- 1Department of Geriatrics, Ruijin Hospital, Shanghai Jiao Tong University School of Medicine, Shanghai, China
- 2Medical Center on Aging of Ruijin Hospital, Shanghai Jiao Tong University School of Medicine, Shanghai, China
- 3Department of Neurology and Institute of Neurology, Ruijin Hospital, Shanghai Jiao Tong University School of Medicine, Shanghai, China
- 4Department of Neurology, Shanghai University of Medicine and Health Sciences Affiliated Sixth People's Hospital South Campus, Shanghai, China
- 5Department of Gastroenterology, Jiading District Central Hospital Affiliated Shanghai University of Medicine and Health Sciences, Shanghai, China
- 6Department of Neurology, Jiading District Central Hospital Affiliated Shanghai University of Medicine and Health Sciences, Shanghai, China
Background: There are many metabolic pathway abnormalities in Alzheimer's disease (AD). Several studies have linked branched-chain amino acid (BCAA) metabolism disorders with AD but have not obtained consistent results. The purpose of this study is to explore the causal association between BCAA concentration and the risk of AD.
Methods: A bidirectional Mendelian randomized (MR) study was applied to explore the causal effect between BCAA level and the risk of AD. Genetic instrumental variables from the genome-wide association study (GWAS) of serum BCAA levels [total BCAAs (115,047 participants), valine (115,048 participants), leucine (115,074 participants), and isoleucine (115,075 participants)] from the UK Biobank and AD (21,982 AD cases and 41,944 controls) from the International Genomics of Alzheimer's Project were applied to explore the causal effect through the inverse variance-weighted (IVW) method, MR-Egger, and weighted median, accompanied by multiple pluripotency and heterogeneity tests.
Results: The forward MR analysis showed that there was no causal effect of total BCAAs (OR: 1.067, 95% CI: 0.838–1.358; p = 0.838), valine (OR: 1.106, 95% CI: 0.917–1.333; p = 0.292), leucine (OR: 1.096, 95% CI: 0.861–1.396; p = 0.659), and isoleucine (OR: 1.457, 95% CI: 1.024–2.742; p = 0.037) levels on the risk of AD. The reverse analysis showed that AD was related to reduced levels of total BCAAs (OR: 0.979, 95% CI: 0.989–0.990; p < 0.001), valine (OR: 0.977, 95% CI: 0.963–0.991; p = 0.001), leucine (OR: 0.983, 95% CI: 0.973–0.994; p = 0.002), and isoleucine (OR: 0.982, 95% CI: 0.971–0.992; p = 0.001).
Conclusion: We provide robust evidence that AD was associated with a decreased level of BCAAs, which can serve as a marker for early diagnosis of AD.
Introduction
Alzheimer's disease (AD), the main type of dementia, becomes one of the most serious public health threats in the world (1). It is estimated that there are currently more than 50 million people worldwide with dementia, which is expected to triple in 2050 (2). Among them, AD accounts for 60–80% of dementia (3). Typical pathological features of AD include extracellular amyloid-β (Aβ) plaques accumulated by Aβ and intracellular neurofibrillary tangles formed by phosphorylated tau (4). However, there is still a lack of accurate explanation of the pathogenesis and effective disease-modifying treatment for AD (5). Increased evidence suggests that the disruption of various metabolic pathways is another important feature of AD (6, 7). Metabolites are the end biochemical products of various biological pathways, such as amino acids, peptides, lipids, and nucleic acids (8). They can reflect the alteration in the complex biological pathways involved in AD caused by the interaction of genetic, environmental, and behavioral factors (6).
Branched-chain amino acids (BCAAs), including leucine (Leu), isoleucine (Ile), and valine (Val), are three types of essential amino acids in the human diet (9). They are not only involved in protein synthesis but also possess various metabolic pathways (10, 11). Altered BCAA metabolism has been shown to be associated with AD in an increasing number of studies (12). However, different studies showed controversial and mixed results. For instance, a previous study of the cerebrospinal fluid (CSF) and plasma amino acid composition has demonstrated a significant reduction of valine in AD patients compared to healthy controls (HC) (13). In a study of sporadic AD patients without receiving any medication, alterations of 23 metabolites were detected, including significantly decreased valine levels (14). In addition, a longitudinal study in APP/PS1 transgenic mice involving profiling of the brain and the plasma metabolome has found seriously disturbed polyamines and BCAA metabolism (15). Notably, the plasma levels of valine were shown to be significantly reduced in AD mice. In a more recent study, lower plasma valine level was shown to correlate with the rate of cognitive decline (16). Nonetheless, there were elevated BCAA concentrations in the serum of AD patients in a small sample study (17). Similarly, elevated isoleucine levels have been observed in patients with mild cognitive impairment (MCI) (18). These heterogeneous results may be related to the susceptibility of metabolites influenced by multiple factors, such as lifestyle and diet, immune response, genetic variations, and gut microbiota (19). These uncontrollable confounding factors make it difficult to distinguish symptoms from causes. Therefore, the use of more reliable research methods will help to elucidate the causal association between BCAA levels and the risk of AD.
Mendelian randomization (MR) study is a methodology that can be applied to explore the causal relationships between exposures (risk factors) and outcomes (diseases) by using genetic variants (20). The genetic variants are only related to the risk factors but are not affected by any confounders (21). In this study, a bidirectional Mendelian randomized approach was applied to explore the causal effects between BCAA levels and the risk of AD.
