- 1Department of Biochemistry and Physiology, Faculty of Pharmacy and Food Sciences, Universitat de Barcelona, Barcelona, Spain
- 2Department of Pharmacy, Faculty of Health Sciences, Universidad San Jorge, Zaragoza, Spain
- 3School of Pharmacy, Università di Camerino, Camerino, Italy
- 4Instituto Agroalimentario de Aragón-IA2, CITA-Universidad de Zaragoza, Zaragoza, Spain
The forest ecosystem is a source of material resources used since ancient times by mankind. Ferns are part of different oriental systems of traditional medicine due to the phytochemical variety of their fronds, which have allowed their traditional use to be validated through ethnopharmacological studies. In Europe, different cultures have used the same fern with a wide variety of applications due to its presence in most European forests. In recent years, studies on the phytocharacterization and biological activity of the fronds of the main European ferns have been published. In this study, the presence of polyphenolic phytochemicals has been evaluated by high-performance liquid chromatography-tandem mass spectrometry (HPLC-MS/MS) in the fronds of two wild ferns together with in vitro activities in non-tumoral and human tumoral cell lines. The polyphenols were extracted from Asplenium trichomanes L. and Ceterach officinarum Willd. by cold maceration using methanol. The main phytochemicals of polyphenolic origin in the extracts of A. trichomanes and C. officinarum determined by HPLC-MS/MS were the flavonol hyperoside and the phenolic acid chlorogenic acid, respectively. This different polyphenolic nature of both extracts contributes to the divergence of the behavior experienced in the biological activities tested, but none of the extracts showed a cytotoxic or phototoxic profile in the different tested cell lines. However, the cytoprotective values in front of the H2O2 oxidative stress induced in the 3T3 and HaCaT cell lines position these extracts as possible candidates for future health applications.
Introduction
Currently, in most countries, forest management is oriented to obtain wood resources. However, in the last decade, there has been an increase in the demand for natural food production, and the global food demand for 2050 is predicted to triplicate the actual demand. Forests may be one via to ensure the food and nutritional security of the population according to the World Food Security (1), especially in industrialized societies due to its lower relationship with nature than rural societies. Bioeconomy is the discipline that covers all activities related to the production, use, and transformation of bioresources, and their functions and principles, among which we find food production, animal feed, and the generation of products, energy, and services (2). Currently, the European Commission’s concept of the bioeconomy incorporates sustainability and circularity with the aim of being respectful with the environment. The food plant resource is one of the main branches of innovations in the bioeconomy due to the broad spectrum of macronutrients and micronutrients in plants compared to foods from animal sources (3).
Pteridophytes (from Greek pteron, meaning “wing” and phyton, meaning “plants”) are the tracheophyta plants with an authentic development of the vascular system and that reproduces sexually by spores (4). Currently, the potential uses of ferns are underestimated as only the properties of a small number of the around 13,000 species of ferns have been studied (5). In 2017, Cao et al. (6) published a review of the valuable reservoir of phytochemicals with the corresponding properties, among which antioxidant, anticancer, antimicrobial, and anti-inflammatory activities have been demonstrated.
Literature reviewing the uses of ferns is irregular worldwide. The continents with the highest number of records in decreasing order are Asia-Tropical, South America, and Asia-Temperate. Nevertheless, Asia-Tropical has approximately three times more reviewed literature uses of ferns than South America (7). Ferns are currently considered a source of polar phytochemicals as phenolic acids and flavonoids with therapeutic and nutritional value in eastern countries, especially in Nepal, India, and China (8–10). This is the case of some ferns described in the traditional Chinese medicine, as the case of Pteridium aquilinum (L.) Kuhn (Dennstaedtiaceae) (10). In addition, the presence of non-polar phytochemicals, such as fatty acids and lipids, has also been described in ferns (11).
Because of the limitations of the floral bioindicator methodology of Ellenberg values, the need for new ecological bioindicators is derived (12). Ferns are susceptible to little environmental changes, and this taxon is positioning itself as an important ecological indicator, as reported in an ecological indicator study in Mexico (13). In Europe, one of the most important families of ferns is the Aspleniaceae family (14), particularly at the Mediterranean coast characterized by its moderated temperatures and high humidity (15). The phenotype studies classified as Aspleniaceae family (from Greek a, meaning “without” and splen, derivate to “spleen” in reference to its use to treat ailments of that organ), known as “spleenwort,” in the eupolypods II group (16, 17). The sporophytes of this taxon are reported in different forms, but they have the common character to be epipetric ferns. Because they grow in rock habits they are also popularly known as “rock ferns”. Nowadays, in some areas of Europe, the Pteridophytes distribution has been characterized as the case of Sicily (18). In Spain, researchers have determined the distribution of Iberian Peninsula ferns (19), that is, the case of the Banco de Datos de Biodiversidad de Cataluña (20), being the Asplenium trichomanes L. (Aspleniaceae) (21) and Ceterach officinarum Willd. (Aspleniaceae) (22) the predominant fern species in the Prades mountains (Tarragona, Spain). Asplenium ceterach L. is a synonym name of Ceterach officinarum Willd.
The sporophyte is the principal characteristic part in the identification taxonomy of ferns (23). The A. trichomanes and C. officinarum fronds present different morphological features that facilitate the difference between both species, as shown in Image 1. The frond of A. trichomanes has a long black petiole with parallel oval pinnas not directly united with the rachis. While the frond of C. officinarum has a short petiole with the pinnas attached directly to the rachis alternately forming a saw. In addition, the soris of C. officinarum has the characteristic of being covered with reddish scales on the underside frond (24). Through the ambivalence of the Latin names used in the past text and the different names attributed to the same plant in different regions, A. trichomanes has been sometimes confused with another Aspleniaceae ferns as C. officinarum (16).
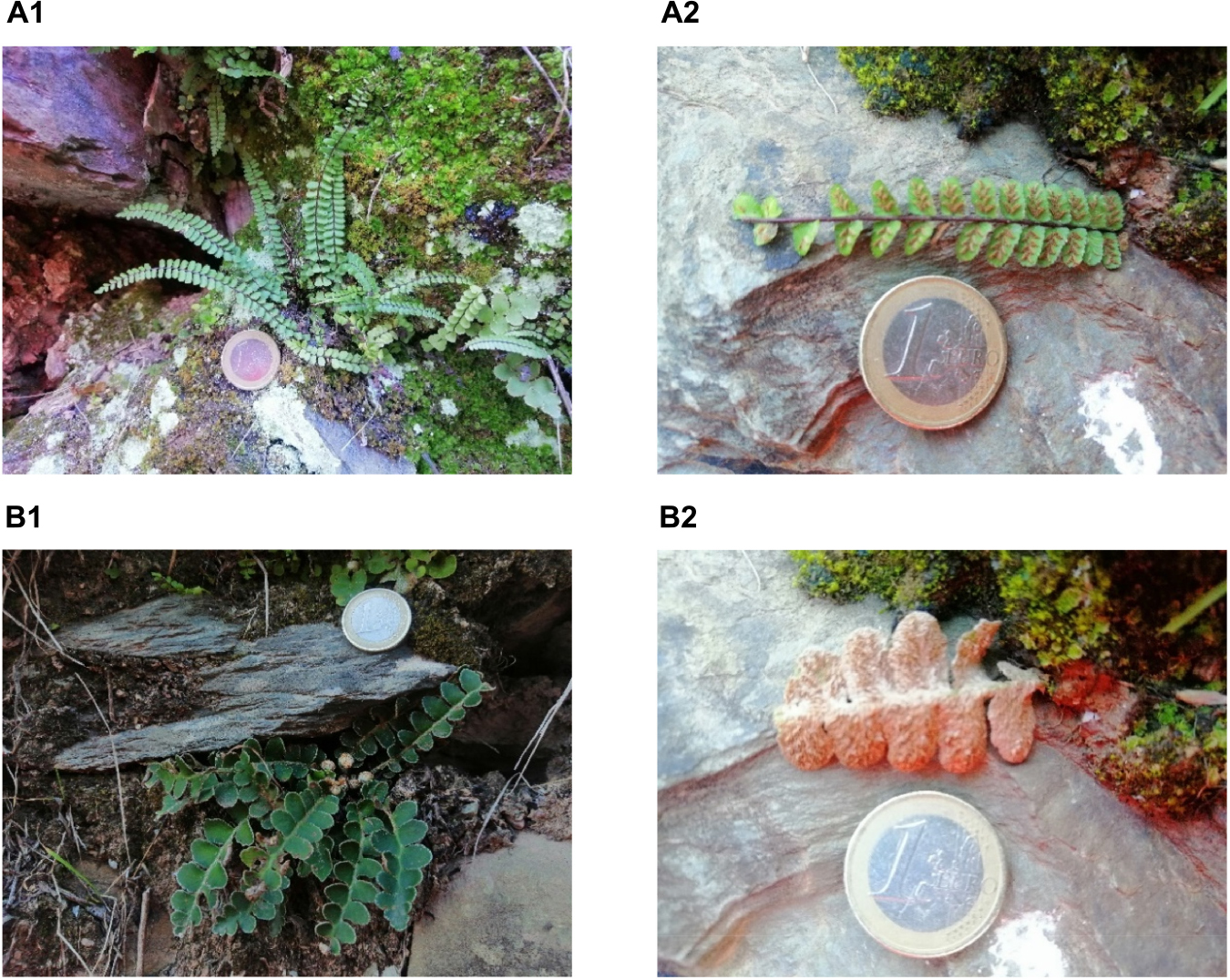
Image 1. Face fronds (1) and underside frond (2) of fresh Asplenium trichomanes L. (Aspleniaceae) (A) and fresh Ceterach officinarum Willd. (Aspleniaceae) (B) by Adrià Farràs at Prades mountains. The euro coin referents the dimension of the frond (A2, B2).
In Catalonia, the popular name of A. trichomanes is falzia vermella and for C. officinarum is dauradella (24). In English literature, these ferns are popularly known as “maidenhair spleenwort” for A. trichomanes (25) and “golden herb” for C. officinarum (26). Previous studies have considered the fronds of A. trichomanes and C. officinarum as reservoirs of flavonols (kaempferol and its glycosides) (27) and cinnamic acids (as chlorogenic acid) (28), respectively. The fact that leads to position these ferns as valuable resources of polyphenolic phytochemicals for nutritional applications. The reported traditional uses of A. trichomanes and C. officinarum in Pyrenees have been used for respiratory tract diseases. The fronds tisane from both ferns has been used for its anticatarrhal properties and specifically A. trichomanes as an antitussive (29, 30). These ethnopharmacological studies highlight the presence of phytochemicals in both species of these two active ferns against respiratory tract infectious diseases. Positioning both ferns as possible functional foods in the prevention of colds (31). Other described uses of the fronds of A. trichomanes are for the treatment of alopecia and kidney stones (16). In the case of the fronds of C. officinarum, in Catalonia, it has also been described as a vasotonic (32) in Alt Empordà and for the treatment of cold sores in the Prades mountains (33).
In this article prior to the study of the in vitro activity against situations of oxidative stress of the methanolic extracts of A. trichomanes fronds (ATM) and C. officinarum fronds (COM) harvested in the Prades mountains, we have evaluated the cytotoxicity and phytocharacterization of both extracts.
