- 1School of Medicine, Federal University of Uberlandia (UFU), Uberlandia, Minas Gerais, Brazil
- 2School of Science and the Environment, University of Worcester, Worcester, United Kingdom
Omega-3 polyunsaturated fatty acids (n-3 PUFA) play a significant role in the prevention and management of cardiometabolic diseases associated with a mild chronic pro-inflammatory background, including type 2 diabetes, hypertension, hypertriglyceridaemia, and fatty liver disease. The effects of n-3 PUFA supplements specifically, remain controversial regarding reducing risks of cardiovascular events. n-3 PUFA supplements come at a cost for the consumer and can result in polypharmacy for patients on pharmacotherapy. Sardines are a well-known, inexpensive source of n-3 PUFA and their consumption could reduce the need for n-3 PUFA supplementation. Moreover, sardines contain other cardioprotective nutrients, although further insights are crucial to translate a recommendation for sardine consumption into clinical practice. The present review discusses the matrix of nutrients contained in sardines which confer health benefits for cardiometabolism, beyond n-3 PUFA. Sardines contain calcium, potassium, magnesium, zinc, iron, taurine, arginine and other nutrients which together modulate mild inflammation and exacerbated oxidative stress observed in cardiovascular disease and in haemodynamic dysfunction. In a common serving of sardines, calcium, potassium, and magnesium are the minerals at higher amounts to elicit clinical benefits, whilst other nutrients are present in lower but valuable amounts. A pragmatic approach towards the consumption of such nutrients in the clinical scenario should be adopted to consider the dose–response relationship effects on physiological interactions. As most recommendations currently available are based on an indirect rationale of the physiological actions of the nutrients found in sardines, randomised clinical trials are warranted to expand the evidence on the benefits of sardine consumption.
1. Introduction
Omega-3 polyunsaturated fatty acids (n-3 PUFA) are recognised as functional nutrients with multifaceted effects in preventing and managing cardiometabolic diseases with pro-inflammatory backgrounds, such as type 2 diabetes (T2D), hypertension, hypertriglyceridaemia, and fatty liver disease (1–5). Extensive research conducted in the past decades has confirmed the beneficial effects of fish oil supplementation as a source of n-3 PUFA, mainly eicosapentaenoic acid (EPA) and docosahexaenoic acid (DHA) (6–9).
Long-term adherence to fish oil supplementation however is currently a matter for debate. With large segments of the general population, particularly older age groups, relying on prescribed medication for a range of health conditions, such a valuable nutritional approach when mismanaged can result in polypharmacy (10–13).
The proposed therapeutic dosage for fish oil supplementation (EPA + DHA ≥ 3 g/d) can lead to a higher intake of capsules, posing a challenge in a chronic treatment plan. Moreover, side-effects including eructation related to fishy taste and nausea are common (14). The gastric capacity of the individual cannot be neglected; as a sufficient volume of water must be ingested with the capsules to allow efficient gastric mixture, aged patients and those suffering from gastroesophageal reflux disease may experience difficulty in complying with the ideal dosage both on fasting or postprandially.
A range of foodstuffs are important sources of n-3 PUFA, but unlike fish oil supplementation, an impasse remains when translating therapeutic n-3 PUFA dosage to actual food intake due to the broad range and varied concentration across dietary sources of n-3 PUFA (15).
Oily fish is the most relevant dietary source of n-3 PUFA (16, 17); however, the n-3 PUFA content substantially varies depending on the fish (18). Sardines are an inexpensive type of fish with high n-3 PUFA content (19, 20). Moreover, sardines are a source of many essential nutrients, including minerals, vitamins and amino acids, whose considerable amounts may improve clinical outcomes in patients affected by cardiometabolic diseases (21, 22).
Guidelines suggest sardine consumption as a source of dietary n-3 PUFA; nevertheless, most evidence available is based on observational studies (23, 24). Despite a lack of scientific robustness, a few randomised clinical trials (RCTs) investigating the effects of sardine consumption have been published recently (25, 26), but the need to infer the current clinical applicability for practitioners remains. The aims of the present study were (1) to appraise existing RCTs that have investigated sardine consumption, providing an indirect comparison with other dietary sources of n-3 PUFA, in order to draw pragmatic conclusions, and (2) to expand the clinical rationale related to functional nutrients contained in sardines that can elicit cardiovascular benefits.
2. Micronutrients from sardines in cardiovascular disease
Sardines are not only a relevant source of n-3 PUFA, but also a source of calcium, presenting a higher amount of both nutrients compared to other fish (Table 1). The calcium content in 100 g of sardines is equivalent to the amount found in ~ 400 ml of milk (27), thus being an alternative for individuals who cannot tolerate dairy products or milk at that volume, and also an option for those in need of complementing their total calcium intake in general. More specifically, 100 g sardines offer 382 mg/d of calcium, which accounts for 38% of the recommended dietary allowance (RDA) (1,000 mg/d in general) (28).
Calcium is a fundamental nutrient in cardiovascular biochemical pathways (30), as evidenced by its major role as signalling ion and paramount for myocardial contractility (31). Calcium is indispensable for bone health (32), and approximately 99% of the calcium bodily content is contained in bone tissue, with the remaining 1% stored in other tissues and with varied but similarly important biochemical roles. Aside from calcium, sardines are an important source of magnesium, phosphorus, and vitamin D, which collectively contribute to bone metabolism (33, 34) and are similarly important for cardiovascular health (35–37).
Sardines, as a dietary source of n-3 PUFA, have shown benefits for blood pressure (BP) and lipid profile normalisation (38, 39). Moreover, sardines are a source of potassium, magnesium, and zinc, which are nutrients with observed BP lowering properties, as well as niacin and zinc, which in turn are candidates for improving circulating levels of lipids and lipoproteins (40–44).
Given that the overall dietary potassium intake corresponds to 1.6 to 5.9 g/d (45), sardines can be considered a relevant source of potassium, at 490 mg in a 100 g serving (Table 1). Adequate potassium intake can not only normalise elevated BP by increasing natriuresis when the cause of hypertension is high sodium levels, it also favours optimal endothelial function and nitric oxide release (46). Furthermore, endothelial hyperpolarization induced by the cell membrane potassium channels decreases cytosolic smooth muscle cell calcium, with subsequent vasodilatation induction and ultimately decreasing BP (46). Through similar biochemical actions, potassium can attenuate sympathetic activity, triggering vascular muscle relaxation and hence lowering elevated BP (46). Considering such relevant actions, current guidelines consider the increase in dietary potassium as a Grade A recommendation to reduce BP (47). A dose–response meta-analysis found an inverse association between potassium intake and stroke risk at a dose of ~ 3,500 mg/d, which may be reached by a balanced diet (48). Overall, sardines can be deemed as a food candidate to contribute to optimal dietary potassium intake (49).
Sardines contain a considerable amount of iron (2.9 mg/100 g), higher than other commonly consumed types of fish (Table 1). Sardine iron content is comparable to that of meat, the most popular source of iron worldwide (50). Thus, sardine consumption is an option to reach the RDA for iron, 8 mg/d for all age groups (51), and particularly helpful for those who do not eat meat. Given the well-established association between iron-deficiency anaemia and cardiovascular diseases, iron intake should not be neglected when managing cardiovascular patients. Iron deficiency is a contributing factor for negative outcomes in patients with coronary artery disease, heart failure and pulmonary hypertension, with the elderly and those with coexisting chronic diseases being more vulnerable (52).
Iron supplementation may be necessary in specific cases, but at the same time sardine consumption could be an ideal option to be included in the diet plan. On the other hand, excessive iron intake must be avoided as excess iron is detrimental to cardiovascular health due to its very high reactivity and increased iron-catalysed free radical-mediated oxidative stress. Iron intake derived from sardine consumption, as well as from other iron-containing foods, do not cause such undesired effects, unlike iron oral supplementation which can more easily lead to iron overload and exacerbate haemochromatosis when mismanaged (53, 54).