Methods
Study design overview
We performed a bidirectional Mendelian randomized study to assess the causal effect between BCAA levels and the risk of AD (Figure 1). The bidirectional Mendelian randomization study was built on the following three assumptions: First of all, the instrumental variables (IVs) were not related to the confounders. Second, there was a strong correlation between IVs and exposure. Third, IVs can affect outcomes (AD) only through exposure and not through other pathways. The analysis of this study was based on genome-wide association study (GWAS) data of BCAAs and Alzheimer's disease in the public database. Therefore, ethics approval and consent are not required for this study.
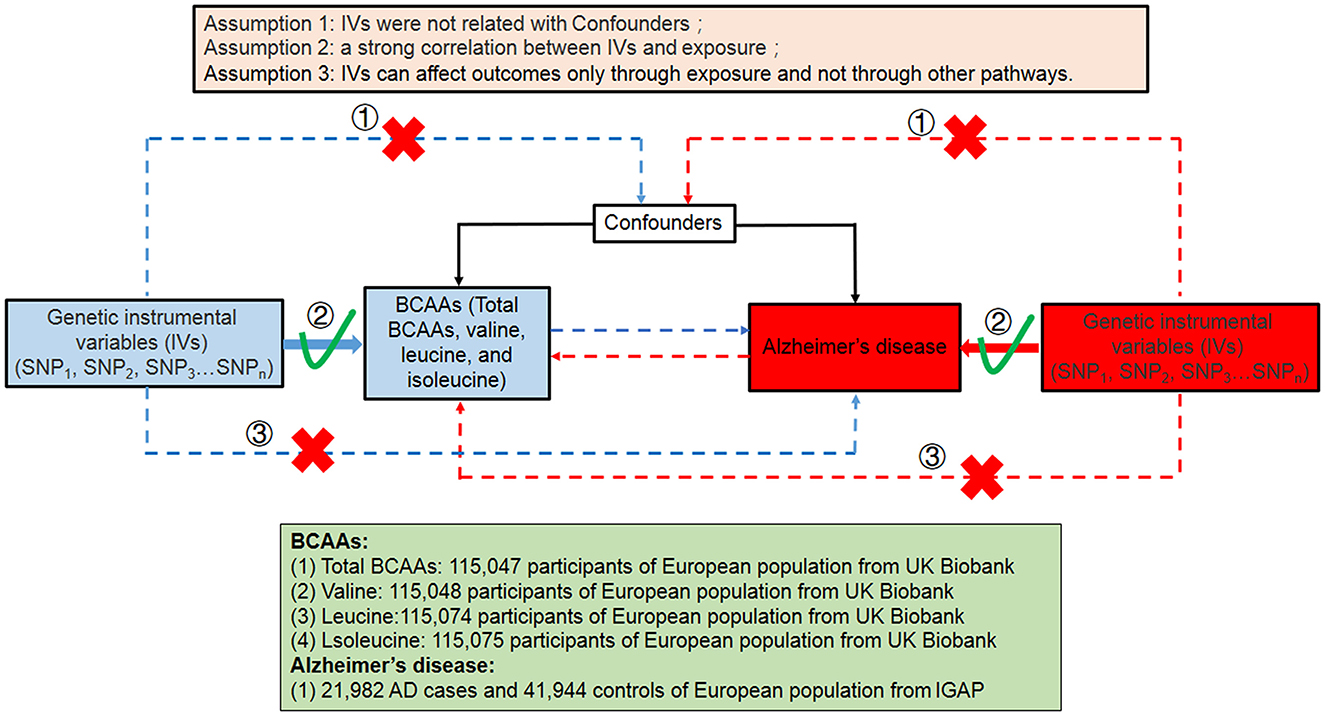
Figure 1. Summary of this bidirectional MR study between the level of BCAAs and the risk of AD in this study. SNP, single-nucleotide polymorphism; BCAAs, branched-chain amino acids; AD, Alzheimer's disease.
Data sources and selection of genetic instruments
The genetic summary statistics of AD (21,982 AD cases and 41,944 controls) were accessed from the International Genomics of Alzheimer's Project (IGAP), which was composed of four consortia: Cohorts for Heart and Aging Research in Genomic Epidemiology Consortium (CHARGE), the European Alzheimer's Disease Initiative (EADI), Alzheimer's Disease Genetics Consortium (ADGC), and Genetic and Environmental Risk in AD/Defining Genetic, Polygenic and Environmental Risk for Alzheimer's Disease Consortium (GERAD/PERADES) (22). For BCAAs (total BCAAs, valine, leucine, and isoleucine), the GWAS statistics were obtained from the MRC IEU OpenGWAS project (https://gwas.mrcieu.ac.uk/), including 115,047 participants for total BCAAs (GWAS ID “met-d-Total_BCAA”), 115,048 participants for valine (GWAS ID “met-d-Val”), 115,074 participants for leucine (GWAS ID “met-d-Leu”), and 115,075 participants for isoleucine (GWAS ID “met-d-Ile”) from the UK Biobank. All participants were of European ancestry.
Instrumental variables (IVs) for AD and BCAAs were extracted under the same criteria. To be specific, the GWAS statistical difference threshold of all relevant SNPs from each GWAS was set to P < 5 × 10−8. The PLINK clumping algorithm was applied to prune for the independence of SNPs in linkage disequilibrium under the threshold of r2 < 0.001 in a 10,000 kb window (23). The palindromic SNPs with a minor allele frequency (MAF) of <0.01 were excluded from the aforementioned instrument SNPs. The PhenoScanner GWAS database (http://phenoscanner.medschl.cam.ac.uk) was applied to remove possible confounding of the exposure–outcome associations (24). In this study, gender, age, diabetes, and cardiovascular disease were selected as confounders. The SNPs associated with them were excluded. Furthermore, the F statistic was calculated to assess the strength of the selected genetic variants (25). The SNPs used as IVs in this study are presented in Supplementary Table S1. The F statistic is presented in Supplementary Table S2.
