- 1College of Textile and Clothing Engineering, Soochow University, Suzhou, China
- 2Shanghai Jing Rui Yang Industrial Co., Ltd, Shanghai, China
- 3Shanghai Nutri-woods Bio-Technology Co., Ltd, Shanghai, China
- 4Shanghai Pechoin Daily Chemical Co., Ltd, Shanghai, China
- 5Shanghai Institute of Spacecraft Equipment, Shanghai, China
Extensive exposure to nanomaterials causes oxidative stress and inflammation in various organs and leads to an increased risk of adverse health outcomes; therefore, how to prevent the toxic effects are of great concern to human. Alpha-lipoic acid (ALA) has anti-oxidant and anti-inflammatory activities, suggesting it may be effective to prevent nanomaterial-induced toxicity. However, the results obtained in individual studies remained controversial. We aimed to comprehensively evaluate the effects of ALA supplementation on nanomaterial-induced toxicity by performing a meta-analysis. Databases of PubMed, EMBASE, and Cochrane Library were searched up to May 2022. STATA 15.0 software was used for statistical analysis. Twelve studies were included. Meta-analysis of eight in vivo studies showed ALA supplementation could exert significant effects on nanomaterial-induced oxidative stress (by reducing MDA, ROS and increasing GSH, CAT, GPx, and SOD), inflammation (by downregulating NO, IgG, TNF-α, IL-6, and CRP), apoptosis (by activation of pro-apoptotic caspase-3), DNA damage (by a reduction in the tail length) and organ damage (by a decrease in the liver biomarker ALT and increases in brain neuron biomarker AChE and heart biomarker CPK). Pooled analysis of four in vitro studies indicated ALA intervention increased cell viability, decreased ROS levels, inhibited cell apoptosis and chelated metal ions. Subgroup analyses revealed changing the levels of GSH, IL-6, and metal ions were the main protective mechanisms of ALA supplementation because they were not changed by any subgroup factors. In conclusion, ALA supplementation may represent a potential strategy for the prevention of the toxicity induced by nanomaterials.
Introduction
Nanomaterials have been widely utilized in several commercial products, such as electronics, fabrics, drugs, food additive, paint, cosmetics, and sunscreens (1–4). The mass production and consumption of nanomaterials inevitably leads to increased occupational and environmental exposure (5, 6). Nanomaterials can be inhaled, absorbed, ingested, or injected into the body and then transported to various tissues and organs via the bloodstream (7, 8). These studies indicate evaluation of their safety and development of prevention measures are challenges encountered by the scientists.
There have been human, in vitro and in vivo studies to demonstrate the toxic effects of nanomaterials, with the main mechanisms of inflammatory responses and oxidative damages (9–12). Bello et al. reported exposure to nano-enabled products induced moderate upper airway inflammation and stronger systemic inflammation in healthy operators, evidenced by upregulation of interleukin (IL)-1β, tumor necrosis factor (TNF)-α and interferon-γ (13). The levels of pro-inflammatory leukotrienes type B4, E4 and TNF-α in exhaled breath condensate (EBC) as well as the percentages of chronic bronchitis were higher in nanomaterial workshop employees compared with office employees (14). Multiple regression analysis detected a strongly positive association between occupational exposure in the nano-titanium dioxide (TiO2) production facility and EBC level of lipid oxidation marker malonaldehyde (MDA) (15). Nanoparticle (NP) exposure changed the levels of enzymes and molecules involved in oxidative stress [including increased MDA and reduced superoxide dismutase (SOD), glutathione (GSH), glutathione peroxidase (GPx), and catalase (CAT)] (16) and inflammation (TNF-α; IL-6; C-reactive protein, CRP) (17), and cause damages in liver (increased alanine aminotransferase, ALT) (18), kidney (increased creatinine, urea, uric acid) (11), heart (increased creatine phosphokinase, CPK) (17), testes (decreased sperm counts and motility) (19) and brain (decreased AChE activity) (19) in murine models compared with controls. In vitro publications showed cell viability was substantially suppressed and cell apoptosis was significantly increased after nanoparticle exposure, which was associated with elevated reactive oxygen species (ROS) (20–22). These findings suggest attenuation of oxidant stress and inflammation may act as potential strategies for preventing the negative effects of nanomaterials.
Recently, nutraceuticals, food or components isolated from food, has been reported to provide health and medical benefits (23). Alpha-lipoic acid (ALA; also known as thioctic acid) is a commonly used nutraceutical that possesses strong anti-oxidant properties because it is an essential cofactor for enzyme complexes involved in mitochondrial oxidative metabolism (24). ALA is also considered to improve inflammatory conditions in the human body (24, 25). Thus, it is hypothesized that supplementation with ALA may represent a cost-effective and safe tool for the prevention of the toxicity induced by nanomaterials. This hypothesis had been demonstrated by some in vivo and in vitro experiments (17, 21, 26). However, some conflicting results were also identified. Deore et al. did not find significant differences in SOD, GSH, CAT and ROS between ALA + ZnONP- and ZnONP-treated groups (19). Even, Abdelkarem et al. observed that the level of TNF-α was increased in the serum of ZnONP-exposed rats after treatment with ALA (27). Therefore, whether ALA supplementation should be recommended for humans exposed to nanomaterials in the future remains inconclusive.
In the current study, we aimed to perform a comprehensive meta-analysis of all published in vivo and in vitro studies to examine the effects of ALA supplementation on oxidant and inflammatory markers as well as the consequence of cell viability, death, DNA damage and organ damage. Our results may be useful to guide the clinical use of ALA for human exposed to nanomaterials, particularly occupational workers.
Materials and methods
Search strategy
This meta-analysis followed the preferred reporting items for systematic reviews and meta-analysis (PRISMA) 2020 checklist. A systematic search was conducted on electronic databases of PubMed, EMBASE and Cochrane Library up to May 2022 to obtain relevant studies, without language restriction. The search terms included (“nanomaterials” OR “nanoparticle” OR “carbon nanotube” OR “graphene” OR “quantum dot”) AND (“lipoic acid” OR “thioctic acid”). Also, manual checking was done on the reference lists of all relevant studies and previous reviews to identify additional complements.
Inclusion and exclusion criteria
Studies were included according to the participants, interventions, comparisons, outcomes, and study design (PICOS) criteria: (1) participants (P): animals or cells; (2) intervention (I): the experimental group was co-treated with nanomaterials and ALA; (3) comparison (C): the control group was only administered with nanomaterials; (4) outcomes (O): cell viability, cell death (apoptosis rate, necrosis rate, caspase-3 activity), DNA damage (tail length, tail DNA%), oxidative stress (MDA, ROS, GSH, CAT, GPx, SOD), inflammation (NO, nitric oxide; TNF-α, IL-6, CRP; IgG, immunoglobin G), organ damage (ALT, AChE, CPK, body weight, organ weight) and chelation (concentration of metal ions); and (5) study design (S): controlled trials. The exclusion criteria were: (1) duplications; (2) non-original research (i.e., reviews, case reports, abstracts, letter to the editor, conference proceedings and comments); (3) data could not be available or were reported only in one study; and (4) irrelevant topics. Two authors independently screened the literatures and any disagreements were resolved by consultation with a third reviewer.
Data extraction
Data extraction was completed by two reviewers independently, including author, publication date, country, cell or animal type, nanomaterial type, nanomaterial dose, ALA dose, ALA administration route, ALA treatment duration, the number of samples in two groups, sample source for analysis of outcomes and related data in two groups (mean ± standard deviation). The data presented only graphically were extracted by using the Engauge Digitizer digitizing software1. Any disagreements in data extraction were discussed with another author.
Quality assessment
The quality of included in vitro studies was evaluated by the Toxrtool scale (28, 29), with the values of 0 or 1 point allocated for each item. The Toxrtool scores of the study ranged from 0 to 18 points. Studies with a Toxrtool score of ≥ 11 points were considered as high quality. The quality of each in vivo study was evaluated by the SYRCLE risk-of-bias tool based on the following domains: selection, performance, detection, attrition, reporting and other bias (30). Each domain was categorized as ‘low,’ ‘high’ or ‘unclear’ risk of bias if they were labeled as yes, no, unclear to included articles. Quality assessment was performed independently by two reviewers; any disagreements were resolved by a third reviewer.
Statistical analysis
STATA version 15.0 (Stata Corp., College Station, TX, United States) was used for data analysis. Effect size for the meta-analysis was defined as the standardized mean difference (SMD) and 95% confidence interval (CI). Cochrane’s Q-square test and I2 index were used to measure the between-study heterogeneity. A random-effects model was considered to analyze the pooled effect size for the outcomes when significant heterogeneity was present (p < 0.1 and I2 > 50%); otherwise, a fixed-effects model was utilized. Possible sources of heterogeneity were explored using subgroup analyses based on nanomaterials types, ALA dose, ALA route, ALA duration and sample source for variables with five included datasets. Publication bias was examined by Egger’s linear regression test. The trim-and-fill method was applied to adjust the pooled SMD in the presence of publication bias (p < 0.05). Sensitivity analysis with a leave-one-out method was conducted to determine the effect of each study on the overall results.
Results
Search results and study selection
As shown in Figures 1, 2, 265 published articles were initially identified through systematically searching the electronic databases. After removal of 1,607 duplicates, 658 studies were included for the title and abstract screening. Then, 643 studies were excluded because they were reviews (n = 13), case reports (n = 2), and irrelevant topics (n = 628). The full-texts of the remaining 15 studies were downloaded and read to examine their eligibility. Consequently, three studies were further eliminated because of the following reasons: data unavailable (only the mean provided; n = 1) (31); data could not be integrated with other studies (percentage relative controls provided, not specific concentration for outcomes; n = 1) (18); ALA modified in NPs and thus only ALA-NP dose was provided, not ALA dose as other studies (n = 1) (32). Eventually, eight in vivo (11, 17, 19, 26, 27, 33–35) and four in vitro (20, 21, 36, 37) studies were included in our meta-analysis.
