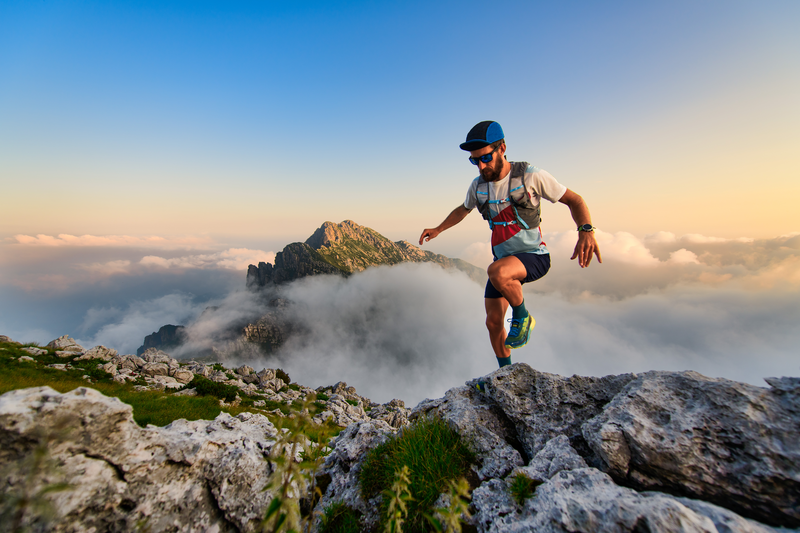
95% of researchers rate our articles as excellent or good
Learn more about the work of our research integrity team to safeguard the quality of each article we publish.
Find out more
ORIGINAL RESEARCH article
Front. Nutr. , 30 March 2023
Sec. Nutrition and Food Science Technology
Volume 10 - 2023 | https://doi.org/10.3389/fnut.2023.1083253
This article is part of the Research Topic Food Sustainability: Challenges and Opportunities for the Future View all 5 articles
Background: Selenium (Se) and zinc (Zn) are essential antioxidant enzyme cofactors. Foliar Se/Zn application is a highly effective method of plant biofortification. However, little is known about the effect of such applications on the concentration of trace elements and phytochemicals with pro-oxidant or antioxidant activity in pea (Pisum sativum L.).
Methods: A 2-year pot experiment (2014/2015) was conducted to examine the response of two pea varieties (Ambassador and Premium) to foliar-administered sodium selenate (0/50/100 g Se/ha) and zinc oxide (0/375/750 g Zn/ha) at the flowering stage. Concentrations of selected trace elements (Fe, Cu, and Mn), total phenolic content (TPC), total flavonoid content (TFC), and total antioxidant activity (ABTS, FRAP) of seeds were determined.
Results and conclusions: Se/Zn treatments did not improve the concentration of trace elements, while they generally enhanced TPC. Among examined treatments, the highest TPC was found in Ambassador (from 2014) treated with 100 g Se/ha and 750 g Zn/ha (2,926 and 3,221 mg/100 g DW, respectively) vs. the control (1,737 mg/100 g DW). In addition, 50 g of Se/ha increased TFC vs. the control (261 vs. 151 mg/100 g DW) in Premium (from 2014), 750 g of Zn/ha increased ABTS vs. the control (25.2 vs. 59.5 mg/100 g DW) in Ambassador (from 2015), and 50 g of Se/ha increased FRAP vs. the control (26.6 vs. 18.0 mmol/100 g DW) in Ambassador (from 2015). In linear multivariable regression models, Zn, Mn, Cu, and TPC best explained ABTS (R = 0.577), while Se, Cu, and TPC best explained the FRAP findings (R = 0.696). This study highlights the potential of foliar biofortification with trace elements for producing pea/pea products rich in bioactive plant metabolites beneficial for human health.
Phenolic compounds or polyphenols are organic (poly)hydroxylated compounds, which are widely abundant bioactive secondary plant metabolites (1). It is estimated that >8,000 phenolic compounds have been identified in plants (2, 3). These are recognized for their many different functions in plants, including their importance for pigmentation and sensory characteristics, growth and reproduction, and resistance against biotic and abiotic stress conditions (4–7). The concentration of phenolic compounds in plant sources depends on numerous factors, such as the biological background (genotype, organ, and ontogeny), cultivation techniques, growing conditions, ripening process, processing, storage conditions (8, 9), as well as extraction methods (10).
The current literature shows that the intake of phenolic compounds originating from various natural sources is related to antioxidant and anti-inflammatory activities, and may protect against a number of chronic diseases, such as cancer, type 2 diabetes, and other cardiometabolic complications, as well as against osteoporosis, pancreatitis, and gastrointestinal problems (11–13). A large body of research shows that the health benefits of phenolic compounds are linked to their antioxidant properties. These are regarded for their free radical scavenging activity, stabilization of divalent cations, and alteration of antioxidant enzymes, e.g., superoxide dismutase (SOD), catalase (CAT), and glutathione peroxidase (GPx), in part via their interactions with transcription factors, such as Nrf-2 (14–16).
Selenium (Se) is an essential trace element for humans. It is an integral component of Se-dependent antioxidant enzymes termed selenoproteins, such as GPx and thioredoxin reductases (TrxR), carrying out a variety of diverse functions. Selenoproteins are involved in antioxidant defense (e.g., within GPx), immune function (e.g., for T-cell proliferation), and thyroid hormone metabolism (e.g., for iodothyronine deiodinase), among others (17–19).
Zinc (Zn) is the second most abundant trace element [after iron (Fe)] and is essential for all living organisms (20). In humans, >300 enzymes and 2,000 transcription factors are known to require Zn. Zinc acts as a cofactor for various antioxidant enzymes, such as SOD and CAT (21). It plays important roles in cellular homeostasis, the immune system, brain and liver function, and metabolic processes, such as carbohydrate, lipid, protein, and nucleic acid synthesis or degradation (21–24).
A low intake of Se (as selenoproteins) and Zn or their low circulating concentrations have been linked with an increased risk of mortality and various chronic illnesses, such as several types of cancer, neurodegenerative diseases, type 2 diabetes, metabolic syndrome, and other cardiometabolic complications (17, 19, 22). Low Se and Zn statuses have also been associated with infectious diseases, including the recent COVID-19 (25, 26).
Pea (Pisum sativum L.) is an important legume widely grown and consumed throughout the world. The crop is used for animal and human nutrition. Pulses (including peas) are insufficiently represented in agricultural research, although they have been recognized for their contributions to sustainable agriculture and environment, biodiversity, public health, and food security (27–29). Peas are an important, inexpensive, and readily available source of proteins (30), carbohydrates, dietary fiber, starch, vitamins, minerals, and phytochemicals, which may be beneficial for human health and wellbeing (1, 31, 32). Phenolic compounds are the best-characterized phytochemicals in peas (31). In peas, at least 115 various polyphenols have been identified so far, constituting mostly glycosylated flavonols, together with biosynthetically related compounds, such as anthocyanins, flavones, flavanols, and flavanones (33). Clinical studies have shown that the consumption of peas and pea constituents may improve metabolic, cardiovascular, and gastrointestinal conditions in humans (31, 34). However, research dedicated to the possible health effects of pea phytochemicals (including phenolic compounds) is scarce. For example, in vitro anti-cancer activities of phenolic compounds from seed coats of pea were reported by Stanisavljević et al. (35). Another study emphasized the anti-cancer potential of flavonoids from pea peels (36).