Mendelian randomized analysis
Two-sample MR was applied to explore the causal effect between BCAA levels and the risk of AD. The inverse variance-weighted (IVW) method was applied to the standard MR analysis. In addition, the MR-Egger and weighted median were also performed to verify the causal effect (21, 26). Mendelian randomization pleiotropy residual sum and outlier (MR-PRESSO) global test was conducted to identify the horizontal pleiotropic of IVs (26). Cochran's Q statistic was applied to assess the heterogeneity of IVW and MR-Egger (27). The MR-Egger intercept test was also used to estimate the potential horizontal pleiotropy of the MR results. In addition, the leave-one-out analysis was also used to eliminate potential pleiotropy by assessing the effects of a single IV on causal effect by removing each IV from the IVW method (28). All analyses in this study were performed through the “two-sample MR” and the “MR-PRESSO” packages in R software (v3.60). As four types of exposures were analyzed, the statistically significant threshold was set as P < 0.0125 after the Bonferroni correction.
Results
Genetically predicted BCAA levels on the risk of AD
The causal effect of BCAA levels on the risk of AD was conducted through the inverse variance-weighted (IVW) MR method. In addition, MR-Egger and weighted median were applied to verify the results of the IVW method. After removing SNPs, the results showed that there were no casual effects on the levels of total BCAAs (IVW, OR: 1.067, 95% CI: 0.838–1.358; p = 0.838), valine (IVW, OR: 1.106, 95% CI: 0.917–1.333; p = 0.292), leucine (IVW, OR: 1.096, 95% CI: 0.861–1.396; p = 0.659), and isoleucine (IVW, OR: 1.457, 95% CI: 1.024–2.742; p = 0.037) on the risk of AD (Figures 2A–D, Table 1). Similarly, the results for MR-Egger and weighted median do not suggest an effect of BCAA levels on the risk of AD. Based on these results, we found no causal effect of BCAA levels on the risk of AD.
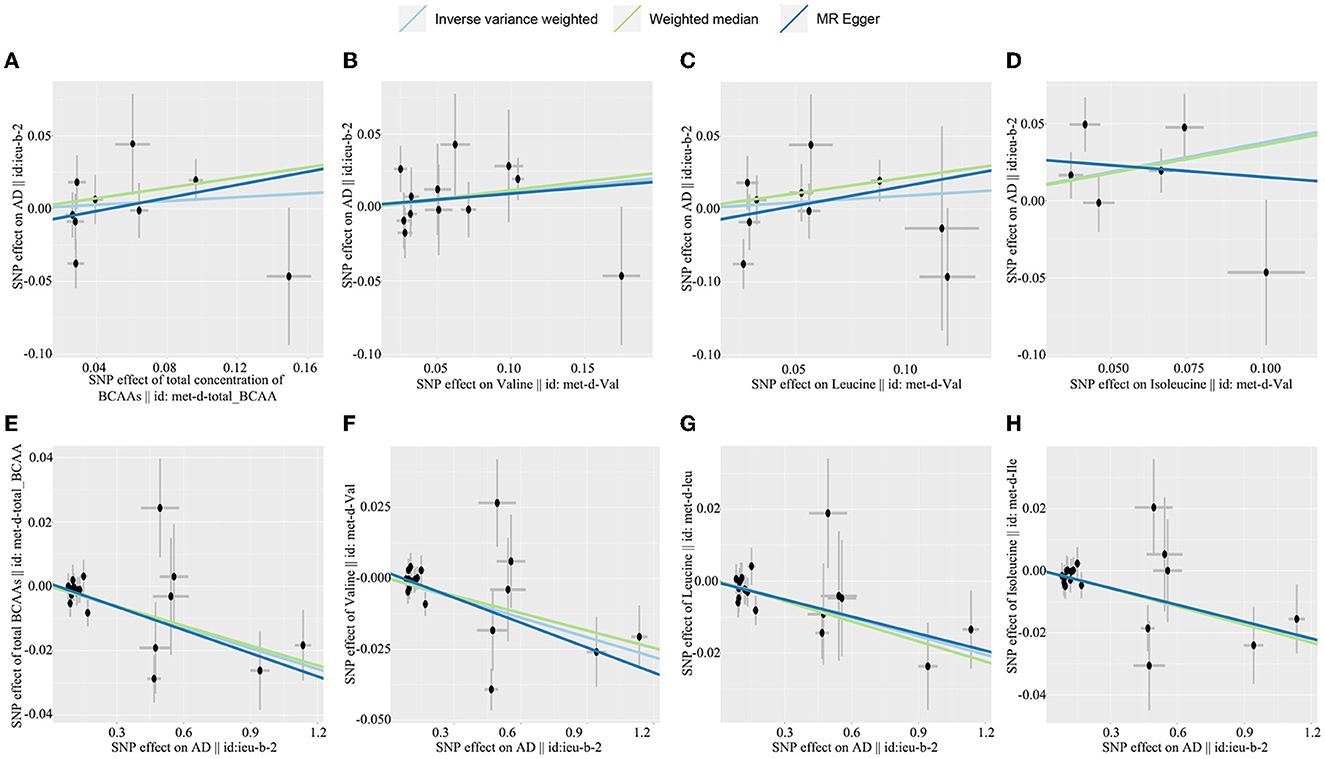
Figure 2. Scatterplot of the genetic causal effect sbetween BCAAs level the risk of AD. (A) Total BCAAs on AD, (B) valine on AD, (C) leucine on AD, (D) isoleucine on AD, (E) AD on total BCAAs, (F) AD on valine, (G) AD on leucine, (H) AD on isoleucine. BCAAs, branched-chain amino acids; AD, Alzheimer's disease.
