- 1Department of Nursing, Ryazan State Medical University, Ryazan, Russia
- 2Department of Pharmacy Management and Economics, Ryazan State Medical University, Ryazan, Russia
Cardiovascular diseases are one of the most important problems of modern medicine. They are associated with a large number of health care visits, hospitalizations and mortality. Prevention of atherosclerosis is one of the most effective strategies and should start as early as possible. Correction of lipid metabolism disorders is associated with definite clinical successes, both in primary prevention and in the prevention of complications of many cardiovascular diseases. A growing body of evidence suggests a multifaceted role for polyunsaturated fatty acids. They demonstrate a variety of functions in inflammation, both participating directly in a number of cellular processes and acting as a precursor for subsequent biosynthesis of lipid mediators. Extensive clinical data also support the importance of polyunsaturated fatty acids, but all questions have not been answered to date, indicating the need for further research.
Introduction
Cardiovascular diseases are one of the most important problems of modern medicine (1). Coronary heart disease, ischemic stroke, and peripheral arterial diseases are clinical manifestations of atherosclerosis of different vascular locations. The increasing prevalence, medical, social and economic consequences of these diseases require new approaches aimed at improving the efficiency of early diagnosis and treatment (2–4). Indeed, atherosclerosis develops over many years, but patients may stay out of the doctor’s sight all this time, as a result, they often first seek medical care only at clinically advanced stages. Atherosclerosis is considered to be a disease whose development is strongly associated with risk factors, such as eating disorders, overweight and obesity, physical inactivity, and smoking (5–7). These modifiable risk factors are an important therapeutic target, which should be treated as early as possible (8). Lipid metabolism disorders play a key role in atherogenesis, and their correction is the basis of drug and non-drug therapy for these patients (9, 10).
A growing body of evidence has made it possible to determine the leading role of impaired lipid metabolism in many processes occurring in the vascular wall in atherosclerosis (11). Fatty acids are essential molecules that exhibit a variety of functions. Because of their diversity, they are involved in a variety of processes.
Depending on the length of the carbon chains, fatty acids are commonly classified as short-chain (have less than 6 carbon atoms), medium-chain (6–12 carbon atoms) and long-chain (more than 12 carbon atoms). In addition, fatty acids are divided by the degree of saturation of the carbon chain with hydrogen atoms. This classification distinguishes saturated fatty acids (SFAs), monounsaturated fatty acids (MUFAs) and polyunsaturated fatty acids (PUFAs). Of clinical importance is another classification that distinguishes ω-3 PUFAs with the end double bond at C3, counting from the methyl end of the hydrocarbon chain, and ω-6 PUFAs with the end double bond at C6.
Clinical interest in PUFAs increased in the 1970s after a series of epidemiological studies were published showing that Greenland Eskimos had a lower risk of coronary heart disease and diabetes compared to the Danish population, which was linked to a high consumption of ω-3 PUFAs derived from marine fish, seals and whales (12, 13). It was shown that the fatty acid structure consumed by the Greenland Eskimos hunters was characterized by a higher content of long-chain polyunsaturated fatty acids (especially eicosapentaenoic acid and docosahexaenoic acid) and a lower content of linoleic and linolenic acids (14). This corresponded to lower plasma concentrations of triglycerides and very low-density lipoproteins in Eskimos than in Western populations (14). Although total polyunsaturated fatty acid concentrations were lower in Greenland Eskimos than in other groups, a higher proportion of palmitic, palmitoleic and eicosapentaenoic acids and a lower concentration of linoleic acid were found in Greenland Eskimos plasma (15).
Japanese people living on Kohama Island in Okinawa have a very low incidence of cardiovascular disease, which is thought to be due to a high intake of eicosapentaenoic and docosahexaenoic acids from fresh fish. In a study of serum from elderly people from this population, higher levels of total eicosapolyenoic acids were found than in mainland people. In addition, a positive correlation was found between serum eicosapentaenoic acid concentrations and high-density lipoprotein (HDL) concentrations (16). Consumption of seafood, a source of eicosapentaenoic and docosahexaenoic acids, was associated with low rates of coronary heart disease mortality in the Inuit population of Nunavik (17).
These and other findings have increased attention to the possible therapeutic value of PUFAs for cardiovascular disease (Figure 1).
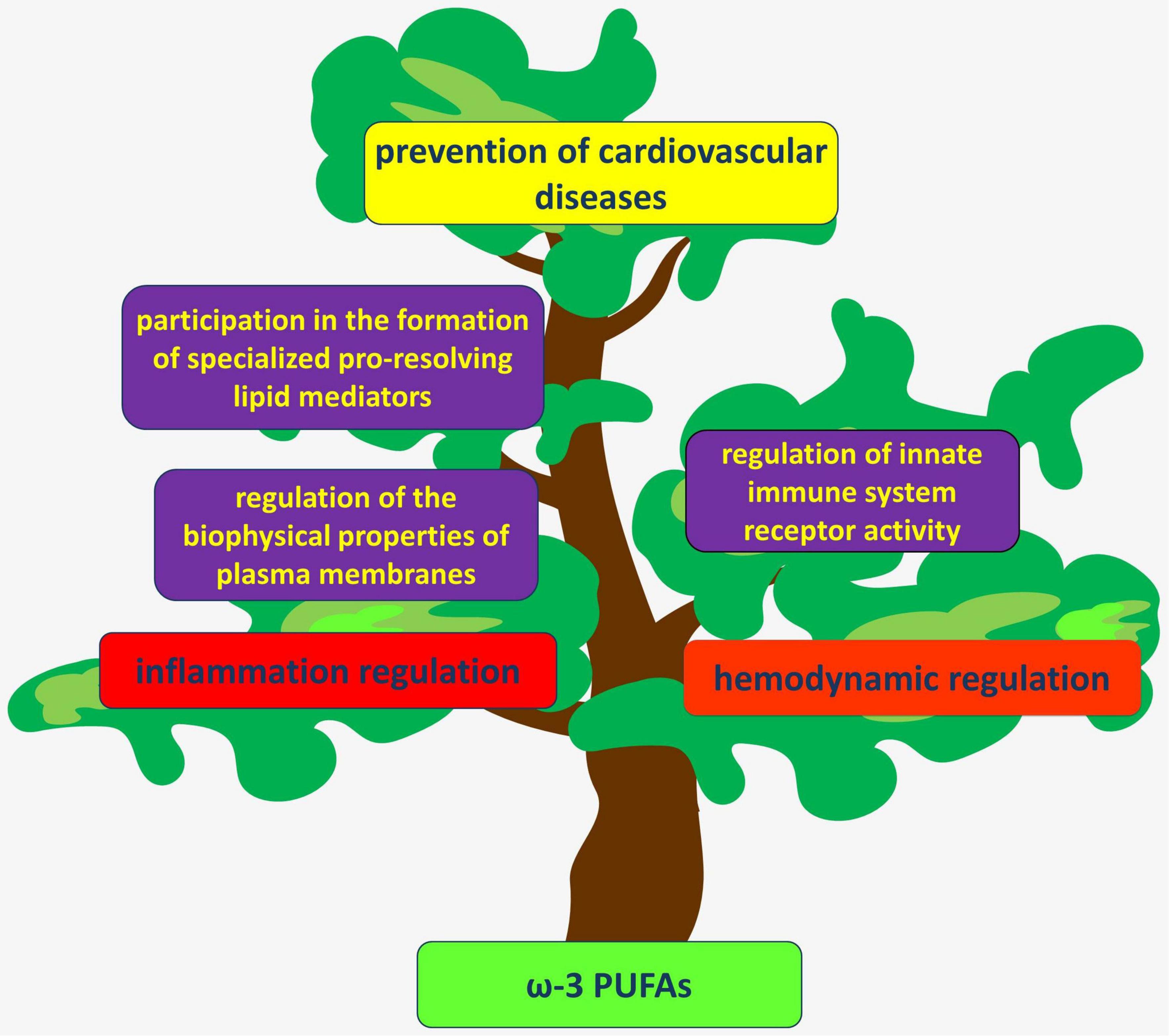
Figure 1. PUFAs act through several mechanisms to regulate hemodynamics and inflammation in the vascular wall. Their anti-inflammatory and atheroprotective effects are considered beneficial for the prevention of various cardiovascular diseases.
Marine fish is a valuable source of polyunsaturated fatty acids. The CALIPSO study showed that eel, salmon, swordfish, and halibut are high in ω-3 PUFAs such as eicosapentaenoic, docosahexaenoic and docosapentaenoic fatty acids (18). In addition, antarctic krill oil was also shown to contain eicosapentaenoic acid and docosahexaenoic acid, which account for over 27% of total fatty acids (19).
Molecular mechanisms in which polyunsaturated fatty acids are involved
Polyunsaturated fatty acids (PUFAs) are important participants in many physiological processes and are associated with the regulation of inflammation, antioxidant protection, the regulation of vascular hemodynamics and other important biological functions (Figure 2).
Participation of polyunsaturated fatty acids in the regulation of inflammation
Participation of polyunsaturated fatty acids in formation of lipid mediators
According to modern concepts, atherosclerosis is an inflammatory disease of arterial intima, in which the balance between the mechanisms of activation and resolution of inflammation is disturbed. PUFAs are substrates for the synthesis of both proinflammatory and specialized pro-resolving lipid mediators (Figure 3) (20).
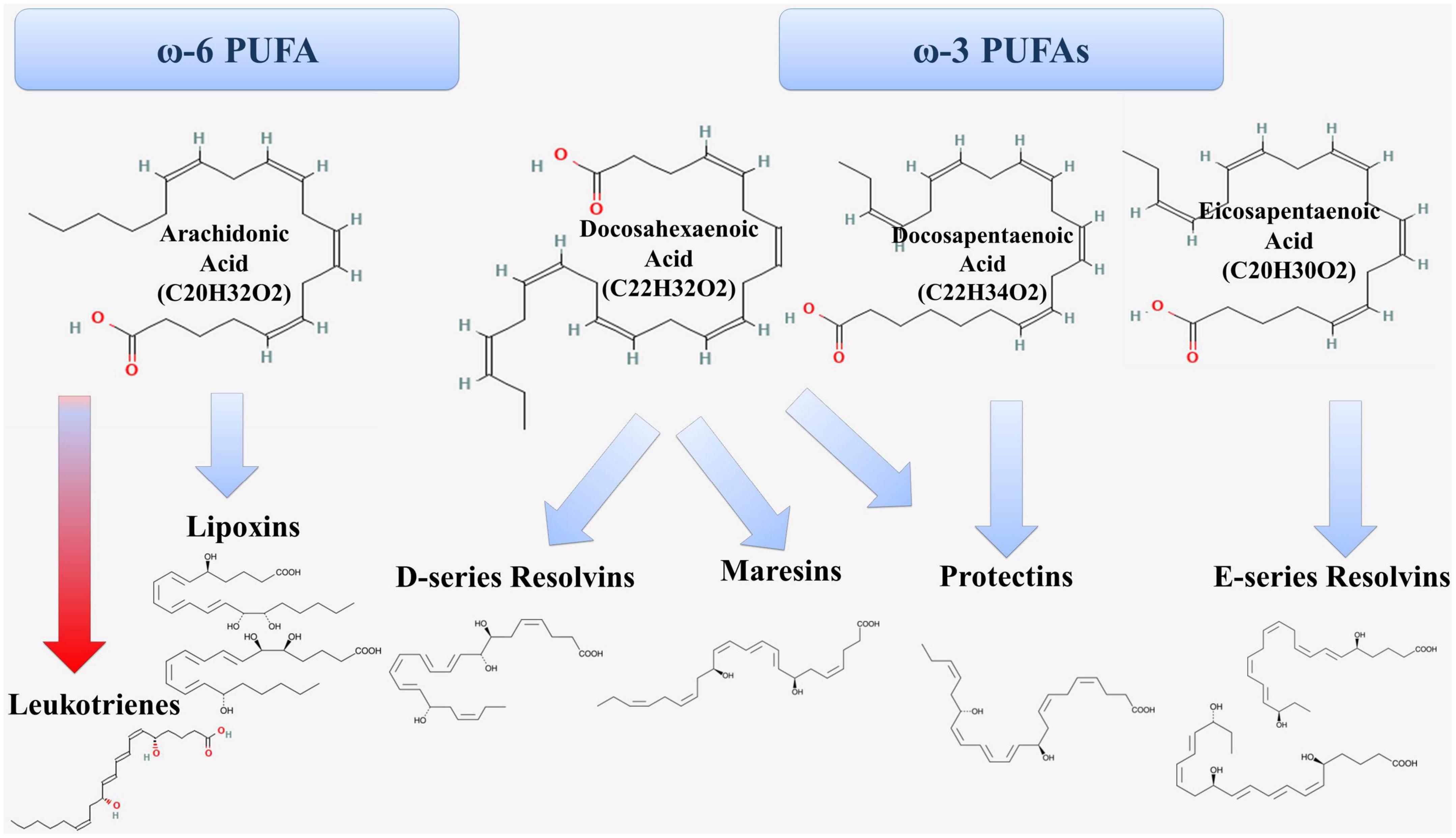
Figure 3. Formation of proinflammatory (red arrow) and anti-inflammatory (blue arrow) lipid mediators from PUFAs.
Arachidonic acid is ω-6 PUFA and is metabolized through the lipoxygenase (LOX) pathway, the cyclooxygenase (COX) pathway and the cytochrome P450 pathway (20). In the lipoxygenase pathway, arachidonic acid is a substrate for the formation of proinflammatory leukotrienes and pro-resolving lipoxins (Figure 1). The 5-LOX enzyme is at the crossroads of the proinflammatory and specialized pro-resolving lipid mediators synthesis pathways (21).
Leukotrienes play an important role in inflammation in atherosclerosis (22, 23). They are mainly produced by macrophages that infiltrate atherosclerotic lesions. Leukotriene (LT) B4 is a potent chemoattractant for monocytes, neutrophils, and lymphocytes. It participates in atherogenesis by promoting leukocyte adhesion to the endothelium, increasing vascular permeability, and promoting proliferation and migration of vascular smooth muscle cells (VSMCs) (24, 25).
Lipoxins belong to the group of specialized pro-resolving lipid mediators and play an important role in atheroprotection. Lipoxin A4 (LXA4) and lipoxin B4 (LXB4) as well as their epimers such as 15-epi-LXA4 and 15-epi-LXB4 are currently known. The formation of lipoxin epimers is related to the action of aspirin on COX-2, leading to the formation of 15R-hydroxyecosatetraenoic acid (15R-HETE), which is then metabolized by 5-LOX to form epi-lipoxins (26). This pathway involves intercellular interactions between leukocytes and endothelial cells (27).
LXA4 and LXB4 show multiple anti-inflammatory effects. They inhibit leukotriene B4-stimulated transendothelial migration of neutrophils and promote the uptake of apoptotic neutrophils (28) by macrophages (29–31). LXA4 has an inhibitory effect on NF-κB, on interleukin (IL) -1β, IL-6, tumor necrosis factor-α (TNF-α) expression, while enhancing IL-10 expression and improving hemodynamic indices, myocardial structure and function (32). 15-epi-lipoxin A4, acting through endothelial nitric oxide synthase (eNOS) and inducible nitric oxide synthase (iNOS) promotes NO production, negatively regulating leukocyte-endothelial interaction (33).
The cyclooxygenase pathway of arachidonic acid metabolism promotes the formation of prostaglandins (PGE2, PGF2, PGD2), thromboxane (TxA2) and prostacyclin (PGI2). Prostaglandins may be involved in inflammation in the vascular wall (34). PGE2 acts through its receptors and induces expression of matrix metalloproteinase, which may contribute to the instability of atherosclerotic plaques (35). PGE2 also demonstrates hemodynamic actions, which are mediated by complex interactions of several receptor subtypes (36, 37).
Thus, arachidonic acid is a precursor for the formation of lipid mediators involved in both the maintenance and resolution of inflammation.
