- College of Food Science and Technology, Henan University of Technology, Zhengzhou, China
Xylooligosaccharide (XOS) are functional oligosaccharides with prebiotic activities, which originate from lignocellulosic biomass and have attracted extensive attention from scholars in recent years. This paper summarizes the strategies used in the production of XOS, and introduces the raw materials, preparation methods, and purification technology of XOS. In addition, the biological characteristics and applications of XOS are also presented. The most commonly recommended XOS production strategy is the two-stage method of alkaline pre-treatment and enzymatic hydrolysis; and further purification by membrane filtration to achieve the high yield of XOS is required for prebiotic function. At the same time, new strategies and technologies such as the hydrothermal and steam explosion have been used as pre-treatment methods combined with enzymatic hydrolysis to prepare XOS. XOS have many critical physiological activities, especially in regulating blood glucose, reducing blood lipid, and improving the structure of host intestinal flora.
Introduction
XOS are functional oligosaccharides, which are composed of 2–7 xylose molecules linked by β-1, 4-glycosidic bonds, and the relative molecular weight is generally about 200–300 kDa (1, 2). XOS have excellent physical and chemical properties, such as high heat and acid resistance (3). The sweetness of XOS is about 40%-50% of sucrose (4). The viscosity of XOS is lower than other oligosaccharides, which can reduce the water activity and improve the ability to hold water in water solution (5).
In addition to excellent physical and chemical properties, XOS are also the research hotspots of scholars from all walks of life as prebiotics (6). A large number of animal experiments have proved the beneficial effects of XOS in preventing caries, regulating blood glucose, reducing blood lipid, reducing cholesterol, preventing inflammatory, improving immunity, preventing oxidation, promoting calcium absorption, which are relating to the ability of regulating intestinal flora structure of XOS (7–16). In addition, XOS could effectively prevent obesity, cardiovascular disease, atherosclerosis, and intestinal diseases (17, 18). The International Association of probiotics and prebiotics (ISAPP) identified XOS as emergent prebiotic oligosaccharides in its latest update of its prebiotic definition (19).
Most XOS are prepared by degradation of agicultural biomass (20, 21). Typical raw materials for preparation of XOS include crop straws such as wheat and sugarcane, as well as processing byproducts such as corncob and rice husk (22). XOS can also be produced from the cotton stalk, corn straw, sugarcane bagasse, and other common agricultural wastes (23). There are three main methods of extracting XOS: autohydrolysis, acid hydrolysis, and enzymatic hydrolysis (24–26). At present, enzymatic hydrolysis preparation of XOS is the primary method (27).
XOS have great potential as food ingredients due to their price competitiveness, thermal stability and pH stability, sensory properties and multidimensional effects on human health and livestock compared with other prebiotics (28–30). Globally, XOS are mainly used in the feed industry (49.6%), followed by health and medical products (25.4%), food and beverage (23.2%), and other applications (1.8%) (31). In addition, the industry's interest in XOS is reflected in an increasing number of XOS patent applications (20). The global prebiotic ingredient market is estimated to be 4.07 billion in 2017, expected to reach $7.37 billion by 2023. The compound annual growth rate (CAGR) is 10.4% (32), and the Asia Pacific region, including China, India, and Japan, are expected to have the highest increase, exceeding 9.5% (33).
This article summarizes the research progress of preparation and purification methods of XOS in recent years and introduces the physiological activities and applications of XOS to provide the basis for the further development and application of XOS.
Preparation of XOS
Raw materials for XOS preparation
Figure 1 showed the schematic representation of the lignocellulosic biomass composition. Lignocellulose biomass are the non-starch part of renewable and abundant plant materials. Lignocellulose materials are mainly cellulose, hemicellulose, and lignin (35). The composition of lignocellulose varies, with an average of cellulose (30–50%), hemicellulose (20–40%), and lignin (15–25%) in the total dry matter (36). Cellulose is composed of a glucose molecular chain, which forms hydrogen bonds between different layers of polysaccharides and forms crystalline conformation. Xylan, the main component of hemicellulose, is the critical target of XOS production.
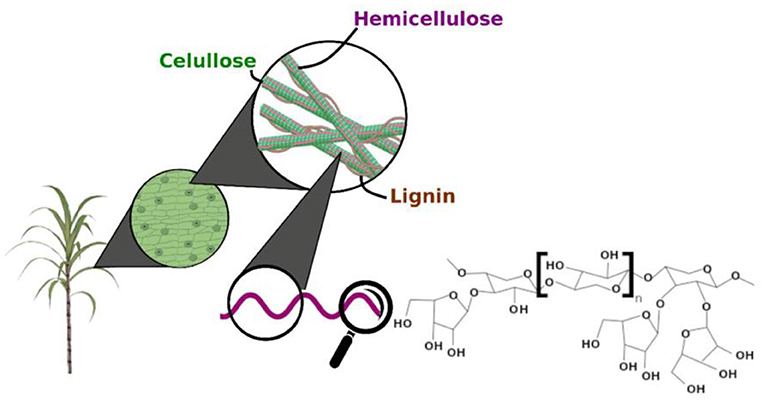
Figure 1. Schematic representation of the lignocellulosic biomass composition [Adopted from Capetti et al. (34)].
Figure 2 showed the structure of xylan. Xylan is the main component of hemicellulose (60–90%), a heteropolysaccharide with a degree of polymerization (DP) between 50 and 200, containing acetyl, 4-o-methyl-dglucouronosyl, and α- substituents of arabinofuranyl residues, related to the main chain of β-1,4-linked xylopyranose units (23, 37). Table 1 lists the composition of several common lignocellulose raw materials. The higher the xylan content of the raw materials, the lower the cost of XOS production. Among these lignocellulose biomass, the hemicelluose content of corncob, sugarcane bagasse, and wheat straw are relatively high, which are ideal raw materials for XOS industrial production.
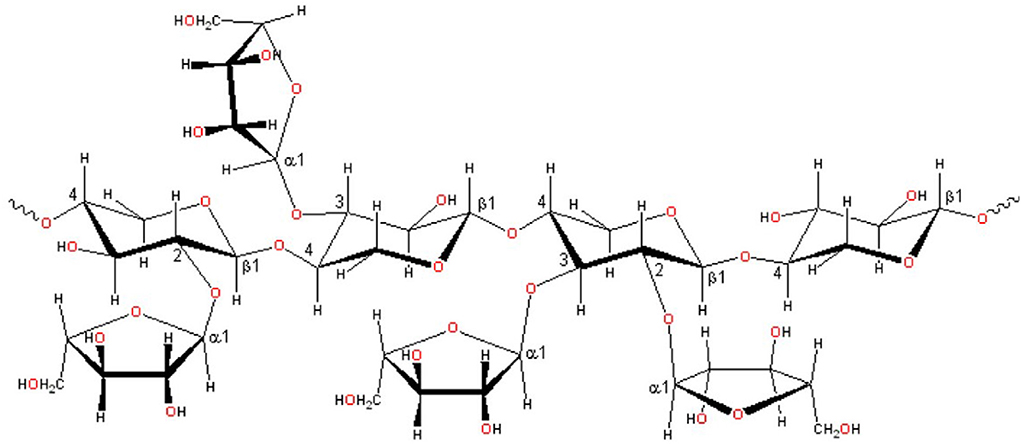
Figure 2. Xylan structure shows different intermolecular bonds [Adopted from Otieno et al. (7)].
Preparation of XOS
Acid hydrolysis
Xylan can be hydrolyzed into soluble XOS under acidic conditions. Generally, dilute hydrochloric acid and sulfuric acid are used to hydrolyze xylan with a high degree of polymerization to produce XOS. The purpose of acid treatment is to improve the hydrolysis degree of hemicellulose, to improve the yield of XOS. Hemicellulose is separated into oligosaccharides and monosaccharides with a wide range of DP through the breaking of glycosidic bonds of xylose (37, 47). However, acid hydrolysis leads to equipment corrosion, which limits its use. In addition, acid hydrolysis will produce excess xylose and other toxic reaction products at high temperatures, such as furfural and hydroxymethylfurfural (HMF), which are harmful to food applications (48, 49).
It was reported that the yield of XOS obtained by hydrolyzing poplar wood with 5% acetic acid at 170°C was 39.8% (50). Ying et al. elucidated that the increase of sulfuric acid dosage enhanced the lignin removal of poplar pretreated with hydrogen peroxide acetic acid (51). The maximum XOS yield was 68.5% when XOS were produced by hydrolyzing corncob with 5% propionic acid at 170°C for 50 min (52). It was reported that the product obtained by hydrolyzing brewer's grains with 1.85% sulfuric acid for 19.5 min contains 6.6 g/L arabinoxylooligosaccharide (AXOS) (53). The acetic acid pre-treatment of poplar could effectively produce XOS, with a yield of 55.8%, and acetylation degradation of lignin occurred after acetic acid pre-treatment (54).
Acid hydrolysis to obtain XOS has been widely used because it was a fast and easy technology (21). High XOS yield could be obtained by hydrolysis with sulfuric acid of lignocellulose biomass. However, the yield of oligomers is lower than monomers, mainly due to the higher yield of xylose (6, 55). Even some methods change the acid conditions, improve the yield of XOS and optimize the existing preparation process; but the acid hydrolysis efficiency is still not high; there are still many impurities in the prepared products; and the content of XOS is still low.
Autohydrolysis
Agricultural plant biomass rich in xylan can also be directly hydrolyzed under high temperature and high pressure to produce XOS. Autohydrolysis is a non-chemical process, which refers to the deacetylation of D-xylan at high temperature in the presence of water (56). Autohydrolysis occurs under slightly acidic conditions due to the partial cleavage of acetyl groups in plant cell walls to form acetic acid (57). In the process of autohydrolysis, XOS are typical reaction intermediate, and their concentration depends on the balance between the decomposition of polymer hemicellulose into XOS and their further decomposition into monomer xylose. Therefore, under medium conditions, the yield of XOS will be higher. Treatment with increased severity resulted in decreased DP and increased decomposition of XOS into xylose. Hemicellulose is easily affected by water under high pressure and high temperature. Exposure of lignocellulosic biomass to water causes hemicellulose to penetrate the cellular structure, resulting in cellulose hydration and hemicellulose depolymerization. The action mode of hydrothermal treatment of lignocellulosic biomass was in the subcritical region of water (100–374°C) (58).
Autohydrolysis is heat treatment with steam or liquid water at high temperature or high pressure (55, 56). Under the autohydrolysis, the autoionization of water will produce ions, which leads to the depolymerization effect of hemicellulose (59). Acetic acid is usually added during autohydrolysis to increase the formation of hydrogen ions (23). The yield of XOS is the high under moderately severe operating conditions (60). It was reported that the maximum yield of XOS (55.3 wt%) was obtained by hydrothermal treatment of pecan shells at 160°C for 2 h. At the same time, high temperature (220°C) and short time (0.5 h) were helpful in hydrolyzing XOS with high DP, in which the yield of XOS (DP2-6) was 37.5 wt% (61). The autohydrolysis of almond shells (200°C, 5 min) resulted in low DP, and the concentration of XOS (xylobiose and xylotriose) was only 3.5% (38). Small-scale (150 tons of brewery waste grain per day) biological refineries could make profits by valuing the waste grain produced by large breweries and applying high-solid hydrothermal technology to produce high-value products xylitol and XOS (62). It was also elucidated that the recovery rate of high-purity polymeric hemicellulose with molecular weight (21–30 kDa) was 35–37% when high-purity hemicellulose (xylan) was partially extracted from wood waste by alkali mediated hydrothermal method; the separated hemicellulose could be chemically transformed into high-value commercial products, such as prebiotics (XOS) (63).
The main advantage of autohydrolysis method is that it has low or no requirements for corrosive compounds and is marked as an environmentally friendly process (36). In the past decades, hydrothermal treatment has been widely studied as the first step of biorefinery because of its environmentally friendly advantages and the selectivity of dissolving hemicellulose as oligosaccharides over other treatments (64, 65). Hydrothermal pre-treatment is considered an ecologically friendly and inexpensive alternative method to treat lignocellulose (66, 67). Autohydrolysis technology automatically ionizes water into hydrogen ions, allowing hemicellulose compounds to be released from lignocellulose, such as acetyl groups in acetic acid. This organic acid acts as a mild catalyst during the reaction, which is conducive to the subsequent dissolution of other hemicellulose-derived compounds (68, 69). Therefore, hydrothermal treatment is a technology to reduce the corrosion effect and cost of different solvents, and has high selectivity for hemicellulose.
Although the consumption of chemicals is low, due to the high pressure and temperature conditions, autohydrolysis process requires high energy consumption. The green characteristics of autohydrolysis will also depend on the energy used (70). It was reported that the most common temperature range to achieve high yield and minimum degradation of compounds was about 160–180°C (42, 71). The high temperature usually causes the release of many monomers (xylose) and impurities, such as furfural and HMF produced by sugar degradation, as well as the phenolic compounds produced by lignin (72, 73). The acidic hydrogen ion is formed due to the release of acetyl group in lignocellulosic biomass; acetyl group is the catalyst for hemicellulose depolymerization (71). The depolymerization of oligomers begins with the random breaking of the bond of xylose, producing oligomers short enough to be extracted from the biomass structure (74).
The main disadvantage of heat treatment is still to produce a large number of unwanted byproducts, such as other oligomers, monosaccharides, acetic acid, furfural, HMF, formic acid, levulinic acid, phenolic compounds, etc., (30, 75). It was observed that the degradation compounds released from the mixed biomass of hydrothermal treatment, had a significant inhibitory effect on the growth of Lactobacillus brevis. The dissolved lignin concentration of 1 g/L inhibited the growth of Lactobacillus brevis. After the adsorption purification step using Amberlite XAD 16N resin, the purified XOS showed the exact cell yield and product yield as commercial XOS (76). In general, a separation process is required to remove unwanted compounds. The existence of the former compound in XOS mixture leads to serious purification difficulties, and then increases the production cost (56).
