- 1Health Management Center, The Third Xiangya Hospital, Central South University, Changsha, China
- 2Department of General Surgery, The Third Xiangya Hospital, Central South University, Changsha, China
Background: Non-alcoholic fatty liver disease (NAFLD), especially lean NAFLD is associated with an increased risk of atherosclerotic cardiovascular disease (CVD). It is not currently known which clinical phenotypes of NAFLD contribute most to individual subclinical atherosclerosis risk. We examined the relationship between body mass index (BMI), the metabolically healthy status, and subclinical atherosclerosis in the NAFLD population.
Methods: Data from asymptomatic NAFLD subjects who participated in a routine health check-up examination were collected. Participants were stratified by BMI (cutoff values: 24.0–27.9 kg/m2 for overweight and ≥28.0 kg/m2 for obesity) and metabolic status, which was defined by Adult Treatment Panel III criteria. Subclinical atherosclerosis was evaluated by brachial-ankle pulse wave velocity (baPWV) in 27,738 participants and by carotid plaque in 14,323 participants.
Results: Within each BMI strata, metabolically unhealthy subjects had a significantly higher prevalence of subclinical atherosclerosis than metabolically healthy subjects, whereas fewer differences were observed across subjects within the same metabolic category. When BMI and metabolic status were assessed together, a metabolically unhealthy status was the main contributor to the association of clinical phenotypes with the subclinical atherosclerosis burden (all p < 0.001). When BMI and metabolic abnormalities were assessed separately, the incidence of subclinical disease did not increase across BMI categories; however, it increased with an increase in the number of metabolic abnormalities (0, 1, 2 and ≥3).
Conclusion: A metabolically healthy status in NAFLD patients was closely correlated with subclinical atherosclerosis, beyond that of the BMI-based obesity phenotype. The application of metabolic phenotyping strategies could enable more precise classification in evaluating cardiovascular risk in NAFLD.
Introduction
Non-alcoholic fatty liver disease (NAFLD) is currently the most common hepatic disease and a highly heterogeneous metabolic disorder (1, 2). The prognosis of NAFLD is not as benign as thought and confers substantial increases in morbidity and mortality in those individuals who are affected. Evolving data from meta-analyses and cohort studies support the notion that the most common cause of death in the NAFLD population is cardiovascular disease (CVD), followed by extrahepatic malignancies and liver-related complications (3–5).
Although NAFLD is particularly common among subjects with obesity, it is increasingly being identified in lean individuals. The prevalence of lean NAFLD in the NAFLD population ranges from 10% to 20%, with the highest prevalence seen in Asian people (4). In addition, NAFLD interacts with the regulation of multiple metabolic pathways and is bidirectionally linked with components of metabolic syndrome (MetS). In 2020, a panel of international experts proposed that the nomenclature be changed from NAFLD to metabolic dysfunction-associated fatty liver disease (MAFLD) (6). However, the relationship between BMI and metabolic abnormalities is not generally uniform in the NAFLD population (1). There is a subgroup of individuals with obesity who are resistant to metabolic abnormalities, while there is another subset of subjects with normal weight who are prone to metabolic disturbances.
Beyond the association of NAFLD with CVD events, substantial epidemiological evidence links it to subclinical atherosclerosis, including carotid artery intima-media thickness (CIMT), carotid plaque, brachial-ankle pulse wave velocity (baPWV), coronary artery calcification (CAC) and brachial arterial flow-mediated dilation (FMD) (7, 8). However, no studies have investigated the impact of body size and shape combined with metabolic status on cardiovascular risk in the NAFLD population. The underlying relationship between obesity, poor metabolic health and subclinical atherosclerosis remains poorly understood. Awareness of the association is important for clinical practice and could provide proper risk stratification for early lifestyle intervention, which includes a healthy dietary composition in fruits and vegetables, legumes, whole grains, a minimal intake of trans-fats, ultra-processed food, red meat, sugar-sweetened beverages and regular physical activity, and so on improve long-term clinical outcomes (9).
In view of the aforementioned gaps, we examined the associations among the BMI-based obesity phenotype, metabolic health phenotype and subclinical CVD burden, including increased arterial wall stiffness and the presence of carotid plaque, in a large sample of asymptomatic NAFLD subjects without known cardiovascular disease.
Methods
Study design and population
We identified 96,963 adults aged 18–90 years who underwent routine health examinations at the Third Xiangya Hospital of Central South University in Changsha between August 2017 and July 2021. The population consisted of a mix of urban and rural residents. After excluding participants with incomplete information, prior cardiovascular disease, and malignancy and those not meeting the definition of NAFLD, 27,738 NAFLD subjects remained for the cross-sectional study 1 analyses of the risk associated with arterial stiffness. We further excluded participants with no carotid vascular ultrasonography, leaving a final sample of 14,323 NAFLD participants for the cross-sectional study 2 analyses of the risk associated with carotid plaque (Figure 1). Informed consent and the protocol of the overall physical examination were reviewed and approved by the institutional review board at the Third Xiangya Hospital (No. 2018-S393).
Clinical characteristics
All participants completed a computerized National Physical Examination Questionnaire (10, 11). Personal details (demographic characteristics, health-related habits, family history, current medication information from pill bottles, previous medical diagnoses, etc.) were recorded according to standard protocols. The assessment and definitions of lifestyle factors, hypertension and diabetes are detailed in the Online Appendix.
Physical examination and laboratory measurements
The detailed methods used for physical examinations and laboratory evaluations have been previously described (11). Briefly, body weight, height, waist circumference (WC), heart rate and blood pressure were measured. BMI was calculated as weight in kilograms divided by height in meters squared (kg/m2).
After the participants fasted overnight for at least 8 h, blood samples were collected and immediately processed and analyzed for alanine aminotransferase (ALT), aspartate aminotransferase (AST), albumin, total bilirubin, uric acid, creatinine, glucose, lipids and platelet count at the clinical laboratory of the Third Xiangya Hospital. The sample analysis was performed in accordance with the manufacturer’s specifications. FIB-4 was used as a noninvasive and accurate marker of fibrosis based on the following formula: FIB-4 index = age × AST(U/L)/platelet count (×109/L) (12). The estimated glomerular filtration rate (eGFR) was used as an index of renal disease based on the modification of diet in renal disease formula for Chinese subjects: eGFR = 175 × Scr−1.234 × age−0.179 [if female, ×0.79] (13).
Determination of NAFLD and clinical phenotypes
NAFLD was defined as the presence of hepatic steatosis without excessive alcohol consumption (≥30 g/d in men and ≥20 g/d in women) or concomitant liver disease (14). The method used for detecting hepatic steatosis was hepatic ultrasound (Logiq 9, GE Medical System, Milwaukee, WI, United States). Positive abdominal ultrasound findings included the following 5 criteria: (1) parenchymal brightness, (2) liver-to-kidney contrast, (3) deep beam attenuation, (4) bright vessel walls and (5) gallbladder wall definition (15). Subjects with at least two abnormal findings were diagnosed with hepatic steatosis (16).
In accordance with the Working Group on Obesity in China (17), BMIs of 18.5–23.9 kg/m2 were defined as normal weight; BMIs of 24.0–27.9 kg/m2 were defined as overweight and ≥28.0 kg/m2 were defined as obesity. The MetS definition in the National Cholesterol Education Program-Adult Treatment Panel III International Diabetes Federation (IDF) criteria (18) included elevated triglyceride (TG) levels (≥1.69 mmol/L) or the use of lipid-lowering drugs, low high-density lipoprotein (HDL) cholesterol levels (<1.03 mmol/L in men and <1.29 mmol/L in women), elevated systolic blood pressure (≥130 mmHg) or diastolic blood pressure (≥85 mmHg) or the use of antihypertensive drugs, and elevated blood glucose levels (≥5.6 mmol/L) or the use of any medications for diabetes (insulin or oral glucose-lowering medications). WC was not included because of collinearity with BMI. Individuals with one or fewer of these components were deemed metabolically healthy (MH), with two or more being deemed metabolically unhealthy (MU) according to the Framingham Heart Study (19).