Foliar spraying is a very effective method of plant biofortification for Se and Zn (37, 38). However, little information is available regarding the potential effects of trace elements on phytochemical antioxidants in pea that may contribute to health benefits in humans. Owing to their implications in many metabolic pathways, increased Se and Zn bioavailability in the plant could impact the accumulation of trace elements and bioactive secondary metabolites in plant tissues.
The aim of this 2-year pot experiment was to examine the response of pea varieties (Ambassador and Premium) to foliar-applied sodium selenate and zinc oxide at the flowering stage. The experiment consisted of five treatments, including one control (no fertilization) and two levels of foliar applications of both Se and Zn. Accumulation of selected trace elements and phytochemicals in seeds, related to anti-inflammatory and antioxidant processes, together with total antioxidant activity and their respective correlations, were determined.
Zinkuran SC was acquired from Arysta LifeScience Slovakia s.r.o. (Nové Zámky, Slovakia). Sodium selenate was purchased from Alfa Aesar (Karlsruhe, Germany). Nitric acid (HNO3, for trace element analysis) was obtained from LGC Standards (Molsheim, France), and hydrogen peroxide (H2O2) 30% (Suprapur) was obtained from Merck/VWR (Leuven, Belgium). Methanol was purchased from Biosolve (Valkenswaard, the Netherlands). Acetone, sodium carbonate (Na2CO3), and sodium hydroxide (NaOH) were acquired from Merck (Darmstadt, Germany). Ascorbic acid (vitamin C), (+)-catechin, gallic acid, Folin–Ciocalteu's phenol reagent, 2,4,6-tri(2-pyridyl)-s-triazine (TPTZ), 2,2′-azino-bis(3-ethylbenzothiazoline-6-sulfonic acid) (ABTS) diammonium salt, 2–2′-azobis (2-methylpropionamidine) dichloride (AAPH), aluminum chloride (AlCl3), and iron(II) sulfate were obtained from Sigma–Aldrich (St. Louis, MO, USA). Hydrogen chloride (HCl) and sodium nitrite (NaNO2) were obtained from VWR (Leuven, Belgium). Iron(III) chloride 6-hydrate was purchased from Merck (Darmstadt, Germany).
A 2-year outdoor pot experiment was conducted between March and June for two growing seasons (2014 and 2015) in the Botanical Garden of the Slovak University of Agriculture, Nitra, Slovakia (48.305 N, 18.096 E). The experiment was designed with four replicates per treatment, two pea varieties, 2 years, and five different treatments (employing a total of 80 pots), so that three main factors were studied. The chemical and physical properties of the tested soil (including employed methods), and climate conditions are provided in our previous study (39). Two dark-seeded and high-yielding pea varieties were used, namely Ambassador (late variety, restored hybrid) and Premium (early variety, open pollinated). Seeds were obtained from a local farmer. Ten L plastic square pots were filled with soil and protected by a wire mesh against birds. In total, 30 seeds/pot were sown at a depth of 5 cm in two rows in mid-March. Selenium as sodium selenate and Zn as Zinkuran SC (30% ZnO + 6% chelate) were applied in the experiment. No additional fertilization was employed according to the recommendations of pea farmers and earlier studies (40). The five treatments evaluated were control (no fertilization), 50 g of Se/ha (Se1), 100 g of Se/ha (Se2), 375 g of Zn/ha (Zn1), and 750 g of Zn/ha (Zn2). The solutions used contained 0.1 and 0.2 g/L of Se and 0.75 and 1.5 g/L of Zn. Foliar application with Se and Zn was carried out on a single occasion during the time of plant flowering and the absence of rain. The timing of foliar applications was chosen according to the previous investigation (40). A plastic trigger spray bottle was used for the manual application of fertilizers. Irrigation and phytosanitary control were applied regularly, following daily visual controls. No toxic effects of Se and Zn treatments on plants were reported during the experiment. No incidences of pests and diseases were observed. Freshly manually harvested seeds at physiological maturity were immediately lyophilized and homogenized by grinding, and the concentration of Fe, Cu, and Mn, total phenolic content (TPC), total flavonoid content (TFC), and antioxidant activities (ABTS and FRAP) were examined.
Sample aliquots (0.2 g) were mixed with 3.5 ml of each HNO3 (65%) and H2O2 (30%). Then, microwave digestion was carried out using a MARS 6 system (CEM, Orsay Cedex, France, 1,200 W, 10 min at 55°C, 10 min at 75°C, and 45 min at 120°C). Concentrations of Fe, Cu, and Mn [mg/kg dry weight (DW)] were determined through the water-diluted digests via an ICP-OES (Varian Vista MPX, Palo Alto, CA, USA). For quantification, external calibration was applied. Regular measurement of calibrated blanks was carried out to monitor accuracy and precision during sample runs. For this purpose, certified reference materials (rice flour NIST1568a, sea lettuce BCR279, and spinach leaves SRM 1570a) were employed.
The method published by Bouayed et al. (41) was employed to extract phenolic compounds (including flavonoids and ABTS determination) from dry material. In brief, 0.5–1 g of freeze-dried pea material was weighted in a 15 ml screw-cap falcon tube, to which 7.5 ml of 80% (v/v) methanol was added. The tubes were sonicated for 5 min and centrifuged (4,000 g, 5 min, RT, Heraeus Multifuge X3, Thermo Scientific, Leuven). Supernatants were removed and placed into a new 15 ml screw-cap falcon tube. Residues from the first tube were re-extracted once with 3 ml of 80% methanol, mixed, sonicated (5 min), and then centrifuged (4,000 g, 5 min). Both supernatants were combined, and this step was repeated once. The final extract was evaporated under nitrogen using a Turbovap blower (Caliper Life Sciences, Teralfene, Belgium) until ~2 ml remained (if needed, distilled water was added to a certain volume). Methanol (100%) was used to adjust to a final volume (4 ml). Extracts were overlayered with argon and stored at −80°C until further analyses.
The method described by Kaulmann et al. (42) was used. In brief, freeze-dried pea material (100 mg) was weighed into 15 ml plastic tubes, to which 2 ml of acetone was added, vortexed (1 min), sonicated (5 min), put for 5 min on ice, and centrifuged (2 min, 2,500 g, 4°C). The supernatant was removed and placed in a 50 ml plastic tube. The residue was re-extracted (four times with 2 ml of acetone). The combined extracts were mixed briefly, and the volume of acetone was noted down. An aliquot of this volume was filtered through a 0.45 μm nylon filter. Unused samples were stored under argon at −80°C.
Total phenolic content was measured spectrophotometrically, using Folin–Ciocalteu's phenol reagent and employing gallic acid as standard (41). In short, 200 μl of appropriately diluted extract or standard and 200 μl of Folin–Ciocalteu's phenol reagent were added to a 10 ml centrifuge tube containing 1.8 ml of water and vortexed. After 5 min of incubation, 2 ml of 7% (w/v) Na2CO3 was added, and the solution was immediately diluted to 5 ml with water and mixed well. The mixture was incubated (90 min, RT), and the absorbance was measured at 750 nm against the blank. Quantification was based on external calibration curves (gallic acid, n = 6 concentrations between 0 and 100 mg/L) to determine the total phenolic content expressed as gallic acid equivalents (GAE) per 100 g DW.
The total flavonoid content was assessed by spectrophotometry with catechin as a standard (41). In short, 200 μl of appropriately diluted extract or standard was added to 15 ml centrifuge vials containing 800 μl of water. Next, 60 μl of 5% (w/v) NaNO2 was added, and the vial was mixed. After 5 min, 60 μl of 10% AlCl3 was added. After 6 min, 400 μl of NaOH (1 M) was added, and the mixture was diluted to 2 ml with water. The absorbance was measured at 510 nm vs. the blank. Quantification was based on external calibration curves (catechin, n = 6 concentrations between 0 and 100 mg/L), and the total flavonoid content was expressed as catechin equivalents (CE) per 100 g DW.