Eicosopentaenoic acid (EPA, 20:5 ω-3) is a ω-3 PUFA and is the precursor for the formation of E-series resolvins (Figure 3). RvE1 has anti-inflammatory and pro-resolving effects through several mechanisms, including inhibition of the release of inflammatory mediators (38, 39) and activation of the process of efferocytosis of apoptotic neutrophils by macrophages (40, 41). In experimental animal models, RvE1 administration suppressed atherogenesis (42). RvE1 had a local anti-inflammatory effect in the aorta of mice and reduced the area and severity of atherosclerotic lesions without affecting plasma lipids (43).
Docosahexaenoic acid (DHA, 22: 6 ω-3) is also ω-3 PUFA and serves as a precursor for the formation of D-series resolvins, protectins, and maresins (Figure 3). RvD1 inhibits the release of proinflammatory cytokines (IL-6 and TNF-α) (44–46) and enhances the production of anti-inflammatory cytokines in macrophages. In addition, RvD1 affects the stability of the atherosclerotic plaque, promotes the reduction of necroptotic cells, activates the PI3K/Akt pathway, which reduces the negative effects of ischemia and reduces the infarct size (47). Another member of this series of resolvins, RvD2 is involved in the regulation of nitric oxide production as well as modulation of leukocyte adhesion receptor expression, which reduces leukocyte-endothelial interaction (48). In turn, protectin PD1 has antiapoptotic and anti-inflammatory activity. It was shown that immediately after the onset of myocardial infarction the levels of protectins increased, which is part of the early anti-inflammatory response after ST-elevation myocardial infarction (49).
Maresins are formed in macrophages from docosahexaenoic acid and have anti-inflammatory and reparative effects (50). The mechanism of anti-inflammatory action of maresins is to decrease the levels of inflammatory mediators such as IL-6, IL-1β, TNF-α (51, 52) and to increase the production of anti-inflammatory mediators (IL-10) (53, 54). In addition, maresins contribute to the enhancement of phagocytosis and efferocytosis by macrophages and help to limit the infiltration of polymorphonuclear leukocytes (55–60).
Fatty acid esters of hydroxy fatty acids (FAHFAs) represent another class of endogenous lipids with various biological effects (61). The hydroxy fatty acid esters of docosahexaenoic acid (FAHFAs) have anti-inflammatory properties (62). 13-DHAHLA (13-docosahexaenoic acid hydroxy linoleic acid) exhibits anti-inflammatory and pro-resolving properties by reducing lipopolysaccharide-induced macrophage activation and enhancing phagocytosis of zymosan particles (62). At the same time, levels of 13-DHAHLA correlated with the patients’ serum omega-3 index (62). 12-EPAHSA (eicosapentaenoic acid esterified 12-hydroxy stearic acid) increased the expression of Nrf2-dependent antioxidant enzyme genes such as NQO1, GCLM, GCLC, SOD-1 and HO-1 (61).
Thus, PUFAs are an important source of both proinflammatory and anti-inflammatory mediators, the balance of which is important for atherogenesis.
Regulation of the innate immune system
The innate immune system plays an important role in atherogenesis. As part of an evolutionarily ancient system of identification and response to pathogen-associated molecular patterns (PAMP), the innate immune system relies on a large family of pattern recognition receptors (PRRs). These receptors, whose role is well known in atherogenesis, include Toll-like receptor 4 (TLR4), which detects the lipopolysaccharide of Gram-negative bacteria. The TLR4 signaling pathway is closely related to lipid rafts, where TLR4 is recruited upon activation. Fatty acids can influence these processes. It was shown that saturated lauric acid induced dimerization and recruitment of TLR4 to lipid rafts (63). In contrast to saturated fatty acids, unsaturated fatty acids did not activate TLR4 (64). In contrast, docosahexaenoic acid suppressed NF-κB activation and COX-2 expression induced by TLR agonist 2, 3, 4, 5, or 9 in a macrophage cell line (RAW264.7) (65). Thus, docosahexaenoic acid can inhibit TLR4 activation induced by saturated fatty acids. In addition, it was shown that by binding to MD2 (which is a TLR4 co-receptor), arachidonic acid can prevent its association with lipopolysaccharide (LPS), reducing LPS-induced inflammation (66).
It also seems important that while saturated fatty acids can promote NLRP3 inflammasome activation, PUFAs inhibit its activity (67). Dietary PUFAs reduced LPS-induced IL-1β secretion in mice in vivo (68). This is important given the data on the role of NLRP3-inflosome and IL-1β in the pathogenesis of atherosclerosis (69, 70).
Other anti-inflammatory mechanisms
It has been shown that eicosapentaenoic acid can decrease iNOS activity (71). In addition, in mouse macrophages stimulated with interferon-γ plus LPS, docosahexaenoic acid inhibited iNOS mRNA accumulation (72). This seems interesting given the role of macrophage iNOS in the overproduction of NO, which in high concentrations can have cytotoxic effects.
Participation of polyunsaturated fatty acids in hemodynamic regulation
One of the most important advances in vascular biology has been the understanding that endothelial cells can detect changes in blood flow and participate in its regulation through the production of vasoactive substances. One of the key molecules involved in vasodilation is nitric oxide. Nitric oxide exhibits several functions in the vascular wall and is produced by endothelial cells via eNOS. Deficiency in NO production is considered an important marker of endothelial dysfunction, which plays a key role in atherogenesis. eNOS localizes in caveolae, specific structural and functional lipid domains of the plasma membrane. Studies emphasize the important role of endothelial caveolae in the regulation of eNOS activation. In an experiment on rats, it has been shown that destruction of caveolae leads to a decrease in endothelial NO release (73).
It was found that fatty acids can affect the bioavailability of nitric oxide (74). Eicosapentaenoic acid-treated cells increase NO production while decreasing ONOO- release. Cell exposure to docosahexaenoic acid increased NO production but had no effect on ONOO- release. In turn, exposure to arachidonic acid had no effect on NO and ONOO- release (74).
Eicosapentaenoic acid and docosahexaenoic acid (6:1) were shown to induce phosphorylation of Src, Akt, and eNOS in Ser 1177 and induce endothelium-dependent NO-mediated relaxation in pig coronary artery in the experiment (75).
In addition to NO, lipid mediators derived from PUFAs play an active role in the regulation of vascular hemodynamics. The crosslinks between hemodynamics and lipid mediators are due to the fact that shear stress can activate cytosolic phospholipase A2, which promotes the release of arachidonic acid from phospholipids of the plasma membrane (76). It is also suggested that NO can enhance COX2 activity through S-nitrosylation, resulting in increased prostaglandin production (77). PGI2 is formed from arachidonic acid and promotes relaxation of VSMCs, resulting in a marked vasodilator effect.
In addition, arachidonic acid is metabolized by cytochrome P450s to hydroxyeicosatetraenoic acids (HETEs) and epoxyeicosatrienoic acids (EETs). 20-HETE is formed by ω-hydroxylation of arachidonic acid in VSMCs and is a potent vasoconstrictor (78, 79). 20-HETE acts on ion channels (79, 80). The G-protein receptor 75 (GPR75) has been identified as a target for 20-HETE and mediates its involvement in the development of hypertension (81, 82). 20-HETE has been shown to uncouple eNOS and reduce NO production and to stimulate NF-κB activation and inflammatory cytokine production in human endothelial cells (83, 84). In turn, 20-HETE formation in vascular smooth muscle is inhibited by nitric oxide (85–87). This is because NO can inhibit heme-containing proteins such as CYP (88). Elevated 20-HETE causes endothelial dysfunction and impairs coronary collateral growth in the metabolic syndrome (89). Urinary excretion of 20-HETE has also been shown to be associated with endothelial dysfunction (90).
Endothelial cells in the renal, cerebral, and coronary arteries produce EET from arachidonic acid (80, 91–95). They have vasodilators and are known as endothelium-derived hyperpolarizing factor (EDHF), which hyperpolarizes vascular smooth muscle cells (VSMCs) by activating large-conductance calcium-activated K + channels (79, 93, 96). Thus, ω-3 PUFAs enhance endothelium-dependent vasorelaxation through enhanced release of EDRF and vasodilatory prostaglandins (97). 20-HETE has been shown to inhibit and EETs to activate renal microvascular smooth muscle cell large-conductance calcium-activated K + channels (80).
Interestingly, an analysis of the prognostic impact of PUFA metabolites on clinical outcomes in coronary heart disease showed that the incidence of future myocardial infarction was more frequent in patients with higher baseline levels of 8- HETE, 9-HETE, 11-HETE, 12-HETE, 15-HETE, 19-HETE, 20-HETE, 5,6-EET, 8,9-EET, 11,12-EET and 14-15-EET (98).
In another study it was shown that prostanoids derived from EPA exerts an endothelium-independent vasorelaxant effect in the isolated aorta of Wistar Kyoto (WKY) rats by activating ATP-sensitive potassium channels (KATP) (99). Docosahexaenoic acid can be metabolized to epoxidocosapentaenoic acids via CYP450, which can activate KATP at high concentrations of docosahexaenoic acid, whereas low concentrations act through Ca2 + -mediated modulation (100). EPA can also directly modulate intracellular Ca2 + signaling in VSMCs, which contributes to a vasodilatory effect (101).
Thus, ω-3 PUFAs exhibit endothelium-independent vasodilatory effects that are associated with the opening of large conductance calcium-activated potassium channels, KATP and members of the Kv7 family of voltage-gated potassium channels in VSMCs (102). The resulting effect is hyperpolarization and relaxation of VSMCs and, consequently, a vasodilatory effect (102).
Role of polyunsaturated fatty acids in the biophysics of plasma membranes
Fatty acids that are part of plasma membrane lipids are involved in the regulation of membrane biophysical properties and physiological functions (103). Chain length and degree of unsaturation are important factors determining the role of ω-3 PUFAs in cell membranes. The lipid bilayer of the plasma membrane has a complex structural organization in which the leading role is assigned to the spatial orientation of cholesterol molecules as well as the fatty acid tails of phospholipids, whose unsaturation can significantly influence such biophysical properties as fluidity (104). At the same time, the biophysical properties of plasma membranes provide functions for many membrane proteins (105, 106). Lipid ordering is known to affect the possibility of necessary conformational changes in proteins to perform their functions. Fatty acids in membrane phospholipids are involved in maintaining the biophysical properties of plasma membranes, providing, on the one hand, optimal fluidity to allow proteins to perform the conformational changes required for their function and, on the other hand, providing sufficient viscosity, which is required for their membrane localization (107, 108). Phospholipid tails of saturated fatty acids maintain lipid organization, whereas phospholipids containing polyunsaturated fatty acids are significantly more disordered. ω-3 PUFAs are incorporated into phospholipids of lipid domains, displacing cholesterol and affecting the molecular order of lipid microdomains (109). This is due to the fact that PUFAs chains have many rapidly changing conformations that push away the rigid steroid moiety of cholesterol molecule, affecting the lipid ordering of the membrane (109, 110). Meanwhile, unordered PUFA-rich domains coexist with highly ordered lipid rafts enriched in sphingolipids and cholesterol (111). The incorporation of ω-3 PUFAs into phospholipids of plasma membranes has enormous potential to change the organization of their molecular architecture, to remodel lipid-protein interactions and the functions of membrane proteins (109).
It was shown that eicosapentaenoic acid changes the lipid composition in caveolae and induces eNOS translocation from caveolae to soluble fractions, which was accompanied by a stimulated ability to produce NO in cells (112). In turn, docosahexaenoic acid changes the microenvironment of caveolae not only by changing the lipid composition of the membrane but also by changing the distribution of basic structural proteins, such as caveolin-1, and also promotes eNOS displacement from caveolae (113). Moreover, treatment of cells with docosahexaenoic acid significantly increases eNOS activity compared to the control (113). In addition, docosahexaenoic acid was shown to enhance eNOS and Akt activity, increase HSP90 (heat shock protein 90) expression, and increase NO bioavailability in response to Akt-kinase activation (114).
Interestingly, PUFAs can have different effects on the lipid organization of membranes, depending on the chain length of the fatty acids and the degree of unsaturation. Eicosapentaenoic acid largely avoids interactions within lipid rafts and prefers a disordered non-raft region, whereas docosahexaenoic acid can be incorporated lipid rafts, modifying their biophysical organization (115). Eicosapentaenoic acid has been shown to decrease membrane fluidity, inhibit cholesterol domain formation, and normalize bilayer width in atherosclerotic-like model membranes (116). At the same time, docosahexaenoic acid had no effect on membrane width, but induced formation of cholesterol domains and increased fluidity of the model membrane (116). Thus, exposure to docosahexaenoic acid may increase the fluidity of the plasma membrane of endothelial cells to a greater extent than eicosapentaenoic acid (117). This effect may be related to the greater ability of docosahexaenoic acid to reduce membrane cholesterol content or the molar ratio of cholesterol to phospholipids (117). Thus, eicosapentaenoic acid and docosahexaenoic acid can mutually counter-regulate each other’s physiological effects in different tissues (115).
Docosahexaenoic acid has 22 carbon atoms and 6 double bonds, being the most unsaturated and one of the longest ω-3 PUFAs, thus having a significant effect on the biophysical properties of plasma membranes (110). It has been shown that docosahexaenoic acid molecules can spontaneously assemble into nanoclusters and localize in liquid-disordered membrane domains (118). Docosahexaenoic acid-rich membranes are fluid, have increased permeability, and are thin, due to the high disorder of the acyl chains and their reduced effective length (110). In addition, docosahexaenoic acid makes simulated bilayer phospholipids less sensitive to applied surface tension than saturated phospholipids, which, suggests a decrease in membrane elasticity, i.e., area elastic modulus, bending rigidity (119). Most tissues typically contain docosahexaenoic acid well below 5 mol% of the total acyl chains, but this amount increases with PUFA supplementation (110).
Numerous data indicate the effect of PUFAs on cardiac ion channels, which affects the occurrence of arrhythmias. Moreover, the effect of PUFAs on the biophysical properties of ion channels and cardiac electrophysiology may differ significantly during acute and long-term administration of PUFAs, when they are gradually incorporated into plasma membranes (120). It was found that acute administration of ω3-PUFAs reduces the cardiac sodium current (INa), which corresponds to a reduction in excitability and a slowing of ventricular conduction, whereas membrane incorporated ω-3 PUFAs do not alter INa (121–127). The ability of docosahexaenoic acid, eicosapentaenoic acid and alpha-linolenic acid to block cardiac sodium currents was found to correlate with their ability to increase membrane fluidity of isolated adult rat ventricular myocytes (123).
In addition, acute administration of ω-3 PUFAs to ventricular myocytes reduced voltage-gated L-type calcium currents (ICa,L), lowering the plateau of the action potential (128, 129). At the same time, ω-3 PUFAs incorporated into the sarcolemma also reduce ICa,L and shortened pig ventricular action potentials (126). Spontaneous calcium release from the sarcoplasmic reticulum, which underlies after-depolarization associated arrhythmias in heart failure, was also found to be reduced after acute administration of eicosapentaenoic acid to isolated ventricular myocytes in rabbits with heart failure (120, 130, 131). The inhibitory effect of ω-3 PUFAs on cardiac Ito (transient outward potassium current) and IK (delayed rectifier potassium current) was also shown to be less effective compared with their effect on cardiac Na and Ca currents (132).
These data indicate a significant effect of PUFAs on cell membrane biophysical properties and cardiac electrophysiology. However, acute and long-term administration to PUFAs differs. At the same time, PUFAs may have both pro- and antiarrhythmic effects (126).
Thus, ω-3 PUFAs, when incorporated into membrane phospholipids, may influence the biophysical properties of membranes and the function of membrane proteins, which, in addition to their role as lipid mediators, is a promising area for future research. The extensive data available to date demonstrate the multifaceted role of PUFAs in many physiological and pathological processes. As has been shown in many studies ω-3 PUFAs demonstrate predominantly atheroprotective effects, which formed the basis for evaluating their preventive and therapeutic efficacy. Dietary and circulating eicosapentaenoic acid and docosahexaenoic acid have been shown to be inversely associated with the incidence of cardiovascular disease. No significant associations with cardiovascular disease were observed for alpha-linolenic acid and ω-6 PUFAs (linoleic acid, arachidonic acid) (133).
