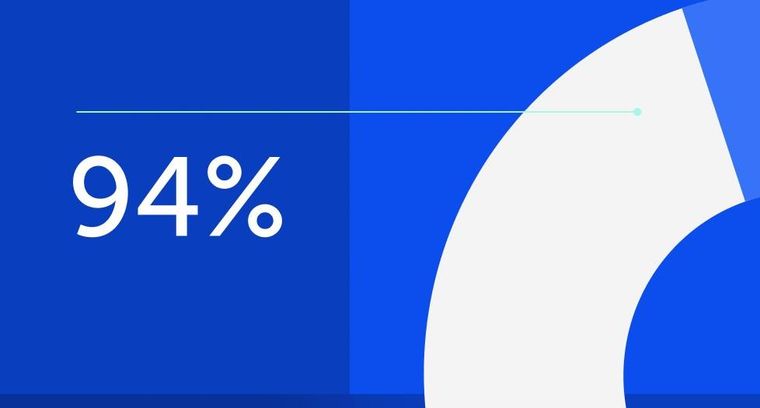
94% of researchers rate our articles as excellent or good
Learn more about the work of our research integrity team to safeguard the quality of each article we publish.
Find out more
ORIGINAL RESEARCH article
Front. Nutr., 23 August 2022
Sec. Nutrition and Microbes
Volume 9 - 2022 | https://doi.org/10.3389/fnut.2022.988517
Pleurotus eryngii mushrooms are commercially cultivated and widely consumed due to their organoleptic properties, and the low caloric and high nutritional value. In addition, they contain various biologically active and health-promoting compounds; very recently, their genoprotective effect in Caco-2 cells after their fermentation by the human fecal microbiota was also documented. In the current study, the effect of P. eryngii pre- and post-fermentation supernatants in micronuclei formation was evaluated in human lymphocytes. In addition, the genoprotective properties of increasing concentrations of aqueous extracts from P. eryngii mushrooms (150, 300, 600 mg/kg) against the cyclophosphamide-induced DNA damage were studied in young and elderly female and male mice in bone marrow and whole blood cells. The ability of the highest dose (600 mg/kg) to regulate the main cellular signaling pathways was also evaluated in gut and liver tissues of female animals by quantifying the mRNA expression of NrF2, Nfkβ, DNMT1, and IL-22 genes. P. eryngii post-fermentation, but not pre-fermentation, supernatants were able to protect human lymphocytes from the mitomycin C-induced DNA damage in a dose-dependent manner. Similarly, genoprotection was also observed in bone marrow cells of mice treated by gavage with P. eryngii extract. The effect was observed in all the experimental groups of mice (young and elderly, male and female) and was more potent in young female mice. Overexpression of all genes examined was observed in both tissues, mainly among the elderly animals. In conclusion, P. eryngii mushrooms were shown to maintain genome integrity through protecting cells from genotoxic insults. These beneficial effects can be attributed to their antioxidant and immunomodulatory properties, as well as their ability to regulate the cell’s epigenetic mechanisms and maintain cell homeostasis.
The beneficial health properties of edible mushrooms have been known since ancient times. Mushrooms are a rich source of polysaccharides, mainly β-glucans, peptidoglucans, and proteoglucans (1, 2). They also contain a plethora of bioactive components, including proteins, chitins, unsaturated fatty acids, vitamins, triterpenic acids, lectins, statins, alkaloids, and polyphenolic compounds (1, 2). Mushrooms were found to be beneficial in preventing and treating chronic diseases, such as cardiovascular and neurodegenerative disorders, diabetes mellitus, hyperlipidemia, atherosclerosis, hypercholesterolemia, and cancer. Their biological activities are extensive, ranging from antioxidant, antimicrobial, prebiotic, immunomodulatory and anti-inflammatory to genoprotective, and anticarcinogenic (1–3). The latter properties, have been the focal point of intense research over the last decade.
Aqueous or organic extracts of mushrooms of various origins or their isolated ingredients were shown to have genoprotective properties against the insult of genotoxic and mutagenic carcinogens both in vitro (4–8) and in vivo (9). In a recent study, Chaga mushroom (Inonotus obliquus) polysaccharides exhibited genoprotective effects in UVB-exposed embryonic zebrafish (Danio rerio) through altered expression of DNA repair genes (10).
Mushroom fruit bodies, extracts or purified bioactive compounds have also been found to possess anticarcinogenic properties. Mushroom extracts exhibit in vitro anti-proliferating or pro-apoptotic activity against various cell lines (11–15), whereas mushrooms’ β-glucans, such as lentinan, schizophyllan, grifolan or polysaccharide-protein, and polysaccharide-peptide complexes, prevent oncogenesis by stimulating the immune system (16). A reduction in the density of lung metastases and lower expression of interleukin-6 (IL-6), cyclooxygenase-2 (COX-2), and nitric oxide synthase (NOS) were shown in mice with 4T1 triple-negative breast cancer (estrogen receptor; ER, progesterone receptor; PgR, human epidermal growth factor receptor-2; HER2) treated, orally, with a mixture of mushrooms (17). Furthermore, lentinan, a neutral polysaccharide purified from the extract of Lentinula edodes mycelia, is now approved to be used in clinical trials as cancer adjuvant therapy, and non-small cell lung carcinoma patients treated on chemo-immunotherapy of lentinan were shown to exhibit an increase in proliferation of cytotoxic T cells, accompanied by the rise in tumor necrosis factor-alpha (TNF-α), interferon gamma (IFN-γ), and interleukin-12 (IL-12) levels (18).
The genoprotective and anticarcinogenic properties of fungal bioactive compounds are frequently attributed to their action as radical scavengers (5, 6, 8) or as direct-acting immunomodulators (18). However, different bioactive compounds from mushrooms have been shown to alter the gut microbiome (GM), and recent research has demonstrated the fundamental role of the latter not only in colorectal carcinogenesis but also in almost every cancer (19, 20). Given that the host-microbiome crosstalk is very complex, changes in the gut microbial population caused by the diet or other confounders may promote or inhibit carcinogenesis (21), and microbiome excreted macromolecules, or small metabolites may act as tumor suppressors or promoters. However, studies on the genoprotective or anticarcinogenic properties of mushrooms fermented by the gut microbiota are scarce and focus only on the effects of fermentation of indigestible polysaccharides (22–26).
We recently investigated the genoprotective effects of edible mushrooms (Pleurotus eryngii, Pleurotus ostreatus, and Cyclocybe cylindracea) following their in vitro static batch culture fermentation by fecal inocula from elderly donors. We subsequently documented for the first time that fermented Pleurotus eryngii (and to a lesser extent Pleurotus ostreatus) mushrooms reduce the tert-butyl hydroperoxide (t-BOOH) induced oxidative DNA damage in Caco-2 human colon adenocarcinoma cells (27). In our study, elderly volunteers were recruited because their gradual age-associated decline of biological functions such as metabolism and immune responses make them more prone to developing various pathological conditions such as cancer (28–30). This finding provided substantial evidence that mushrooms may contain ingredients that, after fermentation by the human gut microbiota, protect genome integrity from genotoxic insults and may act as tumor suppressors. It is worth highlighting that precipitates and supernatants from the same in vitro reactions were previously examined for alterations in fecal microbiota composition (quantitative PCR) and in short-chain fatty acids (SCFA) concentrations (Gass Chromatography) respectively, during the 24-h fermentation processes. P. eryngii was shown to induce a strong lactogenic effect, whereas, all lyophilized mushrooms induced higher SCFA production than that induced by the same concentration (w/v) of the prebiotic polysaccharide inulin (3). Therefore, the beneficial effects of whole mushrooms or mushroom extracts need further investigation, specifically regarding their interactions with the gut microbiome and metabolome. In the current study, we extended the previously reported investigation on the genoprotective properties of P. eryngii fermentation supernatants (PE-FS) in human lymphocytes utilizing the Cytokinesis-block micronucleus (MN) assay. MN frequency in peripheral blood lymphocytes is predictive of the risk for various cancer types, making the Micronucleus test the most informative genotoxicity assay (31, 32). Furthermore, the genoprotective effect of an aqueous extract of P. eryngii mushroom (PE-E) was evaluated in vivo, using young (8–9 weeks old) as well as elderly (17–18 months old) mice of both genders. The extract’s ability to intervene and modulate main signaling pathways of the cell was also evaluated in gut and liver tissues of female animals by quantifying the mRNA expression of the hub genes nuclear factor erythroid 2–related factor 2 (NrF2), Nuclear factor-kβ (Nfkβ), DNA methyltransferase 1(DNMT1), and Interleukin-22 (IL-22).
