- 1Department of Experimental and Clinical Medicine, University of Florence, Florence, Italy
- 2Department of Biomedical, Experimental and Clinical Sciences “Mario Serio”, University of Florence, Florence, Italy
- 3Department of Neurosciences, Psychology, Drug Research and Child Health, University of Florence, Florence, Italy
- 4Department of Agrifood Production and Environmental Sciences, University of Florence, Florence, Italy
- 5Interdisciplinary Internal Medicine Unit, Careggi University Hospital, Florence, Italy
- 6Unit of Clinical Nutrition, Careggi University Hospital, Florence, Italy
Background and aim: In recent years, many studies have suggested that ancient wheat products might have beneficial effects on cardiometabolic risk profile, but little is known about their effect on gut microbiota (GM). The aim of the present study was to evaluate whether a replacement diet with pasta made from ancient wheat (AD) could influence the GM composition and its metabolites’ production compared to a replacement diet with pasta made from modern wheat (CD).
Methods: A randomized, double-blinded crossover trial with two intervention phases was conducted on 20 clinically healthy adults (9 females; 11 males; mean age 43.1 ± 12.5 years). Study participants were assigned to consume pasta made using semi-whole flour from organic wheat that was either from ancient or modern control wheat for 8 weeks in a random order. An 8-week washout period was implemented between the interventions. Stool samples were collected from all subjects at the beginning and at the end of each intervention period. GM composition, and short- (SCFAs) and medium- chain fatty acids (MCFAs) production was evaluated.
Results: Dietary interventions did not produce significant diversity in the GM composition at higher ranks (phylum, class, order and family), but only at genus level. In detail, the AD significantly (adj. p < 0.05) changed the abundance of Erysipelatoclostridium spp., Bacteroides_pectinophilus_group spp., CAG-873 spp., and Holdemanella spp. The CD significantly affected the abundance of Akkermansia spp., CAG-873 spp., Hungatella spp., Lachnospiraceae_UCG-008 spp., NK4A214_group spp., Frisingicoccus spp., Megasphaera spp., Synergistes spp., and Tyzzerella spp. Regarding the production of SCFAs and MCFAs, AD resulted in a significant increase of fecal acetic (+0.7%), isobutyric (+30.1%), 2-methylbutyric (+64.2%), and isovaleric (+22.5%) acids. On the other hand, CD resulted in increased levels of isobutyric (+71.4%), 2-methylbutyric (+116.2%), isovaleric (+99%), and valeric (+21.4%) acids, and a reduction of butyric (-31.6%) and hexanoic (-66.4%) acids.
Conclusion: A short-term replacement diet with both ancient and modern wheat pasta determined significant changes in GM composition at the genus level but notably the AD resulted in a greater beneficial impact on anti-inflammatory SCFAs.
Introduction
Wheat (Triticum spp.), the second most produced cereal grain after maize, currently represents the main staple food in many countries, providing a fifth of dietary calories and protein in human diets globally (1). In addition to being an important source of micronutrients, fiber and beneficial phytochemicals, several epidemiological studies have demonstrated an association between whole-wheat consumption and reduced risk of non-communicable diseases (e.g., cardiovascular disease, type 2 diabetes, and some cancers, including colorectal and pancreatic)(2, 3).
Nevertheless, in the last years, many concerns have been raised about grain products because of their potential association with increased cases of food allergy and intolerances, especially autoimmune responses to gluten like celiac disease and related conditions (dermatitis herpetiformis and gluten ataxia), non-celiac gluten (orwheat) sensitivity, and irritable bowel syndrome associated with non-digestible carbohydrates (4–6). Therefore, ancient wheat cultivars (e.g., einkorn, spelt, emmer, Khorasan) have recently been in the spotlight as several clinical studies have suggested that they may represent a healthier choice than modern ones. The main explicative reasons are the reduced presence of immunoreactive products, with fewer amounts and types of reactive prolamins and fructans, and the higher content of minerals and nutraceutical compounds (7, 8).
Although recent studies have documented the beneficial effects of consuming ancient wheat products on pro-inflammatory/antioxidant parameters, as well as on cardiometabolic risk profile and fibromyalgia symptomatology (9) few studies have evaluated their effect on gut microbiota (GM) (8, 10). GM is involved in several metabolic processes and its susceptibility to the general food quality and the specific quality of wheat is well established (11). In particular, considering that GM plays a pivotal role in gut-associated immune system homeostasis, it has been demonstrated that wheat peptides, only partially digested by intestinal proteases, can have an increased or reduced immunogenicity depending on their degrading bacteria, influencing the possibility of developing wheat-related disorders (12). Another core GM activity is carbohydrate fermentation with the consequent production of short- (SCFAs) and medium-chain fatty acids (MCFAs), critical metabolites that can regulate intestinal physiology and modulate immune functions, as well as serving as energy source for colonocytes or other resident bacteria (13).
Therefore, the aim of the present study was to examine whether a replacement diet with pasta made with ancient wheat (AD) could influence the GM composition and its metabolite profile compared to a replacement diet with pasta made from modern wheat (CD).
Materials and methods
Study participants
All the participants were recruited from the Unit of Clinical Nutrition of the Careggi University Hospital, Florence, Italy. The study population was comprised of 20 volunteers (9 females; 11 males). Inclusion criteria to participate in the study were being between 18 and 65 years of age, being in good general health, and not having gastrointestinal disorders (e.g., chronic constipation, diarrhea, inflammatory bowel disease, irritable bowel syndrome, or other chronic gastrointestinal disorders). Subjects were excluded if they were taking medications or probiotics and similar (prebiotics or symbiotics) for any reason, were pregnant or lactating, was affected by celiac disease and non-celiac gluten (or wheat) sensitivity or had a serious illness or unstable condition. The study procedures were approved by the Ethics Committee of the Tuscany Region, Careggi University Hospital and followed the principles of the Declaration of Helsinki. Written informed consent was obtained from all participants before the start of the trial.