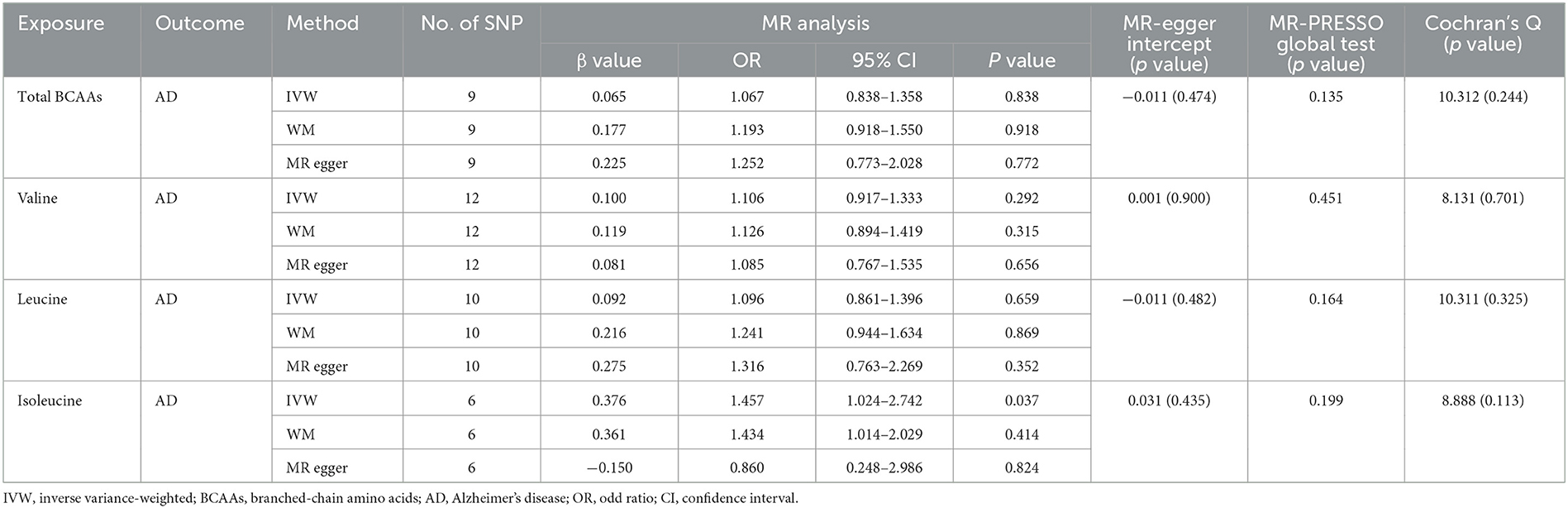
Table 1. Mendelian randomization analysis explore the genetic causal effects of the BCAAs on the risk of AD.
Genetically predicted AD on the BCAA levels
In the reverse MR analysis, we explored the causal effect of AD on the BCAA levels through IVW, MR-Egger, and weighted median. Our investigation revealed that AD was related to a decreased level of total BCAAs (IVW, OR: 0.979, 95% CI: 0.989–0.990, p < 0.001), valine (IVW, OR: 0.977, 95% CI: 0.963–0.991; p = 0.001), leucine (IVW, OR: 0.983, 95% CI: 0.973-−0.994, p = 0.002), and isoleucine (IVW, OR: 0.982, 95% CI: 0.971–0.992, p = 0.001) (Figures 2E–H, Table 2). Similarly, the results of MR-Egger and weighted median were consistent with the results of the IVW method. Taken together, these results revealed that AD was obviously associated with decreased BCAA levels.
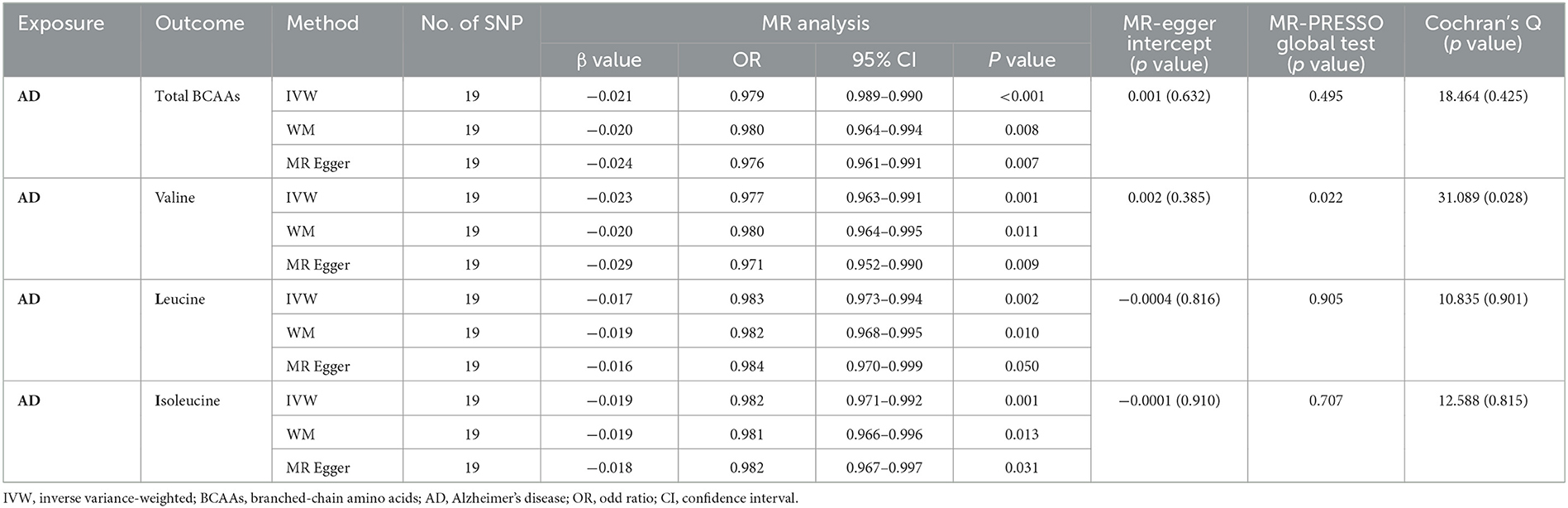
Table 2. Mendelian randomization analysis explore the genetic causal effects of AD on the level of BCAAs.
