- 1Junior Research Group Nutritional Concepts, Institute of Nutritional Sciences, Friedrich Schiller University Jena, Jena, Germany
- 2Competence Cluster for Nutrition and Cardiovascular Health (nutriCARD) Halle-Jena-Leipzig, Jena, Germany
- 3Institute of Medical Statistics, Computer and Data Sciences, Jena University Hospital, Jena, Germany
- 4Institute of Clinical Chemistry and Laboratory Diagnostics, Jena University Hospital, Jena, Germany
- 5Institute of Transfusion Medicine, University Hospital Jena, Jena, Germany
- 6Department of Nutritional Toxicology, Institute of Nutritional Sciences, Friedrich Schiller University Jena, Jena, Germany
- 7Department of Nutritional Biochemistry and Physiology, Institute of Nutritional Sciences, Friedrich Schiller University Jena, Jena, Germany
Background: Regular consumption of the soluble dietary fiber β-glucan is associated with decreased total cholesterol (TC), low-density lipoprotein (LDL) cholesterol and blood glucose. Barley and oat flakes as natural sources of β-glucan were roasted to improve sensory quality. The aim of this study was to investigate whether roasting of barley and oat flakes changes the physiological impact of the β-glucan-rich flakes on glucose and lipid metabolism.
Method: A five-armed randomized crossover trial design was used. The intervention study was conducted from May 2018 to May 2019 and included 32 healthy subjects with moderately increased LDL cholesterol (≥2.5 mmol/L). During the 3-week intervention periods, 80 g of roasted or traditional barley or oat flakes, or four slices of white toast bread per day were consumed for breakfast. At the start and the end of each intervention, fasting and postprandial blood was taken. The intervention periods were separated by 3-week wash-out periods.
Results: During the interventions with the cereal flakes, TC and LDL cholesterol concentrations were significantly reduced compared to baseline values by mean differences of 0.27–0.33 mmol/L and 0.21–0.30 mmol/L, respectively (p < 0.05), while high-density lipoprotein (HDL) cholesterol was only reduced after the intervention with barley flakes (p < 0.05). After the intervention period with toast, TC and HDL cholesterol increased (p < 0.05). The fasting levels of triglycerides, fasting blood glucose and insulin did not change in any group. The effects of traditional and roasted varieties on blood lipids did not differ between the groups.
Conclusion: The regular consumption of traditional or roasted barley and oat flakes contributes to the management of cardiovascular diseases by improving TC and LDL cholesterol.
Clinical trial registration: https://clinicaltrials.gov/ct2/show/NCT03648112, identifier NCT03648112.
1. Introduction
Cardiovascular diseases (CVD), cancer, and diabetes mellitus type 2 (DMT2) are three of the four main non-communicable diseases which are responsible for most of premature deaths (1). These chronic diseases can be prevented by improving lifestyle factors including well-balanced, healthy diet rich in dietary fiber (2, 3). The regular consumption of dietary fiber can reduce blood cholesterol as well as postprandial glucose levels and insulin response (4). Numerous studies have shown that especially the consumption of β-glucan from oat and barley may reduce the risk for the development of CVD or DMT2 (5, 6).
Cereal β-glucan is a soluble dietary fiber and high-molecular non-starch polysaccharide comprised of β-(1–4)- and β-(1–3)-linked β-D-glucopyranosyl subunits in varying proportions. Cell walls of the endosperm and aleurone layer of barley and oat grains contain the highest amounts of β-glucan compared to other cereals (7–9). Oat and barley flakes contain an average of 4.6 and 5.2% β-glucan, respectively (10–12). Numerous studies indicated the health-promoting effects of barley and oat and provide the basis for official health claims by the European Food Safety Authority (EFSA) (13–33). Therefore, 4 g β-glucan from oats or barley for each 30 g of available carbohydrates should be consumed per meal to obtain a reduction of the postprandial glucose level (5, 34). For hypercholesterolemic patients it is suggested to consume 3 g of β-glucans from oats and barley per day to achieve a reduction of blood cholesterol and therefore a reduction of risk factors for CVD (34, 35).
These health-promoting effects of β-glucan depends on its physicochemical properties in particularly high molecular weight, viscosity, and solubility (36–42). Some in vivo studies showed a lower postprandial glucose (37, 38) and serum cholesterol with increasing viscosity of β-glucan (42).
Despite all these health-promoting effects and the fact that barley belongs to the four most cultivated grains worldwide behind maize, rice, and wheat, only less than one percent of cultivated barley is directly used as food in Europe. The largest amount is used for animal feed and as grain for malting and brewing to produce beer and whiskey. Oats are produced in smaller quantities but 14% of the oats produced in Europe are used as human food (43–46).
Roasting is a process that can increase sensory attractivity and the consumer acceptance of foods by improving texture and flavor of foods like nuts and grains. Schlörmann et al. (10, 11) showed in their studies, that scores for taste, texture, odor and appearance increased along with increasing roasting temperatures obtaining an optimal crispy texture and the typical roasted taste and aroma. However, this heat treatment also involves chemical and structural changes such as the formation of Maillard reaction products which are not only responsible for the typical roasted taste but also for the formation of acrylamide (47, 48). Schlörmann et al. (10, 11) have shown in previous studies that health-related compounds such as protein, dietary fiber and especially β-glucan were not affected by roasting barley at a maximum of 170–180°C and oat at a maximum of 160°C, while the sensory quality was improved. However, the physiological effects of roasted barley and oat products regarding the reduction of glucose and cholesterol still need to be investigated. Therefore, our intervention study was conducted to evaluate the effects of a regular consumption of barley and oat flakes in traditional and roasted form on glucose and lipid metabolism.
2. Materials and methods
2.1. Subjects
Thirty-two mildly hypercholesterolemic subjects [low-density lipoprotein (LDL) cholesterol: ≥2.5 mmol/L; aged between 25 and 75 years; 22 women, 10 men] from Thuringia (Germany) entered the study after giving their written informed consent. Subjects receiving lipid-lowering medications or glucocorticoids were not included. Those subjects suffering from gastrointestinal or metabolic diseases (e.g., diabetes mellitus), hypercholesteremic patients with previous familial cardio-vascular events, and subjects taking dietary supplements (e.g., fish oil capsules, vitamin E), having been treated in the previous 3 months with fiber-based dietary supplements and/or probiotics and having known food allergies were also excluded. LDL cholesterol was checked during a screening visit by taking a blood sample.
Subjects could be withdrawn from the study at any time after enrollment for the following reasons: at patient’s request, due to serious infections, or if patient compliance with the study protocol was doubtful. The study was conducted in accordance to the Helsinki Declaration of 1975 as revised in 1983. The study protocol was approved by the Ethical Committee of the Friedrich Schiller University Jena (reference number 5503-04/18) and registered by ClinicalTrials.gov(NCT03648112).
2.2. Study protocol
The randomized five-armed crossover study consisted of five 3-week intervention periods with 3-week wash-out periods in between (Figure 1). We used commercially available oat flakes which are also often termed “oatmeal” or “rolled oats” and comparable barley flakes and labeled these flakes as traditional flakes. The traditional flakes were compared with roasted ones and a control intervention. The sequence of testing was counterbalanced over periods using a 5 × 5 Williams-design. The sequence of intervention was always the same. However, there were five groups always beginning with a different intervention. The sequence of the interventions of each group is presented in Figure 1.
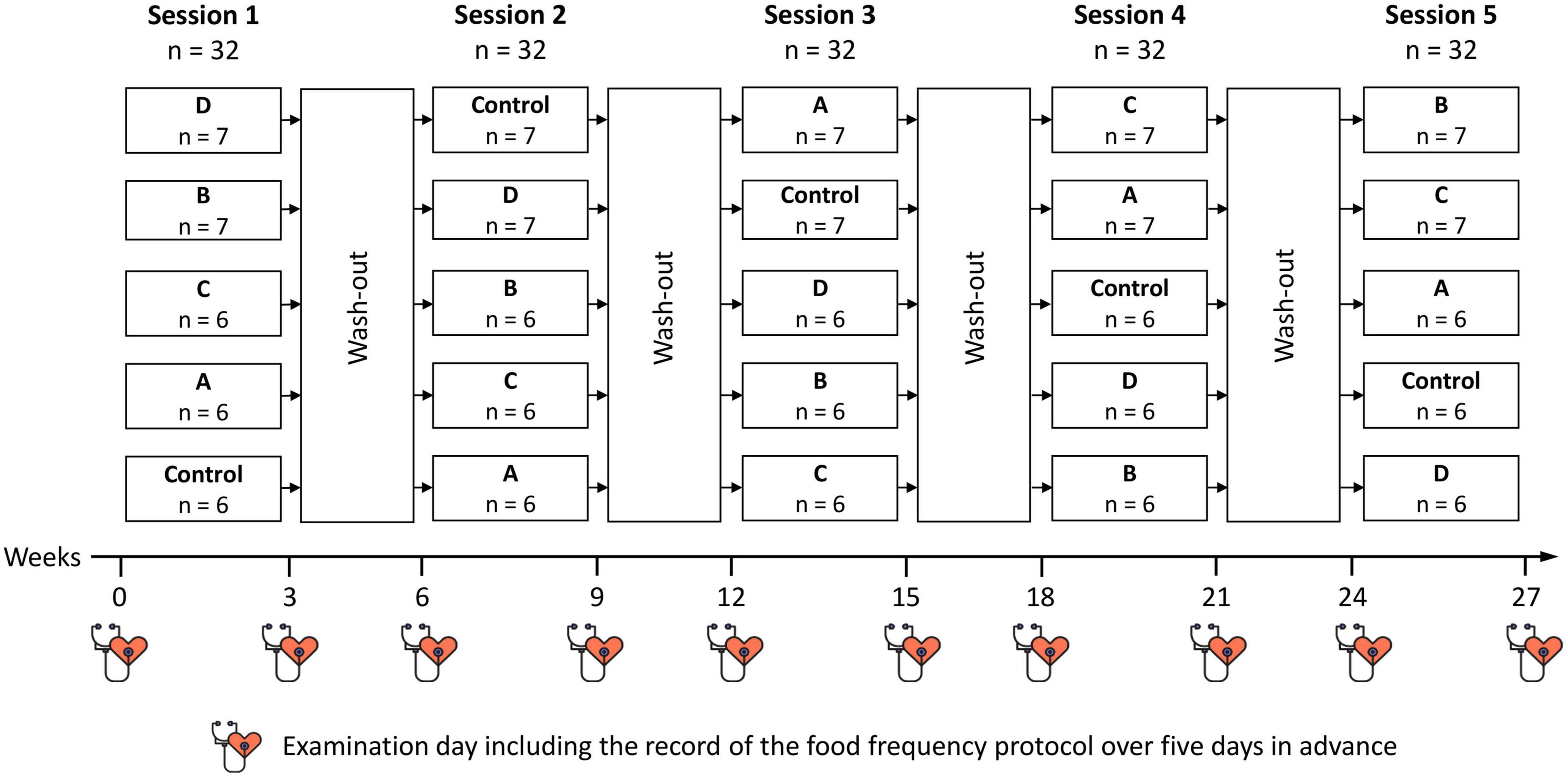
Figure 1. Design of the intervention study. A, roasted oat flakes; B, roasted barley flakes; C, traditional oat flakes; D, traditional barley flakes; Control, white toast bread.