Wheat varieties
The ancient wheat used in the present study was organic Evoldur1, an evolutionary population of durum wheat obtained by crossing 10 ancient Sicilian varieties: Russello, Ruscia, Tunisina, Scavuzza, Urria, Inglesa, Scorza Nera, Crotone, Chiattulidda, and Bidì and two turanic wheat varieties from the USDA collection (PI 125351 and PI 337643). The modern wheat used as control was organic ZetaE. The wheat varieties were grown at four farms that participated in the Tuscany Region-funded GrantSoilBiofert project, aimed at identifying agronomic techniques and selection models to obtain wheat suitable for the soil and climatic conditions of Tuscany (Sottomisura 16.2 - RDP 2014-2020). The farms were Azienda agricola Cini Francesco - il poderino di Asciano (Asciano, Siena), Azienda agricola Vecchioni Giovanna - Tenuta il tesorino (Follonica, Grosseto), Azienda agricola il Sorbo (Granaione, Grosseto), and Azienda agricola Tenute di Fraternita (Pieve al Toppo, Arezzo). The Pastificio Artigiano FABBRI s.a.s. (Strada in Chianti, Firenze) prepared the pasta (with no additives) from both the ancient and modern semolina, according to the artisan manufacturing procedures. To standardize the comparison, all the transformation procedures were identical for both the ancient and the modern wheat.
Study design
The study was a randomized, double-blinded crossover trial with two intervention phases each lasting 8 weeks. After a run-in period of 2-weeks, the participants were divided into two groups which were respectively assigned to consume either ancient wheat (AD group) or modern wheat pasta (CD group) in a random order. An 8-week washout period was implemented between the interventions, in which participants were allowed to eat all foods according to their usual eating habits. In the second intervention period, the group assigned to consume the ancient wheat pasta in the first intervention period was assigned to consume the modern wheat pasta, and vice versa. Participants in both groups received 800 g per week of pasta, with no labels attached to the packages, and were informed that the products were organic and prepared by artisan methods. They were instructed to exclude other type of pasta from their respective diets, which was then “replaced” by ancient or modern pasta during the intervention phases. They were also advised to eat the pasta according to their normal consumption habits, and not to alter their dietary or lifestyle habits.
Both at the beginning and at the end of each intervention period, study participants were evaluated through objective examinations. General information about demographics, personal medical history and use of antibiotics or probiotics in the previous six months were collected. Weight and height were measured using a stadiometer. Body mass index (BMI) was calculated as the weight (kg)/height (m2). Stool samples were collected at the beginning and at the end of each intervention period to evaluate GM composition, SCFAs and MCFAs production.
Gut microbiota characterization
Total DNA was extracted using the DNeasy PowerSoil Pro Kit (Qiagen, Hilden, Germany) from frozen (-80°C) stool samples, according to the manufacturer’s instructions. Briefly, 0.25 g of stool samples were added to a bead beating tube and homogenized with TissueLyser LT (Qiagen, Hilden, Germany) for 5min at 50 Hz. Afterward, DNA was captured on a silica membrane in a spin column format, washed and eluted. The quality and quantity of extracted DNA was assessed with both NanoDrop ND-1000 (Thermo Fisher Scientific, Waltham, MA, United States) and Qubit Fluorometer (Thermo Fisher Scientific, Waltham, MA, United States) and then it was frozen at -20°C. Subsequently, genomic DNA samples were sent to IGA Technology Services (Udine, Italy) where amplicons of the variable V3–V4 region of the bacterial 16S rRNA gene were sequenced in paired-end (2 × 300 cycles) on the Illumina MiSeq platform, according to the Illumina 16S Metagenomic Sequencing Library Preparation protocol.
Demultiplexed sequence reads were processed using QIIME2 2021.4 (14). The sequencing primers were removed using Cutadapt tool (15) while DADA2 (16) was used to perform paired-end reads merging, filtering and chimeras removal steps after trimming nucleotides from forward and reverse reads based on the quality profiles (–p-trunc-len-f 241 and –p-trunc-len-r 201). Hence, amplicon sequence variants (ASVs) were generated and the VSEARCH tool (17) was used for taxonomic assignment using the SILVA database (release 138) as reference, with a 0.99 identity threshold.
Analysis of fecal SCFAs and MCFAs by gas chromatography-mass spectrometry
The qualitative and quantitative evaluation of fecal SCFAs and MCFAs was performed by Agilent gas chromatography-mass spectrometry (GC-MS) system composed with 5971 single quadrupole mass spectrometer, 5890 gas chromatograph and 7673 autosampler, through our previously described GC-MS method (18).
Briefly, just before the analysis, stool samples were thawed and added with sodium bicarbonate 0.25 mM solution (1:1 w/v) in a 1.5 mL centrifuge tube. Then, the obtained suspensions were sonicated for 5 minutes, centrifuged at 5000 rpm for 10 minutes and then the supernatants were collected. The SCFAs were finally extracted as follow: an aliquot of 100μL of sample solution (corresponding to 0.1 mg of stool sample) was added of 50 μL of internal standards mixture, 1 mL of tert-butyl methyl ether and 50 μL of HCl 6 M + 0.5 M NaCl solution in a 1.5 mL centrifuge tube. Subsequently, each tube was shaken in a vortex apparatus for 2 min, centrifuged at 10,000 rpm for 5 min, and lastly the solvent layer was transferred to an autosampler vial and processed three times.
Statistical analysis
Statistical analyses on the bacterial communities were performed in R 4.1.0 with the help of the packages phyloseq 1.38.0, DESeq2 1.32.0 and other packages satisfying their dependencies as vegan 2.5-7. Packages ggplot2 3.3.5 and dendextend 1.15.2 were used to plot data and results. Data were reported as mean ± standard deviation (SD), number and percentage, or median percentage and interquartile range (IQR), as appropriate. Shannon index, Observed ASV richness and Pielou’s evenness were used to estimate bacterial diversity in each sample using the function estimate_richness from phyloseq. The Pielou’s evenness index was calculated using the formula E = S/log(R), where S is the Shannon diversity index and R is the number of ASVs in the sample. Differences in all indices were tested using the Wilcoxon test. PCoAs were performed on proportional count data of each sample, adjusted with square root transformation. At the different taxonomic ranks, the differential analyses of abundance were performed with DESeq2 on raw ASVs data (created using the tax_glom function in phyloseq). In addition, differential abundances of predicted pathways were determined and displayed using linear discriminant analysis (LDA) effect size (LefSe).