Sensitivity analyses
There were no obvious heterogeneities in the causal effect between AD and BCAAs (Tables 1, 2). The intercept of the MR-Egger regression and MR-PRESSO test revealed no obvious horizontal pleiotropy (Tables 1, 2). In addition, the included instrumental variables show apparent symmetry in the funnel plot to exclude the directional pleiotropy (Supplementary Figure S1). Meanwhile, the leave-one-out analysis showed all SNPs contributing to consistent causal estimates (Supplementary Figure S2). The aforementioned analysis proves the reliability of the results of the study.
Discussion
Alzheimer's disease, the most common type of dementia, currently lacks disease-modifying treatment strategies. To make matters worse, the current understanding of the pathogenesis of AD is limited. As a type of essential amino acid, BCAAs have been proven to be closely associated with AD (3, 29). The alteration of BCAA concentration in AD patients is correlated with disease progression and AD-related pathological features (30, 31). However, a causal association between AD and BCAA has not been established. In this study, we attempt to explore the causal effect between AD and BCAAs (total BCAAs, valine, leucine, and isoleucine) using a bidirectional two-sample MR study. The results do not show evidence that BCAA levels are causally related to the risk of AD. Conversely, the reverse MR analysis suggests that AD is significantly associated with decreased BCAA levels, suggesting that AD is the cause but not the result of changed BCAA levels.
Several previous observational cohort studies have linked BCAAs to AD. An earlier study found that there was reduced levels of leucine and valine in the CSF of patients with AD, but no statistically significant differences in plasma (13). In Alzheimer's Disease Neuroimaging Initiative-1 (ADNI-1) cohort, there was a decreased serum valine level in AD patients compared to HC and stable mild cognitive impairment (sMCI) patients (32). Similarly, serum valine levels were lower in patients with progressive MCI (pMCI) than in HC (32). More importantly, serum valine levels in pMCI patients were negatively correlated with Tau and pTau levels in CSF and could be used to predict the risk of progression from MCI to AD (32). In a prospective study of eight cohorts, BCAAs were found to be negatively associated with dementia and the risk of AD (31). However, in another observational study, serum isoleucine levels were elevated in dementia, compared with HC (33). Similarly, elevated blood levels of BCAAs in patients with AD were reported in a small sample study cohort (17). In APP/PS1 mice model, there was an increased plasma BCAA level compared with wide-type mice (34). After high-fat feeding, the plasma BCAA level was significantly upregulated in the WT group, which was not observed in the APP/PS1 group (34). A high saturated fat/glycemic index diet significantly increased CSF BCAA levels in MCI patients but had no significant effect on the HC group (35). Combined with the results of this study, we speculate that these inconsistent results occurred in ordinary observational studies due to the limited sample size and the susceptibility of BCAAs to various confounding factors such as diet. The results of our study explain the causal relationship between AD and BCAAs from the root.
In 2017, Larsson and Markus also reported the results of a study using Mendelian randomization to explore the causal association of BCAAs with the risk of AD (36). The results showed that only higher isoleucine levels were associated with the risk of AD but not leucine and valine (36). Our study differs from other studies in several ways. In this study, the GWAS datasets related to BCAAs are selected with a larger sample size, containing 115,047 participants. From the perspective of methodology, Larsson and Markus (36) only used a single methodology to illustrate the effect of BCAAs on the risk of AD. In our study, IVW, MR-Egger, and weighted median are adopted in the analysis for Mendelian randomization, which provides an important guarantee for the reliability of our research results.
Branched-chain amino acids (BCAAs) are essential amino acids and account for one-third of the total amino acid content of the human body (30). After being absorbed by the gut, BCAAs are widely distributed in many organs for metabolism, mainly including the muscle, brain, liver, and adipose tissue (37). Among them, the muscle is the main metabolic place of BCAAs, accounting for approximately 50% (30, 38). In addition, the brain also absorbs a proportion of BCAAs in the blood (30). In the mammalian brain, BCAAs are involved in multiple functions, including neurotransmitter synthesis, protein synthesis, and energy production (39). In this study, AD patients are found to have reduced BCAA levels, which may be related to BCAA intake and metabolic processes. Circulating BCAAs are derived from diet, proteolysis, and gut microbiota (40). First, changes in gastrointestinal function have been observed in the AD mice model (41, 42). This may lead to reduced absorption of BCAAs from the diet in AD. Second, AD or MCI patients usually experience subclinical malnutrition (43). This resulted in reduced protein reserve and proteolysis levels in AD. Third, a series of studies in recent years have demonstrated the imbalance of gut microbiota in AD (12, 41). This may lead to changes in the abundance of gut microbiota related to BCAAs metabolism and ultimately reduce the level of circulating BCAAs. However, these are just some possible reasons why AD has reduced BCAA levels. Further studies are needed to clarify the specific mechanism. In addition, supplementing seven amino acids (leucine, phenylalanine, lysine hydrochloride, isoleucine, histidine hydrochloride, valine, and tryptophan) can improve cognitive, psychological, and social functions in middle-aged and older adults (44). Furthermore, supplementing L-norvaline (an isoform of valine) for 2 months can reverse cognitive decline and synaptic loss in triple-transgenic (3 × Tg) mice at the age of 4 months (45). Therefore, the supplementation of BCAAs may be one of the strategies to delay cognitive decline in AD patients.