3. Functional amino acids across sardines vs. supplemental therapies
Sardines are an important source of amino acids, including arginine, taurine and others, which play fundamental roles in cardiometabolism, not only as structural biomolecules but also as modulators of antioxidant systems and vascular function (55–57). Regarding arginine, although it is a conditionally essential amino acid, it is vital for BP stability and general vascular health, serving as a substrate in the endothelium-derived nitric oxide synthetic pathway, thereby reducing systemic BP (58, 59). Moreover, arginine can improve renovascular hypertension via the renin-angiotensin system by increasing nitric oxide synthesis and reducing angiotensin II (60).
In general, the average intake of arginine at populational level is close to 4 g daily. For example, the mean arginine intake for the US adult population is approximately 4.4 g/d, with 25% of people consuming less than 2.6 g/d (61). In Finnish men aged 42–60 years, an average arginine intake of 3.8 mg/d was reported (62), while preliminary reporting from the Zutphen Elderly Study, a branch of the Seven Countries Study, found in Dutch men aged 64–84 years a mean arginine intake of 4.4 g/d (63). However, due to the observational nature of their design, those studies do not support the hypothesis that higher arginine intake lowers BP or coronary heart disease mortality (62, 63). Nonetheless, clinical findings suggest that arginine supplementation can reduce BP, but at an oral dosage of 4 to 24 g/d for 2 to 24 weeks, as reported in a meta-analysis of RCT describing reductions of 5.39 mmHg (95% CI −8.54 to −2.25) and 2.66 mmHg (95% CI −3.77 to −1.54) in systolic and diastolic BP, respectively (64).
Despite the lack of robust clinical findings on the relationship between arginine intake and cardiovascular outcomes, habitual sardine consumption can contribute to overall arginine intake. According to the United States Department of Agriculture (USDA) database (29), the arginine content in cooked sardines is 1.47 g/100 g (FDC ID: 175139). However, it must be noted that sardine arginine content is not higher than that of tuna (1.79 g/100 g; FDC ID: 173707), salmon (1.72 g/100 g; FDC ID: 173692) or beef (1.71 g/100 g; FDC ID: 168720) (29).
Taurine is an amino sulfonic acid with a range of biochemical roles, and it has been observed that its antioxidant activity modulates the cardiovascular system, leading to clinical benefits including BP normalisation and amelioration in lipid and glycaemic indices (65–67). Taurine itself does not scavenge reactive oxygen species such as superoxide anion, hydroxyl radical, and hydrogen peroxide (68), but 5-taurinomethyluridine, a derivative of taurine conjugation with uridine on mitochondrial tRNA, modulates mitochondrial protein synthesis and enhances electron transport chain activity (69), protecting the mitochondrion against excessive reactive oxygen and nitrogen species (70, 71). Redox modulation is favourable to blood vessels, but taurine may also lower BP and improve vascular function through additional pathways, for example suppressing renin–angiotensin–aldosterone system activity and augmenting kallikrein activity in blood and peripheral tissues (72).
Cholesterol-lowering properties have been attributed to taurine, including upregulation of the hepatic low-density lipoprotein receptor (LDLR) and facilitation of the binding of LDL particles to LDLR, as well as reduction of hepatic activity of acyl-CoA:cholesterol acyltransferase (ACAT) and enhancement of cholesterol 7alpha-hydroxylase activity (73). ACAT is a membrane-bound enzyme that utilises long-chain fatty acyl-CoA and cholesterol as substrates to synthetise cholesteryl ester, whilst cholesterol 7alpha-hydroxylase catalyses the initial step in cholesterol catabolism and bile acid synthesis (74, 75). Moreover, taurine contributes to glucose homeostasis by modulating gene expression that is essential for glucose-stimulated insulin secretion (i.e., sulfonylurea receptor-1, glucokinase, Glut-2, proconvertase, and pancreas duodenum homeobox-1) and increasing insulin-stimulated tyrosine phosphorylation of the insulin receptor in skeletal muscle and liver, consequently ameliorating peripheral insulin sensitivity and improving overall glycaemic profile (76).
Sardines contain 147 mg taurine/100 g (25), which is not higher than taurine content in tuna (332 mg), beef (344 mg), pork (489 mg), and dark meat chicken (1,355 mg)/100 g (77, 78). As expected, interventions based on a sardine-rich diet increase taurine intake when compared to control groups. For instance, adding 100 g of sardines 5 days a week to a diet plan resulted in a significant increase in taurine intake by 118.5 mg/d, whilst adding 200 g of sardine weekly increased taurine intake by 43.2 mg/d (25, 26). Although sardines are a relevant source of taurine, its content is not compatible with the supplementation dosage that is necessary to elicit clinical benefits; therefore, caution should be exercised not to extrapolate the aforementioned findings. A meta-analysis of RCTs found that the taurine dosage that triggered a mean decrease of ~3 mmHg in both SBP and DBP was 1–6 g/d for 1 day to 12 weeks (79).
4. Mercury and selenium: The ‘yin and yang’
Fish consumption is recommended as part of a healthy diet, but inherent concerns remain regarding mercury-contaminated fish and the toxicological roles of mercury in cardiovascular and neural diseases (80, 81). As a potent and seemingly irreversible inhibitor of the key antioxidant selenoenzymes, mercury hinders the actions of glutathione peroxidases and thioredoxin reductases by binding to selenocysteine at the active site of these enzymes, resulting in impaired antioxidant protection (81). The well-documented toxicological effects of mercury can be partially mitigated by selenium intake, which is incorporated to synthesize new selenoenzymes (81). If this proposed rationale is correct, it is worth noting that sardines contain considerable selenium content and, unlike tuna, are placed at a mid-trophic level, near the base of the food web (82), therefore exposed to lower mercury accumulation as compared to larger predatory fish, which are deemed to contain higher levels of methylmercury (83).
Amongst patients from two American prospective cohorts (51,529 males and 121,700 females), no clinically relevant associations were found between toenail mercury accumulation and higher risk of coronary heart disease, stroke, or total cardiovascular disease (80). Equally important, that study showed that higher fish consumption (≥ 2 servings/week) did not increase the likelihood of cardiovascular events based on toenail mercury concentrations, and that lower fish consumption (< 1 serving/week) did not reduce it (80). Such results contradict the assumption that mercury-induced cardiovascular disease is caused by general fish consumption.
The Prevención con Dieta Mediterránea (PREDIMED) trial, one of the largest prospective RCTs investigating the effects of a Mediterranean diet ever conducted, which recruited 7,477 adults at high risk for cardiovascular disease at baseline (84), showed that mercury exposure from regular fish consumption does not seem to increase cardiovascular disease risk (85). A nested case–control study examining a subpopulation (n = 147) of the PREDIMED trial who had eventually been diagnosed with cardiovascular disease along the course of the trial showed that toenail mercury concentrations detected in that subpopulation were comparable to age- and sex-matched controls (n = 267) (85).
5. The impact of sardine consumption on the omega-3 index
The omega-3 index (O3I), defined as the sum of EPA and DHA in the erythrocyte membrane, expressed as weight percentage of total fatty acids, has been proposed as a risk biomarker for coronary artery disease, particularly helpful following sudden cardiac arrest (86, 87). A meta-analysis (88), investigating 10 cohort studies found an average O3I of 6.1% ± 2.1% across the population included in the studies, with an O3I > 8% identified as protective against coronary heart disease mortality. Moreover, with median first and fifth quintiles at 4.2 vs. 8.3% respectively, the authors found that an O3I < 4% was a higher risk cut-off point (88). Other studies have proposed an O3I > 4 and < 8% as intermediate cut-off points for coronary heart disease risk, sudden cardiac death in particular (89, 90).
Results from the Canadian Health Measures Survey have shown that the average O3I in Canadians aged 20–79 years included in the survey was 4.5%, and that less than 3% of the sample population had a protective O3I of ≥ 8% (89). It is worth mentioning however that the data reported in that study was not obtained through erythrocyte membrane fatty acid chromatographic analyses, but instead indirectly via food diary analyses.
The Mediterranean Diet has traditionally been recognised as featuring moderate to high levels of n-3 PUFA, and the incidence of sudden cardiac death appears to be lower in Spain as compared to some other countries (91). Interestingly, a cross-sectional study involving 198 Spanish patients at high cardiovascular risk, with mean age 66 years and a relatively high 0.9 g/d EPA + DHA intake, showed that the mean O3I was 7.1% (86). Combined, the aforementioned studies suggest careful consideration on reaching an optimal O3I even in a population known for their habitual intake of oily fish and nuts.
