- 1Department of Biotechnology, Jaypee Institute of Information Technology, Noida, India
- 2School of Health Sciences, Pharmaceutical Sciences, The University of Petroleum & Energy Studies (UPES), Dehradun, India
- 3Department of Allied Health Sciences, School of Health Sciences and Technology, The University of Petroleum & Energy Studies (UPES), Dehradun, India
- 4Sunder Deep Pharmacy College, Ghaziabad, India
- 5Department of Pharmacognosy, Parmarth College of Pharmacy, Hapur, India
Probiotics are known as the live microorganisms which upon adequate administration elicit a health beneficial response inside the host by decreasing the luminal pH, eliminating the pathogenic bacteria in the gut as well as producing short chain fatty acids (SCFA). With advancements in research; probiotics have been explored as potential ingredients in foods. However, their use and applications in food industry have been limited due to restrictions of maintaining the viability of probiotic cells and targeting the successful delivery to gut. Encapsulation techniques have significant influence on increasing the viability rates of probiotic cells with the successful delivery of cells to the target site. Moreover, encapsulating techniques also prevent the live cells from harsh physiological conditions of gut. This review discusses several encapsulating techniques as well as materials derived from natural sources and nutraceutical compounds. In addition to this, this paper also comprehensively discusses the factors affecting the probiotics viability and evaluation of successful release and survival of probiotics under simulated gastric, intestinal conditions as well as bile, acid tolerant conditions. Lastly applications and challenges of using encapsulated bacteria in food industry for the development of novel functional foods have also been discussed in detail too. Future studies must include investigating the use of encapsulated bacterial formulations in in-vivo models for effective health beneficial properties as well as exploring the mechanisms behind the successful release of these formulations in gut, hence helping us to understand the encapsulation of probiotic cells in a meticulous manner.
Introduction
World Health Organization (WHO) and the Food and Agriculture Organization (FAO) have defined probiotics as the live microorganisms, including bacteria and yeasts, which upon consumption in adequate amounts confer health promoting effects to the host. Lactobacillus and Bifidobacteria are most commonly used probiotics which are either naturally present in food or added to increase the nutritional as well as functional quality of food (1). The consumption of probiotics have been associated with several therapeutic effects like protection against diarrhoeal diseases, inflammatory disorders, hypercholesterolemia as well as exhibit anticancer, anti-diabetic and anti-oxidative effects by enhancing the host immunity, strengthening the gut barrier and production of immune protective markers (2). Probiotics exists in liquid, paste, powdered form and are available in sachets as well as capsules. These have been widely used up in the food industry in development of novel functional foods, medicinal foods as well as dietary supplements. WHO and FAO suggests that in order to provide satisfactory health benefits to host, the viability of the probiotic cells should be more than 106 log CFU/ml or g in food (3). An emerging solution to compromising viability of ingested probiotics is encapsulation, and is endorsed by research fraternity as well as food industries. However, encapsulation is also not without drawbacks. Probiotic vulnerability to encapsulation parameters and the gut harsh conditions on ingestion—pH, temperature and oxygen tension, are still a serious concern. (4). To overcome these existing problems, several approaches have been proposed to increase the viability rates by decreasing the harmful environmental stresses. For instance; selection of acid and alkaline probiotic strains, using oxygen impermeable vessels, addition of micro and macro nutrients as well as encapsulating the probiotics, are some of the proposed approaches to increase the viability. Amongst these, encapsulation techniques have gathered great attention as this involves the entrapment of material in a matrix of polymeric membrane without affecting its biological activity. In this technique, the active materials are entrapped in the polymeric capsules which further prevent its deterioration from harmful environmental effects, leading to improved viability rates as well as successful release to the target sites. This technique has successfully been utilized in food industry, pharmaceutical industry, textile industry, cosmetics etc., (5). This paper extensively discusses the various different types of techniques involved in encapsulation of probiotics as well as the materials required for encapsulation. Moreover, the factors affecting the viability rates of probiotics during encapsulation have also been discussed in detail. Direct application of encapsulated probiotics in food industry, especially in development of novel functional foods with enhanced health beneficial properties are also comprehensively discussed in this paper.
Encapsulating techniques: Properties, advantages, and disadvantages
Encapsulation is defined as the process of entrapment of one material by another material. The material which is being entrapped is known as active material, filling material and internal material whereas the material which is being used to entrap the former one is called carrier, coating membrane, outer membrane and matrix. Based on the type of encapsulation method, there are majorly three variants of encapsulates: reservoir (microcapsule), matrix (microparticle) as well as coated matrix (multi- wall structure) (6) (Figure 1). Reservoir is defined as the encapsulation method where the active material is being capsuled by the outer layer of carrier material. The other encapsulation method, matrix, has the active material dispersed evenly over and on the surface of the carrier material. The coated matrix is defined as the method of encapsulation, where the capsuled active material is coated from additional coating material (7).
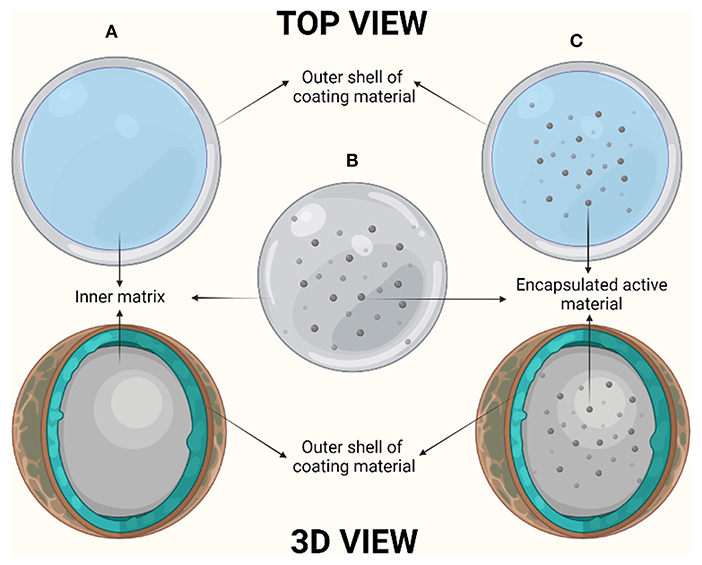
Figure 1. Diagrammatic representation of encapsulation systems: (A) Reservoir, (B) Matrix, and (C) Coated matrix.
Emulsification
Emulsification is a chemical technique to encapsulate active materials using the two phase systems. The discontinuous phase contains cell polymer hydrocolloid suspension of microorganisms whereas the continuous phase contains oil solution to form the emulsions. The discontinuous phase containing the active material is added to larger volume of continuous phase with regular stirring. The mixture of two phases forms the emulsion which is hardened by addition of CaCl2. Once the emulsion is formed, the outer layer must be removed to make tiny particles of encapsulated active material (8). This chemical method of encapsulation has been applied in encapsulating the probiotic cells (Figure 2). The main advantage of this technique is higher survival rates of probiotic bacteria as well as ease of scale-up at industrial levels (9). Moreover, this technique helps in production of encapsulated bacterial capsules with targeted size by optimizing the agitation speed and varying the water:oil ratio to produce the emulsions. Generally, the size of the microcapsules varies from 0.2 μm −8 mm (10). Encapsulating probiotic bacteria using emulsification is a well-established and widely used technique in food industry and has been discussed in later sections. One disadvantage with this technique is the application of additional polymeric layer to protect the encapsulated material to provide added protection. It is known that the classical way of encapsulating probiotics using emulsification makes use of alginate, carrageenan, as well as xanthan gum as outer coverings; which are not acceptable in food industries (11). Hence the solution is to use the milk based proteins as outer coating materials. Additional advantage of using the milk proteins as coating materials is that they have good gelation properties as well as act as natural carriers for probiotics. For gelation purposes, the proteolytic enzyme complex- rennet has been applied which successfully produces the casein micelles by splitting apart k- casein (12). These micelles are known to form gels at temperature above 18°C, hence protecting the encapsulated probiotic cells from outside environment (13). Besides this, using the emulsified encapsulated probiotic cells for fermentation industry with better productivity interfacial polymerization technique is used. In this technique, once the emulsions are formed, the biocompatible agent, like alginate, chitosan, carrageenan and gelatin are added to the continuous phase which encapsulates the bacteria from the discontinuous phase by forming thin layer droplets (14).
Spray drying
Spray drying is another dispersion technique to encapsulate active materials using two phase systems—liquid and air. In this technique, the solution of active material and the dissolved polymer is prepared and homogenized. The prepared solution is atomized using the atomizer to form mist/small liquid droplets which are then dried using hot air or nitrogen gas in the drying chamber at the temperature 150- 250° C (15). This procedure helps in evaporation of the bonded solvent followed by transferring the dried encapsulated material to the cyclone separator for further recovery. Generally, the polymeric solution used in this technique contains maltodextrin, granulated starchy solution, alginate, guar gum, xanthan gum as coating materials to encapsulate the active materials (16). Spray drying method is economic method with minimum energy utilization as compared to any other methods of encapsulation, producing the microcapsules of size 10–150 μm (17). This method has been widely used to encapsulate the probiotic bacteria too (Figure 3). This technique needs proper control and adjustments of inlet and outlet temperatures to maintain the viability of the probiotic cells. The only disadvantage with technique is that it has limited compatibility with only selected strains of probiotics which are tolerant to high range of temperature during the drying step (18). It is known that during the drying process, there is the reduction in the water activity and hence the bacteria become resistant to osmotic stress. The encapsulated bacteria are known to maintain their viability by accumulating the layer of carbohydrates as well as other amines. To provide protection to encapsulated bacteria from higher temperatures, the dryers are often equipped with cooling devices to lower the temperature of prepared microcapsules (19).
