- 1Department of Maternal and Child Health, School of Public Health, Sun Yat-sen University, Guangzhou, China
- 2Institute of Nutrition & Health, Qingdao University, Qingdao, China
- 3Yili Maternal and Infant Nutrition Institute, Inner Mongolia Yili Industrial Group Co., Ltd., Hohhot, China
- 4Inner Mongolia Dairy Technology Research Institute Co., Ltd., Hohhot, China
- 5Nutrition and Health Research Center, National Center of Technology Innovation for Dairy, Hohhot, China
- 6Department of Occupational and Environmental Health, School of Public Health, Sun Yat-sen University, Guangzhou, China
- 7Guangdong Provincial Key Laboratory of Food, Nutrition and Health, School of Public Health, Sun Yat-sen University, Guangzhou, China
Background: The findings of the association between maternal polyunsaturated fatty acid (PUFA) levels during pregnancy and offspring weight status are controversial. Furthermore, few studies have focused on Asian populations or used erythrocyte membranes as biological markers. We aimed to examine the associations between maternal erythrocyte PUFA and offspring weight status within the first 2 years among the Chinese population.
Materials and methods: A total of 607 mother-child pairs were recruited from a birth cohort. Maternal erythrocyte n-3 and n-6 PUFA during pregnancy were measured by gas chromatography, and the ratio of PUFA was calculated. Weight- and body mass index (BMI)-for-age z (WAZ and BAZ) scores were calculated for offspring at 1, 3, 6, 8, 12, 18, and 24 months of age. The risk of overweight and obesity was defined by the WHO criterion. The Generalized Estimating Equation (GEE) model was carried out for repeated anthropometric data within 2 years of age.
Results: Maternal erythrocyte docosapentaenoic acid (DPA, n-3) was inversely associated with offspring BAZ score [tertile 2 vs. tertile 1, β: −0.18 (−0.29, −0.00)]. Higher maternal erythrocyte arachidonic acid (AA) was inversely associated with lower offspring WAZ and BAZ [tertile 3 vs. tertile 1, β: −0.18 (−0.35, −0.02), −0.22 (−0.38, −0.06), respectively]. Furthermore, higher maternal erythrocyte AA [tertile 3 vs. tertile 1, odds ratio [OR]: 0.52 (0.36, 0.75), ptrend < 0.001] and total n-6 PUFA [tertile 3 vs. tertile 1, OR: 0.56 (0.39, 0.81), ptrend = 0.002] were associated with decreased risk of overweight and obesity in offspring. Maternal erythrocyte n-6/n-3 PUFA and AA/eicosapentaenoic acid (EPA) ratios were not associated with offspring weight status.
Conclusion: Maternal erythrocyte PUFA might influence offspring weight status within 2 years of age in the Chinese population. Further Asian studies are still needed.
Introduction
The increasing prevalence of childhood overweight and obesity remains a serious health concern (1). Public prevention of childhood obesity is urgently needed, and the first 1,000 days of life are a unique window (2). The intrauterine nutritional environment might affect fetal growth and the risk of disease later in life by affecting developmental programming (3), among which polyunsaturated fatty acids (PUFA) are critical nutrients for fetal growth and development (4). It was suggested that the maternal PUFA levels during pregnancy have a long-term effect on offspring weight status.
Several in vitro and animal studies have suggested that PUFA could affect fetal adipose tissue accretion. However, the effect may vary by the type and ratio of PUFA (5, 6), especially the composition and ratio of n-3 and n-6 PUFAs. It was suggested that the maternal n-6/n-3 PUFA ratio during pregnancy was more closely associated with fetal metabolic programming (7). Prospective cohort studies also showed conflicting results. The studies from America showed that higher maternal n-3 PUFA was associated with lower total subcutaneous fat mass and lower odds of obesity in children aged 3 years (8). Similarly, another Netherlands study found that lower maternal levels of n-3 PUFA and higher levels of n-6 PUFA were associated with higher total body fat and abdominal fat levels in childhood (9). However, another American study showed that maternal PUFA was not associated with childhood body mass index (BMI) z scores aged 8 years (10). Similarly, results from randomized controlled trials (RCTs) showed inconclusive effects of n-3 PUFA supplement during pregnancy on offspring BMI or body fat percentage (11), because of differences in design, especially the amounts, balance, and type of PUFA given.
Notably, previous studies are almost exclusively from American and European populations, but evidence from Asian populations has been limited. As the Asian population has different genetic variations and dietary patterns when compared with American or European populations (12), more investigation into Asian populations is highly warranted. Moreover, the majority of these studies used plasma as biomarkers or non-objective food frequency questionnaires as exposure measures. Fatty acid composition in erythrocytes could reflect dietary fatty acid intake over the past month or 2 (13), which may be a more stable biomarker than plasma phospholipid fraction. Therefore, we explored the associations between maternal erythrocyte PUFA during pregnancy with offspring weight status within the first 2 years in a Chinese birth cohort.
