include AST to platelet ratio index (APRI) (results based on AST and platelet (PLT) count, APRI >1.5 indicates significant liver fibrosis) (39), Fibrosis-4 (FIB-4) (results based on ALT, AST, PLT and age, FIB-4 > 2.67 suggests that there are NAFLD patients with grade F3-4 or higher hepatic fibrosis) (40, 41) and NAFLD fibrosis score (NFS) (NFS calculated based on albumin, ALT, AST, PLT count, age, DM or impaired fasting blood glucose, and BMI, with NFS > 0.676 suggesting that NAFLD patients have progressive hepatic fibrosis) (42); the above scoring models of formulae for calculation are shown in Supplementary Table S1.
Demographic, physical examination, and laboratory test information in this study are available from the NHANES database, which mainly include age, sex, race, marital status, education level, economic status, BMI, smoking, drinking, homeostasis model assessment of insulin resistance (HOMA-IR), waist circumference and history of hypertension and diabetes. BMI = weight [kg]/height [m2], classified as <25, 25–30, and ≥30 (43); HOMA-IR is fasting insulin (μU/mL) × fasting glucose (mmol/L)/22.5 (44). Hypertension is defined as systolic blood pressure (SBP) ≥ 140 mmHg and diastolic blood pressure (DBP) ≥90 mmHg or the use of antihypertensive drugs. The diagnosis of diabetes mellitus is defined as a self-reported physician diagnosis of diabetes mellitus and/or fasting glucose ≥7.0 mmol/l or glycosylated hemoglobin (HbA1c) ≥6.5% and/or taking diabetes medications (45). We defined current smokers as individuals who have smoked more than 100 cigarettes in their lives and currently smoke on some days or every day; never smokers as people who have smoked less than 100 cigarettes in their lives; and former smokers as people who have smoked more than 100 cigarettes in their lives and now do not smoke at all. We assessed drinking status according to the volume and frequency of alcohol consumption in participants’ self-report questionnaires (46). The races are classified as non-Hispanic white, non-Hispanic black, Mexican-American, and other races. Marital status is divided into married/cohabiting and unmarried. Education level is classified as below high school, high school or the equivalent, and more than high school. Economic status is evaluated by the family income-to-poverty ratio (PIR), which is categorized as <1.0, 1.0–3.0, and >3.0.
Laboratory tests in this study included fasting glucose, fasting insulin, HbA1c, total bilirubin (TBIL), AST, ALT, GGT, alkaline phosphatase (ALP), albumin, creatinine, TG, uric acid, HDL-C, total cholesterol (TC), lactate dehydrogenase (LDH), high sensitivity-C-reactive protein (Hs-CRP), hemoglobin (Hb), white blood cell (WBC) counts, and red blood cell (RBC) count. All routine biochemical tests were performed according to NHANES laboratory/medical technician procedure manual standards (47).
All statistical analyses in this study were performed using R software (version 4.2.02) and Empower Stats software (3X&Y Solutions Inc.). The level of significance of the reported statistical results for all analyses was two-tailed, and p < 0.05 was considered statistically significant. Missing values in the study were processed by directly deleting the participant data if the exposure factor daidzein intake or the outcome indicator MAFLD was missing. The missing values of other confounders are processed by random forest interpolation using the “missForest” R package. In the analysis of participant baseline characteristics, we divided the exposure factor daidzein intake into quartiles and compared statistical differences between quartile groups. We used weighted linear regression analysis to calculate p values for continuous variables, while p values for categorical variables were calculated using a weighted chi-square test. Generalized additive models (GAM) and smoothing curve fitting were used to study whether the exposure variables were nonlinearly related to the outcome variables. The inflection points of the smoothed curves were analyzed by saturation and threshold effects, and the inflection points were calculated by two-stage linear regression analysis. We investigated the relationship between MAFLD status and daidzein intake by multivariate binary logistic regression models, and the relationship between APRI, CAP, LSM, FIB-4, NFS, FLI, HSI, and daidzein intake were assessed, respectively, using multivariate linear regression models. In this study, we constructed three models: crude model: no adjustment for any covariates; model I: adjusted for age, sex, and race; model II: adjusted for covariates of age, sex, race, marital status, education level, PIR, smoking, and alcohol consumption. In addition, we conducted subgroup analyses stratified by variables of interest and used the “forestlater” R package to draw forest plots to show the results of the subgroup analyses.
A total of 1,476 participants were included in this study, with a weighted mean age of 45.24 ± 18.28 years and a weighted ratio of 50% for both males and females. The median level of daidzein intake in our study was 0.18 mg (interquartile range [IQR], 0.02–1.55 mg). Table 1 described the baseline characteristics of participants by daidzein level quartiles. Laboratory findings of participants by quartiles of daidzein intake are shown in Supplementary Table S2. The findings suggest that participants in the third quartile may be younger compared with those in the first quartile of daidzein intake levels (49.00 ± 17.79 years vs. 41.49 ± 19.00 years) and that the daidzein diet intake population concentrated between the ages of 20–65 years. The proportion of daidzein intake was significantly higher among non-Hispanic whites, those with a high school degree or more, those married/cohabiting, and those with higher household income (PIR > 3, i.e., household income above three times the poverty line) than among other populations [e.g., other Hispanics, those below high school level, those unmarried, and those with lower household income (PIR < 1)]. In addition, the proportion of daidzein intake was significantly higher in participants without pre-diabetes, diabetes, and hypertension compared to those with underlying conditions such as these conditions.