Materials and methods
Chemicals and reagents
Sigma-Aldrich (Milan, Italy) served 36 analytical standards (which were gallic acid, neochlorogenic acid, chlorogenic acid, p-hydroxybenzoic acid, 3-hydroxybenzoic acid, caffeic acid, vanillic acid, syringic acid, p-coumaric acid, ferulic acid, 3,5-dicaffeoylquinic acid, ellagic acid, delphinidin-3,5-diglucoside, delphinidin-3-galactoside, cyanidin-3-glucoside, petunidin-3-glucoside, pelargonidin-3-rutinoside, pelargonidin-3-glucoside, malvidin-3-galactoside, rutin, isoquercitrin, quercitrin, myricetin, isorhamnetin, hyperoside, kaempferol, catechin, epicatechin, procyanidin B2, procyanidin A2, phloridzin, phloretin, hesperidin, naringin, resveratrol, and trans-cinnamic acid) of the 38 phytochemicals, determined by high-performance liquid chromatography-tandem mass spectrometry (HPLC-MS/MS). While kaempferol-3-glucoside and quercetin were served by PhytoLab (Vestenbergsgreuth, Germany). The 1,000 mg/mL concentration was used for each stock solution. In which the pure references substance was dissolved by HPLC-grade methanol and then stored at 5°C in glass vials until the evaluation. The HPLC-grade methanol was also used to prepare the diluting stock solutions for the working solutions. The HPLC-grade methanol and formic acid (99% concentration) was informed by Sigma-Aldrich and Merck, respectively. A 0.2 μm polyamide filters, which were used to filter all liquids, were administered by Sartorius Stedim provided (Goettingen, Germany). Before injecting into the HPLC, all samples were filtered by Phenex™ RC 4 mm 0.2 μm syringeless filters (Phenomenex, located in Castel Maggiore, BO, Italy). The ultrapure water (resistivity of >18 MΩ cm) was obtained by A Milli-Q SP Reagent Water System (Millipore, Bedford, MA, United States). The coolant trypan blue (0.4%) dye was obtained and was expedited from Sigma-Aldrich (Madrid, Spain). The reactive viability thiazolyl blue tetrazolium bromide (MTT), dimethyl sulfoxide (DMSO) solvent, the marker 2,7-dichlorodihydrofluorescein diacetate (DCF), and photosensibility chlorpromazine hydrochloride (CPZ, CAS No. 69-09-0) also were supplied by Sigma-Aldrich. The products to maintain proper cell growth except for the HyClone fetal bovine serum (FBS) were purchased from Thermo Scientific (Northumberland, United Kingdom). These products were Dulbecco’s modified Eagle’s medium (DMEM) with and without phenol red, phosphate-buffered saline (PBS), L-glutamine solution (200 mM), and penicillin–streptomycin solution (10,000 U/mL penicillin and 10 mg/mL streptomycin) were supplied by Lonza (Verviers, Belgium). This distributor also provides the trypsin-ethylenediaminetetraacetic acid (EDTA) solution (170,000 U/L trypsin and 0.2 g/L EDTA). The TPP (Trasadingen, Switzerland) supplemented the 75 cm2 culture flasks and 96-well plates.
Plant material
After we verified that A. trichomanes L. and C. officinarum Willd. were located at Prades mountains 41°17′34″N 1°02′42″E geographical coordinates (Tarragona, Spain) by Banco de Datos de Biodiversidad de Cataluña (20), the fronds of these ferns were harvested from this area. We obtained a dried voucher stored at Herbarium of Universidad San Jorge (Zaragoza, Spain), A. trichomanes L. with the voucher n° 005-2016 and C. officinarum Willd. with the voucher n° 006-2016. These ferns were authenticated by Dr. J.A. Vicente Orellana from Universidad CEU San Pablo (Madrid, Spain) using botanical keys.
Preparation of methanolic extract of Asplenium trichomanes L. and Ceterach officinarum Willd. fronds
Once we obtained the dried powdered fronds, these parts were macerated with methanol for 24 h at room temperature. The result was a methanolic extract which was filtered by Whatman N°4 filter paper and evaporated using a rotatory evaporator with a thermostatic bath at 30°C to eliminate the solvent. This process was repeated three times until exhaustion of the plant material. This process is described by us in a previous publication (34). The extracts were kept at −20°C until experimental bioassays. The samples were dissolved in methanol for HPLC analysis or in a culture medium for cell experiments.
Polyphenol characterization in the methanolic extract of Asplenium trichomanes L. and Ceterach officinarum Willd. fronds by high performance liquid chromatography-tandem mass spectrometry
The quantification of all phytochemicals determined was performed using a modified version of our prior designed assay (35). The Agilent 1290 Infinity series and a Triple Quadrupole 6420 bought from Agilent Technology located in Santa Clara (CA, United States) were used to perform the HPLC-MS/MS and linked to an electrospray ionization (ESI) source that operated in negative and positive ionization modes. Using Optimizer Software, the MS/MS parameters of each standard were optimized by using flow injection analysis (FIA). The isolation of phenolic compounds was obtained by the following methodology: a direct injection of diluted sample (1:5) using gradient elution mode on a Phenomenex Synergi Polar–RP C18 column (250 mm × 4.6 mm, 4 μm) using a mixture of water and methanol as solvents A and B, respectively, both with 0.1% formic acid (starting ratio is 80% A and 20% B). For column protection, a polar RP security guard cartridge preceded the column (4 mm × 3 mm ID). The mobile phase composition was a mixture of the following components: 0–1 min, isocratic condition, 20% B; 1–25 min, 20–85% B; 25–26 min, isocratic condition, 85% B; 26–32 min, 85–20% B. The injection volume was 2 μl, and the flow rate was kept at 0.8 mL/min. The selected temperatures for the column and drying gas in the ionization source were 30 and 350°C, respectively; 12 L/min gas flow rate, 55 psi nebulizer pressure, 4,000 V the capillary voltage, and 2 min the specific time window for each compound (Δ retention time) were the technical specifications of the instrument defined for this assay. The peak areas were integrated for quantitation after detection in the dynamic-multiple reaction monitoring (dynamic-MRM) mode. The transition and collision energies for each compound are provided as Supplementary material. The principle product ion was used for quantification, while the rest of the ions were employed for qualitative analysis.
Cell culture
The non-tumoral cell lines (mouse fibroblast NIH 3T3 and the spontaneously immortalized human keratinocyte HaCaT cell lines) were used in all in vitro experiments. In the case of cytotoxic assay, cell viability was also evaluated by the human tumoral cell lines: cervix epitheloid carcinoma HeLa, Caucasian hepatocyte carcinoma HepG2, Caucasian breast adenocarcinoma MCF-7, and Caucasian lung carcinoma A549 cell lines. Sigma-Aldrich, as a worldwide provider of the European Collection of Authenticated Cell Cultures (ECACC), provided the 3T3 and A549. Whereas Eucellbank (Celltec-Universitat de Barcelona) subminister the HaCaT, HeLa, and MCF-7. Kindly, Dr. Borràs of Experimental Toxicology and Ecotoxicology Platform (UTOX-CERETOX) of Parc Científic of Universitat de Barcelona donated the HepG2 cell line. Cell lines were grown in Dulbecco’s Modified Eagle’s medium (DMEM) supplemented with 10% heat-inactivated fetal bovine serum (FBS), 2 mM L-glutamine, and 100 U/mL:100 U/mL streptomycin–penicillin mixture (10% FBS-DMEM) at 37°C in a 5% carbon dioxide (CO2)-humidified incubator. Cells were regularly checked and subsequently subcultured in 75 cm2 flasks. When cells reached 80% of confluence in the flask, culture medium was removed, cells were treated with trypsin-EDTA after cleansing with PBS, to obtain a cell suspension. Cell density was determined by staining an aliquot of the cell suspension with the vital dye trypan blue (0.4%), and the final volume was adjusted according to 100 μL of the cell suspension (1 × 105 cells/mL). Which were seeded in 96-well microplates and incubated overnight (37°C and 5% CO2).
Determination of cell viability by the uptake of the Neutral Red dye and thiazolyl blue tetrazolium bromide assays
Cell viabilities were determined by the uptake of the Neutral Red dye (NRU) and thiazolyl blue tetrazolium bromide assays (MTT) methods after treatments.
Some explained adaptations have been applied to the Borenfreund and Puerner protocol for the determination of cell viability by NRU (36). The supernatant was aspirated from each well and 100 μL of NR solution (0.05 mg/mL in DMEM 0% FBS without phenol red) was added after the treatment incubation time. After 3 h, the supernatant was removed by inversion from the plate, and 100 μL of the developer NR solution was added. In the developer solution, the formaldehyde was replaced by an acidic ethanol solution as defined by Riddell et al. (37). The viable cells corresponded to the quantification of the remnant NR, which is the NRU linked to the lysosomes (38). After shaking the plate (5–10 min), the absorbance was obtained at 550 nm, by Tecan Sunrise® microplate reader (Männedorf, Switzerland).
The slight modifications described by Zanette et al. (39) were applied to the MTT assay, based on the experimental protocol of Mosmann (40). A total of 100 μL of an MTT solution (0.5 mg/mL in 0% FBS-DMEM without phenol red) was incorporated in each well following incubation of the plates for at least 3 h in cell culture incubation conditions (37°C and 5% CO2). When finished the incubation, the culture medium in all assay wells was substituted for 100 μL of the organic dissolvent dimethyl sulfoxide (DMSO), with the objective to dissolve the formazan crystals (41). The amount of soluble formazan is proportional to the number of cells with optimal mitochondrial activity (42). Before reading the absorbance at 550 nm using a Tecan Sunrise® microplate reader (Männedorf, Switzerland), the homogenization content of each well content was realized by gently shaking the microplate (5 min at 100 rpm/min).
Cell viability for NRU and MTT assays was obtained using the following equation:
where Acontrol is the arc of the absorbance of the control, while Asample is the absorbance for each sample.
Cytotoxicity activity of methanolic extract of Asplenium trichomanes L. and Ceterach officinarum Willd. fronds in non-tumoral and tumoral cells lines
Each cell lines assayed were treated for 24 h (37°C and 5% CO2) with the following increment concentrations: 0.01, 0.1, and 1 mg/mL methanolic fronds extracts in 5% FBS-DMEM. In each plate, the negative controls were the untreated cells (maintained with a culture medium). Cytotoxicity of ATM and COM was evaluated by the NRU and MTT methods.
Cytoprotective and cellular repair activity of methanolic extract of Asplenium trichomanes L. and Ceterach officinarum Willd. fronds in non-tumoral cell lines
For the evaluation of the effect of the application of oxidative stress agent after treatment (cytoprotection) or before extract treatment (cellular repair), hydrogen peroxide was selected as the oxidative stress agent (43).
Cytoprotective activity in 3T3 and HaCaT cell lines
Cells were pretreated with the selected methanolic fronds extracts (0.01, 0.1, and 1 mg/mL; 100 μL) dissolved by 5% FBS-DMEM for 24 h following addition of H2O2 (in 5% FBS-DMEM) at a final concentration 2 mM for 2.5 h. Then, cell viability was determined by NRU and MTT assay. In each microplate, negative and positive controls were included. The positive controls for this assay consist of cells treated with H2O2 at 2 mM during 2.5 h without previous pretreatment with the extracts.
Cytoprotective activity was obtained equation as follows:
in which the cell viability for each condition is described in the formula where CV.
Cellular repair activity in 3T3 cell line
The potential cellular repair ability of ferns extracts was evaluated by treating cells with different concentrations of ferns extract (0.01, 0.1, and 1 mg/mL in 5% FBS-DMEM; 100 μL), after induction of oxidative stress with 2 mM H2O2 2.5 h. For each independent experiment and plate, negative and positive controls were included. In this case, positive controls consist of the cells with 2 mM H2O2 treated for 2.5 h but without AAM extract post-treatment. After 24 h incubation, cell viability was assessed by NRU and MTT assays.
Cellular repair activity was calculated as follows equation:
in which the cell viability for each condition is described in the formula where CV.