A study conducted in Norway demonstrated that first-time myocardial infarction-patients without sudden cardiac arrest (n = 185) had a higher O3I compared to out-of-hospital cardiac arrest patients suffering their first myocardial infarction (n = 14; 6.48% vs. 4.59%, respectively; p = 0.002) (87). The same study showed that a 1% increase in the O3I was associated with a 58% reduction in the risk of ventricular fibrillation.
Two recent RCTs have investigated the effects of prolonged sardine consumption on T2D sufferers (25, 26). In one study, 200 g of sardine per week for 12 months resulted in a 1.3% increment in the O3I (6.6 ± 1.2 to 7.9 ± 1.3%) (25), whilst the other study found an increase of 2.7% (5.3 ± 0.3 to 8.0 ± 0.4%) when the participants consumed 100 g of sardines 5 days a week for 6 months (26). Both studies independently revealed that habitual sardine consumption can switch the O3I from intermediate to lower risk of mortality associated with coronary heart disease (25, 26).
Sardine intake appears to increase the O3I in a similar way to the intake of salmon that was grown on feeds containing mainly fish oil or rapeseed oil (92). Likewise, the O3I increase promoted by sardine consumption appears to be similar to that of low-dose fish oil supplementation (< 1 g/d) (93). However, high-dose fish oil supplementation (>4 g/d) is clearly more potent in elevating the O3I (94) (Table 2).
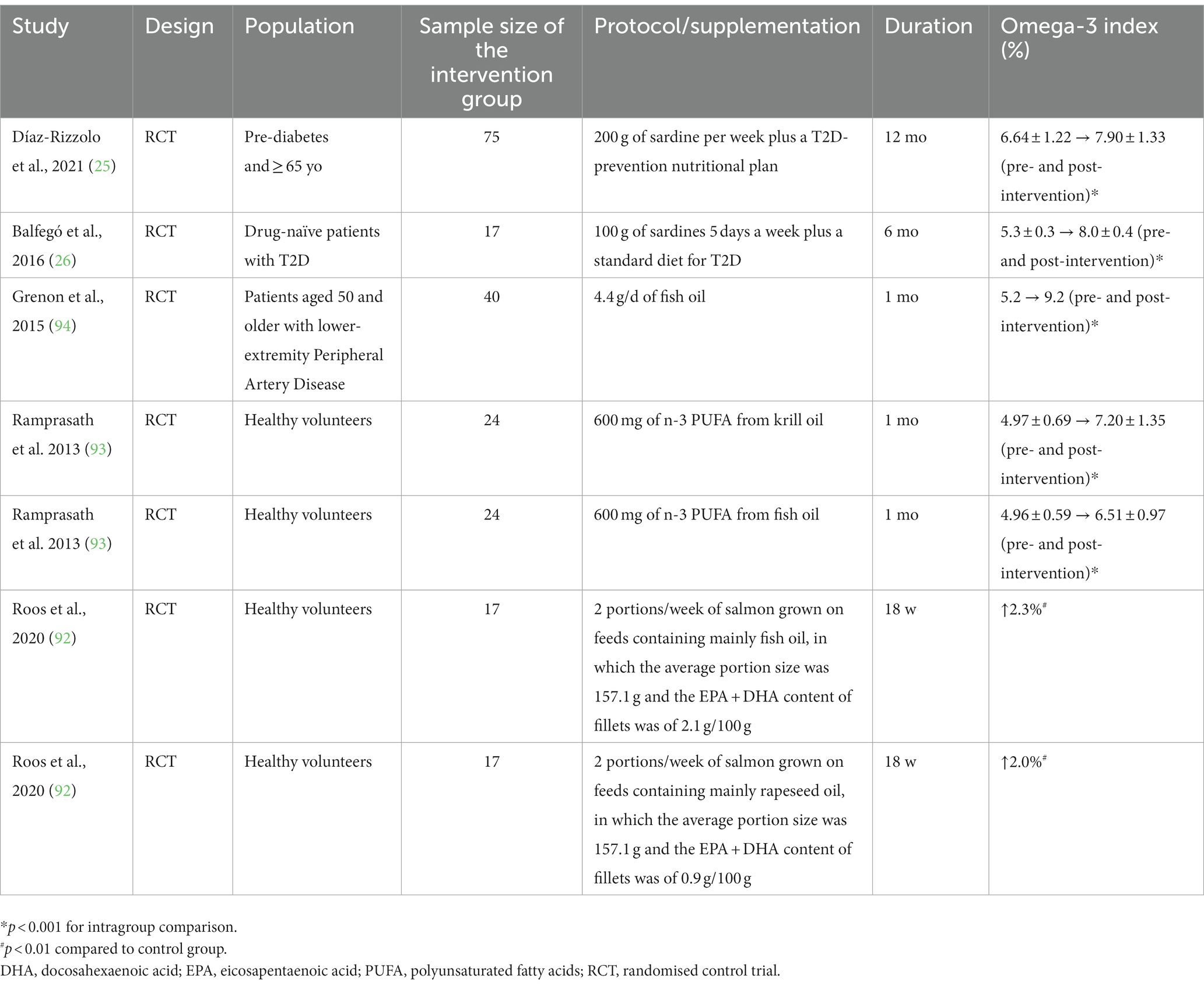
Table 2. The effects of sardine interventions compared to other sources of omega-3 (fish oil supplementation and salmon) on the omega-3 index.
6. Nourishment: Food vs. supplementation
Currently in the United Kingdom, food supplements and their use are regulated by the Department of Health and Social Care Nutrition Legislation, which employs the expertise of various agencies including the Medicines and Healthcare Products Regulatory Agency (MHRA) to ensure medicinal agents regulated by the United Kingdom are not contained in a food product, and the Food Standards Agency (FSA) which is responsible for policy on food safety, food labelling, genetically modified foods, novel foods and food hygiene (95, 96). In the United Kingdom, the governmental definition of food supplements is ‘any food the purpose of which is to supplement the normal diet and which is a concentrated source of a vitamin or mineral or other substance with a nutritional or physiological effect, alone or in combination and is sold in dose form’ (97).
In Europe supplements are regulated under the European Commission’s Directive 2002/46/EC and their definitions of supplementation align closely to those of the United Kingdom. Both bodies of legislation, in the United Kingdom and Europe, are very clear that supplements are intended to correct nutritional deficiencies and help individuals ascertain the necessary nutrients and not to be utilised as medicinal products exerting metabolic action or for the treatment or prevention of diseases (95–97).
It has been noted (98) however that society in general currently sees supplements being utilised with the intent of reducing the risk of chronic diseases such as cardiovascular disease, which is often brought on by an overconsumption of foods associated with sedentary lifestyles, rather than to treat vitamin and nutrient deficiencies as supplements had once done, with classical examples including scurvy and rickets (98–100). The consumption of food supplements to treat chronic diseases when no nutritional deficiency is present remains a matter of relevant debate.
The main reasons why most individuals consume supplements focus on maintenance or improvement of their health. Use of supplements empowers the individual, giving the sense they are actively taking measures to improve their health and longevity (98–100). It is estimated that nearly half of the population in most developed countries are taking supplements (101–103). In the United Kingdom, the 2016 Health Food Manufacturers’ Association (HFMA) National Survey reported that 59% of the adult population take food supplements (103). That was a 4% rise over the previous 2 years (102), and the Coronavirus pandemic marked an even greater increase with now over 70% of United Kingdom adults utilising supplements (103, 104).
Of those adults in the United Kingdom ingesting supplements, almost half of them (45%) consume them five times or more per week (103). Women in most countries are slightly more inclined to consume supplements than men and usage increases with age in both sexes (101–103). The consumption of n-3 PUFA and cod liver oil supplements in the UK is not as wide as multivitamin/mineral (MVM) supplements; however, 35% of the population regularly utilise them and their popularity increase with the age of the consumers (100).
In European countries, the EU Consumer Survey on Food Supplements 2022 reported that of those surveyed who had consumed supplements in the previous year, only 19% consumed n-3 PUFA or fish oils, whilst many consumed vitamin D (46%), vitamin C (36%), magnesium (33%) and MVMs (29%) (102). Of those who did consume n-3 PUFA or fish oil, 63% maintained a regular daily intake of the supplement as opposed to taking it occasionally or seasonally.
