- 1Beijing Ditan Hospital, Capital Medical University, Beijing, China
- 2Beijing Fengtai Hospital, Beijing, China
Background: Citrate refers to an anticoagulant agent commonly used in extracorporeal organ support. Its application is limited in patients with liver failure (LF) due to the increased risk of citrate accumulation induced by liver metabolic dysfunction. This systematic review aims to assess the efficacy and safety of regional citrate anticoagulation in extracorporeal circulation for patients with liver failure.
Methods: PubMed, Web of Science, Embase, and Cochrane Library were searched. Studies regarding extracorporeal organ support therapy for LF were included to assess the efficacy and safety of regional citrate anticoagulation. Methodological quality of included studies were assessed using the Methodological Index for Non-randomized Studies (MINORS). Meta-analysis was performed using R software (version 4.2.0).
Results: There were 19 eligible studies included, involving 1026 participants. Random-effect model showed an in-hospital mortality of 42.2% [95%CI (27.2, 57.9)] in LF patients receiving extracorporeal organ support. The during-treatment incidence of filter coagulation, citrate accumulation, and bleeding were 4.4% [95%CI (1.6-8.3)], 6.7% [95%CI (1.5-14.4)], and 5.0% [95%CI (1.9-9.3)], respectively. The total bilirubin(TBIL), alanine transaminase (ALT), aspartate transaminase(AST), serum creatinine(SCr), blood urea nitrogen(BUN), and lactate(LA) decreased, compared with those before the treatment, and the total calcium/ionized calcium ratio, platelet(PLT), activated partial thromboplastin time(APTT), serum potential of hydrogen(pH), buffer base(BB), and base excess(BE) increased.
Conclusion: Regional citrate anticoagulation might be effective and safe in LF extracorporeal organ support. Closely monitoring and timely adjusting during the process could reduce the risk for complications. More prospective clinical trials of considerable quality are needed to further support our findings.
Systematic review registration: https://www.crd.york.ac.uk/prospero/, identifier CRD42022337767.
1. Introduction
Liver failure (LF) is a critical syndrome commonly seen in clinical practice. Liver failure is a severe liver damage caused by a variety of factors, leading to severe impairment or decompensation of synthetic, detoxification, metabolic and biotransformation functions, and appears as a group of clinical syndromes with jaundice, coagulopathy, hepatorenal syndrome, hepatic encephalopathy, ascites and other major manifestations (1). Studies have demonstrated that LF patients are susceptible to acute kidney injury (AKI) that significantly compromises their prognosis (2). A study by Tujios et al. (3), have found that the morbidity of AKI is approximately 70% in LF patients. Multiple organ failure (MOF) is one of the leading causes of death in critical patients, and extracorporeal organ support (ECOS) could be applied for MOF (4). In the past decades, multiple artificial organ support systems have been applied in the treatment of hepatic and renal failure, such as renal replacement therapy (RRT), molecular adsorbent recirculation system (MARS), and plasma exchange (PE). Extracorporeal blood anticoagulation is the prerequisite for the normal functioning of these systems.
Various anticoagulation strategies have been tried in clinical practice over the years (5). Regional citrate anticoagulation (RCA) is clinically applied since the 1980s (6), and has been receiving increasing attentions. It performs the anticoagulant effect in extracorporeal circulation via chelating calcium ions that present to be a key factor in coagulation cascades (7). As a regional anticoagulation strategy, RCA neither affect the systemic pro-coagulation state, nor disturb the fragile balance of pro- and anti-coagulation in LF patients, which makes it a preferable option for LF patients receiving ECOS (8). Multiple studies have revealed that RCA would be significantly beneficial for prolonging the ECOS lifespan and reducing the risk for hemorrhagic complications (9, 10). Several studies indicate the RCA is safe and effective for RRT in patients with hypohepatia (11–13), especially for continuous renal replacement therapy (CRRT) in which RCA has been recommended as the most proper agent for regional anticoagulation (14). It is safe even for those with severe liver dysfunction (15).
However, the use of RCA in ECOS for LF patients remains controversial. Citrate is physiologically degraded, by the mitochondria of hepatic cells, skeletal muscle cells, and glomerular epithelial cells, into bicarbonate (16). Therefore, LF patients have increased risk of citrate accumulation during RCA-involved ECOS, compared with those un-concomitant with liver dysfunction. Citrate accumulation is characterized by internal environment disturbance, such as hypocalcemia, metabolic acidosis or alkalosis, and Catot/Caion ratio over 2.5 (17). Chelation of citrate with serum calcium ions forms calcium citrate, a soluble complex difficult to be disintegrated, which decreases the serum free calcium so that could induce muscle spasm even cardiac dysfunction (18). Anther common complication of citrate accumulation is metabolic disorder, while the reabsorption of intestinal ammonia would be enhanced in LF patients with metabolic alkalosis leading to severe hyperammonemia so that induces or aggravates hepatic encephalopathy (19). Study by Link et al., (17) has shown that citrate accumulation is associated with decreased liver detoxification function, and is an independent risk factor for 28-day mortality. The Kidney Disease Improving Global Outcomes (KDIGO) lists severe LF as one of a contraindication for citrate-involved anticoagulation (20).
There remain controversies in previous articles on the feasibility of RCA for LF. Therefore, we have summarized RCA in multiple types of ECOS, and have systematically reviewed the efficacy and safety of RCA for the treatment of LF. We expect our findings could provide significant reference for the use of RCA in ECOS. The types of ECOS on which this study mainly focuses are RRT, PE, and MARS.
2. Materials and methods
This systematic review and meta-analysis is conducted and reported in accordance with the Preferred Reporting Items for Systematic Reviews and Meta-Analyses (PRISMA2020) Statement (21), and the study protocol has been registered on PROSPERO (registration No. CRD42022337767). Ethical approval is not required in that this is a review of published studies.
2.1. Search strategy
PubMed, Web of Science, Embase, and Cochrane Library were searched, from January 1st, 2000 to March 8th, 2022, for studies regarding RCA-involved extracorporeal organ support for LF. Each database had specific search strategy, and the search items mainly included “anticoagulation”, “citrate”, “liver failure”, “renal replacement therapy”, “molecular adsorbent recirculation system” and “plasma exchange”. The search strategy was adjusted for different databases. Literature search was conducted by two reviewers independently, and disagreements were settled by a third reviewer. The search items were designed based on the combination of medical subject headings and free words. The author information and reference lists of retrieved articles were manually searched for more study information. We had only included published studies, and no language restriction was set.
2.2. Inclusion and exclusion criteria
Study meeting the following criteria would be included:
➀ Case-control study, cohort study, randomized controlled trial (RCT), or single-arm clinical trial.
➁ Patients were diagnosed with LF, including acute LF (ALF) and chronic LF (CLF). ALF is defined as a sudden loss of liver function with no preexistent liver diseases, such as disturbance of consciousness (encephalopathy) and coagulation disorder (typically INR>1.5, not receiving anticoagulants) (22). CLF refers to decompensated liver cirrhosis primarily characterized by ascites, portal hypertension, and hepatic encephalopathy (23).
➂ Participants were aged over 18.
➃ All patients had received RCA-involved ECOS.
➄ Outcome measures included at least one of the follows: filter lifespan, filter blood coagulation, patients‘ survival, incidence of citrate accumulation, incidence of bleeding, changes in liver and renal function indicators, and results of arterial blood gas analysis.
➅ Reported and published in English.
Exclusion criteria were as follows:
➀ Repeated publication.
➁ Other types of study such as animal study, basic research, case-report, literature review, systematic review, letter, conference summary, and study with irrelevant participants or interventions.
➂ Full-text unavailable.
➃ Irrelevant to the efficacy and safety of RCA for LF.
➄ Sample size less than 5.
➅ Data incomplete, missing, or unextractable.
➆ Reported and published in non-English.
2.3. Data extraction
Data extraction was conducted by two reviewers independently. All standards and required data were identified before study selection and data extraction to ensure the extraction consistency between the two reviewers. Any discrepancy would be settled by the third reviewer. Titles and abstracts of retrieved articles were browsed to preliminarily identify potential eligible studies, and the full-texts of remaining articles were read to identify studies to be included. The data extracted included name of the first author, nationality, study-design, inclusion and exclusion criteria, publication date, study periods, sample size, characteristics of participants, interventions, filters per capita using, outcome measures (incidence of citrate accumulation, filter lifespan, and changes in blood calcium concentration, electrolytes, and liver and renal function indicators), patients‘ survival, and complications. Primary outcome was the efficacy, assessed via filter lifespan, filter coagulation, and patients‘ survival. Second outcome was the safety, assessed by types and incidence of adverse events, incidence of citrate accumulation and bleeding, and changes in liver function, renal function, and acid-base indicators.