The release of degraded compounds depends on the composition of biomass and process conditions. Generally speaking, a separation process is required to remove unwanted compounds. The presence of the former compound in the XOS mixture leads to serious purification difficulties and consequent increased production costs (56). Another disadvantage of using autohydrolysis to produce XOS is that special equipment is required due to high temperature and high pressure (23, 24).
Enzymatic hydrolysis
Figure 3 showed the xylanase hydrolysis of lignocellulose. XOS can also be produced by enzymatic hydrolysis of xylan. Compared with acid hydrolysis and autohydrolysis, enzymatic degradation is an ideal XOS production method because it has specificity and the least byproducts. In addition, enzymatic hydrolysis does not require any special equipment. The current commercial process uses an enzyme process, which has mild operating conditions, and does not use toxic chemicals, so enzymatic hydrolysis is more in line with the viewpoint of biodegradation process. In addition, the use of xylanase is efficient and specific, allowing higher control of DP and reducing the production of unwanted xylose and other byproducts (77).
Enzymatic hydrolysis is usually used for the extraction of XOS due to the mild enzymatic hydrolysis conditions and high product quality (78). However, the isomerization of lignocellulosic materials will be seriously affected by the structure of composite lignin-hemicellulose. Therefore, it is essential to destroy the composite structure to expose more hemicellulose to improve the extraction efficiency. Physical and chemical pre-treatment technology have been used before enzymatic hydrolysis. In addition, the source of materials and xylanase have an impact on enzymatic hydrolysis.
Physical pre-treatment
Physical pre-treatment mainly includes the hydrothermal method, steam explosion method, ultrasonic method, and microwave method.
Steam explosion is instantaneous blasting under high temperature and high pressure. The hemicellulose recovery of corn cob after steam explosion at 196°C for 5 min was 22.8% (79). After a steam explosion at 204°C for 4 min and 180°C for 30 min, the yield of XOS in wheat straw was 8.9 and 13.9/100 g (72, 80). Steam explosion was also applied to the pre-treatment of rice husk, and the final output of XOS was 17.35 mg/mL xylan (81). In addition, after the steam explosion, 40% of xylan was degraded into XOS, and the degree of polymerization of steam explosion hydrolysates had good prebiotic properties (82).
The hydrothermal method processes materials in high-temperature or high-pressure hot water. It was reported that the xylan yield of wheat straw reached 56.2 g/kg after hydrothermal pre-treatment at 180°C for 40 min (39). After hydrothermal pre-treatment at 190°C, 1.8 MPa for 13 min, the extraction rate of xylan from corncob was 18% (6). The extraction rate of xylan was 23.82/100 g from dry corn straw subjected to non-isothermal hydrothermal pre-treatment (83). The extraction of XOS from corn fiber by hydrothermal pre-treatment at 160°C was also reported (84).
Ultrasonic and microwave had been applied to the pre-treatment of lignocellulose materials. Under 121°C ultrasonic pre-treatment, 39% of xylan in corncob was released, which was higher than the conventional extraction method (34%), and the extraction time shortened from 24 h to 43 min (85). After microwave pre-treatment at 185°C for 10 min, the recovery rate of xylan was higher than that of high-pressure steam pre-treatment, because microwave pre-treatment was easy to control the degree of reaction (86).
The results showed that physical pre-treatment was helpful to the release of xylan and XOS. The steam explosion can significantly improve the release of XOS, which has the advantages of simple operation, no pollution, low energy consumption, and a short production cycle. Therefore, the steam explosion is a promising pre-treatment method for XOS extraction in the future.
Chemical pre-treatment
Chemical pre-treatment mainly uses acid and alkali to extract xylan from lignocellulosic materials, and the yield of xylan varies according to the source of materials and extraction conditions. Table 2 lists the material sources, extraction conditions, and pre-treatment methods.
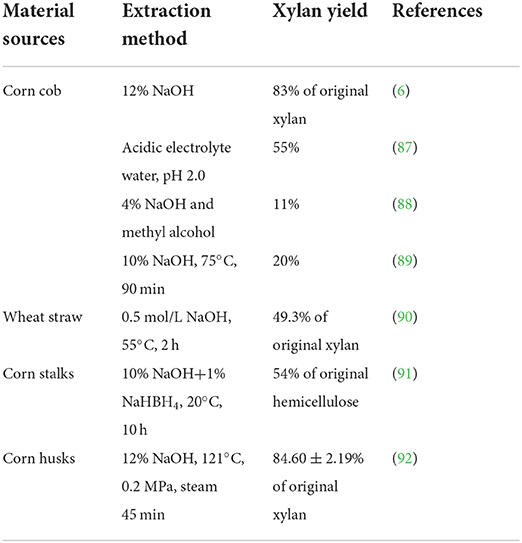
Table 2. Material sources, conditions, and xylan yield of commonly used chemical pre-treatment methods.
As shown in Table 2, the most commonly used chemical pre-treatment method was alkali extraction, which mainly used 5–24% sodium hydroxide and potassium hydroxide as extraction solvents. However, the yield of xylan was relatively low when alkali extraction is used alone. Auxiliary methods such as steam and ultrasound are needed to improve the yield of xylan. Xylan was extracted from corncob with sodium hydroxide and methanol solvent, and the extraction rate reached 11% (89). A similar xylan yield can be obtained when xylan is extracted from dry corncob with 10% sodium hydroxide at 75°C (89). The yields of XOS were 83 and 84.5%, respectively, when xylan were extracted from corncob and corn shells with 12% sodium hydroxide solution with the steam pre-treatment (6, 92). Ultrasonic assisted alkali extraction can significantly improve the yield of XOS in corncob, and the content of related XOS can reach 174.81 mg/g matrix (93).
Due to the low efficiency of acid extraction, acid extraction was rarely used and was usually assisted by physical methods. Xylan was extracted from corncob by acid electrolyte water (pH 2.0) combined with the steam explosion, and the extraction rate was 55% (87). After pretreated corncob with dilute acid and alkali to extract the lignin-saccharide complex, the yeild of XOS was 5.80 ± 0.14 mg/mL from the complex by xylanase hydrolysis (94). According to reports, after being dissolved in 1.0 M H2SO4 for 12 h, XOS were extracted from corncob by high-pressure hydrolysis, and the maximum yield was 67.7% (95).
The alkali extraction method is greatly affected by temperature and the extraction rate increases with the increase of temperature. The alkali extraction method does not produce byproducts, which is better than the acid extraction method, but it have high requirements for equipment. Therefore, it is suggested to use physical processes (such as steam and ultrasonic) and chemical processes to improve the yield of xylan.
Xylanase hydrolysis
Xylanase systems include endo-xylanase and xylose releasing enzyme exo-xylanase, or β-xylosidase, and debranching enzyme (30, 96). For the production of XOS, only endo-xylanase are meaningful. Based on sequence conservation, these enzymes can be found in glycoside hydrolase families (GH) 5, 8, 10, 11, and 43. In addition, exo-xylanase or β-xylosidase preparations with low activities were needed to avoid xylose production (56). Xylanases have been isolated from many different fungi and bacteria, but most commercial xylanase hydrolysates are currently produced by transgenic xylanase strains (97, 98).
It was reported that the main product was xylobiose after the cauliflower stalk was hydrolyzed by natural endo-xylanase extracted from Aspergillus niger TCC9687. The cauliflower stalk XOS showed significantly high antioxidant and antibacterial activities and reduced the viability of human bone cancer MG-63 cells, both alone and in combination with (Lactiplantibacillus plantarum, Bifidobacterium bifidum, Lactobacillus delbrueckiissp. Helveticus); the antibacterial components of cauliflower stalkm XOS were dihydroxybenzoic acid and aspartic acid (99). Abdella et al. reported that after the xylanase produced by Paecilomyces wallichii was applied to beech xylan to produce different types of XOS; when the extract concentration was from 0.1 to 1.5 mg/mL, the antioxidant activity of XOS increased from 15.22 to 70.57% (100). XOS from oil palm empty fruit bunch hemicellulose produced by xylanase from Thermomyces cyanobacterium hydrolysis was composed of xylotriose and xylobiose. XOS was evaluated as the substrate of two probiotics (Lactobacillus plantarum WU-P19 makes better use of XOS than Bifidobacterium TISTR2129) found in the human gastrointestinal tract (101).
Table 3 lists the hydrolysis and yield of different raw materials with endo-xylanase from different sources. It can be seen from Table 3 that the yield of fungal xylanase is high. It is reported that bacteria such as bacillus and streptomyces could also produce xylanase (114). Recombinant xylanase could also obtain a relatively high yield, but large-scale natural production of recombinant enzyme required a highly complex purification process, which significantly increases the cost. Enzymatic hydrolysis of xylan could also be achieved in situ by microbial fermentation. In this process, bacteria were cultured to produce xylanase and secreted into the reaction medium, where the enzyme hydrolyzes xylan to produce XOS (115).
Xylanase hydrolysis process is relatively soft and easy to purify. There is no apparent other production, and the color of XOS is relatively light. XOS prepared by enzymatic hydrolysis have good prebiotic potential and antioxidant performance. In addition, the use of xylanase has high efficiency and specificity, allowing higher control of DP and reducing the production of unwanted xylose and other byproducts. At present, xylanase hydrolysis is the primary method to produce XOS.
To sum up, the existing methods have optimized the preparation of XOS to a certain extent and improved product efficacy. In recent years, the industrial application of XOS has been greatly limited due to high content of impuritie XOS; and the product quality of XOS was not easy to control. Therefore, the refining, separation, and purification of XOS have also become the key to subsequent industrial application. At present, the development of XOS has not reached its peak. As a new generation of functional sugars, XOS have not been fully used. These production optimizations have promoted the application and development of XOS and laid the primary theoretical foundation for large-scale popularization and use in the future.
XOS purification
After XOS production, undesirable compounds and oligosaccharides were produced (116). The presence of unwanted compounds such as glucose and xylose will increase the calorific value of XOS and change their sweetness ability (56). On the other hand, the prebiotic effect of XOS also seems to depend on their purity level. It has been observed that high-purity XOS products have a more significant impact on biological function (57).
To remove unwanted components and obtain high-purity XOS, subsequent purification treatment is required (28). In particular, more components will effect the purity of product when the autohydrolysis method is adopted to treat lignocellulose (117).
The commonly used purification methods include adsorption separation, solvent extraction, membrane separation, and chromatographic separation.
Adsorption on the active solid surface is usually used in combination with the solvent elution step to separate oligomers from monomers and remove other unwanted pollutants. Commonly used adsorbents for purifying XOS include activated carbon, acid clay, bentonite, diatomite, aluminum hydroxide oxide, titanium, silica, and porous synthetic materials (1, 56, 118). Among them, activated carbon is the most commonly used evaluation method, whether in solution or fixed bed adsorption. Activated carbon treatment has proven to be a viable option for removing extract-derived, lignin-derived, and carbohydrate degrading compounds present in XOS mixtures (119). On the other hand, ion exchange resins are combined with different purification strategies to remove salts, heavy metal ions, charged organic compounds and pigments in XOS mixtures (56, 120).
Solvent extraction mainly removes the non-sugar components from the hydrolysate. The recovery and purification degree of the XOS mixture depend on the solvent used for extraction. Ethanol, acetone, and isopropanol are the most common options for refining crude XOS solutions (121–123). In XOS production, solvent extraction is usually used to recover hemicellulose derivatives from pre-treatment (55). In this case, vacuum evaporation is generally used in the first stage to remove volatile compounds and concentrate XOS solution (56). On the other hand, organic solvent precipitation allows the recovery of XOS or xylose while removing phenols and extracting derived compounds.
Chromatographic separation for XOS purification produces analytical grade high-purity components. Gel permeation chromatography (GPC) (124), water-soluble exclusion chromatography (SEC) (125), ion-exchange chromatography (IEC), and centrifugal partition chromatography (CPC) are some standard technologies for purifying XOS (126, 127), Ho et al. used GFC to purify XOS produced by autohydrolysis of agricultural residues. In this cases, GFC could effectively remove high DP oligosaccharides. More importantly, GFC could remove unwanted small molecules, such as monosaccharides, acetic acid, and degradation compounds (furfural, HMF, and phenol) (128).
Membrane separation is another powerful technique commonly used for oligomer purification. Ultrafiltration and nanofiltration based technology is the most promising processing strategy for manufacturing high-purity and concentrated oligosaccharides (55). The popularity of this technology can be attributed to its low energy consumption requirements, relatively easy amplification, and easy operation variables (13, 116, 129–131). Membrane technology is currently considered to be the most promising strategy for industrial production of high-purity XOS. In this case, the ultrafiltration separation of oligosaccharides from high molecular weight compounds has low energy consumption and is easy to operate and enlarge (128). However, its disadvantage is that its performance is poor when small molecules must be removed.
Meanwhile, purification strategies with different properties are often used in combination to improve the purification of XOS. It was reported that a combination of nanofiltration, solvent extraction and dual ion exchange chromatography method could achieve 90.7% XOS purity (132).
Nutritional properties of XOS
Figure 4 showed the main nutritional properties of XOS. XOS have many important physiological activities, especially in regulating blood glucose, reducing blood lipids, improving antioxidant capacity, preventing cancer, preventing dental, and improving the structure of host intestinal flora.
