- 1Department of Cardiology, Shenzhen Bao’an Chinese Medicine Hospital, Guangzhou University of Chinese Medicine, Shenzhen, China
- 2Department of Cardiology, The Eighth Affiliated Hospital, Sun Yat-sen University, Shenzhen, China
- 3Department of Obstetrics, Shenzhen Bao’an Chinese Medicine Hospital, Guangzhou University of Chinese Medicine, Shenzhen, China
- 4Wenhua Community Health Service Center, Shenzhen Luohu Hospital Group, Shenzhen, China
Inflammatory immune response plays a key role in exercise-induced injury and healing; however, the relevant regulatory mechanisms of immune infiltration in exercise-induced injuries remain less studied. In the present study, a highly efficient system for screening immunity-related biomarkers and immunomodulatory ability of natural nutritional supplements was developed by integrating intelligent data acquisition, data mining, network pharmacology, and computer-assisted target fishing. The findings demonstrated that resting natural killer cells showed a higher rate of infiltration after exercise, whereas naive B cells and activated dendritic cells showed higher rate of infiltration before exercise. Four key genes, namely PRF1, GZMB, CCL4, and FASLG, were associated with exercise-induced injuries and inflammatory immune response. In total, 26 natural compounds including echinacoside, eugenol, tocopherol, and casuariin were predicted by using the HERB databases. Molecular docking analysis showed that GZMB, FASLG, and CCL4 bound to echinacoside. In vivo experiments in mice showed that after 30 min swimming, natural killer (NK) cells showed high infiltration rates, and the key genes (GZMB, PRF1, FASLG, and CCL4) were highly expressed; however, echinocandin significantly reduced the level of NK cells and decreased the expression of the four key genes post exercise. This natural nutritional supplement may act to protect against inflammatory injury after exercise by suppressing specific immune infiltration.
Introduction
Exercise and physical activity are effective in the prevention and treatment of a wide range of chronic diseases, and there is considerable evidence for a positive association between long-term exercise and health benefits (1). Physical activity lowers diabetic blood sugar levels (2) and blood pressure (3), reduces the risk of acute cardiovascular disease (4, 5) malignancies such as breast and colon cancer (6), prevents memory and cognitive impairment (7), and enhances immunity to pathogens (8). The Physical Activity Guidelines for Americans (9) recommend that children and adolescents should engage in moderate-to-vigorous physical activity for 60 min or more per day, and adults should engage in at least 150 min of moderate-intensity aerobic activity or at least 75 min of vigorous aerobic activity per week. However, injuries are an inevitable part of exercise and participation in sport; they affect continuation of the sport activity, and the inflammatory response caused by the injury is detrimental to health and may affect athletes’ ability and performance. Thus, the prevention and treatment of sports-related injuries is particularly important. Inflammation is prevalent in the body after exercise; under normal circumstances, this short-term immune response facilitates the removal of necrotic damaged tissue to achieve renewal and repair (10, 11). Inflammation is also one of the main causes of pain and discomfort from post-exercise injuries, and non-steroidal anti-inflammatory drugs (NSAIDs) are used in certain high-intensity competitive sports (12). Delayed-onset muscle soreness is a common negative manifestation of post-exercise discomfort in the body, which was thought to be the result of a combination of factors such as connective tissue damage, muscle damage, lactic acid accumulation, and inflammatory response (13). Chronic inflammation caused by frequent sports-related injuries may place a greater burden on the body than the benefits of exercise. A survey of college athletes showed that 94% had used NSAIDs and 13.9% had overdosed on NSAIDs (14). However, NSAIDs have side effects such as gastrointestinal bleeding, kidney damage, and even inhibition of cartilage proliferation (15). Nutritional therapy is an important strategy to repair sports injuries; it is more widely available than conventional medical care and results in significant cost savings (16). In addition, dietary supplements that relieve pain and discomfort after sports injuries and promote injury repair have fewer side effects than, for example, NSAIDs, and are more effective in improving physical fitness (17). Natural ingredients, such as curcumin, quercetin, and resveratrol, are a great treasure trove and have been found to be effective for sports injuries (18–20). Our previous studies have found that Rhodiola rosea as a natural supplement has a positive effect on exercise capacity and performance, reduces post-exercise pain and skeletal muscle injury, and enhances antioxidant capacity (21). Therefore, we aimed to identify more natural nutritional supplements that are effective in the prevention of and recovery from sports-related injuries. We hope to find some key genes from which to predict some potential natural ingredients that exert anti-inflammatory effects in sports injuries, and use experiments to validate the anti-inflammatory effects of these candidate ingredients, and eventually convert these candidates into natural dietary supplements for use in the anti-inflammatory response to sports injuries.
Materials and methods
Gene expression profiles before and after exercise
Gene expression profiles of individuals before and after exercise was obtained by searching the GEO database; gene IDs were collected and then converted into gene symbols.
Analysis of immune cell infiltration and differentially expressed genes
The CIBERSORT deconvolution method (perm = 1000) was used to analyze immune cell infiltration. The gene expression profiles were screened for differentially expressed genes (DEGs) after normalization using the R limma package based on the cut-off criteria | logFC| ≥ 1 and adjP ≤ 0.05 (22).
Immune-related differentially expressed genes
In addition to immune cell infiltration analysis, we also studied the differential expression of immune-related genes before and after exercise. Immune-related genes were downloaded from the ImmPort database; subsequently, they were compared with DEGs to obtain a list of immune-related differentially expressed genes (ImmDEGs) (23).
Protein–protein interaction, hub genes, and enrichment analyses
We used the STRING database for protein–protein interaction (PPI) analysis of DEGs with confidence level ≥ 0.04 and filtered the top five genes, which were considered to be the hub genes according to Cytoscape’s Cytohubba plugin (Degree algorithm). DEGs were also subjected to gene ontology (GO) enrichment analyses using the R package clusterProfiler (cutoff: P ≤ 0.05 and q ≤ 0.05) (24). Furthermore, to examine the overall gene enrichment more comprehensively, we performed a gene set enrichment analysis (GSEA) of all genes after sorting by logFC descending order using R soft (pvalueCutoff = 0.05, pAdjustMethod = “BH”) (25).
Prediction of key genes and nutrients and molecular docking for validation
Key genes were defined as intersecting genes between ImmDEGs and hub genes. On the basis of the identified key genes, we used the HERB database to back-predict the target nutrients (26). Proteins encoded by key genes were downloaded from the PDB database and subjected to molecular docking analysis using AutoDock software to validate nutrients (Table 1).