Based on the combination of BMI categories and metabolic health status, the clinical phenotypes of NAFLD were then categorized into 6 groups: normal weight-MH, normal weight-MU, overweight-MH, overweight-MU, obese-MH and obese-MU.
Assessment of baPWV and carotid plague
As previously reported (11), baPWV was measured with an automatic waveform analyzer (BP-203 RPE III, Omron Health Medical, Dalian, China). After a minimum rest of 5 min in the supine position, 4 cuffs were wrapped around the extremities (upper arms and ankles) and then connected to the plethysmography sensor (volume pulse form) and an oscillometric pressure sensor. Pressure waveforms were recorded at both the brachial and tibial arteries to assess the transmission time between the initial increases in these waves. The measurements were performed twice, and the mean of the left- and right-side baPWV values were calculated. Moreover, substantial side differences in the baPWV of more than 10 m/s indicated problems with measurement, and the measurement should be repeated. The highest baPWV quartile (>1,590 cm/s) was classified as increased baPWV (arterial stiffness) (20).
As previously reported (21), carotid plaque was assessed using carotid artery sonography (Siemens AcusonSequoiaTM512 Ultrasound System, Mountain View, CA, United States) with a 9 MHz linear array transducer. Experienced sonographers performed carotid examinations, including bilateral visualization of the common, internal, and external carotid arteries. Carotid plaque was defined as a focal wall thickening of at least 0.5 mm or 50% of the surrounding CIMT that encroached into the arterial lumen or a focal region with CIMT greater than 1.5 mm that protruded into any carotid segment (22).
Statistical analyses
Descriptive characteristics are presented as the mean ± SD or median (interquartile range) for continuous variables and as the number (percentage) for categorical variables. The covariates between different groups were compared using a t test or the Mann–Whitney U test for continuous variables and the chi-square test for categorical variables. Multivariate logistic regression models were performed to assess the association of baPWV (the highest quartile versus other quartiles) and carotid plaques (presence versus absence) with (1) the 6 clinical phenotypes above; (2) the 3 BMI categories (normal weight, overweight and obese); (3) the 4 different categories of metabolic abnormalities (0, 1, 2 and ≥3 indices); and (4) individual metabolic abnormality risk factors (elevated blood pressure, triglyceridemia, hyperglycemia levels and low HDL-cholesterol). In addition, multiple linear regression analysis was used to evaluate the associations between baPWV (defined as a continuous variable) and the 6 clinical phenotypes. Potential covariates were adjusted for age, sex, demographic characteristics, lifestyles, WC, heart rate, albumin, total bilirubin, FIB-4, uric acid and eGFR.
Additional sensitivity analysis was performed to replicate our main findings with stricter definitions of metabolically healthy status, including the WC criterion (cutoff points of ≥90 cm for men and ≥85 cm for women) for the definition and defining metabolically healthy participants as having none of the five possible metabolic abnormality risk factors.
A p-value <0.05 was considered statistically significant. Statistical analyses were performed using SAS version 9.4 (SAS Institute, Cary, NC), and graphs were drawn by GraphPad Prism version 6.00 (GraphPad Software, La Jolla California, United States).
Results
Study population
The cross-sectional analysis included 27,738 overall participants (mean age: 49.8 years; 80.3% were male) with complete baPWV information in cross-sectional study sample I. A total of 14,323 individuals (mean age: 60 years; 76.7% were male) with complete carotid plaque information were included in cross-sectional study sample II.
Prevalence and characteristics of body size phenotypes
Of the 27,738 NAFLD participants, 13.2%, 55.1% and 31.7% fell into the normal weight, overweight and obese categories, respectively (Figure 2A). In the normal weight group, the MH subjects accounted for a higher percentage than did the MU subjects (56.3% vs. 43.7%), whereas MU status was predominant in the overweight and obese groups (63.5% vs. 36.5%; 74.8% vs. 25.2%) (Figure 2B).
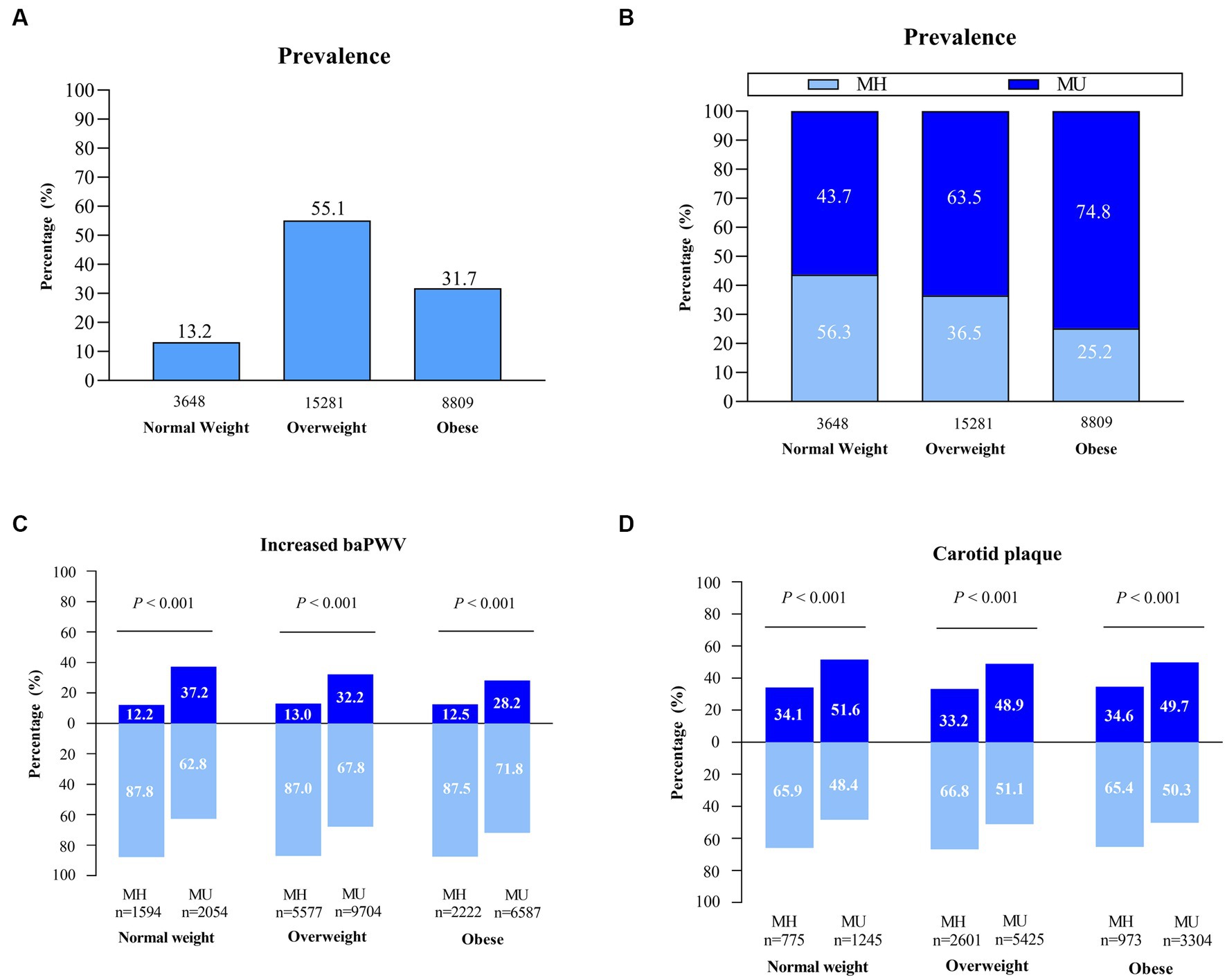
Figure 2. The prevalence of clinical phenotypes and distribution of subclinical atherosclerosis in the NAFLD population. (A–C) Assessment in NAFLD cross-sectional sample I (n = 27,738). (D) Assessment in NAFLD cross-sectional sample II (n = 14,323). MH, metabolically healthy; MU, metabolically unhealthy.