2.4. Quality assessment
Methodological quality of included studies were assessed using the Methodological Index for Non-randomized Studies (MINORS) (24), and this process was conducted by two reviewers independently. MINORS has 12 items, and each item can be scored for 0 to 2 indicating on report, incomplete report, and sufficient report, respectively. The first 8 items can be scored for a maximum of 16, and a total score of 24 after adding the latter 4 items. The specific MINORS items are as follows: (1) a stated aim of the study, (2) inclusion of consecutive patients, (3) prospective collection of data, (4) endpoint appropriate to the study aim, (5) unbiased evaluation of endpoints, (6) follow-up period appropriate to the major endpoint, (7) loss to follow-up not exceeding 5%, and (8) prospective calculation of the sample size. The latter 4 items are designed for studies that have control group, which are (1) a control group having the gold standard intervention, (2) contemporary groups, (3) baseline equivalence of groups, and (4) statistical analyses adapted to the study design. In this meta-analysis, non-comparative studies scored for less than 8, within 9 to 12, and over 13 would be considered of low, medium, and high quality, respectively. Comparative studies scored for less than 12, within 13 to 18, and over 19 would be considered of low, medium, and high quality, respectively.
2.5. Statistical analysis
All data analyses were performed using R software (version 3.3.3, The R Foundation for Statistical Computing, Austria, Vienna). For continuous variables, the median (IRQ) was transformed in to mean difference (MD) ± standard deviation (SD). For classification variables, the incidence was calculated by dividing the total number of patients by the number of events observed. Fixed-effect model and random-effect model were applied for meta-analysis to pool the effect size (ES) and 95% confidence interval (95%CI). Heterogeneity among included studies was assessed using I2 statistic. Generally, an I2<25% indicates low heterogeneity, 25% < I2 < 50% indicates medium heterogeneity, and an I2>50% indicates significant heterogeneity. Random-effect model would be applied if I2>50%, otherwise, fixed-effect model would be used (25). Subgroup analysis would be performed if there were sufficient data, to identify the potential sources of heterogeneity. Sensitivity analysis would be conducted if necessary to assess the effect of individual study on the pooled size. Publication bias was assessed using funnel plot, Egger‘s test and Begg’s test. Descriptive statistics was applied to analyze characteristics of the participants. A p value less than 0.05 indicated statistical significance.
3. Results
3.1. Study selection
There were 3,951 relevant articles retrieved (821 from PubMed, 896 from WOS, 2103 from Embase, and 131 from Cochrane Library). These articles were screened according to the inclusion and exclusion criteria. There were 824 duplicates and 176 non-English articles removed, and 2,951 remained. After browsing the study-design, titles and abstracts, 2,503 irrelevant studies, 883 animal studies, 47 case-reports, 537 reviews, 50 reviews and meta-analyses, 50 letters and conference summaries were excluded, and 986 studies were removed for other reasons (incomplete and missing data, studies of pathological mechanisms, studies on infants and children, and incomplete full-texts). Then, 448 articles remained for full-text reading, and 429 were excluded. Finally, 19 eligible studies were included (11–13, 26–41). Study selection process are shown in Figure 1.
3.2. Characteristics of included studies
There were 19 eligible studies included in this systematic review and meta-analysis, involving 1,026 participants. Among the included studies, 780 patients of 12 studies had received RCA during RRT, 158 patients of 3 studies received RCA during PE, 79 patients of another 3 studies during MARS. Additionally, 9 patients of 1 study had received RCA during RRT-PE combination treatment. Publication date of these studies ranged from 2005 to 2021, with the sample size varying greatly. Only 2 studies recruited over 100 participants. All included studies were observational design, with 8 prospective studies and 11 retrospective studies. As for the nationality, 7 studies were conducted in German, 3 were in China, and the other 9 were in Italy, France, The United States, Brazil, Turkey, India, Belgium, Austria, and Japan, respectively. All these studies were single-centric. Various geographical regions and intervention regimens might be the source of heterogeneity. The primary tool these studies applied for LF severity assessment was the Model for End-Stage Liver Disease (MELD), and Acute Physiology and Chronic Health Evaluation (APACHE-II) and Sequential Organ Failure Assessment (SOFA) were applied to assess the severity of critical patients. Among the 13 RRT-involved studies, CRRT accounted for nearly 80%. Characteristics of included studies are presented in Table 1.
3.3. Quality assessment
Methodological quality of included studies was assessed using MINORS, and the scores are shown in Table 2. There were 3 non-comparative studies assessed via the 8 items, and the other 16 comparative studies assessed via the 4 items. According to different grading standards, 11 studies were graded as low risk of bias, and the total scores indicated high quality. Another 8 studies were graded as medium quality.
3.4. Study results
3.4.1. Efficacy
Efficacy of RCA for LF was assessed according to the mortality, filter lifespan, and filter coagulation. The primary ECOSs in this study included RRT, PE, and MARS.
3.4.2. Mortality
There were 16 studies that reported mortality, and the mortality endpoint subjected to the in-hospital mortality. The overall mortality was 42.2% [95%CI (27.2, 57.9)] (Figure 2A). Mortality in RRT, PE, and MARS group were 45.9% [95%CI (26.1, 66.4)], 19.4% [95%CI (3.4, 43.2)], and 47.7% [95%CI (24.8, 71.0)], respectively. No statistical difference in the mortality among the 3 groups (Chi2 = 3.68, df = 2, p = 0.16), while significant heterogeneity was observed (Chi2 = 372.44, df = 15, P < 0.01, I2 = 96%).
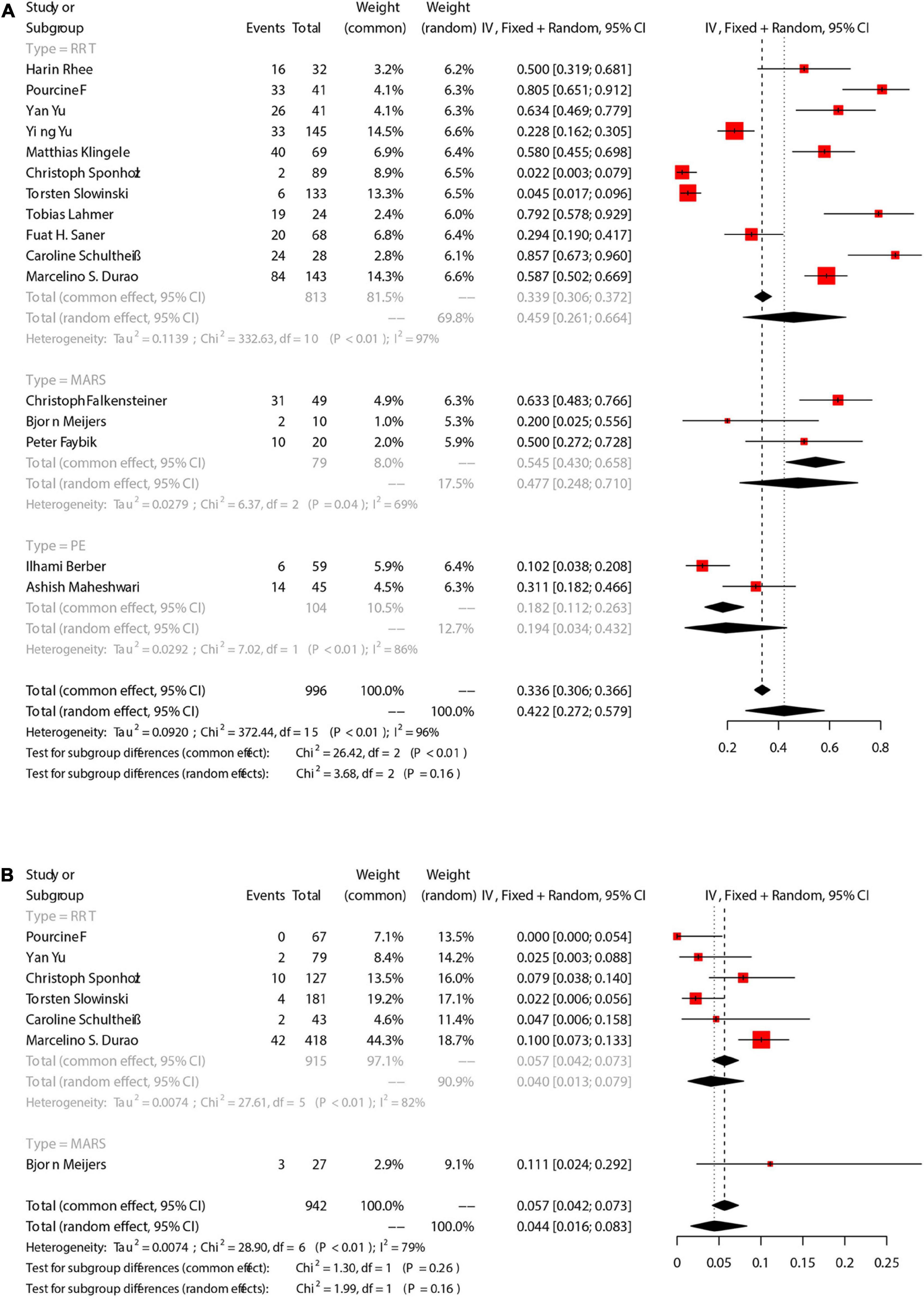
Figure 2. The pooled rates (95% CI) of patient death (A) and filter clotting (B). RRT, renal replacement therapy; MARS, molecular adsorbent recirculation system; PE, plasma exchange; CI, confidence interval.