Materials and methods
Study design and participants
Mothers and infants were from a prospective birth cohort study (registration number: NCT03023293), which was carried out at a hospital in Guangzhou, China. We enrolled pregnant women who were aged 20–45 years and at 20–28 weeks of gestation during 2017 and 2018 and then followed up their offspring for 2 years postpartum. Mothers with pre-existing diabetes mellitus, cardiovascular disease, thyroid disease, hematopathy, polycystic ovary syndrome, pregnancy infection, mental disorder, or multiple pregnancies and infants who did not have follow-up data were excluded from the study.
A total of 691 mother-offspring pairs were enrolled. We further excluded women whose erythrocyte PUFA information was missing (n = 84). Overall, 607 mother-child pairs were finally included in our analysis. This study was approved by the institutional review boards of Sun Yat-sen University. Informed consent forms were obtained from all participants.
Maternal erythrocyte fatty acid analysis
Maternal blood samples were collected during 20–28 weeks of gestation. The venous blood samples were collected by professional nurses in the morning after an overnight fast of at least 10 h. The samples were centrifuged at 841 × g for 15 min to obtain agglutinated blood cells. Blood cell samples were kept at −80°C until later laboratory analysis.
Laboratory analysis of erythrocyte fatty acid was as follows. First, removed the blood samples from the refrigerator and thawed them. In total, 100 μl of blood cells per sample was dropped on the test paper (Omegabandz Inc., China) and shipped to the laboratory after drying. Second, the tris–HCl buffer was added to the sample. After the red blood cells were hemolyzed, we centrifuged them to obtain the bottom layer of milky red blood cell fragments. And then, the lipid component of erythrocyte fragments was extracted with a chloroformmethanol (2:1, v/v) solvent system containing 10 mg/L of butylated-hydroxytoluene (BHT, Sigma Chemical Co., St. Louis, MO, USA) (14). Third, the methyl esters of the fatty acids from the lipid extract were transesterified with H2SO4 in methanol (5%, v/v), together with toluene, in sealed tubes at 70°C for 2 h. The methanol layer was transferred to a new test tube, blown by nitrogen, and then dissolved in hexane. Fourth, the derived fatty acid methyl esters were analyzed by using Agilent 7820 Gas Chromatograph (Agilent Corporation, USA) equipped with a 60 m × 0.25 mm × 0.25 μm fused silica-bonded phase column (DB-23, Agilent Corporation, USA) and a flame ionization detector. The column temperature was firstly programmed from 150 to 180°C at a rate of 10°C/min, with an initial hold time of 2 min; then it was increased to 215 at 2.5°C/min and held for 6 min; and finally, it was increased to 230 at 10°C/min and held for another 5 min. Fatty acids were identified by comparison of retention time with standard mixtures of fatty acid methyl ester (Nu-Chek Prep, Inc., Waterville, MN, USA). Quantification of the fatty acid compositions was achieved by the comparison of peak areas with the internal standard (tricosanoic acid, Nu-Chek Prep, Inc., Waterville, MN, USA), which was added to the samples (1 mg of internal standard in 500 mg sample) prior to extraction.
Polyunsaturated fatty acid levels were expressed as a proportion of the total fatty acids. Based on findings from previous studies, selected PUFAs were total n-3 PUFAs, which included α-linolenic acid (ALA, C18:3 n-3), eicosapentaenoic acid (EPA, C20:5 n-3), docosapentaenoic acid (DPA, C22:5 n-3), and docosahexaenoic acid (DHA, C22:6 n-3), and total n-6 PUFA, which included linoleic acid (LA, C18:2 n-6), γ-linolenic acid (GLA, C18:3 n-6), dihomo-gamma-linolenic acid (DGLA, C20:3 n-6), and arachidonic acid (AA, C20:4 n-6). We also calculated the ratio of total n-6/n-3 PUFA and the ratio of AA/EPA.
Offspring anthropometric measurements
At the age of 1, 3, 6, 8, 12, 18, and 24 months, offspring length and weight without shoes and heavy clothing were measured by the trained nurses. The length was measured to the nearest 0.1 cm by a stadiometer, and weight was measured to the nearest 0.01 kg using an electronic scale. All measurements were performed by trained professionals, and standardized tools were used. BMI was calculated as weight (kg)/height in meters square.
According to the criterion from the World Health Organization Child Growth Standards 2006 (15), offspring weight-for-age z (WAZ) score, length-for-age z (LAZ) score, and BMI-for-age z (BAZ) score were calculated. Furthermore, offspring’s weight status was defined as a normal, possible risk of overweight, or overweight and obesity based on the BAZ score.
