- 1Key Laboratory of Aquatic Product Processing, Ministry of Agriculture and Rural Affairs, National R&D Center for Aquatic Product Processing, South China Sea Fisheries Research Institute, Chinese Academy of Fishery Sciences, Guangzhou, China
- 2Food Safety Laboratory, Ocean University of China, Qingdao, China
- 3Co-Innovation Center of Jiangsu Marine Bio-industry Technology, Jiangsu Ocean University, Lianyungang, China
- 4Collaborative Innovation Center of Seafood Deep Processing, Dalian Polytechnic University, Dalian, China
The active substances derived from plants have received increasing attention owing to their wide range of pharmacological applications, including anti-tumor, anti-allergic, anti-viral, and anti-oxidative activities. The allergy epidemic is a growing global public health problem that threatens human health and safety. Polyphenols from plants have significant anti-allergic effects and are an important source of anti-allergic drug research and development. Here, we describe recent advances in the anti-allergic efficacy of plant polyphenols, including their comprehensive effects on cellular or animal models. The current issues and directions for future development in this field are discussed to provide a theoretical basis for the development and utilization of these active substances as anti-allergic products.
1. Introduction
Allergies are pathological immune responses caused by ingestion of, inhalation of, and contact with harmful foreign substances and are aimed at removing the foreign substances (1, 2). Despite their significance to the body’s defense system, their excessiveness (hypersensitivity reaction) is potentially harmful. Hypersensitivity is divided into type I (immediate hypersensitivity), type II (cytotoxic type of allergy), type III (immune complex type), and type IV (delayed type) reactions (3). An allergy is a type of immediate hypersensitivity reaction (abnormal and excessive immune response), characterized by rapid occurrence and rapid regression of the reaction, leading to systemic or local dysfunction. Common allergy symptoms include hives, asthma, rhinitis, and diarrhea. Substances that cause allergic reactions are called allergens. Allergens are mainly macromolecular substances (4). Common allergens originate from foods (e.g., nuts, milk, eggs, soybeans, wheat, peanuts, fish, and crustaceans), inhalants (e.g., pollen, dust, and mites), and microorganisms (e.g., mold and bacteria). Certain physical factors, such as light, can also cause allergies (5). Upon entry into the body, macromolecular substances directly act as antigens, whereas small-molecular substances act as haptens that combine with substances in the body to form new antigens. Food allergens trigger the most allergic diseases, and 90% of allergic diseases are food allergies (6). Upon initial exposure to allergens, the body triggers a series of reaction processes but does not exhibit allergic symptoms. However, it rapidly develops allergic symptoms upon subsequent exposure (Figure 1A). The use of anti-allergic drugs is currently a common solution for the treatment of allergic diseases, but there are still shortcomings such as adverse reactions and drug resistance. Due to the diversity of plant-derived ingredients and their various effects, they are widely used for food and medicine, and are associated with adequate research and utilization prospects (7). This review summarizes research progress in the field of bioactive polyphenols derived from plants with the aim of providing a reference for the development of anti-allergic functional products.
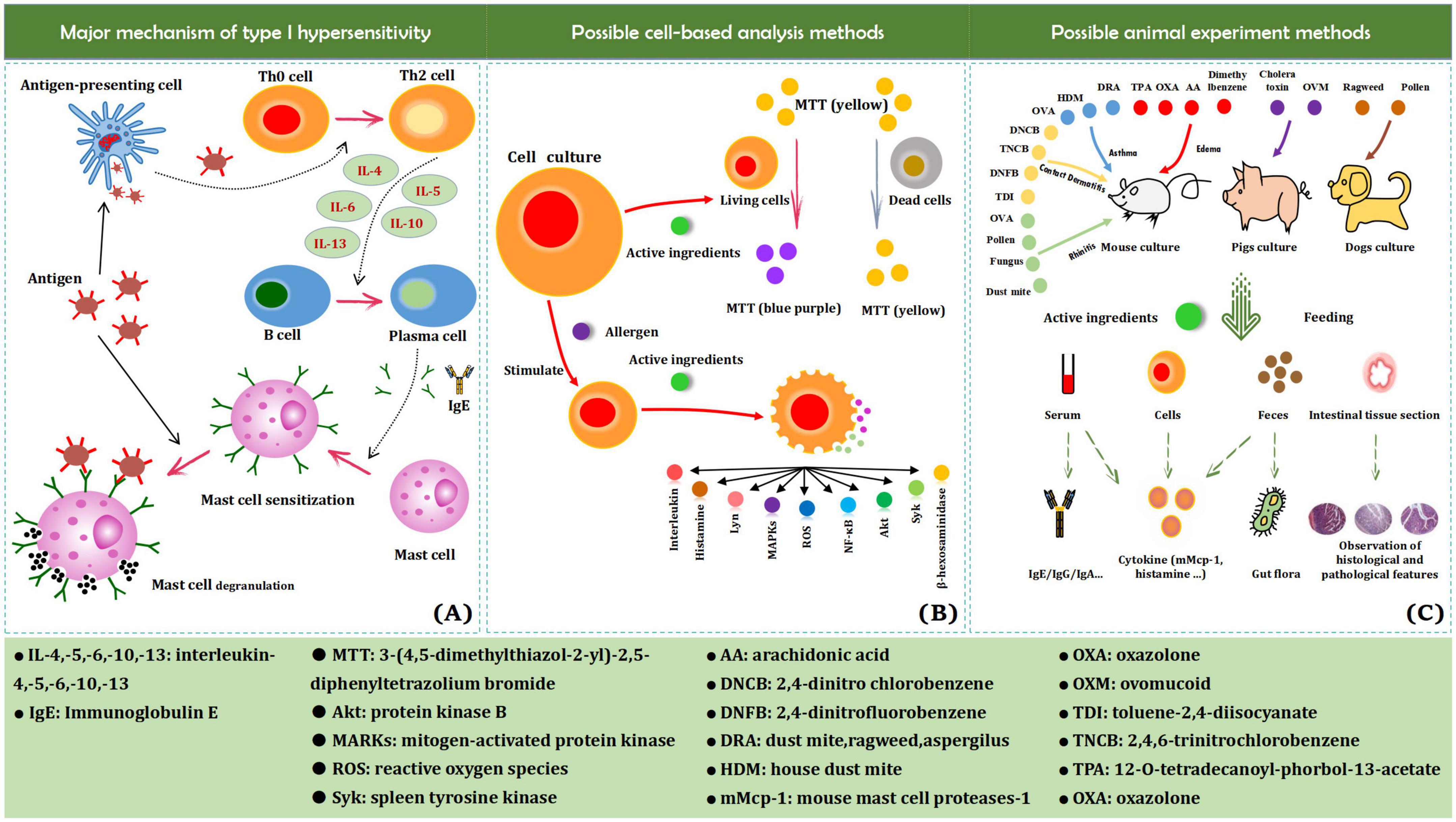
Figure 1. Major mechanism of type I hypersensitivity (A), possible cell-based analysis methods (B), and animal experiment methods (C).
2. Anti-allergic effects of plant polyphenols