7. Sardines vs. n–3 PUFA supplements
Although sardines and oily fish in general are important sources of n-3 PUFA, patient compliance remains a challenge if n-3 PUFA intake is not followed. Interestingly, fish oil supplementation will not necessarily be the solution for all cases. A few studies have demonstrated that n-3 PUFA supplementation achieved by fish oil supplementation will not always significantly reduce the risk of major cardiovascular events, nor will it always be useful for the primary and secondary prevention of cardiovascular events, including all-cause mortality, cardiovascular mortality and cardiovascular events, coronary heart disease mortality, stroke, and arrhythmia (105, 106). A multicentre RCT recruiting 8,179 patients with confirmed cardiovascular disease or diabetes and under statin therapy found that daily supplementation with highly purified eicosapentaenoic acid ethyl ester (icosapent ethyl, Vascepa®; 4 g/d) was more effective in reducing the risk of cardiovascular events as compared to the placebo-receiving group (107). This strategy is considered a pharmacological intervention and should be preferably indicated for patients at high risk of cardiovascular disease, rather than targeted at the general population.
Whilst a more purified form of 3-PUFA along with removal of toxic contaminants is expected in fish-oil-based supplements (108), some level of oxidation is expected for n-3 PUFA in fish intake given its exposure to high temperatures, cooking and preparation methods. Both fried and baked oily fish increase bioactive oxidized n-3 PUFA products, mainly F-4 t-neuroprostanes derived from DHA (109). Cooked samples of oily fish also generate cholesterol oxidation products, in particular 7-ketocholesterol and cholestanetriol—the latter is the most cytotoxic cholesterol oxidation products (110). Moreover, cooked samples of oily fish contain more n-6 PUFA than raw samples, increasing n-6/n-3 PUFA ratios (110).
Besides cooking, the gastrointestinal system per se is another concern due to a profound effect on n-3 PUFA oxidation in both sardines and fish-oil-based supplements, limiting the n-3 PUFA bioavailability (111). Apparently however, such observed oxidation does not nullify the benefits of ingesting n-3 PUFA from fish as several studies have identified beneficial effects (112, 113). Thus, the beneficial effects of n-3 PUFA from fish consumption cannot be neglected for the overall population. Nevertheless, therapeutic strategies based on specific n-3 PUFA supplementation plans, or EPA alone in some cases, can be more useful in patients at higher risk of cardiovascular events and metabolic disease (114–118).
8. The bottom line: What is the recommendation?
According to the 2015–2020 Dietary Guidelines for Americans, 1 to 2 seafood meals per week may be included to reduce the risk of congestive heart failure, coronary heart disease, ischaemic stroke, and sudden cardiac death, especially when seafood replaces the intake of less healthy foods (23). Based on those guidelines, it is reasonable to recommend at least 1–2 servings of sardines per week aiming at cardiovascular benefits. In addition, as discussed earlier, a study observed cardiovascular benefits when participants consumed 100 g of sardines 5 days a week (26), but this amount and frequency tends to be unfeasible in the long term.
A personalised recommendation consisting of sardine consumption higher than the general guidelines of 1–2 portions weekly can be safely followed, depending on the individual’s acceptance and adherence to the diet plan. Noteworthy, a Science Advisory from the American Heart Association recommends n-3 PUFA (EPA + DHA or EPA-only) at a dose of 4 g/d as an effective and safe strategy for hypertriglyceridemia as monotherapy, or as an adjunct to other lipid-lowering drugs (119). An intake of sardines equivalent to 400 g daily would be necessary to reach this n-3 PUFA amount.
9. The issue of satiety
Whilst supplements are a convenient method of achieving a daily dose of n-3 PUFA, if not consumed with food, they are unlikely to achieve satiety the same way a portion of sardines or another oily fish would. Interestingly, several studies investigating the effects of n-3 PUFA and fish oil have demonstrated an increase in appetite in healthy and diseased individuals associated with their supplementation (99, 120, 121). Moreover, n-3 PUFA have been shown to possess orexigenic properties, increasing neuropeptide Y and decreased α-melanocyte-stimulating hormone and serotonin receptor activity in tumour bearing anorexic rats (122).
Satiation is regulated by the gut-brain axis in the form of visceral neural and blood-borne signals that reach the central nervous system to modulate orexigenic and anorexigenic responses. Mechanistically this occurs in the form of gut distension, content composition and rate of emptying, and also through chemoreceptors signalling nutrient composition by way of afferent signals via the vagus nerve to the nucleus of the solitary tract and subsequently to the lateral hypothalamic area. The consumption of supplements without food, or as a replacement for food, would fail to mechanistically modulate appetite in the same way food physically present in the alimentary canal does.
10. Conclusion
Only very few RCTs have investigated the effects of sardine consumption on human health. However, due to their nutritional composition, sardines may be considered a functional food and an adjuvant in the management of cardiometabolic diseases with a pro-inflammatory background. Sardines are an important source of n-3 PUFA in addition to components known for their cardioprotective effects, including calcium, potassium, magnesium, zinc, iron, taurine, and arginine. These nutrients in synergy are essential to modulate inflammation and oxidative stress related to the cardiovascular system and participate in cardiomyocyte and haemodynamic functions.
Notwithstanding the myriad of physiological interactions amongst micronutrients, amino acids and fatty acids discussed here, clinical wisdom must be exercised when making recommendations, as the nutrient amount across the food matrix does not have the same magnitude as therapeutic dosages (i.e., supplementation regimens).
Author contributions
HS conceptualized the idea and drafted the first version of the manuscript. HS, TM, and AB contributed to the literature review, data gathering and discussion, and manuscript writeup. All authors have read and approved the final version of this manuscript.
Funding
HS has been supported by Coordenação de Aperfeiçoamento de Pessoal de Nível Superior (CAPES), Brazil.
Conflict of interest
The authors declare that the research was conducted in the absence of any commercial or financial relationships that could be construed as a potential conflict of interest.
Publisher’s note
All claims expressed in this article are solely those of the authors and do not necessarily represent those of their affiliated organizations, or those of the publisher, the editors and the reviewers. Any product that may be evaluated in this article, or claim that may be made by its manufacturer, is not guaranteed or endorsed by the publisher.
References
1. Wu, JH, Micha, R, Imamura, F, Pan, A, Biggs, ML, Ajaz, O, et al. Omega-3 fatty acids and incident type 2 diabetes: a systematic review and meta-analysis. Br J Nutr. (2012) 107:S214–27. doi: 10.1017/S0007114512001602
2. Mori, TA . Omega-3 fatty acids and hypertension in humans. Clin Exp Pharmacol Physiol. (2006) 33:842–6. doi: 10.1111/j.1440-1681.2006.04451.x
3. Scorletti, E, and Byrne, CD. Omega-3 fatty acids and non-alcoholic fatty liver disease: evidence of efficacy and mechanism of action. Mol Asp Med. (2018) 64:135–46. doi: 10.1016/j.mam.2018.03.001
4. McKenney, JM, and Sica, D. Role of prescription omega-3 fatty acids in the treatment of hypertriglyceridemia. Pharmacotherapy. (2007) 27:715–28. doi: 10.1592/phco.27.5.715
5. Santos, HO, Price, JC, and Bueno, AA. Beyond fish oil supplementation: the effects of alternative plant sources of Omega-3 polyunsaturated fatty acids upon lipid indexes and Cardiometabolic biomarkers-an overview. Nutrients. (2020) 12:3159. doi: 10.3390/nu12103159
6. Siriwardhana, N, Kalupahana, NS, and Moustaid-Moussa, N. Health benefits of n-3 polyunsaturated fatty acids: eicosapentaenoic acid and docosahexaenoic acid. Adv Food Nutr Res. (2012) 65:211–22. doi: 10.1016/B978-0-12-416003-3.00013-5
7. Metcalf, RG, James, MJ, Gibson, RA, Edwards, JR, Stubberfield, J, Stuklis, R, et al. Effects of fish-oil supplementation on myocardial fatty acids in humans. Am J Clin Nutr. (2007) 85:1222–8. doi: 10.1093/ajcn/85.5.1222
8. Lee, JH, O'Keefe, JH, Lavie, CJ, and Harris, WS. Omega-3 fatty acids: cardiovascular benefits, sources and sustainability. Nat Rev Cardiol. (2009) 6:753–8. doi: 10.1038/nrcardio.2009.188
9. Harris, WS . Fish oil supplementation: evidence for health benefits. Cleve Clin J Med. (2004) 71:208–210, 212. doi: 10.3949/ccjm.71.3.208
10. Volpe, M, Chin, D, and Paneni, F. The challenge of polypharmacy in cardiovascular medicine. Fundam Clin Pharmacol. (2010) 24:9–17. doi: 10.1111/j.1472-8206.2009.00757.x
11. Newman, J, Grobman, WA, and Greenland, P. Combination polypharmacy for cardiovascular disease prevention in men: a decision analysis and cost-effectiveness model. Prev Cardiol. (2008) 11:36–41. doi: 10.1111/j.1520-037x.2007.06423.x
12. Louis, C, and Yannopoulos, G. The transposable elements involved in hybrid dysgenesis in Drosophila melanogaster. Oxf Surv Eukaryot Genes. (1988) 5:205–50.