3.4.3. Filter lifespan
Characteristics of different interventions are shown in Table 3. There were 16 studies that reported the number of times of loop filter, so that the per capita use could be calculated. There are 946 participants involved in the 16 studies, and 3340 sets of loop filter were used (3.5 per capita use). The 11 RRT-involved studies, with 754 patients, had used 2715 sets (3.6 per capita use), and the 3 MARS-involved studies, with 79 patients, had used 179 sets (2.3 per capita use). In addition, 2 studies reported a per capita filter use of 3.9 (446/113) during PE. Among the included studies, 9 reported the average filter lifespan of 37.5 hours. Average filter lifespan was 44.2 h during RRT, 14 during MARS, and not reported during PE. Multiple factors could induce the discontinuation of ECOS such as filter coagulation, Catot/Caion ≥ 2.5, emergency surgery, hemorrhage, machine dysfunction, and clinical decision to discontinue. This would explain the different filter lifespan among individuals.
There were 7 studies that reported filter coagulation during ECOS, in which 6 were related to RRT, and 1 was related to MARS (Figure 2B). The overall incidence of filter coagulation was 4.4% [95%CI (1.6, 8.3)], and the incidence during RRT was 4.0% [95%CI (1.3, 7.9)]. There was no statistical difference in the incidence of filter coagulation between RRT group and MARS group, while significant heterogeneity was observed (Chi2 = 28.90, df = 6, P < 0.01, I2 = 79%).
3.4.4. Safety
Safety was assessed according to the incidence of citrate accumulation and bleeding during ECOS, changes of hepatic and renal function indicators, and changes of electrolytes. Likewise, we focused on RRT, PE, and MARS.
3.4.5. Citrate accumulation
There were 9 studies that reported the number of patients with citrate accumulation, in which 8 was RRT and the other 1 was PE. Citrate accumulation was confirmed according to an Catot/Caion ≥ 2.5. The incidence of citrate accumulation was 6.7% [95%CI (1.5, 14.4), Figure 3A]. Grouping analysis was performed for RRT-involved studies, and the pooled results showed that the incidence of citrate accumulation was 4.7% [95%CI (0.8, 10.8)]. There was only 1 study that reported citrate accumulation during PE, and the incidence was 29.6% [95%CI (18.0, 43.6)]. There was statistical difference in the incidence of citrate accumulation between RRT group and PE group (Chi2 = 15.01, df = 1, p < 0.01), and significant heterogeneity existed between the groups (Chi2 = 71.76, df = 8, P < 0.01, I2 = 89%).
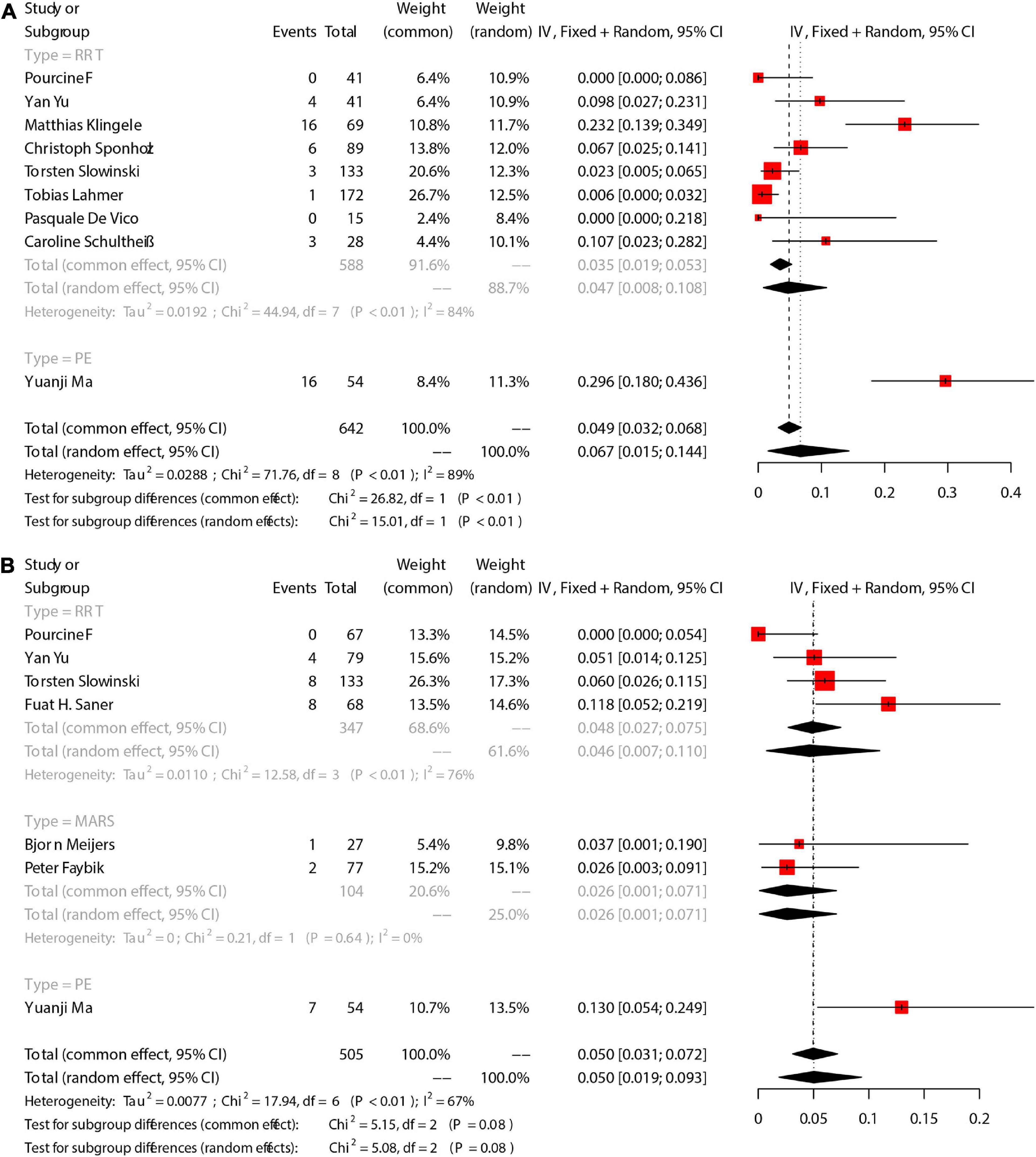
Figure 3. The pooled rates (95% CI) of Citrate accumulation (A) and bleeding (B). RRT, renal replacement therapy; MARS, molecular adsorbent recirculation system; PE, plasma exchange; CI, confidence interval.
On the other hand, 6 studies provided specific changes of Catot/Caion ratio, and we compared the Catot/Caion ratio before ECOS with that after ECOS to further explore the citrate accumulation during RCA. Generally, Catot/Caion ratio slightly increased during ECOS [Mean = 0.141, 95%CI (0.053, 0.229)] (Figure 4). Pooled analysis for 3 RRT-involved studies showed an increased Catot/Caion ratio [Mean = 0.136, 95%CI (0.017, 0.255)], and that for 2 PE-involved studies also showed an increased Catot/Caion ratio [Mean = 0.190, 95%CI (−0.112, 0.492)].