Within each BMI strata, MU individuals were older, had a higher proportion of poor diet scores, had a higher prevalence of diabetes and hypertension, and had higher WC, heart rate, blood pressure, and levels of fasting glucose, triglycerides, liver enzymes and uric acid than MH individuals. In contrast, a greater proportion of MH participants had a university degree, a worker occupation and a short sleep duration, and they had significantly greater levels of HDL-C and total bilirubin than participants with MU (Table 1).
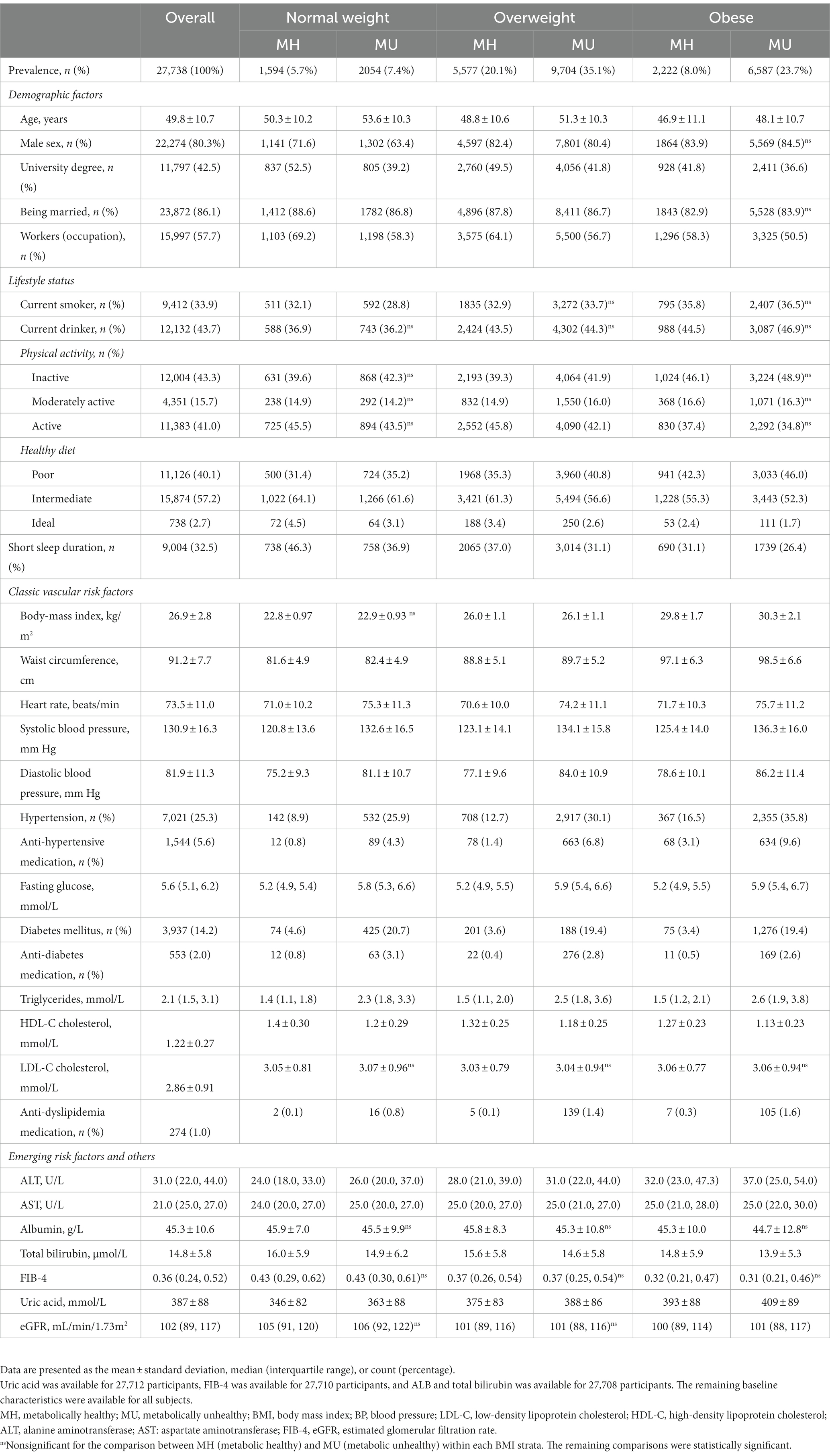
Table 1. Study population clinical characteristics stratified by clinical phenotypes in cross-sectional sample I.
Subclinical atherosclerosis profile
The MU individuals had an obviously higher prevalence of increased baPWV and carotid plaque than the MH individuals across all BMI categories. The extent of subclinical atherosclerosis was similar among MH individuals across BMI categories. For MU individuals, the extension was relatively higher for normal-weight individuals than for overweight and obese individuals (37.2% vs. 32.2% vs. 28.2%; 51.6% vs. 48.9% vs. 49.7%) (Figures 2C,D). A possible reason is that lean NAFLD subjects tend to be older than non-lean NAFLD subjects (52.2 vs. 49.5 years).
Association between subclinical atherosclerosis and clinical phenotypes
Increased baPWV and the presence of carotid plaque were available for the analysis in cross-sectional study sample I and sample II, respectively.
First, we evaluated the association between subclinical atherosclerosis and clinical phenotypes (6 subgroups) after adjusting for potential confounding factors (Table 2). The associations, which were defined according to the highest baPWV quartiles and carotid plaque, were evaluated using multiple logistic regression analysis. With the normal weight-MH subgroup as a reference, among MU subjects, normal weight, overweight and obesity were all significantly associated with a higher risk for increased baPWV and carotid plaque, and the adjusted odds ratio of risk was similar across BMI categories; however, among MH individuals, overweight and obesity showed a much lower but marginally significant risk of increased baPWV but were not associated with carotid plaque. Additionally, we conducted linear regression analysis for baPWV, which was defined as a continuous variable. Similar patterns were found regarding the relationship of subclinical atherosclerosis and MAFLD clinical phenotypes.