Furthermore, the software GraphPad Prism (v.5) was used for the statistical analysis of the fecal SCFAs’ and MCFA’s composition between pre- and post-AD samples and between pre- and post-CD samples. Pairwise differences between groups were assessed using Wilcoxon signed-rank test and p-values less than 0.05 were considered statically significant.
Results
Characteristics of the study population
The mean age of the study population was 43.1 ± 12.5 years. Body weight was 66.3 ± 14.1 kg, while BMI was 23.0 ± 2.7 kg/m2. As shown in Figure 1, 10 participants (6F; 4M) with a mean age of 46.8 ± 14.1 years and a BMI of 24.0 ± 3.0 kg/m2 were assigned to consume ancient wheat pasta (AD group), while the other ten participants (5F; 5M) with a mean age of 39.4 ± 10.0 years and a BMI of 22.0 ± 2.1 kg/m2 were assigned to consume modern wheat pasta (CD group). Subsequently, after the washout period, the study groups were crossed and, because of the retirement of two subjects, ten subjects (5F; 5M) consumed ancient wheat pasta and eight subjects (5F; 3M) consumed modern wheat pasta. No participants reported having used antibiotics or probiotics in the previous six months.
GM composition
First, we evaluated if the replacement diet with pasta made from ancient wheat and the replacement diet with pasta made from modern control wheat had an impact on the GM composition. As shown in Figure 2, no significant differences were reported on the different alpha diversity indices after both dietary interventions.
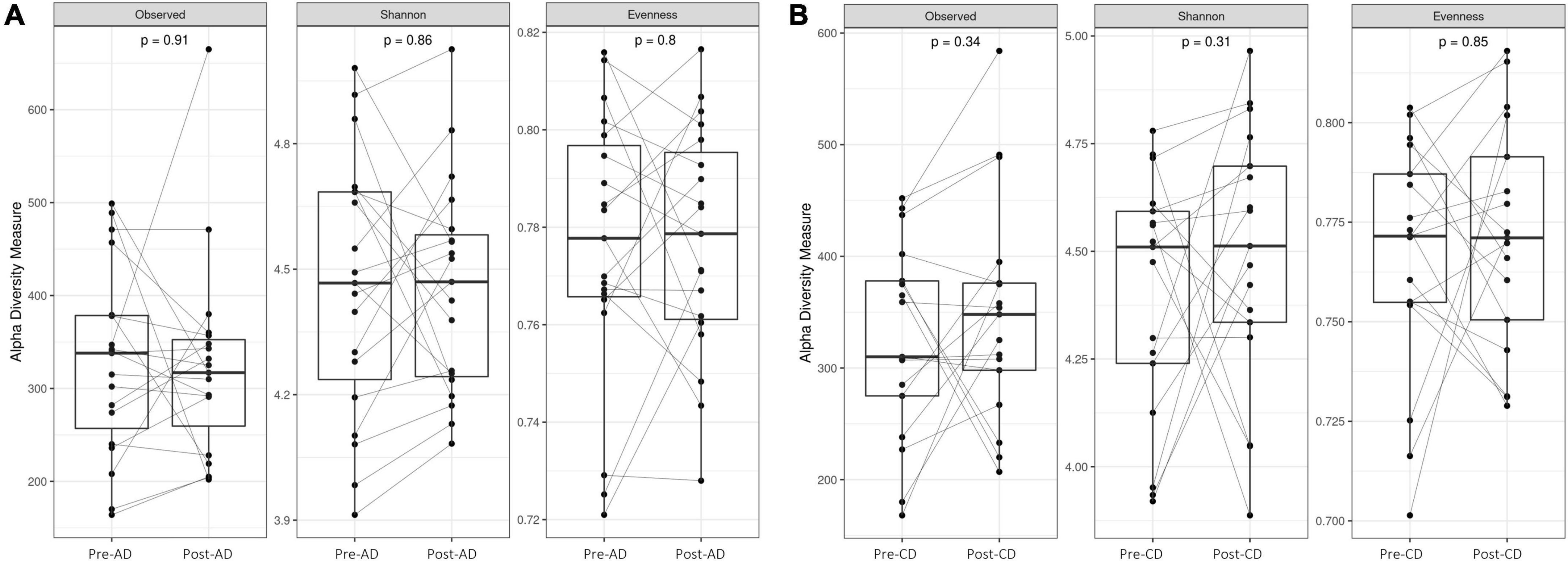
Figure 2. Box plots reporting alpha diversity indices (Observed ASV richness, Shannon index, Pielou’s evenness) pre- and post-AD (A) and pre- and post-CD (B). Lines link paired samples and statistical differences were assessed using the Wilcoxon signed-rank test. p-values less than 0.05 were considered statistically significant. AD, ancient-wheat diet; CD, control-wheat diet.
Similarly, beta diversity analysis, assessed through the Bray–Curtis dissimilarity metric, did not highlighted any clear separation at the genus level between the pre- and post-AD groups (Figure 3A) or among the pre- and post-CD groups (Figure 3B), neither at other taxonomic ranks. To evaluate the impact of both dietary interventions on microbial abundances, a taxonomic composition analysis was performed. While no significant changes were observed at phylum, class, order and family ranks, significant differences were observed at genus level. As shown in Figure 4A, AD determined a significant increase of Erysipelatoclostridium spp. (log2FC = 3.487; adj. p = 7.90e-4) and a significant decrease of [Bacteroides]_pectinophilus_group spp. (log2FC = -11.278; adj. p = 1.30e-4), CAG-873 spp. (log2FC = -25.205; adj. p = 1.60e-17), and Holdemanella spp. (log2FC = -12.099; adj. p = 3.73e-5). On the other hand, CD resulted in a significant increase in the abundance of Akkermansia spp. (log2FC = 3.183; adj. p = 0.015), CAG-873 spp. (log2FC = 19.923; adj. p = 9.64e-10), Hungatella spp. (log2FC = 2.205; adj. p = 0.040), Lachnospiraceae_UCG-008 spp. (log2FC = 2.709; adj. p = 0.026), and NK4A214_group spp. (log2FC = 8.969; adj. p = 0.040), and a decrease of Frisingicoccus spp. (log2FC = -23.318, adj. p = 2.96e-13), Megasphaera spp. (log2FC = -14.384; adj. p = 4.43e-05), Synergistes spp. (log2FC = -25.409; adj. p = 1.67e-15) and Tyzzerella spp. (log2FC = -10.946; adj. p = 0.006) (Figure 4B).