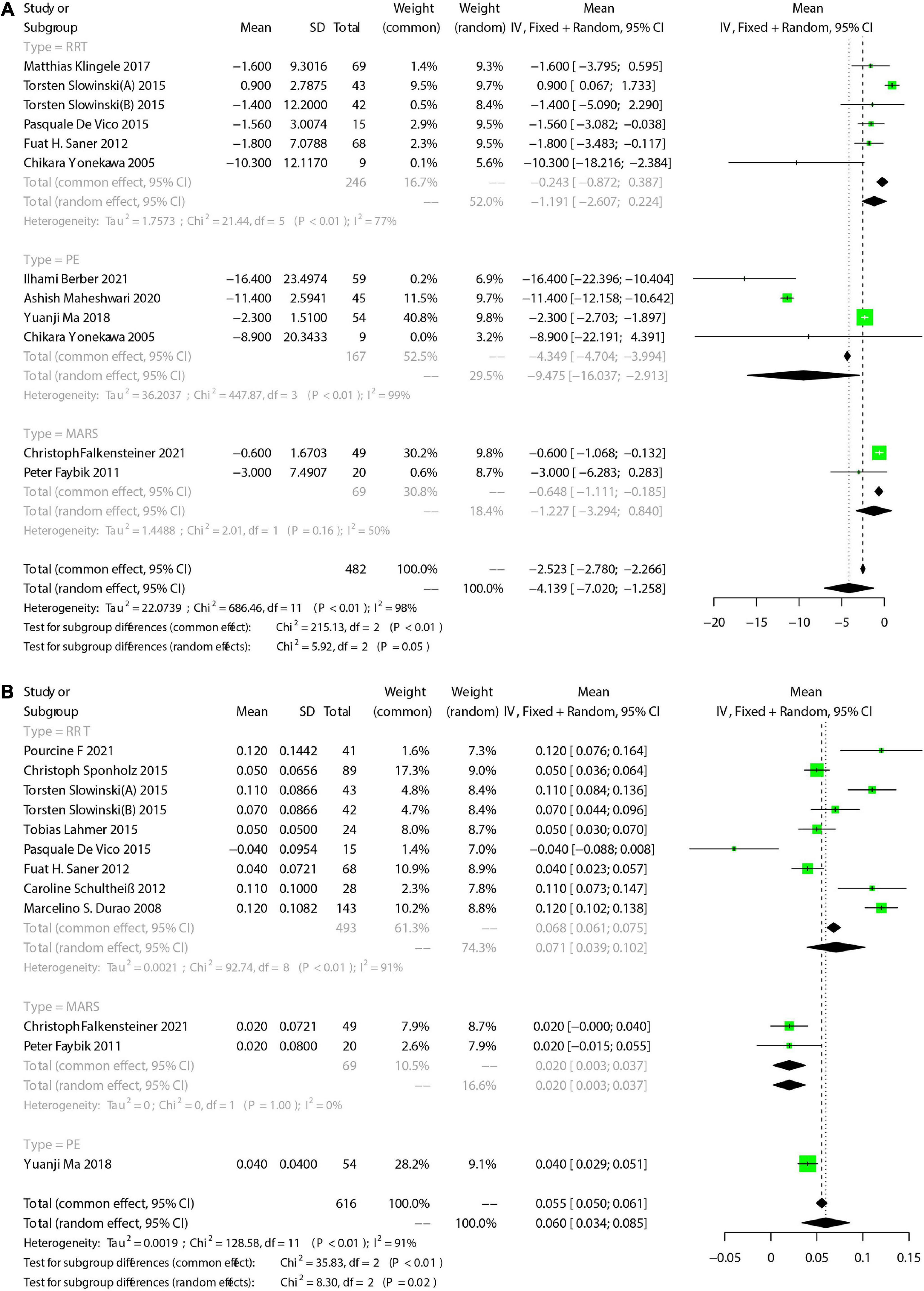
Figure 4. The pooled rates (95% CI) of TBIL (A) and pH (B). RRT, renal replacement therapy; MARS, molecular adsorbent recirculation system; PE, plasma exchange; CI, confidence interval; SD, standard deviation; TBIL, total bilirubin; pH, potential of hydrogen.
3.4.6. Bleeding
There were 7 studies that reported the number of patients with bleeding. The incidence of bleeding was 5.0% [95%CI (1.9, 9.3)] (Figure 3B), and 4 RRT-involved studies showed an incidence of 4.6% [95%CI (0.7, 11)]. Additionally, 2 MARS-involved studies showed an incidence of 2.6% [95%CI (0.1, 7.1)].
3.4.7. Liver function
We focused on total bilirubin (TBIL), alanine transaminase (ALT), and aspartate aminotransferase (AST). There were 11 studies reported TBIL. Meta-analysis showed that the TBIL decreased after ECOS [Mean = −4.139, 95%CI (−7.020, −1.258), Figure 4A]. Subgroup analysis showed no significant change of TBIL in 5 RRT-involved studies [Mean = −1.197, 95%CI (−2.607, 0.224)]. In addition, 2 MARS-involved studies also showed no significant change of TBIL [Mean = −1.227, 95%CI (−3.249, 0.840)]. Pooled 4 PE-involved studies showed a significant decrease of TBIL [Mean = −9.475, 95%CI (−16.037, −2.913)]. We also assessed changes of ALT and AST before and after ECOS, and the results are shown in Table 4. Forest plots are provided in Supplementary Appendix 1, 2.
3.4.8. Renal function
Studies that assessed renal function were RRT-involved. We reviewed data of serum creatinine (SCr) and blood urea nitrogen (BUN), as shown in Table 4. Forest plots are presented in Supplementary Appendix 3, 4. RRT resulted in significantly decreased SCr and BUN.
3.4.9. Coagulation function
Coagulation function-associated indicators were also changed during ECOS. We primarily assessed platelet (PLT), Activated partial thromboplastin time (APTT), and international normalized ratio (INR) (Table 4). There were 7 studies that reported PLT, and the results showed a decrease of PLT after ECOS [Mean = −10.214, 95%CI (−16.865, −3.563), Supplementary Appendix 5]. Subgroup analysis showed that the decrease of PLT was statistically significant only in PE group [Mean = −26.153, 95%CI (−39.229, −13.07)], while not in RRT and MARS group. There were 4 studies that reported the change of APTT, with no statistical significance [Mean = −2.919, 95%CI (−7.659, 1.820), Supplementary Appendix 6]. In addition, 5 studies reported the change of INR, and the pooled results are shown in Table 4.
3.4.10. Acid-base and electrolyte status
The acid-base equilibrium fluctuated during ECOS. Meta-analysis showed that pH [Mean = 0.060, 95%CI (0.034, 0.05), Figure 4B], BE [Mean = 3.284, 95%CI (1.848, 4.719), Supplementary Appendix 7], BE [Mean = 4.501, 95%CI (3.026, 5.976), Supplementary Appendix 8], and SPCO2 [Mean = 0.356, 95%CI (−1.492, 2.205), Supplementary Appendix 9]. The incidence of metabolic acidosis during RCA-ECOS was 22% [95%CI (8.8, 38.8), Supplementary Appendix 10], and that of metabolic alkalosis was 6.5% [95%CI (0.6, 16.9), Supplementary Appendix 11]. Blood lactic acid [Mean = 0.286, 95%CI (-0.793, 0.221), Supplementary Appendix 12]. In addition, we pooled the changes of Na2+, K+, and the incidence of hypercalcemia and hypocalcemia, and the results are shown in Table 4 and Supplementary Appendix 13–16.
3.4.11. Other results
We also assessed the development of sepsis during ECOS and ICU-duration. Meta-analysis showed that the incidence of sepsis during RCA-ECOS was 36.7 [95%CI (24.6, 49.7), Supplementary Appendix 17], and the mean ICU-duration was 16.397 [95%CI (6.789, 26.004), Supplementary Appendix 18].
3.5. Sensitivity analysis and publication bias
We analyzed the sensitivity of the pooling effect of more than 10 articles. The results of sensitivity analysis are shown in Supplementary Appendix 19–22. There was no significant change in the pooled effect, indicating that the results were robust. Publication bias was evaluated using funnel plot and Egger’s test. We assessed the sensitivity and publication bias of the main results of mortality (Figure 5A). A relatively symmetric funnel plot indicated no significant publication bias, which was validated by Egger’s test (p = 0.1025) (Figure 5B). The power of the Egger’s test might be limited if less than 10 studies included, so that the difference between contingency and true asymmetry would not be distinguished. We presented the Egger’s test of more than 10 studies in the Supplementary Appendix 23–26. No potential publication bias was observed in the included studies. Not all tests are available for subset datasets with a small sample size.