Polyphenols, including flavonoids, tannins, phenolic acids, and anthocyanins, are important secondary metabolites that are widely present in plants. Further, they can regulate the activities of most enzymes and cell receptors and have significant anti-allergic effects (8). Plant polyphenols interfere with allergic responses mainly by inhibiting the production of signaling factors and suppressing cytokine production, signal transduction, and gene expression in mast cells, basophils, or T-cells (Table 1). The binding between allergenic proteins and plant polyphenols changes the conformation of the protein, reducing its allergenic potential (9, 10). The activity of polyphenols in inhibiting allergic reactions is related to their structure. Among the many components of epigallocatechin gallate, the component with a pyrogallol structure, a galloyl group on the benzene ring, and a 2,3-cis configuration has the strongest inhibitory activity (11). Functional groups that enhance inhibitory activity often have a synergistic and therefore stronger effect.
Flavonoids are the polyphenols associated with the most outstanding research progress in recent years. They mainly affect the secretion process of immune cells, mitosis, and the interaction between cells and significantly influence the inhibition of allergic reactions (12). Flavonoids inhibit the release of the proteolytic enzyme β-hexosaminidase and histamine from mast cells (13, 14). Certain flavonoids can modulate the expression of genes associated with allergic reactions. Luteolin can promote the expression of IFN-γ in dendritic cells, and quercetin inhibits the transcriptional upregulation of histamine H-1 receptors by inhibiting the protein kinase C-δ/extracellular signal-regulated kinase/poly (ADP-ribose) polymerase-1 signaling pathway mediated transcriptional upregulation of receptors (15, 16). Baicalin, luteolin, isoquercitrin, and other flavonoids can inhibit the release of cell mediators from mast cells, reduce the expression level of Th2 cell molecules, improve the balance between Th1 cells and Th2 cells, and inhibit the release of related enzymes and gene expression levels, thereby exerting anti-allergic effects (17–19).
Flavonoids have a C6-C3-C6 skeleton, two benzene rings, and a heterocyclic ring in the middle (20). Flavonoids with hydroxyl groups on the benzene ring or carbon–carbon double bonds on the heterocycle have more significant anti-allergic effects, and the enhancing effects of hydroxyl groups and carbon–carbon double bonds can be superimposed. Both luteolin and eriodictyol are flavonoids with very similar structures. Luteolin, with a carbon–carbon double bond on the heterocycle, has a significant anti-allergic effect, whereas eriodictyol, without a carbon–carbon double bond on the heterocycle, has almost no anti-allergic effect (21). Notably, the introduction of a hydroxyl group to the heterocycle has no significant effect on the enhancement of anti-allergic efficacy. The glucuronidation reaction is the main metabolic pathway associated with flavonoids (22). However, flavonoids with significant glucuronidation, such as alpinetin, have poor bioavailability in vivo (23). The inhibition of glucuronidation can thus significantly improve the pharmacokinetic profile of flavonoids. In conclusion, flavonoids have significant anti-allergic effects, which are highly correlated with their structure. By modifying the structure of plant-derived flavonoids, their bioavailability and pharmacokinetics in vivo can be improved, and their anti-allergic effects can be enhanced. The development of anti-allergic drugs using flavonoids as raw materials is therefore anticipated. Polyphenols have significant anti-allergic activity, but there are fewer studies on their development for the treatment of allergies, probably because of their low bioavailability in the body. Improving the body’s absorption of polyphenols by modifying their structure has interesting prospects and warrants further study.