Conclusion
This bidirectional MR study indicates that AD is associated with decreased BCAA levels, which can serve as a marker for early diagnosis of AD. Future studies need to further clarify how AD leads to lower levels of BCAAs.
Data availability statement
The original contributions presented in the study are included in the article/Supplementary material, further inquiries can be directed to the corresponding authors.
Author contributions
H-dT and G-yD designed the study and prepared the manuscript. X-hQ and X-lL developed the methodology and analyzed the data. All authors discussed the results and approved the manuscript.
Funding
This study was supported by the National Natural Science Foundation of China [81971014], the Hundred Faculty Talent Pool Program at Shanghai University of Medicine & Health Sciences in China, and the Shanghai Municipal Commission of Health and Family Planning [20184Y0056].
Acknowledgments
We sincerely appreciated the data provided by the MRC IEU OpenGWAS project.
Conflict of interest
The authors declare that the research was conducted in the absence of any commercial or financial relationships that could be construed as a potential conflict of interest.
Publisher's note
All claims expressed in this article are solely those of the authors and do not necessarily represent those of their affiliated organizations, or those of the publisher, the editors and the reviewers. Any product that may be evaluated in this article, or claim that may be made by its manufacturer, is not guaranteed or endorsed by the publisher.
Supplementary material
The Supplementary Material for this article can be found online at: https://www.frontiersin.org/articles/10.3389/fnut.2023.1103303/full#supplementary-material
Supplementary Figure S1. Funnel plot of the causal effect between BCAAs level and the risk of AD. (A) Total BCAAs on AD, (B) valine on AD, (C) leucine on AD, (D) isoleucine on AD, (E) AD on total BCAAs, (F) AD on valine, (G) AD on leucine, (H) AD on isoleucine. Each instrumental SNP was represented by a black dot. SNPs, single-nucleotide polymorphisms; BCAAs, branched-chain amino acids; AD, Alzheimer's disease.
Supplementary Figure S2. Leave-one-out analysis of the causal effect between BCAAs level and the risk of AD. (A) Total BCAAs on AD, (B) valine on AD, (C) leucine on AD, (D) isoleucine on AD, (E) AD on total BCAAs, (F) AD on valine, (G) AD on leucine, (H) AD on isoleucine. BCAAs, branched-chain amino acids; AD, Alzheimer's disease.
Supplementary Table S1. Characteristics of selected SNPs for analysis of the causal effect between BCAA level and the risk of AD from IGAP.
Supplementary Table S2. F statistic in this study.
References
1. Jia L, Quan M, Fu Y, Zhao T, Li Y, Wei C, et al. Dementia in China: epidemiology, clinical management, and research advances. Lancet Neurol. (2020) 19:81–92. doi: 10.1016/S1474-4422(19)30290-X
2. Irwin MR, Vitiello MV. Implications of sleep disturbance and inflammation for Alzheimer's disease dementia. Lancet Neurol. (2019) 18:296–306. doi: 10.1016/S1474-4422(18)30450-2
3. Jasbi P, Shi X, Chu P, Elliott N, Hudson H, Jones D, et al. Metabolic profiling of neocortical tissue discriminates Alzheimer's disease from mild cognitive impairment, high pathology controls, and normal controls. J Proteome Res. (2021) 20:4303–17. doi: 10.1021/acs.jproteome.1c00290
4. Fani L, Ahmad S, Ikram MK, Ghanbari M, Ikram MA. Immunity and amyloid beta, total tau and neurofilament light chain: Findings from a community-based cohort study. Alzheimer's Demen. (2021) 17:446–56. doi: 10.1002/alz.12212
5. Arvanitakis Z, Shah RC, Bennett DA. Diagnosis and management of dementia: review. Jama. (2019) 322:1589–99. doi: 10.1001/jama.2019.4782
6. Varma VR, Oommen AM, Varma S, Casanova R, An Y, Andrews RM, et al. Brain and blood metabolite signatures of pathology and progression in Alzheimer disease: A targeted metabolomics study. PLoS Med. (2018) 15:e1002482. doi: 10.1371/journal.pmed.1002482
7. An Y, Varma VR, Varma S, Casanova R, Dammer E, Pletnikova O, et al. Evidence for brain glucose dysregulation in Alzheimer's disease. Alzheimer's Demen. (2018) 14:318–29. doi: 10.1016/j.jalz.2017.09.011
8. Lord J, Jermy B, Green R, Wong A, Xu J, Legido-Quigley C, et al. Mendelian randomization identifies blood metabolites previously linked to midlife cognition as causal candidates in Alzheimer's disease. Proc Natl Acad Sci U S A. (2021) 118:e2009808118. doi: 10.1073/pnas.2009808118
9. Biswas D, Duffley L, Pulinilkunnil T. Role of branched-chain amino acid-catabolizing enzymes in intertissue signaling, metabolic remodeling, and energy homeostasis. FASEB J. (2019) 33:8711–31. doi: 10.1096/fj.201802842RR