The ABTS-radical scavenging capacity assay was examined using the vitamin C equivalent antioxidant capacity (VCEAC) test (41). In brief, 2.5 mM ABTS was mixed with 1 mM AAPH in phosphate-buffered saline (pH 7.4). The mixture was heated (water bath, at 68°C, ca. 15 min) to receive a blue–green ABTS radical solution. The absorbance of this solution was adjusted to 0.650 ± 0.020 with further phosphate-buffered saline (pH 7.4). Then, 40 μl of diluted extract or standard was mixed with 1,960 μl of ABTS radical solution. This was incubated in the dark at 37°C for 10 min. In the following, the absorbance was read at 734 nm vs. the blank. Quantification was performed by external calibration curves (vitamin C, n = 5 concentrations between 0 and 0.2 mg/L) to determine the antioxidant capacity expressed as vitamin C equivalents (VCE) per 100 g DW.
The ferric-reducing antioxidant power assay (FRAP) method was used based on the protocol of Bouayed et al. (41). In short, the FRAP reagent was prepared freshly by mixing acetate buffer (pH 3.6), 10 mM TPTZ solution in 40 mM HCl, and 20 mM iron(III) chloride solution (10:1:1, v/v/v). It was then warmed up (37°C in a water bath) before the application. Subsequently, 50 μl of the sample (appropriately diluted sample extracts or standard) was mixed with 1.5 ml of the FRAP reagent. After a 4-min incubation period, the absorbance was read at 593 nm vs. the blank. Quantification was carried out by external calibration curves using iron(II) sulfate solution (n = 7 concentrations between 100 and 2,000 μmol), and the antioxidant capacity was expressed as μmol Fe(II) per 100 g DW.
Normal distribution of data and equality of variance were determined through normality plots and box plots, respectively. When needed, data were log-transformed to fulfill the criteria of normal distribution. The effect of three factors on outcomes was studied. Multivariate models were then employed, with the concentration of trace elements, total phenolic content, total flavonoid content, as well as total antioxidant activity, as the observed (dependent) variables, variety (two levels), year (two levels), and treatment type (Se or Zn at two levels plus unamended control, i.e., five levels) as independent fixed factors. Biofortification levels were nested within biofortificant. Following significant Fisher's F-tests, all group-wise comparisons were carried out (Bonferroni post hoc tests). Following significant interactions, the models were further broken down to keep one of the interaction factors constant. A P-value of < 0.05 was regarded as statistically significant (two-sided). All analyses were performed using SPSS (version 19.0, IBM, Chicago, IL, USA).
Bivariate Pearson correlations were employed to study the relationship between total antioxidant activity (ABTS and FRAP) and trace elements, as well as total phenolics and total flavonoids, both for the Ambassador and Premium varieties.
Multivariable linear regression models (one for ABTS and one for FRAP) were created, following a backward elimination procedure starting with variety, total phenolics, total flavonoids, and all trace elements measured. For this purpose, p-values below 0.1 were considered for inclusion in the next step, while parameters with the highest p-values were step-wise excluded. This allowed for obtaining a model from the full set of variables (saturated model), where we automatically removed variables that did not contribute significantly to the R-square in the model.
Combined analysis of variance showed that treatment (pooled years and varieties) significantly affected only total phenolic and total flavonoid contents in seeds. The growing year (pooled treatments and varieties) had a significant effect on all variables except for seed Mn concentration. Variety (pooled treatments and years) showed a significant effect on all variables, with the exception of total flavonoid content in seeds. In some cases, interactions were significant (Table 1).
Table 1. Combined ANOVA for the effects of year, variety, and treatment on Fe, Cu, and Mn concentrations, total phenolic content, total flavonoid content, and total antioxidant activity (ABTS and FRAP) of seeds.
In 2014, treatment did not significantly impact the concentration of Fe vs. controls in both varieties. Ambassador had a significantly higher Fe concentration than Premium for all treatments except for Zn1. In 2015, treatment did not significantly influence the concentration of Fe vs. the control in Ambassador; however, both Se and Zn treatments significantly decreased the concentration of Fe vs. the control in Premium. Ambassador had a significantly higher Fe concentration than Premium for Se1 and Zn2. Growing year showed a significant effect on the concentration of Fe in Premium vs. Ambassador (Table 2).
Table 2. Effect of foliar application of Se and Zn, depending on variety and year, on seed Fe, Cu, and Mn concentrations.
For both 2014 and 2015, treatment did not significantly affect Cu concentration vs. controls in both varieties. Ambassador had significantly higher Cu concentration vs. Premium for all treatments in both years. Growing year had a significant influence on Cu concentration in Ambassador compared with Premium (Table 2).
In 2014, treatment did not significantly impact the concentration of Mn compared with controls in both varieties. Premium showed slightly but significantly higher Mn concentration than Ambassador for Se2 and Zn1. In 2015, treatment failed to show (p = 0.057) a significant influence on the concentration of Mn compared with the control in Ambassador. Both Se and Zn applications significantly reduced the concentration of Mn vs. the control in Premium. Premium exhibited significantly higher Mn concentration than Ambassador for the control. Growing year had no significant impact on the concentration of Mn in both varieties (Table 2).
In 2014, Zn2 followed by Zn1, Se2, and Se1 significantly increased TPC compared with the control in Ambassador, while Zn2 following Zn1 and Se1 significantly increased TPC vs. the control in Premium. Ambassador had significantly higher TPC than Premium for Se2 and Zn2. In 2015, treatment did not significantly affect TPC vs. the control in Premium, while Se1 followed by Se2, Zn1, and Zn2 significantly increased TPC vs. the control in Ambassador. Ambassador also exhibited significantly higher TPC than Premium for Se1 and Se2 (Figure 1A). Growing year showed a significant effect on TPC in both varieties (p < 0.001).
Figure 1. Effect of foliar application of Se and Zn in two growing seasons (2014 and 2015) on total phenolic content (A), total flavonoid content (B), and total antioxidant activity (ABTS and FRAP) [(C, D), respectively] of pea seeds. C (control), without Se and Zn; Se1, 50 g Se/ha; Se2, 100 g Se/ha; Zn1, 375 g Zn/ha; Zn2, 750 g Zn/ha; mean ± SD; n = 4. Bars not sharing the same superscript are significantly different within varieties. P-values on the right side of the figure show the effect of treatment across the two varieties (i.e., Ambassador vs. Premium).
In 2014, treatment did not significantly affect TFC vs. the control in Ambassador, while the Se1 treatment significantly increased TFC vs. the control in Premium. No significant differences were found for TFC between Ambassador and Premium for all treatments. In 2015, treatment did not significantly influence TFC vs. controls in both varieties. Ambassador showed significantly higher TFC than Premium for the control (Figure 1B). Growing year had a significant effect on TFC in both varieties (p < 0.001).
In 2014, treatment did not significantly impact ABTS compared with controls of both varieties. No significant differences were observed for ABTS between Ambassador and Premium for all treatments. In 2015, treatment did not significantly affect ABTS vs. the control of Premium, while the Zn2 treatment significantly increased ABTS vs. the control of Ambassador. Premium showed significantly higher ABTS than Ambassador for all treatments with the exception of Zn2 (Figure 1C). Growing year had a significant effect on ABTS of Ambassador (p = 0.029) and Premium (p < 0.001).