Covariates
Information on maternal age, pre-pregnancy weight and height, educational level (high school or below/junior college/college or above), monthly household income (<4,000/4,000–6,000/6,000–10,000/≥10,000 RMB), and passive smoking during pregnancy were obtained by a face-to-face questionnaire survey in the baseline investigation. Maternal pre-pregnancy BMI was calculated from weight (kg) divided by height squared (m2). In addition, all pregnant women were scheduled for a 75-g oral glucose tolerance test between 20 and 28 weeks of gestation. Women were diagnosed with gestational diabetes (GDM) when meeting the criteria of the International Association of Diabetes and Pregnancy Study Groups (16). Offspring sex, birth weight, and other delivery information were obtained from the hospital birth records. Offspring feeding status at 6 months (breastfeeding/formula feeding/mixed feeding) was obtained at the age of 6 months by a structured questionnaire.
Statistical analysis
All statistical analyses were carried out using SAS statistical software package (version 9.4; SAS Institute Inc., Cary, NC, USA). PUFA levels were divided into three levels based on the tertiles of each PUFA. Continuous variables were reported as the mean ± standard deviation (SD) or median (25th–75th percentile), and categorical variables were expressed as percentages.
Considering the within-subject correlation due to repeated measures, the Generalized Estimating Equation (GEE) (17) was selected to correct for children’s repeated anthropometric measurements at 1, 3, 6, 8, 12, 18, and 24 months of age because the method takes this within-subject correlation into account. After comparing the quasi-likelihood under the independence model criterion (QIC) value, the GEE with six dependent correlation matrices was used to estimate the relationship between the maternal PUFA and offspring WAS score, LAZ score, BAZ score (continuous), and risk of overweight and obesity (ordered categorical) within the first 2 years. The model was adjusted for confounding factors from mothers and infants, which included maternal age, educational level, family income, GDM, pre-pregnancy BMI, passive smoking during pregnancy, infant age, sex, and breastfeeding. p < 0.05 was considered significant.
Results
Subject characteristics
Table 1 and Supplementary Table 1 show the characteristics of pregnant women and their offsprings. A total of 607 mother-offspring pairs were included in our analyses. The median age of the women was 30.51 years and the median pre-pregnancy BMI was 20.0 kg/m2.
Table 2 shows the maternal erythrocyte PUFA levels in mid-pregnancy. The median maternal erythrocyte total n-3 and n-6 PUFA levels were 9.69 and 36.33%, respectively. Among the individual PUFAs, the content of AA was the highest (17.48%), followed by LA, DHA, DGLA, and DPA (15.32, 7.29, 2.43, and 1.25%, respectively). In addition, the ratios of total n-6/n-3 PUFA and AA/EPA were 3.61, and 18.41, respectively.
Maternal erythrocyte polyunsaturated fatty acid ratios and offspring weight status
Table 3 shows that there was an inverse association between medium-level maternal erythrocyte DPA and offspring BAZ scores [tertile 2 vs. tertile 1, β (95% CI): −0.18 (−0.29, −0.00)]. Higher maternal AA levels were associated with lower offspring WAZ score in the adjusted models [tertile 3 vs. tertile 1, β (95% CI): −0.18 (−0.35, −0.02), ptrend = 0.028]. Moreover, higher maternal erythrocyte AA was associated with lower offspring BAZ score [tertile 3 vs. tertile 1, β (95% CI): −0.22 (−0.38, −0.06), ptrend = 0.006]. We did not find associations between other n-3 PUFA or n-6 PUFA with offspring’s WAS score or BAZ score. In addition, Supplementary Tables 2, 3 show the associations of maternal erythrocyte PUFA with the risk of low birth weight and LAZ score in offspring, respectively.
Table 4 shows that higher maternal erythrocyte total n-6 PUFA and AA were associated with decreased risk of overweight and obesity in offspring (tertile 3 vs. tertile 1, OR (95% CI): 0.56 (0.39, 0.81), ptrend = 0.002; tertile 3 vs. tertile 1, OR (95% CI): 0.52 (0.36, 0.75), ptrend < 0.001, respectively). Non-significant associations were observed between other maternal PUFAs with offspring’s risk of overweight and obesity.