Materials and experimental animals and treatment
According to the predicted results, we selected echinacoside for in vitro validation. Echinacoside was purchased from Chengdu HerbSubstance Co., Ltd. (Chengdu, China) and the percentage purity of echinacoside was 99.85%. Echinacoside was dissolved in 25 mg/mL dimethyl sulfoxide (DMSO) and diluted to 10mg/mL with phosphate-buffered saline. The final concentration of DMSO was less than 10%; the solution was then divided into 1.5 mL aliquots and stored at −80 °C until further use. Specific pathogen-free male C57BL/6 mice (body weight: 20 ± 2 g, age: 56–62 days, n = 12) were purchased from the Guangdong Medical Laboratory Animal Center (Guangdong, China). The mice were maintained under controlled temperature (22 ± 1 °C), humidity (50%), and lighting (12:12 h light/dark) conditions. After 7 days of habituation, the mice were randomly divided into four groups (n = 3 per group; Figure 3D). In the normal control group (NC group), mice were gavaged with distilled water (10 mL/kg) once a day for 1 week. In the model group, mice were gavaged with distilled water (10 mL/kg) once a day for 1 week and subjected to passive swimming activity for 30 min on the last day. In the long-term echinacoside group (LE group), mice were gavaged with echinacoside (100 mg/kg) (27) once a day for 1 week and subjected to passive swimming for 30 min on the last day. In the short-term echinacoside group (SE group), mice were gavaged with distilled water (10 mL/kg) once a day for 6 days; on the seventh day, the mice were gavaged with echinacoside (100mg/kg) (27) and, after 30 min, subjected to passive swimming for 30 min. Peripheral blood and muscle tissues were harvested immediately after the swimming session. All animal experiments were approved by the Animal Care and Use Committee of Affiliated Hospital of Guangzhou University of Chinese Medicine (approval number:20200331016), and experiments were performed in accordance with the Guide for the Care and Use of Laboratory Animals published by the US National Institutes of Health.
Flow cytometry analysis
Flow cytometry was used to detect the percentage of natural killer (NK) cells and dendritic cell (DC) subsets in the peripheral blood of mice. Peripheral blood was collected into tubes containing ethylenediaminetetraacetic acid. Peripheral blood mononuclear cells (PBMCs) were obtained from whole blood by density gradient centrifugation. PBMCs (1 × 106) were incubated with fluorescein isothiocyanate-conjugated rat anti-mouse antibodies against CD45/PE, NK1.1/APC, CD11C/PE-CY5, and CD3 for 60 min in the dark. The antibodies were purchased from Tonbo (Beijing, China). The cell samples were detected on Novocyte D2060R (Agilent, CA, USA), and the percentage of CD3–NK1.1+ NK cell subset and CD11C+NK1.1– DC subset was analyzed using NovoExpress 1.4.1.
Quantitative reverse transcription PCR
Total RNA was extracted from muscle tissues using TRIzol reagent. cDNA was synthesized using the EvoM-MLV kits. Quantitative reverse transcription-PCR (RT-qPCR) was performed using 2X SYBR Green qPCR Master Mix (K1070-500, APExBIO, US) on a CFX96 Real-Time PCR Detection System (Bio-Rad Laboratories) following the manufacturer’s protocol and analyzed using the 2–ΔΔCt method. The following optimized thermal conditions were used: 95°C for 30 s, 95°C for 5 s, and 40 cycles at 60°C for 5 s. The levels of mRNA were normalized to endogenous GAPDH, and the expression of target genes was analyzed using the 2–ΔΔCt method. The experiments were repeated three times independently. The primer sequences used in this study are listed in Table 2.
Statistical analysis
Data were expressed as mean ± standard error of the mean (SEM). GraphPad Prism 8.0 software was used to perform statistical analysis and construct histograms. The differences between groups were evaluated by t-tests or one-way analysis of variance (ANOVA) with appropriate post hoc tests; and P < 0.05 was considered to indicate statistically significant differences.
Results
Gene expression profiles