Lyophilisation (freeze drying)
This is the most commonly used technique for encapsulation of probiotics. This method involves higher freezing rates to avoid the damage to probiotic cells from small ice crystals. Freeze drying involves three steps of freezing, primary drying and secondary drying. In first stage, at lower freezing temperatures, the chemical and osmotic changes occur due to crystallization of water. The frozen water crystals are removed in primary drying due to sublimation process under vacuum conditions. The residual unfrozen water is again removed by desorption during secondary drying (20). Though this method is very convenient to carry out and provides microcapsules with larger surface area as compared to spray dried capsules, but the formation of ice crystals often damages probiotic cells' membranes cellular and surface proteins as well as reduces the water content of the cell, compromising the cellular viability (21). Moreover, this method requires more energy consumption and longer processing time (22). To overcome the demerits of this technique, some of the cryo- protectants have been added to during the process of freeze drying. The carbohydrates rich compounds like lactose, polyethylene glycol and sucrose have been extensively used as cryo-protectants which increase the unfrozen water and provide more space to probiotic cells to survive. These are also known to reduce the cellular damage caused by physical and osmotic stress (23). Another disadvantage of freeze drying is the necessity to select probiotic bacteria tolerant for low process temperatures. Researchers recommend a pre-stress conditioning phase, exposing bacteria to low temperatures to acclimate them as well as optimizing the process conditions to ensure viability of the cells (24).
Extrusion
Extrusion is another dispersion technique that is low cost and widely used. It involves mixing of probiotic cells into the polymeric hydrocolloid solution. The mix is passed through the nozzle of spray machine and the small droplets of the mix are projected into a solution of CaCl2 which results in gelation by cross linking mechanism. Though this technique is easy to scale up at industrial levels, there are several demerits of this technique (25). The number of process variables—temperature, concentration, flow rate and viscosity of the polymer and probiotic mix, diameter of the nozzle and drop height, to be controlled is high and affects the size of microcapsules formed (Figure 4). To overcome these disadvantages, advanced extrusion technique—prilling was introduced. In this technique, the droplets are formed under the controlled environmental conditions by applying vibrations to the nozzle sprayer. Prilling provides the control on the size of the beads by fluctuating the applied electric potential. This also reduces the cost by minimizing the dependency on additional organic solvents. Another advanced extrusion technique is centrifugal extrusion which involves the application of concentric orifices at the outlet of the nozzle sprayer and hence producing the microcapsules of desired size (26).
Electrospraying
Electrospraying offsets the disadvantages of using solvents and higher temperatures in previously mentioned techniques. In this technique, high speed and voltage is applied to form the microcapsules. This reduces the chances of toxicity of encapsulated material caused due to usage of solvents. The electric field is applied on the polymeric solution containing the probiotics to form capsules of size ranging from nano to micrometer, without the requirement of higher temperatures (27). There are several advantages associated with this technique like high versatility, simplicity and easy scale up. Since, there is no involvement of heat; there is minimum thermal damage to encapsulated probiotic cells in this technique (Table 1) (36).
Potential encapsulating materials in food system
The choice for encapsulation material is a challenging task. Apart from economic considerations the material should be edible and chosen such that it ensures the viability of probiotics at all stages of its development: from processing to storage to its targeted delivery in the GI tract. It has been commonly observed that a matrix showing gel like properties with high content of dry matter are generally more suitable (37). Nature of encapsulating material has a crucial role in determining encapsulation efficiency. A number of polymeric materials including polysaccharide hydrocolloids (alginate, xanthum, chitosan, carrageenan, cellulose), fats and proteins have been used for microencapsulation (24). Alginate is one of the most widely used materials due to its characteristics property to resist pH 2–2.5 (gastric pH) and expand at neutral to alkaline pH (38) (Table 2). However, the application of alginate is limited by high porosity. Therefore, use of other co-encapsulating materials has been proposed in a number of studies. A study by Iqbal and co-workers shows the potential use of chitosan or sodium alginate along with whey protein to form a double coating improving the viability of Bifidobacterium bifidum ATCC 35914 in simulated gastric environment from <1.87 log units of free cells after 1.5 h to more than 106Log CFU/mL after 2 h incubation (pH 2) (41). Polysaccharides have been classified into five distinct categories (46) on the basis of their structural role in microcapsulation as:
1. Polysaccharides that can be induced to form gels in presence of ions such as alginate, pectin in presence of Ca2+ ions or carrageenan via K+ ions
2. Polysaccharides that reinforce the structure and are resistant to acid and enzyme actions such as gellan gum
3. Polysaccharides that are selectively dissolute only in enteric environment such as cellulose acetate propionate
4. Polysaccharides with charges that can interact with opposite charges such as chitosan combining with alginate or pectin
5. Polysaccharides with prebiotic potential.
It should also be taken into consideration that food is a complex matrix where presence of many components such as antimicrobial compounds, additives, polyphenols, prebiotics and many more can have a direct effect on probiotics survivability especially during storage (4). A recent study shows that the viability of Lactobaccilus plantarum decreased in rose petal jam due to inherent low pH and water activity of jam. However, microencapsulation with 3.5% or 5% Arabic gum and 2% sodium alginate improved the storage stability of these probiotics and were detected at acceptable levels even after 90 days of storage at both room and refrigerated temperatures (45).
Prebiotics is defined as “a substrate that is selectively utilized by host microorganisms conferring a health benefit” (4). Some of the commonly used prebiotics are fructooligisaccharides (FOS), inulin, fructans, galacto-oligosaccharide. However, newer prebiotic sources are also being constantly explored such as dietary fibers, carbohydrates with different glycosidic linkages or monosaccharide content that can be used with or without modifications (4), exopolysaccharides such as mucilages, natural bioactive polysaccharides such as lentinan, psyllium and many more (46). Extracts of natural plants are also been increasingly explored for co-encapsulation in microencapsulating system such as onion extract (47), blueberry extract (48) for enhanced functional properties. Nami and co-workers studied the effect of incorporation of two prebiotics FOS and inulin in an alginate-persian gum-based hydrogel system for probiotic incorporation of Lactococcus lacti ABRIINW- N19 in orange juice. The study indicates that prebiotics got integrated in the polymeric two layered structure formed by the hydrogel. Both the prebiotics had good encapsulation efficiency, GI survivability and storage stability with decreased malic acid formation and sugar consumption and increased buffering capacity in comparison to free cells in orange juice. The viability decrease in encapsulated cells was only 1.46 log CFU/g compared to 6.52 log CGU/g for free cells after 2 h of simulated GI conditions (pH 2.5). However, in terms of release the dense structure of inulin used at higher percentages resulted in slow release that is observed completely only after 2 h (40). In another study, FOS isolated from banana peel was used along with sodium alginate showed an improvement in encapsulation efficiency of Lactobacillus rhamnosus. Besides, the incorporation of FOS in sodium alginate at 50 percent significantly enhanced viability with log reduction of 1.4 log CFU/mL as compared to 7.5 log CFU/mL for free cells (49).
In a recent interesting study, use of black waxy rice was explored as a prebiotic source in place of rice starch with an aim to utilize its' resistant starch which has slow digestibility and additionally has bioactive anthocyanins. These prebiotics have previously been reported to enhance growth of good bacteria particularly Bifidobaterium and Lactobacillus species and also impart favorable sensorial characteristics during Lactobacillus based fermentation. Therefore, the enzyme and heat moisture treated black waxy rice was used to encapsulate Lactobacillus plantarum and then incorporated in yogurt. Increased short chain fatty acid production and favorable growth of other probiotic species in yogurt suggests that resistant starch of rice could be further explored as prebiotic in synbiotic yogurt development (50).
In recent years, focus has also been on developing encapsulation material providing improved targeted delivery. The ability of certain materials to adhere to mucosal membranes can provide enhanced retention of probiotics in GI tract and thus can have direct impact on the bioavailabilty, administration frequency and targeted action of probiotics (51). An interesting study by Phuong and co-workers explored the potential of different materials including chitosan, chitosan coated alginate, alginate and alginate-resistant starch on gastric adhesion of probiotic bacteria Lactobacillus plantarum. The chitosan coated alginate showed mucoadhesive properties similar to chitosan and thus can be used as a system for targeted delivery of probiotic to gastric epithelium (52).
Factors affecting probiotics viability during encapsulation
Evidence suggests nearly 106 fold decline in the colony forming units in several commercial probiotic formulations within the first 5 min of exposure to simulated gastro-intestinal tract (GIT) conditions (53). Vulnerability to the harsh conditions during food processing/formulation, storage and in the GIT are key factors compromising the viability of beneficial probiotic rendering them ineffective. Further, emphasis is also placed on the three dimensional characteristics of the encapsulated particle in influencing the viable cell count. Herein, we discuss the various factors compromising the viable cell count:
Heat
Largest fraction of commercial strains of probiotics known today have established thermal susceptibility, as the optimal temperature for probiotic viability evolved to be the human body temperature. Mechanistic introspection of this declined viability due to low versus high temperatures exposure manifests via exacerbated membrane porosity leading to leakage of intra-cellular contents, and thermal stress mediated inactivation of critical molecular machinery (polymerases), respectively (54–56).