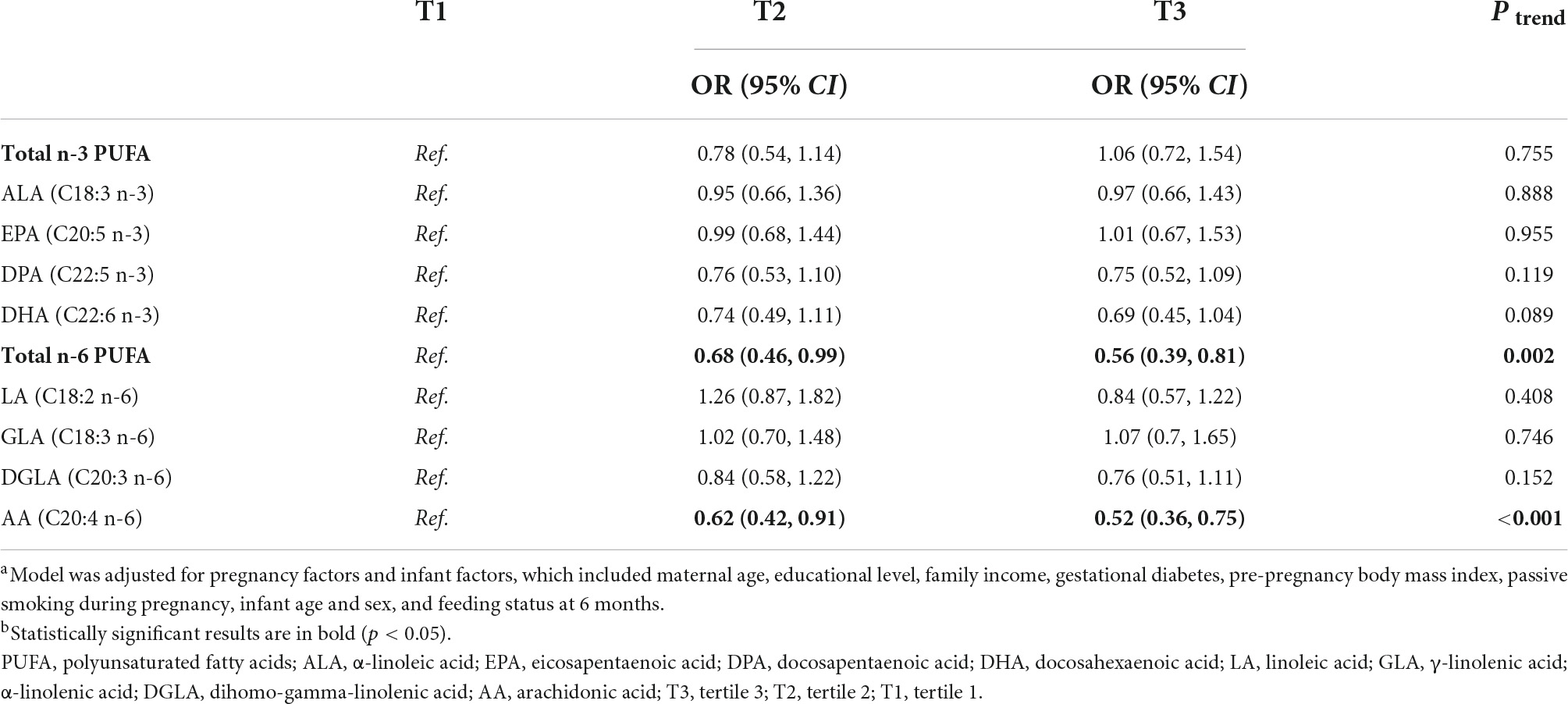
Table 4. Association of maternal erythrocyte PUFA during pregnancy with the risk of overweight and obesity in offspringa,b.
Maternal erythrocyte polyunsaturated fatty acid ratios and offspring weight status
Figure 1 shows that maternal total n-6/n-3 ratios and AA/EPA ratios were not associated with offspring WAZ score, BAZ score, or the risk of overweight and obesity in the adjusted GEE model.
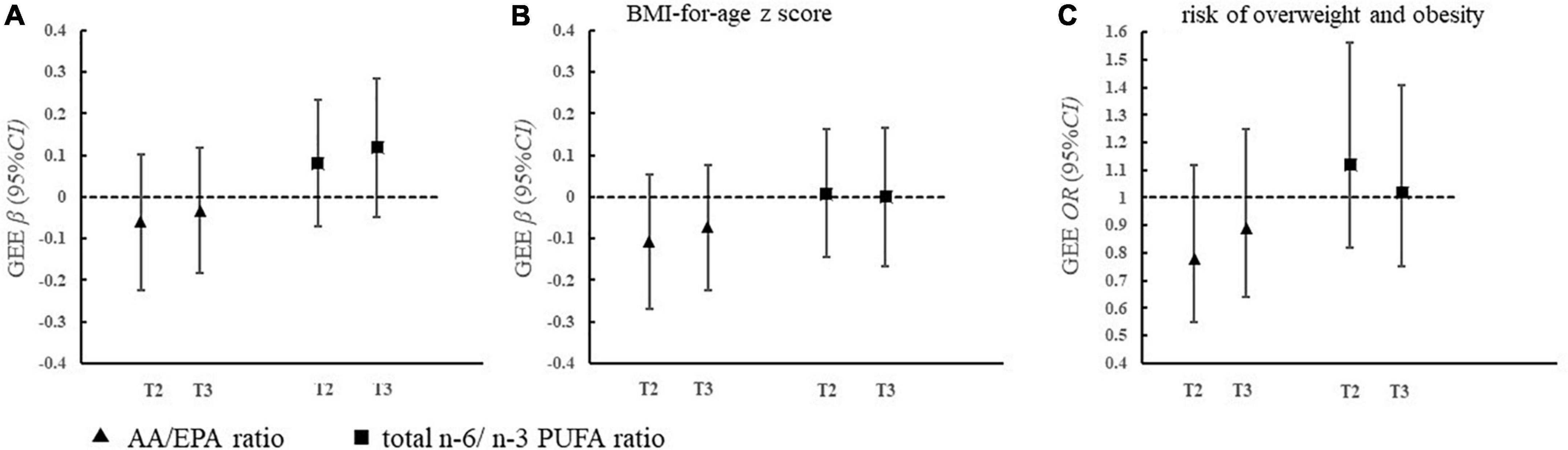
Figure 1. Association of maternal erythrocyte PUFA ratio during pregnancy with offspring weight status (A–C). (A) AA/EPA ratio
total n-6/n-3 PUFA ratio. (B) Model was adjusted for pregnancy factors and infant factors, which included maternal age, educational level, family income, gestational diabetes, pre-pregnancy body mass index, passive smoking during pregnancy, infant age and sex, and feeding status at 6 months. PUFA, polyunsaturated fatty acids; EPA, eicosapentaenoic acid; AA, arachidonic acid; T3, tertile 3; T2, tertile 2; T1, tertile 1.