Optimizing the intestinal flora
Figure 5 showed the role of XOS in regulating intestinal flora. XOS can change the composition of intestinal microorganisms, increase the number of probiotics and produce healthy fatty acids. It was found that XOS from giant awn could increase the number of Bifidobacteria, Lactobacillus and Escherichia coli without affecting the number of pathogenic bacteria such as Clostridium perfringens (134). XOS extracted from corn straw also had a significant effect on the proliferation of Lactobacillus and Bacteroides (80). The addition of XOS during fattening period would increase the concentration of acetic acid, linear fatty acids and short-chain fatty acids in pig intestinal contents, and change the composition and metabolic activity of intestinal flora (135). The intake of XOS could significantly increase the number of Bifidobacteria in human intestine (136). It was elucidated that in vitro fermentation of XOS from birch could significantly proliferated the number of Bifidobacteria, Staphylococcus, especially Staphylococcus hominis, which could produce bacteriostasis and inhibit corresponding pathogenic bacteria such as Staphylococcus aureus and Helicobacter pylori (137). Hald et al. elucidated that after ingestion of arabinoxylan, the number of Bifidobacteria in feces increased significantly, while the number of Lactobacillus, Clostridium, and Akmann mucophilus did not change significantly (138).
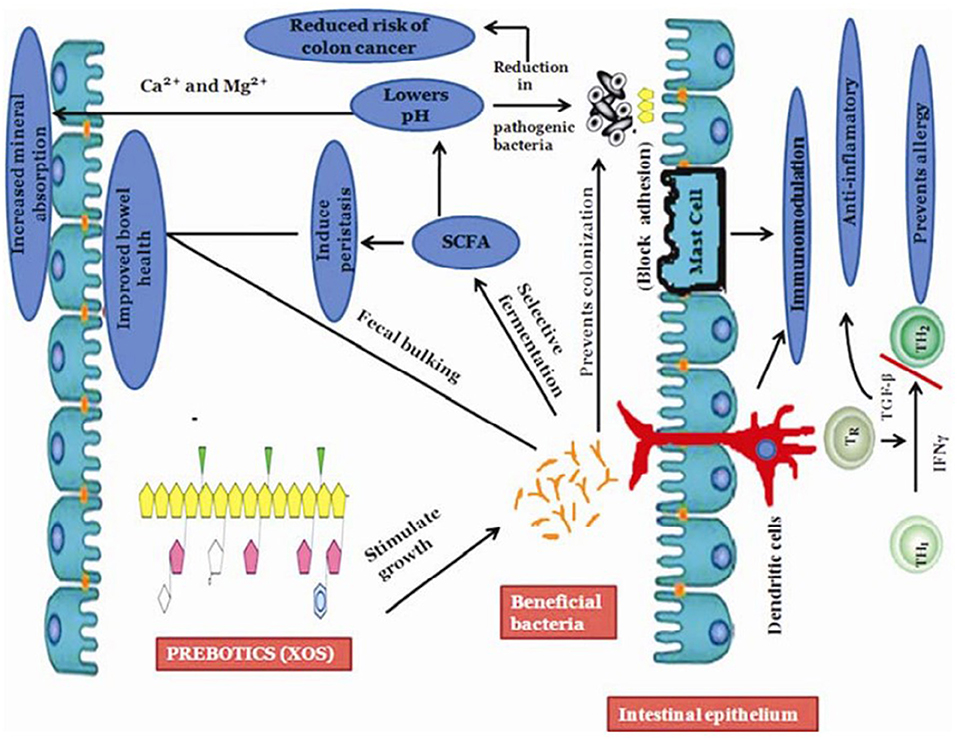
Figure 5. Illustration of benefits incurred by prebiotics on immune system: Stimulates growth of the beneficial bacterium in the large intestine that prevents colonization of harmful bacteria; increases production of short-chain fatty acids (SCFA) and helps in improving bowel health that reduces risks of colon cancer [Adopt from Slizewska et al. (133)].
The reason that XOS selectively proliferate beneficial bacteria such as Bifidobacteria in the intestine is related to the production of vitamins and immune stimulation (139). The proliferation of Bifidobacteria in the intestine will also inhibit the growth and reproduction of pathogenic bacteria, produce some digestive enzymes and help the body rebuild the intestinal flora (140). The effect of XOS on intestinal health is also reflected in the large production of organic acids, such as short-chain fatty acids, acetic acid, propionic acid, and butyric acid, as well as other organic acids such as lactic acid, succinic acid, formic acid, isobutyric acid, valeric acid, caproic acid, and isohexanoic acid (99, 141). These organic acids play essential roles in preventing various intestinal diseases. The increase in acetic acid concentration was particularly significant after ingestion of Arabinoxylooligosaccharide (136). It was reported that the proliferation of Bifidobacteria caused by the intake of XOS is an essential reason for maintaining intestinal health and preventing intestinal diseases (30). The study found that after the intake of XOS, the number of Enterobacteriaceae and Clostridium perfringens decreased significantly, which effectively reduced the incidence of intestinal diseases caused by harmful bacteria (88, 142).
XOS can selectively proliferate beneficial bacteria for three reasons: (1) Providing energy materials for beneficial bacteria (143). (2) Proliferating beneficial bacteria form a microbial barrier to prevent pathogen colonization (144). (3) XOS are fermented and utilized by Bifidobacteria and other microorganisms in the intestine, and the organic acids produced reduce the pH of the intestine, and most pathogenic bacteria are suitable to grow in a neutral environment to inhibit the growth and reproduction of pathogenic microorganisms (145).
Regulating blood sugar
XOS have unique molecular structure of β-1, 4 glycosidic bonds so that the enzymes in the digestive tract in the body cannot decompose them and cannot be directly absorbed and utilized by the human body, so they do not affect the blood glucose concentration. XOS cannot be digested and absorbed by the animal gastrointestinal tract but can be fermented and utilized by beneficial bacteria such as Bifidobacteria in the intestine in the large intestine and produce a large number of organic acids such as short-chain fatty acids (146). It was found that type 2 diabetic patients had significantly lower blood sugar levels after 8 weeks of XOS intake (147). The intake of 5% XOS could significantly reduced the blood glucose of obese mice (146). XOS from cereals could effectively improve the blood glucose level of mammals (148).
It was reported that XOS regulated blood glucose and lipid metabolism in mammals depending on their fermentation process in the colon (148). Some researchers argued that XOS could improve glucose tolerance by reducing plasma glucose levels and enhancing insulin sensitivity (149). It was reported that after XOS were ingested by mammals, a large amount of propionic acid produced by the fermentation of beneficial bacteria in the intestine could stimulate the production of glucagon like peptide 1 (GLP-1), which stimulates the secretion of insulin, thereby increasing the synthesis of liver glycogen and reducing the level of plasma glucose (150).
To sum up, XOS play a regulatory role in blood glucose levels. For people with high glucose, diabetes, or impaired glucose tolerance, XOS intake has a positive effect on lowering blood sugar levels, which is consistent with most studies.
Reducing blood lipids
Many studies have shown that XOS could effectively reduce the lipid levels of obese people. For example, it was found that after 8 weeks of continuous intake of 4 g/d xylose, fat in patients with type 2 diabetes decreased significantly (147). XOS could reduce the levels of total cholesterol, low-density lipoprotein, triglyceride, and increase the level of high-density lipoprotein in obese mice with a high-fat diets (146). XOS could also reduce the fat level of broilers (151).
The intake of oligosaccharides will reduce the levels of total auxin and acylated auxin. In contrast, the reduction of acylated auxin will reduce food intake to improve obesity and control metabolism. It was found that the short-chain fatty acids produced by microbial fermentation of XOS in the intestine affect the metabolism of cholesterol, in which propionic acid was absorbed by the intestine and entered the blood through the portal vein to the liver to reduce the synthesis of cholesterol in the liver and improve the sensitivity of insulin to regulate the body's lipid metabolism (152). In addition, some studies have explained the mechanism of XOS reducing blood lipid from the perspective of bile acid. It was found that the mechanism that XOS reduced the cholesterol level of patients with high cholesterol was the excessive excretion of bile acids (153).
Improving antioxidant capacity
XOS also have antioxidant activity (154). The antioxidant activities of XOS are mainly reflected in increasing the content of non-enzymatic antioxidant substances and improving the activity and level of antioxidant enzymes (147). It was found that XOS could significantly reduce the levels of oxidized glutathione (GSH) and malondialdehyde (MDA) in serum, heart and liver of high-fat diet mice, and normal mice (146). XOS intake could significantly increase the activity and level of antioxidant enzymes such as SOD (superoxide dismutase), CAT (catalase), and GSH PX (glutathione peroxidase) in the heart of mice with a high-fat diet (155). Abasubong et al. reported that 5% XOS (the mass fraction of 1.5%) could significantly improve the growth performance, antioxidant capacity, innate immunity, and hydrophilic bacilli resistance of Sparus macrocephalus (156). XOS could increase the contents of Lactobacillus and Bifidobacterium in mouse feces and reduce the contents of Enterococcus, Enterobacter, and Clostridium. The vitro antioxidant results showed that the conbination of XOS and Lactobacillus plantarum had free radical scavenging activity (154).
Preventing cancer
Short-chain fatty acids and other organic acids produced by XOS fermentation in the intestine have a specific role in preventing cancer, and their immune regulation in the body are essential means to prevent cancer (146, 157). Studies have shown that XOS could change the intestinal microbiota of mice and improve the intestinal barrier (158). It was demonstrated that XOS could reduce systemic inflammation, increase trabecular thickness, reduce osteoclasts and active erosive surfaces, and restore the rate of mineral deposition and bone formation in male Wistar rats (159). Yin et al. found that the inflammatory state and intestinal barrier of XOS-fed piglets improved significantly (160). Many reports have reported that the addition of oligosaccharides could reduce the expression of TNF-α (tumor necrosis factor) and NF-κβ (proinflammatory nuclear transcription factor protein) in the colon (161). It was also verified the anti-inflammatory activity of wheat arabinose oligosaccharides (162). XOS could also significantly increase the activation potential of T cells and B cells in tumor-bearing mice, as well as the immune ability in body fluids and cellular mediators, and play an anti-tumor role. It was also reported that the taking of XOS could minimize the risk of colon cancer, produce cytotoxic effects on leukemia cells, improve the immune system, and has a positive effect on type 2 diabetes (40, 163–165).
Beneficial bacteria can regulate immune factors and antibodies by using organic acids produced in the process of XOS to improve the immune function of the body (166). Bifidobacterium can increase the number of peripheral leukocytes so that the immune function is enhanced through the proliferation of Bifidobacteria (154). At the same time, XOS can increase the number of blood monocytes, serum alkaline phosphatase activity, and lysozyme activity. As an immune adjuvant, Bifidobacterium can recognize PP lymph nodes, activate intestinal lymph nodes, and induce lymphocyte outflow through lymphatic vessels; the immune system of the body is activated through lymphatic circulation (167). In addition, oligosaccharides directly bind to sugar receptors on the surface of immune cells to stimulate immune cell differentiation and increase activity; XOS can also be used as foreign antigens to effectively and permanently stimulate the immune system and promote the cell division and development of immune organs (153).
Other nutritional properties of XOS
At the same time, XOS can also prevent dental caries (168). XOS can not be decomposed by Streptococcus mutans and other bacteria in the mouth. The absorption rate of calcium was improved when XOS and calcium were ingested simultaneously (161). Kobayashi et al. found that the use of acidic XOS in mice with iron deficiency anemia significantly reduced the contents of ferritin in the liver and iron transporter in the small intestine, indicating that XOS could improve the body's iron absorption capacity and promote the body's absorption of minerals. XOS could promote the proliferation of beneficial bacteria in the intestine; and the enzymes (such as phytase) produced by the beneficial bacteria promoted the dissociation of mineral ions in the intestine and improved the intestinal absorption rate of the mineral (169).
Application of XOS
Figure 6 showed the different application of XOS. In the food industry, XOS are commonly used as gelling agents, viscosity regulators, foam stabilizers, and tablet adhesives (170). In addition, the use of XOS as fat substitute in dairy products improves the elasticity and hardness of low-fat cheese and improves the storage stability of the cheese (171, 172). Except in the food industry, XOS are also widely used in medicine, agriculture and feed and other field because of their good physical and chemical properties.
Application of XOS in food
Application of XOS in baked food
XOS are widely used in baked foods because of their excellent acid and thermal stability. The moisture retention and water retention of XOS can change the rheological properties of dough and control the best moisture effect to change the taste and appearance of baked food. It was reported that the partial substitution of 5% XOS for sucrose would not change the physical and chemical properties of biscuits but would triple the content of crude fiber and increase the content of dietary fiber by 35%; and the biscuits had the functions of storage stability and prebiotic function (173). The addition of XOS increased the baking characteristics of biscuits, which increased caramel flavor, darker color, and more brittle texture (174). The addition of XOS also increased the sweetness and overall taste intensity of biscuits, indicating that XOS play a role in flavor enhancement in baked products. XOS have proved to be a promising new substitute which can increase the dietary fiber content of cereal biscuits.
Application of XOS in beverages
With the addition of XOS to orange juice, pomegranate juice, and whey beverage, the overall sensory acceptance generally improved (170, 175). The strawberry whey beverage with XOS showed an inhibitory effect on the enzymes controlling hypertension and diabetes in vitro. With the addition of XOS, the viscosity of the beverage increased, which was attributed to the substantial network formed by hydrogen bonding between XOS and protein and the strong water holding capacity of XOS (176).
Application of XOS in fodder
XOS could promote the improvement of food-specific characteristics and have been incorporated into animal feed to improve health (20).