Table 2 showed the relationship between MAFLD incidence and daidzein intake evaluated by three univariate and multivariate binary logistic regression models. In the crude model, there was a negative linear correlation between daidzein intake and MAFLD incidence (OR for Q4 versus Q1 was 0.63, 95% CI: 0.46–0.86, p for trend was 0.0024). Multivariate adjusted model II also revealed a significant negative association between daidzein intake and the incidence of MAFLD with an OR of 0.98 (0.97, 0.99) and a value of p of 0.0039. Similarly, participants in the highest quartile had an OR of 0.65, 95% CI: 0.46–0.86, compared to those in the lowest quartile of daidzein intake, and statistical significance remained. In addition, we observed a dose–response relationship between daidzein intake and MAFLD incidence, and MAFLD incidence decreased with increasing daidzein intake independent of gender (Figures 2A, 3A; Table 3).
Table 2. Relationship between MAFLD prevalence, APRI, CAP, LSM, FIB-4, NFS, FLI, HSI and daidzein intake in a multiple regression model.
Figure 2. The relationship between daidzein intake and MAFLD prevalence, CAP, LSM, HSI, FLI, NFS, FIB-4, and APRI, respectively. The generalized additive model (GAM) was used to assess the relationship between daidzein intake and MAFLD prevalence (A), CAP (B), LSM (C), HSI (D), FLI (E), NFS (F), FIB-4 (G), and APRI (H), respectively. The red dashed line represents the smoothed curve fit between the variables. The blue dashed line represents the 95% confidence interval of the fit. Age, sex, and race/ethnicity were adjusted in the model. MAFLD, metabolic associated fatty liver disease; CAP, controlled attenuation parameter; LSM, liver stiffness measurement; HSI, hepatic steatosis index; FLI, fatty liver index; FIB-4, fibrosis-4; NFS, NAFLD fibrosis score; APRI, AST to platelet ratio index.
Figure 3. The relationship between daidzein intake and MAFLD prevalence, CAP, LSM, HSI, FLI, NFS, FIB-4, and APRI stratified by gender, respectively. The relationship between daidzein intake and MAFLD prevalence (A), CAP (B), LSM (C), HSI (D), FLI (E), NFS (F), FIB-4 (G), and APRI (H) stratified by gender, respectively. Age and race/ethnicity were adjusted in the model. MAFLD, metabolic associated fatty liver disease; CAP, controlled attenuation parameter; LSM, liver stiffness measurement; HSI, hepatic steatosis index; FLI, fatty liver index; FIB-4, fibrosis-4; NFS, NAFLD fibrosis score; APRI, AST to platelet ratio index.
Table 3. Threshold effect analysis of daidzein intake using two-segment linear regression models for MAFLD prevalence, APRI, CAP, LSM, FIB-4, NFS, FLI, and HSI, respectively.
Table 2 also showed the relationship between CAP, APRI, LSM, FIB-4, NFS, FLI, HSI, and daidzein intake by three different linear regression models. We found that CAP was negatively related to daidzein intake in the crude model. Similar results were present in the model I (after adjusting for age, sex, and race, β = −0.40, 95% CI: −0.66 to −0.14, p = 0.0024) and model II (after adjusting for age, sex, race, education level, marital status, PIR, smoking, and alcohol use, β = −0.37, 95% CI: −0.63 to −0.12, p = 0.0046). Stratified by quartiles of daidzein intake levels, trend analysis of the association between daidzein intake and CAP remained significant (p for trend = 0.0054). We also found a negative correlation between HSI, FLI, NFS, and daidzein intake, and the detailed results were shown in Table 2. In model I, the β values between daidzein intake and HSI, FLI, and NFS were −0.06 (95% CI: −0.10 to −0.03, p = 0.0012), −0.24 (95% CI: −0.37 to −0.11, p = 0.0003), −0.01 (95% CI: −0.01 to 0.00, p = 0.0005), respectively. In model II, the β values between daidzein intake and HSI, FLI, and NFS were −0.06 (95% CI: −0.10 to −0.02, p = 0.0027), −0.22 (95% CI: −0.35 to −0.09, p = 0.0008), −0.01 (95% CI, −0.01to 0.00, p = 0.0006), respectively. LSM was negatively associated with daidzein intake, but p > 0.05, with no significant statistical differences. The correlation between APRI, FIB-4, and daidzein intake was not strong (although p < 0.05, all β values were 0).
In Figures 2, 3 and Table 3, we further assessed the dose–response relationships between each outcome variable and daidzein intake by generalized additive modeling and smoothed curve fitting. With this dose–response relationship, we also used a log-likelihood ratio-based test to assess the presence of a saturation threshold effect and used a two-step recursive method to determine the inflection point of the smoothing curve. In Table 3, if the log-likelihood ratio test p > 0.05, it indicates a linear correlation between daidzein intake and the outcome variable, and the curve inflection point is not significant, referring to the results of Model I. If p < 0.05, it indicates a curvilinear relationship between daidzein intake and the outcome variable, and the inflection point is significantly present, referring to the results of Model II. Therefore, Table 3 and Figure 2 show a linear relationship between MAFLD, CAP, LSM, FIB-4, NFS, FLI, HSI, and daidzein intake, except for APRI, where the presence of two inflection points is a non-linear relationship. In addition, we performed interaction tests for gender and found that the value of ps for all interactions after adjusting for variables were >0.05, which indicated that the relationship between daidzein intake and each outcome variable was not significantly dependent on gender (Figure 3; Table 3). Figure 4 further stratifies by age, sex, race, education level, marital status, PIR, alcohol use, and smoking to determine the independent relationship between MAFLD prevalence and daidzein intake. We observed that higher daidzein intake was related to lower MAFLD prevalence among those aged 20–65 years, married/cohabiting, with less than high school level, with PIR between 1 and 3, and who never smoked (Figure 4).