Discussion
To the best of our knowledge, this is the first prospective study to explore the association between maternal erythrocyte PUFA during pregnancy with offspring weight status in the Chinese population. We found that higher maternal erythrocyte DPA during pregnancy was associated with lower offspring BAZ score within 2 years old. Higher maternal erythrocyte AA was associated with lower offspring WAZ and BAZ scores. Similarly, maternal erythrocyte AA and total n-6 PUFAs were associated with decreased risk of overweight and obesity.
An appropriate supply of n-3 PUFA during pregnancy has an effect on optimal fetal development (4), but whether the effect can persist into childhood is not clear. DPA is one of the n-3 PUFAs, which can play an independent role. We found a negative association between maternal DPA and offspring BAZ score. Consistent with our results, Vdakovic et al. also observed that higher maternal plasma DPA levels were associated with lower childhood total body fat percentage in the Netherlands (9). The underlying possible mechanisms were as followed. Firstly, it is known that overweight and obesity are characterized by chronic low-grade inflammation, while DPA is the precursor of a large panel of lipid mediators (protectins and resolvins) principally implicated in the pro-resolution of the inflammation, with specific effects (18). Secondly, it has been confirmed that DPA inhibits the process of adipocyte differentiation through the inhibition of the activity of the cyclooxygenase enzymes (19), leading the decreased fat accumulation and expression of inflammatory markers (20). Thus, it is possible that maternal DPA may have a long-lasting effect on offspring growth and development through multiple potential mechanism pathways. It is necessary to further advance the critical window of opportunity for the prevention of lifelong obesity (21).
It is worth mention that we found no association of maternal DHA or EPA with offspring weight or BAZ score. In line with our results, Moon et al. found a non-significant relationship between maternal plasma DHA or EPA and offspring growth (22). Moreover, a recent meta-analysis suggested a null correlation between maternal DHA and EPA supplementation in pregnancy and offspring BMI z score at 0–4 years of age (23). However, in contrast with our results, Donahue et al. found that an enhanced maternal DHA and EPA status was associated with lower childhood adiposity in the American population (8). These discrepancies might be partly explained by differences in the n-3 PUFA levels in various populations. DHA and EPA levels of our population were higher than that of American women in whom differences were found (24) but close to the reference interval for healthy Norwegian pregnant women (25). This indicated that the variation of DHA or EPA in our population might not be large enough, which makes it more difficult to find significant associations. Furthermore, the possible interactions of environmental pollutants with DHA and EPA have complex effects on offspring growth and development (26). Therefore, a larger sample size and diverse research designs may be needed for the Chinese population to further confirm the association of maternal DHA and EPA with offspring WAZ or BAZ scores.
Interestingly, we observed that maternal erythrocyte AA, an n-6 PUFA, was related to lower offspring WAZ score, BAZ score, and decreased risk of overweight and obesity. Consistent with our results (9), Much et al. found that higher maternal AA during pregnancy was associated with lower BMI in offspring at 1 year of age in the German population (27). Similarly, Al-Hinai et al. suggested that Mexican maternal intake of AA during mid-pregnancy was inversely associated with offspring linear growth (25). Nevertheless, the hypothesis that higher maternal AA during pregnancy promotes offspring adiposity was confirmed in some European and American mother-offspring pairs (9, 22). The main reason for the inconsistent results might be that AA played different roles at different levels. Although excess AA can serve as a substrate for the production of many pro-inflammatory mediators, (28) optimal AA during pregnancy is beneficial for fetal brain and immune system development (29). Moreover, AA-derived metabolites also have roles in the resolution of inflammation (30). Compared with the American and European populations, the absolute intake of AA in the Chinese population was lower than the Chinese Recommended Nutrient Intakes (RNIS) and North American recommendations for fat intake. (31). Therefore, it might be possible that appropriate AA during pregnancy promotes offspring growth rather than fat accumulation.