Prior to the study, 132 subjects were screened for enrollment. A total of 32 participants met the inclusion criteria. For assigning the subjects to the first supplement to start with, a randomization list was generated with R studio (Figure 2). The allocation ratio of the study groups was 1:1:1:1:1. By applying the crossover design, all subjects ran through each intervention. The entire net duration of the study was 27 weeks (five 3-weeks interventions plus four 3-weeks wash-out phases in between). The wash out period during Christmas time was extended to 6 weeks to prevent bias by changes in dietary patterns (49).
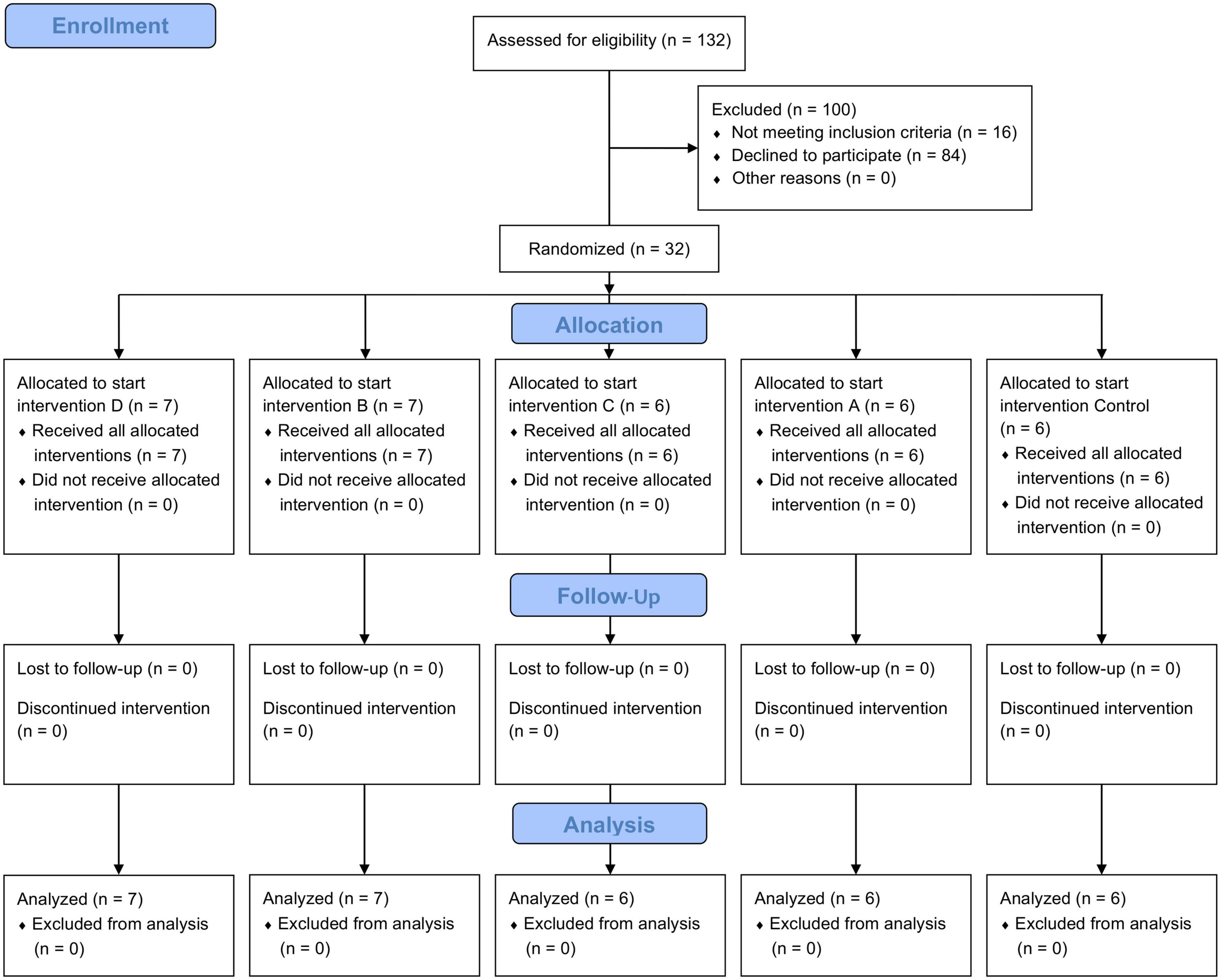
Figure 2. Flowchart diagram of the selection procedure of study participants. 132 subjects were screened for enrollment. Of them, 32 met the inclusion criteria and were randomized into five groups, each of them starting with another intervention. The other 100 subjects were excluded because they did not meet the inclusion criteria or declined to participate. All subjects completed the study and were included in the analysis.
At the beginning and at the end of each intervention period the subjects visited the study center at the University Hospital of Jena for collection of anthropometric data and taking fasting and postprandial blood samples. The subjects arrived in the morning following a 12-h overnight fasting. Height was only obtained during the first visit of the first period without shoes using a stadiometer (Seca 213, Hamburg, Germany). Body weight was measured using a digital floor scale (BC-313, Tanita Europe B.V., Amsterdam, Netherlands) with all subjects without shoes and waist circumference was determined using a measuring tape for circumference (Seca 201, Hamburg, Germany). Seated, resting blood pressure was obtained after the subject had been seating for at least 10 min and by using an automatic blood pressure device (boso-medicus uno, BOSCH & SOHN, Jungingen, Germany). During the examination, the subjects received a test meal to evaluate postprandial blood glucose and insulin levels. The test meal consisted of the respective intervention supplement and 200 ml whole milk (3.5% total fat). After obtaining the fasting blood samples, the subjects consumed the test meal within 20 min. Next blood samples were taken at 30, 60, 120, and 180 min after the fasting blood sample. During this 3-h examination, subjects were only allowed to drink water. Before the start and at the end of each study period, subjects documented their normal nutritional habits over 5 days in a food frequency protocol (FFP). The FFP originated from the Prodi® version 6.1 software (Nutri-Science, Freiburg, Germany). This protocol includes common foods with usual portion sizes as a check list. Subjects kept this tally sheet over 5 days. The daily energy and nutrient intake was then calculated by the software Prodi®.
2.3. Study diet
Subjects consumed either 80 g of roasted oat flakes (supplement A), roasted barley flakes (supplement B), traditional oat flakes (supplement C), or traditional barley flakes (supplement D) daily during the intervention periods. The fifth intervention consisted of the control supplement which was four slices of white toast bread (Lieken, Lutherstadt Wittenberg, Germany). Barley flakes were obtained from a special cultivar with low amylose but high β-glucan content (5.0/100 g) (50). Beta-glucan content of oat flakes was 4.0/100 g. Both traditional flakes varieties are commercially available and were provided by Peter Kölln, Elmshorn, Germany (oat flakes) and Dieckmann cereals, Rinteln, Germany, (beta®-barley flakes).1 These traditional flakes varieties were also used for the roasting process.
Roasting of barley and oat flakes was performed by Probat von Gimborn Maschinenfabrik (Emmerich am Rhein, Germany). Oat flakes were roasted at 150°C and barley flakes 160°C for approx. 20 min. These temperatures were chosen because of previous studies conducted by Schlörmann et al. who investigated the effects of different roasting conditions on the chemical composition and sensory quality of oat and barley products (10, 11). A good sensory quality and acrylamide levels below the benchmark levels of acrylamide in breakfast cereals as defined by the European Commission (150 μg/kg for spelt, maize, oat, barley, and rice-based products) was decisive for the roasting conditions (51).
The four slices of white toast bread provided comparable amounts of energy and total carbohydrates but only 2.8 g fiber per serving (Table 1).
All flakes were offered in neutral packages which were packed by unblinded study staff. The interventions with cereal flakes (traditional vs. roasted) were double-blinded, and the subjects and the blinded researches were not informed about the flakes they received or dispensed. During the intervention periods the subjects received recipes for breakfast for 20 days according to their energy intake (Table 2). Energy intake was determined by using the table for energy intake by the German Nutrition Society (52). The recipes included all 80 g of oat or barley flakes or four slices of white toast bread. They served as suggestions for preparations, but the participants were free to add or change ingredients as long as all flakes or slices of white toast bread were consumed. Further thermic treatment of the flakes and consumption of other β-glucan containing products was prohibited. The FFP and a query how many flakes or toast breads were left over after the intervention period served as intake control of the study products.
2.4. Blood collection and analytical methods
On examination days, subjects arrived at the Institute of Transfusion Medicine, Jena University Hospital at 8:00 h following a 12-h overnight fast. An intravenous catheter (Sarstedt, Nümbrecht, Germany) was fitted into antecubital vein (retrograde) for blood collection. The fasting and postprandial venous blood was collected and centrifuged (10 min, 4°C, 1,300 × g and 2,500 × g, respectively) for separation of plasma and serum. Study parameters were analyzed immediately after blood sampling at the Institute of Clinical Chemistry and Laboratory Diagnostics, Jena University Hospital. The laboratory analyses were performed by trained personnel to assess total cholesterol (TC), high-density lipoprotein (HDL), LDL cholesterol and triglycerides as primary outcomes as well as biomarkers for diabetes risk (fasting glucose and insulin), high-sensitive c-reactive protein (hs-CRP), and small blood count as secondary outcomes. The analyses of chemical parameters in plasma, serum or whole blood were measured by applying an Abbott Architect CI 16200 analyzer (Abbott, Wiesbaden, Germany), XN 1000 (Sysmex, Norderstedt, Germany), or Tosoh HLC-723G11 (Sysmex) according to the manufacturer’s recommendations at the Institute of Clinical Chemistry and Laboratory Diagnostics, Jena University Hospital (Supplementary Table 1). HOMA-IR was calculated according to the formula: fasting insulin (mU/L) × fasting glucose (mmol/L)/22.5.
2.5. Calculations
The postprandial responses over 3 h after a test meal were determined by using the incremental area under the curve (AUC). The AUCs were calculated using the linear trapezoidal rule and the concentration above the fasting values measured at the time points 0, 30, 60, 120, and 180 min.
2.6. Statistical analysis
The sample size calculation was based on data previously published by Behall et al. (53, 54) and determined by using a power-analysis (SAS proc power, version 9.4.). In this 5 × 5 Williams-design with four interventions and one control, a mean difference of 0.3 mmol/L was estimated between interventions and control.