In 2014, treatment did not significantly influence FRAP vs. controls of both varieties. Ambassador had significantly higher FRAP than Premium for controls. In 2015, treatment did not significantly impact FRAP vs. the control of Premium, while the Se1 treatment significantly increased FRAP vs. the control of Ambassador. The latter showed significantly elevated FRAP vs. Premium for Se1, Se2, and Zn2 (Figure 1D). Growing year showed a significant influence on FRAP of Premium (p < 0.001) compared with Ambassador.
Overall, the strongest positive correlations were generally found between Mn and Fe concentrations, for both years and varieties, though not always reaching statistical significance. Furthermore, Zn concentration and ABTS always correlated positively, though not always significantly. In addition, significant positive correlations were observed between total phenolics and Cu concentration, and between total flavonoids and Se concentration for Premium from the 2015 growing season, between Fe and Cu concentrations for Premium from the 2014 growing season, and between Mn and Cu and Fe concentrations for Ambassador from the 2015 growing season (Figure 2). The full matrix of correlations is shown in Supplementary Table S2.
Figure 2. Bivariate correlations between Mn and Fe concentrations for Ambassador from the 2015 growing season (A), between Cu and Mn concentrations for Ambassador from the 2015 growing season (B), between Cu and Fe concentrations for Premium from the 2014 growing season (C), between Mn and Fe concentrations for Premium from the 2015 growing season (D), between total phenolics and Cu concentration for Premium from the 2015 growing season (E), and between total flavonoids and Se concentration for Premium from the 2015 growing season (F).
For Ambassador, Cu concentration was significantly and negatively correlated with seed dry matter (r2 = 0.1998), number of seeds/pod (r2 = 0.1927), pod length (r2 = 0.1544), and pod perimeter (r2 = 0.3091). A significant negative correlation was also observed between total flavonoids and seed dry matter (r2 = 0.2079), number of seeds/pod (r2 = 0.1232), pod length (r2 = 0.1109), and pod perimeter (r2 = 0.3919). Total phenolics showed a significant and positive correlation with the number of seeds/pod (r2 = 0.3158), pod length (r2 = 0.0980), and pod perimeter (r2 = 0.3709). For Premium, seed dry matter was significantly negatively correlated with Fe concentration (r2 = 0.1274) and ABTS (r2 = 0.3003). Pod length showed a significant and negative correlation with FRAP (r2 = 0.1436). Pod perimeter was significantly positively correlated with total phenolics (r2 = 0.4692) and FRAP (r2 = 0.3434), while it was significantly negatively correlated with Fe concentration (r2 = 0.4122), Cu concentration (r2 = 0.1253), total flavonoids (r2 = 0.1459), and ABTS (r2 = 0.4032) (Supplementary Table S3).
The variety of pea seeds did not result in a statistically significant effect in both models (ABTS and FRAP), thus combined results were analyzed. For ABTS, the final model included a constant, Zn, Mn, Cu, and total phenolics, with a regression coefficient R of 0.577 (Table 3). For FRAP, the final model included a constant, Se, Cu, and total phenolics, with a regression coefficient R of 0.696 (Table 4).
Table 3. Regression model for the association between trace elements, total phenolics and ABTS based on multivariable linear regression modeling.
Table 4. Regression model for the association between trace elements, total phenolics and FRAP based on multivariable linear regression modeling.
In the present study, we examined the influence of the foliar application of selenate and zinc oxide at the flowering stage on two pea varieties. The concentration of selected trace elements, total phenolic content, total flavonoid content, as well as the total antioxidant activity in seeds, were determined. Though treatments did not improve concentrations of trace elements, they generally enhanced total phenolic content and in part (though not consistently) total flavonoid content and total antioxidant activity (Tables 1, 2, Figure 1).
Pea was selected as it represents an important legume and nutritious staple with global importance for food security. The varieties, Ambassador and Premium, were chosen, on the one hand, due to their high-yielding performance, and on the other hand, because studies have suggested that dark-seeded varieties of pea seeds have a high potential to accumulate phenolics (43–46). Selenium and Zn solutions were administered via foliar application, as this approach reduced the effect of soil properties on interactions between the examined minerals (47). Previous findings employing foliar Se and Zn fertilization suggested that field peas, especially due to their higher protein concentration, may show a larger potential for Se and Zn uptake and therefore seed accumulation than cereals (48, 49). Selenium as selenate was applied due to its recognized high efficacy for foliar uptake (50). Although the essentiality of Se to higher plants is still controversial, beneficial effects of Se applications at low concentrations have been demonstrated, including for plant growth, development and yield, as well as enhanced resistance to abiotic stresses (51). Zinc oxide was employed following recommendations by the agro-industry. In contrast to Se, Zn is an essential trace element needed for plant growth and quality (52). Concentrations of trace elements and bioactive compounds were examined, as these are also needed for plant growth and/or their intakes have been related to human health outcomes.
Our previous study highlighted that foliar-applied sodium selenate and zinc oxide had minimal overall effects on the growth parameters of pea (39). This study further showed that selenate improved seed Se accumulation in both employed varieties in a dose-dependent fashion. Premium accumulated greater amounts of Se in seeds than Ambassador. The most pronounced Se accumulation was found in seeds of Premium treated with 100 g Se/ha (7.84 mg/kg DW) vs. the control (0.16 mg/kg). Contrarily, zinc oxide did not significantly affect seed Zn accumulation (Supplementary Table S1).
The present study revealed that selenate and zinc oxide did not improve concentrations of Fe, Cu, and Mn in pea seeds (Tables 1, 2), suggesting that Se and Zn did not impair the transport of the other trace elements. In theory, at low concentrations, Se may act as an antioxidant in plants, reducing lipid peroxidation, whereas at higher levels, it could act as a pro-oxidant, increasing the accumulation of lipid peroxidation products (53–55). Feng et al. (56) indicated that there can be associations between Se, Fe, and lipid peroxidation in plants, and suggested that the ambivalent effects of Se on plants may occur through the downregulation of Fe at low Se doses and the upregulation of Fe at high Se doses, as Fe may constitute a pro-oxidant following the Haber–Weiss reaction (57).
However, similar to the present findings, Poblaciones and Rengel demonstrated that foliar-applied selenate and zinc sulfate individually and in several combinations (at early seed filling) did not significantly affect Fe concentration in pea seeds (48, 49). Similarly, Kayan et al. (58) found that Fe concentration in seeds of chickpea was slightly, though not significantly, increased following foliar-applied Zn (zinc chelate and zinc sulfate) vs. controls. In contrast, another study by Poblaciones and Rengel (59) that examined the effect of all combinations of soil/foliar applications (before flowering and at the early seed-filling stage) of zinc sulfate on field pea, showed that seed Fe concentrations were significantly reduced (vs. the control) by soil Zn application. It could be argued that this may have been due to competitive reasons, as Fe and Zn can compete for similar absorption pathways in the plant root, as reviewed previously (60, 61). The uptake of trace elements by plants is affected by multiple transporters, many of which may transport more than one. For example, the Fe-regulated transporter (IRT), expressed in roots, is induced mainly by Fe deficiency (also by Zn application during Fe deficiency), and can transport Fe, Mn, Cu, Zn, and probably other divalent cations (62–64). Sharing similar uptake/transport systems by these ions may cause competition among them, resulting in antagonism and reduced accumulation (64). It is also worth noting that grain Zn and Fe concentrations may correlate significantly and positively with grain protein concentration (65, 66), which is in line with our recent study on pea seeds (47), indicating that genes influencing the accumulations of Zn, Fe, and protein synthesis are closely linked (67).
