- 1Department of Neurology, Beijing Friendship Hospital, Capital Medical University, Beijing, China
- 2Department of Neurology, Dehui People's Hospital, Jilin, China
Introduction: Epilepsy is a highly prevalent neurological disease whose treatment has always been challenging. Hence, it is crucial to explore the molecular mechanisms underlying epilepsy inhibition. Inflammation and oxidative stress are important pathophysiological changes in epilepsy that contribute to the development of spontaneous seizures and cognitive deficits. In recent years, altered gut microbiota composition was found to be involved in epilepsy, but the underlying mechanism remains unclear. Modulation of the gut microbiota showed a positive impact on the brain by regulating oxidative stress and inflammation. Hence, this study evaluated the effect of modulating gut dysbiosis by treating epileptic rats with prebiotics, probiotics, and synbiotics and investigated the underlying molecular mechanism.
Materials and methods: Epileptic rat models were established by injecting 1 μl of kainic acid (KA, 0.4 μg/μl) into the right amygdalae. The rats were divided into Sham, KA, KA+prebiotic [inulin:1 g/kg body weight (bw)/day], KA+probiotics (10 × 109cfu of each bacteria/kg, bw/day), and KA+synbiotic groups (1:1 mixture of prebiotics and probiotics). Seizures were monitored, and cognitive function was assessed in all rats. Biochemical indicators, namely, oxidative stress, DNA damage, glutamate levels, and inflammation markers, were also determined.
Results: The KA-induced status epilepticus (SE) rats exhibited spontaneous seizures and cognitive deficits. This was accompanied by the activation of glial cells, the inflammatory response (IL-1 β, IL-6, and TNF-α), lipid peroxidation (MDA), DNA damage (8-OHdG), the release of glutamate, and a decline in total antioxidant ability (GSH). These changes were alleviated by partial treatment with prebiotics, probiotics, and synbiotics.
Conclusion: Modulating gut dysbiosis ameliorates spontaneous seizures and cognitive deficits in rats with KA-induced status epilepticus. The underlying mechanism may potentially involve the inhibition of inflammation and oxidative stress.
Introduction
Epilepsy is a highly prevalent neurological disease affecting millions of people worldwide (1). Patients with recurrent seizures usually have disorders in cognition, sleep, and neuropsychiatry, which result in a low quality-of-life (2–4). Despite various anti-seizure medications (ASMs) used in the clinical treatment of epilepsy, the seizures of 30% of patients cannot be well-controlled, leading to the development of refractory epilepsy (5). It is not sufficient to control seizures by using ASMs only; the ultimate goal of epilepsy treatment is to find a breakthrough in inhibiting its occurrence and development. Neuroinflammation and oxidative stress are important pathophysiological changes in epilepsy and are associated with an enhanced risk of developing the disease (6). Hence, targeting inflammation and oxidative stress are crucial therapeutic approaches to inhibit the development of epilepsy and its associated comorbidities.
The microbiota-gut-brain axis is gradually recognized to play an important role in the central nervous system. The bidirectional connection between the brain and gut is mediated by the immune system, enteric nervous system (ENS), vagus nerve, and microbial metabolites (7). Some studies have reported the presence of an altered gut microbiota composition in drug-resistant patients, suggesting that gut dysbiosis might be involved in the mechanism of epilepsy (8, 9). Host bacteria have been found to regulate the maturation and function of microglia and the microglia-mediated inflammatory response (10, 11). In addition, oxidative stress during seizures is mainly caused by NADPH oxidases, especially those expressed in activated microglia (12, 13). The effect of gut bacteria on regulating neuroinflammation and oxidative stress has been directly confirmed in models of Alzheimer's disease (14). Therefore, modulating the gut microbiome may be a potential intervention strategy to inhibit the development of epilepsy.
The addition of probiotics, prebiotics, and synbiotics can be used to modulate gut microbiota. Probiotics are live microorganisms that exert beneficial effects on body health by improving intestinal and immune homeostasis (15, 16). Lactobacilli and bifidobacteria are well-known probiotic strains that have shown positive effects on several neurological and psychological diseases (17–19). Prebiotics, regarded as non-digestible food fibers, can improve the health of the host by selectively increasing the growth and activity of gut microbes, especially Lactobacillus and Bifidobacterium (20). Synbiotics are a combination of prebiotics and probiotics, in which the prebiotic components are beneficial for the growth and metabolism of the probiotics (20). In this study, we aimed to investigate the effects of prebiotics, probiotics, and synbiotics on kainic acid-induced status epilepticus in rats and elucidate the underlying mechanism of their action.
Materials and methods
Animals
Male Wistar rats (Beijing Vital River Laboratory Animal Technology Co. Ltd, China) weighing 280–300 g were used in this study. All experimental rats were raised in a standard environment with a 12-h light/dark cycle, constant temperature (22 ± 2°C), and free access to water and chow. All procedures in the experiment followed guidelines on the care and use of laboratory animals by the Capital Medical University (China). The ethics approval for this study was obtained from the Animal Studies Subcommittee of Capital Medical University (China).
