- 1Graduate Program in Child and Adolescent Health (PPGSCA), Medical School of Universidade Federal do Rio Grande do Sul (UFRGS), Hospital de Clínicas de Porto Alegre (HCPA), Porto Alegre, Brazil
- 2Graduate Program in Food, Nutrition and Health, Medical School of Universidade Federal do Rio Grande do Sul (UFRGS), Hospital de Clínicas de Porto Alegre (HCPA), Porto Alegre, Brazil
Background and aims: Early child interventions focused on the family prevented neurodevelopmental and behavioral delays and can provide more knowledge regarding responsive feeding, thus creating learning opportunities to promote better quality nutrition and preventing failure to thrive. The aim is to verify the impact of a continuous program of early home-based intervention on the body composition of preschool infants who were born preterm with very low birth weight (VLBW).
Methods: This is a longitudinal analysis from a randomized controlled trial, including VLBW preterm children, born in a tertiary hospital in Southern Brazil and followed up at the high-risk institutional ambulatory clinic. Participants were divided into the intervention group (IG): skin-to-skin care with the mother (kangaroo care), breastfeeding policy, and tactile-kinesthetic stimulation by mothers until hospital discharge. Subsequently, they received a program of early intervention with orientation and a total of 10 home visits, independently from the standard evaluation and care that was performed following the 18 months after birth; conventional group (CG): standard care according to the routine of the newborn intensive care unit (NICU), which includes kangaroo care, and attending to their needs in the follow-up program. Body composition estimation was performed using bioelectrical impedance analyses (BIA), and physical activity and feeding practices questionnaires were evaluated at preschool age, as well as anthropometric measurements and biochemical analysis.
Results: Data of 41 children at 4.6 ± 0.5 years old were evaluated (CG n = 21 and IG n = 20). Body weight, height, body mass index, waist and arm circumferences, and triceps and subscapular skinfold did not differ between groups. The IG presented higher segmented fat-free mass (FFM) when compared to the CG (right arm FFM: 0.74 vs. 0.65 kg, p = 0.040; trunk FFM: 6.86 vs. 6.09 kg, p = 0.04; right leg FFM: 1.91 vs. 1.73 kg, p = 0.063). Interaction analyses showed that segmented FFM and FFM Index were associated with higher iron content in the IG. In the CG, interaction analyses showed that increased visceral fat area was associated with higher insulin resistance index.
Conclusion: An early intervention protocol from NICU to a home-based program performed by the mothers of VLBW preterm children of low-income families presents a small effect on FFM.
Introduction
Preterm birth is considered a health problem worldwide, with a higher incidence in low-income countries, with an average of 12%, compared to 9% in high-income countries (1). Despite preterm birth being the leading cause of death of children aged under 5 years, the improvement of neonatal care and follow-up programs to support this population is increasing survival rates, allowing more children to enter adulthood (2). Furthermore, preterm infants, mainly those born extremely preterm, present a higher incidence of developmental deficits (cognitive, motor, behavioral, communicative, learning, and sensory disorders) and a higher risk for delayed neurodevelopment, a condition that demands early implementation of multidisciplinary actions that may prevent negative outcomes (3, 4). Moreover, preterm infants present a higher risk for chronic and metabolic diseases with aging (5, 6).
Among the factors that increase their vulnerability to developing growth and developmental issues (5) is body composition, which has been associated with neurodevelopment in very low birth weight (VLBW) infants (7, 8). A higher rate of fat-free mass (FFM) was associated with improved cognitive and motor scores at 12 months of corrected age (9), and, on the other hand, a deficit of FFM was associated with neurological impairment in VLBW infants at 24 months of corrected age (10). Body composition studies have shown that preterm infants reach full-term equivalent age with less FFM and a higher percentage of total body fat (%TBF) when compared to their full-term equivalent counterparts (11, 12), as well as lower bone mineral density (13, 14) and increased abdominal adipose tissue (15). Since body growth and mineral accretion occur mainly in the last trimester of gestation, reduced muscle mass and skeletal mineralization could be related to preterm infants (12). Moreover, body fat and FFM gains in preterm infants are associated with several areas of cognitive function (16). Thus, early intervention, follow-up care for preventive action, and timely detection of possible adverse health outcomes are critical for preterm infants’ growth health.
The “first 1,000 days,” from conception to 24 months (17), are characterized as a window of opportunity to stimulate a child’s developmental domains, such as physical, language, cognitive, and social–emotional (18, 19). A systematic review showed that early intervention significantly affects child development, but it does not affect linear growth, which is more associated with nutritional intervention (20). The literature describes that nutritional intervention promotes short- and long-term health effects after preterm birth (21). Moreover, early tactile and kinesthetic stimulation in VLBW preterm children promoted a borderline higher psychomotor development and increased cognitive development assessed at 2 years corrected age, which did not affect weight, length, and head circumference (22). Early physical therapy intervention also presented a positive impact on VLBW preterm, hence reducing the incidence of motor delay (23). However, it is still not clear if an early intervention program could affect the body composition of the preterm population with advanced age. A single-blind cluster randomized controlled trial showed that early physical activity in the first months of life in term-born children promoted a reduced sum of skinfold, when compared to the non-stimulated group, without any differences in motor development (24).
There are few studies evaluating body composition in preschool VLBW preterm children subjected to early intervention and continuous clinical follow-up. Thus, our main goal is to investigate if a protocol of early home-based intervention program during the first 18 months of corrected age in VLBW preterm affected the body composition once they reach preschool age, comparing them with a group subjected to conventional care protocol. Also, this study investigated if the body composition results in response to the intervention protocol were related to neonatal, growth, and biochemical characteristics evaluated during these follow-ups.
Materials and methods
Design and study population
This was a longitudinal analysis from a randomized controlled trial that investigated preschool VLBW preterm children that were subjected to an early, continuous, and global intervention with a parent’s orientation program in the first 12–18 months of corrected age. This program was a randomized clinical trial (RCT), performed from 2016 to 2019, previously described in the study protocol (4), with children born in the Hospital de Clínicas de Porto Alegre (HCPA), a level-3 referral center for high-risk neonates in South Brazil, with regular follow-up until 5 years of age. The study population was preterm children born at less than 32 weeks of gestational age (GA) or children of birth weight less than 1,500 g during the 48 h after childbirth. Newborns with a major congenital malformation or inborn errors of metabolism, STORCH complex infections, HIV, or autoimmune conditions were excluded.
For the follow-up study, the parents were invited to accompany their children, aged 3 and 5 years, to perform a body composition analysis (Figure 1). Exclusion criteria for this investigation were children that died before reaching preschool age, children with severe motor, cognitive, or organic sequelae that prevented the use of bioimpedance scale (cerebral palsy, autism spectrum disorder, use of orthoses, tracheostomized children, and gastrostomy), and children who did not complete 3 years of age during the study period. The recruitment was performed by phone calls and use of social media to locate the parents with no updated phone numbers in the medical records.
The preschool children evaluated in this study were those who were randomized to one of the two groups from the previous clinical trial (4). The intervention group (IG) was characterized by skin-to-skin care (kangaroo care), breastfeeding policy, and massage therapy made by the mothers until hospital discharge. After discharge, mothers received orientation for continuous global stimulation at home plus 10 home visits by the research team, regardless of the standard evaluation and care provided in the follow-up clinic to all preterm children. The systematic early intervention program was based on developmental milestones, anticipating by a month the evolutionary step acquisition of motor and/or cognitive expected for corrected age. The conventional group (CG) was characterized as receiving standard care according to the routine care of the newborn intensive care unit (NICU) and according to the subject’s needs in the follow-up program. The follow-up program used in this study is an extension of neonatal and perinatal care, in which the research team provides conditions to monitor growth, development, and common morbidities with a multidisciplinary team who can fully assess the child and the caregivers, parents, family members, and the school.
Outcome measures
Physical exam during newborn intensive care unit and follow-up at an institutional ambulatory clinic
Physical exams for measurements of weight (kg), height (cm), head circumference (cm), and body mass index (BMI, kg/m2) were performed at birth, at NICU discharge, and at monthly and annual assessments as a routine appointment at the ambulatory clinic until the appointment for bioelectrical impedance analysis (BIA). All measurements in the ambulatory clinic were performed by a Neonatologist, using standard techniques and calibrated instruments (electronic scale and stadiometer). Z-scores of weight-for-age (W/A), length-for-age (L/A), and BMI-for-age (BMI/A) were evaluated using the Anthro® software (25), considering overweight: BMI/A Z-score > + 1, obesity: BMI/A Z-score > + 2, and underweight: BMI/A Z-score < –2.