Ham’s F-10 was purchased from Biosera (Nuaille, France), mitomycin C and cytochalasin B were purchased from Cayman chemical company (Ann Arbor, MI, United States), Mushroom and Yeast Beta-Glucan assay kit (K-YBGL) was purchased from Megazyme (Bray, Ireland), Luna Universal PCR mix was purchased from New England Biolabs Inc. (Ipswich, MA, United States), Giemsa’s azur eosin methylene blue solution was purchased from Panreac Applichem (Castella del Valles, Spain), cyclophosphamide, DPX mounting medium, methanol, glacial acetic acid and formalin solution (neutral buffered, 10%) were purchased from Merck (Darmstadt, Germany), Fetal Bovine Serum (FBS), L-glutamine, M-MLV reverse transcriptase, Phytohemagglutinin M (PHA-M), and Trizol reagent were purchased from Thermo Fisher Scientific (Paisley, United Kingdom).
Pure cultures of Pleurotus eryngii (PE) strain LGAM216 (Basidiomycota, Agaricales) are maintained in the Fungal Culture Collection of the Laboratory of General and Agricultural Microbiology (Agricultural University of Athens). Mushroom cultivation and batch in vitro fermentation were performed as previously described (3, 27). For the extraction, 50 g of lyophilized PE powder were suspended in 1 L H2O at 95°C for 24 h, under continuous stirring. The mixture was centrifuged at 4,500 rpm for 1 h; the supernatant was transferred to a new tube and evaporated using a rotary evaporator at 50°C. The concentrated supernatant was lyophilized to dryness, and the semisolid material obtained was kept at 4°C until use. α- and β-glucan content of P. eryngii extract (PE-E) was determined by K-YBGL kit (Megazyme) according to the manufacturer’s instructions. The extraction procedure resulted in an aqueous extract with total glucan content 43 ± 3.3% (w/w), α-glucan content 11 ± 0.7% (w/w), and β-glucan content 32 ± 2.7% (w/w). The lyophilized extract was dissolved in water at appropriate concentrations for the in vivo experiments.
Five asymptomatic fecal donors (>60 years; three males, two females) were recruited, and the field study was conducted as previously described (3, 27). The study was performed according to the guidelines laid down in the Declaration of Helsinki and under the approval of the Bioethics Committee of Harokopio University, Athens, Greece (62-03/07/2018). Written informed consent was obtained from all fecal donors before their inclusion in the study.
Whole peripheral blood samples were obtained by venous puncture from four donors (two males, two females), for performing the in vitro micronucleus assay. The donors were asymptomatic, non-smoking volunteers of Greek nationality and ethnicity, 25–35 years old. Subjects had not been exposed to radiation or drugs 6 months before the study and were interviewed for the possible influence of confounding factors. The study was approved by the 6/30-05-2022 NHRF’s Research Ethics Committee.
For the in vitro static batch culture fermentation, lyophilized P. eryngii powder was added to fresh fecal inocula obtained from all five volunteers, suspended in basal medium. Inoculum samples without the addition of mushroom powder from each volunteer were also included as negative controls (NC). Pre- and post-fermentation supernatants (FS) were obtained as described elsewhere (3, 27).
Five out of the eight matched pre- and post-fermentation supernatants (FS) obtained from the previous studies (3, 27) were examined for their modulatory effect on the genotoxicity of mitomycin C (reference genotoxic agent) in human lymphocytes using the Cytokinesis- block micronucleus assay. Whole blood from each of the four donors was treated independently with the PE-FS or NC-FS as previously described (33). Therefore, twenty independent experiments (five fecal donors × four blood donors) were performed for each of the conditions tested (pre-fermentation, post-fermentation). The procedure was as follows: 0.5 ml of peripheral blood was added to 8.3 ml Ham’s F-10 culture medium supplemented with 20% Fetal Bovine Serum, 1 mM L-glutamine and 4% v/v Phytohemagglutinin-M. The cultures were incubated at 37°C in a humidified incubator with 5% CO2 for 24 h. Pre- and post- fermentation supernatants were added at 1% v/v concentration in combination with the genotoxic agent mitomycin C (0.05 μg/ml). Cells treated with the medium or with only the genotoxic agent were also included as negative and positive controls, respectively. The cultures were incubated for 20 h, and then 6 μg/ml of the cytostatic agent cytochalasin B was added. The samples were collected 28 h later and centrifuged at 1,500 rpm for 10 min. Cell pellets were resuspended in 3 ml of hypotonic solution (Ham’s F-10: H2O, 3:1) and 5 ml of fixative solution (methanol: acetic acid, 5:1), under vigorous stirring. The samples were centrifuged at 1,500 rpm for 10 min and the cell pellets were resuspended in 5 ml of fixative solution. This procedure was repeated three times. Samples for microscopic observation were obtained by carefully dropping the cell suspension onto clean wet slides. Microscopic slides were stained with 10% Giemsa solution and covered with mounting media DPX and coverslips. The frequency of micronuclei in one thousand binucleated lymphocytes was evaluated under a light microscope for each sample.
CD1 mice purchased from Charles River were housed in the certified Animal Lab Facility of the National Hellenic Research Foundation (EL 25 BIO 031-033) under constant photoperiod cycle (12 h light, 12 h dark), humidity (55–60%), room temperature (22 ± 2°C) and free access to tap water and normal chow. Animal handling followed the regulations of the Federation of the European Laboratory Animal Science Association (FELASA) and the experimental design was approved by the Veterinary Office of Attica Prefecture (82837-30/01/2020) and the 7/15-06-2022 NHRF’s Research Ethics Committee.
This study was performed in accordance with the OECD guideline for the testing of chemicals #474 (1997): mammalian erythrocyte micronucleus test, as was updated in 2014 and published in 2016 (34). The genoprotective effects of P. eryngii hot water extract (PE-E) were assessed in young and elderly mice of both sexes. Seventy-two young mice aged 8–9 weeks (36 male and 36 female) and 72 elderly mice aged 17–18 months (36 male and 36 female) were separated by age and gender into groups of six animals. The animals were treated orally by gavage, once daily, with the mushroom’s hot water extract at doses of 150, 300, and 600 mg/kg for 14 days. Body weights were measured every 2 days, and the dose was adjusted to body weight changes. On the 14th day, the genotoxic agent cyclophosphamide (80 mg/kg) was also administered intraperitoneally for micronuclei formation. Twenty-four hours after CP administration, the animals were anesthetized for blood collection and killed by cervical dislocation for bone marrow isolation and tissue removal. Animals treated only with water (vehicle), cyclophosphamide, or PE-E for the same period were also included as controls.
Liver and colon tissues were obtained only from elderly male and female mice of (a) the control group (C: 150 μl water administration, every day for 14 days), (b) the cyclophosphamide treated group (CP: 150 μl H2O for 14 days + 80 mg/kg cyclophosphamide at the 14th day) and (c) the group treated with the highest dose of PE-E and cyclophosphamide (CP + PE-E: 600 mg/kg mushroom extract for 14 days + 80 mg/kg cyclophosphamide at the 14th day). Tissues from each group were processed for histopathological examination. Liver and gut tissues were kept in 10% formalin solution before being embedded in paraplast, sectioned using a microtome (4 μm sections) and stained with Hematoxylin-Eosin (H/E). Three pathologists examined the tissue sections twice in a Nikon E400 microscope independently at different time points.
The Micronucleus assay in bone marrow was executed as previously described (35). Briefly, both femurs of the cervical dislocated animals were removed, and bone marrow cells were washed in fetal bovine serum. Bone marrow smears were prepared on clean glass slides, air-dried, fixed with absolute methanol, and stained with 10% Giemsa solution.
The Micronucleus assay in peripheral blood cells was performed according to the method described by Hayashi et al. (36). Briefly, peripheral blood was obtained from the superficial temporal vein, and blood smears were prepared instantly on clean glass slides, air-dried, fixed with absolute methanol, and stained with 10% Giemsa solution.
The micronucleus frequency in the bone marrow and whole blood cells was determined by counting the micronuclei in 1,000 polychromatic erythrocytes (PCEs) under a light microscope.
Total RNA was extracted using the Trizol reagent and reversed transcribed. The RNA quality was confirmed by agarose gel electrophoresis and the Agilent 4,150 Tapestation system. Real–time PCR was performed in 96-well plates using the Luna universal PCR mix in a CFX96 PCR thermal cycler (Biorad). The thermal cycling conditions comprised an initial denaturation step at 95°C for 60 s followed by 42 cycles at 95°C for 15 s and 60°C for 30 s. The comparative Ct method was used to calculate the relative gene expression (37). Expression levels of the genes of interest were normalized to the respective levels of GAPDH. Supplementary Table 1 shows the sets of real-time qPCR (RT-qPCR) primers used for each gene whose expression was studied.