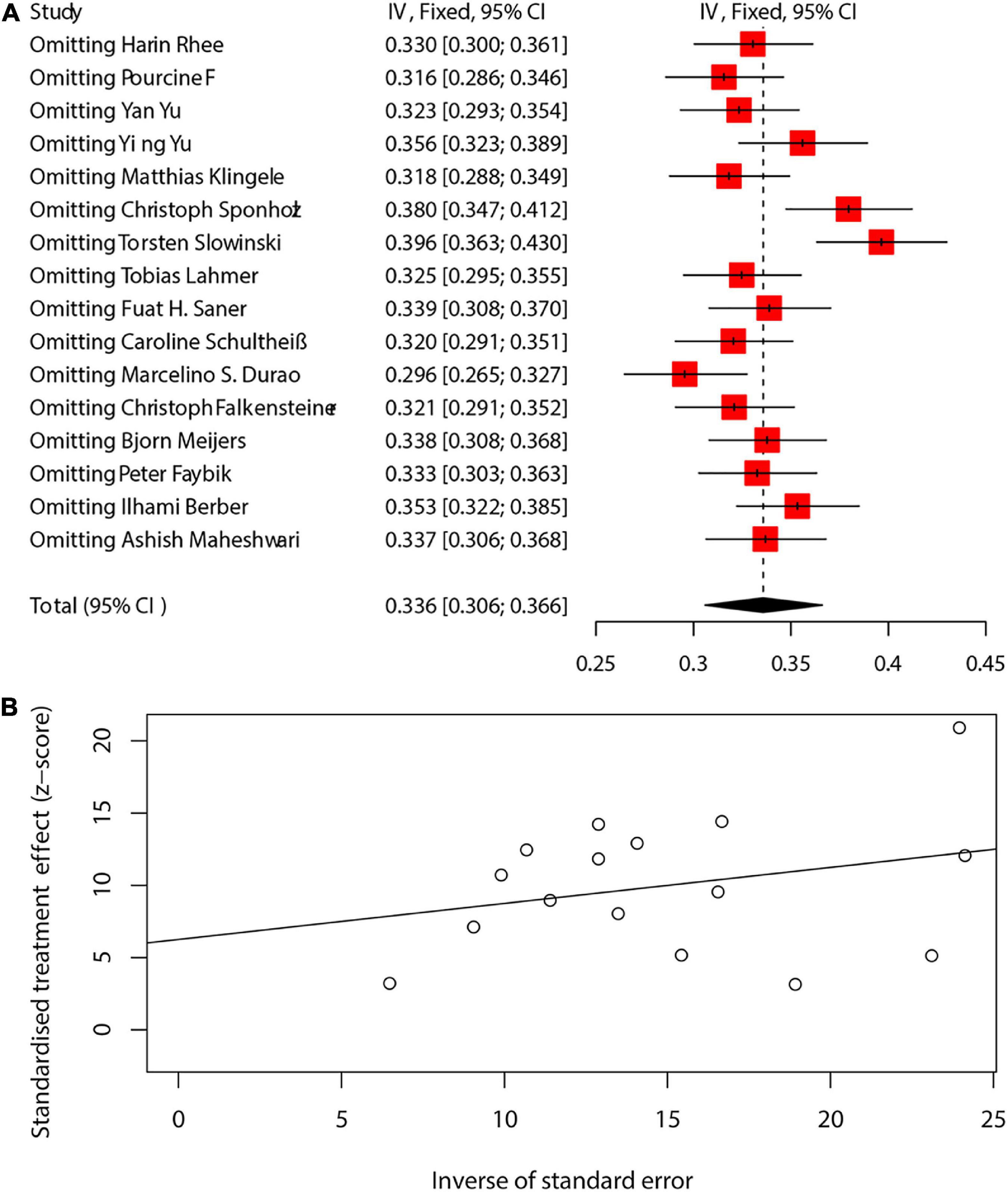
Figure 5. The sensitivity and publication bias of the main results of mortality (A,B). CI, confidence interval.
4. Discussion
Liver failure is a clinical syndrome commonly seen in clinical practice. It is of rapid progression and high mortality (42). The primary treatment strategies include comprehensive physical treatment, artificial liver support, stem cell transplantation, and liver transplantation (42). Studies have shown that the incidence of AKI ranges from 40% to 85% in LF patients (22, 43), and ECOSs such as RRT, PE, and MARS are recommended, for patients with treatment failure or waiting for transplantation, to alleviate the capacity load, remove the solute of small molecules, correct the acid-base imbalance and electrolyte disturbance, so as to stabilize the internal environment. ECOS can create conditions for patients to recover or transition to liver transplantation, combined liver and kidney transplantation and other further treatment. There are multiple patterns of bleeding and coagulation disorders in patients with liver failure. Except calcium ion and coagulation factor VIII, other coagulation factors are synthesized in the liver. In patients with liver insufficiency, the synthesis of coagulation factors and anticoagulants decreased, and the coagulation system of patients with liver failure was rebalanced, but the balance was brittle balance, which was easily broken by hemostasis or anticoagulation therapy, causing bleeding or hypercoagulability (44, 45). Moreover, the contact of blood with biomaterial of filter during ECOS could cause bioincompatible reaction (46), including the activation of coagulation cascade and cell-mediated inflammatory response (47). Therefore, one major problem of ECOS is the anticoagulation in extracorporeal circulation. unfractionated heparin (UFH) and low molecular weight heparin (LMWH) remain the preferred coagulants in most trial centers. However, heparin would probably induce systemic anticoagulation so that aggravate LF-related coagulation disorder (48). In the past years, citrate has become an effective alternative to heparin for ECOS anticoagulation (49).
It presents a challenge for the ECOS anticoagulation in LF patients admitted to ICU. Here we have reviewed the results of 19 studies, in which 12 applied RCA during RRT, 3 during PE, 3 during MARS, and 1 during RRT-PE combination. Citrate is a vital intermediate substance for tricarboxylic acid cycle in mitochondria, and its final metabolites are carbon dioxide and water. Citrate usually exists in mitochondria-rich organs such as liver, kidney, and muscles due to its metabolism demanding oxygen (50). Exogenous citrate serves as a calcium ion chelating agent in anticoagulation, and calcium ion is an important cofactor in coagulation cascade. This makes RCA a highly effective anticoagulant in ECOS. Several studies have found that the use of citrate for anticoagulation in ICU-admitted patients could reduce bleeding-associated complications and prolong the filter lifespan, and might reduce mortality (51) When RCA is used in ECOS, calcium citrate complex can be partially removed by filter, and tricarboxylic acid cycle is mainly carried out in the liver after the rest of the calcium citrate complex enters the body. Therefore, the use of RCA in patients with LF is controversial (52). The reason might be the complexity of citrate. It requires specific citrate solution/alternative solution. One the other hand, LF patients might have increased risk for citrate accumulation and unpredictable metabolic complications (such as acid-base imbalance and electrolyte disturbance).
Liver failure is a heterogeneous disease state, and multiple liver support technologies have been developed to attenuate the accumulation of endogenous toxins so as to improve the outcome of LF (53). Currently, PE and MARS are the most widely applied treatments (54). Moreover, other organ dysfunction often occurs in LF patients, such as previously mentioned end-stage liver disease and liver transplantation. RRT would be necessary in that these complications could cause AKI (55). CRRT is applied in most cases in ICU. It is usually the most preferable RRT for patients with AKI (56). In 2003, a pharmacokinetic study, which involved a cohort of 16 patients with liver cirrhosis, aroused interest in the use of RCA for patients with liver dysfunction (52). RCA-RRT has been acclaimed in recent years (57), and has been recommended by KDIGO guideline for anticoagulation (14, 58, 59). According to the guideline released by European Association for the Study of the Liver (EASL), RRT should be performed as early as possible for patients with sustained hyperammonemia, hyponatremia, metabolic disturbance, and abnormal body temperature (60). Multiple studies have demonstrated the feasibility of RCA in LF patients receiving RRT (12, 27–29). Citrate-based RRT can reduce the risk of bleeding, complications, and extracorporeal coagulation in patients with LF-induced coagulation dysfunction (28), and closely monitoring during RCA-RRT can probably avoid citrate accumulation (12, 27).
MARS is an adjuvant treatment system for liver diseases, mainly by removing endogenous and exogenous toxins to block the vicious circle, improve the balance of internal environment, reduce the continued destruction of hepatocytes, and promote the regeneration of hepatocytes and the functional recovery of critical hepatocytes (39, 61). MARS can not only reduce albumin and non-albumin binding toxins (62), but improve hemodynamics (63) and decrease bilirubin level in LF patients (64). Recent studies have shown that RCA could be safely applied in patients receiving MARS (38, 40). An observational study on 20 LF patients indicate that RCA would be safe, and no episodes of coagulation or bleeding observed during MARS (40). Another prospective study has revealed that RCA-MARS would be safe and feasible under close metabolic monitoring in LF patients, and would be more effective than non-RCA-MARS (38). As for survival, a study by Gerth et al. has shown that MARS could reduce the 28-day mortality of LF patients (61), which is supported by some other studies (39). However, there are some studies that hold different view on the survival benefit of MRAS (65). Two studies indicate MARS brings no benefits to the survival of LF patients, and a study report a higher 28-day mortality in patients receiving MARS over 4 times, compared with those receiving MARS less than 4 times (66).
Plasma exchange is also a commonly used artificial liver support treatment, which uses a plasma separator to separate and discard patient’s plasma that contains pathological components, and supplement 2,000-3,000 ml of fresh frozen plasma that contains essential substances such as protein and coagulation factors (67). This process filters out plenty of bilirubin, endotoxin, and virus, and rapidly improves the internal environment so as to alleviate liver injury and improve the regeneration environment of hepatocytes (22, 68). Studies have shown that PE could reduce the risk of bleeding in ALF patients via correcting the coagulation function (69). PE can also improve cerebral blood and oxygen support (70). In recent years, there are not only animal studies that have validated the efficacy and safety of citrate-based PE (71), but clinical reports regarding the use of citrate in PE. Betz et al. applied Fresenius Ci-Ca, (72) and provide intravenous injection of calcium as needed under the mode of fixed citrate and calcium ratio, which provide a feasible approach for the use of PE in patients with high risk of bleeding. The main complication of PE is hypocalcemia. Fresh frozen plasma contains a large amount of citric acid preparation, which increases the risk of hypocalcemia and citrate accumulation (73). Slow intravenous administration (15-30 min) of 10% calcium chloride injection or continuous intravenous infusion of calcium gluconate after PE initiation can prevent citrate toxicity (74).
