- 1Maternal and Childhood Obesity Research Center, Urmia University of Medical Sciences, Urmia, Iran
- 2Department of Nutrition, School of Medicine, Urmia University of Medical Sciences, Urmia, Iran
- 3Food and Beverages Safety Research Center, Urmia University of Medical Sciences, Urmia, Iran
- 4School of Public Health, Urmia University of Medical Sciences, Urmia, Iran
- 5Department of Infectious Diseases and Dermatology, Urmia University of Medical Sciences, Urmia, Iran
- 6Cancer Prevention and Control Program, Department of Epidemiology and Biostatistics, Arnold School of Public Health, University of South Carolina, Columbia, SC, United States
Background: Inflammation and cytokine storm have been reported to be the main cause of acute symptoms of coronavirus disease (COVID-19). Diet-induced inflammation may affect the condition of patients with COVID-19. Therefore, this study aimed to investigate the relationship between disease severity, inflammatory and immune system biomarkers, and the dietary inflammatory index (DII) in patients with COVID-19.
Methods: This cross-sectional study was conducted on 500 adult patients with COVID-19. Patients were divided into mild, moderate, and severe conditions based on clinical and laboratory evidence. A validated food frequency questionnaire (FFQ) was used to determine DII and energy-adjusted DII (E-DII) scores. The serum C-reactive protein (CRP) level and blood cell count were measured for all patients. Multiple linear regression was used to explore the association between DII and E-DII and CRP, blood cell counts, and hospitalization in patients with COVID-19.
Results: Coronavirus disease (COVID-19) patients with higher DII had higher consumption of fat and carbohydrate and lower intakes of protein, anti-inflammatory nutrients, garlic, caffeine, tea, onion, and fiber (P < 0.05). There was a positive association between DII and CRP (β = 1.024, P < 0.001), hospitalization (β = 1.062, P < 0.001), WBC count (β = 0.486, P < 0.009), neutrophil count (β = 0.565, P < 0.001), and neutrophil-to-lymphocyte ratio (β = 0.538, P < 0.001) and a negative association between DII and the lymphocyte count (β = −0.569, P < 0.001). There was a positive association between E-DII and hospitalization (β = 1.645, P < 0.001), WBC count (β = 0.417, P < 0.02), and neutrophil-to-lymphocyte ratio (β = 0.35, P < 0.03).
Conclusion: There is a positive correlation between DII and inflammation, immune hyperactivation, and length of hospital stay in patients with COVID-19. Further longitudinal studies are necessary.
Introduction
Since 2019, the world has been grappling with a major health crisis caused by severe acute respiratory syndrome virus 2 (SARS-CoV-2). To date, ~579 million confirmed cases of COVID-19 and 6.4 million deaths have been reported worldwide (1). A COVID-19 infection can present as an asymptomatic infection, or patients can present with a mild upper respiratory tract illness that includes cough, chills, fever, fatigue, and shortness of breath. In severe cases, the most common complications are acute respiratory distress syndrome (ARDS), heart failure, and septic shock. However, severe viral pneumonia with respiratory failure can potentially lead to death (2). Multiple organ dysfunction is probably caused by uncontrolled acute inflammation due to cytokine storm (3).
It has been reported that the severity of the disease is related to socioeconomic status, demographic factors such as age (age over 60 years) and male gender, having an underlying disease, time of initiation of treatment, quality of health care (4), ethnicity (black and South Asian) (5, 6), nutritional and lifestyle items (such as diet, lack of vitamin D level, and obesity) (7–9), smoking (10), and viral load (11).
Many studies have shown that inflammatory status is associated with dietary patterns in patients with COVID-19 (12–16). Adequate intake of fruits, vegetables, olive oil, nuts, and whole grains, which are components of the Mediterranean diet, is negatively associated with inflammation in patients with COVID-19 (12, 17, 18). Moreover, some of the nutrients including vitamins D and A, zinc, selenium, flavonoids, and unsaturated fatty acids can reverse inflammatory conditions in these patients, especially by affecting nuclear factor kappa-B (NF-κB), which is the main production regulator of pro-inflammatory cytokines such as interleukin-6 (IL-6) and tumor necrosis factor-alpha (TNF-α). However, foods with higher glycemic load, simple carbohydrates, saturated fatty acids, processed foods, and red meats found in Western diets are positively associated with inflammation in COVID-19 (19–22).
The dietary inflammatory index (DII) was designed to assess the inflammatory potential of the diet by Shivapa et al. The DII scores the diet based on the intake of 45 foods that affect the concentration of certain inflammatory biomarkers such as IL-6, TNF-α, and C-reactive protein (CRP) or anti-inflammatory biomarkers such as IL-10 and IL-4 (23–26). Recently, studies have shown that DII had a positive relationship with the amount of fibrinogen and white blood cells (lymphocyte, monocyte, neutrophil, neutrophil-to-lymphocyte ratio, eosinophil, and basophil) (24, 27).
To the best of our knowledge, only Moludi et al. have reported that energy-adjusted DII (E-DII) was related to an increased risk of developing COVID-19, as well as CRP and severity of the disease. However, their sample size was low, and studied biomarkers were limited (13). As a result, the present study was conducted to determine the relationship between DII and E-DII, disease severity, and inflammatory and immune system biomarkers in patients with COVID-19.
Methods
Study population
The study population of the present consecutive cross-sectional study was adult patients with COVID-19 who were referred to Taleghani Hospital in Urmia, Iran. Based on convenience sampling, all patients with COVID-19 diagnosed with clinical symptoms and quantitative polymerase chain reaction (qPCR) testing from March 2021 to September 2021 were included in the study (28). Patients with obesity (BMI ≥ 30) (29), pregnancy (30), and surgery (31) were excluded. The sample size was calculated based on the study of Shivappa et al. (32) with the correlation coefficient r = 0.12. Taking into account the confidence interval of 95% and the study power of 80%, the sample size of 462 was calculated. Considering a 10% dropout rate, the sample size was calculated as 500 patients.
After the diagnosis of COVID-19, the patients were divided into inpatient and outpatient groups based on evidence such as arterial oxygen saturation, respiratory rate, clinical symptoms, and lung involvement in CT scans. The criteria for dividing individuals into mild, moderate, severe, and critical [intensive care unit (ICU)] categories have been previously reported (2).
The Ethics Committee of Urmia University of Medical Sciences, Urmia, Iran, approved the study protocol (ethics code IR.UMSU.REC.1399.367). This research complies with the standards of the Declaration of Helsinki and current ethical guidelines.
Dietary inflammatory index
Regarding the assessment of dietary intake, the validated 147-item food frequency questionnaire (FFQ) (33) was filled out by phone call, based on the recommendations of the ethics committee, which did not allow direct contact between the researchers and the patients with COVID-19. Food intake analysis was then performed after converting all units to grams using Nutritionist-IV software (version 7.0; NSquared computing, Salem, OR, USA) to check the intake of the year before the disease. Before filling out the questionnaire, images containing food units were sent to the patients' mobile phones through applications or e-mail. After the full explanation of the food units, a phone call was made to the patient and the questionnaire was completed.
After completing the FFQ, Shivappa et al.'s method (34) was used to determine DII scores. They reported a total of 45 specific nutrients and food items that affect the concentration of some inflammatory biomarkers such as interleukin-6 (IL-6), tumor necrosis factor-alpha (TNF-α), IL-1β, and C-reactive protein (CRP) or anti-inflammatory biomarkers such as IL-10 and IL-4. Accordingly, the inflammatory potential of each food was evaluated according to either the increase in inflammatory factors, the decrease in anti-inflammatory factors, or the lack of effect on inflammation. In the first step, the mean and standard deviation for each of the 45 food parameters were estimated based on data that were then linked to a regionally representative global database. A z-score was calculated by subtracting the “standard report mean” from the “standard global mean” and then dividing it by its standard deviation. The calculated value is converted to a percentile score to minimize the effect of ‘right skewing’, and then, this value is multiplied by the respective inflammatory effect score to derive the overall DII score. A total of 28 nutritional parameters including energy, carbohydrates, total fat, protein, saturated fat, cholesterol, polyunsaturated fatty acids (PUFAs), monounsaturated fatty acids (MUFAs), fiber, vitamins A, C, D, and E, beta-carotene, B vitamins (B1, B2, B3, B6, B9, and B12), iron, magnesium, zinc, selenium, garlic, caffeine, tea, and onion were available for the current FFQ to calculate DII. Energy-adjusted DII (E-DII) was calculated by derived DII per 1,000 kcal.