Food processing operations constituting probiotics are varied and expose the constituting microorganisms to widely different temperatures. At one end of the temperature spectrum are procedures such as freeze drying where cells are exposed to extremely low temperatures (up to −40°C), whereas spray drying, sterilization and pasteurization involve exposure to temperatures more than 60°C (57, 58). Diminished decimal reduction time and a 4–6 log reduction is reported in fruit juice inoculated with various species of Lactobacillus and Bifidobacterium on a 30 s exposure to 76°C (59). In fact, compromised viability is observed despite the encapsulation - Lactobacillus reuteri capsules dried at 55 °C led to decrease in viability from 1.6 × 109 CFU g −1 to 2.5 × 107 CFU g−1 (60). Composition of the encapsulating matrix is a key determinant of the thermal stress and cell death post-encapsulation. Bifidobacterium pseudocatenulatum CECT 7765 is an emerging probiotic bacterium with demonstrated health benefits in alleviating inflammation, vascular and neuroendocrine distress, metabolic syndrome incidence etc, but it is particularly heat sensitive (61, 62). Alehosseini and co-workers prepared agarose-based freeze-dried capsules via an “oil induced biphasic hydrogel formation” technique (agarose in combination with alginate, whey protein concentrate, gelatin) of Bifidobacterium pseudocatenulatum. Post-encapsulation, freeze drying conferred a 40–75% enhanced survival of the Bifidobacterium pseudocatenulatum CECT 7765 as compared to directly freeze-dried probiotic solution. Especially the combination of agarose with whey protein led to a marginal 1 log unit decline in cell viability over 2 months (63). Whey protein, milk proteins and gelatin have been demonstrated to confer protection to probiotic bacteria against thermal stress as well as other adverse ambient conditions in lieu of their amino acid composition which limits heat transfer at high temperatures and ability to form a viscous cell coating, protecting against ice crystal puncturing at low temperatures such as in lyophilization or freeze drying (64–66). Inclusion of sugars like lactose and trehalose has also been demonstrated to have protective abilities against thermal stress due to their ability to form stabilizing hydrogen bonding with the structural proteins constituting the plasma membrane and maintain its integrity despite displacement of water molecules due to thermal stress, during spray drying and storage (67, 68). Inclusion of low-melting-temperature fats into the encapsulating matrix limits heat transfer to the probiotic cells in the shell, as the fats melts and absorbs the heat, limiting the high temperature shock (69).
An alternate emerging strategy to counter thermal inactivation of probiotics during encapsulation is to expose the probiotic culture to an acclimatizing heat treatment at 50–52 °C for approximately 15 min to trigger the heat shock proteins and eventually confer protection against near-lethal temperature during food processing operations. The method has shown promising results in various heat sensitive strains of Lactobacillus spp (70).
A novel potential category of probiotic bacteria is soil-based organisms, Bacillus spp. being a prime example. The bacteria and its spores are heat resistant, and confer several health advantages (reviewed elsewhere by Lee and co-workers) (71). Survivability of Bacillus coagulans post-encapsulation is higher than the more common Lactobacillus spp. during spray drying as well as freeze drying. Nonetheless the material of the encapsulating matrix is the determinant in survivability during the processing procedures, skimmed milk conferring maximum protection and xanthan gum the least (72).
Oxygen toxicity
Differential oxygen tolerance of probiotic bacteria was reported as far back as in 1969 in different strains of Bifidobacteria (73). The microaerophilic or anaerobic nature of gut bacteria is attributed to the absence of electron transport chain, the ultimate cellular electron sump, leading to partial reduction of oxygen to form the cytotoxic hydrogen peroxide. This is more popularly known as oxygen toxicity (74). High levels of oxygen incorporated in food products during processing and preparation is an accepted factor compromising the viability of probiotic formulations. However, the physical isolation of microbial cells during encapsulation shields the cells from direct exposure to high oxygen levels permeating the formulation. Enhanced oxygen tolerance in encapsulated probiotics has been demonstrated in several investigations, notably, in the dairy industry for products such as yogurt, ice-cream, cheese etc (24, 75). Alginate encapsulated L. acidophilus and Bifidobacterium spp. are reported to have anoxic regions at the center of the encapsulated particle, creating a favorable microenvironment for survival of the cells. Significantly higher viable cell counts were observed in encapsulated cells vs. free cells, as a proof of concept that encapsulation shields from toxicity (76, 77).
An alternative strategy to enhance the oxygen tolerance of encapsulated cells can be to co-encapsulate an oxygen-consuming bacterial strain such as Streptococcus thermophilus, and create a favorable microenvironment for microaerophilic/anaerobic bacterial survival (24, 78). Additional precautions can be taken by setting up anaerobic environment during the encapsulation process, deoxygenating the encapsulating matrix in liquid phase (79) and adding anti-oxidants such as L-cysteine to sustain the microaerophilic and anaerobic organisms (77).
pH
The optimal pH for the viability and growth of probiotic bacteria is pH 6–7, the pH in the human colon microenvironment. The gastric pH, compromises probiotic survival by raising the cytosolic concentration of H+ ions which further interfere with activity of ATPase as well as denaturing the structural proteins and enzymes (80).
Alginate is a much-favored encapsulating matrix for probiotic formulations due to its pH responsive nature (81). Interestingly, a synbiotic formulation of a multi-particulate system comprising of poly (d,l-lactic-co-glycolic acid) (PLGA) microcapsules containing galacto-oligosaccharide in an alginate-chitosan matrix with Bifidobacteria enhanced the viable cell counts several log higher than a simple alginate-chitosan microencapsulation system (82). Recently, Pupa and co-workers demonstrated the efficacy of three double-microencapsulating formulations in preserving the probiotic viability of various Lactobacillus plantarum strains, Pediococcus pentosaceus 77F, and P. acidilactici 72N in the face of acid stress, bile stress and anti-bacterial activity. Namely, the double encapsulation comprised of alginate (1.5%) and chitosan (0.5%) and the microcapsules were formed via extrusion, emulsion, and spray drying. Spray-dried double microencapsulated formulation emerged as the most efficacious in preserving probiotic viability even after 6 months of storage at room temperature (83).
Bile stress
Besides the low pH, the GIT enzymes—lipases, proteolytic enzymes and amylases, in combination with the bile salts in the small intestine contribute to the harsh environment compromising the viability of cells in probiotic microencapsulated formulations (84). Bile acids in the small intestine are anti-bacterial and have detergent action which disrupts the bacterial cell membranes and membrane localized proteins, besides damaging the cellular nucleic acids (85). Differential bile tolerance amongst different probiotic bacteria relies on the expression of bile salt hydrolase enzyme (BSH). BSH positive bacteria are more tolerant to bile exposure. Besides BSH, bile salt efflux system also exist in various probiotic bacteria as a mechanism to cope with bile stress during passage via the small intestine (54, 86, 87). An interesting correlation between bile tolerance and antibiotic susceptibility of lactobacilli has also been reported, bile exposure renders lactobacilli susceptible to clinical regimes of antibiotics (88). Thus, shielding the probiotic bacteria from bile exposure is a favorable approach.
Khosravi Zanjani and coworkers formulated Lactobacillus casei and Bifidobacterium bifidum into alginate-gelatinize starch microcapsules with chitosan coating and observed significant protection from the simulated intestinal juice composed of pancreatin and 4.5% bile salts at pH 8.0 (89). Spray dried casein based emulsion of Bifidobacterium spp. and Lactobacillus acidophilus and several other commercial probiotic supplements was reported to have higher bile tolerance than non-encapsulated bacteria (90). Singh and co-workers formulated exo-polysacchride, alginate microcapsules containing L. acidophillus and other probiotic strains, and demonstrated enhanced viable cell counts in the simulated intestinal conditions (91).
Applications in functional food development and challenges
Probiotics have been considered as an important addition to food since ancient times. The selection of the probiotic strains often depends on the health beneficial properties as well as characteristics of the final product. Several strains of probiotics have been added traditionally to dairy products like cheese, buttermilk as well as dairy drink to carry out the fermentation process. Apart from the dairy products, probiotics have also been added to non- dairy food products like meat, cereals, vegetables and fruit juices as well as bakery items (92). As discussed in previous sections, the process of encapsulation of probiotics in food is a crucial decisive step to ensure viability and functionality of probiotics in functional foods and nutraceuticals. Herein we discuss examples of commercialized food products where encapsulated probiotics have been incorporated successfully: (Table 3).