10. Dullius A, Fassina P, Giroldi M, Goettert MI, Volken CF, de Souza. A biotechnological approach for the production of branched chain amino acid containing bioactive peptides to improve human health: A review. Food Res Int. (2020) 131:109002. doi: 10.1016/j.foodres.2020.109002
11. Sivanand S, Vander Heiden MG. Emerging roles for branched-chain amino acid metabolism in cancer. Cancer Cell. (2020) 37:147–56. doi: 10.1016/j.ccell.2019.12.011
12. Qian XH, Song XX, Liu XL, Chen SD, Tang HD. Inflammatory pathways in Alzheimer's disease mediated by gut microbiota. Ageing Res Rev. (2021) 68:101317. doi: 10.1016/j.arr.2021.101317
13. Basun H, Forssell LG, Almkvist O, Cowburn RF, Eklöf R, Winblad B, et al. Amino acid concentrations in cerebrospinal fluid and plasma in Alzheimer's disease and healthy control subjects. J Neural Transm Parkinson's Dis Dement Section. (1990) 2:295–304. doi: 10.1007/BF02252924
14. González-Domínguez R, García-Barrera T, Gómez-Ariza JL. Metabolite profiling for the identification of altered metabolic pathways in Alzheimer's disease. J Pharm Biomed Anal. (2015) 107:75–81. doi: 10.1016/j.jpba.2014.10.010
15. Pan X, Nasaruddin MB, Elliott CT, McGuinness B, Passmore AP, Kehoe PG, et al. Alzheimer's disease-like pathology has transient effects on the brain and blood metabolome. Neurobiol Aging. (2016) 38:151–63. doi: 10.1016/j.neurobiolaging.2015.11.014
16. Toledo JB, Arnold M, Kastenmüller G, Chang R, Baillie RA, Han X, et al. Metabolic network failures in Alzheimer's disease: A biochemical road map. Alzheimer's Demen. (2017) 13:965–84. doi: 10.1016/j.jalz.2017.01.020
17. Li H, Ye D, Xie W, Hua F, Yang Y, Wu J, et al. Defect of branched-chain amino acid metabolism promotes the development of Alzheimer's disease by targeting the mTOR signaling. Biosci Rep. (2018) 38:BSR20180127. doi: 10.1042/BSR20180127
18. Wang X, Sun G, Feng T, Zhang J, Huang X, Wang T, et al. Sodium oligomannate therapeutically remodels gut microbiota and suppresses gut bacterial amino acids-shaped neuroinflammation to inhibit Alzheimer's disease progression. Cell Res. (2019) 29:787–803. doi: 10.1038/s41422-019-0216-x
19. Horgusluoglu E, Neff R, Song WM, Wang M, Wang Q, Arnold M, et al. Integrative metabolomics-genomics approach reveals key metabolic pathways and regulators of Alzheimer's disease. Alzheimer's Demen. (2022) 18:1260–78. doi: 10.1002/alz.12468
20. Skrivankova VW, Richmond RC, Woolf BAR, Yarmolinsky J, Davies NM, Swanson SA. Strengthening the reporting of observational studies in epidemiology using mendelian randomization: The STROBE-MR statement. Jama. (2021) 326:1614–21. doi: 10.1001/jama.2021.18236
21. Bowden J, Davey Smith G, Burgess S. Mendelian randomization with invalid instruments: effect estimation and bias detection through Egger regression. Int J Epidemiol. (2015) 44:512–25. doi: 10.1093/ije/dyv080
22. Kunkle BW, Grenier-Boley B, Sims R, Bis JC, Damotte V, Naj AC, et al. Genetic meta-analysis of diagnosed Alzheimer's disease identifies new risk loci and implicates Aβ, tau, immunity and lipid processing. Nat Genet. (2019) 51:414–30. doi: 10.1038/s41588-019-0358-2
23. Purcell S, Neale B, Todd-Brown K, Thomas L, Ferreira MA, Bender D, et al. PLINK: a tool set for whole-genome association and population-based linkage analyses. Am J Hum Genet. (2007) 81:559–75. doi: 10.1086/519795
24. Staley JR, Blackshaw J, Kamat MA, Ellis S, Surendran P, Sun BB, et al. PhenoScanner: a database of human genotype-phenotype associations. Bioinformatics. (2016) 32:3207–9. doi: 10.1093/bioinformatics/btw373
25. Lawlor DA, Harbord RM, Sterne JA, Timpson N, Davey Smith G. Mendelian randomization: using genes as instruments for making causal inferences in epidemiology. Stat Med. (2008) 27:1133–63. doi: 10.1002/sim.3034
26. Verbanck M, Chen CY, Neale B, Do R. Detection of widespread horizontal pleiotropy in causal relationships inferred from Mendelian randomization between complex traits and diseases. Nat Genet. (2018) 50:693–8. doi: 10.1038/s41588-018-0099-7
27. Hemani G, Bowden J, Davey Smith G. Evaluating the potential role of pleiotropy in Mendelian randomization studies. Hum Mol Genet. (2018) 27:R195–r208. doi: 10.1093/hmg/ddy163
28. Hemani G, Zheng J, Elsworth B, Wade KH, Haberland V, Baird D, et al. The MR-Base platform supports systematic causal inference across the human phenome. Elife. (2018) 7:e34408. doi: 10.7554/eLife.34408
29. Jia L, Yang J, Zhu M, Pang Y, Wang Q, Wei Q, et al. A metabolite panel that differentiates Alzheimer's disease from other dementia types. Alzheimer's Demen. (2022) 18:1345–56. doi: 10.1002/alz.12484