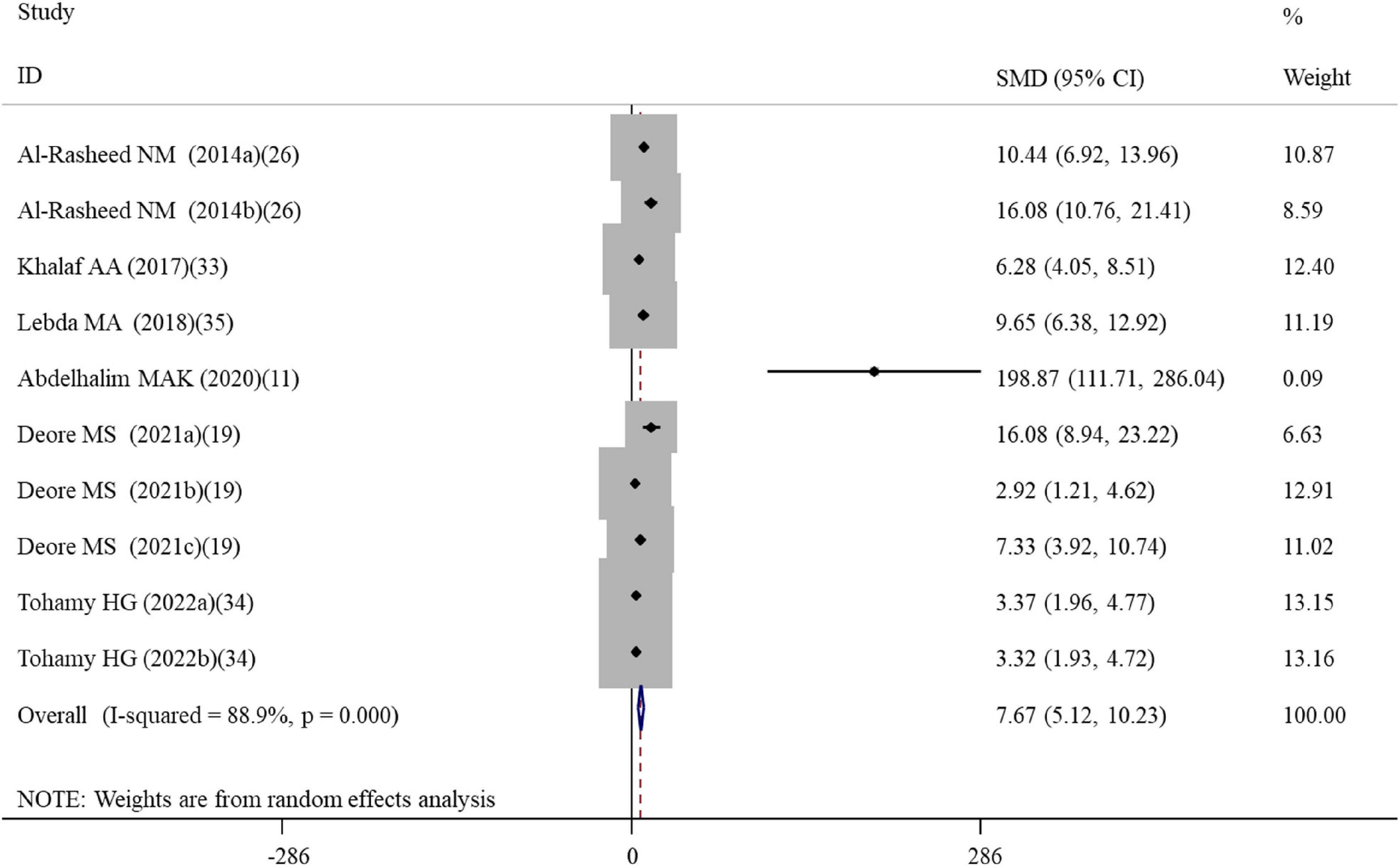
Figure 2. Forest plots assessing the effect of alpha-lipoic acid supplementation on the level of GSH compared with the nanomaterial exposure group. a, b of the study of Al-Rasheed et al. (26) represent treatment with 600 and 1000 mg/kg of ZnONPs; a, b of the study of Tohamy et al. (34) represent the assay of GSH and total GSH; a, b, c of the study of Deore et al. (19) represent the level of GSH in the brain, spleen, and testis tissues. GSH, glutathione; SMD, standardized mean difference; CI, confidence interval.
Study characteristics
Study characteristics of these 12 eligible articles are shown in Table 1. All in vivo studies were performed in the rat model and published from 2013 to 2022; five studies were conducted in Egypt, two in Saudi Arabia and one in the India; four studies investigated the protective roles of ALA for the toxicity induced by zinc oxide nanoparticles (ZnONPs), two for silver nanoparticles (AgNPs), one for copper nanoparticles (CNPs) and gold nanoparticles (GNPs), respectively; the daily doses of ALA were 5, 100, and 200 mg orally or intravenously; the treatment duration ranged from 1 to 8 weeks. In vitro studies were published from 2009 to 2021; three studies were conducted in China and one in Canada; each one study was included to explore the protective roles of ALA for the toxicity induced by ZnONPs, AgNPs, cobalt nanoparticles (CoNPs) and quantum dot, respectively. The exposed cells included normal (primary dorsal root ganglia cells, mouse fibroblast cell line Balb/3T3, human pancreatic ductal cell line CRL-4023, human hepatic stellate cells LX-2, human aortic endothelial cells) and malignant types (rat pheochromocytoma cell PC12, human pancreatic ductal adenocarcinoma cell lines BxPc-3, PANC-1, MIA-PaCa2, and BxGEM). The exposed dose of ALA ranged from 50 to 1000 μM and treatment duration ranged from 6 to 24 h.
Quality assessment
None of the in vivo studies reported sequence generation, allocation concealment, blinding of investigators, random outcome assessment and blinding of outcome assessor; thus, an unclear risk of bias was assigned for them. However, they were at a low risk of bias in terms of baseline characteristics, random housing, incomplete outcome data, selective outcome reporting and others. Therefore, the overall quality of in vivo studies was considered to be relatively low. According to the Toxrtool score, all the in vitro studies also had high quality scores (Supplementary Table S1).
Meta-analysis results
Since multiple ALA doses, treatment durations, tissue samples and cell types were designed for some studies, the number of datasets for meta-analysis was larger than the actual number of included articles. The detailed data that were extracted from in vivo and in vitro studies for each variable are summarized in Supplementary Tables S2, S3, respectively.
Effects of alpha-lipoic acid supplementation on oxidative stress in rats exposed to nanomaterials
A total of four, two, ten, five, three, and five datasets examined the effects of ALA supplementation on the levels of oxidative stress-related indicators MDA, ROS, GSH, CAT, GPx, and SOD, respectively (Supplementary Table S2). The pooled analysis using a random-effects model showed that compared with the nanomaterial-exposed group, treatment with ALA induced significant reductions in the levels of pro-oxidant MDA (SMD = –5.53; 95%CI, –8.39 –2.66; p < 0.001) and ROS (SMD = –2.84; 95%CI, –5.01 –0.66; p = 0.011), while significant increases in the levels of anti-oxidant GSH (SMD = 7.68; 95%CI, 5.12 – 10.23; p < 0.001; Figure 2), CAT (SMD = 6.31; 95%CI, 3.61 – 9.00; p < 0.001), GPx (SMD = 5.63; 95%CI, 1.70 – 9.55; p = 0.005) and SOD (SMD = 4.88; 95%CI, 2.37 – 7.38; p < 0.001) (Table 2). The significant beneficial effects of ALA treatment on oxidative stress-related indicators were still present in most of subgroups (except the levels of CAT and SOD in the brain were not improved by ALA supplementation), especially GSH which was not changed by any subgroup variables (Table 3). Although the heterogeneity was still present, it had been decreased by the nanomaterial type and sample source (Table 3), indicating they may be potential sources of heterogeneity.