Phototoxicity activity of methanolic extract of Asplenium trichomanes L. and Ceterach officinarum Willd. fronds in 3T3 and HaCaT cell lines
The potential phototoxic activity of ATM and COM was evaluated following the Organization for Economic Cooperation and Development (OECD) TG 432 (2019) (44) with some adaptions. Briefly, 3T3 and HaCaT cells were seeded at a density to form monolayers at 1 × 105 cells/mL (100 μL) in a 96-well microplate in 10% FBS-DMEM for 24 h. Then, pre-incubated with 0.01, 0.1, and 1 mg/mL methanolic fronds extracts (100 μL). The 0% FBS-DMEM without phenol red was the dissolvent to the samples, while positive and negative controls (cells no treated and treated with chloropromazine, 37.5 μg/mL chlorpromazine, respectively) were dissolved by 0% FBS-DMEM without phenol red. After the 1 h incubation, one plate remains in the dark, and other was exposed to 1.8 J/cm2 of ultraviolet A (UVA) light. When finished the irradiation process, the medium was replaced with 100 μL of fresh medium (10% FBS-DMEM) to determine the cell viability after 24 h of incubation by the NRU and MTT colorimetric assays.
Light exposure was performed in a photostability UV chamber (58 cm × 34 cm × 28 cm) with three UVA lamps Actinic BL TL/TL-D/T5 (Philips, 43 V, 352 nm, 15 W) as described by Martínez et al. (45). The dosage and time exposition of cells to UVA light was regularly settled by a photoradiometer Delta OHM provided with a UVA probe (HD2302—Italy). We followed the following equation:
where ultraviolet dose is E, represents the time expressed in seconds is t and the lamp potency is P.
Intracellular reactive oxygen species induced by H2O2 of methanolic extract of Asplenium trichomanes L. and Ceterach officinarum Willd. fronds in 3T3 and HaCaT cell lines
For the ROS assay, we followed the methodology of Ferreira et al. (46). After the pretreatment cells with the different concentrations of the methanolic fronds extracts (0.01, 0.1, and 1 mg/mL) for 24 h, cells were washed twice with PBS, and DCF (100 μM) was applied to each well for 45 min (37°C and 5% CO2). To remove the DCF which no penetrated cells, this was removed by washing twice with a cell culture medium, and then H2O2 (1 and 2 mM) was added into each well to induce oxidative stress. The fluorescence intensity of the oxidized product of DCF was registered ((λexcitation 480 nm; λemision 530 nm) at 0, 1, 2, and 3 h by a plate reader ThermoFisher SCIENTIFIC VARIOSKAN LUX (ThermoFisher SCIENTIFIC, Waltham, MA, United States). The fluorescence intensity (FI), which have adimensional units, was used to represent the results. The FIz h Vs 0h was calculated as follows equation:
where FIz h is the intensity of fluorescence at z h (z as 1, 2, or 3 h) of incubation and FI0 h is the amount of fluorescence intensity at 0 h.
The FI for each specific time was calculated using this formula:
The ΔROS, which has adimensional units for FI, was obtained using the following formula:
Statistical analysis
All experiments were executed in triplicates, and almost three independent experiments were assayed, on different days, except for the cytoprotection ferns extract for HaCaT against 2 mM H2O2 (2.5 h) MTT for which the results correspond to n = 2 experiments. Statistical analysis for MTT cell viability and fluorescence intensity (FI) was performed using GraphPad Prism version 7, San Diego, CA, United States. All data were expressed as mean ± standard error. Activities have been compared using a two-way analysis of variance (ANOVA) by Bonferroni. The results were considered significantly different when p ≤ 0.05 (*), p ≤ 0.01 (**), p ≤ 0.001 (***), and p ≤ 0.0001 (****).
Results
Phytochemical characterization of methanolic extract of Asplenium trichomanes L. and Ceterach officinarum Willd. fronds by high performance liquid chromatography-tandem mass spectrometry
Both methanolic extracts present a similar profile of phytochemicals except for neochlorogenic acid, pelargonidin-3-rutinoside, phloridzin, and naringin. Phloridzin was detected for the first time in A. trichomanes in low amounts. The phytochemicals neochlorogenic acid and naringin have only been detected in COM, while pelargonidin-3-rutinoside and phloridzin have only been found in ATM. A clearly higher content of phenolic species has been determined in the extract of C. officinarum (55,490.94 mg polyphenolic species/kg dry extract) than in the extract of A. trichomanes (4,637.30 mg polyphenolic species/kg dry extract), as represented in Table 1. In the C. officinarum extract, the content of the majority phytochemical prevails hugely front the rest of all other phytochemicals. This phytochemical is chlorogenic acid and constitutes 78.95% of the total phytochemicals determined in the extract. In the case of the A. trichomanes extract, the two major phytochemicals are those that present a higher quantity than the other phytochemicals determined in the extract. These two phytochemicals are flavonols (one type of flavonoids) hyperoside and isoquercitrin, which constitute 54.72% of the determined phytochemicals in the extract.
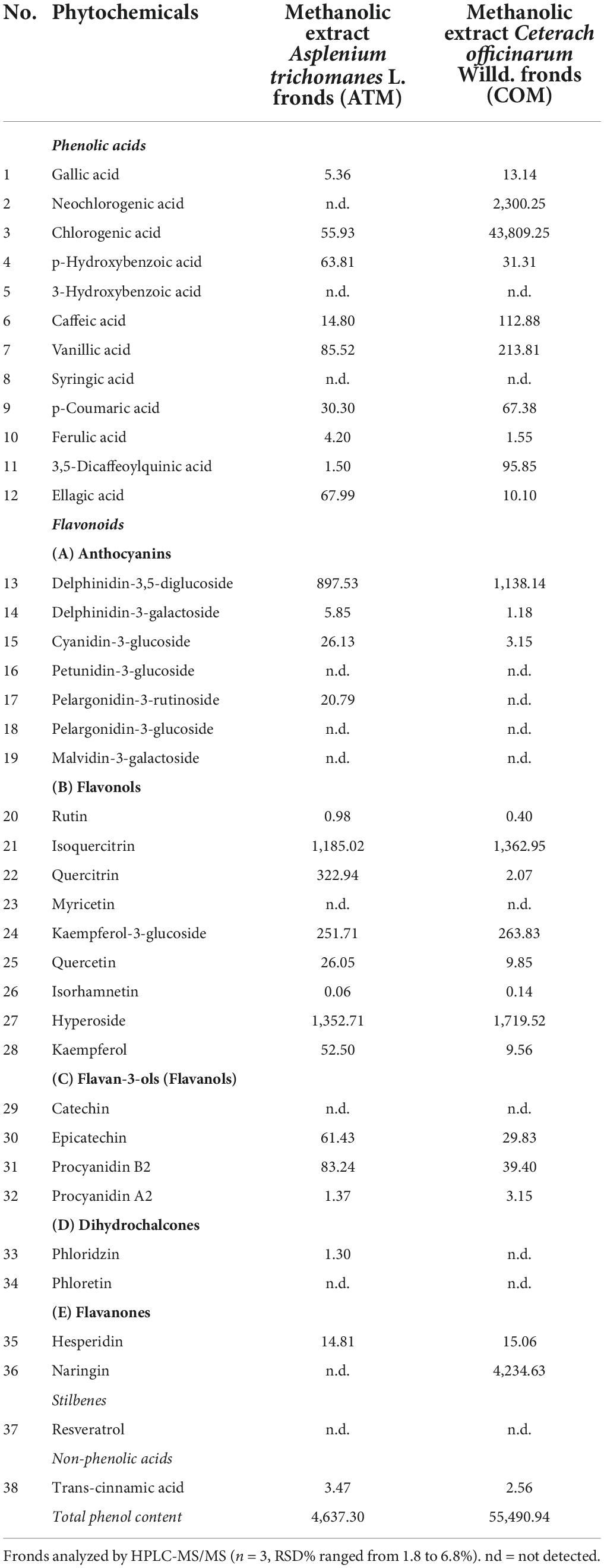
Table 1. Content (mg/kg of dry extract) of 38 polyphenolic phytochemicals in the methanolic extract of Asplenium trichomanes L. and Ceterach officinarum Willd. fronds.
When considering all the phytochemicals determined in HPLC-MS/MS, the different nature of both extracts is increased. Thus, when determining the global nature of the polyphenolic species, it is observed that in the extract of A. trichomanes, flavonoids (92.82% total phytochemicals determined) prevail over phenolic acids (7.10% total phytochemicals determined). While in the case of C. officinarum, phenolic acids prevail (84.08% total phytochemicals determined) over flavonoids (15.92% total phytochemicals determined).
The phenolic acids 3-hydroxybenzoic acid and syringic acid were not detected in both extracts. Some determined flavonoids represent a low proportion of the total phytochemicals determined for both extracts, as in the case of rutin, isorhamnetin, and procyanidin A2. The flavonoids petunidin-3-glucoside, pelargonidin-3-glucoside, malvidin-3-galactoside, myricetin, catechin, and phloretin were not found in any of the samples. The stilbene resveratrol wasn’t identified either.
In vitro cell assays
The results obtained with the NRU test did not show differences regardless of the conditions tested (data not shown).
Cytotoxicity activity of methanolic extract of Asplenium trichomanes L. and Ceterach officinarum Willd. fronds in non-tumoral and tumoral cell lines
The 3T3 and HaCaT (which are the non-tumoral cell lines assayed), Figures 1A,B, are characterized by the absence of increased cell viability greater than the corresponding negative control. The increment of the ATM concentration leads to a decline in cell viability for 3T3 and HaCaT cell lines, in which ATM is more sensible to 3T3 than HaCaT. While in the case of COM, similar behavior is observed for the two non-tumoral cell lines except at 1 mg/mL COM. The situation in which obtained the lowest cell viability (67.2% cell viability) for the six cell lines assayed. This situation was the only cytotoxic comportment for the six cell lines analyzed as a consequence that cell viability is ≤70%.
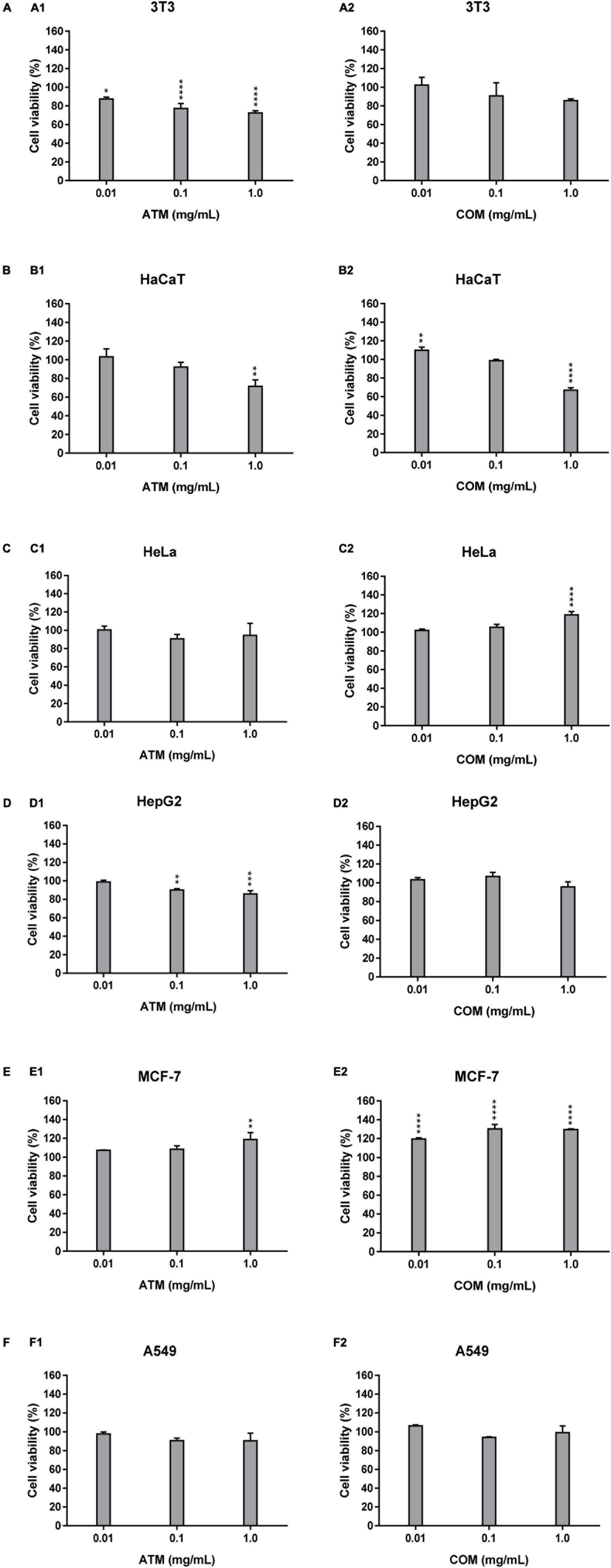
Figure 1. Cytotoxicity activity of ATM (1) and COM (2) in 3T3 (A) and HaCaT (B), HeLa (C), HepG2 (D), MCF-7 (E), and A549 (F) cell lines by MTT assay and expressed as a percentage of cell viability respect to control cells Results are expressed as mean ± standard error of n = 3. Control cells were maintained only with a culture medium. A two-way analysis of variance (ANOVA) and a Bonferroni post hoc assay have been performed. Statistical differences were considered as follows: *p ≤ 0.05, **p ≤ 0.01, ***p ≤ 0.001, and ****p ≤ 0.0001 comparison with no treated cells (negative control).
