- 1Laboratorio de Biología Celular y Tisular, Departamento de Morfología, Centro de Ciencias Básicas, Universidad Autónoma de Aguascalientes, Aguascalientes, Mexico
- 2Laboratorio de Biotecnología, Departamento Ingeniería Bioquímica, Centro de Ciencias Básicas, Universidad Autónoma de Aguascalientes, Aguascalientes, Mexico
- 3Laboratorio de Estudios Ambientales, Departamento de Fisiología y Farmacología, Centro de Ciencias Básicas, Universidad Autónoma de Aguascalientes, Aguascalientes, Mexico
Mexico is one of the largest guava producers in the world, so it has access to a huge amount of waste and byproducts obtained after the industrial processing of the fruit. This review discusses the potential recovery of this residue for its application as an antimicrobial agent, considering the phytochemical composition, the bioactivity reported in-vivo and in-vitro, and the toxicology of the plant. Nowadays there is a growing demand for more natural and safer products, so the use of guava extracts is an interesting initiative, especially due to its availability in the country, its wide variety of traditional uses, and its phytochemical profile. This review highlights the importance and potential antimicrobial use of this plant in today's world.
1. Introduction
Psidium guajava L. is a native American shrub that can grow in tropical environments around the world (1). It can reach up to 7 m in height and 25 cm in diameter in the trunk when it reaches maturity. Its bark is smooth, thin and coppery brown, while its leaves are green, perennial, coriaceous, and with short petioles. This plant also has white flowers with 4–5 petals and numerous stamens, characteristic of the Myrtaceae family (2).
However, its economic importance lies mainly in its fruit, the guava, a juicy berry with numerous seeds and a slightly acid taste. Its shape and color depend on the variety, so we can find guavas from yellow to bright pink and round, ovoid, or pear-shaped (2). The morphological characteristics of P. guajava L. are illustrated in Figure 1.
The guava belongs in the Myrtaceae family, known for having numerous species with high antioxidant activity (3). On the other hand, the Psidium genus has ~ 150 species of shrubs, of which Psidium guajava L. is the best known and distributed worldwide (4). Regarding its phenology, this shrub flowers mainly in spring and fruiting can appear throughout the year, mostly during the summer months (5).
Guava worldwide production is estimated to be around 40 million tons. Even if fresh guava trade is limited internationally, the marketing of a wide variety of products derived from its fruit such as preserves, jams, jellies and syrups is becoming more common (6). The highest production of guava can be found in India, Thailand, Brazil, and Mexico (7).
Mexico is a key guava producer in the international market, whose export volume increased more than 150% in < 10 years, from 4,306 tons of guava exported in 2009, to 10,850 tons exported in 2018 (5). Unfortunately, the Mexican agricultural sector presents serious post-harvest problems, generating enormous fruit losses during the different stages of the production chain. Guava is one of the most affected fruits with a waste of more than 50% of its national production (8).
Specifically, in the industrial processing of guava, a heterogeneous mixture of peels, seeds and pulp is generated, and it that can represent up to 30% of the total mass. Guava leaves and bark are other byproducts that are obtained, mainly during the harvest of the fruit (9). Approximately 80 kg of waste per metric ton of fresh fruit is produced during guava processing (10). Unfortunately, and in most cases, the waste generated is thrown into landfills or is incinerated, increasing the environmental load and the total cost of production due to the handling and transportation that these residues require (9, 11).
The aim of this updated review is to expose the potential of Psidium guajava L. as an antimicrobial agent to promote the valorization of guava agro-industrial waste and byproducts in Mexico.
2. Review methodology
The search engines Google scholar, Elsevier, Springer, MDPI and Science Direct were used to find research articles and reviews related to Psidium guajava L. Documents from 2000 to 2022 in Spanish and English were included, focusing in the most recent articles. Articles published before the 2000s were excluded. Book chapters, official sites of the Mexican government and Mexican companies were also consulted. The keywords used were “Psidium guajava L.,” “traditional uses,” “phytochemistry,” “biological activities,” “toxicology,” “In-vivo evaluation,” and “antimicrobial effects” “diabetes.”
3. Traditional uses
Psidium guajava L. has been traditionally used as a medicinal plant in different places around the world. Its applications are highly diverse and range from the treatment of gastrointestinal diseases such as vomiting and simple diarrhea to the treatment of wounds, caries, and cough (12–15) as we can see in Figure 2.
All parts of the bush have been used to treat different disorders (16). For example, the leaves and fruit are used to treat respiratory and digestive problems, while the seeds and the leaves have been used as an antispasmodic, anti-inflammatory and even in the control of hypertension and diabetes (17).
Its use also varies depending on geographical location. It has been reported that in Trinidad, Fiji, China and distinct parts of Latin America, guava leaves are used to treat diarrhea and stomach pain (12). On the other hand, in Uruguay they are used to wash the vagina and uterus, especially leucorrhoea cases; in the Cook Islands, guava leaves are used for sores, cuts, sprains, and boils; while in India they are used together with the root to treat rheumatism, seizures, and fevers (12). In the Amazonia this plant is used for the treatment of menstrual disorders, stomach pain, and vertigo, in Cuba, for colds, dysentery, dyspepsia, diarrhea, and hypertension. Finally, in Haiti, guava is used for epilepsy, diarrhea and different skin disorders (15).
Thanks to scientific research, novel applications of guava have been discovered in immune and endocrine systems diseases, as well as in cancer and diabetes. In addition, studies have reported that guava leaf extracts can be used to improve skin problems such as acne and hyperpigmentation (18).
3.1. Traditional uses of Psidium guajava L. in Mexico
In Mexico, herbalism is an ancient practice that has been used to this day and has great cultural and economic importance. However, in most cases, the active ingredients that provide the beneficial effect are unknown and, therefore, it is a field of study of high scientific interest (19). Guava leaves have been used for medicinal purposes in the country since very remote times, their presence in historical documents on indigenous herbalism has been constant for at least five hundred years (20).
This shrub used to be called by the ancient Mexicans as “xalxócotl,” a word in Nahuatl that refers to a fruit that has a “hard and acid shell (xócotl) and a sandy texture (xalli)”, due to its abundant seeds (20). Guava can be called in diverse ways depending on the Mexican state, for example, in Chiapas, this plant is usually called pata, pocscuy, potok, pox, or sumbadam; in Michoacán it is known as enendi, in Nayarit as caaru, in Morelos as coloc or jaljocote pichi and in Veracruz as asiwit, cuympatan, or pitchcuy (21).
The uses of P. guajava L. in the country are as diverse as its names, however, its most common medicinal use is to treat stomach pain. Diarrhea, dysentery, fever, and cough are treated with infusions of guava and its leaves; in the case of skin problems, the leaves are cooked and applied locally. The infusion of guava buds and leaves are used as a de-wormer and guava tea is used to cure scares, using it to give short baths (21).
The medicinal use of guava can also vary slightly according to the geographical location in the country, for example, while in southern Veracruz it has been reported that the plant is used to treat diarrhea (22), in the Huasteca Potosina it is also used to treat herpes, wounds, toothache, gastritis and rashes (13). On the other hand, in Guerrero, the infusion of guava leaves is used to cure cough, fever, flu and stomach pain (14). Figure 3 represents and summarizes the different names and traditional uses of guava in Mexico.
4. Current importance of guava in Mexico
In Mexico there are twenty entities that harvest this fruit, with Michoacán being the main producing state, followed by Aguascalientes and Zacatecas (23). In 2020, a planted area of 29,872.67 hectares was reported, from which a production of 287,273.02 tons of guava was obtained. This meant an economic spill of $1,664,607.62 billion Mexican pesos (24). The most harvested variety of guava in the country is undoubtedly the “media-china,” while the “china” and the “criolla” are produced in far less quantity (24).
It should be noted that, as of 2008, fresh guava started being sent to the United States of America (25). This is interesting given that, traditionally, the export of this fruit was conducted in its dehydrated or processed versions; however, its nutritional and exotic appeal increased the interest of developed countries like United States, Canada, and Japan (26).
A wide variety of guava processed products such as candies, rolls, ates, ice cream, jams, jellies, juices, and even “guava mole,” a highly seasoned sauce, can be found in Mexico. However, its importance lies not only in its alimentary use, but it also has great relevance for its cosmetic and medicinal applications. In the Mexican market we can also find multiple products that contain guava leaves, like extracts, pills, concentrates, and food supplements that are presented as a natural remedy for stomach pain or as an antioxidant and anti-aging agent. The most relevant example is a drug to relieve the symptoms of colitis called QG5, based on a dry extract of Psidium guajava L. which is sold in pharmacies throughout the country. The effect of QG5 is attribute to the presence of quercetin (27).
The composition of the extracts marketed in the country is not clear and, in general, their beneficial effects are attributed to what is already known for its traditional uses. Hence the importance of knowing which compounds are responsible for such effects, and thus be able to move from tradition to science. This will allow us to give a better use to the extract, obtaining a better, and safer beneficial effect and we can even get to know interesting new bioactivities.
5. Phytochemical composition
Psidium guajava L. chemical composition includes compounds such as tannins, phenols, flavonoids, saponins, carbohydrates, alkaloids, sterols, and terpenoids (28). It is important to consider that the type and abundance of phytochemicals can vary depending on the microclimate and soil conditions of the habitat (29), but also depending on the plant tissue and seasonal changes (30).