Yogurt
Yogurt is one of the first dairy products used to incorporate probiotic bacteria. Several studies have been reported in literature making use of encapsulated probiotic cells without affecting the traditional way of yogurt preparation. However, the survivability of incorporated bacteria is often affected by high acidic environmental conditions. Hence, the better alternate is to use micro capsulated probiotic cells. For instance, L. acidophilus was incorporated in the yogurt during its preparation. The addition of free probiotic showed that the bacterial survival decreased from 9.97 log cfu/ml on first day to 6.12 log cfu/ml on day 28. To increase the viability of probiotics, the bacteria were encapsulated using sodium alginate and carrageenan using the extrusion technique. Encapsulated bacteria were released into the yogurt and it was observed that encapsulation using sodium alginate as well as carrageenan decreased the viability of encapsulated bacteria from 9.91 log cfu/ml to 8.74 log cfu/ml and 9.89 log cfu/ml to 8.39 log cfu/ml respectively from first day to day 28. The results showed that in both the cases the viability of probiotic bacteria was decreasing. However, the decrease in viability of bacteria was less after encapsulation as compared to free bacteria. In addition to this, the free probiotic cells showed poor survival rates under the simulated gastro- intestinal conditions as compared to encapsulated bacteria. This study also proved that sodium alginate was better encapsulating material for probiotics in comparison to carrageenan (93). Another study also investigated the effect of encapsulating bacteria on the sensory properties of yogurt. It was observed that the incorporation of alginate based encapsulated bacteria did not change sensory properties of yogurt including odor as well as color. However, minute textural change was observed in the yogurt containing the encapsulated bacteria. The encapsulated cells resulted in formation of grittiness in the final product and affecting the textural properties of yogurt (112). In addition to this, the incorporation of encapsulated probiotics in yogurt has been observed to increase the viscosity as well as mouth feel properties of the final product. The size of the encapsulated beads containing viable cells is directly linked to sensory characteristics. To illustrate, the microencapsulated beads with larger size are directly linked with more inferior sensory characteristics of the final product. Apart from using single material system for encapsulation, the three layered system for encapsulation has been studied too. To justify, xanthan- chitosan- xanthan multi-layered system was used to encapsulate the probiotic bacteria- Bifidobacterium BB01 in yogurt. The viability of encapsulated probiotic bacteria was investigated during the shelf life of yogurt for 21 days. Chitosan was used as the middle layer to encapsulate the target probiotic which was layered between two xanthan layers. The results depicted from this study showed that bacterial viability improved by encapsulation and also helped the bacteria to remain viable during gastric digestion for longer time as compared to free bacteria (94).
There are several probiotics strains which have been studied as microencapsulated bacteria incorporated in yogurt. These probiotics include L. casei, L. rhamnosus, L. plantarum, B. longum and B. bifidum. Apart from using the encapsulated material for enhancing the survival of probiotics, addition of cereals and milk proteins can increase the survival rates of probiotics too (113). Addition of encapsulated probiotics along with fruit juices often results in increasing the acidic environment of the food matrix and hence affecting the survival rate of probiotics. Hence, the better alternate is to use the nutritionally rich additives to support the viability of encapsulated bacteria. Prebiotics and carbohydrates sources are widely used additive in yogurt which controls the formation of acidic environment during milk fermentation process (114).
Cheese
Cheese is another dairy product which has been successfully utilized to incorporate the encapsulated probiotic bacteria. The milk proteins present in the cheese matrix provide the suitable environment as well as the nourishment to the probiotic cells. These milk proteins along with other carbohydrate sources are also known to provide the protection to the cells against the harsh stressed conditions of the gut (115). Amongst several variants of cheese, cheddar cheese is considered as the best carrier material for probiotics. It is known that the physiochemical properties of cheddar cheese provide the appropriate environment for survival of bacteria. Low acidic environment of cheddar cheese supports the bacterial survival (116). In one such study, low fat cream cheese has been developed as a successful delivery vehicle for Lactobacillus rhamnosus. The free and carbohydrate encapsulated (β-glucan and phytosterol) forms of probiotics were incorporated in the cheese; encapsulation using these sources provided the protection as well as the nourishment. It was observed that addition of encapsulated bacteria showed more survival as compared to free probiotic strains by the end of 35 days. Moreover, the encapsulated bacteria resulted in formation of firm and thicker cheese, with much higher consumer acceptability as compared to the cheese formed by free probiotic bacteria (117). Probiotics are often added as the starter cultures during the cheese making or as additives to enhance the nutritive and sensory properties of the final product. However, addition of probiotics as an additive is always considered as the better option. During the cheese making process, the ripening and storage of cheese often results in lowering the pH of the cheese matrix. As a result of this, the starter culture probiotic bacteria do no survive for long. Encapsulation of probiotics and consuming the bacterial cells as an additive resulted in more viability of probiotic cells (118). Apart from using polysaccharides as the encapsulating materials, milk proteins and wheat starch have been used up to encapsulate the probiotic bacteria. Results revealed that encapsulation of probiotics while using these novel matrices enhanced the probiotic survival under simulated gastro-intestinal conditions. Moreover, the cheese produced using wheat starch and milk protein exhibited acceptable taste, aroma and flavor. It is known that proteins and other carbohydrates sources offer great gelation property, non-reactive nature toward gel as well as offer good buffering capacity; hence favoring the survival of probiotics under harsh environmental conditions (119). Different probiotic strains have been involved in cheese making depending upon the desired flavor and health benefits. There are several probiotics which have been encapsulated using different techniques and have been incorporated into cheese making. The applications of encapsulated probiotics in cheese making have been summarized in Table 3. Another study includes the application of enzyme based gelation using skim milk powder and rennet, skim milk powder and transglutaminase as well as transglutaminase and sodium caseinate, for encapsulating the targeted probiotic strain. For the study, Lactobacillus paracasei was used for encapsulation and it was observed that rennet-based encapsulation was most efficient which resulted in more viable cells at the end of storage period and overall better textural properties of the final product (120).
Bakery
The incorporation of probiotics into bakery products is an emerging area for functional food development domain. The bakery products which have been developed with added probiotics include breads, buns, cookies, soufflés and cakes. However, the main challenge in developing the probiotic rich bakery product includes the maintenance of viability of probiotic cells. Higher temperature during the baking process often results in declining viability of live cells. In addition to this, the longer shelf lives of the bakery products do not always comply with long term viability of probiotic strains. In addition to this, the complexity of food matrix of the bakery product including physio-chemical environment as well as occurrence of milliard reaction upon storage; affects the survival of probiotics (121). Hence, the better alternate is to encapsulate the probiotic strains with suitable materials. In one such study, the use of heat resistant probiotic- Lactobacillus acidophilus LA-5 was examined. The selected probiotic strain was encapsulated using sodium alginate and additional substances, which enhanced the survival of probiotic bacteria during baking (102). In baking industry, the encapsulated bacteria have been involved in food products using three main techniques: encapsulated bacteria as outer coating, as additive during dough preparation and as additive in creams as well as fillers. To illustrate, Lactobacillus plantarum ATCC8014 was encapsulated using sodium alginate emulsions which were incorporated during dough baking. The emulsions were further coated with maltodextrin and pectin to provide additional protection to live cells during higher temperatures of baking, leading to successful survival of probiotics under simulated gastric conditions. Moreover, usage of prebiotic components also helped in creating denser network which enhanced the survival of probiotics under harsh conditions (103). Using the first application, the encapsulated probiotics (Limosilactobacillus fermentum NKN51 and Lactobacillus brevis NKN52) have been applied during the wheat buns making. The encapsulation was achieved by sodium caseinate and chia mucilage. The coating of encapsulated bacteria resulted in increased shelf life of live cells by 3 weeks under cold storage and by 2 weeks at room temperature (104).
Beverages
The potential health benefits of probiotics have led to its incorporation into the fermented as well as non- fermented beverages. Several fruits and prebiotics rich drinks have been developed with desired probiotic strains. These prebiotic contents include inulin, maltodextrin, lactulose, sorbitol and phytosterol; providing the extra protection to the viable cells. The pH as well as acidity of the fermented and non- fermented beverages affects the probiotic survival. Hence, the better alternate is to encapsulate probiotic bacteria using various techniques like spray drying, emulsions, electro- spraying and extrusion. To illustrate, three probiotic strains namely- Lactobacillus rhamnosus, Lactobacillus casei and Lactobacillus plantarum have been used to incorporate into the juice mix of acerola and ciriguela. The probiotic bacteria were encapsulated before addition in the fruit juice using spray drying technique. The encapsulating material was a prebiotic rich component- maltodextrin which helped in providing support and nourishment to the probiotic cells during encapsulation. The juice powders produced at the end of the study, showed maximum probiotic viability without affecting the physio- chemical, textural and sensory properties of the final product (107). Another study also demonstrated the incorporation of encapsulating materials like inulin and Tiliacora triandra gum to encapsulate Lactobacillus casei 01 and Lactobacillus acidophilus LA5 for development of probiotic rich maoluang juice. The effects of encapsulated probiotic bacteria have been examined and the results showed that free bacteria were not able to survive for longer duration due to environmental stress of the simulated gastric conditions. However, the encapsulated bacteria showed great survival rates as well as enhanced the accumulation of short chain fatty acids, lactic acid and decline in growth of other pathogenic bacteria too (108). Incorporation of three different prebiotic rich sources has also been used to encapsulate probiotic bacteria using spray drying technique. Encapsulated L. plantarum has been used to develop functional litchi juice. The encapsulation using the selected prebiotic strains showed even particle size of the microcapsule, hence enhancing the acceptability of final product. As mentioned earlier, more capsule size results in more grittiness texture of the product. Thus, selection of appropriate particle size as well as considering the viability of probiotics is the most critical step during encapsulation. In addition to this, the selected combination of prebiotics enhanced the survival of encapsulated probiotics under simulated gastric conditions (109).