At the evaluation appointment for BIA in the preschool-age children, all anthropometric measurements were performed in duplicate for which the average results were considered: waist circumference (cm) measured with an inelastic measuring tape (in cm) placed at the umbilical scar level at the end of the child’s exhale in orthostatic position; arm circumference (cm), measured in the mid-point of the upper arm; triceps skinfold thickness (mm), measured by the midpoint between the acromion and the olecranon; and, subscapular skinfold thickness (mm), measured diagonally below the inferior angle of the scapula. Skinfold measurements (triceps and subscapular) were assessed using a skinfold caliper (Lange®, Ann Arbor, Michigan, USA).
Socioeconomic status
Data related to the mother’s schooling level (in years) and household income (in BRL per month) were assessed on the day of the follow-up appointment when participants reached preschool age, on the same day of BIA.
Neonatal and follow-up data
Neonatal information from the NICU stay, follow-ups, and clinical appointments was collected from in-hospital patient records. During the NICU period, maternal variables were collected (maternal age, preeclampsia, gestational diabetes, and use of corticosteroid). The neonatal variables were gestational age (GA—evaluated by last menstrual period and confirmed by early obstetrical ultrasound and neonatal clinical examination), birth weight, gender, the status of small for gestational age (SGA; defined as birth weight < 10th percentile) (26), 5-min Apgar score, length of hospital stay, necrotizing enterocolitis, central nervous system injury (periventricular hemorrhage and/or leukomalacia), bronchopulmonary dysplasia as oxygenotherapy at 36 weeks corrected age, retinopathy of prematurity, and packed red blood cell transfusion. Anthropometric measures were collected at NICU discharge.
In addition to physical exams in the follow-up clinic, information regarding breastfeeding practices was examined and classified as exclusive breastfeeding, exclusive infant formula, or combination feeding. Exclusive breastfeeding is the action of feeding solely with human milk coming from the mother’s breast, with no other liquids or solids (27).
A biochemical exam was also assessed in the records in the follow-up consultation at the 24th month of corrected age. Venous blood sampling (12 h fasting) was collected for routine blood tests according to the follow-up program for measuring hemogram; serum iron (μg/dL); total iron binding capacity (μg/dL); ferritin and transferrin (mg/dL); total cholesterol; LDL-cholesterol; HDL-cholesterol; non-HDL cholesterol and triglycerides (mg/dL); glucose (mg/dL); insulin (μU/mL); and cortisol (μg/dL). Dyslipidemia was determined when one or more lipid markers presented values, such as total cholesterol > 200 mg/dl; LDL-cholesterol > 130 mg/dL; HDL-cholesterol < 40 mg/dL; non-HDL cholesterol > 145 mg/dL; and triglycerides > 100 mg/dL (28). The insulin resistance index was calculated as a homeostatic model assessment for insulin resistance (HOMA-IR) [(glucose (mg/dL) × insulin (μU/mL))/405].
Body composition, feeding practices, and physical activity at preschool age
Body composition was evaluated using a multi-frequency bioelectrical impedance analysis (BIA) using InBody 770® (Biospace, South Korea), a tetrapolar electrode configuration system. Parents were instructed to fast their children for at least 3 h before the measurement, and diapers were changed before children got up on the scale. Each participant was positioned in an orthostatic position on a platform with lower electrodes for the feet and the hands holding onto upper electrodes, in which children were to hold this position for 1 min until completion of the measurement. This evaluation accurately measures body weight (BW in kg), the body water content in liters (total body water (TBW), water inside and outside cells, and water in the segments), fat mass (FM; in kg), total body fat (%TBF), fat-free mass (FFM = BW–fat mass, in kg; segmented FFM of arms, legs, and trunk—the head is not considered in the segmented measurements), FFM Index (FFMI, in kg/m2), lean mass (LM = water + proteins + non-osseous mineral; in kg), skeletal muscle mass (in kg), proteins and minerals (in kg), bone mineral content (BMC = osseous mineral; in kg), visceral fat area (in cm2), cellular body mass (in kg), arm circumference (in cm), and basal metabolic rate (BMR, in kCal).
Physical activity levels were measured using a structured questionnaire that estimates the sedentary and active time of children, considering activities on weekdays or weekends and day shifts (morning, afternoon, and night), presented as total hours of activity per week. The measure of physical activity is expressed by the daily time of participation in games and outdoor play while the measure of sedentary behavior is based on the time spent watching television.
Data analysis
Descriptive statistics are shown as mean with standard deviation (± SD) or median with interquartile range for continuous variables, according to the Shapiro-Wilk normality test, and counts with proportions for categorical variables. Between-group comparisons were performed with Student’s t-test, Mann-Whitney U-test, Pearson’s Chi-squared test, or Fisher’s exact test. Cohen’s d was used to measure effect size for quantitative variables. The power to compare the FFM segment averages between conventional and intervention groups was calculated considering a 5% significance level and the sample size of this study (IG = 20 and CG = 21). First, the relationship between neonatal data (GA, birth weight, and head circumference), physical exam results at 12 months (body weight, height, and BMI/A), and biochemical variables with body composition were explored (TBW, FM, FFM, and BMC) by Pearson’ or Spearman’ correlation (a hypothesis-generating analyses). Analysis of covariance (ANCOVA) was performed to compare body composition outcomes between groups and to analyze the relationship between them and the biochemical variables. They were adjusted for gender due to literature differences between outcomes by gender (29–31). P-values under 0.05 were considered statistically significant. All analyses were performed using the SPSS (Statistical Package for the Social Science) Program Version 18.0 (IBM SPSS Statistical for Windows, Armonk, New York, USA).
Results
Our investigation evaluated 41 VLBW preterm children at preschool age who participated previously in a clinical trial of an early intervention program developed from February 2016 to December 2019. Briefly, of the 134 children from the previous clinical trial, 84 were eligible to participate in this new investigation (Figure 1). For the current study, BIA and physical exam results were collected from December 2020 to June 2022 from the children whose guardians have accepted to participate.
Table 1 shows the characteristics of infants of the general sample population, as well as their respective groups, CG (n = 21) and IG (n = 20). The mean age of children when BIA was performed was 4.7 ± 0.5 years, 17 (41%) girls and 24 (59%) boys. There were no differences in anthropometric variables, such as body weight, height, waist and arm circumferences, and skinfold measurements from the children of both groups. Overall, 22% of the sample were classified as overweight or obese and 7% as underweight, without any significant differences between groups. Maternal schooling level and family income did not differ between groups. Regarding neonatal data of the preterm children randomly assigned in each group, the mean gestational age was 28 ± 2 weeks, birth weight 1,073 ± 318 g, head circumference 25.5 ± 2.4 cm, and 4 (11%) were SGA without statistical differences between groups. Also, neonatal comorbidities were not statistically different between groups. In both groups, birth weight correlated positively with TBW (IG: r = 0.462, p = 0.041; CG: r = 0.501, p = 0.021) and with FFM (IG: r = 0.461, p = 0.041; CG: r = 0.487, p < 0.025). Birth weight also correlated positively with FM only in CG: r = 0.472, p = 0.031 (IG r = 0.289, p = 0.22), and no significant association with BMC was observed. GA did not present a correlation with TBW, FFM, FM, and BMC when analyzed in each group separately (p > 0.05).