Figure 4. Subgroup analysis of the association between daidzein intake and the prevalence of MAFLD plotted in (A) and (B). MAFLD, metabolic associated fatty liver disease; Q, quartile; PIR, family income-to-poverty ratio.
A previous study from China supported a negative association between daidzein intake in isoflavones and the incidence of NAFLD (27). However, a number of growing evidence has supported that MAFLD diagnostic criteria are more useful than NAFLD for recognizing patients with a fatty liver at high risk of progressive disease (10–12). It was also necessary to study the association of daidzein intake with MAFLD in different ethnic groups. We found a relatively high proportion of daidzein intake among non-Hispanic white participants who had a high school degree or higher, were married/cohabiting, had higher household income, and did not have underlying conditions such as prediabetes, diabetes, and hypertensive disease. We also observed that the incidence of MAFLD decreased with increasing levels of daidzein intake and was not affected by gender, and more specifically, it is likely that this relationship was more significant among those aged 20–65 years, married/cohabiting, with education below high school level, with a PIR between 1 and 3 and never having smoked. We also found that CAP, HSI, FLI, and NFS were negatively associated with daidzein intake, but the correlation between daidzein intake and LSM, APRI, and FIB-4 was not strong.
The previous 2001–2004 NHANES study showed that income and race were associated with differences in dietary intake, particularly among individuals from low-income households and non-Hispanic blacks, who consumed less fruits, vegetables, grains, legumes, dairy, etc. (48). Another study from 2011 to 2016 NHANES data demonstrated the association of flavonoid intake in the US population with higher socioeconomic status (49). Our findings were similar, revealing a higher daidzein intake among non-Hispanic white, higher household income participants. Daidzein is protective against certain diseases related to estrogen regulation like prostate cancer, breast cancer, diabetes, and cardiovascular diseases (23–25). In addition, studies have shown that daidzein has the potential to prevent metabolic syndromes such as cardiovascular disease and diabetes (26), which may well explain the lower risk of diabetes and hypertension in participants with a high percentage of daidzein intake in this study. In mice, daidzein may ameliorate insulin resistance in obesity via direct modulation of hepatic ab initio adipogenesis and insulin signaling, and alter adipocyte metabolism to indirectly control obesity and reduce NAFLD by regulating adipokine expression through PPAR γ (50, 51). Another study also showed that daidzein improved lipopolysaccharide (LPS)-induced damage to hepatocytes by inhibiting inflammation and oxidative stress in mice (52). The MAFLD definition emphasizes more metabolic disorders and hepatic steatosis. Thus, the above-mentioned basic research further explains the negative correlation between daidzein intake and the prevalence of MAFLD.
A prospective cohort study from Guangzhou, China, included 2,694 participants and assessed the association between dietary flavonoid intake and NAFLD status using a food frequency questionnaire with face-to-face interviews, and revealed that higher flavonoid intake was related to a lower risk of progression of NAFLD in an elderly Chinese population (53). Our study also showed that the higher the daidzein intake in the middle-aged and elderly population, the more significant the reduction in the incidence of MAFLD. Another study from Tehran showed that education level and marital status were related to cardiovascular risk factors and dietary intake (54), and married women in the Hong Kong research had significantly higher intakes of vegetables, soy products, and fish than single women (55). Smoking was also considered an independent risk factor for poor prognosis in various chronic liver diseases (56–58). The result of a recent nationally representative cohort study from Thailand showed that smoking also increases the risk of all-cause mortality in patients with NAFLD, and this association was more pronounced in women with NAFLD (59). These studies also explained well that increased daidzein intake in the nonsmoking population in our study reduced the occurrence of MAFLD.
The pathophysiology of NAFLD/MAFLD has evolved from a “first strike” characterized by increased hepatic fat to a “second strike” consisting of adipokines, inflammatory cytokines, oxidative stress, and mitochondrial dysfunction; and the “multiple strikes” hypothesis consisting of insulin resistance, inflammation, lipotoxicity, cytokine imbalance, innate immune activation and microbiota disorders in the background of genetic and environmental factors (60–62). However, the accumulation of hepatic fat caused by insulin resistance remains the first and central striking factor (63). The current diagnosis of MAFLD is the basis of the presence of hepatic steatosis, which can be assessed clinically by non-invasive (blood biomarkers and imaging) and invasive means (liver biopsy) (64). Liu et al. (65). evaluated the accuracy of five commonly non-invasive hepatic steatosis algorithms such as FLI, HSI, Visceral Adiposity Index (VAI), NAFLD-liver fat score (NAFLD-LFS), and Steato text (ST) in the diagnosis of MAFLD using NHANES III, and the result showed that FLI had the highest diagnostic performance in the diagnosis of MAFLD. In this study, we also used three non-invasive liver fat assessment methods such as FLI, HSI, and CAP assess the relationship between daidzein intake and MAFLD, and the results showed that FLI, HSI, and CAP were negatively associated with daidzein intake, implying that daidzein may improve hepatic steatosis.