Conclusion
Probiotics ingestion is considered a better alternative to medicine and treatments for maintaining a healthier lifestyle. Due to the numerous health-beneficial properties, probiotic bacteria have been incorporated into food items offering consumers a variety of choices from dairy to non-dairy food products. But the major challenge is to maintain the viability of probiotic strains during longer shelf lives of products with desired health beneficial properties exhibited by probiotics upon consumption. Microencapsulation as a technique has a potential to support the survivability of microorganisms in their unique environment. Therefore, if exploited to its full potential it can be used for effective delivery of important health beneficial microbiota via the food matrix. However, despite several studies, the present microencapsulation techniques do not completely support probiotic survival. Proper selection of encapsulating material as well as encapsulation technique is essential criteria to maintain longer viability of probiotic strains. Future studies must include research focused on the exploration of novel cost-effective encapsulating materials and techniques for industrial applications in functional food development. In addition, with increasing number of probiotic strains being explored it is essential that more research be directed to encapsulation of these novel strains as well.
Author contributions
SSi and SG contributed to conception and design of the study. SSi, RG, SG, SChaw, MS, RT, SU, SSh, and SChan wrote the first draft of the manuscript. SG and PG edited and revised the manuscript. All authors contributed to manuscript revision, read, and approved the submitted version.
Funding
This work was supported by Jaypee Institute of Information Technology, Noida, for which authors are deeply obliged.
Acknowledgments
The authors are grateful to Department of Biotechnology, Ministry of Science and Technology, New Delhi to award DBT-JRF fellowship to RG.
Conflict of interest
The authors declare that the research was conducted in the absence of any commercial or financial relationships that could be construed as a potential conflict of interest.
Publisher's note
All claims expressed in this article are solely those of the authors and do not necessarily represent those of their affiliated organizations, or those of the publisher, the editors and the reviewers. Any product that may be evaluated in this article, or claim that may be made by its manufacturer, is not guaranteed or endorsed by the publisher.
Supplementary material
The Supplementary Material for this article can be found online at: https://www.frontiersin.org/articles/10.3389/fnut.2022.971784/full#supplementary-material
References
1. Martín R, Langella P. Emerging health concepts in the probiotics field: streamlining the definitions. Front Microbiol. (2019) 10:1047. doi: 10.3389/fmicb.2019.01047
2. Minj J, Chandra P, Paul C, Sharma RK. Bio-functional properties of probiotic Lactobacillus: current applications and research perspectives. Crit Rev Food Sci Nutr. (2021) 61:2207–24. doi: 10.1080/10408398.2020.1774496
3. Valero-Cases E, Cerdá-Bernad D, Pastor JJ, Frutos MJ. Non-dairy fermented beverages as potential carriers to ensure probiotics, prebiotics, and bioactive compounds arrival to the gut and their health benefits. Nutrients. (2020) 12:1666. doi: 10.3390/nu12061666
4. Terpou A, Papadaki A, Lappa IK, Kachrimanidou V, Bosnea LA, Kopsahelis N. Probiotics in food systems: Significance and emerging strategies towards improved viability and delivery of enhanced beneficial value. Nutrients. (2019) 11:1591. doi: 10.3390/nu11071591
5. Onwulata CI. Microencapsulation and functional bioactive foods. J Food Proces Preserv. (2013) 37:510–32. doi: 10.1111/j.1745-4549.2012.00680.x
6. ÐDordević V, Balanč B, Belščak-Cvitanović A, Lević S, Trifković K, Kalušević A, et al. Trends in encapsulation technologies for delivery of food bioactive compounds. Food Eng Rev. (2015) 7:452–90. doi: 10.1007/s12393-014-9106-7
7. Radosavljević M, Lević S, Pejin J, Mojović L, Nedović V. Encapsulation technology of lactic acid bacteria in food fermentation. In: Lactic Acid Bacteria in Food Biotechnology. Amsterdam: Elsevier (2022). p. 319–47
8. Amin T, Thakur M, Jain SC. Microencapsulation-the future of probiotic cultures. J Microbiol Biotechnol Food Sci. (2021) 3:35–43. Available online at: https://www.jmbfs.org/wp-content/uploads/2013/07/jmbfs-0315-amin.pdf
9. Dong QY, Chen MY, Xin Y, Qin XY, Cheng Z, Shi LE, et al. Alginate-based and protein-based materials for probiotics encapsulation: a review. Int J Food Sci Technol. (2013) 48:1339–51. doi: 10.1111/ijfs.12078
10. Kanellopoulos A, Giannaros P, Palmer D, Kerr A, Al-Tabbaa A. Polymeric microcapsules with switchable mechanical properties for self-healing concrete: synthesis, characterisation and proof of concept. Smart Mat Struct. (2017) 26:045025. doi: 10.1088/1361-665X/aa516c
11. Asgari S, Pourjavadi A, Licht TR, Boisen A, Ajalloueian F. Polymeric carriers for enhanced delivery of probiotics. Adv Drug Deliv Rev. (2020) 161:1–21. doi: 10.1016/j.addr.2020.07.014
12. El-Salam A, Mohamed H, El-Shibiny S. Preparation and properties of milk proteins-based encapsulated probiotics: a review. Dairy Sci Technol. (2015) 95:393–412. doi: 10.1007/s13594-015-0223-8
13. Liu H, Cui SW, Chen M, Li Y, Liang R, Xu F, et al. Protective approaches and mechanisms of microencapsulation to the survival of probiotic bacteria during processing, storage and gastrointestinal digestion: a review. Crit Rev Food Sci Nutr. (2019) 59:2863–78. doi: 10.1080/10408398.2017.1377684
14. Schoebitz M, López MD, Roldán A. Bioencapsulation of microbial inoculants for better soil–plant fertilization. A review. Agron Sustain Dev. (2013) 33:751–65. doi: 10.1007/s13593-013-0142-0
15. Ray S, Raychaudhuri U, Chakraborty R. An overview of encapsulation of active compounds used in food products by drying technology. Food Biosci. (2016) 13:76–83. doi: 10.1016/j.fbio.2015.12.009
16. Rojas-Barboza DY. Recovery and Microencapsulation of Jujube (Ziziphus Jujuba Li) Bioactive Compounds Using Glandless Cottonseed Meal Protein Isolate as Carrier Agent. Doctoral dissertation. Las Cruces, NM: New Mexico State University (2020).
17. El-Kader A, Abu Hashish H. Encapsulation techniques of food bioproduct. Egypt J Chem. (2020) 63:1881–909. doi: 10.21608/ejchem.2019.16269.1993
18. Martín MJ, Lara-Villoslada F, Ruiz MA, Morales ME. Microencapsulation of bacteria: A review of different technologies and their impact on the probiotic effects. Innov Food Sci Emerg Technol. (2015) 27:15–25. doi: 10.1016/j.ifset.2014.09.010
19. Misra S, Pandey P, Mishra HN. Novel approaches for co-encapsulation of probiotic bacteria with bioactive compounds, their health benefits and functional food product development: a review. Trends Food Sci Technol. (2021) 109:340–51. doi: 10.1016/j.tifs.2021.01.039
20. Fonseca F, Cenard S, Passot S. Freeze-drying of lactic acid bacteria. In: Cryopreservation and Freeze-Drying Protocols. New York, NY: Springer (2015). p. 477–88.
21. Rathore S, Desai PM, Liew CV, Chan LW, Heng PWS. Microencapsulation of microbial cells. J Food Eng. (2013) 116:369–81. doi: 10.1016/j.jfoodeng.2012.12.022
22. Broeckx G, Vandenheuvel D, Claes IJ, Lebeer S, Kiekens F. Drying techniques of probiotic bacteria as an important step towards the development of novel pharmabiotics. Int J Pharm. (2016) 505:303–18. doi: 10.1016/j.ijpharm.2016.04.002
23. Tripathi MK, Giri SK. Probiotic functional foods: Survival of probiotics during processing and storage. J Funct Foods. (2014) 9:225–41. doi: 10.1016/j.jff.2014.04.030
24. Misra S, Pandey P, Dalbhagat CG, Mishra HN. Emerging technologies and coating materials for improved probiotication in food products: a review. Food Bioproc Tech. (2022) 15:998–1039. doi: 10.1007/s11947-021-02753-5
25. Vandamme TF, Gbassi GK, Nguyen TL, Li X. Microencapsulation of probiotics. Encapsulation and Controlled Release Technologies in Food Systems. New York, NY: Wiley-Blackwell (2016). p. 97–128.