Participation of polyunsaturated fatty acids in other processes
Disorder of reverse cholesterol transport is considered to be an important part of the pathogenesis of atherosclerosis. A large number of data indicate a role of ABCA1 and ABCG1 in the regulation of cholesterol transport from macrophages and HDL formation. A decrease in the expression and functional activity of these transporters is associated with the formation of “foam cells” and the progression of atherosclerosis. Unsaturated fatty acids such as palmitoleic, oleic, and linoleic acids have been shown to reduce ABCA1 expression at transcriptional as well as posttranscriptional levels, reducing cholesterol efflux (134, 135). Eicosapentaenoic and oleic acids also reduced ABCA1 promoter activity in macrophages. Unsaturated fatty acids also suppressed ABCG1 gene expression through a mechanism that involves LXR/RXR binding to promoters (136). In addition, inclusion of eicosapentaenoic acid in the membrane impairs ABCA1-dependent cholesterol efflux through the protein kinase A signaling pathway in primary human macrophages (137). Inclusion of eicosapentaenoic acid in the membrane impairs cholesterol efflux from cholesterol-laden human macrophages also by reducing the mobilization of cholesterol ester from lipid droplets (138). On the other hand, incorporation of eicosapentaenoic acid into the membranes increased ABCA1 functionality in cholesterol normal human THP-1 macrophages, probably through a combination of different mechanisms (139). In addition, dietary fish consumption and ω-3 PUFAs were associated with levels of ABCA1 leukocyte DNA methylation (140).
Interestingly, the heart tissue of mice on a high-fat diet (HFD) showed an increase in arachidonic, linoleic, and docosahexaenoic acids and a decrease in eicosapentaenoic acid (141). The incorporation of PUFAs into the cell membrane can change the biophysical properties of the membrane and affect cardiac function (141).
Linoleic acid is a biochemical precursor of arachidonic acid, which is used to synthesize proinflammatory lipid mediators (142). Linoleic acid can be converted to arachidonic acid, and α-linolenic acid can be metabolized to eicosapentaenoic acid and docosahexaenoic acid (143, 144). In addition, linoleic acid is a substrate for the synthesis of bioactive oxidized metabolites such as 9- and 13-hydroxyoctadecadienoic acid (9- and 13-HODE), which may be involved in various processes related to atherogenesis (145–147). We found that the level of 9-HODE obtained from LDL of young patients with atherosclerosis was increased 20-fold compared with samples taken from healthy individuals (146). 9-HODE and 13-HODE stimulate the release of IL-1β from macrophages (148). In addition, linoleic acid exposure increases the transport of human LDL through cultured endothelial monolayers (149).
Atheroma samples were shown to contain a higher proportion of linoleic acid (150). Consumption of more linoleic acid increases its amount in complicated aortic plaques (151). On the other hand, 13-HODE was found to induce cholesterol efflux from macrophages through the PPAR-LXRa-ABCA1/SR-BI pathway (152).
Thus, PUFAs are involved in various physiological and pathological processes, whose complex relationships may be disturbed during atherogenesis.
Clinical significance of polyunsaturated fatty acids
Accumulating evidence suggests a potential benefit of ω-3 PUFAs in the prevention of cardiovascular disease. Fish oil supplementation in healthy volunteers was associated with improved endothelial function and decreased resting heart rate (153). In addition, ω-3 PUFA supplementation significantly improved endothelial function and reduced inflammatory markers in the offspring of patients with type 2 diabetes (154). Dietary supplements containing marine ω-3 PUFAs improved endothelium-dependent dilatation of large arteries in patients with hypercholesterolemia but had no effect on endothelium-independent dilatation (155). Fish oil supplements increased the number of endothelial progenitor cells and decreased the number of endothelial microparticles, potentially contributing to endothelial integrity (156).
A randomized, placebo-controlled trial including 53 mildly hypertriacylglycerolemic subjects evaluated the potential benefit of daily ω-3 PUFA supplementation for improving cardiovascular health. After 10 weeks, subjects taking ω-3 PUFAs at 3 grams per day had significantly increased ω-3 PUFA levels in plasma and erythrocytes. In addition, the EPA-derived mediators PGE3, 12- HEPE, 15- HEPE, and 18-HEPE increased in plasma. This was consistent with improvements in cardiovascular risk factors such as HDL levels, triacylglycerides, arachidonic acid/eicosapentaenoic acid ratio, and ω-3 index (157).
Results from a recent pooled analysis of 4 international cohort trials including 191,558 individuals from 58 countries showed that a minimum fish consumption of 175 grams (approximately 2 servings) per week was associated with a lower risk of major cardiovascular disease and mortality among patients with prior cardiovascular disease, but not in the general population (158).
A comprehensive review of 34 meta-analyses of prospective cohort studies on the association of fish consumption and the risk of cardiovascular disease found that an increase in fish consumption of 100 grams per day was associated with a lower risk of all-cause mortality (summary relative risk (SRR): 0.92; 95% confidence interval (CI): 0.87, 0.97), cardiovascular mortality (SRR: 0.75; 95% CI: 0.65, 0.87), risk of coronary heart disease (SRR: 0.88; 95% CI: 0.79, 0.99), myocardial infarction (SRR: 0.75; 95% CI: 0.65, 0.93), stroke (SRR: 0.86; 95% CI: 0.75, 0.99), and heart failure (SRR: 0.80; 95% CI: 0.67, 0.95) (159).
An analysis of 49 randomized controlled trials, with durations ranging from one to eight years and included 24,272 participants found that increasing PUFA intake had little no effect on all-cause mortality or cardiovascular disease (risk 7.8 vs 7.6%, risk ratio (RR) 0.98, 95% CI 0.89–1.07). However, it slightly reduces the risk of coronary heart disease (from 14.2 to 12.3%, RR 0.87, 95% CI 0.72 -1.06) and cases of cardiovascular disease events (from 14.6 to 13.0%, RR 0.89, 95% CI 0.79–1.01), and may also slightly reduce the risk of mortality from coronary heart disease (6.6–6.1%, RR 0.91, 95% CI 0.78–1.06) (160).
The INTERMAP (The International Study of Macro- and Micro-nutrients and Blood Pressure) trial showed that ω-3 PUFA intake was inversely related to blood pressure, including in non-hypertensive individuals (161). A meta-analysis of 7 randomized controlled trials showed that EPA + DHA intake lowered blood pressure, most significantly in patients with untreated hypertension (162). In this group of patients, the decrease in systolic blood pressure was 4.51 mm Hg (95% CI =−6.12 to–2.83 mm Hg) and the decrease in diastolic blood pressure was 3.05 mm Hg (95% CI =−4.35 to −1.74 mm Hg). Another study, although it did not confirm the role of ω-6 or ω-3 PUFA intake in changes in blood pressure over time, did show a possible interaction of ω-3 PUFA with the CYP4F2 V433M genotype (163). In a study of 81,579 participants with arterial hypertension, fish oil intake was associated with an 8% reduction in cardiometabolic multimorbidity risk (95% CI 0.89–0.96, p < 0.001) and a 10% reduction in all-cause mortality (95% CI 0.85–0.95, p < 0.001) (164). Fish oil was associated with a low incidence of sustained ventricular fibrillation episodes in the monkey (165).
A meta-analysis of 13 studies (GISSI-P, JELIS, GISSI-HF, DOIT, SU.FOL.OM3, Alpha Omega, OMEGA, ORIGIN, R&P, AREDS-2, VITAL, ASCEND, REDUCE-IT) including a total of 127,477 patients showed that marine ω-3 PUFAs supplementation significantly reduced risk of myocardial infarction (RR 0.92, 95% CI 0.86–0.99, p = 0.020), coronary heart disease (RR 0.95, 95% CI 0.91–0.99, p = 0.008) and overall cardiovascular morbidity (RR 0.97, 95% CI 0.94–0.99, p = 0.015) (166). A meta-analysis of 38 randomized controlled trials involving 149,051 participants showed that ω-3 PUFAs reduce cardiovascular mortality (RR, 0.93 95% CI 0.88–0.98, p = 0.01) and improve cardiovascular outcomes. The risk of non-fatal myocardial infarction (RR, 0.87 95% CI 0.81–0.93, p = 0.0001), coronary heart disease events (RR, 0.91 95% CI 0.87–0.96, p = 0.0002) was shown to decrease. The reduction in cardiovascular risk was more pronounced with eicosapentaenoic acid monotherapy than with docosahexaenoic acid (167). Importantly, ω-3 PUFAs increased the incidence of atrial fibrillation (OR 1.26 [1.08–1.48]), and eicosapentaenoic acid monotherapy compared with the control group was associated with a higher risk of total bleeding (OR: 1.49 [1.20–1.84]) and atrial fibrillation (OR: 1.35 [1.10–1.66]) (167).
Thus, there is evidence for the promising use of PUFAs in clinical practice due to their diverse functions. At the same time, the data on the clinical efficacy of PUFAs vary.
While earlier studies showed predominantly positive effects, more recent studies have shown no significant clinical effects of PUFA supplementation and, in some cases, negative results. In a large cohort of patients (12,513) with multiple cardiovascular risk factors, daily treatment with ω-3 PUFAs did not reduce cardiovascular mortality or morbidity compared with placebo (olive oil) [11,7% vs 11,9% (RR 0.97, 95% CI 0.88–1.08, p = 0.58)] (168). Treatment with ω-3 PUFAs for 3 months did not improve endothelial function in patients with type 2 diabetes and very high cardiovascular risk (169). Consumption of ω-3 PUFAs at a dose ≤ 1.8 g/d for 12 months also did not improve endothelial function in healthy adults (170).
In addition to examining the role of ω-3 PUFAs, there is increasing interest in finding the optimal ratio of ω-6/ω-3 PUFAs (171). The significance of ω-6 PUFAs for cardiovascular disease has also been the subject of much research over a long period of time (172). Linoleic acid is an essential ω-6 fatty acid because it cannot be synthesized by humans (173). It is the most common PUFAs in the human diet, as significant amounts of linoleic acid are present in vegetable oils.
Linoleic acid has been shown to reduce LDL cholesterol levels (174). And higher intake of linolenic acid was associated with a lower risk of atherosclerotic plaques in the carotid arteries (175). A meta-analysis of 30 prospective studies involving 68,659 participants found that higher concentrations of linolenic acid were significantly associated with lower risks of overall cardiovascular disease (hazard ratio (HR) 0.93, 95% CI 0.88–0.99), cardiovascular mortality (HR 0.78, 95% CI 0.70–0.85), and risks of ischemic stroke (HR 0.88, 95% CI 0.79–0.98) (176). An analysis of 13 cohort studies with a total of 310,602 participants showed that dietary intake of linoleic acid was inversely associated with the risk of coronary heart disease in a dose-dependent manner (177). On the other hand, evaluation of reconstructed data from the Sydney Dietary Heart Study and an updated meta-analysis showed that dietary linoleic acid use from safflower oil increased the risk of death from coronary heart disease and cardiovascular disease (178). The study, which included 20,000 middle-aged men and women in the Netherlands, found that linoleic acid intake was not associated with the ratio of total cholesterol to HDL cholesterol, and no association was found between linoleic acid intake and the incidence of CHD over a 10-year follow-up period (179).
Cost-effectiveness analysis of long-term treatment with ω-3 PUFAs after myocardial infarction showed comparable results with other drugs for secondary prevention after myocardial infarction (180). Another five-country study showed that adding highly concentrated ω-3 PUFAs to standard treatment for secondary prevention of myocardial infarction was cost-effective compared with standard treatment alone (181). Combination therapy with eicosapentaenoic acid and statins showed acceptable cost-effectiveness for secondary prevention of cardiovascular disease in patients with hypercholesterolemia in Japan (182). Adding ω-3 PUFAs to optimal drug therapy for patients with heart failure may also be cost-effective (183). Treatment with icosapent etyl has been associated with both higher costs and higher benefits (184). Omacor has also been shown to be cost-effective as a prophylactic after myocardial infarction, both after 4 years and during life (185).
Thus, the clinical significance of PUFAs for cardiovascular disease is the subject of research and debate, but all questions have not been answered to date.
The significance of polyunsaturated fatty acids in coronary heart disease
A significant number of studies have analyzed the diagnostic, prophylactic and therapeutic value of PUFAs. It has been shown that patients with CHD have lower levels of ω-6 and ω-3 PUFAs, especially eicosapentaenoic acid, in the blood (186). In a study including 2,692 American adults aged 74 ± 5 years, high individual and overall levels of circulating ω-3 PUFAs (eicosapentaenoic acid, docosapentaenoic acid, and docosahexaenoic acid) were found to be associated with lower overall mortality, especially mortality from CHD (187).
In the GISSI-Prevenzione trial, dietary supplements with ω-3 PUFAs in patients who had had a myocardial infarction resulted in clinically important effects, providing the basis for their therapeutic use (188). However, in a mortality risk assessment study of 3,114 men under age 70 with angina who ate fatty fish or fish oil, no beneficial effect was shown. Moreover, men who ate this diet had a higher risk of cardiac death (189). In a multicenter trial including 4,837 patients aged 60–80 years (78% men) who had had a myocardial infarction in addition to current antihypertensive, antithrombotic, and lipid-modifying therapies and received various combinations of marine ω-3 fatty acids eicosapentaenoic acid and docosahexaenoic acid and plant alpha linolenic acid and placebo. The results of the study showed no significant reduction in the incidence of serious cardiovascular complications when low-dose eicosapentaenoic acid-docosahexaenoic acid or alpha-linolenic acid were taken (190).
The JELIS (Japan EPA Lipid Intervention Study) evaluated the effect of eicosapentaenoic acid on major coronary events in 18,645 patients with hypercholesterolemia. It found that unstable angina and non-fatal coronary events were significantly reduced in the eicosapentaenoic acid group. There were no differences in sudden cardiac death and coronary death (191).
In the OMEGA-REMODE randomized clinical trial, administration of high-dose omega-3 fatty acids for 6 months to patients undergoing acute myocardial infarction was associated with a reduction in adverse left ventricular remodeling as well as non-infarct myocardial fibrosis and serum biomarkers of systemic inflammation (192). More recently, genetic profiling of fatty acid desaturase 2 (FADS2) polymorphisms has been shown to identify patients who may benefit from high-dose omega-3 PUFAs for cardiac remodeling after acute myocardial infarction (193).
An analysis of 86 randomized controlled clinical trials (162,796 participants) that lasted at least 12 months showed little or no effect of increasing omega-3 PUFA intake on all-cause mortality, cardiovascular mortality, and cardiovascular events. However, ω-3 PUFA intake may marginally reduce the risk of mortality from coronary heart disease and complications of coronary heart disease (194).
A recent meta-analysis including 14 randomized controlled clinical trials (135,291 patients) showed that taking ω-3 PUFAs reduced the risk of major adverse cardiovascular events, cardiovascular death, and myocardial infarction, but had no significant effect on all-cause mortality (195). And taking ω-3 PUFAs can prevent myocardial infarction regardless of the stage of CHD (195).
Thus, data from various studies demonstrate different clinical efficacy of ω-3 PUFAs in the prevention and treatment of coronary heart disease, which requires additional research.
The significance of polyunsaturated fatty acids in peripheral arterial disease
Peripheral artery disease (PAD) is another important clinical problem associated with endothelial dysfunction, inflammation, and atherosclerosis. PAD was found to be deficient in ω-3 PUFAs in the erythrocyte membrane and to have a lower ratio of eicosapentaenoic acid to arachidonic acid (196). In a randomized, placebo-controlled, double-blind trial in 70 patients with peripheral arterial disease, endothelial function was shown to improve without affecting pain-free walking distance after 3 months of treatment with 4 grams of ω-3 PUFAs (197).