We downloaded a gene expression matrix for GSE14642 from the GEO database. This data matrix contains data on gene expression levels in peripheral blood of 20 young women before and after 30 min cycle ergometry exercise (28). The work rate was calculated to 50% of the work rate between the anaerobic threshold and the peak oxygen uptake Individually.
Immune cell infiltration and differentially expressed genes
There were marked differences in the immune cell infiltration in these women before and after exercise (Figure 1A). Subsequently, Wilcoxon tests showed that resting NK cells were more highly infiltrated after exercise (P = 0.001), whereas naive B cells and activated DCs were more highly infiltrated before exercise (P = 0.001 and P = 0.013, respectively; Figure 1B). Moreover, 61 DEGs were identified: 8 genes were downregulated, and 53 were upregulated (Figures 1C,D).
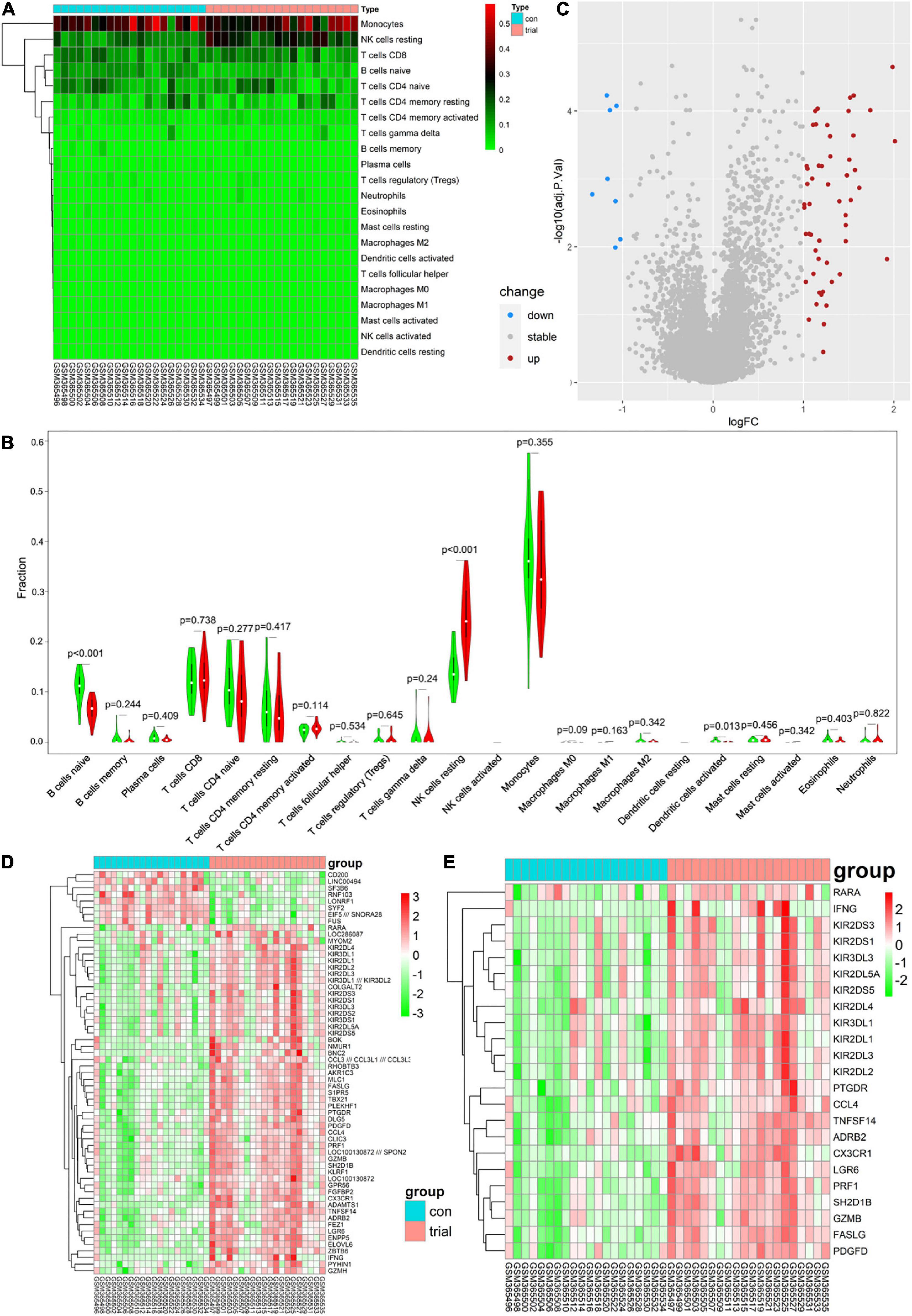
Figure 1. (A) Each column represents a sample, and each row represents a type of immune cells. Color transition from green to red represents an increase in immune cell infiltration level. Con group = before exercise; trial group = after exercise. (B) Green and red violin columns represent immune cell infiltration levels before and after exercise, respectively. The vertical axis represents the ratio of immune cell infiltration responsible for total immune cell infiltration. P value, obtained using the Wilcoxon test, represents the statistical significance of the difference in the immune cell infiltration levels before and after exercise. (C) Upregulated and downregulated differentially expressed genes (DEGs) are highlighted in red and blue, respectively. Criteria: |logFC| ≥ 1 and adjP ≤ 0.05. (D) Expression levels of 61 DEGs are shown; the darker the red color, the higher the expression level, and the darker the green color, the lower the expression level. Con group = before exercise; trial group = after exercise. (E) Expression levels of 23 immune-related differentially expressed genes are shown; the darker the red color, the higher the expression level, and the darker the green color, the lower the expression level. Con group = pre-exercise; trial group = post-exercise.
Immune-related differentially expressed genes
Analysis of immune-related gene expression levels identified 23 ImmDEGs: all of them (e.g., PRF1) were highly expressed after exercise (Figure 1E).
Protein–protein interaction network construction, hub gene selection, and enrichment analysis
Five hub genes (PRF1, GZMB, KLRF1, CCL4, and FASLG) were identified on the basis of PPI analysis (Figures 2A,B). GO enrichment analysis revealed that DEGs were enriched in 52 biological processes (GO-BP) mainly associated with, for example, NK cell-mediated immunity and cellular defense response. Similarly, DEGs were enriched in eight molecular functions (GO-MF), with the major categories being immune receptor activity and chemokine activity (Figures 2C,D). However, cellular components were not significantly enriched. GSEA enrichment analysis showed that eight pathways were enriched; six pathways including NK cell-mediated cytotoxicity were upregulated after exercise, and two pathways including ECM-receptor interaction were downregulated after exercise (Figure 2E and Table 3).
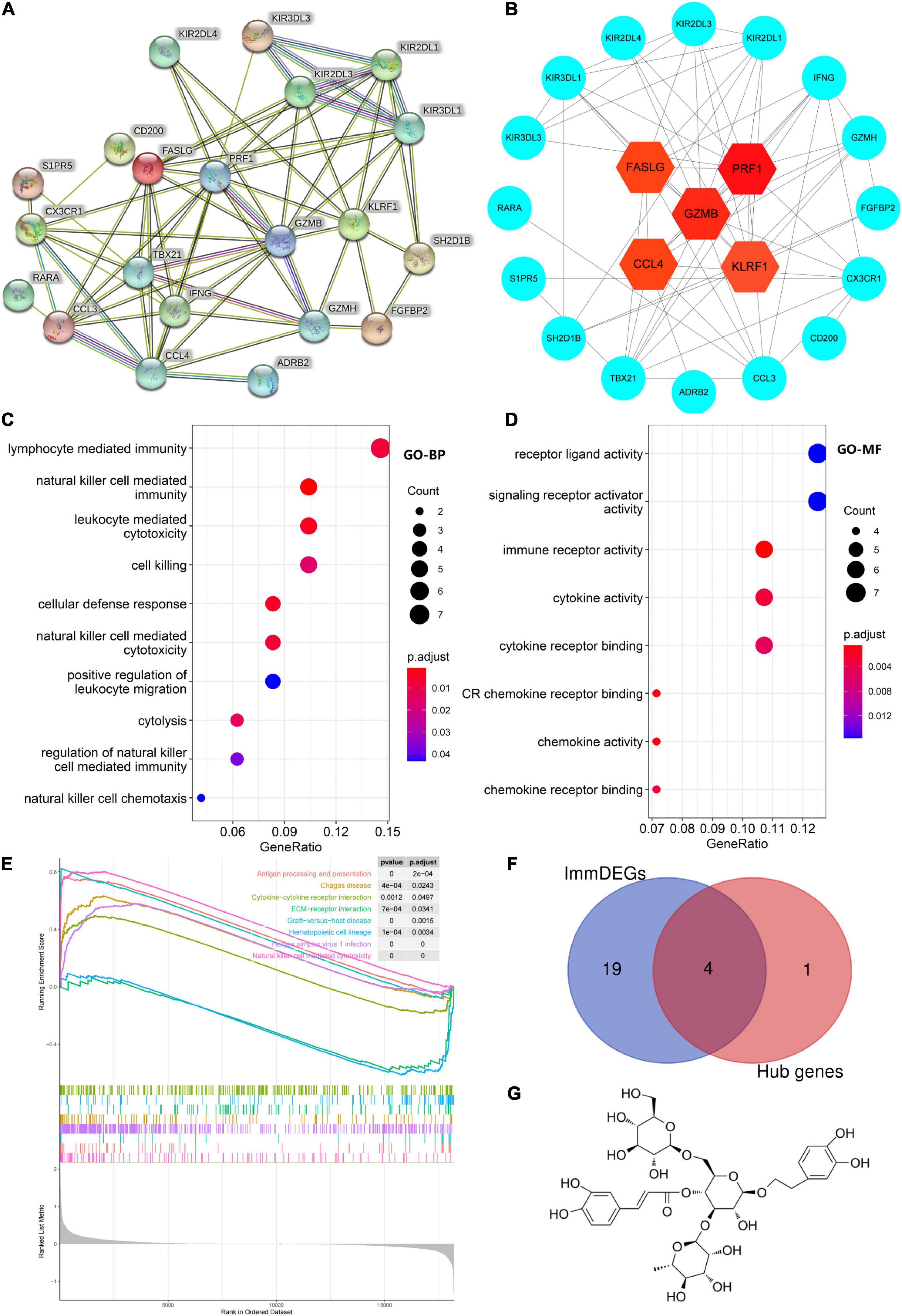
Figure 2. (A) Protein–protein interaction network of differentially expressed genes (DEGs). (B) Hub gene map, the darker the red color of the node, the stronger the protein interaction. (C,D) Top 10 biological processes and all molecular functions observed to be enriched in gene ontology enrichment analysis. The horizontal axis represents the gene ratio, that is, the ratio of the number of DEGs to number of total genes. Dot size is proportional to the gene ratio, and dot color transition from blue to red indicates that the adjusted P value is getting smaller. (E) Gene set enrichment analysis results, with each colored line representing an enriched pathway. (F) Intersection of immune-related differentially expressed genes (ImmDEGs) and hub genes; the genes common between the two groups were GZMB, FASLG, CCL4, and PRF1. (G) The two-dimensional structure of echinacoside.