In poultry feed, XOS could effectively increase the number of Lactobacillus in the ruminant intestine and improve animal digestibility. It was elucidated that adding XOS to grain could improve the feed conversion rate by adjusting the nutrient digestibility and ileal morphology of laying hens, which might be due to the increase of bacterial richness and the change of microbial composition, especially the enrichment of Lactobacillus and short-chain fatty acid-producing bacteria and the decrease of Bacteroides abundance (177). The addition of XOS to the diet had a positive effect on the growth performance, nutrient digestibility, and SCFA ratio of broilers attacked by coccidia (178). Ribeiro et al. studied the effect of XOS on the performance of broilers and reported that the dietary supplementation of XOS increased the nutritional value of the wheat diet, and the improvement of animal performance was accompanied by the transfer of microbial population settled in the upper gastrointestinal tract (179).
In terms of livestock feed, the addition of 100 g/t XOS in a grain diet could increase the height of jejunal villus, the abundance of Lactobacillus and Bifidobacterium, as well as the concentration of acetic acid and short-chain fatty acids, and could significantly improve the intestinal ecosystem of Weaned Piglets (180). The addition of XOS had a positive impact on the growth performance, nutrient digestibility, and the proportion of short-chain fatty acids of pigs (181). The supplement of 100 g/t XOS in the growth completion stage of pigs would increase the relative abundance of Lactobacillus and short-chain fatty acids and biogenic amines (135). Yin et al. elucidated that the addition of XOS to the diet significantly enhanced the α-diversityof the intestinal microbiota of weaned piglets (157). The addition of XOS increased the villus height: crypt depth ratio in jejunum of weanling piglets. The addition of XOS alone (200 mg/kg) could improve the apparent digestibility of dry matter, nitrogen, and total energy on the 14th day, improve trypsin activity and reduce fecal NH3 concentration. On day 14, taking XOS reduced the number of E. coli in feces and increased the number of Lactobacilli (182).
In terms of aquatic feed, XOS could promote the growth of aquatic animals, reduce the content of serum cholesterol and triglyceride, effectively control blood glucose level and enhance the immunity of aquatic animals. It was reported that after fed Caspian white fish with 3% XOS for 8 weeks, the antibacterial activity and total protein level of skin mucus was significantly improved. After taking XOS into the diet, the total number of intestinal heterotrophic bacteria and lactic acid bacteria increased significantly, which proved the beneficial effect of XOS on different skin mucosal immune parameters (183). Feeding juvenile triploid O. mykiss 5.0–10.0 g/kg XOS could increase the number of Lactobacillus by promoting intestinal development, limiting intestinal injury and inflammation, and regulating the structure of the intestinal microbial community (182). The high-fat diet supplemented with 1–3% XOS promoted the growth of carp fed with high-fat diet; and XOS could improve the growth, digestive enzymes, antioxidants, and immune response of carp fed with a high-fat diet (184). The appropriate level of XOS supplement could improve the growth performance of grass carp, increase the number of Lactobacillus and Bifidobacterium and the concentration of short-chain fatty acids, improve the growth performance of grass carp and prevent intestinal cell apoptosis (185). It was reported that added 5% XOS to the diet could significantly improve the growth performance, antioxidant capacity, innate immunity, and hydrophilic bacilli resistance of Megalobrama amblycephala (156). Adding XOS to feed European bass (Dicentrarchusla-brax) could significantly increase body weight and protein efficiency ratio and feed conversion rate, improve growth, stimulate immunity, and enhance anti-infection ability (186).
Application of XOS in medical treatment
The unique physiological activities of XOS makes them widely used in medicine for the treatment and prevention of a variety of diseases. In medical care, XOS can be an option in preventing cardiovascular, tumor, and endocrine diseases. XOS can be used as anti-tumor stabilizers, immune stimulants, antioxidants, and drugs. XOS can also be widely added to health care products as functional factors to assist in treating of some human diseases. Through clinical verification, XOS can be combined with traditional Chinese medicine extracts or added to the formula of Western medicine to replace some auxiliary materials such as starch and dextrin, which can strengthen the efficacy of drugs. In addition, XOS can also directly develop health products and enhance the body's physique. According to clinical trials, XOS play an important role in treating diabetes, hypertension, hyperlipidemia, chronic hepatitis, irritable bowel syndrome, chronic gastroenteritis, osteoporosis, pruritus, and otitis (187). Sheu and other studies reported that 8 weeks of XOS as a dietary supplement could effectively improve blood glucose and lipid levels in type 2 diabetes (147). It was found that XOS was beneficial to the reversible change of intestinal microflora in diabetic patients, such as decreasing the growth of Enterorhabdus, Howardella, SLackia, and so on (48). At the same time, XOS could also reduce the OGTT-2h (2 h oral glucose tolerance test) of prediabetic patients. It was reported that ID-HWS1000 composed of Lactobacillus and Bifidobacterium, XOS, and dietary fiber directly improved the discomfort related to defecation, reduced the proportion of vertebrates, increased the proportion of Bacteroides, improved the perception of intestinal activity in patients with functional constipation, and produced positive changes (188). XOS supplementation could also improve intestinal function, calcium absorption and lipid metabolism, as well as reduce cardiovascular disease, and colon cancer (23).
Application of XOS in agriculture
XOS were also known as plant growth regulators (189). XOS could also be used as fertilizers to improve soil activity and promote crop growth. When XOS were used as soil conditioner, the number of soil microorganisms and enzyme activity increased significantly, and the soil ecosystem was improved (190). In XOS treatment, the content of Brassinolide (BRS) increased significantly. Some researchers pointed out that BRS could induce the accumulation of zeatin nucleoside in plants to enhance photoprotection by accumulating many cold shock proteins and effectively prevent the accumulation of cold induced proteins (191). Finally, the resistance of plants to low temperature increased (192). Chen et al. reported the effect of XOS on improving salt tolerance of Chinese cabbage (193). XOS had a force to increase root biomass (increased by 69.5%), and the absorption of auxin also increased significantly (194).
Other applications of XOS
It was reported that adding 3% XOS to a snakehead ball could increase the elasticity of the fishball by 1.32 times without changing the hardness (195). XOS were excellent food additives and could be used as sucrose substitutes in the hydrostatic preparation of high protein meat products. Maillard reaction of soybean protein isolate and XOS could prepare new antioxidant wall materials (196). Soy isolate protein and oligosaccharide conjugate based on Maillard reaction showed excellent potential in microencapsulation of probiotics. Neves and other studies reported that the spray drying blue coloring agent using XOS has shown great potential in many foods as functional ingredients, replacing artificial blue coloring agents and combining the prebiotic characteristics (197). XOS could induce stomatal closure through the production of reactive oxygen species (ROS) and nitric oxide (NO) mediated by salicylic acid signal (198). In addition, in the cosmetics industry, the antioxidant, moisturizing, stabilizer, and emulsifier capabilities of XOS, as well as their ability to restore the microflora, making XOS very attractive (20). Brazil International Flavor Association reported that probiotics and prebiotics were one of the most important active ingredients in the cosmetics market, which could promote the balance of skin microbiota, improve skin resistance, replenish water and alleviate irritation. It was elucidated that XOS could avoid protein denaturation during frozen storage; shrimp soaked in XOS solution (3.0% w/v) had better water retention, stability of myofibrillar protein, and excellent texture characteristics (199). The mechanism of protein stability was described by the hydrogen bond between XOS and the polar residues of muscle protein and by limiting the fluidity of water to avoid the growth of ice crystals.
Conclusion
XOS are highly effective prebiotic nutritional oligosaccharudes, which can be produced by hydrolysis of hemicellulose, a rich component in agricultural residues rich in xylan. At present, the preparation of XOS mainly includes acid hydrolysis, autohydrolysis, and chemical enzyme synthesis. Although XOS can be produced by chemical hydrolysis; enzymatic hydrolysis has significant advantages because it usually does not produce byproducts, which is very important for the application of XOS. In the industrial environment, the need for biomass pre-treatment and the relatively low efficiency of subsequent enzymatic hydrolysis limit the yield of XOS. Therefore, the successful production of XOS requires strict and optimized conditions.
The existing methods optimize the preparation of XOS to a certain extent and improve the preparation efficiency. In recent years, the industrial application of xylan, a byproducts of agricultural products, has been greatly limited due to its high content of impurities, and the product quality is not easy to control. Therefore, the refining, separation, and purification of XOS have also become the key to their subsequent industrial application. At present, the development of XOS has not reached its peak, and as a new generation of functional sugars, XOS have not been fully used. These production optimizations have promoted the application and development of XOS and laid the primary theoretical foundation for large-scale popularization and use in the future.
The existing physiological activity studies of XOS are carried out in animals and can only speculate on the effect on human body according to the obtained data. The actual effects need further experimental verification. In the preparation process of XOS, the effects of different raw materials on the structure and physiological activity of XOS cannot be determined; further research is needed.
XOS have an auspicious future. With the continuous in-depth development and promotion of the health care industry, there are more and more customers' needs. The advantages of XOS are less addition, good stability, and high selectivity, which is in line with the general demand that capsules, tablets, and other dosage forms are easier to carry and take. These advantages are unmatched by other oligosaccharides. In addition, as relatively new feed additive, they also have excellent performance in bacteriostasis. XOS can further maintain the health and productivity of animals. With many countries have enacted laws, and more and more antibiotics are avoided outside the scope of feed additives, including the continuous improvement of various policies and the gradual diversification and innovation of industrial development, there will be a new opportunity for the sustainable development of the XOS industry.
Author contributions
FY: conceptualization, funding acquisition, and writing—original draft. ST: formal analysis, investigation, resources, and writing—review and editing. KD: investigation and resources. XX: investigation, methodology, and supervision. PG: investigation and methodology. ZC: investigation and supervision. All authors contributed to the article and approved the submitted version.
Funding
This research was supported by the Cultivation Programme for Young Backbone Teachers in Henan Province (2021GGJS059) and funded by Natural Science Innovation Fund Support Program from Henan University of Technology (2021ZKCJ12).
Conflict of interest
The authors declare that the research was conducted in the absence of any commercial or financial relationships that could be construed as a potential conflict of interest.
Publisher's note
All claims expressed in this article are solely those of the authors and do not necessarily represent those of their affiliated organizations, or those of the publisher, the editors and the reviewers. Any product that may be evaluated in this article, or claim that may be made by its manufacturer, is not guaranteed or endorsed by the publisher.
References
1. Chapla D, Pandit P, Shah A. Production of xylooligosaccharides from corncob xylan by fungal xylanase and their utilization by probiotics. Bioresour Technol. (2012) 115:215–21. doi: 10.1016/j.biortech.2011.10.083
2. Yang H, Wang K, Song X, Xu F. Production of xylooligosaccharides by xylanase from Pichia stipitis based on xylan preparation from triploid Populas tomentosa. Bioresour Technol. (2011) 102:7171–6. doi: 10.1016/j.biortech.2011.03.110
3. Bouxin F, Marinkovic S, Bras JL, Estrine B. Direct conversion of xylan into alkyl pentosides. Carbohydr Res. (2010) 345:2469–73. doi: 10.1016/j.carres.2010.09.003
4. Samanta AK, Senani S, Kolte AP, Sridhar M, Sampath K, Jayapal TN, et al. Production and in vitro evaluation of xylooligosaccharides generated from corn cobs. Food Bioprod Process. (2012) 90:466–74. doi: 10.1016/j.fbp.2011.11.001
5. Peng P, Peng F, Bian J, Xu F, Sun R. Studies on the starch and hemicelluloses fractionated by graded ethanol precipitation from bamboo Phyllostachys bambusoides f. shouzhu Yi. Food Chem. (2011) 59:2680–8. doi: 10.1021/jf1045766
6. Samanta AK, Jayapal N, Kolte AP, Senani S, Sridhar M, Suresh KP, et al. Enzymatic production of xylooligosaccharides from alkali solubilized xylan of natural grass (Sehima nervosum). Bioresour Technol. (2012) 112:199–205. doi: 10.1016/j.biortech.2012.02.036
7. Otieno DO, Ahring BK. The potential for oligosaccharide production from the hemicellulose fraction of biomasses through pretreatment processes: xylooligosaccharides (XOS), arabinooligosaccharides (AOS), mannooligosaccharides (MOS). Carbohydr Res. (2012) 360:84–92. doi: 10.1016/j.carres.2012.07.017
8. Akpinar O, Erdogan K, Bakir U, Yilmaz L. Comparison of acid and enzymatic hydrolysis of tobacco stalk xylan for preparation of xylooligosaccharides. LWT Food Sci Technol. (2010) 43:119–25. doi: 10.1016/j.lwt.2009.06.025
9. Ahmed S, Riaz S, Jamil A. Molecular cloning of fungal xylanases: an overview. Appl Microbiol Biotechnol. (2009) 84:19–35. doi: 10.1007/s00253-009-2079-4
10. Kay H, Carola F. Novel xylan gels prepared from oat spelts. Macromol Symp. (2010) 294:141–50. doi: 10.1002/masy.200900169
11. Ochs M, Muzard M, Plantier-Royon ERB, Remond C. Enzymatic synthesis of alkyl b-D-xylosides and oligoxylosides from xylans and from hydrothermally pretreated wheat bran. Green Chem. (2011) 13:2380–8. doi: 10.1039/c1gc15719a
12. Brienzo M, Carvalho W, Milagres AMF. Xylooligosaccharides production from alkali-pretreated sugarcane bagasse using xylanases from Thermoascus aurantiacus. Appl Biochem Biotechnol. (2010) 162:1195–205. doi: 10.1007/s12010-009-8892-5
13. Nabarlatz D, Torras C, Garcia-Valls R, Montané D. Xylooligosaccharides production from alkali-pretreated sugarcane bagasse using xylanases from thermoascus aurantiacus. Sep Purif Technol. (2007) 53:235–43. doi: 10.1016/j.seppur.2006.07.006
14. Ahring O. A thermochemical pretreatment process to produce xylooligosaccharides (XOS), arabinooligosaccharides (AOS) and mannooligosaccharides (MOS) from lignocellulosic biomasses. Bioresour Technol. (2012) 112:285–92.