13. Abolbashari, M, Macaulay, TE, Whayne, TF, Mukherjee, D, and Saha, S. Polypharmacy in cardiovascular medicine: problems and promises! Cardiovasc Hematol Agents Med Chem. (2017) 15:31–9. doi: 10.2174/1871525715666170529093442
14. Appel, LJ, Miller, ER 3rd, Seidler, AJ, and Whelton, PK. Does supplementation of diet with 'fish oil' reduce blood pressure? A meta-analysis of controlled clinical trials. Arch Intern Med. (1993) 153:1429–38. doi: 10.1001/archinte.1993.00410120017003
15. Nichols, PD, Petrie, J, and Singh, S. Long-chain omega-3 oils-an update on sustainable sources. Nutrients. (2010) 2:572–85. doi: 10.3390/nu2060572
16. Helland, A, Bratlie, M, Hagen, IV, Mjos, SA, Sornes, S, Ingvar Halstensen, A, et al. High intake of fatty fish, but not of lean fish, improved postprandial glucose regulation and increased the n-3 PUFA content in the leucocyte membrane in healthy overweight adults: a randomised trial. Br J Nutr. (2017) 117:1368–78. doi: 10.1017/S0007114517001234
17. Rajaram, S, Haddad, EH, Mejia, A, and Sabate, J. Walnuts and fatty fish influence different serum lipid fractions in normal to mildly hyperlipidemic individuals: a randomized controlled study. Am J Clin Nutr. (2009) 89:1657S–63S. doi: 10.3945/ajcn.2009.26736S
18. Torris, C, Molin, M, and Smastuen, MC. Lean fish consumption is associated with beneficial changes in the metabolic syndrome components: a 13-year follow-up study from the Norwegian Tromso study. Nutrients. (2017) 9:247. doi: 10.3390/nu9030247
19. Patin, RV, Vitolo, MR, Valverde, MA, Carvalho, PO, Pastore, GM, and Lopez, FA. The influence of sardine consumption on the omega-3 fatty acid content of mature human milk. J Pediatr. (2006) 82:63–9. doi: 10.2223/JPED.1439
20. Bispo, P, Batista, I, Bernardino, RJ, and Bandarra, NM. Preparation of triacylglycerols rich in omega-3 fatty acids from sardine oil using a Rhizomucor miehei lipase: focus in the EPA/DHA ratio. Appl Biochem Biotechnol. (2014) 172:1866–81. doi: 10.1007/s12010-013-0616-1
21. Castro Gonzalez, MI, Perez-Gil Romo, F, Carranco Jauregui, ME, Montano Benavides, S, and Silencio Barrita, JL. Vitamins and minerals from sardine in tomato sauce, from the Mexican Pacific fish zones. Arch Latinoam Nutr. (1999) 49:379–83.
22. Simat, V, Hamed, I, Petricevic, S, and Bogdanovic, T. Seasonal changes in free amino acid and fatty acid compositions of sardines, Sardina pilchardus (Walbaum, 1792): implications for nutrition. Foods. (2020) 9:867. doi: 10.3390/foods9070867
23. Rimm, EB, Appel, LJ, Chiuve, SE, Djousse, L, Engler, MB, Kris-Etherton, PM, et al. Seafood long-chain n-3 polyunsaturated fatty acids and cardiovascular disease: a science advisory from the American Heart Association. Circulation. (2018) 138:e35–47. doi: 10.1161/CIR.0000000000000574
24. Piepoli, MF, Hoes, AW, Agewall, S, Albus, C, Brotons, C, Catapano, AL, et al. 2016 European guidelines on cardiovascular disease prevention in clinical practice: the sixth joint task Force of the European Society of Cardiology and Other Societies on cardiovascular disease prevention in clinical practice (constituted by representatives of 10 societies and by invited experts)developed with the special contribution of the European Association for Cardiovascular Prevention & rehabilitation (EACPR). Eur Heart J. (2016) 37:2315–81. doi: 10.1093/eurheartj/ehw106
25. Diaz-Rizzolo, DA, Serra, A, Colungo, C, Sala-Vila, A, Siso-Almirall, A, and Gomis, R. Type 2 diabetes preventive effects with a 12-months sardine-enriched diet in elderly population with prediabetes: an interventional, randomized and controlled trial. Clin Nutr. (2021) 40:2587–98. doi: 10.1016/j.clnu.2021.03.014
26. Balfego, M, Canivell, S, Hanzu, FA, Sala-Vila, A, Martinez-Medina, M, Murillo, S, et al. Effects of sardine-enriched diet on metabolic control, inflammation and gut microbiota in drug-naive patients with type 2 diabetes: a pilot randomized trial. Lipids Health Dis. (2016) 15:78. doi: 10.1186/s12944-016-0245-0
27. Misselwitz, B, Pohl, D, Fruhauf, H, Fried, M, Vavricka, SR, and Fox, M. Lactose malabsorption and intolerance: pathogenesis, diagnosis and treatment. United European Gastroenterol J. (2013) 1:151–9. doi: 10.1177/2050640613484463
29. USDA . National Nutrient Database for standard reference. (2018). Available at: https://fdc.nal.usda.gov/ (Accessed January 20, 2022).
30. Penther, P, Boschat, J, Etienne, Y, Le Potier, J, and Gilard, M. Tight calcified aortic valve stenosis in adults aged from 50 to 69. Anatomical study of 50 cases. Arch Mal Coeur Vaiss. (1988) 81:149–55. [Les stenoses aortiques orificielles serrees et calcifiees de l'adulte de 50 a 69 ans. Etude anatomique de 50 observations.
31. Dewenter, M, von der Lieth, A, Katus, HA, and Backs, J. Calcium signaling and transcriptional regulation in Cardiomyocytes. Circ Res. (2017) 121:1000–20. doi: 10.1161/CIRCRESAHA.117.310355
32. Marks, AR . Calcium and the heart: a question of life and death. J Clin Invest. (2003) 111:597–600. doi: 10.1172/JCI18067
33. Allgrove, J . Physiology of calcium, phosphate, magnesium and vitamin D. Endocr Dev. (2015) 28:7–32. doi: 10.1159/000380990
34. Mimouni, FB, Mandel, D, Lubetzky, R, and Senterre, T. Calcium, phosphorus, magnesium and vitamin D requirements of the preterm infant. World Rev Nutr Diet. (2014) 110:140–51. doi: 10.1159/000358463
35. Gutierrez, OM . The connection between dietary phosphorus, cardiovascular disease, and mortality: where we stand and what we need to know. Adv Nutr. (2013) 4:723–9. doi: 10.3945/an.113.004812
36. Surdu, AM, Pinzariu, O, Ciobanu, DM, Negru, AG, Cainap, SS, Lazea, C, et al. Vitamin D and its role in the lipid metabolism and the development of atherosclerosis. Biomedicine. (2021) 9:172. doi: 10.3390/biomedicines9020172.