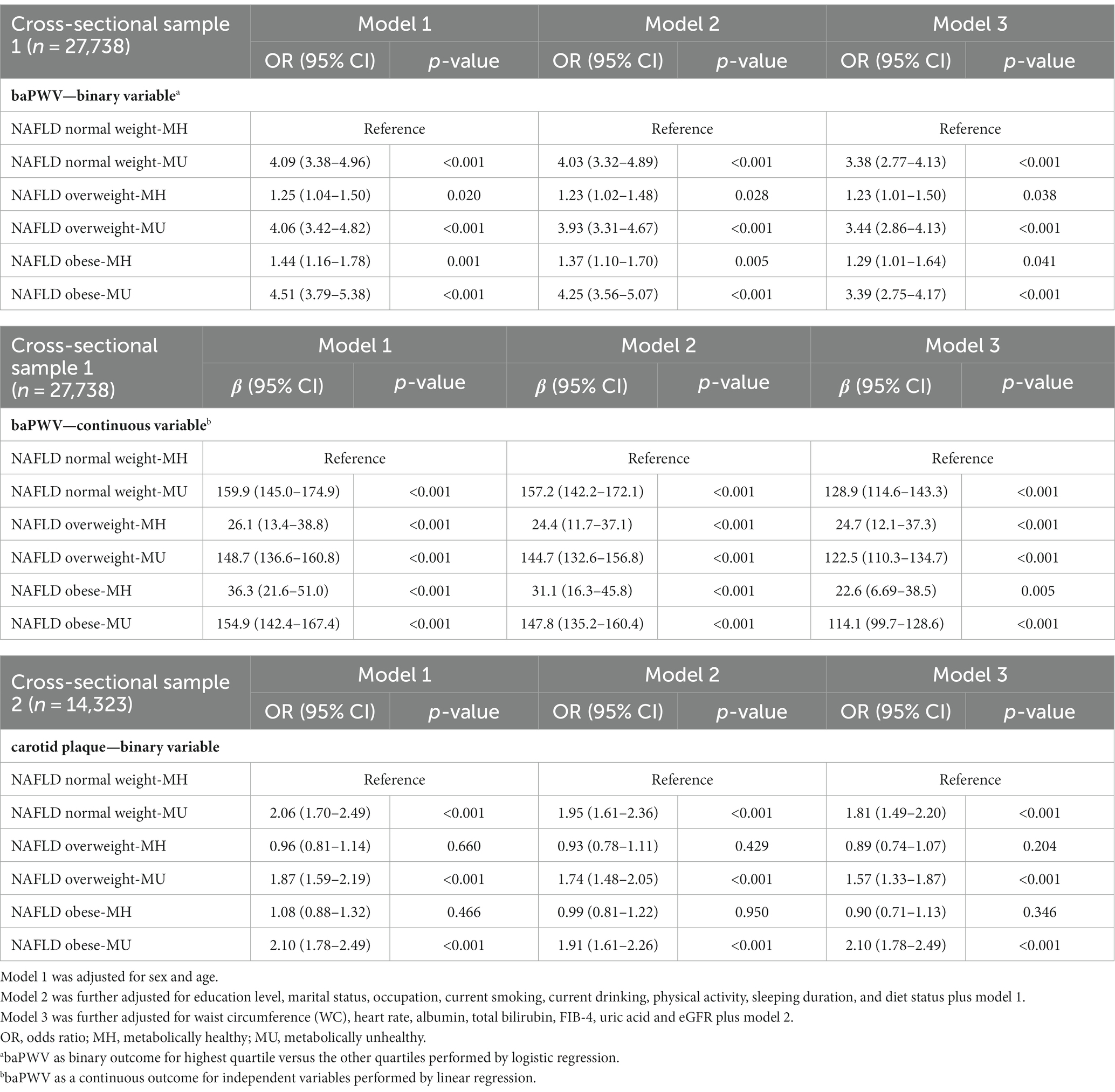
Table 2. The associations of clinical phenotypes with increased arterial stiffness in cross-sectional study sample I and carotid plaque in cross-sectional study sample II.
Second, to further explore whether the increased risk was mediated by metabolic abnormalities but not by increased BMI, we performed separate analyses to further assess the association between subclinical atherosclerosis and body size phenotypes after adjustment for confounding variables (Figures 3A,B,D,E). Whereas increased baPWV and carotid plaque increased with the increase in the number of metabolic abnormalities, there was no increase in the incidence of subclinical disease across BMI categories.
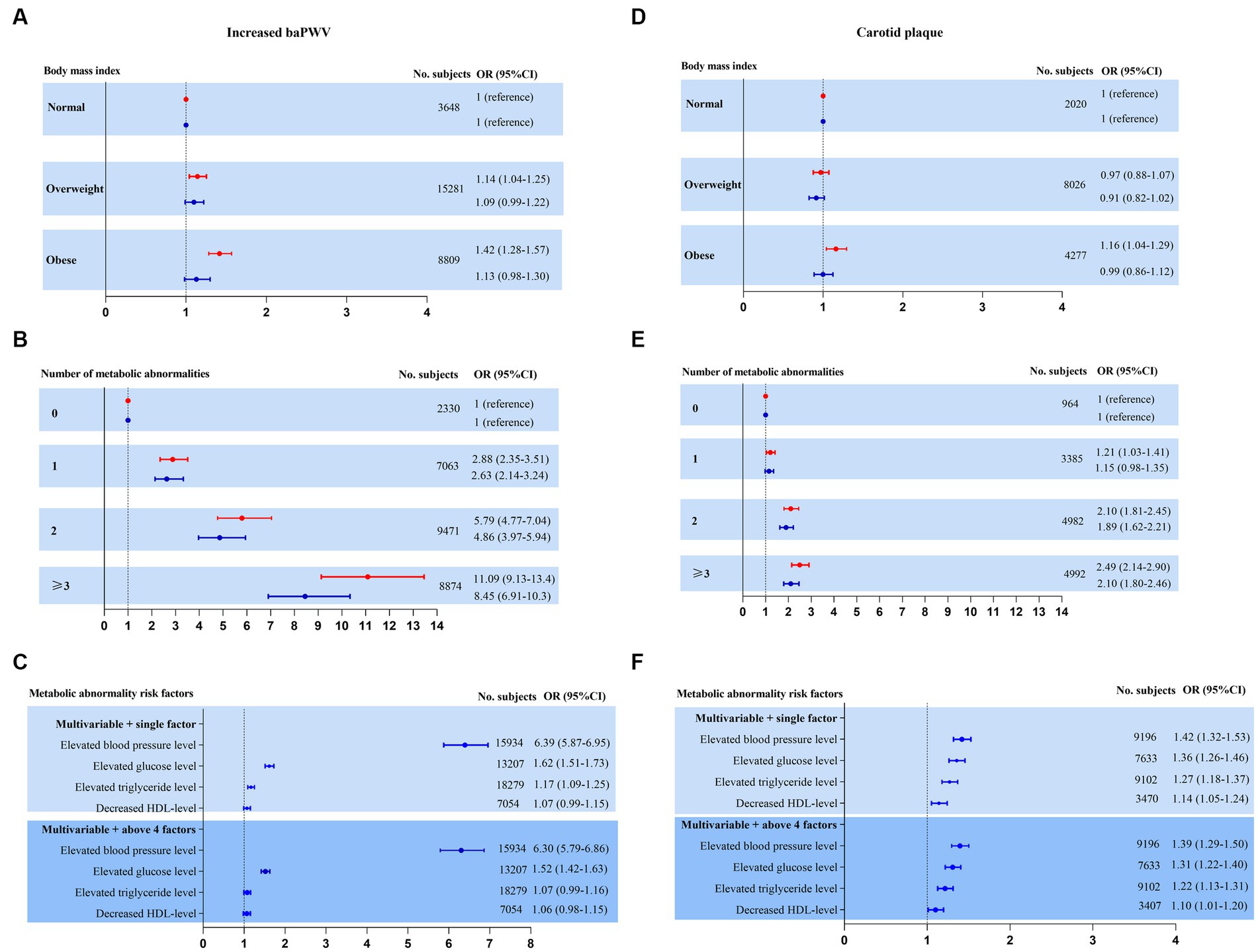
Figure 3. Risk for subclinical atherosclerosis stratified by body mass index, number of metabolic abnormalities and individual metabolic risk factor categories. Adjusted model 1 (red estimates) and fully adjusted model 2 (blue estimates) are both reported. Multivariate model 1 was adjusted for age and sex. Multivariate model 2 was further adjusted for education level, marital status, occupation, current smoking, current drinking, physical activity, sleeping duration, diet status, waist circumference, heart rate, ALB, total bilirubin, FIB-4, uric acid and eGFR plus model 1. (A–C) Increased baPWV was performed as a binary outcome for the highest quartile versus the other quartiles by logistic regression in NAFLD cross-sectional sample 1 (n = 27,738). (D–F) Carotid plaque as a binary outcome for presence versus absence by logistic regression in NAFLD cross-sectional sample 2 (n = 14,323).
