- Department of Life and Consumer Sciences, College of Agriculture and Environmental Sciences, University of South Africa, Florida, South Africa
Obesity and type 2 diabetes (T2D) are chronic conditions with detrimental impacts on the overall health of individuals. Presently, the use of pharmacological agents in obesity and T2D offers limited benefits and pose side effects. This warrant studies on remedies that are less toxic and inexpensive while effective in ameliorating secondary complications in obesity and T2D. Plant-based remedies have been explored increasingly due to their remarkable properties and safety profile. We searched for pre-clinical evidence published from inception until 2023 on PubMed, Scopus, Google, and Semantic scholar on Corchorus olitorius (C. olitorius) in both obesity and T2D. Our focus was to understand the beneficial impact of this plant-based remedy on basic glycemic, lipid, inflammatory, and biomarkers of oxidative stress. The evidence gathered in this review suggests that C. olitorius treatment may significantly reduce blood glucose, body weight, total cholesterol, triglycerides, and low-density lipoprotein (LDL) in concomitant with increasing high-density lipoprotein-cholesterol (HDL-c) in rodent models of obesity and T2D. Interestingly, this effect was consistent with the reduction of malonaldehyde, superoxide dismutase and catalases, tumor necrosis factor-alpha, interleukins, and leptin. Some of the mechanisms by which C. olitorius reduces blood glucose levels is through stimulation of insulin secretion, increasing β-cell proliferation, thus promoting insulin sensitivity; the process which is mediated by ascorbic acid present in this plant. C. olitorius anti-hyperlipidemia is attributable to the content of ferulic acid found in this plant, which inhibits 3-Hydroxy-3-methyl glutaryl coenzyme A (HMG-CoA) reductase inhibitors and thus results in reduced synthesis of cholesterol and increased hepatic LDL-c receptor expression, respectively. The present review provides extensive knowledge and further highlights the potential benefits of C. olitorius on basic metabolic parameters, lipid profile, inflammation, and oxidative stress in rodent models of obesity and T2D.
1. Introduction
Diabetes mellitus (DM), a chronic metabolic disorder, is characterized by an increased level of glucose in the blood. In an International Diabetes Federation (IDF) report of 2021, 537 million people were living with DM globally (1). Hyperglycemia observed in DM induces oxidative stress (2, 3) through stimulation of mitochondrial enzymes, resulting in the accumulation of reactive oxygen species (ROS) (4), which impair organ function (5). Furthermore, DM is also associated with high levels of triglycerides (TG), homocysteinemia, low-density lipoprotein-cholesterol (LDL-c), and reduced levels of high-density lipoprotein-cholesterol (HDL-c) (6, 7), which predispose type 2 diabetes (T2D) to the development of cardiovascular diseases (CVD) (6). In animal models of obesity or diabetes induced by a high-fat diet (HFD), a substantial increase in oxidative stress is present from the early stages of the disease (8, 9). This is accompanied by inflammation, impaired insulin secretion, action, and CVD development. In obesity, increased leptin and adipocytes are associated with an imbalance between pro and anti-inflammatory cytokines. Concomitant to that, resulting in inflammation, insulin resistance, and hyperglycemia (10) (Figure 1). Moreover, there is an impaired secretion of adipokines in obese states, and this increases the risk of CVD (11). There is an association between hyperglycemia, hyperglycemic-induced oxidative stress, inflammation, and the development and progression of T2D. Various reports have shown that chronic low-grade inflammation is associated with the risk of developing T2D and that sub-clinical inflammation contributes to insulin resistance and is linked to the characteristics of metabolic syndrome which include hyperglycemia. Oxidative stress induces the generation of inflammatory cytokines, and inflammation and further enhances the production of ROS (12, 13).
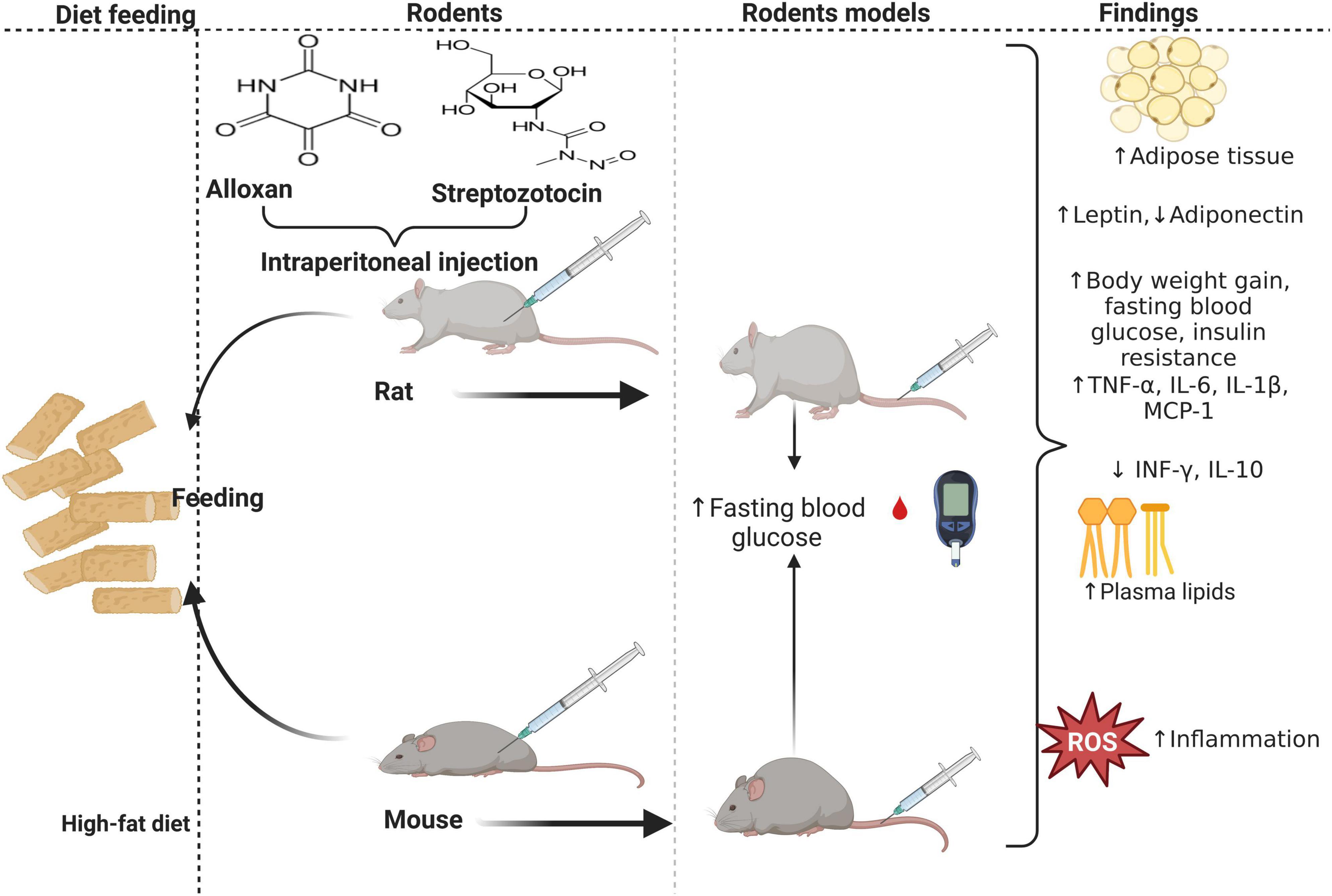
Figure 1. The effect of a high-fat diet (HFD) on inflammation and insulin resistance during obesity. HFD-fed mice develop while rats fed HFD concomitant to intraperitoneal injection of alloxan monohydrate or streptozotocin (STZ) develop diabetes. Rodents with diet-induced obesity or diabetes have enlarged adipocytes, elevating pro-inflammatory cytokines, adipokines, and lipid profiles, concomitant to decreased anti-inflammatory cytokines and adipokines.
Notably, when exploring the efficacy of anti-diabetic drugs or therapies, paying attention to their antioxidant properties and effectiveness in improving the impaired lipid profile and glycemic control is crucial. The widely used T2D and dyslipidemic drugs such as Glucophage (metformin) (14) and statins (15) play a significant role in controlling these conditions; however, they are less tolerated in other patients due to their toxicity and severe adverse effects experienced by other patients (16, 17). Moreover, despite their availability, the rate of CVD complications associated with T2D is alarming globally, suggesting their limitations in cardiometabolic diseases (1, 18, 19). Hence, this warrants more investigation into alternative therapy and naturally occurring products that can regulate hyperglycemia and dyslipidemia with fewer side effects (20–23). Antioxidants are considered effective treatment due to their ability to neutralize free oxygen radicals (24, 25). Genistein, a polyphenolic isoflavone when used as monotherapy or in combination with metformin exhibits anti-inflammatory effects as demonstrated by decreased serum interleukin-6 (IL-6), tumor necrosis factor-α (TNF-α) and C-reactive protein (CRP) (26).
Corchorus olitorius L (jute mallow leaves) has been investigated extensively due to its antioxidant properties (27) in pre-clinical studies as an alternative treatment for T2D (28, 29). Previous evidence suggests that C. olitorius may exhibit protective effects against T2D, obesity, inflammation, and oxidative stress partly due to its chemical composition, such as vitamins A and ascorbic acid (AA) (30, 31), iron, calcium, and fibers (32, 33). Therefore, the C. olitorius antioxidant and anti-inflammatory properties may be a relevant target in treating different CVD and metabolic diseases. A study by Olusanya et al. (34) confirmed the anti-diabetic properties of C. olitorius in a rodent model of T2D. Interestingly, other studies have also shown a beneficial effect of C. olitorius on glucose and lipid level profiles in the same model animal of T2D (35, 36). Most recently, Lee and team (37, 38) demonstrated the anti-dyslipidemic properties of C. olitorius extract and reduced body weight in rodent models of obesity. However, while such benefits are widely reported, Aikawa (39) reported conflicting findings as C. olitorius aqueous solution showed no effect on FBG, insulin, and lipid profile in obese rodents. These contradicting findings show the limitation of C. olitorius as an agent to ameliorate hyperglycemia and dyslipidemia; therefore, this warrants more extensive research to understand the beneficial impact of this plant in obesity and T2D. Interestingly, C. olitorius extract given in a short period has proven safe and well-tolerated without side effects among T2D rodents (34). Although no clinical studies have been conducted to investigate the beneficial impacts of these plants on obesity and T2D, the present review aimed to review extensively and highlight the effects of C. olitorius in rodent models of obesity and T2D, focusing on basic metabolic parameters, glycemic control, lipid profile, inflammation, and oxidative stress.
2. Literature search, inclusion, exclusion criteria, and selection process
A comprehensive search for pre-clinical evidence published from inception to 2023 was done, and this was updated on the 11th March 2023 using PubMed, Scopus, Semantic, and Google Scholar using medical subheading (MesH) terms and text words such as “Jute leaves,” “Corchorus olitorius,” “molokhia,” “obesity,” and “diabetes.” There was no language restriction applied in the search in all databases. One hundred and thirteen records were identified from the databases. Pre-clinical studies were considered relevant if they evaluated the effect of C. olitorius on obesity or diabetes. Different doses and duration of intervention of C. olitorius in obese and diabetes rodent models were included. However, studies not on rodent models of obesity or diabetes were excluded. Before the screening process, 26 records were identified as duplicates by the Mendeley Reference Manager and were excluded. Following the initial screening of the title, abstract, and keyword, 30 records were excluded as the titles and abstract were irrelevant to the review. Out of the 57 records retrieved, eight were not on obese or diabetes rodent models, one was in human subjects, 13 were in phytochemical studies, seven were in vitro evidence, and 12 were reviews. Therefore, only 16 studies were found relevant; an additional four were retrieved by manually screening relevant studies. Therefore 20 relevant pre-clinical studies exploring the potential of C. olitorius extract as an anti-diabetic, anti-dyslipidemic, and anti-inflammatory agent in the rodent models of obesity and T2D were included in the review (Supplementary Figure 1 and Supplementary Tables 1A–F).