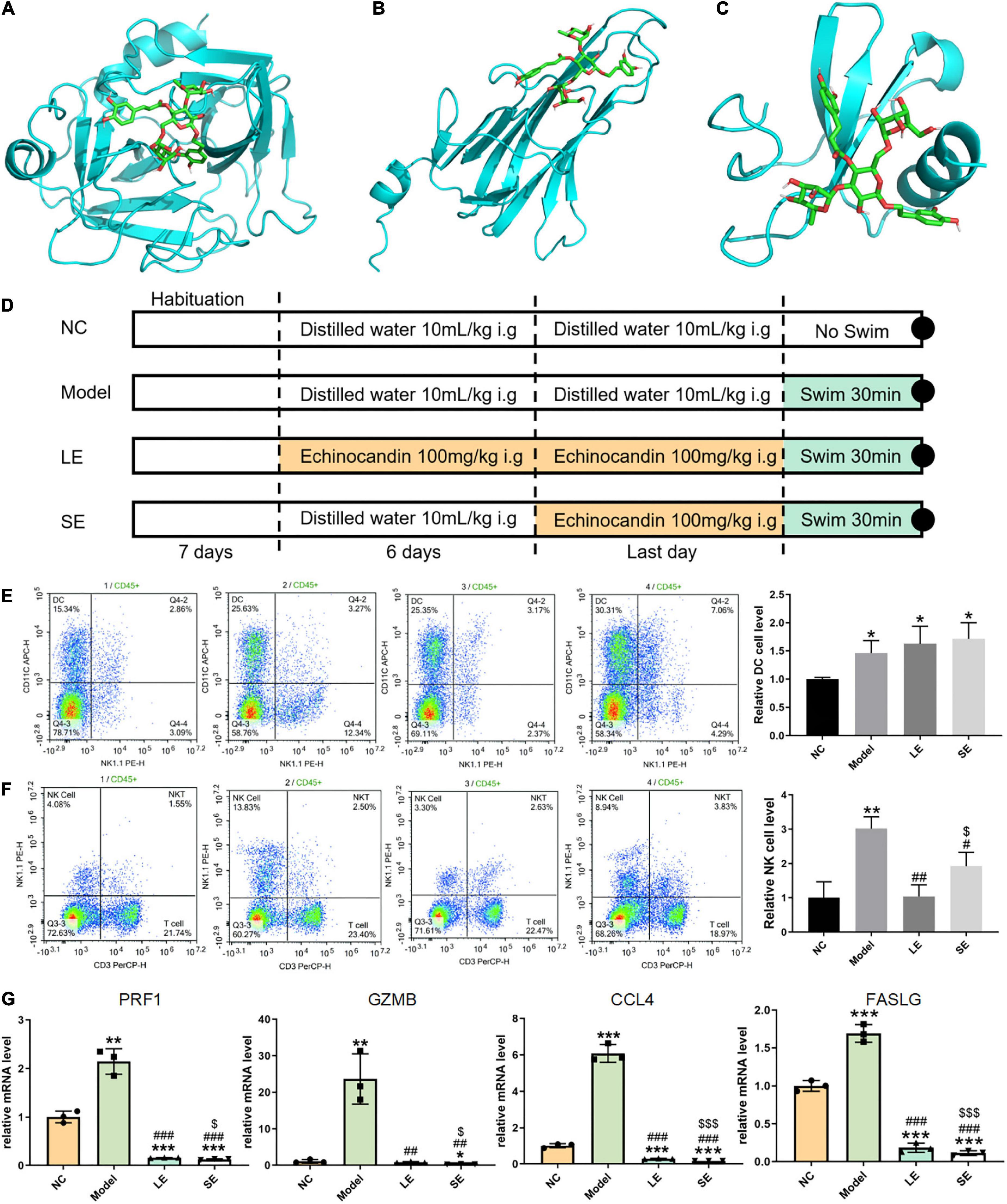
Figure 3. (A–C) Lowest-free-energy docking models of echinacoside with GZMB, FASLG, and CCL4, respectively. (D) Depiction of the control and experimental groups. (E,F) Results of flow cytometry analysis of dendritic cells (DCs) and natural killer (NK) cells, respectively. (G) Quantitative reverse transcription-PCR results for key gene expression levels averaged over three independent experiments. Data are shown as mean ± standard error of the mean. *p < 0.05, **p < 0.01, ***p < 0.001 vs. NC; #p < 0.05, ##p < 0.01, ###p < 0.001 vs. Model; $p < 0.05, p < 0.01, p < 0.001 vs. LE.
Prediction of key genes and nutrients and molecular docking
Four common genes (PRF1, GZMB, CCL4, and FASLG) were obtained by intersecting the sets of ImmDEGs and hub genes, which were considered as key genes (Figure 2F). The top five natural Chinese herbs with the lowest P value predicted on the basis of key genes in the HERB database were selected (Table 4). The 26 candidate (Table 5) ingredients were identified by the intersection of the related target ingredients directly predicted based on key genes by the HERB database and the active ingredients of above-mentioned Chinese herbs. However, PRF1 failed to predict natural Chinese medicine by the database. Of these 26 candidates, echinacoside (Figure 2G) was the most interesting to us, as this compound is the active ingredient in our predicted herb, Herba Cistanches, which have been used in China for thousands of years and are still used to this day to enhance physical functions. Based on this fact, we consider echinacoside to be the highest priority research candidate. Molecular docking of selected compound to key genes showed that GZMB, FASLG, and CCL4 bind tightly to echinacoside with their lowest binding free energies of −8.0kcal/mol, −7.2kcal/mol, −6.9kcal/mol, respectively (Figures 3A–C). However, we were unable to analyze the molecular docking of PRF1 with echinacoside because its 3D structure was not accessible.