For reaching a power of 80% with a significance level of 0.05, six subjects per group and time point were required (n total = 30). To consider potential dropouts of 7%, a total of 32 subjects were included.
Outlier detection for each variable was performed by descriptive statistics and histograms. For each variable, the differences between baseline values at the week before starting the intervention (week 0) and values during the last week of the intervention (week 3) were determined by Wilcoxon signed-rank test.
To prevent a possible carry-over effect, the study includes a 3-week wash-out period between the interventions. Nevertheless, for each variable, a pre-test for carry-over was performed prior to assessing treatment efficacy by using the baseline values. There was no statistically significant evidence for carry-over from the comparison of values immediately prior to each intervention period for any parameter.
Analog, the treatment effects were tested using a mixed model with random intercept. For all variables, we calculated the difference between the values from week 3 and the baseline values. Then we tested, if treatment and sequence of interventions have a significant influence. In case of significance, pairwise comparisons of differences (end values minus baseline) were done with Tukey-Kramer correction (55, 56).
Statistical analyses were performed using SAS Enterprise Guide version 9.4 (Cary, NC, USA) and the software R (version 4.1.1; The R Foundation, Indianapolis, IN, USA). All tests were considered significant at p < 0.05.
3. Results
3.1. Subjects and compliance
A total of 32 subjects (men: 10; women: 22; mean age 51.0 years) underwent randomization and started the first of the five 3-weeks interventional periods starting from May 2018, Six or seven participants set up a group and started with the same intervention (Figures 1, 2). No participant dropped out during the whole study period. Until May 2019 all 32 participants had completed all five intervention periods. Table 3 shows the characteristics of the study participants at baseline.
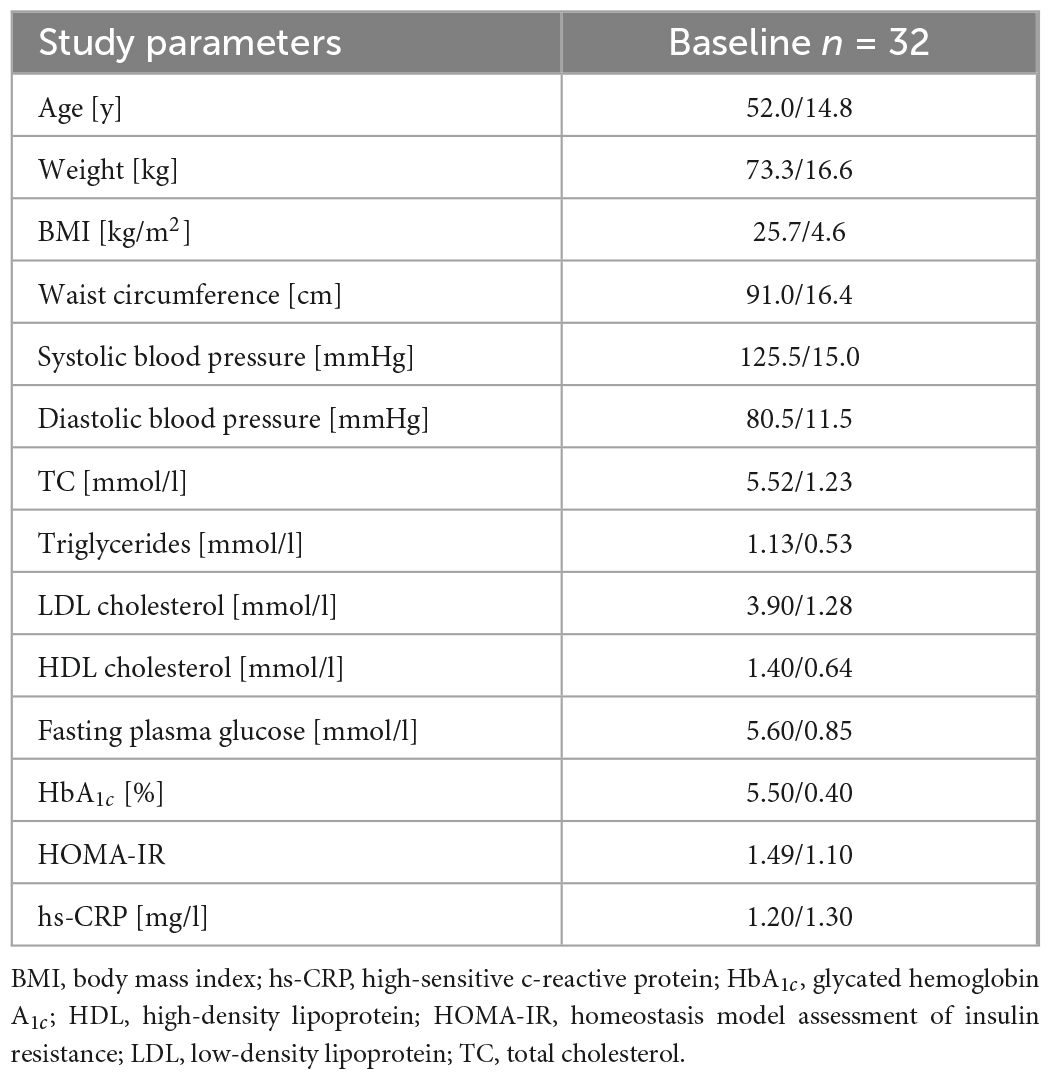
Table 3. Baseline characteristics of the study subjects expressed as median and interquartile range (IQR).
3.2. Dietary intake
The study products were completely consumed by all participants. In nine cases, participants reported on flatulence at the begin of the interventions with flakes. The FFPs showed that the participants’ usual diet provided between 1,962 and 2,108 kcal/day, 22–24 g/day dietary fiber, 75–82 g/day proteins, 207–220 g/day carbohydrates, 68–80 g/day fats, with 28–32 g/day saturated fatty acids (SFA), 23–29 g/day monounsaturated fatty acids (MUFA), and 11–14 g/day polyunsaturated fatty acids (PUFA) (Table 4). By consuming the cereal flakes, the intake of dietary fiber increased significantly between 3.63 and 6.47 g/day compared to the baseline diet (A: p < 0.001; B: p = 0.014; C: p = 0.012; D: p < 0.001) as well as the intake of carbohydrates (during the intervention with barley flakes; B: p = 0.029; D: p < 0.001), potassium (during the intervention with oat flakes; A: p = 0.009; C: p = 0.034), magnesium (A: p < 0.001; C: p = 0.002; D: p = 0.018), and vitamin B12 (during the intervention with traditional oat flakes; C: p = 0.034). The energy intake was significantly increased during the intervention with traditional barley flakes (p = 0.016) compared to the baseline diet. During the intervention with oat flakes, the intake of sodium (A: p = 0.003) and cholesterol (A: p = 0.038; C: p = 0.036) decreased compared to the baseline diet. In contrast, while consuming the control product, energy intake (p < 0.001), the intake of carbohydrates (p < 0.001), fat (p = 0.006), oleic acid (p = 0.028), SFA (p < 0.001), MUFA (p = 0.014), minerals (p = 0.038), and sodium (p = 0.032) was increased compared to the baseline diet. These differences resulted in a treatment effect for fat (p = 0.016), oleic acid (p = 0.042), SFA (p = 0.001), MUFA (p = 0.029), sodium (p < 0.001), and cholesterol (p = 0.034) (Table 4).
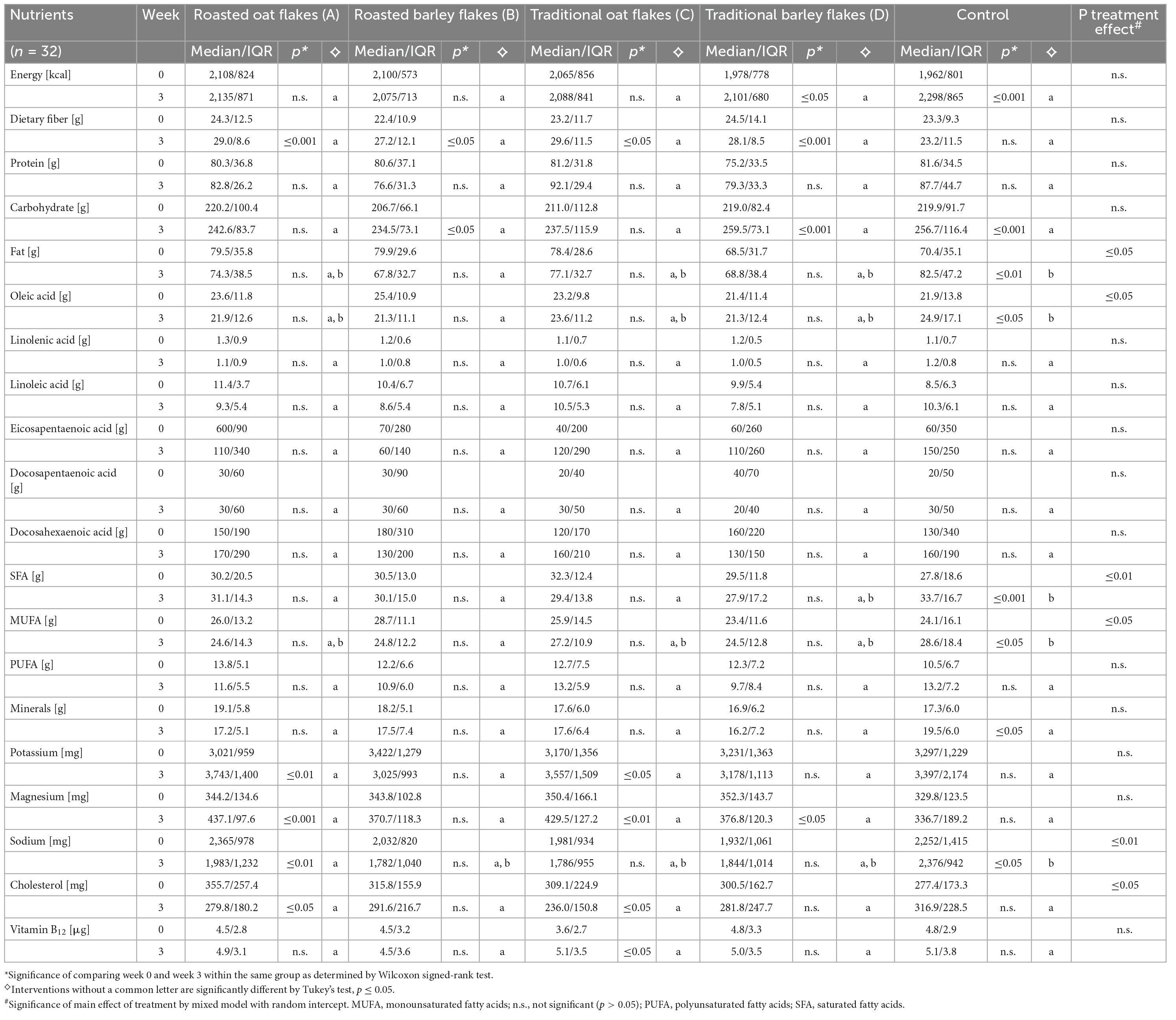
Table 4. Consumption of nutrients according to FFP (5 days) in the week before starting the intervention (week 0) and during the last week of the intervention (week 3) expressed as median and IQR (n = 32).