Drug preparation
Kainic acid (KA; Abcam, Cambridge, UK) was used to induce status epilepticus brain insults. To create a working solution, 0.4 μg KA was dissolved in 1 μl phosphate-buffered saline (PBS). Inulin (1 g/kg bw/day; Chicory, Sigma-Aldrich, MO, USA) was used as the prebiotic supplement. Probiotics containing Bifidobacterium and Lactobacillus were provided by Shanxi Sciphar Natural Products Co., Ltd. Approximately 10 × 109 cfu of each probiotic per kg bodyweight was used for treating the experimental rats. The synbiotics used were a 1:1 mixture of prebiotics and probiotics.
Seizure induction and experimental groups
Rat epilepsy models were anesthetized and positioned in a stereotaxic frame. Then, 1 μl KA solution was injected into the right amygdalae (2.5 mm caudal to the bregma; 4.5 mm right of the midline; 8.5 mm from the surface of the skull) to establish status epilepticus (SE) insult. Only rats that reached the fifth seizure stage (21) and were maintained for 1 h were allowed in this study to ensure consistency of brain injury and minimize suffering. Diazepam (8 mg/kg; Sigma-Aldrich) was used to terminate the seizures.
Rats were randomly divided into five groups. The sham group was injected with 1 μl PBS in the right amygdala and was fed orally with 1 mL PBS per day. The KA group was injected with 1 μl KA solution into the right amygdala and orally fed with 1 ml PBS daily. The KA+prebiotic group was injected with 1 μl KA solution into the right amygdala and orally fed with 1 ml PBS containing calculated prebiotics per day. The KA+probiotics group was injected with 1 μl KA solution into the right amygdala and orally fed with 1 ml PBS containing calculated probiotics per day. At last, the KA+synbiotic group was injected with 1 μl KA solution into the right amygdala and orally fed with 1 mL PBS containing calculated synbiotics per day.
All the experimental rats were monitored via video for spontaneous seizures from days 1 to 28. Latency (the time from SE induction to the first seizure episode), frequency of spontaneous seizures, the duration of the seizures, and scores of the seizures were recorded and evaluated for analysis.
Morris water maze (MWM) test
The experimental rats were tested for spatial learning and memory ability using an MWM 14 days following seizure induction. This experimental device included a circular tank (divided into four quadrants), a platform (placed 1 cm below the water surface in one quadrant), and visual cues (placed inside the pool). Data were tracked and monitored using the Viewer 2 tracking software (China).
The rats were trained for five consecutive days (four 90-s trials per day) in the water maze. They were required to find the hidden platform within 90 s and were placed on different starting points for each trial. If the rats could not complete the goal within 90 s, they were directed and positioned on the platform for 10 s. The time each rat spent reaching the platform was recorded as the escape latency, with 90 s being the maximum time. On the sixth day, the learning ability and memory of the rats were assessed with a 90-s trial. In this trial, the platform was removed, and all rats were positioned in the quadrant opposite to the previous target quadrant. The escape latency, number of platform crossings, and time each rat spent in the target quadrant were recorded.
Brain sample collection
In total, 3 days following SE induction, all experimental rats were sacrificed, their hippocampal tissues were extracted, and placed in ice-cold saline. The hippocampal samples were blotted and weighed, and 100 mg of wet tissue was homogenized in 1 ml phosphate buffer (0.1 M, pH 7.4). The homogenate was centrifuged (3,000 rpm, 4°C, 15 min), and the supernatant was collected and stored at −80°C for biochemical assays.
Detection of biochemical parameters
The presence of the nucleotide 8-hydroxy-2-deoxyguanosine (8-OhdG) is an indicator of DNA damage. The concentrations of 8-OHdG were detected using an 8-OHdG assay kit (Nanjing Jiancheng Biotechnology Co., Ltd., China). Glutamate is an important excitatory neurotransmitter in the central nervous system, which was measured by using a Glutamate measurement kit (Nanjing Jiancheng Biotechnology Co., Ltd.). Malondialdehyde (MDA), a marker of lipid peroxidation, was detected using a thiobarbituric acid (TBA)-based spectrophotometric assay kit (Nanjing Jiancheng Biotechnology Co., Ltd.). The level of intracellular reduced glutathione (GSH) was measured using a GSH assay kit according to the manufacturer's protocol (Nanjing Jiancheng Biotechnology Co., Ltd.).
Concentrations of interleukin (IL)-1β, IL-6, interferon (IFN)-γ, and tumor necrosis factor (TNF)-α in the hippocampus were detected using ELISA kits according to the manufacturer's protocols (Nanjing Jiancheng Biotechnology Co., Ltd.). Ionized calcium-binding adapter protein-1 (Iba-1) and glial fibrillary acidic protein (GFAP) represent the activation of microglia and astrocytes, respectively. The levels of Iba-1 (Shanghai Runyu Biotechnology Co., Ltd., China) and GFAP (provided by Shanghai Zeye Technology Co., Ltd., China) were measured using ELISA kits according to the respective manufacturer's instructions.
Statistical analysis
All the experimental data were analyzed using SPSS statistical software (version 21.0) and GraphPad Prism software (version 7.0). The data are expressed as the mean ± standard deviation (SD). Differences between groups were compared using Student's t-test or repeated-measures ANOVA. Tukey's test was used as a post-hoc analysis for multiple comparisons. Statistical significance was set as follows: * P < 0.05, **P < 0.01.