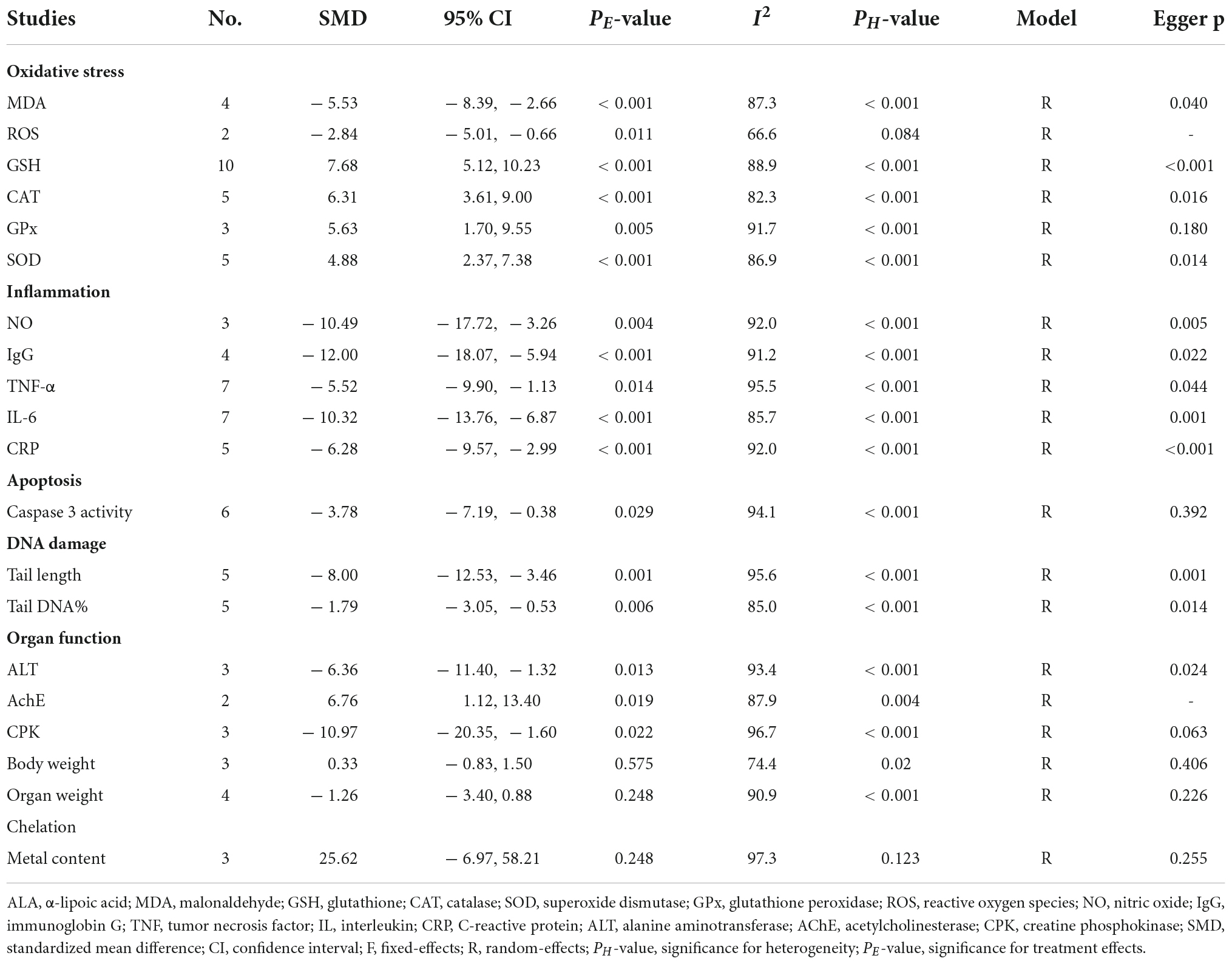
Table 2. Meta-analysis with in vivo studies to assess effects of ALA for nanomaterial-induced toxicity.
Effects of alpha-lipoic acid supplementation on inflammation in rats exposed to nanomaterials
Overall, three, four, seven, seven, and five datasets respectively measured the levels of inflammation factors NO, IgG, TNF-α, IL-6 and CRP in nanomaterial-exposed and ALA treatment groups (Supplementary Table S2). The results of meta-analysis using a random-effects model showed that ALA supplementation significantly decreased the levels of NO (SMD = –10.49; 95%CI, –17.72 –3.26; p = 0.004), IgG (SMD = –12.00; 95%CI, –18.07 –5.94; p < 0.001), TNF-α (SMD = –5.52; 95%CI, –9.90 –1.13; p = 0.014), IL-6 (SMD = –10.32; 95%CI, –13.76 –6.87; p = 0.014; Figure 3) and CRP (SMD = –6.28; 95%CI, –9.57 –2.99; p < 0.001) (Table 2). The inhibiting effect of ALA supplementation on the level of IL-6 was still significant in any subgroups and the heterogeneity was also decreased. The effects of ALA supplementation on the levels of TNF-α seemed to be only significant in the early stage with a low-dose (duration ≤ 2 weeks, p = 0.014) (Table 3).
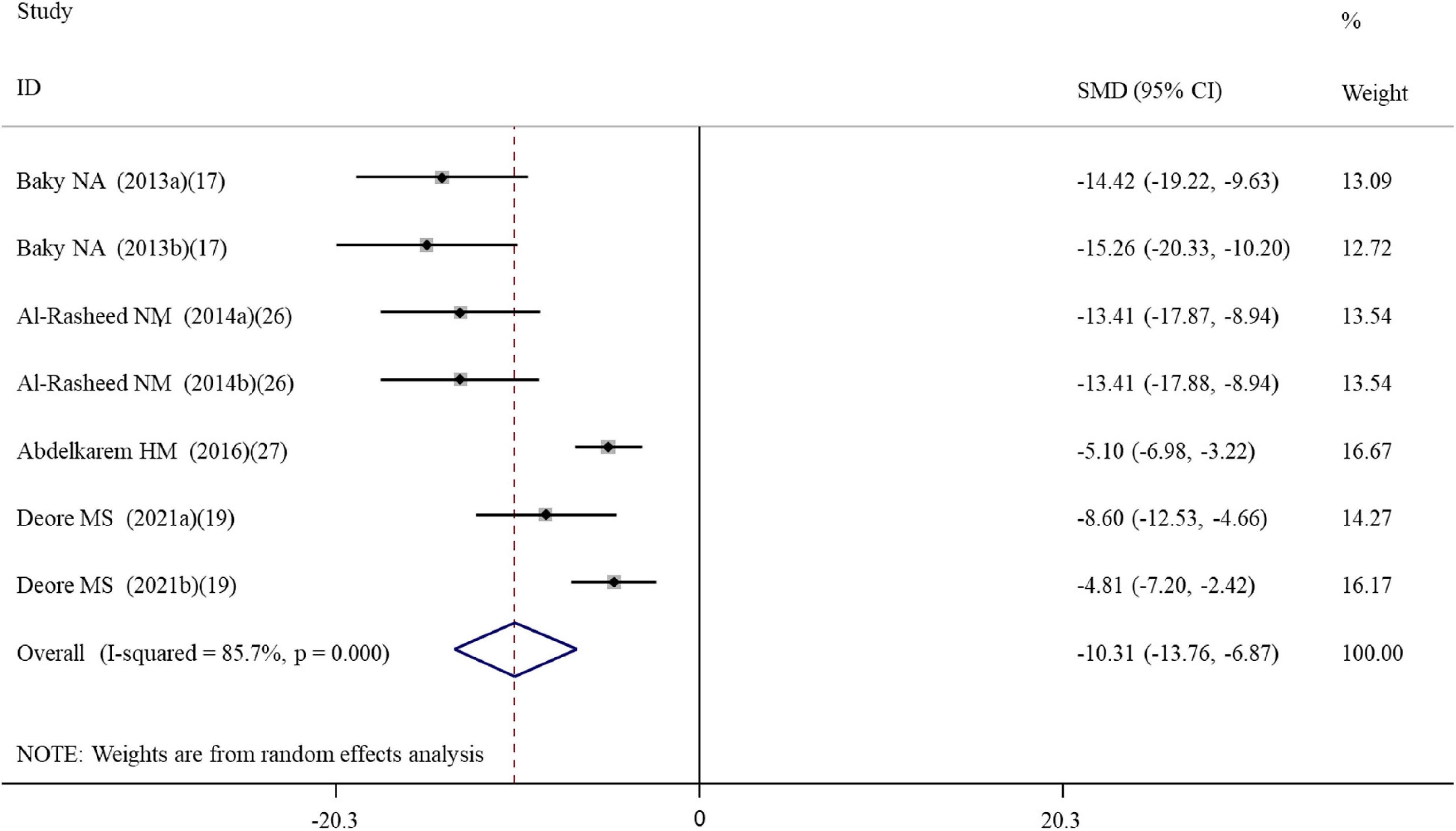
Figure 3. Forest plots assessing the effect of alpha-lipoic acid supplementation on the level of IL-6 compared with the nanomaterial exposure group. a, b of the study of Baky et al. (17) and Al-Rasheed et al. (26) represent treatment with 600 and 1000 mg/kg of ZnONPs; a, b of the study of Deore et al. (19) represent the level of IL-6 in the brain and spleen tissues. IL, interleukin; SMD, standardized mean difference; CI, confidence interval.
Effects of alpha-lipoic acid supplementation on apoptosis in rats exposed to nanomaterials
Six datasets assessed the caspase-3 activity following ALA administration to rats (Supplementary Table S2). Results pooled from the random-effects model showed that compared with the controls, ALA supplementation could remarkably decrease the activity of caspase-3 (SMD = –3.78; 95%CI, –7.19 –0.38; p = 0.029) (Table 2 and Figure 4). Subgroup analysis showed that the protective roles of low-dose and short-duration (p = 0.927) ALA supplementation on the apoptosis of cardiac tissues (p = 0.143) may be limited (Table 3).