Biochemical measures
According to the protocol for the management of COVID-19, after referring to the hospital and confirming the inclusion criteria, the serum CRP level and blood cell count were performed for all patients.
Statistical analysis
In this study, data analysis was performed by SPSS software (version 25, Chicago, IL, USA). A normality test was done using the Kolmogorov–Smirnov test and descriptive data (mean and SD, skewness, kurtosis) (35). Fisher's exact test analyzed the distribution of qualitative variables among the DII and E-DII quartiles and different stages of COVID-19. A one-way analysis of variance (ANOVA) (the Kruskal–Wallis test for non-normally distributed data) was used to determine the difference in quantitative variables among the DIIs and E-DII quartiles. Post-hoc analyses were performed based on Tukey's test. A correlation test was performed to investigate the relationship between the severity of the disease, inflammatory and immune system biomarkers, and the DII and E-DII values. Multiple linear regression was used to explore the association between DII and E-DII and CRP, blood cell counts, and hospitalization in patients with COVID-19 after adjusting for age, gender, literacy level, job, economic level, smoking, dietary supplement, drug history, disease history, BMI, and physical activity. In the cases of skewed distribution, log transformation was performed. The regression assumptions including residual normality, residual independence, homogeneity of residual variances, and co-linearity were confirmed by performing a normal probability plot, Durbin-Watson statistics (1.5–2.5), residual vs. predicted value plot, and variance inflation factor (VIF < 5). P-values <0.05 were considered statistically significant in all analyses.
Results
Baseline characteristics of patients
The flowchart of the study process is shown in Figure 1. Finally, 500 patients were examined. As shown in Table 1, patients in the severe and ICU groups had higher age, weight, and body mass index (BMI) compared to the moderate and mild groups (P < 0.05). Moreover, the grouping of patients based on the DII and E-DII quartiles showed that patients with increasing age (mean age 40.33 years in quartile I vs. 45.2 years in quartile IV), weight (mean weight 72.27 kg in quartile I vs. 75.44 kg in quartile IV), and BMI (mean BMI 24.85 kg/m2 in quartile I vs. 26.22 kg/m2 in quartile IV) were placed in a higher subgroup of the DII quartile (P < 0.05). There was a similar trend for the E-DII quartiles (P < 0.05). There were no significant differences between patients with different disease severity in terms of gender and smoking status (Table 1). These findings were shown for patients in different quartiles of DII and E-DII (P > 0.05). As reported in Table 1, 25.5% of ICU patients with COVID-19 had a medical history such as heart disease, hypertension, diabetes mellitus, fatty liver disease, hypothyroidism, anemia, asthma, and depression; however, this rate was 13.8% in mild patients (P < 0.05). This trend was similar in DII (P = 0.003) and E-DII quartiles (P = 0.001); therefore, patients in the highest quartiles of DII (29.6% vs. 11.2%) and E-DII (32% vs. 11.2%) had the highest rate of disease history compared to the lowest quartiles. Moreover, ICU patients with COVID-19 had the highest rate of medication use (22.4% vs. 11.3%) compared to mild patients (Table 1). In the DII and E-DII quartiles, there were no significant differences in terms of dietary supplement history (P > 0.05).
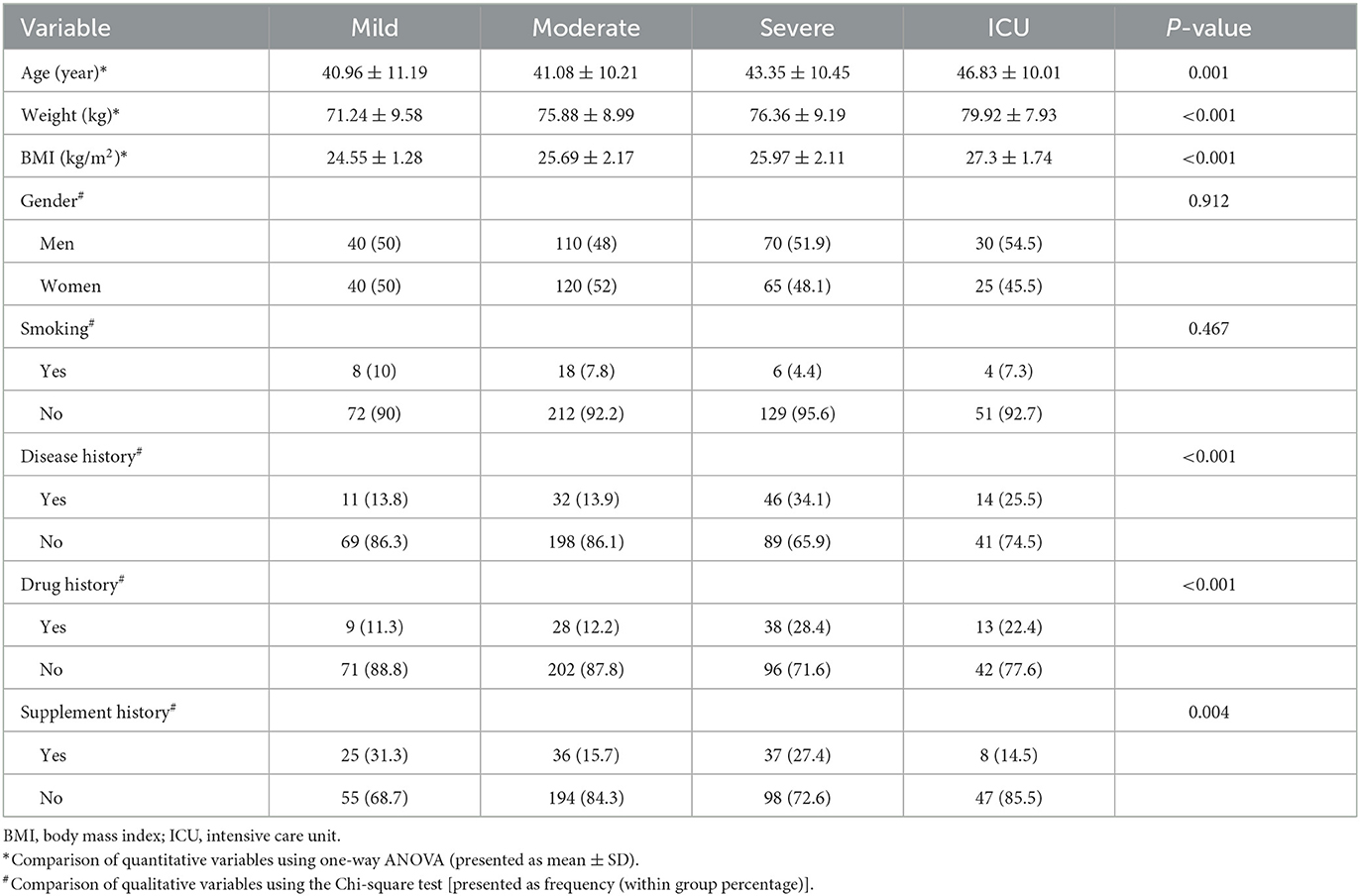
Table 1. Comparison of baseline characteristics between different stages of COVID-19 (Ntotal = 500).
Relationship between biochemical variables, dietary factors, and disease severity, and DII and E-DII
As reported in Tables 2, 3, patients with COVID-19 in quartile IV of DII and E-DII significantly had higher CRP, neutrophil percent, and NLR and lower lymphocyte percent (P < 0.05). For WBC, a significant difference was shown only in the DII quartiles. Post-hoc analyses based on Tukey's test showed that all subgroups had significant differences two by two.