Given the current state of treatment for NAFLD/MAFLD, daidzein may be a potential therapeutic option. Recent research has demonstrated that soy protein concentrates and related isoflavones play an important role in lowering blood lipids, reducing hepatic steatosis, and improving the symptoms of NAFLD (66, 67). A cross-section study of 17,685 US adults also showed a negative association between the consumption of flavonoids and FLI (68). A randomized controlled trial from Iran found that 8 weeks of soy milk consumption and a low-calorie diet showed significant improvements in blood pressure and insulin resistance-related indicators in patients with NAFLD (69). Canadian food guidelines also recommend that the diet of soy foods or supplements may be a beneficial strategy to reduce the burden of disease and prevalence of MAFLD (70).
NAFLD can develop from simple steatosis to NASH, progressive hepatic fibrosis, cirrhosis, hepatocellular carcinoma, and liver failure (71, 72). NAFLD is related to increased mortality from hepatic and cardiovascular events, in which advanced fibrosis is considered an essential predictor of prognosis in NAFLD patients (72, 73). Liver biopsy is the golden standard for diagnosis of NAFLD fibrosis but is limited by the invasive nature of liver biopsy. Therefore, non-invasive assessments such as VCTE, point shear wave elastography (pSWE), magnetic resonance elastography (MRE), FIB-4, NFS, APRI, and other tests are used for screening fibrosis in NAFLD (74). In this study, we assessed the relationship between daidzein intake and hepatic fibrosis using four methods, including FIB-4, NFS, APRI, and LSM, and the findings revealed a negative correlation between NFS and daidzein intake levels, but no strong between daidzein intake and LSM, APRI, and FIB-4. There is insufficient evidence for the ability of daidzein to alleviate hepatic fibrosis. A previous study reported a significant inhibitory effect of high-dose soy isoflavones on thioacetamide-induced hepatic fibrosis in rats, which may be related to the inhibition of hepatic stellate cell activation and proliferation (75). The future may require us to investigate the association between daidzein intake and MAFLD-associated hepatic fibrosis in greater depth.
The advantages of our study are the use of representative national NHANES data, the multi-stage stratified sampling and the collection of relevant information by trained technical staff, the relatively large size of the sample included studies and the adjustment for multiple potential confounders to improve the reliability of the findings. However, our study also has some limitations. First, the cross-sectional study design of the study could not conclude whether there was a causal association between daidzein intake and MAFLD; therefore, further validation in a prospective cohort study is necessary. Second, the diagnosis of MAFLD in this study was defined as hepatic steatosis based on a CAP ≥248 dB/m measured by VCTE rather than by liver biopsy, which inevitably leads to a biased diagnosis of MAFLD. Also, non-invasive assessment methods do not perfectly predict the presence of hepatic steatosis and fibrosis in liver biopsies. Third, in our study, we tried to incorporate as many confounding factors as possible that were relevant to the study results, but we still could not completely rule out the possibilities of other confounding factors causing bias in the conclusions. Finally, due to limitations in the design of the USDA Food and Nutrient Dietary Study Database (FNDDS), it does not have data addressing the relationship between daidzein intake and calories, fat, protein, and dietary fiber. Therefore, we also do not know whether the daidzein intake is associated with a reduced intake of calories, fat, and protein. We will further investigate the relationship between daidzein intake and energy, lipid and protein metabolism, and dietary fiber, to elaborate more deeply on the relationship between daidzein intake and MAFLD.
In conclusion, we found that the prevalence of MAFLD decreased with increasing daidzein intake and that CAP, HSI, and FLI were negatively correlated with daidzein intake, suggesting that daidzein intake may have improved hepatic steatosis. Therefore, dietary patterns of soy food or supplement consumption may be a beneficial strategy to reduce the disease burden and prevalence of MAFLD. However, the correlation between daidzein intake and hepatic fibrosis indicators such as LSM, APRI, and FIB-4 was not strong, and we may need to investigate the association between daidzein intake and MAFLD-related hepatic fibrosis more deeply in the future.
The raw data supporting the conclusions of this article will be made available by the authors, without undue reservation.
ZY, YS, and QH conceptualized the study idea and conducted the interpretation, manuscript writing, and final approval. DG, XH, and FH performed the data analysis and collection, as well as the linguistic polishing. All authors contributed to the article and approved the submitted version.
This work was supported by Jingzhou Science and Technology Bureau Plan Project (2021CC28-04 to ZY) and the Natural Science Foundation of Hubei Province (2019CFB567 to YS).
We are very grateful to the NHANES working group and all participants for their valuable contributions to this study.
The authors declare that the research was conducted in the absence of any commercial or financial relationships that could be construed as a potential conflict of interest.
All claims expressed in this article are solely those of the authors and do not necessarily represent those of their affiliated organizations, or those of the publisher, the editors and the reviewers. Any product that may be evaluated in this article, or claim that may be made by its manufacturer, is not guaranteed or endorsed by the publisher.