26. Ngwuluka NC, Abu-Thabit NY, Uwaezuoke OJ, Erebor JO, Ilomuanya MO, Mohamed RR, et al. Natural polymers in micro-and nanoencapsulation for therapeutic and diagnostic applications: part I: lipids and fabrication techniques. Nano Microencapsul Tech Appl. (2021) 3–54. doi: 10.5772/intechopen.94856
27. Bhushani JA, Anandharamakrishnan C. Electrospinning and electrospraying techniques: potential food based applications. Trends Food Sci Technol. (2014) 38:21–33. doi: 10.1016/j.tifs.2014.03.004
28. Kakran M, Antipina MN. Emulsion-based techniques for encapsulation in biomedicine, food and personal care. Curr Opin Pharmacol. (2014) 18:47–55. doi: 10.1016/j.coph.2014.09.003
29. Piñón-Balderrama CI, Leyva-Porras C, Terán-Figueroa Y, Espinosa-Solís V, Álvarez-Salas C, Saavedra-Leos MZ. Encapsulation of active ingredients in food industry by spray-drying and nano spray-drying technologies. Processes. (2020) 8:889. doi: 10.3390/pr8080889
30. Estevinho BN, Rocha F, Santos L, Alves A. Microencapsulation with chitosan by spray drying for industry applications–A review. Trends Food Sci Technol. (2013) 31:138–55. doi: 10.1016/j.tifs.2013.04.001
31. Ozkan G, Franco P, De Marco I, Xiao J, Capanoglu E. A review of microencapsulation methods for food antioxidants: Principles, advantages, drawbacks and applications. Food Chem. (2019) 272:494–506. doi: 10.1016/j.foodchem.2018.07.205
32. Rezvankhah A, Emam-Djomeh Z, Askari G. Encapsulation and delivery of bioactive compounds using spray and freeze-drying techniques: a review. Drying Technol. (2020) 38:235–58. doi: 10.1080/07373937.2019.1653906
33. Fangmeier M, Lehn DN, Maciel MJ, Volken de Souza CF. Encapsulation of bioactive ingredients by extrusion with vibrating technology: advantages and challenges. Food Bioprocess Technol. (2019) 12:1472–86. doi: 10.1007/s11947-019-02326-7
34. Suganya V, Anuradha V. Microencapsulation and nanoencapsulation: a review. Int J Pharm Clin Res. (2017) 9:233–9. doi: 10.25258/ijpcr.v9i3.8324
35. Tanhaei A, Mohammadi M, Hamishehkar H, Hamblin MR. Electrospraying as a novel method of particle engineering for drug delivery vehicles. J Controlled Release. (2021) 330:851–65. doi: 10.1016/j.jconrel.2020.10.059
36. Haffner FB, Diab R, Pasc A. Encapsulation of probiotics: insights into academic and industrial approaches. AIMS Mat Sci. (2016) 3:114–36. doi: 10.3934/matersci.2016.1.114
37. Ta LP, Bujna E, Antal O, Ladányi M, Juhász R, Szécsi A, et al. Effects of various polysaccharides (alginate, carrageenan, gums, chitosan) and their combination with prebiotic saccharides (resistant starch, lactosucrose, lactulose) on the encapsulation of probiotic bacteria Lactobacillus casei 01 strain. Int J Biol Macromol. (2021) 183:1136–44. doi: 10.1016/j.ijbiomac.2021.04.170
38. Djaenudin Saepudin E, Nasir M. Microencapsulation of probiotic Lactobacillus casei based on alginate and chitosan materials. Asian J Appl Sci. (2021) 9:149–57. doi: 10.24203/ajas.v9i2.6564
39. Qi G, Shidong L, Jiaxin T, Shuaidan C, Liyue Q, Gengan D, et al. Microencapsulation of Lactobacillus plantarum by spray drying: protective effects during simulated food processing, gastrointestinal conditions, and in kefir. Int J Biol Macromol. (2022) 194:539–45. doi: 10.1016/j.ijbiomac.2021.11.096
40. Nami Y, Lornezhad G, Kiani A, Abdullah N, Haghshenas B. Alginate-Persian Gum-Prebiotics microencapsulation impacts on the survival rate of Lactococcus lactis ABRIINW-N19 in orange juice. LWT. (2020) 124:109190. doi: 10.1016/j.lwt.2020.109190
41. Iqbal R, Zahoor T, Huma N, Jamil A, Ünlü G. In-vitro GIT Tolerance of microencapsulated Bifidobacterium bifidum ATCC 35914 using polysaccharide-protein matrix. Probio Antimicrob Proteins. (2019) 11:830–9. doi: 10.1007/s12602-017-9384-5
42. Kiani A, Nami Y, Hedayati S, Jaymand M, Samadian H, Haghshenas B. Tarkhineh as a new microencapsulation matrix improves the quality and sensory characteristics of probiotic Lactococcus lactis KUMS-T18 enriched potato chips. Sci Rep. (2021) 11:12599. doi: 10.1038/s41598-021-92095-1
43. Hossain MN, Ranadheera CS, Fang Z, Ajlouni S. Impact of encapsulating probiotics with cocoa powder on the viability of probiotics during chocolate processing, storage, and in vitro gastrointestinal digestion. J Food Sci. (2021) 86:1629–41. doi: 10.1111/1750-3841.15695
44. Muzzafar A, Sharma V. Microencapsulation of probiotics for incorporation in cream biscuits. Food Measure. (2018) 12:2193–201. doi: 10.1007/s11694-018-9835-z
45. Shoaei F, Heshmati A, Mahjub R, Garmakhany AD, Taheri M. The assessment of microencapsulated Lactobacillus plantarum survivability in rose petal jam and the changes in physicochemical, textural and sensorial characteristics of the product during storage. Sci Re. (2022) 12:6200. doi: 10.1038/s41598-022-10224-w
46. Liu H, Xie M, Nie S. Recent trends and applications of polysaccharides for microencapsulation of probiotics. Food Front. (2020) 1: 1–15. doi: 10.1002/fft2.11
47. Ashwanandhini G, Reshma R, Preetha R. Synbiotic microencapsulation of Enterococcus faecium Rp1: a potential probiotic isolated from ragi porridge with antiproliferative property against colon carcinoma cell line. J Food Sci Technol. (2022) 1–7. doi: 10.1007/s13197-022-05415-2
48. Neuenfeldt NH, Farias CAA, Mello RO, Robalo SS, Barin JS, de Silva LP, et al. Effects of blueberry extract co-microencapsulation on the survival of Lactobacillus rhamnosus. LWT. (2022) 155:112886. doi: 10.1016/j.lwt.2021.112886
49. Azam M, Saeed M, Pasha I, Shahid M. A prebiotic-based biopolymeric encapsulation system for improved survival of Lactobacillus rhamnosus. Food Biosci. (2020) 37:100679. doi: 10.1016/j.fbio.2020.100679
50. Wattananapakasem I, van Valenberg HJF, Fogliano V. Synbiotic microencapsulation from slow digestible colored rice and its effect on yoghurt quality. Food Bioprocess Technol. (2018) 11:1111–24. doi: 10.1007/s11947-018-2068-7
51. Razavi S, Janfaza S, Tasnim N, Gibson DL, Hoorfar M. Nanomaterial-based encapsulation for controlled gastrointestinal delivery of viable probiotic bacteria. Nanoscale Adv. (2021) 3:2699–709. doi: 10.1039/D0NA00952K
52. Phuong Ta L, Bujna E, Kun S, Charalampopoulos D, Khutoryanskiy VV. Electrosprayed mucoadhesive alginate-chitosan microcapsules for gastrointestinal delivery of probiotics. Int J Pharm. (2021) 597:120342. doi: 10.1016/j.ijpharm.2021.120342
53. Dodoo CC, Wang J, Basit AW, Stapleton P, Gaisford S. Targeted delivery of probiotics to enhance gastrointestinal stability and intestinal colonisation. Int J Pharm 15. (2017) 530:224–9. doi: 10.1016/j.ijpharm.2017.07.068
54. Wendel U. Assessing viability and stress tolerance of probiotics-a review. Front Microbiol. (2022) 12:818468. doi: 10.3389/fmicb.2021.818468
55. Smelt JP, Brul S. Thermal inactivation of microorganisms. Crit Rev Food Sci Nutr. (2014) 54:1371–85. doi: 10.1080/10408398.2011.637645
56. Santivarangkna C, Kulozik U, Foerst P. Inactivation mechanisms of lactic acid starter cultures preserved by drying processes. J Appl Microbiol. (2008) 105:1–13. doi: 10.1111/j.1365-2672.2008.03744.x
57. Massounga Bora AF, Li X, Zhu Y, Du L. Improved viability of microencapsulated probiotics in a freeze-dried banana powder during storage and under simulated gastrointestinal tract. Probio Antimicrob Prot. (2019) 11:1330–9. doi: 10.1007/s12602-018-9464-1