3. General overview of the included pre-clinical studies
From the evidence gathered, the studies were conducted in different countries; for instance, nine were conducted in Nigeria, 2 in India, Japan, and Korea, and one from Bangladesh, Egypt, Iraq, Northern Cyprus, and Côte d’Ivoire. The studies were conducted in different rodent strains, including Wistar rats, Albino rats, Sprague–Dawley rats, mice of C57BL/6N or J strain, Long Evans rats, and Otsuka Long-Evans Tokushima Fatty (OLETF) rats. Researchers induced these metabolic disorders through different methods, such as HFD and intraperitoneal injection of either streptozotocin (STZ) or alloxan monohydrate at different doses (Tables 1, 2). The special focus was centered on understanding how plants affect fasting blood glucose (FBG), body weight, and lipid metabolism and the mechanisms involved in inflammation and oxidative stress. T2D can lead to CVD (40); hence various rodent models have been used to understand the pathways and mechanisms implicated in obesity, T2D, and CVD. Most importantly, these experimental models are used to explore the properties of several pharmacological compounds against secondary complications associated with obesity and T2D (41).
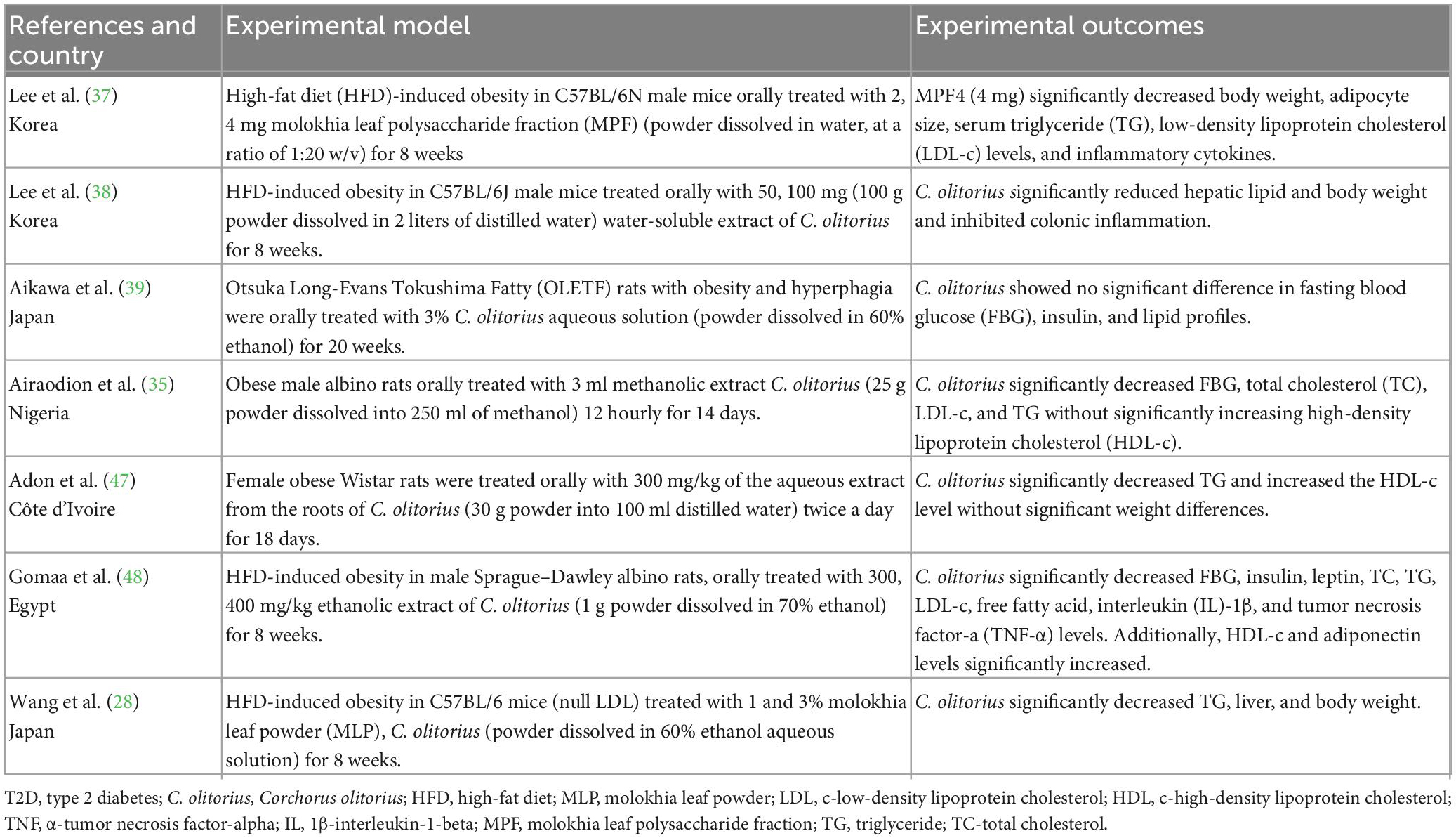
Table 1. A summary of studies evaluating the impact of Corchorus olitorius in a rodent model of obesity.
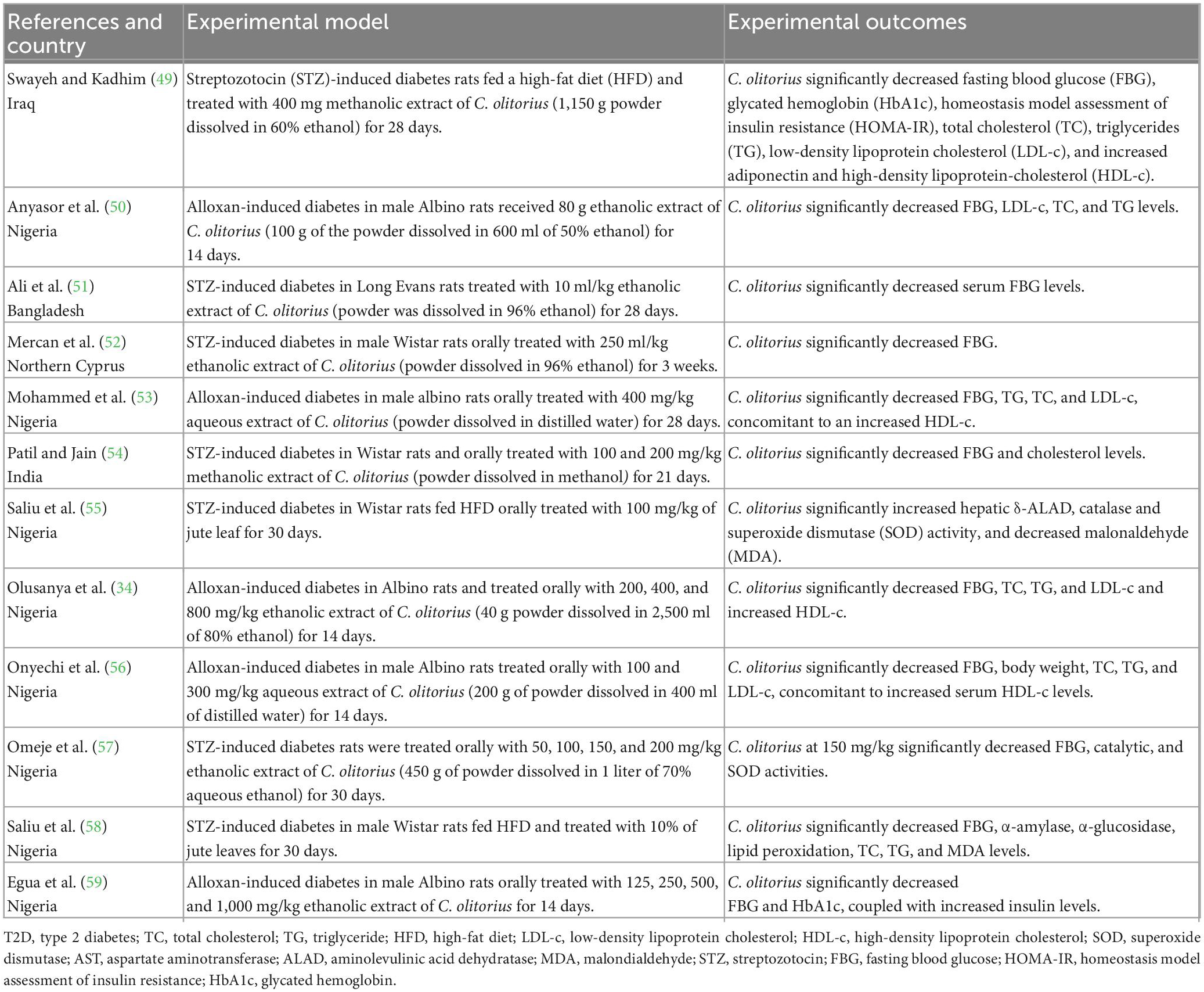
Table 2. A summary of studies evaluating the impact of Corchorus olitorius in rodent models of diabetes.
4. An overview of C. olitorius, components, bioavailability, and functional properties
Corchorus olitorius is a naturally occurring plant used as a vegetable in different African and Asian communities and belongs to the family of Malvaceae. For example, at least 97.7% of individuals in Uganda use C. olitorius for consumption (42). However, it is cultivated in other parts, including Australia, South America, and Europe, to be used in food products as additives and industrial applications. The nutritious quality and value of C. olitorius are attributable to its high level of vitamins, minerals, and phenolics (43). All components of the C. olitorius plant are useful; for example, dried leaves are used as herbal tea, green and fresh leaves are used as vegetables, and seeds are used as a flavoring agent.
Bioavailability refers to the amount of substance absorbed and reaching the circulation for body use (44). However, knowledge of the bioavailability of C. olitorius and its derivatives is limited. Considering the abundance of active compounds it contains, it is likely that its absorption mechanism would not differ from that of the active chemical compound it contains. This plant is rich in carbohydrates, fats, protein, iron, calcium, potassium, sodium, phosphorus, beta-carotene, thiamine, riboflavin, niacin, AA, triterpenes, sterols, fatty acid, phenolics, ionones, oxidase, chlorogenic acid, glycosides, saponins, tannins, and flavones (45). Its high content of various compounds (Figure 2) makes it a unique leafy vegetable with anti-diabetic, cardioprotective, and anti-inflammatory (46).