Results
Modulating the gut microbiota ameliorates spontaneous seizures in epileptic rats
The effects of modulating gut microbiota on the characteristics of spontaneous seizures in rats following SE were investigated in this study (Figure 1). After SE induction, treatment with prebiotics, probiotics, and synbiotics extended the latency period (prebiotics, P < 0.05; probiotics, P < 0.01; synbiotics, P < 0.01), decreased the frequency of seizures (prebiotics, P < 0.05; probiotics, P < 0.01; synbiotics, P < 0.01), and decreased the duration of seizures (prebiotics, P < 0.05; probiotics, P < 0.01; synbiotics, P < 0.01). In particular, the effect of synbiotics on the latency period and frequency of seizures was more obvious. Regarding the severity of seizures, treatment with synbiotics significantly decreased the scores for spontaneous seizures (P < 0.05).
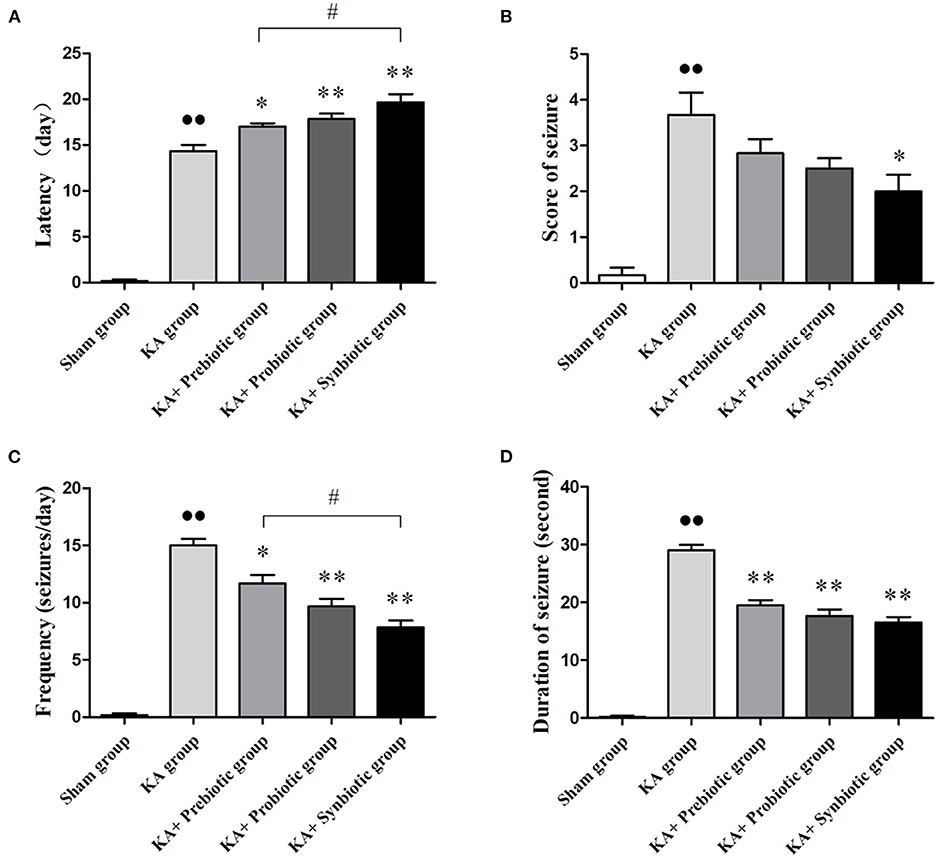
Figure 1. Effect of prebiotics, probiotics, and synbiotics on spontaneous seizures in rats following status epilepticus induction. (A) Latency. (B) Scores of seizures. (C) Frequency of seizures. (D) Duration of seizures. ∙∙ P < 0.01 vs. the Sham group, *P < 0.05 vs. the KA group, **P < 0.01 vs. the KA group, # P < 0.05 vs. the KA+synbiotic group. KA, kainic acid.
Modulating the gut microbiota improves cognitive deficit in epileptic rats
The MWM test was used to evaluate the learning and memory abilities of the experimental rats. As shown in Figure 2, the escape latency of rats from the five groups gradually reduced during the five-day trial. On the fifth day, the escape latency of rats in the KA group was much longer than that in the sham group (P < 0.01); treatment with prebiotics (P < 0.01), probiotics (P < 0.01), or synbiotics (P < 0.01) significantly reduced escape latency (Figure 2A).