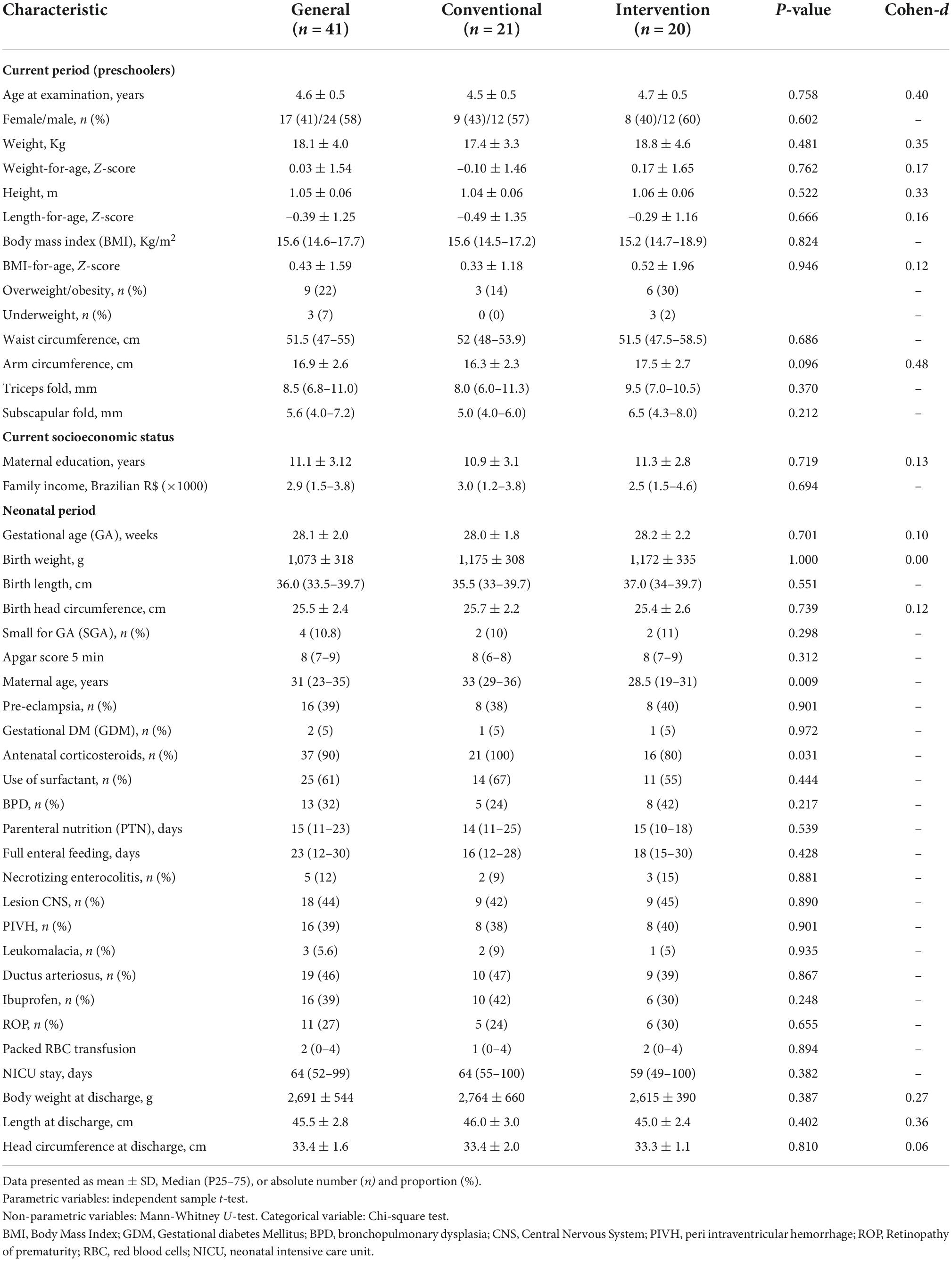
Table 1. Current and neonatal characteristics of preterm children subjected to the early intervention program compared to conventional care.
Physical examination performed during the first follow-up appointment after NICU discharge and 12 months corrected age did not show a statistical difference between groups. The nutrition offered to the preterm children in the first weeks of life at home did not vary between groups (Table 2). Biochemical analyses performed at 24 months of corrected age did not show statistical differences among groups (Table 3).
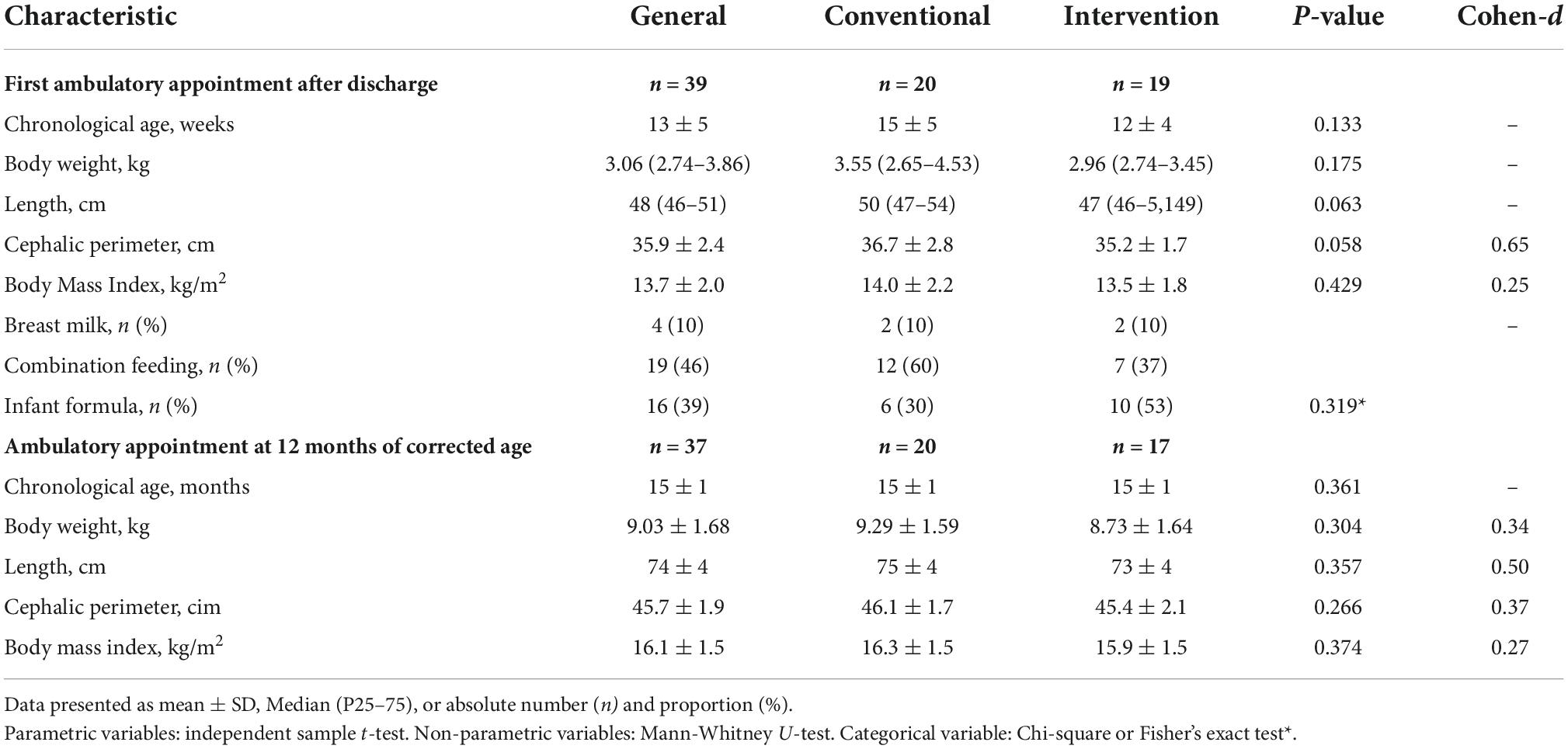
Table 2. Physical exam in the first ambulatory follow-up visit after NICU discharge and 12 months corrected age of preterm children subjected to early intervention program compared to conventional care.
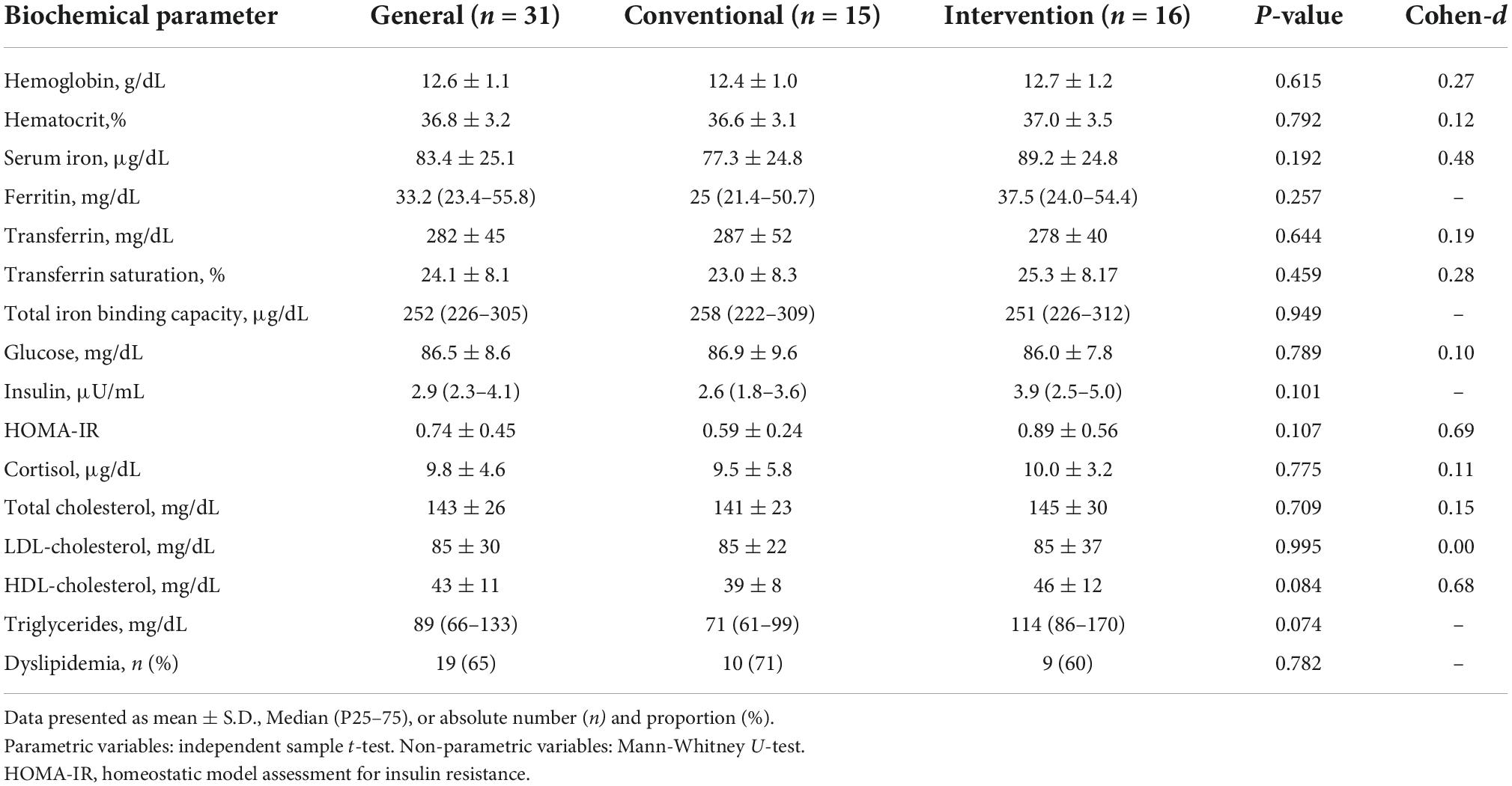
Table 3. Biochemical analysis in the follow-up ambulatory care at 24 months corrected the age of preterm infants subjected to an early intervention program compared to conventional care.