Several studies have shown that changing the contents of anti-allergic active substances in food can reduce the incidence of food allergy. An epidemiological survey in Finland showed that the incidence of allergic asthma could be reduced by the relatively high intake of flavonoids (24). Another study showed that cocoa intake of more than 7 g per day can reduce the incidence of food allergy, which might be related to the flavonoids contained in this food (25). In conclusion, modifying the anti-allergic active ingredients in food to reduce the incidence of food allergy is feasible.
3. In vitro and in vivo research methods
3.1. In vitro studies
In vitro studies mainly use enzymes and cells as research tools. Hyaluronidase, an enzyme that inhibits the activity of hyaluronic acid, is strongly associated with allergic reactions (42). Hyaluronic acid is a mucopolysaccharide widely distributed in epithelial tissue, which plays an important role in maintaining the physiological function of the skin or mouth. The activation of hyaluronidase will lead to the decomposition of hyaluronic acid, change the permeability of capillaries and inflammatory cells, and induce allergic reactions (43). Certain drugs for the treatment of allergic reactions have strong inhibitory effects on hyaluronidase activity (44); therefore, hyaluronidase is often used to test the anti-allergic activity of active compounds.
In recent years, the use of cell experiments has been integrated into the field of anti-allergic research. Most cell models are associated with similar experimental methods, including the use of MTT to measure cell viability to prove the safety of the test material, as well as the effect of active substances on cell degranulation, the release of histamine, intracellular reactive oxygen species, and the secretion of cytokines (Figure 1B). Mast cells are the main effector cells in allergic diseases, but the use of suitable sub-cultured mast cell lines for research is lacking (45). The high-affinity IgE receptor (FcεRI) is present on the surface of RBL-2H3 cells, which have numerous characteristics and functions of mast cells. More importantly, RBL-2H3 cells can be passaged more stably than mast cells. Therefore, RBL-2H3 cells have been commercialized as an alternative model of mast cells for the study of allergic diseases (46). The protein kinases Fyn, Lyn, and Syk in RBL-2H3 cells are activated in the initial stage of degranulation signaling; besides, Fyn and Lyn can further activate sphingosine kinase, and Syk mediates the degranulation of RBL-2H3 cells via the production of phosphatidylinositol triphosphate (47). Compound 48/80 is commonly used to induce the activation and degranulation of RBL-2H3 cells (48). The release rate of β-hexosaminidase and the amount of histamine released are markers used to detect the degranulation of RBL-2H3 cells (49). However, RBL-2H3 cells are only suitable for studying IgE-mediated degranulation and are not representative of other irritants (Compound 48/80, etc.) (50). Thus, RBL-2H3 cells might not be suitable for establishing anaphylactoid reaction models.
The RBL-2H3 cell model has been used to study the anti-allergic activity of different polyphenol combinations. One study showed that curcumin or quercetin combined with luteolin could significantly inhibit TNF-α and IL-8 mRNA expression. In addition, the combination of luteolin and quercetin inhibits the β- activity of hexosaminidase (51). Persicaria perfoliata (L.) H. Gross is commonly used as an anti-allergic drug in traditional medicine. The RBL-2H3 cell model was used to show that an aqueous extract of P. perfoliata could inhibit the release of β-hexosaminidase in a dose-dependent manner, without causing cytotoxicity. Treatment with an aqueous extract of P. perfoliata also dose-dependently inhibited the phosphorylation of extracellular regulatory kinase (ERK) and c-Jun N-terminal kinase (JNK) in cells (52).
The P815 cell line is a mast cell model. Compared with RBL-2H3 cells, P815 cells have a shorter degranulation time and a more severe response to the same external stimulus, and the percentage and degranulation ratio of Annexin V-positive cells among P815 cells were found to be substantially higher than those in RBL-2H3 cells. The use of P815 cells has shown that stem cell factors have an important effect on cytokine production by mast cells (significantly increased IL-13 production, marked ERK phosphorylation, and CREB activation) (53). The P815 cell model was further used to show that zinc inhibits mast cell hypersensitivity through the IL-33/ST2 pathway (decreased expression of IL-33, ST2, p38, and p65 proteins) (54).
Basophils are a type of white blood cells that express the high-affinity IgE receptor (Fc&RI) on their surface. After basophils come into contact with allergens, they release histamine, leukotriene C4, IL-4, and IL-13 (55). Further, basophils express various markers such as CD45, CD63, and CD203c on their surface (56). Therefore, they are sometimes used in allergy research. When basophils are stimulated, they become activated, the basophilic granule membrane fuses with the cell membrane, and CD63 is exposed (57). Therefore, the degranulation of activated basophils can be detected via flow cytometry. CD203c expression increases when basophils are activated and thus can be used as an indicator of basophil activation through flow cytometry.
In summary, in vitro anti-allergic studies are primarily conducted using enzymes and cells. Experiments using enzymes are simple, whereas those using cells provide an in-depth explanation of the mechanism by which active compounds exert anti-allergic effects. At present, the commonly used cell models still need improvements with respect to stability between cell passages and the reproducibility of experimental data, in addition to reducing interference with experimental results owing to cell instability.