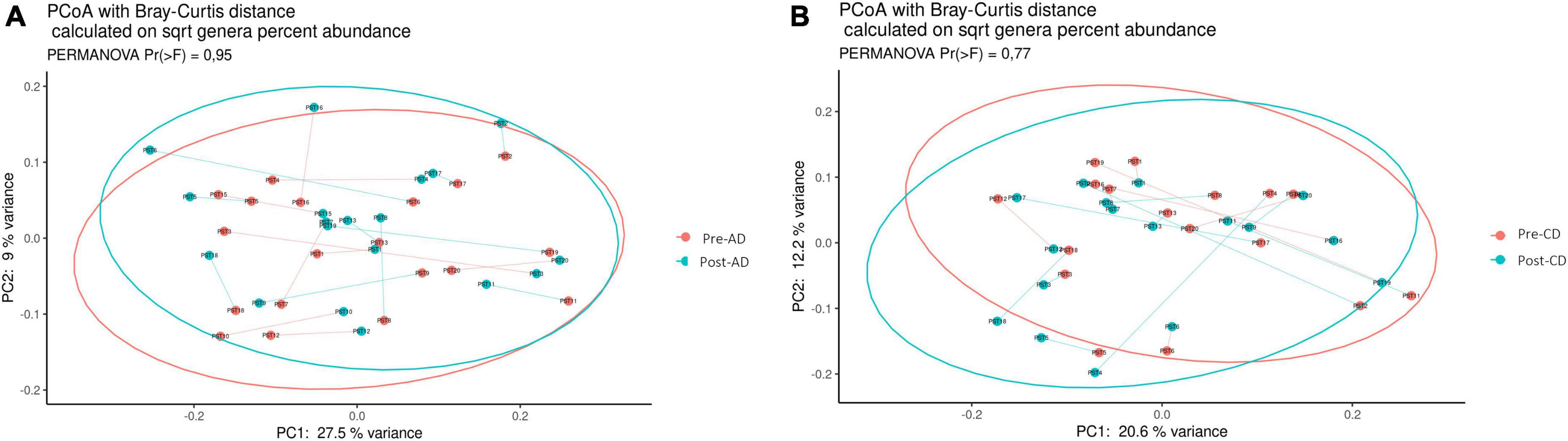
Figure 3. Principal coordinates analysis (PCoA), according to the Bray-Curtis beta-diversity metric, of pre- and post-AD samples (A) and pre- and post-CD samples (B). Results of the permutational multivariate analysis of variance (PERMANOVA) are also shown based on the first two coordinates. AD, ancient-wheat diet; CD, control-wheat diet.
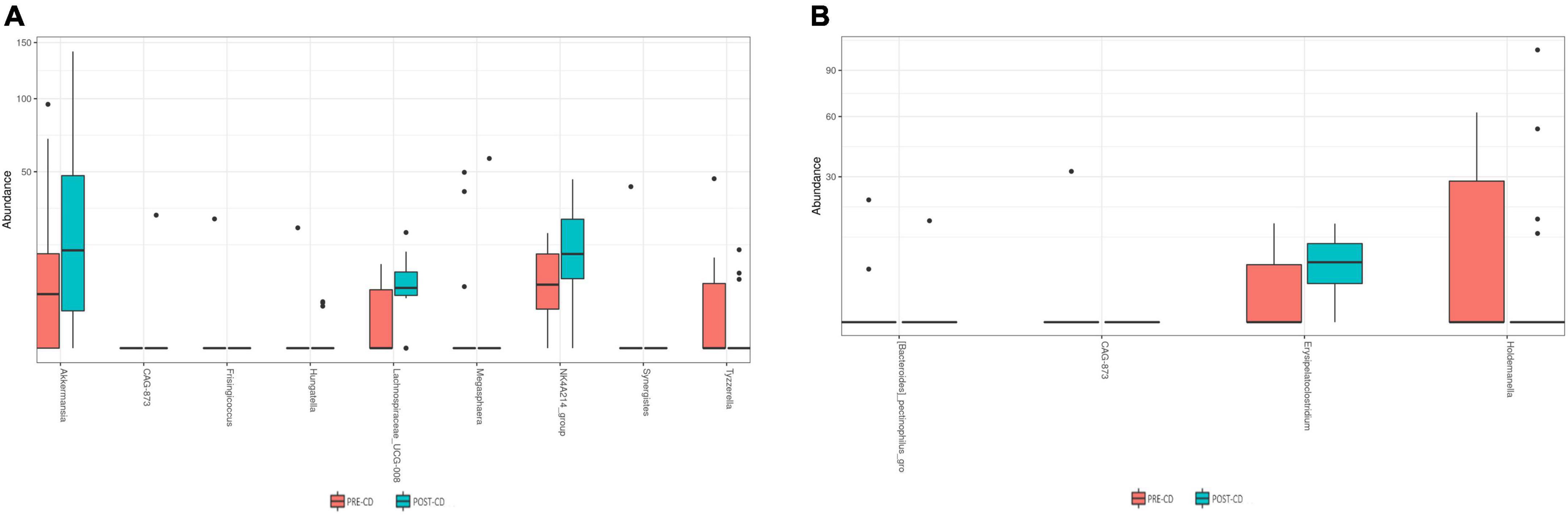
Figure 4. Box plots showing significant differentially abundant genera among pre- and post-AD (A) and pre- and post-CD (B) samples. AD, ancient-wheat diet; CD, control-wheat diet.