Our study showed that selenate enhanced total phenolic content and in part (though not consistently) total flavonoid content in pea seeds (Table 1, Figures 1A, B). Previous research also demonstrated that foliar-applied selenate positively affected total phenolic content in pea seeds (68), curly endive (69), and cauliflower florets (70), as well as total flavonoid content in Indian mustard leaves (71) and tomato fruits (72). The present study further revealed that selenate improved in part (though not consistently) the total antioxidant activity (FRAP) of pea seeds (Table 1, Figures 1C, D). Beneficial effects of foliar-applied selenate on total antioxidant activity were also reported previously for pea seeds (68) and cauliflower florets (70), although different tests were employed (2,2-diphenyl-1-picrylhydrazyl; DPPH and/or photochemiluminescence).
D'Amato et al. (73) emphasized that stress in the plant, induced by increased Se levels, activates the phenylpropanoid pathway involved in the biosynthesis of phenolic compounds (including phenolic acids), which may enhance the scavenging capacity of reactive oxygen species (ROS). The authors also indicated that these effects depend on plant species and plant growth stage, as well as Se treatments and forms. Our study confirmed not only the role of Se treatments but also of variety on the accumulation of total phenolics in pea seeds (Figure 1A). Groth et al. (74), in their study on apples, highlighted an inverse correlation between polyphenol oxidase (PPO) activity and total phenolic content, as the enzyme catalyzes the oxidation of phenolic compounds, which could explain the effect of Se biofortification, as well as the variety-specific differences. It is also worth stressing that Zhu et al. (72) indicated that proteins involved in the phenylpropanoid biosynthetic pathway, such as NAD-dependent epimerase (P230), oxidoreductase zinc-binding dehydrogenase (P242), and acyl-CoA synthetase (P252), were upregulated in Se-enriched tomato fruit, resulting in an increased total flavonoid content.
Our study further demonstrated that zinc oxide did not improve total flavonoid content, while it enhanced total phenolic content and in part (though not consistently) total antioxidant activity (ABTS) of pea seeds (Table 1, Figure 1). The fact that zinc oxide did not improve the accumulation of Zn, while it improved the accumulation of phenolics in seeds (Supplementary Table S1, Figure 1A) may suggest that exposure of pea plants to Zn was a sufficient stimulus to induce phenolic biosynthesis pathways. It has been revealed that Zn application is associated with the increased activity of enzymes of secondary plant metabolite pathways, namely shikimate dehydrogenase, phenylalanine ammonialyase (PAL), and PPO (75). Song et al. (76) showed, though on Zn-deficient soil, that foliar-applied zinc sulfate improves the accumulation of total phenols, flavonoids, flavanols, tannins, and anthocyanins in grape berries. DFR and LDOX genes, key genes for polyphenol formation, can catalyze the production of proanthocyanidins and anthocyanidins (77, 78). In the study by Song et al. (76), zinc sulfate significantly affected the expression of genes involved in phenolic (especially flavonol and anthocyanin) biosynthetic pathways (VvPAL, VvSTS29, VvCHS, VvCHI, VvF3H, VvFLS4, VvDFR, VvLDOX, and VvMYBF1) throughout berry development, fostering phenolic accumulation. The authors also pointed out that sucrose is a positive regulator of the biosynthesis of phenolics, particularly flavonoids (79), and the beneficial effect of Zn application on photosynthesis and sugar accumulation may improve the biosynthesis of flavonoids.
Finally, the present study showed significant relationships, among others, between Mn, Cu, and Fe concentrations, as well as between Fe and Cu concentrations in pea seeds (Figures 2A–D, Supplementary Table S2). The association between micro-minerals in plants may be affected by a number of factors that include species of the plant as well as genotype, dose and form of fertilizer, route of fertilizer application, cultivation aspects, characteristics of the soil, and antagonism and/or synergism between various elements, as emphasized by Malka et al. (47). Imbalance of trace minerals such as Fe, Cu, Mn, Zn, and Se, which are cofactors for antioxidant enzymes (51, 80), may affect the presence of bioactive compounds and total antioxidant activity of plants. Indeed, our research revealed significant positive relationships between total phenolics and Cu concentration (Figure 2E), as well as between total flavonoids and Se concentration (Figure 2F) for Premium seeds. Based on the linear multivariable regression models, Zn, Mn, Cu, and total phenolics were the best explanatory parameters for ABTS (Table 3), while Se, Cu, and total phenolics were the best explanatory variables for FRAP (Table 4), demonstrating the general importance of these trace elements and total phenolics for the total antioxidant activity of pea seeds. Previous studies on apples (81) and baby mustard (82) also showed that total phenolics were associated with total antioxidant activity (ABTS and FRAP). Furthermore, our previous study revealed that other compounds, including Ca, Mg, K, Na, soluble solids, protein, chlorophyll a and b, total chlorophyll, total carotenoids, and total condensed tannins, did not contribute substantially to the total antioxidant activity (ABTS and FRAP) of pea seeds (47).
Taken together, our study highlighted that selenate and zinc oxide, foliar applied at the flowering stage, generally enhanced total phenolic content in pea seeds. In addition, in part (though not consistently), selenate improved total flavonoid content and FRAP, while zinc oxide increased ABTS of seeds. Furthermore, the importance of trace elements for total phenolics and antioxidant activity was emphasized by multivariable regression models. Additional investigations are required to reveal molecular mechanisms regulating the accumulation of phenolic compounds in pea upon foliar-applied Se/Zn. Furthermore, although growing season is expected to play a role, the evaluation of the importance of climate conditions on the investigated parameters requires additional experiments under field conditions, which was not the case in the present study. This research is important in terms of producing pea/pea products rich in secondary plant metabolites with additional human health benefits. We conclude that foliar biofortification with trace elements may have the potential to boost phytochemical antioxidants in crops.
The original contributions presented in the study are included in the article/Supplementary material, further inquiries can be directed to the corresponding author.
MM designed and carried out the experiments, as well as prepared the first version of the manuscript. TB was involved in the analysis, statistical interpretation, and manuscript writing. GK was involved in performing the analysis. GD was involved in the conceptualization, supervision of the project, and writing of the manuscript. AH organized, designed, and supervised the experiments in Nitra. All authors have read and agreed to the published version of the manuscript.
This study was supported by the Ministry of Education, Science, Research and Sport of the Slovak Republic (grant: VEGA 1/0105/14) and the Luxembourg Institute of Science and Technology.
We thank the staff of the Slovak University of Agriculture in Nitra for helping with the organization of experiments and Radosław Marcinkowski (RADEC GmbH) for the transportation of the samples.
The authors declare that the research was conducted in the absence of any commercial or financial relationships that could be construed as a potential conflict of interest.
All claims expressed in this article are solely those of the authors and do not necessarily represent those of their affiliated organizations, or those of the publisher, the editors and the reviewers. Any product that may be evaluated in this article, or claim that may be made by its manufacturer, is not guaranteed or endorsed by the publisher.