Table 4 shows the results of the BIA in preschool age. FM and %TBF did not differ significantly between CG and IG groups. Although the IG presented a non-significant increase in total FFM, FFMI, and skeletal muscle mass when compared to the GC group, they demonstrated a significant increase in segmented FFM in the children subjected to early intervention (right arm (p = 0.040), left arm (p = 0.053), trunk (p = 0.040), and an overall tendency in right leg (p = 0.063) and left leg (p = 0.054). The statistical power to test if there were a minimal difference of 0.09 kg in the mean of right arm FFM between groups was 57%. For the means of trunk FFM, a power of 53% was calculated, considering a minimal difference of 0.77 kg between groups. Moreover, the means of left leg FFM yielded a 51% power, considering a minimal difference of 0.19 kg among groups. These power values were obtained considering a 5% statistical level. TBW content also showed an increased pattern, as observed in the FFM from IG, although not significant. BMC, BMR, and visceral fat area were similar between groups. Finally, we observed that TBW/FFM ratio in preterm children was 74.3% (95% CI 74.0–74.5).
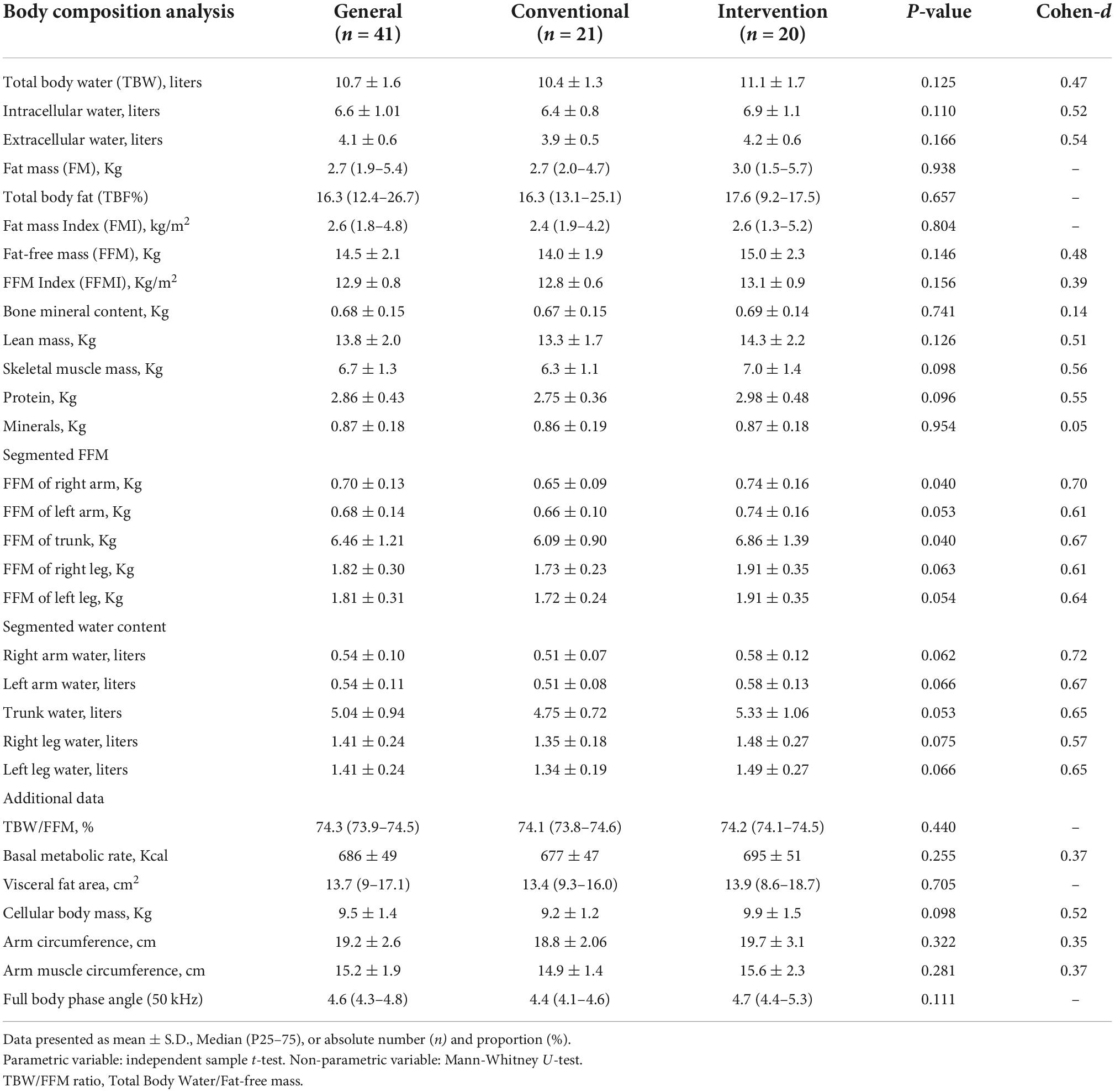
Table 4. Body composition in preterm infants subjected to early intervention program compared to conventional care.
Regarding physical activity, total active hours during the week were significantly higher in CG (median 16 h (14–19.5) when compared to IG [10 h (6–18); p = 0.015)] (data not shown).
Analyses of interaction were performed to investigate if the association between body composition and biochemical analyses differed among groups. Overall, Figure 2 shows that FFM with iron content differed between IG and CG, even after adjusting by gender. IG group showed that FFMI increased by 0.26 kg/m2 (95%CI: 0.002–0.049; p = 0.037) per unit of increased serum iron, but no increase was observed in CG. Moreover, IG showed an increase of segmented FFM per unit of iron content (adjusted by gender): right arm: 0.004 kg (95%CI: 0.000–0.008; p = 0.036), left arm 0.004 kg (95%CI: 0.000–0.008; p = 0.051), trunk 0.025 kg (95%CI: 0.008–0.058; p = 0.132), right leg 0.009 kg (95%CI: 001–0.017; p = 0.037), and left leg 0.009 kg (95%CI: 0.00–0.017; p = 0.043) per unit of iron content. Visceral fat area interaction significantly decreased to 37 cm2 (95%CI: –50 to –25; p < 0.001) per unit of HOMA-IR in IG, an opposite response when compared to CG (Figure 2E) (removing the two outliers, interaction is still significant, p = 0.005**).