Fecal SCFAs and MCFAs profiles
The abundances of fecal SCFAs and MCFAs before and after both dietary interventions are reported in Table 1. In AD group, a significant increase (p < 0.05) was observed in the abundance of acetic (+0.7%), isobutyric (+30.1%), 2-methylbutyric (+64.2%), and isovaleric (+22.5%) acids. On the other hand, CD resulted in increased levels of isobutyric (+71.4%), 2-methylbutyric (+116.2%), isovaleric (+99%), and valeric (+21.4%) acids, and a reduction of butyric (-31.6%) and hexanoic (-66.4%) acids.
PICRUST analysis
Finally, the PICRUSt2 (phylogenetic investigation of communities by reconstruction of unobserved states) predictive metabolism approach (19) was used on the 16S rRNA gene sequencing data to assess functional and metabolic changes of microbial communities after both dietary interventions. Based on MetaCyc metabolic pathway database,1 we found no metabolic differences after AD, while CD determined a significant reduction of the pathway PWY-7254 (TCA_cycle_VII_acetate_producers_) (LDA score > 2.0; p = 0.046) (Figure 5).
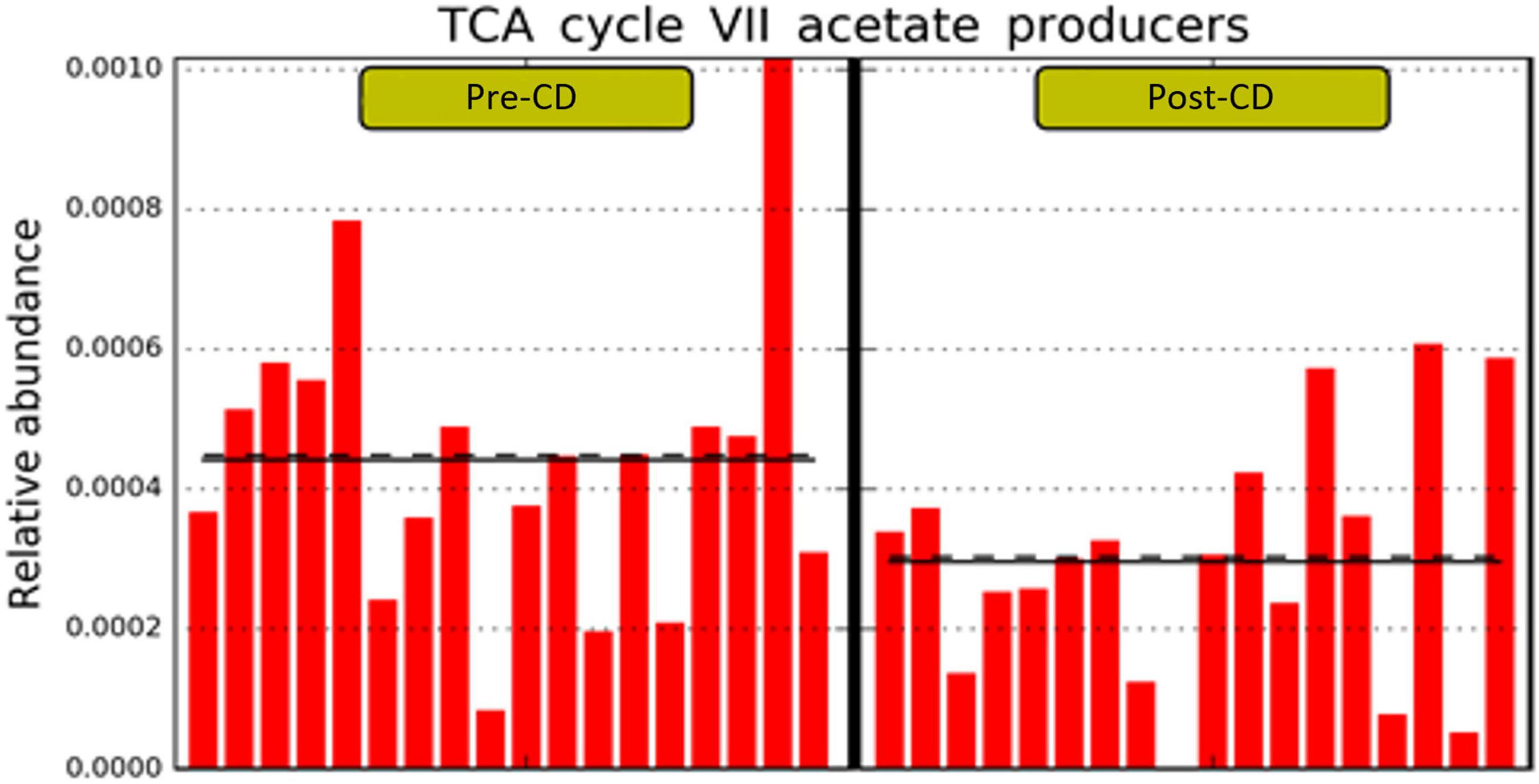
Figure 5. Statistically significant different predicted pathway with LDA score > 2.0 between pre- and post-CD. LDA, linear discriminant analysis; CD, control wheat diet.
Discussion
Ancient wheat varieties have gained increasing interest for their nutritional profile but especially for the supposed ability to reduce the risk of chronic diseases compared with modern wheat. Nevertheless, the mechanisms by which they confer protective effects on human health and their potential influence on GM composition are still unclear. In this scenario, we have conducted randomized, double-blind crossover trial to explore if a replacement diet with pasta made with ancient wheat could modify the GM composition and production of bacterial metabolites, such as SCFAs and MCFAs, compared to a replacement diet with pasta made from modern wheat.
In agreement with Saa and colleagues (20), who found no changes in the GM composition of healthy volunteers after a 3-month diet with Khorasan wheat or whole durum wheat, we observed that the two interventions did not significantly modify either the structure or the microbial diversity. In addition, Carroccio and colleagues recently found no statistically significant differences in alpha diversity indices after a 30-day intervention with ancient and modern wheat-based foods (21). These findings are not surprising, since a considerable GM stability and resilience have also been demonstrated with other nutritional interventions (22).