15. Lin YS, Tseng MJ, Lee WC. Production of xylooligosaccharides using immobilized endo-xylanase of Bacillus halodurans. Process Biochem. (2011) 46:2117–21. doi: 10.1016/j.procbio.2011.08.008
16. Dekker R, Barbosa AM, Giese EC. Bioactive Oligosaccharides: Production, Biological Functions, and Potential Commercial Applications. Nova Science Publishers, Incorporated (2010).
17. Nabarlatz D, Montané D, Kardošová A, Bekešová S, Hríbalová V, Ebringerová A. Almond shell xylo-oligosaccharides exhibiting immunostimulatory. Carbohydr Res. (2007) 342:1122–8. doi: 10.1016/j.carres.2007.02.017
18. Zhou S, Liu X, Guo Y, Qiang W, Peng D, Cao L. Comparison of the immunological activities of arabinoxylans from wheat bran with alkali and xylanase-aided extraction. Carbohydr Polym. (2010) 81:784–9. doi: 10.1016/j.carbpol.2010.03.040
19. Swanson KS, Gibson GR, Hutkins RW. The International Scientific Association for Probiotics and Prebiotics (ISAPP) consensus statement on the definition and scope of synbiotics. Nature Reviews Gastroenterol Hepatol. (2020) 17: 1–15. doi: 10.1038/s41575-020-0344-2
20. Amorim C, Silvério SC, Prather KLJ, Rodrigues LR. From lignocellulosic residues to market: production and commercial potential of xylooligosaccharides. Biotechnol Adv. (2019) 37:107397. doi: 10.1016/j.biotechadv.2019.05.003
21. Akpinar O, Erdogan K, Bostanci S. Enzymatic production of xylooligosaccharide from selected agricultural wastes. Food Bioprod Process. (2009) 87:145–51. doi: 10.1016/j.fbp.2008.09.002
22. Wang J, Sun B, Cao Y, Wang C. In vitro fermentation of xylooligosaccharides from wheat bran insoluble dietary fiber by Bifidobacteria. Carbohydr Polym. (2010) 82:419–23. doi: 10.1016/j.carbpol.2010.04.082
23. Samanta AK, Jayapal N, Jayaram C, Roy S, Kolte AP, Senani S, et al. Xylooligosaccharides as prebiotics from agricultural byproducts: production and applications. Bioact Carbohydr Diet. (2015) 5:62–71. doi: 10.1016/j.bcdf.2014.12.003
24. Jayapal N, Samanta AK, Kolte AP, Kolte A, Senani S, Sridhar M, et al. Value addition to sugarcane bagasse: xylan extraction and its process optimization for xylooligosaccharides production. Ind Crops Prod. (2013) 42:14–24. doi: 10.1016/j.indcrop.2012.05.019
25. Terrasan C, Temer B, Duarte M, Carmona E. Production of xylanolytic enzymes by Penicillium janczewskii. Bioresour Technol. (2010) 101:4139–43. doi: 10.1016/j.biortech.2010.01.011
26. Miller PS, Blum PH. Extremophile inspired strategies for enzymatic biomass saccharification. Environ Technol. (2010) 31:1005–15. doi: 10.1080/09593330903536113
27. Almeida JRM, Runquist D, Nogué VS, Lidén G, Gorwa-Grauslund MF. Stress-related challenges in pentose fermentation to ethanol by the yeast Saccharomyces cerevisiae. Biotechnol J. (2011) 6:286–99. doi: 10.1002/biot.201000301
28. Australian Industry Development Corporation Inc. PreticX-Optimize Your Flora. Australian Industry Development Corporation Inc (2017). Availabler online at: http://storefronts.supplysideshow.com/~/media/Files/Storefronts/
29. Courtin CM, Swennen K, Verjans P, Delcour JA. Heat and pH stability of prebiotic arabinoxylooligosaccharides, xylooligosaccharides and fructooligosaccharides. Food Chem. (2009) 112:831–7. doi: 10.1016/j.foodchem.2008.06.039
30. Aachary AA, Prapulla SG. Xylooligosaccharides (XOS) as an emerging prebiotic: microbial synthesis, utilization, structural characterization, bioactive properties, and applications. Rev Food Sci Food Saf. (2011) 10:2–16. doi: 10.1111/j.1541-4337.2010.00135.x
31. Ahmad R. Better health with plant oligosaccharides: trends and future. In: Proceeding of the 1st International Conference on Food and Agriculture (2019).
32. Markets and Markets™. Prebiotic Ingredients Market Worth 7.37 Billion USD by 2023. Markets and Markets™ (2018). Available online at: https://www.prnewswire.com/news-releases/prebiotic-ingredients-market-worth-737-billion-usd-by-2023-670471503
33. The Market Watch. Prebiotics Market Annual Consumption Will Cross 1.4 Million Tonsby 2024. The Market Watch (2018). Available online at: https://www.marketwatch.com/press-release/prebiotics-market-annual-consumption-will-cross-14-million-tons-by-2024-2018-09-10
34. Capetti CCDM, Vacilotto MM, Dabul ANG, Sepulchro AGV, Pellegrini VOA, Polikarpov I. Recent advances in the enzymatic production and applications of xylooligosaccharides. J Microbiol Biotechnol. (2021) 37:1–12. doi: 10.1007/s11274-021-03139-7
35. Perlack RD. Biomass as Feedstock for a Bioenergy and Bioproducts Industry: The Technical Feasibility of a Billion-Ton Annual Supply. Oak Ridge National Laboratory (2005). doi: 10.2172/885984
36. Otieno DO, Ahring BK. A thermochemical pretreatment process to produce xylooligosaccharides (XOS), arabinooligosaccharides (AOS) and mannooligosaccharides (MOS) from lignocellulosic biomasses. Bioresour Technol. (2012) 112:285–92. doi: 10.1016/j.biortech.2012.01.162
37. Poletto P, Pereira GN, Monteiro CRM, Pereira MAF, Oliveira DD. Xylooligosaccharides: transforming the lignocellulosic biomasses into valuable 5-carbon sugar prebiotics. Process Biochem. (2020) 91:352–63. doi: 10.1016/j.procbio.2020.01.005
38. Singh RD, Gracy C, Muir J, Arora A. Green and clean process to obtain low degree of polymerisation xylooligosaccharides from almond shell. J Clean Prod. (2019) 241:118237. doi: 10.1016/j.jclepro.2019.118237
39. Huang X, Ouyang X, Hendriks BMS, Gonzalez OMM, Zhu J, IKorányi T, et al. Selective production of mono-aromatics from lignocellulose over Pd/C catalyst: the influence of acid co-catalysts. Faraday Discuss. (2017) 202:141–56. doi: 10.1039/C7FD00039A
40. Santibáñez L, Henríquez C, Corro-Tejeda R, Bernal S, Armijo B, Salazar O. Xylooligosaccharides from lignocellulosic biomass: a comprehensive review. Carbohydr Polym. (2021) 251:117118. doi: 10.1016/j.carbpol.2020.117118
41. Jnawali P, Kumar V, Tanwar B, Hirdyani H, Gupta P. Enzymatic production of xylooligosaccharides from brown coconut husk treated with sodium hydroxide. Waste Biomass Valorization. (2018) 9:1757–66. doi: 10.1007/s12649-017-9963-4
42. Gullón B, Eibes G, Dávila I, Moreira MT, Labidi J, Gullón P. Hydrothermal treatment of chestnut shells (Castanea sativa) to produce oligosaccharides and antioxidant compounds. Carbohydr Polym. (2018) 192:75–83. doi: 10.1016/j.carbpol.2018.03.051
43. Surek E, Buyukkileci AO. Production of xylooligosaccharides by autohydrolysis of hazelnut (Corylus avellana L.) shell. Carbohydr Polym. (2017) 174:565–71. doi: 10.1016/j.carbpol.2017.06.109
44. Miranda I, Simões R, Medeiros B, Nampoothiri KM, Sukumaran RK, Rajan D, et al. Valorization of lignocellulosic residues from the olive oil industry by pro-duction of lignin, glucose and functional sugars. Bioresour Technol. (2019) 292:121936. doi: 10.1016/j.biortech.2019.121936
45. Banerjee S, Patti AF, Ranganathan V, Arora A. Hemicellulose based biorefinery from pineapple peel waste : xylan extraction and its conversion into xylooligosaccharides. Food Bioprod Process. (2019) 117:38–50. doi: 10.1016/j.fbp.2019.06.012
46. Rico X, Gullón B, Alonso JL, Parajó JC, Yáñez R. Valorization of peanut shells :manufacture of bioactive oligosaccharides. Carbohydr Polym. (2018) 183:21–8. doi: 10.1016/j.carbpol.2017.11.009
47. Freitas CD, Carmona E, Brienzo M. Xylooligosaccharides production process from lignocellulosic biomass and bioactive effects. Bioact Carbohydr Diet Fibre. (2019) 18:100184. doi: 10.1016/j.bcdf.2019.100184
48. Yang J, Summanen PH, Henning SM, Mark H, Heiman L, Huang J, et al. Xylooligosaccharide supplementation alters gut bacteria in both healthy and prediabetic adults: a pilot study. Front Physiol. (2015) 6:216. doi: 10.3389/fphys.2015.00216
49. Biely P. Microbial xylanolytic systems. Trends Biotechnol. (1985) 3:286–90. doi: 10.1016/0167-7799(85)90004-6
50. Wen P, Zhang T, Wei L, Wang J, Zhang J. Effect of dilute acetic acid hydrolysis on xylooligosaccharide production and the inhibitory effect of cellulolytic enzyme lignin from poplar. ACS Sustainable Chem Eng. (2021) 9:11361–71. doi: 10.1021/acssuschemeng.1c02937
51. Ying W, Xu Y, Zhang J. Effect of sulfuric acid on production of xylooligosaccharides and monosaccharides from hydrogen peroxide-acetic acid-pretreated poplar. Bioresour Technol. (2021) 321:124472. doi: 10.1016/j.biortech.2020.124472
52. Liao H, Xu Y, Zhang J. Efficient production of xylooligosaccharides and fermentable sugars from corncob by propionic acid and enzymatic hydrolysis. Bioresour Technol. (2021) 342:125680. doi: 10.1016/j.biortech.2021.125680
53. Bedo S, Rozbach M, Nagy L, Fehér A, Fehér C. Optimised fractionation of brewer's spent grain for a biorefinery producing sugars, oligosaccharides, and bioethanol. Processes. (2021) 9:366. doi: 10.3390/pr9020366
54. Wang J, Xu Y, Meng X, Pu Y, Ragauskas A, Zhang J. Production of xylo-oligosaccharides from poplar by acetic acid pretreatment and its impact on inhibitory effect of poplar lignin. Bioresour Technol. (2021) 323:124593. doi: 10.1016/j.biortech.2020.124593
55. Qing Q, Li H, Kumar R, Wyman CE. Xylooligosaccharides production, quantification, and characterization in context of lignocellulosic biomass pretreatment, aqueous pretreatment. Plant Biomass Biol Chem Conver Fuels Chem. (2013) 19:391–415. doi: 10.1002/9780470975831.ch19
56. Vázquez MJ, Alonso JL, Dominguez H, Parajó JC. Xylooligosaccharides: manufacture and applications. Trends Food Sci Technol. (2000) 11:387–93. doi: 10.1016/S0924-2244(01)00031-0
57. Huang C, Wang X, Liang C, Jiang X, Yong Q. A sustainable process for procuring biologically active fractions of high-purity xylooligosaccharides and water-soluble lignin from moso bamboo prehydrolyzate. Biotechnol Biofuels. (2019) 12:1–13. doi: 10.1186/s13068-019-1527-3
58. Ruiz HA, Rodríguez-Jasso RM, Fernandes BD, Vicente AA, Teixeira JA. Hydrothermal processing, as an alternative for upgrading agriculture residues and marine biomass according to the biorefinery concept: a review. Renew Sust Energ Rev. (2013) 21:35–51. doi: 10.1016/j.rser.2012.11.069
59. Garrote G, Domi'nguez H, Parajó JC. Autohydrolysis of corncob: study of non-isothermal operation for xylooligosaccharide production. J Food Eng. (2002) 52:211–8. doi: 10.1016/S0260-8774(01)00108-X
60. Moure A, Gullón P, Domínguez H, Parajó JC. Advances in the manufacture, purification and applications of xylo-oligosaccharides as food additives and nutraceuticals. Process Biochem. (2006) 41:1913–23. doi: 10.1016/j.procbio.2006.05.011
61. Wang ZK, Huang C, Zhong JL, Wang Y, Tang L, Li B, et al. Valorization of Chinese hickory shell as novel sources for the efficient production of xylooligosaccharides. Biotechnol Biofuels. (2021) 14:1–13. doi: 10.1186/s13068-021-02076-9
62. Swart LJ, Metersen PA, Bedzo O, Bedzo OKK, Grgens JF. Techno?conomic analysis of the valorisation of brewers spent grains: production of xylitol and xylo, ligosaccharides. J Chem. Technol Biotechnol. (2021) 96:1632–44. doi: 10.1002/jctb.6683
63. Ghosh D, Tanner J, Lavoie JM, Gil G, Antonio AF. An integrated approach for hemicellulose extraction from forest residue. BioResources. (2021) 16:2524–47. doi: 10.15376/biores.16.2.2524-2547
64. Pablo G, Domínguez VD, Domínguez E, Gullón P, Gullón B, Garrote G, et al. Comparative study of biorefinery processes for the valorization of fast-growing paulownia wood. Bioresour Technol. (2020) 314:123722. doi: 10.1016/j.biortech.2020.123722
65. Romaní A, Tomaz PD, Garrote G, Teixeira JA, Domingues L. Combined alkali and hydrothermal pretreatments for oat straw valorization within a biorefinery concept. Bioresour Technol. (2016) 220:323–32. doi: 10.1016/j.biortech.2016.08.077
66. Pablo G, Gomes-Dias JS, Rocha CMR, Romaní A, Garrote G, Domingues L. Recent trends on seaweed fractionation for liquid biofuels production. Bioresour Technol. (2020) 299:122613. doi: 10.1016/j.biortech.2019.122613
67. Domínguez E, Nóvoa T, Pablo G, Garrote G. Sequential two-stage autohydrolysis biorefinery for the production of bioethanol from fast-growing paulownia biomass. Energy Convers Manage. (2020) 226:113517. doi: 10.1016/j.enconman.2020.113517