The most analyzed part of this shrub is undoubtedly its leaves, given its frequent use as a medicinal remedy. Shabbir et al. (31) reported the approximate composition of guava leaves: 82.47% moisture, 3.64% ash, 0.62% fat, 18.53% protein, 12.74% carbohydrates, 103 mg of ascorbic acid (vitamin C), and 1,717 mg of total phenolic compounds [mg of gallic acid equivalents (GAE)/g]. It should be noted that Shabbir et al. (31) also observed a higher concentration of phenolic compounds in the leaves than in the seeds and fruits of guava.
Guava leaves are also a rich source of vitamins and minerals, such as calcium, potassium, sodium, magnesium, iron, sulfur, vitamin B, and C (32). Even, Thomas et al. (33) mentioned that the leaves have a higher concentration of vitamin B (14.80 mg/100 g), calcium (1,660 mg/100 g), magnesium (440 mg/100 g), phosphorus (360 mg/100 g), and iron (13.50 mg/100 g) compared to the fruits, however, the fruit is richer in vitamin C (228.3 mg/100 g) and potassium (417 mg/100 g).
The higher concentration of calcium in guava leaves (1,660 mg/100 g) compared to the concentration in the fruit (18 mg/100 g) may be since its transpiration rate is higher (34). Similarly, there is a trend toward major magnesium allocation in transpiring organs such as leaves (440 mg/100 g) and flowers rather than roots and fruits (22 mg/100 g) (35).
Furthermore, it is important to consider that the nutrient content varies considerably between different plant organs, as well as by the age of the tissue in question. Beyond that, the nutrient content largely depends on optimal uptake and soil quality (36).
The polysaccharides found in guava leaves have also had great relevance in recent years since they have been found to be beneficial for the treatment of diabetes mellitus symptoms, as will be discussed in more depth later, and they can also be used as antioxidant additives in food (37).
Díaz-de-Cerio et al. (38) identified more than 70 phenolic compounds in guava leaves by HPLC-DAD-QTOF-MS using hydro-alcoholic extracts obtained by sonication. Table 1 shows the main compounds identified in extracts of Psidium guajava L. reported by different authors.
The recovery of the unused flesh, peels, seeds, flowers, bark, and leaves is a great opportunity to guava producing countries, considering the variety of bioactive compounds that can be extracted (47).
Nonetheless, an aspect to consider during the use of industrial byproducts is the possible presence of food additives, as is the case of the pulp extract used by dos Santos et al. (44), which contained different additives, among them, the antioxidant BHT, which may cause allergies, eczema, rash, and angioedema, therefore BHT cannot exceed an ADI of 0.5 mg/kg (48). As the presence of these additives probably remains after the extraction and can affect the activity of the extract, it is important to determine in what concentrations are they found in the plant material and what are the possible toxicological effects that they may cause depending on the route of administration.
On the other hand, several authors have reported that the main component of the extracts or essential oils of guava leaves is β-Caryophyllene (45, 49, 50), which has recently gained attention due to its potential application for the treatment of various disorders such as cancer, chronic pain, and inflammation (51). In addition, many of the plant extracts where β-Caryophyllene is found have antimicrobial effects, notwithstanding the role that this compound plays in said activity is still unclear. One of the proposed modes of action is that it alters the permeability of the bacterial membrane causing the formation of non-selective pores (52).
Even if the mechanism of action and the interactions between all the components of the Psidium guajava L. extracts is ambiguous, many researchers around the world have identify different beneficial effects of their components, for example, their anti-inflammatory, antioxidant, and antimicrobial activity. Table 2 shows some properties attributed to compounds found in the different guava extracts.
Observing the properties of some of its components (Table 2), it is not surprising that numerous studies have reported the potential of different extracts of Psidium guajava L. as an antimicrobial agent, so in this section we will dedicate ourselves to present the most relevant discoveries of recent times on this topic to create an overview of the different applications that we can get from this plant and what needs to be done to make these benefits available to society.
6. Antimicrobial effects of Psidium guajava L.
We are currently facing a serious global problem regarding microbial resistance, which has been aggravated during the COVID-19 pandemic. In Mexico, it has already been reported that strains such as Staphylococcus aureus, Klebsiella pneumoniae and Enterococcus faecium increased their resistance during the pandemic (65). Consequently, there is an urgent need for new and better antimicrobials, where the use of phytochemicals has drawn the attention of many researchers around the world.
Table 3 summarizes various evaluations of the antimicrobial effect of Psidium guajava L. carried out in recent years. Different solvents, plant parts and tests were used. Overall, the results indicate that guava extracts have potential activity on multiple bacteria strains and on yeasts of the genus Candida.
It should be noted that a proper comparison between studies cannot be made, even if similar methodologies were used, since each author established the MIC differently, for example, Soliman et al. (41) defined MIC as the lowest concentration that had granular appearing micro-colonies of growth instead of filamentous radiating colonies on solid agar; while for Hemeg et al. (68) MIC was the lowest concentration of extract that resulted in no visible growth on the surface of the agar. In addition, we can also find multiple differences between the methods to measure the antimicrobial effect, for example, dos Santos et al. (44) used a 0.1% resazurin solution, while Festus et al. (67) analyzed bacterial growth based on turbidity.
Due to all these variants, it is difficult to interpret the results in a general way and the fact that different solvents, types of extraction, and parts of the plant were used, makes everything more complex, because the results depend on a great variety of factors. The selection of controls is also a factor to improve, given that in some studies negative controls were used but not positive ones and vice-versa. In addition, the part of the plant used to perform the extraction of phytochemicals and the location where the plant material was collected should be clear and precise.
However, the highest dose used in the studies analyzed was 160 mg/ml (160,000 ug/ml) by Festus et al. (67), but the highest reported MIC was 40 mg /ml (40,000 ug/ml) for the bacteria Pseudomonas aeruginosa, Bacillus cereus, and Escherichia coli (67).
6.1. Anti-biofilm effects of guava extracts
Biofilms are architectural elements embedded in self-produced extracellular polymeric substances that adhere to inert or biological surfaces. These elements allow microorganisms to adapt to their environment and on certain occasions give them the ability to escape host defense systems and may even confer resistance to antibiotics given the difficult penetration of molecules into the matrix of extracellular polymeric substances (69).
Therefore, finding substances that can inhibit the formation and growth of biofilms is of special interest, especially if they are substances of natural origin, such as phytochemicals, which can be substitutes for synthetic drugs without presenting significant secondary effects (28).
Unfortunately, recent studies of the potential antibiofilm activity of P. guajava L. are not numerous and focus on the effect on S. aureus. Therefore, research on multiple microorganisms is needed to evaluate the spectrum of antibiofilm activity of guava extracts. Table 4 presents a summary of the results obtained and the possible mechanism of action proposed by the authors.
The MBEC (Minimum Biofilm Eradication Concentration) reported by dos Santos et al. (44) (250 μg/ml) is lower than those reported by Dutta et al. (69) (1,000–2,100 μg/ml). This could indicate that probably the guava leaf extract has a higher activity compared to the compound Benzyl isocyanate (isolated from guava leaves) on its own. The interaction of the components of the extract and a possible synergy may be involved in this fact, but future research is needed to clarify and verify this issue.
The antibiofilm mechanism exhibit by the phytochemicals presents in plant extracts are highly diverse, and among them are the decrease in the production of virulence factors, the inhibition of the formation of the polymeric matrix, the suppression of cell adhesion and the alteration of quorum sensing (72).
The lack of antibiofilm evaluations with extracts from another part of the plant, since those published focus mainly on the leaves and their derivatives, shows that there is still much to discover on this topic and a large field of study opens for those interested in this area.
6.2. Antiviral effects of Psidium guajava L.
Nowadays, researchers have been particularly interested in the study of medicinal plants as a therapeutic option for the treatment of viral infections, based on traditional ethnomedical wisdom and taking advantage of their molecules to develop antiviral drugs (73). Different studies have revealed that Psidium guajava L. extracts may have antiviral activity and some of them are summarized below.
The infusion of guava leaves was able to inhibit the growth of isolated influenza A (H1N1) virus. Likewise, it was able to inhibit viral hemagglutination and sialidase activity (IC50= 0.44 ± 0.05–7.50 ± 0.49) (74). Therefore, it appears to be effective for the control of pandemic and epidemic influenza viruses, including oseltamivir-resistant strains (75).
Sharma et al. (76) reported that the guava leaves extract and its nanoparticles reduce/stop chikungunya virus replication in the Vero cell line. Specifically, the authors reported a viability of 84.21% of the cells treated with the guava extract, a viability of 64.40% of the cells treated with the nanoparticles of the extract, and a viability of 24.03% of the positive control (virus + cells). The authors conclude that in the absence of vaccines and antivirals, the extracts of P. guajava L. can be an alternative treatment.
The antiviral effect of the crude aqueous extract of 10 plants used in traditional medicine in the Philippines, including the leaves of Psidium guajava L., was analyzed by Vista et al. (77). The authors reported that guava leaves were one of the best candidates against ZIKA virus (ZIKV) along with M. charantia, V. negundo, and B. balsamifera.