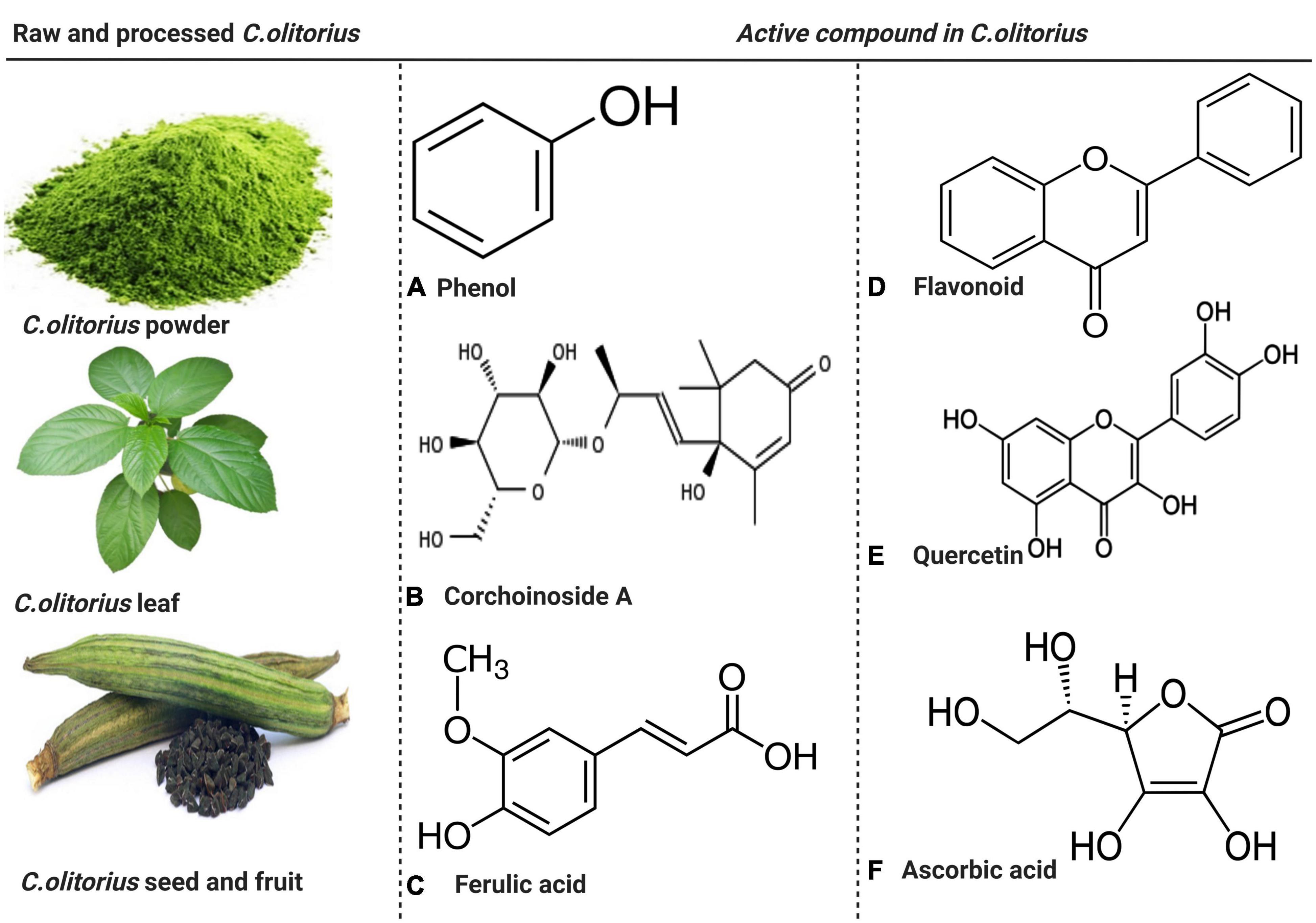
Figure 2. The raw and processed parts of Corchorus olitorius and active compound present in C. olitorius. Both raw and processed Corchorus olitorius contains active compounds such as phenol, corchoinoside A, ferulic acid, flavonoids, quercetin, and ascorbic acid.
5. Effect of C. olitorius on the body weight and glucose metabolism in rodent models of T2D
Diabetes is characterized by hyperglycemia which is often coupled with body weight gain. This is observed in humans and the animal model impaired glucose tolerance (3, 60, 61). It is public knowledge that correct regulation of blood glucose levels and effective control of other primary metabolic indices such as body weight and insulin levels are crucial when controlling and managing T2D. Experimental evidence supports this antioxidant, C. olitorius, in regulating blood glucose levels (Table 2). For example, Anyasor et al. (50) demonstrated that using 80 g ethanolic extract of C. olitorius leaf for 13 days in T2D rats could improve glucose metabolism, as revealed by a significant decrease in plasma glucose in the treated group (99.0 mg/dL) compared to 313.5 mg/dL, p < 0.05 in the control group. Similarly, Mercan et al. (52) reported that the administration of 96% ethanolic extract of C. olitorius (250 mg/kg) for 3 weeks in STZ-induced T2D Wistar albino rats could significantly decrease FBG (297.83 mg/dL) when compared to untreated STZ rats (419.00 mg/dL, p < 0.05). The same study revealed a 0.44-fold decrease in FBG when baseline and post-treatment results were compared. Additionally, in Albino rats, treatment with 3 ml of methanolic extract of C. olitorius decreases FBG (35). Interestingly, FBG was significantly reduced when methanolic extract of C. olitorius was administered at 100 mg/kg (118.00 mg/dL), p < 0.005, or 200 mg/kg (120.00 mg/dL), p < 0.05 compared to control group 397.00 mg/dL (54). The effect was more predominant when a lower dose (100 mg/kg) of the methanolic extract was administered. Similar trends were observed in T2D Albino rats, as FBG was significantly reduced to 9.43 mg/dL at 200 mg/kg, 7.88 mg/dL at 400 mg/kg, and 6.05 mg/dL at 800 mg/kg of C. olitorius extract compared to the diabetic group without treatment (20.41 mg/kg), p < 0.05 (34). Similarly, Mohammed et al. (53) revealed a significant effect of 400 mg/kg of C. olitorius extract on reducing FBG (5.20 mg/dL) compared to diabetic control (10.30 mg/dL), p < 0.05. Another study that induced T2D through STZ in Wistar rats and administered 10% of C. olitorius also revealed a significant reduction in FBG levels (p < 0.05) (58). Consistently, Omeje et al. (57) also revealed that oral administration of ethanol extract of C. olitorius in rats at low or high doses (50, 100, 150, and 200 mg/kg) for 30 days still decreases FBG compared to the untreated group (138.1 mg/dL). However, a pronounced decrease was observed using 200 mg/dL of ethanol extract (96.5 mg/dL), which was deemed a high dose according to the study. Of interest was one study that demonstrated a significant decrease in various parameters associated with glycemic control. This study showed a reduction in FBG (271.50 mg/dL, p < 0.001), glycated hemoglobin (HbA1c) (4.23%, p < 0.001) when 400 mg/kg methanolic extract of C. olitorius compared to untreated T2D rats whose FBG and HbA1c were 408.90 mg/dL and 4.98%, respectively (49). A similar trend was observed by Egua et al. (59) as they demonstrated a significant decrease in FBG and HbA1c concomitant to an increased insulin level in Alloxan-induced T2D. This study used different doses, including 100, 250, 500, and 1,000 mg/kg, and all extracts showed a significant decrease (p < 0.05) in FBG. However, at 100 mg/dL, there was a pronounced decrease of 157.2 mg/dL compared to the control 183.6 mg/dL, p < 0.05.
According to the results by Onyechi et al. (56), C. olitorius administration in T2D Wistar significantly reduced FBG post-treatment (p < 0.05), and this was more predominant when 300 mg/kg instead of 100 mg/kg was administered. Moreover, the same study revealed a decrease in body weight in 100 and 300 mg/kg of C. olitorius extract 143.12 and 155.98 g, respectively, compared to the control group 245.65 g (Figure 3).
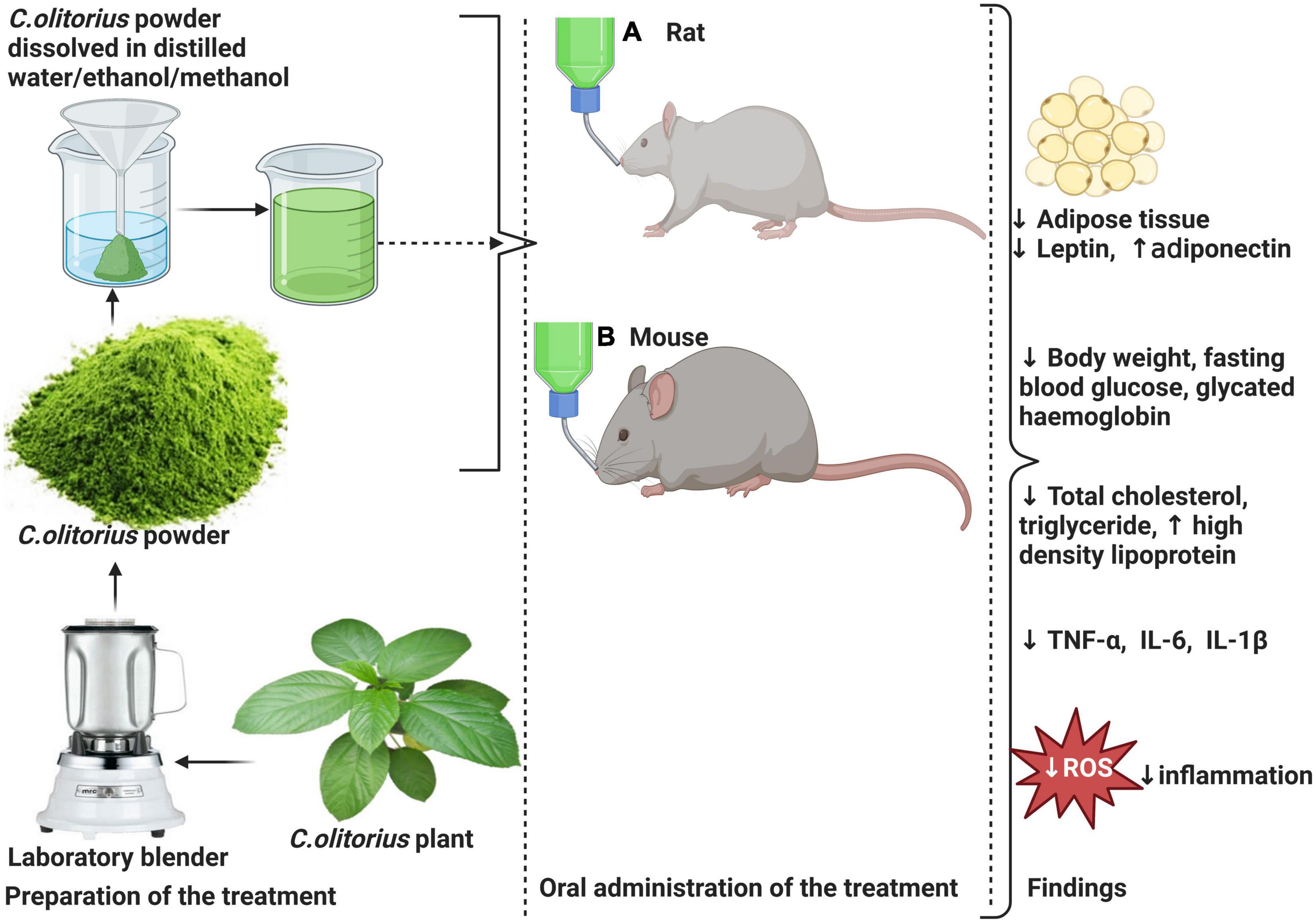
Figure 3. Effects of Corchorus olitorius in a rodent model of diabetes induced by HFD and intraperitoneal injection of streptozotocin (STZ) or monohydrate alloxan in rodents. This is followed by a moderate decrease in the size of adipose tissue and a subsequent decrease in leptin concomitant to increased adiponectin levels. C. olitorius reduce inflammation through the reduction of pro-inflammatory markers such as tumor necrosis factor-alpha (TNF-α), interleukin-6 (IL-6), and interleukin-1-beta (IL-1β). The anti-hyperglycemic potential of C. olitorius is demonstrated by a decrease in total cholesterol levels, triglyceride, low-density lipoprotein-cholesterol (LDL-c), and increased high-density lipoprotein (HDL-c). Also, reduced blood glucose and glycated hemoglobin demonstrate its effect on basic glycemic parameters.