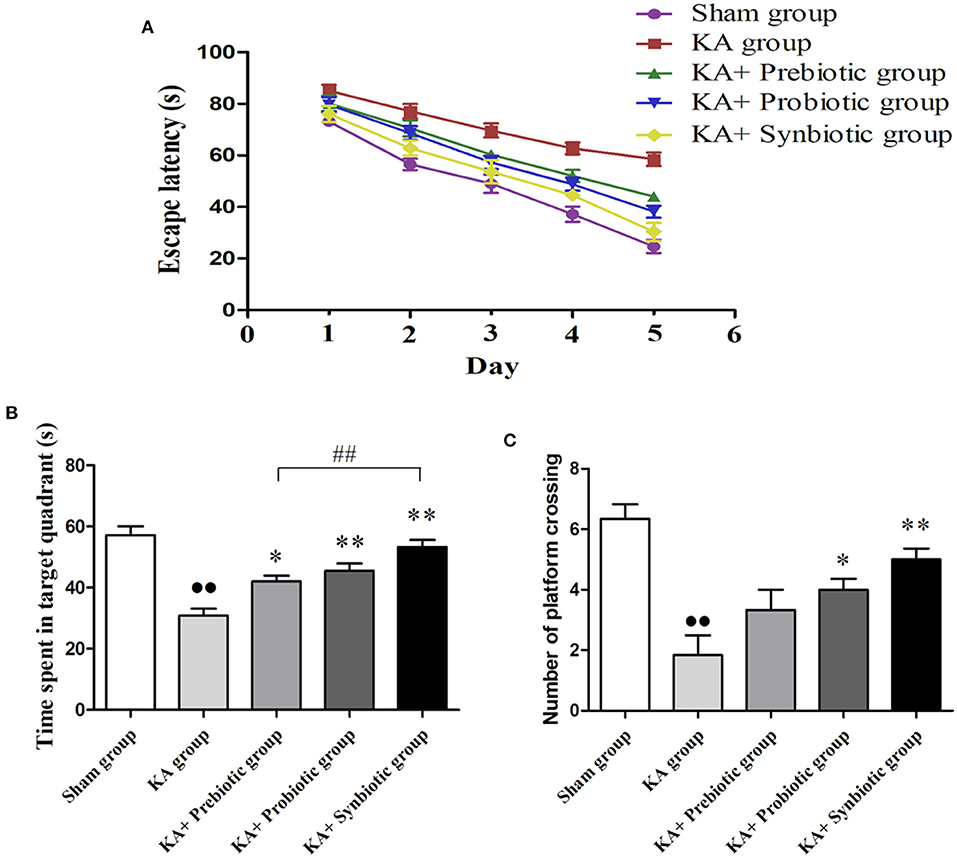
Figure 2. Effect of prebiotics, probiotics, and synbiotics on cognitive function in rats following status epilepticus induction. (A) Escape latency. (B) Time spent in the target quadrant. (C) Number of platform crossings. ∙∙ P < 0.01 vs. the Sham group, *P < 0.05 vs. the KA group, **P < 0.01 vs. the KA group, ## P < 0.01 vs. the KA+synbiotic group. KA, kainic acid.
On the sixth day of the spatial probe test, rats in the KA group spent less time in the target quadrant (P < 0.01, Figure 2B) and crossed fewer platforms (P < 0.01, Figure 2C) compared with the sham group. Administration of prebiotics (P < 0.05), probiotics (P < 0.01), or synbiotics (P < 0.01) significantly increased the time spent in the target quadrant (Figure 2B). The number of platform crossings (Figure 2C) was significantly increased by treatment with probiotics (P < 0.05) or synbiotics (P < 0.01).
Modulating the gut microbiota mitigates oxidative stress, DNA damage, and glutamate release following SE
The role of modulating the gut microbiota in oxidative stress, DNA damage, and glutamate in rats following SE was evaluated. As shown in Figure 3, the rats in the KA group exhibited significantly higher levels of MDA (P < 0.01), 8-OHdG (P < 0.01), and glutamate (P < 0.01) and lower levels of GSH (P < 0.01) than those in the sham group. Administration of prebiotics, probiotics, and synbiotics significantly decreased the levels of MDA (prebiotics, P < 0.05; probiotics, P < 0.05; synbiotics, P < 0.01), 8-OHdG (prebiotics, P < 0.01; probiotics, P < 0.01; synbiotics, P < 0.01), and glutamate (prebiotics, P < 0.05; probiotics, P < 0.05; synbiotics, P < 0.01), and increased the levels of GSH (prebiotics, P < 0.05; probiotics, P < 0.01; synbiotics, P < 0.01) in KA-induced rats.