Trujillo-Correa et al. (78) reported that four compounds isolated from guava bark extracts, specifically gallic acid, quercetin, naringin, and catechin, inhibited dengue virus (DENV-2) replication. Of these, catechin was the most promising compound. The authors also mention that the anti-DENV effect of the P. guajava L. extract could be related to the inhibition of the enzyme α-glucosidase, since it has been described as essential for the correct folding of viral glycoproteins and for the assembly of the virion.
Shin et al. (79) studied the potential of BEN815, a natural nutraceutical composed of extracts of guava leaves (Psidium guajava L.), green tea leaves (Camellia sinensis), and rose petals (Rosa hybrida) to treat COVID-19. The authors reported that BEN815 showed antiviral activity against SARS-CoV-2, with an IC50= 34.38 μg/ml. In addition, it is mentioned that of the compounds found in the nutraceutical, EGCG (Epigalocatechin gallate) plays a key role in the antiviral effect against SARS-CoV-2.
7. Potential mechanisms of action of antimicrobial activity
The exact mechanism of antibacterial activity is still not clear, mainly because phytochemicals have highly variable structures, generating multiple possible modes of action. Moreover, plant extracts contain a complex mixture of compounds whose interaction can influence the mechanism (80). Therefore, the mode of action depends on the type of extract or essential oil and the microorganism used (81).
Nonetheless, it has been shown that phenolic compounds can interact with bacterial cell walls, leading to their rupture and the release of cellular components (80), they can also suppress several microbial virulence factors (among them biofilm formation and toxin production), inhibit the synthesis of nucleic acids and the activity of enzymes (82). In addition, it has been reported that gram-negative bacteria are more resistant to phenolic compounds, probably due to the presence of an outer membrane and enzymes in the periplasmic space that can damage molecules that enter the bacteria (80).
Similarly, the mode of action of terpenes, also an important component of guava extracts, remains largely unknown, however, it has been observed that most terpenoids can inhibit two essential processes for microbial survival: oxygen uptake and oxidative phosphorylation. Thus, terpene interaction leads to alteration in cellular respiration which later causes uncoupling of oxidative phosphorylation in the microbe (83).
On the other hand, the proposed mode of action of β-caryophyllene, a bicyclic sesquiterpene considered by multiple authors as the main component of guava leaf extract (45, 49, 50), is through altering the bacterial membrane permeability and causing non-selective pore formation. This induces the intracellular content leakage leading to damage and loss of the membrane integrity and may eventually lead to cell death (52). Figure 4 summarizes the possible mechanisms of action for the bactericidal activity.
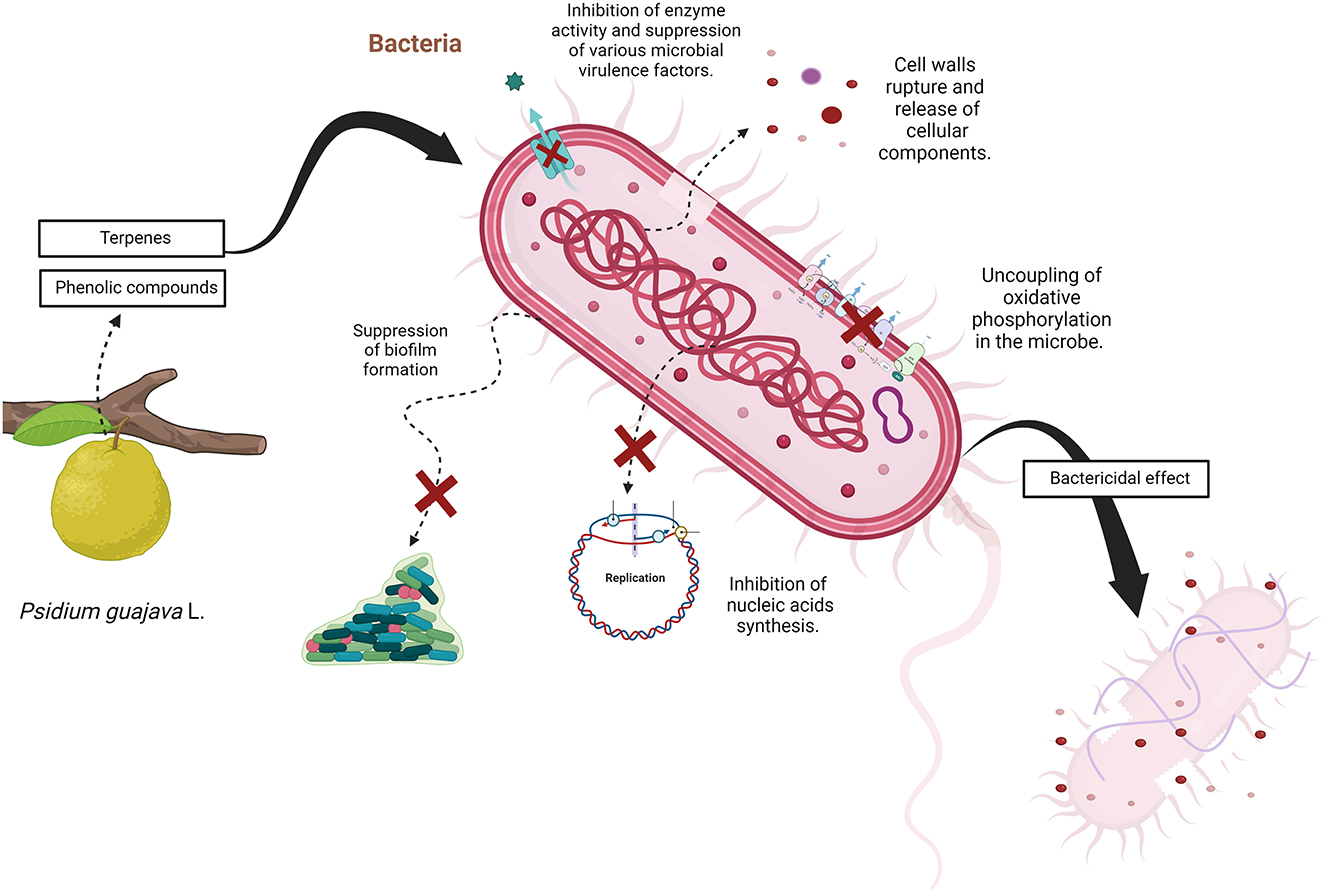
Figure 4. The possible antibacterial mechanisms of action of Psidium guajava L., adapted and modified from Bankour et al. (84).
Regarding the antiviral activity exerted by phenolic compounds, the mechanisms proposed are the suppression of the infection process and/or the repression of viral replication. In this regard, reported antiviral modes of action include viral DNA/protein damage and/or inhibition of viral enzymes (85).
8. Antidiabetic activity
Diabetes mellitus is a chronic disease that causes an increase in blood glucose levels, type 1 is caused by the loss of beta cells from the pancreatic islets, so the pancreas is no longer capable of producing insulin, while type 2, is caused by insulin resistance, so the body cannot effectively use the insulin it produces (86).
In 2021, 13% of deaths in Mexico were due to diabetes, and of the deceased, 74.9% were not insulin dependent (87). In addition, the country ranks second in Latin America with the highest prevalence of diabetes with 11.5 million cases (88).
Although, in Mexico there are different treatments available such as insulin and oral hypoglycemics, a large part of the population continues to use and even prefers the use of medicinal plants (86, 89). In addition, it should be noted that many current treatments have side effects such as gastrointestinal problems, heart failure, weight gain, edema, impaired kidney function, pancreatitis, and genital infections (90).
Multiple hydrolytic enzymes are involved in carbohydrate digestion, including α-amylase, which hydrolyzes starch, glycogen, and many oligosaccharides into maltose, maltotriose, and oligoglycans, which are further hydrolyzed by α-glucosidase to glucose, more suitable for absorption, increasing blood glucose levels (91, 92). Thus, inhibition of these enzymes can decrease postprandial glucose uptake and is therefore a key therapeutic target in the management of type 2 diabetes mellitus (91). Table 5 presents different studies that have analyzed the inhibition of these enzymes by extracts of P. guajava L. and their results.
As we can see in Table 6. multiple studies have analyzed the effect of Psidium guajava L. on the inhibition of α-amylase and α-glucosidase, obtaining good in-vitro results; this may be due to the presence of quercetin in the extracts, a competitive inhibitor of α-glucosidase, since the hydroxyl present in the 3, 3′ and 4′ carbons in its structure can interact with the Asp214 and Glu276 present in the enzymatic active site (91). In addition, tannins have also been shown to be good inhibitors of α-glucosidase with Ki values similar to those of synthetic inhibitors such as acarbose and voglibose, which are currently used as treatment to control type 2 diabetes (101). The variations in the IC50values can be due to multiple factors, among them, the location and season of the year in which the biological material was collected, the extraction method, possible differences in the methodologies and in the enzymes used, for example, Beidokhti et al. (93) used a porcine α-amylase, while Samejima et al. (94) a bacterial one.
Xu et al. (102) administered 800 mg/kg of guava leaf extract daily to diabetic rats and observed a significant reduction in total cholesterol, Low-density lipoprotein cholesterol (LDL-C), and glycosylated serum protein (precise intermediate marker of glycemia) compared with the control group (diabetic rats that received only distilled water). Exposing that guava leaves have antihyperglycemic and antihyperlipidemic effects.