In the four human tumoral cell lines, both extracts show a similar behavior only for MCF-7 (Figure 1E). In which cell lines for both extracts present slightly higher cell viability than the negative control. In the case of the remaining tumoral cell lines (HeLa, HepG2, and A549), Figures 1C,D,F, the behavior is similar for each extract. ATM for these three tumoral cell lines, there is a mild decrease in cell viability up to 0.1 mg/mL ATM in which cell viability is stabilized. The COM does not show a clear affectation in the cell viability for HeLa, HepG2, and A549 cell lines at assayed extract concentrations. However, only a slight increase in HeLa cell viability is observed at 1 mg/mL COM. The situation in which there is observed an increment in cell viability less than 20% of the corresponding negative control.
When comparing the behavior of both extracts in non-tumoral versus tumoral cell lines, the only cell line in which a similar behavior has been determined has been in 3T3 and HepG2 for ATM.
Cytoprotective activity of methanolic extract of Asplenium trichomanes L. and Ceterach officinarum Willd. fronds in 3T3 and HaCaT cell lines
Prior to carrying out the cytoprotection assays, the cellular viability of HaCaT was determined for a range of concentrations-time of H2O2. To define the ideal conditions for the evaluation of the cytoprotective effect of ATM and COM in non-tumoral lines (data not shown). The ideal conditions for the oxidative stress-inducing agent selected were 2 mM H2O2 for 2.5 h.
In Table 2, cytoprotective results are shown at 2 mM H2O2 for 2.5 h. In Figure 2A, cytoprotection of ATM and COM in 3T3 cells against 2 mM H2O2 for 2.5 h is also demonstrated. Under these same cytoprotection conditions in the case of COM, this extract does not imply statistically significant modifications with respect to the corresponding positive control.
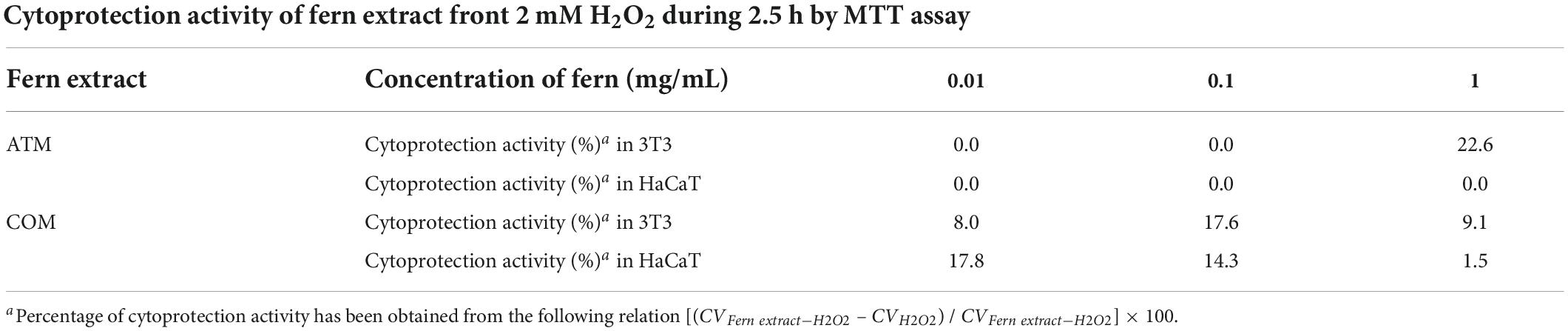
Table 2. Cytoprotection activity of ATM and COM in 3T3 and HaCaT cell lines for 2 mM H2O2 during 2.5 h by MTT assay.
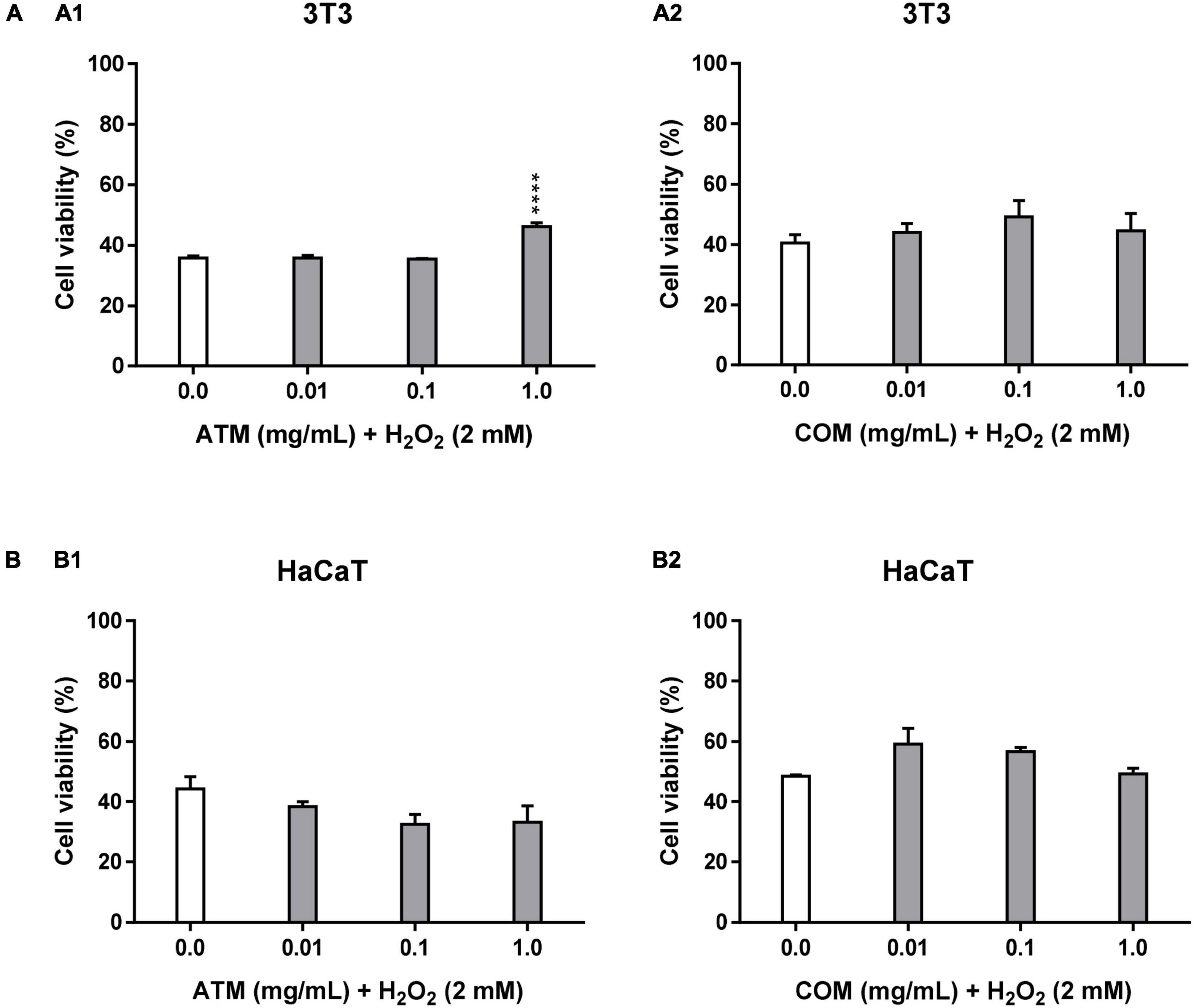
Figure 2. Cytoprotective activity of ATM (1) and COM (2) in 3T3 (A) and HaCaT (B) cell lines for 2 mM H2O2 during 2.5 h by MTT assay and expressed as a percentage of cell viability with respect to untreated cells control. H2O2 cell viability was used as a positive control. Results are expressed as mean ± standard error of n = 3 (3T3) and n = 2 (HaCaT). A two-way analysis of variance (ANOVA) and a Bonferroni post hoc assay have been performed. Statistical differences were considered as follows: ****p ≤ 0.0001 comparison with positive control.
Figure 2B shows the behavior of HaCaT in the cytoprotection assay of each of the extracts against 2 mM H2O2 for 2.5 h. The evolution of cell viability in relation to the increase in ATM concentrations does not present a clear trend. All cell viabilities for ATM concentrations are lower than the corresponding positive control. Nevertheless, COM is the only extract that shows a clary trend in decreasing cell viability with increasing concentration. Without obtaining effective cytoprotection values for COM. No statistically significant cell viability values have been obtained for both extracts in this assay.
Cellular repair activity of methanolic extract of Asplenium trichomanes L. and Ceterach officinarum Willd. fronds in 3T3 cell line
The 3T3 cell viability of the positive controls in ATM and COM in the cellular repair assay (23.8 and 20.4% viability, respectively) is lower than the corresponding ones in the cytoprotection assay (35.7 and 40.5% viability, respectively). This variability is the consequence that the oxidizing agent in the cellular repair assay is applied one day earlier than in the cytoprotective assay (described in methodology).
In the cellular repair assay, due to the absence of a cellular pre-treatment with the extract, which is carried out in the cytoprotection assay, it is expected to obtain lower cell viability than in the corresponding cytoprotection assays. In Figure 3, cellular repair assay in 3T3 for ATM and COM against the conditions of the agent inducing oxidative stress (2 mM H2O2 for 2.5 h), when compared with the corresponding cell viabilities of the assay of cytoprotection (Figure 2A) except for 1 mg/mL COM. In which cell viability in both cases are similar (Cytoprotective 3T31 mg/mL COM = 44.5% cell viability; Cellular repair 3T31 mg/mL COM = 44.6% cell viability).