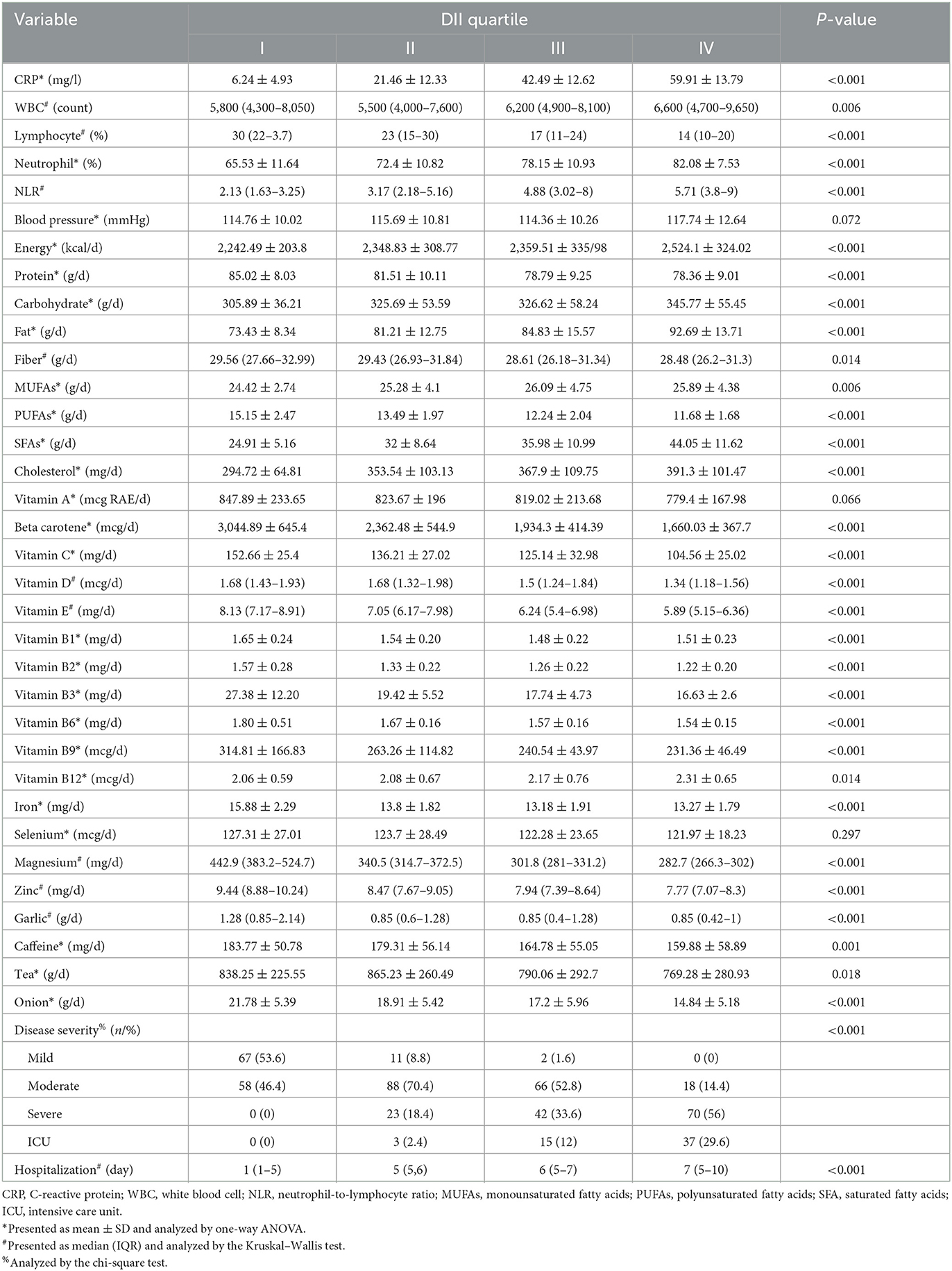
Table 2. Biochemical measures, dietary factors, and disease severity among patients with different DII quartiles.
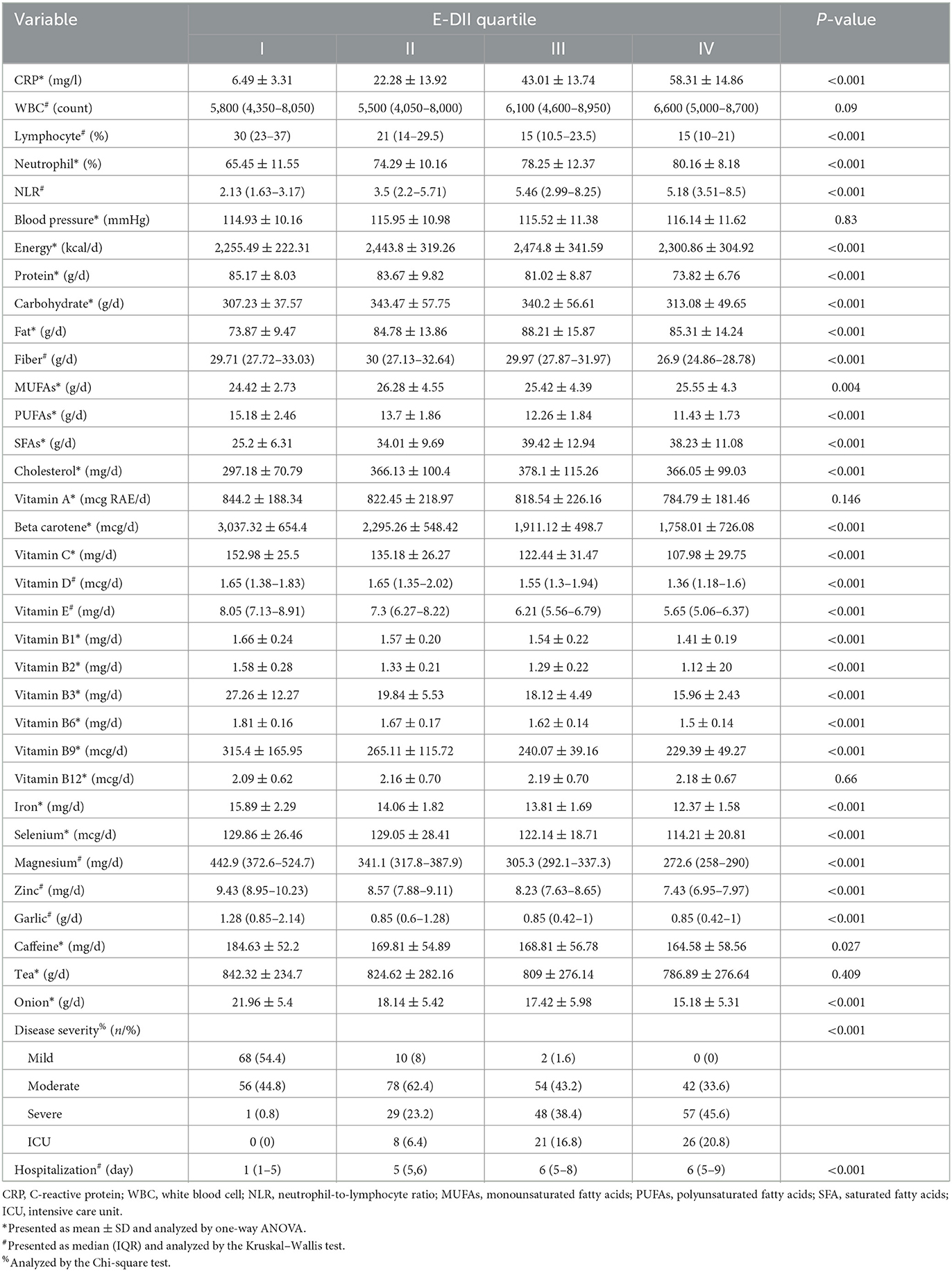
Table 3. Biochemical measures, dietary factors, and disease severity among the energy-adjusted dietary inflammatory index (E-DII) quartiles.