Some significant variations, on the other hand, have been reported at genus level. The ancient wheat pasta administration determined a significant increase of Erysipelatoclostridium spp. and a significant decrease of the genera [Bacteroides]_pectinophilus_group, CAG-873 and Holdemanella. In this regard, Martin-Gallausiaux and colleagues have documented that members of genus Erysipelatoclostridium are bacteria producing butyrate, a crucial SCFA with renowned and potent anti-inflammatory properties (23). In addition, the [Bacteroides]_pectinophilus_group is well known as a genus constituted by complex carbohydrate degrading bacteria (24). As to Holdemanella spp., Pujo and collaborators showed its anti-inflammatory properties in ulcerative colitis patients (25).
Regarding the control pasta intervention, it determined a significant increase of Akkermansia spp., CAG-87 spp., Hungatella spp., Lachnospiraceae_UCG-008 spp., and NK4A214_group spp. and a significant decrease of the genera Frisingicoccus, Megasphaera, Synergistes, and Tyzzerella. According to recent findings, Akkermansia spp. may exert anti-inflammatory properties in the intestinal tract, and enhances the intestinal barrier integrity and function (26); on the contrary, the genus Hungatella, which includes trimethylamine-producers members, have been associated with cardiovascular diseases (27). Also increased levels of Lachnospiraceae_UCG-008 spp. Tyzzerella spp. have been associated with chronic intestinal inflammation (28, 29), while a high abundance of Frisingicoccus spp. have been reported in patients affected by Parkinson’s disease (30).
Furthermore, we evaluated the production of bacteria-derived metabolites, by analyzing fecal SCFAs and MCFAs profiles. As is well known, the SCFAs are mainly produced by bacterial anaerobic fermentation of indigestible polysaccharides and can either be absorbed by the colonic epithelium providing energy or enter the bloodstream and play a relevant role in the regulation of fatty acids, glucose, and cholesterol metabolism (31). In addition, they mediate the beneficial effects of dietary fiber, i.e., protection against cardiovascular disease, colorectal cancer, obesity, and diabetes (32). According to recent studies, branched SCFAs such as isovaleric and isobutyric acids may also exert a beneficial influence on host metabolism and especially immunity, preventing inflammatory diseases and metabolic disorders (33). In our study, the ancient wheat pasta intervention caused a significant increase of acetic, isobutyric, 2-methylbutyric and isovaleric acids. These findings are in agreement with those of the, previously reported, study of Saa and colleagues documenting an increased SCFAs’ levels after a 3-month Khorasan based diet in healthy individuals (20). In addition, we have previously documented increased SCFAs’ production in patients with fibromyalgia syndrome who consumed Khorasan wheat (34). Rios-Covian and colleagues, on the other hand, reported an association between branched SCFAs and lower fiber consumption, hypothesizing that the reduced availability of fermentable carbohydrates by the GM promoted a shift to increased protein fermentation (35). Regarding control modern wheat pasta, the intervention resulted in increased levels of isobutyric, 2-methylbutyric, isovaleric and valeric acids, and a reduction of hexanoic and butyric acids. Notably, butyric acid is one of the most abundant SCFAs produced by intestinal bacteria that, being (i) a potent activator of both G protein-coupled receptors (35) and peroxisome proliferator-activated receptors (36) and (ii) a potent inhibitor of histone deacetylases (37), exerts multiple beneficial effects such as the modulation of visceral sensitivity and immune responses, and the expression regulation of some critical genes (36).
Finally, we used the PICRUSt2 to assess eventual functional change of microbial communities after both dietary interventions. While no metabolic differences were found with the ancient wheat pasta, a significant reduction of the TCA_cycle_VII_acetate_producers_ pathway was observed with the modern wheat pasta. The TCA (tricarboxylic acid cycle) pathway is usually used as a reference for its central role in energy generation. Indeed, it is present in most aerobic living organisms that use this cycle to generate NADH and FADH2 and utilize these molecules as the reductive potential required for ATP synthesis during oxidative phosphorylation (37). In contrast to our findings, Carroccio and colleagues showed that the GM ability to metabolize carbohydrates increased only after a diet containing ancient grain products, and not with a modern wheat-based diet (21).
Surely our study has some limitations, such as the restricted number of participants, the limited study duration, and the low taxonomical resolution of 16S rRNA sequencing. Furthermore, it was not possible to consider any lifestyle changes that might have affected the study, even though participants were under strict instruction to maintain their usual lifestyle patterns while consuming ancient and modern wheat pasta. We are aware that 8 weeks of intervention is a limited period and permits only the suggestion of the possible interpretation of the results. However, the present study shows different strengths, including the double-blind randomized cross-over design, the good compliance of participants to the assigned diets, and finally the fact that data on ancient wheat consumption and GM modifications are currently very uncommon.
In conclusion, a short-term replacement diet with ancient and modern wheat pasta did not modify either the structure or the microbial diversity of gut microbiota. However, both interventions determined significant changes in GM composition at the genus level. As to bacteria-derived metabolites, the administration of ancient wheat pasta resulted in a greater beneficial impact on anti-inflammatory SCFAs. Anyway, further studies with larger number of subjects and longer periods of dietary intervention are needed to confirm these preliminary results.
Data availability statement
The data presented in this study are deposited in the NCBI Gene Expression Omnibus (GEO) repository, accession number GSE206807.
Ethics statement
The studies involving human participants were reviewed and approved by Ethics Committee of the Tuscany Region, Careggi University Hospital. The patients/participants provided their written informed consent to participate in this study.
Author contributions
SBa, MD, AA, and FS conceived and designed the study and drafted the manuscript. SBa and LC acquired experimental data of microbiota. MD, GP, BC, and FS were involved in subjects’ enrolment. SBa, MP, and GP performed gas chromatography-mass spectrometer analysis. LD and MR performed bioinformatic and statistical analysis of microbiota. SBa, MD, GP, BC, LC, LD MP, MR, and GB analyzed and interpreted the data. SBe, AA, and FS critically revised the manuscript. All authors contributed to the article and approved the submitted version.