The same year, Luo et al. (103) worked with diabetic mice induced by streptozotocin and high-fat diets and reported an improvement in body weight, decrease in fasting blood glucose, total triglycerides and total cholesterol levels with the daily intragastric administration of polysaccharides isolated from guava leaves (100 and 200 mg/kg) for 4 weeks. They even observed protective effects on the kidney, since the creatine content, whose excretion is an indicator of the organ's metabolism and whose accumulation indicates impaired functioning, decreased after the administration of the polysaccharides. Similarly, Glycated serum protein levels decreased in a dose-dependent manner.
On the other hand, Eidenberger et al. (104) analyzed the absorption of guava leaf extract in the CaCo-2 cell line, widely used to investigate nutrient absorption, and concluded that the extract can exert its observed effect in-vitro after being administered orally. Although there are results that indicate a potential antidiabetic effect, there is still much to be investigated, especially the activity and safety in humans, the possible mechanisms of action and the long-term effects.
9. Toxicity
Numerous health benefits of plants and their extracts have been reported, for example, as potential antioxidant, anticancer, antimicrobial, and anti-inflammatory agents. However, even though they may exhibit excellent in-vitro activity, we cannot take advantage of these benefits until they are proven to have no harmful side effects. The use of a plant for a certain purpose must not only be effective, but also safe for the consumer (105).
In-vivo and in-vitro toxicology studies are conducted to determine a drug's short and long term functional and morphologic adverse effects. There is a large variety of toxicological studies, among them, the acute toxicity studies are carried out to determine the short-term adverse effects of a single dose of a drug (or multiple doses during a period of 24 h) and the subacute toxicity studies, performed to evaluate the possible adverse effects of a new drug after a treatment period of 2–4 weeks duration (106). Table 6 shows some of the toxicological studies carried out with guava extracts.
Manekeng et al. (96) evaluated the toxicity of orally administered Psidium guajava L. bark methanolic extract in Wistar rats, the authors observed no abnormalities or mortality in rats at a dose of 5,000 mg/kg, however, they mention that the repeated administration of high doses (1,000 mg/kg or more) could exhibit mild organ toxicity. Similarly, Atik et al. (97) in their acute toxicity test observed no side effects in Swiss Webster mice after oral treatment with 2,000 and 5,000 mg/kg of guava ethanolic extract.
Babatola et al. (98) evaluated the acute toxicity of aqueous extracts of guava leaves of three different varieties (white, red, and pink guava), none of the extracts caused death or generated any symptoms of pathology at concentrations of 50, 500, and 5,000 mg/kg. These results are interesting because we must remember that the composition of the extracts can vary due to many factors, among them the variety and therefore, the toxicity can also be affected; this study was not the case, but it is important to consider this fact to avoid generalizing the results.
Bautista et al. (99) conducted the Brine Shrimp Lethality Assay (BSLA), a simple and inexpensive bioassay used for evaluating the efficacy of phytochemical present in the plant extracts. This assay is based on the ability to kill a simple zoological organism on 24 h (107). The authors evaluated the toxicity of aqueous extracts of guava bark and leaves and concluded that bark leaf extract is more toxic compared to leaf extract.
The toxicological evaluation is very important, especially considering that in many parts of the world, including Mexico, it is thought that herbal products, thanks to their natural origin, are not toxic and are used indiscriminately, nonetheless, everything depends on the dose and the consumer. Unfortunately, in recent years an increase in cases of adverse effects of herbal products has been reported (98), which reflects the need for better and more complete toxicological evaluations of plant extracts.
10. Bioavailability
Bioavailability is the ability of certain compound to reach the circulatory system and be distributed throughout the body. While bioaccessibility, another important concept, refers to the amount of compound that is available for absorption in the gastrointestinal tract (108).
The digestion of phenolic compounds is a complex and not fully understood process, however, today we know that the release of phenolic compounds begins with mastication (chewing) and the low pH of the stomach, which generates the disintegration of the food matrix. Subsequently, depolymerization begins, also in the stomach, where the phenolic compounds are degraded into small structures so that they can be absorbed. Uptake of small phenolic compounds, particularly aglycones, occurs by passive diffusion (lactase phloridizin hydrolase activity) or active transport by sodium-dependent glucose transporter (cytosolic β-glucosidase). Unabsorbed phenolic compounds now move to the colon, where the gut microbiota can facilitate absorption of phenolic compounds, nevertheless, the variability within individual gut microbiota is a determinant factor to increase the bioaccessibility of phenolic compounds (108).
The bioavailability of phenolic compounds depends on several factors, including release from the matrix during gastrointestinal digestion, bioaccessibility, cellular uptake, metabolism, and further transport in the circulatory system. Unfortunately, most phenolic compounds are poorly absorbed in the small intestine, as they are either heavily metabolized or rapidly eliminated. In addition, polyphenols can be associated with the dietary fiber matrix, through covalent bonds or hydrophobic interactions, which means that they are not bioavailable in the human intestine (109).
Similarly, terpenes have low water solubility and low bioavailability. Furthermore, it has been noticed that terpenes have a highly lipophilic behavior that influences their solubility in the aqueous phase of the intestinal lumen and, therefore, their bioavailability according to their incorporation into a lipid phase, either during digestion or during food processing (110).
To enable phytochemicals compounds, like polyphenols and terpenes, applications as antimicrobial agents, it is necessary to improve its bioavailability. One of the most promising strategies, which can also increase their stability, is the use of delivery systems, such as liposomes, nanoemulsions, and polymeric/biopolymeric nanoparticles (111). For example, Vasconcelos et al. (112) generated a self-emulsifying drug delivery system containing purified lycopene from red guava.
11. Clinical trials
Birdi et al. (113) conducted a clinical trial with 109 patients diagnosed with diarrhea. The authors reported that a decoction of guava leaves was a safe and simple treatment for common diarrhea. However, some trial limitations must be considered, first, the study was conducted with adults, so the safety and effectiveness in children, who are more susceptible to this pathology, remains to be proven. Moreover, since bacteriological examination of the stools was not undertaken at screening to identify causative organism, the laboratory results could not be verified clinically from the patients in this trial.
In addition, Nayak et al. (114) conducted a double blind randomized, placebo controlled clinical trial with subjects with moderate to severe chronic gingivitis. The authors evaluated the potential activity of an hydroethanolic extract of guava leaves incorporated in a mouthrinse in 56 patients aged between 18 and 40 years who were evaluated for 3 months. Although the guava mouthrinse group showed beneficial results, like reductions in plaque scores and improvement in gingival health, the results were not statistically significant. In addition, further randomized clinical trials should be carried out for an extended period to substantiate its long-term effects.
Similarly, Amaliya et al. (115) evaluated the effect of guava and vitamin C supplementation on gingivitis. The randomized clinical trial had the participation of 48 persons; however, it was also carried out in a short period of time (4-week) which did not allow to make firm conclusions.
Pongsakornpaisan et al. (116) conducted a study on the efficacy of their anti-sebum toner based on guava leaf extract with 10 volunteers. The authors observed that guava toner suppressed the sebum level in the nose and forehead area after 28 days of treatment and concluded that guava may be a good agent for cosmetic products. Although, the number of participants was small and long-term effects need to be assessed.
König et al. (117) reported that guava extract obtained by supercritical CO2 extraction reduced postprandial blood glucose levels in a randomized, double-blind, parallelized clinical study conducted with 31 young and healthy participants (20 female and 11 male, ages 19–29) from the Paracelsus Medical University Salzburg. After an overnight fast, each participant underwent an oral glucose tolerance test, in which members of the control group each received a glucose solution (75 g glucose) and members of the intervention group each received a glucose solution that also contained 2.5 ml of extract. However, it should be considered that the number of participants was small and only healthy young people were considered, so the effect on adults, children, and people with chronic diseases, including diabetes, is unknown. In addition, a low concentration of the extract was used, so possible side effects at higher concentrations and after long periods of administration were not reported. An important fact also mentioned by the authors is that the effect of the extract decreased after storage, therefore, it is necessary to consider the possible instability of the extracts over time for future applications.
Another similar clinical trial was carried out by Kumari (118), which had 45 young and healthy participants (31 male and 14 females, 18–25 years). The trial was conducted for 4 months, with 6 weeks of guava supplementation. Fifteen subjects were supplemented with 400 g of ripe guava with peel, another 15 with 400 g guava without peel and 15 subjects were the control group, which did not receive any supplement. After supplementation with ripe guava without peel a drop in blood sugar was observed. The authors attribute this effect to the high fiber content in the guava pulp, since it delays the intestinal absorption of glucose; the presence of flavonoid glycosides that improve insulin sensitivity, as well as the inhibition of the α-glucosidase enzyme. In addition, there was also a decrease in total cholesterol and triglycerides and an increase in high-density lipoproteins (HDLc). However, supplementation with ripe guava fruits with peel gave different results, since it increased blood glucose levels, cholesterol, triglyceride, and low-density lipoprotein (LDLc) levels. According to the authors, this effect may be since the peels have a low concentration of magnesium, necessary for the activity of multiple enzymes and which favors insulin-dependent glucose absorption; however, this explanation is ambiguous, and more studies are necessary to clarify this phenomenon.
More detailed clinical trials need to be conducted to establish the efficacy of the guava extracts.
12. Perspectives and conclusions
The potential of Psidium guajava L. and its different extracts is evident, however, there is still much to investigate so the different benefits can have an industrial application and therefore can reach society.