3.2. In vivo studies
In vivo studies of allergic reactions mainly use animals as experimental subjects. Animal experiments are widely used in life science research and play an important role therein (58). Guinea pigs, BALB/c mice, Wistar rats, Sprague Dawley rats, Brown Norway rats, piglets, and dogs have been used in experimental anti-allergic studies (59–65). Rodents, such as Guinea pigs, rats, and mice, have short reproductive cycles, are less costly, and can be easily bred, with mature commercial models available, making them more suitable as anti-allergic research animal models (66). Mouse models are mainly used to obtain serum data, excreta data, and tissue section data (Figure 1C).
Mice are the most commonly used animal models, and various mouse models have been developed for anti-allergic experiments, including BALB/c, C57BL/6, and A/J mice. The BALB/c mouse is the most commonly used mouse model and is a highly IgE-responsive strain. Mice sensitized with a high dose of tropomyosin exhibit signs of anaphylaxis, including puffiness around the eyes and snout, inactivity, tremor, and convulsions, after the challenge (67). After the intraperitoneal injection of ovalbumin (OVA), BALB/c mice show mild allergic signs, such as piloerection and rapid breathing, increased serum levels of OVA sIgE, sIgG, HIS, and mMCP-1, and an increase in the percentage of Th2 cells that differentiate among splenic lymphocytes, indicating a Th2-type allergic reaction (68). Some scholars have explored the feasibility of using BALB/c mice as anti-allergic research animal models and found that these mice exhibit different levels of sensitization to various allergens and can be used to distinguish sensitization to different food proteins (69).
In conclusion, in vivo experiments on active compounds are mainly conducted on animals. Paradoxically, animals that are similar to humans tend to have long life cycles and high testing costs, and conclusions obtained from animals that are easy to use as experimental subjects are often difficult to reproduce in humans. The direct use of humans as experimental subjects is very dangerous and can lead to unknown consequences. This necessitates the identification of new and more suitable animal models or the improvement of existing animal models to further optimize the efficiency of animal experiments and the accuracy of results. In addition, an increasing number of studies are based on animal models to study the relationship between intestinal microorganisms and food allergy, which is a promising research field.
4. Conclusion
Plant polyphenols have remarkable effects and limited toxic and side effects, but at present, plant medicines are not widely used for allergy treatment. The main problems are as follows. First, research still focuses on plant polyphenols that are known to have anti-allergic activity, such as quercetin and paeonol. Second, most anti-allergic activity studies stay at the level of activity characterization and verification; there are few studies on the molecular targeting mechanism and even fewer on their deep application to drug development. Third, there are few studies on the structure–activity relationship of plant polyphenols and the key functional groups and spatial structures that characterize their efficacy. There are also limited studies on plant polyphenols and even fewer toxicological studies on them, all of which require more in-depth research. Finally, plant polyphenols have inherent cross-reactivity with many reported allergens, which severely affects their application. A possible solution to this is to suppress the effects of cross-allergic allergens by mixing various plant polyphenols. Another possible solution is to separate and purify the components of plant polyphenols, separating proteins from other components, thereby reducing the possibility of cross-reactions with other allergens. Conversely, as almost all drugs have side effects, if the inhibitory effect of plant polyphenols on allergic reactions is weaker than the allergic symptoms caused by allergens produced by the plant itself, then the effect is of no value.
In the background of the current allergic disease epidemic sweeping the world and affecting the health and life of numerous humans, the market demand for anti-allergic drugs will increase. At the same time, the continuous introduction of new foods and the continuous refinement of food processing will continue to generate new allergens, and the demand for allergy-preventing foods and drugs that can broadly inhibit allergic reactions is increasing. Currently marketed anti-allergic drugs, such as loteprednol and jasmonic acid, have single effects and marked toxic and side effects, which limit the application of chemically synthetized anti-allergic drugs. In contrast, traditional Chinese medicines have fewer side effects, a wide range of effects, and better applications. However, their compositions are complex, their mechanisms are difficult to demonstrate, and they are difficult to market as drugs. Therefore, in-depth studies on the structure–activity relationship, mechanisms of action, efficacy, toxicology, and clinical application potential of anti-allergic active substances should be carried out to make active substances more attractive drugs. Future research should focus more on improving the bioavailability of natural plant polyphenols for practical applications that suppress the prevalence of food allergies. The development of natural active compounds as food components or pharmaceutical formulation components could be a viable approach.
Author contributions
TW and ZL: writing—original draft preparation. YZ and YaW: conceptualization. XY: funding acquisition and supervision. YZ, XY, LL, and SC: writing—review and editing. TW, BQ, YuW, and CL: visualization. All authors read and agreed to the published version of the manuscript.
Funding
We are grateful for the financial support from the National Key R&D Program of China (2018YFD0901006), China Agriculture Research System of MOF and MARA (CARS-50), and Central Public-interest Scientific Institution Basal Research Fund, CAFS (2020TD73).
Conflict of interest
The authors declare that the research was conducted in the absence of any commercial or financial relationships that could be construed as a potential conflict of interest.