3.3. Anthropometric parameters
After the intervention with roasted barley flakes (B), body weight (p = 0.028) and body mass index (BMI) were reduced compared to baseline (p = 0.035; Table 5). Moreover, the systolic and diastolic blood pressure decreased also by 4.6% (p = 0.041) and 6.7% (p = 0.035), respectively. The further treatments did not have any effects on the anthropometric parameters, and there was no significant effect between the periods (Table 5).
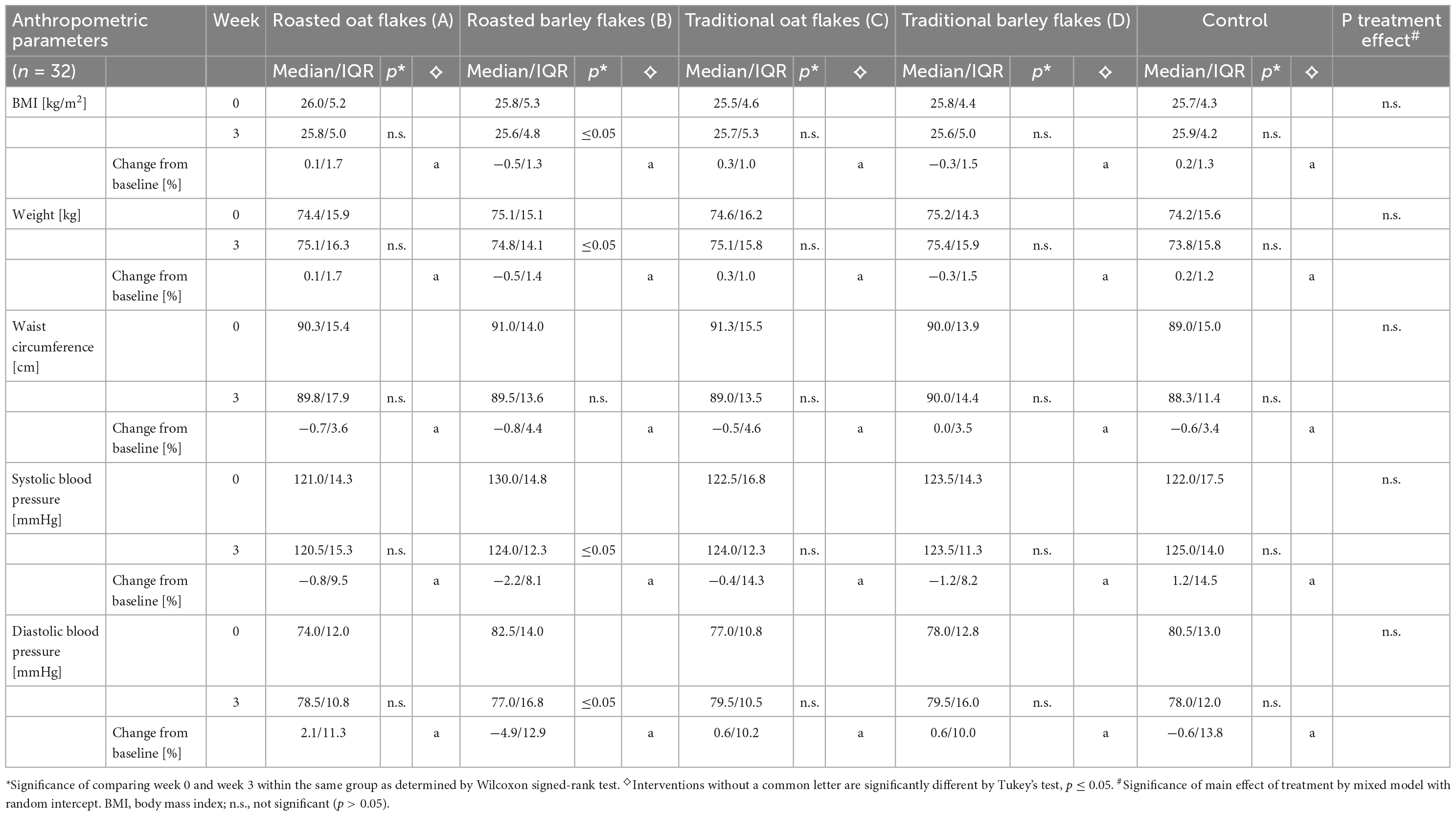
Table 5. Anthropometric parameters at baseline (week 0) and at the end of the intervention periods (week 3) expressed as median and IQR (n = 32).
3.4. Fasting biochemical parameters
During all interventions with oat and barley flakes, TC and LDL cholesterol were reduced by in average 4.8% for TC (A: p = 0.004; B: p = 0.015; C: p = 0.006; D: p = 0.006) and 6.5% for LDL cholesterol (A: p = 0.002; B: p = 0.009; C: p = 0.012; D: p = 0.004), respectively, compared to baseline (Table 6). In contrast, TC increased by 3.9% (p = 0.012) in the control period. The regular consumption of traditional and roasted barley flakes was associated with a reduction of HDL cholesterol by 4.8% (p = 0.049) and 5.4% (p = 0.001), respectively (Table 6). After the control intervention, HDL cholesterol increased by 3.7% (p = 0.012). This resulted in a treatment effect for HDL cholesterol (p < 0.001). Moreover, there was a treatment effect for TC (p < 0.001) and LDL cholesterol (p = 0.004) as these parameters decreased significantly due to consumption of oat and barley flakes (Table 6). Triglycerides were not affected by any intervention.
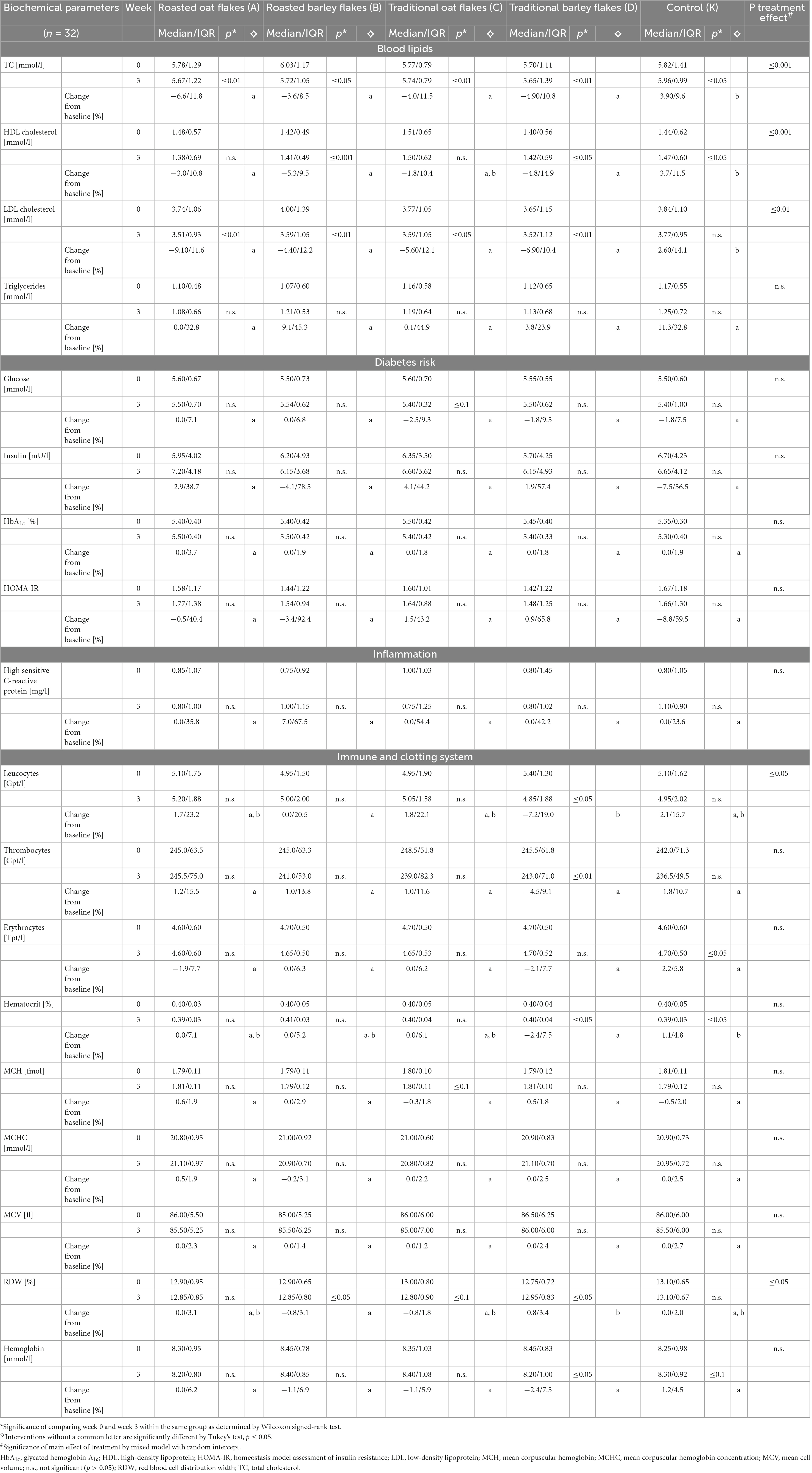
Table 6. Fasting biochemical parameters at baseline (week 0) and at the end of the intervention periods (week 3) expressed as median and IQR (n = 32).
Except a trend for a reduction of fasting glucose after the intervention with traditional oat flakes (p = 0.083), the analyzed diabetes risk markers and hs-CRP were not influenced by any intervention (Table 6).
For blood count, there were differences between the interventions with roasted and traditional barley flakes observed for leukocytes and red blood cell distribution width (RDW) (p = 0.028 and p = 0.019, respectively). Other parameters [leukocytes (p = 0.046), thrombocytes (p = 0.008), hematocrit (p = 0.022), and hemoglobin (p = 0.025)] decreased in response to the regular consumption of traditional barley flakes whereas the concentration of erythrocytes (p = 0.041) and hematocrit (p = 0.026) increased in the control period (Table 6).