The reduced hyperglycemia in these rodent models may be attributed to the phytochemical content of C. olitorius, such as flavonoids (62–65), saponins (66), and thiamine (67), which are proven to have hypoglycemic properties. Moreover, C. olitorius is rich in polyphenols, which have inhibitory effects on α-amylase and glucosidase, enzymes essential for glucose catabolism (30, 55). Slow intake, digestion, and absorption of a starch-containing diet remain important mechanisms for reducing blood glucose. Therefore, inhibition of pancreatic α-amylase and glucosidase reduces the breakdown of carbohydrates into glucose and absorption of glucose in the small intestine, thereby reducing hyperglycemia (Figure 4) (68, 69).
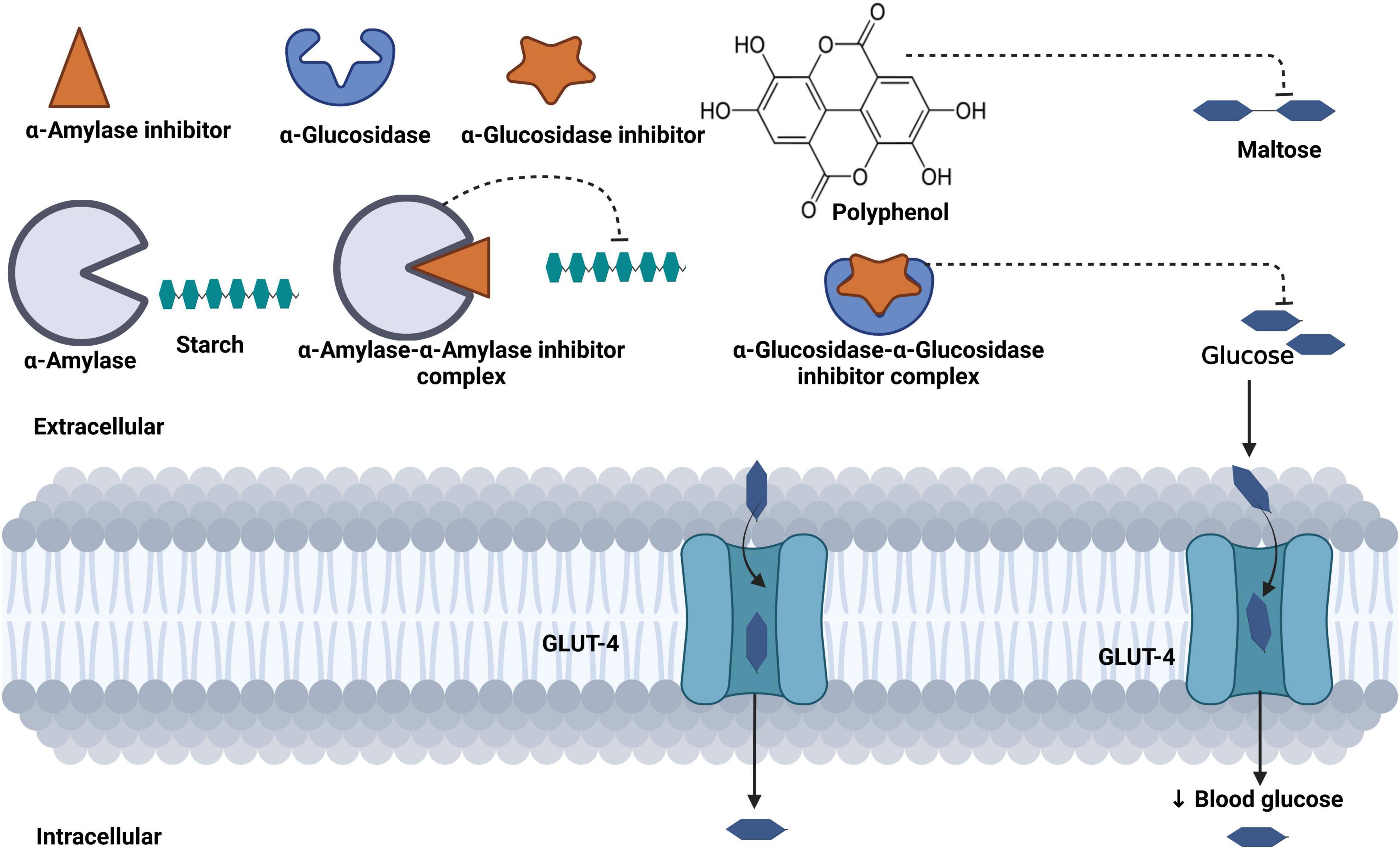
Figure 4. Mechanism of blood glucose regulation mediated by phenol content in Corchorus olitorius. Polyphenols inhibit starch-digesting enzymes (α-amylase, β-glucosidase), reduce the breakdown of starch into maltose, and further reduce the transportation of glucose in the bloodstream.
6. Effect of C. olitorius on the body weight, adipocytes, leptin, adiponectin, and glucose metabolism in a rodent model of obesity
According to the Centers for Disease Control and Prevention (CDC), obesity is defined by increased body mass index (30 kg/m2) (70). It is characterized by an excessive accumulation or hypertrophy of adipocytes, partly due to increased calorie intake, without physical activities. An overview of evidence showing the effects of C. olitorius on basic glycemic control is presented in Table 2. According to a study by Lee et al. (38), the use of HFD to induce obesity in C57BL/6J mice and subsequent administration of 100 mg C. olitorius extract showed a significant decrease in body weight. The same group revealed that administration of C. olitorius treatment at 4 mg/kg molokhia leaf polysaccharide fraction for 8 weeks reduces body weight in C57BL/6N mice fed HFD (37). However, this time around, the effects were accompanied by a significant decrease in the size of adipocytes. This reflects the potential effect of this antioxidant plant during obesity.
Consistently, adipocyte in obesity is associated with the recruitment of monocytes and inflammation, which are central to the development of insulin resistance and further CVD (71). Therefore, a reduced adipocyte size following C. olitorius administration could minimize the risk of insulin resistance in obesity and alleviate secondary complications. Additionally, in Sprague–Dawley rats fed HFD to induce obesity, the administration of C. olitorius at either a dose of 300 mg/kg or 400 mg/kg showed a significant decrease in serum glucose and insulin (48). The same study also showed a significant reduction in leptin concomitant to an increase in adiponectin; this is partly because of the direct relationship between adipocytes in obesity and its influence on leptin levels. The high content of phytol found in C. olitorius reduces leptin, which is associated with the reversal of insulin resistance in obesity. This action is mediated by the inhibition of inflammatory cytokines that trigger insulin resistance. According to a study by Yagishita et al. (72), hypothalamic-related oxidative stress induces leptin resistance, which results in insulin resistance and obesity.
Conversely, adipocyte macrophages contribute to increased levels of inflammatory cytokines such as TNF-α, IL-1β, and IL-6. These inflammatory cytokines induce insulin resistance by activating the suppressors of cytokine signaling proteins in insulin-target tissues (73). Both leptin and adiponectin are important in obesity through indirect regulation of food intake and body weight (74). In fact, leptin reduces body weight by decreasing food intake. However, obesity is associated with an elevated level of leptin, which can stimulate hunger, increase food intake and impair metabolism; this exacerbates obesity the latter results in extra body weight gain in the form of adipose storage (75).
On the other hand, adiponectin, an adipokine produced by adipose, exhibits antioxidant and anti-inflammatory properties that regulate blood glucose, lipid metabolism, and insulin sensitivity (76). Notably, alteration in the adiponectin gene expression and its receptors in obesity is associated with decreased levels and sensitivity; the latter results in insulin resistance, exacerbating hyperinsulinemia. In C57BL/6 with knocked low-density lipoprotein, receptor (LDLR-/-) mice fed HFD to induce obesity when given 1 and 3% C. olitorius for 8 weeks showed a significant decrease in body 26.7 and 27.2 g, respectively, when compared to control 28.3 g, p = 0.032 (28). In addition, the same study revealed a significant decrease in liver weight at both doses, 1.5 and 1.43 g, compared to the control, 1.74 g, p = 0.001. Obese status is associated with fat accumulation in the liver, resulting in liver hypertrophy, chronic inflammation, and cirrhosis.
In a recent study by Airadion et al. (35), 3 ml oral administration of C. olitorius in obese Albino rats; significantly decreased FBG when compared to the control (p < 0.05). Similarly, the intergroup difference also revealed a significant decrease in FBG post-treatment compared to pre-treatment (p < 0.05). It is assumed that the potential effect of C. olitorius in ameliorating hyperglycemia may be mediated by AA found in C. olitorius, as it improves insulin sensitivity (77). AA is an antioxidant, and a previous meta-analysis has shown that AA effectively reduces blood glucose (78).
Despite other studies showing the beneficial effects of C. olitorius on body weight, FBG, and insulin sensitivity, conflicting results were reported by Aikawa et al. (39), who found that administration of a 3% C. olitorius in obese rats had no significant effect on FBG and insulin. However, it is important to note that this study used an obese-hyperphagia model of OLETF rats (39). Therefore, their findings may be influenced by the hyperphagia stage as it is associated with increased ghrelin hormone in obesity (79, 80). Ghrelin is known to increase appetite (81), and therefore, it is likely to exacerbate obesity and insulin resistance despite the administration of C. olitorius.
Similarly, another study found contrasting findings as it reported no evidential improvement in hyperglycemia and body weight following the administration of C. olitorius. For example, 18 days of 300 mg/kg of C. olitorius in Wistar rats showed moderate body weight gain (+ 0.11 g) compared to control (+ 0.48 g) despite showing consecutive weight loss at days 6, 9, and 12. However, this was not statistically significant (p > 0.05) (47). Although these studies did not show a significant difference per se, the results may be explained partly by the gender and the strain and number of the rats used; for instance, other studies reported that they used males, while this researcher used female rats. A study by Taraschenko et al. (82) reported that female rats, when fed HFD, do not become obese and do not respond to treatment compared to male rats. Additionally, the small sample size (n = 3 rats per group) might have influenced the statistical power of the investigation. It has been shown previously that a small sample size affects the power and effect size of experiments in pre-clinical and clinical studies (83).