Finally, the adjusted risk for subclinical disease was also calculated according to individual and joint metabolic risk factors. Both the presence of elevated blood pressure and elevated glucose levels were revealed to be obviously significant covariates in predicting subclinical disease. Elevated triglyceride levels and decreased HDL levels were borderline or not significantly associated with subclinical disease (Figures 3C,F).
Sensitivity analyses
Using a strict definition of metabolic health led to a slight reinforcement of the magnitude of the associations. However, this did not generally affect the trend of these associations (Supplementary Table S1 and Supplementary Figure S1).
Discussion
Main findings
We report here, for the first time, evidence of an association between clinical phenotypes and subclinical atherosclerosis in light of two different indices. We found a wide spectrum of clinical phenotypes in the NAFLD population. The poor metabolic profile was noticeable in overweight and obese individuals. Compared with metabolically healthy subjects, metabolically unhealthy subjects had a higher burden of subclinical atherosclerosis regardless of their BMI phenotypes. Unhealthy metabolic status, but not BMI-based body size, was the main contributor to the associations of clinical phenotypes with subclinical atherosclerosis. An increasing number of comorbid MetS traits were linked with a higher risk in this cohort. Among the MetS components, elevated blood pressure and blood glucose levels were stronger risk factors associated with the prevalence of subclinical diseases. Our results held true not only when we used a clinical definition of metabolic healthy status (presence of one or fewer of the 4 metabolic risk factors) but also when we used a more stringent definition (presence of none of the 5 cardiometabolic risk factors).
Comparison with previous studies
The presence of carotid plaque was validated as an excellent marker of atherosclerotic lesions and structural abnormalities, reflecting generalized atherosclerosis. Increased baPWV is a reliable index of early vascular functional stiffness. A growing body of studies has reported that NAFLD is associated with the two markers of preclinical atherosclerosis, notably independent of established CVD risk factors (8, 23). However, these previous studies have several limitations. First, the most frequently included populations were hospital-based or outpatient cohorts, and some patients were diagnosed with liver biopsy, which resulted in a relatively small sample and led to selection bias. Hence, the results might lead to an overestimation of the effect (24–28). Second, the subjects among whom this link was observed were simply NAFLD vs. non-NAFLD. Thus, the findings can be interpreted that the NAFLD population could be recognized as having a high risk of atherosclerotic CVD. However, it is important to determine how risk discrimination can be performed within the NAFLD population. In our study, the metabolic profile was the greatest contributor to the relationship of subclinical atherosclerosis with different clinical phenotypes. This was illustrated by the effect of risk in subclinical atherosclerosis being concentrated among subjects in the metabolic abnormality subgroups, suggesting a major role for metabolic health in comparison with BMI ranges. Our findings highlight the message that precise metabolic phenotyping assessment beyond BMI could enable proper classification of NAFLD patients.
Several previous studies have reported an association between clinical phenotypes and the prognosis of NAFLD. In Gut, Younes et al. (29) reported a multicenter study comparing the long-term prognosis, including the onset of diabetes and long-term CVD events, between lean and non-lean NAFLD populations. They found that hepatic and non-hepatic clinical complications of the two subgroups did not differ significantly over a decade of follow-up. In addition, they found that 77.5% of the lean NAFLD patients stayed at a normal weight at the end follow-up, which implied that the longitudinal progression to obesity did not contribute to the disease outcomes in such lean subjects. Another study (30) investigated the effect of metabolic abnormalities on the risk of hepatic prognosis among patients with NAFLD over a 9 years period. Among lean NAFLD patients with one or zero metabolic risk factors, the future risk is very low. Therefore, these studies challenged that a BMI-driven strategy for screening NAFLD subjects for cardiovascular or liver damage outcomes can be misleading and should be reevaluated as metabolic derangements.
Potential mechanisms
Although BMI is an acceptable marker for overall adiposity, it cannot distinguish between muscle and fat and does not capture assessments of body-fat distribution, such as visceral and subcutaneous adipose tissue (31). Lean NAFLD is a common phenotype among Asian people, especially as they seem to have higher amounts of central fat deposition than White people and develop NAFLD and metabolic complications within a low BMI range (32, 33). Findings from body composition studies showed that increased BMI was a poor surrogate for increased visceral adipose tissue and intrahepatic fat, both of which are strongly associated with NAFLD and cardiometabolic risk factors (34–37). In contrast, several studies revealed that WC was a reliable anthropometric index of visceral adiposity. Additionally, it was recommended that health professionals should be trained to properly perform this simple measurement and consider it an important “vital sign” in clinical practice. Therefore, importantly, BMI might be a suboptimal parameter for evaluating the association between obesity and subclinical atherosclerosis in the NAFLD population. Further studies that include precise quantification of different fat distributions could help to resolve this issue.
In this study, MetS itself or components of metabolic syndrome were consistent correlates of subclinical atherosclerosis. A complicated and dynamic interaction between a multitude of factors, including genetic, epigenetic, nutrition and dietary factors, lifestyle factors, and gut microbiota, is likely to shape individual metabolic profiles (1). In addition, NAFLD and MetS share a bidirectional association as a cause and a consequence (38). NAFLD is accompanied by impaired insulin-mediated suppression of hepatic glucose production, leading to liver steatosis, hyperglycemia and dyslipidemia. In turn, selective hepatic insulin resistance is thought to result in the development of both hepatic and peripheral metabolic dysfunction. Several types of pathophysiological crosstalk between NAFLD and MetS, including insulin resistance, abnormal lipoprotein metabolism, chronic low-grade inflammation, lipotoxicity and excessive oxidative stress, exert a proatherogenic effect on blood vessels (7). Similarly, Lee et al. (39) and Wang et al. (40) present evidence from large cohort studies from Korea and China, respectively, on the utility of the MAFLD definition for incident CVD and the risk of all-cause deaths. As expected from the positive definition (with a set of accompanying metabolic abnormalities), it is appealing to cover more patients with CVD and death under the umbrella term MAFLD compared with NAFLD. However, a dissociation between NAFLD and insulin resistance/dyslipidemia/CVD is present. NAFLD patients with a specific “liver-genetic” background have a lower risk of CVD, which may partially explain why a subgroup of patients with NAFLD are metabolically healthy (41). In addition, NAFLD impacts metabolism and CVD risk through hepatokines, such as fetuin-A, ANGPTL3 (angiopoietin-related protein 3), FGF21 (fibroblast growth factor 21), SHBG (sex hormone-binding globulin), selenoprotein P, fetuin-B and follistatin (42).