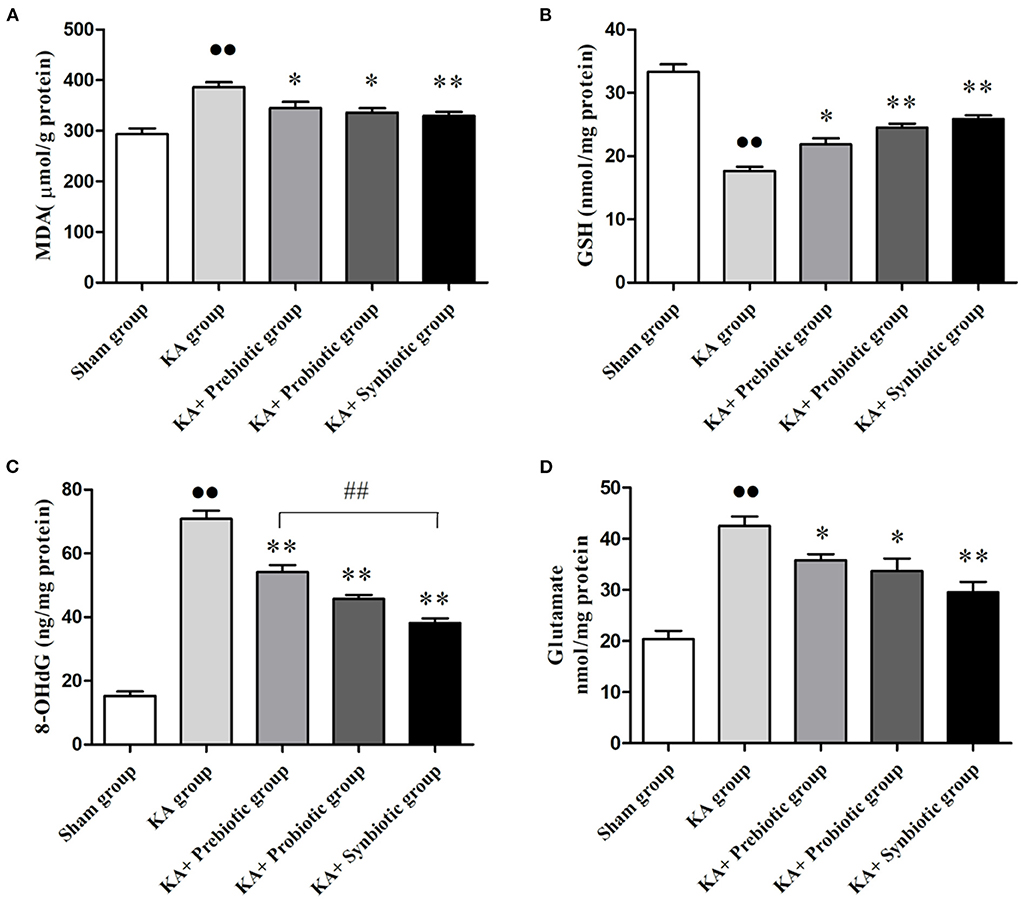
Figure 3. Treatment with prebiotics, probiotics, and synbiotics reduced the levels of MDA, 8-OHdG, and glutamate and increased the level of GSH in the hippocampus of rats after status epilepticus induction. (A) MDA. (B) GSH. (C) 8-OHdG. (D) Glutamate. ∙∙ P < 0.01 vs. the Sham group, *P < 0.05 vs. the KA group, **P < 0.01 vs. the KA group, ## P < 0.01 vs. the KA+synbiotic group. KA, kainic acid.
Modulating the gut microbiota inhibits the activation of astrocytes and microglia and reduces inflammation following SE
The role of modulating the gut microbiota on glial cells and inflammatory cytokines in rats following SE was also investigated, the results of which are shown in Figure 4. Rats in the KA group presented the activation of microglia (P < 0.01) and astrocytes (P < 0.01) and higher levels of IL-1β (P < 0.01), IL-6 (P < 0.01), and TNF-α (P < 0.01) compared with those in sham group. Treatment with prebiotics, probiotics, or synbiotics significantly inhibited the activation of microglia (prebiotics, P < 0.05; probiotics, P < 0.01; synbiotics, P < 0.01) and astrocytes (prebiotics, P < 0.05; probiotics, P < 0.05; synbiotics, P < 0.01) and reduced the levels of IL-1β (prebiotics, P < 0.05; probiotics, P < 0.01; synbiotics, P < 0.01), IL-6 (prebiotics, P < 0.01; probiotics, P < 0.01; synbiotics, P < 0.01), and TNF-α (prebiotics, P < 0.01; probiotics, P < 0.01; synbiotics, P < 0.01). No statistically significant difference was observed in IFN-γ levels between these groups.
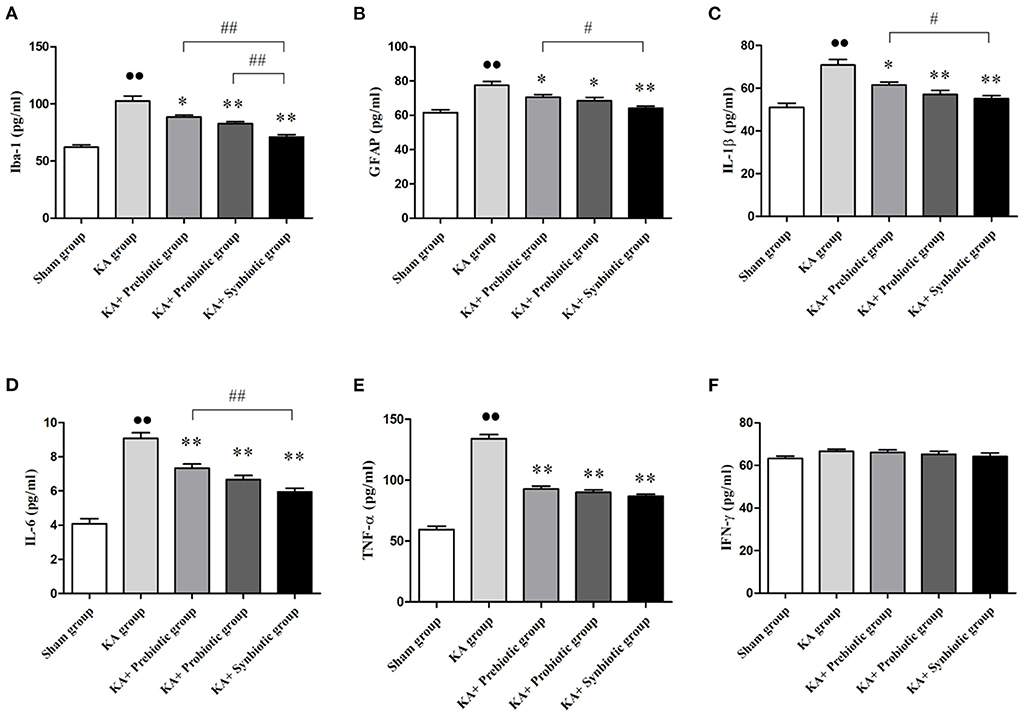
Figure 4. Treatment with prebiotics, probiotics, and synbiotics decreased the levels of Iba-1 and GFAP and inhibited the release of inflammatory cytokines such as IL-1 β, IL-6, and TNF- α. (A) Iba-1. (B) GFAP. (C) IL-1β. (D) IL-6. (E) TNF- α. (F) IFN- γ. ∙∙ P < 0.01 vs. the Sham group, *P < 0.05 vs. the KA group, **P < 0.01 vs. the KA group, ## P < 0.01 vs. the KA+synbiotic group. KA, kainic acid.