3.5. Postprandial kinetics
Insufficient volume of blood was collected for the determination of glucose in two cases and for the determination of other parameters (TC, triglycerides, LDL and HDL cholesterol) in one case, respectively. This resulted in different group sizes. During the intervention with traditional oat flakes, the AUC of glucose was significantly reduced [median/IQR: 1,045.5/205.9 to 1,005.0/156.4 (mmol/L) × min; p = 0.018, Table 7]. The AUC of insulin and triglycerides was not affected by any intervention (Table 7). The AUC of TC and LDL cholesterol was significantly reduced during all interventions with cereal flakes between 3.4–6.7% (TC; A: p = 0.006; B: p < 0.001; C: p = 0.005; D: p = 0.007) and 3.4–9.6% (LDL; A: p = 0.001; B: p = 0.021; C: p = 0.006; D: p = 0.005) (Table 7), resulting in a significant treatment effect (p < 0.001 and p = 0.019, Table 7). The AUC of HDL cholesterol significantly decreased during the intervention with roasted barley flakes by 12.75/35.85 (mmol/L) × min (p < 0.001). In contrast, the AUC of HDL cholesterol significantly increased during the intervention with toast bread by 10.50/33.04 (mmol/L) × min (p = 0.032). For both interventions the intervention effect was significant (p < 0.001, Table 7).
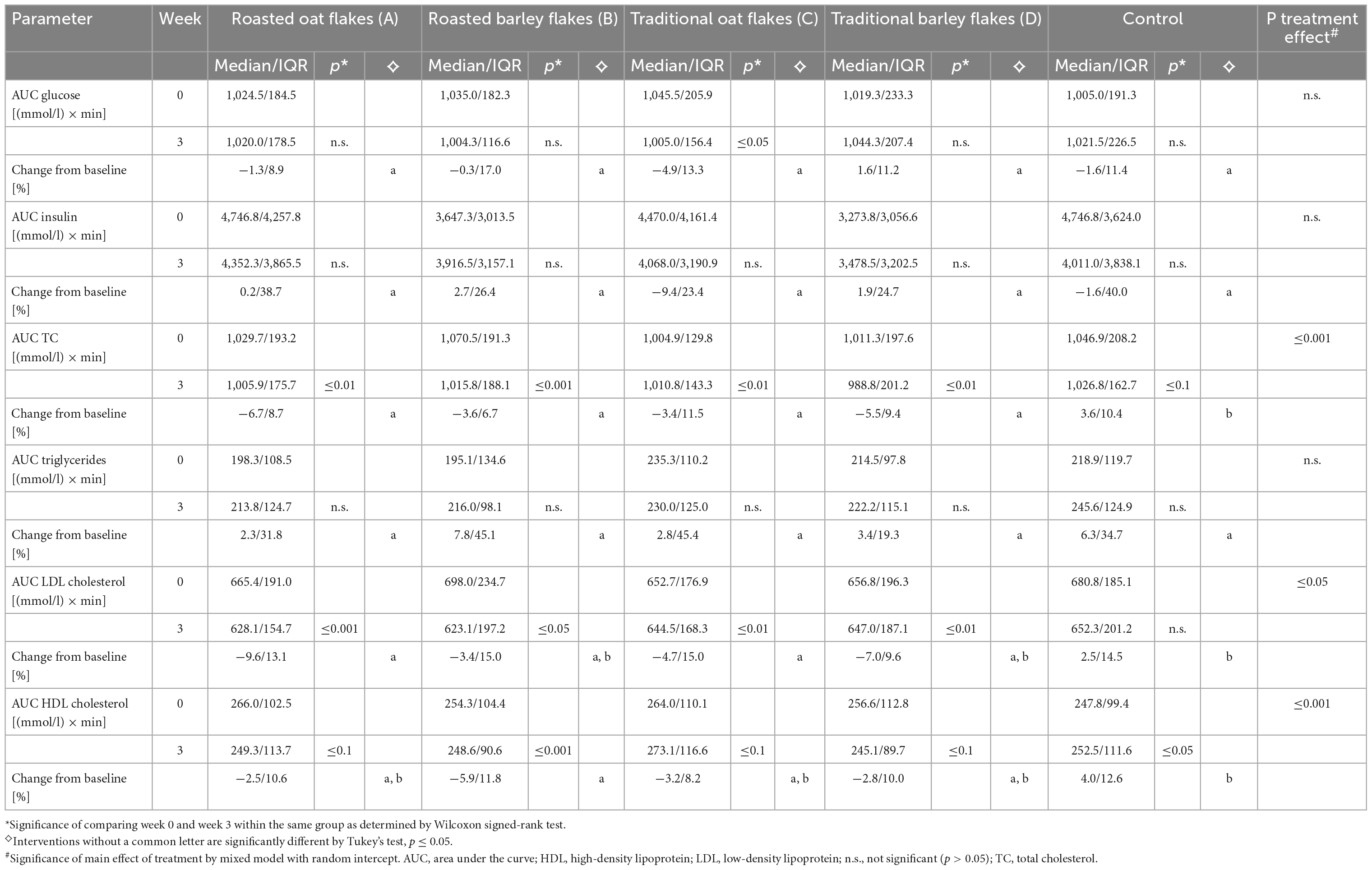
Table 7. Area under the curve (AUCs) at baseline (week 0) and at the end of the intervention periods (week 3) expressed as median and IQR (n = 31).
4. Discussion
In a sample of mildly hypercholesterolemic subjects, the regular consumption of 80 g traditional or roasted oat or barley flakes for breakfast per day resulted in a significant reduction of TC and LDL cholesterol. The interventions mostly failed to detect any significant changes in the analyzed biomarkers for diabetes and hs-CRP. The data from the postprandial kinetics reflect the results after a regular consumption of the investigated cereal flakes over 3 weeks (Tables 6, 7). In all interventions with cereal flakes, fasting glucose showed a decreasing trend, whereby the regular consumption of traditional oat flakes seemed to induce a slightly stronger effect than the other cereal flakes.
Similar results on TC, LDL cholesterol and on glucose metabolism were also obtained during the BELT study which investigated the effect of 3 g/day oat β-glucan on lipid profile, glycemia and intestinal health in 83 Italian with moderate hypercholesterolemia (LDL cholesterol: ≥3.36 mmol/L) (57). In the 8-week study period the participants received a proprietary formulation of β-glucans. LDL cholesterol concentrations were reduced by 12.2% from baseline after 4 weeks of supplementation and by 15.1% after 8 weeks of supplementation. TC and non-HDL cholesterol were also significantly reduced during both follow-up visits. With 4–9%, the relative LDL cholesterol reduction was smaller in the present study. This fact might be attributed by the shorter intervention period and the higher variability of LDL cholesterol concentrations at baseline. In addition, participants with higher LDL cholesterol concentrations at baseline were enrolled in the BELT study (≥3.36 and ≤4.91 mmol/L). However, the mean reduction of TC and LDL cholesterol by 0.29 and 0.24 mmol/L respectively, is exact in the range of further studies which investigated the cholesterol-lowering effects of ≥3 g oat β-glucan (42, 58, 59).
The effect of oat and barley β-glucan on HDL cholesterol is not conclusive as the results show trends for a reduction that are only significant for the intervention with barley flakes (Tables 6, 7). This is also in line with the meta-analysis of Whitehead et al. (57) and study of Xu (42, 60) who showed no effect on HDL cholesterol or a significant heterogeneity among studies (57).
Our findings on fasting plasma glucose are also in accordance with the results from the BELT study. Like in our study, euglycemic participants were enrolled in the BELT study (57). Meta-analyses already showed that the intake of oat β-glucan is more effective in subjects with DMT2 (61, 62). Nevertheless, we observed a slight reduction for the AUC of glucose after the regular consumption of traditional oat flakes (p = 0.018; Table 7). Stronger effects were presented by Wolever et al. (63), as a significant dose-response effect of low amounts of oat β-glucan on glycemic response was also shown. Thereby, each gram of oat β-glucan reduced AUC by 7%. Nevertheless, for a significant reduction in the AUC of glucose, 2.77 g β-glucan per 16.2 g available carbohydrates were necessary which is in accordance with the EFSA health claim (34).
The study interventions with oat and barley flakes had no effects on triglycerides. This is in line with findings of several other studies, as was shown in a meta-analysis of randomized controlled trials by Whitehead et al. (58). The authors reported that adding ≥3 g/day oat β-glucan to the diet reduces LDL cholesterol and TC without changing triglycerides. However, there are hints that β-glucan might reduce triglycerides in healthy people with a normal lipid profile, but also in overweight people or those with diabetes or metabolic syndrome. In a recent systematic review six out of 17 included studies reported significant improvements in triglyceride concentrations (64). However, often higher doses, longer study duration, and the combination with long-chain omega-3 fatty acids or calorie reduction resulted in these stronger effects (64).
Different physicochemical properties of β-glucan can also result in varying study results in human trails (16, 65). Water-solubility of dietary fiber is already known to positively affect serum cholesterol as highly water-soluble β-glucan is more effective in reducing serum cholesterol than poorly water-soluble β-glucan (16, 65). Moreover, especially soluble fiber have a functional relationship with the gut microbiota in the large intestine (66) by exerting a prebiotic effect on the gut microbiota which in turn can regulate cholesterol homeostasis in the host (67, 68). β-Glucan solubility depends on its molecular fine structure, which is different in oat and barley because of different ratios of cellotriosyl to cellotetraosyl units (69). Several studies already demonstrated that barley β-glucans show lower solubility than oat β-glucans (69–71). In the present study, these effects can be evidenced by the mean differences before and after the interventions in the LDL cholesterol AUCs. These were slightly greater (but not significantly) in oat flakes [roasted: −55 (mmol/L) × min; traditional: −61 (mmol/L) × min] than in barley flakes [roasted: −38 (mmol/L) × min, traditional: −35 (mmol/L) × min]. The fact that these differences were not reflected in the fasting concentrations is indicative for a faster postprandial LDL cholesterol clearance after a time of adaptation to an oat-rich diet which is necessary when microbiota need to adapt (72). However, these mechanisms need to be more evaluated and further studies with especially barley β-glucan are warranted.
A further important determinant of the physicochemical characteristics of β-glucan is its molecular weight. The molecular weight of β-glucan affects its water-solubility and viscosity which are responsible for its hypocholesterolemic and hypoglycemic properties (73–75). The molecular weight of barley β-glucan is around 0.15–2.5 × 106 g/mol and of oat β-glucan around 0.065–3.0 × 106 g/mol (76), and can vary due to the diversity in its source, extraction protocol, and methodology employed for its determination (75). In the present study, roasted oat flakes seemed to show a higher AUC of insulin compared to traditional and roasted barley flakes (Table 7). A significant reduction of insulin AUCs only by barley (p < 0.005), and not by oat, was also found in a study by Behall et al. (24). They used barley of a special cultivar (Prowashonupana cultivar) with a very high β-glucan content (15 g/100 g) so that the β-glucan content of the barley products was no longer comparable to the oat products (3.23 g β-glucan for oat test meal vs. 12.1 g β-glucan for barley test meal). The higher concentration and a different molecular weight of β-glucan could be responsible for the greater reduction in insulin AUC (61, 70). In contrast, Hallfrisch et al. (17) found no differences between barley and oat on glucose and insulin responses on 22 non-diabetic men and women who consumed 1 g/kg body weight of carbohydrate as glucose or 0.33 g/kg of body weight as oat bran, barley flour, oat or barley extract in a Latin square design after consuming controlled diets for 3 days. AUCs of glucose and insulin responses to oats, barley, and both extracts, were significantly lower than responses to the glucose solution (17). However, the sources of β-glucan were no flakes, what can be again indicative for different physicochemical properties.