In this study, we have analyzed the results of 19 studies from 4 databases to assess the efficacy and safety of citrate as anticoagulant in ECOS. Firstly, citrate anticoagulation can provide adequate anticoagulation for blood during cardiopulmonary bypass, thereby minimizing coagulation disorders associated with contact activation while maintaining systemic coagulation (75). The liver contributes essentially to exogenous citric acid metabolism, and the clearance of endogenous citric acid is decreased in LF patients (52). As a result, metabolic acidosis and increased anion gaps may occur. According to previous studies, a serum Catot/Caion ratio greater than or equal to 2.5 may be the critical threshold for potential citrate accumulation (76). Secondly, although the use of ECOS is relatively safe, a considerable number of studies have reported the incidence of bleeding-associated complications during the treatment, ranging from 9% to 40% (77). A study of citrate anticoagulation in RRT reported no bleeding complications and no blood transfusion within 24 h after RRT (28). In addition, the effect of ECOS on renal function is also evident. RRT can improve renal function. At the same time, the MARS device is combined with routine hemodialysis for RRT. Therefore, creatinine and urea nitrogen levels were reduced during MARS treatment. Studies on changes in biochemical markers also reported at least a decrease in creatinine levels during the use of MARS (78). However, RRT is more effective in reducing SCr and BUN, compared with the 8-h course recommended by MARS, which might be attributed to the longer treatment duration. In current studies, MARS usually operates in CVVHD mode, and the effectiveness of renal replacement may be lower than that of CVVHD mode.
This is the first systematic review and meta-analysis conducted to assess the efficacy and safety of citrate anticoagulation in ECOSs, including RRT, MARS and PE. However, some limitations exist. There are differences in the basic characteristics of patients, such as disease severity, gender, age, regional population, and treatment regimen, leading to significant clinical heterogeneity among the studies. There are also a variety of models to be selected even in RRT. We failed to provide specific treatment, but unified as one study. In addition, the study-design are not unified. The inherent limitations of non-randomized studies in Meta-analysis may also affect our results. More prospective RCTs are needed to further validate our findings. The evaluation criteria for results may vary depending on the type of treatment used, combination therapy, the degree of liver failure, and the duration of ECOS treatment, especially in different countries. We tried to use random-effect model and subgroup analysis method to solve these problems. Meta-analysis is also limited by the quality of included studies. Because it is in a relatively new period of exploration and research, the potential publication bias could not be ignored. Considering the above limitations, the results of our meta-analysis should be interpreted prudently.
5. Conclusion
Citrate-based anticoagulation would be safe and effective in ECOS treatment for LF patients. Close monitoring and timely adjustment are needed during the process to avoid potential complications. Due to the quantitative and qualitative limitations in this study, more prospective clinical trials of high quality are needed to further validate our findings so as to provide more robust evidence for the anticoagulation strategy in ECOS for LF patients. Moreover, due to the interactions between organs, multi-organ support therapy can provide joint support for different organ failure, and the integrated multi-organ support platform is the future development direction, which makes it necessary to select the most preferable anticoagulation strategy.
Data availability statement
The original contributions presented in this study are included in the article/supplementary material, further inquiries can be directed to the corresponding authors.
Author contributions
BP and JLu wrote the main manuscript and fully participated in all analyses. AL and JLi contributed to the study concept and design. HG and JLu participated in literature search, data extraction, and quality assessment. All authors read and approved the final manuscript.
Funding
This work was supported by Beijing Municipal Administration of Hospitals Key Medical Professional Development Plan – “Yangfan Plan” (ZYLX201802).
Acknowledgments
We would like to thank the researchers and study participants for their contributions.
Conflict of interest
The authors declare that the research was conducted in the absence of any commercial or financial relationships that could be construed as a potential conflict of interest.
Publisher’s note
All claims expressed in this article are solely those of the authors and do not necessarily represent those of their affiliated organizations, or those of the publisher, the editors and the reviewers. Any product that may be evaluated in this article, or claim that may be made by its manufacturer, is not guaranteed or endorsed by the publisher.
Supplementary material
The Supplementary Material for this article can be found online at: https://www.frontiersin.org/articles/10.3389/fnut.2023.1031796/full#supplementary-material
References
1. Alberto S, Pires S, Figueiredo A, Deus J. [Acute liver failure]. Acta Med Port. (2009) 22:809–20.
2. Moreau R, Lebrec D. Acute renal failure in patients with cirrhosis: perspectives in the age of MELD. Hepatology. (2003) 37:233–43.
3. Tujios S, Hynan L, Vazquez M, Larson A, Seremba E, Sanders C, et al. Risk factors and outcomes of acute kidney injury in patients with acute liver failure. Clin Gastroenterol Hepatol. (2015) 13:352–9. doi: 10.1053/jhep.2003.50084
4. Ronco C, Ricci Z, Husain-Syed F. From multiple organ support therapy to extracorporeal organ support in critically ill patients. Blood Purif. (2019) 48:99–105. doi: 10.1016/j.cgh.2014.07.011
5. Davenport A. What are the anticoagulation options for intermittent hemodialysis? Nat Rev Nephrol. (2011) 7:499–508. doi: 10.1159/000490694
6. Pinnick R, Wiegmann T, Diederich D. Regional citrate anticoagulation for hemodialysis in the patient at high risk for bleeding. N Engl J Med. (1983) 308:258–61. doi: 10.1038/nrneph.2011.88
7. Oudemans-van Straaten H, Ostermann M. Bench-to-bedside review: citrate for continuous renal replacement therapy, from science to practice. Crit Care. (2012) 16:249. doi: 10.1056/NEJM198302033080506
8. Wonnacott R, Josephs B, Jamieson JCRRT. Regional anticoagulation using citrate in the liver failure and liver transplant population. Crit Care Nurs Q. (2016) 39:241–51. doi: 10.1186/cc11645
9. Morabito S, Pistolesi V, Tritapepe L, Zeppilli L, Polistena F, Strampelli E, et al. Regional citrate anticoagulation in cardiac surgery patients at high risk of bleeding: a continuous veno-venous hemofiltration protocol with a low concentration citrate solution. Crit Care. (2012) 16:R111. doi: 10.1097/CNQ.0000000000000118
10. Zarbock A, Küllmar M, Kindgen-Milles D, Wempe C, Gerss J, Brandenburger T, et al. Effect of regional citrate anticoagulation vs systemic heparin anticoagulation during continuous kidney replacement therapy on dialysis filter life span and mortality among critically ill patients with acute kidney injury: a randomized clinical trial. JAMA. (2020) 324:1629–39. doi: 10.1186/cc11403
11. Schultheiß C, Saugel B, Phillip V, Thies P, Noe S, Mayr U, et al. Continuous venovenous hemodialysis with regional citrate anticoagulation in patients with liver failure: a prospective observational study. Crit Care. (2012) 16:R162. doi: 10.1001/jama.2020.18618
12. Lahmer T, Messer M, Rasch S, Beitz A, Schnappauf C, Schmid R, et al. Sustained low-efficiency dialysis with regional citrate anticoagulation in medical intensive care unit patients with liver failure: a prospective study. J Crit Care. (2015) 30:1096–100. doi: 10.1186/cc11485
13. Slowinski T, Morgera S, Joannidis M, Henneberg T, Stocker R, Helset E, et al. Safety and efficacy of regional citrate anticoagulation in continuous venovenous hemodialysis in the presence of liver failure: the liver citrate anticoagulation threshold (L-CAT) observational study. Critical care. (2015) 19:349. doi: 10.1016/j.jcrc.2015.06.006
14. Stevens PE, Levin A. Section 5: dialysis interventions for treatment of AKI. Kidney Int Suppl. (2012) 2:89–115. doi: 10.1186/s13054-015-1066-7
15. Balogun R, Turgut F, Caldwell S, Abdel-Rahman E. Regional citrate anticoagulation in critically ill patients with liver and kidney failure. J Nephrol. (2012) 25:113–9. doi: 10.1038/kisup.2011.35
16. Kindgen-Milles D, Brandenburger T, Dimski T. Regional citrate anticoagulation for continuous renal replacement therapy. Curr Opin Crit Care. (2018) 24:450–4. doi: 10.5301/JN.2011.8363
17. Link A, Klingele M, Speer T, Rbah R, Pöss J, Lerner-Gräber A, et al. Total-to-ionized calcium ratio predicts mortality in continuous renal replacement therapy with citrate anticoagulation in critically ill patients. Crit Care. (2012) 16:R97. doi: 10.1097/MCC.0000000000000547
18. Morgera S, Scholle C, Voss G, Haase M, Vargas-Hein O, Krausch D, et al. Metabolic complications during regional citrate anticoagulation in continuous venovenous hemodialysis: single-center experience. Nephron Clin Pract. (2004) 97:c131–6. doi: 10.1186/cc11363
19. Weissenborn K. Hepatic encephalopathy: definition, clinical grading and diagnostic principles. Drugs. (2019) 79(Suppl. 1):5–9. doi: 10.1159/000079171
20. Jha V, Arici M, Collins A, Garcia-Garcia G, Hemmelgarn B, Jafar T, et al. Understanding kidney care needs and implementation strategies in low- and middle-income countries: conclusions from a “Kidney Disease: Improving Global Outcomes” (KDIGO) Controversies Conference. Kidney Int. (2016) 90:1164–74. doi: 10.1007/s40265-018-1018-z
21. Page M, McKenzie J, Bossuyt P, Boutron I, Hoffmann T, Mulrow C, et al. The PRISMA 2020 statement: an updated guideline for reporting systematic reviews. BMJ. (2021) 372:n71.