First, the repertoire of strains used in the analysis of antibiofilm effects must be expanded, moreover, most of the published studies about antimicrobial effects are in-vitro evaluations (antibiograms, microdilution method, etc.), therefore, in-vivo studies should be encouraged in the coming years.
On the other hand, the literature focuses mostly on the bioactivity of guava leaves, however, if the aim is to use agro-industrial residues, it will probably be difficult to separate the leaves from the rest of the waste (heterogeneous mixture of leaves, bark, seeds, and pulp), so it would be interesting to analyze the effect of an extract obtained from this combination. Another inconvenience that should be considered when using waste and byproducts from the agroindustry is the possible presence of food additives and pesticides.
Besides, it is important to improve the long-term stability of the extract, since in most studies the conservation of the extracts is carried out by freezing, refrigeration, or drying, which would not be very viable in industrial applications. In addition, it is known that phenolic compounds have low bioavailability, so it is necessary to find a way to protect them and make them more available, for example, through encapsulation.
More detailed clinical trials need to be conducted to establish the efficacy of guava extracts, and it would be interesting to analyze their effect in the treatment of other diseases than diarrhea or oral problems, for example, flu and acne.
Finally, the possible variation in phytochemicals present in the extracts due to changes in climate, season, and other factors such as the presence of insects can cause a lack of homogeneity in the final product, which should also be considered if commercialization is sought.
The recovery and valorization of guava waste can bring economic benefits to the agroindustry and can represent an alternative to the use of traditional antimicrobials, helping the problem of microbial resistance in Mexico, which has easy access to this residue. In addition, it can reduce environmental problems caused by leaching and emission of greenhouse gases generated during the treatment of agro-industrial waste.
Author contributions
DGM was the main writer of the review. ALGB is the main head of the laboratory. NACV and FJAG review the manuscript. IGOG also participate in the redaction of the review. All authors contributed to the article and approved the submitted version.
Funding
This work was supported by internal project PIB22-3 of the Universidad Autonoma de Aguascalientes. DGM was supported by CONACYT CVU 1091197.
Conflict of interest
The authors declare that the research was conducted in the absence of any commercial or financial relationships that could be construed as a potential conflict of interest.
Publisher's note
All claims expressed in this article are solely those of the authors and do not necessarily represent those of their affiliated organizations, or those of the publisher, the editors and the reviewers. Any product that may be evaluated in this article, or claim that may be made by its manufacturer, is not guaranteed or endorsed by the publisher.
References
1. Rajan S, Hudedamani U. Genetic resources of guava: importance, uses and prospects. In:Rajasekharan PE, Ramanatha Rao V, , editors. Conservation and Utilization of Horticultural Genetic Resources. Singapore: Springer (2019). p. 363–83. doi: 10.1007/978-981-13-3669-0_11
2. Hussain SZ, Naseer B, Qadri T, Fatima T, Bhat TA. Fruits Grown in Highland Regions of the Himalayas. Cham: Springer International Publishing (2021).
3. Bouchoukh I, Hazmoune T, Boudelaa M, Bensouici C, Zellagui A. Anticholinesterase antioxidant activities of foliar extract from a tropical species: Psidium guajava L. (Myrtaceae) grown in Algeria. Curr Issues Pharm Med Sci. (2019) 32:160–7. doi: 10.2478/cipms-2019-0029
4. Mendes-Pereira F, Usman M, Mayer N, Costa-Nachtigal J, Mbongeni-Maphanga R, Willemse S. Advances in guava propagation. Rev Bras Frutic. (2016) 39. doi: 10.1590/0100-29452017358
5. Landrum LR, Mitra S. Psidium guajava L.: taxonomy, relatives and possible origin. In: Guava: Botany, Production and Uses. CABI (2021).
6. Angulo-López JE, Flores-Gallegos AC, Torres-León C, Ramírez-Guzmán KN, Martínez GA, Aguilar CN. Guava (Psidium guajava L.) fruit and valorization of industrialization by-products. Processes. (2021) 9:1075. doi: 10.3390/pr9061075
7. Sánchez E, Mora Newcomer E, Barrantes Santamaría W. Aislamiento de ADN de alta calidad en Psidium guajava L. para estudios genómicos. Agron Mesoam. (2021) 32:638–49. doi: 10.15517/am.v32i2.41606
8. González-Arias S, Zúñiga-Moreno A, García-Morales R, Elizalde-Solis O, Verónico-Sánchez FJ, Flores-Valle SO. Gasification of Psidium guajava L. Waste using supercritical water: evaluation of feed ratio and moderate temperatures. Energies. (2021) 14:2555. doi: 10.3390/en14092555
9. Kuila A, Mukhopadhyay M. Biorefinery Production Technologies for Chemicals and Energy. Kuila A, Mukhopadhyay M, editors. Hoboken, NJ: Wiley (2020).
10. Lim SY, Tham PY, Lim HYL, Heng WS, Chang YP. Potential functional byproducts from guava purée processing. J Food Sci. (2018) 83:1522–32. doi: 10.1111/1750-3841.14155
11. Khalid M, Sajad A, Shabir A. Handbook of Fruit Wastes and By-Products: Chemistry, Processing Technology, and Utilization. 1st edition. Boca Raton, FL: CRC Press (2022).
12. Gutiérrez RMP, Mitchell S, Solis RV. Psidium guajava: a review of its traditional uses, phytochemistry and pharmacology. J Ethnopharmacol. (2008) 117:1–27. doi: 10.1016/j.jep.2008.01.025
13. Alonso-Castro A, Jose Maldonado-Miranda J, Zarate-Martinez A, Jacobo-Salcedo M, del R, Fernández-Galicia C, et al. Medicinal plants used in the huasteca potosina, México. J Ethnopharmacol. (2012) 143:292–8. doi: 10.1016/j.jep.2012.06.035
14. Juárez-Vázquez MC, Carranza-Álvarez C, Alonso-Castro AJ, González-Alcaraz VF, Bravo-Acevedo E, Chamarro-Tinajero FJ, et al. Ethnobotany of medicinal plants used in xalpatlahuac, guerrero, México. J Ethnopharmacol. (2013) 148:521–7. doi: 10.1016/j.jep.2013.04.048
15. Omayio DG, Abong GO, Okoth MW, Gachuiri CK, Mwang'ombe AW. Current status of guava (Psidium guajava L.) production, utilization, processing and preservation in Kenya: a review. Cur Agric Res J. (2019) 7:318–31. doi: 10.12944/CARJ.7.3.07
16. Borah A, Pandey SK, Haldar S, Lal M. Chemical composition of leaf essential oil of Psidium guajava L. from North East India. J Essent Oil Bear Plants. (2019) 22:248–53. doi: 10.1080/0972060X.2019.1574213
17. Barbalho SM, Farinazzi-Machado FM, de Alvares Goulart R, Brunnati ACS, Otoboni AM, Ottoboni JMAP, et al. Psidium guajava (guava): a plant of multipurpose medicinal applications. Med Aromat Plants. (2012) 1:1–6. doi: 10.4172/2167-0412.1000104
18. Díaz-de-Cerio E, Verardo V, Gómez-Caravaca A, Fernández-Gutiérrez A, Segura-Carretero A. Health effects of Psidium guajava L. leaves: an overview of the last decade. Int J Mol Sci. (2017) 18:897. doi: 10.3390/ijms18040897
19. Guzmán H, Díaz-Huacuz RS, González-Chavira M. Plantas Medicinales, La Realidad De Una Tradición Ancestral. Celaya: Instituto Nacional de Investigaciones Forestales Agrícolas y Pecuaria (2017).
20. Rivera-Arce E, Chávez-Soto MA, Gattuso M, Lozoya-Legorreta X. La hoja del guayabo en el tratamiento de afecciones gastrointestinales. Rev Fitoterapia. (2003) 3:101–11.
21. Fonseca-Chávez RE, Rivera-Levario LA, Vázquez-García L. Guía Ilustrada de Plantas Medicinales en el Valle de México. Meixico: Instituto Nacional de los Pueblos Indígenas (2020).
22. Leonti M, Fernando RR, Sticher O, Heinrich M. Medicinal flora of the Popoluca, Mexico: a botanical systematical perspective. Econ Bot. (2003) 57:218–30. doi: 10.1663/0013-0001(2003)057[0218:MFOTPM]2.0.CO;2
23. Servicio de Información Agroalimentaria y Pesquera. El Poder de la Guayaba. (2019). Available online at: https://www.gob.mx/siap/articulos/el-poder-de-la-guayaba (accessed May 5, 2021).
24. SIACON NG. Información Agroalimentaria y Pesquera S. (2021). Available online at: https://www.gob.mx/siap/documentos/siacon-ng-161430 (accessed February 15, 2021).
25. Quintero Ramirez JM, Omaña Silvestre JM, Sangerman-Jarquín D. Modelo de optimización para la distribución de guayaba de México hacia Estados Unidos de América. Rev Mex De Cienc Agric. (2019) 10:1709–20. doi: 10.29312/remexca.v10i8.2151
26. Morales-Troncoso C. La exportación de Guayaba Mexicana… Un Incipiente Agronegocio Con Excelentes Posibilidades. (2009). Available online at: http://fcaenlinea1.unam.mx/anexos/1526/1526_u11_act1 (accessed February 16, 2021).