The Supplementary material for this article can be found online at: https://www.frontiersin.org/articles/10.3389/fnut.2023.1113789/full#supplementary-material
1. Asrani, SK, Devarbhavi, H, Eaton, J, and Kamath, PS. Burden of liver diseases in the world. J Hepatol. (2019) 70:151–71. doi: 10.1016/j.jhep.2018.09.014
2. Younossi, Z, and Henry, L. Contribution of alcoholic and nonalcoholic fatty liver disease to the burden of liver-related morbidity and mortality. Gastroenterology. (2016) 150:1778–85. doi: 10.1053/j.gastro.2016.03.005
3. Younossi, Z, Anstee, QM, Marietti, M, Hardy, T, Henry, L, Eslam, M, et al. Global burden of NAFLD and Nash: trends, predictions, risk factors and prevention. Nat Rev Gastroenterol Hepatol. (2018) 15:11–20. doi: 10.1038/nrgastro.2017.109
4. Paik, JM, Golabi, P, Younossi, Y, Mishra, A, and Younossi, ZM. Changes in the global burden of chronic liver diseases from 2012 to 2017: the growing impact of NAFLD. Hepatology. (2020) 72:1605–16. doi: 10.1002/hep.31173
5. Sepanlou, S, Safiri, S, Bisignano, C, and Ikuta, K. The global, regional, and national burden of cirrhosis by cause in 195 countries and territories, 1990-2017: a systematic analysis for the global burden of disease study 2017. Lancet Gastroenterol Hepatol. (2020) 5:245–66. doi: 10.1016/S2468-1253(19)30349-8
6. Younossi, ZM, Koenig, AB, Abdelatif, D, Fazel, Y, Henry, L, and Wymer, M. Global epidemiology of nonalcoholic fatty liver disease-meta-analytic assessment of prevalence, incidence, and outcomes. Hepatology. (2016) 64:73–84. doi: 10.1002/hep.28431
7. Younossi, ZM, Stepanova, M, Lawitz, E, Charlton, M, Loomba, R, Myers, RP, et al. Improvement of hepatic fibrosis and patient-reported outcomes in non-alcoholic steatohepatitis treated with selonsertib. Liver Int. (2018) 38:1849–59. doi: 10.1111/liv.13706
8. Eslam, M, Sanyal, AJ, and George, J. MAFLD: a consensus-driven proposed nomenclature for metabolic associated fatty liver disease. Gastroenterology. (2020) 158:e1:1999–2014. doi: 10.1053/j.gastro.2019.11.312
9. Eslam, M, Newsome, PN, Sarin, SK, Anstee, QM, Targher, G, Romero-Gomez, M, et al. A new definition for metabolic dysfunction-associated fatty liver disease: an international expert consensus statement. J Hepatol. (2020) 73:202–9. doi: 10.1016/j.jhep.2020.03.039
10. Lin, S, Huang, J, Wang, M, Kumar, R, Liu, Y, Liu, S, et al. Comparison of MAFLD and NAFLD diagnostic criteria in real world. Liver Int. (2020) 40:2082–9. doi: 10.1111/liv.14548
11. van Kleef, LA, Ayada, I, Alferink, L, Pan, Q, and de Knegt, RJ. Metabolic dysfunction-associated fatty liver disease improves detection of high liver stiffness: the Rotterdam study. Hepatology. (2022) 75:419–29. doi: 10.1002/hep.32131
12. Yamamura, S, Eslam, M, Kawaguchi, T, Tsutsumi, T, Nakano, D, Yoshinaga, S, et al. MAFLD identifies patients with significant hepatic fibrosis better than NAFLD. Liver Int. (2020) 40:3018–30. doi: 10.1111/liv.14675
13. Younossi, Z, Tacke, F, Arrese, M, Chander, SB, Mostafa, I, Bugianesi, E, et al. Global perspectives on nonalcoholic fatty liver disease and nonalcoholic steatohepatitis. Hepatology. (2019) 69:2672–82. doi: 10.1002/hep.30251
14. Pickett-Blakely, O, Young, K, and Carr, RM. Micronutrients in nonalcoholic fatty liver disease pathogenesis. Cell Mol Gastroenterol Hepatol. (2018) 6:451–62. doi: 10.1016/j.jcmgh.2018.07.004
15. Berná, G, Álvarez-Amor, L, and Martín, F. Geometry of nutrition: nutrients and NAFLD progression In: NAFLD and NASH. Cham: Springer (2020). 49–67.
16. Gillespie, J. “You are what you eat”: the role of dietary macronutrients and micronutrients in MAFLD. Clin Liver Dis (Hoboken). (2021) 18:67–71. doi: 10.1002/cld.1083
17. Wang, K, Tan, W, Liu, X, Deng, L, Huang, L, Wang, X, et al. New insight and potential therapy for NAFLD: cyp2e1 and flavonoids. Biomed Pharmacother. (2021) 137:111326. doi: 10.1016/j.biopha.2021.111326
18. Peluso, I, Miglio, C, Morabito, G, Ioannone, F, and Serafini, M. Flavonoids and immune function in human: a systematic review. Crit Rev Food Sci Nutr. (2015) 55:383–95. doi: 10.1080/10408398.2012.656770
19. Krizova, L, Dadakova, K, Kasparovska, J, and Kasparovsky, T. Isoflavones. Molecules. (2019) 24:1076. doi: 10.3390/molecules24061076
20. Setchell, KD, Brown, NM, and Lydeking-Olsen, E. The clinical importance of the metabolite equol-a clue to the effectiveness of soy and its isoflavones. J Nutr. (2002) 132:3577–84. doi: 10.1093/jn/132.12.3577
21. Coward, L, Barnes, NC, Setchell, KD, and Barnes, S. Genistein, daidzein, and their. Beta.-glycoside conjugates: antitumor isoflavones in soybean foods from American and Asian diets. J Agric Food Chem. (1993) 41:1961–7. doi: 10.1021/jf00035a027
22. Alshehri, MM, Sharifi-Rad, J, Herrera-Bravo, J, Jara, EL, Salazar, LA, Kregiel, D, et al. Therapeutic potential of isoflavones with an emphasis on daidzein. Oxidative Med Cell Longev. (2021) 2021:6331630. doi: 10.1155/2021/6331630
23. Sathyamoorthy, N, and Wang, TT. Differential effects of dietary phyto-oestrogens daidzein and equol on human breast cancer mcf-7 cells. Eur J Cancer. (1997) 33:2384–9. doi: 10.1016/s0959-8049(97)00303-1
24. Adjakly, M, Ngollo, M, Boiteux, JP, Bignon, YJ, Guy, L, and Bernard-Gallon, D. Genistein and daidzein: different molecular effects on prostate cancer. Anticancer Res. (2013) 33:39–44.