Publisher’s note
All claims expressed in this article are solely those of the authors and do not necessarily represent those of their affiliated organizations, or those of the publisher, the editors and the reviewers. Any product that may be evaluated in this article, or claim that may be made by its manufacturer, is not guaranteed or endorsed by the publisher.
References
1. Mitsias D, Xepapadaki P, Makris M, Papadopoulos N. Immunotherapy in allergic diseases—improved understanding and innovation for enhanced effectiveness. Curr Opin Immunol. (2020) 66:1–8. doi: 10.1016/j.coi.2020.02.005
2. Wubben R, Efstathiou C, Stevenson N. Chapter One–The interplay between the immune system and viruses. In: G Litwack editor. Vitamins and hormones. hormones, regulators and viruses. Cambridge, MA: Academic Press (2021). p. 1–15.
3. Bølling A, Sripada K, Becher R, Bekö G. Phthalate exposure and allergic diseases: review of epidemiological and experimental evidence. Environ Int. (2020) 139:105706. doi: 10.1016/j.envint.2020.105706
4. Militello M, Hu S, Laughter M, Dunnick C. American contact dermatitis society allergens of the year 2000 to 2020. Dermatol Clin. (2020) 38:309–20.
5. De Gruijl F. The sun’s vitamin against sun allergy. Br J Dermatol. (2011) 165:2–3. doi: 10.1111/j.1365-2133.2011.10421.x
6. Lehtimäki J, Thorsen J, Rasmussen M, Hjelmsø M, Shah S, Mortensen M, et al. Urbanized microbiota in infants, immune constitution; and later risk of atopic diseases. J Allergy Clin Immunol. (2021) 148:234–43. doi: 10.1016/j.jaci.2020.12.621
7. Faccio G. Plant complexity and cosmetic innovation. iScience. (2020) 23:101358. doi: 10.1016/j.isci.2020.101358
8. Bessa C, Francisco T, Dias R, Mateus N, Freitas V, Perez-Gregorio R. Use of polyphenols as modulators of food allergies. From chemistry to biological implications. Front Sustain Food Syst. (2021) 5:623611. doi: 10.3389/fsufs.2021.623611
9. Pan T, Wu Y, He S, Wu Z, Jin R. Food allergenic protein conjugation with plant polyphenols for allergenicity reduction. Curr Opin Food Sci. (2022) 43:36–42. doi: 10.1016/j.cofs.2021.10.002
10. Yang T, Li C, Xue W, Huang L, Wang Z. Natural immunomodulating substances used for alleviating food allergy. Crit Rev Food Sci Nutr. (2021). [Epub ahead of print]. doi: 10.1080/10408398.2021.1975257
11. Tamura S, Yoshihira K, Fujiwara K, Murakami N. New inhibitors for expression of IgE receptor on human mast cell. Bioorg Med Chem Lett. (2010) 20:2299–302. doi: 10.1016/j.bmcl.2010.01.160
12. Kaur R, Kaur K, Arora R, Saini B, Arora S. Natural fused heterocyclic flavonoids: potent candidates as anti-inflammatory and anti-allergic agents in the treatment of asthma. Curr Bioact Compd. (2021) 17:28–40. doi: 10.2174/1573407215666191120125608
13. Jo B, Bong S, Jegal J, Kim S, Yang M. Antiallergic effects of phenolic compounds isolated from Stellera chamaejasme on RBL-2H3 cells. Nat Prod Commun. (2020) 15:1–7. doi: 10.1177/1934578X20942352
14. Santos F, Malafaia C, Simas D, Paulino A, Muzitano M, Simas N, et al. Phenolic compounds from Tocoyena bullata mart (Rubiaceae) with inhibitory activity in mast cells degranulation. Nat Prod Res. (2020) 34:3295–8. doi: 10.1080/14786419.2018.1560281
15. Han L, Sakane I, Mizuno M. Synergistic anti-allergy activity using a combination of Enterococcus faecalis IC-1 and luteolin. Food Biosci. (2021) 41:100924. doi: 10.1016/j.fbio.2021.100924
16. Hattori M, Mizuguchi H, Baba Y, Ono S, Nakano T, Zhang Q, et al. Quercetin inhibits transcriptional up-regulation of histamine H-1 receptor via suppressing protein kinase C-delta/extracellular signal-regulated kinase/poly(ADP-ribose) polymerase-1 signaling pathway in HeLa cells. Int Immunopharmacol. (2013) 15:232–9. doi: 10.1016/j.intimp.2012.12.030
17. Chen S, Chen G, Shu S, Xu Y, Ma X. Metabolomics analysis of baicalin on ovalbumin-sensitized allergic rhinitis rats. R Soc Open Sci. (2019) 6:181081. doi: 10.1098/rsos.181081