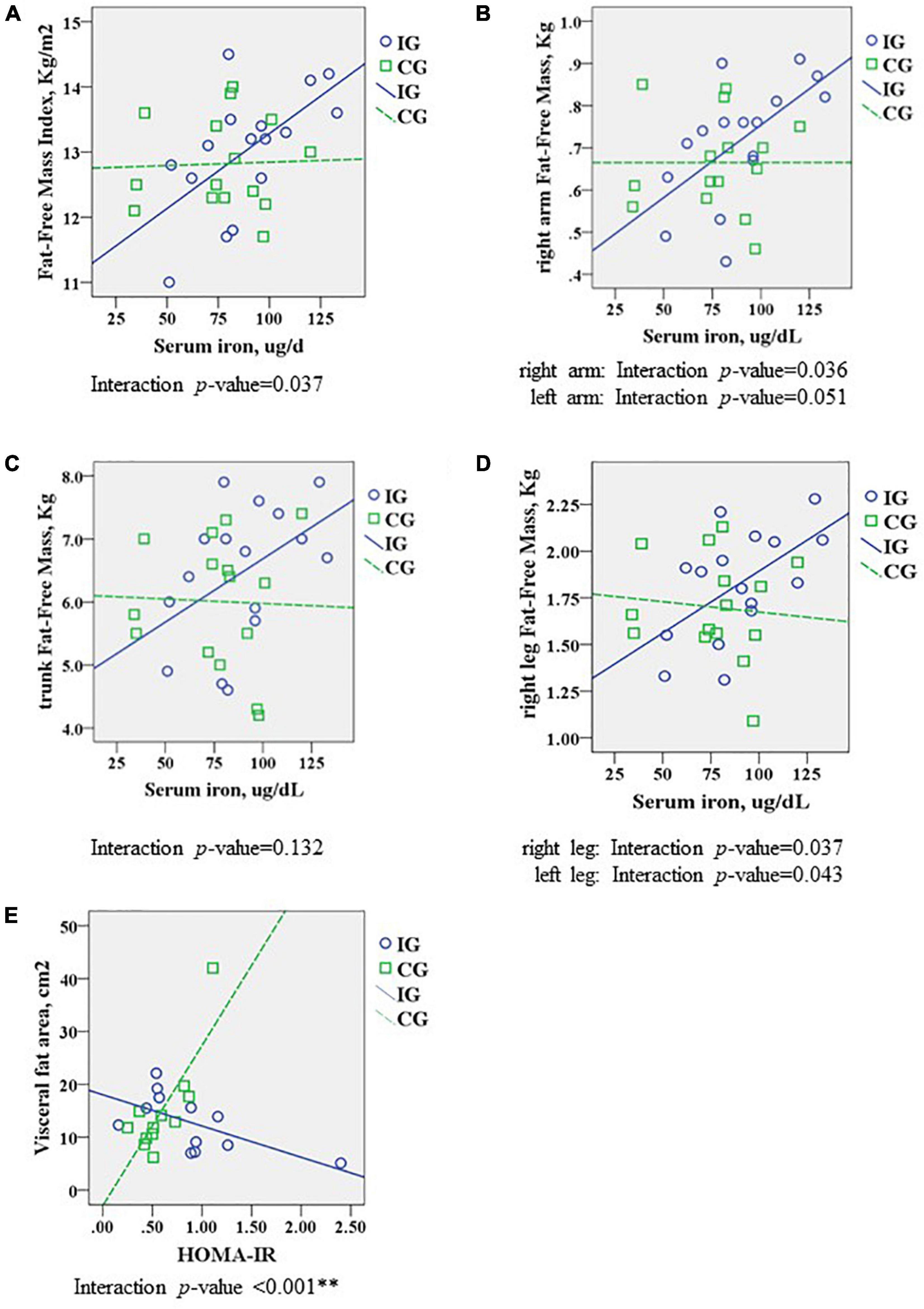
Figure 2. Comparison of body composition analysis at preschool age between interventional group (IG) and conventional group (CG), analyzing the interaction with biochemical blood results (blood exam at 24 months of corrected age), adjusted by gender. Association of (A) FFM Index and serum iron, (B) right arm FFM and serum iron, (C) trunk FFM and serum iron, (D) right leg FFM and serum iron, and (E) visceral fat area and HOMA-IR. Data were analyzed using ANCOVA, presenting interaction significant with a p-value < 0.05. FFM, fat-free mass; HOMA-IR, homeostatic model assessment for insulin resistance. **Removing the two outliers, interaction is still significant (p = 0.005).
There was no significant interaction between the FFMI and segmented FFM with physical activity when adjusted by gender. Also, no association was established between feeding practices and FFM (data not shown).
Discussion
For this study, we investigated the body composition of preterm VLBW children at preschool age who were subjected to a protocol of skin-to-skin care and global stimulation in a home-based program for 18 months. This investigation observed that early intervention may increase FFM in the body segments. On the other hand, despite stimulation implementation and closer family monitoring, no change in fat mass was observed. Nonetheless, this protocol may present a positive effect on reducing the relationship between visceral fat mass and insulin resistance.
Early life exposures to certain environmental factors during critical periods of development and growth may have significant short- and long-term consequences on an individual’s health: for that reason, early intervention is a strategy to improve growth and developmental outcomes (32, 33). In preterm infants, studies have shown that early intervention can improve cognition, increases growth and global development (34, 35), and attenuate the decrease in bone strength that may reduce the risk of osteopenia (36). Moreover, it presents a positive effect on motor skills through environmental enrichment (37). However, there are few early interventions implemented in the “first 1,000 days” and limited data on these effects in VLBW children regarding body composition across childhood.
Growth assessment is generally based on anthropometric measurements, which gives insufficient attention to growth quality. Thus, the assessment of body composition through BIA provides additional information on the relationship between growth and development (12). In a cohort study that compared extremely preterm children with full-term, in whose body composition was measured by DEXA, it was observed that preterm children presented the same height and weight as full-term. However, the preterm group presented lower values for muscle mass [0.9 kg (95%CI: 0.3–1.5)], total bone mineral density z-score [0.30 units (95%CI: 0.13–0.52)] and fat mass ratio [0.14 units (95%CI 0.06–0.21)] (13). In our study, the early stimulation protocol was performed anticipating, by a month, the motor and cognitive acquisition steps expected for corrected age, approaching near-term development; the usual growth evaluations (BMI, body circumferences, and skinfolds) did not present differences over the first year between intervention and conventional groups. Nonetheless, BIA showed that the early intervention program caused a small, but significant increase of segmented FFM, a body component comprised of skeletal muscle mass, body cell mass, total body water, connective tissue, and bone mineral mass. The use of a multi-frequency BIA provides a more direct portrayal of water compartments, increasing results reliability (38). Skeletal muscle accounts for a large proportion of the FFM, and it is known that physical activity positively affects FFM accretion from birth onward (39). Loss of muscle mass is associated with poor prognosis, reduced quality of life, and increased mortality, thus highlighting the muscle as an important component of whole-body metabolism, glucose homeostasis, as well as overall health and wellbeing (40). A systematic review showed that preterm infants present less lean tissue but a similar fat mass than full-term infants (11). Muscle growth can be activated by mechanical, oxidative, and energetic distress, and influenced by the availability of nutrients, growth factors, and cytokines (41). Moreover, an experimental study showed that mechano-signaling pathways stimulated by passive movements can control myofibrillar protein synthesis (42). Our finding showed that early intervention increased FFM, corroborating a previous investigation in which motor physical therapy in preterm in NICU increased lean mass (43). The preterm infants from the intervention group presented more FFM, despite performing less physical activity during the week compared to CG. The evaluation was done by physical questionnaire and supported our hypothesis that early intervention may contribute to an increase in the components of FFM. Moreover, an observational study showed that the gain of FFM in the first 4 years of the life of preterm children was associated with higher full-scale IQ and processing speed performance, which may enhance preschool cognitive performance (16).
Evidence from the classical birth cohorts from Pelotas, South Brazil showed that prematurity was associated with decreased total body fat and FFM, but with higher fat mass in adulthood (in male) (44). Higher fat mass is associated with an increased risk for metabolic syndrome in the preterm population (45). The early intervention protocol did not impact fat mass or bone mineral content within our intervention group. The metabolic bone mineral disease of prematurity is highly prevalent in VLBW preterm as it may occur due to loss of mineral transfer from the placental in the latest trimester and the reduced mechanical stimulation from the fetus against the uterine wall. This deficiency could be prevented by minerals and vitamin D intake, as well as physical activity (46–48). We did not observe changes in bone mineral content in response to early intervention protocol. However, a study with motor stimulation in preterm infants (26–34 weeks), with birth weight < 1,600 g, was able to increase bone mineral content evaluated by DEXA (43). A clinical study with extreme preterm-born young adults presented reduced area bone mineral density when compared to sex- and age-matched full-term controls, showing the long-term consequences of bone health (14).
Biochemical analysis performed in the follow-up clinic, at 24 months of corrected age, may support the structural findings observed in response to the early stimulation protocol. A significant relationship between iron content and FFM was observed only in IG, suggesting that the mechanical and global stimulus positively affects the components of FFM, such as the skeletal muscle which is increased by 700 g on average when compared to the CG group. Iron is an essential component of hemoglobin and myoglobin, in which iron supports muscle metabolism and healthy connective tissue, and is essential for physical growth, neurological development, and cellular function (49). Since iron is a micronutrient necessary for early development, it could be postulated that implementing an early intervention protocol of 18 months could improve muscle function, thus promoting iron homeostasis in the muscle system (50). Although no difference was observed in iron content between groups, a recent experimental study of a neurological disease demonstrated that regular physical exercise modulates iron homeostasis, in which dysregulation of iron metabolism leads to pathophysiological pathways (51).