The Supplementary Material for this article can be found online at: https://www.frontiersin.org/articles/10.3389/fnut.2023.1083253/full#supplementary-material
1. Singh B, Singh JP, Kaur A, Singh N. Phenolic composition and antioxidant potential of grain legume seeds: a review. Food Res Int. (2017) 101:1–16. doi: 10.1016/j.foodres.2017.09.026
2. Lecour S, Lamont KT. Natural polyphenols and cardioprotection. Mini-Rev Med Chem. (2011) 11:1191–9. doi: 10.2174/13895575111091191
3. Ouchemoukh S, Amessis-Ouchemoukh N, Gómez-Romero M, Aboud F, Giuseppe A, Fernández-Gutiérrez A, et al. Characterisation of phenolic compounds in Algerian honeys by RP-HPLC coupled to electrospray time-of-flight mass spectrometry. LWT Food Sci Technol. (2017) 85:460–9. doi: 10.1016/j.lwt.2016.11.084
4. Bravo L. Polyphenols: chemistry, dietary sources, metabolism, and nutritional significance. Nutr Rev. (1998) 56:317–33.
5. Alasalvar C, Grigor JM, Zhang D, Quantick PC, Shahidi F. Comparison of volatiles, phenolics, sugars, antioxidant vitamins, and sensory quality of different colored carrot varieties. J Agric Food Chem. (2001) 49:1410–6. doi: 10.1021/jf000595h
6. Naumovski N. Bioactive composition of plants and plant foods. In:Scarlett CJ, Vuong QV, , editors. Plant Bioactive Compounds for Pancreatic Cancer Prevention and Treatment. New York, NY: Nova Science Publishers (2015). p. 81–115.
7. Vagiri M, Johansson E, Rumpunen K. Phenolic compounds in black currant leaves: an interaction between the plant and foliar diseases? J Plant Interact. (2017) 12:193–9. doi: 10.1080/17429145.2017.1316524
8. Naczk M, Shahidi F. Phenolics in cereals, fruits and vegetables: occurrence, extraction and analysis. J Pharm Biomed Anal. (2006) 41:1523–42. doi: 10.1016/j.jpba.2006.04.002
9. Ksouri R, Megdiche W, Falleh H, Trabelsi N, Boulaaba M, Smaoui A, et al. Influence of biological, environmental and technical factors on phenolic content and antioxidant activities of Tunisian halophytes. Comptes Rendus Biol. (2008) 331:865–73. doi: 10.1016/j.crvi.2008.07.024
10. Zargoosh Z, Ghavam M, Bacchetta G, Tavili A. Effects of ecological factors on the antioxidant potential and total phenol content of Scrophularia striata Boiss. Sci Rep. (2019) 9:16021. doi: 10.1038/s41598-019-52605-8
11. Bhuyan DJ, Basu A. Phenolic compounds: potential health benefits and toxicity. In:Vuong QV, , editor. Utilisation of Bioactive Compounds from Agricultural and Food Waste. Boca Raton: CRC Press (2017). p. 27–59. doi: 10.1201/9781315151540-3
12. Cory H, Passarelli S, Szeto J, Tamez M, Mattei J. The role of polyphenols in human health and food systems: a mini-review. Front Nutr. (2018) 5:87. doi: 10.3389/fnut.2018.00087
13. Shahidi F, Yeo JD. Bioactivities of phenolics by focusing on suppression of chronic diseases: a review. Int J Mol Sci. (2018) 19:1573. doi: 10.3390/ijms19061573
14. Ozcan T, Akpinar-Bayizit A, Yilmaz-Ersan L, Delikanli B. Phenolics in human health. Int J Chem Eng Appl. (2014) 5:393–396. doi: 10.7763/IJCEA.2014.V5.416
15. Zhao D, Shi D, Sun J, Li H, Zhao M, Sun B. Quantification and cytoprotection by vanillin, 4-methylguaiacol and 4-ethylguaiacol against AAPH-induced abnormal oxidative stress in HepG2 cells. RSC Adv. (2018) 8:35474–84. doi: 10.1039/C8RA06505E
16. Chen J, He F, Liu S, Zhou T, Baloch S, Jiang C, et al. Cytoprotective Effect of ligustrum robustum polyphenol extract against hydrogen peroxide-induced oxidative stress via Nrf2 signaling pathway in Caco-2 cells. Evid Based Compl Altern Med. (2019) 2019:6458. doi: 10.1155/2019/5026458
17. Rayman MP. Selenium and human health. Lancet. (2012) 379:1256–68. doi: 10.1016/S0140-6736(11)61452-9
18. Zoidis E, Seremelis I, Kontopoulos N, Danezis GP. Selenium-dependent antioxidant enzymes: actions and properties of selenoproteins. Antioxidants. (2018) 7:66. doi: 10.3390/antiox7050066
19. Barchielli G, Capperucci A, Tanini D. The role of selenium in pathologies: an updated review. Antioxidants. (2022) 11:251. doi: 10.3390/antiox11020251
20. Kambe T, Tsuji T, Hashimoto A, Itsumura N. The physiological, biochemical, and molecular roles of zinc transporters in zinc homeostasis and metabolism. Physiol Rev. (2015) 95:749–84. doi: 10.1152/physrev.00035.2014
21. Marreiro DDN, Cruz KJC, Morais JBS, Beserra JB, Severo JS, de Oliveira ARS. Zinc and oxidative stress: current mechanisms. Antioxidants. (2017) 6:24. doi: 10.3390/antiox6020024
22. Kaur K, Gupta R, Saraf SA, Saraf SK. Zinc: The metal of life. Compr Rev Food Sci Food Saf. (2014) 13:358–76. doi: 10.1111/1541-4337.12067
23. Lee SR. Critical role of zinc as either an antioxidant or a prooxidant in cellular systems. Oxid Med Cell Longev. (2018) 2018:6285. doi: 10.1155/2018/9156285