68. Fujimoto S, Inoue S, Yoshida M. High solid concentrations during the hydrothermal pretreatment of eucalyptus accelerate hemicellulose decomposition and subsequent enzymatic glucose production. Bioresour Technol Rep. (2018) 4:16–20. doi: 10.1016/j.biteb.2018.09.006
69. Vargas F, Domínguez E, Vila C, Rodríguez A, Garrote G. Biorefinery scheme for residual biomass using autohydrolysis and organosolv stages for oligomers and bioethanol production. Energy Fuels. (2016) 30:8236–45. doi: 10.1021/acs.energyfuels.6b00277
70. Naidu DS, Hlangothi SP, John MJ. Bio-based products from xylan: a review. Carbohydr. Polym. (2018) 179:28–41. doi: 10.1016/j.carbpol.2017.09.064
71. Gallina G, Cabeza Á, Grénman H, Biasi P, García-Serna J, Salmi T. Hemicellulose extraction by hot pressurized water pretreatment at 160 °C for 10 different woods: yield and molecular weight. J Supercrit Fluids. (2018) 133:716–25. doi: 10.1016/j.supflu.2017.10.001
72. Álvarez C, González A, Negro MJ, Ballesteros I, Oliva JM, Sáez F. Optimized use of hemicellulose within a biorefinery for processing high value-added xylooligosaccharides. Ind Crops Prod. (2017) 99:41–8. doi: 10.1016/j.indcrop.2017.01.034
73. Dávila I, Gordobil O, Labidi J, Gullón P. Assessment of suitability of vine shoots for hemicellulosic oligosaccharides production through aqueous processing. Bioresour Technol. (2016) 211:636–44. doi: 10.1016/j.biortech.2016.03.153
74. Cocero MJ, Cabeza A, Abad N, Adamovic T, Vaquerizo L, Martínez CM, et al. Understanding biomass fractionation in subcritical & supercritical water. J Supercrit Fluids. (2018) 133:550–65. doi: 10.1016/j.supflu.2017.08.012
75. Chen MH, Bowman MJ, Dien BS, Rausch KD, Tumbleson ME, Singh V. Autohydrolysis of miscanthus x giganteus for the production of xylooligosaccharides (XOS): kinetics, characterization and recovery. Bioresour Technol. (2014) 155:359–65. doi: 10.1016/j.biortech.2013.12.050
76. Hong C, Corbett D, Venditti R, Jameel H, Park S. Xylooligosaccharides as prebiotics from biomass autohydrolyzate. LWT Food Sci Technol. (2019) 111:703–10. doi: 10.1016/j.lwt.2019.05.098
77. Akpinar O, Ak O, Kavas A, Bakir U, Yilmaz L. Enzymatic production of xylooligosaccharides from cotton stalks. Food Chem. (2007) 55:5544–51. doi: 10.1021/jf063580d
78. Karlsson EN, Schmitz E, Linares-Pastén JA, Adlercreutz P. Endo-xylanases as tools for production of substituted xylooligosaccharides with prebiotic properties. Appl Microbiol Biotechnol. (2018) 102:9081–8. doi: 10.1007/s00253-018-9343-4
79. Teng C, Yan Q, Jiang Z, Fan G, Bo S. Production of xylooligosaccharides from the steam explosion liquor of corncobs coupled with enzymatic hydrolysis using a thermostable xylanase. Bioresour Technol. (2010) 101:7679–82. doi: 10.1016/j.biortech.2010.05.004
80. Álvarez C, González A, Alonso JL, Sáeza F, Negro MJ, Gullón B. Xylooligosaccharides from steam-exploded barley straw: structural features and assessment of bifidogenic properties. Food Bioprod Process. (2020) 124:131–42. doi: 10.1016/j.fbp.2020.08.014
81. Khat-udomkiri N, Sivamaruthi BS, Sirilun S, Narissara L, Sartjin P, Chaiyavat C. Optimization of alkaline pretreatment and enzymatic hydrolysis for the extraction of xylooligosaccharide from rice husk. AMB Express. (2018) 8:115. doi: 10.1186/s13568-018-0645-9
82. Carvalho AFA, Marcondes WF, Pedro DON, Pastore GM, Saddler JN, Arantes V. The potential of tailoring the conditions of steam explosion to produce xylo-oligosaccharides from sugarcane bagasse. Bioresour Technol. (2018) 250:221–9. doi: 10.1016/j.biortech.2017.11.041
83. Buruiana CT, Gómez B, Vizireanu C, Garrote G. Manufacture and evaluation of xylooligosaccharides from corn stover as emerging prebiotic candidates for human health. LWT Food Sci Technol. (2017) 77:449–59. doi: 10.1016/j.lwt.2016.11.083
84. Kim Y, Hendrickson R, Hosier N, Ladisch MR. Plug-flow reactor for continuous hydrolysis of glucans and xylans from pretreated corn fiber. Energy Fuels. (2005) 19:2189–200. doi: 10.1021/ef050106l
85. Yang W, Ajapur VK, Krishnamurthy K, Feng H, Yang R, Rababah TM. Expedited extraction of xylan from corncob by power ultrasound. Int J Agric Biol Eng. (2009) 2:76–83. Available online at: http://www.ijabe.org
86. Immerzeel P, Falck P, Galbe M, Adlercreutz P, Karlsson EN. Extraction of water-soluble xylan from wheat bran and utilization of enzymatically produced xylooligosaccharides by Lactobacillus, Bifidobacterium and Weissella spp. LWT Food Sci Technol. (2014) 56:321–7. doi: 10.1016/j.lwt.2013.12.013
87. Zhang H, Xu Y, Yu S. Co-production of functional xylooligosaccharides and fermentable sugars from corncob with effective acetic acid prehydrolysis. Bioresour Technol. (2017) 234:343–9. doi: 10.1016/j.biortech.2017.02.094
88. Oliveira EE, Silva AE, Júnior TN, Gomes MCS, Aguiar LM, Marcelino HR, et al. Xylan from corn cobs, a promising polymer for drug delivery: production and characterization. Bioresour Technol. (2010) 101:5402–6. doi: 10.1016/j.biortech.2010.01.137
89. Cai W, Chen Q, Xie L, Yang L, Zhang R. Extraction, sulfonation and anticoagulant activity of xylan from corncob. Eur Food Res Technol. (2015) 240:969–75. doi: 10.1007/s00217-014-2401-y
90. Ruzene DS, Silva DP, Vicente AA, Gonalves AR, Teixeira JA. An alternative application to the Portuguese agro-industrial residue: wheat straw. Appl Biochem Biotechno. (2007) 147:453–64. doi: 10.1007/978-1-60327-526-2_43
91. Egüés I, Sanchez C, Mondragon II, Labidi J. Effect of alkaline and autohydrolysis processes on the purity of obtained hemicelluloses from corn stalks. Bioresour Technol. (2012) 103:239–48. doi: 10.1016/j.biortech.2011.09.139
92. Samanta AK, Kolte AP, Elangovan AV, Dhali A, Roy S. Value addition of corn husks through enzymatic production of xylooligosaccharides. Braz Arch Biol Technol. (2016) 59. doi: 10.1590/1678-4324-2016160078
93. Kawee-ai A, Srisuwun A, Tantiwa N, Nontaman W, Boonchuay P, Kuntiy A, et al. Eco-friendly processing in enzymatic xylooligosaccharides production from corncob: influence of pretreatment with sonocatalytic–synergistic fenton reaction and its antioxidant potentials. Ultrason Sonochem. (2016) 31:184–92. doi: 10.1016/j.ultsonch.2015.12.018
94. Kumar R, Wyman CE. The impact of dilute sulfuric acid on the selectivity of xylooligomer depolymerization to monomers, the impact of dilute sulfuric acid on the selectivity of xylooligomer depolymerization to monomers. Carbohydr Res. (2008) 343:290–300. doi: 10.1016/j.carres.2007.10.022
95. Yang R, Xu S, Wang Z, Wang Y. Aqueous extraction of corncob xylan and production of xylooligosaccharides. LWT Food Sci Technol. (2005) 38:677–82. doi: 10.1016/j.lwt.2004.07.023
96. Mano M, Neri-Numa IA, Silva J, Paulino BN, Pessoa MG, Pastore GM. Oligosaccharide biotechnology: an approach of prebiotic revolution on the industry. Appl Biochem Biotechnol. (2018) 102:17–37. doi: 10.1007/s00253-017-8564-2
97. Yadav SK. Technological advances and applications of hydrolytic enzymes for valorization of lignocellulosic biomass. Bioresour Technol. (2017) 245:1727–39. doi: 10.1016/j.biortech.2017.05.066
98. Mussatto SI, Teixeira JA. Lignocellulose as Raw Material in Fermentation Processes. (2010). Available online at: http://hdl.handle.net/1822/16762
99. Li Z, Summanen PH, Komoriya TM. In vitro study of the prebiotic xylooligosaccharide (XOS) on the growth of Bifidobacterium spp and Lactobacillus spp. Int J Food Sci Nutr. (2015) 66:919–22. doi: 10.3109/09637486.2015.1064869
100. Abdella A, Ramadan S, Hamouda RA, Saddiq AA, Alhazmi NM, Al-Saman MA. Paecilomyces variotii xylanase production, purification and characterization with antioxidant xylo-oligosaccharides production. Sci Rep. (2021) 11:16468. doi: 10.1038/s41598-021-95965-w
101. Rathamat Z, Choorit W, Chisti Y, Prasertsan P. Two-step isolation of hemicellulose from oil palm empty fruit bunch fibers and its use in production of xylooligosaccharide prebiotic. Ind Crops Prod. (2021) 160:113124. doi: 10.1016/j.indcrop.2020.113124
102. Chaiyaso T, Boonchuay P, Takenaka S, Techapun C, Watanabe M. Efficient enzymatic process for mulberry paper production: an approach for xylooligosaccharide production coupled with minimizing bleaching agent doses. Waste Biomass Valorization. (2021) 12:5347–60. doi: 10.1007/s12649-021-01416-y
103. Su Y, Fang L, Wang P, Lai C, Huang C, Ling Z, et al. Efficient production of xylooligosaccharides rich in xylobiose and xylotriose from poplar by hydrothermal pretreatment coupled with post-enzymatic hydrolysis. Bioresour Technol. (2021) 342:125955. doi: 10.1016/j.biortech.2021.125955
104. Majumdar S, Bhattacharyya DK, Bhowal J. Evaluation of nutraceutical application of xylooligosaccharide enzymatically produced from cauliflower stalk for its value addition through a sustainable approach. Food Funct. (2021) 12:5501–23. doi: 10.1039/D0FO03120H
105. Seekram P, Thammasittirong A, Thammasittirong NR. Evaluation of spent mushroom substrate after cultivation of Pleurotus ostreatus as a new raw material for xylooligosaccharides production using crude xylanases from Aspergillus flavus KUB2. 3 Biotech. (2021) 11:1–9. doi: 10.1007/s13205-021-02725-8
106. Gufe C, Ngenyoung A, Rattanarojpong T, Khunra P. Investigation into the effects of CbXyn10C and Xyn11A on xylooligosaccharide profiles produced from sugarcane bagasse and rice straw and their impact on probiotic growth. Bioresour Technol. (2022) 344:126319. doi: 10.1016/j.biortech.2021.126319
107. Fujii Y, Kobayashi M, Miyabe Y, Kishimura H, Hatanaka T, Kumagai Y. Preparation of β(1→ 3)/β(1→ 4)-xylooligosaccharides from red alga dulse by two xylanases from Streptomyces thermogriseus. Bioresour Biopro. (2021) 8:1–10. doi: 10.1186/s40643-021-00390-6
108. Surek E, Buyukkileci AO, Yegin S. Processing of hazelnut (Corylus avellana L.) shell autohydrolysis liquor for production of low molecular weight xylooligosaccharides by Aureobasidium pullulans NRRL Y−2311–1 xylanase. Ind Crops Prod. (2021) 161:113212. doi: 10.1016/j.indcrop.2020.113212
109. Cristina L, Alberto G, Ignacio B, Negro MJ. Production of xylooligosaccharides, bioethanol, and lignin from structural components of barley straw pretreated with a steam explosion. Bioresour Technol. (2021) 342:125953. doi: 10.1016/j.biortech.2021.125953
110. Jaichakan P, Nakphaichit M, Rungchang S, Weerawatanakorn M, Phongthai S, Klangpetch W. Two-stage processing for xylooligosaccharide recovery from rice by-products and evaluation of products: promotion of lactic acid-producing bacterial growth and food application in a high-pressure process. Food Res Int. (2021) 147:110529. doi: 10.1016/j.foodres.2021.110529
111. Gautério GV, Hübner T, Ribeiro TDR, Ziotti APM, Kalil SJ. Xylooligosaccharide production with low xylose release using crude xylanase fromaureobasidium pullulans: effect of the enzymatic hydrolysis parameters. Appl Biochem Biotechnol. (2021) 194:862–81. doi: 10.1007/s12010-021-03658-x
112. Forsan CF, Freitas CD, Masarin F, Brienzo M. Xylooligosaccharide production from sugarcane bagasse and leaf using aspergillus versicolor endoxylanase and diluted acid. Biomass Convers Biorefin. (2021) 1–16. doi: 10.1007/s13399-021-01403-2
113. Valladares-Diestra KK, Vandenberghe L, Soccol CR. A biorefinery approach for enzymatic complex production for the synthesis of xylooligosaccharides from sugarcane bagasse. Bioresour Technol. (2021) 333:125174. doi: 10.1016/j.biortech.2021.125174
114. Álvarez-Cervantes J. Mycosphere essay 10: properties and characteristics of microbial xylanases. Mycosphere. (2016) 7:1600–19. doi: 10.5943/mycosphere/si/3b/12
115. Azzouz Z, Bettache A, Boucherba N, Eugenio LD. Optimization of β-1,4-endoxylanase production by an Aspergillus niger. Strain growing on wheat straw and application in xylooligosaccharides production. Molecules. (2020) 26:2527. doi: 10.3390/molecules26092527
116. Gullón P, Moura P, Esteves MP, Girio FM, Domínguez H, Parajó JC. Assessment on the fermentability of xylooligosaccharides from rice husks by probiotic bacteria. J Agric Food Chem. (2008) 56:7482–7. doi: 10.1021/jf800715b
117. Illanes A, Guerrero C, Vera C, Wilson L, Conejeros R, Scott F. Lactose-Derived Prebiotics: A Process Perspective. Academic Press (2016).
