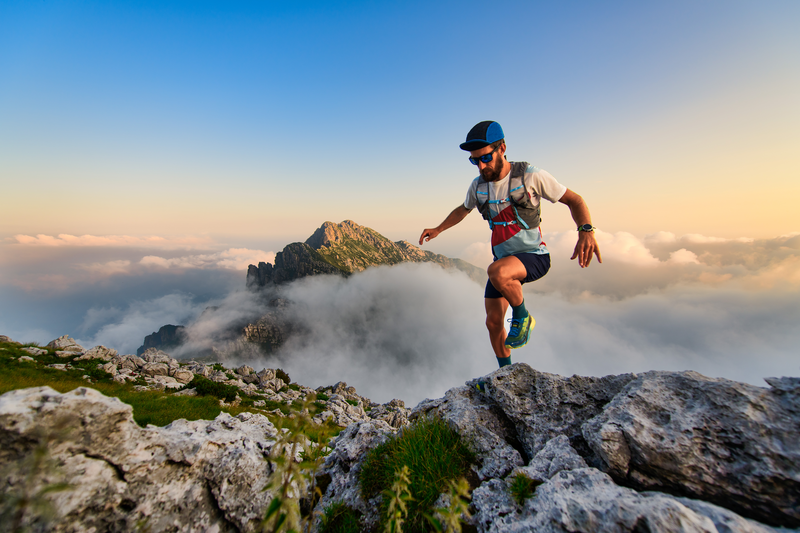
94% of researchers rate our articles as excellent or good
Learn more about the work of our research integrity team to safeguard the quality of each article we publish.
Find out more
ORIGINAL RESEARCH article
Front. Nutr. , 02 September 2022
Sec. Clinical Nutrition
Volume 9 - 2022 | https://doi.org/10.3389/fnut.2022.970729
Background: Increasing life expectancy of coronary artery bypass grafting (CABG) remains to be the major concern of cardiac surgeons. However, few studies have investigated the effect of postoperative skeletal muscle index (SMI) loss on prognosis. This study aims to evaluate the prognostic role of postoperative SMI loss ≥ 5% after CABG, in order to develop a novel nomogram to predict overall survival (OS).
Methods: Patients underwent CABG via midline sternotomy from December 2015 to March 2021 were recruited in this study. Preoperative and postoperative 3 months chest computed tomography (CT) images were compared to assess changes in SMI at T12 level. Based on this, patients were classified into the presence or absence of SMI loss ≥ 5%. The association between postoperative SMI loss ≥ 5% and OS was then analyzed by the Kaplan-Meier curves and Cox model. A novel nomogram incorporating independent clinical prognostic variables was also developed.
Results: The study enrolled 506 patients receiving CABG, of whom 98 patients experienced T12 SMI loss ≥ 5% and had a significantly worse OS (P < 0.0001). Multivariate regression analysis showed that T12 SMI per cent change (%T12 SMI-change) was an independent prognostic factor for OS (HR = 0.809, 95% CI = 0.749–0.874). The nomogram incorporating %T12 SMI-change with other variables was accurate for predicting OS. Besides, we also found that postoperative oral nutritional supplement (ONS) can rescue T12 SMI loss.
Conclusion: Postoperative SMI loss can predict survival outcome after CABG. The nomogram incorporating changes in SMI provides a superior performance than existing systems.
Coronary artery disease (CAD) is a global burden in terms of high mortality, morbidity and economic loss (1, 2). As a preferred treatment for patients with 3-vessel disease and left main CAD, coronary artery bypass grafting (CABG) provides surgical collateralization and prolong life (3, 4). However, preventing postoperative complications and increasing life expectancy still arouse cardiac surgeons’ concerns (5). Previous studies on improving the prognosis of patients underwent CABG have mainly focused on treatment-related factors, such as the selection of grafts, surgical approach methods, and the establishment of extracorporeal circulation (6–9). Conversely, some patient-related modifiable factors, such as postoperative weight loss, sarcopenia, etc., have not been studied extensively, and corresponding measures to improve these factors are lacking.