All statistical tests were performed by Sigmaplot 14 software. All data distributions passed the Shapiro–Wilk normality test. In vitro comparisons were analyzed using paired samples t-test. In vivo micronucleus assay data were analyzed by one-way ANOVA, post hoc Dunnett’s test for multiple comparisons. Gene expression comparisons were performed by student’s t-test with 95% confidence interval. *p ≤ 0.05 was considered as statistically significant.
Peripheral blood mononuclear cells obtained from four blood donors (2 male, 2 female) were treated with 1% v/v fermentation supernatants in combination with the genotoxic agent mitomycin C (0.05 μg/ml). Five out of the eight matched pre- and post-fermentation supernatants (FS) obtained from previous studies (3, 27) were examined. Therefore, twenty independent experiments (five fecal donors × four blood donors) were performed for both PE treated (PE-FS) and untreated controls (NC-FS), and for each of the conditions tested (pre-fermentation, post-fermentation). Micronuclei formation was calculated in 1,000 binucleated lymphocytes per blood and fecal donor. Figure 1A represents the genoprotective effects of post-fermentation PE-FS. There was a consistent, highly significant (t = 7.5; p < 0.001) reduction of the micronuclei frequency formed (mean = −28.1%; SD = 15.4) for the PE-FS relative to the corresponding NC-FS. The genoprotective effect was fecal and blood donor independent as shown in Supplementary Figures 1A,B, ranging from −23.3 to −39.3% depending on the blood donor.
Figure 1. Genoprotective and genotoxic effects of (A) post- and (B) pre- fermentation supernatants in human lymphocytes. Human peripheral blood cells were treated with 1% v/v post- and pre- fermentation supernatants of NC and PE in combination with the mutagenic agent mitomycin C. Micronuclei formation was evaluated in 1,000 binucleated lymphocytes for each blood and fecal donor. (A) Genoprotective effect of 1% v/v post- fermentation supernatants of PE per blood and fecal donor (t = 24 h); (B) genotoxic effect of 1% v/v pre- fermentation supernatants of PE per blood and fecal donor (t = 0 h). NC-FS, fermentation supernatants without any additional carbon source; PE-FS, fermentation supernatants of Pleurotus eryngii mushroom; D1–D4, blood donors.
As previously observed in Caco-2 cells and batch in vitro fermentation of P. eryngii and other edible mushrooms (27), the pre-fermentation supernatants (PE-FS) exhibited a slight genotoxic effect (mean = 13.4%; SD = 17.0) when compared to the NC-FS (p = 0.003) (Figure 1B). However, the effect seems to be fecal and blood donor dependent as shown in Supplementary Figures 1A,B. These results indicate that P. eryngii and other mushrooms contain water-soluble constituents that are either genotoxic or become genotoxic through interactions with inoculum ingredients. These compounds are either degraded by the gut microorganisms or/and inactivated by their metabolic products that may exert genoprotective action.
There were no significant changes in body weight during the 14-day treatment period at any experimental group (data not shown). Histopathological examination of colon and liver tissues of the controls CP and CP + PE-E treated elderly male and female mice did not exhibit any significant tissue alterations (Figures 2A,B). A slight increase in the number of eosinophil leucocytes in the mucosal enteric crypts of the colon was sporadically observed in all groups (Figure 2C). Some of the CP + PE-E- treated animals also showed a mild liver sinusoidal/canalicular dilatation (Figure 2D).
Figure 2. Histopathological analysis of colon and liver tissues of elderly mice. At the end of the treatment period, the mice were anesthetized and killed by cervical dislocation for tissue removal. Liver and gut tissues were kept in 10% formalin solution before being embedded in paraplast, sectioned using a microtome (4 μm sections) and stained with Hematoxylin-Eosin (H/E). The tissue sections were examined twice in a Nikon E400 microscope by three pathologists independently at different time points. (A) Colon and (B) liver tissues without any histopathological alterations; (C) colon tissue with small number of eosinophil leucocytes in the mucosal enteric crypts (arrows); (D) liver tissue with mild liver sinusoidal/canalicular dilatation (arrows).
The frequency of micronuclei formation in the bone marrow cells of young and elderly mice treated with different concentrations of P. eryngii hot water extract (PE-E) in combination with the alkylating agent cyclophosphamide is shown in Table 1. Administration of PE-E alone did not increase the number of micronuclei in polychromatic erythrocytes compared to the group which received water only. Young mice of both genders were more sensitive to the cyclophosphamide-induced genotoxicity and micronuclei formation than old mice of the same gender (p < 0.05). All groups treated with the highest concentration of the PE-E (600 mg/kg) showed a significant reduction in MN formation compared to the positive control group (CP) (p < 0.05) and exhibited a linear trend for micronuclei reduction as the dose increases which indicates that PE-E has genoprotective activities in mice of both gender and age groups. The genoprotective effect seems to be both age- and gender-dependent, being more potent among young and female mice, respectively. It should be noted that among the young females, statistically significant genoprotection was apparent even at the lower concentration of 150 mg/kg (Table 1).
Table 1. Number of micronucleated polychromatic erythrocytes (MNPCEs) per 1,000 polychromatic erythrocytes (PCEs) in bone marrow.
The frequency of micronuclei formation in whole blood cells of young mice treated with different concentrations of PE-E was also examined. The differences observed in micronuclei formation were not significant (Supplementary Table 2), mainly due to the high deviations observed between animals of the same group. For that reason, the micronuclei assay in whole blood cells of the older animals was not performed.
In order to investigate the possible involvement of antioxidant or immunoregulatory mechanisms in the genoprotective ability of P. eryngii mushroom extract (PE-E), the mRNA expression levels of the hub genes NrF2 and Nfkβ for the respective processes were quantified in gut and liver tissues. The RNA quantitation analysis was performed only for the female mice, which showed more responsiveness to the PE-E in the micronuclei assay. The groups selected were the control C (H2O), PE-E (600 mg/kg), CP and CP + PE-E (600 mg/kg). Figures 3A,B represent the expression levels of NrF2 and Nfkβ in young and elderly female mice. The effect of PE-E in the expression of NrF2 appears to be both tissue and age-independent (Figure 3A). Administration of the PE-E alone or in combination with CP significantly increased the levels of NrF2 mRNA in both tissues relative to those of the control groups C and CP, respectively. The expression of NrF2 gene was reduced in the gut of CP treated elderly mice (p < 0.05), which however was restored to approximately the control levels in mice of the CP + PE-E group. The effect of PE-E in the expression of Nfkβ appeared to be age-dependent and was more pronounced among the elderly mice (Figure 3B). In young mice, administration of the PE-E alone or in combination with CP did not alter the levels of Nfkβ relative to the control groups, C and CP, respectively. Among the young mice, only the CP-treated group showed in both tissues elevated expression of Nfkβ relative to all the other groups. In elderly female mice, administration of PE-E alone upregulated Nfkβ in the liver, whereas its administration in combination with CP significantly increased the levels of Nfkβ mRNA in both tissues relative to those of the respective control CP-treated groups (Figure 3B).
Figure 3. mRNA expression levels of (A) NrF2 and (B) Nfkβ in gut and liver tissues of young and elderly female mice. C, negative control group treated with water for 14 days; PE-E, Pleurotus eryngii control group treated with 600 mg/kg P. eryngii mushroom extract for 14 days; CP, positive control group treated with water for 14 days and 80 mg/kg cyclophosphamide the 14th day; CP+PE-E, administration of 600 mg/kg P. eryngii mushroom extract for 14 days and 80 mg/kg cyclophosphamide the 14th day. All values are expressed as the mean ± SD of six mice in each group. *p < 0.05 compared to control (water) (t-test).
The ability of the extract to regulate epigenetic mechanisms, such as DNA methylation, was also evaluated by quantification of the mRNA expression levels of the DNMT1 gene, which is responsible for the maintenance and possibly the de novo CpG methylation of transposable elements (38). An age-dependent effect of PE-E treatment was also observed for DNMT1 expression levels with the young animals showing no differences in DNMT1 expression between PE-E treated and relative control groups in both tissues (Figure 4A). Among the young mice only the CP treated group showed elevated expression of DNMT1 in the liver relative to all the other groups. On the contrary, treatments with PE-E alone or in combination with CP caused a significant induction in DNMT1 expression levels in gut and liver tissues of elderly female mice compared to the respective controls (Figure 4B). In addition, as also shown in Figure 4B, PE-E seems to have the ability in the CP + PE-E group of animals to restore the reduced expression levels of DNMT1 in the gut of elderly females treated only with CP.