In another study, administration of ω-3 PUFAs for 3 months to patients with PAD, caused a marked improvement in endothelial function but had no effect on inflammatory status (198). The OMEGA-PAD I Trial, which evaluated the effect of short-term high-dose ω-3 PUFAs, showed no difference in endothelial function between the treatment and placebo groups. However, there was an increase in production of markers of specialized pro-resolving mediators, in patients with PAD (199). The OMEGA-PAD II Trial, which was conducted to evaluate the effect of high-dose oral ω-3 PUFA supplementation for 3 months on inflammation, endothelial function, and walking ability in patients with PAD, showed increased SPMs in the plasma of patients with PAD (200).
Significance of polyunsaturated fatty acids in atrial fibrillation
Atrial fibrillation (AF) is an urgent medical problem due to its high prevalence and impact on prognosis. This information strengthens the understanding of the importance of atrial fibrillation prevention.
Increased serum concentrations of long-chain ω-3 PUFAs have been shown to be associated with a lower risk of AF. A prospective population-based study of coronary heart disease risk factors, including 2,174 men followed for 17.7 years, showed that increased serum concentrations of long-chain ω-3 PUFAs may protect against AF. Serum docosahexaenoic acid concentration showed the greatest effect on the risk of AF (201). Another study of 3,326 men and women aged ≥ 65 years found that higher plasma levels of total long-chain ω-3 PUFAs and docosahexaenoic acid were associated with a lower risk of AF (202).
Animal studies have provided evidence for a positive role of ω-3 PUFAs for the prevention of atrial fibrillation. Eicosapentaenoic acid prevented atrial fibrillation associated with heart failure in a rabbit model (203). Dietary ω-3 fatty acids reduced atrial infiltration by inflammatory cells and reduced the inducibility of postoperative atrial fibrillation in a canine cardiac surgery model (204).
However, many clinical studies have failed to confirm the positive role of PUFA supplementation for the prevention of AF.
The STRENGTH randomized clinical trial included 13,078 patients with high cardiovascular risk treated with statins. Adding omega-3 eicosapentaenoic acid and docosahexaenoic acid to conventional background therapy compared with corn oil did not result in a significant difference in a composite outcome of major adverse cardiovascular events. However, the ω-3 PUFA group (24.7%) had a higher incidence of gastrointestinal adverse events compared with patients receiving corn oil (14.7%). Interestingly, patients taking ω-3 PUFA had a higher incidence of first-time atrial fibrillation (2.2% vs. 1.3%) compared with corn oil (205).
Another trial, including 25,119 participants aged 50 years or older, evaluated the effect of long-term ω-3 fatty acid (eicosapentaenoic acid and docosahexaenoic acid) and vitamin D supplementation on the incidence of AF. The results of the study showed no significant difference in the risk of AF over a follow-up period of more than 5 years among the groups taking eicosapentaenoic acid – docosahexaenoic acid or vitamin D3 compared with placebo (206).
In the REDUCE-IT trial, patients who received highly purified ethyl ester of eicosapentaenoic acid (icosapent ethyl) had a higher risk of hospitalization for AF compared with placebo (3.1% versus 2.1%) over a median of 4.9 years (207). Moreover, the risk of ischemic events, including cardiovascular death, was significantly lower among patients taking icosapent ethyl than among those receiving placebo (207).
In the OMEMI (Omega-3 Fatty acids in Elderly with Myocardial Infarction) randomized clinical trial, elderly patients after myocardial infarction received omega-3 fatty acids (a combination of eicosapentaenoic acid and docosahexaenoic acid) or corn oil for 2 years. Results showed no reduction in clinical events, whereas 7.2% of patients in the omega-3 fatty acid group versus 4.0% in the corn oil group developed atrial fibrillation (208). A secondary analysis of the OMEMI trial showed that a greater increase in eicosapentaenoic acid during treatment was associated with a higher risk of first-onset AF (209).
The Danish cohort study (n = 54,737) showed no association between ω-3 or ω-6 PUFAs and atrial fibrillation (210). A randomized, double-blind, multicenter trial of ω-3 PUFAs showed no efficacy in preventing arrhythmia recurrence after electrical cardioversion of chronic persistent atrial fibrillation (211).
These data suggest that ω-3 fatty acid intake does not show a reduced risk of atrial fibrillation. In addition, in another large trial [The Omega-3 Fatty Acids for Prevention of Post-operative Atrial Fibrillation (OPERA)] in which patients received perioperative ω-3-PUFA supplementation before heart surgery found no reduction in the risk of postoperative AF compared with placebo (212).
A meta-analysis of randomized controlled trials showed that preoperative PUFA treatment had no effect on the incidence of postoperative atrial fibrillation in patients undergoing open-heart surgery (213). In addition, a recent systematic review and meta-analysis of 7 studies involving 81,210 patients showed that long-term supplementation with marine ω-3 PUFAs was associated with an increased risk of atrial fibrillation (HR 1.25, 95% CI 1.07-1.46, p = 0.013). The increased risk of atrial fibrillation was most significant with omega-3 PUFA supplementation at doses > 1 g/day (214).
The findings suggest that other studies are needed to evaluate the molecular mechanisms linking the incidence of AF to PUFAs intake.
It should be noted that ω-3 PUFAs demonstrate both antiarrhythmic and proarrhythmic effects through their action on different mechanisms (120). Given that different arrhythmogenic mechanisms are characteristic of different patient subgroups, the effects of ω-3 PUFAs on different types of arrhythmias require further study (120).
The importance of polyunsaturated fatty acids in heart failure
Heart failure is a complex syndrome that is the final outcome of the progression of many cardiovascular diseases, such as coronary heart disease and arterial hypertension. Heart failure is an important cause of reduced quality of life, hospitalizations, and death. The rate of progression of heart failure depends on many factors and is an important target for therapeutic interventions, primarily regarding its causes. Because of the great medical and social significance of heart failure, new effective therapies are required. Numerous studies have investigated the possible benefits of taking ω-3 PUFAs as a means of preventing and treating heart failure.
In the MESA (Multi-Ethnic Study of Atherosclerosis) trial, in 6,562 participants aged 45–84 years, followed for 13.0 years, higher plasma eicosapentaenoic acid levels were significantly associated with a reduced risk of heart failure in both patients with reduced and preserved ejection fractions (215). Over a follow-up period of 15.6 years, higher plasma levels of ω-3 fatty acids were shown to be associated with fewer long-term cardiovascular events. And the absolute reduction in the incidence of cardiovascular disease with higher levels of ω-3 PUFAs was more evident with higher coronary artery calcium levels (216). In addition, another study showed that the eicosapentaenoic acid/arachidonic acid ratio in the blood was an independent predictor of cardiac mortality in patients with heart failure (217). And the most pronounced effect of the eicosapentaenoic acid/arachidonic acid ratio on cardiac mortality was among patients shown to be taking statins.
In a recent meta-analysis that included 13 studies, it was shown that high dietary intake of ω-3 PUFAs was associated with a lower risk of heart failure in 8 of those studies, which included more than 300,000 participants (218). Another six studies involving 17,163 participants showed an association of higher concentrations of circulating ω-3 PUFAs with a lower risk of heart failure (218). In addition, high circulating concentrations of docosahexaenoic acid have been shown to be associated with a reduced risk of heart failure, while no significant association was found for eicosapentaenoic and docosapentaenoic acids (218).
Interestingly, eicosapentaenoic acid, but not docosahexaenoic acid, prevents fibrosis in pressure overload-induced heart failure, due to a potential role of the free fatty acid receptor 4 (Ffar4) linked to G-protein (219). Eicosapentaenoic acid prevents cardiac fibrosis and diastolic dysfunction in heart failure with preserved ejection fraction signaling through Ffar4 (220). The cardioprotective mechanisms of eicosapentaenoic acid in heart failure are related to its ability to activate NRF2 (nuclear factor erythropoietin 2 related factor 2), Ffar4 receptor (free fatty acid receptor 4), or GPR120 fibroblast receptors. This activation inhibits cardiac fibrosis and protects the heart from heart failure (221). NRF2 is regulated by specialized pro-resolving mediators derived from docosahexanoic acid and eicosapentaenoic acid (222). NRF2 is a transcription factor that participates in the control of oxidative stress, thus providing myocardial protection against the occurrence of fibrosis (222). In addition, Nrf2 was shown to suppress TNF-α-induced monocyte chemoattractant protein (MCP)-1 and VCAM-1 mRNA and protein expression in a dose-dependent manner and inhibited TNF-α-induced adhesion of human monocytic U937 cells to human aortic endothelial cells (HAEC) (223).
These data suggest that the molecular mechanisms and clinical effects of various PUFAs need to be better understood. Clinical, and corresponding pathophysiological differences in heart failure forms, should be considered in studies to evaluate the role of different PUFAs.
Conclusion
The problem of cardiovascular disease remains one of the most urgent topics in modern medicine. A large number of patients, a significant impact on the quality of life, ability to work and prognosis, is a serious economic and social burden for patients, their families, society and health care systems in many countries. The importance of the problem is also underscored by the fact that a significant number of cardiovascular diseases today have limited therapeutic prospects. This and other information reinforces the importance of preventive measures that could reduce the risks of cardiovascular complications. ω-3 PUFAs have been considered for a relatively long time as promising agents for the prevention and treatment of several cardiovascular diseases.
Accumulated evidence suggests that the cardioprotective properties of ω-3 PUFAs are associated with their anti-inflammatory effects, participation in the regulation of the biophysical properties of plasma membranes, modification of signaling pathways and cardiac ion channels, and improvement of endothelial function.
Despite the large number of studies conducted, many of the data obtained are contradictory and, when analyzed in combination, do not give confidence in the full therapeutic efficacy of ω-3 PUFAs (Table 1). Moreover, analysis of many recent studies has shown that the benefit of PUFAs in preventing cardiovascular events may be exaggerated. In particular, an increased risk of atrial fibrillation with PUFAs has been shown.
At the same time, it should be noted that many studies do not take into account the influence of concomitant factors, including physical activity levels and patients’ attitudes toward treatment. In addition, the importance of other nutritional factors, including other oils taken by patients, which may have influenced the outcome of the studies, must be considered.
Interestingly, salmon (Salmo salar L), which is a good dietary source of PUFAs, itself suffers from coronary atherosclerosis (224, 225). And a diet enriched in cholesterol led to a worsening of lipid indices and progression of lesions (226). These and other data underscore the need for a comprehensive evaluation of various factors.
Thus, PUFAs are involved in the regulation of a large number of mechanisms that have complex cross-linkages and are associated with cardiovascular disease. This requires new research that would expand the boundaries of our understanding of the processes that occur in the vascular wall and how these processes can be effectively influenced.
Author contributions
SK and AK contributed to the conception of research and wrote the manuscript. SK contributed to the revision of the manuscript. Both authors approved the final manuscript for submission.
Conflict of interest
The authors declare that the research was conducted in the absence of any commercial or financial relationships that could be construed as a potential conflict of interest.
Publisher’s note
All claims expressed in this article are solely those of the authors and do not necessarily represent those of their affiliated organizations, or those of the publisher, the editors and the reviewers. Any product that may be evaluated in this article, or claim that may be made by its manufacturer, is not guaranteed or endorsed by the publisher.
References
1. Herrington W, Lacey B, Sherliker P, Armitage J, Lewington S. Epidemiology of atherosclerosis and the potential to reduce the global burden of atherothrombotic disease. Circul Res. (2016) 118:535–46. doi: 10.1161/CIRCRESAHA.115.307611
2. Song P, Fang Z, Wang H, Cai Y, Rahimi K, Zhu Y, et al. Global and regional prevalence, burden, and risk factors for carotid atherosclerosis: a systematic review, meta-analysis, and modelling study. Lancet Global Health. (2020) 8:e721–9. doi: 10.1016/S2214-109X(20)30117-0
3. Peters SAE, den Ruijter HM, Bots ML, Moons KGM. Improvements in risk stratification for the occurrence of cardiovascular disease by imaging subclinical atherosclerosis: a systematic review. Heart. (2012) 98:177–84. doi: 10.1136/heartjnl-2011-300747
4. Costa J, Alarcão J, Araujo F, Ascenção R, Caldeira D, Fiorentino F, et al. The burden of atherosclerosis in portugal. EurHeart J Qual Care Clin Outcomes. (2020) 7:154–62. doi: 10.1093/ehjqcco/qcaa060
5. Bian L, Xia L, Wang Y, Jiang J, Zhang Y, Li D, et al. Risk factors of subclinical atherosclerosis and plaque burden in high risk individuals: results from a community-based study. Front Physiol. (2018) 9:739. doi: 10.3389/fphys.2018.00739
6. Visseren FLJ, Mach F, Smulders YM, Carballo D, Koskinas KC, Bäck M, et al. 2021 Esc guidelines on cardiovascular disease prevention in clinical practice. Eur Heart J. (2021) 42:3227–337. doi: 10.1093/eurheartj/ehab484
7. Visseren FLJ, Mach F, Smulders YM, Carballo D, Koskinas KC, Bäck M, et al. 2021 Esc guidelines on cardiovascular disease prevention in clinical practice: developed by the task force for cardiovascular disease prevention in clinical practice with representatives of the European society of cardiology and 12 medical societies with the special contribution of the European association of preventive cardiology. (Eapc). Eur J Prev Cardiol. (2021) 29:5–115. doi: 10.1093/eurjpc/zwab154
8. van Trier TJ, Mohammadnia N, Snaterse M, Peters RJG, Jørstad HT, Bax WA. Lifestyle management to prevent atherosclerotic cardiovascular disease: evidence and challenges. Netherlands Heart J. (2022) 30:3–14. doi: 10.1007/s12471-021-01642-y
9. Mach F, Baigent C, Catapano AL, Koskinas KC, Casula M, Badimon L, et al. 2019 Esc/Eas guidelines for the management of dyslipidaemias: lipid modification to reduce cardiovascular risk: the task force for the management of dyslipidaemias of the European Society of Cardiology. (Esc) and European Atherosclerosis Society. (Eas). Eur Heart J. (2019) 41:111–88. doi: 10.1093/eurheartj/ehz455
10. Catapano AL, Ray KK, Tokgözoglu L. Prevention guidelines and Eas/Esc guidelines for the treatment of dyslipidaemias: a look to the future. Atherosclerosis. (2022) 340:51–2. doi: 10.1016/j.atherosclerosis.2021.11.021
11. Rafieian-Kopaei M, Setorki M, Doudi M, Baradaran A, Nasri H. Atherosclerosis: process, indicators, risk factors and new hopes. Int J Prev Med. (2014) 5:927–46.