118. Zhu Y, Kim TH, Lee YY, Chen R, Elander RT. Enzymatic production of xylooligosaccharides from corn stover and corn cobs treated with aqueous ammonia. Appl Biochem Biotechnol. (2006) 130:586–98. doi: 10.1385/ABAB:130:1:586
119. Montané D, Nabarlatz D, Martorell A, Torné-Fernández V, Fierro V. Removal of lignin and associated impurities from xylo-oligosaccharides by activated carbon adsorption. Ind Eng Chem Res. (2006) 45:2294–302. doi: 10.1021/ie051051d
120. Chen MH, Bowman MJ, Cotta MA, Dien BS, Iten LB, Whitehead TR, et al. Miscanthus× giganteus xylooligosaccharides: purification and fermentation. Carbohydr Polym. (2016) 140:96–103. doi: 10.1016/j.carbpol.2015.12.052
121. Gullón P, Gullón B, Moure A, Alonso JL, Parajó JC. Manufacture of prebiotics from biomass sources. In: Prebiotics and Probiotics Science and Technology. (2009). p. 535–89. doi: 10.1007/978-0-387-79058-9_14
122. Vegas R, Alonso JL, Domínguez H, Parajó JC. Manufacture and refining of oligosaccharides from industrial solid wastes. Ind Eng Chem Res. (2005) 44:614–20. doi: 10.1021/ie049289+
123. Swennen K, Courtin CM, Bruggen BV, Vandecasteele C, Delcour JA. Ultrafiltration and ethanol precipitation for isolation of arabinoxylooligosaccharides with different structures. Carbohydr Polym. (2005) 62:283–92. doi: 10.1016/j.carbpol.2005.08.001
124. Sun HJ, Yoshida S, Park NH, Kusakabe I. Preparation of (1→ 4)-β-D-xylooligosaccharides from an acid hydrolysate of cotton-seed xylan: suitability of cotton-seed xylan as a starting material for the preparation of (1→ 4)-β-D-xylooligosaccharides. Carbohydr Res. (2002) 337:657–61. doi: 10.1016/S0008-6215(02)00031-9
125. Jacobs A, Palm M, Zacchi G, Dahlman O. Isolation and characterization of water-soluble hemicelluloses from flax shive. Carbohydr Res. (2003) 338:1869–76. doi: 10.1016/S0008-6215(03)00308-2
126. Marchal L, Legrand J, Foucault A. Centrifugal partition chromatography: a survey of its history, and our recent advances in the field. Chem Rec. (2003) 3:133–43. doi: 10.1002/tcr.10057
127. Lau CS, Bunnell KA, Clausen EC, Thoma GJ, Lay JO, Gidden J, et al. Separation and purification of xylose oligomers using centrifugal partition chromatography. Ind Microbiol Biotechnol. (2011) 38:363–70. doi: 10.1007/s10295-010-0799-1
128. Ho AL, Carvalheiro F, Duarte LC, Roseiro LB, Charalampopoulos D, Rastall RA. Production and purification of xylooligosaccharides from oil palm empty fruit bunch fibre by a non-isothermal process. Bioresour Technol. (2014) 152:526–9. doi: 10.1016/j.biortech.2013.10.114
129. Pinelo M, Jonsson G, Meyer AS. Membrane technology for purification of enzymatically produced oligosaccharides: molecular and operational features affecting performance. Sep Purif Technol. (2009) 70:1–11. doi: 10.1016/j.seppur.2009.08.010
130. Cano À, Palet C. Xylooligosaccharide recovery from agricultural biomass waste treatment with enzymatic polymeric membranes and characterization of products with MALDI-TOF-MS. J Memb Sci. (2007) 291:96–105. doi: 10.1016/j.memsci.2006.12.048
131. Yuan QP, Zhang H, Qian ZM, Yang XJ. Pilot-plant production of xylo-oligosaccharides from corncob by steaming, enzymatic hydrolysis and nanofiltration. J Chem Technol Biotechnol. (2004) 79:1073–9. doi: 10.1002/jctb.1071
132. Vegas R, Luque S, Alvarez JR, Alonso JL, Domínguez H, Parajó JC. Membrane-Assisted processing of xylooligosaccharide-containing liquors. J Agric Food Chem. (2006) 54:5430–6. doi: 10.1021/jf060525w
133. Slizewska K, Kapuśniak J, Barczyńska R, Jochym K. Riesistant dextrins as prebiotic. In: Carbohydrates–Comprehensive Studies on Glycobiology and Glycotechnology. (2012). p. 261. doi: 10.5772/51573
134. Chen MH, Swanson KS, Fahey GCJ, Dien BS, Beloshapka AN, Bauer LL, et al. In vitro fermentation of xylooligosaccharides produced from Miscanthus× giganteus by human fecal microbiota. J Agric Food Chem. (2016) 64:262–7. doi: 10.1021/acs.jafc.5b04618
135. Pan J, Yin J, Zhan K, Xie P, Ding H, Huang X, et al. Dietary xylo-oligosaccharide supplementation alters gut microbial composition and activity in pigs according to age and dose. Amb Express. (2019) 9:1–10. doi: 10.1186/s13568-019-0858-6
136. Maki KC, Gibson GR, Dickmann RS, Kendall CWCC, Chen YO, Costabile A, et al. Digestive and physiologic effects of a wheat bran extract, arabino-xylan-oligosaccharide in breakfast cereal. Nutrition. (2012) 28:1115–21. doi: 10.1016/j.nut.2012.02.010
137. Nieto-Domínguez M, Eugenio LI, York-Durán MJ, Rodríguez-Colinas B, Plou FJ, Chenoll E, et al. Prebiotic effect of xylooligosaccharides produced from birchwood xylan by a novel fungal GH11 xylanase. Food Chem. (2017) 232:105–13. doi: 10.1016/j.foodchem.2017.03.149
138. Hald S, Schioldan AG, Moore ME, Dige A, Lærke HN, Agnholt J, et al. Effects of arabinoxylan and resistant starch on intestinal microbiota and short-chain fatty acids in subjects with metabolic syndrome: a randomised crossover study. PLoS ONE. (2016) 11:e0159223. doi: 10.1371/journal.pone.0159223
139. Roberfroid M, Gibson GR, Hoyles L, Mccartney AL, Meheust A. Prebiotic effects: metabolic and health benefits. Br J Nutr. (2010) 104:1–63. doi: 10.1017/S0007114510003363
140. Grootaert C, Abbeele PV, Marzorati M, Broekaert WF, Courtin CM, Delcour JA, et al. Comparison of prebiotic effects of arabinoxylan oligosaccharides and inulin in a simulator of the human intestinal microbial ecosystem. FEMS Microbiol Ecol. (2009) 69:231–42. doi: 10.1111/j.1574-6941.2009.00712.x
141. Geraylou Z, Souffreau C, Rurangwa E, Meester LD, Courtin CM, Delcour JA, et al. Effects of dietary arabinoxylan-oligosaccharides (AXOS) and endogenous probiotics on the growth performance, non-specific immunity and gut microbiota of juvenile Siberian sturgeon (Acipenserábaerii). Fish Shellfish Immunol. (2013) 35:766–75. doi: 10.1016/j.fsi.2013.06.014
142. Cheng L, Zhang X, Hong Y, Li Z, Li C, Gu Z. Characterisation of physicochemical and functional properties of soluble dietary fibre from potato pulp obtained by enzyme-assisted extraction. Int J Biol Macromol. (2017) 101:1004–11. doi: 10.1016/j.ijbiomac.2017.03.156
143. Soleimani A, Taghizadeh M, Bahmani F, Badroj N, Asemi Z. Metabolic response to omega-3 fatty acid supplementation in patients with diabetic nephropathy: a randomized, double-blind, placebo-controlled trial. Clin Nutr. (2017) 36:79–84. doi: 10.1016/j.clnu.2015.11.003
144. Haenen D, Silva CS, Zhang J, Koopmans SJ, Bosch G, Vervoort J, et al. Resistant starch induces catabolic but suppresses immune and cell division pathways and changes the microbiome in the proximal colon of male pigs. Nutrition. (2013) 143:1889–98. doi: 10.3945/jn.113.182154
145. Gibson GR, Scott KP, Rastall RA, Tuohy KM, Hotchkiss A, Dubert-Ferrandon A, et al. Dietary prebiotics: current status and new definition. Food Sci Technol Bull Funct Foods. (2010) 7:1–19. doi: 10.1616/1476-2137.15880
146. Wang J, Cao Y, Wang C, Sun B. Wheat bran xylooligosaccharides improve blood lipid metabolism and antioxidant status in rats fed a high-fat diet. Carbohydr Polym. (2011) 86:1192–7. doi: 10.1016/j.carbpol.2011.06.014
147. Sheu WHH, Lee IT, Chen W, Chan YC. Effects of xylooligosaccharides in type 2 diabetes mellitus. J Nutr Sci Vitaminol. (2008) 54:396–401. doi: 10.3177/jnsv.54.396
148. Broekaert WF, Courtin CM, Verbeke K, Tom V, Verstraete W, Delcour JA. Prebiotic and other health-related effects of cereal-derived arabinoxylans, arabinoxylan-oligosaccharides, and xylooligosaccharides. Crit Rev Food Sci Nutr. (2011) 51:178–94. doi: 10.1080/10408390903044768
149. Strader AD, Woods SC. Gastrointestinal hormones and food intake. Gastroenterology. (2005) 128:175–91. doi: 10.1053/j.gastro.2004.10.043
150. Delzenne NM, Cani PD, Neyrinck AMJ. Modulation of glucagon-like peptide 1 and energy metabolism by inulin and oligofructose: experimental data. Nutrition. (2007) 137:2547–51. doi: 10.1093/jn/137.11.2547S
151. Frost GS, Brynes AE, Dhillo WS, Bloom SR, Mcburney MI. The effects of fiber enrichment of pasta and fat content on gastric emptying, GLP-1, glucose, and insulin responses to a meal. Eur J Clin Nutr. (2003) 57:293–8. doi: 10.1038/sj.ejcn.1601520
152. Ooi LG, Liong MT. Cholesterol-lowering effects of probiotics and prebiotics: a review of in vivo and in vitro findings. Int J Mol Sci. (2010) 11:2499–522. doi: 10.3390/ijms11062499
153. Gunness P, Williams BA, Gerrits WJJ, Bird AR, Kravchuk O, Gidley MJ. Circulating triglycerides and bile acids are reduced by a soluble wheat arabinoxylan via modulation of bile concentration and lipid digestion rates in a pig model. Nutr Food Res. (2016) 60:642–51. doi: 10.1002/mnfr.201500686
154. Gobinath D, Madhu AN, Prashant G, Srinivasan K, Prapulla SG. Beneficial effect of xylo-oligosaccharides and fructo-oligosaccharides in streptozotocin-induced diabetic rats. Br J Nutr. (2010) 104:40–7. doi: 10.1017/S0007114510000243
155. Veenashri BR, Muralikrishna G. In vitro antioxidant activity of xylooligosaccharides derived from cereal and millet bran-A comparative study. Food Chem. (2011) 126:1475–81. doi: 10.1016/j.foodchem.2010.11.163
156. Abasubong KP, Liu WB, Zhang DD, Yuan XY, Xia SL, Xu C, et al. Fishmeal replacement by rice protein concentrate with xylooligosaccharides supplement benefits the growth performance, antioxidant capability and immune responses against aeromonas hydrophila in blunt snout bream (Megalobrama amblycephala). Fish Shellfish Immunol. (2018) 78:177–86. doi: 10.1016/j.fsi.2018.04.044
157. Hromádková Z, Paulsen BS, Polovka M, Koštálová Z, Ebringerová A. Structural features of two heteroxylan polysaccharide fractions from wheat bran with anti-complementary and antioxidant activities. Carbohydr Polym. (2013) 93:22–30. doi: 10.1016/j.carbpol.2012.05.021
158. Hansen CHF, Larsen CS, Petersson HO, Zachariassen LF, Vegge A, Lauridsen C, et al. Targeting gut microbiota and barrier function with prebiotics to alleviate autoimmune manifestations in NOD mice. Diabetologia. (2019) 62:1689–700. doi: 10.1007/s00125-019-4910-5
159. Eaimworawuthikul S, Tunapong W, Chunchai T, Suntornsaratoon P, Charoenphandhu N, Thiennimitr P, et al. Altered gut microbiota ameliorates bone pathology in the mandible of obese–insulin-resistant rats. Eur J Nutr. (2020) 59:1453–62. doi: 10.1007/s00394-019-02002-8
160. Yin J, Li F, Kong XC, Wen Q, Guo L, Zhang W, et al. Dietary xylo-oligosaccharide improves intestinal functions in weaned piglets. Food Funct. (2019) 10:2701–9. doi: 10.1039/C8FO02485E