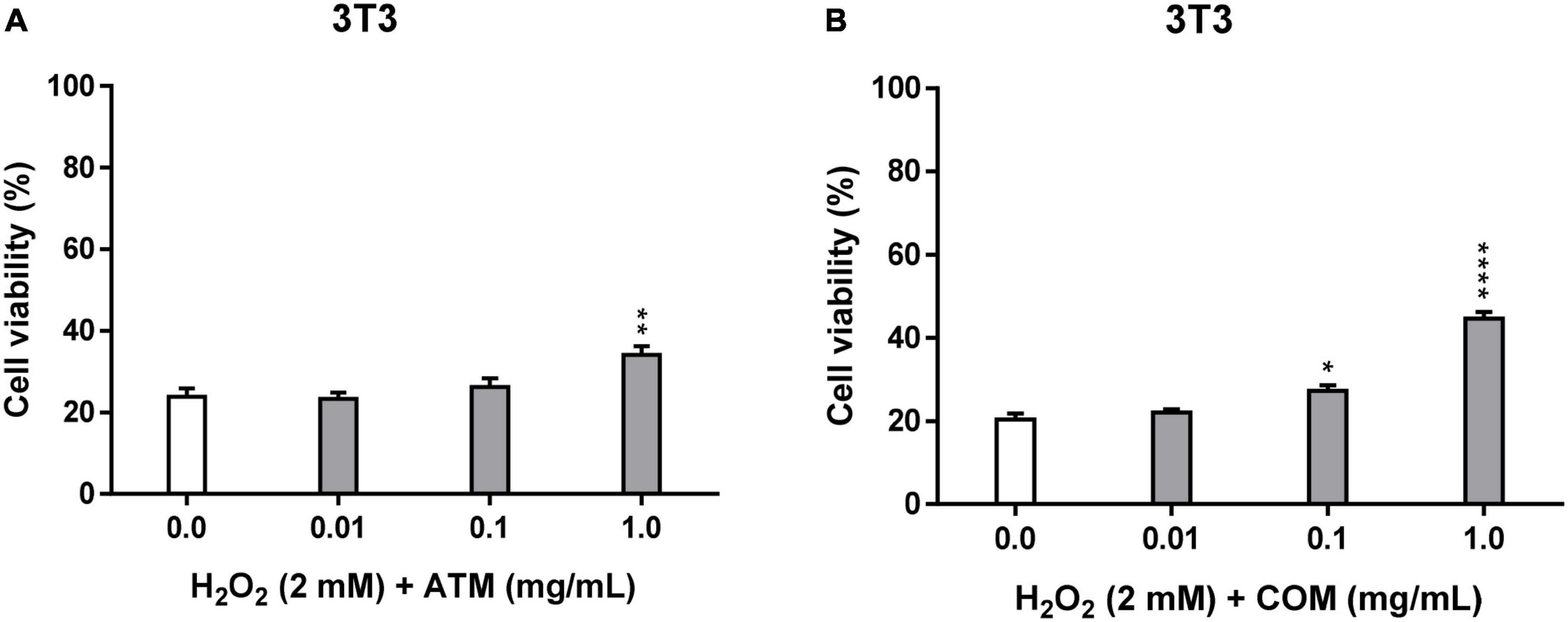
Figure 3. Cellular repair activity of ATM (A) and COM (B) in 3T3 cell line for 2 mM H2O2 during 2.5 h by MTT assay and expressed as a percentage of cell viability with respect to untreated cells control. H2O2 cell viability was used as a positive control. Results are expressed as mean ± standard error of n = 3. A two-way analysis of variance (ANOVA) and a Bonferroni post hoc assay have been performed. Statistical differences were considered as follows: *p ≤ 0.05, **p ≤ 0.01, and ****p ≤ 0.0001 comparison with positive control.
Both extracts have generally exhibited a greater protective effect in the cellular repair assay than in the corresponding cytoprotective assay. The viability of the samples in the cellular repair assay is always higher than the corresponding positive control in COM (statistically significant values from 0.1 mg/mL COM), while in ATM, it is only from 0.1 mg/mL extract (3T30.1 mg/mL ATM = 26.1% cell viability), in which the only statistically significant values for ATM are at 1 mg/mL ATM. The trend of the evolution of 3T3 cell viability experienced for ATM in the cytoprotective assay is like that experienced in the cellular repair assay, in which the trend of increasing cell viability as a function of extract concentration is observed clearly from 0.1 to 1 mg/mL ATM. In the case of COM, the evolution of cell viability in the cell repair extract is different from that experienced in the cytoprotective assay (increasing the concentration of extract leads to an increase in cell viability). Result that no clary trend has experienced cell viability with the increasing COM concentration in cellular repair assay.
In the cellular repair assay for both extracts, a percentage of effective cellular repair is obtained only at 1 mg/mL extract. But COM seems clearly superior compared with ATM (Table 3).

Table 3. Cellular repair activity of ATM and COM in 3T3 cell line for 2 mM H2O2 during 2.5 h by MTT assay.
Phototoxicity activity of methanolic extract of Asplenium trichomanes L. and Ceterach officinarum Willd. fronds in 3T3 and HaCaT cell lines
Irradiated DMEM control versus non-irradiated DMEM control gates a decrease of around 30% cell viability for 3T3 and HaCaT cell lines (Table 4). One possible bias of the low ratio of this control has been eliminated, obtaining the corresponding viabilities with respect to the non-irradiated DMEM (in the graphic representation of the phototoxicity test, the cell viability is represented as a function of the non-irradiated DMEM for the non-irradiated ones and the irradiated DMEM for irradiated).
The positive control shows a high phototoxic effect of irradiated chlorpromazine compared to the corresponding non-irradiated situation. Obtaining a greater sensitivity of the oxidative stress inducing agent in HaCaT than in 3T3, which is the contrast obtained in the positive cytoprotection control at 2 mM H2O2 for 2.5 h.
Figure 4 represents the phototoxicity test at 1.8 J/cm2 for 3T3 and HaCaT using MTT for ATM and COM. In none of the concentrations tested for both extracts for 3T3 have phototoxic behavior been obtained (Figure 4A). Presenting both extracts a similar behavior to 0.01 and 0.1 mg/mL extract with higher cell viability in the irradiated homologue than in the non-irradiated one. The fact that by presenting an increase in the cell viability of the irradiated compared to the non-irradiated one ≥20% in ATM at 0.1 mg/mL extract and in COM at 0.01 and 0.1 mg/mL extract, a possible photoprotective effect is considered. At 1 mg/mL COM, no irradiated decrease in cell viability is manifested in comparison to CPZ no irradiated. A situation that confirms the previous cytotoxic comportment of this extract in 3T3 at the highest concentration assayed.
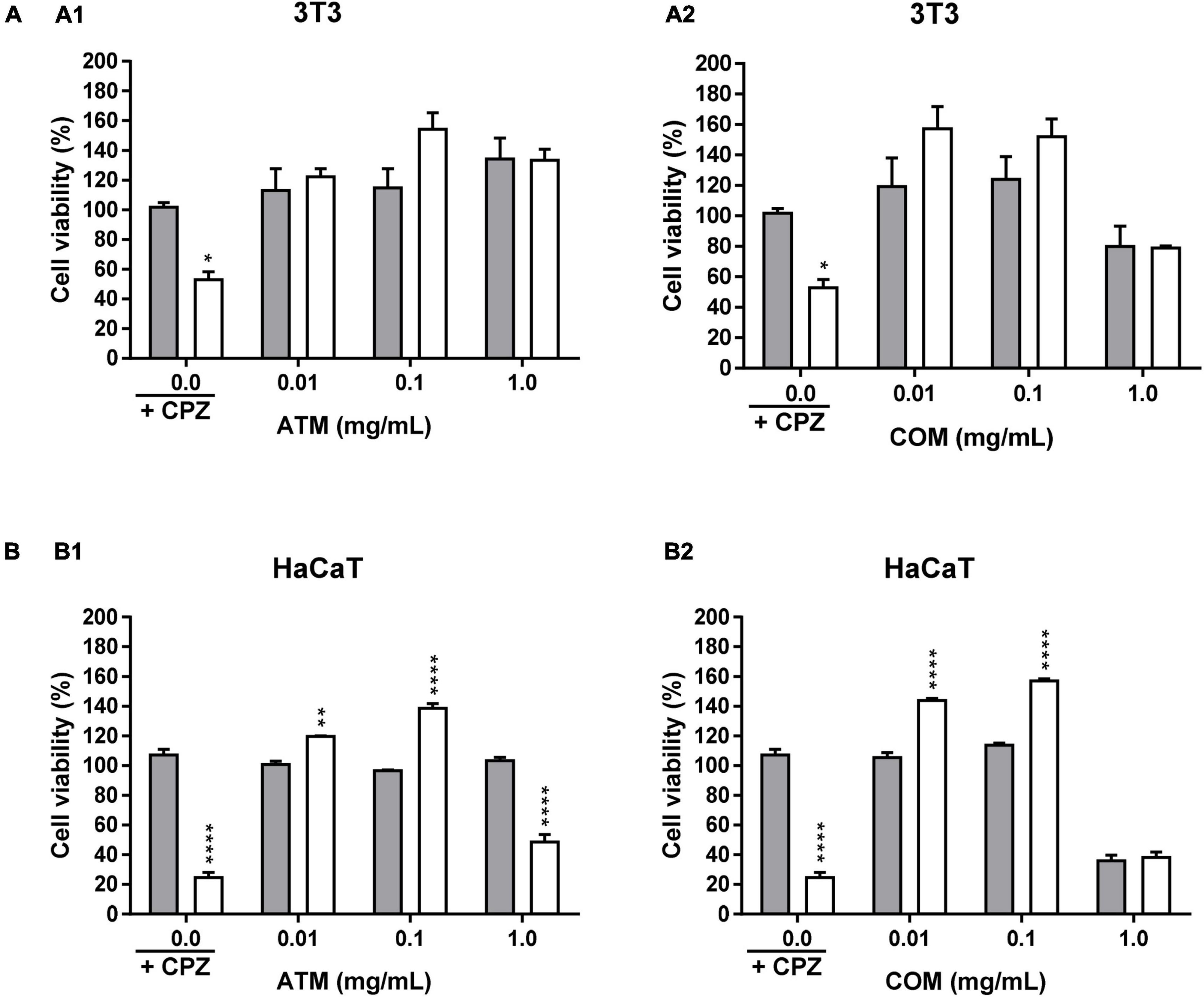
Figure 4. Phototoxicity activity of ATM (1) and COM (2) in 3T3 (A) and HaCaT (B) cell lines by MTT assay and expressed as a percentage of cell viability with respect to the correspondent control cells Chlorpromazine cell viability was used as positive control. Gray columns correspond to cells nonexposed to UVA light and white columns correspond to cells exposed to 1.8 J/cm2 of UVA light. Results are expressed as mean ± standard error of n = 3. A two-way analysis of variance (ANOVA) and a Bonferroni post hoc assay have been performed. Statistical differences were considered as follows: *p ≤ 0.05, **p ≤ 0.01, and ****p ≤ 0.0001 comparison with the equivalent nonirradiated condition homologue.
Figure 4B represents the phototoxicity test at 1.8 J/cm2 for the ATM and COM extracts in HaCaT by MTT. At 0.01 and 0.1 mg/mL extract, the same behavior is experienced as in the corresponding situation as in 3T3. Wherein the viability between homologues is higher in the irradiated compared to the corresponding non-irradiated. However, in 1 mg/mL COM, the effects previously observed in cytotoxicity assay prevail. While ATM, despite not observing a significant decrease in cell viability in the non-irradiated 1 mg/mL extract (it is not a cytotoxic extract at assayed conditions), in the corresponding irradiated situation the extract does not have the ability to reverse the photosensitizing effect induced by UVA.
Intracellular ROS induced by H2O2 of methanolic extract of Asplenium trichomanes L. and Ceterach officinarum Willd. fronds in 3T3 and HaCaT cell lines
The ROS value does not undergo significant modifications over time. Rather, time only slightly increases ROS values without modifying the profile experienced in each of the times analyzed (1, 2, and 3 h). The reason why only ROS is represented at 2 h (ROS at 1 h and 3 h not represented).