7. Effect of C. olitorius on lipid profile in rodent models of T2D
The lipid profile encompasses TC, TG, LDL-c, and HDL-c. These remain the primary markers of dyslipidemia in obesity and diabetes. The evidence gathered in this review suggests the potential of C. olitorius as anti-hyperlipidemia. The general overview of the studies reporting on the impact of C. olitorius in alleviating hepatic lipid accumulation to improve dyslipidemia in experimental models of T2D is presented in Table 2. An impaired lipid profile in T2D is associated with dyslipidemia, atherosclerosis, and an increased risk of CVD (61). According to Anyasor et al. (50), 80 g of C. olitorius in T2D Albino rats improved triglyceride (82.44 mg/dl), TC (108.6 mg/dl), and LDL-c (55.98 mg/dL) when compared to control TG (127.03 mg/dl), TC (137.51 mg/dl), LDL-c (76.82 mg/dl), p < 0.05. Similarly, when Wistar rats were given about 100–200 mg/kg of methanolic extract of C. olitorius for 21 days, they showed a decrease in TC level (54). However, only 200 mg/kg of the extract demonstrated a significant decrease in TC (125.0 mg/kg) compared to the control 210.0 mg/kg, p < 0.05. A 14-day administration of either 200, 400, or 800 mg/kg of C. olitorius in T2D Albino rats also showed a significant decrease (p < 0.05) in TC, TG, LDL-c, total protein, concomitant to an increased HDL-c (34). Consistently, treatment with C. olitorius in HFD-fed STZ-induced diabetic rats, TC and TG levels significantly decreased when compared to an untreated group (p < 0.05) (58). The findings are corroborated by Onyechi et al. (56) who reported a decrease in serum TC, TG, and LDL-c, concomitant to increased HDL-c levels (p < 0.05). A similar trend was observed by Swayer and Kadhim (49), who also demonstrated a significant decrease in TC (73.05 mg/dL), TG (85.4 mg/dL), LDL-c (36.07 mg/dL), and coupled with increased HDL-c (19.9 mg/dL) (p < 0.05).
According to the evidence synthesized in this review, we are of the opinion that C. olitorius has the potential for use as an alternative treatment to curb hyperlipidemia in T2D (Figure 4). While this evidence is obtained from pre-clinical studies, it would be better to understand the mode of action of this plant in ameliorating hyperlipidemia. While such benefits are acknowledged in ameliorating dyslipidemia, it seems they are attributable to ferulic acid found in this plant, partly because it can inhibit 3-Hydroxy-3-methyl glutaryl coenzyme A (HMG-CoA) reductase inhibitors and thus resulting in reduced synthesis of cholesterol and hence low levels of TC and LDL-c. Moreover, the antioxidant effect of ferulic acid may also decrease lipid peroxidation, causing decreases in the oxidized LDL-c (84).
8. Effect of C. olitorius on lipid profile in rodent models of obesity
The general summary showing the potential of C. olitorius as anti-hyperlipidemia is presented in Table 2. For example, a study by Gomaa et al. (48) demonstrated that C. olitorius at 300 and 400 mg/kg doses for 8 weeks significantly reduced plasma TG levels in 8.27 and 6.28 mg/dL, respectively, compared to untreated HFD-fed Sprague–Dawley obese rats 152.25 mg/dL, p < 0.05. Another study also corroborates the prior findings as reported that C. olitorius supplementation at 300 mg/kg for 18 days could reduce TG (0.56 mg/dL) levels concomitant to an increase in the HDL-c (0.26 mg/dL) level compared to control (0.8 and 0.39 mg/dL), p < 0.05, respectively (47). In C57BL/6N, male mice on 8-week HFD for induction of obesity showed that 4 mg/kg of C olitorius is effective as it significantly reduced serum TG and LDL-c levels (p < 0.05) (37). C57BL/6 (LDLR-/-) mice fed HFD for 8 weeks and treated with 3% of C. olitorius revealed significantly decreased TG levels 192 mg/dL compared to control (262 mg/dL), p = 0.009 (28). Albino rats administered with 3 ml of C. olitorius also demonstrated a beneficial impact by reducing TG, TC, and LDL-c without significant changes in HDL-c levels, p < 0.05 (35).
Contradicting results were reported by Lee et al. (38) who revealed no significant difference in TG and TC (p > 0.05) following treatment with 100 mg of C. olitorius in HFD-fed C57BL/6J mice. Similarly, another study showed limited potential effects of C. olitorius extract and showed no significant difference in serum and liver TG, TC (p > 0.05) in the obese-hyperphagia model of OLETF rats (39). Nevertheless, experimental evidence summarized in this review suggests that C. olitorius may improve lipid metabolism. It seems C. olitorius can ameliorate hyperlipidemia through various mechanisms; for example, the beneficial effects on lipid profile could be attributed to C. olitorius phenolic content, with antioxidative properties, which include stimulation of lipid metabolism in the liver, removal of excess fat in adipocytes and the liver, and lower plasma and hepatic lipid levels, resulting in an anti-obesity effect (28). In addition, the saponins content in the root of C. olitorius may contribute to its anti-hyperlipidemic and anti-hypercholesterolemia (35, 85). Furthermore, evidence suggests that C. olitorius’s ability to reduce the TC and TG levels in the body is attributable to its alkaloid content, which increases the hepatic LDL-c receptor expression and inhibits lipid synthesis in the liver (86–88).
9. The general overview of C. olitorius on oxidative stress and inflammation in rodent models of obesity and T2D
Chronic inflammation induced by obesity can result in β-cell dysfunction, insulin resistance, and T2D (89). Primary pro-inflammatory markers such as TNF-α, IL-6, and IL-8 induce inflammation and are central to the pathogenesis of CVD associated with obesity and T2D. In a homeostatic state, there is a general balance between the ROS produced and the ability of the body to neutralize these active molecules. However, excessive ROS generation can damage the structure and triggers an inflammatory response and oxidative stress that initiates the development of T2D (90) and cardiovascular disease (91). Important widely used biomarkers of oxidative stress include malonaldehyde (MDA), superoxide dismutase (SOD) and enzyme δ-aminolevulinic acid dehydratase (ALAD) were reported in the gathered evidence. Antioxidants are known to counteract the reactive response generated by ROS; this ameliorates oxidative stress by scavenging free radical molecules (92). A report by Saliu and the team showed a significant decrease in MDA (p < 0.05) following treatment with 100 mg/kg C. olitorius extract in T2D (55). However, the same study revealed a significant increase in catalase, SOD, and ALAD (p < 0. 05) compared to the untreated T2D group. Moreover, another study reported a significant decrease (p < 0.05) in MDA when 10% jute leave was administered in T2D rats (58).
Similar observations were reported by another group of researchers who showed decreased SOD activities in T2D rats treated with 150 mg/kg ethanolic extract of C. olitorius (118.3 IU/L) compared to the untreated group (216.1 IU/L). Interestingly when the baseline was compared to post-treatment, the same study at 150 mg/kg registered a 0.5-fold decrease (230.8 IU/L) in SOD activity (57). The same study reported a decrease in catalase activity in the treated group (97.8 IU/L) compared to the untreated (100.6 IU/L). The activities of these enzymes are crucial in protecting cell integrity from oxidative stress. Therefore, the evidence gathered in this review suggests that C. olitorius may also reduce oxidative stress in T2D individuals. It has been confirmed in rodent models that C. olitorius significantly ameliorates oxidative stress (29, 55, 93). The antioxidant property of C. olitorius is attributable to flavonoids, AA (31, 94), carotenoids (45), and phenols contents (95).
With regards to inflammation, a study by Lee et al. (37) showed that 8-week treatment with 4 mg/kg of C. olitorius extract in the HFD-induced obese C57BL/6N mouse model significantly (p < 0.05) decreases inflammatory markers. These results are further supported by Gomaa et al. (48), who reported a significant decrease in IL-1β (p < 0.01) and TNF-α (p < 0.01) in obese Sprague–Dawley albino rats treated with C. olitorius extract at a dose of 300 or 400 mg/kg for 8 weeks (48). These results support the potential effect of this plant as an anti-inflammatory agent (96), and the effects seem to be mediated by active compounds found in this plant, such as thiamine (97) and riboflavin (98). These compounds reduce inflammation by inhibiting nitric oxide (NO), cyclo-oxygenase (COX), and ROS tyrosine enzymes (99). On the other hand, AA present in C. olitorius attenuates inflammation by inhibiting oxidative stress, Iκκ-αβ, NADPH oxidase, NF-κβ, and cytokine storm (Figure 5). Additionally, the ionone and glycosides found in the leaves of C. olitorius also show an inhibitory effect on histamine, thus reducing inflammation (100). C. olitorius reduces inflammation by inhibiting NO production, and this mechanism is mediated by corchori fatty acids present in this plant (101). The evidence suggests that C. olitorius leaves may be an excellent alternative nutraceutical supplement against T2D-related inflammation (Figure 5 and Table 1).
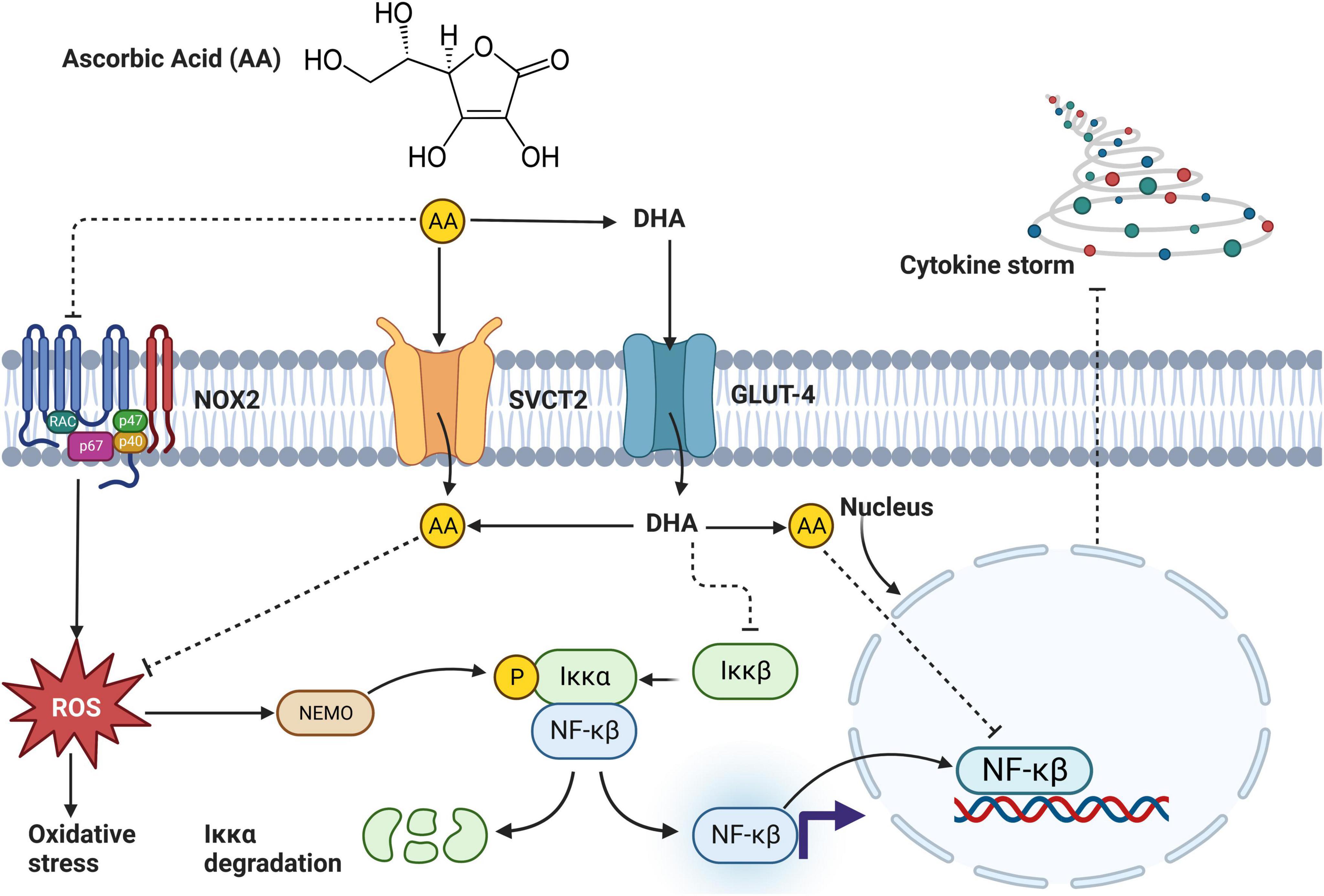
Figure 5. An overview mechanism by which ascorbic acid (AA) found in Corchorus olitorius reduces inflammation. AA enters the cell through sodium-vitamin C cotransporter 2 (SVCT2) or indirectly as dehydroascorbic acid (DHA). In the cell, DHA is converted to AA and directly inhibits activation of NF-κβ or through a series of pathways involving suppressing reactive oxygen species (ROS) generation and slowing down oxidative stress. Alternatively, AA blocks NADPH oxidase (NOX2) activation, further inhibiting ROS generation, and oxidative stress. Inhibition of NF-κβ directly inhibits inflammation (102).