24. Vallee BL. Zinc: biochemistry, physiology, toxicology and clinical pathology. Biofactors. (1988) 1:31–6.
25. Alexander J, Tinkov A, Strand TA, Alehagen U, Skalny A, Aaseth J. Early nutritional interventions with zinc, selenium and vitamin D for raising anti-viral resistance against progressive COVID-19. Nutrients. (2020) 12:2358. doi: 10.3390/nu12082358
26. Du Laing G, Petrovic M, Lachat C, De Boevre M, Klingenberg GJ, Sun Q, et al. Course and survival of COVID-19 patients with comorbidities in relation to the trace element status at hospital admission. Nutrients. (2021) 13:3304. doi: 10.3390/nu13103304
27. Powers SE, Thavarajah D. Checking agriculture's pulse: field pea (P. sativum L.), sustainability, and phosphorus use efficiency. Front Plant Sci. (2019) 10:1489. doi: 10.3389/fpls.2019.01489
28. Ferreira H, Pinto E, Vasconcelos MW. Legumes as a cornerstone of the transition toward more sustainable agri-food systems and diets in Europe. Front Sustain Food Syst. (2021) 5:694121. doi: 10.3389/fsufs.2021.694121
29. Sahruzaini NA, Rejab NA, Harikrishna JA, Khairul Ikram NK, Ismail I, Kugan HM, et al. Pulse crop genetics for a sustainable future: where we are now and where we should be heading. Front Plant Sci. (2020) 11:531. doi: 10.3389/fpls.2020.00531
30. Ge J, Sun CX, Corke H, Gul K, Gan RY, Fang Y. The health benefits, functional properties, modifications, and applications of pea (P. sativum L.) protein: current status, challenges, and perspectives. Compr Rev Food Sci Food Saf. (2020) 19:1835–76. doi: 10.1111/1541-4337.12573
31. Dahl WJ, Foster LM, Tyler RT. Review of the health benefits of peas (P. sativum L.). Br J Nutr. (2012) 108:S3–S10. doi: 10.1017/S0007114512000852
32. Hegedusová A, Mezeyová I, Timoracká M, Šlosár M, Musilová J, Juríková T. Total polyphenol content and antioxidant capacity changes in dependence on chosen garden pea varieties. Potravinarstvo. (2015) 9:1–8. doi: 10.5219/412
33. Fahim JR, Attia EZ, Kamel MS. The phenolic profile of pea (P. sativum): a phytochemical and pharmacological overview. Phytochem Rev. (2019) 18:173–98. doi: 10.1007/s11101-018-9586-9
34. Kumari T, Deka SC. Potential health benefits of garden pea seeds and pods: a review. Legum Sci. (2021) 3:e82. doi: 10.1002/leg3.82
35. Stanisavljević NS, Ilić MD, Matić IZ, Jovanović ŽS, Cupić T, Dabić D, et al. Identification of phenolic compounds from seed coats of differently colored european varieties of pea (P. sativum L.) and characterization of their antioxidant and in vitro anticancer activities. Nutr Cancer. (2016) 68:988–1000. doi: 10.1080/01635581.2016.1190019
36. El-Feky AM, Elbatanony MM, Mounier MM. Anti-cancer potential of the lipoidal and flavonoidal compounds from P. sativum and Vicia faba peels. Egy J Basic Appl Sci. (2018) 5:258–64. doi: 10.1016/j.ejbas.2018.11.001
37. Delaqua D, Carnier R, Berton RS, Corbi FCA, Coscione AR. Increase of selenium concentration in wheat grains through foliar application of sodium selenate. J Food Compos Anal. (2021) 99:103886. doi: 10.1016/j.jfca.2021.103886
38. Sattar A, Wang X, Ul-Allah S, Sher A, Ijaz M, Irfan M, et al. Foliar application of zinc improves morpho-physiological and antioxidant defense mechanisms, and agronomic grain biofortification of wheat (Triticum aestivum L.) under water stress. Saudi J Biol Sci. (2021) 29:1699–706. doi: 10.1016/j.sjbs.2021.10.061
39. Malka M, Du Laing G, Li J, Bohn T. Separate foliar sodium selenate and zinc oxide application enhances Se but not Zn accumulation in pea (P. sativum L.) seeds. Front Plant Sci. (2022) 13:968324. doi: 10.3389/fpls.2022.968324
40. Poblaciones MJ, Rodrigo S, Santamaría O. Evaluation of the potential of peas (P. sativum L.) to be used in selenium biofortification programs under mediterranean conditions. Biol Trace Elem Res. (2013) 151:132–7. doi: 10.1007/s12011-012-9539-x
41. Bouayed J, Hoffmann L, Bohn T. Total phenolics, flavonoids, anthocyanins and antioxidant activity following simulated gastro-intestinal digestion and dialysis of apple varieties: bioaccessibility and potential uptake. Food Chem. (2011) 128:14–21. doi: 10.1016/j.foodchem.2011.02.052
42. Kaulmann A, Jonville MC, Schneider YJ, Hoffmann L, Bohn T. Carotenoids, polyphenols and micronutrient profiles of Brassica oleraceae and plum varieties and their contribution to measures of total antioxidant capacity. Food Chem. (2014) 155:240–50. doi: 10.1016/j.foodchem.2014.01.070
43. Troszyńska A, Ciska E. Phenolic Compounds of seed coats of white and coloured varieties of pea (P. sativum L.) and their total antioxidant activity. Czech J Food Sci. (2002) 20:15–22. doi: 10.17221/3504-CJFS
44. Dueñas M, Estrella I, Hernández T. Occurrence of phenolic compounds in the seed coat and the cotyledon of peas (P. sativum L.). Eur Food Res Technol. (2004) 219:116–23. doi: 10.1007/s00217-004-0938-x
45. Xu BJ, Yuan SH, Chang SKC. Comparative analyses of phenolic composition, antioxidant capacity, and color of cool season legumes and other selected food legumes. J Food Sci. (2007) 72:S167–77. doi: 10.1111/j.1750-3841.2006.00261.x
46. Campos-Vega R, Loarca-Piña G, Oomah BD. Minor components of pulses and their potential impact on human health. Food Res Int. (2010) 43:461–82. doi: 10.1016/j.foodres.2009.09.004
47. Malka M, Du Laing G, Bohn T. Separate effects of foliar applied selenate and zinc oxide on the accumulation of macrominerals, macronutrients and bioactive compounds in two pea (P. sativum L.) seed varieties. Plants. (2022) 11:2009. doi: 10.3390/plants11152009
48. Poblaciones MJ, Rengel Z. The effect of processing on P. sativum L. biofortified with sodium selenate. J Plant Nutr Soil Sci. (2018) 181:932–7. doi: 10.1002/jpln.201800251
49. Poblaciones MJ, Rengel Z. Combined foliar selenium and zinc biofortification in field pea (P. sativum): accumulation and bioavailability in raw and cooked grains. Crop Pasture Sci. (2017) 68:265–71. doi: 10.1071/CP17082
50. Ros GH, van Rotterdam AMD, Bussink DW, Bindraban PS. Selenium fertilization strategies for bio-fortification of food: an agro-ecosystem approach. Plant Soil. (2016) 404:99–112. doi: 10.1007/s11104-016-2830-4
51. Hasanuzzaman M, Bhuyan MB, Raza A, Hawrylak-Nowak B, Matraszek-Gawron R, Al Mahmud J, et al. Selenium in plants: boon or bane? Environ Exp Bot. (2020) 178:104170. doi: 10.1016/j.envexpbot.2020.104170
52. Hacisalihoglu G. Zinc (Zn): the last nutrient in the alphabet and shedding light on Zn efficiency for the future of crop production under suboptimal Zn. Plants. (2020) 9:1471. doi: 10.3390/plants9111471
53. Cartes P, Gianfreda L, Mora ML. Uptake of selenium and its antioxidant activity in ryegrass when applied as selenate and selenite forms. Plant Soil. (2005) 276:359–67. doi: 10.1007/s11104-005-5691-9
54. Hartikainen H, Xue T, Piironen V. Selenium as an anti-oxidant and pro-oxidant in ryegrass. Plant Soil. (2000) 225:193–200. doi: 10.1023/A:1026512921026
55. Mora ML, Pinilla L, Rosas A, Cartes P. Selenium uptake and its influence on the antioxidative system of white clover as affected by lime and phosphorus fertilization. Plant Soil. (2008) 303:139–49. doi: 10.1007/s11104-007-9494-z
56. Feng R, Wei C, Tu S, Wu F. Effects of Se on the uptake of essential elements in Pteris vittata L. Plant Soil. (2009) 325:123–32. doi: 10.1007/s11104-009-9961-9
57. Ranieri A, Castagna A, Baldan B, Soldatini GF. Iron deficiency differently affects peroxidase isoforms in sunflower. J Exp Bot. (2001) 52:25–35. doi: 10.1093/jexbot/52.354.25