27. Marcas.GenommaLab. Es QG5 para ti? (2022). Available online at: https://marcas.genommalab.com/qg5/es-qg5-para-ti?hsLang=es (accessed March 23, 2022).
28. Millones-Gómez PA, Maurtua-Torres D, Bacilio-Amaranto R, Calla-Poma RD, Requena-Mendizabal MF, Valderrama-Negron AC, et al. Antimicrobial activity and antiadherent effect of peruvian Psidium guajava (guava) leaves on a cariogenic biofilm model. J Contemp Dent Pract. (2020) 21:733–40. doi: 10.5005/jp-journals-10024-2893
29. Lavola A, Salonen A, Virjamo V, Julkunen-Tiitto R. Phytochemical variation in the plant-part specific phenols of wild crowberry (Empetrum hermaphroditum Hagerup) populations. Phytochem Lett. (2017) 21:11–20. doi: 10.1016/j.phytol.2017.05.016
30. Hardege J. Chemical ecology. In: Encyclopedia of Life Support Systems; Earth and Atmospheric. EOLSS Publications (2009).
31. Shabbir H, Kausar T, Noreen S, Rehman H, Hussain A, Huang Q, et al. In vivo screening and antidiabetic potential of polyphenol extracts from guava pulp, seeds and leaves. Animals. (2020) 10:1714. doi: 10.3390/ani10091714
32. Adrian JAL, Arancon NQ, Mathews BW, Carpenter JR. Mineral composition and soil-plant relationships for common guava (Psidium guajava L.) and yellow strawberry guava (Psidium cattleianum var. lucidum) tree parts and fruits. Commun Soil Sci Plant Anal. (2015) 46:1960–79. doi: 10.1080/00103624.2015.1069310
33. Thomas LAT, Anitha T, Lasyaja AB, Suganya M, Gayathri P, Chithra S. Biochemical and mineral analysis of the undervalued leaves–Psidium guajava L. Int J Adv Sci Res. (2017) 2:16–21.
34. Gilliham M, Dayod M, Hocking BJ, Xu B, Conn SJ, Kaiser BN, et al. Calcium delivery and storage in plant leaves: exploring the link with water flow. J Exp Bot. (2011) 62:2233–50. doi: 10.1093/jxb/err111
35. Barker AV, Pilbeam DJ. Handbook of Plant Nutrition. 2nd edition. CRC Press (2015). doi: 10.1201/b18458
36. Mengel K, Kikby EA. Principios de Nutrición Vegetal. Suiza: Instituto Internacional de la Potasa (2000).
37. Kumar M, Tomar M, Amarowicz R, Saurabh V, Nair MS, Maheshwari C, et al. Guava (Psidium guajava L.) leaves: nutritional composition, phytochemical profile, health-promoting bioactivities. Foods. (2021) 10:752. doi: 10.3390/foods10040752
38. Díaz-de-Cerio E, Verardo V, Gómez-Caravaca A, Fernández-Gutiérrez A, Segura-Carretero A. Exploratory characterization of phenolic compounds with demonstrated anti-diabetic activity in guava leaves at different oxidation states. Int J Mol Sci. (2016) 17:699. doi: 10.3390/ijms17050699
39. Bezerra CF, Rocha JE, Nascimento Silva MK, de Freitas TS, de Sousa AK, dos Santos ATL, et al. Analysis by UPLC-MS-QTOF and antifungal activity of guava (Psidium guajava L). Food Chem Toxicol. (2018) 119:122–32. doi: 10.1016/j.fct.2018.05.021
40. Silva EAJ, Estevam EBB, Silva TS, Nicolella HD, Furtado RA, Alves CCF, et al. Antibacterial and antiproliferative activities of the fresh leaf essential oil of Psidium guajava L. (Myrtaceae). Braz J Biol. (2019) 79:697–702. doi: 10.1590/1519-6984.189089
41. Soliman FM, Fathy MM, Salama MM, Saber FR. Comparative study of the volatile oil content and antimicrobial activity of Psidium guajava L. and Psidium cattleianum sabine leaves. Bull Fac Pharm Cairo Univer. (2016) 54:219–25. doi: 10.1016/j.bfopcu.2016.06.003
42. Shaheena S, Chintagunta AD, Dirisala VR, Sampath Kumar NS. Extraction of bioactive compounds from Psidium guajava and their application in dentistry. AMB Express. (2019) 9:208. doi: 10.1186/s13568-019-0935-x
43. Kapoor S, Gandhi N, Tyagi SK, Kaur A, Mahajan BVC. Extraction and characterization of guava seed oil: a novel industrial byproduct. LWT. (2020) 132:109882. doi: 10.1016/j.lwt.2020.109882
44. dos Santos RM, Costa G, Cerávolo IP, Dias-Souza MV. Antibiofilm potential of Psidium guajava and Passiflora edulis pulp extracts against Staphylococcus aureus, cytotoxicity, and interference on the activity of antimicrobial drugs. Fut J Pharm Sci. (2020) 6:48. doi: 10.1186/s43094-020-00056-8
45. Sherazi STH, Mahesar SA, Arain A, Uddin S. Guava (Psidium guajava) oil. In:Ramadan MF, , editor. Fruit Oils: Chemistry and Functionality. Cham: Springer International Publishing (2019). p. 541–59. doi: 10.1007/978-3-030-12473-1_27
46. Fernandes CC, Rezende JL, Silva EAJ, Silva FG, Stenico L, Crotti AEM, et al. Chemical composition and biological activities of essential oil from flowers of Psidium guajava (Myrtaceae). Braz J Biol. (2021) 81:728–36. doi: 10.1590/1519-6984.230533
47. Liu X, Yan X, Bi J, Liu J, Zhou M, Wu X, et al. Determination of phenolic compounds and antioxidant activities from peel, flesh, seed of guava (Psidium guajava L.). Electrophoresis. (2018) 39:1654–62. doi: 10.1002/elps.201700479
48. Bensid A, El Abed N, Houicher A, Regenstein JM, Özogul F. Antioxidant and antimicrobial preservatives: properties, mechanism of action and applications in food – a review. Crit Rev Food Sci Nutr. (2022) 62:2985–3001. doi: 10.1080/10408398.2020.1862046
49. De Souza WFC, de Lucena FA, de Castro RJS, de Oliveira CP, Quirino MR, Martins LP. Exploiting the chemical composition of essential oils from Psidium cattleianum and Psidium guajava and its antimicrobial and antioxidant properties. J Food Sci. (2021) 86:4637–49. doi: 10.1111/1750-3841.15889
50. Jassal K, Kaushal S. Phytochemical and antioxidant screening of guava (Psidium guajava) leaf essential oil. Agric Res J. (2019) 56:528. doi: 10.5958/2395-146X.2019.00082.6
51. Maffei ME. Plant natural sources of the endocannabinoid (E)-β-caryophyllene: a systematic quantitative analysis of published literature. Int J Mol Sci. (2020) 21:6540. doi: 10.3390/ijms21186540
52. Moo CL, Yang SK, Osman MA, Yuswan MH, Loh JY, Lim WM, et al. Antibacterial activity and mode of action of β-caryophyllene on. Polish J Microbiol. (2020) 69:49–54. doi: 10.33073/pjm-2020-007
53. Sampath Kumar NS, Sarbon NM, Rana SS, Chintagunta AD, Prathibha S, Ingilala SK, et al. Extraction of bioactive compounds from Psidium guajava leaves and its utilization in preparation of jellies. AMB Express. (2021) 11:36. doi: 10.1186/s13568-021-01194-9
54. Murthy N, Bapat A. Bioactive Compounds in Underutilized Fruits and Nuts. Murthy HN, Bapat AV, editors. Cham: Springer International Publishing (2020). doi: 10.1007/978-3-030-06120-3
55. Bae J, Kim N, Shin Y, Kim SY, Kim J. Activity of catechins and their applications. Biomed Dermatol. (2020) 4:8. doi: 10.1186/s41702-020-0057-8
56. Evtyugin DD, Magina S, Evtuguin D. Recent advances in the production and applications of ellagic acid and its derivatives. A review. Molecules. (2020) 25:2745. doi: 10.3390/molecules25122745
57. Mendes de Lacerda Leite G, de Oliveira Barbosa M, Pereira Lopes MJ, de Araújo Delmondes G, Bezerra DS, Araújo IM, et al. Pharmacological and toxicological activities of α-humulene and its isomers: a systematic review. Trends Food Sci Technol. (2021) 115:255–74. doi: 10.1016/j.tifs.2021.06.049