12. Bang HO, Dyerberg J, Aon N. Plasma lipid and lipoprotein pattern in greenlandic west-coast eskimos. Lancet. (1971) 297:1143–6. doi: 10.1016/S0140-6736(71)91658-8
13. Kromann N, Green A. Epidemiological studies in the upernavik district, Greenland. Acta Med Scand. (1980) 208:401–6. doi: 10.1111/j.0954-6820.1980.tb01221.x
14. Bang HO, Dyerberg J, Hjørne N. The composition of food consumed by Greenland Eskimos. Acta Med Scand. (1976) 200:69–73. doi: 10.1111/j.0954-6820.1976.tb08198.x
15. Dyerberg J, Bang HO, Hjørne N. Fatty acid composition of the plasma lipids in Greenland Eskimos. Am J Clin Nutr. (1975) 28:958–66. doi: 10.1093/ajcn/28.9.958
16. Kagawa Y, Nishizawa M, Suzuki M, Miyatake T, Hamaoto T, Goto K, et al. Eicosapolyenoic acids of serum lipids of Japanese Islanders with low incidence of cardiovascular diseases. J Nutr Sci Vitaminol. (1982) 28:441–53. doi: 10.3177/jnsv.28.441
17. Dewailly E, Blanchet C, Lemieux S, Sauvé L, Gingras S, Ayotte P, et al. N−3 fatty acids and cardiovascular disease risk factors among the Inuit of Nunavik. Am J Clin Nutr. (2001) 74:464–73. doi: 10.1093/ajcn/74.4.464
18. Sirot V, Oseredczuk M, Bemrah-Aouachria N, Volatier J-L, Leblanc J-C. Lipid and fatty acid composition of fish and seafood consumed in france: calipso study. J Food Composit Anal. (2008) 21:8–16. doi: 10.1016/j.jfca.2007.05.006
19. Wen C, Jiang M, Huang W, Liu S. Antarctic krill oil attenuates oxidative stress via the keap1-Nrf2 signaling in patients with coronary heart disease. Evid Based Complement Alternat Med. (2020) 2020:9534137. doi: 10.1155/2020/9534137
20. Kotlyarov S, Kotlyarova A. Involvement of fatty acids and their metabolites in the development of inflammation in atherosclerosis. Int J Mol Sci. (2022) 23:1308. doi: 10.3390/ijms23031308
21. Samuelsson B. The discovery of the leukotrienes. Am J Respir Crit Care Med. (2000) 161(Suppl_1):S2–6. doi: 10.1164/ajrccm.161.supplement_1.ltta-1
22. Riccioni G, Bäck M, Capra V. Leukotrienes and atherosclerosis. Curr Drug Targets. (2010) 11:882–7. doi: 10.2174/138945010791320881
23. Riccioni G, Zanasi A, Vitulano N, Mancini B, D’Orazio N. Leukotrienes in atherosclerosis: new target insights and future therapy perspectives. Mediat Inflamm. (2009) 2009:737282. doi: 10.1155/2009/737282
24. Stanke-Labesque F, Pépin J-L, de Jouvencel T, Arnaud C, Baguet J-P, Petri MH, et al. Leukotriene B4 Pathway Activation and Atherosclerosis in Obstructive Sleep Apnea[S]. J Lipid Res. (2012) 53:1944–51. doi: 10.1194/jlr.P022814
25. Riccioni G, Bäck M. Leukotrienes as modifiers of preclinical atherosclerosis? Sci World J. (2012) 2012:490968. doi: 10.1100/2012/490968
26. Chandrasekharan JA, Sharma-Walia N. Lipoxins: nature’s way to resolve inflammation. J Inflamm Res. (2015) 8:181–92. doi: 10.2147/JIR.S90380
27. Clària J, Serhan CN. Aspirin triggers previously undescribed bioactive eicosanoids by human endothelial cell-leukocyte interactions. Proc Natl Acad Sci USA. (1995) 92:9475–9. doi: 10.1073/pnas.92.21.9475
28. Papayianni A, Serhan CN, Brady HR. Lipoxin A4 and B4 inhibit leukotriene-stimulated interactions of human neutrophils and endothelial cells. J Immunol. (1996) 156:2264–72.
29. Godson C, Mitchell S, Harvey K, Petasis NA, Hogg N, Brady HR. Cutting edge: lipoxins rapidly stimulate nonphlogistic phagocytosis of apoptotic neutrophils by monocyte-derived macrophages. J Immunol. (2000) 164:1663–7. doi: 10.4049/jimmunol.164.4.1663
30. McMahon B, Mitchell S, Brady HR, Godson C. Lipoxins: revelations on resolution. Trends Pharmacol Sci. (2001) 22:391–5. doi: 10.1016/S0165-6147(00)01771-5
31. Higgins G, Ringholz F, Buchanan P, McNally P, Urbach V. Physiological impact of abnormal lipoxin a4 production on cystic fibrosis airway epithelium and therapeutic potential. Biomed Res Int. (2015) 2015:781087. doi: 10.1155/2015/781087
32. Chen Z, Wu Z, Huang C, Zhao Y, Zhou Y, Zhou X, et al. Effect of Lipoxin A4 on myocardial ischemia reperfusion injury following cardiac arrest in a rabbit model. Inflammation. (2013) 36:468–75. doi: 10.1007/s10753-012-9567-x
33. Paul-Clark MJ, van Cao T, Moradi-Bidhendi N, Cooper D, Gilroy DW. 15-Epi-Lipoxin A4–mediated induction of nitric oxide explains how aspirin inhibits acute inflammation. J Exp Med. (2004) 200:69–78. doi: 10.1084/jem.20040566
34. Gomez I, Foudi N, Longrois D, Norel X. The role of prostaglandin E2 in human vascular inflammation. Prostagland Leukotr Essential Fatty Acids. (2013) 89:55–63. doi: 10.1016/j.plefa.2013.04.004
35. Gómez-Hernández A, Sánchez-Galán E, Martín-Ventura JL, Vidal C, Blanco-Colio LM, Ortego M, et al. Atorvastatin reduces the expression of prostaglandin E2 receptors in human carotid atherosclerotic plaques and monocytic cells: potential implications for plaque stabilization. J Cardiovasc Pharmacol. (2006) 47:60–9. doi: 10.1097/01.fjc.0000194252.38683.68
36. Audoly LP, Tilley SL, Goulet J, Key M, Nguyen M, Stock JL, et al. Identification of specific Ep Receptors Responsible for the Hemodynamic Effects of Pge2. Am J Physiol. (1999) 277:H924–30. doi: 10.1152/ajpheart.1999.277.3.H924
37. Zhang Y, Guan Y, Schneider A, Brandon S, Breyer RM, Breyer MD. Characterization of murine vasopressor and vasodepressor prostaglandin E receptors. Hypertension. (2000) 35:1129–34. doi: 10.1161/01.hyp.35.5.1129
38. Seki H, Fukunaga K, Arita M, Arai H, Nakanishi H, Taguchi R, et al. The anti-inflammatory and proresolving mediator resolvin e1 protects mice from bacterial pneumonia and acute lung injury. J Immunol. (2010) 184:836–43. doi: 10.4049/jimmunol.0901809
39. El Kebir D, Gjorstrup P, Filep JG. Resolvin E1 promotes phagocytosis-induced neutrophil apoptosis and accelerates resolution of pulmonary inflammation. Proc Natl Acad Sci USA. (2012) 109:14983–8. doi: 10.1073/pnas.1206641109
40. Schwab JM, Chiang N, Arita M, Serhan CN. Resolvin E1 and protectin D1 activate inflammation-resolution programmes. Nature. (2007) 447(7146):869–74. doi: 10.1038/nature05877
41. Oh SF, Pillai PS, Recchiuti A, Yang R, Serhan CN. Pro-resolving actions and stereoselective biosynthesis of 18s E-Series resolvins in human leukocytes and murine inflammation. J Clin Investig. (2011) 121:569–81. doi: 10.1172/JCI42545
42. Hasturk H, Abdallah R, Kantarci A, Nguyen D, Giordano N, Hamilton J, et al. Resolvin E1. (Rve1) attenuates atherosclerotic plaque formation in diet and inflammation-induced atherogenesis. Arterioscler Thromb Vasc Biol. (2015) 35:1123–33. doi: 10.1161/atvbaha.115.305324
43. Salic K, Morrison MC, Verschuren L, Wielinga PY, Wu L, Kleemann R, et al. Resolvin E1 attenuates atherosclerosis in absence of cholesterol-lowering effects and on top of atorvastatin. Atherosclerosis. (2016) 250:158–65. doi: 10.1016/j.atherosclerosis.2016.05.001
44. Duffield JS, Hong S, Vaidya VS, Lu Y, Fredman G, Serhan CN, et al. Resolvin D series and protectin D1 mitigate acute kidney injury. J Immunol. (2006) 177:5902–11. doi: 10.4049/jimmunol.177.9.5902
45. Serhan CN, Hong S, Gronert K, Colgan SP, Devchand PR, Mirick G, et al. Resolvins a family of bioactive products of omega-3 fatty acid transformation circuits initiated by aspirin treatment that counter proinflammation signals. J Exp Med. (2002) 196:1025–37.
46. Wang B, Gong X, Wan J-Y, Zhang L, Zhang Z, Li H-Z, et al. Resolvin D1 protects mice from lps-induced acute lung injury. Pulm Pharmacol Therapeut. (2011) 24:434–41. doi: 10.1016/j.pupt.2011.04.001
47. Bazan HA, Lu Y, Jun B, Fang Z, Woods TC, Hong S. Circulating inflammation-resolving lipid mediators rvd1 and dha are decreased in patients with acutely symptomatic carotid disease. Prostaglandins Leukot Essent Fatty Acids. (2017) 125:43–7. doi: 10.1016/j.plefa.2017.08.007
48. Spite M, Norling LV, Summers L, Yang R, Cooper D, Petasis NA, et al. Resolvin D2 is a potent regulator of leukocytes and controls microbial sepsis. Nature. (2009) 461:1287–91. doi: 10.1038/nature08541
49. Fosshaug LE, Colas RA, Anstensrud AK, Gregersen I, Nymo S, Sagen EL, et al. Early Increase of Specialized Pro-Resolving Lipid Mediators in Patients with St-Elevation Myocardial Infarction. eBioMedicine. (2019) 46:264–73. doi: 10.1016/j.ebiom.2019.07.024
50. Serhan CN, Yang R, Martinod K, Kasuga K, Pillai PS, Porter TF, et al. Maresins: novel macrophage mediators with potent antiinflammatory and proresolving actions. J Exp Med. (2009) 206:15–23. doi: 10.1084/jem.20081880
51. Serhan CN. Treating inflammation and infection in the 21st century: new hints from decoding resolution mediators and mechanisms. Faseb J. (2017) 31:1273–88. doi: 10.1096/fj.201601222R
52. Spite M, Clària J, Serhan CN. Resolvins, specialized proresolving lipid mediators, and their potential roles in metabolic diseases. Cell Metab. (2014) 19:21–36. doi: 10.1016/j.cmet.2013.10.006
53. Jetten N, Roumans N, Gijbels MJ, Romano A, Post MJ, de Winther MP, et al. Wound administration of M2-polarized macrophages does not improve murine cutaneous healing responses. PLoS One. (2014) 9:e102994. doi: 10.1371/journal.pone.0102994
54. Tang S, Wan M, Huang W, Stanton RC, Xu Y. Maresins: specialized proresolving lipid mediators and their potential role in inflammatory-related diseases. Mediat Inflamm. (2018) 2018:2380319. doi: 10.1155/2018/2380319
55. Hwang S-M, Chung G, Kim YH, Park C-K. The role of maresins in inflammatory pain: function of macrophages in wound regeneration. Int J Mol Sci. (2019) 20:5849. doi: 10.3390/ijms20235849
56. Rodriguez AR, Spur BW. First total synthesis of the macrophage derived anti-inflammatory and pro-resolving lipid mediator maresin 2. Tetrahedron Lett. (2015) 56:256–9. doi: 10.1016/j.tetlet.2014.11.082
57. Serhan CN, Dalli J, Colas RA, Winkler JW, Chiang N. Protectins and maresins: new pro-resolving families of mediators in acute inflammation and resolution bioactive metabolome. Biochim Biophys Acta. (2015) 1851:397–413. doi: 10.1016/j.bbalip.2014.08.006
58. Li Y, Dalli J, Chiang N, Baron RM, Quintana C, Serhan CN. Plasticity of leukocytic exudates in resolving acute inflammation is regulated by microrna and proresolving mediators. Immunity. (2013) 39:885–98. doi: 10.1016/j.immuni.2013.10.011
59. Serhan CN, Chiang N, Dalli J. The resolution code of acute inflammation: novel pro-resolving lipid mediators in resolution. Semin Immunol. (2015) 27:200–15. doi: 10.1016/j.smim.2015.03.004
60. Viola JR, Lemnitzer P, Jansen Y, Csaba G, Winter C, Neideck C, et al. Resolving lipid mediators maresin 1 and resolvin d2 prevent atheroprogression in mice. Circul Res. (2016) 119:1030–8. doi: 10.1161/CIRCRESAHA.116.309492
61. Gowda B, Sg Fuda H, Tsukui T, Chiba H, Hui S-P. Discovery of eicosapentaenoic acid esters of hydroxy fatty acids as potent Nrf2 activators. Antioxidants. (2020) 9:397. doi: 10.3390/antiox9050397
62. Kuda O, Brezinova M, Rombaldova M, Slavikova B, Posta M, Beier P, et al. Docosahexaenoic acid-derived fatty acid esters of hydroxy fatty acids. (Fahfas) with anti-inflammatory properties. Diabetes. (2016) 65:2580–90. doi: 10.2337/db16-0385
63. Wong SW, Kwon M-J, Choi AMK, Kim H-P, Nakahira K, Hwang DH. Fatty Acids Modulate Toll-Like Receptor 4 Activation through Regulation of Receptor Dimerization and Recruitment into Lipid Rafts in a Reactive Oxygen Species-Dependent Manner. The Journal of biological chemistry. (2009) 284(40):27384–92. doi: 10.1074/jbc.m109.044065
64. Lee JY, Sohn KH, Rhee SH, Hwang D. Saturated fatty acids, but not unsaturated fatty acids, induce the expression of cyclooxygenase-2 mediated through toll-like receptor 4. J Biol Chem. (2001) 276:16683–9. doi: 10.1074/jbc.M011695200
65. Lee JY, Zhao L, Youn HS, Weatherill AR, Tapping R, Feng L, et al. Saturated fatty acid activates but polyunsaturated fatty acid inhibits toll-like receptor 2 dimerized with toll-like receptor 6 or 1 *. J Biol Chem. (2004) 279:16971–9. doi: 10.1074/jbc.M312990200
66. Zhang Y, Chen H, Zhang W, Cai Y, Shan P, Wu D, et al. Arachidonic acid inhibits inflammatory responses by binding to myeloid differentiation factor-2. (Md2) and preventing Md2/toll-like receptor 4 signaling activation. Biochim Biophys Acta. (2020) 1866:165683. doi: 10.1016/j.bbadis.2020.165683
67. Ralston JC, Lyons CL, Kennedy EB, Kirwan AM, Roche HM. Fatty acids and Nlrp3 inflammasome-mediated inflammation in metabolic tissues. Annu Rev Nutr. (2017) 37:77–102. doi: 10.1146/annurev-nutr-071816-064836
68. Shen L, Yang Y, Ou T, Key C-CC, Tong SH, Sequeira RC, et al. Dietary pufas attenuate Nlrp3 inflammasome activation via enhancing macrophage autophagy. J Lipid Res. (2017) 58:1808–21. doi: 10.1194/jlr.M075879
69. Karasawa T, Takahashi M. Role of Nlrp3 inflammasomes in atherosclerosis. J Atheroscl Thromb. (2017) 24:443–51. doi: 10.5551/jat.RV17001
70. Ridker PM, Everett BM, Thuren T, MacFadyen JG, Chang WH, Ballantyne C, et al. Antiinflammatory therapy with canakinumab for atherosclerotic disease. N Engl J Med. (2017) 377:1119–31. doi: 10.1056/NEJMoa1707914
71. Sherratt SCR, Dawoud H, Libby P, Bhatt DL, Malinski T, Mason P. Eicosapentaenoic acid inhibits lipopolysaccharide. (lps)-induced macrophage activation in a manner that is enhanced with colchicine. Atherosclerosis. (2021) 331:e71. doi: 10.1016/j.atherosclerosis.2021.06.205
72. Khair-El-Din T, Sicher SC, Vazquez MA, Chung GW, Stallworth KA, Kitamura K, et al. Transcription of the murine inos gene is inhibited by docosahexaenoic acid, a major constituent of fetal and neonatal sera as well as fish oils. J Exp Med. (1996) 183:1241–6. doi: 10.1084/jem.183.3.1241
73. Al-Brakati AY, Kamishima T, Dart C, Quayle JM. Caveolar disruption causes contraction of rat femoral arteries via reduced basal no release and subsequent closure of Bkca channels. PeerJ. (2015) 3:e966. doi: 10.7717/peerj.966
74. Sherratt SCR, Dawoud H, Bhatt DL, Malinski T, Mason RP. Omega-3 and omega-6 fatty acids have distinct effects on endothelial fatty acid content and nitric oxide bioavailability. Prostaglandins Leukotrienes Essential Fatty Acids. (2021) 173:102337. doi: 10.1016/j.plefa.2021.102337
75. Zgheel F, Alhosin M, Rashid S, Auger C, Schini-Kerth VB. The Epa:Dha 6:1-evoked endothelium-dependent no-mediated relaxation in the coronary artery involves a copper-dependent pro-oxidant response triggering the pi3-kinase/Akt-mediated activation of Enos. Eur Heart J. (2013) 34(Suppl_1):578. doi: 10.1093/eurheartj/eht307.P578
76. Pearce MJ, McIntyre TM, Prescott SM, Zimmerman GA, Whatley RE. Shear stress activates cytosolic phospholipase A2. (Cpla2) and map kinase in human endothelial cells. Biochem Biophys Res Commun. (1996) 218:500–4. doi: 10.1006/bbrc.1996.0089
77. Kim SF. The role of nitric oxide in prostaglandin biology; update. Nitric Oxide. (2011) 25:255–64. doi: 10.1016/j.niox.2011.07.002
78. Tunctan B, Korkmaz B, Nihal Sari A, Kacan M, Unsal D, Sami Serin M, et al. A novel treatment strategy for sepsis and septic shock based on the interactions between prostanoids, nitric oxide, and 20-hydroxyeicosatetraenoic acid. Anti Inflamm Anti Aller Agents Med Chem. (2012) 11:121–50. doi: 10.2174/187152312803305759
79. Roman RJ. P-450 metabolites of arachidonic acid in the control of cardiovascular function. Physiol Rev. (2002) 82:131–85. doi: 10.1152/physrev.00021.2001
80. Imig JD. Epoxyeicosatrienoic Acids, 20-Hydroxyeicosatetraenoic Acid, and Renal Microvascular Function. Prostaglandins Other Lipid Mediat. (2013) 10(4–105):2–7. doi: 10.1016/j.prostaglandins.2013.01.002
81. Garcia V, Gilani A, Shkolnik B, Pandey V, Zhang FF, Dakarapu R, et al. 20-hete signals through G-protein-coupled receptor Gpr75. (G(Q)) to affect vascular function and trigger hypertension. Circ Res. (2017) 120:1776–88. doi: 10.1161/circresaha.116.310525
82. Agostinucci K, Hutcheson R, Hossain S, Pascale JV, Villegas E, Zhang F, et al. Blockade of 20-hydroxyeicosatetraenoic acid receptor lowers blood pressure and alters vascular function in mice with smooth muscle-specific overexpression of Cyp4a12-20-hete synthase. J Hypertens. (2022) 40:498–511. doi: 10.1097/HJH.0000000000003038
83. Cheng J, Wu CC, Gotlinger KH, Zhang F, Falck JR, Narsimhaswamy D, et al. 20-Hydroxy-5,8,11,14-eicosatetraenoic acid mediates endothelial dysfunction via ikappab kinase-dependent endothelial nitric-oxide synthase uncoupling. J Pharmacol Exp Ther. (2010) 332:57–65. doi: 10.1124/jpet.109.159863
84. Ishizuka T, Cheng J, Singh H, Vitto MD, Manthati VL, Falck JR, et al. 20-hydroxyeicosatetraenoic acid stimulates nuclear factor-kappab activation and the production of inflammatory cytokines in human endothelial cells. J Pharmacol Exp Ther. (2008) 324:103–10. doi: 10.1124/jpet.107.130336
85. Miyata N, Roman RJ. Role of 20-hydroxyeicosatetraenoic acid. (20-Hete) in vascular system. J Smooth Muscle Res. (2005) 41:175–93. doi: 10.1540/jsmr.41.175
86. Sun CW, Alonso-Galicia M, Taheri MR, Falck JR, Harder DR, Roman RJ. Nitric oxide-20-hydroxyeicosatetraenoic acid interaction in the regulation of k+ channel activity and vascular tone in renal arterioles. Circ Res. (1998) 83:1069–79. doi: 10.1161/01.res.83.11.1069
87. Oyekan AO, Youseff T, Fulton D, Quilley J, McGiff JC. Renal cytochrome p450 omega-hydroxylase and epoxygenase activity are differentially modified by nitric oxide and sodium chloride. J Clin Invest. (1999) 104:1131–7. doi: 10.1172/jci6786
88. Minamiyama Y, Takemura S, Imaoka S, Funae Y, Tanimoto Y, Inoue M. Irreversible inhibition of cytochrome p450 by nitric oxide. J Pharmacol Exp Ther. (1997) 283:1479–85.