10. The safety profile of C. olitorius for consumption or treatment
Although the toxicological reports are limited, the available evidence suggests that the C. olitorius plant is safe, as no severe adverse events have been reported in rodent models of obesity and T2D. For instance, experiments that investigated the toxicity of C. olitorius extract using different doses (50, 100, 200, and 400 mg/kg) in Swiss albino mice showed no adversity in all doses, and there were no changes in the behavioral pattern or any mortality reported (103, 104). Thus, it is evident that the C. olitorius treatment is not toxic, as low and high doses were used in the experiments and still showed no evidence of adversity. Our current review showed that no cases of adversities were reported when C. olitorius was administered at a low or high dose.
11. Overall evidence, concluding remarks, and future directions
Although there are widely used anti-diabetic pharmaceutical drugs, remedies derived from natural antioxidant plants increasingly show positive outcomes when regulating glycemic and lipid metabolism, thus gaining scientific interest. This is attributable to their antioxidant properties and the fact that they are not toxic and have no side effects compared to widely used diabetic drugs. Evidence from pre-clinical experiments in the current review stipulates that C. olitorius reduced various pro-inflammatory cytokines, hyperglycemia, and oxidative stress to ameliorate obese and T2D-associated secondary complications. Evidence presented in Table 2 supports the view that C. olitorius administration at a low dose of between 2 and 4 mg/kg for 8 weeks in obese could improve inflammation by reducing inflammatory cytokines such as TNF-α, IL-6, and IL-8 concomitant to reducing lipid peroxidation. Similarly, a high dose of 300–400 mg/kg of C. olitorius for 8 weeks in obese mice could improve inflammation by significantly reducing plasma IL-1β and TNF-α. Consistent results showed a beneficial effect of C. olitorius in regulating glucose metabolism, as demonstrated by a significant reduction in FBG.
Interestingly, C. olitorius administration in obese and T2D models improved lipid metabolism, as revealed by a significant decrease in TG, TC, and LDL-c. These results are interesting, considering that many individuals with obesity and T2D develop CVD-related complications, which remain at high risk for mortality worldwide. Therefore, using a natural treatment with anti-hyperglycemic, inflammatory, while reducing dyslipidemia and oxidative stress without side effects, may help reduce the burden of CVD in the obese and T2D population. Although the anti-hyperglycemic effect of this plant was notable in T2D rodents, conflicting results are still observed in the obese model. According to our knowledge, no clinical evidence has been published exploring the effect of this plant on obese and diabetic patients. Hence, it would be essential to examine the antioxidant properties of this plant in these populations, given that the results obtained in pre-clinical studies are promising. While such therapeutic benefits and efficacy are acknowledged in pre-clinical studies, additional evidence is still required to establish the safety profile of C. olitorius in rodent models of obesity and diabetes. Moreover, research is still warranted to establish methods to effectively improve its absorption and bioavailability, including establishing the desirable doses and ascertaining its efficacy and safety in obesity and T2D population.
Author contributions
KM: conceptualization, writing—original draft, data curation, and methodology. KM and WP: writing—review and editing. Both authors approved the final version of the manuscript.
Acknowledgments
KM thank Profs. Anna Tsotetsi-Khambule and F. Tabit of the University of South Africa for mentorship.
Conflict of interest
The authors declare that the research was conducted in the absence of any commercial or financial relationships that could be construed as a potential conflict of interest.
Publisher’s note
All claims expressed in this article are solely those of the authors and do not necessarily represent those of their affiliated organizations, or those of the publisher, the editors and the reviewers. Any product that may be evaluated in this article, or claim that may be made by its manufacturer, is not guaranteed or endorsed by the publisher.
Supplementary material
The Supplementary Material for this article can be found online at: https://www.frontiersin.org/articles/10.3389/fnut.2023.1099880/full#supplementary-material
References
1. International Diabetes Federation. IDF Diabetes Atlas 10th Edition. (2021). Available online at: www.diabetesatlas.org (accessed December 3, 2022).
2. Yaribeygi H, Sathyapalan T, Atkin S, Sahebkar A. Molecular mechanisms linking oxidative stress and diabetes mellitus. Oxid Med Cell Longev. (2020) 2020:8609213. doi: 10.1155/2020/8609213
3. Ahangarpour A, Oroojan A, Khorsandi L, Kouchak M, Badavi M. Hyperglycemia-induced oxidative stress in isolated proximal tubules of mouse: the in vitro effects of myricitrin and its solid lipid nanoparticle. Arch Physiol Biochem. (2021) 127:422–8. doi: 10.1080/13813455.2019.1647250
4. Eftekharpour E, Fernyhough P. Oxidative stress and mitochondrial dysfunction associated with peripheral neuropathy in type 1 diabetes. Antioxid Redox Signal. (2022) 37:578–96. doi: 10.1089/ars.2021.0152
5. Ogura S, Shimosawa T. Oxidative stress and organ damages. Curr Hypertens Rep. (2014) 16:452. doi: 10.1007/s11906-014-0452-x
6. Warraich H, Rana J. Dyslipidemia in diabetes mellitus and cardiovascular disease. Cardiovasc Endocrinol. (2017) 6:27–32. doi: 10.1097/XCE.0000000000000120
7. Wu L, Parhofer K. Diabetic dyslipidemia. Metabolism. (2014) 63:1469–79. doi: 10.1016/j.metabol.2014.08.010
8. Luc K, Schramm-Luc A, Guzik T, Mikolajczyk T. Oxidative stress and inflammatory markers in prediabetes and diabetes. J Physiol Pharmacol. (2019) 70:809–24. doi: 10.26402/jpp.2019.6.01
9. Azemi A, Mokhtar S, Hou L, Sharif S, Rasool A. Model for type 2 diabetes exhibits changes in vascular function and structure due to vascular oxidative stress and inflammation. Biotech Histochem. (2021) 96:498–506. doi: 10.1080/10520295.2020.1823480
10. Zatterale F, Longo M, Naderi J, Raciti G, Desiderio A, Miele C, et al. Chronic adipose tissue inflammation linking obesity to insulin resistance and type 2 diabetes. Front Physiol. (2020) 10:1607. doi: 10.3389/fphys.2019.01607
11. Landecho M, Tuero C, Valentí V, Bilbao I, de la Higuera M, Frühbeck G. Relevance of leptin and other adipokines in obesity-associated cardiovascular risk. Nutrients. (2019) 11:2664. doi: 10.3390/nu11112664
12. Sahakyan G, Vejux A, Sahakyan N. The role of oxidative stress-mediated inflammation in the development of T2DM-induced diabetic nephropathy: possible preventive action of tannins and other oligomeric polyphenols. Molecules. (2022) 27:9035. doi: 10.3390/molecules27249035
13. Rehman K, Akash M. Mechanism of generation of oxidative stress and pathophysiology of type 2 diabetes mellitus: how are they interlinked? J Cell Biochem. (2017) 118:3577–85. doi: 10.1002/jcb.26097
14. Hostalek U, Campbell I. Metformin for diabetes prevention: update of the evidence base. Curr Med Res Opin. (2021) 37:1705–17. doi: 10.1080/03007995.2021.1955667
15. Bonfim M, Oliveira A, do Amaral S, Monteiro H. Treatment of dyslipidemia with statins and physical exercises: recent findings of skeletal muscle responses. Arq Bras Cardiol. (2015) 104:324–31. doi: 10.5935/abc.20150005
16. Infante M, Leoni M, Caprio M, Fabbri A. Long-term metformin therapy and vitamin B12 deficiency: an association to bear in mind. World J Diabetes. (2021) 12:916–31. doi: 10.4239/wjd.v12.i7.916
17. Urina-Jassir M, Pacheco-Paez T, Paez-Canro C, Urina-Triana M. Statin associated adverse reactions in Latin America: a scoping review. BMJ Open. (2021) 11:e050675. doi: 10.1136/bmjopen-2021-050675
18. Mosenzon O, Alguwaihes A, Leon J, Bayram F, Darmon P, Davis T, et al. CAPTURE: a multinational, cross-sectional study of cardiovascular disease prevalence in adults with type 2 diabetes across 13 countries. Cardiovasc Diabetol. (2021) 20:154. doi: 10.1186/s12933-021-01344-0
19. Hong T, Yan Z, Li L, Tang W, Qi L, Ye J, et al. The prevalence of cardiovascular disease in adults with type 2 diabetes in china: results from the cross-sectional CAPTURE study. Diabetes Ther. (2022) 13:969–81. doi: 10.1007/s13300-022-01243-x
20. Glil Alkushi A. Alternative natural management of dyslipidemia. In: SI McFarlane editor. Dyslipidemia. Vienna: IntechOpen (2019). doi: 10.5772/intechopen.82430
21. Houston M. The role of nutraceutical supplements in the treatment of dyslipidemia. J Clin Hypertens. (2012) 14:121–32. doi: 10.1111/j.1751-7176.2011.00576.x
22. Xu L, Li Y, Dai Y, Peng J. Natural products for the treatment of type 2 diabetes mellitus: pharmacology and mechanisms. Pharmacol Res. (2018) 130:451–65. doi: 10.1016/j.phrs.2018.01.015
23. Putra I, Fakhrudin N, Nurrochmad A, Wahyuono S. Antidiabetic activity of Coccinia grandis (L.) Voigt: bioactive constituents, mechanisms of action, and synergistic effects. J Appl Pharm Sci. (2022) 12:041–054. doi: 10.7324/JAPS.2021.120103
24. Khutami C, Sumiwi S, Khairul Ikram N, Muchtaridi M. The effects of antioxidants from natural products on obesity, dyslipidemia, diabetes and their molecular signaling mechanism. Int J Mol Sci. (2022) 23:2056. doi: 10.3390/ijms23042056
25. Leh H, Lee L. Lycopene: a potent antioxidant for the amelioration of type ii diabetes mellitus. Molecules. (2022) 27:2335. doi: 10.3390/molecules27072335
26. Rehman K, Ali M, Akash M. Genistein enhances the secretion of glucagon-like peptide-1 (GLP-1) via downregulation of inflammatory responses. Biomed Pharmacother. (2019) 112:108670. doi: 10.1016/j.biopha.2019.108670
27. Oboh G, Raddatz H, Henle T. Characterization of the antioxidant properties of hydrophilic and lipophilic extracts of Jute (Corchorus olitorius) leaf. Int J Food Sci Nutr. (2009) 60(Suppl. 2):124–34. doi: 10.1080/09637480902824131
28. Wang L, Yamasaki M, Katsube T, Sun X, Yamasaki Y, Shiwaku K. Antiobesity effect of polyphenolic compounds from molokheiya (Corchorus olitorius L.) leaves in LDL receptor-deficient mice. Eur J Nutr. (2011) 50:127–33. doi: 10.1007/s00394-010-0122-y
29. Yabani D, Adotey G. Antioxidant activity of Corchorus olitorius and its effect on lipid peroxidation in mice. Food Sci. (2018) 114:49526–30.