18. Liang K, Yu S, Huang W, Yen H. Luteolin attenuates allergic nasal inflammation via inhibition of interleukin-4 in an allergic rhinitis mouse model and peripheral blood from human subjects with allergic rhinitis. Front Pharmacol. (2020) 11:291. doi: 10.3389/fphar.2020.00291
19. Park J. Anti-anaphylactic activity of isoquercitrin (Quercetin-3-O-beta-D-Glucose) in the cardiovascular system of animals. Biomedicines (2020) 8:139. doi: 10.3390/biomedicines8060139
20. Kuppusamy U, Khoo H, Das N. Structure-activity studies of flavonoids as inhibitors of hyaluronidase. Biochem Pharmacol. (1990) 40:397–401. doi: 10.1016/0006-2952(90)90709-T
21. Noshita T, Miura K, Ikeda K, Ouchi H, Matsumoto T, Tai A. Structure-activity relationships of flavanones, flavanone glycosides, and flavones in anti-degranulation activity in rat basophilic leukemia RBL-2H3 cells. J Nat Med Tokyo. (2018) 72:551–6. doi: 10.1007/s11418-017-1169-3
22. Tang L, Singh R, Liu Z, Hu M. Structure and concentration changes affect characterization of UGT isoform-specific metabolism of isoflavones. Mol Pharm. (2009) 6:1466–82. doi: 10.1021/mp8002557
23. Zhao G, Tong Y, Luan F, Zhu W, Zhan C, Qin T, et al. Alpinetin: a review of its pharmacology and pharmacokinetics. Front Pharmacol. (2022) 13:814370. doi: 10.3389/fphar.2022.814370
24. Knekt P, Kumpulainen J, Jarvinen R, Rissanen H, Heliovaara M, Reunanen A, et al. Flavonoid intake and risk of chronic diseases. Am J Clin Nutr. (2002) 76:560–8. doi: 10.1093/ajcn/76.3.560
25. Rodriguez-Lagunas MJ, Vicente F, Pereira P, Castell M, Perez-Cano FJ. Relationship between cocoa intake and healthy status: a pilot study in university students. Molecules. (2019) 24:812. doi: 10.3390/molecules24040812
26. Li J, Lin X, Liu X, Ma Z, Li Y. Baicalin regulates Treg/Th17 cell imbalance by inhibiting autophagy in allergic rhinitis. Mol Immunol. (2020) 125:162–71. doi: 10.1016/j.molimm.2020.07.008
27. Zheng M, Guo X, Pan R, Gao J, Zang B, Jin M. Hydroxysafflor yellow a alleviates ovalbumin-induced asthma in a guinea pig model by attenuateing the expression of inflammatory cytokines and signal transduction. Front Pharmacol. (2019) 10:328. doi: 10.3389/fphar.2019.00328
28. Liu R, Zhao T, Che D, Cao J, Wang J, Lv Y, et al. The anti-anaphylactoid effects of hydroxysafflor yellow a on the suppression of mast cell Ca2+ influx and degranulation. Phytomedicine. (2018) 48:43–50. doi: 10.1016/j.phymed.2018.05.009
29. Dong J, Xu O, Wang J, Shan C, Ren X. Luteolin ameliorates inflammation and Th1/Th2 imbalance via regulating the TLR4/NF-κB pathway in allergic rhinitis rats. Immunopharmacol Immunotoxicol. (2021) 43:319–27. doi: 10.1080/08923973.2021.1905659
30. Wang S, Wuniqiemu T, Tang W, Teng F, Bian Q, Yi L, et al. Luteolin inhibits autophagy in allergic asthma by activating PI3K/Akt/ mTOR signaling and inhibiting Beclin-1-PI3KC3 complex. Int Immunopharmacol. (2021) 94:107460. doi: 10.1016/j.intimp.2021.107460
31. Xing Y, Tan C, Luo Y, Liu W. Effect of quercetin on rhinitis via inflammatory pathway. Pak J Zool. (2021) 53:619–26. doi: 10.17582/journal.pjz/20190925070938
32. Ding Y, Li C, Zhang Y, Ma P, Zhao T, Che D, et al. Quercetin as a Lyn kinase inhibitor inhibits IgE-mediated allergic conjunctivitis. Food Chem Toxicol. (2020) 135:110924. doi: 10.1016/j.fct.2019.110924
33. Feng H, Xiong X, Xu Q, Zhang Z, Feng J, Wu Y. Study on the immunomodulatory effect of quercetin nanoparticles loaded with chitosan on a mouse model of ovalbumin-induced food allergy. Nanosci Nanotechnol Lett. (2020) 12:915–20. doi: 10.1166/nnl.2020.3197
34. Hwang K-A, Hwang Y-J, Song J. Anti-allergic effect of aster yomena on ovalbumin-sensitized mouse and RHL-2H3 Cells via Th1/Th2 cytokine balance. J Funct Food. (2018) 44:1–8. doi: 10.1016/j.jff.2018.02.026