25. Vitale, DC, Piazza, C, Melilli, B, Drago, F, and Salomone, S. Isoflavones: estrogenic activity, biological effect and bioavailability. Eur J Drug Metab Pharmacokinet. (2013) 38:15–25. doi: 10.1007/s13318-012-0112-y
26. Yamagata, K, and Yamori, Y. Potential effects of soy isoflavones on the prevention of metabolic syndrome. Molecules. (2021) 26:5863. doi: 10.3390/molecules26195863
27. Wang, X, Wang, Y, Xu, W, Lan, L, Li, Y, Wang, L, et al. Dietary isoflavones intake is inversely associated with non-alcoholic fatty liver disease, hyperlipidaemia and hypertension. Int J Food Sci Nutr. (2022) 73:60–70. doi: 10.1080/09637486.2021.1910630
28. Zipf, G, Chiappa, M, Porter, KS, Ostchega, Y, Lewis, BG, and Dostal, J. National health and nutrition examination survey: plan and operations, 1999-2010. Vital Health Stat. (2013) 1:1–37.
29. National Health and Nutrition Examination Survey (2020) “2017–2018 Data Documentation, Codebook, and Frequencies.” Available at: https://wwwn.cdc.gov/Nchs/Nhanes/2017-2018/DR1IFF_J.htm (Accessed November 09, 2022).
30. U.S. Department of Agriculture, Agricultural Research Service. (2022). Flavonoid values for USDA survey foods and beverages 2017-2018. Food Surveys Research Group Home Page. Available at: www.ars.usda.gov/nea/bhnrc/fsrg. (Accessed November 16, 2022).
31. Centers for Disease Control and Prevention (CDC). (2022). About the National Health and Nutrition Examination Survey 2017-2018. Available at: https://wwwn.cdc.gov/nchs/nhanes/Default.aspx (Accessed November 9, 2022).
32. U.S. Department of Agriculture. (2022). Agricultural Research Service. Food Data Central [Modified 2022 April 18]. Available at: https://fdc.nal.usda.gov. (Accessed May 31, 2022)
33. Centers for Disease Control and Prevention: National Health and Nutrition Examination Survey (NHANES). Liver ultrasound transient elastography procedures manual. (2018). Available at: https://wwwn.cdc.gov/nchs/data/nhanes/2017-2018/manuals/2018_Liver_Ultrasound_Elastography_Procedures_Manual.pdf (Accessed November 19, 2022).
34. Karlas, T, Petroff, D, Sasso, M, Fan, JG, Mi, YQ, de Ledinghen, V, et al. Individual patient data meta-analysis of controlled attenuation parameter (CAP) technology for assessing steatosis. J Hepatol. (2017) 66:1022–30. doi: 10.1016/j.jhep.2016.12.022
35. Ciardullo, S, Monti, T, and Perseghin, G. Prevalence of liver steatosis and fibrosis detected by transient elastography in adolescents in the 2017-2018 national health and nutrition examination survey. Clin Gastroenterol Hepatol. (2021) 19:384–390.e1. doi: 10.1016/j.cgh.2020.06.048
36. Eddowes, PJ, Sasso, M, Allison, M, Tsochatzis, E, Anstee, QM, Sheridan, D, et al. Accuracy of fibroscan controlled attenuation parameter and liver stiffness measurement in assessing steatosis and fibrosis in patients with nonalcoholic fatty liver disease. Gastroenterology. (2019) 156:1717–30. doi: 10.1053/j.gastro.2019.01.042
37. Bedogni, G, Bellentani, S, Miglioli, L, Masutti, F, Passalacqua, M, Castiglione, A, et al. The fatty liver index: a simple and accurate predictor of hepatic steatosis in the general population. BMC Gastroenterol. (2006) 6:1–7. doi: 10.1186/1471-230X-6-33
38. Lee, JH, Kim, D, Kim, HJ, Lee, CH, Yang, JI, Kim, W, et al. Hepatic steatosis index: a simple screening tool reflecting nonalcoholic fatty liver disease. Dig Liver Dis. (2010) 42:503–8. doi: 10.1016/j.dld.2009.08.002
39. Wai, CT, Greenson, JK, Fontana, RJ, Kalbfleisch, JD, Marrero, JA, Conjeevaram, HS, et al. A simple noninvasive index can predict both significant fibrosis and cirrhosis in patients with chronic hepatitis C. Hepatology. (2003) 38:518–26. doi: 10.1053/jhep.2003.50346
40. Sterling, RK, Lissen, E, Clumeck, N, Sola, R, Correa, MC, Montaner, J, et al. Development of a simple noninvasive index to predict significant fibrosis in patients with HIV/HCV coinfection. Hepatology. (2006) 43:1317–25. doi: 10.1002/hep.21178
41. Lee, J, Vali, Y, Boursier, J, Spijker, R, Anstee, QM, Bossuyt, PM, et al. Prognostic accuracy of fib-4, NAFLD fibrosis score and APRI for NAFLD-related events: a systematic review. Liver Int. (2021) 41:261–70. doi: 10.1111/liv.14669
42. Angulo, P, Hui, JM, Marchesini, G, Bugianesi, E, George, J, Farrell, GC, et al. The NAFLD fibrosis score: a noninvasive system that identifies liver fibrosis in patients with NAFLD. Hepatology. (2007) 45:846–54. doi: 10.1002/hep.21496