22. Squires J, McKiernan P, Squires R. Acute liver failure: an update. Clin Liver Dis. (2018) 22:773–805. doi: 10.1136/bmj.n71
23. Ferstl P, Trebicka J. Acute decompensation and acute-on-chronic liver failure. Clin Liver Dis. (2021) 25:419–30. doi: 10.1016/j.cld.2018.06.009
24. Slim K, Nini E, Forestier D, Kwiatkowski F, Panis Y, Chipponi J. Methodological index for non-randomized studies (minors): development and validation of a new instrument. ANZ J Surg. (2003) 73:712–6. doi: 10.1016/j.cld.2021.01.009
25. Higgins J, Thompson S, Deeks J, Altman D. Measuring inconsistency in meta-analyses. BMJ. (2003) 327:557–60. doi: 10.1046/j.1445-2197.2003.02748.x
26. Yu Y, Peng S, Cen Z, Cai J, Wang W, Tang Y, et al. Applying regional citrate anticoagulation in continuous renal replacement therapy for acute kidney injury patients with acute liver dysfunction: a retrospective observational study. Kidney Blood Press Res. (2018) 43:1065–74. doi: 10.1136/bmj.327.7414.557
27. De Vico P, Messino V, Tartaglione A, Beccaris C, Buonomo C, Talarico D, et al. Safety and efficacy of citrate anti-coagulation continuous renal replacement therapies in post-cardiac surgery patients with liver dysfunction. Ther Apher Dial. (2015) 19:272–8. doi: 10.1159/000491057
28. Pourcine F, Vong L, Chelly J, Rollin N, Sy O, Jochmans S, et al. Sustained low-efficiency dialysis with regional citrate anticoagulation for patients with liver impairment in intensive care unit: a single-center experience. Ther Apher Dial. (2021) 25:211–7. doi: 10.1111/1744-9987.12280
29. Rhee H, Berenger B, Mehta R, Macedo E. Regional citrate anticoagulation for continuous kidney replacement therapy with calcium-containing solutions: a cohort study. Am J Kidney Dis (2021) 78:550.e–9.e. doi: 10.1111/1744-9987.13538
30. Yu Y, Bai M, Ma F, Zhang W, Li Y, Zhao L, et al. Regional citrate anticoagulation versus no-anticoagulation for continuous venovenous hemofiltration in patients with liver failure and increased bleeding risk: a retrospective case-control study. PLoS One. (2020) 15:e0232516. doi: 10.1053/j.ajkd.2021.01.017
31. Sponholz C, Settmacher U, Bauer M, Kortgen A. Regional citrate anticoagulation for continuous renal replacement therapy in the perioperative care of liver transplant recipients: a single center experience. Ther Apher Dial. (2015) 19:8–15. doi: 10.1371/journal.pone.0232516
32. Saner F, Treckmann J, Geis A, Lösch C, Witzke O, Canbay A, et al. Efficacy and safety of regional citrate anticoagulation in liver transplant patients requiring post-operative renal replacement therapy. Nephrol Dial Transplant. (2012) 27:1651–7. doi: 10.1111/1744-9987.12196
33. Durão M, Monte J, Batista M, Oliveira M, Iizuka I, Santos B, et al. The use of regional citrate anticoagulation for continuous venovenous hemodiafiltration in acute kidney injury. Crit Care Med. (2008) 36:3024–9. doi: 10.1093/ndt/gfr510
34. Ma Y, Xu Y, Chen F, Wang Y, Bai L, Tang H. Good tolerance of citrate accumulation due to plasma exchange among patients with acute-on-chronic liver failure: a prospective, observational study. Can J Gastroenterol Hepatol. (2018) 2018:4909742. doi: 10.1097/CCM.0b013e31818b9100
35. Berber I, Cagin Y, Erdogan M, Ataman E, Gozukara H, Erkurt M, et al. Early therapeutic plasma exchange may improve treatment outcomes in severe acute toxic hepatitis. Transfus Apher Sci. (2021) 60:103250. doi: 10.1155/2018/4909742
36. Maheshwari A, Bajpai M, Patidar G. Effects of therapeutic plasma exchange on liver function test and coagulation parameters in acute liver failure patients. Hematol Transfus Cell Ther. (2020) 42:125–8. doi: 10.1016/j.transci.2021.103250
37. Meijers B, Laleman W, Vermeersch P, Nevens F, Wilmer A, Evenepoel P. A prospective randomized open-label crossover trial of regional citrate anticoagulation vs. anticoagulation free liver dialysis by the molecular adsorbents recirculating system. Crit Care. (2012) 16:R20. doi: 10.1016/j.htct.2019.05.003
38. Falkensteiner C, Kortgen A, Leonhardt J, Bauer M, Sponholz C. Comparison of albumin dialysis devices molecular adsorbent recirculating system and ADVanced organ support system in critically ill patients with liver failure-A retrospective analysis. Ther Apher Dial. (2021) 25:225–36. doi: 10.1186/cc11180
39. Faybik P, Hetz H, Mitterer G, Krenn C, Schiefer J, Funk G, et al. Regional citrate anticoagulation in patients with liver failure supported by a molecular adsorbent recirculating system. Critical Care Med. (2011) 39:273–9. doi: 10.1111/1744-9987.13533
40. Yonekawa C, Nakae H, Tajimi K, Asanuma Y. Effectiveness of combining plasma exchange and continuous hemodiafiltration in patients with postoperative liver failure. Artif Organs. (2005) 29:324–8. doi: 10.1097/CCM.0b013e3181fee8a4
41. Klingele M, Stadler T, Fliser D, Speer T, Groesdonk H, Raddatz A. Long-term continuous renal replacement therapy and anticoagulation with citrate in critically ill patients with severe liver dysfunction. Critical care. (2017) 21:294. doi: 10.1111/j.1525-1594.2005.29054.x
42. Arroyo V, Moreau R, Jalan R. Acute-on-chronic liver failure. N Engl J Med. (2020) 382:2137–45. doi: 10.1186/s13054-017-1870-3
43. Betrosian A, Agarwal B, Douzinas E. Acute renal dysfunction in liver diseases. World J Gastroenterol. (2007) 13:5552–9. doi: 10.1056/NEJMra1914900
44. Dhar A, Mullish B, Thursz M. Anticoagulation in chronic liver disease. J Hepatol. (2017) 66:1313–26. doi: 10.3748/wjg.v13.i42.5552
45. Ribic C, Crowther M. Thrombosis and anticoagulation in the setting of renal or liver disease. Hematol Am Soc Hematol Educ Program. (2016) 2016:188–95. doi: 10.1016/j.jhep.2017.01.006
46. Kissling S, Wilson P, Ridel C, Burnier M, Vogt B. [What reasonable applications for regional citrate anticoagulation in renal replacement therapy?]. Rev Med Suisse. (2012) 8:452–6. doi: 10.1182/asheducation-2016.1.188
47. Bartels P, Schoorl M, Schoorl M, Wiering J, Nubé M. Activation of coagulation during treatment with haemodialysis. Scand J Clin Lab Invest. (2000) 60:283–90.