We also tried to disentangle the effects of individual metabolic traits and found no correlation or only a weak effect of low HDL levels and hypertriglyceridemia on the risk of NAFLD atherosclerosis. Among the indices of lipid metabolism, only HDL cholesterol and triglyceride levels are used to define MetS. Nevertheless, total cholesterol and LDL cholesterol are risk factors for atherosclerotic lesions and structural abnormalities, which are not included in the definition.
Implications
This study adds an important facet to the NAFLD cardiometabolic risk factor research spectrum in which, within the heterogeneity of body shape phenotype, metabolic health is more closely related to subclinical atherosclerosis, above and beyond adiposity defined by BMI. Our findings add to evidence that metabolic health conferred important clues to cardiometabolic risk that BMI may not explain.
In regard to the risk stratification implications, the strategy is solely stratified by BMI, and lean subjects with NAFLD may be misclassified as low risk and “metabolically healthy.” Obese NAFLD subjects who are “metabolically healthy” will be misclassified as being at higher risk. Accordingly, screening at-risk CVD populations instead of considering obesity or lean NAFLD as particular subgroups and performing a comprehensive assessment of specific metabolic profiles should be recommended. In other words, a novel definition of MH may be better suited to stratify subjects based on cardiometabolic risk parameters. In regard to the intervention management implications, lifestyle interventions remain the cornerstone of treatment. Weight loss has been shown substantial benefits not only for intrahepatic fat loss but also for improvement in metabolic parameters of glucose control and insulin sensitivity, whether NAFLD with overweight or normal weight (43). Several diet regimens were recommended, such as Mediterranean diet, low-carbohydrate diet, a low-fat diet and intermittent fasting over a regular diet (9, 44, 45). High adherence to the Mediterranean diet is associated with better profile of MetS features in NAFLD subjects owing to its close association with CVD (46). Nonetheless, further research is needed for its contribution on the development of subclinical atherosclerosis. In addition, some evidence showed that drinking three or more cups of coffee daily, dietary intake of vitamins E and C and phenolic acids had a protective association with NAFLD and its metabolic factors (47–49).
Strengths and limitations
The strengths of our study include many NAFLD participants; data on both structural and functional markers of subclinical disease; rich covariable adjustments encompassing sociodemographic factors and related CVD risk factors; and additional sensitivity analysis with strict MetS criteria, which added robustness to our findings.
Moreover, our results need to be interpreted with certain limitations. First, our cross-sectional design fails to determine causal relationships between clinical phenotypes and the development of subclinical atherosclerosis. Second, this study population consisted mostly of men, which potentially limits the generalizability of the results. Third, although we used two different versions of the MetS definition, impaired glucose tolerance and the inflammatory index, which are important components of new metabolic dysfunction criteria, were not included in this study (50). Fourth, recent data suggest that clinical phenotypes might be transient for a large proportion of individuals (19). Thus, the presence of phenotypes during one clinical examination could not capture the natural course of the variability within individuals, which remains controversial regarding the findings. Fifth, we chose only two markers of subclinical atherosclerosis, and other surrogate markers, such as coronary artery calcification (CAC) and brachial arterial flow-mediated dilation (FMD), were not included in our analysis. Sixth, we did not include information on emerging “nontraditional” CVD risk factors, such as decreased vitamin D and adiponectin levels, which may influence the relationship (51, 52). Lastly, carotid plaque indexes (such as carotid plaque burden and maximum carotid plaque thickness) are better predictors of cardiovascular disease risk than CIMT, and we did not obtain quantitative indicators of CIMT in our study (53).
Conclusion
In this health checkup-based study, different clinical phenotypes were associated with a greater subclinical CVD burden evaluated in subjects with NAFLD. However, this association remained significant only for the metabolic traits above and beyond the contribution of BMI-based body size. Our findings highlight the importance of metabolic status in screening fatty liver subjects at higher CVD risk. Although several treatments for NAFLD are currently in the pipeline, it would be preferable to establish treatment strategies with known benefits for improving both fat distribution and metabolic abnormalities in to achieve a better cardiovascular prognosis in the future.
Data availability statement
The raw data supporting the conclusions of this article will be made available by the authors, without undue reservation.
Ethics statement
The studies involving human participants were reviewed and approved by institutional review board at the Third Xiangya Hospital (No. 2018-S393). The patients/participants provided their written informed consent to participate in this study. Written informed consent was obtained from the individual(s) for the publication of any potentially identifiable images or data included in this article.
Author contributions
YW: study design, acquisition of clinical data, statistical analysis, and writing the manuscript. TY, SD, XZ, YD, and XL: acquisition of clinical data. LL and CW: acquisition of clinical data and modification of the manuscript. All authors contributed to the article and approved the submitted version.
Funding
This study was funded by the Changsha Natural Science Foundation (kq2208351), the National Natural Science Foundation of China (82001338), Hunan Provincial Natural Science Foundation (2020JJ4854, 2021JJ40934, and 2021JJ30989) and the Special Funding for the Construction of Innovative Provinces in Hunan (2020SK2055).
Acknowledgments
The authors would like to thank all of the participants for their support.
Conflict of interest
The authors declare that the research was conducted in the absence of any commercial or financial relationships that could be construed as a potential conflict of interest.
Publisher’s note
All claims expressed in this article are solely those of the authors and do not necessarily represent those of their affiliated organizations, or those of the publisher, the editors and the reviewers. Any product that may be evaluated in this article, or claim that may be made by its manufacturer, is not guaranteed or endorsed by the publisher.
Supplementary material
The Supplementary material for this article can be found online at: https://www.frontiersin.org/articles/10.3389/fnut.2023.1104859/full#supplementary-material
References
1. Eslam, M, Fan, JG, and Mendez-Sanchez, N. Non-alcoholic fatty liver disease in non-obese individuals: the impact of metabolic health. Lancet Gastroenterol Hepatol. (2020) 5:713–5. doi: 10.1016/S2468-1253(20)30090-X
2. Diehl, AM, and Day, C. Cause pathogenesis, and treatment of nonalcoholic steatohepatitis. N Engl J Med. (2017) 377:2063–72. doi: 10.1056/NEJMra1503519
3. Zou, B, Yeo, YH, Nguyen, VH, Cheung, R, Ingelsson, E, and Nguyen, MH. Prevalence, characteristics and mortality outcomes of obese, nonobese and lean NAFLD in the United States, 1999–2016. J Intern Med. (2020) 288:139–51. doi: 10.1111/joim.13069
4. Ye, Q, Zou, B, Yeo, YH, Li, J, Huang, DQ, Wu, Y, et al. Global prevalence, incidence, and outcomes of non-obese or lean non-alcoholic fatty liver disease: a systematic review and meta-analysis. Lancet Gastroenterol Hepatol. (2020) 5:739–52. doi: 10.1016/s2468-1253(20)30077-7
5. Lu, FB, Zheng, KI, Rios, RS, Targher, G, Byrne, CD, and Zheng, MH. Global epidemiology of lean non-alcoholic fatty liver disease: a systematic review and meta-analysis. J Gastroenterol Hepatol. (2020) 35:2041–50. doi: 10.1111/jgh.15156
6. Eslam, M, Newsome, PN, Sarin, SK, Anstee, QM, Targher, G, Romero-Gomez, M, et al. A new definition for metabolic dysfunction-associated fatty liver disease: an international expert consensus statement. J Hepatol. (2020) 73:202–9. doi: 10.1016/j.jhep.2020.03.039
7. Adams, LA, Anstee, QM, Tilg, H, and Targher, G. Non-alcoholic fatty liver disease and its relationship with cardiovascular disease and other extrahepatic diseases. Gut. (2017) 66:1138–53. doi: 10.1136/gutjnl-2017-313884
8. Zhou, YY, Zhou, XD, Wu, SJ, Fan, DH, van Poucke, S, Chen, YP, et al. Nonalcoholic fatty liver disease contributes to subclinical atherosclerosis: a systematic review and meta-analysis. Hepatol Commun. (2018) 2:376–92. doi: 10.1002/hep4.1155
9. Younossi, ZM, Zelber-Sagi, S, Henry, L, and Gerber, LH. Lifestyle interventions in nonalcoholic fatty liver disease. Nat Rev Gastroenterol Hepatol. (2023). doi: 10.1038/s41575-023-00800-4 [Epub ahead of print].