161. Rodríguez-Cabezas ME, Galvez J, Lorente MD, Concha A, Camuesco D, Azzouz S, et al. Dietary fiber down-regulates colonic tumor necrosis factor α and nitric oxide production in trinitrobenzenesulfonic acid-induced colitic rats. J Nutr. (2002) 132:3263–71. doi: 10.1093/jn/132.11.3263
162. Mendis M, Simsek S. Arabinoxylans and human health. Food Hydrocolloids. (2014) 42:239–43. doi: 10.1016/j.foodhyd.2013.07.022
163. López-Almela I, Romaní-Pérez M, Bullich-Vilarrubias C, Benítez-Páez A, Pulgar EMGD, Francés R, et al. Bacteroides uniformis combined with fiber amplifies metabolic and immune benefits in obese mice. Gut Microbes. (2021) 13:1–20. doi: 10.1080/19490976.2020.1865706
164. Palaniappan A, Antony U, Emmambux MN. Current status of xylooligosaccharides: production, characterization, health benefits and food application. Trends Food Sci Technol. (2021) 111:506–19. doi: 10.1016/j.tifs.2021.02.047
165. Remón J, Li T, Chuck CJ, Matharu AS, Clark JH. Toward renewable-based, food-applicable prebiotics from biomass: a one-step, additive-free, microwave-assisted hydrothermal process for the production of high purity xylo-oligosaccharides from beech wood hemicellulose. ACS Sustainable Chem Eng. (2019) 7:16160–72. doi: 10.1021/acssuschemeng.9b03096
166. Annison G, Topping DL. Nutritional role of resistant starch: chemical structure vs physiological function. Ann Rev Nutr. (1994) 14:297–320. doi: 10.1146/annurev.nu.14.070194.001501
167. Lavin JH, Read NW. The effect on hunger and satiety of slowing the absorption of glucose: relationship with gastric emptying and postprandial blood glucose and insulin responses. Appetite. (1995) 25:89–96. doi: 10.1006/appe.1995.0043
168. Antov MG, Dordević TR. Environmental-friendly technologies for the production of antioxidant xylooligosaccharides from wheat chaff. Food Chem. (2017) 235:175–80. doi: 10.1016/j.foodchem.2017.05.058
169. Kobayashi Y, Ohbuchi T, Fukuda T, Etsuko W, Risa Y, Mai H, et al. Acidic xylooligosaccharide preserves hepatic iron storage level in adult female rats fed a low-iron diet. J Nutr Sci Vitaminol. (2011) 57:292–7. doi: 10.3177/jnsv.57.292
170. Hesam F, Tarzi BG, Honarvar M, Jahadi M. Valorization of sugarcane bagasse to high value-added xylooligosaccharides and evaluation of their prebiotic function in a synbiotic pomegranate juice. Biomass Convers Biorefin. (2020) 1–13. doi: 10.1007/s13399-020-01095-0
171. Ferrão LL, Ferreira MVS, Cavalcanti RNA, Carvalho FA, Pimentel TC, Silva HLA, et al. The xylooligosaccharide addition and sodium reduction in requeijão cremoso processed cheese. Food Res Int. (2018) 107:137–47. doi: 10.1016/j.foodres.2018.02.018
172. Leddomado LS, Silva R, Guimarães JT, Carvalho AFA, Pimentel TC, Silva HLA, et al. Technological benefits of using inulin and xylooligosaccharide in dulce de leche. Food Hydrocolloids. (2021) 110:106158. doi: 10.1016/j.foodhyd.2020.106158
173. Ayyappan P, Abirami A, Anbuvahini N, Kumaran PST, Naresh M, Malathi D, et al. Physicochemical properties of cookies enriched with xylooligosaccharides. Food Sci Technol Int. (2016) 22:420–8. doi: 10.1177/1082013215617567
174. Juhász R, Penksza P, Sipos L. Effect of xylo-oligosaccharides (XOS) addition on technological and sensory attributes of cookies. Food Sci Nutr. (2020) 8:5452–60. doi: 10.1002/fsn3.1802
175. Silva EK, Arruda HS, Pastore GM, Meireles MAA, Saldana MD. Xylooligosaccharides chemical stability after high-intensity ultrasound processing of prebiotic orange juice. Ultrason Sonochem. (2020) 63:104942. doi: 10.1016/j.ultsonch.2019.104942
176. Souza FP, Balthazar CF, Guimarães JT, Pimentel TC, Esmerino EA, Freitas MQ, et al. The addition of xyloligoosaccharide in strawberry-flavored whey beverage. LWT Food Sci Technol. (2019) 109:118–22. doi: 10.1016/j.lwt.2019.03.093
177. Zhou J, Wu S, Qi G, Fu Y, Wang W, Zhang H, et al. Dietary supplemental xylooligosaccharide modulates nutrient digestibility, intestinal morphology, and gut microbiota in laying hens. Anim Nutr. (2021) 7:152–62. doi: 10.1016/j.aninu.2020.05.010
178. Craig AD, Khattak F, Hastie P, Bedford MR, Olukosi OA. Dietary supplemental xylooligosaccharide modulates nutrient digestibility, intestinal morphology, and gut microbiota in laying hens. PLoS ONE. (2021) 7:152–62.
179. Ribeiro T, Cardoso V, Ferreira LMA, Lordelo MMS, Coelho E, Moreira ASP, et al. Xylo-oligosaccharides display a prebiotic activity when used to supplement wheat or corn-based diets for broilers. Fontes Poult Sci. (2018) 97:4330–41. doi: 10.3382/ps/pey336
180. Su J, Zhang W, Ma C, Xie P, Blachier F, Kong X. Dietary supplementation with xylo-oligosaccharides modifies the intestinal epithelial morphology, barrier function and the fecal microbiota composition and activity in weaned piglets. Front Vet Sci. (2021) 8:680208. doi: 10.3389/fvets.2021.680208
181. Liu JB, Cao SC, Liu J, Xie YN, Zhang HF. Asian-Australas. Effect of probiotics and xylo-oligosaccharide supplementation on nutrient digestibility, intestinal health and noxious gas emission in weanling pigs. J Anim Sci. (2018) 31:1660. doi: 10.5713/ajas.17.0908
182. Wang C, Xu Z, Lu S, Jiang H, Li J, Wang L, et al. Effects of dietary xylooligosaccharide on growth, digestive enzymes activity, intestinal morphology, and the expression of inflammatory cytokines and tight junctions genes in triploid oncorhynchus mykiss fed a low fishmeal diet. Aquacult Rep. (2022) 22:100941. doi: 10.1016/j.aqrep.2021.100941
183. Hoseinifar SH, Sharifian M, Vesaghi MJ, Khalili M, Esteban MÁ. The effects of dietary xylooligosaccharide on mucosal parameters, intestinal microbiota and morphology and growth performance of Caspian white fish (Rutilus frisii kutum) fry. Fish Shellfish Immunol. (2014) 39:231–6. doi: 10.1016/j.fsi.2014.05.009
184. Abasubong KP, Li XF, Adjoumani JJY, Jiang GZ, Desouky HE, Liu WB. Effects of dietary xylooligosaccharide prebiotic supplementation on growth, antioxidant and intestinal immune-related genes expression in common carp cyprinus carpio fed a high-fat diet. Anim Nutr. (2022) 106:403–18. doi: 10.1111/jpn.13669
185. Sun C, Liu Y, Feng L, Jiang W, Wu P, Jiang J, et al. Xylooligosaccharide supplementation improved growth performance and prevented intestinal apoptosis in grass carp. Aquaculture. (2021) 535:736360. doi: 10.1016/j.aquaculture.2021.736360
186. Abdelmalek BE, Driss D, Kallel F, Guargouri M, Missaoui H, Chaabouni SE, et al. Effect of xylan oligosaccharides generated from corncobs on food acceptability, growth performance, haematology and immunological parameters of Dicentrarchus labrax fingerlings. Fish Physiol Biochem. (2015) 41:1587–96. doi: 10.1007/s10695-015-0110-5
187. Mhetras N, Mapre V, Gokhale D. Xylooligosaccharides (XOS) as emerging prebiotics: its production from lignocellulosic material. Adv Microbiol. (2019) 9:14–20. doi: 10.4236/aim.2019.91002
188. Kim MC, Lee S, Park JK, Park J, Lee D, Park J, et al. Effects of ID-HWS1000 on the perception of bowel activity and microbiome in subjects with functional constipation: a randomized, double-blind placebo-controlled study. J Med Food. (2021) 24:883–93. doi: 10.1089/jmf.2020.4746
189. Belorkar SA, Gupta AK. Oligosaccharides: a boon from nature's desk. Amb Express. (2016) 6:1–11. doi: 10.1186/s13568-016-0253-5
190. Katapodis P, Kavarnou A, Kintzios S, Pistola E, Kekos D, Macris BJ, et al. Production of acidic xylo-oligosaccharides by a family 10 endoxylanase from Thermoascus aurantiacus and use as plant growth regulators. Biotechnol Lett. (2002) 24:1413–6. doi: 10.1023/A:1019898414801
191. Fang P, Yan M, Chi C, Wang M, Zhou Y, Zhou J, et al. Brassinosteroids act as a positive regulator of photoprotection in response to chilling stress. Plant Physiol. (2019) 180:2061–76. doi: 10.1104/pp.19.00088
192. Ahammed GJ, Li X, Liu A, Chen S. Brassinosteroids in plant tolerance to abiotic stress. J Plant Growth Regul. (2020) 39:1451–4. doi: 10.1007/s00344-020-10098-0
193. Chen W, Chen G, Hussain S, Zhu B, Wu L. Role of xylo-oligosaccharides in protection against salinity-induced adversities in Chinese cabbage. Sci Pollut Res. (2016) 23:1254–64. doi: 10.1007/s11356-015-5361-2
194. He J, Han W, Wang J, Qian Y, Saito M, Bai W, et al. Functions of oligosaccharides in improving tomato seeding growth and chilling resistance. J Plant Growth Regul. (2021) 41:535–45. doi: 10.1007/s00344-021-10319-0
195. Liu H, Li Y, Tang B, Peng Y, Wu X, Che L, et al. Effects of xylooligosaccharide on angiotensin I-converting enzyme inhibitory activity of fish actomyosin and quality of snakehead balls with or without high hydrostatic pressure treatment. LWT Food Sci Technol. (2021) 140:110803. doi: 10.1016/j.lwt.2020.110803
196. Zhong SR, Li MF, Zhang ZH, Zong MH, Wu XL, Lou WY. Novel antioxidative wall materials for lactobacillus casei microencapsulation via the maillard reaction between the soy protein isolate and prebiotic oligosaccharides. J Agric Food Chem. (2021) 69:13744–53. doi: 10.1021/acs.jafc.1c02907
197. Neves MIL, Strieder MM, Prata AS, Silva EK, Meireles MAA. Xylooligosaccharides as an innovative carrier matrix of spray-dried natural blue colorant. Food Hydrocolloids. (2021) 121:107017. doi: 10.1016/j.foodhyd.2021.107017
198. Zhang C, Song Z, Jin P, Zhou X, Zhang H. Xylooligosaccharides induce stomatal closure via salicylic acid signaling-regulated reactive oxygen species and nitric oxide production in Arabidopsis. Physiol Plant. (2021) 172:1908–18. doi: 10.1111/ppl.13403
Keywords: xylooligosaccharide (XOS), xylanase, nutritional properties, agricultural and forestry byproducts, application
Citation: Yan F, Tian S, Du K, Xue X, Gao P and Chen Z (2022) Preparation and nutritional properties of xylooligosaccharide from agricultural and forestry byproducts: A comprehensive review. Front. Nutr. 9:977548. doi: 10.3389/fnut.2022.977548
Received: 24 June 2022; Accepted: 11 August 2022;
Published: 13 September 2022.
Edited by:
Bin Li, Shenyang Agricultural University, ChinaReviewed by:
Yanglei Yi, Northwest A&F University, ChinaParipok Phitsuwan, King Mongkut's University of Technology Thonburi, Thailand
Haisong Wang, Dalian Polytechnic University, China
Copyright © 2022 Yan, Tian, Du, Xue, Gao and Chen. This is an open-access article distributed under the terms of the Creative Commons Attribution License (CC BY). The use, distribution or reproduction in other forums is permitted, provided the original author(s) and the copyright owner(s) are credited and that the original publication in this journal is cited, in accordance with accepted academic practice. No use, distribution or reproduction is permitted which does not comply with these terms.
*Correspondence: Shuangqi Tian, dGlhbnNodWFuZ3FpQGhhdXQuZWR1LmNu; Zhicheng Chen, Y2hlbl8xOTU4QDE2My5jb20=