Previous studies have shown that an increase in the n-6/n-3 ratio has accelerated the risk for obesity (32). Over the past few decades, the intake of n-6 PUFA is increased while n-3 PUFA is decreased in the modern Western diet, which has pushed the n-6/n-3 ratios from 1:1 to 15:1 (33). Donahue et al. have found that a higher ratio of cord plasma n-6/n-3 PUFA was associated with a higher risk of obesity in American children (8). However, we did not find the association of maternal n-6/n-3 PUFA ratio or AA/EPA ratio with offspring weight status, consistent with the results of the study conducted in Germany (34). A potential explanation for the discrepant findings was the various levels of n-6/n-3 PUFA ratio in the participants. The ratio of maternal erythrocyte n-6/n-3 PUFAs in our population was 3.61, within the reference interval for pregnant women (25). Studies also suggested that a ratio of n-6/n-3 PUFA lower than 5 could reduce the risk of adverse inflammation (35). The n-6/n-3 PUFA ratio in our study was at an appropriate level and might not cause pathological inflammation in the fetus, which helps us to understand the null association between maternal n-6/n-3 PUFA and offspring weight gain or obesity. Based on the above evidence, maternal n-6 PUFAs, especially AA, during pregnancy may benefit offspring growth and development within 2 years of age in the Chinese population when the ratio of n-6/n-3 PUFAs falls within the appropriate range. However, further studies are still needed to explore whether the association of maternal PUFA ratio with offspring weight status varies by different n-6/n-3 PUFA levels in other populations.
Our study has several limitations. First, although the observational study could not establish an exact causal relationship, our study was a prospective cohort study and we have performed an extensive adjustment for the potential maternal and childhood confounders. Second, due to the difficulty in measuring body composition in young children, we have only measured offspring anthropometric index as the outcome but did not provide information on body composition, which can reflect the fat distribution (36). Nevertheless, the BAZ score of children can still predict obesity in childhood and even in adulthood accurately. Third, the sample size of our study was moderate when compared to previous literature. It was possible that the statistical power for individual fatty acids might be insufficient. Therefore, more future studies are still needed. Finally, the levels of PUFA in pregnant women might change with the prolongation of pregnancy. Our study only measured the maternal PUFA in the second trimester, lacking information about the third trimester. However, the second trimester is a critical period for fetal adipocyte development (37), and the level of PUFA in the third trimester was shown to be similar to those in the second trimester (38). Therefore, it is theoretically appropriate to select the second trimester as the exposure window for our study.
Conclusion
The maternal erythrocyte DPA, AA, and total n-6 PUFA might influence offspring weight status within 2 years old in the Chinese population. No significant associations were found between maternal n-6/n-3 PUFA or AA/EPA ratio and offspring weight status. Further Asian studies are still needed to assess the effects of maternal PUFA on offspring weight status throughout childhood.
Data availability statement
The datasets presented in this article are not readily available because they are from an ongoing cohort. Requests to access the datasets should be directed to LC, Y2FpbGk1QG1haWwuc3lzdS5lZHUuY24=.
Ethics statement
The studies involving human participants were reviewed and approved by the Ethics Committee of the School of Public Health of Sun Yat-sen University. Written informed consent to participate in this study was provided by the participants’ legal guardian/next of kin.
Author contributions
LC conceived the study. SW performed data curation, statistical analysis, and prepared the manuscript draft. FZ and YH provided guidance on the process of fatty acid detection. YC performed the investigation and carried out quality control. LC, XW, and LL revised the initial manuscript. TH, SD, and IS critically reviewed this manuscript. All authors contributed to the manuscript revision and approved the submitted version.
Funding
This work was supported by the Key-Area Research and Development Program of Guangdong Province (2019B030335001) and Huhhot Science & Technology Plan (No. 2021-National Center of Technology innovation for Dairy-4).
Acknowledgments
We thank all the participating families and the research assistants involved in our cohort study.
Conflict of interest
Authors TH, GF, and IS were employed by Inner Mongolia Yili Industrial Group Co., Ltd. Authors TH, SD, and GF were employed by Inner Mongolia Dairy Technology Research Institute Co., Ltd.
The remaining authors declare that the research was conducted in the absence of any commercial or financial relationships that could be construed as a potential conflict of interest.
Publisher’s note
All claims expressed in this article are solely those of the authors and do not necessarily represent those of their affiliated organizations, or those of the publisher, the editors and the reviewers. Any product that may be evaluated in this article, or claim that may be made by its manufacturer, is not guaranteed or endorsed by the publisher.
Supplementary material
The Supplementary Material for this article can be found online at: https://www.frontiersin.org/articles/10.3389/fnut.2022.978679/full#supplementary-material
References
1. Pan XF, Wang L, Pan A. Epidemiology and determinants of obesity in China. Lancet Diabetes Endocrinol. (2021) 9:373–92.
2. Pietrobelli A, Agosti M, MeNu G. Nutrition in the first 1000 days: ten practices to minimize obesity emerging from published science. Int J Environ Res Public Health. (2017) 14:1491. doi: 10.3390/ijerph14121491
4. Collins CT, Gibson RA, McPhee AJ, Makrides M. The role of long chain polyunsaturated fatty acids in perinatal nutrition. Semin Perinatol. (2019) 43:151156.