89. Joseph G, Soler A, Hutcheson R, Hunter I, Bradford C, Hutcheson B, et al. Elevated 20-hete impairs coronary collateral growth in metabolic syndrome via endothelial dysfunction. Am J Physiol Heart Circ Physiol. (2017) 312:H528–40. doi: 10.1152/ajpheart.00561.2016
90. Ward NC, Rivera J, Hodgson J, Puddey IB, Beilin LJ, Falck JR, et al. Urinary 20-hydroxyeicosatetraenoic acid is associated with endothelial dysfunction in humans. Circulation. (2004) 110:438–43. doi: 10.1161/01.Cir.0000136808.72912.D9
91. Harder DR, Gebremedhin D, Narayanan J, Jefcoat C, Falck JR, Campbell WB, et al. Formation and action of a p-450 4a metabolite of arachidonic acid in cat cerebral microvessels. Am J Physiol. (1994) 266(5 Pt 2):H2098–107. doi: 10.1152/ajpheart.1994.266.5.H2098
92. Harder DR, Roman RJ, Gebremedhin D, Birks EK, Lange ARA. Common pathway for regulation of nutritive blood flow to the brain: arterial muscle membrane potential and cytochrome p450 metabolites. Acta Physiol Scand. (1998) 164:527–32. doi: 10.1111/j.1365-201x.1998.tb10702.x
93. Campbell WB, Gebremedhin D, Pratt PF, Harder DR. Identification of Epoxyeicosatrienoic Acids as Endothelium-Derived Hyperpolarizing Factors. Circ Res. (1996) 78:415–23. doi: 10.1161/01.res.78.3.415
94. Rosolowsky M, Campbell WB. Role of Pgi2 and Epoxyeicosatrienoic Acids in Relaxation of Bovine Coronary Arteries to Arachidonic Acid. Am J Physiol. (1993) 264(2 Pt 2):H327–35. doi: 10.1152/ajpheart.1993.264.2.H327
95. Rosolowsky M, Falck JR, Willerson JT, Campbell WB. Synthesis of lipoxygenase and epoxygenase products of arachidonic acid by normal and stenosed canine coronary arteries. Circ Res. (1990) 66:608–21. doi: 10.1161/01.res.66.3.608
96. Frömel T, Fleming I. Whatever happened to the epoxyeicosatrienoic acid-like endothelium-derived hyperpolarizing factor? The identification of novel classes of lipid mediators and their role in vascular homeostasis. Antioxid Redox Signal. (2015) 22:1273–92. doi: 10.1089/ars.2014.6150
97. Lawson DL, Mehta JL, Saldeen K, Mehta P, Saldeen TG. Ω-3 polyunsaturated fatty acids augment endothelium-dependent vasorelaxation by enhanced release of Edrf and vasodilator prostaglandins. Eicosanoids. (1991) 4:217–23.
98. Huang C-C, Chang M-T, Leu H-B, Yin W-H, Tseng W-K, Wu Y-W, et al. Association of arachidonic acid-derived lipid mediators with subsequent onset of acute myocardial infarction in patients with coronary artery disease. Sci Rep. (2020) 10:8105. doi: 10.1038/s41598-020-65014-z
99. Engler MB, Engler MM, Browne A, Sun YP, Sievers R. Mechanisms of vasorelaxation induced by eicosapentaenoic acid. (20:5n-3) in Wky rat aorta. Br J Pharmacol. (2000) 131:1793–9. doi: 10.1038/sj.bjp.0703754
100. Engler MB, Engler MM. Docosahexaenoic acid – induced vasorelaxation in hypertensive rats: mechanisms of action. Biol Res Nurs. (2000) 2:85–95. doi: 10.1177/109980040000200202
101. Engler MB, Ma YH, Engler MM. Calcium-mediated mechanisms of eicosapentaenoic acid-induced relaxation in hypertensive rat aorta. Am J Hypertens. (1999) 12(12 Pt 1–2):1225–35. doi: 10.1016/s0895-7061(99)90060-2
102. Bercea C-I, Cottrell GS, Tamagnini F, McNeish AJ. Omega-3 polyunsaturated fatty acids and hypertension: a review of vasodilatory mechanisms of docosahexaenoic acid and eicosapentaenoic acid. Br J Pharmacol. (2021) 178:860–77. doi: 10.1111/bph.15336
103. Kotlyarov S. Diversity of lipid function in atherogenesis: a focus on endothelial mechanobiology. Int J Mol Sci. (2021) 22:11545. doi: 10.3390/ijms222111545
104. Marguet D, Lenne P-F, Rigneault H, He H-T. Dynamics in the plasma membrane: how to combine fluidity and order. EMBO J. (2006) 25:3446–57. doi: 10.1038/sj.emboj.7601204
105. Zabroski IO, Nugent MA. Lipid raft association stabilizes vegf receptor 2 in endothelial cells. Int J Mol Sci. (2021) 22:798. doi: 10.3390/ijms22020798
106. Grouleff J, Irudayam SJ, Skeby KK, Schiøtt B. The influence of cholesterol on membrane protein structure, function, and dynamics studied by molecular dynamics simulations. Biochim Biophys Acta. (2015) 1848:1783–95. doi: 10.1016/j.bbamem.2015.03.029
107. Axelrod D. Lateral motion of membrane proteins and biological function. J Membr Biol. (1983) 75:1–10. doi: 10.1007/BF01870794
108. Phillips R, Ursell T, Wiggins P, Sens P. Emerging roles for lipids in shaping membrane-protein function. Nature. (2009) 459:379–85. doi: 10.1038/nature08147
109. Shaikh SR, Kinnun JJ, Leng X, Williams JA, Wassall SR. How polyunsaturated fatty acids modify molecular organization in membranes: insight from Nmr studies of model systems. Biochim Biophys Acta. (2015) 1848(1, Pt B):211–9. doi: 10.1016/j.bbamem.2014.04.020
110. Wassall SR, Stillwell W. Polyunsaturated fatty acid–cholesterol interactions: domain formation in membranes. Biochim Biophys Acta. (2009) 1788:24–32. doi: 10.1016/j.bbamem.2008.10.011
111. Williams Justin A, Batten Shawn E, Harris M, Rockett Benjamin D, Shaikh Saame R, Stillwell W, et al. Docosahexaenoic and eicosapentaenoic acids segregate differently between raft and nonraft domains. Biophys J. (2012) 103:228–37. doi: 10.1016/j.bpj.2012.06.016
112. Li Q, Zhang Q, Wang M, Zhao S, Ma J, Luo N, et al. Eicosapentaenoic acid modifies lipid composition in caveolae and induces translocation of endothelial nitric oxide synthase. Biochimie. (2007) 89:169–77. doi: 10.1016/j.biochi.2006.10.009
113. Li Q, Zhang Q, Wang M, Liu F, Zhao S, Ma J, et al. Docosahexaenoic acid affects endothelial nitric oxide synthase in caveolae. Arch Biochem Biophys. (2007) 466:250–9. doi: 10.1016/j.abb.2007.06.023
114. Stebbins CL, Stice JP, Hart CM, Mbai FN, Knowlton AA. Effects of dietary decosahexaenoic acid. (Dha) on enos in human coronary artery endothelial cells. J Cardiovasc Pharmacol Ther. (2008) 13:261–8. doi: 10.1177/1074248408322470
115. Pal A, Metherel AH, Fiabane L, Buddenbaum N, Bazinet RP, Shaikh SR. Do eicosapentaenoic acid and docosahexaenoic acid have the potential to compete against each other? Nutrients. (2020) 12:3718. doi: 10.3390/nu12123718
116. Mason RP, Jacob RF, Shrivastava S, Sherratt SCR, Chattopadhyay A. Eicosapentaenoic acid reduces membrane fluidity, inhibits cholesterol domain formation, and normalizes bilayer width in atherosclerotic-like model membranes. Biochim Biophys Acta. (2016) 1858:3131–40. doi: 10.1016/j.bbamem.2016.10.002
117. Hashimoto M, Hossain S, Yamasaki H, Yazawa K, Masumura S. Effects of eicosapentaenoic acid and docosahexaenoic acid on plasma membrane fluidity of aortic endothelial cells. Lipids. (1999) 34:1297–304. doi: 10.1007/s11745-999-0481-6
118. Li X, Zhou S, Lin X. Molecular view on the impact of dha molecules on the physical properties of a model cell membrane. J Chem Informat Model. (2022) 62:2421–31. doi: 10.1021/acs.jcim.2c00074
119. Huber T, Rajamoorthi K, Kurze VF, Beyer K, Brown MF. Structure of docosahexaenoic acid-containing phospholipid bilayers as studied by 2h Nmr and molecular dynamics simulations. J Am Chem Soc. (2002) 124:298–309. doi: 10.1021/ja011383j
120. Den Ruijter HM, Berecki G, Opthof T, Verkerk AO, Zock PL, Coronel R. Pro- and antiarrhythmic properties of a diet rich in fish oil. Cardiovasc Res. (2007) 73:316–25. doi: 10.1016/j.cardiores.2006.06.014
121. Xiao YF, Kang JX, Morgan JP, Leaf A. Blocking effects of polyunsaturated fatty acids on Na+ channels of neonatal rat ventricular myocytes. Proc Natl Acad Sci USA. (1995) 92:11000–4. doi: 10.1073/pnas.92.24.11000
122. Xiao YF, Wright SN, Wang GK, Morgan JP, Leaf A. Fatty acids suppress voltage-gated na+ currents in hek293t cells transfected with the alpha-subunit of the human cardiac Na+ channel. Proc Natl Acad Sci USA. (1998) 95:2680–5. doi: 10.1073/pnas.95.5.2680
123. Leifert WR, McMurchie EJ, Saint DA. Inhibition of cardiac sodium currents in adult rat myocytes by N-3 polyunsaturated fatty acids. J Physiol. (1999) 520(Pt 3):671–9. doi: 10.1111/j.1469-7793.1999.00671.x
124. Kang JX, Xiao YF, Leaf A. Free, long-chain, polyunsaturated fatty acids reduce membrane electrical excitability in neonatal rat cardiac myocytes. Proc Natl Acad Sci USA. (1995) 92:3997–4001. doi: 10.1073/pnas.92.9.3997
125. Dhein S, Michaelis B, Mohr F-W. Antiarrhythmic and electrophysiological effects of long-chain Ω-3 polyunsaturated fatty acids. Naunyn-Schmiedeberg’s Arch Pharmacol. (2005) 371:202–11.
126. Verkerk AO, van Ginneken AC, Berecki G, den Ruijter HM, Schumacher CA, Veldkamp MW, et al. Incorporated sarcolemmal fish oil fatty acids shorten pig ventricular action potentials. Cardiovasc Res. (2006) 70:509–20. doi: 10.1016/j.cardiores.2006.02.022
127. Leifert WR, Jahangiri A, Saint DA, McMurchie EJ. Effects of dietary N-3 fatty acids on contractility, na+ and k+ currents in a rat cardiomyocyte model of arrhythmia. J Nutr Biochem. (2000) 11:382–92. doi: 10.1016/s0955-2863(00)00094-2
128. Xiao YF, Gomez AM, Morgan JP, Lederer WJ, Leaf A. Suppression of voltage-gated L-Type Ca2+ currents by polyunsaturated fatty acids in adult and neonatal rat ventricular myocytes. Proc Natl Acad Sci USA. (1997) 94:4182–7. doi: 10.1073/pnas.94.8.4182
129. Ferrier GR, Redondo I, Zhu J, Murphy MG. Differential effects of docosahexaenoic acid on contractions and l-type ca2+ current in adult cardiac myocytes. Cardiovasc Res. (2002) 54:601–10. doi: 10.1016/s0008-6363(02)00275-4
130. Baartscheer A, Schumacher CA, Belterman CN, Coronel R, Fiolet JW Sr. Calcium handling and calcium after-transients in a rabbit model of heart failure. Cardiovasc Res. (2003) 58:99–108. doi: 10.1016/s0008-6363(02)00854-4
131. Verkerk AO, Veldkamp MW, Baartscheer A, Schumacher CA, Klöpping C, van Ginneken AC, et al. Ionic mechanism of delayed afterdepolarizations in ventricular cells isolated from human end-stage failing hearts. Circulation. (2001) 104:2728–33. doi: 10.1161/hc4701.099577
132. Xiao YF, Morgan JP, Leaf A. Effects of polyunsaturated fatty acids on cardiac voltage-activated K(+) currents in adult ferret cardiomyocytes. Sheng Li Xue Bao. (2002) 54:271–81.