Discussion
This study found that modulating the gut microbiota had a positive impact on seizure control and cognitive deficits in KA-induced SE rats. Moreover, changes in oxidative stress parameters, DNA damage, glutamate release, activation of microglia and astrocytes, and levels of inflammatory cytokines in the hippocampal tissues were measured to study the role of modulating gut microbiota in their anti-seizure and cognition-enhancing effects.
Gut dysbiosis has gradually been recognized to be associated with epilepsy (22). Peng et al. studied alterations in gut microbiome composition between patients with drug-resistant epilepsy (DRE) and healthy individuals using 16S rDNA sequencing and found an increased abundance of rare flora in patients with DRE (8). In addition, those with four or more seizures per year showed lower levels of Bifidobacteria and Lactobacillus than those with fewer than four seizures per year (8). This study provides evidence that restoring the gut microbiota may be a novel therapeutic strategy for treating DRE. We used two bacterial strains, Bifidobacterium and Lactobacillus, as probiotics and inulin as a prebiotic, which were beneficial to the growth and activity of these probiotic strains. Our results showed that treatment with prebiotics, probiotics, and synbiotics ameliorated spontaneous seizures and cognitive deficits in KA-induced SE rats. As a matter of interest, synbiotics seemed to have a better curative effect than either prebiotics or probiotics alone.
Glial cell-mediated inflammation plays an important role in the development of epilepsy (23). A dysfunction in glial cells leads to the abnormal regulation of water, ions, and neurotransmitters, promoting hyperexcitability and hypersynchrony in the brain and increasing susceptibility to epilepsy (24). Uncontrolled glial-mediated immune reactions could cause a sustained inflammatory response and facilitate the development of epilepsy (24). Gut dysbiosis might be involved in the inflammatory mechanisms underlying epilepsy. Gut bacteria can affect neural networks by releasing neurotransmitters or their precursors, such as γ-aminobutyric acid (GABA) and glutamate (25, 26). The imbalance between excitatory and inhibitory neurotransmitters caused by gut dysbiosis contributes to the development of epilepsy. In addition, under normal conditions, gut bacteria can induce anti-inflammatory activity in the brain by stimulating the afferent neurons of the ENS via the vagus nerve. Disturbing normal bacterial abundance or function could disrupt the microbiota balance and lead to excess inflammation (27). Moreover, there may be a “leakage” in the integrity of the barrier system that participates in epileptogenesis, namely, the blood-brain barrier and intestinal mucosal barrier, which could also be affected by the gut microbiota. Gut dysbiosis may also induce excessive LPS production, which increases the permeability of the intestinal immune barrier, leading to epilepsy (27, 28). In the present study, we observed that modulating the gut microbiota via treatment with prebiotics, probiotics, and synbiotics effectively inhibited the inflammatory response and release of glutamate in the hippocampal tissues of rats following SE induction.
Oxidative stress is an important factor in the pathogenesis of epilepsy (29). Seizure activity can increase lipid peroxidation and decrease total antioxidant ability (30). The excessive generation of reactive oxygen species (ROS) can cause neuronal cell damage, which is associated with spontaneous seizures and cognitive deficits (29, 31). The gut microbiota may also influence the oxidative state in the central nervous system by interfering with the levels of ROS and the antioxidant system (32, 33). Abnormal changes in various metabolites, such as short-chain fatty acids, vitamins, and absorbable vitamins, can also regulate the oxidative state of the brain. These mechanisms have mostly been inferred from previous studies but still lack direct evidence. The present study confirmed that modulating the gut microbiota decreased lipid peroxidation, inhibited DNA damage, and enhanced total antioxidant ability, providing direct evidence that the gut microbiota affects the oxidative state during epilepsy.
Conclusion
The present study demonstrated that modulating the gut microbiota by treatment with prebiotics, probiotics, and synbiotics exerts an anti-seizure effect and ameliorates cognitive impairment in KA-induced epileptic rats. Moreover, the results highlighted that modulating the gut microbiota inhibited oxidative stress and the inflammatory response in the hippocampus of epileptic rats, suggesting that modulating the gut microbiota could be a new therapeutic strategy to inhibit the development of epilepsy.
Data availability statement
The original contributions presented in the study are included in the article/supplementary material, further inquiries can be directed to the corresponding author.
Ethics statement
The animal study was reviewed and approved by Animal Studies Subcommittee of the Capital Medical University.