5. Oosting A, Kegler D, Boehm G, Jansen HT, van de Heijning BJ, van der Beek EM. N-3 long-chain polyunsaturated fatty acids prevent excessive fat deposition in adulthood in a mouse model of postnatal nutritional programming. Pediatr Res. (2010) 68:494–9. doi: 10.1203/PDR.0b013e3181f74940
6. Ailhaud G, Massiera F, Weill P, Legrand P, Alessandri JM, Guesnet P. Temporal changes in dietary fats: role of n-6 polyunsaturated fatty acids in excessive adipose tissue development and relationship to obesity. Prog Lipid Res. (2006) 45:203–36. doi: 10.1016/j.plipres.2006.01.003
7. Heerwagen MJ, Stewart MS, de la Houssaye BA, Janssen RC, Friedman JE. Transgenic increase in N-3/n-6 fatty acid ratio reduces maternal obesity-associated inflammation and limits adverse developmental programming in mice. PLoS One. (2013) 8:e67791. doi: 10.1371/journal.pone.0067791
8. Donahue SM, Rifas-Shiman SL, Gold DR, Jouni ZE, Gillman MW, Oken E. Prenatal fatty acid status and child adiposity at age 3 y: results from a US pregnancy cohort. Am J Clin Nutr. (2011) 93:780–8. doi: 10.3945/ajcn.110.005801
9. Vidakovic AJ, Gishti O, Voortman T, Felix JF, Williams MA, Hofman A, et al. Maternal plasma PUFA concentrations during pregnancy and childhood adiposity: the generation R study. Am J Clin Nutr. (2016) 103:1017–25. doi: 10.3945/ajcn.115.112847
10. Maslova E, Rifas-Shiman SL, Olsen SF, Gillman MW, Oken E. Prenatal n-3 long-chain fatty acid status and offspring metabolic health in early and mid-childhood: results from project viva. Nutr Diabetes. (2018) 8:29. doi: 10.1038/s41387-018-0040-2
11. Vahdaninia M, Mackenzie H, Dean T, Helps S. The effectiveness of omega-3 polyunsaturated fatty acid interventions during pregnancy on obesity measures in the offspring: an up-to-date systematic review and meta-analysis. Eur J Nutr. (2019) 58:2597–613. doi: 10.1007/s00394-018-1824-9
12. Mathias RA, Pani V, Chilton FH. Genetic variants in the FADS gene: implications for dietary recommendations for fatty acid intake. Curr Nutr Rep. (2014) 3:139–48. doi: 10.1007/s13668-014-0079-1
13. Hodson L, Skeaff CM, Fielding BA. Fatty acid composition of adipose tissue and blood in humans and its use as a biomarker of dietary intake. Prog Lipid Res. (2008) 47:348–80.
14. Folch J, Lees M, Sloane Stanley GH. A simple method for the isolation and purification of total lipides from animal tissues. J Biol Chem. (1957) 226:497–509.
15. WHO. WHO child growth standards based on length/height, weight and age. Acta Paediatr. (2006) 450:76–85.
16. Metzger BE, Gabbe SG, Persson B, Buchanan TA, Catalano PA, Damm P, et al. International association of diabetes and pregnancy study groups recommendations on the diagnosis and classification of hyperglycemia in pregnancy. Diabetes Care. (2010) 33:676–82.
17. Schwinger C, Fadnes LT, Van den Broeck J. Using growth velocity to predict child mortality. Am J Clin Nutr. (2016) 103:801–7.
18. Drouin G, Rioux V, Legrand P. The n-3 docosapentaenoic acid (DPA): a new player in the n-3 long chain polyunsaturated fatty acid family. Biochimie. (2019) 159:36–48. doi: 10.1016/j.biochi.2019.01.022
19. Kim HK, Della-Fera M, Lin J, Baile CA. Docosahexaenoic acid inhibits adipocyte differentiation and induces apoptosis in 3T3-L1 preadipocytes. J Nutr. (2006) 136:2965–9.
20. Wojcik C, Lohe K, Kuang C, Xiao Y, Jouni Z, Poels E. Modulation of adipocyte differentiation by omega-3 polyunsaturated fatty acids involves the ubiquitin-proteasome system. J Cell Mol Med. (2014) 18:590–9. doi: 10.1111/jcmm.12194