Immune cell infiltration after echinacoside intervention detected by flow cytometry
The frequency of infiltrated immune cells in each group were detected by flow cytometry (Figures 3E,F). The percentage of infiltrating NK cells significantly increased after swimming. LE and SE groups both reduced the percentage of infiltrating NK cells with model group. Long-term supplementation with echinacoside restricted the infiltration of NK cells more significantly than short-term supplementation with echinacoside. However, in contrast, the percentage of DCs was significantly increased after swimming. Long-term or short-term supplementation with echinacoside did not significantly decrease the percentage of DCs after swimming.
Expression of key genes after echinacoside intervention detected by quantitative reverse transcription PCR
The mRNA levels of key genes were determined by quantitative reverse transcription PCR, which showed that the mRNA levels of all key genes were significantly increased after swimming (Figure 3G). Both long-term or short-term supplementation with echinacoside significantly reduced the expression level of key genes. GZMB, PRF1, and CCL4 were markedly downregulated in the SE group compared with the LE group.
Discussion
Our analysis of immune cell infiltration in the peripheral blood before and 30 min after exercise showed that NK cells showed a higher rate of infiltration post exercise, whereas naive B cells and activated DCs showed a low rate of infiltration (see Figure 1B). DCs, the most effective antigen-presenting cells, play an important role in immune homeostasis, bridging the gap between innate and adaptive immunity (29). They rapidly perform uptake and presentation functions when foreign antigens are present, activating T cells to produce an immune clearance response and inducing immune tolerance in the presence of self-antigens (30). Naive B cells are immature B cells that play an immunomodulatory role in inflammation by secreting cytokines and other pro-inflammatory indicators (31). Both of these cells are adaptive immune cells. NK cells are innate immune cells that determine the inflammatory microenvironment and are important in the removal of foreign infectious agents and own necrotic tissue (32). Therefore, the immune infiltration results do not signify an increase in immunity after 30 min of exercise but a sterile inflammation resulting from exercise-induced injury. We found that 53 genes were upregulated and 8 genes were downregulated post exercise (see Figures 1C,D). Notably, a PPI analysis of these differentially expressed genes showed that four of the top five genes (PRF1, GZMB, CCL4, and FASLG) were immune-related genes (see Figure 2F). GMZB, PRF1, and FASLG are key cytotoxic proteins in the secretory lysosomes in NK cells and typically produce cytotoxic effects in the inflammation zone (33). Thus, the findings suggest that the women developed immune dysregulation after 30 min of exercise, which was primarily associated with abnormally high levels of NK cells.
PRF1 is a potent component of NK cells; PRF1 expression levels are positively correlated with the inflammatory toxic effects of NK cells, and PRF1 targeting regulates (enhances or decreases) the activity and function of NK cells (34–37). Thus, the high expression of PRF1 after exercise is consistent with the high rate of NK cell infiltration. GZMB is a component of NK cell endolytic granules and is known for its apoptosis-promoting function (38, 39). It is an important mediator of the inflammatory response, and some studies have found that silencing GZMB reduces tissue damage caused by inflammation, for example, in a rat model of rheumatoid arthritis, GZMB silencing significantly reduced the degree of swelling in the ankle joint and reduced joint soft tissue damage (40, 41). FASLG, a member of the tumor necrosis factor family, is a transmembrane protein that forms an apoptotic cascade with FAS that is important for cell proliferation homeostasis (42, 43). This factor is widely involved in the inflammatory response and is highly expressed in inflammatory injury responses such as neurological injury, acute lung injury, kidney injury, and traumatic brain injury, and its expression is significantly associated with the extent of acute burns (44–47). Similar to PRF1, the high GZMB and FASLG expression was consistent with a high infiltration rate of NK cells, suggesting an inflammatory response following exercise. CCL4 is also an inflammatory chemokine that mediates the inflammatory immune response by recruiting lymphocytes, NK cells, and eosinophils, among others (48, 49). Several previous studies have found that the expression of this chemokine is significantly elevated in the inflammatory immune response following exercise (50, 51). It has also been found that CCL4 is a key mediator of neuroinflammatory pain following nerve injury (52). Overall, the high expression of these key genes reflects the fact that inflammatory damage does occur after acute exercise.
Notably, we predicted 26 natural immunomodulatory compounds on the basis of these immune infiltration findings. Echinacoside is a key natural component of the traditional Chinese medicinal herb Herba Cistanches. This compound remains little studied in the field of exercise, but it is widely used in traditional Chinese medicine because it is believed to play a key role in regulating human body functions. Interestingly, the substance is homologous to food and medicine; therefore, we intended to investigate whether it regulates inflammation after sports-related injury and further examine whether it is a potential sports supplement. Therefore, we selected this natural ingredient, echinacoside, as the optimal candidate compound and as the experimentally verified ingredient. Firstly, we verified the tightness of binding between echinacoside and GZMB using molecular docking and we found a minimum binding free energy of −8.0kcal/mol between them, which indicating a very tight binding. Furthermore, although echinacoside are not among the other key genetically predicted natural ingredients, we wanted to explore whether this component could bind tightly to key genes other than GZMB, such as FASLG. Surprisingly, we found that the binding free energy of echinacoside to FASLG and CCL4 was also very low, at −7.2kcal/mol and −6.9kcal/mol, respectively, suggesting that this natural ingredient also binds tightly to FASLG and CCL4. Finally, Experiments in mice were used to verify the modulatory effect of echinacoside on immune imbalance after sports-related injury. Remarkably, the flow cytometry results showed that mice gavaged with echinacoside had significantly lower levels of NK cells in their peripheral blood; the LE group showed the lowest NK cell levels, equivalent to those in the NC group, followed by the SE group, whereas the Model group, which was not supplemented with echinacoside, showed the highest levels of NK cells in the peripheral blood (see Figure 3F). However, for DCs, there was no statistical difference in the DC levels between the Model, LE, and SE groups, which all showed significant higher DC levels than those in the NC group. On the basis of this finding, we analyzed the factors affecting the expression levels of DCs in peripheral blood. DCs maintain overall immune homeostasis through their own active or passive regulation during an inflammatory response occurring after exercise; for example, in tissue damage inflammation, vimentin prevents autoimmune damage by inhibiting DCs (53). The mode of energy supply is another important factor affecting DC expression. Hypoxia or changes in the nutritional status affect DC metabolism, aerobic glycolysis significantly promotes DC activation, and inhibition of glycolytic efficiency directly prevents DC activation (54, 55). It is difficult to identify which factor most influences the function of DCs, but these factors together lead to the dynamic regulation of DCs.