Measurement of body composition and its impact on outcomes after cardiac surgery are gaining increasing attention. Our study has previously confirmed that preoperative sarcopenia is associated with unfavorable short- and long-term prognosis of patients receiving CABG (10). Estimating preoperative skeletal muscle index (SMI) has a predictive effect and allows for timely therapeutic intervention for better postoperative outcomes. However, many patients underwent CABG experience muscle wasting during the recovery period, which results in reduced muscle mass, functional capacity and quality of life, and increased mortality, especially in patients older than 50 years (11, 12). Surgery-induced stress, inflammation, and protein depletion all contribute to disturbed metabolism and postoperative skeletal muscle loss (13, 14). Considering the dynamic change of SMI during perioperative period, it may inadequate to merely assess the state of skeletal muscle before surgery to predict the clinical outcomes of the patients and formulate postoperative rehabilitation programs. Nevertheless, the few existing studies mainly focused on the impact of preoperative skeletal muscle assessment on the prognosis of CABG patients (10, 15), and the relationship between postoperative skeletal muscle loss and clinical outcomes in these patients is unclear.
In this study, we explored the factors affecting SMI loss after CABG, and investigated the predictive role of postoperative SMI loss on long-term outcomes in CABG patients. The secondary objective was to develop a novel nomogram with body composition-related parameters for predicting overall survival (OS) and verify its predictive effect in patients underwent CABG.
Between December 2015 and March 2021, patients aged ≥ 18 years who underwent CABG through midline sternotomy at the Department of Cardio-Thoracic Surgery, Shanghai Tenth People’s Hospital were recruited in this study. Patients with emergency surgery, a history of previous cardiothoracic surgery, insufficient chest computed tomography (CT) data, or in-hospital mortality were excluded. All the patients signed written informed consent. This study was conducted following the Helsinki Declaration of the World Medical Association, and the research approach was approved by the ethics committee of Shanghai Tenth People’s Hospital and registered in Chinese Clinical Trial Registry (ChiCTR2000037875).
For each patient, the following clinical data were collected by trained surgeons: (1) preoperative characteristics, including general information, cardiac function-related information, existing comorbidity, laboratory tests and nutritional risk assessment [evaluated by prognostic nutritional index (PNI) and geriatric nutritional risk index (GNRI)] during preoperative period; (2) operative features, including type of surgery, number of bypassed vessels, and operative time; (3) postoperative characteristics, including postoperative complications graded by Clavien-Dindo classification (16) (Grade ≥ II were analyzed), severe complications (Grade ≥ III) and the use of oral nutritional supplement (ONS). According to the doctor’s recommendation, patients with reduced eating were prescribed with Ruineng® (Sino-Swed Pharmaceutical Corp. Ltd.) for 5 days after CABG. This ONS product is a nutritionally balanced enteral nutritional emulsion, containing approximately 650 kcal energy, 29.3 g protein, 36 g fat, 52 g of carbohydrate, and vitamins and minerals per 500 ml. Patients receiving the advice of physician took ONS after CABG, and the expected daily intake of ONS was 500 ml. And patients did not routinely receive exercise rehabilitation training after CABG.
The chest CT images performed within 2 weeks before and 3 months after CABG were collected, respectively. Then we analyzed these collected images at the 12th thoracic vertebra (T12) level by INFINITT PACS software (version 3.0.11.3, Seoul, Korea) to identify skeletal muscle in the range of –29 to + 150 Hounsfield unit (HU). Skeletal muscles evaluated at T12 level contained the rectus abdominis, external oblique, internal oblique, latissimus dorsi, intercostal, and erector spinae muscles. The skeletal muscle area was normalized by height (m2) to determine the SMI (cm2/m2) at T12. Consistent with our previous study, referring to a large-scale study, low SMI was defined as T12 SMI < 28.8 cm2/m2 for male and < 20.8 cm2/m2 for female (10, 17). T12 SMI per cent change (%T12 SMI-change) was calculated as (postoperative T12 SMI—preoperative T12 SMI)/preoperative T12 SMI × 100%. Since multiple studies have demonstrated that SMI loss ≥ 5% is associated with adverse clinical outcomes (18–21), we used this threshold to divide patients into T12 SMI loss ≥ 5% and T12 SMI loss < 5% groups to compare OS according to SMI change in this study.