30. Polis B, Samson AO. Role of the metabolism of branched-chain amino acids in the development of Alzheimer's disease and other metabolic disorders. Neural Regen Res. (2020) 15:1460–70. doi: 10.4103/1673-5374.274328
31. Tynkkynen J, Chouraki V, van der Lee SJ, Hernesniemi J, Yang Q, Li S, et al. Association of branched-chain amino acids and other circulating metabolites with risk of incident dementia and Alzheimer's disease: A prospective study in eight cohorts. Alzheimer's Demen. (2018) 14:723–33. doi: 10.1016/j.jalz.2018.01.003
32. Xiong YL, Therriault J, Ren SJ, Jing XJ, Zhang H. The associations of serum valine with mild cognitive impairment and Alzheimer's disease. Aging Clin Exp Res. (2022) 34:1807–17. doi: 10.1007/s40520-022-02120-0
33. Socha E, Kośliński P, Koba M, Madra-Gackowska K, Kedziora-Kornatowska K, Gackowski M, et al. Amino acid levels as potential biomarker of elderly patients with dementia. Brain Sci. (2020) 10:917. doi: 10.3390/brainsci10120914
34. Ruiz HH, Chi T, Shin AC, Lindtner C, Hsieh W, Ehrlich M, et al. Increased susceptibility to metabolic dysregulation in a mouse model of Alzheimer's disease is associated with impaired hypothalamic insulin signaling and elevated BCAA levels. Alzheimer's Demen. (2016) 12:851–61. doi: 10.1016/j.jalz.2016.01.008
35. Russin KJ, Nair KS, Montine TJ, Baker LD, Craft S. Diet effects on cerebrospinal fluid amino acids levels in adults with normal cognition and mild cognitive impairment. J Alzheimer's Dis. (2021) 84:843–53. doi: 10.3233/JAD-210471
36. Larsson SC, Markus HS. Branched-chain amino acids and Alzheimer's disease: a Mendelian randomization analysis. Sci Rep. (2017) 7:13604. doi: 10.1038/s41598-017-12931-1
37. Brosnan JT, Brosnan ME. Branched-chain amino acids: enzyme and substrate regulation. J Nutr. (2006) 136:207s–11s. doi: 10.1093/jn/136.1.207S
38. Trautman ME, Richardson NE, Lamming DW. Protein restriction and branched-chain amino acid restriction promote geroprotective shifts in metabolism. Aging Cell. (2022) 21:e13626. doi: 10.1111/acel.13626
39. J.D. Fernstrom. Branched-chain amino acids and brain function. J Nutr. (2005) 135:1539s–46s. doi: 10.1093/jn/135.6.1539S
40. McGarrah RW, White PJ. Branched-chain amino acids in cardiovascular disease. Nat Rev Cardiol. (2023) 20:77–89. doi: 10.1038/s41569-022-00760-3
41. Qian XH, Liu XL, Chen G, Chen SD, Tang HD. Injection of amyloid-β to lateral ventricle induces gut microbiota dysbiosis in association with inhibition of cholinergic anti-inflammatory pathways in Alzheimer's disease. J Neuroinflam. (2022) 19:236. doi: 10.1186/s12974-022-02599-4
42. Pellegrini C, Fornai M, D'Antongiovanni V, Antonioli L, Bernardini N, Derkinderen P. The intestinal barrier in disorders of the central nervous system. Lancet Gastroenterol Hepatol. (2023) 8:66–80. doi: 10.1016/S2468-1253(22)00241-2
43. S Ogawa. Nutritional management of older adults with cognitive decline and dementia. Geriatr Gerontol Int. (2014) 14:17–22. doi: 10.1111/ggi.12252
44. Suzuki H, Yamashiro D, Ogawa S, Kobayashi M, Cho D, Iizuka A, et al. Intake of seven essential amino acids improves cognitive function and psychological and social function in middle-aged and older adults: A double-blind, randomized, placebo-controlled trial. Front Nutr. (2020) 7:586166. doi: 10.3389/fnut.2020.586166
Keywords: Alzheimer's disease, branched-chain amino acids, valine, leucine, isoleucine, Mendelian randomized study
Citation: Qian X-h, Liu X-l, Zhang B, Lin Y, Xu J-h, Ding G-y and Tang H-d (2023) Investigating the causal association between branched-chain amino acids and Alzheimer's disease: A bidirectional Mendelian randomized study. Front. Nutr. 10:1103303. doi: 10.3389/fnut.2023.1103303
Received: 20 November 2022; Accepted: 06 March 2023;
Published: 31 March 2023.
Edited by:
Lais Bhering Martins, University of Texas Health Science Center at Houston, United StatesReviewed by:
Wen Liu, Capital Medical University, ChinaBaruh Polis, Yale University, United States
Andrew C. Shin, Texas Tech University, United States
Copyright © 2023 Qian, Liu, Zhang, Lin, Xu, Ding and Tang. This is an open-access article distributed under the terms of the Creative Commons Attribution License (CC BY). The use, distribution or reproduction in other forums is permitted, provided the original author(s) and the copyright owner(s) are credited and that the original publication in this journal is cited, in accordance with accepted academic practice. No use, distribution or reproduction is permitted which does not comply with these terms.
*Correspondence: Gang-yu Ding, jonathandin@126.com; Hui-dong Tang, thd10495@rjh.com.cn
†These authors have contributed equally to this work