35. Cao J, Wang Y, Hu S, Ding Y, Jia Q, Zhu J, et al. Kaempferol ameliorates secretagogue-induced pseudo-allergic reactions via inhibiting intracellular calcium fluctuation. J Pharm Pharmacol. (2020) 72:1221–31. doi: 10.1111/jphp.13312
36. Molitorisova M, Sutovska M, Kazimierova I, Barborikova J, Joskova M, Novakova E, et al. The anti-asthmatic potential of flavonol kaempferol in an experimental model of allergic airway inflammation. Eur J Pharmacol. (2021) 891:173698. doi: 10.1016/j.ejphar.2020.173698
37. Chiu Y, Wu Y, Hung J, Chen M. Epigallocatechin gallate/L-ascorbic acid-loaded poly-γ-glutamate microneedles with antioxidant, anti-inflammatory, and immunomodulatory effects for the treatment of atopic dermatitis. Acta Biomater. (2021) 130:223–33. doi: 10.1016/j.actbio.2021.05.032
38. Kim C-Y, Kim J-W, Kim J-H, Jeong J-S, Lim J-O, Ko J-W, et al. Inner shell of the chestnut (Castanea Crenatta) suppresses inflammatory responses in ovalbumin-induced allergic asthma mouse model. Nutrients. (2022) 14:2067. doi: 10.3390/nu14102067
39. Sugiura Y, Katsuzaki H, Imai K, Amano H. The anti-allergic and anti-inflammatory effects of phlorotannins from the edible brown algae, Ecklonia sp. and Eisenia sp. Nat Prod Commun. (2021) 16:1934578X211060924. doi: 10.1177/1934578X211060924
40. Liang Z, Wu L, Deng X, Liang Q, Xu Y, Deng R, et al. The antioxidant rosmarinic acid ameliorates oxidative lung damage in experimental allergic asthma via modulation of NADPH oxidases and antioxidant enzymes. Inflammation. (2020) 43:1902–12. doi: 10.1007/s10753-020-01264-3
41. Shakeri F, Eftekhar N, Roshan N, Rezaee R, Moghimi A, Boskabady M. Rosmarinic acid affects immunological and inflammatory mediator levels and restores lung pathological features in asthmatic rats. Allergol Immunopath. (2019) 47:16–23. doi: 10.1016/j.aller.2018.04.004
42. Lei W, Liu C, Pan L, Peng C, Wang J, Zhou H. Screening of probiotic Lactobacilli with potential anti-allergic activity based on hyaluronidase inhibition and degranulation of RBL-2H3 cells in vitro. LWT. (2021) 140:110707. doi: 10.1016/j.lwt.2020.110707
43. Vasvani S, Kulkarni P, Rawtani D. Hyaluronic acid: a review on its biology, aspects of drug delivery, route of administrations and a special emphasis on its approved marketed products and recent clinical studies. Int J Biol Macromol. (2020) 151:1012–29. doi: 10.1016/j.ijbiomac.2019.11.066
44. Kakegawa H, Matsumoto H, Satoh T. Activation of hyaluronidase by metallic salts and compound 48/80, and inhibitory effect of anti-allergic agents on hyaluronidase. Chem Pharm Bull. (1985) 33:642–6. doi: 10.1248/cpb.33.642
45. Kolkhir P, Elieh-Ali-Komi D, Metz M, Siebenhaar F, Maurer M. Understanding human mast cells: lesson from therapies for allergic and non-allergic diseases. Nat Rev Immunol. (2021) 22:294–308. doi: 10.1038/s41577-021-00622-y
46. Falcone F, Wan D, Barwary N, Sagi-Eisenberg R. RBL cells as models for in vitro studies of mast cells and basophils. Immunol Rev. (2018) 282:47–57. doi: 10.1111/imr.12628
47. Amir-Moazami O, Alexia C, Charles N, Launay P, Monteiro R, Benhamou M. Phospholipid scramblase 1 modulates a selected set of IgE receptor-mediated mast cell responses through LAT-dependent pathway. J Biol Chem. (2008) 283:25514–23. doi: 10.1074/jbc.M705320200
48. Xu Y, Yang F, Xie J, Li W, Liu B, Chen J, et al. Human umbilical cord mesenchymal stem cell therapy mitigates interstitial cystitis by inhibiting mast cells. Med Sci Monitor. (2021) 27:e930001. doi: 10.12659/MSM.930001
49. Do H, Oh T. Effect of Ulmus macrocarpa Hance Ethanol extract (Ulmus) on improvement of allergic responses in RBL-2H3 mast Cells. J Herb Form Sci. (2021) 29:191–203. doi: 10.14374/HFS.2021.29.4.191
50. Xiaoyu Z, Ruijuan Q, Ximeng L, Yixin H, Qiaoling F, Runlan C, et al. RBL-2H3 cells not suitable for pseudo-allergic reaction model. Chin Pharmacol Bull. (2020) 36:438–43.