21. Simeoni U, Armengaud JB, Siddeek B, Tolsa JF. Perinatal origins of adult disease. Neonatology. (2018) 113:393–9.
22. Moon RJ, Harvey NC, Robinson SM, Ntani G, Davies JH, Inskip HM, et al. Maternal plasma polyunsaturated fatty acid status in late pregnancy is associated with offspring body composition in childhood. J Clin Endocrinol Metab. (2013) 98:299–307. doi: 10.1210/jc.2012-2482
23. Ren X, Vilhjalmsdottir BL, Rohde JF, Walker KC, Runstedt SE, Lauritzen L, et al. Systematic literature review and meta-analysis of the relationship between polyunsaturated and trans fatty acids during pregnancy and offspring weight development. Front Nutr. (2021) 8:625596. doi: 10.3389/fnut.2021.625596
24. Williams MA, Frederick IO, Qiu C, Meryman LJ, King IB, Walsh SW, et al. Maternal erythrocyte omega-3 and omega-6 fatty acids, and plasma lipid concentrations, are associated with habitual dietary fish consumption in early pregnancy. Clin Biochem. (2006) 39:1063–70. doi: 10.1016/j.clinbiochem.2006.09.008
25. Araujo P, Kjellevold M, Nerhus I, Dahl L, Aakre I, Moe V, et al. Fatty acid reference intervals in red blood cells among pregnant women in Norway-cross sectional data from the ‘little in Norway’ cohort. Nutrients. (2020) 12:2950. doi: 10.3390/nu12102950
26. Cano-Sancho G, Casas M. Interactions between environmental pollutants and dietary nutrients: current evidence and implications in epidemiological research. J Epidemiol Commun Health. (2021) 75:108–13. doi: 10.1136/jech-2020-213789
27. Much D, Brunner S, Vollhardt C, Schmid D, Sedlmeier EM, Brüderl M, et al. Effect of dietary intervention to reduce the n-6/n-3 fatty acid ratio on maternal and fetal fatty acid profile and its relation to offspring growth and body composition at 1 year of age. Eur J Clin Nutr. (2013) 67:282–8. doi: 10.1038/ejcn.2013.2
28. Djuricic I, Calder PC. Beneficial outcomes of omega-6 and omega-3 polyunsaturated fatty acids on human health: an update for 2021. Nutrients. (2021) 13:2421. doi: 10.3390/nu13072421
29. Innis SM. Essential fatty acid transfer and fetal development. Placenta. (2005) 26(Suppl. A):S70–5.
30. Serhan CN, Chiang N, Dalli J. The resolution code of acute inflammation: novel pro-resolving lipid mediators in resolution. Semin Immunol. (2015) 27:200–15. doi: 10.1016/j.smim.2015.03.004
31. Barbarich BN, Willows ND, Wang L, Clandinin MT. Polyunsaturated fatty acids and anthropometric indices of children in rural China. Eur J Clin Nutr. (2006) 60:1100–7. doi: 10.1038/sj.ejcn.1602424
32. Simopoulos AP. An increase in the omega-6/omega-3 fatty acid ratio increases the risk for obesity. Nutrients. (2016) 8:128.
33. Simopoulos AP. The importance of the ratio of omega-6/omega-3 essential fatty acids. Biomed Pharmacother. (2002) 56:365–79.
34. Brei C, Stecher L, Much D, Karla MT, Amann-Gassner U, Shen J, et al. Reduction of the n-6: n-3 long-chain PUFA ratio during pregnancy and lactation on offspring body composition: follow-up results from a randomized controlled trial up to 5 y of age. Am J Clin Nutr. (2016) 103:1472–81. doi: 10.3945/ajcn.115.128520
35. Wei Y, Meng Y, Li N, Wang Q, Chen L. The effects of low-ratio n-6/n-3 PUFA on biomarkers of inflammation: a systematic review and meta-analysis. Food Funct. (2021) 12:30–40. doi: 10.1039/d0fo01976c
36. Shah NR, Braverman ER. Measuring adiposity in patients: the utility of body mass index (BMI), percent body fat, and leptin. PLoS One. (2012) 7:e33308. doi: 10.1371/journal.pone.0033308
37. Poissonnet CM, Burdi AR, Bookstein FL. Growth and development of human adipose tissue during early gestation. Early Hum Dev. (1983) 8:1–11.
Keywords: polyunsaturated fatty acid, pregnancy, offspring, weight status, birth cohort
Citation: Wu S, Zhao F, He Y, He T, Duan S, Feng G, Chen Y, Wang X, Szeto IM-Y, Lin L and Cai L (2022) Association between maternal erythrocyte polyunsaturated fatty acid levels during pregnancy and offspring weight status: A birth cohort study. Front. Nutr. 9:978679. doi: 10.3389/fnut.2022.978679
Received: 26 June 2022; Accepted: 30 August 2022;
Published: 29 September 2022.
Edited by:
Zhiyong Zou, Peking University, ChinaReviewed by:
Kaixiong Ye, University of Georgia, United StatesHans Demmelmair, Ludwig Maximilian University of Munich, Germany
Copyright © 2022 Wu, Zhao, He, He, Duan, Feng, Chen, Wang, Szeto, Lin and Cai. This is an open-access article distributed under the terms of the Creative Commons Attribution License (CC BY). The use, distribution or reproduction in other forums is permitted, provided the original author(s) and the copyright owner(s) are credited and that the original publication in this journal is cited, in accordance with accepted academic practice. No use, distribution or reproduction is permitted which does not comply with these terms.
*Correspondence: Li Cai, Y2FpbGk1QG1haWwuc3lzdS5lZHUuY24=