Furthermore, we examined the expression levels of key genes (GZMB, PRF1, FASLG, and CCL4) in each mouse group by RT-qPCR and found that the expression levels of these genes in the LE and SE groups were similar to those in the NC group, significantly lower than those in the Model group, and the lowest in the SE group (see Figure 3G). Overall, the flow cytometry results for NK cells were consistent with the expression levels of key genes. However, unexpectedly, the expression of key genes was lower in the SE group than in the LE group. We then reviewed the pharmacokinetics of echinacoside and found that this natural active ingredient is extremely rapidly absorbed in the gastrointestinal tract, with a peak blood concentration time of 15 min and a short half-life of 74 min (56). The changes in the expression levels of these key genes intervened by echinacoside are relatively consistent with the pharmacokinetics of echinacoside. This may therefore explain the lowest expression of key genes in the SE group. Therefore, our preliminary findings suggest that echinacoside administration for a longer period is more effective in regulating peripheral blood NK cell levels after exercise and that this natural supplement is relatively more effective in reducing inflammation when administered 30 min before exercise.
In summary, echinacoside effectively modulates the inflammatory immune response following exercise, primarily by regulating NK cell levels. We report, for the first time to our knowledge, that echinacoside, a promising natural compound, may be used as a dietary supplement to reduce inflammatory damage due to excessive NK cell infiltration following exercise by reducing the elevated NK cell levels and lowering the levels of NK cell-associated cytotoxic proteins such as GZMB, PRF1, FASLG, and CCL4 in the peripheral blood.
In this study, our approach has pioneered a very novel screening model for natural nutritional candidates, a systematic model that allows for rapid screening of a number of potential target natural compounds, greatly improving research efficiency (see Figure 4). The results of our study, echinacoside, which can bring preventive and therapeutic effects to patients with sports injuries, are of great clinical application. Particularly in the context of the apparent underdevelopment of anti-inflammatory dietary supplements for the prevention and mitigation of sports injuries, both our research model and echinacoside show strong potential in this area.
Data availability statement
The original contributions presented in this study are included in the article/Supplementary material, further inquiries can be directed to the corresponding author.
Ethics statement
The animal study was reviewed and approved by Animal Care and Use Committee of Affiliated Hospital of Guangzhou University of Chinese Medicine.
Author contributions
FL and RY designed and planned the study and identified, and selected original studies. FJ and DX drafted the manuscript. FL and YS revised the final manuscript. LL and RL performed most experiments with some help from FL and RY. FL performed all the bioinformatics analyses. LX and MT contributed to data analysis. FL and ZZ provided advice during the study and manuscript preparation. All authors read and approved the final manuscript.
Funding
This study was supported by the National Natural Science Foundation of China (82004320), the Natural Science Foundation of Guangdong Province of China (2021A1515011095, 2022A1515011710, and 2022A1515010679), the Science and Technology Project of Shenzhen City of China (JCYJ20190807115201653), and Shenzhen Bao’an Chinese Medicine Hospital Research Program (BAZYY20220702).
Acknowledgments
We would like to thank the native English speaking scientists of Elixigen Company (Huntington Beach, California) for editing our manuscript.
Conflict of interest
The authors declare that the research was conducted in the absence of any commercial or financial relationships that could be construed as a potential conflict of interest.
Publisher’s note
All claims expressed in this article are solely those of the authors and do not necessarily represent those of their affiliated organizations, or those of the publisher, the editors and the reviewers. Any product that may be evaluated in this article, or claim that may be made by its manufacturer, is not guaranteed or endorsed by the publisher.
Supplementary material
The Supplementary Material for this article can be found online at: https://www.frontiersin.org/articles/10.3389/fnut.2022.987545/full#supplementary-material
References
1. Chaput JP, Willumsen J, Bull F, Chou R, Ekelund U, Firth J, et al. 2020 WHO guidelines on physical activity and sedentary behaviour for children and adolescents aged 5-17 years: summary of the evidence. Int J Behav Nutr Phys Act. (2020) 17:141. doi: 10.1186/s12966-020-01037-z
2. Smith AD, Crippa A, Woodcock J, Brage S. Physical activity and incident type 2 diabetes mellitus: a systematic review and dose–response meta-analysis of prospective cohort studies. Diabetologia. (2016) 59:2527–45. doi: 10.1007/s00125-016-4079-0
3. Börjesson M, Onerup A, Lundqvist S, Dahlöf B. Physical activity and exercise lower blood pressure in individuals with hypertension: narrative review of 27 RCTs. Br J Sports Med. (2016) 50:356–61. doi: 10.1136/bjsports-2015-095786
4. Jeong SW, Kim SH, Kang SH, Kim HJ, Yoon CH, Youn TJ, et al. Mortality reduction with physical activity in patients with and without cardiovascular disease. Eur Heart J. (2019) 40:3547–55. doi: 10.1093/eurheartj/ehz564
5. Fiuza-Luces C, Santos-Lozano A, Joyner M, Carrera-Bastos P, Picazo O, Zugaza JL, et al. Exercise benefits in cardiovascular disease: beyond attenuation of traditional risk factors. Nat Rev Cardiol. (2018) 15:731–43. doi: 10.1038/s41569-018-0065-1
6. Schmitz KH, Campbell AM, Stuiver MM, Pinto BM, Schwartz AL, Morris GS, et al. Exercise is medicine in oncology: engaging clinicians to help patients move through cancer. CA Cancer J Clin. (2019) 69:468–84. doi: 10.3322/caac.21579