Clinical studies indicate that preterm individuals have physiological disease pathways that differ from those born at full-term (52). In this same context of different mechanisms between preterm and full-term individuals, we observed that the hydration factor (TBW/FFM) in our preschool preterm children was 74.3%, a higher percentage than the assumed value for euhydrated individuals, which is set as 73.2%. A systematic review showed that preterm newborns present a higher TBW percentage compared to full-term individuals (73.8%). TBW of preterm reached up to 90% at 26 weeks of gestation, dropping to 75% at 36 weeks of gestation, and dropping 1.44% per week after birth (53). Estimation of total body water by the 2H2O dilution method from healthy individuals (children to adults) showed that prepubescent children have a higher aqueous fraction of their fat-free body mass when compared to young adults (72.7 ± 1.6% vs. 70.8 ± 1.2%; p < 0.01) (54). Lohman et al. described the chemical composition of FFM changes during childhood and they were both ages- and sex-specific (55). Therefore, our data contribute to characterizing the TBW/FFM in VLBW preterm, since there is still a paucity of data in this population during growth ex utero (53).
Another interesting finding of early stimulation in the VLBW preterm population was the relationship between visceral fat area and insulin resistance, showing that CG presented higher insulin resistance with more visceral fat, a relation not observed in the IG group. Prematurity has been considered a risk factor for cardiovascular and metabolic diseases (5, 56), in which preterm-born individuals presented a higher incidence of hypertension (57), glucose intolerance (52, 58), as well as metabolic syndromes (59, 60). The inverse association observed in our study agrees with the observation that young adults born extremely preterm present a higher number of risk factors for cardiometabolic disorders unrelated to each other as observed in the control term group (52). Although our results are not strong enough to infer many interpretations, we believe that the protective response observed in IG could be related to the light increase of FFM components, such as skeletal muscle mass, which is insulin sensitive and regulates glucose metabolism, and contributes in the prevention of cardiometabolic disorders (61). Since early stimulation can positively affect the central nervous system, thus improving neurodevelopment, it can also positively affect the other systems, as is observed in exercising: it can improve whole-body glucose tolerance, lipid handling, and insulin sensitivity in humans (62), as well as in rodents (63).
Our follow-up study presents limitations. Although we have been using in our cohort of children born preterm the research grade InBody 770®, a multi-frequency BIA that presents more reliable results of water compartments (38), we should be careful with the data interpretation. However, many studies have shown that BIA results correlate well with the gold standard method DEXA scan. This implies that BIA demonstrated a strong accuracy and reliability when compared to DEXA (64, 65) which has been recently used in children of preschool age (66–68). Moreover, the use of this equipment is advantageous for clinical use and large-scale epidemiological studies since it is simple, rapid, non-invasive, accessible, reliable, and requires little training. This will allow an appropriate follow-up of the preterm population growth and development from our cohort (69). A study with the purpose to examine the validity of body composition showed that skeletal muscle mass was reliably measured using a multi-frequency BIA method in preschool children (70). Using BIA in preschool-aged children can be difficult due to the child’s movement during measurement; the evaluation was considered complete if the child was able to stay still for 60 s over the scale holding the electrodes. Further studies from our preterm cohort population and additional follow-up evaluations, at both preschool and school ages, will contribute to confirming these preliminary results. Another limitation of this study was the small sample size due to unreachable subjects, as well as the disinterest of the legal guardians (parents) to participate in the study due to the pandemic and socio-economic-related factors.
Conclusion
This study was developed to investigate if an early intervention program could positively affect body composition in VLBW preterm children. The intervention indicates that it can probably increase FFM and modify the relationship between fat and the endocrine system, which may contribute to better health with advancing age in VLBW preterm children. Nonetheless, further longitudinal studies and follow-ups of these preterm children are required to establish the clinical significance and prolonged impact of early intervention in this population.
Data availability statement
The data that support the findings of this study are available on request from the corresponding authors JB, amJlcm5hcmRpQGhjcGEuZWR1LmJy and RF, cm9sZmVybmFuZGVzQGhjcGEuZWR1LmJy. The data are not publicly available due to their containing information that could compromise the privacy of research participants.
Ethics statement
The ethical approval to conduct this study was granted by the Research Ethics Committee of the Hospital de Clínicas de Porto Alegre (number HCPA: 2019-0809; Certificate of Presentation for Ethical Appreciation (CAAE): 27358019.1.0000.5327). The guardians of the participants who met the eligibility criteria were invited to participate in the study and were included after signing the informed consent form.
Author contributions
RF and JB conceptualized and designed the study, performed data analyses, and wrote the manuscript. JF and FG participated in data collection and conducted the study. RP and RS conceptualized and designed the study, reviewed critically the manuscript, and obtained funding. All authors revised and finalized the manuscript.
Funding
This work was supported in part by Bill & Melinda Gates Foundation, Grand Challenges Brazil: All Children Thriving (OPP1142172); National Council for Scientific and Technological Development (CNPq); Departamento de Ciência e Tecnologia (DECIT)/Ministério da Saúde do Brasil; and Fundo de Incentivo à Pesquisa (FIPE) of Hospital de Clínicas de Porto Alegre (HCPA).
Acknowledgments
We thank all the research groups, the multi-professional team from the Ambulatory of Neonatology of HCPA, the assistance of the Biostatistics Team of HCPA, and the contribution in data collection and review of the students Maria Eduarda Pinko Santa Helena, Laurem Oliveira e Silva and Merly Innocent, and the will of all participants. We also thank the Bill & Melinda Gates Foundation and the Fundo de Incentivo à Pesquisa (FIPE/HCPA) for funding; and the National Postdoctoral Program (PNPD) of Coordenação de Aperfeiçoamento de Pessoal de Nível Superior—Brasil (CAPES) for the financial support (scholarship—finance code 001).
Conflict of interest
The authors declare that the research was conducted in the absence of any commercial or financial relationships that could be construed as a potential conflict of interest.
Publisher’s note
All claims expressed in this article are solely those of the authors and do not necessarily represent those of their affiliated organizations, or those of the publisher, the editors and the reviewers. Any product that may be evaluated in this article, or claim that may be made by its manufacturer, is not guaranteed or endorsed by the publisher.