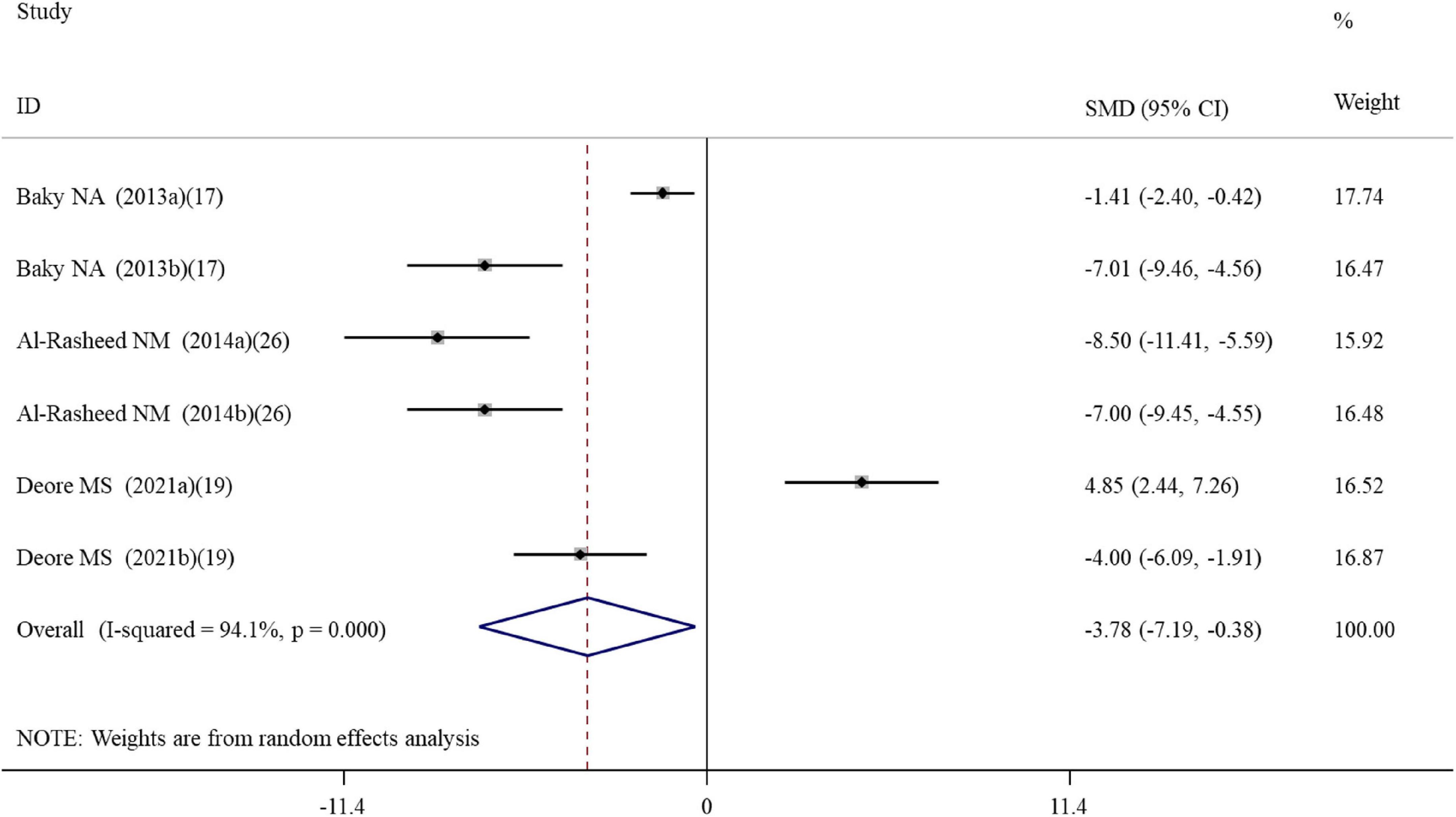
Figure 4. Forest plots assessing the effect of alpha-lipoic acid supplementation on the caspase-3 activity compared with the nanomaterial exposure group. a, b of the study of Al-Rasheed et al. (26) represent treatment with 600 and 1000 mg/kg of ZnONPs; a, b of the study of Deore et al. (19) represent the caspase-3 activity in the brain and spleen tissues. SMD, standardized mean difference; CI, confidence interval.
Effects of alpha-lipoic acid supplementation on DNA damage in rats exposed to nanomaterials
Five datasets (Supplementary Table S2) reported the effect of ALA supplementation on DNA damage which used the tail DNA content and the tail length measured by comet assay as metrics. The combined results revealed that ALA consumption resulted in significant decreases in the tail length (SMD = –8.00; 95%CI, –12.53 –3.46; p < 0.001; Figure 5) and tail DNA% (SMD = –1.79; 95%CI, –3.05 –0.53; p = 0.006) (Table 2). The beneficial effects of ALA treatment on the tail length remained significant after the subgroup analysis based on sample sources (Table 3).
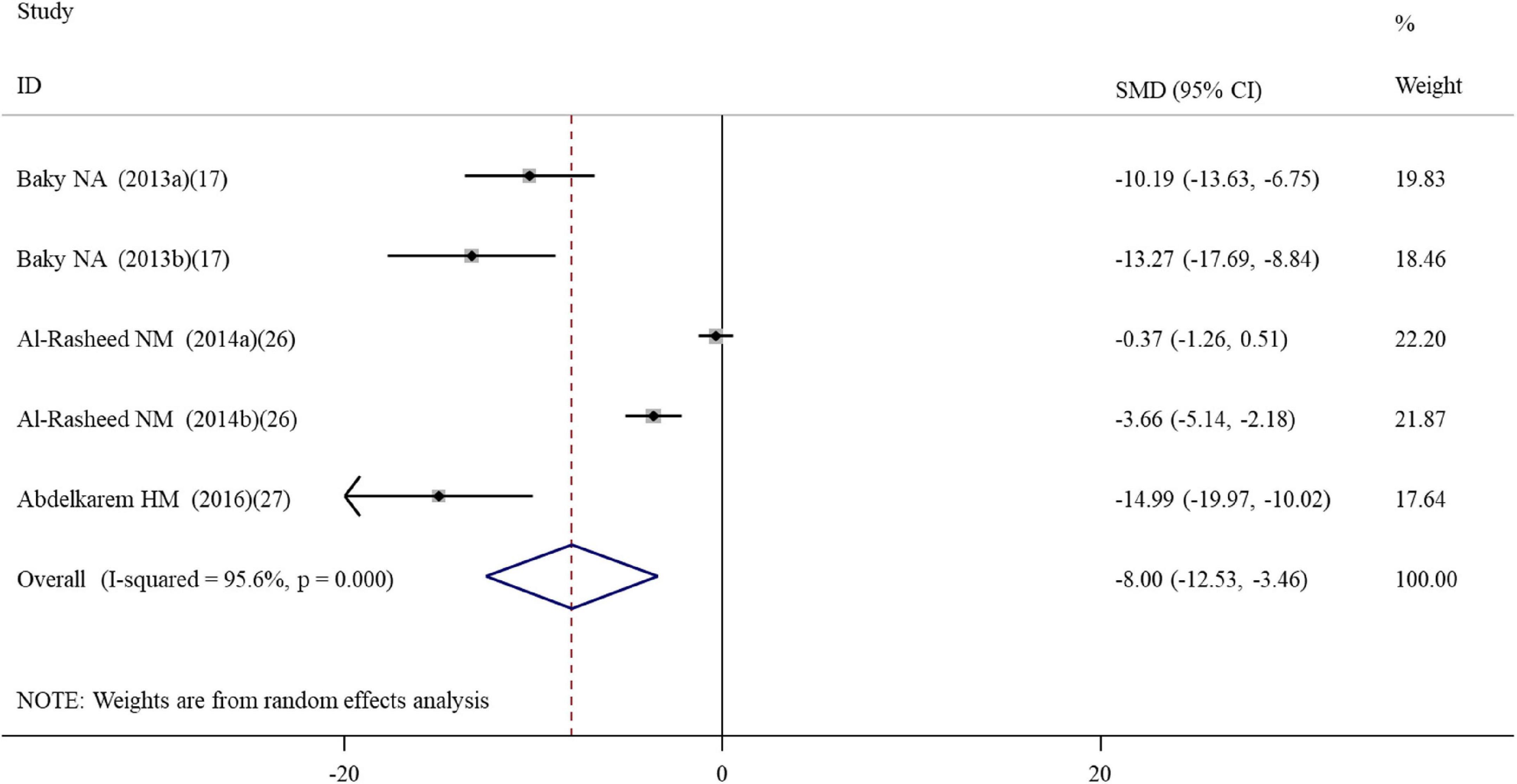
Figure 5. Forest plots assessing the effect of alpha-lipoic acid supplementation on the tail length compared with the nanomaterial exposure group. a, b of the study of Al-Rasheed et al. (26) represent treatment with 600 and 1000 mg/kg of ZnONPs. SMD, standardized mean difference; CI, confidence interval.
Effects of alpha-lipoic acid supplementation on organ function in rats exposed to nanomaterials
Liver function biomarker ALT, brain neuron biomarker AChE and heart function biomarker CPK were analyzed in three, two and three datasets, respectively (Supplementary Table S2). Body weight and organ weight were also recorded in three and four datasets to assess the overall damage (Supplementary Table S2). The pooled results demonstrated that ALA supplementation caused a significant change in the levels of ALT (SMD = –6.36; 95%CI, –11.40 –1.32; p = 0.013), AChE (SMD = 6.76; 95%CI, 1.12 – 13.40; p = 0.019) and CPK (SMD = –10.97; 95%CI, –20.35 –1.60; p = 0.022) (Table 2). Body weight (p = 0.575) and organ weight (p = 0.248) were not significantly changed by ALA supplementation (Table 2).
Effects of alpha-lipoic acid supplementation on metal content in rats exposed to nanomaterials
Metal oxides NP exposure often induced metal ions accumulation in cells or tissues and led to adverse effects. Thus, clearance of metal ions was also an important mechanism to prevent their induced damages. Three datasets detected the concentration of copper, silver and zinc in rats after exposure to CNPs, AgNPs, and ZnONPs as well as the effects of ALA supplementation (Supplementary Table S2). The pooled results showed ALA supplementation did not exhibit an excellent effect of chelating metal ions (SMD = 25.62; 95%CI, –6.97 – 58.21; p = 0.248) (Table 2).
Effects of alpha-lipoic acid supplementation on the viability of cells exposed to nanomaterials
A total of 22 datasets investigated the effects of ALA supplementation on the cell viability (Supplementary Table S3). The pooled results showed that ALA treatment could significantly enhance the viability of cells compared with the nanomaterial exposure group (SMD = 2.06; 95%CI, 1.14 – 2.98; p < 0.001) (Table 4 and Figure 6). Except of quantum dot, subgroup analysis further confirmed this significant effect of ALA treatment on the cell viability (Table 5).