51. Mizuno M, Yamashita S, Hashimoto T. Enhancement of anti-inflammatory and anti-allergic activities with combination of luteolin and quercetin in in vitro co-culture system. Food Sci Technol Res. (2017) 23:811–8. doi: 10.3136/fstr.23.811
52. Seo Y, Park C. Inhibitory effect of Persicaria perfoliata (L.) H. gross on IgE mediated allergic responses in RBL-2H3 cells. J Korean Soc Integr Med. (2020) 8:163–9. doi: 10.1155/2016/8742562
53. Wang Y, Ma H, Tao X, Luo Y, Wang H, He J, et al. SCF promotes the production of IL-13 via the MEK-ERK-CREB signaling pathway in mast cells. Exp Ther Med. (2019) 18:2491–6. doi: 10.3892/etm.2019.7866
54. Wen W, Yang F, Shen X, Feng N, Ha H, Ma R. Regulatory role of zinc in allergic rhinitis through the IL-33/ST2 pathway. J Healthc Eng. (2022) 2022:3718317. doi: 10.1155/2022/3718317
55. Kleine-Tebbe J, Erdmann S, Knol E, MacGlashan D, Poulsen L, Gibbs B. Diagnostic tests based on human basophils: potentials, pitfalls and perspectives. Int Arch Allergy Immunol. (2006) 141:79–90. doi: 10.1159/000094495
56. Vella A, Bjorklund G, Chirumbolo S. Basophil activation test in amiodarone hypersensitivity and Non-IgE-Mediated allergy. J Invest Allergol Clin Immunol. (2019) 29:401–2. doi: 10.18176/jiaci.0416
57. Ebo D, Elst J, van Houdt M, Pintelon I, Timmermans J, Horiuchi T, et al. Flow cytometric basophil activation tests: staining of exteriorized basophil granule matrix by fluorescent avidin versus appearance of CD63. Cytometry B Clin Cytom. (2020) 98:483–90. doi: 10.1002/cyto.b.21868
58. Kanzler S, Krabbe J, Forkmann T, Tolba R, Steitz J. Animal experiments in biomedical research: knowledge, self-evaluation and attitudes of biology and medical students. Lab Anim. (2022) 56:455–65. doi: 10.1177/00236772221080833
59. van Esch B, van Bilsen J, Gros-van Hest M, Kleinjans L, Belzer C, Jeurink P, et al. A multi-center assessment to compare residual allergenicity of partial hydrolyzed whey proteins in a murine model for cow’s milk allergy - Comparison to the single parameter guinea pig model. Toxicol Lett. (2020) 333:312–21. doi: 10.1016/j.toxlet.2020.05.020
60. Muhandes L, Chapsa M, Pippel M, Behrendt R, Ge Y, Dahl A, et al. Low threshold for cutaneous allergen sensitization but no spontaneous dermatitis or atopy in FLG-Deficient mice. J Invest Dermatol. (2021) 141:2611. doi: 10.1016/j.jid.2021.02.763
61. Aglas L, Bethanis A, Chrusciel P, Stolz F, Gruen M, Jaakkola U, et al. In vivo induction of functional inhibitory igG antibodies by a hypoallergenic bet v 1 variant. Front Immunol. (2020) 11:2118. doi: 10.3389/fimmu.2020.02118
62. Yigit S, Hallaj N, Sugarman J, Chong L, Roman S, Abu-Taleb L, et al. Toxicological assessment and food allergy of silk fibroin derived from Bombyx mori cocoons. Food Chem Toxicol. (2021) 151:112117. doi: 10.1016/j.fct.2021.112117
63. Ballegaard A, Madsen C, Bogh K. An animal model for wheat allergy skin sensitisation: a comparative study in naive versus tolerant brown Norway rats. Int Arch Allergy Immunol. (2019) 178:106–18. doi: 10.1159/000493802
64. Radcliffe J, Brito L, Reddivari L, Schmidt M, Herman E, Schinckel AP. A swine model of soy protein-induced food allergenicity: implications in human and swine nutrition. Anim Front. (2019) 9:52–9. doi: 10.1093/af/vfz025
65. Imanishi I, Uchiyama J, Mizukami K, Kamiie J, Kurata K, Iyori K, et al. IgE reactivity to fish allergens from Pacific cod (Gadus macrocephalus) in atopic dogs. BMC Vet Res. (2020) 16:341. doi: 10.1186/s12917-020-02559-1
66. Benede S, Berin M. Applications of mouse models to the study of food allergy. Methods Cell Biol. (2021) 2223:1–17. doi: 10.1007/978-1-0716-1001-5_1
67. Laly S, Sankar T, Panda S. In vivo characterization of histological and immunological response of allergic protein of Metapenaeus dobsonii using an adjuvant free BALB/c mice model. Immunol Lett. (2020) 217:133–9. doi: 10.1016/j.imlet.2019.12.001
68. Xu J, Ye Y, Ji J, Sun J, Wang J, Sun X. Untargeted metabolomic profiling reveals changes in gut microbiota and mechanisms of its regulation of allergy in OVA-Sensitive BALB/c mice. J Agric Food Chem. (2022) 70:3344–56. doi: 10.1021/acs.jafc.1c07482
Keywords: anti-allergic, allergen, plant polyphenols, active substances, mechanism, immune activity
Citation: Wu T, Li Z, Wu Y, Yang X, Li L, Chen S, Qi B, Wang Y, Li C and Zhao Y (2023) Exploring plant polyphenols as anti-allergic functional products to manage the growing incidence of food allergy. Front. Nutr. 10:1102225. doi: 10.3389/fnut.2023.1102225
Received: 18 November 2022; Accepted: 03 April 2023;
Published: 09 June 2023.
Edited by:
Linglin Fu, Zhejiang Gongshang University, ChinaReviewed by:
Michele Maffia, University of Salento, ItalyCopyright © 2023 Wu, Li, Wu, Yang, Li, Chen, Qi, Wang, Li and Zhao. This is an open-access article distributed under the terms of the Creative Commons Attribution License (CC BY). The use, distribution or reproduction in other forums is permitted, provided the original author(s) and the copyright owner(s) are credited and that the original publication in this journal is cited, in accordance with accepted academic practice. No use, distribution or reproduction is permitted which does not comply with these terms.
*Correspondence: Yongqiang Zhao, zhaoyq@scsfri.ac.cn