References
1. Blencowe H, Cousens S, Oestergaard MZ, Chou D, Moller AB, Narwal R, et al. National, regional, and worldwide estimates of preterm birth rates in the year 2010 with time trends since 1990 for selected countries: a systematic analysis and implications. Lancet. (2012) 379:2162–72. doi: 10.1016/S0140-6736(12)60820-4
2. Rysavy MA, Horbar JD, Bell EF, Li L, Greenberg LT, Tyson JE, et al. Assessment of an updated neonatal research network extremely preterm birth outcome model in the vermont oxford network. JAMA Pediatr. (2020) 174:e196294. doi: 10.1001/jamapediatrics.2019.6294
3. Voller SMB. Follow-Up Care for High-Risk Preterm Infants. Pediatr Ann. (2018) 47:e142–6. doi: 10.3928/19382359-20180325-03
4. Silveira RC, Mendes EW, Fuentefria RN, Valentini NC, Procianoy RS. Early intervention program for very low birth weight preterm infants and their parents: a study protocol. BMC Pediatr. (2018) 18:268. doi: 10.1186/s12887-018-1240-6
5. Luu TM, Katz SL, Leeson P, Thébaud B, Nuyt AM. Preterm birth: risk factor for early-onset chronic diseases. CMAJ. (2016) 188:736–46. doi: 10.1503/cmaj.150450
6. Möllers LS, Yousuf EI, Hamatschek C, Morrison KM, Hermanussen M, Fusch C, et al. Metabolic-endocrine disruption due to preterm birth impacts growth, body composition, and neonatal outcome. Pediatr Res. (2021) 91:1350–60. doi: 10.1038/s41390-021-01566-8
7. Pfister KM, Zhang L, Miller NC, Ingolfsland EC, Demerath EW, Ramel SE. Early body composition changes are associated with neurodevelopmental and metabolic outcomes at 4 years of age in very preterm infants. Pediatr Res. (2018) 84:713–8. doi: 10.1038/s41390-018-0158-x
8. Skinner AM, Narchi H. Preterm nutrition and neurodevelopmental outcomes. World J Methodol. (2021) 11:278–93. doi: 10.5662/wjm.v11.i6.278
9. Ramel SE, Gray HL, Christiansen E, Boys C, Georgieff MK, Demerath EW. Greater Early gains in fat-free mass, but not fat mass, are associated with improved neurodevelopment at 1 year corrected age for prematurity in very low birth weight preterm infants. J Pediatr. (2016) 173:108–15. doi: 10.1016/j.jpeds.2016.03.003
10. Frondas-Chauty A, Simon L, Flamant C, Hanf M, Darmaun D, Rozé JC. Deficit of fat free mass in very preterm infants at discharge is associated with neurological impairment at age 2 years. J Pediatr. (2018) 196:301–4. doi: 10.1016/j.jpeds.2017.12.017
11. Johnson MJ, Wootton SA, Leaf AA, Jackson AA. Preterm birth and body composition at term equivalent age: a systematic review and meta-analysis. Pediatrics. (2012) 130:e640–9. doi: 10.1542/peds.2011-3379
12. Andrews ET, Beattie RM, Johnson MJ. Measuring body composition in the preterm infant: Evidence base and practicalities. Clin Nutr. (2019) 38:2521–30. doi: 10.1016/j.clnu.2018.12.033
13. Engan M, Vollsæter M, Øymar K, Markestad T, Eide GE, Halvorsen T, et al. Comparison of physical activity and body composition in a cohort of children born extremely preterm or with extremely low birth weight to matched term-born controls: a follow-up study. BMJ Paediatr Open. (2019) 3:e000481. doi: 10.1136/bmjpo-2019-000481
14. Xie LF, Alos N, Cloutier A, Béland C, Dubois J, Nuyt AM, et al. The long-term impact of very preterm birth on adult bone mineral density. Bone Rep. (2019) 10:100189. doi: 10.1016/j.bonr.2018.100189
15. Uthaya S, Thomas EL, Hamilton G, Doré CJ, Bell J, Modi N. Altered adiposity after extremely preterm birth. Pediatr Res. (2005) 57:211–5. doi: 10.1203/01.PDR.0000148284.58934.1C
16. Scheurer JM, Zhang L, Plummer EA, Hultgren SA, Demerath EW, Ramel SE. Body composition changes from infancy to 4 years and associations with early childhood cognition in preterm and full-term children. Neonatology. (2018) 114:169–76. doi: 10.1159/000487915
17. Blake-Lamb TL, Locks LM, Perkins ME, Woo Baidal JA, Cheng ER, Taveras EM. Interventions for childhood obesity in the first 1,000 days a systematic review. Am J Prev Med. (2016) 50:780–9. doi: 10.1016/j.amepre.2015.11.010
18. Cunha AJ, Leite Á, Almeida IS. The pediatrician’s role in the first thousand days of the child: the pursuit of healthy nutrition and development. J Pediatr (Rio J). (2015) 91(6 Suppl 1):S44–51. doi: 10.1016/j.jped.2015.07.002
19. Pascoali Rodovanski G, Bêz Reus BA, Cechinel Damiani AV, Franco Mattos K, Moreira RS, Neves Dos Santos A. Home-based early stimulation program targeting visual and motor functions for preterm infants with delayed tracking: Feasibility of a Randomized Clinical Trial. Res Dev Disabil. (2021) 116:104037. doi: 10.1016/j.ridd.2021.104037
20. Prado EL, Larson LM, Cox K, Bettencourt K, Kubes JN, Shankar AH. Do effects of early life interventions on linear growth correspond to effects on neurobehavioural development? A systematic review and meta-analysis. Lancet Glob Health. (2019) 7:e1398–413. doi: 10.1016/S2214-109X(19)30361-4
21. Ruys CA, van de Lagemaat M, Rotteveel J, Finken MJJ, Lafeber HN. Improving long-term health outcomes of preterm infants: how to implement the findings of nutritional intervention studies into daily clinical practice. Eur J Pediatr. (2021) 180:1665–73. doi: 10.1007/s00431-021-03950-2
22. Procianoy RS, Mendes EW, Silveira RC. Massage therapy improves neurodevelopment outcome at two years corrected age for very low birth weight infants. Early Hum Dev. (2010) 86:7–11. doi: 10.1016/j.earlhumdev.2009.12.001
23. Cameron EC, Maehle V, Reid J. The effects of an early physical therapy intervention for very preterm, very low birth weight infants: a randomized controlled clinical trial. Pediatr Phys Ther. (2005) 17:107–19. doi: 10.1097/01.PEP.0000163073.50852.58
24. de Vries AG, Huiting HG, van den Heuvel ER, L’Abée C, Corpeleijn E, Stolk RP. An activity stimulation programme during a child’s first year reduces some indicators of adiposity at the age of two-and-a-half. Acta Paediatr. (2015) 104:414–21. doi: 10.1111/apa.12880
25. Who Multicentre Growth Reference Study Group. WHO Child Growth Standards based on length/height, weight and age. Acta Paediatr Suppl. (2006) 450:76–85. doi: 10.1111/j.1651-2227.2006.tb02378.x
26. Fenton TR, Kim JH. A systematic review and meta-analysis to revise the Fenton growth chart for preterm infants. BMC Pediatr. (2013) 13:59. doi: 10.1186/1471-2431-13-59
27. WHO. The optimal duration of exclusive breastfeeding: results of a WHO systematic review. Indian Pediatr. (2001) 38:565–7.
28. de Ferranti SD, Daniels SR, Gillman M, Vernacchio L, Plutzky J, Baker AL. NHLBI integrated guidelines on cardiovascular disease risk reduction: can we clarify the controversy about cholesterol screening and treatment in childhood? Clin Chem. (2012) 58:1626–30. doi: 10.1373/clinchem.2012.182089
29. Taylor RW, Gold E, Manning P, Goulding A. Gender differences in body fat content are present well before puberty. Int J Obes Relat Metab Disord. (1997) 21:1082–4. doi: 10.1038/sj.ijo.0800522
30. Domellöf M, Lönnerdal B, Dewey KG, Cohen RJ, Rivera LL, Hernell O. Sex differences in iron status during infancy. Pediatrics. (2002) 110:545–52. doi: 10.1542/peds.110.3.545
31. Kirchengast S. Gender differences in body composition from childhood to old age: an evolutionary point of view. J Life Sci. (2010) 2:1–10. doi: 10.1080/09751270.2010.11885146
32. Barker DJ. The origins of the developmental origins theory. J Intern Med. (2007) 261:412–7. doi: 10.1111/j.1365-2796.2007.01809.x
33. Benzies KM, Magill-Evans JE, Hayden KA, Ballantyne M. Key components of early intervention programs for preterm infants and their parents: a systematic review and meta-analysis. BMC Pregnancy Childbirth. (2013) 13(Suppl 1):S10. doi: 10.1186/1471-2393-13-S1-S10
34. Melnyk BM, Alpert-Gillis L, Feinstein NF, Fairbanks E, Schultz-Czarniak J, Hust D, et al. Improving cognitive development of low-birth-weight premature infants with the COPE program: a pilot study of the benefit of early NICU intervention with mothers. Res Nurs Health. (2001) 24:373–89. doi: 10.1002/nur.1038
35. Field T. Preterm infant massage therapy studies: an American approach. Semin Neonatol. (2002) 7:487–94. doi: 10.1053/siny.2002.0153
36. Litmanovitz I, Dolfin T, Friedland O, Arnon S, Regev R, Shainkin-Kestenbaum R, et al. Early physical activity intervention prevents decrease of bone strength in very low birth weight infants. Pediatrics. (2003) 112(1Pt 1):15–9. doi: 10.1542/peds.112.1.15
37. Morgan C, Novak I, Badawi N. Enriched environments and motor outcomes in cerebral palsy: systematic review and meta-analysis. Pediatrics. (2013) 132:e735–46. doi: 10.1542/peds.2012-3985
38. Moonen HPFX, Van Zanten ARH. Bioelectric impedance analysis for body composition measurement and other potential clinical applications in critical illness. Curr Opin Crit Care. (2021) 27:344–53. doi: 10.1097/MCC.0000000000000840
39. McGee M, Unger S, Hamilton J, Birken CS, Pausova Z, Vanderloo LM, et al. Lean mass accretion in children born very low birth weight is significantly associated with estimated changes from sedentary time to light physical activity. Pediatr Obes. (2020) 15:e12610. doi: 10.1111/ijpo.12610
40. Vainshtein A, Sandri M. Signaling pathways that control muscle mass. Int J Mol Sci. (2020) 21:4759. doi: 10.3390/ijms21134759
41. You JS, McNally RM, Jacobs BL, Privett RE, Gundermann DM, Lin KH, et al. The role of raptor in the mechanical load-induced regulation of mTOR signaling, protein synthesis, and skeletal muscle hypertrophy. FASEB J. (2019) 33:4021–34. doi: 10.1096/fj.201801653RR
42. Corpeno Kalamgi R, Salah H, Gastaldello S, Martinez-Redondo V, Ruas JL, Fury W, et al. Mechano-signalling pathways in an experimental intensive critical illness myopathy model. J Physiol. (2016) 594:4371–88. doi: 10.1113/JP271973
43. Vignochi CM, Miura E, Canani LH. Effects of motor physical therapy on bone mineralization in premature infants: a randomized controlled study. J Perinatol. (2008) 28:624–31. doi: 10.1038/jp.2008.60
44. Bortolotto CC, Santos IS, Dos Santos Vaz J, Matijasevich A, Barros AJD, Barros FC, et al. Prematurity and body composition at 6, 18, and 30 years of age: Pelotas (Brazil) 2004, 1993, and 1982 birth cohorts. BMC Public Health. (2021) 21:321. doi: 10.1186/s12889-021-10368-w
45. Liao L, Deng Y, Zhao D. Association of low birth weight and premature birth with the risk of metabolic syndrome: a meta-analysis. Front Pediatr. (2020) 8:405. doi: 10.3389/fped.2020.00405
46. Sharp M. Bone disease of prematurity. Early Hum Dev. (2007) 83:653–8. doi: 10.1016/j.earlhumdev.2007.07.009
47. Faienza MF, D’Amato E, Natale MP, Grano M, Chiarito M, Brunetti G, et al. Metabolic bone disease of prematurity: diagnosis and management. Front Pediatr. (2019) 7:143. doi: 10.3389/fped.2019.00143
48. Stalnaker KA, Poskey GA. Osteopenia of prematurity: does physical activity improve bone mineralization in preterm infants? Neonatal Netw. (2016) 35:95–104. doi: 10.1891/0730-0832.35.2.95
49. Aggett PJ, Iron. In: Erdman JW, Macdonald IA, Zeisel SH editors. Present Knowledge in Nutrition 10th ed. Hoboken, NJ: Wiley-Blackwell (2012).