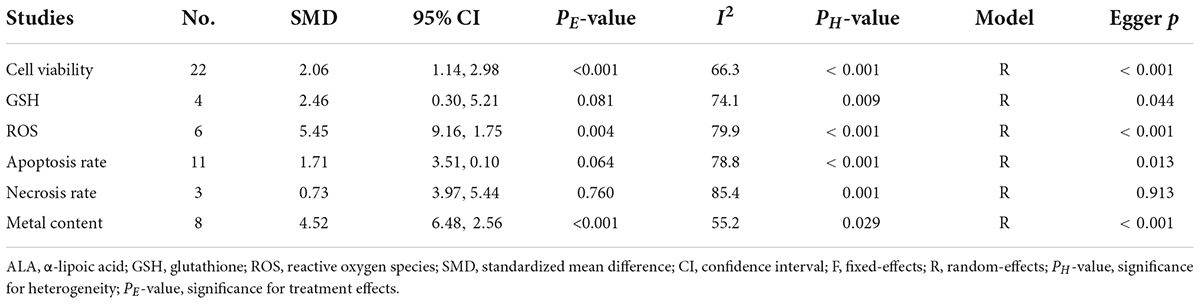
Table 4. Meta-analysis with in vitro studies to assess effects of ALA for nanomaterial-induced toxicity.
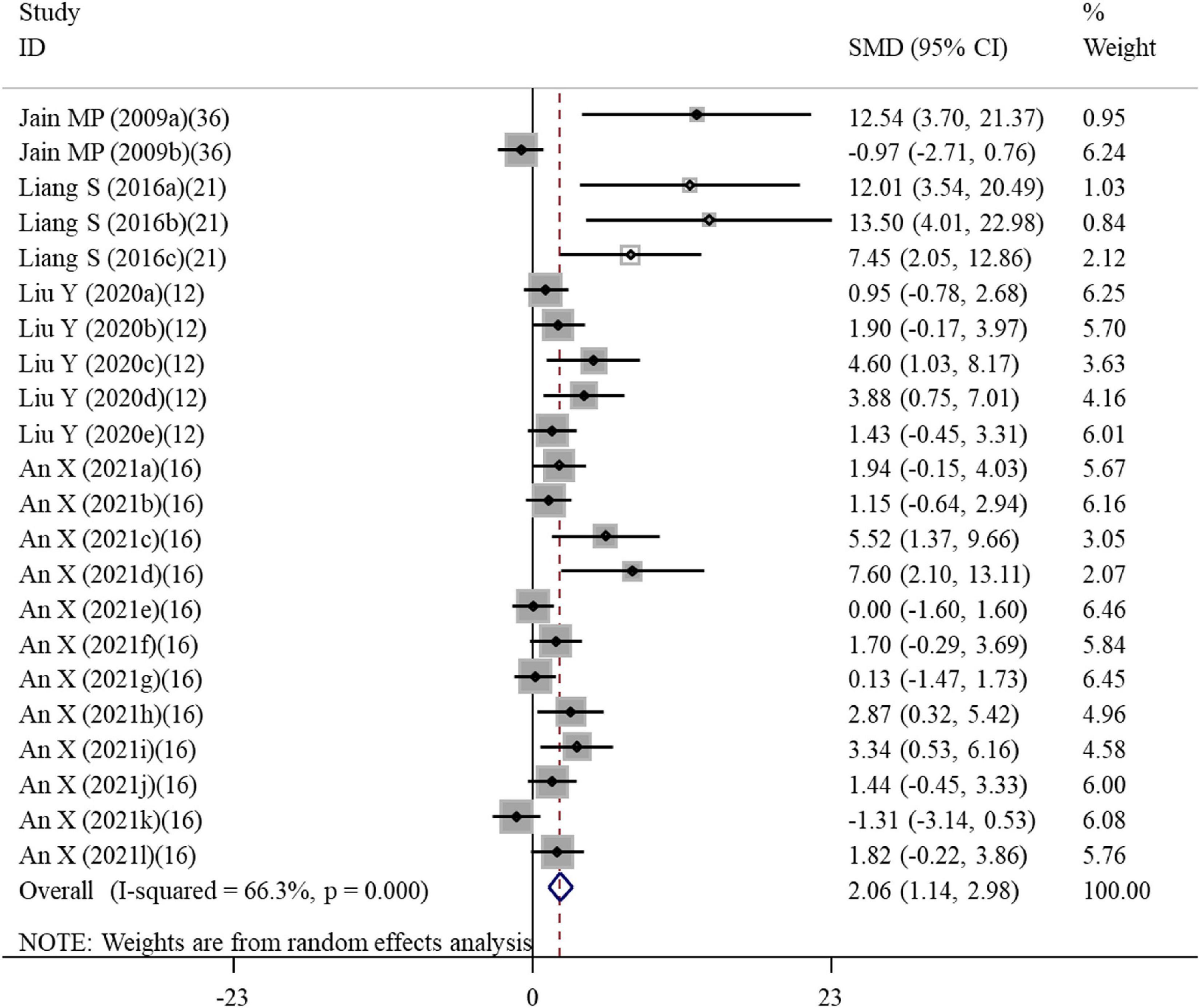
Figure 6. Forest plots assessing the effect of alpha-lipoic acid supplementation on the cell viability compared with the nanomaterial exposure group. a, b of the study of Jain et al. (36) represent the effects on PC12 and DRG cells; a, b, c of the study of Liang et al. (21) represent the effects on HAECs for 6, 12, and 24 h; a, b, c, d, e of the study of Liu et al. (12) represent treatment with 50, 100, 200, 400, and 600 μM alpha-lipoic acid; a, b, c, d, e, f, g, h, I, j, k, l of the study of An et al. (16) represent treatment with 500 and 1000 μM alpha-lipoic acid for CRL-4023, LX-2, BxPc-3, BxGEM, PANC-1, and MIA-PaCa2 cells. SMD, standardized mean difference; CI, confidence interval.
Effects of alpha-lipoic acid supplementation on oxidative stress of cells exposed to nanomaterials
Four and six studies assessed the effects of ALA supplementation on the level of GSH and ROS in cells exposed to nanomaterials, respectively (Supplementary Table S3). The pooled results showed that the level of GSH in cells was only increased by ALA supplementation at a marginal significance (p = 0.081), while the level of ROS was statistically decreased by ALA (SMD = –5.45; 95%CI, –9.16 –1.75; p = 0.004) (Table 4). Subgroup analysis further demonstrated the protective effects of ALA treatment on ROS, particularly for non-malignant cells (Table 5).
Effects of alpha-lipoic acid supplementation on death of cells exposed to nanomaterials
Apoptosis and necrosis rates determined by flow cytometry were recorded in eleven and three datasets, respectively (Supplementary Table S3). As shown in Table 4, the overall SMD of ALA treatment compared with the control was –1.71 (95% CI: –3.51 to 0.1) for the apoptosis rate and 0.73 (95% CI: –3.97 to 5.44) for the necrosis rate, indicative of no statistical difference between these two groups. However, a significant inhibitory effect of ALA treatment on the cell apoptosis was observed in the subgroup with a low dose (≤400 μM; SMD = –4.03; 95%CI, –6.76 –1.29; p = 0.004) under a fixed-effect model (Table 5), implying supplementation with low-dose ALA may be effective to prevent the apoptosis of cells exposed to nanomaterials.
Effects of alpha-lipoic acid supplementation on metal content in cells exposed to nanomaterials
Eight datasets detected the concentration of cadmium, cobalt and silver in cells after exposure to cadmium-quantum dot, CoNPs and AgNPs as well as the effects of ALA supplementation (Supplementary Table S3). The pooled results showed that ALA supplementation significantly cleared the metal ions caused by nanomaterials (SMD = –4.52; 95%CI, –6.48 –2.56; p < 0.001) (Table 4). This chelating effect was still significant in all subgroup analyses (Table 5).
Publication bias and sensitivity analysis
Egger’s test showed no evidence of publication bias for analysis of GPx (p = 0.180), caspase 3 activity (p = 0.392), CPK (p = 0.063), body weight (p = 0.406), organ weight (p = 0.226), and necrosis rate (p = 0.913), while publication bias existed for analysis of MDA (p = 0.04), rat GSH (p < 0.001), CAT (p = 0.016), SOD (p = 0.014), NO (p = 0.005), IgG (p = 0.022), TNF-α (p = 0.044), IL-6 (p = 0.001), CRP (p < 0.001), tail length (p = 0.001), tail DNA% (p = 0.014), ALT (p = 0.024), cell viability (p < 0.001), cell GSH (p = 0.044), cell ROS (p < 0.001), and apoptosis rate (p = 0.013). Therefore, the trim and fill method was used to adjust the pooled SMD for these variables with publication bias. The results showed the SMD for MDA, NO, IgG, TNF-α, IL-6, CRP, tail length, tail DNA%, ALT, ROS and apoptosis was the same as the results before correction. Although SMD was decreased, a statistical difference was still present for rat GSH (SMD = 4.15; 95%CI, 1.37 – 6.92; p = 0.003), CAT (SMD = 4.36; 95%CI, 1.44 – 7.27; p = 0.003), SOD (SMD = 2.80; 95%CI, 0.07 – 5.53; p = 0.044) and cell viability (SMD = 1.04; 95%CI, 0.01 – 2.06; p = 0.047). The level of GSH in cells was still demonstrated not to be significantly changed by ALA after correction (SMD = 0.98; 95%CI, –1.73 – 3.69; p = 0.477). The leave-one-out sensitivity analysis indicated that removing each of the dataset had no significant effect on the pooled effect size (Figure 7).