In terms of macronutrients and energy, patients with COVID-19 in quartile IV of DII had the highest consumption of energy, fat, and carbohydrate and the lowest protein intake (P < 0.05) (Table 2). Post hoc analysis revealed that there were no significant differences between quartiles 2 and 3 in terms of energy and macronutrients. Despite the significant difference, there is no clear trend for energy and macronutrient values in the E-DII quartiles (Table 3). However, by decreasing the consumption of anti-inflammatory nutrients (vitamin D, vitamin E, vitamin A, vitamin C, beta-carotene, magnesium, zinc, selenium, PUFAs, B1, B2, B3, and B6), garlic, caffeine, tea, onion, and fiber, the DII and E-DII values increased (Tables 2, 3). Vitamin B12 intake was not different among the E-DII quartiles (P = 0.66) (Table 3). However, with the increase in the DII quartiles, B12 intake increased significantly (P = 0.014) (Table 2). Iron and vitamin B9 intakes were significantly higher in the first DII and E-DII quartiles (Table 2). However, post-hoc analysis revealed that iron and vitamin B9 intakes in other quartiles of DII were not significantly different. The median day of hospitalization in quartile IV of DII and E-DII was 7 and 6 days, respectively. However, the median day of hospitalization in the first quartile of DII and E-DII was only one day (P < 0.05) (Tables 2, 3). Most of the patients with mild and moderate COVID-19 had low DII and E-DII. However, ICU patients and patients with severe COVID-19 consumed a diet with higher DII and E-DII (P < 0.05) (Tables 2, 3).
Based on correlation tests, significant positive correlations were found between CRP, neutrophil, WBC, NLR, hospitalization, BMI, weight, B12, MUFAs, SFA, energy, cholesterol, fat, carbohydrate intakes, and DII values. However, significant negative correlations were reported between DII values and lymphocyte, intakes of anti-inflammatory nutrients, iron, protein, garlic, caffeine, onion, and fiber intakes (P < 0.05) (Supplementary Table 1). Significant relationships regarding vitamin A, vitamin B12, WBC, carbohydrates, and MUFAs disappeared following energy adjustment for DII (Supplementary Table 2).
The results of the multiple linear regression found a positive association between DII and CRP (β = 1.024, P < 0.001), hospitalization (β = 1.062, P < 0.001), WBC count (β = 0.486, P < 0.009), neutrophil count (β = 0.565, P < 0.001), and neutrophil-to-lymphocyte ratio (β = 0.538, P < 0.001) and a negative association between DII and the lymphocyte count (β = −0.569, P < 0.001) in patients with COVID-19 after adjusting for age, gender, literacy level, job, economic level, smoking, dietary supplement, drug history, disease history, BMI, and physical activity (Table 4). Regarding the association of E-DII with inflammatory and immune system biomarkers, a positive association was found between E-DII and hospitalization (β = 1.645, P < 0.001), WBC count (β = 0.417, P < 0.02), and neutrophil-to-lymphocyte ratio (β = 0.35, P < 0.03) in patients with COVID-19 after adjusting for age, gender, literacy level, job, economic level, smoking, dietary supplement, drug history, disease history, BMI, and physical activity (Table 5).
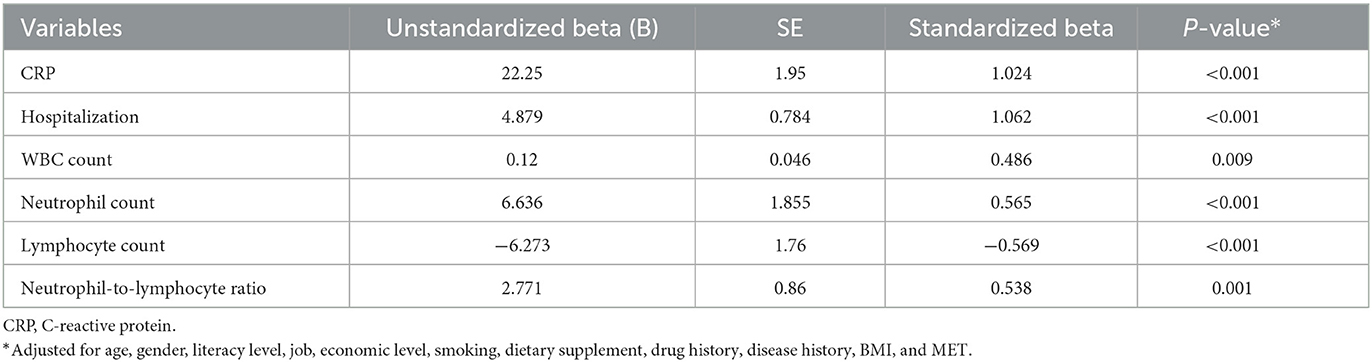
Table 4. Association of inflammatory and immune system biomarkers with dietary inflammatory index (DII) in patients with COVID-19 based on multiple linear regression.
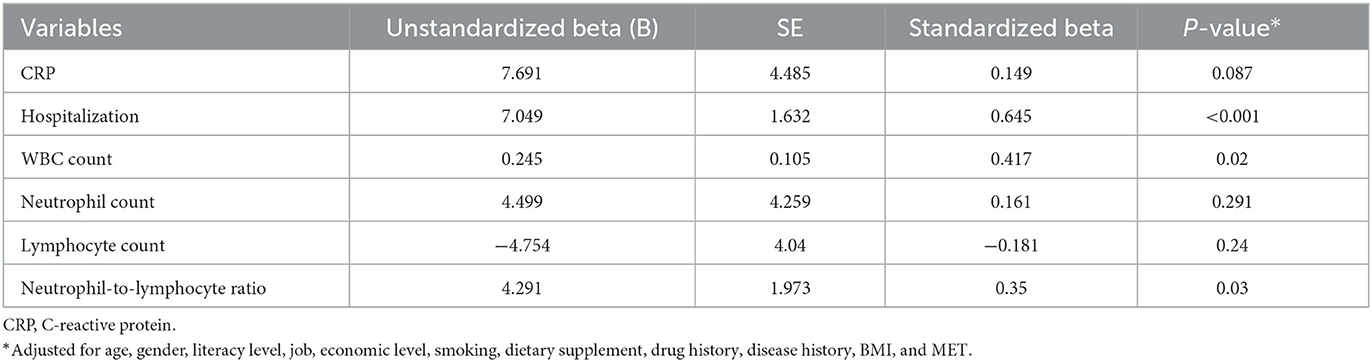
Table 5. Association of inflammatory and immune system biomarkers with energy-adjusted dietary inflammatory index (E-DII) in patients with COVID-19 based on multiple linear regression.
Discussion
This study aimed to evaluate the association between DII and inflammatory biomarkers, immune system biomarkers, and disease severity in patients with COVID-19. Our results showed that there were positive significant relationships between DII and CRP, hospitalization, neutrophil, WBC, NLR, and disease severity. Similar to our results, various observational studies have reported that higher DII increases the risk of lung-related inflammatory conditions such as asthma (36, 37) and COVID-19 (13, 38). Similarly, studies conducted on other health conditions have reported positive associations between CRP and immune system biomarkers with DII values (39, 40). In terms of hospitalization, DII and E-DII values were positively related to hospitalization. In line with our findings, DII was a determinant of hospitalization length in other diseases (41, 42).
In a mechanistic review study conducted by Maiorino et al. (43), the proposed mechanisms of the beneficial effect of the Mediterranean dietary pattern (including whole grains, vegetables and fruits, fish, and “healthy” fats, including MUFA and PUFA) for controlling COVID-19 were inhibition of inflammation caused by COVID-19, characterized by increased CRP, TNF-α, and interleukin-6, as well as infection through supplying of vitamin D, which reduces the expression of angiotensin-converting enzyme 2 (ACE2) receptor and thus decreases the entry of pathogens into lung cells. Finally, it was suggested that prospective studies must be conducted to prove the beneficial effect of the Mediterranean dietary pattern on the control of COVID-19 infection in large populations (43). Butler et al. suggested that following a Western diet that was associated with more consumption of red meats, saturated fats, and refined sugars leads to activation of the innate immune system and impairment of acquired immunity, subsequently leading to chronic inflammation and disruption of the host's defense against viruses (44).