37. Severino, P, Netti, L, Mariani, MV, Maraone, A, D'Amato, A, Scarpati, R, et al. Prevention of cardiovascular disease: screening for magnesium deficiency. Cardiol Res Pract. (2019) 2019:1–10. doi: 10.1155/2019/4874921
38. Minihane, AM, Armah, CK, Miles, EA, Madden, JM, Clark, AB, Caslake, MJ, et al. Consumption of fish oil providing amounts of Eicosapentaenoic acid and Docosahexaenoic acid that can be obtained from the diet reduces blood pressure in adults with systolic hypertension: a retrospective analysis. J Nutr. (2016) 146:516–23. doi: 10.3945/jn.115.220475
39. Mori, TA, Vandongen, R, Beilin, LJ, Burke, V, Morris, J, and Ritchie, J. Effects of varying dietary fat, fish, and fish oils on blood lipids in a randomized controlled trial in men at risk of heart disease. Am J Clin Nutr. (1994) 59:1060–8. doi: 10.1093/ajcn/59.5.1060
40. Santos, HO, Teixeira, FJ, and Schoenfeld, BJ. Dietary vs. pharmacological doses of zinc: a clinical review. Clin Nutr. (2019) 39:1345–53. doi: 10.1016/j.clnu.2019.06.024
41. Santos, HO, Kones, R, Rumana, U, Earnest, CP, Izidoro, LFM, and Macedo, RCO. Lipoprotein(a): current evidence for a physiologic role and the effects of Nutraceutical strategies. Clin Ther. (2019) 41:1780–97. doi: 10.1016/j.clinthera.2019.06.002
42. Houston, MC . The importance of potassium in managing hypertension. Curr Hypertens Rep. (2011) 13:309–17. doi: 10.1007/s11906-011-0197-8
43. Zhang, X, Li, Y, Del Gobbo, LC, Rosanoff, A, Wang, J, Zhang, W, et al. Effects of magnesium supplementation on blood pressure: a meta-analysis of randomized double-blind placebo-controlled trials. Hypertension. (2016) 68:324–33. doi: 10.1161/HYPERTENSIONAHA.116.07664
44. Ganji, SH, Kamanna, VS, and Kashyap, ML. Niacin and cholesterol: role in cardiovascular disease (review). J Nutr Biochem. (2003) 14:298–305. doi: 10.1016/S0955-2863(02)00284-X
45. Lanham-New, SA, Lambert, H, and Frassetto, L. Potassium. Adv Nutr. (2012) 3:820–1. doi: 10.3945/an.112.003012
46. Ekmekcioglu, C, Elmadfa, I, Meyer, AL, and Moeslinger, T. The role of dietary potassium in hypertension and diabetes. J Physiol Biochem. (2016) 72:93–106. doi: 10.1007/s13105-015-0449-1
47. Leung, AA, Nerenberg, K, Daskalopoulou, SS, McBrien, K, Zarnke, KB, Dasgupta, K, et al. Hypertension Canada's 2016 Canadian hypertension education program guidelines for blood pressure measurement, diagnosis, assessment of risk, prevention, and treatment of hypertension. Can J Cardiol. (2016) 32:569–88. doi: 10.1016/j.cjca.2016.02.066
48. Vinceti, M, Filippini, T, Crippa, A, de Sesmaisons, A, Wise, LA, and Orsini, N. Meta-analysis of potassium intake and the risk of stroke. J Am Heart Assoc. (2016) 5. doi: 10.1161/JAHA.116.004210
49. Castro, H, and Raij, L. Potassium in hypertension and cardiovascular disease. Semin Nephrol. (2013) 33:277–89. doi: 10.1016/j.semnephrol.2013.04.008
50. Czerwonka, M, and Tokarz, A. Iron in red meat-friend or foe. Meat Sci. (2017) 123:157–65. doi: 10.1016/j.meatsci.2016.09.012
51. Institute of Medicine (US) . Dietary Reference Intakes For Vitamin A, Vitamin K, Arsenic, Boron, Chromium, Copper, Iodine, Iron, Manganese, Molybdenum, Nickel, Silicon, Vanadium, and Zinc. Washington (DC): The National Academies Press. (2001).
52. von Haehling, S, Jankowska, EA, van Veldhuisen, DJ, Ponikowski, P, and Anker, SD. Iron deficiency and cardiovascular disease. Nat Rev Cardiol. (2015) 12:659–69. doi: 10.1038/nrcardio.2015.109
53. Zacharski, LR, Chow, BK, Howes, PS, Shamayeva, G, Baron, JA, Dalman, RL, et al. Reduction of iron stores and cardiovascular outcomes in patients with peripheral arterial disease: a randomized controlled trial. JAMA. (2007) 297:603–10. doi: 10.1001/jama.297.6.603
54. Geissler, C, and Singh, M. Iron, meat and health. Nutrients. (2011) 3:283–316. doi: 10.3390/nu3030283
55. Xu, YJ, Arneja, AS, Tappia, PS, and Dhalla, NS. The potential health benefits of taurine in cardiovascular disease. Exp Clin Cardiol. (2008) 13:57–65.
56. Wu, G, and Meininger, CJ. Arginine nutrition and cardiovascular function. J Nutr. (2000) 130:2626–9. doi: 10.1093/jn/130.11.2626
57. Santos, HO, Tinsley, GM, da Silva, GAR, and Bueno, AA. Pharmaconutrition in the clinical management of COVID-19: a lack of evidence-based research but clues to personalized prescription. J Pers Med. (2020) 10:145. doi: 10.3390/jpm10040145
58. Vasdev, S, and Gill, V. The antihypertensive effect of arginine. Int J Angiol. (2008) 17:07–22. doi: 10.1055/s-0031-1278274
59. Gokce, N . L-arginine and hypertension. J Nutr. (2004) 134:2807S–11S. doi: 10.1093/jn/134.10.2807S
60. Gouvea, SA, Bissoli, NS, Moyses, MR, Cicilini, MA, Pires, JG, and Abreu, GR. Activity of angiotensin-converting enzyme after treatment with L-arginine in renovascular hypertension. Clin Exp Hypertens. (2004) 26:569–79. doi: 10.1081/CEH-200031837
61. King, DE, Mainous, AG 3rd, and Geesey, ME. Variation in L-arginine intake follow demographics and lifestyle factors that may impact cardiovascular disease risk. Nutr Res. (2008) 28:21–4. doi: 10.1016/j.nutres.2007.11.003
62. Venho, B, Voutilainen, S, Valkonen, VP, Virtanen, J, Lakka, TA, Rissanen, TH, et al. Arginine intake, blood pressure, and the incidence of acute coronary events in men: the Kuopio Ischaemic heart disease risk factor study. Am J Clin Nutr. (2002) 76:359–64. doi: 10.1093/ajcn/76.2.359
63. Oomen, CM, van Erk, MJ, Feskens, EJ, Kok, FJ, and Kromhout, D. Arginine intake and risk of coronary heart disease mortality in elderly men. Arterioscler Thromb Vasc Biol. (2000) 20:2134–9. doi: 10.1161/01.ATV.20.9.2134
64. Dong, JY, Qin, LQ, Zhang, Z, Zhao, Y, Wang, J, Arigoni, F, et al. Effect of oral L-arginine supplementation on blood pressure: a meta-analysis of randomized, double-blind, placebo-controlled trials. Am Heart J. (2011) 162:959–65. doi: 10.1016/j.ahj.2011.09.012
65. Inam, UL, Piao, F, Aadil, RM, Suleman, R, Li, K, Zhang, M, et al. Ameliorative effects of taurine against diabetes: a review. Amino Acids. (2018) 50:487–502. doi: 10.1007/s00726-018-2544-4
66. Militante, JD, and Lombardini, JB. Treatment of hypertension with oral taurine: experimental and clinical studies. Amino Acids. (2002) 23:381–93. doi: 10.1007/s00726-002-0212-0
67. Zhang, M, Bi, LF, Fang, JH, Su, XL, Da, GL, Kuwamori, T, et al. Beneficial effects of taurine on serum lipids in overweight or obese non-diabetic subjects. Amino Acids. (2004) 26:267–71. doi: 10.1007/s00726-003-0059-z
68. Schaffer, SW, Azuma, J, and Mozaffari, M. Role of antioxidant activity of taurine in diabetes. Can J Physiol Pharmacol. (2009) 87:91–9. doi: 10.1139/Y08-110