Figure 4. mRNA expression levels of DNMT1 in gut and liver tissues of (A) young and (B) elderly female mice. C, negative control group treated with water for 14 days; PE-E, Pleurotus eryngii control group treated with 600 mg/kg P. eryngii mushroom extract for 14 days; CP, positive control group treated with water for 14 days and 80 mg/kg cyclophosphamide the 14th day; CP+PE-E, administration of 600 mg/kg P. eryngii mushroom extract for 14 days and 80 mg/kg cyclophosphamide the 14th day. All values are expressed as the mean ± SD of six mice in each group. *p < 0.05 compared to control (water) (t-test).
Pleurotus eryngii mushrooms potential in maintaining cell homeostasis was evaluated by the quantification of IL-22 mRNA expression levels in gut tissues only (Figure 5), since its expression in the liver was not detectable (data not shown). Once more the age effect was prominent with the young animals showing only α minor non-significant increase (p > 0.05) in IL-22 expression in PE-E treated animals relative to the respective controls. On the contrary, treatments with PE-E alone or in combination with CP caused overexpression of IL-22 compared to the control groups in elderly females. The induction in the expression levels of IL-22 in the CP + PE-E relative to the CP group was however not significant (p = 0.150) due to the high interindividual variations in the expression levels among the animals of the same group.
Figure 5. mRNA expression levels of IL-22 in gut tissue of (A) young and (B) elderly female mice. C, negative control group treated with water for 14 days; PE-E, Pleurotus eryngii control group treated with 600 mg/kg P. eryngii mushroom extract for 14 days; CP, positive control group treated with water for 14 days and 80 mg/kg cyclophosphamide the 14th day; CP+PE-E, administration of 600 mg/kg P. eryngii mushroom extract for 14 days and 80 mg/kg cyclophosphamide the 14th day. All values are expressed as the mean ± SD of six mice in each group. *p < 0.05 compared to control (water) (t-test).
Edible mushrooms contain a variety of bioactive compounds, such as polysaccharides, sterols, coumarins, and polyphenols, with numerous biological activities (22, 39, 40). The genoprotective and anticarcinogenic properties of fungal bioactive compounds are frequently attributed to their direct action as radical scavengers or immunomodulators (5, 6, 18). However, over the last years, an indirect mode of action that implicates the gut microbiome as a mediator is gaining considerable ground (19, 20). Although not adequately addressed so far, the mushrooms’ anticancer properties may be due to their fermentation by the human gut microbiota and the metabolites produced in the process (41, 42).
Pleurotus eryngii mushroom powder was fermented in vitro by fecal inocula of healthy elderly volunteers for 24 h. Pre- and post-fermentation supernatants were collected, and their effect on DNA damage induced by the genotoxic agent mitomycin C was assessed by Cytokinesis block micronucleus assay in human lymphocytes. According to our results, even at the low concentrations used (1% v/v), post-fermentation supernatants decrease remarkably (28.1%) the micronuclei frequency in human lymphocytes. The reduction of micronuclei was consistent throughout all the experiments and fecal and blood donor-independent (Supplementary Figure 1). On the contrary, the pre-fermentation supernatants of PE (PE-FS) exhibited a moderate genotoxic effect (13.4%) compared to the NC pre-fermentation supernatants (NC-FS); the effect was fecal and blood donor dependent (Supplementary Figure 2). We have previously demonstrated using the comet assay that post-fermentation supernatants of P. eryngii mushroom exhibit genoprotective effects against the genotoxic agent tert-butyl-hydroperoxide (t-BOOH) in human small intestinal cancer cells (Caco-2) (27). In the same study, we showed that pre-fermentation supernatants of another mushroom, Cyclocybe cylindracea, were significantly genotoxic in Caco-2 cells. At the same time, the respective P. eryngii fermentation supernatant was also moderately but not significantly genotoxic. Therefore, P. eryngii mushrooms may contain water-soluble ingredients that are either genotoxic in vitro or ameliorate the well-known genotoxic activity of the aqueous phase of human stool (43).
Little is known about the protective role of mushrooms on DNA damage, after their fermentation in the gut (22–26), and the main question arising is which metabolites are being associated with the genoprotective effect. During the fermentation process of Pleurotus eryngii mushrooms or their extracts by gut microbiota, short chain fatty acids (SCFAs) such as acetate, propionate and butyrate are produced (44). SCFAs are involved in many biological processes and might be associated with genoprotective properties (23), such as those we observed for fermented P. eryngii. The matched pre- and post-fermentation supernatants (FS) examined in the current study and their respective precipitates were previously examined for alterations in short-chain fatty acids (SCFA) concentration and in fecal microbiota composition respectively, during the 24-h fermentation processes (3, 27). P. eryngii was shown to induce higher SCFA production than similar concentrations (w/v) of the prebiotic polysaccharide inulin (3) possibly because it exhibits a strong lactogenic effect. Furthermore, our preliminary untargeted NMR-based metabolomics analysis of PE-FS showed a remarkable increase of short-chain fatty acids (SCFAs), namely acetate, propionate, butyrate, and formate, and enhanced production of trimethylamine (TMA) as well as of the neurotransmitter gamma-aminobutyric acid (GABA) (27).
T he genoprotective effect of P. eryngii hot water extract (PE-E) was investigated in young (8–9 weeks old) and elderly mice of both sexes in bone marrow cells. The old mice were 17-18 months old, corresponding to 56–60 human years. DNA damage was induced by cyclophosphamide (CP), an alkylating agent, which is metabolized in the liver by cytochromes P450. CP is an established genotoxic agent since it can cause DNA damage when metabolized, leading to chromosome breaks, micronuclei (MN) formation, and cell death (45, 46). In the present work, cyclophosphamide was administered intraperitoneally at a dose of 80 mg/kg. This dosing regimen induces micronuclei formation without causing any tissue damage, as confirmed by histopathological examination of liver and gut tissues. All animal groups treated with PE-E only (highest dose; 600 mg/kg), exhibited micronuclei frequency similar to the controls, indicating that P. eryngii mushroom extract is not genotoxic in vivo. In addition, histopathological examination of randomly selected animals (groups of old mice) did not show significant gut or liver alterations. The small increase in the number of eosinophil leucocytes in the mucosal enteric crypts of the colon and the mild liver sinusoidal/canalicular dilatation observed in some animals could be attributed to their old age. The number of micronuclei after CP administration was lower in aged mice compared to young and in aged females compared to aged male mice. Variations on DNA damage between genders have been previously reported by the Collaborative study group for the micronucleus test (47). According to this study, male CD-1 mice treated with cyclophosphamide were more prone to micronuclei formation compared to females. All groups treated with the highest concentration of the extract (CP + PE-E 600 mg/kg) showed a significant reduction in MN formation compared to the positive control group (CP) (p < 0.05) (Table 1). Furthermore, administration of the P. eryngii extract at doses of 150, 300, and 600 mg/kg/day for 14 days prevented the formation of MN in the bone marrow cells of CP-treated mice of all groups in a dose-dependent manner as the p values for linear trend indicated. Therefore, when administered by gavage in mice, PE-E exerts genoprotective activities in both male and female, young and elderly animals.
Our results are in agreement with two recent studies, where extracts of the mushrooms Phellinus baumii and Pleurotus ostreatus (ethanolic and aqueous extracts, respectively) prevented the formation of MN in the bone marrow cells of CP-treated mice in a dose-dependent manner (39, 48).
Moreover, significant age- and gender-dependent differences in the genoprotection were observed between the groups. PE-E exhibited higher genoprotection in the female and young mice than in male and old, respectively. It has been reported that the response to genotoxic agents is strain-, sex- and tissue-dependent, and the differences observed between males and females are attributed to the differential expression of genes involved in DNA repair mechanisms, such as Mpg, Xrcc1, and Mgmt (49). A similar gender-dependent response to genoprotective agents may exist. Another possible explanation for these differences may be the influence of sex hormones on cytochrome P450 (50).
It is generally known that aging affects various biological functions of the organism, enhancing the accumulation of DNA adducts and the formation of micronuclei. Contrary to the above, our experiments showed a reduction in micronuclei formation in the bone marrow cells of CP-treated elderly mice compared to younger mice. These differences may be due to the liver’s reduced xenobiotic (including CP) metabolizing capacity upon aging (51). We also found that the mushroom extract does not protect older mice from CP induced- DNA damage at the same level as younger mice. This finding was also observed in (1→3) (1→6)-β-D-glucan botryosphaeran treated mice (9) and could be attributed to the decreased gut microbiota diversity and increased immunosenescence that comes with aging (52).