133. de Oliveira Otto MC, Wu JHY, Baylin A, Vaidya D, Rich SS, Tsai MY, et al. Circulating and dietary omega-3 and omega-6 polyunsaturated fatty acids and incidence of cvd in the multi-ethnic study of atherosclerosis. J Am Heart Assoc. (2013) 2:e000506. doi: 10.1161/JAHA.113.000506
134. Uehara Y, Engel T, Li Z, Goepfert C, Rust S, Zhou X, et al. Polyunsaturated fatty acids and acetoacetate downregulate the expression of the atp-binding cassette transporter A1. Diabetes. (2002) 51:2922–8. doi: 10.2337/diabetes.51.10.2922
135. Wang Y, Oram JF. Unsaturated fatty acids inhibit cholesterol efflux from macrophages by increasing degradation of atp-binding cassette transporter A1 *. J Biol Chem. (2002) 277:5692–7. doi: 10.1074/jbc.M109977200
136. Uehara Y, Miura S, von Eckardstein A, Abe S, Fujii A, Matsuo Y, et al. Unsaturated fatty acids suppress the expression of the Atp-binding cassette transporter G1. (Abcg1) and Abca1 Genes Via an Lxr/Rxr responsive element. Atherosclerosis. (2007) 191:11–21. doi: 10.1016/j.atherosclerosis.2006.04.018
137. Fournier N, Tardivel S, Benoist JF, Vedie B, Rousseau-Ralliard D, Nowak M, et al. Eicosapentaenoic acid membrane incorporation impairs Abca1-dependent cholesterol efflux via a protein kinase a signaling pathway in primary human macrophages. Biochim Biophys Acta. (2016) 1861:331–41. doi: 10.1016/j.bbalip.2016.01.002
138. Fournier N, Sayet G, Vedie B, Nowak M, Allaoui F, Solgadi A, et al. Eicosapentaenoic acid membrane incorporation impairs cholesterol efflux from cholesterol-loaded human macrophages by reducing the cholesteryl ester mobilization from lipid droplets. Biochim Biophys Acta Mol Cell Biol Lipids. (2017) 1862(10 Pt A):1079–91. doi: 10.1016/j.bbalip.2017.07.011
139. Dakroub H, Nowak M, Benoist JF, Noël B, Vedie B, Paul JL, et al. Eicosapentaenoic acid membrane incorporation stimulates Abca1-mediated cholesterol efflux from human Thp-1 macrophages. Biochim Biophys Acta Mol Cell Biol Lipids. (2021) 1866:159016. doi: 10.1016/j.bbalip.2021.159016
140. Fujii R, Yamada H, Munetsuna E, Yamazaki M, Mizuno G, Ando Y, et al. Dietary fish and Ω-3 polyunsaturated fatty acids are associated with leukocyte Abca1 DNA methylation levels. Nutrition. (2021) 81:110951. doi: 10.1016/j.nut.2020.110951
141. Pakiet A, Jakubiak A, Mierzejewska P, Zwara A, Liakh I, Sledzinski T, et al. The effect of a high-fat diet on the fatty acid composition in the hearts of mice. Nutrients. (2020) 12:824. doi: 10.3390/nu12030824
142. Shramko VS, Polonskaya YV, Kashtanova EV, Stakhneva EM, Ragino YI. The short overview on the relevance of fatty acids for human cardiovascular disorders. Biomolecules. (2020) 10:1127. doi: 10.3390/biom10081127
143. Burdge GC, Jones AE, Wootton SA. Eicosapentaenoic and docosapentaenoic acids are the principal products of α-linolenic acid metabolism in young men. Br J Nutr. (2002) 88:355–63. doi: 10.1079/BJN2002662
144. Salvador-Montanes O, Gomez-Gallanti A, Garofalo D, Noujaim SF, Peinado R, Filgueiras-Rama D. Polyunsaturated fatty acids in atrial fibrillation: looking for the proper candidates. Front Physiol. (2012) 3:370. doi: 10.3389/fphys.2012.00370
145. Ramsden CE, Ringel A, Feldstein AE, Taha AY, MacIntosh BA, Hibbeln JR, et al. Lowering dietary linoleic acid reduces bioactive oxidized linoleic acid metabolites in humans. Prostaglandins Leukot Essent Fatty Acids. (2012) 87:135–41. doi: 10.1016/j.plefa.2012.08.004
146. Jira W, Spiteller G, Carson W, Schramm A. Strong increase in hydroxy fatty acids derived from linoleic acid in human low density lipoproteins of atherosclerotic patients. Chem Phys Lipids. (1998) 91:1–11. doi: 10.1016/s0009-3084(97)00095-9
147. DiNicolantonio JJ, O’Keefe JH. Omega-6 vegetable oils as a driver of coronary heart disease: the oxidized linoleic acid hypothesis. Open Heart. (2018) 5:e000898. doi: 10.1136/openhrt-2018-000898
148. Jira W, Spiteller G, Richter A. Increased levels of lipid oxidation products in low density lipoproteins of patients suffering from rheumatoid arthritis. Chem Phys Lipids. (1997) 87:81–9. doi: 10.1016/s0009-3084(97)00030-3
149. Hennig B, Shasby DM, Spector AA. Exposure to fatty acid increases human low density lipoprotein transfer across cultured endothelial monolayers. Circ Res. (1985) 57:776–80. doi: 10.1161/01.res.57.5.776
150. Carpenter KL, Taylor SE, Ballantine JA, Fussell B, Halliwell B, Mitchinson MJ. Lipids and oxidised lipids in human atheroma and normal aorta. Biochim Biophys Acta. (1993) 1167:121–30. doi: 10.1016/0005-2760(93)90151-x
151. Dayton S, Hashimoto SAM, Pearce ML. Influence of a diet high in unsaturated fat upon composition of arterial tissue and atheromata in man. Circulation. (1965) 32:911–24. doi: 10.1161/01.CIR.32.6.911
152. Kämmerer I, Ringseis R, Biemann R, Wen G, Eder K. 13-hydroxy linoleic acid increases expression of the cholesterol transporters Abca1, Abcg1 and Sr-Bi and stimulates Apoa-I-dependent cholesterol efflux in raw264.7 macrophages. Lipids Health Dis. (2011) 10:222. doi: 10.1186/1476-511x-10-222
153. Shah AP, Ichiuji AM, Han JK, Traina M, El-Bialy A, Meymandi SK, et al. Cardiovascular and endothelial effects of fish oil supplementation in healthy volunteers. J Cardiovasc Pharmacol Ther. (2007) 12:213–9. doi: 10.1177/1074248407304749
154. Rizza S, Tesauro M, Cardillo C, Galli A, Iantorno M, Gigli F, et al. Fish oil supplementation improves endothelial function in normoglycemic offspring of patients with type 2 diabetes. Atherosclerosis. (2009) 206:569–74. doi: 10.1016/j.atherosclerosis.2009.03.006
155. Goodfellow J, Bellamy MF, Ramsey MW, Jones CJ, Lewis MJ. Dietary supplementation with marine omega-3 fatty acids improve systemic large artery endothelial function in subjects with hypercholesterolemia. J Am Coll Cardiol. (2000) 35:265–70. doi: 10.1016/s0735-1097(99)00548-3
156. Wu SY, Mayneris-Perxachs J, Lovegrove JA, Todd S, Yaqoob P. Fish-oil supplementation alters numbers of circulating endothelial progenitor cells and microparticles independently of Enos genotype. Am J Clin Nutr. (2014) 100:1232–43. doi: 10.3945/ajcn.114.088880
157. Dawczynski C, Massey KA, Ness C, Kiehntopf M, Stepanow S, Platzer M, et al. Randomized placebo-controlled intervention with n-3 lc-pufa-supplemented yoghurt: effects on circulating eicosanoids and cardiovascular risk factors. Clin Nutr. (2013) 32:686–96. doi: 10.1016/j.clnu.2012.12.010
158. Mohan D, Mente A, Dehghan M, Rangarajan S, O’Donnell M, Hu W, et al. Associations of fish consumption with risk of cardiovascular disease and mortality among individuals with or without vascular disease from 58 countries. JAMA Intern Med. (2021) 181:631–49. doi: 10.1001/jamainternmed.2021.0036
159. Jayedi A, Shab-Bidar S. Fish consumption and the risk of chronic disease: an umbrella review of meta-analyses of prospective cohort studies. Adv Nutr. (2020) 11:1123–33. doi: 10.1093/advances/nmaa029
160. Abdelhamid AS, Martin N, Bridges C, Brainard JS, Wang X, Brown TJ, et al. Polyunsaturated fatty acids for the primary and secondary prevention of cardiovascular disease. Cochrane Database Syst Rev. (2018) 11:Cd012345. doi: 10.1002/14651858.CD012345.pub3
161. Ueshima H, Stamler J, Elliott P, Chan Q, Brown IJ, Carnethon MR, et al. Food omega-3 fatty acid intake of individuals. (total, linolenic acid, long-chain) and their blood pressure: intermap study. Hypertension. (2007) 50:313–9. doi: 10.1161/hypertensionaha.107.090720
162. Miller PE, Van Elswyk M, Alexander DD. Long-chain omega-3 fatty acids eicosapentaenoic acid and docosahexaenoic acid and blood pressure: a meta-analysis of randomized controlled trials. Am J Hypertens. (2014) 27:885–96. doi: 10.1093/ajh/hpu024
163. Tagetti A, Ericson U, Montagnana M, Danese E, Almgren P, Nilsson P, et al. Intakes of omega-3 polyunsaturated fatty acids and blood pressure change over time: possible interaction with genes involved in 20-hete and eets metabolism. Prostaglandins Other Lipid Mediat. (2015) 120:126–33. doi: 10.1016/j.prostaglandins.2015.05.003
164. Ma T, He L, Luo Y, Li J, Zhang G, Cheng X, et al. Associations of baseline use of fish oil with progression of cardiometabolic multimorbidity and mortality among patients with hypertension: a prospective study of Uk biobank. Eur J Nutr. (2022) 61:3461–70. doi: 10.1007/s00394-022-02889-w
165. McLennan PL, Bridle TM, Abeywardena MY, Charnock JS. Dietary lipid modulation of ventricular fibrillation threshold in the marmoset monkey. Am Heart J. (1992) 123:1555–61. doi: 10.1016/0002-8703(92)90809-a
166. Hu Y, Hu FB, Manson JE. Marine omega-3 supplementation and cardiovascular disease: an updated meta-analysis of 13 randomized controlled trials involving 127 477 participants. J Am Heart Assoc. (2019) 8:e013543. doi: 10.1161/JAHA.119.013543
167. Khan SU, Lone AN, Khan MS, Virani SS, Blumenthal RS, Nasir K, et al. Effect of omega-3 fatty acids on cardiovascular outcomes: a systematic review and meta-analysis. EclinicalMedicine. (2021) 38:100997. doi: 10.1016/j.eclinm.2021.100997
168. Roncaglioni MC, Tombesi M, Avanzini F, Barlera S, Caimi V, Longoni P, et al. N-3 fatty acids in patients with multiple cardiovascular risk factors. N Engl J Med. (2013) 368:1800–8. doi: 10.1056/NEJMoa1205409
169. Siniarski A, Haberka M, Mostowik M, Gołȩbiowska-Wiatrak R, Porȩba M, Malinowski KP, et al. Treatment with omega-3 polyunsaturated fatty acids does not improve endothelial function in patients with type 2 diabetes and very high cardiovascular risk: a randomized, double-blind, placebo-controlled study. (Omega-Fmd). Atherosclerosis. (2018) 271:148–55. doi: 10.1016/j.atherosclerosis.2018.02.030
170. Sanders TA, Hall WL, Maniou Z, Lewis F, Seed PT, Chowienczyk PJ. Effect of low doses of long-chain n-3 pufas on endothelial function and arterial stiffness: a randomized controlled trial. Am J Clin Nutr. (2011) 94:973–80. doi: 10.3945/ajcn.111.018036
171. Mariamenatu AH, Abdu EM. Overconsumption of Omega-6 Polyunsaturated Fatty Acids. (Pufas) versus deficiency of omega-3 pufas in modern-day diets: the disturbing factor for their “balanced antagonistic metabolic functions” in the human body. J Lipids. (2021) 2021:8848161. doi: 10.1155/2021/8848161
172. Willett WC. The role of dietary n-6 fatty acids in the prevention of cardiovascular disease. J Cardiovasc Med. (2007) 8(Suppl 1):S42–5. doi: 10.2459/01.Jcm.0000289275.72556.13
173. Saini RK, Keum YS. Omega-3 and omega-6 polyunsaturated fatty acids: dietary sources, metabolism, and significance – a review. Life Sci. (2018) 203:255–67. doi: 10.1016/j.lfs.2018.04.049
174. Rassias G, Kestin M, Nestel PJ. Linoleic acid lowers ldl cholesterol without a proportionate displacement of saturated fatty acid. Eur J Clin Nutr. (1991) 45:315–20.