43. Global BMC Di Angelantonio, E, Bhupathiraju, S, Wormser, D, Gao, P, Kaptoge, S, et al. Body-mass index and all-cause mortality: individual-participant-data meta-analysis of 239 prospective studies in four continents. Lancet. (2016) 388:776–86. doi: 10.1016/S0140-6736(16)30175-1
44. Matthews, DR, Hosker, JP, Rudenski, AS, Naylor, BA, Treacher, DF, and Turner, RC. Homeostasis model assessment: insulin resistance and beta-cell function from fasting plasma glucose and insulin concentrations in man. Diabetologia. (1985) 28:412–9. doi: 10.1007/BF00280883
45. American Diabetes Association. 2. Classification and Diagnosis of Diabetes: Standards of medical care in diabetes-2020. Diabetes Care. (2020) 43:S14–31. doi: 10.2337/dc20-S002
46. Ruhl, CE, and Everhart, JE. Joint effects of body weight and alcohol on elevated serum alanine aminotransferase in the United States population. Clin Gastroenterol Hepatol. (2005) 3:1260–8. doi: 10.1016/s1542-3565(05)00743-3
48. Kirkpatrick, SI, Dodd, KW, Reedy, J, and Krebs-Smith, SM. Income and race/ethnicity are associated with adherence to food-based dietary guidance among us adults and children. J Acad Nutr Diet. (2012) 112:624–635.e6. doi: 10.1016/j.jand.2011.11.012
49. Vieux, F, Maillot, M, Rehm, CD, and Drewnowski, A. Flavonoid intakes in the US diet are linked to higher socioeconomic status and to tea consumption: analyses of NHANES 2011-16 data. J Nutr. (2020) 150:2147–55. doi: 10.1093/jn/nxaa145
50. Kim, MH, Park, JS, Jung, JW, Byun, KW, Kang, KS, and Lee, YS. Daidzein supplementation prevents non-alcoholic fatty liver disease through alternation of hepatic gene expression profiles and adipocyte metabolism. Int J Obes. (2011) 35:1019–30. doi: 10.1038/ijo.2010.256
51. Sakamoto, Y, Naka, A, Ohara, N, Kondo, K, and Iida, K. Daidzein regulates proinflammatory adipokines thereby improving obesity-related inflammation through PPAR gamma. Mol Nutr Food Res. (2014) 58:718–26. doi: 10.1002/mnfr.201300482
52. Yu, Z, Yang, L, Deng, S, and Liang, M. Daidzein ameliorates LPS-induced hepatocyte injury by inhibiting inflammation and oxidative stress. Eur J Pharmacol. (2020) 885:173399. doi: 10.1016/j.ejphar.2020.173399
53. Zhong, QW, Wu, YY, Xiong, F, Liu, M, Liu, YP, Wang, C, et al. Higher flavonoid intake is associated with a lower progression risk of non-alcoholic fatty liver disease in adults: a prospective study. Br J Nutr. (2021) 125:460–70. doi: 10.1017/S0007114520002846
54. Parvin, M, Fatemeh, M, Sima, A, and Fereidoun, A. Association of educational level and marital status with dietary intake and cardiovascular risk factors in Tehranian adults: Tehran lipid and glucose study (TLGS). Nutr Res. (2002) 22:1365–75. doi: 10.1016/S0271-5317(02)00440-2
55. Woo, J, Leung, SS, Ho, SC, Sham, A, Lam, TH, and Janus, ED. Influence of educational level and marital status on dietary intake, obesity and other cardiovascular risk factors in a Hong Kong Chinese population. Eur J Clin Nutr. (1999) 53:461–7. doi: 10.1038/sj.ejcn.1600777
56. Zein, CO, Unalp, A, Colvin, R, Liu, YC, and McCullough, AJ. Smoking and severity of hepatic fibrosis in nonalcoholic fatty liver disease. J Hepatol. (2011) 54:753–9. doi: 10.1016/j.jhep.2010.07.040
57. Corpechot, C, Gaouar, F, Chretien, Y, Johanet, C, Chazouilleres, O, and Poupon, R. Smoking as an independent risk factor of liver fibrosis in primary biliary cirrhosis. J Hepatol. (2012) 56:218–24. doi: 10.1016/j.jhep.2011.03.031
58. Mantaka, A, Koulentaki, M, Samonakis, D, Sifaki-Pistolla, D, Voumvouraki, A, Tzardi, M, et al. Association of smoking with liver fibrosis and mortality in primary biliary cholangitis. Eur J Gastroenterol Hepatol. (2018) 30:1461–9. doi: 10.1097/MEG.0000000000001234
59. Charatcharoenwitthaya, P, Karaketklang, K, and Aekplakorn, W. Cigarette smoking increased risk of overall mortality in patients with non-alcoholic fatty liver disease: a nationwide population-based cohort study. Front Med (Lausanne). (2020) 7:604919. doi: 10.3389/fmed.2020.604919
60. Day, CP, James OF. Steatohepatitis: a tale of two “hits”? Gastroenterology. (1998) 114:842–5. doi: 10.1016/s0016-5085(98)70599-2
61. Fang, YL, Chen, H, Wang, CL, and Liang, L. Pathogenesis of non-alcoholic fatty liver disease in children and adolescence: from “two hit theory” to “multiple hit model”. World J Gastroenterol. (2018) 24:2974–83. doi: 10.3748/wjg.v24.i27.2974