30. Ademiluyi A, Oboh G, Aragbaiye F, Oyeleye S, Ogunsuyi O. Antioxidant properties and in vitro α-amylase and α-glucosidase inhibitory properties of phenolics constituents from different varieties of Corchorus spp. J Taibah Univ Med Sci. (2015) 10:278–87. doi: 10.1016/j.jtumed.2014.11.005
31. Mohammed Z, Milala M, Imam S. Determination of vitamin A and vitamin C in Corchorus olitorius (bush okra) using high performance liquid chromatography. Biology. (2019) 3:376–84.
32. Choudhary S, Kumari N, Sharma H, Ojha P, Uraon J. Bioactive compounds of mallow leaves (Corchorus species). In: HN Murthy, KY Paek editors. Bioactive Compounds in Underutilized Vegetables and Legumes. Cham: Springer (2021). doi: 10.1007/978-3-030-57415-4_9
33. Ndlovu J, Afolayan A. Nutritional analysis of the South African wild vegetable Corchorus olitorius. Asian J Plant Sci. (2008) 7:615–8. doi: 10.3923/ajps.2008.615.618
34. Olusanya AR, Ifeoluwa BS, Aboyewa Jumoke A, Khadijat B. “Antidiabetic and safety properties of ethanolic leaf extract of Corchorus olitorius in alloxan-induced diabetic rats. In: Diabetes Food Plan. London: InTech (2018). p. 57–70. doi: 10.5772/intechopen.71529
35. Airaodion A, Akinmolayan J, Ogbuagu E, Airaodion E, Ogbuagu U, Awosanya O. Effect of methanolic extract of Corchorus olitorius leaves on hypoglycemic and hypolipidaemic activities in albino rats. Asian Plant Res J. (2019) 2:1–13. doi: 10.9734/aprj/2019/v2i430054
36. Nduka A, Oluwatoyin A, Temitope A, Odutola O. Hypoglycemic and hypolipidemic effects of Corchorus olitorius leaves as a food supplement on rats with alloxan-induced diabet. Ukr Biochem J. (2020) 92:63–9. doi: 10.15407/ubj92.04.063
37. Lee H, Oh M, Do M, Kim Y, Choi I, Kim Y, et al. Dietary rhamnogalacturonan-I rich extracts of molokhia ameliorate high fat diet-induced obesity and gut dysbiosis. J Nutr Biochem. (2022) 103:108954. doi: 10.1016/j.jnutbio.2022.108954
38. Lee H, Oh M, Do M, Kim Y, Park H. Molokhia leaf extract prevents gut inflammation and obesity. J Ethnopharmacol. (2020) 257:112866. doi: 10.1016/j.jep.2020.112866
39. Aikawa Y, Wakasugi Y, Yoneda M, Narukawa T, Sugino K, Yamashita T. Effect of Corchorus olitorius on glucose metabolism, lipid metabo-lism, and bone strength in a rat model of obesity with hyperphagia. Int J Anal Bio Sci. (2020)8:93–101.
40. Joseph J, Deedwania P, Acharya T, Aguilar D, Bhatt D, Chyun D, et al. Comprehensive management of cardiovascular risk factors for adults with type 2 diabetes: a scientific statement from the American Heart Association. Circulation. (2022) 145:e722–59. doi: 10.1161/CIR.0000000000001040
41. Nakaziba R, Anyolitho MK, Amanya SB, Sesaazi CD, Byarugaba F, Ogwal-Okeng J, et al. Traditional medicinal vegetables in northern Uganda: an ethnobotanical survey. Int J Food Sci. (2021) 2021:5588196. doi: 10.1155/2021/5588196
42. Nakaziba R, Kenneth Anyolitho M, Bright Amanya S, Ogwal-Okeng J, Alele P. Traditional Uses of Corchorus olitorius L in Oyam District, Northern Uganda: A Cross-Sectional Ethnobotanical Survey. (2020). doi: 10.21203/rs.3.rs-18590/v1
43. Giro A, Ferrante A. Yield and quality of Corchorus olitorius baby leaf grown in a floating system. J Horticult Sci Biotechnol. (2016) 91:603–10. doi: 10.1080/14620316.2016.1200955
44. Holst B, Williamson G. Nutrients and phytochemicals: from bioavailability to bioefficacy beyond antioxidants. Curr Opin Biotechnol. (2008) 19:73–82. doi: 10.1016/j.copbio.2008.03.003
45. Mahbubul Islam MD. Biochemistry, medicinal and food values of jute (Corchorus capsularis L. and C. olitorius L.) leaf: a review. Int J Enhanc Res Sci Technol Eng. (2013) 2:35–44.
46. Nyadanu D, Lowor S. Promoting competitiveness of neglected and underutilized crop species: comparative analysis of nutritional composition of indigenous and exotic leafy and fruit vegetables in Ghana. Genet Resour Crop Evol. (2015) 62:131–40. doi: 10.1007/s10722-014-0162-x
47. Adon A, Ackah B, Yayé G, Okou C, Brahima R, Djaman J. Evaluation of the effects of Corchorus olitorius L. and Carapa procera in the treatment of obesity. Int J Res Med Sci. (2018) 6:1078. doi: 10.18203/2320-6012.ijrms20181259
48. Gomaa A, El-Sers D, Al-Zokeim N, Gomaa M. Amelioration of experimental metabolic syndrome induced in rats by orlistat and Corchorus olitorius leaf extract; role of adipo/cytokines. J Pharm Pharmacol. (2019) 71:281–91. doi: 10.1111/jphp.13032
49. Swayeh N, Kadhim H. Effects of methanol extract of Corchorus olitorius cultivated in Iraq on high fat diet plus streptozotocin-induced type ii diabetes in rats. Int J Drug Deliv Technol. (2022) 12:754–9. doi: 10.25258/ijddt.12.2.51
50. Anyasor GN, Adekanye O, Adeyemi O, Osilesi O. Hypoglycemic and hypolipidemic effects of Corchorus olitorius leaves as a food supplement on rats with alloxan-induced diabet. Ukr Biochem J. (2020) 92:63–9.
51. Ali M, Asrafuzzaman M, Tusher M, Rahman M, Rahman M, Roy B, et al. Comparative study on antidiabetic effect of ethanolic extract of jute leaf on neonatal streptozotocin- induced type-2 diabetic model rat. J Pharm Res Int. (2020) 32:60–71. doi: 10.9734/jpri/2020/v32i3130922
52. Mercan N, Toros P, Söyler G, Hanoglu A, Kükner A. Effects of Corchorus olitorius and protacatechuic acid on diabetic rat testis tissue. Int J Morphol. (2020) 38:1330–5.
53. Mohammed A, Luka C, Ngwen A, Omale O, Yaknan B. Evaluation of the effect of aqueous leaf of jute mallow Corchorus olitorius on some biochemical parameters in alloxan-induced diabetic rats. Eur J Pharm Med Res. (2019) 6:652–8.
54. Patil D, Jain A. In-vivo antidiabetic activity of methanolic extract of Corchorus olitorius for the management of type 2 diabetes. J Pharmacogn Phytochem. (2019) 8:3213–8.
55. Saliu J, Ademiluyi A, Boligon A, Oboh G, Schetinger M, Rocha J. Dietary supplementation of jute leaf (Corchorus olitorius) modulates hepatic delta-aminolevulinic acid dehydratase (δ-ALAD) activity and oxidative status in high-fat fed/low streptozotocin-induced diabetic rats. J Food Biochem. (2019) 43:e12949. doi: 10.1111/jfbc.12949
56. Onyechi AU, Ibeanu V, Maduforo A, Ugwuonah A, Nsofor L. Effect of Corchorus olitorius, Myrianthus arboreus and Annona muricata aqueous leaves extract of bodyweight levels, blood glucose and lipid profile of alloxan-induced diabetic rats. J Diet Assoc Nigeria. (2018) 9:2635–3326.
57. Omeje K, Ezike T, Omeje H, Ezema B. Effect of ethanol extract of Corchorus olitorus leaf on glucose level and antioxidant enzymes of streptozotocin-induced hyperglycemic rat. Nigerian Soc Exp Biol. (2016) 28:121–7. doi: 10.13140/RG.2.2.32748.51844
58. Saliu JA, Oboh G, Schetinger MR, Stefanello N, Rocha JBT. Antidiabetic potentials of jute leaf (Corchorus olitorius) on type-2 diabetic rats. J Emerg Trends Eng Appl Sci. (2015) 6:223–30.
59. Egua MO, Etuk U, Bello S, Sanusi WH. Anti diabetic activity of ethanolic seed extract of Corchorus olitorius. Int J Sci. (2013) 12:8–21.
60. Mokgalaboni K, Dludla P, Mkandla Z, Mutize T, Nyambuya T, Mxinwa V, et al. Differential expression of glycoprotein IV on monocyte subsets following high-fat diet feeding and the impact of short-term low-dose aspirin treatment. Metabol Open. (2020) 7:100047. doi: 10.1016/j.metop.2020.100047
61. Mokgalaboni K, Dludla P, Nyambuya T, Yakobi S, Mxinwa V, Nkambule B. Monocyte-mediated inflammation and cardiovascular risk factors in type 2 diabetes mellitus: a systematic review and meta-analysis of pre-clinical and clinical studies. JRSM Cardiovasc Dis. (2020) 9:2048004019900748. doi: 10.1177/2048004019900748
62. Nakagawa K, Kishida H, Arai N, Nishiyama T, Mae T. Licorice flavonoids suppress abdominal fat accumulation and increase in blood glucose level in obese diabetic KK-A(y) mice. Biol Pharm Bull. (2004) 27:1775–8. doi: 10.1248/bpb.27.1775
63. Al-Ishaq R, Abotaleb M, Kubatka P, Kajo K, Büsselberg D. Flavonoids and their anti-diabetic effects: cellular mechanisms and effects to improve blood sugar levels. Biomolecules. (2019) 9:430. doi: 10.3390/biom9090430
64. Jubaidi F, Zainalabidin S, Taib I, Hamid Z, Budin S. The potential role of flavonoids in ameliorating diabetic cardiomyopathy via alleviation of cardiac oxidative stress, inflammation and apoptosis. Int J Mol Sci. (2021) 22:5094. doi: 10.3390/ijms22105094
65. Zhou T, Luo D, Li X, Luo Y. Hypoglycemic and hypolipidemic effects of flavonoids from lotus (Nelumbo nuficera Gaertn) leaf in diabetic mice. J Med Plants Res. (2009) 3:290–3.
66. Choudhary N, Khatik G, Suttee A. The possible role of saponin in type-II diabetes- a review. Curr Diabetes Rev. (2021) 17:107–21. doi: 10.2174/1573399816666200516173829
67. Qamar T, Saleem S, Fatima K, Pak A. (2011). Effect of thiamine on glycemic control in diabetic rats effect of thiamine on glycemic control in diabetic rats. Ann Pak Inst Med Sci. 7:213–6.