10. Wang, Y, Li, L, Li, Y, Liu, M, Gan, G, Zhou, Y, et al. The impact of dietary diversity, lifestyle, and blood lipids on carotid atherosclerosis: a cross-sectional study. Nutrients. (2022) 14:815. doi: 10.3390/nu14040815
11. Lu, Y, Pechlaner, R, Cai, J, Yuan, H, Huang, Z, Yang, G, et al. Trajectories of age-related arterial stiffness in Chinese men and women. J Am Coll Cardiol. (2020) 75:870–80. doi: 10.1016/j.jacc.2019.12.039
12. Zhou, F, Zhou, J, Wang, W, Zhang, XJ, Ji, YX, Zhang, P, et al. Unexpected rapid increase in the burden of NAFLD in China from 2008 to 2018: a systematic review and meta-analysis. Hepatology. (2019) 70:1119–33. doi: 10.1002/hep.30702
13. Teo, BW, Zhang, L, Guh, JY, Tang, SCW, Jha, V, Kang, DH, et al. Glomerular filtration rates in Asians. Adv Chronic Kidney Dis. (2018) 25:41–8. doi: 10.1053/j.ackd.2017.10.005
14. European Association for the Study of the Liver (EASL), European Association for the Study of Diabetes (EASD) and European Association for the Study of Obesity (EASO). EASL-EASD-EASO Clinical Practice Guidelines for the management of non-alcoholic fatty liver disease. J Hepatol. (2016) 64:1388–402. doi: 10.1016/j.jhep.2015.11.004
15. National Health and Nutrition Examination Survey (NHANES) III. (2010). Hepatic steatosis ultrasound images assessment procedures manual. National Health and Nutrition Examination Survey (NHANES) III.
16. Farrell, GC, Chitturi, S, Lau, GK, and Sollano, JD, Asia-Pacific Working Party on NAFLD1. Guidelines for the assessment and management of non-alcoholic fatty liver disease in the Asia-Pacific region: executive summary. J Gastroenterol Hepatol. (2007) 22:775–7. doi: 10.1111/j.1440-1746.2007.05002.x
17. Pan, XF, Wang, L, and Pan, A. Epidemiology and determinants of obesity in China. Lancet Diabetes Endocrinol. (2021) 9:373–92. doi: 10.1016/S2213-8587(21)00045-0
18. Expert Panel on Detection, Evaluation, and Treatment of High Blood Cholesterol in Adults. Treatment of high blood cholesterol in executive summary of the third report of the National Cholesterol Education Program (NCEP) expert panel on detection, evaluation, and treatment of high blood cholesterol in adults (adult treatment panel III). JAMA. (2001) 285:2486–97. doi: 10.1001/jama.285.19.2486
19. Echouffo-Tcheugui, JB, Short, MI, Xanthakis, V, Field, P, Sponholtz, TR, Larson, MG, et al. Natural history of obesity subphenotypes: dynamic changes over two decades and prognosis in the Framingham Heart Study. J Clin Endocrinol Metab. (2019) 104:738–52. doi: 10.1210/jc.2018-01321
20. Lee, YH, Shin, MH, Choi, JS, Rhee, JA, Nam, HS, Jeong, SK, et al. HbA1c is significantly associated with arterial stiffness but not with carotid atherosclerosis in a community-based population without type 2 diabetes: the Dong-gu study. Atherosclerosis. (2016) 247:1–6. doi: 10.1016/j.atherosclerosis.2016.01.032
21. Zhu, X, Chen, Z, Yang, P, Liu, L, Wu, L, and Wang, Y. The association of subclinical atherosclerosis with prediabetes is stronger in people with dyslipidaemia than in those with normoglycaemia: a cross-sectional study in Chinese adults. Prim Care Diabetes. (2020) 14:760–7. doi: 10.1016/j.pcd.2020.07.007
22. Mancia, G, Fagard, R, Narkiewicz, K, Redon, J, Zanchetti, A, Böhm, M, et al. 2013 ESH/ESC guidelines for the management of arterial hypertension: the task force for the Management of Arterial Hypertension of the European Society of Hypertension (ESH) and of the European Society of Cardiology (ESC). Eur Heart J. (2013) 34:2159–219. doi: 10.1093/eurheartj/eht151
23. Kapuria, D, Takyar, VK, Etzion, O, Surana, P, O'Keefe, JH, and Koh, C. Association of hepatic steatosis with subclinical atherosclerosis: systematic review and meta-analysis. Hepatol Commun. (2018) 2:873–83. doi: 10.1002/hep4.1199
24. Kang, JH, Cho, KI, Kim, SM, Lee, JY, Kim, JJ, Goo, JJ, et al. Relationship between nonalcoholic fatty liver disease and carotid artery atherosclerosis beyond metabolic disorders in non-diabetic patients. J Cardiovasc Ultrasound. (2012) 20:126–33. doi: 10.4250/jcu.2012.20.3.126
25. Brea, A, Mosquera, D, Martin, E, Martín, E, Arizti, A, Cordero, JL, et al. Nonalcoholic fatty liver disease is associated with carotid atherosclerosis: a case-control study. Arterioscler Thromb Vasc Biol. (2005) 25:1045–50. doi: 10.1161/01.ATV.0000160613.57985.18
26. Chung, GE, Choi, SY, Kim, D, Kwak, MS, Park, HE, Kim, MK, et al. Nonalcoholic fatty liver disease as a risk factor of arterial stiffness measured by the cardioankle vascular index. Medicine. (2015) 94:e654. doi: 10.1097/MD.0000000000000654
27. Kim, BJ, Kim, NH, Kim, BS, and Kang, JH. The association between nonalcoholic fatty liver disease, metabolic syndrome and arterial stiffness in nondiabetic, nonhypertensive individuals. Cardiology. (2012) 123:54–61. doi: 10.1159/000341248
28. Taharboucht, S, Guermaz, R, Brouri, M, and Chibane, A. Subclinical atherosclerosis and arterial stiffness in nonalcoholic fatty liver disease: a case-control study in Algerian population. J Med Vasc. (2021) 46:129–38. doi: 10.1016/j.jdmv.2021.03.008
29. Younes, R, Govaere, O, Petta, S, Miele, L, Tiniakos, D, Burt, A, et al. Caucasian lean subjects with non-alcoholic fatty liver disease share long-term prognosis of non-lean: time for reappraisal of BMI-driven approach? Gut. (2021) 71:382–90. doi: 10.1136/gutjnl-2020-322564
30. Kanwal, F, Kramer, JR, Li, L, Dai, J, Natarajan, Y, Yu, X, et al. Effect of metabolic traits on the risk of cirrhosis and hepatocellular cancer in nonalcoholic fatty liver disease. Hepatology. (2020) 71:808–19. doi: 10.1002/hep.31014
31. Neeland, IJ, Ross, R, Després, JP, Matsuzawa, Y, Yamashita, S, Shai, I, et al. Visceral and ectopic fat, atherosclerosis, and cardiometabolic disease: a position statement. Lancet Diabetes Endocrinol. (2019) 7:715–25. doi: 10.1016/S2213-8587(19)30084-1
32. Fan, JG, Kim, SU, and Wong, VW. New trends on obesity and NAFLD in Asia. J Hepatol. (2017) 67:862–73. doi: 10.1016/j.jhep.2017.06.003