48. Tripodi A, Mannucci P. The coagulopathy of chronic liver disease. N Engl J Med. (2011) 365:147–56. doi: 10.1080/003655100750046440
49. Morgera S. Regional anticoagulation with citrate: expanding its indications. Crit Care Med. (2011) 39:399–400. doi: 10.1056/NEJMra1011170
50. Chappell J. The oxidation of citrate, isocitrate and cis-aconitate by isolated mitochondria. Biochem J. (1964) 90:225–37. doi: 10.1097/CCM.0b013e318205c500
51. Meersch M, Küllmar M, Wempe C, Kindgen-Milles D, Kluge S, Slowinski T, et al. Regional citrate versus systemic heparin anticoagulation for continuous renal replacement therapy in critically ill patients with acute kidney injury (RICH) trial: study protocol for a multicentre, randomised controlled trial. BMJ Open. (2019) 9:e024411. doi: 10.1042/bj0900225
52. Kramer L, Bauer E, Joukhadar C, Strobl W, Gendo A, Madl C, et al. Citrate pharmacokinetics and metabolism in cirrhotic and noncirrhotic critically ill patients. Crit Care Med. (2003) 31:2450–5. doi: 10.1136/bmjopen-2018-024411
53. Mitzner S. Extracorporeal liver support-albumin dialysis with the molecular adsorbent recirculating system (MARS). Ann Hepatol. (2011) 10 Suppl 1:S21–8. doi: 10.1097/01.CCM.0000084871.76568.E6
54. Leckie P, Davenport A, Jalan R. Extracorporeal liver support. Blood Purif. (2012) 34:158–63. doi: 10.1016/S1665-2681(19)31602-3
55. Weber M, Ibrahim H, Lake J. Renal dysfunction in liver transplant recipients: evaluation of the critical issues. Liver Transplant. (2012) 18:1290–301. doi: 10.1159/000342065
56. Schefold J, von Haehling S, Pschowski R, Bender T, Berkmann C, Briegel S, et al. The effect of continuous versus intermittent renal replacement therapy on the outcome of critically ill patients with acute renal failure (CONVINT): a prospective randomized controlled trial. Crit Care. (2014) 18:R11. doi: 10.1002/lt.23522
57. Hetzel G, Schmitz M, Wissing H, Ries W, Schott G, Heering P, et al. Regional citrate versus systemic heparin for anticoagulation in critically ill patients on continuous venovenous haemofiltration: a prospective randomized multicentre trial. Nephrol, Dial Transplant. (2011) 26:232–9. doi: 10.1186/cc13188
58. Claure-Del Granado R, Macedo E, Soroko S, Kim Y, Chertow G, Himmelfarb J, et al. Anticoagulation, delivered dose and outcomes in CRRT: the program to improve care in acute renal disease (PICARD). Hemodial Int. (2014) 18:641–9. doi: 10.1093/ndt/gfq575
59. Honore P, Spapen H. Evolution of vascular access and anticoagulation. Contrib Nephrol. (2018) 194:15–24. doi: 10.1111/hdi.12157
60. Wendon J, Cordoba J, Dhawan A, Larsen F, Manns M, Samuel D, et al. EASL clinical practical guidelines on the management of acute (fulminant) liver failure. J Hepatol. (2017) 66:1047–81. doi: 10.1159/000485597
61. Gerth H, Pohlen M, Thölking G, Pavenstädt H, Brand M, Hüsing-Kabar A, et al. Molecular adsorbent recirculating system can reduce short-term mortality among patients with acute-on-chronic liver failure-a retrospective analysis. Crit Care Med. (2017) 45:1616–24. doi: 10.1016/j.jhep.2016.12.003
62. Sponholz C, Matthes K, Rupp D, Backaus W, Klammt S, Karailieva D, et al. Molecular adsorbent recirculating system and single-pass albumin dialysis in liver failure–a prospective, randomised crossover study. Crit Care. (2016) 20:2. doi: 10.1097/CCM.0000000000002562
63. Laleman W, Wilmer A, Evenepoel P, Elst I, Zeegers M, Zaman Z, et al. Effect of the molecular adsorbent recirculating system and Prometheus devices on systemic haemodynamics and vasoactive agents in patients with acute-on-chronic alcoholic liver failure. Crit Care. (2006) 10:R108. doi: 10.1186/s13054-015-1159-3
64. Al-Chalabi A, Matevossian E, Ak V, Luppa P, Neiss A, Schuster T, et al. Evaluation of the Hepa Wash ® treatment in pigs with acute liver failure. BMC Gastroenterol. (2013) 13:83. doi: 10.1186/cc4985
65. Kribben A, Gerken G, Haag S, Herget-Rosenthal S, Treichel U, Betz C, et al. Effects of fractionated plasma separation and adsorption on survival in patients with acute-on-chronic liver failure. Gastroenterology. (2012) 142:782.e–9.e. doi: 10.1186/1471-230X-13-83
66. Bañares R, Ibáñez-Samaniego L, Torner J, Pavesi M, Olmedo C, Catalina M, et al. Meta-analysis of individual patient data of albumin dialysis in acute-on-chronic liver failure: focus on treatment intensity. Ther Adv Gastroenterol. (2019) 12:1756284819879565. doi: 10.1053/j.gastro.2011.12.056
67. Tan E, Wang M, Pang J, Lee G. Plasma exchange in patients with acute and acute-on-chronic liver failure: a systematic review. World J Gastroenterol. (2020) 26:219–45. doi: 10.1177/1756284819879565
68. Schwartz J, Padmanabhan A, Aqui N, Balogun R, Connelly-Smith L, Delaney M, et al. Guidelines on the use of therapeutic apheresis in clinical practice-evidence-based approach from the writing committee of the American society for apheresis: the seventh special issue. J Clin Apher. (2016) 31:149–62. doi: 10.3748/wjg.v26.i2.219
69. Singer A, Olthoff K, Kim H, Rand E, Zamir G, Shaked A. Role of plasmapheresis in the management of acute hepatic failure in children. Ann Surg. (2001) 234:418–24. doi: 10.1002/jca.21470
70. Clemmesen J, Kondrup J, Nielsen L, Larsen F, Ott P. Effects of high-volume plasmapheresis on ammonia, urea, and amino acids in patients with acute liver failure. Am J Gastroenterol. (2001) 96:1217–23. doi: 10.1097/00000658-200109000-00015
71. Francey T, Schweighauser A. Membrane-based therapeutic plasma exchange in dogs: prescription, anticoagulation, and metabolic response. J Vet Intern Med. (2019) 33:1635–45. doi: 10.1111/j.1572-0241.2001.03706.x
72. Betz C, Buettner S, Geiger H, Jung O. Regional citrate anticoagulation in therapeutic plasma exchange with fresh frozen plasma–a modified protocol. Int J Artif Organs. (2013) 36:803–11. doi: 10.1111/jvim.15528
73. Weinstein R. Hypocalcemic toxicity and atypical reactions in therapeutic plasma exchange. J Clin Apher. (2001) 16:210–1. doi: 10.5301/ijao.5000245
74. Weinstein R. Prevention of citrate reactions during therapeutic plasma exchange by constant infusion of calcium gluconate with the return fluid. J Clin Apher. (1996) 11:204–10. doi: 10.1002/jca.10000
75. Evenepoel P, Maes B, Vanwalleghem J, Kuypers D, Messiaen T, Vanrenterghem Y. Regional citrate anticoagulation for hemodialysis using a conventional calcium-containing dialysate. Am J Kidney Dis. (2002) 39:315–23. doi: 10.1002/(SICI)1098-1101(1996)11:4<204::AID-JCA5>3.0.CO;2-F
76. Bauer E, Derfler K, Joukhadar C, Druml W. Citrate kinetics in patients receiving long-term hemodialysis therapy. Am J Kidney Dis. (2005) 46:903–7. doi: 10.1053/ajkd.2002.30551
77. Tan H, Yang W, Choong H, Wong K. Albumin dialysis without anticoagulation in high-risk patients: an observational study. Artif Organs. (2012) 36:E83–8. doi: 10.1053/j.ajkd.2005.07.041
Keywords: anticoagulation, citrate, liver failure, extracorporeal organ support, renal replacement therapy, molecular adsorbent recirculation system, plasma exchange
Citation: Peng B, Lu J, Guo H, Liu J and Li A (2023) Regional citrate anticoagulation for replacement therapy in patients with liver failure: A systematic review and meta-analysis. Front. Nutr. 10:1031796. doi: 10.3389/fnut.2023.1031796
Received: 01 September 2022; Accepted: 09 January 2023;
Published: 16 February 2023.
Edited by:
Silvia Lai, Sapienza University of Rome, ItalyReviewed by:
Gianina Flocco, Cleveland Clinic, United StatesThananda Trakarnvanich, Navamindradhiraj University, Thailand
Copyright © 2023 Peng, Lu, Guo, Liu and Li. This is an open-access article distributed under the terms of the Creative Commons Attribution License (CC BY). The use, distribution or reproduction in other forums is permitted, provided the original author(s) and the copyright owner(s) are credited and that the original publication in this journal is cited, in accordance with accepted academic practice. No use, distribution or reproduction is permitted which does not comply with these terms.
*Correspondence: Jingyuan Liu, ZHR5eWljdUBjY211LmVkdS5jbg==; Ang Li,
bGlhbmdAY2NtdS5lZHUuY24=
†These authors have contributed equally to this work