Funding
This research received a grant from the Tuscany Region PSR Toscana 2014-2020 – Progetti Integrati di Filiera (PIF) Agroalimentare, Sottomisura 16.2. Project: “Tecniche Agronomiche e modelli di Selezione per ottenere frumenti adatti alle condizioni pedo-climatiche della Toscana” – GrAnt SoilBioFert. Moreover, it was also supported by MUR under the umbrella of the European Joint Program Initiative “A Healthy Diet for a Healthy Life” (JPI–HDHL) and of the ERA–NET Cofund ERA–HDHL, ID: 1523 (GA N 696295 of the EU HORIZON 2020 Research and Innovation Programme).
Conflict of interest
The authors declare that the research was conducted in the absence of any commercial or financial relationships that could be construed as a potential conflict of interest.
Publisher’s note
All claims expressed in this article are solely those of the authors and do not necessarily represent those of their affiliated organizations, or those of the publisher, the editors and the reviewers. Any product that may be evaluated in this article, or claim that may be made by its manufacturer, is not guaranteed or endorsed by the publisher.
Footnotes
References
1. Shiferaw B, Smale M, Braun H-J, Duveiller E, Reynolds M, Muricho G. Crops that feed the world 10. Past successes and future challenges to the role played by wheat in global food security. Food Secur. (2013) 5:291–317.
2. Reynolds A, Mann J, Cummings J, Winter N, Mete E, Te Morenga L. Carbohydrate quality and human health: a series of systematic reviews and meta-analyses. Lancet. (2019) 393:434–45. doi: 10.1016/S0140-6736(18)31809-9
3. Marshall S, Petocz P, Duve E, Abbott K, Cassettari T, Blumfield M, et al. The effect of replacing refined grains with whole grains on cardiovascular risk factors: a systematic review and meta-analysis of randomized controlled trials with GRADE clinical recommendation. J Acad Nutr Diet. (2020) 120:1859–83.e31.
4. Bradauskiene V, Vaiciulyte-Funk L, Martinaitiene D, Andruskiene J, Verma AK, Lima JPM, et al. Wheat consumption and prevalence of celiac disease: correlation from a multilevel analysis. Crit Rev Food Sci Nutr. (2021) 62:1–15. doi: 10.1080/10408398.2021.1939650
5. Gibson PR, Varney J, Malakar S, Muir JG. Food components and irritable bowel syndrome. Gastroenterology. (2015) 148:1158–74.e4.
6. Brouns F, van Rooy G, Shewry P, Rustgi S, Jonkers D. Adverse reactions to wheat or wheat components. Compr Rev Food Sci Food Saf. (2019) 18:1437–52.
7. Shewry PR. Do ancient types of wheat have health benefits compared with modern bread wheat? J Cereal Sci. (2018) 79:469–76.
8. Dinu M, Whittaker A, Pagliai G, Benedettelli S, Sofi F. Ancient wheat species and human health: biochemical and clinical implications. J Nutr Biochem. (2018) 52:1–9. doi: 10.1016/j.jnutbio.2017.09.001
9. Baldi S, Pagliai G, Dinu M, Di Gloria L, Nannini G, Curini L, et al. Effect of ancient Khorasan wheat on gut microbiota, inflammation, and short-chain fatty acid production in patients with fibromyalgia. World J Gastroenterol. (2022) 28:1965–80. doi: 10.3748/wjg.v28.i18.1965
10. Pagliai G, Venturi M, Dinu M, Galli V, Colombini B, Giangrandi I, et al. Effect of consumption of ancient grain bread leavened with sourdough or with baker’s yeast on cardio-metabolic risk parameters: a dietary intervention trial. Int J Food Sci Nutr. (2021) 72:367–74. doi: 10.1080/09637486.2020.1799956
11. Spisni E, Imbesi V, Giovanardi E, Petrocelli G, Alvisi P, Valerii MC. Differential physiological responses elicited by ancient and heritage wheat cultivars compared to modern ones. Nutrients. (2019) 11:2879. doi: 10.3390/nu11122879
12. Cristofori F, Francavilla R, Capobianco D, Dargenio VN, Filardo S, Mastromarino P. Bacterial-based strategies to hydrolyze gluten peptides and protect intestinal mucosa. Front Immunol. (2020) 11:567801. doi: 10.3389/fimmu.2020.567801
13. Marchesi JR, Adams DH, Fava F, Hermes GD, Hirschfield GM, Hold G, et al. The gut microbiota and host health: a new clinical frontier. Gut. (2016) 65:330–9. doi: 10.1136/gutjnl-2015-309990
14. Bolyen E, Rideout JR, Dillon MR, Bokulich NA, Abnet CC, Al-Ghalith GA, et al. Reproducible, interactive, scalable and extensible microbiome data science using QIIME 2. Nat Biotechnol. (2019) 37:852–7.
15. Martin M. Cutadapt removes adapter sequences from high-throughput sequencing reads. EMBnet J. (2011) 17:10–2. doi: 10.1089/cmb.2017.0096
16. Callahan BJ, McMurdie PJ, Rosen MJ, Han AW, Johnson AJ, Holmes SP. DADA2: High-resolution sample inference from Illumina amplicon data. Nat Methods. (2016) 13:581–3.
17. Rognes T, Flouri T, Nichols B, Quince C, Mahé F. VSEARCH: a versatile open source tool for metagenomics. PeerJ. (2016) 4:e2584. doi: 10.7717/peerj.2584
18. Niccolai E, Baldi S, Ricci F, Russo E, Nannini G, Menicatti M, et al. Evaluation and comparison of short chain fatty acids composition in gut diseases. World J Gastroenterol. (2019) 25:5543–58.
19. Douglas GM, Maffei VJ, Zaneveld JR, Yurgel SN, Brown JR, Taylor CM, et al. PICRUSt2 for prediction of metagenome functions. Nat Biotechnol. (2020) 38:685–8.
20. Taneyo Saa D, Turroni S, Serrazanetti DI, Rampelli S, Maccaferri S, Candela M, et al. Impact of Kamut® Khorasan on gut microbiota and metabolome in healthy volunteers. Food Res Int. (2014) 63:227–32.