58. Assadpour E, Jafari SM. Advances in spray-drying encapsulation of food bioactive ingredients: from microcapsules to nanocapsules. Annu Rev Food Sci Technol. (2019) 10:103–31. doi: 10.1146/annurev-food-032818-121641
59. Sheehan VM, Ross P, Fitzgerald GF. Assessing the acid tolerance and the technological robustness of probiotic cultures for fortification in fruit juices. Innov Food Sci Emerg Technol. (2007) 8:279–84. doi: 10.1016/j.ifset.2007.01.007
60. Jantzen M, Göpel A, Beermann C. Direct spray drying and microencapsulation of probiotic Lactobacillus reuteri from slurry fermentation with whey. J Appl Microbiol. (2013) 115:1029–36. doi: 10.1111/jam.12293
61. Moya-Pérez A, Neef A, Sanz Y. Bifidobacterium pseudocatenulatum CECT 7765 reduces obesity-associated inflammation by restoring the lymphocyte-macrophage balance and gut microbiota structure in high-fat diet-fed mice. PLoS ONE. (2015) 10:e0126976. doi: 10.1371/journal.pone.0126976
62. Simpson PJ, Stanton C, Fitzgerald GF, Ross RP. Intrinsic tolerance of Bifidobacterium species to heat and oxygen and survival following spray drying and storage. J Appl Microbiol. (2005) 99:493–501. doi: 10.1111/j.1365-2672.2005.02648.x
63. Alehosseini A, del Pulgar E–MG, Fabra MJ, Gómez-Mascaraque LG, Benítez-Páez A, Sarabi-Jamab M, et al. Agarose-based freeze-dried capsules prepared by the oil-induced biphasic hydrogel particle formation approach for the protection of sensitive probiotic bacteria. Food Hydrocolloids. (2019) 87:487–96 doi: 10.1016/j.foodhyd.2018.08.032
64. Arslan S, Topuz A, Tontul I, Erbas M. Microencapsulation of probiotic Saccharomyces cerevisiae var. boulardii with different wall materials by spray drying. Technol Food Sci Technol. (2015) 63:685–90. doi: 10.1016/j.lwt.2015.03.034
65. Wang SY, Ho YF, Chen YP, Chen MJ. Effects of a novel encapsulating technique on the temperature tolerance and anti-colitis activity of the probiotic bacterium Lactobacillus kefiranofaciens M1. Food Microbiol. (2015) 46:494–500. doi: 10.1016/j.fm.2014.09.015
66. de Araújo Etchepare M, Nunes GL, Nicoloso BR, Barin JS, Flores EMM, de Oliveira Mello R, et al. Improvement of the viability of encapsulated probiotics using whey proteins. LWT. (2020) 117:108601 doi: 10.1016/j.lwt.2019.108601
67. Lapsiri W, Bhandari B, Wanchaitanawong P. Viability of Lactobacillus plantarum TISTR 2075 in different protectants during spray drying and storage. Dry Technol. (2012) 30:1407–12. doi: 10.1080/07373937.2012.684226
68. Sinkiewicz-Enggren G, Skurzynska A, Sandberg T. Stabilization of Lactobacillus reuteri by encapsulation of bacterial cells through spray drying. Am J Biomed. (2015) 3:432–43. doi: 10.18081/2333-5106/015-07/432-443
69. Huang S, Vignolles ML, Chen XD, Loir YL, Jan G, Schuck P, et al. Spray drying of probiotics and other food-grade bacteria: A review. Trends Food Sci Technol. (2017) 63:1–17. doi: 10.1016/j.tifs.2017.02.007
70. Paéz R, Lavari L, Vinderola G, Audero G, Cuatrin A, Zaritzky N, et al. Effect of heat treatment and spray drying on lactobacilli viability and resistance to simulated gastrointestinal digestion. Food Res Int. (2012) 48:748–54. doi: 10.1016/j.foodres.2012.06.018
71. Lee NK, Kim WS, Paik HD. Bacillus strains as human probiotics: characterization, safety, microbiome, and probiotic carrier. Food Sci Biotechnol. (2019) 28:1297–305. doi: 10.1007/s10068-019-00691-9
72. Pandey KR, Vakil BV. Encapsulation of probiotic bacillus coagulans for enhanced shelf life. J App Biol Biotech. (2017) 5:057–65. doi: 10.7324/JABB.2017.50409
73. de Vries W, Stouthamer AH. Factors determining the degree of anaerobiosis of Bifidobacterium strains. Arch Mikrobiol. (1969) 65:275–87. doi: 10.1007/BF00407109
74. Talwalkar A, Kailasapathy K. The role of oxygen in the viability of probiotic bacteria with reference to L. acidophilus and Bifidobacterium spp. Curr Issues Intest Microbiol. (2004) 5:1–8.
75. Talwalkar A, Kailasapathy K. A review of oxygen toxicity in probiotic yogurts: influence on the survival of probiotic bacteria and protective techniques. Compr Rev Food Sci Food Saf. (2004) 3:117–24. doi: 10.1111/j.1541-4337.2004.tb00061.x
76. Talwalkar A, Kailasapathy K. Effect of microencapsulation on oxygen toxicity in probiotic bacteria. Aust J Dairy Technol. (2003) 58:36–9. Available online at: https://www.proquest.com/docview/199359432?pq-origsite=gscholar&fromopenview=true
77. Rodrigues D, Sousa S, Rocha-Santos T, Silva JP, Sousa Lobo JM, Costa P, et al. Influence of l-cysteine, oxygen and relative humidity upon survival throughout storage of probiotic bacteria in whey protein-based microcapsules. Int Dairy J. (2011) 21:869–76. doi: 10.1016/j.idairyj.2011.05.005
78. Lourens-Hattingh A, Viljoen BC. Yogurt as probiotic carrier food. Int Dairy J. (2001) 11:1–17. doi: 10.1016/S0958-6946(01)00036-X
79. Chen J, Wang Q, Liu CM, Gong J. Issues deserve attention in encapsulating probiotics: Critical review of existing literature. Crit Rev Food Sci Nutr. (2017) 57:1228–38. doi: 10.1080/10408398.2014.977991
80. Cotter PD, Hill C. Surviving the acid test: responses of gram-positive bacteria to low pH. Microbiol Mol Biol Rev. (2003) 67:429–53. doi: 10.1128/MMBR.67.3.429-453.2003
81. Allan-Wojtas P, Hansen LT, Paulson AT. Microstructural studies of probiotic bacteria-loaded alginate microcapsules using standard electron microscopy techniques and anhydrous fixation. LWT-Food Sci Technol. (2008) 41:101–8. doi: 10.1016/j.lwt.2007.02.003
82. Cook MT, Tzortzis G, Charalampopoulos D, Khutoryanskiy VV. Microencapsulation of a synbiotic into PLGA/alginate multiparticulate gels. Int J Pharm. (2014) 466:400–8. doi: 10.1016/j.ijpharm.2014.03.034
83. Pupa P, Apiwatsiri P, Sirichokchatchawan W. The efficacy of three double-microencapsulation methods for preservation of probiotic bacteria. Sci Rep. (2021) 11:13753. doi: 10.1038/s41598-021-93263-z
84. Yao M, Xie J, Du H, McClements DJ, Xiao H, Li L. Progress in microencapsulation of probiotics: a review. Compr Rev Food Sci Food Saf. (2020) 19:857–74. doi: 10.1111/1541-4337.12532
85. Gou X, Zhang L, Zhao S, Ma W, Yang Z. Application of the combination of soybean lecithin and whey protein concentrate 80 to improve the bile salt and acid tolerance of probiotics. J Microbiol Biotechnol. (2021) 31:840–6. doi: 10.4014/jmb.2103.03017
86. Ruiz L, Margolles A, Sánchez B. Bile resistance mechanisms in Lactobacillus and Bifidobacterium. Front Microbiol. (2013) 4:396. doi: 10.3389/fmicb.2013.00396
87. Begley M, Hill C, Gahan CG. Bile salt hydrolase activity in probiotics. Appl Environ Microbiol. (2006) 72:1729–38. doi: 10.1128/AEM.72.3.1729-1738.2006
88. Hyacinta M, Hana KS, Andrea B, Barbora C. Bile tolerance and its effect on antibiotic susceptibility of probiotic Lactobacillus candidates. Folia Microbiol (Praha). (2015) 60:253–7. doi: 10.1007/s12223-014-0365-8
89. Khosravi Zanjani MA, Ghiassi Tarzi B, Sharifan A, Mohammadi N. Microencapsulation of probiotics by calcium alginate-gelatinized starch with chitosan coating and evaluation of survival in simulated human gastro-intestinal condition. Iran J Pharm Res. (2014) 13:843–52.