7. Valenzuela PL, Castillo-García A, Morales JS, de la Villa P, Hampel H, Emanuele E, et al. Exercise benefits on Alzheimer’s disease: state-of-the-science. Ageing Res Rev. (2020) 62:101108. doi: 10.1016/j.arr.2020.101108
8. Chastin SFM, Abaraogu U, Bourgois JG, Dall PM, Darnborough J, Duncan E, et al. Effects of regular physical activity on the immune system, vaccination and risk of community-acquired infectious disease in the general population: systematic review and meta-analysis. Sports Med. (2021) 51:1673–86. doi: 10.1007/s40279-021-01466-1
9. Piercy KL, Troiano RP, Ballard RM, Carlson SA, Fulton JE, Galuska DA, et al. The physical activity guidelines for Americans. JAMA. (2018) 320:2020–8. doi: 10.1001/jama.2018.14854
10. Mohr M, Draganidis D, Chatzinikolaou A, Barbero-Álvarez JC, Castagna C, Douroudos I, et al. Muscle damage, inflammatory, immune and performance responses to three football games in 1 week in competitive male players. Eur J Appl Physiol. (2016) 116:179–93. doi: 10.1007/s00421-015-3245-2
11. Lee EC, Fragala MS, Kavouras SA, Queen RM, Pryor JL, Casa DJ. Biomarkers in sports and exercise: tracking health, performance, and recovery in athletes. J Strength Cond Res. (2017) 31:2920–37. doi: 10.1519/jsc.0000000000002122
12. Matava MJ. Injectable nonsteroidal anti-inflammatory drugs in sport. Clin J Sport Med. (2018) 28:443–50. doi: 10.1097/jsm.0000000000000602
13. Cheung K, Hume P, Maxwell L. Delayed onset muscle soreness : treatment strategies and performance factors. Sports Med. (2003) 33:145–64. doi: 10.2165/00007256-200333020-00005
14. O’Connor S, McCaffrey N, Whyte E, Moran K, Lacey P. Nonsteroidal anti-inflammatory drug use, knowledge, and behaviors around their use and misuse in Irish collegiate student-athletes. Phys Sports Med. (2019) 47:318–22. doi: 10.1080/00913847.2018.1553468
15. Bedi A, Trinh TQ, Olszewski AM, Maerz T, Ramme AJ. Nonbiologic injections in sports medicine. JBJS Rev. (2020) 8:e0052. doi: 10.2106/jbjs.Rvw.19.00052
16. Smith-Ryan AE, Hirsch KR, Saylor HE, Gould LM, Blue MN. Nutritional considerations and strategies to facilitate injury recovery and rehabilitation. J Athl Train. (2020) 55:918–30. doi: 10.4085/1062-6050-550-19
17. Rawson ES, Miles MP, Larson-Meyer DE. Dietary supplements for health, adaptation, and recovery in athletes. Int J Sport Nutr Exerc Metab. (2018) 28:188–99. doi: 10.1123/ijsnem.2017-0340
18. Fernández-Lázaro D, Mielgo-Ayuso J, Seco Calvo J, Córdova Martínez A, Caballero García A, Fernandez-Lazaro CI. Modulation of exercise-induced muscle damage, inflammation, and oxidative markers by curcumin supplementation in a physically active population: a systematic review. Nutrients. (2020) 12:501. doi: 10.3390/nu12020501
19. Huang CC, Lee MC, Ho CS, Hsu YJ, Ho CC, Kan NW. Protective and recovery effects of resveratrol supplementation on exercise performance and muscle damage following acute plyometric exercise. Nutrients. (2021) 13:3217. doi: 10.3390/nu13093217
20. Borghi SM, Pinho-Ribeiro FA, Fattori V, Bussmann AJ, Vignoli JA, Camilios-Neto D, et al. Quercetin inhibits peripheral and spinal cord nociceptive mechanisms to reduce intense acute swimming-induced muscle pain in mice. PLoS One. (2016) 11:e0162267. doi: 10.1371/journal.pone.0162267
21. Lu Y, Deng B, Xu L, Liu H, Song Y, Lin F. Effects of Rhodiola rosea supplementation on exercise and sport: a systematic review. Front Nutr. (2022) 9:856287. doi: 10.3389/fnut.2022.856287
22. Shen S, Kong J, Qiu Y, Yang X, Wang W, Yan L. Identification of core genes and outcomes in hepatocellular carcinoma by bioinformatics analysis. J Cell Biochem. (2019) 120:10069–81. doi: 10.1002/jcb.28290
23. Liu Y, Zhang X, Zhang J, Tan J, Li J, Song Z. Development and validation of a combined ferroptosis and immune prognostic classifier for hepatocellular carcinoma. Front Cell Dev Biol. (2020) 8:596679. doi: 10.3389/fcell.2020.596679
24. Sun Q, Li X, Xu M, Zhang L, Zuo H, Xin Y, et al. Differential expression and bioinformatics analysis of circRNA in non-small cell lung cancer. Front Genet. (2020) 11:586814. doi: 10.3389/fgene.2020.586814
25. Subramanian A, Tamayo P, Mootha VK, Mukherjee S, Ebert BL, Gillette MA, et al. Gene set enrichment analysis: a knowledge-based approach for interpreting genome-wide expression profiles. Proc Natl Acad Sci U.S.A. (2005) 102:15545–50. doi: 10.1073/pnas.0506580102
26. Fang S, Dong L, Liu L, Guo J, Zhao L, Zhang J, et al. HERB: a high-throughput experiment- and reference-guided database of traditional Chinese medicine. Nucleic Acids Res. (2021) 49:D1197–206. doi: 10.1093/nar/gkaa1063
27. Liu J, Yang L, Dong Y, Zhang B, Ma X. Echinacoside, an inestimable natural product in treatment of neurological and other disorders. Molecules. (2018) 23:1213. doi: 10.3390/molecules23051213
28. Radom-Aizik S, Zaldivar F Jr, Leu SY, Cooper DM. A brief bout of exercise alters gene expression and distinct gene pathways in peripheral blood mononuclear cells of early- and late-pubertal females. J Appl Physiol. (2009) 107:168–75. doi: 10.1152/japplphysiol.00121.2009
29. Balan S, Saxena M, Bhardwaj N. Dendritic cell subsets and locations. Int Rev Cell Mol Biol. (2019) 348:1–68. doi: 10.1016/bs.ircmb.2019.07.004
30. Waisman A, Lukas D, Clausen BE, Yogev N. Dendritic cells as gatekeepers of tolerance. Semin Immunopathol. (2017) 39:153–63. doi: 10.1007/s00281-016-0583-z
31. Frasca D, Diaz A, Romero M, Garcia D, Blomberg B. B cell immunosenescence. Annu Rev Cell Dev Biol. (2020) 36:551–74. doi: 10.1146/annurev-cellbio-011620-034148
32. Li Y, Wang F, Imani S, Tao L, Deng Y, Cai Y. Natural killer cells: friend or foe in metabolic diseases? Front Immunol. (2021) 12:614429. doi: 10.3389/fimmu.2021.614429
33. Lettau M, Kabelitz D, Janssen O. Lysosome-related effector vesicles in T lymphocytes and NK cells. Scand J Immunol. (2015) 82:235–43. doi: 10.1111/sji.12337
34. Romero CA, Sánchez IP, Gutierrez-Hincapié S, Álvarez-Álvarez JA, Pereañez JA, Ochoa R, et al. A novel pathogenic variant in PRF1 associated with hemophagocytic lymphohistiocytosis. J Clin Immunol. (2015) 35:501–11. doi: 10.1007/s10875-015-0169-x