Ultimately, the already discussed characteristics, water-solubility, and molecular weight, affect the viscosity. There is evidence, that an increasing solubility and molecular weight of β-glucan is accompanied with higher viscosity. An increased viscosity in the gastrointestinal tract delays the gastric emptying as well as the starch digestion, that in turn, led to reduced postprandial hyperglycemia. The particular microenvironment with high viscosity in the small intestine acts as a physical barrier and prevents the absorption and reabsorption of cholesterol and bile acids, so that more bile acids are excreted which promotes the de novo synthesis of bile acids from cholesterol leading to reduced serum cholesterol concentrations (77, 78). In a study, which measured bile acid excretion within 24 h of β-glucan consumption, it revealed that this is increased. Entrapment of whole micelles in the gut owing to higher viscosity is held to be responsible for this effect (79). Therefore, the viscosity is an important determinant of the postprandial glucose regulation and cholesterol lowering effect of β-glucan (37, 38, 42, 65, 67). However, viscosity is further influenced by the concentration of β-glucan and the surrounding food matrix, and these factors can be altered again by food processing and storage (39, 42, 80, 81). The flakes used in this study were already examined on their viscosity by Schlörmann et al. (10, 11). For both flakes the viscosity significantly decreased with roasting. However, regarding postprandial glucose regulation or cholesterol levels, we observed no differences between the roasted and the traditional flakes. As discussed by Schlörmann et al. (11) the reduced viscosities from 255 to 98 mPas may not interfere with these physiological effects. This fact agrees with the studies by Wood et al. (32, 82) and a review by Lazaridou and Biliaderis (74), whereby a huge range of viscosities from 20 to 5,000 mPas is able to improve postprandial glucose and insulin responses. Thus, the gentle treatment of roasting used for our flakes did not have an impact on the physiological effects but improved the sensory quality (10, 11).
Next to the different properties of β-glucans the underlying mechanisms reflected on serum metabolomics linking β-glucan intake and the reduction of TC and LDL cholesterol are under investigation. Therefore, Xu et a. also conducted a study involving healthy adults with mild hypercholesterolemia consuming 80 g oats daily and implemented an untargeted serum metabolomics. They could reveal underlying metabolic pathways such as glycerophospholipids, alanine, aspartate and glutamate, sphingolipid, and retinol metabolism which regulate those beneficial effects on risk factors for CVD (83).
In the present study white toast bread was used as control intervention, because this typical component of German breakfast is low in dietary fiber. As limitation for comparability the side dishes of toast like butter, sausages, or jam often contain more energy, fat, and carbohydrates than the side dishes for cereal flakes like fruits and yogurt. For standardization, subjects also received recipes for the breakfast with toast. The energy content was similar between the flakes and control recipes, but the content of carbohydrates and dietary fiber was lower in the control recipes (Table 2). Subjects did not have to cook exactly following the recipes as long they ate the 80 g of flakes or the four slices of white toast bread. In contrast to the nutritional value of the recipes, results from the FFP showed, that subjects significantly consumed more energy, carbohydrates, fat, oleic acid, SFA, MUFA, sodium, and minerals during the control intervention (Table 4). This may have contributed also to significantly increased TC and LDL cholesterol concentrations (Table 4) (84, 85). The increased intake of energy, carbohydrates, and fats during the control period might also result from a lower satiety because of the regular consumption of the toast bread (86).
The present study was conducted to study the effect of a regular consumption of traditional and roasted oat and barley flakes on blood lipids and glucose metabolism. The data show a small unfavorable effect of roasting on glucose metabolism as the significant decrease of AUC glucose after consumption of traditional oat flakes was not observed after test meal with roasted oat flakes. On the other side, advantages were detected for regularly intake of roasted barley flakes which resulted in significant decreases of body weight, BMI, as well as systolic and diastolic blood pressure. These reductions on cardiovascular risk factors were not identified after consumption of traditional barley flakes. We assume that the increased sensory quality by roasting improved compliance with the study intervention which results in these valuable effects. In this context, it must also be mentioned that the daily consumption of roasted barley flakes resulted in a significant decrease of AUC HDL cholesterol. This was not observed for traditional barley flakes.
To our knowledge, this is the first study, which directly compared the physiological effects of a regular consumption of traditional and roasted barley and oat flakes. Although the consumption of all cereal flakes reduced TC and LDL cholesterol concentrations significantly within 3 weeks after a daily consumption, TC and LDL cholesterol returned to basal values after 3 weeks of wash-out. This highlights the importance of a regular consumption of β-glucan-rich cereal flakes to achieve clinically relevant reductions of cardiovascular risk factors in the long-term. The improved sensory quality after roasting represents a promising alternative to increase consumption and long-term compliance (10, 11). Nevertheless, oat and barley flakes have a high susceptibility to oxidation, which may result in off-flavors. Roasted variants of flakes showed the same positive physiological effects as the traditional forms, but the improved sensory quality had to be ensured by an optimal storage at low moisture, low temperature, and low oxygen concentrations (10, 87). For the future, more studies are needed which specifically investigate the effects of molecular weight, viscosity, water-solubility, and the surrounding food matrix of roasted β-glucan-rich cereal flakes on glucose and lipid metabolism.
5. Conclusion
This study confirmed that the regular consumption of 80 g traditional and roasted barley and oat flakes daily for breakfast for 3 weeks effectively reduces fasting and postprandial TC and LDL cholesterol concentrations. The reducing effect on postprandial glucose response after consumption of roasted oat flakes was mitigated compared to traditional oat flakes. Roasting did not reduce the physiological effects on blood lipids. This indicates that processing like roasting with dry heat does not affect the health impact of β-glucan-rich roasted cereal flakes on lipid metabolism of moderately increased LDL cholesterol healthy subjects.
Data availability statement
The raw data supporting the conclusions of this article will be made available by the authors, without undue reservation.
Ethics statement
The study involving human participants was reviewed and approved by Ethical Commission of the Friedrich Schiller University, Jena. The patients/participants provided their written informed consent to participate in this study.
Author contributions
MG, WS, SL, and CD: conceptualization, methodology, funding acquisition, and supervision. SRe, SH, and CD: validation and data curation. LW and SRe: formal analysis and statistical analysis. SRe, SH, MK, AK, SRu, and CD: investigation. SL, MK, and CD: resources. SRe: writing—original draft preparation. MG, SL, WS, and CD: writing—review and editing. SRe: visualization. MG, WS, and CD: project administration. All authors have read and agreed to the published version of the manuscript.
Funding
This IGF project of the FEI was supported via AiF within the program for promoting the Industrial Collective Research (IGF) of the German Ministry of Economic Affairs and Climate Action (BMWi), based on a resolution of the German Parliament. We acknowledge the support by the German Research Foundation in this IGF Project (19351 BR). We also acknowledge support by the German Research Foundation Project-Nr. 512648189 and the Open Access Publication Fund of the Thueringer Universitaets-und Landesbibliothek Jena.
Acknowledgments
We would like to thank Dieckmann Cereals GmbH (Rinteln, Germany) and Peter Kölln GmbH & Co. KGaA (Elmshorn, Germany) for the allocation of the traditional thin barley and oat flakes. We also thank Probat-Werke von Gimborn Maschinenfabrik GmbH (Emmerich am Rhein, Germany) for roasting the oat and barley flakes, especially Thomas Koziorowski and Thomas Elshoff. We thank all participants of this study for participating on the trial. Many thanks go to the staff of the Institute of Transfusion Medicine, Jena University Hospital, for taking the blood samples.
Conflict of interest
The authors declare that the research was conducted in the absence of any commercial or financial relationships that could be construed as a potential conflict of interest.
Publisher’s note
All claims expressed in this article are solely those of the authors and do not necessarily represent those of their affiliated organizations, or those of the publisher, the editors and the reviewers. Any product that may be evaluated in this article, or claim that may be made by its manufacturer, is not guaranteed or endorsed by the publisher.
Supplementary material
The Supplementary Material for this article can be found online at: https://www.frontiersin.org/articles/10.3389/fnut.2023.1095245/full#supplementary-material
Footnotes
References
1. WHO. World Health Statistics 2020: Monitoring Health for the Sdgs, Sustainable Development Goals. Geneva: World Health Organization (2020).