68. Sun L, Warren F, Gidley M. Natural products for glycaemic control: polyphenols as inhibitors of alpha-amylase. Trends Food Sci Technol. (2019) 91:262–73. doi: 10.1016/j.tifs.2019.07.009
69. Ćorković I, Gašo-Sokač D, Pichler A, Šimunović J, Kopjar M. Dietary polyphenols as natural inhibitors of α-amylase and α-glucosidase. Life. (2022) 12:1692. doi: 10.3390/life12111692
70. World Health Organization. WHO European Regional Obesity?: Report 2022. (2022). Available online at: https://apps.who.int/iris/handle/10665/353747 (accessed January 24, 2023).
71. Chait A, den Hartigh L. Adipose tissue distribution, inflammation and its metabolic consequences, including diabetes and cardiovascular disease. Front Cardiovasc Med. (2020) 7:22. doi: 10.3389/fcvm.2020.00022
72. Yagishita Y, Uruno A, Fukutomi T, Saito R, Saigusa D, Pi J, et al. Nrf2 improves leptin and insulin resistance provoked by hypothalamic oxidative stress. Cell Rep. (2017) 18:2030–44. doi: 10.1016/j.celrep.2017.01.064
73. Khodabandehloo H, Gorgani-Firuzjaee S, Panahi G, Meshkani R. Molecular and cellular mechanisms linking inflammation to insulin resistance and β-cell dysfunction. Transl Res. (2016) 167:228–56. doi: 10.1016/j.trsl.2015.08.011
74. Agostinis-Sobrinho C, Vicente S, Norkiene S, Rauckienë-Michaelsson A, Kievisienë J, Dubey V, et al. Is the leptin/adiponectin ratio a better diagnostic biomarker for insulin resistance than leptin or adiponectin alone in adolescents? Children. (2022) 9:1193. doi: 10.3390/children9081193
75. Izquierdo A, Crujeiras A, Casanueva F, Carreira M. Leptin, obesity, and leptin resistance: where are we 25 years later? Nutrients. (2019) 11:2704. doi: 10.3390/nu11112704
76. Balsan G, Vieira J, Oliveira A, Portal V. Relationship between adiponectin, obesity and insulin resistance. Rev Assoc Med Bras. (2015) 61:72–80. doi: 10.1590/1806-9282.61.01.072
77. Mason S, Rasmussen B, van Loon L, Salmon J, Wadley G. Ascorbic acid supplementation improves postprandial glycaemic control and blood pressure in individuals with type 2 diabetes: findings of a randomized cross-over trial. Diabetes Obes Metab. (2019) 21:674–82. doi: 10.1111/dom.13571
78. Ashor A, Werner A, Lara J, Willis N, Mathers J, Siervo M. Effects of vitamin C supplementation on glycaemic control: a systematic review and meta-analysis of randomised controlled trials. Eur J Clin Nutr. (2017) 71:1371–80. doi: 10.1038/ejcn.2017.24
79. DelParigi A, Tschöp M, Heiman M, Salbe A, Vozarova B, Sell S, et al. High circulating ghrelin: a potential cause for hyperphagia and obesity in Prader-Willi syndrome. J Clin Endocrinol Metab. (2002) 87:5461–4. doi: 10.1210/jc.2002-020871
80. Fittipaldi A, Hernández J, Castrogiovanni D, Lufrano D, De Francesco P, Garrido V, et al. Plasma levels of ghrelin, des-acyl ghrelin and LEAP2 in children with obesity: correlation with age and insulin resistance. Eur J Endocrinol. (2020) 182:165–75. doi: 10.1530/EJE-19-0684
81. Jiao Z, Luo Q. Molecular mechanisms and health benefits of ghrelin: a narrative review. Nutrients. (2022) 14:4191. doi: 10.3390/nu14194191
82. Taraschenko O, Maisonneuve I, Glick S. Sex differences in high fat-induced obesity in rats: effects of 18-methoxycoronaridine. Physiol Behav. (2011) 103:308–14. doi: 10.1016/j.physbeh.2011.02.011
83. Serdar C, Cihan M, Yücel D, Serdar M. Sample size, power and effect size revisited: simplified and practical approaches in pre-clinical, clinical and laboratory studies. Biochem Med. (2021) 31:010502. doi: 10.11613/BM.2021.010502
84. Bumrungpert A, Lilitchan S, Tuntipopipat S, Tirawanchai N, Komindr S. Ferulic acid supplementation improves lipid profiles, oxidative stress, and inflammatory status in hyperlipidemic subjects: a randomized, double-blind, placebo-controlled clinical trial. Nutrients. (2018) 10:713. doi: 10.3390/nu10060713
85. Marrelli M, Conforti F, Araniti F, Statti G. Effects of saponins on lipid metabolism: a review of potential health benefits in the treatment of obesity. Molecules. (2016) 21:1404. doi: 10.3390/molecules21101404
86. Kou S, Han B, Wang Y, Huang T, He K, Han Y, et al. Synergetic cholesterol-lowering effects of main alkaloids from rhizoma coptidis in HepG2 cells and hypercholesterolemia hamsters. Life Sci. (2016) 151:50–60. doi: 10.1016/j.lfs.2016.02.046
87. Al-Fartosy AJ, Zearah SA, Alwan NA. Total antioxidant capacity and antihyperlipidemic activity of alkaloid extract from aerial part of Anethum graveolens L plant. Eur Sci J. (2013) 9:413–23.
88. Brusq J, Ancellin N, Grondin P, Guillard R, Martin S, Saintillan Y, et al. Inhibition of lipid synthesis through activation of AMP kinase: an additional mechanism for the hypolipidemic effects of berberine. J Lipid Res. (2006) 47:1281–8. doi: 10.1194/jlr.M600020-JLR200
89. Rohm T, Meier D, Olefsky J, Donath M. Inflammation in obesity, diabetes, and related disorders. Immunity. (2022) 55:31–55. doi: 10.1016/j.immuni.2021.12.013
90. He L, He T, Farrar S, Ji L, Liu T, Ma X. Antioxidants maintain cellular redox homeostasis by elimination of reactive oxygen species. Cell Physiol Biochem. (2017) 44:532–53. doi: 10.1159/000485089
91. Moris D, Spartalis M, Spartalis E, Karachaliou G, Karaolanis G, Tsourouflis G, et al. The role of reactive oxygen species in the pathophysiology of cardiovascular diseases and the clinical significance of myocardial redox. Ann Transl Med. (2017) 5:326. doi: 10.21037/atm.2017.06.27
92. Poljsak B, Šuput D, Milisav I. Achieving the balance between ROS and antioxidants: when to use the synthetic antioxidants. Oxid Med Cell Longev. (2013) 2013:956792. doi: 10.1155/2013/956792
93. Al-Khalifah A, Ahmed F. Corchorus olitorius L. leaf extract protects rats from acrylamide-induced hepatic injury. Curr Res Nutr Food Sci. (2021) 9:833–40. doi: 10.12944/CRNFSJ.9.3.11
94. Aluwong T, Ayo J, Kpukple A, Oladipo O. Amelioration of hyperglycaemia, oxidative stress and dyslipidaemia in alloxan-induced diabetic Wistar rats treated with probiotic and vitamin C. Nutrients. (2016) 8:151. doi: 10.3390/nu8050151
95. Guzzetti L, Panzeri D, Ulaszewska M, Sacco G, Forcella M, Fusi P, et al. Assessment of dietary bioactive phenolic compounds and agricultural sustainability of an african leafy vegetable Corchorus olitorius L. Front Nutr. (2021) 8:667812. doi: 10.3389/fnut.2021.667812
96. Owoyele B, Oyewole A, Alimi M, Sanni S, Oyeleke S. Anti-inflammatory and antipyretic properties of Corchorus olitorius aqueous root extract in Wistar rats. J Basic Clin Physiol Pharmacol. (2015) 26:363–8. doi: 10.1515/jbcpp-2013-0166
97. Menezes R, Godin A, Rodrigues F, Coura G, Melo I, Brito A, et al. Thiamine and riboflavin inhibit production of cytokines and increase the anti-inflammatory activity of a corticosteroid in a chronic model of inflammation induced by complete Freund’s adjuvant. Pharmacol Rep. (2017) 69:1036–43. doi: 10.1016/j.pharep.2017.04.011
98. Mazur-Bialy A, Pocheć E. Riboflavin reduces pro-inflammatory activation of adipocyte-macrophage co-culture. Potential application of vitamin B2 enrichment for attenuation of insulin resistance and metabolic syndrome development. Molecules. (2016) 21:1724. doi: 10.3390/molecules21121724
99. Chen L, Deng H, Cui H, Fang J, Zuo Z, Deng J, et al. Inflammatory responses and inflammation-associated diseases in organs. Oncotarget. (2018) 9:7204–18. doi: 10.18632/oncotarget.23208
100. Yokoyama S, Hiramoto K, Fujikawa T, Kondo H, Konishi N, Sudo S, et al. Topical application of Corchorus olitorius leaf extract ameliorates atopic dermatitis in NC/Nga mice. Dermatol Aspects. (2014) 2:3. doi: 10.7243/2053-5309-2-3
101. Yoshikawa M, Murakami T, Shimada H, Yoshizumi S, Saka M, Yamahara J, et al. Medicinal foodstuffs. XIV. On the bioactive constituents of moroheiya. (2): new fatty acids, corchorifatty acids A, B, C, D, E, and F, from the leaves of Corchorus olitorius L. (Tiliaceae): structures and inhibitory effect on NO production in mouse peritoneal macrophages. Chem Pharm Bull. (1998) 46:1008–14. doi: 10.1248/cpb.46.1008
102. Fujii T, Fowler R, Vincent J. Vitamin C and thiamine for sepsis: time to go back to fundamental principles. Intensive Care Med. (2020) 46:2061–3. doi: 10.1007/s00134-020-06242-9
103. Haridy L, Shakerali S, Alghamdi R. Protective role of Corchorus olitorius L leaves extract against experimentally-induced hepatotoxicity. Int J Pharm Phytopharmacol Res. (2020) 10:50–60.
Keywords: Corchorus olitorius, diabetes, obesity, anti-diabetic compounds, inflammation, dyslipidemia, antioxidant
Citation: Mokgalaboni K and Phoswa WN (2023) Corchorus olitorius extract exhibit anti-hyperglycemic and anti-inflammatory properties in rodent models of obesity and diabetes mellitus. Front. Nutr. 10:1099880. doi: 10.3389/fnut.2023.1099880
Received: 16 November 2022; Accepted: 22 March 2023;
Published: 05 April 2023.
Edited by:
Michael Mathai, Victoria University, AustraliaReviewed by:
Parames C. Sil, Bose Institute, IndiaMlindeli Gamede, University of Pretoria, South Africa
Phetole Mangena, University of Limpopo, South Africa
Muhammad Sajid Hamid Akash, Government College University Faisalabad, Pakistan
Copyright © 2023 Mokgalaboni and Phoswa. This is an open-access article distributed under the terms of the Creative Commons Attribution License (CC BY). The use, distribution or reproduction in other forums is permitted, provided the original author(s) and the copyright owner(s) are credited and that the original publication in this journal is cited, in accordance with accepted academic practice. No use, distribution or reproduction is permitted which does not comply with these terms.
*Correspondence: Kabelo Mokgalaboni, bW9rZ2FrQHVuaXNhLmFjLnph