69. Jong, CJ, Sandal, P, and Schaffer, SW. The role of Taurine in mitochondria health: more than just an antioxidant. Molecules (Basel, Switzerland). (2021) 26:4913. doi: 10.3390/molecules26164913
70. Jong, CJ, Azuma, J, and Schaffer, S. Mechanism underlying the antioxidant activity of taurine: prevention of mitochondrial oxidant production. Amino Acids. (2012) 42:2223–32. doi: 10.1007/s00726-011-0962-7
71. Oliveira, MW, Minotto, JB, de Oliveira, MR, Zanotto-Filho, A, Behr, GA, Rocha, RF, et al. Scavenging and antioxidant potential of physiological taurine concentrations against different reactive oxygen/nitrogen species. Pharmacol Rep. (2010) 62:185–93. doi: 10.1016/S1734-1140(10)70256-5
72. Sun, Q, Wang, B, Li, Y, Sun, F, Li, P, Xia, W, et al. Taurine supplementation lowers blood pressure and improves vascular function in prehypertension. Monograph (American Heart Association). (2016) 67:541–9. doi: 10.1161/HYPERTENSIONAHA.115.06624
73. Murakami, S, Kondo, Y, Toda, Y, Kitajima, H, Kameo, K, Sakono, M, et al. Effect of taurine on cholesterol metabolism in hamsters: up-regulation of low density lipoprotein (LDL) receptor by taurine. Life Sci. (2002) 70:2355–66. doi: 10.1016/S0024-3205(02)01507-2
74. Chang, TY, Li, BL, Chang, CC, and Urano, Y. Acyl-coenzyme a:cholesterol acyltransferases. Am J Physiol Endocrinol Metab. (2009) 297:E1–9. doi: 10.1152/ajpendo.90926.2008
75. Pullinger, CR, Eng, C, Salen, G, Shefer, S, Batta, AK, Erickson, SK, et al. Human cholesterol 7alpha-hydroxylase (CYP7A1) deficiency has a hypercholesterolemic phenotype. J Clin Invest. (2002) 110:109–17. doi: 10.1172/JCI0215387
76. Carneiro, EM, Latorraca, MQ, Araujo, E, Beltra, M, Oliveras, MJ, Navarro, M, et al. Taurine supplementation modulates glucose homeostasis and islet function. J Nutr Biochem. (2009) 20:503–11. doi: 10.1016/j.jnutbio.2008.05.008
77. Laidlaw, SA, Grosvenor, M, and Kopple, JD. The taurine content of common foodstuffs. JPEN J Parenter Enteral Nutr. (1990) 14:183–8. doi: 10.1177/0148607190014002183
78. Stapleton, PP, Charles, RP, Redmond, HP, and Bouchier-Hayes, DJ. Taurine and human nutrition. Clin Nutr. (1997) 16:103–8. doi: 10.1016/S0261-5614(97)80234-8
79. Waldron, M, Patterson, SD, Tallent, J, and Jeffries, O. The effects of Oral Taurine on resting blood pressure in humans: a meta-analysis. Curr Hypertens Rep. (2018) 20:81. doi: 10.1007/s11906-018-0881-z
80. Mozaffarian, D, Shi, P, Morris, JS, Spiegelman, D, Grandjean, P, Siscovick, DS, et al. Mercury exposure and risk of cardiovascular disease in two U.S. cohorts. N Engl J Med. (2011) 364:1116–25. doi: 10.1056/NEJMoa1006876
81. Gochfeld, M, and Burger, J. Mercury interactions with selenium and sulfur and the relevance of the se:hg molar ratio to fish consumption advice. Environ Sci Pollut Res Int. (2021) 28:18407–20. doi: 10.1007/s11356-021-12361-7
82. Bode, A, Carrera, P, González-Nuevo, G, Nogueira, E, Riveiro, I, and Santos, MB. A trophic index for sardine (Sardina pilchardus) and its relationship to population abundance in the southern Bay of Biscay and adjacent waters of the NE Atlantic. Prog Oceanogr. (2018) 166:139–47. doi: 10.1016/j.pocean.2017.08.005
83. Lescord, GL, Johnston, TA, Branfireun, BA, and Gunn, JM. Percentage of methylmercury in the muscle tissue of freshwater fish varies with body size and age and among species. Environ Toxicol Chem. (2018) 37:2682–91. doi: 10.1002/etc.4233
84. Estruch, R, Ros, E, Salas-Salvado, J, Covas, MI, Corella, D, Aros, F, et al. Retraction and republication: primary prevention of cardiovascular disease with a Mediterranean diet. N Engl J Med. (2018) 378:2441–2. doi: 10.1056/NEJMc1806491
85. Downer, MK, Martinez-Gonzalez, MA, Gea, A, Stampfer, M, Warnberg, J, Ruiz-Canela, M, et al. Mercury exposure and risk of cardiovascular disease: a nested case-control study in the PREDIMED (PREvention with MEDiterranean diet) study. BMC Cardiovasc Disord. (2017) 17:9. doi: 10.1186/s12872-016-0435-8
86. Sala-Vila, A, Harris, WS, Cofan, M, Perez-Heras, AM, Pinto, X, Lamuela-Raventos, RM, et al. Determinants of the omega-3 index in a Mediterranean population at increased risk for CHD. Br J Nutr. (2011) 106:425–31. doi: 10.1017/S0007114511000171
87. Aarsetoey, H, Aarsetoey, R, Lindner, T, Staines, H, Harris, WS, and Nilsen, DW. Low levels of the omega-3 index are associated with sudden cardiac arrest and remain stable in survivors in the subacute phase. Lipids. (2011) 46:151–61. doi: 10.1007/s11745-010-3511-3
88. Harris, WS, Del Gobbo, L, and Tintle, NL. The Omega-3 index and relative risk for coronary heart disease mortality: estimation from 10 cohort studies. Atherosclerosis. (2017) 262:51–4. doi: 10.1016/j.atherosclerosis.2017.05.007
90. Harris, WS . The omega-3 index as a risk factor for coronary heart disease. Am J Clin Nutr. (2008) 87:1997S–2002S. doi: 10.1093/ajcn/87.6.1997S
91. Marrugat, J, Elosua, R, and Gil, M. Epidemiology of sudden cardiac death in Spain. Rev Esp Cardiol. (1999) 52:717–25. doi: 10.1016/S0300-8932(99)74993-6
92. de Roos, B, Wood, S, Bremner, D, Bashir, S, Betancor, MB, Fraser, WD, et al. The nutritional and cardiovascular health benefits of rapeseed oil-fed farmed salmon in humans are not decreased compared with those of traditionally farmed salmon: a randomized controlled trial. Eur J Nutr. (2021) 60:2063–75. doi: 10.1007/s00394-020-02396-w
93. Ramprasath, VR, Eyal, I, Zchut, S, and Jones, PJ. Enhanced increase of omega-3 index in healthy individuals with response to 4-week n-3 fatty acid supplementation from krill oil versus fish oil. Lipids Health Dis. (2013) 12:178. doi: 10.1186/1476-511X-12-178
94. Grenon, SM, Owens, CD, Nosova, EV, Hughes-Fulford, M, Alley, HF, Chong, K, et al. Short-term, high-dose fish oil supplementation increases the production of Omega-3 fatty acid-derived mediators in patients with peripheral artery disease (the OMEGA-PAD I trial). J Am Heart Assoc. (2015) 4:e002034. doi: 10.1161/JAHA.115.002034
95. GOV, UK . Guidance nutrition legislation information sheet. (2021). Available at: https://www.gov.uk/government/publications/nutrition-legislation-information-sources/nutrition-legislation-information-sheet--2#:~:text=2.3%20Prohibited%20ingredients%20in%20food%20supplements%20in%20the%20UK&text=Before%20you%20place%20your%20product. Accessed October 16, 2022.
96. UK Department of Health . Food supplements. (2011). Available at: https://assets.publishing.service.gov.uk/government/uploads/system/uploads/attachment_data/file/204303/Supplements_Summary__Jan_2012__DH_FINAL.doc.pdf (Accessed October 16, 2022).