2. Reynolds A, Mann J, Cummings J, Winter N, Mete E, Te Morenga L. Carbohydrate quality and human health: a series of systematic reviews and meta-analyses. Lancet. (2019) 393:434–45. doi: 10.1016/s0140-6736(18)31809-9
3. Reynolds A, Akerman A, Mann J. Dietary fibre and whole grains in diabetes management: systematic review and meta-analyses. PLoS Med. (2020) 17:e1003053. doi: 10.1371/journal.pmed.1003053
4. Anderson J, Baird P, Davis R Jr, Ferreri S, Knudtson M, Koraym A, et al. Health benefits of dietary fiber. Nutr Rev. (2009) 67:188–205. doi: 10.1111/j.1753-4887.2009.00189.x
5. Tosh S. Review of human studies investigating the post-prandial blood-glucose lowering ability of oat and barley food products. Eur J Clin Nutr. (2013) 67:310–7. doi: 10.1038/ejcn.2013.25
6. AbuMweis S, Jew S, Ames N. Beta-glucan from barley and its lipid-lowering capacity: a meta-analysis of randomized, controlled trials. Eur J Clin Nutr. (2010) 64:1472–80. doi: 10.1038/ejcn.2010.178
7. Charalampopoulos D, Wang R, Pandiella S, Webb C. Application of cereals and cereal components in functional foods: a review. Int J Food Microbiol. (2002) 79:131–41. doi: 10.1016/S0168-1605(02)00187-3
8. Wood P. Oat B -glucan-physicochemical properties and physiological effects. Trends Food Sci Technol. (1991) 2:311–4. doi: 10.1016/0924-2244(91)90733-Y
9. Cui W, Wood P, Blackwell B, Nikiforuk J. Physicochemical properties and structural characterization by two-dimensional Nmr spectroscopy of wheat B -D-glucan—comparison with other cereal B -D-glucans. Carbohydr Polym. (2000) 41:249–58. doi: 10.1016/S0144-8617(99)00143-5
10. Schlörmann W, Zetzmann S, Wiege B, Haase N, Greiling A, Lorkowski S, et al. Impact of different roasting conditions on sensory properties and health-related compounds of oat products. Food Chem. (2020) 307:125548. doi: 10.1016/j.foodchem.2019.125548
11. Schlörmann W, Zetzmann S, Wiege B, Haase N, Greiling A, Lorkowski S, et al. Impact of different roasting conditions on chemical composition, sensory quality and physicochemical properties of waxy-barley products. Food Funct. (2019) 10:5436–45. doi: 10.1039/c9fo01429b
12. Wolever T, Johnson J, Jenkins A, Campbell J, Ezatagha A, Chu Y. Impact of oat processing on glycaemic and insulinaemic responses in healthy humans: a randomised clinical trial. Br J Nutr. (2019) 121:1264–70. doi: 10.1017/S0007114519000370
13. Pick M, Hawrysh Z, Gee M, Toth E. Barley bread products improve glycemic control of type 2 subjects. Int J Food Sci Nutr. (1998) 49:71–8. doi: 10.3109/09637489809086406
14. Urooj A, Vinutha S, Puttaraj S, Leelavathy K, Rao P. Effect of barley incorporation in bread on its quality and glycemic responses in diabetics. Int J Food Sci Nutr. (1998) 49:265–70. doi: 10.3109/09637489809089397
15. Tapola N, Karvonen H, Niskanen L, Mikola M, Sarkkinen E. Glycemic responses of oat bran products in type 2 diabetic patients. Nutr Metab Cardiovasc Dis. (2005) 15:255–61. doi: 10.1016/j.numecd.2004.09.003
16. Biörklund M, van Rees A, Mensink R, Onning G. Changes in serum lipids and postprandial glucose and insulin concentrations after consumption of beverages with beta-glucans from oats or barley: a randomised dose-controlled trial. Eur J Clin Nutr. (2005) 59:1272–81. doi: 10.1038/sj.ejcn.1602240
17. Hallfrisch J, Scholfield D, Behall K. Physiological responses of men and women to barley and oat extracts (Nu-Trimx). Ii. Comparison of glucose and insulin responses. Cereal Chem. (2003) 80:80–3. doi: 10.1094/CCHEM.2003.80.1.80
18. Hallfrisch J, Scholfield D, Behall K. Diets containing soluble oat extracts improve glucose and insulin responses of moderately hypercholesterolemic men and women. Am J Clin Nutr. (1995) 61:379–84. doi: 10.1093/ajcn/61.2.379
19. Kabir M, Oppert J, Vidal H, Bruzzo F, Fiquet C, Wursch P, et al. Four-week low-glycemic index breakfast with a modest amount of soluble fibers in type 2 diabetic men. Metab Clin Exp. (2002) 51:819–26. doi: 10.1053/meta.2002.33345
20. Li J, Kaneko T, Qin L, Wang J, Wang Y. Effects of barley intake on glucose tolerance, lipid metabolism, and bowel function in women. Nutrition. (2003) 19:926–9. doi: 10.1016/S0899-9007(03)00182-5
21. Pick M, Hawrysh Z, Gee M, Toth E, Garg M, Hardin R. Oat bran concentrate bread products improve long-term control of diabetes: a pilot study. J Am Diet Assoc. (1996) 96:1254–61. doi: 10.1016/s0002-8223(96)00329-x
22. EFSA. Consolidated List of Article 13 Health Claims List of References Received by Efsa Part 2 Ids 1001-2000. (2011). Available online at: https://www.efsa.europa.eu/sites/default/files/topic/ndaart13ref02.pdf (accessed August 16, 2022).
23. Battilana P, Ornstein K, Minehira K, Schwarz J, Acheson K, Schneiter P, et al. Mechanisms of action of beta-glucan in postprandial glucose metabolism in healthy men. Eur J Clin Nutr. (2001) 55:327–33. doi: 10.1038/sj.ejcn.1601160
24. Behall K, Scholfield D, Hallfrisch J. Comparison of hormone and glucose responses of overweight women to barley and oats. J Am Coll Nutr. (2005) 24:182–8. doi: 10.1080/07315724.2005.10719464
25. Braaten J, Scott F, Wood P, Riedel K, Wolynetz M, Brule D, et al. High beta-glucan oat bran and oat gum reduce postprandial blood glucose and insulin in subjects with and without type 2 diabetes. Diabet Med. (1994) 11:312–8. doi: 10.1111/j.1464-5491.1994.tb00277.x
26. Granfeldt Y, Liljeberg H, Drews A, Newman R, Bjorck I. Glucose and insulin responses to barley products: influence of food structure and amylose-amylopectin ratio. Am J Clin Nutr. (1994) 59:1075–82. doi: 10.1093/ajcn/59.5.1075
27. Holm J, Koellreutter B, Wursch P. Influence of sterilization, drying and oat bran enrichment of pasta on glucose and insulin responses in healthy subjects and on the rate and extent of in vitro starch digestion. Eur J Clin Nutr. (1992) 46:629–40.
28. Liljeberg H, Granfeldt Y, Bjorck I. Metabolic responses to starch in bread containing intact kernels versus milled flour. Eur J Clin Nutr. (1992) 46:561–75.
29. Liljeberg H, Granfeldt Y, Bjorck I. Products based on a high fiber barley genotype, but not on common barley or oats, lower postprandial glucose and insulin responses in healthy humans. J Nutr. (1996) 126:458–66. doi: 10.1093/jn/126.2.458
30. Östman E, Rossi E, Larsson H, Brighenti F, Björck I. Glucose and insulin responses in healthy men to barley bread with different levels of (1→3;1→4)-B -glucans; predictions using fluidity measurements of in vitro enzyme digests. J Cereal Sci. (2006) 43:230–5. doi: 10.1016/j.jcs.2005.11.001
31. Ostman E, Liljeberg Elmstahl H, Bjorck I. Barley bread containing lactic acid improves glucose tolerance at a subsequent meal in healthy men and women. J Nutr. (2002) 132:1173–5. doi: 10.1093/jn/132.6.1173
32. Wood P, Beer M, Butler G. Evaluation of role of concentration and molecular weight of oat beta-glucan in determining effect of viscosity on plasma glucose and insulin following an oral glucose load. Br J Nutr. (2000) 84:19–23.
33. Yokoyama W. Effect of barley beta-glucan in durum wheat pasta on human glycemic response. Cereal Chem. (1997) 74:293–6. doi: 10.1094/CCHEM.1997.74.3.293
34. EFSA. Scientific opinion on the substantiation of health claims related to beta-glucans from oats and barley and maintenance of normal blood Ldl-cholesterol concentrations (Id 1236, 1299), increase in satiety leading to a reduction in energy intake (Id 851, 852), reduction of post-prandial glycaemic responses (Id 821, 824), and “digestive function” (Id 850) pursuant to article 13 of regulation (Ec) No 1924/2006. EFSA J. (2011) 9:2207. doi: 10.2903/j.efsa.2011.2207
35. Ripsin C, Keenan J, Jacobs D Jr., Elmer P, Welch R, Van Horn L, et al. Oat products and lipid lowering. A meta-analysis. JAMA. (1992) 267:3317–25.
36. Marasca E, Boulos S, Nystrom L. Bile acid-retention by native and modified oat and barley beta-glucan. Carbohydr Polym. (2020) 236:116034. doi: 10.1016/j.carbpol.2020.116034
37. Regand A, Tosh S, Wolever T, Wood P. Physicochemical properties of beta-glucan in differently processed oat foods influence glycemic response. J Agric Food Chem. (2009) 57:8831–8. doi: 10.1021/jf901271v
38. Regand A, Chowdhury Z, Tosh S, Wolever T, Wood P. The molecular weight, solubility and viscosity of oat beta-glucan affect human glycemic response by modifying starch digestibility. Food Chem. (2011) 129:297–304. doi: 10.1016/j.foodchem.2011.04.053
39. Wang Q, Ellis P. Oat beta-glucan: physico-chemical characteristics in relation to its blood-glucose and cholesterol-lowering properties. Br J Nutr. (2014) 112(Suppl. 2):S4–13. doi: 10.1017/s0007114514002256
40. Kim H, White P. Impact of the molecular weight, viscosity, and solubility of beta-glucan on in vitro oat starch digestibility. J Agric Food Chem. (2013) 61:3270–7. doi: 10.1021/jf305348j
41. Tosh S, Brummer Y, Miller S, Regand A, Defelice C, Duss R, et al. Processing affects the physicochemical properties of beta-glucan in oat bran cereal. J Agric Food Chem. (2010) 58:7723–30. doi: 10.1021/jf904553u
42. Wolever T, Tosh S, Gibbs A, Brand-Miller J, Duncan A, Hart V, et al. Physicochemical properties of oat beta-glucan influence its ability to reduce serum Ldl cholesterol in humans: a randomized clinical trial. Am J Clin Nutr. (2010) 92:723–32. doi: 10.3945/ajcn.2010.29174
43. Gangopadhyay N, Hossain M, Rai D, Brunton NPA. Review of extraction and analysis of bioactives in oat and barley and scope for use of novel food processing technologies. Molecules. (2015) 20:10884–909. doi: 10.3390/molecules200610884
44. Eurostat. Agricultural Production – Crops. (2021). Available online at: https://ec.europa.eu/eurostat/statistics-explained/index.php?title=Agricultural_production_-_crops (accessed August 16, 2022).
45. United States Department of Agriculture. Grain: World Markets and Trade. (2021). Available online at: https://apps.fas.usda.gov/psdonline/circulars/grain.pdf (accessed August 16, 2022).
46. Bundesministerum für Ernährung und Landwirtschaft. Versorgungsbilanzen Zu Getreide: BMEL. (2021). Available online at: https://www.bmel-statistik.de/ernaehrung-fischerei/versorgungsbilanzen/getreide/ (accessed August 16, 2022).
47. Alamprese C, Ratti S, Rossi M. Effects of roasting conditions on hazelnut characteristics in a two-step process. J Food Eng. (2009) 95:272–9. doi: 10.1016/j.jfoodeng.2009.05.001
48. Ranganathan V, Nunjundiah I, Bhattacharya S. Effect of roasting on rheological and functional properties of sorghum flour. Food Sci Technol Int. (2014) 20:579–89. doi: 10.1177/1082013213497210
49. Vedel-Krogh S, Kobylecki C, Nordestgaard B, Langsted A. The Christmas holidays are immediately followed by a period of hypercholesterolemia. Atherosclerosis. (2019) 281:121–7. doi: 10.1016/j.atherosclerosis.2018.12.011
50. Dieckmann Cereals GmbH. Beta-Glucan-Gerste Mit Wertvollen Nährstoffen. (2022). Available online at: https://www.gerstoni.de/naehrwert-tabelle (accessed July 12, 2022).