The micronucleus assay is widely used in bone marrow cells and, to a lesser extent in peripheral blood cells (53, 54). Therefore, we also examined the frequency of micronuclei formation in whole blood cells among young mice. Due to the high deviations observed in the micronuclei frequency among the animals of the same group and the non-significant changes (Supplementary Table 2), the experiment was not repeated for the elderly mice groups. It is generally known that polychromatic erythrocytes (PCEs) need more time to appear in the circulating blood compared to the PCEs in the bone marrow. Since animals were killed 24 h after CP administration, an appropriate time point for the evaluation of the CP’s acute effect in bone marrow (55, 56), the frequency of micronucleated polychromatic erythrocytes was low and not sufficient to produce reliable results.
To investigate the mechanisms involved in the genoprotective ability of P. eryngii extract (PE-E), the mRNA expression levels of the genes NrF2 and Nfkβ, DNMT1 and IL-22 were quantified in gut and liver tissues in the female mice, which were shown to be more responsive to the genoprotective activity of PE-E. NrF2 (nuclear factor erythroid 2-related factor 2) is a major transcriptional factor involved in antioxidant response pathways. Upon activation by oxidative or genotoxic stressors, NrF2 accumulates into the nucleus and binds to antioxidant response elements (ARE), thus regulating the expression of antioxidant genes (57). Nfkβ is an essential transcriptional factor in main cellular responses, such as immunity, inflammation, homeostasis, and cell death. During activation by various factors, such as DNA damage agents, Nfkβ translocates to the nucleus, binds to specific gene promoters, and regulates the expression of hundreds of genes (58). DNMT1 is one of the hub genes that orchestrate one of the most important epigenetic modifications, CpG DNA methylation, which plays key roles in regulating gene expression, genomic imprinting, X chromosome inactivation, and tumorigenesis. DNMT1 gene is responsible for the maintenance of CpG methylation across the genome and possibly the de novo CpG methylation of transposable elements (38). IL-22 is a member of the IL-10 family of cytokines. It is produced mainly by T cell subsets and type 3 innate lymphoid cells and is an important regulator of the immune response, protection of intestinal barrier, and maintenance of gut homeostasis and microbial composition (59).
The effect of administration of PE-E alone or combined with CP on the expression of the genes mentioned above, NrF2 exempted, was age-dependent. The effect was significant only among the elderly mice. This was not surprising since, upon external stimuli, an age-dependent differential gene expression response is commonly observed in both animal and human studies (60–62). Furthermore, differential age-related mRNAs expression was also observed despite the similar micronuclei frequency observed in both age groups treated with CP and 600 mg/kg PE-E. Similarly, Thalacker-Mercer et al. (63) reported differential genomic responses in old vs. young humans despite similar levels of modest muscle damage after resistance loading.
Pre-treatment with PE-E alone or combined with CP caused a remarkable induction in NrF2 expression levels in both tissues of young and elderly female mice compared to the respective control groups (water and CP) (Figure 3A). In addition, NrF2 expression after CP administration was significantly reduced in the gut of elderly animals. However, it was restored to the control levels in the animals in which CP was co-administered with PE-E. Although our results are restricted only at the gene expression level, they support previous studies, which showed that mushroom extracts possess strong antioxidant properties through their ability to regulate the NrF2 signaling pathway and to ameliorate the oxidative effects caused by CP administration by regulating the transcription of glutathione peroxidase (GSH-Px), nicotinamide adenine dinucleotide phosphate [NAD(P)H] quinine oxidoreductase-1 (NQO-1), heme oxygenase-1 (HO-1), superoxide dismutase 2 (SOD2), and catalase (CAT) antioxidant enzymes (64, 65).
In elderly mice, administration of the PE-E alone upregulated Nfkβ expression in the liver, whereas its administration in combination with CP significantly increased the levels of Nfkβ in both tissues relative to those of the CP treated control groups (Figure 3B). According to previous studies, various hot water extracts of mushrooms possess immunomodulatory properties through the stimulation of immune cells, such as macrophages (66–70), dendritic (71) or B cells (72) via activation of toll-like receptor 4 (TLR4), mitogen activated protein kinase (MAPK) and Nfkβ signaling pathways. Our results indicate that P. eryngii could probably modulate the expression of genes involved in immune regulation through the activation of Nfkβ signaling pathway, which is in agreement with very recent findings by our group. We reported that the in vitro fermentation of P. eryngii mushrooms by fecal microbiota of healthy elderly volunteers clearly affected immune response, as indicated by the altered gene expression and secretion levels of pro- (TNF-α, IL-1β) and anti-inflammatory cytokines (IL-10, IL-1Rα) in human macrophages while fermentation supernatants induced immunophenotypic changes in T helper 2 (Th2) and myeloid lineage cells in their peripheral blood mononuclear cells (PBMCs) (73).
Treatments with PE-E alone or in combination with CP caused a significant induction in DNMT1 expression levels in gut and liver tissues of elderly female mice compared to the respective controls (C and CP) (Figure 4B). It has recently been shown that alterations in gut microbiota and its metabolites’ composition may affect the epigenetic mechanisms of gene expression. Gut microbiota has recently been identified as a critical mediator of the intestinal inflammatory response through epigenetic reprograming (74). It was also shown that SCFAs produced by gut microbiota regulate endothelial function and reduce inflammation by inhibiting the histone deacetylases (HDACs), a key epigenetic enzyme (75, 76). The current study, to the best of our knowledge, is the first study showing that ingestion of a mushroom extract causes alterations in DNMT1 expression in mice. Furthermore, it is worth noting that in our study PE-E, when co-administered with CP, seem to have the ability to restore the reduced expression levels of DNMT1 in the gut of CP-treated elderly animals. The only partially relevant work is that of Sharma and Tollefsbol (77) where a mixture of two dietary phytochemicals (Sulforaphane and Genistein) and butyrate inhibits DNMT1 expression in MDA-MB-231 and MCF-7 breast cancer cells.
In the present work, treatments of elderly animals with PE-E alone or in combination with CP caused overexpression of IL-22 in the gut compared to the respective control groups (Figure 5). Recent studies have revealed that upon exposure to genotoxic agents, IL-22 promotes the activation of Ataxia Telangiectasia Mutated pathway (ATM) and the initiation of DNA damage response (DDR) in colon stem cells. In addition, it has been shown that innate lymphocytes regulate the expression of IL-22 and, consequently, the activation of DDR through the AhR expression in response to genotoxic dietary factors (78). Butyrate promotes IL-22 production, enhancing butyrate’s protective effect in intestinal inflammation in mice (79). Considering the aforementioned, the induction of IL-22 expression in our experiments probably promotes the activation of the DDR, which is highly upregulated in the CP + PE-E groups, resulting in the inhibition of the DNA damage caused by CP and the reduction in MN formation. These effects could be associated with the production of SCFAs, since their production affects T cell populations (80), and consequently, IL-22 levels.
The current study focused on exploring the genotoxic/genoprotective properties of the mushroom P. eryngii. Our results indicate that P. eryngii, fermented by fecal inocula in vitro or ingested in vivo, exerts genoprotective properties against the genotoxic insult of well-known DNA damaging agents. In order to elucidate the role of the microbiome and its metabolome in the observed genoprotection of fermented PE, extensive 16S Next Generation Sequencing (NGS) metagenomic analysis and full-scale NMR-based metabolomics analysis in both the in vitro and in vivo experiments are currently underway. The quantification of mRNA expression of the hub genes NrF2, Nfkβ, DNMT1, and IL-22 in mice gut and liver tissues may suggest possible mechanisms for the observed genoprotection via modulation of the antioxidant and immunosurveillance responses of the cells, alterations in epigenetic mechanisms, maintenance of cell homeostasis, and promotion of the DNA damage response pathways.
The original contributions presented in this study are included in the article/Supplementary material, further inquiries can be directed to the corresponding author.
The studies involving human participants were reviewed and approved by Bioethics Committee of Harokopio University, Athens, Greece (62-03/07/2018) and by NHRF’s Research Ethics Committee. The patients/participants provided their written informed consent to participate in this study. The animal study was reviewed and approved by the Veterinary Office of Attica Prefecture (82837-30/01/2020) and the 7/15-06-2022 NHRF’s Research Ethics Committee.
AB contributed to the conceptualization, data curation, methodology, validation, formal analysis, and writing the original draft. PM, G-MS, ES, CV, GS, EM, GK, GZ, and AK contributed to the formal analysis, data curation, and methodology. VP contributed to the conceptualization, funding acquisition, project administration, and reviewing and editing the manuscript. PG contributed to the conceptualization, methodology, supervision, investigation, validation, and writing, reviewing, and editing the manuscript. All authors contributed to the article and approved the submitted version.