Our results showed that higher intakes of anti-inflammatory micro-nutrients (vitamins A, D, and E as fat-soluble vitamins, vitamins C, B1, B2, B3, B6, and B9 as water-soluble vitamins, beta-carotene, iron, magnesium, zinc, and selenium) led to the lower DII and E-DII values. Similarly, Özbey et al. reported that intakes of vitamins A, E, and C had a negative relationship with DII values (36). Kim et al. also demonstrated that dietary vitamins A, D, E, C, B1, B2, B3, B6, and B9, and beta-carotene, zinc, selenium, and magnesium were negatively associated with DII values (45).
Different studies have investigated the anti-inflammatory mechanisms of vitamin D (46), vitamin E (47), vitamin A (48), vitamin C (49), beta-carotene (50), magnesium (51), zinc (52), selenium (53), and B vitamins (54). However, an overload of some minerals such as iron leads to inflammatory responses (55). Some anti-inflammatory features have been proposed for vitamin B12 in COVID-19 (56). An insignificant relationship between E-DII and vitamin B12 in our analysis showed that a significant positive association between dietary vitamin B12 and DII might be biased and an energy-adjusted correlation could fix this bias. Moreover, there was a positive correlation between the CRP level and the B12 level in the first 2 days of ICU admission (57). Therefore, additional studies must be done to clear the association between B12 and inflammation in patients with COVID-19.
In terms of macronutrients, negative relationships between protein, PUFA, and fiber intakes with DII and E-DII values were shown in our study. However, energy, SFA, cholesterol, fat, and carbohydrate intakes resulted in higher DII and E-DII values. Dietary intake of MUFAs was only positively related to DII and not E-DII. In a study by Aghababayan et al., only dietary intakes of total fat, cholesterol, and SFA were significantly higher in the upper tertile of DII (58). In another study, dietary intakes of carbohydrates, fibers, and PUFAs were higher in the lowest tertile of DII; however, dietary intakes of cholesterol, SFAs, and MUFAs were higher in the third quartile of DII (59).
The anti-inflammatory effects of PUFAs have been described in another study (60). Short-chain fatty acids (SCFAs) produced by microflora in the intestinal duct are the main contributors to reduced inflammation following fiber intake (61). In a large cohort study, higher protein intake, particularly from plant-based foods, was inversely associated with inflammation (62). However, Koelman et al. reported that both high-protein and low-protein diets lead to a decrease in the CRP level. However, this decrease in the high-protein group was more pronounced (63). High dietary methionine content was reported to be associated with higher inflammation (64, 65). Most of the studies that addressed the positive effect of high-protein intake on inflammation have pointed to the high consumption of protein from plant or dairy sources (66, 67). However, the positive effect of dairy products may be related to the bacterial content of fermented dairy products or bioactive peptides (68). Various studies have reported the beneficial effect of MUFAs on inflammation (69, 70). The positive association between MUFAs and DII values in the current study was lost by adjusting the effect of energy. As a result, the positive relationship between MUFAs and DII could be mainly due to the confounding effect of energy consumption on MUFA intake. Numerous studies have demonstrated that SFAs induce adipose tissue inflammation mediated by toll-like receptors (TLRs) as the main receptors in the innate immune system. It has been suggested that this stimulation is mediated by the SFA effect on TLR receptor numbers and dimerization, the balance between SFAs and USFA, and lipid rafts (71). In vivo studies have revealed that high dietary cholesterol leads to increased adipocyte size, expression of pro-inflammatory cytokines, and macrophage infiltration (72, 73). Karimi et al. reported that high carbohydrate intake had a positive association with monocyte chemoattractant protein-1 (MCP-1) and transforming growth factor beta (TGF-β) that were related to increased expression of NF-κB as the main regulator of pro-inflammatory cytokine expression (74).
Our investigation showed that dietary intakes of garlic, onion, caffeine, and tea were also negatively related to DII and E-DII values. These functional foods may have anti-inflammatory effects due to their flavonoid components (75, 76).
It must be noted that the dietary patterns of people during the COVID-19 pandemic were changed due to quarantine rules. In a study by Górnicka et al. in Poland, 34%, 33%, and 18% of respondents reported an increase in total food, sweets, and alcohol consumption, respectively. A total of 53% of respondents did not change their eating habits during the COVID-19 pandemic (77). Sidor et al. reported an increase in total energy intake in people from Poland during the COVID-19 quarantine, which was associated with less consumption of vegetables, fruits, and legumes and a tendency to consume more meat, dairy, and fast foods (78). Hu et al. reported that only 30% of people in China increased vegetable and fruit consumption during the COVID-19 quarantine (79).
Regression analysis was implemented to explore potential predictors of CRP and blood cell counts. Possessing higher DII and E-DII values, being of the male gender, having higher education, and being a smoker were associated with higher CRP in patients with COVID-19. Lee et al. reported that men have higher CRP levels than women (80). However, this trend is ethnically specific and does not generalize to the entire population (81). Consistent with our results, large observational studies also found that socioeconomic status is associated with CRP levels (82–84). We also found that DII, gender, and hospitalization were the common main predictors of blood cell counts. Having higher DII, being of the male gender, and having a longer hospitalization led to higher WBC and neutrophil counts and NLR but a lower lymphocyte count in patients with COVID-19. A long hospitalization period can lead to unwanted acute hospital infections, which affect the immune system and inflammation.
There were some limitations in the present study. First, it would be better to conduct a case–control design to obtain more reliable results. However, healthy individuals to be included in the control group may have been infected with COVID-19, and there was no possibility of performing high-volume PCR testing. Second, only CRP was tested as the main biomarker of systemic inflammation due to the limited budget allocated to the project. Further studies should include other inflammatory biomarkers such as pro-inflammatory cytokines. Third, limitations of the FFQ including the limited structure and response options, and reliance on respondents' memory should be considered (85).
Conclusion
A positive correlation between DII and E-DII with hyperactivation of the inflammatory and innate immune system and length of hospitalization in patients with COVID-19 was obtained from the present study. However, higher DII and E-DII led to dysfunction in the acquired immune system. The number of patients with severe COVID-19 was higher in the upper DII and E-DII quartiles. More intakes of anti-inflammatory nutrients, protein, garlic, onion, caffeine, tea, and fiber led to lower DII and E-DII values. In contrast, SFA, cholesterol, fat, and carbohydrate intakes led to higher DII and E-DII values. Dietary intake of MUFAs was only positively related to DII and not E-DII.
Data availability statement
The datasets presented in this article are not readily available because this research is complied with the standards of the Declaration of Helsinki and current ethical guidelines. Requests to access the datasets should be directed to SD, https://orcid.org/0000-0002-6769-1577.
Ethics statement
The studies involving human participants were reviewed and approved by the Ethics Committee of Urmia University of Medical Sciences, Urmia, Iran (Ethics Code IR.UMSU.REC.1399.367). The patients/participants provided their written informed consent to participate in this study.
Author contributions
All authors listed have made a substantial, direct, and intellectual contribution to the work and approved it for publication.
Conflict of interest
The authors declare that the research was conducted in the absence of any commercial or financial relationships that could be construed as a potential conflict of interest.
Publisher's note
All claims expressed in this article are solely those of the authors and do not necessarily represent those of their affiliated organizations, or those of the publisher, the editors and the reviewers. Any product that may be evaluated in this article, or claim that may be made by its manufacturer, is not guaranteed or endorsed by the publisher.