90. Dianawati D, Mishra V, Shah NP. Viability, acid and bile tolerance of spray dried probiotic bacteria and some commercial probiotic supplement products kept at room temperature. J Food Sci. (2016) 81:M1472–9. doi: 10.1111/1750-3841.13313
91. Singh P, Saini P, Dubey S, Srivastava U, Singh A, Kaur D, et al. Microencapsulation of probiotics by exopolysaccharides-sodium alginate and evaluation of their survival in simulated gi conditions. Current Nutr Food Sci. (2020). 16:1141–6. doi: 10.2174/1573401316666200123095452
92. De Prisco A, Mauriello G. Probiotication of foods: a focus on microencapsulation tool. Trends Food Sci Technol. (2016) 48:27–39. doi: 10.1016/j.tifs.2015.11.009
93. Afzaal M, Khan AU, Saeed F, Ahmed A, Ahmad MH, Maan AA, et al. Functional exploration of free and encapsulated probiotic bacteria in yogurt and simulated gastrointestinal conditions. Food Sci Nutr. (2019) 7:3931–40. doi: 10.1002/fsn3.1254
94. Chen L, Yang T, Song Y, Shu G, Chen H. Effect of xanthan-chitosan-xanthan double layer encapsulation on survival of Bifidobacterium BB01 in simulated gastrointestinal conditions, bile salt solution and yogurt. LWT-Food Sci Technol. (2017) 81:274–80. doi: 10.1016/j.lwt.2017.04.005
95. Romero-Chapol OO, Varela-Pérez A, Castillo-Olmos AG, García HS, Singh J, García-Ramírez PJ, et al. Encapsulation of Lacticaseibacillus rhamnosus GG: probiotic survival, in vitro digestion and viability in apple juice and yogurt. Appl Sci. (2022) 12:2141. doi: 10.3390/app12042141
96. Kia EM, Ghasempour Z, Ghanbari S, Pirmohammadi R, Ehsani A. Development of probiotic yogurt by incorporation of milk protein concentrate (MPC) and? microencapsulated Lactobacillus paracasei? in gellan-caseinate mixture. Br Food J. (2018). 120:1516–28. doi: 10.1108/BFJ-12-2017-0668
97. Fazilah NF, Hamidon NH, Ariff AB, Khayat ME, Wasoh H, Halim M. Microencapsulation of Lactococcus lactis Gh1 with gum arabic and synsepalum dulcificum via spray drying for potential inclusion in functional yogurt. Molecules. (2019) 24:1422. doi: 10.3390/molecules24071422
98. Hain AB, Gomes IT, Gomes RG, Bergamasco RDC. Effect of β-cyclodextrin on the survival of alginate–xanthan microencapsulated bifidobacterium-BB12 in simulated digestive conditions and frozen yogurt. J Culinary Sci Technol. (2021) 1–12. doi: 10.1080/15428052.2021.1884997
99. Afzaal M, Saeed F, Ateeq H, Ahmed A, Ahmad A, Tufail T, et al. Encapsulation of Bifidobacterium bifidum by internal gelation method to access the viability in cheddar cheese and under simulated gastrointestinal conditions. Food Sci Nutr. (2020) 8:2739–47. doi: 10.1002/fsn3.1562
100. Mudgil P, Aldhaheri F, Hamdi M, Punia S, Maqsood S. Fortification of Chami (traditional soft cheese) with probiotic-loaded protein and starch microparticles: characterization, bioactive properties, and storage stability. LWT. (2022) 158:113036. doi: 10.1016/j.lwt.2021.113036
101. Mirković M, Mirković N, Miočinović J, Radulović A, Paunović D, Ilić M, et al. Probiotic yogurt and cheese from ultrafiltered milk: Sensory quality and viability of free-living and spray dried Lactiplantibacillus plantarum 564 and Lactiplantibacillus plantarum 299v. J Food Process Preserv. (2021) 45:e15713. doi: 10.1111/jfpp.15713
102. Hadidi M, Majidiyan N, Jelyani AZ, Moreno A, Hadian Z, Mousavi Khanegah A. Alginate/fish gelatin-encapsulated Lactobacillus acidophilus: a study on viability and technological quality of bread during baking and storage. Foods. (2021) 10:2215. doi: 10.3390/foods10092215
103. Dong LM, Luan NT, Thuy DTK. Enhancing the viability rate of probiotic by co-encapsulating with prebiotic in alginate microcapsules supplemented to cupcake production. Microbiol Biotechnol Letters. (2020) 48:113–20. doi: 10.4014/mbl.1910.10015
104. Semwal A, Ambatipudi K, Navani NK. Development and characterization of sodium caseinate based probiotic edible film with chia mucilage as a protectant for the safe delivery of probiotics in functional bakery. Food Hydrocoll Health. (2022) 2:100065. doi: 10.1016/j.fhfh.2022.100065
105. Ghasemi L, Nouri L, Mohammadi Nafchi A, Al-Hassan AA. The effects of encapsulated probiotic bacteria on the physicochemical properties, staling, and viability of probiotic bacteria in gluten-free bread. J Food Process Preserv. (2022) 46:e16359. doi: 10.1111/jfpp.16359
106. Zhang L, Chen XD, Boom RM, Schutyser MA. Survival of encapsulated Lactobacillus plantarum during isothermal heating and bread baking. LWT. (2018) 93:396–404. doi: 10.1016/j.lwt.2018.03.067
107. Souza M, Mesquita A, Veríssimo C, Grosso C, Converti A, Maciel MI. Microencapsulation by spray drying of a functional product with mixed juice of acerola and ciriguela fruits containing three probiotic lactobacilli. Drying Technol. (2022) 40:1185–95. doi: 10.1080/07373937.2020.1862182
108. Chaikham P, Kemsawasd V, Seesuriyachan P. Spray drying probiotics along with maoluang juice plus Tiliacora triandra gum for exposure to the in vitro gastrointestinal environments. LWT. (2017) 78:31–40. doi: 10.1016/j.lwt.2016.12.013
109. Kalita D, Saikia S, Gautam G, Mukhopadhyay R, Mahanta CL. Characteristics of synbiotic spray dried powder of litchi juice with Lactobacillus plantarum and different carrier materials. LWT. (2018) 87:351–60. doi: 10.1016/j.lwt.2017.08.092
110. Dias CO, de Almeida JDSO, Pinto SS, de Oliveira Santana FC, Verruck S, Müller CMO, et al. Development and physico-chemical characterization of microencapsulated bifidobacteria in passion fruit juice: a functional non-dairy product for probiotic delivery. Food Biosci. (2018) 24:26–36. doi: 10.1016/j.fbio.2018.05.006
111. Mokhtari S, Jafari SM, Khomeiri M. Survival of encapsulated probiotics in pasteurized grape juice and evaluation of their properties during storage. Food Sci Technol Int. (2019) 25:120–9. doi: 10.1177/1082013218801113
112. Ortakci FATIH, Sert S. Stability of free and encapsulated Lactobacillus acidophilus ATCC 4356 in yogurt and in an artificial human gastric digestion system. J Dairy Sci. (2012) 95:6918–25. doi: 10.3168/jds.2012-5710
113. Rokka S, Rantamäki P. Protecting probiotic bacteria by microencapsulation: challenges for industrial applications. Eur Food Res Technol. (2010) 231:1–12. doi: 10.1007/s00217-010-1246-2
114. Fazilah NF, Ariff AB, Khayat ME, Rios-Solis L, Halim M. Influence of probiotics, prebiotics, synbiotics and bioactive phytochemicals on the formulation of functional yogurt. J Funct Foods. (2018) 48:387–99. doi: 10.1016/j.jff.2018.07.039
115. Chávarri M, Marañón I, Villarán MC. Encapsulation technology to protect probiotic bacteria. In: Probiotics. London: IntechOpen (2012).
116. Meira QGS, Magnani M, de Medeiros Júnior FC, do Egito RDCR, Madruga MS, Gullón B, et al. Effects of added Lactobacillus acidophilus and Bifidobacterium lactis probiotics on the quality characteristics of goat ricotta and their survival under simulated gastrointestinal conditions. Food Res Int. (2015) 76:828–38. doi: 10.1016/j.foodres.2015.08.002
117. Ningtyas DW, Bhandari B, Bansal N, Prakash S. The viability of probiotic Lactobacillus rhamnosus (non-encapsulated and encapsulated) in functional reduced-fat cream cheese and its textural properties during storage. Food Control. (2019) 100:8–16. doi: 10.1016/j.foodcont.2018.12.048
118. Boylston TD, Vinderola CG, Ghoddusi HB, Reinheimer JA. Incorporation of bifidobacteria into cheeses: challenges and rewards. Int Dairy J. (2004) 14:375–87. doi: 10.1016/j.idairyj.2003.08.008
119. Nahum V, Domb AJ. Recent developments in solid lipid microparticles for food ingredients delivery. Foods. (2021) 10:400. doi: 10.3390/foods10020400
120. Moghaddas Kia E, Alizadeh M, Esmaiili M. Development and characterization of probiotic UF Feta cheese containing Lactobacillus paracasei microencapsulated by enzyme based gelation method. J Food Sci Technol. (2018) 55:3657–64. doi: 10.1007/s13197-018-3294-8
Keywords: probiotics, encapsulation, nutraceutical, functional food, viability
Citation: Singh S, Gupta R, Chawla S, Gauba P, Singh M, Tiwari RK, Upadhyay S, Sharma S, Chanda S and Gaur S (2022) Natural sources and encapsulating materials for probiotics delivery systems: Recent applications and challenges in functional food development. Front. Nutr. 9:971784. doi: 10.3389/fnut.2022.971784
Received: 20 June 2022; Accepted: 17 August 2022;
Published: 21 September 2022.
Edited by:
Kandi Sridhar, Agrocampus Ouest, FranceReviewed by:
Suhas, Gurukul Kangri Vishwavidyalaya, IndiaPraveen Dikkala, Jawaharlal Nehru Technological University, Kakinada, India
Copyright © 2022 Singh, Gupta, Chawla, Gauba, Singh, Tiwari, Upadhyay, Sharma, Chanda and Gaur. This is an open-access article distributed under the terms of the Creative Commons Attribution License (CC BY). The use, distribution or reproduction in other forums is permitted, provided the original author(s) and the copyright owner(s) are credited and that the original publication in this journal is cited, in accordance with accepted academic practice. No use, distribution or reproduction is permitted which does not comply with these terms.
*Correspondence: Smriti Gaur, smriti.gaur@jiit.ac.in