50. Raffaeli G, Manzoni F, Cortesi V, Cavallaro G, Mosca F, Ghirardello S. Iron homeostasis disruption and oxidative stress in preterm newborns. Nutrients. (2020) 12:1554. doi: 10.3390/nu12061554
51. Belaya I, Kucháriková N, Górová V, Kysenius K, Hare DJ, Crouch PJ, et al. Regular physical exercise modulates iron homeostasis in the 5xFAD mouse model of Alzheimer’s Disease. Int J Mol Sci. (2021) 22:8715. doi: 10.3390/ijms22168715
52. Flahault A, Paquette K, Fernandes RO, Delfrate J, Cloutier A, Henderson M, et al. Increased incidence but lack of association between cardiovascular risk factors in adults born preterm. Hypertension. (2020) 75:796–805. doi: 10.1161/HYPERTENSIONAHA.119.14335
53. Young A, Brown LK, Ennis S, Beattie RM, Johnson MJ. Total body water in full-term and preterm newborns: systematic review and meta-analysis. Arch Dis Child Fetal Neonatal Ed. (2021) 106:542–8. doi: 10.1136/archdischild-2020-321112
54. Hewitt MJ, Going SB, Williams DP, Lohman TG. Hydration of the fat-free body mass in children and adults: implications for body composition assessment. Am J Physiol. (1993) 265(1Pt 1):E88–95. doi: 10.1152/ajpendo.1993.265.1.E88
55. Lohman TG, Hingle M, Going SB. Body composition in children. Pediatr Exerc Sci. (2013) 25:573–90. doi: 10.1123/pes.25.4.573
56. Paquette K, Fernandes RO, Xie LF, Cloutier A, Fallaha C, Girard-Bock C, et al. Kidney size, renal function, ang (angiotensin) peptides, and blood pressure in young adults born preterm. Hypertension. (2018) 72:918–28. doi: 10.1161/HYPERTENSIONAHA.118.11397
57. Bertagnolli M, Luu TM, Lewandowski AJ, Leeson P, Nuyt AM. Preterm birth and hypertension: is there a link? Curr Hypertens Rep. (2016) 18:28. doi: 10.1007/s11906-016-0637-6
58. Hofman PL, Regan F, Jackson WE, Jefferies C, Knight DB, Robinson EM, et al. Premature birth and later insulin resistance. N Engl J Med. (2004) 351:2179–86. doi: 10.1056/NEJMoa042275
59. Sipola-Leppänen M, Vääräsmäki M, Tikanmäki M, Matinolli HM, Miettola S, Hovi P, et al. Cardiometabolic risk factors in young adults who were born preterm. Am J Epidemiol. (2015) 181:861–73. doi: 10.1093/aje/kwu443
60. Heidemann LA, Procianoy RS, Silveira RC. Prevalence of metabolic syndrome-like in the follow-up of very low birth weight preterm infants and associated factors. J Pediatr (Rio J). (2019) 95:291–7. doi: 10.1016/j.jped.2018.02.009
61. Merz KE, Thurmond DC. Role of skeletal muscle in insulin resistance and glucose uptake. Compr Physiol. (2020) 10:785–809. doi: 10.1002/cphy.c190029
62. Conn VS, Koopman RJ, Ruppar TM, Phillips LJ, Mehr DR, Hafdahl AR. Insulin sensitivity following exercise interventions: systematic review and meta-analysis of outcomes among healthy adults. J Prim Care Commun Health. (2014) 5:211–22. doi: 10.1177/2150131913520328
63. Musial B, Fernandez-Twinn DS, Duque-Guimaraes D, Carr SK, Fowden AL, Ozanne SE, et al. Exercise alters the molecular pathways of insulin signaling and lipid handling in maternal tissues of obese pregnant mice. Physiol Rep. (2019) 7:e14202. doi: 10.14814/phy2.14202
64. Lim JS, Hwang JS, Lee JA, Kim DH, Park KD, Jeong JS, et al. Cross-calibration of multi-frequency bioelectrical impedance analysis with eight-point tactile electrodes and dual-energy X-ray absorptiometry for assessment of body composition in healthy children aged 6-18 years. Pediatr Int. (2009) 51:263–8. doi: 10.1111/j.1442-200X.2008.02698.x
65. Seo YG, Kim JH, Kim Y, Lim H, Ju YS, Kang MJ, et al. Validation of body composition using bioelectrical impedance analysis in children according to the degree of obesity. Scand J Med Sci Sports. (2018) 28:2207–15. doi: 10.1111/sms.13248
66. Bell LA, Vuillermin P, Timperio A, Ponsonby AL, Tang MLK, Hesketh KD, et al. Physical activity and adiposity in preschool children: The Barwon Infant Study. Pediatr Obes. (2022) 17:e12853. doi: 10.1111/ijpo.12853
67. Keating SE, Mielke GI, King-Dowling S, Timmons BW, Kwan M, Cairney J. Associations between fitness, physical activity, and fatness in preschool children with typical and atypical motor coordination. Front Pediatr. (2022) 10:756862. doi: 10.3389/fped.2022.756862
68. Berglund NR, Lewis JI, Michaelsen KF, Mølgaard C, Renault KM, Carlsen EM. Birthweight z-score and fat-free mass at birth predict body composition at 3 years in Danish children born from obese mothers. Acta Paediatr. (2022) 111:1427–34. doi: 10.1111/apa.16346
69. Rush EC, Bristow S, Plank LD, Rowan J. Bioimpedance prediction of fat-free mass from dual-energy X-ray absorptiometry in a multi-ethnic group of 2-year-old children. Eur J Clin Nutr. (2013) 67:214–7. doi: 10.1038/ejcn.2012.182
Keywords: premature birth, very low birth weight (VLBW), early intervention, body composition, blood chemical analysis, preschool child
Citation: Fernandes RO, Bernardi JR, da Fonseca JD, Gomes da Silva F, Procianoy RS and Silveira RC (2022) The impact of an early intervention home-based program on body composition in preterm-born preschoolers with very low birth weight. Front. Nutr. 9:981818. doi: 10.3389/fnut.2022.981818
Received: 29 June 2022; Accepted: 21 September 2022;
Published: 20 October 2022.
Edited by:
Roberto Fernandes Da Costa, Federal University of Rio Grande do Norte, BrazilReviewed by:
Daniel Hoffman, Rutgers, The State University of New Jersey, United StatesJose Geraldo Mill, Universidade Federal do Espírito Santo, Brazil
Leigh C. Ward, The University of Queensland, Australia
Copyright © 2022 Fernandes, Bernardi, da Fonseca, Gomes da Silva, Procianoy and Silveira. This is an open-access article distributed under the terms of the Creative Commons Attribution License (CC BY). The use, distribution or reproduction in other forums is permitted, provided the original author(s) and the copyright owner(s) are credited and that the original publication in this journal is cited, in accordance with accepted academic practice. No use, distribution or reproduction is permitted which does not comply with these terms.
*Correspondence: Rafael Oliveira Fernandes, cm9sZmVybmFuZGVzQGhjcGEuZWR1LmJy; Juliana Rombaldi Bernardi, amJlcm5hcmRpQGhjcGEuZWR1LmJy
†These authors have contributed equally to this work and share first authorship