58. Kayan N, Gulmezoglu N, Kaya MD. The optimum foliar zinc source and level for improving Zn content in seed of chickpea. Legum Res. (2015) 38:826–31. doi: 10.18805/lr.v38i6.6731
59. Poblaciones MJ, Rengel Z. Soil and foliar zinc biofortification in field pea (P. sativum L.): grain accumulation and bioavailability in raw and cooked grains. Food Chem. (2016) 212:427–33. doi: 10.1016/j.foodchem.2016.05.189
60. Fan X, Zhou X, Chen H, Tang M, Xie X. Cross-talks between macro- and micronutrient uptake and signaling in plants. Front Plant Sci. (2021) 12:663477. doi: 10.3389/fpls.2021.663477
61. Wang Q, Chen M, Hao Q, Zeng H, He Y. Research and progress on the mechanism of iron transfer and accumulation in rice grains. Plants. (2021) 10:2610. doi: 10.3390/plants10122610
62. Sinclair SA, Krämer U. The zinc homeostasis network of land plants. Biochim Biophys Acta. (2012) 1823:1553–67. doi: 10.1016/j.bbamcr.2012.05.016
63. Connolly EL, Fett JP, Guerinot ML. Expression of the IRT1 metal transporter is controlled by metals at the levels of transcript and protein accumulation. Plant Cell. (2002) 14:1347–57. doi: 10.1105/tpc.001263
64. Bindraban PS, Dimkpa C, Nagarajan L, Roy A, Rabbinge R. Revisiting fertilisers and fertilisation strategies for improved nutrient uptake by plants. Biol Fertil Soils. (2015) 51:897–911. doi: 10.1007/s00374-015-1039-7
65. Ghasemi S, Khoshgoftarmanesh AH, Afyuni M, Hadadzadeh H. The effectiveness of foliar applications of synthesized zinc-amino acid chelates in comparison with zinc sulfate to increase yield and grain nutritional quality of wheat. Eur J Agron. (2013) 45:68–74. doi: 10.1016/j.eja.2012.10.012
66. Cakmak I, Pfeiffer WH, McClafferty B. Biofortification of durum wheat with zinc and iron. Cereal Chem. (2010) 87:10–20. doi: 10.1094/CCHEM-87-1-0010
67. Cakmak I, Torun A, Millet E, Feldman M, Fahima T, Korol A, et al. Triticum dicoccoides: an important genetic resource for increasing zinc and iron concentration in modern cultivated wheat. Soil Sci Plant Nutr. (2004) 50:1047–54. doi: 10.1080/00380768.2004.10408573
68. Hegedusová A, Mezeyová I, Hegedus O, Andrejiová A, Juríková T, Golian M, et al. Increasing of selenium content and qualitative parameters in garden pea (P. sativum L.) after its foliar application. Acta Sci Pol Hortorum Cultus. (2017) 16:3–17. doi: 10.24326/asphc.2017.6.1
69. Sabatino L, Ntatsi G, Iapichino G, D'Anna F, De Pasquale C. Effect of selenium enrichment and type of application on yield, functional quality and mineral composition of curly endive grown in a hydroponic system. Agronomy. (2019) 9:207. doi: 10.3390/agronomy9040207
70. Saeedi M, Soltani F, Babalar M, Izadpanah F, Wiesner-Reinhold M, Baldermann S. Selenium fortification alters the growth, antioxidant characteristics and secondary metabolite profiles of cauliflower (Brassica oleracea var. botrytis) cultivars in hydroponic culture. Plants. (2021) 10:1537. doi: 10.3390/plants10081537
71. Golubkina N, Kekina H, Caruso G. Yield, quality and antioxidant properties of Indian mustard (Brassica juncea L.) in response to foliar biofortification with selenium and iodine. Plants. (2018) 7:80. doi: 10.3390/plants7040080
72. Zhu Z, Zhang Y, Liu J, Chen Y, Zhang X. Exploring the effects of selenium treatment on the nutritional quality of tomato fruit. Food Chem. (2018) 252:9–15. doi: 10.1016/j.foodchem.2018.01.064
73. D'Amato R, Fontanella MC, Falcinelli B, Beone GM, Bravi E, Marconi O, et al. Selenium biofortification in rice (Oryza sativa L.) sprouting: effects on se yield and nutritional traits with focus on phenolic acid profile. J Agric Food Chem. (2018) 66:4082–90. doi: 10.1021/acs.jafc.8b00127
74. Groth S, Budke C, Neugart S, Ackermann S, Kappenstein FS, Daum D, et al. Influence of a selenium biofortification on antioxidant properties and phenolic compounds of apples (Malus domestica). Antioxidants. (2020) 9:187. doi: 10.3390/antiox9020187
75. Majdoub N, El-Guendouz S, Rezgui M, Carlier J, Costa C, Kaab LBB, et al. Growth, photosynthetic pigments, phenolic content and biological activities of Foeniculum vulgare Mill, Anethum graveolens L and Pimpinella anisum L (Apiaceae) in response to zinc. Ind Crops Prod. (2017) 109:627–36. doi: 10.1016/j.indcrop.2017.09.012
76. Song CZ, Liu MY, Meng JF, Chi M, Xi ZM, Zhang ZW. Promoting effect of foliage sprayed zinc sulfate on accumulation of sugar and phenolics in berries of Vitis vinifera cv. Merlot growing on zinc deficient soil. Molecules. (2015) 20:2536–54. doi: 10.3390/molecules20022536
77. He F, Mu L, Yan GL, Liang NN, Pan QH, Wang J, et al. Biosynthesis of anthocyanins and their regulation in colored grapes. Molecules. (2010) 15:9057–91. doi: 10.3390/molecules15129057
78. Wang H, Fan W, Li H, Yang J, Huang J, Zhang P. Functional characterization of dihydroflavonol-4-reductase in anthocyanin biosynthesis of purple sweet potato underlies the direct evidence of anthocyanins function against abiotic stresses. PLoS ONE. (2013) 8:e78484. doi: 10.1371/journal.pone.0078484
79. Solfanelli C, Poggi A, Loreti E, Alpi A, Perata P. Sucrose-specific induction of the anthocyanin biosynthetic pathway in Arabidopsis. Plant Physiol. (2006) 140:637–46. doi: 10.1104/pp.105.072579
80. Rajput VD, Harish S, Singh RK, Verma KK, Sharma L, Quiroz-Figueroa FR, et al. Recent developments in enzymatic antioxidant defence mechanism in plants with special reference to abiotic stress. Biology. (2021) 10:267. doi: 10.3390/biology10040267
81. Bouayed J, Hoffmann L, BohnT. Antioxidative mechanisms of whole-apple antioxidants employing different varieties from Luxembourg. J Med Food. (2011) 14:1631–7. doi: 10.1089/jmf.2010.0260
Keywords: oxidative stress, micronutrients, trace elements, polyphenols, legume biofortification, food security, mineral deficiency, foliar Se/Zn application
Citation: Malka M, Du Laing G, Kurešová G, Hegedüsová A and Bohn T (2023) Enhanced accumulation of phenolics in pea (Pisum sativum L.) seeds upon foliar application of selenate or zinc oxide. Front. Nutr. 10:1083253. doi: 10.3389/fnut.2023.1083253
Received: 28 October 2022; Accepted: 13 February 2023;
Published: 30 March 2023.
Edited by:
Monika Thakur, Amity University, IndiaReviewed by:
Karuna Singh, Sharda University, IndiaCopyright © 2023 Malka, Du Laing, Kurešová, Hegedüsová and Bohn. This is an open-access article distributed under the terms of the Creative Commons Attribution License (CC BY). The use, distribution or reproduction in other forums is permitted, provided the original author(s) and the copyright owner(s) are credited and that the original publication in this journal is cited, in accordance with accepted academic practice. No use, distribution or reproduction is permitted which does not comply with these terms.
*Correspondence: Torsten Bohn, VG9yc3Rlbi5Cb2huQGxpaC5sdQ==
Disclaimer: All claims expressed in this article are solely those of the authors and do not necessarily represent those of their affiliated organizations, or those of the publisher, the editors and the reviewers. Any product that may be evaluated in this article or claim that may be made by its manufacturer is not guaranteed or endorsed by the publisher.
Research integrity at Frontiers
Learn more about the work of our research integrity team to safeguard the quality of each article we publish.