58. Chen S-P, Su C-H, Huang-Liu R, Lee M-W, Chinag C-Y, Chen W-Y, et al. Protective effect of nerolidol on lipopolysaccharide-induced acute lung injury through the inhibition of NF-?B activation by the reduction of p38 MAPK and JNK phosphorylation. J Func Food. (2020) 69:103943. doi: 10.1016/j.jff.2020.103943
59. Salehi B, Upadhyay S, Erdogan Orhan I, Kumar Jugran ALD, Jayaweera SA, Dias D, et al. Therapeutic potential of α- and β-pinene: a miracle gift of nature. Biomolecules. (2019) 9:738. doi: 10.3390/biom9110738
60. Vijayakumar K, Manigandan V, Jeyapragash D, Bharathidasan V, Anandharaj B, Sathya M. Eucalyptol inhibits biofilm formation of Streptococcus pyogenes and its mediated virulence factors. J Med Microbiol. (2020) 69:1308–18. doi: 10.1099/jmm.0.001253
61. Ortega JT, Estrada O, Serrano ML, Contreras W, Orsini G, Pujol FH, et al. Glycosylated flavonoids from psidium guineense as major inhibitors of HIV-1 replication in vitro. Nat Prod Commun. (2017) 12:1934578X1701200. doi: 10.1177/1934578X1701200712
62. Prabu GR, Gnanamani A, Sadulla S. Guaijaverin – a plant flavonoid as potential antiplaque agent against Streptococcus mutans. J Appl Microbiol. (2006) 101:487–495. doi: 10.1111/j.1365-2672.2006.02912.x
63. Kamatou GPP, Viljoen AM. A review of the application and pharmacological properties of α -bisabolol and α -bisabolol-rich oils. J Am Oil Chem Soc. (2010) 87:1–7. doi: 10.1007/s11746-009-1483-3
64. Chang ST, Wang SY, Kuo YH. Resources and bioactive substances from Taiwania (Taiwania cryptomerioides). J Wood Sci. (2003) 49:0001–4. doi: 10.1007/s100860300000
65. López-Jácome LE, Fernández-Rodríguez D, Franco-Cendejas R, Camacho-Ortiz A, Morfin-Otero MDR, Rodríguez-Noriega E, et al. Increment antimicrobial resistance during the COVID-19 pandemic: results from the invifar network. Microbiol Drug Resistance. (2022) 28:338–45. doi: 10.1089/mdr.2021.0231
66. Pandey M, Qidwai A, Kumar R, Pandey A, Shukla SK, Pathak A, et al. pharmacological and antibacterial aspect of Psidium guajava L. against acne vulgaris. Int J Pharm Sci Res. (2017) 8:145. doi: 10.13040/IJPSR.0975-8232.8(1).145-50
67. Festus FI, Goodness OO, Temilade OP. Effect of different extraction solvents on the antimicrobial activity of Psidium guajava (guava) leaves against multi-drug resistant bacteria implicated in nosocomial infections. Int J Biotechnol. (2020) 9:24–37. doi: 10.18488/journal.57.2020.91.24.37
68. Hemeg HA, Moussa IM, Ibrahim S, Dawoud TM, Alhaji JH, Mubarak AS, et al. Antimicrobial effect of different herbal plant extracts against different microbial population. Saudi J Biol Sci. (2020) 27:3221–7. doi: 10.1016/j.sjbs.2020.08.015
69. Dutta K, Karmakar A, Jana D, Ballav S, Shityakov S, Panda AK, et al. Benzyl isocyanate isolated from the leaves of Psidium guajava inhibits Staphylococcus aureus biofilm formation. Biofouling. (2020) 36:1000–17. doi: 10.1080/08927014.2020.1842877
70. Vasavi HS, Arun AB, Rekha PD. Anti-quorum sensing activity of Psidium guajava L. flavonoids against Chromobacterium violaceum and Pseudomonas aeruginosa PAO1. Microbiol Immunol. (2014) 58:286–93. doi: 10.1111/1348-0421.12150
71. Lakshmana Prabu S, Umamaheswari A, Grace Felciya SJ. Investigation on the biofilm eradication potential of selected medicinal plants against methicillin-resistant Staphylococcus aureus. Biotechnol Rep. (2020) 28:e00523. doi: 10.1016/j.btre.2020.e00523
72. Asma ST, Imre K, Morar A, Herman V, Acaroz U, Mukhtar H, et al. An overview of biofilm formation–combating strategies and mechanisms of action of antibiofilm agents. Life. (2022) 12:1110. doi: 10.3390/life12081110
73. Cáceres A, Cáceres S. Principales plantas medicinales disponibles en guatemala con actividad contra virus respiratorios que infectan al ser humano – revisión narrativa. Ciencia Tecnol Salud. (2020) 7:412–41. doi: 10.36829/63CTS.v7i3.978
74. Sriwilaijaroen N, Fukumoto S, Kumagai K, Hiramatsu H, Odagiri T, Tashiro M, et al. Antiviral effects of Psidium guajava Linn. (guava) tea on the growth of clinical isolated H1N1 viruses: its role in viral hemagglutination and neuraminidase inhibition. Antiviral Res. (2012) 94:139–46. doi: 10.1016/j.antiviral.2012.02.013
75. Ishimine RG, Vega JR, Loza MGL. Plantas medicinales antivirales: una revisión enfocada en el COVID-19. Med Nat. (2021) 15:38–45.
76. Sharma Y, Kawatra A, Sharma V, Dhull D, Kaushik S, Yadav JP, et al. In-vitro and in-silico evaluation of the anti-chikungunya potential of Psidium guajava leaf extract and their synthesized silver nanoparticles. Virus Dis. (2021) 32:260–65. doi: 10.1007/s13337-021-00685-4
77. Vista FES, Dalmacio LMM, Corales LGM, Salem GM, Galula JU, Chao, et al.-Y. Antiviral effect of crude aqueous extracts from ten philippine medicinal plants against zika virus. Acta Medica Philippina. (2020) 54:1501. doi: 10.47895/amp.v54i2.1501
78. Trujillo-Correa AI, Quintero-Gil DC, Diaz-Castillo F, Quiñones W, Robledo SM, Martinez-Gutierrez M. In vitro and in silico anti-dengue activity of compounds obtained from Psidium guajava through bioprospecting. BMC Complement Alternat Med. (2019) 19:298. doi: 10.1186/s12906-019-2695-1
79. Shin JA, Oh S, Jeong JM. The potential of BEN815 as an anti-inflammatory, antiviral and antioxidant agent for the treatment of COVID-19. Phytomed Plus. (2021) 1:100058. doi: 10.1016/j.phyplu.2021.100058
80. Efenberger-Szmechtyk M, Nowak A, Czyzowska A. Plant extracts rich in polyphenols: antibacterial agents and natural preservatives for meat and meat products. Crit Rev Food Sci Nutr. (2021) 61:149–78. doi: 10.1080/10408398.2020.1722060
81. Guimarães AC, Meireles LM, Lemos MF, Guimarães MCC, Endringer DC, Fronza M, et al. Antibacterial activity of terpenes and terpenoids present in essential oils. Molecules. (2019) 24:2471. doi: 10.3390/molecules24132471
82. Takó M, Kerekes EB, Zambrano C, Kotogán A, Papp T, Krisch J, et al. Plant phenolics and phenolic-enriched extracts as antimicrobial agents against food-contaminating microorganisms. Antioxidants. (2020) 9:165. doi: 10.3390/antiox9020165
83. Mahizan NA, Yang SK, Moo CL, Song AL, Chong CM, Chong CW, et al. Terpene derivatives as a potential agent against antimicrobial resistance (AMR) pathogens. Molecules. (2019) 24:2631. doi: 10.3390/molecules24142631
84. Bakour M, Laaroussi H, Ousaaid D, El Ghouizi A, Es-Safi I, Mechchate H, et al. Bee bread as a promising source of bioactive molecules and functional properties: an up-to-date review. Antibiotics. (2022) 11:203. doi: 10.3390/antibiotics11020203
85. Rashad Y, Aseel D, Hammad S. Phenolic compounds against fungal and viral plant diseases. In:Lone R, Shuab R, Kamili AN, , editors. Plant Phenolics in Sustainable Agriculture. Singapore: Springer (2020). p. 201–19. doi: 10.1007/978-981-15-4890-1_9
86. Huerta-Reyes M, Tavera-Hernández R, Alvarado-Sansininea JJ, Jiménez-Estrada M. Selected species of the cucurbitaceae family used in mexico for the treatment of diabetes mellitus. Molecules. (2022) 27:3440. doi: 10.3390/molecules27113440