Discussion
Due to the anti-oxidant and anti-inflammatory effects of ALA, oral supplementation of ALA has been recommended in clinic for the prevention and treatment of several oxidant- and inflammatory-related diseases (i.e., diabetes, metabolic syndrome, cardiovascular disease, rheumatoid arthritis, et al.) and meta-analysis of randomized clinical trials also confirmed its beneficial effects (25, 38–41). However, only in vitro and in vivo studies focused on the roles of ALA supplementation on nanomaterial-induced oxidant and inflammatory injuries currently and controversial results were reported. Therefore, our study, for the first time, attempted to comprehensively evaluate the effects of ALA for cells and animals exposed to nanomaterials by performing a meta-analysis, which may provide supporting evidence for the clinical use in the future. As a result, our meta-analysis of eight in vivo studies showed that ALA administration could exert significant effects on nanomaterial-induced oxidative stress (manifested by reducing MDA, ROS and increasing GSH, CAT, GPx and SOD), inflammation (evidenced by downregulating NO, IgG, TNF-α, IL-6, and CRP), apoptosis (manifested by activation of pro-apoptotic caspase-3), DNA damage (represented by a reduction in the tail length) and organ damage (represented by a decrease in the liver biomarker ALT and increases in brain biomarker AChE and heart biomarker CPK). Pooled analysis of four in vitro studies also indicated ALA intervention increased cell viability, decreased ROS levels and inhibited cell apoptosis. These results confirmed the protective roles of ALA for nanomaterial-induced toxicity by reduction of both inflammatory and oxidative mediators, which were in line with previous meta-analysis studies (25, 39, 40, 42).
It is evident that the heterogeneity was present for analysis of several variables; thus, subgroup analyses were performed to explore the influence of various factors. The results of in vivo studies showed that the beneficial effects of ALA supplementation on the levels of GSH and IL-6 were not changed by any subgroup factors, suggesting improvement on these two variables may be the main mechanisms of ALA supplementation for nanomaterial-induced toxicity. ALA-induced increase of the GSH content may be, on one hand, attributed to its ability to supply the cysteine precursor of GSH by metabolic reduction of lipoic acid to dihydrolipoic acid; on the other hand, ALA could promote the regeneration of GSH by activating nuclear factor erythroid 2-related factor 2 (Nrf2)/antioxidant responsive element signaling pathway (43–45) and then upregulating the Nrf2-downstream genes γ-glutamate-cysteine ligase (46) and glutathione reductase (34), both of which are key enzymes for GSH synthesis. The beneficial effect of ALA against IL-6 was associated with the increased GSH concentrations (47, 48). ALA was also reported to enhance the Nrf2/heme oxygenase 1 pathway signaling and inhibit the activation of nuclear factor-kappa B (NF-κB), ultimately markedly suppressing the transcription of NF-κB downstream genes pro-inflammatory cytokine IL-6 (49, 50). The subgroup analysis of TNF-α showed that ALA supplementation exerted a significant inhibition effect on the level of TNF-α at the early stage (duration ≤ 2 weeks). This result may be associated with the fact that TNF-α is located upstream of the cytokine cascade (51). Therefore, at the late stage, the level of TNF-α may be similar in two groups, while downstream cytokine IL-6 was abundantly produced and thus, the effect may be more obvious for IL-6.
In addition to anti-oxidant and anti-inflammatory effects, ALA was considered as a metal chelator to prevent metal ion-induced toxicity (19, 20, 33, 35, 37). Hereby, in this study, we also analyzed the metal ions of NPs in animals and cells. The meta-analysis of in vitro studies (n = 8) demonstrated the significant metal-chelating capacity of ALA, while no statistical effects were observed for analysis of in vivo studies (n = 3). This negative metal detoxification effect indicated by in vivo studies may result from a meta-analysis with a small sample size. Furthermore, the different binding affinities of ALA to different ions may also explain this difference between in vitro and in vivo analysis results (52).
Clinically, ALA is commonly used for the treatment of diseases related to the nervous system (53, 54). Although the neuroprotective mechanisms may be complex, anti-oxidant and anti-inflammatory effects of ALA play main roles (53–57), indicating ALA supplementation may antagonize nanomaterial-induced neurotoxicity. In line with the expectation, we found the AchE, an enzyme that mediates the neuromuscular impulse transmission and promotes regeneration of neurites, in rats exposed to nanomaterials was significantly increased by ALA treatment, which was accompanied by improved pathological changes of cerebrum neuron (19, 35). Autonomic neuropathy causes a wide range of cardiac disorders and thus ALA therapy was also attempted for patients with cardiac dysfunction (58, 59) and animal models (60, 61), with the results of improving cardiac functions through suppressing oxidative and inflammatory mediators. Similar to these findings, we also found ALA had a protective role for nanomaterial-induced cardiotoxicity, with CPK significantly decreased (17). Liver tissue is the primary site for detoxification and therefore liver damage is one of the most common consequences after exposure to the toxins, including nanomaterials (33). Prevention of hepatotoxicity was an important goal for the use of ALA. As anticipated, serum ALT (a liver biomarker) enzyme activity in rats that received nanomaterials was significantly reduced after administration of ALA, with the mechanisms associated with an improvement of oxidative and inflammatory status (26, 33).
Oxidative and inflammatory mediators contribute to the ultimate damages in organs since they could react with DNA molecule to cause single-, double-strand breaks and alkali-labile sites (62, 63) and DNA damage could subsequently trigger cell apoptosis via regulating signal transducers including CHK2, p53, E2F-1 and caspases (64). During comet assay, DNA fragmentation migrates into the electric field and forms the comet’s tail; hereby, the tail length reflects the degree of DNA damage. Reduction of the tail length and cell apoptosis (caspase-3 activity) in our results may illustrate the beneficial effects of ALA administration for nanomaterial-induced toxicity.
The present meta-analysis has some limitations. First is the limited number of included in vivo and in vitro studies, which may affect the pooled effect size for some indicators (such as GSH in cells and metal ion content in rats) and result in the effects on other tissue (i.e., lung, kidney, testes) damages that could not be evaluated. Second, the eligible studies were heterogeneous, and the between-study heterogeneity could not be eliminated by the subgroup analysis, which made our conclusion be interpreted with great caution. Third, included animal studies were at an unclear risk of bias during the quality assessment of domains involving the randomization, allocation concealment, and blinding of outcome assessment. Fourth, the majority of data were extracted from the bar graphs, which may lead to some differences from the real data. Fifth, ALA exists in the form of two different optical isomers [(+) or (-) enantiomers]. There was evidence that (+)-ALA was more active than (±)- or (-)-enantiomers in reducing oxidative stress and may be more effective against nanomaterial-induced injuries (55, 56). However, no included studies reported the isomer information of used ALA. Sixth, although our subgroup analyses suggested the ALA dose seemed not to influence the anti-oxidant, anti-inflammatory and chelation effects, it was known that ALA was also not completely free from side effects, particularly (–) enantiomer-treated animals that were found to display more marked organ toxicity signs (57). Thus, low-dose ALA should be recommended theoretically. However, a limitation was that the dose varied even if they were under the range set by us (≤100 mg/kg in animals or ≤400 μM in cells). Based on these limitations, we consider more experiments are needed to confirm the protective effects of ALA (with specific dose, isomer, duration, et al.) on nanomaterial-induced toxicity before it is recommended for clinical application. We also consider a bibliometric analysis by searching the Web of Science with more broad keywords and using Bibliometrix, VOSviewer, or CiteSpace software to find out the future research hotspot, such as other nutritional components which may be suitable to combine with ALA to lower ALA dose and ALA-brought toxicity.
Conclusion
Findings of the current meta-analysis suggest that consumption of ALA may provide the beneficial effects on reducing the inflammatory mediator IL-6, increasing the anti-oxidant GSH and chelating metal ions, which ultimately prevents the cell death, DNA and organ damages induced by nanomaterials.
Data availability statement
The original contributions presented in the study are included in this article/Supplementary material, further inquiries can be directed to the corresponding author/s.
Author contributions
XL and JH conceived the idea and designed the study. XL and DX collected the data, performed the statistical analysis, and wrote the manuscript. TW, WX, QM, and KC contributed to the interpretation of the results. JH revised the manuscript. All authors approved the final version of the manuscript.
Funding
Financial support from National Key Research and Development Program of China (2017YFA0204600) and Project funded by China Postdoctoral Science Foundation (2017M621322 and 2018T110324) is greatly acknowledged.
Conflict of interest
TW was employed by Shanghai Jing Rui Yang Industrial Co., Ltd. WX was employed by Shanghai Nutri-woods Bio-Technology Co., Ltd. Author QM was employed by Shanghai Pechoin Daily Chemical Co., Ltd.
The remaining authors declare that the research was conducted in the absence of any commercial or financial relationships that could be construed as a potential conflict of interest.
Publisher’s note
All claims expressed in this article are solely those of the authors and do not necessarily represent those of their affiliated organizations, or those of the publisher, the editors and the reviewers. Any product that may be evaluated in this article, or claim that may be made by its manufacturer, is not guaranteed or endorsed by the publisher.