The ROS value for both 3T3 positive controls (at 1 and 2 mM H2O2; for each concentration of H2O2 are the same positive controls for both extracts) is higher than the corresponding HaCaT values (ROS positive control for 1 mM H2O2: ROS1 mM H2O2 for 3T3 = 168.0 and ROS1 mM H2O2 for HaCaT = 82.3; ROS positive control for 2 mM H2O2: ROS2 mM H2O2 for 3T3 = 153.9 and ROS2 mM H2O2 for HaCaT = 110.0). However, the fact that in 3T3 higher ROS has been determined in 1 mM H2O2 than in 2 mM H2O2 is due to possible cell death at 2 mM H2O2. This is in line with the low cell viability of the positive controls in the cytoprotection at 2 mM H2O2 for 2.5 h assays in 3T3 compared to the corresponding ones in HaCaT. Because protective activity and ROS values are inversely related (less protective activity of cell line entails higher ROS values).
In Figure 5A, ROS assay in 3T3 for ATM and COM, a different evolution of ROS in relation to the increase in the concentration of each of the extracts is observed by both conditions of oxidative stress (1 and 2 mM H2O2). In all the tested conditions, the ROS value is always higher in ATM with respect to the corresponding COM except for 0.1 mg/mL COM at 2 mM H2O2. In the ATM extract, no clear trend is observed. In the case of COM, an increase in ROS to 0.01 and 0.1 mg/mL extract at 2 mM H2O2 stands out, possibly due to the increment of oxidative stress. Finally, at 1 mg/mL COM for both concentrations of H2O2 tested, the cytotoxic effects of the COM prevail, as observed in the cytotoxicity assay, producing decreases in the increase of ROS due to cell death itself.
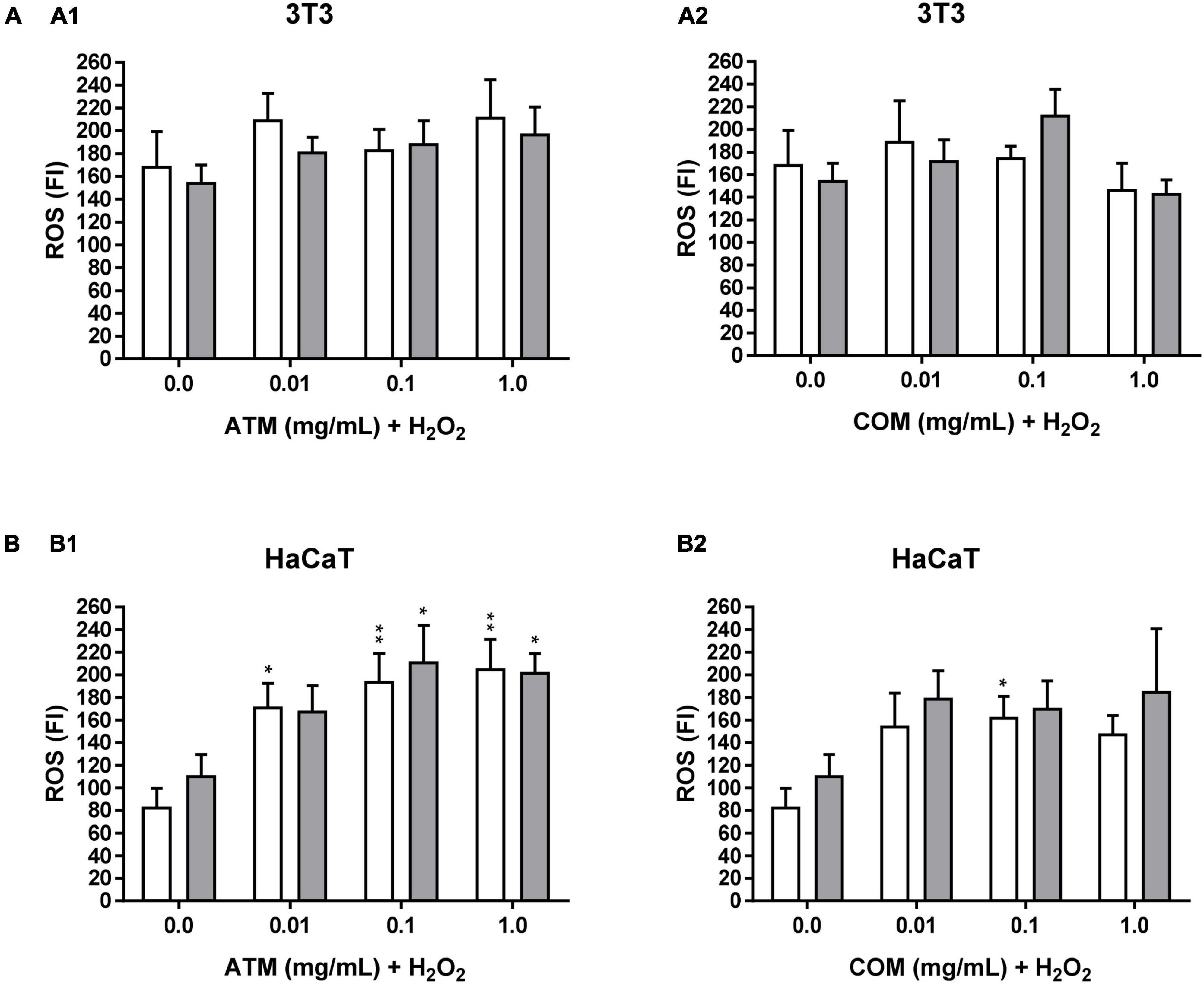
Figure 5. Intracellular ROS induced by 1 and 2 mM H2O2 for 2 h treatment with ATM (1) and COM (2) in 3T3 (A) and HaCaT (B) cells H2O2 cell viability was used as a positive control. White columns correspond to 1 mM H2O2, and gray columns correspond to 2 mM H2O2. Results are expressed as mean ± standard error of n = 3. A two-way analysis of variance (ANOVA) and a Bonferroni post hoc assay have been performed. Statistical differences were considered as follows: *p ≤ 0.05 and **p ≤ 0.01 comparison with the corresponding positive control.
In Figure 5B, ROS assay in HaCaT for ATM and COM, the same trend is determined for both H2O2 conditions applied in each extract assay. Where ROS increases with respect to the corresponding positive controls. Causing the majority of ROS increases to be positive (Table 5). A clear trend has been observed that increasing the ATM concentration carries an increase in ROS value. While in the case of COM, there is a decline in ROS from 0.1 mg/mL extract in both oxidative stress conditions assayed. This coincides is the case in the corresponding cytotoxicity assays in Figure 1B (cytotoxicity of both extracts in HaCaT).
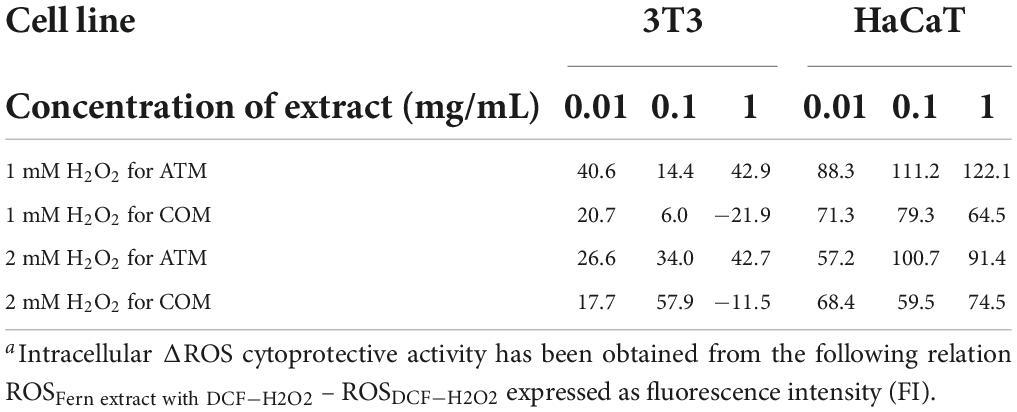
Table 5. Intracellular ΔROSa induced by 1 and 2 mM H2O2 for 2 h with respect to 0 h at different concentrations of ATM and COM in 3T3 and HaCaT.
Discussion
Although ferns have been poorly studied from a phytochemical and nutritional approach, fronds are the main part of the ferns used to make medicinal preparations by the Malayalis in Kolli hills, India (9). The election of the extraction solvent is a relevant aspect in the phytochemical determination of plant drugs. Selectivity (based on polarity target phytochemical), safety, neutral, and easy to separate from the rest of no target phytochemicals, low viscosity, low boiling temperature, and economical are the criteria for selecting the extraction solvent (47). Currently, most plant matrices are obtained from organic solvents compared to other solvents such as green solvents (environmentally friendly). For the in vitro studies that we have carried out on A. trichomanes and C. officinarum extracts, we have used the methanolic extract of their fronds while the correspondence hexane extract was discarded due to the lower presence of total polyphenol content (TPC) than the corresponding methanolic extract in a previous study (34).
In the Russian Far East, there are a minimum of 13 fronds of edible fern species, where P. aquilinum predominates (11). The degree of maturation of the fronds (young fronds, as known fern shots or fiddleheads, or mature fronds) and the way in which the fronds are preserved (by freezing, canning, salting, or drying) are important to determine the potential toxicity of edible ferns. For example, different government dossiers regard the safeguard of public health against P. aquilinum to whose toxins affect human populations and animals. The main toxin that triggers the carcinogenesis of P. aquilinum is ptaquiloside (an illudane, a subclass of sesquiterpenes) (48, 49). Currently, ferns are not incorporated into the diet of European countries. However, the publication of nutritional properties of plant matrices of ferns may be an incentive to incorporate ferns in European diets (14).
We have considered the methanolic extracts of the fronds of A. trichomanes and C. officinarum as potential reservoirs of phytochemicals. Derived that other Aspleniaceae species have been described as reservoirs of phytochemicals. Such as the ferns Asplenium adiantum-nigrum L. (Aspleniaceae) and Asplenium ruta-muraria L. (Aspleniaceae) (50), of which the methanolic extract of the fronds of both ferns is the part with the highest total phenol content (TPC) and total flavonoid content (TFC). And specifically, the content of flavonoids in the fronds is relevant due to one of the functions of the flavonoids in the fronds, which are the neutralization of UV radiation and ROS (51). In the phytocharacterization by HPLC-MS/MS of ATM fronds, a greater quantity of flavonoids, especially flavonols related to kaempferol, was obtained, as Dall’Acqua et al. (52) described. In our phytocharacterization, the main phytochemical isolated from ATM has been hyperoside, another flavonol. We have also isolated kaempferol and kaempferol glycoside (kaempferol-3-glucoside). Resulting that flavonols are the main polyphenolic species determined in ATM, representing 68.8% of the total phytochemicals determined by HPLC-MS/MS. In the case of COM, flavonoids are minor phytochemicals detected by HPLC-MS/MS compared to phenolic acids. These results are equivalent to that obtained by Zivkovic et al. (53). In contrast to Zivkovic et al. (53), we have detected fractions of rutin in the fronds of C. officinarum. In this study, chlorogenic acid is the main phytochemical determined in COM, while the other cinnamic acids such as caffeic acid are only detected in traces. This observation has already been previously reported by Tomou et al. (28). Phytocharacterization by HPLC-MS/MS of both extracts coincides with the nature of the phytochemicals determined by TLC in a previous study (34).
Durdevic et al. (54) determined that the content of phenolic species in the ethylacetate extracts of A. trichomanes and C. officinarum is higher in the frond than in the correspondence rhizome. And the predominance of aglycone phytochemicals (no sugar phytochemicals) than glycone phytochemicals (sugar phytochemicals) in the ethylacetate frond extract. We have determined a higher amount of TPC in the methanolic extract of the fronds of C. officinarum than in the methanolic extract of the fronds of A. trichomanes, as described by Durdevic et al. (54).