87. INEGI. Comunicado de Prensa Num. 6576/22. Estadística a Propósito del Día Mundial De La Diabetes. INEGI (2022).
88. Quiroga-Garza A, Garza-Cisneros AN, Elizondo-Omaña RE, Vilchez-Cavazos JF, de-Oca-Luna RM, Villarreal-Silva E, et al. Research barriers in the global South: Mexico. J Glob Health. (2022) 12:03032. doi: 10.7189/jogh.12.03032
89. Bahrani AHM, Zaheri H, Soltani N, Kharazmi F, Keshavarz M, Kamalinajad M. Effect of the administration of psidium guava leaves on blood glucose, lipid profiles and sensitivity of the vascular mesenteric bed to phenylephrine in streptozotocin-induced diabetic rats. J Diabetes Mellitus. (2012) 02:138–45. doi: 10.4236/jdm.2012.21023
90. Lee J, Noh S, Lim S, Kim B. Plant extracts for type 2 diabetes: from traditional medicine to modern drug discovery. Antioxidants. (2021) 10:81. doi: 10.3390/antiox10010081
91. Uuh-Narvaez JJ, Tamayo MAG, Segura-Campos MR. A study on nutritional and functional study properties of mayan plant foods as a new proposal for type 2 diabetes prevention. Food Chem. (2020) 341:128247. doi: 10.1016/j.foodchem.2020.12824
92. Quan N, Tran HD, Xuan T, Ahmad A, Dat T, Khanh T, et al. Momilactones A and B are α-amylase and α-glucosidase inhibitors. Molecules. (2019) 24:482. doi: 10.3390/molecules24030482
93. Beidokhti MN, Eid HM, Villavicencio MLS, Jäger AK, Lobbens ES, Rasoanaivo PR, et al. Evaluation of the antidiabetic potential of Psidium guajava L. (Myrtaceae) using assays for α-glucosidase, α-amylase, muscle glucose uptake, liver glucose production, and triglyceride accumulation in adipocytes. J Ethnopharmacol. (2020) 257:112877. doi: 10.1016/j.jep.2020.112877
94. Samejima H, Park BJ. Inhibition activity of guava (Psidium guajava L.) leaf extract against collagenase, elastase, hyaluronidase, and carbohydrate digestion enzymes.? Trop Agricult Develop. (2019) 63:12–7. doi: 10.11248/jsta.63.12
95. Liu CW, Wang YC, Lu HC, Chiang WD. Optimization of ultrasound-assisted extraction conditions for total phenols with anti-hyperglycemic activity from Psidium guajava leaves. Proc Biochem. (2014) 49:1601–5. doi: 10.1016/j.procbio.2014.06.009
96. Manekeng HT, Mbaveng AT, Ntyam Mendo SA, Agokeng AJD, Kuete V. Evaluation of acute and subacute toxicities of Psidium guajava methanolic bark extract: a botanical with in vitro antiproliferative potential. Evid Based Complement Alternat Med. (2019) 2019:8306986. doi: 10.1155/2019/8306986
97. Atik N, Muda I, Rahmadi AR, Achadiyani A, Djunaedi DD. Phytochemical screening and histology appearance of acute oral toxicity study on ethanol extract of Psidium guajava linn. Fruit in mice. Asian J Pharm Clin Res. (2019) 12:351. doi: 10.22159/ajpcr.2019.v12i1.30118
98. Babatola LJ, Oboh G, Ademiluyi AO. Toxicological evaluation of aqueous extract of different varieties of guava (Psidium guajava Linn) leaves. Comparat Clin Pathol. (2019) 28:1689–97. doi: 10.1007/s00580-019-03002-0
99. Bautista ML, Pomer P, Salonga JJ. Comparison of toxic effects of Psidium guajava leaf and bark extracts against brine shrimp (Artemia salina). Int J Agric Technol. (2018) 14:1033–8.
100. Ashraf A, Sarfraz RA, Rashid MA, Mahmood A, Shahid M, Noor N. Chemical composition, antioxidant, antitumor, anticancer and cytotoxic effects of Psidium guajava leaf extracts. Pharm Biol. (2016) 54:1971–81. doi: 10.3109/13880209.2015.1137604
101. Cao H, Ou J, Chen L, Zhang Y, Szkudelski T, Delmas D, et al. Dietary polyphenols and type 2 diabetes: human study and clinical trial. Crit Rev Food Sci Nutr. (2019) 59:3371–9. doi: 10.1080/10408398.2018.1492900
102. Xu C, Li X, Zeng D, Liu Y, Gao Y, Tsunoda M, et al. Amino acid profiling study of Psidium guajava L. leaves as an effective treatment for type 2 diabetic rats. Evid Based Complement Alternat Med. (2020) 2020:9784382. doi: 10.1155/2020/9784382
103. Luo Y, Peng B, Wei W, Tian X, Wu Z. Antioxidant and anti-diabetic activities of polysaccharides from guava leaves. Molecules. (2019) 24:1343. doi: 10.3390/molecules24071343
104. Eidenberger T, Selg M, Krennhuber K. Inhibition of dipeptidyl peptidase activity by flavonol glycosides of guava (Psidium guajava L.): a key to the beneficial effects of guava in type II diabetes mellitus. Fitoterapia. (2013) 89:74–9. doi: 10.1016/j.fitote.2013.05.015
105. Amos TN, Bashir L, Saba SE, Saba MA, Mohammed BM, Abdulsalam IH, et al. Phytochemicals and acute toxicity profile of aqueous and methanolic extracts of Crateva adansonii leaves in Swiss albino rats. Asian J Biochem. (2015) 10:173–9. doi: 10.3923/ajb.2015.173.179
106. Colerangle JB. Preclinical development of nononcogenic drugs (small and large molecules). In:Faqui As, , editor. A Comprehensive Guide to Toxicology in Nonclinical Drug Development. Elsevier (2017). p. 659–83. doi: 10.1016/B978-0-12-803620-4.00025-6
107. Sarah QS, Anny FC, Misbahuddin M. Brine shrimp lethality assay. Bangladesh J Pharmacol. (2017) 12:32796. doi: 10.3329/bjp.v12i2.32796
108. Lorenzo JM, Estévez M, Barba FJ, Thirumdas R, Franco D, Munekata PES. Polyphenols: Bioaccessibility and bioavailability of bioactive components. In:Barba FJ, Saraiva JMA, Cravatto G, Lorenzo JM, , editors. Innovative Thermal and Non-Thermal Processing, Bioaccessibility and Bioavailability of Nutrients and Bioactive Compounds. Elsevier (2019). p. 309–32. doi: 10.1016/B978-0-12-814174-8.00011-1
109. Blancas-Benitez FJ, González-Aguilar GA, Sáyago-Ayerdi SG. Guava (Psidium guajava). In:Yahia EM, , editor. Fruit and Vegetable Phytochemicals. Chichester: John Wiley & Sons, Ltd (2017). p. 1067–76. doi: 10.1002/9781119158042.ch53
110. Niaz K, Shah MA, Khan F, Saleem U, Vargas C, Panichayupakaranant P. Bioavailability and safety of phytonutrients. In: Phytonutrients in Food. Elsevier (2020). p. 117–36. doi: 10.1016/B978-0-12-815354-3.00003-4
111. Câmara JS, Albuquerque BR, Aguiar J, Corrêa RCG, Gonçalves JL, Granato D, et al. Food bioactive compounds and emerging techniques for their extraction: polyphenols as a case study. Foods. (2020) 10:37. doi: 10.3390/foods10010037
112. Vasconcelos AG, Barros ALA0N, Cabral WF, Moreira DC, da Silva IGM, Silva Carvalho AE, et al. Promising self-emulsifying drug delivery system loaded with lycopene from red guava (Psidium guajava L.): in vivo toxicity, biodistribution and cytotoxicity on DU-145 prostate cancer cells. Cancer Nanotechnol. (2021) 12:30. doi: 10.1186/s12645-021-00103-w
113. Birdi T, Krishnan GG, Kataria S, Gholkar M, Daswani P. A randomized open label efficacy clinical trial of oral guava leaf decoction in patients with acute infectious diarrhoea. J Ayurveda Integrat Med. (2020) 11:163–72. doi: 10.1016/j.jaim.2020.04.001
114. Nayak N, Varghese J, Shetty S, Bhat V, Durgekar T, Lobo R, et al. Evaluation of a mouthrinse containing guava leaf extract as part of comprehensive oral care regimen- a randomized placebo-controlled clinical trial. BMC Complement Alternati Med. (2019) 19:327. doi: 10.1186/s12906-019-2745-8
115. Amaliya A, Risdiana AS, van der Velden U. Effect of guava and vitamin C supplementation on experimental gingivitis: a randomized clinical trial. J Clin Periodontol. (2018) 45:959–967. doi: 10.1111/jcpe.12922
116. Pongsakornpaisan P, Lourith N, Kanlayavattanakul M. Anti-sebum efficacy of guava toner: a split-face, randomized, single-blind placebo-controlled study. J Cosmet Dermatol. (2019) 18:1737–41. doi: 10.1111/jocd.12943
117. König A, Schwarzinger B, Stadlbauer V, Lanzerstorfer P, Iken M, Schwarzinger C, et al. Guava (Psidium guajava) fruit extract prepared by supercritical CO2 extraction inhibits intestinal glucose resorption in a double-blind, randomized clinical study. Nutrients. (2019) 11:1512. doi: 10.3390/nu11071512
Keywords: guava, extracts, antimicrobial, phenolic compounds, biofilm, byproducts recovery
Citation: Gutierrez-Montiel D, Guerrero-Barrera AL, Chávez-Vela NA, Avelar-Gonzalez FJ and Ornelas-García IG (2023) Psidium guajava L.: From byproduct and use in traditional Mexican medicine to antimicrobial agent. Front. Nutr. 10:1108306. doi: 10.3389/fnut.2023.1108306
Received: 01 December 2022; Accepted: 02 January 2023;
Published: 24 January 2023.
Edited by:
Mohamed Fawzy Ramadan, Umm al-Qura University, Saudi ArabiaReviewed by:
Dejan S. Stojkovic, Siniša Stanković Institute for Biological Research, University of Belgrade, SerbiaHassan Laaroussi, Sidi Mohamed Ben Ab-dellah University, Morocco
Copyright © 2023 Gutierrez-Montiel, Guerrero-Barrera, Chávez-Vela, Avelar-Gonzalez and Ornelas-García. This is an open-access article distributed under the terms of the Creative Commons Attribution License (CC BY). The use, distribution or reproduction in other forums is permitted, provided the original author(s) and the copyright owner(s) are credited and that the original publication in this journal is cited, in accordance with accepted academic practice. No use, distribution or reproduction is permitted which does not comply with these terms.
*Correspondence: Alma L. Guerrero-Barrera, alguerre@correo.uaa.mx