Author contributions
XW wrote the draft of this manuscript. CY conducted the experiments. LY analyzed the data of this study. YZ revised the manuscript and gave the final approval. All authors contributed to the article and approved the submitted version.
Acknowledgments
We would thank Editage (www.editage.cn) for English language editing.
Conflict of interest
The authors declare that the research was conducted in the absence of any commercial or financial relationships that could be construed as a potential conflict of interest.
Publisher's note
All claims expressed in this article are solely those of the authors and do not necessarily represent those of their affiliated organizations, or those of the publisher, the editors and the reviewers. Any product that may be evaluated in this article, or claim that may be made by its manufacturer, is not guaranteed or endorsed by the publisher.
References
1. Banerjee PN, Filippi D, Allen Hauser W. The descriptive epidemiology of epilepsy-a review. Epilepsy Res. (2009) 85:31–45. doi: 10.1016/j.eplepsyres.2009.03.003
2. Yadav J, Singh P, Dabla S, Gupta R. Psychiatric comorbidity and quality of life in patients with epilepsy on antiepileptic monotherapy and polytherapy. Tzu Chi Med J. (2022) 34:226–31. doi: 10.4103/tcmj.tcmj_34_21
3. Pastorino GMG, Operto FF, Padovano C, Vivenzio V, Scuoppo C, Pastorino N, et al. Social cognition in neurodevelopmental disorders and epilepsy. Front Neurol. (2021) 12:658823. doi: 10.3389/fneur.2021.658823
4. Bhanudeep S, Madaan P, Sankhyan N, Saini L, Malhi P, Suthar R, et al. Long-term epilepsy control, motor function, cognition, sleep and quality of life in children with West syndrome. Epilepsy Res. (2021) 173:106629. doi: 10.1016/j.eplepsyres.2021.106629
5. Dalic L, Cook MJ. Managing drug-resistant epilepsy: challenges and solutions. Neuropsychiatr Dis Treat. (2016) 12:2605–16. doi: 10.2147/NDT.S84852
6. Terrone G, Balosso S, Pauletti A, Ravizza T, Vezzani A. Inflammation and reactive oxygen species as disease modifiers in epilepsy. Neuropharmacology. (2020) 167:107742. doi: 10.1016/j.neuropharm.2019.107742
7. Suganya K, Koo BS. Gut-brain axis: role of gut microbiota on neurological disorders and how probiotics/prebiotics beneficially modulate microbial and immune pathways to improve brain functions. Int J Mol Sci. (2020) 21:7551. doi: 10.3390/ijms21207551
8. Peng A, Qiu X, Lai W, Li W, Zhang L, Zhu X, et al. Altered composition of the gut microbiome in patients with drug-resistant epilepsy. Epilepsy Res. (2018) 147:102–7. doi: 10.1016/j.eplepsyres.2018.09.013
9. Lee H, Lee S, Lee DH, Kim DW, A. comparison of the gut microbiota among adult patients with drug-responsive and drug-resistant epilepsy: an exploratory study. Epilepsy Res. (2021) 172:106601. doi: 10.1016/j.eplepsyres.2021.106601
10. Cryan JF, Dinan TG. Gut microbiota: microbiota and neuroimmune signalling-Metchnikoff to microglia. Nat Rev Gastroenterol Hepatol. (2015) 12:494–6. doi: 10.1038/nrgastro.2015.127
11. Erny D, Hrabě de. Angelis AL, Jaitin D, Wieghofer P, Staszewski O, David E, et al. hosts Host microbiota constantly control maturation and function of microglia in the CNS. Nat Neurosci. (2015) 18:965–77. doi: 10.1038/nn.4030
12. Haslund-Vinding J, Mcbean G, Jaquet V, Vilhardt F, NADPH. oxidases in oxidant production by microglia: activating receptors, pharmacology and association with disease. Br J Pharmacol. (2017) 174:1733–49. doi: 10.1111/bph.13425
13. Ho YH, Lin YT, Wu CW, Chao YM, Chang AY, Chan JY. Peripheral inflammation increases seizure susceptibility via the induction of neuroinflammation and oxidative stress in the hippocampus. J Biomed Sci. (2015) 22:46. doi: 10.1186/s12929-015-0157-8
14. Mehrabadi S, Sadr SS. Assessment of probiotics mixture on memory function, inflammation markers, and oxidative stress in an Alzheimer's disease model of rats. Iran Biomed J. (2020) 24:220–8. doi: 10.29252/ibj.24.4.220
15. Reid G, Younes JA, Van Der Mei HC, Gloor GB, Knight R, Busscher HJ. Microbiota restoration: natural and supplemented recovery of human microbial communities. Nat Rev Microbiol. (2011) 9:27–38. doi: 10.1038/nrmicro2473
16. Azad MAK, Sarker M, Li T, Yin J. Probiotic species in the modulation of gut microbiota: an overview. BioMed Res Int. (2018) 2018:9478630. doi: 10.1155/2018/9478630