Currently, there is a great discrepancy in the cytotoxicity of ferns, derived from the fact that they are a widely diversified taxon. However, in the cytotoxic determination of methanolic extracts, different species of ferns have concluded greater cytotoxicity in the frond than the corresponding rhizome (55). For this reason, it is important that cytotoxicity assays have been performed in a wide range of concentrations. The most common assays to determine cell viability are the reduction in the tetrazole salt or MTT, the uptake of the Neutral Red dye (NRU), or the release of lactate dehydrogenase among others (42). The measurements of most of these techniques are end point and present advantages and disadvantages. In this sense, MTT is usually the method of choice in different cytotoxic studies (38), because it has proven to be valid with different cell lines and is relatively straightforward and useful when conditions are optimized. Moreover, the MTT assay has been used to characterize the cytotoxic profile of different plant extracts including ferns (34). Actually, MTT is regarded as a gold standard of initial cytotoxicity assays as it is highly sensitive and a high-throughput screening assay together with its low economic cost (56). Nevertheless, the NRU test failed to be sensitive in our case. We are also aware that in our research there may be potential interferences of the extracts in the assays by colorimetric methods. It is described that some phytochemicals can interact with MTT producing false-positive cell viabilities (57). For this reason, the absence of interference has been verified by the MTT assay for the in vitro concentrations of the extracts tested. Petkov et al. 2021 (58) analyzed the cytotoxicity of methanolic fronds extracts of three Aspleniaceae ferns by MTT, of which two were A. trichomanes and C. officinarum. The comparison of our cytotoxicity results with the results of Petkov et al. 2021 (58) is relevant due to the same methodology for obtaining the frond extract (frond methanolic extract) and for determination of cell viability (MTT assay) for A. trichomanes and C. officinarum. In the initial toxicity studies of the pharmaceutical industry, assays with a high sensitivity against cytotoxicity with reasonable cost are used, in which various hepatic cell lines are generally used, such as HepG2 (59). The absence of cytotoxicity of the extracts in HepG2 confirms the safety of these extracts.
A diet rich in antioxidants and polyphenols contributes to reducing the risk of diseases resulting from oxidative damage. However, the employment of synthetic antioxidants, as in the case of butylated hydroquinone, in recent years, has been decreased for safety reasons (51). For this reason, investigations of new plant matrices with a high content of antioxidants have increased (60). Despite the low number of studies on ferns, the methanolic extract of their fronds is considered plant parts with a high amount of antioxidant phytochemicals (61, 62). Currently, the extracts of Polypodiaceae ferns such as Polypodium leucotomos (aqueous extract) and Polypodium vulgare L. (methanolic extract) have been reported as antioxidant and cytoprotective agents in vitro (63, 64). However, few ferns of the Aspleniaceae family, despite being the main ferns in Europe, have been studied as plant matrices for the contribution of antioxidant phytochemicals (14). For example, in the methanolic extract of A. adiantum-nigrum and C. officinarum (both species are Aspleniaceae family), mangiferins and mangiferin-related phytochemicals have also been isolated among other polyphenolic phytochemicals (50, 53). This fact demonstrates the potential of the Aspleniaceae as a reservoir of polyphenolic phytochemicals.
Conclusion
The methanolic extracts of the fronds of the two main species of ferns from the Prades mountains, A. trichomanes and C. officinarum, present interesting phytochemicals of different nature without being cytotoxic in the mouse fibroblast 3T3, human keratinocyte HaCaT, cervical human cancer HeLa, liver human cancer HepG2, breast human cancer MCF-7, and lung human cancer A549 cell lines at the assayed concentrations. This fact provides new evidence for considering certain ferns of the Aspleniaceae family as plant matrices for the extraction of phytochemicals of pharmaceutical or nutritional interest.
Data availability statement
The raw data supporting the conclusions of this article will be made available by the authors, without undue reservation.
Author contributions
MM and VL: conceptualization, writing-review and editing, and supervision. AF, MM, and FM: methodology. AF, FM, GC, and MM: analysis. AF, MM, and VL: investigation. MM, MV, and VL: resources. AF: writing—original draft preparation. MM and MV: funding acquisition. All authors have read and agreed to the published version of the manuscript.
Funding
This work was financially supported by project 307,629 of Fundació Bosch & Gimpera - Universitat de Barcelona.
Acknowledgments
We acknowledge the technical support of Universitat de Barcelona and Universidad San Jorge.
Conflict of interest
The authors declare that the research was conducted in the absence of any commercial or financial relationships that could be construed as a potential conflict of interest.
Publisher’s note
All claims expressed in this article are solely those of the authors and do not necessarily represent those of their affiliated organizations, or those of the publisher, the editors and the reviewers. Any product that may be evaluated in this article, or claim that may be made by its manufacturer, is not guaranteed or endorsed by the publisher.
Supplementary material
The Supplementary Material for this article can be found online at: https://www.frontiersin.org/articles/10.3389/fnut.2022.994215/full#supplementary-material
Glossary
3T3 = NIH 3T3 | NIH 3T3 mouse fibroblast cell line |
A549 | Human Caucasian lung carcinoma |
ATM | Methanolic extract of Asplenium trichomanes L. fronds |
COM | Methanolic extract of Ceterach officinarum L. fronds |
CO2 | Carbon dioxide |
CPZ | Chlorpromazine hydrochloride |
CV | Cell Viability |
E | Ultraviolet dose |
DCF | 2,7-dichlorodihydrofluorescein diacetate |
DMEM | Dulbecco’s Modified Eagle’s Medium |
DMSO | Dimethyl sulfoxide |
ECACC | European Collection of Authenticated Cell Cultures |
EDTA | Ethylenediaminetetraacetic acid |
FBS | Fetal Bovine Serum |
FI | Fluorescence Intensity |
HaCaT | Spontaneously immortalized human keratinocyte cell line |
HeLa | Human cervix epitheloid carcinoma |
HepG2 | Human Caucasian hepatocyte carcinoma |
HPLC-MS/MS | High performance liquid chromatography-tandem mass spectrometry |
H2O2 | Hydrogen peroxide |
MCF-7 | Human Caucasian breast adenocarcinoma |
MTT | 2,5-diphenyl-3-(4,5-dimethyl-2-thiazolyl) tetrazolium bromide or thiazolyl blue tetrazolium bromide |
NR | Neutral Red |
NRU | Neutral Red Uptake or uptake of the Neutral Red dye |
OECD | Organisation for Economic Cooperation and Development |
PBS | Phosphate Buffered Saline |
PLE | Aqueous extract of Polypodium leucotomos fronds |
ROS | Reactive Oxygen Species |
TLC | Thin Layer Chromatography |
TFC | Total Flavonoids Content |
TPC | Total Phenol/Polyphenol Content |
UV | Ultraviolet |
UVA | Ultraviolet A |
References
1. Chamberlain JL, Darr D, Meinhold K. Rediscovering the contributions of forests and trees to transition global food systems. Forests. (2020) 11:21. doi: 10.3390/f11101098
2. Jankovsky M, García-Jácome SP, Dvorák J, Nyarko I, Hájek M. Innovations in forest bioeconomy: A bibliometric analysis. Forests. (2021) 12:17. doi: 10.3390/f12101392
4. Nagalingum NS. Seedless land plants, evolution and diversification of. In: RM Kliman editor. Encyclopedia of Evolutionary Biology (Sydney, NSW: Elsevier) (2016). doi: 10.1016/B978-0-12-800049-6.00255-9
5. Baskaran XR, Geo Vigila AV, Zhang SZ, Feng SX, Liao WB. A review of the use of pteridophytes for treating human ailments. J Zhejiang Univ Sci B. (2018) 19:1–35. doi: 10.1631/jzus.B1600344
6. Cao H, Chai TT, Wang X, Morais-Braga MFB, Yang JH, Wong FC, et al. Phytochemicals from fern species: Potential for medicine applications. Phytochem Rev. (2017) 6:379–440. doi: 10.1007/s11101-016-9488-7
7. Muhammad M, Ismail ZS, Schneider H, Hawkins JA. Medicinal use of ferns: An ethnobotanical review. Sains Malaysiana. (2020) 49:1003–14. doi: 10.17576/jsm-2020-4905-05
8. Ojha R, Prasad Devkota H. Edible and medicinal pteridophytes of Nepal: A review. Ethnobot Res Appl. (2021) 22:16. doi: 10.32859/era.22.16.1-16
9. Sureshkumar J, Silambarasan R, Bharati KA, Krupa J, Amalraj S, Ayyanar M. A review on ethnomedicinally important pteridophytes of India. J Ethnopharmacol. (2018) 219:269–87. doi: 10.1016/j.jep.2018.03.024
10. Wang YL, Gao SS, He XY, Li Y, Zhang Y, Chen W. Response of total phenols, flavonoids, minerals, and amino acids of four edible fern species to four shading treatments. PeerJ. (2020) 8:18. doi: 10.7717/peerj.8354
11. Nekrasov EV, Svetashev VI. Edible far eastern ferns as a dietary source of long-chain polyunsaturated fatty acids. Foods. (2021) 10:16. doi: 10.3390/foods10061220
12. Tyler T, Herbertsson L, Olofsson J, Olsson PA. Ecological indicator and traits values for Swedish vascular plants. Ecol Indic. (2021) 120:13. doi: 10.1016/j.ecolind.2020.106923
13. Silva VL, Mehltreter K, Schmitt JL. Ferns as potential ecological indicators of edge effects in two types of Mexican forests. Ecol Indic. (2018) 93:669–76. doi: 10.1016/j.ecolind.2018.05.029
14. Langhansova L, Pumprova K, Haisel D, Ekrt L, Pavicic A, Zajickova M, et al. European ferns as rich sources of antioxidants in the human diet. Food Chem. (2021) 356:129637. doi: 10.1016/j.foodchem.2021.129637
15. Bystriakova N, Peregrym M, Dragicevic S. Effect of environment on distributions of rock ferns in the Mediterranean climate: The case of the genus Asplenium in Montenegro. Flora. (2015) 215:84–91. doi: 10.1016/j.flora.2015.07.003
16. Fiorin E, Saez L, Malgosa A. Ferns as healing plants in medieval Mallorca, Spain? Evidence from human dental calculus. Int J Osteoarchaeol. (2019) 29:82–90. doi: 10.1002/oa.2718
17. Li CX, Yang Q. Notes on the divergence time of the fern genus Asplenium from fossil and molecular evidence. Cretac Res. (2013) 45:352–5. doi: 10.1016/j.cretres.2013.06.005
18. Rizzo F. The distribution of Pteridophytes (ferns and lycophytes) in Sicily. Biodivers J. (2021) 12:91–120. doi: 10.31396/Biodiv.Jour.2021.12.1.91.120
19. Ramos-Gutiérrez I, Lima H, Pajarón S, Romero-Zarco C, Sáez L, Pataro L, et al. Atlas of the vascular flora of the Iberian Peninsula biodiversity hotspot (AFLIBER). Glob Ecol Biogeogr. (2021) 30:1951–7.
20. Font Castell X, de Càceres M, Quadrada R, Moreno J, Martí D. Banco de datos de biodiversidad de Cataluña. Barcelona: Generalidad de Cataluña (2015).
21. The World Flora Online. Asplenium trichomanes L. World Flora Online (WFO) (2022). Available online at: http://www.worldfloraonline.org/taxon/wfo-0001109364
22. The World Flora Online. Ceterach officinarum Willd. World Flora Online (WFO) (2022). Available online at: http://www.worldfloraonline.org/taxon/wfo-0001114265
23. Vasco A, Moran RC, Ambrose BA. The evolution, morphology, and development of fern leaves. Front Plant Sci. (2013) 4:16. doi: 10.3389/fpls.2013.00345
24. Bolòs O, Vigo J. Flora dels països catalans I. Polipodiàcies: Polypodium vulgare L., Barcino editor. Barcelona: Editorial Barcino (1984). p. 188–90.