51. European Commission. Commission regulation (Eu) 2017/2158 of 20 November 2017 establishing mitigation measures and benchmark levels for the reduction of the presence of acrylamide in food. Off J Eur Union. (2017) 304:24–44.
52. Deutsche Gesellschaft für Ernährung e.V. Energie. (2015) Available online at: https://www.dge.de/wissenschaft/referenzwerte/energie/?L=0 (accessed December 26, 2022).
53. Behall K, Scholfield D, Hallfrisch J. Diets containing barley significantly reduce lipids in mildly hypercholesterolemic men and women. Am J Clin Nutr. (2004) 80:1185–93. doi: 10.1093/ajcn/80.5.1185
54. Behall K, Scholfield D, Hallfrisch J. Lipids significantly reduced by diets containing barley in moderately hypercholesterolemic men. J Am Coll Nutr. (2004) 23:55–62. doi: 10.1080/07315724.2004.10719343
55. Brown H, Prescott R. Applied Mixed Models in Medicine. 3 ed. New York, NY: John Wiley & Sons Inc (2015).
56. Jones B, Kenward M. Design and Analysis of Cross-over Trials. 3rd ed. New York, NY: Chapman and Hall/CRC (2014).
57. Cicero A, Fogacci F, Veronesi M, Strocchi E, Grandi E, Rizzoli E, et al. A randomized placebo-controlled clinical trial to evaluate the medium-term effects of oat fibers on human health: the beta-glucan effects on lipid profile, glycemia and intestinal health (belt) study. Nutrients. (2020) 12:686. doi: 10.3390/nu12030686
58. Whitehead A, Beck E, Tosh S, Wolever T. Cholesterol-lowering effects of oat β-glucan: a meta-analysis of randomized controlled trials. Am J Clin Nutr. (2014) 100:1413–21. doi: 10.3945/ajcn.114.086108
59. Kerckhoffs D, Hornstra G, Mensink R. Cholesterol-lowering effect of beta-glucan from oat bran in mildly hypercholesterolemic subjects may decrease when beta-glucan is incorporated into bread and cookies. Am J Clin Nutr. (2003) 78:221–7. doi: 10.1093/ajcn/78.2.221
60. Xu D, Feng M, Chu Y, Wang S, Shete V, Tuohy K, et al. The prebiotic effects of oats on blood lipids, gut microbiota, and short-chain fatty acids in mildly hypercholesterolemic subjects compared with rice: a randomized, controlled trial. Front Immunol. (2021) 12:787797. doi: 10.3389/fimmu.2021.787797
61. Zurbau A, Noronha J, Khan T, Sievenpiper J, Wolever T. The effect of oat β-glucan on postprandial blood glucose and insulin responses: a systematic review and meta-analysis. Eur J Clin Nutr. (2021) 75:1540–54. doi: 10.1038/s41430-021-00875-9
62. He L, Zhao J, Huang Y, Li Y. The difference between oats and beta-glucan extract intake in the management of hba1c, fasting glucose and insulin sensitivity: a meta-analysis of randomized controlled trials. Food Funct. (2016) 7:1413–28. doi: 10.1039/C5FO01364J
63. Wolever T, Jenkins A, Prudence K, Johnson J, Duss R, Chu Y, et al. Effect of adding oat bran to instant oatmeal on glycaemic response in humans – a study to establish the minimum effective dose of oat β-glucan. Food Funct. (2018) 9:1692–700. doi: 10.1039/C7FO01768E
64. Amerizadeh A, Ghaheh H, Vaseghi G, Farajzadegan Z, Asgary S. Effect of oat (Avena sativa L.) consumption on lipid profile with focus on triglycerides and high-density lipoprotein cholesterol (Hdl-C): an updated systematic review. Curr Probl Cardiol. (2022) 101153. doi: 10.1016/j.cpcardiol.2022.101153
65. Theuwissen E, Mensink R. Water-soluble dietary fibers and cardiovascular disease. Physiol Behav. (2008) 94:285–92. doi: 10.1016/j.physbeh.2008.01.001
66. He B, Nohara K, Ajami N, Michalek R, Tian X, Wong M, et al. Transmissible microbial and metabolomic remodeling by soluble dietary fiber improves metabolic homeostasis. Sci Rep. (2015) 5:10604. doi: 10.1038/srep10604
67. Joyce S, Kamil A, Fleige L, Gahan C. The cholesterol-lowering effect of oats and oat beta glucan: modes of action and potential role of bile acids and the microbiome. Front Nutr. (2019) 6:171. doi: 10.3389/fnut.2019.00171
68. Connolly M, Tzounis X, Tuohy K, Lovegrove J. Hypocholesterolemic and prebiotic effects of a whole-grain oat-based granola breakfast cereal in a cardio-metabolic “at risk” population. Front Microbiol. (2016) 7:1675. doi: 10.3389/fmicb.2016.01675
69. Mikkelsen M, Jespersen B, Larsen F, Blennow A, Engelsen S. Molecular structure of large-scale extracted β-glucan from barley and oat: identification of a significantly changed block structure in a high β-glucan barley mutant. Food Chem. (2013) 136:130–8. doi: 10.1016/j.foodchem.2012.07.097
70. Lambo A, Öste R, Nyman M. Dietary fibre in fermented oat and barley β-glucan rich concentrates. Food Chem. (2005) 89:283–93. doi: 10.1016/j.foodchem.2004.02.035
71. Lazaridou A, Biliaderis C, Micha-Screttas M, Steele BRA. Comparative study on structure–function relations of mixed-linkage (1→3), (1→4) linear B -D-glucans. Food Hydrocoll. (2004) 18:837–55. doi: 10.1016/j.foodhyd.2004.01.002
72. O’Keefe S. Nutrition and colonic health: the critical role of the microbiota. Curr Opin Gastroenterol. (2008) 24:51–8. doi: 10.1097/MOG.0b013e3282f323f3
73. Rimsten L, Stenberg T, Andersson R, Andersson A, Åman P. Determination of A -glucan molecular weight using sec with calcofluor detection in cereal extracts. Cereal Chem. (2003) 80:485–90. doi: 10.1094/CCHEM.2003.80.4.485
74. Lazaridou A, Biliaderis C. Molecular aspects of cereal β-glucan functionality: physical properties, technological applications and physiological effects. J Cereal Sci. (2007) 46:101–18. doi: 10.1016/j.jcs.2007.05.003
75. Du B, Meenu M, Liu H, Xu BA. Concise review on the molecular structure and function relationship of β-glucan. Int J Mol Sci (2019) 20:4032. doi: 10.3390/ijms20164032
76. Tiwari U, Cummins E. Factors influencing β-glucan levels and molecular weight in cereal-based products. Cereal Chem. (2009) 86:290–301. doi: 10.1094/CCHEM-86-3-0290
77. Bashir K, Choi J. Clinical and physiological perspectives of β-glucans: the past, present, and future. Int J Mol Sci. (2017) 18:1906.
78. Gunness P, Michiels J, Vanhaecke L, De Smet S, Kravchuk O, Van de Meene A, et al. Reduction in circulating bile acid and restricted diffusion across the intestinal epithelium are associated with a decrease in blood cholesterol in the presence of oat β-glucan. FASEB J. (2016) 30:4227–38. doi: 10.1096/fj.201600465R
79. Ellegård L, Andersson H. Oat bran rapidly increases bile acid excretion and bile acid synthesis: an ileostomy study. Eur J Clin Nutr. (2007) 61:938–45. doi: 10.1038/sj.ejcn.1602607
80. Ames N, Tosh S. Effects of processing on physicochemical properties and efficacy of β-glucan from oat and barley. CFW Plexus. (2013) 60:4–8. doi: 10.1094/CPLEX-2013-1226-59W
81. Tosh S, Chu Y. Systematic review of the effect of processing of whole-grain oat cereals on glycaemic response. Br J Nutr. (2015) 114:1256–62. doi: 10.1017/S0007114515002895
82. Wood P, Braaten J, Scott F, Riedel K, Wolynetz M, Collins M. Effect of dose and modification of viscous properties of oat gum on plasma glucose and insulin following an oral glucose load. Br J Nutr. (1994) 72:731–43. doi: 10.1079/bjn19940075
83. Xu D, Wang S, Feng M, Shete V, Chu Y, Kamil A, et al. Serum metabolomics reveals underlying mechanisms of cholesterol-lowering effects of oat consumption: a randomized controlled trial in a mildly hypercholesterolemic population. Mol Nutr Food Res. (2021) 65:e2001059. doi: 10.1002/mnfr.202001059
84. Li Y, Hruby A, Bernstein A, Ley S, Wang D, Chiuve S, et al. Saturated fats compared with unsaturated fats and sources of carbohydrates in relation to risk of coronary heart disease: a prospective cohort study. J Am Coll Cardiol. (2015) 66:1538–48. doi: 10.1016/j.jacc.2015.07.055
85. Farvid M, Ding M, Pan A, Sun Q, Chiuve S, Steffen L, et al. Dietary linoleic acid and risk of coronary heart disease: a systematic review and meta-analysis of prospective cohort studies. Circulation. (2014) 130:1568–78. doi: 10.1161/circulationaha.114.010236
86. Rebello C, O’Neil C, Greenway F. Dietary fiber and satiety: the effects of oats on satiety. Nutr Rev. (2015) 74:131–47. doi: 10.1093/nutrit/nuv063
Keywords: oat, barley, roasting, β-glucan, blood lipids, cardiovascular risk factors
Citation: Reiners S, Hebestreit S, Wedekind L, Kiehntopf M, Klink A, Rummler S, Glei M, Lorkowski S, Schlörmann W and Dawczynski C (2023) Effect of a regular consumption of traditional and roasted oat and barley flakes on blood lipids and glucose metabolism–A randomized crossover trial. Front. Nutr. 10:1095245. doi: 10.3389/fnut.2023.1095245
Received: 10 November 2022; Accepted: 17 January 2023;
Published: 02 February 2023.
Edited by:
Marija Takic, University of Belgrade, SerbiaReviewed by:
Siti Raihanah Shafie, Universiti Putra Malaysia, MalaysiaCaio Eduardo Gonçalves Reis, University of Brasilia, Brazil
Copyright © 2023 Reiners, Hebestreit, Wedekind, Kiehntopf, Klink, Rummler, Glei, Lorkowski, Schlörmann and Dawczynski. This is an open-access article distributed under the terms of the Creative Commons Attribution License (CC BY). The use, distribution or reproduction in other forums is permitted, provided the original author(s) and the copyright owner(s) are credited and that the original publication in this journal is cited, in accordance with accepted academic practice. No use, distribution or reproduction is permitted which does not comply with these terms.
*Correspondence: Christine Dawczynski, Q2hyaXN0aW5lLmRhd2N6eW5za2lAdW5pLWplbmEuZGU=