17. Kwok LY, Wang L, Zhang J, Guo Z, Zhang H, A. pilot study on the effect of Lactobacillus casei Zhang on intestinal microbiota parameters in Chinese subjects of different age. Benef Microbes. (2014) 5:295–304. doi: 10.3920/BM2013.0047
18. Rinaldi E, Consonni A, Guidesi E, Elli M, Mantegazza R, Baggi F. Gut microbiota and probiotics: novel immune system modulators in myasthenia gravis? Ann N Y Acad Sci. (2018) 1413:49–58. doi: 10.1111/nyas.13567
19. Mitrea L, Nemeş SA, Szabo K, Teleky BE, Vodnar DC. Guts imbalance imbalances the brain: a review of gut microbiota association with neurological and psychiatric disorders. Front Med (Lausanne). (2022) 9:813204. doi: 10.3389/fmed.2022.813204
20. Pandey KR, Naik SR, Vakil BV. Probiotics, prebiotics and Synbiotics- a review. J Food Sci Technol. (2015) 52:7577–87. doi: 10.1007/s13197-015-1921-1
21. Racine RJ. Modification of seizure activity by electrical stimulation. II Motor seizure Electroencephalogr. Clin Neurophysiol. (1972) 32:281–94. doi: 10.1016/0013-4694(72)90177-0
22. Lum GR, Olson CA, Hsiao EY. Emerging roles for the intestinal microbiome in epilepsy. Neurobiol Dis. (2020) 135:104576. doi: 10.1016/j.nbd.2019.104576
23. Wetherington J, Serrano G, Dingledine R. Astrocytes in the epileptic brain. Neuron. (2008) 58:168–78. doi: 10.1016/j.neuron.2008.04.002
24. Devinsky O, Vezzani A, Najjar S, De Lanerolle NC, Rogawski MA. Glia and epilepsy: excitability and inflammation. Trends Neurosci. (2013) 36:174–84. doi: 10.1016/j.tins.2012.11.008
25. Bravo JA, Forsythe P, Chew MV, Escaravage E, Savignac HM, Dinan TG, et al. Ingestion of Lactobacillus strain regulates emotional behavior and central GABA receptor expression in a mouse via the vagus nerve. Proc Natl Acad Sci U S A. (2011) 108:16050–5. doi: 10.1073/pnas.1102999108
26. O'Mahony SM, Clarke G, Borre YE, Dinan TG, Cryan JF. Serotonin, tryptophan metabolism and the brain-gut-microbiome axis. Behav Brain Res. (2015) 277:32–48. doi: 10.1016/j.bbr.2014.07.027
27. Blander JM, Longman RS, Iliev ID, Sonnenberg GF, Artis D. Regulation of inflammation by microbiota interactions with the host. Nat Immunol. (2017) 18:851–60. doi: 10.1038/ni.3780
28. Chatzikonstantinou S, Gioula G, Kimiskidis VK, Mckenna J, Mavroudis I, Kazis D. The gut microbiome in drug-resistant epilepsy. Epilepsia Open. (2021) 6:28–37. doi: 10.1002/epi4.12461
29. Méndez-Armenta M, Nava-Ruíz C, Juárez-Rebollar D, Rodríguez-Martínez E, Gómez PY. Oxidative stress associated with neuronal apoptosis in experimental models of epilepsy. Oxid Med Cell Longev. (2014) 2014:293689. doi: 10.1155/2014/293689
30. Abdel-Wahab BA, Al-Qahtani JM, El-Safty SA. Omega-3 polyunsaturated fatty acids in large doses attenuate seizures, cognitive impairment, and hippocampal oxidative DNA damage in young kindled rats. Neurosci Lett. (2015) 584:173–7. doi: 10.1016/j.neulet.2014.10.003
31. Mishra P, Mittal AK, Rajput SK, Sinha JK. Cognition and memory impairment attenuation via reduction of oxidative stress in acute and chronic mice models of epilepsy using antiepileptogenic nux vomica. J Ethnopharmacol. (2021) 267:113509. doi: 10.1016/j.jep.2020.113509
32. Jones RM, Mercante JW, Neish AS. Reactive oxygen production induced by the gut microbiota: pharmacotherapeutic implications. Curr Med Chem. (2012) 19:1519–29. doi: 10.2174/092986712799828283
Keywords: epilepsy, gut dysbiosis, oxidative stress, inflammation, cognition
Citation: Wang X, Yang C, Yang L and Zhang Y (2022) Modulating the gut microbiota ameliorates spontaneous seizures and cognitive deficits in rats with kainic acid-induced status epilepticus by inhibiting inflammation and oxidative stress. Front. Nutr. 9:985841. doi: 10.3389/fnut.2022.985841
Received: 04 July 2022; Accepted: 01 August 2022;
Published: 29 August 2022.
Edited by:
David Vauzour, University of East Anglia, United KingdomReviewed by:
Yuri Zilberter, INSERM U1106 Institut de Neurosciences des Systèmes, FranceUfuk Okkay, Atatürk University, Turkey
Copyright © 2022 Wang, Yang, Yang and Zhang. This is an open-access article distributed under the terms of the Creative Commons Attribution License (CC BY). The use, distribution or reproduction in other forums is permitted, provided the original author(s) and the copyright owner(s) are credited and that the original publication in this journal is cited, in accordance with accepted academic practice. No use, distribution or reproduction is permitted which does not comply with these terms.
*Correspondence: Yongbo Zhang, eW9uZ2JvemhhbmcmI3gwMDA0MDtjY211LmVkdS5jbg==