33. Lear, SA, and Lesser, IA. A review of obesity and body fat distribution and its relationship to cardio-metabolic risk in men and women of Chinese origin. Cardiovasc Hematol Disord Drug Targets. (2012) 12:113–8. doi: 10.2174/1871529x11202020113
34. Lee, JJ, Pedley, A, Hoffmann, U, Massaro, JM, Levy, D, and Long, MT. Visceral and intrahepatic fat are associated with cardiometabolic risk factors above other ectopic fat depots: the Framingham Heart Study. Am J Med. (2018) 131:684–692.e12. doi: 10.1016/j.amjmed.2018.02.002
35. Stefan, N. Causes, consequences, and treatment of metabolically unhealthy fat distribution. Lancet Diabetes Endocrinol. (2020) 8:616–27. doi: 10.1016/S2213-8587(20)30110-8
36. Bril, F, Barb, D, Portillo-Sanchez, P, Biernacki, D, Lomonaco, R, Suman, A, et al. Metabolic and histological implications of intrahepatic triglyceride content in nonalcoholic fatty liver disease. Hepatology. (2017) 65:1132–44. doi: 10.1002/hep.28985
37. Stefan, N, Haring, HU, and Cusi, K. Non-alcoholic fatty liver disease: causes, diagnosis, cardiometabolic consequences, and treatment strategies. Lancet Diabetes Endocrinol. (2019) 7:313–24. doi: 10.1016/S2213-8587(18)30154-2
38. Cariou, B, Byrne, CD, Loomba, R, and Sanyal, AJ. Nonalcoholic fatty liver disease as a metabolic disease in humans: a literature review. Diabetes Obes Metab. (2021) 23:1069–83. doi: 10.1111/dom.14322
39. Lee, H, Lee, YH, Kim, SU, and Kim, HC. Metabolic dysfunction-associated fatty liver disease and incident cardiovascular disease risk: a nationwide cohort study. Clin Gastroenterol Hepatol. (2021) 19:2138–2147.e10. doi: 10.1016/j.cgh.2020.12.022
40. Wang, X, Wu, S, Yuan, X, Chen, S, Fu, Q, Sun, Y, et al. Metabolic dysfunction-associated fatty liver disease and mortality among Chinese adults: a prospective cohort study. J Clin Endocrinol Metab. (2022) 107:e745–55. doi: 10.1210/clinem/dgab644
41. Stefan, N, and Cusi, K. A global view of the interplay between non-alcoholic fatty liver disease and diabetes. Lancet Diabetes Endocrinol. (2022) 10:284–96. doi: 10.1016/S2213-8587(22)00003-1
42. Stefan, N, Schick, F, Birkenfeld, AL, Häring, HU, and White, MF. The role of hepatokines in NAFLD. Cell Metab. (2023) 35:236–52. doi: 10.1016/j.cmet.2023.01.006
43. Younossi, ZM, Corey, KE, and Lim, JK. AGA clinical practice update on lifestyle modification using diet and exercise to achieve weight loss in the management of nonalcoholic fatty liver disease: expert review. Gastroenterology. (2021) 160:912–8. doi: 10.1053/j.gastro.2020.11.051
44. Houttu, V, Csader, S, Nieuwdorp, M, Holleboom, AG, and Schwab, U. Dietary interventions in patients with non-alcoholic fatty liver disease: a systematic review and meta-analysis. Front Nutr. (2021) 8:716783. doi: 10.3389/fnut.2021.716783
45. Yin, C, Li, Z, Xiang, Y, Peng, H, Yang, P, Yuan, S, et al. Effect of intermittent fasting on non-alcoholic fatty liver disease: systematic review and meta-analysis. Front Nutr. (2021) 8:709683. doi: 10.3389/fnut.2021.709683
46. Montemayor, S, Mascaró, CM, Ugarriza, L, Casares, M, Llompart, I, Abete, I, et al. Adherence to Mediterranean diet and NAFLD in patients with metabolic syndrome: the FLIPAN study. Nutrients. (2022) 14:3186. doi: 10.3390/nu14153186
47. Chen, YP, Lu, FB, Hu, YB, Xu, LM, Zheng, MH, and Hu, ED. A systematic review and a dose-response meta-analysis of coffee dose and nonalcoholic fatty liver disease. Clin Nutr. (2019) 38:2552–7. doi: 10.1016/j.clnu.2018.11.030
48. Sanyal, AJ, Chalasani, N, Kowdley, KV, McCullough, A, Diehl, AM, Bass, NM, et al. Pioglitazone, vitamin E, or placebo for nonalcoholic steatohepatitis. N Engl J Med. (2010) 362:1675–85. doi: 10.1056/NEJMoa0907929
49. Xie, ZQ, Li, HX, Tan, WL, Yang, L, Ma, XW, Li, WX, et al. Association of serum vitamin C with NAFLD and MAFLD among adults in the United States. Front Nutr. (2022) 8:795391. doi: 10.3389/fnut.2021.795391
50. Stefan, N, Häring, HU, Hu, FB, and Schulze, MB. Metabolically healthy obesity: epidemiology, mechanisms, and clinical implications. Lancet Diabetes Endocrinol. (2013) 1:152–62. doi: 10.1016/S2213-8587(13)70062-7
51. Bugianesi, E, Pagotto, U, Manini, R, Vanni, E, Gastaldelli, A, de Iasio, R, et al. Plasma adiponectin in nonalcoholic fatty liver is related to hepatic insulin resistance and hepatic fat content, not to liver disease severity. J Clin Endocrinol Metab. (2005) 90:3498–504. doi: 10.1210/jc.2004-2240
52. Kwok, RM, Torres, DM, and Harrison, SA. Vitamin D and nonalcoholic fatty liver disease (NAFLD): is it more than just an association? Hepatology. (2013) 58:1166–74. doi: 10.1002/hep.26390
Keywords: cross-sectional study, metabolic status, obesity phenotype, subclinical atherosclerosis, non-alcoholic fatty liver disease
Citation: Wang Y, Yuan T, Deng S, Zhu X, Deng Y, Liu X, Liu L and Wang C (2023) Metabolic health phenotype better predicts subclinical atherosclerosis than body mass index-based obesity phenotype in the non-alcoholic fatty liver disease population. Front. Nutr. 10:1104859. doi: 10.3389/fnut.2023.1104859
Edited by:
Ellen E. Blaak, Maastricht University, NetherlandsReviewed by:
Norbert Stefan, University of Tübingen, GermanyHaseeb Sattar, Huazhong University of Science and Technology, China
Copyright © 2023 Wang, Yuan, Deng, Zhu, Deng, Liu, Liu and Wang. This is an open-access article distributed under the terms of the Creative Commons Attribution License (CC BY). The use, distribution or reproduction in other forums is permitted, provided the original author(s) and the copyright owner(s) are credited and that the original publication in this journal is cited, in accordance with accepted academic practice. No use, distribution or reproduction is permitted which does not comply with these terms.
*Correspondence: Lei Liu, bGl1bGVpMTk4OTA0MDNAMTYzLmNvbQ==; Changfa Wang, d29uZ2RvdmVAMTYzLmNvbQ==
†These authors have contributed equally to this work
‡ORCID: Lei Liu orcid.org/0000-0002-3448-4264