25. Singh S, Kharkwal K, Kant R, Sinha BK, Ambrish K. Studies on ethnobotanical aspects of pteridophytes in great himalayan national park, kullu, himachal pradesh. Phytotaxonomy. (2017) 17:43–9.
26. Pekgöz AK, Cinbilgel I. Phytochemical contents and antioxidant activities of fern, Asplenium ceterach L. In different altitudes. Bangladesh J Bot. (2019) 48:315–20.
27. Imperato F. Flavonol Glycosides from Ferns of the Genera Asplenium and Cheilanthes. Biochem Syst Ecol. (1989) 17:161–6. doi: 10.1016/0305-1978(89)90074-4
28. Tomou EM, Skaltsa H. Phytochemical investigation of the fern Asplenium ceterach (Aspleniaceae). Nat Prod Commun. (2018) 13:849–50.
29. Bonet MÀ, Agelet A, Vallès J, Villar Pérez L. Contribution à la connaissance ethnobotanique des ptéridophytes dans les Pyrénées. Bocconea. (2001) 13:605–12.
30. Rigat M, Vallès J, Iglésias J, Garnatje T. Traditional and alternative natural therapeutic products used in the treatment of respiratory tract infectious diseases in the eastern Catalan Pyrenees (Iberian Peninsula). J Ethnopharmacol. (2013) 148:411–22. doi: 10.1016/j.jep.2013.04.022
31. Pappalardo G, Lusk JL. The role of beliefs in purchasing process of functional foods. Food Qual Prefer. (2016) 53:151–8. doi: 10.1016/j.foodqual.2016.06.009
32. Parada M, Carrió E, Vallès J. Ethnobotany of food plants in the Alt Empordà region (Catalonia, Iberian Peninsula). J Appl Bot Food Qual. (2011) 84:11–25.
33. Batet D, Cartanyà J, Castells R, Piñas I, Salat X. Entrevista n°13. Etnobotànica a les Muntanyes de Prades, Centre d’Història Natural de la Conca de Barberà editor. Montblanc: Centre d’Història Natural de la Conca de Barberà (2011).
34. Farràs A, Cásedas G, Les F, Terrado EM, Mitjans M, López V. Evaluation of anti-tyrosinase and antioxidant properties of four fern species for potential cosmetic applications. Forests (2019) 10:14. doi: 10.3390/f10020179
35. Mustafa AM, Angeloni S, Abouelenein D, Acquaticci L, Xiao JB, Sagratini G, et al. A new HPLC-MS/MS method for the simultaneous determination of 36 polyphenols in blueberry, strawberry and their commercial products and determination of antioxidant activity. Food Chem. (2022) 367:11. doi: 10.1016/j.foodchem.2021.130743
36. Borenfreund E, Puerner JA. Toxicity determined in vitro by morphological alterations and neutral red absorption. Toxicol Lett. (1985) 24:119–24. doi: 10.1016/0378-4274(85)90046-3
37. Riddell RJ, Clothier RH, Balls M. An evaluation of 3 in vitro cytotoxicity assays. Food Chem Toxicol. (1986) 24:469–71. doi: 10.1016/0278-6915(86)90095-5
38. Weyermann J, Lochmann D, Zimmer A. A practical note on the use of cytotoxicity assays. Int J Pharm. (2005) 288:369–76. doi: 10.1016/j.ijpharm.2004.09.018
39. Zanette C, Pelin M, Crosera M, Adami G, Bovenzi M, Larese FF, et al. Silver nanoparticles exert a long-lasting antiproliferative effect on human keratinocyte HaCaT cell line. Toxicol Vitro. (2011) 25:1053–60. doi: 10.1016/j.tiv.2011.04.005
40. Mosmann T. Rapid colorimetric assay for cellular growth and survival – application to proliferation and cyto-toxicity assays. J Immunol Methods. (1983) 65:55–63. doi: 10.1016/0022-1759(83)90303-4
41. Präbst K, Engelhardt H, Ringgeler S, Hübner H. Basic colorimetric proliferation assays: MTT, WST, and resazurin. In: Gilbert , DF O Friedrich editor. Cell viability assays. Methods and protocols. New York, NY: Springer (2017). doi: 10.1007/978-1-4939-6960-9_1
42. Fotakis G, Timbrell JA. In vitro cytotoxicity assays: Comparison of LDH, neutral red, MTT and protein assay in hepatoma cell lines following exposure to cadmium chloride. Toxicol Lett. (2006) 160:171-177. doi: 10.1016/j.toxlet.2005.07.001
43. Cásedas G, Les F, Choya-Foces C, Hugo M, López V. The metabolite urolithin-A ameliorates oxidative stress in neuro-2a cells, becoming a potential neuroprotective agent. Antioxidants. (2020) 9:16. doi: 10.3390/antiox9020177
44. Organization for Economic Cooperation and Development [OECD]. OECD test No. 432: In vitro 3T3 NRU phototoxicity test. In: OECD guidelines for the testing of chemicals, section 4. Paris: Organization for Economic Cooperation and Development (OECD) (2019). doi: 10.1787/9789264071162-en
45. Martínez V, Galbiati V, Corsini E, Martin-Venegas R, Vinardell MP, Mitjans M. Establishment of an in vitro photoassay using THP-1 cells and IL-8 to discriminate photoirritants from photoallergens. Toxicol Vitro. (2013) 27:1920-1927. doi: 10.1016/j.tiv.2013.06.013
46. Ferreira I, Silva A, Martins JD, Neves BM, Cruz MT. Nature and kinetics of redox imbalance triggered by respiratory and skin chemical sensitizers on the human monocytic cell line THP-1. Redox Biol. (2018) 16:75-86. doi: 10.1016/j.redox.2018.02.002
47. Hikmawanti NPE, Ramadon D, Jantan I, Mun’im A. Natural deep eutectic solvents (NADES): Phytochemical extraction performance enhancer for pharmaceutical and nutraceutical product development. Plants Basel. (2021) 10:2091. doi: 10.3390/plants10102091
48. da Costa RMG, Bastos MMSM, Oliveira PA, Lopes C. Bracken-associated human and animal health hazards: Chemical, biological and pathological evidence. J Hazard Mater. (2012) 203:1-12. doi: 10.1016/j.jhazmat.2011.12.046
49. O’Connor PJ, Alonso-Amelot ME, Roberts SA, Povey AC. The role of bracken fern illudanes in bracken fern-induced toxicities. Mutat Res Rev Mutat Res. (2019) 782:39. doi: 10.1016/j.mrrev.2019.05.001
50. Zivkovic S, Milutinovic M, Maksimovi V, Ciric A, Ivanov M, Bozunovic J, Banjanac T, Misic D. Antioxidant and antimicrobial activity of two Asplenium species. S Afr J Bot. (2020) 132:180-187. doi: 10.1016/j.sajb.2020.03.034
51. Lai HY, Lim YY, Tan SP. Antioxidative, tyrosinase inhibiting and antibacterial activities of leaf extracts from medicinal ferns. Biosci Biotechnol Biochem. (2009) 73:1362–6. doi: 10.1271/bbb.90018
52. Dall’Acqua S, Tomè F, Vitalini S, Agradi E, Innocenti G. In vitro estrogenic activity of Asplenium trichomanes L. extracts and isolated compounds. J Ethnopharmacol. (2009) 122:424–9. doi: 10.1016/j.jep.2009.02.012
53. Zivkovic S, Skoric M, Siler B, Dmitrovic S, Filipovic B, Nikolic T, et al. Phytochemical characterization and antioxidant potential of rustyback fern (Asplenium ceterach L.). Lekovite Sirovine. (2017) 37:15–20. doi: 10.5937/leksir1737015Z
54. Durdevic L, Mitrovic M, Pavlovic P, Bojovic S, Jaric S, Oberan L, et al. Total phenolics and phenolic acids content in leaves, rhizomes and rhizosphere soil under Ceterach officinarum D.C., Asplenium trichomanes L. and A. adiantum nigrum L. in the gorge of sicevo (SERBIA). Ekologia. (2007) 26:164–73.
55. Valizadeh H, Sonboli A, Mahmoodi Kordi F, Babak Bahadori M. Cytotoxicity, antioxidant activity and phenolic content of eight fern species from North of Iran. Pharm Sci. (2015) 21:18–24. doi: 10.15171/PS.2015.12
56. van Tonder A, Joubert AM, Cromarty AD. Limitations of the 3-(4,5-dimethylthiazol-2-yl)-2,5-diphenyl-2H-tetrazolium bromide (MTT) assay when compared to three commonly used cell enumeration assays. BMC Res Notes. (2015) 8:47. doi: 10.1186/s13104-015-1000-8
57. Karakas D, Ari F, Ulukaya E. The MTT viability assay yields strikingly false-positive viabilities although the cells are killed by some plant extracts. Turk J Biol. (2017) 41:919–25. doi: 10.3906/biy-1703-104
58. Petkov V, Batsalova T, Stoyanov P, Mladenova T, Kolchakova D, Argirova M, et al. Selective anticancer properties, proapoptotic and antibacterial potential of three Asplenium species. Plants Basel. (2021) 10:14. doi: 10.3390/plants10061053
59. Richter LHJ, Beck A, Flockerzi V, Maurer HH, Meyer MR. Cytotoxicity of new psychoactive substances and other drugs of abuse studied in human HepG2 cells using an adopted high content screening assay. Toxicol Lett. (2019) 301:79–89. doi: 10.1016/j.toxlet.2018.11.007
60. Brewer MS. Natural antioxidants: Sources, compounds, mechanisms of action, and potential applications. Compr Rev Food Sci Food Saf. (2011) 10:221–47. doi: 10.1111/j.1541-4337.2011.00156.x
61. Ding ZT, Fang YS, Tai ZG, Yang MH, Xu YQ, Li F, et al. Phenolic content and radical scavenging capacity of 31 species of ferns. Fitoterapia. (2008) 79:581–3. doi: 10.1016/j.fitote.2008.01.011
62. Gupta SK, Mitali G, Rajib B, Saha BK, Das AP, Palash M. Evaluation of in vitro antioxidant activity of methanolic extracts of some ferns from Mawsynram of Meghalaya, India. Int J Curr Sci. (2014) 12:87–97.
63. Berman B, Ellis C, Elmets C. Polypodium leucotomos – An overview of basic investigative findings. J Drugs Dermatol. (2016) 15:224–8.
Keywords: bioeconomy, cytoprotection, cytotoxicty, ethnopharmacology, ferns, functional food, polyphenolic phytochemicals
Citation: Farràs A, Mitjans M, Maggi F, Caprioli G, Vinardell MP and López V (2022) Exploring wild Aspleniaceae ferns as safety sources of polyphenols: The case of Asplenium trichomanes L. and Ceterach officinarum Willd.. Front. Nutr. 9:994215. doi: 10.3389/fnut.2022.994215
Received: 14 July 2022; Accepted: 04 August 2022;
Published: 12 September 2022.
Edited by:
Márcio Carocho, Centro de Investigação de Montanha (CIMO), PortugalReviewed by:
Gokhan Zengin, Selcuk University, TurkeyUroš M. Gašić, University of Belgrade, Serbia
Harald Schneider, Xishuangbanna Tropical Botanical Garden (CAS), China
Copyright © 2022 Farràs, Mitjans, Maggi, Caprioli, Vinardell and López. This is an open-access article distributed under the terms of the Creative Commons Attribution License (CC BY). The use, distribution or reproduction in other forums is permitted, provided the original author(s) and the copyright owner(s) are credited and that the original publication in this journal is cited, in accordance with accepted academic practice. No use, distribution or reproduction is permitted which does not comply with these terms.
*Correspondence: Víctor López, ilopez@usj.es