35. Huang Q, Ding J, Gong M, Wei M, Zhao Q, Yang J. Effect of miR-30e regulating NK cell activities on immune tolerance of maternal-fetal interface by targeting PRF1. Biomed Pharmacother. (2019) 109:1478–87. doi: 10.1016/j.biopha.2018.09.172
36. Lu CC, Wu TS, Hsu YJ, Chang CJ, Lin CS, Chia JH, et al. NK cells kill mycobacteria directly by releasing perforin and granulysin. J Leukoc Biol. (2014) 96:1119–29. doi: 10.1189/jlb.4A0713-363RR
37. Chang CJ, Chen YY, Lu CC, Lin CS, Martel J, Tsai SH, et al. Ganoderma lucidum stimulates NK cell cytotoxicity by inducing NKG2D/NCR activation and secretion of perforin and granulysin. Innate Immun. (2014) 20:301–11. doi: 10.1177/1753425913491789
38. Oboshi W, Watanabe T, Hayashi K, Nakamura T, Yukimasa N. QPY/RAH haplotypes of the GZMB gene are associated with natural killer cell cytotoxicity. Immunogenetics. (2018) 70:29–36. doi: 10.1007/s00251-017-1014-6
39. Turner CT, Lim D, Granville DJ. Granzyme B in skin inflammation and disease. Matrix Biol. (2019) 75-76:126–40. doi: 10.1016/j.matbio.2017.12.005
40. Velotti F, Barchetta I, Cimini FA, Cavallo MG. Granzyme B in inflammatory diseases: apoptosis, inflammation, extracellular matrix remodeling, epithelial-to-mesenchymal transition and fibrosis. Front Immunol. (2020) 11:587581. doi: 10.3389/fimmu.2020.587581
41. Bao CX, Chen HX, Mou XJ, Zhu XK, Zhao Q, Wang XG. GZMB gene silencing confers protection against synovial tissue hyperplasia and articular cartilage tissue injury in rheumatoid arthritis through the MAPK signaling pathway. Biomed Pharmacother. (2018) 103:346–54. doi: 10.1016/j.biopha.2018.04.023
42. Ji W, Xin Y, Zhang L, Liu X. ALG2 influences T cell apoptosis by regulating FASLG intracellular transportation. Biochem J. (2020) 477:3105–21. doi: 10.1042/bcj20200028
43. Magerus A, Bercher-Brayer C, Rieux-Laucat F. The genetic landscape of the FAS pathway deficiencies. Biomed J. (2021) 44:388–99. doi: 10.1016/j.bj.2021.06.005
44. Li Y, Sun Y, Cai M, Zhang H, Gao N, Huang H, et al. FAS Ligand Gene (FASLG) plays an important role in nerve degeneration and regeneration after rat sciatic nerve injury. Front Mol Neurosci. (2018) 11:210. doi: 10.3389/fnmol.2018.00210
45. Jiang H, Wang S, Hou L, Huang JA, Su B. Resveratrol inhibits cell apoptosis by suppressing long noncoding RNA (lncRNA) XLOC_014869 during lipopolysaccharide-induced acute lung injury in rats. J Thorac Dis. (2021) 13:6409–26. doi: 10.21037/jtd-21-1113
46. Ortiz A, Lorz C, Egido J. The FAS ligand/FAS system in renal injury. Nephrol Dial Transplant. (1999) 14:1831–4. doi: 10.1093/ndt/14.8.1831
47. Beer R, Franz G, Schöpf M, Reindl M, Zelger B, Schmutzhard E, et al. Expression of FAS and FAS ligand after experimental traumatic brain injury in the rat. J Cereb Blood Flow Metab. (2000) 20:669–77. doi: 10.1097/00004647-200004000-00004
48. Mukaida N, Sasaki SI, Baba T. CCL4 signaling in the tumor microenvironment. Adv Exp Med Biol. (2020) 1231:23–32. doi: 10.1007/978-3-030-36667-4_3
49. Kobayashi Y, Konno Y, Kanda A, Yamada Y, Yasuba H, Sakata Y, et al. Critical role of CCL4 in eosinophil recruitment into the airway. Clin Exp Allergy. (2019) 49:853–60. doi: 10.1111/cea.13382
50. Muders K, Pilat C, Deuster V, Frech T, Krüger K, Pons-Kühnemann J, et al. Effects of Traumeel (Tr14) on recovery and inflammatory immune response after repeated bouts of exercise: a double-blind RCT. Eur J Appl Physiol. (2017) 117:591–605. doi: 10.1007/s00421-017-3554-8
51. Peake JM, Roberts LA, Figueiredo VC, Egner I, Krog S, Aas SN, et al. The effects of cold water immersion and active recovery on inflammation and cell stress responses in human skeletal muscle after resistance exercise. J Physiol. (2017) 595:695–711. doi: 10.1113/jp272881
52. Saika F, Kiguchi N, Kobayashi Y, Fukazawa Y, Kishioka S. CC-chemokine ligand 4/macrophage inflammatory protein-1β participates in the induction of neuropathic pain after peripheral nerve injury. Eur J Pain. (2012) 16:1271–80. doi: 10.1002/j.1532-2149.2012.00146.x
53. Yu MB, Guerra J, Firek A, Langridge WHR. Extracellular vimentin modulates human dendritic cell activation. Mol Immunol. (2018) 104:37–46. doi: 10.1016/j.molimm.2018.09.017
54. O’Neill LA, Pearce EJ. Immunometabolism governs dendritic cell and macrophage function. J Exp Med. (2016) 213:15–23. doi: 10.1084/jem.20151570
55. Kelly B, O’Neill LA. Metabolic reprogramming in macrophages and dendritic cells in innate immunity. Cell Res. (2015) 25:771–84. doi: 10.1038/cr.2015.68
56. Jia C, Shi H, Wu X, Li Y, Chen J, Tu P. Determination of echinacoside in rat serum by reversed-phase high-performance liquid chromatography with ultraviolet detection and its application to pharmacokinetics and bioavailability. J Chromatogr B Analyt Technol Biomed Life Sci. (2006) 844:308–13. doi: 10.1016/j.jchromb.2006.07.040
Keywords: sport injury, nutritional supplement, immune infiltration, inflammation, echinacoside
Citation: Jiang F, Yang R, Xue D, Li R, Tan M, Zeng Z, Xu L, Liu L, Song Y and Lin F (2022) Effects of a natural nutritional supplement on immune cell infiltration and immune gene expression in exercise-induced injury. Front. Nutr. 9:987545. doi: 10.3389/fnut.2022.987545
Received: 06 July 2022; Accepted: 29 August 2022;
Published: 16 September 2022.
Edited by:
Mihnea-Alexandru Gãman, Carol Davila University of Medicine and Pharmacy, RomaniaReviewed by:
Rajeev K. Singla, Sichuan University, ChinaMohammad Amin Khazeei Tabari, Mazandaran University of Medical Sciences, Iran
Copyright © 2022 Jiang, Yang, Xue, Li, Tan, Zeng, Xu, Liu, Song and Lin. This is an open-access article distributed under the terms of the Creative Commons Attribution License (CC BY). The use, distribution or reproduction in other forums is permitted, provided the original author(s) and the copyright owner(s) are credited and that the original publication in this journal is cited, in accordance with accepted academic practice. No use, distribution or reproduction is permitted which does not comply with these terms.
*Correspondence: Fengxia Lin, c3psaW5meEAxNjMuY29t
†These authors have contributed equally to this work