This research has been co-financed by the European Union and Greek national funds through the Operational Program Competitiveness, Entrepreneurship and Innovation, under the call RESEARCH–CREATE–INNOVATE (project code: T1EDK-03404).
We would like to thank Margarita Bekyrou for technical assistance and proofreading the manuscript. We also thank Marigoula Vlassopoulou, Paraschos Christodoulou, and Sofia Vidaly for their contribution in animal Unit during sacrifices and tissues removal. We would also like to acknowledge Efthymia Kitraki for the hospitality in her laboratory and the guidance in preparing the tissue samples for the histopathological analysis.
The authors declare that the research was conducted in the absence of any commercial or financial relationships that could be construed as a potential conflict of interest.
All claims expressed in this article are solely those of the authors and do not necessarily represent those of their affiliated organizations, or those of the publisher, the editors and the reviewers. Any product that may be evaluated in this article, or claim that may be made by its manufacturer, is not guaranteed or endorsed by the publisher.
The Supplementary Material for this article can be found online at: https://www.frontiersin.org/articles/10.3389/fnut.2022.988517/full#supplementary-material
1. Valverde ME, Hernández-Pérez T, Paredes-López O. Edible mushrooms: improving human health and promoting quality life. Int J Microbiol. (2015) 2015:376387. doi: 10.1155/2015/376387
2. Yadav D, Negi PS. Bioactive components of mushrooms: processing effects and health benefits. Food Res Int. (2021) 148:110599. doi: 10.1016/j.foodres.2021.110599
3. Mitsou EK, Saxami G, Stamoulou E, Kerezoudi E, Terzi E, Koutrotsios G, et al. Effects of rich in β-glucans edible mushrooms on aging gut microbiota characteristics: an in vitro study. Molecules. (2020) 25:2806. doi: 10.3390/molecules25122806
4. Barbosa AM, Steluti RM, Dekker RF, Cardoso MS, Corradi da Silva ML. Structural characterization of botryosphaeran: a (1–>3;1–>6)-beta-D-glucan produced by the ascomyceteous fungus, Botryosphaeria sp. Carbohydr Res. (2003) 338:1691–8. doi: 10.1016/s0008-6215(03)00240-4
5. Kosanic M, Petrović N, Milosevic-Djordjevic O, Grujičić D, Tubic J, Marković A, et al. The health promoting effects of the fruiting bodies extract of the peppery milk cap mushroom Lactarius piperatus (Agaricomycetes) from Serbia. Int J Med Mushrooms. (2020) 22:347–57. doi: 10.1615/IntJMedMushrooms.2020034167
6. Živković L, Bajić V, Bruić M, Borozan S, Popić K, Topalović D, et al. Antigenotoxic and antioxidant potential of medicinal mushrooms (Immune Assist) against DNA damage induced by free radicals-an in vitro study. Mutat Res Genet Toxicol Environ Mutagen. (2019) 845:403078. doi: 10.1016/j.mrgentox.2019.06.008
7. Al-Habib MN, Holliday J, Aladahmy MS. Psathyrella candolleana and Agaricus bisporus extracts provide protection against DNA oxidative damage induced by doxorubicin. Int J Med Mushrooms. (2018) 20:749–59. doi: 10.1615/IntJMedMushrooms.2018027002
8. Knežević A, Stajić M, Živković L, Milovanović I, Spremo-Potparević B, Vukojević J. Antifungal, antioxidative, and genoprotective properties of extracts from the blushing bracket mushroom, Daedaleopsis confragosa (Agaricomycetes). Int J Med Mushrooms. (2017) 19:509–20. doi: 10.1615/IntJMedMushrooms.v19.i6.30
9. Silva-Sena GG, Malini M, Delarmelina JM, Dutra JCV, Gervásio SV, Leal MAS, et al. In vivo antimutagenic and antiatherogenic effects of the (1→3)(1→6)-β-d- glucan botryosphaeran. Mutat Res Genet Toxicol Environ Mutagen. (2018) 826:6–14. doi: 10.1016/j.mrgentox.2017.12.008
10. Eid JI, Al-Tuwaijri MM, Mohanty S, Das B. Chaga mushroom (Inonotus obliquus) polysaccharides exhibit genoprotective effects in UVB-exposed embryonic zebrafish (Danio rerio) through coordinated expression of DNA repair genes. Heliyon. (2021) 7:e06003. doi: 10.1016/j.heliyon.2021.e06003
11. Khatua S, Gupta SS, Ghosh M, Tripathi S, Acharya K. Exploration of nutritional, antioxidative, antibacterial and anticancer status of Russula alatoreticula: towards valorization of a traditionally preferred unique myco-food. J Food Sci Technol. (2021) 58:2133–47. doi: 10.1007/s13197-020-04723-9
12. Finimundy TC, Abreu RMV, Bonetto N, Scariot FJ, Dillon AJP, Echeverrigaray S, et al. Apoptosis induction by Pleurotus sajor-caju (Fr.) Singer extracts on colorectal cancer cell lines. Food Chem Toxicol. (2018) 112:383–92. doi: 10.1016/j.fct.2018.01.015
13. Finimundy TC, Gambato G, Fontana R, Camassola M, Salvador M, Moura S, et al. Aqueous extracts of Lentinula edodes and Pleurotus sajor-caju exhibit high antioxidant capability and promising in vitro antitumor activity. Nutr Res. (2013) 33:76–84. doi: 10.1016/j.nutres.2012.11.005
14. Ozmen A, Degirmenci EH. In vitro anticancer and apoptotic activity of edible mushroom Lepista nuda (Bull.) Cooke on leukemia and breast cancer compared with protocatechuic acid, paclitaxel and doxorubicin. Indian J Exp Biol. (2021) 59:147–52.
15. Awadasseid A, Hou J, Gamallat Y, Xueqi S, Eugene KD, Musa Hago A, et al. Purification, characterization, and antitumor activity of a novel glucan from the fruiting bodies of Coriolus Versicolor. PLoS One. (2017) 12:e0171270. doi: 10.1371/journal.pone.0171270
16. Pandya U, Dhuldhaj U, Sahay NS. Bioactive mushroom polysaccharides as antitumor: an overview. Nat Prod Res. (2019) 33:2668–80. doi: 10.1080/14786419.2018.1466129
17. Roda E, Luca F, Iorio CD, Ratto D, Siciliani S, Ferrari B, et al. Novel medicinal mushroom blend as a promising supplement in integrative oncology: a multi-tiered study using 4T1 triple-negative mouse breast cancer model. Int J Mol Sci. (2020) 21:3479. doi: 10.3390/ijms21103479
18. Wang XE, Wang YH, Zhou Q, Peng M, Zhang J, Chen M, et al. Immunomodulatory effect of lentinan on aberrant T subsets and cytokines profile in non-small cell lung cancer patients. Pathol Oncol Res. (2020) 26:499–505. doi: 10.1007/s12253-018-0545-y
19. Pickard JM, Zeng MY, Caruso R, Núñez G. Gut microbiota: role in pathogen colonization, immune responses, and inflammatory disease. Immunol Rev. (2017) 279:70–89. doi: 10.1111/imr.12567
20. Armstrong H, Bording-Jorgensen M, Dijk S, Wine E. The complex interplay between chronic inflammation, the microbiome, and cancer: understanding disease progression and what we can do to prevent It. Cancers. (2018) 10:83. doi: 10.3390/cancers10030083
21. Vivarelli S, Salemi R, Candido S, Falzone L, Santagati M, Stefani S, et al. Gut microbiota and cancer: from pathogenesis to therapy. Cancers. (2019) 11:38. doi: 10.3390/cancers11010038
22. Nowak A, Śiżewska K, Otlewska A. Antigenotoxic activity of lactic acid bacteria, prebiotics, and products of their fermentation against selected mutagens. Regul Toxicol Pharmacol. (2015) 73:938–46. doi: 10.1016/j.yrtph.2015.09.021
23. Christophersen CT, Petersen A, Licht TR, Conlon MA. Xylo-oligosaccharides and inulin affect genotoxicity and bacterial populations differently in a human colonic simulator challenged with soy protein. Nutrients. (2013) 5:3740–56. doi: 10.3390/nu5093740
24. Allsopp P, Possemiers S, Campbell D, Oyarzábal IS, Gill C, Rowland I. An exploratory study into the putative prebiotic activity of fructans isolated from Agave angustifolia and the associated anticancer activity. Anaerobe. (2013) 22:38–44. doi: 10.1016/j.anaerobe.2013.05.006
25. Burns AJ, Rowland IR. Antigenotoxicity of probiotics and prebiotics on faecal water-induced DNA damage in human colon adenocarcinoma cells. Mutat Res. (2004) 551:233–43. doi: 10.1016/j.mrfmmm.2004.03.010
26. Munjal U, Scharlau D, Glei M. Gut fermentation products of inulin-type fructans modulate the expression of xenobiotic-metabolising enzymes in human colonic tumor cells. Anticancer Res. (2012) 32:5379–86.