97. European Food Safety Authority . Food supplements. (2018). Available at: https://www.efsa.europa.eu/en/topics/topic/food-supplements (Accessed October 16, 2022).
98. Lentjes, MAH . The balance between food and dietary supplements in the general population. Proc Nutr Soc. (2019) 78:97–109. doi: 10.1017/s0029665118002525
99. Yehuda, S, Rabinovitz, S, and Mostofsky, DI. Mixture of essential fatty acids lowers test anxiety. Nutr Neurosci. (2005) 8:265–7. doi: 10.1080/10284150500445795
100. Food Standards Agency (FSA) . Food supplements consumer research. (2018). Available at: https://www.food.gov.uk/sites/default/files/media/document/food-supplements-consumer-research.pdf (Accessed October 16, 2022).
101. Mishra, S, Stierman, B, Gahche, JJ, and Potischman, N. Dietary supplement use among adults: United States, 2017-2018. NCHS Data Brief. (2021) 1–8. doi: 10.15620/cdc:101131
102. Iłowiecka, K, Maślej, M, Czajka, M, Pawłowski, A, Więckowski, P, Styk, T, et al. Lifestyle, eating habits, and health behaviors among dietary supplement users in three European countries. Front Public Health. (2022) 10:892233. doi: 10.3389/fpubh.2022.892233
103. Food Supplements Europe (FSE) . Consumer survey on food supplements in the EU. (2022). Available at: https://foodsupplementseurope.org/wp-content/uploads/2022/07/FSE-Consumer_Survey-Ipsos-2022.pdf (Accessed October 16, 2022).
104. Lordan, R . Dietary supplements and nutraceuticals market growth during the coronavirus pandemic–implications for consumers and regulatory oversight. PharmaNutrition. (2021) 18:100282. doi: 10.1016/j.phanu.2021.100282
105. Manson, JE, Cook, NR, Lee, I-M, Christen, W, Bassuk, SS, Mora, S, et al. Marine n-3 fatty acids and prevention of cardiovascular disease and cancer. N Engl J Med. (2019) 380:23–32. doi: 10.1056/NEJMoa1811403
106. Abdelhamid, AS, Brown, TJ, Brainard, JS, Biswas, P, Thorpe, GC, Moore, HJ, et al. Omega-3 fatty acids for the primary and secondary prevention of cardiovascular disease. Cochrane Database Syst Rev. (2018) 7:CD003177. doi: 10.1002/14651858.CD003177.pub3
107. Bhatt, DL, Steg, PG, Miller, M, Brinton, EA, Jacobson, TA, Ketchum, SB, et al. Cardiovascular risk reduction with Icosapent ethyl for hypertriglyceridemia. N Engl J Med. (2019) 380:11–22. doi: 10.1056/NEJMoa1812792
108. Nevigato, T, Masci, M, and Caproni, R. Quality of fish-oil-based dietary supplements available on the Italian market: a preliminary study. Molecules (Basel, Switzerland). (2021) 26:5015. doi: 10.3390/molecules26165015
109. Leung, KS, Galano, JM, Durand, T, and Lee, JC. Profiling of omega-polyunsaturated fatty acids and their oxidized products in Salmon after different cooking methods. Antioxidants (Basel, Switzerland). (2018) 7:96. doi: 10.3390/antiox7080096
110. Echarte, M, Zulet, MA, and Astiasaran, I. Oxidation process affecting fatty acids and cholesterol in fried and roasted salmon. J Agric Food Chem. (2001) 49:5662–7. Epub 2001/11/21. doi: 10.1021/jf010199e
111. Floros, S, Toskas, A, Pasidi, E, and Vareltzis, P. Bioaccessibility and oxidative stability of Omega-3 fatty acids in supplements, sardines and enriched eggs studied using a static in vitro gastrointestinal model. Molecules (Basel, Switzerland). (2022) 27:415. doi: 10.3390/molecules27020415
112. Galli, C, and Rise, P. Fish consumption, omega 3 fatty acids and cardiovascular disease. The science and the clinical trials. Nutr Health. (2009) 20:11–20. doi: 10.1177/026010600902000102
113. Petsini, F, Fragopoulou, E, and Antonopoulou, S. Fish consumption and cardiovascular disease related biomarkers: a review of clinical trials. Crit Rev Food Sci Nutr. (2019) 59:2061–71. doi: 10.1080/10408398.2018.1437388
114. Musa-Veloso, K, Venditti, C, Lee, HY, Darch, M, Floyd, S, West, S, et al. Systematic review and meta-analysis of controlled intervention studies on the effectiveness of long-chain omega-3 fatty acids in patients with nonalcoholic fatty liver disease. Nutr Rev. (2018) 76:581–602. doi: 10.1093/nutrit/nuy022
115. O'Mahoney, LL, Matu, J, Price, OJ, Birch, KM, Ajjan, RA, Farrar, D, et al. Omega-3 polyunsaturated fatty acids favourably modulate cardiometabolic biomarkers in type 2 diabetes: a meta-analysis and meta-regression of randomized controlled trials. Cardiovasc Diabetol. (2018) 17:98. doi: 10.1186/s12933-018-0740-x
116. Sekikawa, A, Mahajan, H, Kadowaki, S, Hisamatsu, T, Miyagawa, N, Fujiyoshi, A, et al. Association of blood levels of marine omega-3 fatty acids with coronary calcification and calcium density in Japanese men. Eur J Clin Nutr. (2019) 73:783–92. doi: 10.1038/s41430-018-0242-7
117. Feuchtner, G, Langer, C, Barbieri, F, Beyer, C, Dichtl, W, Friedrich, G, et al. The effect of omega-3 fatty acids on coronary atherosclerosis quantified by coronary computed tomography angiography. Clin Nutr. (2021) 40:1123–9. doi: 10.1016/j.clnu.2020.07.016
118. Mori, TA . Omega-3 fatty acids and cardiovascular disease: epidemiology and effects on cardiometabolic risk factors. Food Funct. (2014) 5:2004–19. doi: 10.1039/C4FO00393D
119. Skulas-Ray, AC, Wilson, PWF, Harris, WS, Brinton, EA, Kris-Etherton, PM, Richter, CK, et al. Omega-3 fatty acids for the Management of Hypertriglyceridemia: a science advisory from the American Heart Association. Circulation. (2019) 140:e673–91. doi: 10.1161/CIR.0000000000000709
120. Moradi, S, Alivand, M, KhajeBishak, Y, AsghariJafarabadi, M, Alipour, M, Chilibeck, PD, et al. The effect of omega3 fatty acid supplementation on PPARγ and UCP2 expressions, resting energy expenditure, and appetite in athletes. BMC Sports Sci Med Rehab. (2021) 13:48. doi: 10.1186/s13102-021-00266-4
121. Irving, GF, Freund-Levi, Y, Eriksdotter-Jönhagen, M, Basun, H, Brismar, K, Hjorth, E, et al. Omega-3 fatty acid supplementation effects on weight and appetite in patients with Alzheimer's disease: the omega-3 Alzheimer's disease study. J Am Geriatr Soc. (2009) 57:11–7. doi: 10.1111/j.1532-5415.2008.02055.x
Keywords: sardines, fish oil, omega-3, lipids, cardiovascular disease, calcium
Citation: Santos HO, May TL and Bueno AA (2023) Eating more sardines instead of fish oil supplementation: Beyond omega-3 polyunsaturated fatty acids, a matrix of nutrients with cardiovascular benefits. Front. Nutr. 10:1107475. doi: 10.3389/fnut.2023.1107475
Edited by:
Arunaksharan Narayanankutty, St. Joseph's College (Autonomous), Devagiri, IndiaReviewed by:
Philip Calder, University of Southampton, United KingdomPatroklos Vareltzis, Aristotle University of Thessaloniki, Greece
Copyright © 2023 Santos, May and Bueno. This is an open-access article distributed under the terms of the Creative Commons Attribution License (CC BY). The use, distribution or reproduction in other forums is permitted, provided the original author(s) and the copyright owner(s) are credited and that the original publication in this journal is cited, in accordance with accepted academic practice. No use, distribution or reproduction is permitted which does not comply with these terms.
*Correspondence: Heitor O. Santos, aGVpdG9yb2xpdmVpcmFzYW50b3NAZ21haWwuY29t