Supplementary material
The Supplementary Material for this article can be found online at: https://www.frontiersin.org/articles/10.3389/fnut.2023.1075061/full#supplementary-material
References
1. Doaei S, Gholami S, Rastgoo S, Gholamalizadeh M, Bourbour F, Bagheri SE, et al. The effect of omega-3 fatty acid supplementation on clinical and biochemical parameters of critically ill patients with COVID-19: a randomized clinical trial. J Transl Med. (2021) 19:1–9. doi: 10.1186/s12967-021-02795-5
2. Krishnan A, Hamilton JP, Alqahtani SA, Woreta TA. COVID-19: an overview and a clinical update. World J Clin Cases. (2021) 9:8–23. doi: 10.12998/wjcc.v9.i1.8
3. Wang J, Yang X, Li Y, Huang J-A, Jiang J, Su N. Specific cytokines in the inflammatory cytokine storm of patients with COVID-19-associated acute respiratory distress syndrome and extrapulmonary multiple-organ dysfunction. Virol J. (2021) 18:1–12. doi: 10.1186/s12985-021-01588-y
4. Gao Yd, Ding M, Dong X, Zhang JJ, Kursat Azkur A, Azkur D, et al. Risk factors for severe and critically ill COVID-19 patients: a review. Allergy. (2020) 76:428–55. doi: 10.1111/all.14657
5. Wendel Garcia PD, Fumeaux T, Guerci P, Heuberger DM, Montomoli J, Roche-Campo F, et al. Prognostic factors associated with mortality risk and disease progression in 639 critically ill patients with COVID-19 in Europe: initial report of the international RISC-19-ICU prospective observational cohort. eClinicalMedicine. (2020) 25:100449. doi: 10.1016/j.eclinm.2020.100449
6. Williamson EJ, Walker AJ, Bhaskaran K, Bacon S, Bates C, Morton CE, et al. Factors associated with COVID-19-related death using OpenSAFELY. Nature. (2020) 584:430–6. doi: 10.1038/s41586-020-2521-4
7. Grant WB, Lahore H, McDonnell SL, Baggerly CA, French CB, Aliano JL, et al. Evidence that vitamin D supplementation could reduce risk of influenza and COVID-19 infections and deaths. Nutrients. (2020) 12:988. doi: 10.3390/nu12040988
8. Klang E, Kassim G, Soffer S, Freeman R, Levin MA, Reich DL. Severe obesity as an independent risk factor for COVID-19 mortality in hospitalized patients younger than 50. Obesity (Silver Spring). (2020) 28:1595–9. doi: 10.1002/oby.22913
9. Bousquet J, Anto JM, Iaccarino G, Czarlewski W, Haahtela T, Anto A, et al. Is diet partly responsible for differences in COVID-19 death rates between and within countries? Clin Transl Allergy. (2020) 10:16. doi: 10.1186/s13601-020-00323-0
10. Haddad C, Bou Malhab S, Sacre H, Salameh P. Smoking and COVID-19: a scoping review. Tob Use Insights. (2021) 14:1179173x21994612. doi: 10.1177/1179173X21994612
11. Liu Y, Yan L-M, Wan L, Xiang T-X, Le A, Liu J-M, et al. Viral dynamics in mild and severe cases of COVID-19. Lancet Infec. (2020) 20:656–7. doi: 10.1016/S1473-3099(20)30232-2
12. Perez-Araluce R, Martinez-Gonzalez M, Fernández-Lázaro C, Bes-Rastrollo M, Gea A, Carlos S. Mediterranean diet and the risk of COVID-19 in the 'Seguimiento Universidad de Navarra'cohort. Clin Nutr. (2021) 41:3061–8. doi: 10.1016/j.clnu.2021.04.001
13. Moludi J, Qaisar SA, Alizadeh M, Jafari Vayghan H, Naemi M, Rahimi A, et al. The relationship between Dietary Inflammatory Index and disease severity and inflammatory status: a case-control study of COVID-19 patients. Brit J Nutr. (2022) 127:773–81. doi: 10.1017/S0007114521003214
14. Ebrahimzadeh A, Taghizadeh M, Milajerdi A. Major dietary patterns in relation to disease severity, symptoms, and inflammatory markers in patients recovered from COVID-19. Front Nutr. (2022) 9:929384. doi: 10.3389/fnut.2022.929384
15. Eva M, Islam K, Afrin M, Islam A, Bayazid B. Role of dietary habit and nutritional status on the severity of COVID-19 treatment: a hospital based cross-sectional study. J Curr Med Pract. 2022:58.
16. Majidi N, Bahadori E, Shekari S, Gholamalizadeh M, Tajadod S, Ajami M, et al. Effects of supplementation with low-dose group B vitamins on clinical and biochemical parameters in critically ill patients with COVID-19: a randomized clinical trial. Exp Rev Anti-infect Ther. (2022) 30:1–7. doi: 10.1080/14787210.2022.2125867
17. Majidi N, Rabbani F, Gholami S, Gholamalizadeh M, BourBour F, Rastgoo S, et al. The effect of Vitamin C on pathological parameters and survival duration of critically ill coronavirus disease 2019 patients: a randomized clinical trial. Front Immun. (2021) 12:717–816. doi: 10.3389/fimmu.2021.717816
18. Hajipour A, Afsharfar M, Jonoush M, Ahmadzadeh M, Gholamalizadeh M, Hassanpour Ardekanizadeh N, et al. The effects of dietary fiber on common complications in critically ill patients; with a special focus on viral infections: a systematic review. Immun Inflamm Dis. (2022) 10:e613. doi: 10.1002/iid3.613
19. Faghfouri AH, Baradaran B, Khabbazi A, Khaje Bishak Y, Zarezadeh M, Tavakoli-Rouzbehani OM, et al. Profiling inflammatory cytokines following zinc supplementation: a systematic review and meta-analysis of controlled trials. Br J Nutr. (2021) 126:1441–50. doi: 10.1017/S0007114521000192
20. Faghfouri AH, Zarrin R, Maleki V, Payahoo L, Khajebishak Y. A comprehensive mechanistic review insight into the effects of micronutrients on toll-like receptors functions. Pharmacol Res. (2020) 152:104619. doi: 10.1016/j.phrs.2019.104619
21. Calder PC, Ahluwalia N, Brouns F, Buetler T, Clement K, Cunningham K, et al. Dietary factors and low-grade inflammation in relation to overweight and obesity. Br J Nutr. (2011) 106 Suppl 3:S5–78. doi: 10.1017/S0007114511005460
22. Zabetakis I, Lordan R, Norton C, Tsoupras A. COVID-19: the inflammation link and the role of nutrition in potential mitigation. Nutrients. (2020) 12:1466. doi: 10.3390/nu12051466
23. Shivappa N, Steck SE, Hurley TG, Hussey JR, Ma Y, Ockene IS, et al. A population-based dietary inflammatory index predicts levels of C-reactive protein in the seasonal variation of blood cholesterol study (SEASONS). Public Health Nutr. (2014) 17:1825–33. doi: 10.1017/S1368980013002565
24. Shivappa N, Hébert JR, Rietzschel ER, De Buyzere ML, Langlois M, Debruyne E, et al. Associations between dietary inflammatory index and inflammatory markers in the Asklepios Study. Brit J Nutr. (2015) 113:665–71. doi: 10.1017/S000711451400395X
25. Vahid F, Bourbour F, Gholamalizadeh M, Shivappa N, Hébert JR, Babakhani K, et al. A pro-inflammatory diet increases the likelihood of obesity and overweight in adolescent boys: a case-control study. Diabetol Metab Syndr. (2020) 12:1–8. doi: 10.1186/s13098-020-00536-0
26. Aminnejad B, Roumi Z, Hasanpour Ardekanizadeh N, Vahid F, Gholamalizadeh M, Kalantari N, et al. Association of dietary antioxidant index with body mass index in adolescents. Obes Sci Pract. (2022) 2022:1–8. doi: 10.1002/osp4.639
27. Wirth MD, Sevoyan M, Hofseth L, Shivappa N, Hurley TG, Hébert JR. The Dietary Inflammatory Index is associated with elevated white blood cell counts in the National Health and Nutrition Examination Survey. Brain Behav Immun. (2018) 69:296–303. doi: 10.1016/j.bbi.2017.12.003