Supplementary material
The Supplementary Material for this article can be found online at: https://www.frontiersin.org/articles/10.3389/fnut.2022.991524/full#supplementary-material
Footnotes
References
1. Li J, Xin M, Ma Z, Shi Y, Pan L. Nanomaterials and their applications on bio-inspired wearable electronics. Nanotechnology. (2021) 32:472002. doi: 10.1088/1361-6528/abe6c7
2. Luo X, Liang Y, Weng W, Hu Z, Zhu M. Polypyrrole-coated carbon nanotube/cotton hybrid fabric with high areal capacitance for flexible quasi-solid-state supercapacitors. Eenergy Storage Mater. (2020) 33:11–7. doi: 10.1016/j.ensm.2020.07.036
3. Ranjha M, Shafique B, Rehman A, Mehmood A, Ali A, Zahra SM, et al. Biocompatible nanomaterials in food science, technology, and nutrient drug delivery: recent developments and applications. Front Nutr. (2022) 8:778155. doi: 10.3389/fnut.2021.778155
4. Bhat BB, Kamath PP, Chatterjee S, Bhattacherjee R, Nayak UY. Recent updates on nanocosmeceutical skin care and anti-aging products. Curr Pharm Des. (2022) 28:1258–71. doi: 10.2174/1381612828666220321142140
5. Fonseca AS, Viitanen AK, Kanerva T, Säämänen A, Aguerre-Chariol O, Fable S, et al. Occupational exposure and environmental release: the case study of pouring TiO(2) and filler materials for paint production. Int J Environ Res Public Health. (2021) 18:418. doi: 10.3390/ijerph18020418
6. Boccuni F, Ferrante R, Tombolini F, Natale C, Gordiani A, Sabella S, et al. Occupational exposure to graphene and silica nanoparticles. Part I: workplace measurements and samplings. Nanotoxicology. (2020) 14:1280–300. doi: 10.1080/17435390.2020.1834634
7. Singla R, Sharma C, Shukla AK, Acharya A. Toxicity concerns of therapeutic nanomaterials. J Nanosci Nanotechnol. (2019) 19:1889–907. doi: 10.1166/jnn.2019.16502
8. Puisney C, Baeza-Squiban A, Boland S. Mechanisms of uptake and translocation of nanomaterials in the lung. Adv Exp Med Biol. (2018) 1048:21–36. doi: 10.1007/978-3-319-72041-8_2
9. Omari Shekaftik S, Shirazi FH, Yarahmadi R, Rasouli M, Ashtarinezhad A. Investigating the relationship between occupational exposure to nanomaterials and symptoms of nanotechnology companies’ employees. Arch Environ Occup Health. (2022) 77:209–18. doi: 10.1080/19338244.2020.1863315
10. Guo NL, Bello D, Ye Q, Tagett R, Chanetsa L, Singh D, et al. Pilot deep RNA sequencing of worker blood samples from Singapore printing industry for occupational risk assessment. NanoImpact. (2020) 19:100248. doi: 10.1016/j.impact.2020.100248
11. Abdelhalim MAK, Qaid HA, Al-Mohy YH, Ghannam MM. The protective roles of vitamin E and α-Lipoic acid against nephrotoxicity, lipid peroxidation, and inflammatory damage induced by gold nanoparticles. Int J Nanomedicine. (2020) 15:729–34. doi: 10.2147/IJN.S192740
12. Liu X, Lu B, Fu J, Zhu X, Song E, Song Y. Amorphous silica nanoparticles induce inflammation via activation of NLRP3 inflammasome and HMGB1/TLR4/MYD88/NF-kb signaling pathway in HUVEC cells. J Hazard Mater. (2021) 404:124050. doi: 10.1016/j.jhazmat.2020.124050
13. Bello D, Chanetsa L, Cristophi CA, Poh TY, Singh D, Setyawati MI, et al. Chronic upper airway and systemic inflammation from copier emitted particles in healthy operators at six Singaporean workplaces. NanoImpact. (2021) 22:100325. doi: 10.1016/j.impact.2021.100325
14. Pelclova D, Zdimal V, Komarc M, Vlckova S, Fenclova Z, Ondracek J, et al. Deep airway inflammation and respiratory disorders in nanocomposite workers. Nanomaterials. (2018) 8:731. doi: 10.3390/nano8090731
15. Pelclova D, Zdimal V, Kacer P, Komarc M, Fenclova Z, Vlckova S, et al. Markers of lipid oxidative damage among office workers exposed intermittently to air pollutants including nanoTiO2 particles. Rev Environ Health. (2017) 32:193–200. doi: 10.1515/reveh-2016-0030
16. An H, Ling C, Xu M, Hu M, Wang H, Liu J, et al. Oxidative damage induced by nano-titanium dioxide in rats and mice: a systematic review and meta-analysis. Biol Trace Elem Res. (2020) 194:184–202. doi: 10.1007/s12011-019-01761-z
17. Baky NA, Faddah LM, Al-Rasheed NM, Al-Rasheed NM, Fatani AJ. Induction of inflammation, DNA damage and apoptosis in rat heart after oral exposure to zinc oxide nanoparticles and the cardioprotective role of α-lipoic acid and vitamin E. Drug Res. (2013) 63:228–36. doi: 10.1055/s-0033-1334923
18. Abdelhalim MAK, Moussa SAA, Qaid HA, Al-Ayed MS. Potential effects of different natural antioxidants on inflammatory damage and oxidative-mediated hepatotoxicity induced by gold nanoparticles. Int J Nanomedicine. (2018) 13:7931–8. doi: 10.2147/IJN.S171931
19. Deore MS, Keerthana S, Naqvi S, Kumar A, Flora SJS. Alpha-lipoic acid protects co-exposure to lead and zinc oxide nanoparticles induced neuro, immuno and male reproductive toxicity in rats. Front Pharmacol. (2021) 12:626238. doi: 10.3389/fphar.2021.626238
20. Liu Y, Zhu W, Ni D, Zhou Z, Gu JH, Zhang W, et al. Alpha lipoic acid antagonizes cytotoxicity of cobalt nanoparticles by inhibiting ferroptosis-like cell death. J Nanobiotechnology. (2020) 18:141. doi: 10.1186/s12951-020-00700-8
21. Liang S, Sun K, Wang Y, Dong S, Wang C, Liu L, et al. Role of Cyt-C/caspases-9,3, Bax/Bcl-2 and the FAS death receptor pathway in apoptosis induced by zinc oxide nanoparticles in human aortic endothelial cells and the protective effect by alpha-lipoic acid. Chem Biol Interact. (2016) 258:40–51. doi: 10.1016/j.cbi.2016.08.013
22. Kad A, Pundir A, Arya SK, Puri S, Khatri M. Meta-analysis of in-vitro cytotoxicity evaluation studies of zinc oxide nanoparticles: paving way for safer innovations. Toxicol In Vitro. (2022) 83:105418. doi: 10.1016/j.tiv.2022.105418
23. Sachdeva V, Roy A, Bharadvaja N. Current prospects of nutraceuticals: a review. Curr Pharm Biotechnol. (2020) 21:884–96. doi: 10.2174/1389201021666200130113441
24. Yoon J, Lee H, Lim JW, Kim H. Inhibitory effect of alpha-lipoic acid on mitochondrial dysfunction and interleukin-8 expression in interleukin-1beta-stimulated ataxia teleangiectasia fibroblasts. J Physiol Pharmacol. (2020) 71:155–65.
25. Haghighatdoost F, Hariri M. The effect of alpha-lipoic acid on inflammatory mediators: a systematic review and meta-analysis on randomized clinical trials. Eur J Pharmacol. (2019) 849:115–23. doi: 10.1016/j.ejphar.2019.01.065
26. Al-Rasheed NM, Al-Rasheed NM, Abdel Baky NA, Faddah LM, Fatani AJ, Hasan IH, et al. Prophylactic role of α-lipoic acid and vitamin E against zinc oxide nanoparticles induced metabolic and immune disorders in rat’s liver. Eur Rev Med Pharmacol Sci. (2014) 18:1813–28.
27. Abdelkarem HM, Fadda LM, Kaml OR. Alleviation of bone markers in rats induced nano-zinc oxide by qurecetin and α-lipolic acid. Toxicol Mech Methods. (2016) 26:692–9. doi: 10.1080/15376516.2016.1236424
28. Schneider K, Schwarz M, Burkholder I, Kopp-Schneider A, Edler L, Kinsner-Ovaskainen A, et al. ”ToxRTool”, a new tool to assess the reliability of toxicological data. Toxicol Lett. (2009) 189:138–44. doi: 10.1016/j.toxlet.2009.05.013
29. Sanches PL, Geaquinto LRO, Cruz R, Schuck DC, Lorencini M, Granjeiro JM, et al. Toxicity evaluation of TiO(2) nanoparticles on the 3D skin model: a systematic review. Front Bioeng Biotechnol. (2020) 8:575. doi: 10.3389/fbioe.2020.00575
30. Hooijmans CR, Rovers MM, de Vries RB, Leenaars M, Ritskes-Hoitinga M, Langendam MW. SYRCLE ’s risk of bias tool for animal studies. BMC Med Res Methodol. (2014) 14:43. doi: 10.1186/1471-2288-14-43
31. Andriychuk NJ, Vlasyk LI. Study of protective effect of alpha lipoic acid in conditions of subacute action of decahedron silver nanoparticles. Ukrainian J Occup Health. (2015) 4:38–43. doi: 10.33573/ujoh2015.01.038
32. Sun A, Qian D, Wang Z, Xu Y, Yan CH. Protective effect of lipoic acid modification on brain dysfunctions of mice induced by mesoporous silica nanoparticles. Chem Eng J. (2021) 415:128957. doi: 10.1016/j.cej.2021.128957
33. Khalaf AA, Zaki AR, Galal MK, Ogaly HA, Ibrahim MA, Hassan A. The potential protective effect of α-lipoic acid against nanocopper particle-induced hepatotoxicity in male rats. Hum Exp Toxicol. (2017) 36:881–91. doi: 10.1177/0960327116674526
34. Tohamy HG, Lebda MA, Sadek KM, Elfeky MS, El-Sayed YS, Samak DH, et al. Biochemical, molecular and cytological impacts of alpha-lipoic acid and Ginkgo biloba in ameliorating testicular dysfunctions induced by silver nanoparticles in rats. Environ Sci Pollut Res Int. (2022) 29:38198–211. doi: 10.1007/s11356-021-18441-y
35. Lebda MA, Sadek KM, Tohamy HG, Abouzed TK, Shukry M, Umezawa M, et al. Potential role of α-lipoic acid and Ginkgo biloba against silver nanoparticles-induced neuronal apoptosis and blood-brain barrier impairments in rats. Life Sci. (2018) 212:251–60. doi: 10.1016/j.lfs.2018.10.011