62. Xian, YX, Weng, JP, Xu, F, and Mafld, V. S. NAFLD: shared features and potential changes in epidemiology, pathophysiology, diagnosis, and pharmacotherapy. Chin Med J. (2020) 134:8–19. doi: 10.1097/CM9.0000000000001263
63. Cusi, K, Sanyal, AJ, Zhang, S, Hartman, ML, Bue-Valleskey, JM, Hoogwerf, BJ, et al. Non-alcoholic fatty liver disease (NAFLD) prevalence and its metabolic associations in patients with type 1 diabetes and type 2 diabetes. Diabetes Obes Metab. (2017) 19:1630–4. doi: 10.1111/dom.12973
64. Zhang, JZ, Cai, JJ, Yu, Y, She, ZG, and Li, H. Nonalcoholic fatty liver disease: an update on the diagnosis. Gene Expr. (2019) 19:187–98. doi: 10.3727/105221619X15553433838609
65. Liu, Y, Liu, S, Huang, J, Zhu, Y, and Lin, S. Validation of five hepatic steatosis algorithms in metabolic-associated fatty liver disease: a population based study. J Gastroenterol Hepatol. (2022) 37:938–45. doi: 10.1111/jgh.15799
66. Xiao, CW, and Hendry, A. Hypolipidemic effects of soy protein and isoflavones in the prevention of non-alcoholic fatty liver disease-a review. Plant Foods Hum Nutr. (2022) 77:319–28. doi: 10.1007/s11130-022-00984-1
67. Hakkak, R, Spray, B, Borsheim, E, and Korourian, S. Diet containing soy protein concentrate with low and high isoflavones for 9 weeks protects against non-alcoholic fatty liver steatosis using obese Zucker rats. Front Nutr. (2022) 9:913571. doi: 10.3389/fnut.2022.913571
68. Mazidi, M, Katsiki, N, and Banach, M. A higher flavonoid intake is associated with less likelihood of nonalcoholic fatty liver disease: results from a multiethnic study. J Nutr Biochem. (2019) 65:66–71. doi: 10.1016/j.jnutbio.2018.10.001
69. Maleki, Z, Jazayeri, S, Eslami, O, Shidfar, F, Hosseini, AF, Agah, S, et al. Effect of soy milk consumption on glycemic status, blood pressure, fibrinogen and malondialdehyde in patients with non-alcoholic fatty liver disease: a randomized controlled trial. Complement Ther Med. (2019) 44:44–50. doi: 10.1016/j.ctim.2019.02.020
70. Health Canada (2019) Canada’s Food Guide. https://food-guide.canada.ca/en/
71. Pierantonelli, I, and Svegliati-Baroni, G. Nonalcoholic fatty liver disease: basic pathogenetic mechanisms in the progression from NAFLD to Nash. Transplantation. (2019) 103:e1–e13. doi: 10.1097/TP.0000000000002480
72. Farrell, GC, and Larter, CZ. Nonalcoholic fatty liver disease: from steatosis to cirrhosis. Hepatology. (2006) 43:S99–S112. doi: 10.1002/hep.20973
73. Stal, P. Liver fibrosis in non-alcoholic fatty liver disease - diagnostic challenge with prognostic significance. World J Gastroenterol. (2015) 21:11077–87. doi: 10.3748/wjg.v21.i39.11077
74. Heyens, L, Busschots, D, Koek, GH, Robaeys, G, and Francque, S. Liver fibrosis in non-alcoholic fatty liver disease: from liver biopsy to non-invasive biomarkers in diagnosis and treatment. Front Med (Lausanne). (2021) 8:615978. doi: 10.3389/fmed.2021.615978
Keywords: daidzein, MAFLD, NHANES, hepatic steatosis, hepatic fibrosis
Citation: Yang Z, Gong D, He X, Huang F, Sun Y and Hu Q (2023) Association between daidzein intake and metabolic associated fatty liver disease: A cross-sectional study from NHANES 2017–2018. Front. Nutr. 10:1113789. doi: 10.3389/fnut.2023.1113789
Received: 01 December 2022; Accepted: 26 January 2023;
Published: 13 February 2023.
Edited by:
Jianbo Xiao, University of Vigo, SpainReviewed by:
Aaron Koenig, The Ohio State University, United StatesCopyright © 2023 Yang, Gong, He, Huang, Sun and Hu. This is an open-access article distributed under the terms of the Creative Commons Attribution License (CC BY). The use, distribution or reproduction in other forums is permitted, provided the original author(s) and the copyright owner(s) are credited and that the original publication in this journal is cited, in accordance with accepted academic practice. No use, distribution or reproduction is permitted which does not comply with these terms.
*Correspondence: Yi Sun, ✉ anp6eHl5c3lAMTYzLmNvbQ==; Qinming Hu, ✉ aHVxaW5taW5nXzE5NjRAMTYzLmNvbQ==
Disclaimer: All claims expressed in this article are solely those of the authors and do not necessarily represent those of their affiliated organizations, or those of the publisher, the editors and the reviewers. Any product that may be evaluated in this article or claim that may be made by its manufacturer is not guaranteed or endorsed by the publisher.
Research integrity at Frontiers
Learn more about the work of our research integrity team to safeguard the quality of each article we publish.