175. Djoussé L, Folsom AR, Province MA, Hunt SC, Ellison RC. Dietary linolenic acid and carotid atherosclerosis: the national heart, lung, and blood institute family heart study. Am J Clin Nutr. (2003) 77:819–25. doi: 10.1093/ajcn/77.4.819
176. Marklund M, Wu JHY, Imamura F, Del Gobbo LC, Fretts A, de Goede J, et al. Biomarkers of dietary omega-6 fatty acids and incident cardiovascular disease and mortality. Circulation. (2019) 139:2422–36. doi: 10.1161/circulationaha.118.038908
177. Farvid MS, Ding M, Pan A, Sun Q, Chiuve SE, Steffen LM, et al. Dietary linoleic acid and risk of coronary heart disease: a systematic review and meta-analysis of prospective cohort studies. Circulation. (2014) 130:1568–78. doi: 10.1161/CIRCULATIONAHA.114.010236
178. Ramsden CE, Zamora D, Leelarthaepin B, Majchrzak-Hong SF, Faurot KR, Suchindran CM, et al. Use of dietary linoleic acid for secondary prevention of coronary heart disease and death: evaluation of recovered data from the sydney diet heart study and updated meta-analysis. BMJ. (2013) 346:e8707. doi: 10.1136/bmj.e8707
179. de Goede J, Geleijnse JM, Boer JM, Kromhout D, Verschuren WM. Linoleic acid intake, plasma cholesterol and 10-year incidence of chd in 20,000 middle-aged men and women in the Netherlands. Br J Nutr. (2012) 107:1070–6. doi: 10.1017/s0007114511003837
180. Franzosi MG, Brunetti M, Marchioli R, Marfisi RM, Tognoni G, Valagussa F. Cost-effectiveness analysis of N-3 polyunsaturated fatty acids. (Pufa) after myocardial infarction: results from gruppo Italiano Per Lo Studio Della Sopravvivenza Nell’infarto. (Gissi)-Prevenzione Trial. Pharmacoeconomics. (2001) 19:411–20. doi: 10.2165/00019053-200119040-00008
181. Lamotte M, Annemans L, Kawalec P, Zoellner YA. Multi-country health economic evaluation of highly concentrated N-3 polyunsaturated fatty acids in secondary prevention after myocardial infarction. Pharmacoeconomics. (2006) 24:783–95. doi: 10.2165/00019053-200624080-00005
182. Kodera S, Morita H, Kiyosue A, Ando J, Komuro I. Cost-effectiveness of statin plus eicosapentaenoic acid combination therapy for cardiovascular disease prevention in japanese patients with hypercholesterolemia - an analysis based on the Japan eicosapentaenoic acid lipid intervention study. (Jelis). Circ J. (2018) 82:1076–82. doi: 10.1253/circj.CJ-17-0995
183. Cowie MR, Cure S, Bianic F, McGuire A, Goodall G, Tavazzi L. Cost-effectiveness of highly purified omega-3 polyunsaturated fatty acid ethyl esters in the treatment of chronic heart failure: results of markov modelling in a Uk setting. Eur J Heart Fail. (2011) 13:681–9. doi: 10.1093/eurjhf/hfr023
184. Gao L, Moodie M, Li S-C. The cost-effectiveness of omega-3 polyunsaturated fatty acids – the Australian healthcare perspective. Eur J Internal Med. (2019) 67:70–6. doi: 10.1016/j.ejim.2019.07.001
185. Quilici S, Martin M, McGuire A, Zoellner YA. Cost-effectiveness analysis of N-3 Pufa. (Omacor) treatment in post-mi patients. Int J Clin Pract. (2006) 60:922–32. doi: 10.1111/j.1742-1241.2006.01009.x
186. Wang C, Enssle J, Pietzner A, Schmöcker C, Weiland L, Ritter O, et al. Essential polyunsaturated fatty acids in blood from patients with and without catheter-proven coronary artery disease. Int J Mol Sci. (2022) 23:766. doi: 10.3390/ijms23020766
187. Mozaffarian D, Lemaitre RN, King IB, Song X, Huang H, Sacks FM, et al. Plasma phospholipid long-chain ω-3 fatty acids and total and cause-specific mortality in older adults. Ann Internal Med. (2013) 158:515–25. doi: 10.7326/0003-4819-158-7-201304020-00003
188. Gissi-Prevenzione Investigators. Dietary supplementation with N-3 polyunsaturated fatty acids and vitamin e after myocardial infarction: results of the gissi-prevenzione trial. Lancet. (1999) 354:447–55. doi: 10.1016/S0140-6736(99)07072-5
189. Burr ML, Ashfield-Watt PA, Dunstan FD, Fehily AM, Breay P, Ashton T, et al. Lack of benefit of dietary advice to men with angina: results of a controlled trial. Eur J Clin Nutr. (2003) 57:193–200. doi: 10.1038/sj.ejcn.1601539
190. Kromhout D, Giltay EJ, Geleijnse JM. N-3 fatty acids and cardiovascular events after myocardial infarction. N Engl J Med. (2010) 363:2015–26. doi: 10.1056/NEJMoa1003603
191. Yokoyama M, Origasa H, Matsuzaki M, Matsuzawa Y, Saito Y, Ishikawa Y, et al. Effects of eicosapentaenoic acid on major coronary events in hypercholesterolaemic patients. (jelis): a randomised open-label, blinded endpoint analysis. Lancet. (2007) 369:1090–8. doi: 10.1016/s0140-6736(07)60527-3
192. Heydari B, Abdullah S, Pottala JV, Shah R, Abbasi S, Mandry D, et al. Effect of omega-3 acid ethyl esters on left ventricular remodeling after acute myocardial infarction: the omega-remodel randomized clinical trial. Circulation. (2016) 134:378–91. doi: 10.1161/circulationaha.115.019949
193. Kwong RY, Heydari B, Ge Y, Abdullah S, Fujikura K, Kaneko K, et al. Genetic profiling of fatty acid desaturase polymorphisms identifies patients who may benefit from high-dose omega-3 fatty acids in cardiac remodeling after acute myocardial infarction-post-hoc analysis from the omega-remodel randomized controlled trial. PLoS One. (2019) 14:e0222061. doi: 10.1371/journal.pone.0222061
194. Abdelhamid AS, Brown TJ, Brainard JS, Biswas P, Thorpe GC, Moore HJ, et al. Omega-3 fatty acids for the primary and secondary prevention of cardiovascular disease. Cochrane Database Syst Rev. (2020) 3:Cd003177. doi: 10.1002/14651858.CD003177.pub5
195. Shen S, Gong C, Jin K, Zhou L, Xiao Y, Ma L. Omega-3 fatty acid supplementation and coronary heart disease risks: a meta-analysis of randomized controlled clinical trials. Front Nutr. (2022) 9:809311. doi: 10.3389/fnut.2022.809311
196. Ramirez JL, Zahner GJ, Spaulding KA, Khetani SA, Hills NK, Gasper WJ, et al. Peripheral artery disease is associated with a deficiency of erythrocyte membrane N-3 polyunsaturated fatty acids. Lipids. (2019) 54:211–9. doi: 10.1002/lipd.12140
197. Hammer A, Moertl D, Schlager O, Matschuck M, Seidinger D, Koppensteiner R, et al. Effects of N-3 pufa on endothelial function in patients with peripheral arterial disease: a randomised, placebo-controlled, double-blind trial. Br J Nutr. (2019) 122:698–706. doi: 10.1017/S0007114519001582
198. Schiano V, Laurenzano E, Brevetti G, De Maio JI, Lanero S, Scopacasa F, et al. Omega-3 polyunsaturated fatty acid in peripheral arterial disease: effect on lipid pattern, disease severity, inflammation profile, and endothelial function. Clin Nutr. (2008) 27:241–7. doi: 10.1016/j.clnu.2007.11.007
199. Grenon SM, Owens CD, Nosova EV, Hughes-Fulford M, Alley HF, Chong K, et al. Short-term, high-dose fish oil supplementation increases the production of omega-3 fatty acid-derived mediators in patients with peripheral artery disease. (the Omega-Pad I Trial). J Am Heart Assoc. (2015) 4:e002034. doi: 10.1161/JAHA.115.002034
200. Ramirez JL, Gasper WJ, Khetani SA, Zahner GJ, Hills NK, Mitchell PT, et al. Fish oil increases specialized pro-resolving lipid mediators in Pad. (the Omega-Pad Ii Trial). J Surg Res. (2019) 238:164–74. doi: 10.1016/j.jss.2019.01.038
201. Virtanen JK, Mursu J, Voutilainen S, Tuomainen TP. Serum long-chain N-3 polyunsaturated fatty acids and risk of hospital diagnosis of atrial fibrillation in men. Circulation. (2009) 120:2315–21. doi: 10.1161/circulationaha.109.852657
202. Wu JHY, Lemaitre RN, King IB, Song X, Sacks FM, Rimm EB, et al. Association of plasma phospholipid long-chain Ω-3 fatty acids with incident atrial fibrillation in older adults: the cardiovascular health study. Circulation. (2012) 125:1084–93. doi: 10.1161/CIRCULATIONAHA.111.062653
203. Kitamura K, Shibata R, Tsuji Y, Shimano M, Inden Y, Murohara T. Eicosapentaenoic acid prevents atrial fibrillation associated with heart failure in a rabbit model. Am J Physiol Heart Circ Physiol. (2011) 300:H1814–21. doi: 10.1152/ajpheart.00771.2010
204. Mayyas F, Sakurai S, Ram R, Rennison JH, Hwang E-S, Castel L, et al. Dietary Ω3 fatty acids modulate the substrate for post-operative atrial fibrillation in a canine cardiac surgery model. Cardiovasc Res. (2011) 89:852–61. doi: 10.1093/cvr/cvq380
205. Nicholls SJ, Lincoff AM, Garcia M, Bash D, Ballantyne CM, Barter PJ, et al. Effect of high-dose omega-3 fatty acids Vs corn oil on major adverse cardiovascular events in patients at high cardiovascular risk: the strength randomized clinical trial. JAMA. (2020) 324:2268–80. doi: 10.1001/jama.2020.22258
206. Albert CM, Cook NR, Pester J, Moorthy MV, Ridge C, Danik JS, et al. Effect of marine omega-3 fatty acid and vitamin d supplementation on incident atrial fibrillation: a randomized clinical trial. JAMA. (2021) 325:1061–73. doi: 10.1001/jama.2021.1489
207. Bhatt DL, Steg PG, Miller M, Brinton EA, Jacobson TA, Ketchum SB, et al. Cardiovascular risk reduction with icosapent ethyl for hypertriglyceridemia. N Engl J Med. (2019) 380:11–22. doi: 10.1056/NEJMoa1812792
208. Kalstad AA, Myhre PL, Laake K, Tveit SH, Schmidt EB, Smith P, et al. Effects of N-3 fatty acid supplements in elderly patients after myocardial infarction. Circulation. (2021) 143:528–39. doi: 10.1161/CIRCULATIONAHA.120.052209
209. Myhre PL, Kalstad AA, Tveit SH, Laake K, Schmidt EB, Smith P, et al. Changes in eicosapentaenoic acid and docosahexaenoic acid and risk of cardiovascular events and atrial fibrillation: a secondary analysis of the omemi trial. J Intern Med. (2022) 291:637–47. doi: 10.1111/joim.13442
210. Mortensen LM, Lundbye-Christensen S, Schmidt EB, Calder PC, Schierup MH, Tjønneland A, et al. Long-chain N-3 and N-6 polyunsaturated fatty acids and risk of atrial fibrillation: results from a danish cohort study. PLoS One. (2017) 12:e0190262. doi: 10.1371/journal.pone.0190262
211. Bianconi L, Calò L, Mennuni M, Santini L, Morosetti P, Azzolini P, et al. N-3 polyunsaturated fatty acids for the prevention of arrhythmia recurrence after electrical cardioversion of chronic persistent atrial fibrillation: a randomized, double-blind, multicentre study. Europace. (2011) 13:174–81. doi: 10.1093/europace/euq386
212. Mozaffarian D, Marchioli R, Macchia A, Silletta MG, Ferrazzi P, Gardner TJ, et al. Fish oil and postoperative atrial fibrillation: the omega-3 fatty acids for prevention of post-operative atrial fibrillation. (opera) randomized trial. JAMA. (2012) 308:2001–11. doi: 10.1001/jama.2012.28733
213. Zhang B, Zhen Y, Tao A, Bao Z, Zhang G. Polyunsaturated fatty acids for the prevention of atrial fibrillation after cardiac surgery: an updated meta-analysis of randomized controlled trials. J Cardiol. (2014) 63:53–9. doi: 10.1016/j.jjcc.2013.06.014
214. Gencer B, Djousse L, Al-Ramady OT, Cook NR, Manson JE, Albert CM. Effect of long-term marine ω-3 fatty acids supplementation on the risk of atrial fibrillation in randomized controlled trials of cardiovascular outcomes: a systematic review and meta-analysis. Circulation. (2021) 144:1981–90. doi: 10.1161/CIRCULATIONAHA.121.055654
215. Block RC, Liu L, Herrington DM, Huang S, Tsai MY, O’Connell TD, et al. Predicting risk for incident heart failure with omega-3 fatty acids: from mesa. JACC Heart Fail. (2019) 7:651–61. doi: 10.1016/j.jchf.2019.03.008
216. Alfaddagh A, Kapoor K, Dardari ZA, Bhatt DL, Budoff MJ, Nasir K, et al. Omega-3 fatty acids, subclinical atherosclerosis, and cardiovascular events: implications for primary prevention. Atherosclerosis. (2022) 353:11–9. doi: 10.1016/j.atherosclerosis.2022.06.1018
217. Watanabe S, Yoshihisa A, Kanno Y, Takiguchi M, Yokokawa T, Sato A, et al. Associations with eicosapentaenoic acid to arachidonic acid ratio and mortality in hospitalized heart failure patients. J Card Fail. (2016) 22:962–9. doi: 10.1016/j.cardfail.2016.04.017
218. Zheng S, Qiu M, Wu JHY, Pan XF, Liu X, Sun L, et al. Long-chain omega-3 polyunsaturated fatty acids and the risk of heart failure. Ther Adv Chronic Dis. (2022) 13:20406223221081616. doi: 10.1177/20406223221081616
219. Eclov JA, Qian Q, Redetzke R, Chen Q, Wu SC, Healy CL, et al. Epa, Not Dha, prevents fibrosis in pressure overload-induced heart failure: potential role of free fatty acid receptor 4. J Lipid Res. (2015) 56:2297–308. doi: 10.1194/jlr.M062034
220. O’Connell TD, Block RC, Huang SP, Shearer GC. Ω3-polyunsaturated fatty acids for heart failure: effects of dose on efficacy and novel signaling through free fatty acid receptor 4. J Mol Cell Cardiol. (2017) 103:74–92. doi: 10.1016/j.yjmcc.2016.12.003
221. Oppedisano F, Mollace R, Tavernese A, Gliozzi M, Musolino V, Macrì R, et al. Pufa supplementation and heart failure: effects on fibrosis and cardiac remodeling. Nutrients. (2021) 13:2965.
222. Kang GJ, Kim EJ, Lee CH. Therapeutic effects of specialized pro-resolving lipids mediators on cardiac fibrosis via Nrf2 activation. Antioxidants. (2020) 9:1259. doi: 10.3390/antiox9121259
223. Chen X-L, Dodd G, Thomas S, Zhang X, Wasserman MA, Rovin BH, et al. Activation of Nrf2/are pathway protects endothelial cells from oxidant injury and inhibits inflammatory gene expression. Am J Physiol Heart Circ Physiol. (2006) 290:H1862–70. doi: 10.1152/ajpheart.00651.2005
224. Saunders RL, Farrell AP. Coronary arteriosclerosis in atlantic salmon. no regression of lesions after spawning. Arteriosclerosis. (1988) 8:378–84. doi: 10.1161/01.atv.8.4.378
225. Dalum AS, Kristthorsdottir KH, Griffiths DJ, Bjørklund K, Poppe TT. Arteriosclerosis in the ventral aorta and epicarditis in the bulbus arteriosus of atlantic salmon. (Salmo Salar L). J Fish Dis. (2017) 40:797–809. doi: 10.1111/jfd.12561
226. Farrell AP, Saunders RL, Freeman HC, Mommsen TP. Arteriosclerosis in atlantic salmon: effects of dietary cholesterol and maturation. Arteriosclerosis Thromb Vasc Biol. (1986) 6:453–61. doi: 10.1161/01.atv.6.4.453
227. Bosch J, Gerstein HC, Dagenais GR, Díaz R, Dyal L, Jung H, et al. N-3 fatty acids and cardiovascular outcomes in patients with dysglycemia. N Engl J Med. (2012) 367:309–18. doi: 10.1056/NEJMoa1203859
228. Bowman L, Mafham M, Wallendszus K, Stevens W, Buck G, Barton J, et al. Effects of N-3 fatty acid supplements in diabetes mellitus. N Engl J Med. (2018) 379:1540–50. doi: 10.1056/NEJMoa1804989
Keywords: atherosclerosis, cardiovascular disease, polyunsaturated fatty acids, inflammation, lipid metabolism
Citation: Kotlyarov S and Kotlyarova A (2022) Clinical significance of polyunsaturated fatty acids in the prevention of cardiovascular diseases. Front. Nutr. 9:998291. doi: 10.3389/fnut.2022.998291
Received: 19 July 2022; Accepted: 20 September 2022;
Published: 06 October 2022.
Edited by:
Manohar Garg, The University of Newcastle, AustraliaReviewed by:
Arrigo Francesco Giuseppe Cicero, University of Bologna, ItalyPhilip Calder, University of Southampton, United Kingdom
Copyright © 2022 Kotlyarov and Kotlyarova. This is an open-access article distributed under the terms of the Creative Commons Attribution License (CC BY). The use, distribution or reproduction in other forums is permitted, provided the original author(s) and the copyright owner(s) are credited and that the original publication in this journal is cited, in accordance with accepted academic practice. No use, distribution or reproduction is permitted which does not comply with these terms.
*Correspondence: Stanislav Kotlyarov, SKMR1@yandex.ru