21. Carroccio A, Celano G, Cottone C, Di Sclafani G, Vannini L, D’Alcamo A, et al. WHOLE-meal ancient wheat-based diet: effect on metabolic parameters and microbiota. Dig Liver Dis. (2021) 53:1412–21. doi: 10.1016/j.dld.2021.04.026
22. Fassarella M, Blaak EE, Penders J, Nauta A, Smidt H, Zoetendal EG. Gut microbiome stability and resilience: elucidating the response to perturbations in order to modulate gut health. Gut. (2021) 70:595–605. doi: 10.1136/gutjnl-2020-321747
23. Martin-Gallausiaux C, Marinelli L, Blottière HM, Larraufie P, Lapaque N. SCFA: mechanisms and functional importance in the gut. Proc Nutr Soc. (2021) 80:37–49.
24. Hullar MAJ, Jenkins IC, Randolph TW, Curtis KR, Monroe KR, Ernst T, et al. Associations of the gut microbiome with hepatic adiposity in the multiethnic cohort adiposity phenotype study. Gut Microbes. (2021) 13:1965463. doi: 10.1080/19490976.2021.1965463
25. Pujo J, Petitfils C, Le Faouder P, Eeckhaut V, Payros G, Maurel S, et al. Bacteria-derived long chain fatty acid exhibits anti-inflammatory properties in colitis. Gut. (2021) 70:1088–97. doi: 10.1136/gutjnl-2020-321173
26. Naito Y, Uchiyama K, Takagi T. A next-generation beneficial microbe: Akkermansia muciniphila. J Clin Biochem Nutr. (2018) 63:33–5. doi: 10.3164/jcbn.18-57
27. Ramireddy L, Tsen HY, Chiang YC, Hung CY, Chen FC, Yen HT. The gene expression and bioinformatic analysis of choline trimethylamine-lyase (CutC) and its activating enzyme (CutD) for gut microbes and comparison with their TMA production levels. Curr Res Microb Sci. (2021) 2:100043. doi: 10.1016/j.crmicr.2021.100043
28. Vacca M, Celano G, Calabrese FM, Portincasa P, Gobbetti M, De Angelis M. The Controversial role of human gut Lachnospiraceae. Microorganisms. (2020) 8:573. doi: 10.3390/microorganisms8040573
29. Chen YJ, Wu H, Wu SD, Lu N, Wang YT, Liu HN, et al. Parasutterella, in association with irritable bowel syndrome and intestinal chronic inflammation. J Gastroenterol Hepatol. (2018) 33:1844–52. doi: 10.1111/jgh.14281
30. Romano S, Savva GM, Bedarf JR, Charles IG, Hildebrand F, Narbad A. Meta-analysis of the Parkinson’s disease gut microbiome suggests alterations linked to intestinal inflammation. NPJ Parkinsons Dis. (2021) 7:27. doi: 10.1038/s41531-021-00156-z
31. Natarajan N, Pluznick JL. From microbe to man: the role of microbial short chain fatty acid metabolites in host cell biology. Am J Physiol Cell Physiol. (2014) 307:C979–85. doi: 10.1152/ajpcell.00228.2014
32. Cani PD. Human gut microbiome: hopes, threats and promises. Gut. (2018) 67:1716–25. doi: 10.1136/gutjnl-2018-316723
33. Taormina VM, Unger AL, Schiksnis MR, Torres-Gonzalez M, Kraft J. Branched-chain fatty acids-an underexplored class of dairy-derived fatty acids. Nutrients. (2020) 12:2875. doi: 10.3390/nu12092875
34. Pagliai G, Colombini B, Dinu M, Whittaker A, Masoni A, Danza G, et al. Effectiveness of a Khorasan wheat-based replacement on pain symptoms and quality of life in patients with fibromyalgia. Pain Med. (2020) 21:2366–72. doi: 10.1093/pm/pnaa134
35. Rios-Covian D, González S, Nogacka AM, Arboleya S, Salazar N, Gueimonde M, et al. An overview on fecal branched short-chain fatty acids along human life and as related with body mass index: associated dietary and anthropometric factors. Front Microbiol. (2020) 11:973. doi: 10.3389/fmicb.2020.0097
36. D’Souza WN, Douangpanya J, Mu S, Jaeckel P, Zhang M, Maxwell JR, et al. Differing roles for short chain fatty acids and GPR43 agonism in the regulation of intestinal barrier function and immune responses. PLoS One. (2017) 12:e0180190. doi: 10.1371/journal.pone.0180190
Keywords: gut microbiota, ancient wheat, modern wheat, SCFAs, MCFAs, pasta
Citation: Baldi S, Dinu M, Pagliai G, Colombini B, Di Gloria L, Curini L, Pallecchi M, Ramazzotti M, Bartolucci G, Benedettelli S, Amedei A and Sofi F (2022) Effect of ancient wheat pasta on gut microbiota composition and bacteria-derived metabolites: A randomized controlled trial. Front. Nutr. 9:971666. doi: 10.3389/fnut.2022.971666
Received: 17 June 2022; Accepted: 01 July 2022;
Published: 04 August 2022.
Edited by:
Reza Rastmanesh, American Physical Society, United StatesReviewed by:
Maria Chiara Valerii, AOU S. Orsola-Malpighi, ItalyEnzo Spisni, University of Bologna, Italy
Copyright © 2022 Baldi, Dinu, Pagliai, Colombini, Di Gloria, Curini, Pallecchi, Ramazzotti, Bartolucci, Benedettelli, Amedei and Sofi. This is an open-access article distributed under the terms of the Creative Commons Attribution License (CC BY). The use, distribution or reproduction in other forums is permitted, provided the original author(s) and the copyright owner(s) are credited and that the original publication in this journal is cited, in accordance with accepted academic practice. No use, distribution or reproduction is permitted which does not comply with these terms.
*Correspondence: Amedeo Amedei, aamedei@unifi.it; Francesco Sofi, francesco.sofi@unifi.it
†These authors have contributed equally to this work and share first authorship
‡These authors have contributed equally to this work and share senior authorship