27. Boulaka A, Christodoulou P, Vlassopoulou M, Koutrotsios G, Bekiaris G, Zervakis GI, et al. Genoprotective properties and metabolites of β-glucan-rich edible mushrooms following their in vitro fermentation by human faecal microbiota. Molecules. (2020) 25:3554. doi: 10.3390/molecules25153554
28. Nagpal R, Mainali R, Ahmadi S, Wang S, Singh R, Kavanagh K, et al. Gut microbiome and aging: physiological and mechanistic insights. Nutr Healthy Aging. (2018) 4:267–85. doi: 10.3233/NHA-170030
29. O’Toole PW, Jeffery IB. Gut microbiota and aging. Science. (2015) 350:1214–5. doi: 10.1126/science.aac8469
30. Van Herck Y, Feyaerts A, Alibhai S, Papamichael D, Decoster L, Lambrechts Y, et al. Is cancer biology different in older patients? Lancet Healthy longev. (2021) 2:e663–77. doi: 10.1016/S2666-7568(21)00179-3
31. Bonassi S, El-Zein R, Bolognesi C, Fenech M. Micronuclei frequency in peripheral blood lymphocytes and cancer risk: evidence from human studies. Mutagenesis. (2011) 26:93–100. doi: 10.1093/mutage/geq075
32. Kwon M, Leibowitz ML, Lee JH. Small but mighty: the causes and consequences of micronucleus rupture. Exp Mol Med. (2020) 52:1777–86. doi: 10.1038/s12276-020-00529-z
33. Fenech M. Cytokinesis-block micronucleus cytome assay. Nat Protoc. (2007) 2:1084–104. doi: 10.1038/nprot.2007.77
34. OECD. Mammalian Erythrocyte Micronucleus Test. OECD Guidelines for the Testing of Chemicals, Section 4. Paris: OECD Publishing (2016). doi: 10.1787/9789264264762-en
36. Hayashi M, MacGregor JT, Gatehouse DG, Adler ID, Blakey DH, Dertinger SD, et al. In vivo rodent erythrocyte micronucleus assay. II. Some aspects of protocol design including repeated treatments, integration with toxicity testing, and automated scoring. Environ Mol Mutagen. (2000) 35:234–52.
37. Schmittgen TD, Livak KJ. Analyzing real-time PCR data by the comparative C(T) method. Nat Protoc. (2008) 3:1101–8. doi: 10.1038/nprot.2008.73
38. Haggerty C, Kretzmer H, Riemenschneider C, Kumar AS, Mattei AL, Bailly N, et al. Dnmt1 has de novo activity targeted to transposable elements. Nat Struct Mol Biol. (2021) 28:594–603. doi: 10.1038/s41594-021-00603-8
39. Yoo JH, Lee YS, Ku S, Lee HJ. Phellinus baumii enhances the immune response in cyclophosphamide-induced immunosuppressed mice. Nutr Res. (2020) 75:15–31. doi: 10.1016/j.nutres.2019.12.005
40. Elhusseiny SM, El-Mahdy TS, Awad MF, Elleboudy NS, Farag MMS, Yassein MA, et al. Proteome analysis and in vitro antiviral, anticancer and antioxidant capacities of the aqueous extracts of Lentinula edodes and Pleurotus ostreatus edible mushrooms. Molecules. (2021) 26:4623. doi: 10.3390/molecules26154623
41. Su J, Su L, Li D, Shuai O, Zhang Y, Liang H, et al. Antitumor activity of extract from the sporoderm-breaking spore of Ganoderma lucidum: restoration on exhausted cytotoxic T cell with gut microbiota remodeling. Front Immunol. (2018) 9:1765. doi: 10.3389/fimmu.2018.01765
42. Khan I, Huang G, Li XA, Liao W, Leong WK, Xia W, et al. Mushroom polysaccharides and jiaogulan saponins exert cancer preventive effects by shaping the gut microbiota and microenvironment in ApcMin/+ mice. Pharmacol Res. (2019) 148:104448. doi: 10.1016/j.phrs.2019.104448
43. Turunen KT, Pletsa V, Georgiadis P, Triantafillidis JK, Karamanolis D, Kyriacou A. Impact of β-glucan on the fecal water genotoxicity of polypectomized patients. Nutr Cancer. (2016) 68:560–7. doi: 10.1080/01635581.2016.1156713
44. Ma G, Xu Q, Du H, Muinde Kimatu B, Su A, Yang W, et al. Characterization of polysaccharide from Pleurotus eryngii during simulated gastrointestinal digestion and fermentation. Food Chem. (2022) 370:131303. doi: 10.1016/j.foodchem.2021.131303
45. Mahmoud AM, Germoush MO, Alotaibi MF, Hussein OE. Possible involvement of Nrf2 and PPARγ up-regulation in the protective effect of umbelliferone against cyclophosphamide-induced hepatotoxicity. Biomed Pharmacother. (2017) 86:297–306. doi: 10.1016/j.biopha.2016.12.047
46. El-Sheikh AA, Morsy MA, Okasha AM. Inhibition of NF-κB/TNF-α pathway may be involved in the protective effect of resveratrol against cyclophosphamide-induced multi-organ toxicity. Immunopharmacol Immunotoxicol. (2017) 39:180–7. doi: 10.1080/08923973.2017.1318913
47. The Collaborative Study Group for the Micronucleus Test. Sex difference in the micronucleus test. Mutat Res. (1986) 172:151–63.
48. Farhan EM, Chechan RA. Evaluating the ability of Pleurotus ostreatus aqueous extract to modulate genotoxicity induced by cyclophosphamide in mice bone marrow cells. Iraqi J Agric Sci. (2020) 51:1405–12. doi: 10.36103/ijas.v51i5.1150
49. Lewis L, Chappell GA, Kobets T, O’Brian BE, Sangaraju D, Kosyk O, et al. Sex-specific differences in genotoxic and epigenetic effects of 1,3-butadiene among mouse tissues. Arch Toxicol. (2019) 93:791–800. doi: 10.1007/s00204-018-2374-x
50. Fortoul TI, Moncada-Hernández S, Saldivar-Osorio L, Espejel-Maya G, Mussali-Galante P, del Carmen Avila-Casado M, et al. Sex differences in bronchiolar epithelium response after the inhalation of lead acetate (Pb). Toxicology. (2005) 207:323–30. doi: 10.1016/j.tox.2004.10.004
51. Schmucker DL. Age-related changes in liver structure and function: implications for disease ? Exp Gerontol. (2005) 40:650–9. doi: 10.1016/j.exger.2005.06.009
52. Lee KA, Luong MK, Shaw H, Nathan P, Bataille V, Spector TD. The gut microbiome: what the oncologist ought to know. Br J Cancer. (2021) 125:1197–209. doi: 10.1038/s41416-021-01467-x
53. Kim NS, Shin S, Shin GG, Bang OS. Genotoxicity evaluation of a Phragmitis rhizoma extract using a standard battery of in vitro and in vivo assays. J Ethnopharmacol. (2019) 241:112025. doi: 10.1016/j.jep.2019.112025
54. Salmani A, Kosari AA, Pirouzi A, Omidi M, Mohsenzadeh M. Protective effect of methanolic extracts of Thymus vulgaris L. against cyclophosphamide-induced DNA damage in mouse bone marrow cells using the micronucleus test. Trends Pharmacol Sci. (2015) 1:243–50.