28. Wiersinga WJ, Rhodes A, Cheng AC, Peacock SJ, Prescott HC. Pathophysiology, transmission, diagnosis, and treatment of coronavirus disease 2019 (COVID-19): a review. JAMA. (2020) 324:782–93. doi: 10.1001/jama.2020.12839
29. Visser M, Bouter LM, McQuillan GM, Wener MH, Harris TB. Elevated C-reactive protein levels in overweight and obese adults. JAMA. (1999) 282:2131–5. doi: 10.1001/jama.282.22.2131
30. Gaillard R, Rifas-Shiman SL, Perng W, Oken E, Gillman MW. Maternal inflammation during pregnancy and childhood adiposity. Obesity. (2016) 24:1320–7. doi: 10.1002/oby.21484
31. Woeste G, Müller C, Bechstein WO, Wullstein C. Increased serum levels of C-reactive protein precede anastomotic leakage in colorectal surgery. World J Surg. (2010) 34:140–6. doi: 10.1007/s00268-009-0304-z
32. Shivappa N, Schneider A, Hebert JR, Koenig W, Peters A, Thorand B. Association between dietary inflammatory index, and cause-specific mortality in the MONICA/KORA Augsburg Cohort Study. Eur J Public Health. (2018) 28:167–72. doi: 10.1093/eurpub/ckx060
33. Malekshah A, Kimiagar M, Saadatian-Elahi M, Pourshams A, Nouraie M, Goglani G, et al. Validity and reliability of a new food frequency questionnaire compared to 24 h recalls and biochemical measurements: pilot phase of Golestan cohort study of esophageal cancer. Eur J Clin Nutr. (2006) 60:971–7. doi: 10.1038/sj.ejcn.1602407
34. Shivappa N, Steck SE, Hurley TG, Hussey JR, Hébert JR. Designing and developing a literature-derived, population-based dietary inflammatory index. Public Health Nutr. (2014) 17:1689–96. doi: 10.1017/S1368980013002115
35. Mishra P, Pandey CM, Singh U, Gupta A, Sahu C, Keshri A. Descriptive statistics and normality tests for statistical data. Ann Card Anaesth. (2019) 22:67–72. doi: 10.4103/aca.ACA_157_18
36. Özbey Ü, Uçar A, Shivappa N, Hebert JR. The relationship between dietary inflammatory index, pulmonary functions and asthma control in asthmatics. Iran J Allergy Asthma Immunol. (2019) 18:605–14. doi: 10.18502/ijaai.v18i6.2173
37. Wood LG, Shivappa N, Berthon BS, Gibson PG, Hebert JR. Dietary inflammatory index is related to asthma risk, lung function and systemic inflammation in asthma. Clin Exp Allergy. (2015) 45:177–83. doi: 10.1111/cea.12323
38. Firoozi D, Masoumi SJ, Ranjbar S, Shivappa N, Hebert JR, Zare M, et al. The association between energy-adjusted dietary inflammatory index, body composition, and anthropometric indices in COVID-19-infected patients: a case-control study in Shiraz, Iran. Int J Clin Pract. (2022) 2022:5452488. doi: 10.1155/2022/5452488
39. Phillips CM, Shivappa N, Hébert JR, Perry IJ. Dietary inflammatory index and biomarkers of lipoprotein metabolism, inflammation and glucose homeostasis in adults. Nutrients. (2018) 10:1033. doi: 10.3390/nu10081033
40. Ren Z, Zhao A, Wang Y, Meng L, Szeto IM-Y, Li T, et al. Association between dietary inflammatory index, C-reactive protein and metabolic syndrome: a cross-sectional study. Nutrients. (2018) 10:831. doi: 10.3390/nu10070831
41. Shivappa N, Hébert JR, Veronese N, Caruso MG, Notarnicola M, Maggi S, et al. The relationship between the dietary inflammatory index (DII(®)) and incident depressive symptoms: a longitudinal cohort study. J Affect Disord. (2018) 235:39–44. doi: 10.1016/j.jad.2018.04.014
42. Galas A, Kulig P, Kulig J. Dietary inflammatory index as a potential determinant of a length of hospitalization among surgical patients treated for colorectal cancer. Eur J Clin Nutr. (2014) 68:1168–74. doi: 10.1038/ejcn.2014.120
43. Maiorino MI, Bellastella G, Longo M, Caruso P, Esposito K. Mediterranean diet and COVID-19: hypothesizing potential benefits in people with diabetes. Front Endocrinol. (2020) 11:574315. doi: 10.3389/fendo.2020.574315
44. Butler MJ, Barrientos RM. The impact of nutrition on COVID-19 susceptibility and long-term consequences. Brain Behav Immun. (2020) 87:53–4. doi: 10.1016/j.bbi.2020.04.040
45. Kim D, Park Y. Association between the dietary inflammatory index and risk of frailty in older individuals with poor nutritional status. Nutrients. (2018) 10:1363. doi: 10.3390/nu10101363
46. Laird E, Rhodes J, Kenny RA. Vitamin D and inflammation: potential implications for severity of Covid-19. Ir Med J. (2020) 113:81.
47. Mosca A, Crudele A, Smeriglio A, Braghini MR, Panera N, Comparcola D, et al. Antioxidant activity of hydroxytyrosol and vitamin E reduces systemic inflammation in children with paediatric NAFLD. Dig Liver Dis. (2021) 53:1154–8. doi: 10.1016/j.dld.2020.09.021
48. Reifen R. Vitamin A as an anti-inflammatory agent. Proc Nutr Soc. (2002) 61:397–400. doi: 10.1079/PNS2002172
49. Ellulu MS, Rahmat A, Patimah I, Khaza'ai H, Abed Y. Effect of vitamin C on inflammation and metabolic markers in hypertensive and/or diabetic obese adults: a randomized controlled trial. Drug Des Devel Ther. (2015) 9:3405–12. doi: 10.2147/DDDT.S83144
50. Kang H, Kim H. Astaxanthin and β-carotene in Helicobacter pylori-induced gastric inflammation: a mini-review on action mechanisms. J Cancer Prev. (2017) 22:57. doi: 10.15430/JCP.2017.22.2.57
51. Nielsen FH. Magnesium deficiency and increased inflammation: current perspectives. J Inflamm Res. (2018) 11:25. doi: 10.2147/JIR.S136742
52. Zarezadeh M, Faghfouri AH, Aghapour B, Rostamkhani H, Malekahmadi M, Naemi Kermanshahi M, et al. Investigation of the clinical efficacy of Zn supplementation in improvement of oxidative stress parameters: a systematic review and dose-response meta-analysis of controlled clinical trials. Int J Clin Pract. (2021) 75:e14777. doi: 10.1111/ijcp.14777
53. Duntas L. Selenium and inflammation: underlying anti-inflammatory mechanisms. Horm Metab Res. (2009) 41:443–7. doi: 10.1055/s-0029-1220724
54. Spinas E, Saggini A, Kritas S, Cerulli G, Caraffa A, Antinolfi P, et al. Crosstalk between vitamin B and immunity. J Biol Regul Homeost Agents. (2015) 29:283–8. doi: 10.6084/M9.FIGSHARE.3860199.V1
55. Wessling-Resnick M. Iron homeostasis and the inflammatory response. Annu Rev Nutr. (2010) 30:105–22. doi: 10.1146/annurev.nutr.012809.104804
56. dos Santos LMJ. Can vitamin B12 be an adjuvant to COVID-19 treatment? GSC Biol Pharmaceut Sci. (2020) 11:001–5. doi: 10.30574/gscbps.2020.11.3.0155
57. Romain M, Sviri S, Linton DM, Stav I, van Heerden PV. The role of Vitamin B12 in the critically ill—a review. Anaesth Intensive Care. (2016) 44:447–52. doi: 10.1177/0310057X1604400410
58. Aghababayan S, Sheikhi Mobarakeh Z, Qorbani M, Tiznobeyk Z, Aminianfar A, Sotoudeh G. Higher dietary inflammatory index scores are associated with increased odds of benign breast diseases in a case-control study. J Inflamm Res. (2020) 13:61–9. doi: 10.2147/JIR.S232157
59. Meneguelli TS, Hinkelmann JV, Novaes J, Rosa CdOB, Filgueiras M, Silveira BKS. Dietary inflammatory index is associated with excessive body weight and dietary patterns in subjects with cardiometabolic risk. J Food Nutr Res. (2019) 7:491–9. doi: 10.12691/jfnr-7-7-2