36. Jain MP, Choi AO, Neibert KD, Maysinger D. Probing and preventing quantum dot-induced cytotoxicity with multimodal alpha-lipoic acid in multiple dimensions of the peripheral nervous system. Nanomedicine. (2009) 4:277–90. doi: 10.2217/nnm.09.3
37. An X, Liu L, Schaefer M, Yan B, Scholz C, Hillmer S, et al. Alpha-lipoic acid prevents side effects of therapeutic nanosilver without compromising cytotoxicity in experimental pancreatic cancer. Cancers. (2021) 13:4770. doi: 10.3390/cancers13194770
38. Saboori S, Falahi E, Eslampour E, Zeinali Khosroshahi M, Yousefi Rad E. Effects of alpha-lipoic acid supplementation on C-reactive protein level: a systematic review and meta-analysis of randomized controlled clinical trials. Nutr Metab Cardiovasc Dis. (2018) 28:779–86. doi: 10.1016/j.numecd.2018.04.003
39. Akbari M, Ostadmohammadi V, Tabrizi R, Mobini M, Lankarani KB, Moosazadeh M, et al. The effects of alpha-lipoic acid supplementation on inflammatory markers among patients with metabolic syndrome and related disorders: a systematic review and meta-analysis of randomized controlled trials. Nutr Metab. (2018) 15:39. doi: 10.1186/s12986-018-0274-y
40. Rahimlou M, Asadi M, Banaei Jahromi N, Mansoori A. Alpha-lipoic acid (ALA) supplementation effect on glycemic and inflammatory biomarkers: a systematic review and meta- analysis. Clin Nutr ESPEN. (2019) 32:16–28. doi: 10.1016/j.clnesp.2019.03.015
41. Mahmoudi-Nezhad M, Vajdi M, Farhangi MA. An updated systematic review and dose-response meta-analysis of the effects of α-lipoic acid supplementation on glycemic markers in adults. Nutrition. (2021) 82:111041. doi: 10.1016/j.nut.2020.111041
42. Moeinian M, Abdolghaffari AH, Nikfar S, Momtaz S, Abdollahi M. Effects of alpha lipoic acid and its derivative “andrographolid-lipoic acid-1” on ulcerative colitis: a systematic review with meta-analysis of animal studies. J Cell Biochem. (2019) 120:4766–82. doi: 10.1002/jcb.27807
43. Gu L, Li S, Bai J, Zhang Q, Han Z. α-Lipoic acid protects against microcystin-LR induced hepatotoxicity through regeneration of glutathione via activation of Nrf2. Environ Toxicol. (2020) 35:738–46. doi: 10.1002/tox.22908
44. Shi C, Zhou X, Zhang J, Wang J, Xie H, Wu Z. α-Lipoic acid protects against the cytotoxicity and oxidative stress induced by cadmium in HepG2 cells through regeneration of glutathione by glutathione reductase via Nrf2/ARE signaling pathway. Environ Toxicol Pharmacol. (2016) 45:274–81. doi: 10.1016/j.etap.2016.06.003
45. Zhang J, Zhou X, Wu W, Wang J, Xie H, Wu Z. Regeneration of glutathione by α-lipoic acid via Nrf2/ARE signaling pathway alleviates cadmium-induced HepG2 cell toxicity. Environ Toxicol Pharmacol. (2017) 51:30–7. doi: 10.1016/j.etap.2017.02.022
46. Xu Y, Zhou X, Shi C, Wang J, Wu Z. α-Lipoic acid protects against the oxidative stress and cytotoxicity induced by cadmium in HepG2 cells through regenerating glutathione regulated by glutamate-cysteine ligase. Toxicol Mech Methods. (2015) 25:596–603.
47. Tsai CH, Yang SF, Chen YJ, Chu SC, Hsieh YS, Chang YC. Regulation of interleukin-6 expression by arecoline in human buccal mucosal fibroblasts is related to intracellular glutathione levels. Oral Dis. (2004) 10:360–4. doi: 10.1111/j.1601-0825.2004.01041.x
48. Allameh A, Ahmadi-Ashtiani HR, Maleki N. Glutathione-related inflammatory signature in hepatocytes differentiated from the progenitor mesenchymal stem cells. Heliyon. (2020) 6:e04149. doi: 10.1016/j.heliyon.2020.e04149
49. Van Nguyen T, Piao CH, Fan YJ, Shin DU, Kim SY, Song HJ, et al. Anti-allergic rhinitis activity of α-lipoic acid via balancing Th17/Treg expression and enhancing Nrf2/HO-1 pathway signaling. Sci Rep. (2020) 10:12528. doi: 10.1038/s41598-020-69234-1
50. Li M, Wu X, Zou J, Lai Y, Niu X, Chen X, et al. Dietary α-lipoic acid alleviates deltamethrin-induced immunosuppression and oxidative stress in northern snakehead (Channa argus) via Nrf2/NF-κB signaling pathway. Fish Shellfish Immunol. (2022) 127:228–37. doi: 10.1016/j.fsi.2022.06.029
51. Vanden Berghe W, Vermeulen L, De Wilde G, De Bosscher K, Boone E, Haegeman G. Signal transduction by tumor necrosis factor and gene regulation of the inflammatory cytokine interleukin-6. Biochem Pharmacol. (2000) 60:1185–95. doi: 10.1016/S0006-2952(00)00412-3
52. Smirnova J, Kabin E, Järving I, Bragina O, Tõugu V, Plitz T, et al. Copper(I)-binding properties of de-coppering drugs for the treatment of Wilson disease. α-Lipoic acid as a potential anti-copper agent. Sci Rep. (2018) 8:1463. doi: 10.1038/s41598-018-19873-2
53. Nádró B, Lõrincz H, Molnár Á, Szentpéteri A, Zöld E, Seres I. Effects of alpha-lipoic acid treatment on serum progranulin levels and inflammatory markers in diabetic neuropathy. J Int Med Res. (2021) 49:3000605211012213. doi: 10.1177/03000605211012213
54. Khan H, Singh TG, Dahiya RS, Abdel-Daim MM. α-Lipoic acid, an organosulfur biomolecule a novel therapeutic agent for neurodegenerative disorders: an mechanistic perspective. Neurochem Res. (2022) 47:1853–64. doi: 10.1007/s11064-022-03598-w
55. Pacini A, Tomassoni D, Trallori E, Micheli L, Amenta F, Ghelardini C, et al. Comparative assessment of the activity of racemic and dextrorotatory forms of thioctic (alpha-lipoic) acid in low back pain: preclinical results and clinical evidences from an open randomized trial. Front Pharmacol. (2021) 12:607572. doi: 10.3389/fphar.2021.607572
56. Tomassoni D, Amenta F, Di Cesare Mannelli L, Ghelardini C, Nwankwo IE, Pacini A. Neuroprotective activity of thioctic acid in central nervous system lesions consequent to peripheral nerve injury. Biomed Res Int. (2013) 2013:985093. doi: 10.1155/2013/985093
57. Lucarini E, Trallori E, Tomassoni D, Amenta F, Ghelardini C, Pacini A, et al. Toxicological profile of the pain-relieving antioxidant compound thioctic acid in its racemic and enantiomeric forms. Antioxidants. (2020) 9:749. doi: 10.3390/antiox9080749
58. Serhiyenko VA, Serhiyenko LM, Sehin VB, Serhiyenko AA. Effect of alpha-lipoic acid on arterial stiffness parameters in type 2 diabetes mellitus patients with cardiac autonomic neuropathy. Endocr Regul. (2021) 55:224–33. doi: 10.2478/enr-2021-0024
59. Hegazy SK, Tolba OA, Mostafa TM, Eid MA, El-Afify DR. Alpha-lipoic acid improves subclinical left ventricular dysfunction in asymptomatic patients with type 1 diabetes. Rev Diabet Stud. (2013) 10:58–67. doi: 10.1900/RDS.2013.10.58
60. Gholami S, Mokhtari B, Badalzadeh R. Alpha-lipoic acid potentiates the anti-arrhythmic effects of ischemic postconditioning in the setting of cardiac ischemia/reperfusion injury in diabetic rats. J Diabetes Metab Disord. (2022) 21:707–16. doi: 10.1007/s40200-022-01034-y
61. Li CJ, Zhang QM, Li MZ, Zhang JY, Yu P, Yu DM. Attenuation of myocardial apoptosis by alpha-lipoic acid through suppression of mitochondrial oxidative stress to reduce diabetic cardiomyopathy. Chin Med J. (2009) 122:2580–6.
62. Lee J, Kim Y, Lim S, Jo K. Single-molecule visualization of ROS-induced DNA damage in large DNA molecules. Analyst. (2016) 141:847–52. doi: 10.1039/C5AN01875G
63. Matsuya Y, Hamada N, Yachi Y, Satou Y, Ishikawa M, Date H, et al. Inflammatory signaling and DNA damage responses after local exposure to an insoluble radioactive microparticle. Cancers. (2022) 14:1045. doi: 10.3390/cancers14041045
Keywords: nanomaterials, oxidative stress, inflammation, alpha-lipoic acid, meta-analysis
Citation: Luo X, Xie D, Wu T, Xu W, Meng Q, Cao K and Hu J (2022) Evaluation of the protective roles of alpha-lipoic acid supplementation on nanomaterial-induced toxicity: A meta-analysis of in vitro and in vivo studies. Front. Nutr. 9:991524. doi: 10.3389/fnut.2022.991524
Received: 11 July 2022; Accepted: 17 August 2022;
Published: 06 September 2022.
Edited by:
Mohd Imtiaz Nawaz, King Saud University, Saudi ArabiaReviewed by:
Mohamed M. Abdel-Daim, Suez Canal University, EgyptLorenzo Di Cesare Mannelli, University of Florence, Italy
Copyright © 2022 Luo, Xie, Wu, Xu, Meng, Cao and Hu. This is an open-access article distributed under the terms of the Creative Commons Attribution License (CC BY). The use, distribution or reproduction in other forums is permitted, provided the original author(s) and the copyright owner(s) are credited and that the original publication in this journal is cited, in accordance with accepted academic practice. No use, distribution or reproduction is permitted which does not comply with these terms.
*Correspondence: Xiaogang Luo, eGdsdW9Ac3VkYS5lZHUuY24=; Jianchen Hu, aHVqaWFuY2hlbkBzdWRhLmVkdS5jbg==