55. Hatanaka Y, Kitagawa Y, Toyoda Y, Kawata T, Ando N, Kawabata Y, et al. Micronucleus test with cyclophosphamide using mouse peripheral blood reticulocytes. Mutat Res. (1992) 278:99–101. doi: 10.1016/0165-1218(92)90216-m
56. Delmanto RD, de Lima PL, Sugui MM, da Eira AF, Salvadori DM, Speit G, et al. Antimutagenic effect of Agaricus blazei Murrill mushroom on the genotoxicity induced by cyclophosphamide. Mutat Res. (2001) 496:15–21. doi: 10.1016/s1383-5718(01)00228-5
57. He F, Ru X, Wen T. NRF2, a transcription factor for stress response and beyond. Int J Mol Sci. (2020) 21:4777. doi: 10.3390/ijms21134777
58. Wang W, Mani AM, Wu ZH. DNA damage-induced nuclear factor-kappa B activation and its roles in cancer progression. J Cancer Metastasis Treat. (2017) 3:45–59. doi: 10.20517/2394-4722.2017.03
59. Keir M, Yi Y, Lu T, Ghilardi N. The role of IL-22 in intestinal health and disease. J Exp Med. (2020) 217:e20192195. doi: 10.1084/jem.20192195
60. Yoshii H, Tatsumi S, Matsuoka T, Suehiro S, Konishi J, Yanagihara Y, et al. Immunopharmacological actions of an extract isolated from inflamed skin of rabbits inoculated with Vaccinia virus (Neurotropin)–II. Restorative effect on immune responses through the recovery of IL-2 production in aging mice. Int J Immunopharmacol. (1989) 11:543–50. doi: 10.1016/0192-0561(89)90184-7
61. Van Bussel IP, Jolink-Stoppelenburg A, De Groot CP, Müller MR, Afman LA. Differences in genome-wide gene expression response in peripheral blood mononuclear cells between young and old men upon caloric restriction. Genes Nutr. (2016) 11:13. doi: 10.1186/s12263-016-0528-0
62. Broustas CG, Duval AJ, Amundson SA. Impact of aging on gene expression response to x-ray irradiation using mouse blood. Sci Rep. (2021) 11:10177. doi: 10.1038/s41598-021-89682-7
63. Thalacker-Mercer AE, Dell’Italia LJ, Cui X, Cross JM, Bamman MM. Differential genomic responses in old vs. young humans despite similar levels of modest muscle damage after resistance loading. Physiol Genomics. (2010) 40:141–9. doi: 10.1152/physiolgenomics.00151.2009
64. Lin S, Hao G, Long M, Lai F, Li Q, Xiong Y, et al. Oyster (Ostrea plicatula Gmelin) polysaccharides intervention ameliorates cyclophosphamide-Induced genotoxicity and hepatotoxicity in mice via the Nrf2-ARE pathway. Biomed Pharmacother. (2017) 95:1067–71. doi: 10.1016/j.biopha.2017.08.058
65. Liu Y, Yang A, Qu Y, Wang Z, Zhang Y, Liu Y, et al. Ameliorative effects of Antrodia cinnamomea polysaccharides against cyclophosphamide-induced immunosuppression related to Nrf2/HO-1 signaling in BALB/c mice. Int J Biol Macromol. (2018) 116:8–15. doi: 10.1016/j.ijbiomac.2018.04.178
66. Abdullah N, Abdulghani R, Ismail SM, Abidin MH. Immune-stimulatory potential of hot water extracts of selected edible mushrooms. Food Agric Immunol. (2017) 28:374–87. doi: 10.1080/09540105.2017.1293011
67. Hu Q, Du H, Ma G, Pei F, Ma N, Yuan B, et al. Purification, identification and functional characterization of an immunomodulatory protein from Pleurotus eryngii. Food Funct. (2018) 9:3764–75. doi: 10.1039/c8fo00604k
68. Guo M, Meng M, Zhao J, Wang X, Wang C. Immunomodulatory effects of the polysaccharide from Craterellus cornucopioides via activating the TLR4-NFκB signaling pathway in peritoneal macrophages of BALB/c mice. Int J Biol Macromol. (2020) 160:871–9. doi: 10.1016/j.ijbiomac.2020.05.270
69. Yuan Q, Zhang X, Ma M, Long T, Xiao C, Zhang J, et al. Immunoenhancing glucuronoxylomannan from Tremella aurantialba Bandoni et Zang and its low-molecular-weight fractions by radical depolymerization: properties, structures and effects on macrophages. Carbohydr Polym. (2020) 238:116184. doi: 10.1016/j.carbpol.2020.116184
70. Ma G, Kimatu BM, Yang W, Pei F, Zhao L, Du H, et al. Preparation of newly identified polysaccharide from Pleurotus eryngii and its anti-inflammation activities potential. J Food Sci. (2020) 85:2822–31. doi: 10.1111/1750-3841.15375
71. Lin YL, Liang YC, Tseng YS, Huang HY, Chou SY, Hseu RS, et al. An immunomodulatory protein, Ling Zhi-8, induced activation and maturation of human monocyte-derived dendritic cells by the NF-kappaB and MAPK pathways. J Leukoc Biol. (2009) 86:877–89. doi: 10.1189/jlb.0708441
72. Yang SF, Zhuang TF, Si YM, Qi KY, Zhao J. Coriolus versicolor mushroom polysaccharides exert immunoregulatory effects on mouse B cells via membrane Ig and TLR-4 to activate the MAPK and NF-κB signaling pathways. Mol Immunol. (2015) 64:144–51. doi: 10.1016/j.molimm.2014.11.007
73. Vlassopoulou M, Paschalidis N, Savvides AL, Saxami G, Mitsou EK, Kerezoudi EN, et al. Immunomodulating activity of Pleurotus eryngii mushrooms following their in vitro fermentation by human fecal microbiota. J Fungi. (2022) 8:329. doi: 10.3390/jof8040329
74. Ansari I, Raddatz G, Gutekunst J, Ridnik M, Cohen D, Abu-Remaileh M, et al. The microbiota programs DNA methylation to control intestinal homeostasis and inflammation. Nat Microbiol. (2020) 5:610–9. doi: 10.1038/s41564-019-0659-3
75. Vinolo MA, Rodrigues HG, Nachbar RT, Curi R. Regulation of inflammation by short chain fatty acids. Nutrients. (2011) 3:858–76. doi: 10.3390/nu3100858
76. Li M, van Esch BCAM, Henricks PAJ, Folkerts G, Garssen J. The anti-inflammatory effects of short chain fatty acids on lipopolysaccharide- or Tumor Necrosis Factor α-stimulated endothelial cells via activation of GPR41/43 and Inhibition of HDACs. Front Pharmacol. (2018) 9:533. doi: 10.3389/fphar.2018.00533
77. Sharma M, Tollefsbol TO. Combinatorial epigenetic mechanisms of sulforaphane, genistein and sodium butyrate in breast cancer inhibition. Exp Cell Res. (2022) 416:113160. doi: 10.1016/j.yexcr.2022.113160
78. Gronke K, Hernández PP, Zimmermann J, Klose CSN, Kofoed-Branzk M, Guendel F, et al. Interleukin-22 protects intestinal stem cells against genotoxic stress. Nature. (2019) 566:249–53. doi: 10.1038/s41586-019-0899-7
79. Yang W, Yu T, Huang X, Bilotta AJ, Xu L, Lu Y, et al. Intestinal microbiota-derived short-chain fatty acids regulation of immune cell IL-22 production and gut immunity. Nat Commun. (2020) 11:4457. doi: 10.1038/s41467-020-18262-6
Keywords: mushroom, genoprotection, anticancer, CpG methylation, immunomodulation, antioxidative mechanisms, DNA damage response, fecal microbiota
Citation: Boulaka A, Mantellou P, Stanc G-M, Souka E, Valavanis C, Saxami G, Mitsou E, Koutrotsios G, Zervakis GI, Kyriacou A, Pletsa V and Georgiadis P (2022) Genoprotective activity of the Pleurotus eryngii mushrooms following their in vitro and in vivo fermentation by fecal microbiota. Front. Nutr. 9:988517. doi: 10.3389/fnut.2022.988517
Received: 07 July 2022; Accepted: 08 August 2022;
Published: 23 August 2022.
Edited by:
Phisit Seesuriyachan, Chiang Mai University, ThailandReviewed by:
Utoomporn Surayot, Chiang Mai University, ThailandCopyright © 2022 Boulaka, Mantellou, Stanc, Souka, Valavanis, Saxami, Mitsou, Koutrotsios, Zervakis, Kyriacou, Pletsa and Georgiadis. This is an open-access article distributed under the terms of the Creative Commons Attribution License (CC BY). The use, distribution or reproduction in other forums is permitted, provided the original author(s) and the copyright owner(s) are credited and that the original publication in this journal is cited, in accordance with accepted academic practice. No use, distribution or reproduction is permitted which does not comply with these terms.
*Correspondence: Panagiotis Georgiadis, cGFub3NnQGVpZS5ncg==
Disclaimer: All claims expressed in this article are solely those of the authors and do not necessarily represent those of their affiliated organizations, or those of the publisher, the editors and the reviewers. Any product that may be evaluated in this article or claim that may be made by its manufacturer is not guaranteed or endorsed by the publisher.
Research integrity at Frontiers
Learn more about the work of our research integrity team to safeguard the quality of each article we publish.