60. Kavyani Z, Musazadeh V, Fathi S, Faghfouri AH, Dehghan P, Sarmadi B. Efficacy of the omega-3 fatty acids supplementation on inflammatory biomarkers: an umbrella meta-analysis. Int Immunopharmacol. (2022) 111:109104. doi: 10.1016/j.intimp.2022.109104
61. Swann OG, Kilpatrick M, Breslin M, Oddy WH. Dietary fiber and its associations with depression and inflammation. Nutr Rev. (2019) 78:394–411. doi: 10.1093/nutrit/nuz072
62. Hruby A, Jacques PF. Dietary protein and changes in biomarkers of inflammation and oxidative stress in the Framingham heart study offspring cohort. Curr Dev Nutr. (2019) 3:nzz019. doi: 10.1093/cdn/nzz019
63. Koelman L, Markova M, Seebeck N, Hornemann S, Rosenthal A, Lange V, et al. Effects of high and low protein diets on inflammatory profiles in people with morbid obesity: a 3-week intervention study. Nutrients. (2020) 12:3636. doi: 10.3390/nu12123636
64. Bárcena C, Quirós PM, Durand S, Mayoral P, Rodríguez F, Caravia XM, et al. Methionine restriction extends lifespan in progeroid mice and alters lipid and bile acid metabolism. Cell Rep. (2018) 24:2392–403. doi: 10.1016/j.celrep.2018.07.089
65. Sharma S, Dixon T, Jung S, Graff EC, Forney LA, Gettys TW, et al. Dietary methionine restriction reduces inflammation independent of FGF21 action. Obesity (Silver Spring). (2019) 27:1305–13. doi: 10.1002/oby.22534
66. Zemel MB, Sun X, Sobhani T, Wilson B. Effects of dairy compared with soy on oxidative and inflammatory stress in overweight and obese subjects. Am J Clin Nutr. (2010) 91:16–22. doi: 10.3945/ajcn.2009.28468
67. Silveira BKS, Oliveira TMS, Andrade PA, Hermsdorff HHM, Rosa COB, Franceschini S. Dietary pattern and macronutrients profile on the variation of inflammatory biomarkers: scientific update. Cardiol Res Pract. (2018) 2018:4762575. doi: 10.1155/2018/4762575
68. Lomax AR, Calder PC. Probiotics, immune function, infection and inflammation: a review of the evidence from studies conducted in humans. Curr Pharm Des. (2009) 15:1428–518. doi: 10.2174/138161209788168155
69. Ravaut G, Légiot A, Bergeron K-F, Mounier C. Monounsaturated fatty acids in obesity-related inflammation. Int J Mol Sci. (2021) 22:330. doi: 10.3390/ijms22010330
70. Rocha DM, Bressan J, Hermsdorff HH. The role of dietary fatty acid intake in inflammatory gene expression: a critical review. São Paulo Med J. (2017) 135:157–68. doi: 10.1590/1516-3180.2016.008607072016
71. Chait A, Kim F. Saturated fatty acids and inflammation: who pays the toll? Arterioscler Thromb Vasc Biol. (2010) 30:692–3. doi: 10.1161/ATVBAHA.110.203984
72. Subramanian S, Han CY, Chiba T, McMillen TS, Wang SA, Haw A 3rd, et al. Dietary cholesterol worsens adipose tissue macrophage accumulation and atherosclerosis in obese LDL receptor-deficient mice. Arterioscler Thromb Vasc Biol. (2008) 28:685–91. doi: 10.1161/ATVBAHA.107.157685
73. Louie AY, Tingling J, Dray E, Hussain J, McKim DB, Swanson KS, et al. Dietary cholesterol causes inflammatory imbalance and exacerbates morbidity in mice infected with influenza A virus. J Immunol. (2022) 208:2523–39. doi: 10.4049/jimmunol.2100927
74. Karimi E, Yarizadeh H, Setayesh L, Sajjadi SF, Ghodoosi N, Khorraminezhad L, et al. High carbohydrate intakes may predict more inflammatory status than high fat intakes in pre-menopause women with overweight or obesity: a cross-sectional study. BMC Res Notes. (2021) 14:279. doi: 10.1186/s13104-021-05699-1
75. Dwyer JT, Peterson J. Tea and flavonoids: where we are, where to go next. Am J Clin Nutr. (2013) 98:1611s−8s. doi: 10.3945/ajcn.113.059584
76. Soto C, González R, Sance M, Galmarini C. Organosulfur and phenolic content of garlic (Allium sativum L.) and onion (Allium cepa L.) and its relationship with antioxidant activity. Acta Horticult. (2016) 2016:277–90. doi: 10.17660/ActaHortic.2016.1143.39
77. Górnicka M, Drywień ME, Zielinska MA, Hamułka J. Dietary and lifestyle changes during COVID-19 and the subsequent lockdowns among Polish adults: a cross-sectional online survey PLifeCOVID-19 study. Nutrients. (2020) 12:2324. doi: 10.3390/nu12082324
78. Sidor A, Rzymski P. Dietary choices and habits during COVID-19 lockdown: experience from Poland. Nutrients. (2020) 12:1657. doi: 10.3390/nu12061657
79. Hu Z, Lin X, Kaminga AC, Xu H. Impact of the COVID-19 epidemic on lifestyle behaviors and their association with subjective well-being among the general population in mainland China: cross-sectional study. J Med Int Res. (2020) 22:e21176. doi: 10.2196/21176
80. Lee YJ, Lee JH, Shin YH, Kim JK, Lee HR, Lee DC. Gender difference and determinants of C-reactive protein level in Korean adults. Clin Chem Lab Med. (2009) 47:863–9. doi: 10.1515/CCLM.2009.196
81. Cartier A, Côté M, Lemieux I, Pérusse L, Tremblay A, Bouchard C, et al. Sex differences in inflammatory markers: what is the contribution of visceral adiposity? Am J Clin Nutr. (2009) 89:1307–14. doi: 10.3945/ajcn.2008.27030
82. Nazmi A, Oliveira IO, Horta BL, Gigante DP, Victora CG. Lifecourse socioeconomic trajectories and C-reactive protein levels in young adults: findings from a Brazilian birth cohort. Soc Sci Med. (2010) 70:1229–36. doi: 10.1016/j.socscimed.2009.12.014
83. Davillas A, Benzeval M, Kumari M. Socio-economic inequalities in C-reactive protein and fibrinogen across the adult age span: findings from Understanding Society. Sci Rep. (2017) 7:2641. doi: 10.1038/s41598-017-02888-6
84. Alley DE, Seeman TE, Ki Kim J, Karlamangla A, Hu P, Crimmins EM. Socioeconomic status and C-reactive protein levels in the US population: NHANES IV. Brain Behav Immun. (2006) 20:498–504. doi: 10.1016/j.bbi.2005.10.003
Keywords: dietary inflammatory index, inflammation, immune system, hospitalization, COVID-19
Citation: Barania Adabi S, Daneghian S, Khalkhali H, Nejadrahim R and Shivappa N (2023) The association between inflammatory and immune system biomarkers and the dietary inflammatory index in patients with COVID-19. Front. Nutr. 10:1075061. doi: 10.3389/fnut.2023.1075061
Received: 20 October 2022; Accepted: 07 February 2023;
Published: 31 March 2023.
Edited by:
Margarida Castell, University of Barcelona, SpainReviewed by:
Roman Acevedo-Espinola, Universidad Peruana de Ciencias Aplicadas, PeruTorsten Bohn, Luxembourg Institute of Health, Luxembourg
Copyright © 2023 Barania Adabi, Daneghian, Khalkhali, Nejadrahim and Shivappa. This is an open-access article distributed under the terms of the Creative Commons Attribution License (CC BY). The use, distribution or reproduction in other forums is permitted, provided the original author(s) and the copyright owner(s) are credited and that the original publication in this journal is cited, in accordance with accepted academic practice. No use, distribution or reproduction is permitted which does not comply with these terms.
*Correspondence: Sevana Daneghian, RGFuZWdoaWFuLnMmI3gwMDA0MDt1bXN1LmFjLmly
†ORCID: Sevana Daneghian orcid.org/0000-0002-6769-1577