All patients were followed up every 3 months for the first 2 years, and every 6 months after that by telephone interviews or outpatient visits. OS was calculated from the date of operation until the date of death from any cause or the last follow-up date for live patients. The latest follow-up date was January 31, 2022.
Depend on the normality of distribution, continuous variables were presented as the mean ± standard deviation (SD) and compared using the Student’s t-test, or median (p25–p75) and compared using the Mann-Whitney U-test or Kruskal-Wallis test. While categorical data were expressed as numbers (percentages), using Chi-squared or Fisher’s exact test for comparison. Univariate Cox regression analysis was performed to determine probable risk factors of OS. Factors with P < 0.10 were included in the multivariate Cox analysis by a backward stepwise selection methodology. Kaplan-Meier curves and Cox proportional hazards model were used to analyze long-term survival. The variables screened by multivariate Cox regression were incorporated to develop a nomogram for OS prediction. Random resampling of the study population with a 75% ratio was performed to simulate outsource validation cohort. C-index, the area under receiver operating characteristic curve (AUC) and calibration curve were performed to evaluated the discriminative ability and predictive accuracy of the novel nomogram. All tests were two-sided and P < 0.05 was regarded as statistically significant. All statistical analysis was conducted by SPSS software version 26.0 (Armonk, NY, United States) and R software version 4.1.3 (Vienna, Austria).
Initially, 548 patients underwent CABG via midline sternotomy met the inclusion criteria. And excluding unavailable postoperative chest CT scans in 42 patients, a total of 506 patients were finally analyzed in our study. The patient flow chart was shown in Figure 1.
Patient characteristics were presented in Table 1. Based on the preoperative and postoperative chest CT scans of each patient, 98 (19.37%) patients demonstrated T12 SMI loss ≥ 5%, while 408 (80.63%) patients had T12 SMI loss < 5%. The two groups were comparable in terms of age, gender, comorbidities, C-reactive protein, red blood cells, hemoglobin, neutrophil-to-lymphocyte ratio (NLR), albumin, blood urea nitrogen (BUN), creatinine, preoperative T12 SMI, surgical details, and postoperative complications. However, ≥ 5% T12 SMI loss was associated with lower body mass index (BMI) (P = 0.035) and postoperative T12 SMI (P < 0.001). In contrast, patients with T12 SMI ≥ 5% loss had higher white blood cells (P = 0.020). Although the left ventricular ejection (LVEF) and postoperative hospital stays of two groups were quite close, patients who exhibited ≥ 5% T12 SMI loss had higher LVEF (P = 0.045) and longer postoperative hospital stays (P = 0.045). For the risk score of cardiac surgery, EuroSCORE II score was higher in T12 SMI loss ≥ 5% group than in T12 SMI loss < 5% group (P = 0.023).
As shown in Supplementary Table 1, preoperative low SMI (P = 0.003), BMI (P = 0.023), LVEF (P = 0.013), hypoproteinemia (P < 0.001), PNI (P < 0.001), and GNRI (P = 0.013) may be essential preoperative factors affecting postoperative SMI change. However, postoperative complications did not affect postoperative %T12 SMI-change. In addition, of the 506 patients, 190 (37.5%) patients received doctor’s recommendation to take ONS after surgery. The median of %T12 SMI-change was –0.67 [–4.15–3.44]% and 1.12 [–3.82–4.54]% for patients without and with ONS, respectively (Figure 2). And there was significant difference in %T12 SMI-change between the two groups (P = 0.015).
During a median of 3.38 years follow-up, 43 (8.5%) patients died. The survival curves demonstrated that patients with T12 SMI ≥ 5% loss had a significantly worse OS relative to those with < 5% T12 SMI loss (Figure 3, Log-rank: P < 0.0001). Univariate and multivariate Cox regression analyses for OS were presented in Table 2. In the multivariate analysis, Preoperative low SMI (P = 0.003) and %T12 SMI-change (P < 0.001) were independent prognostic factor for OS in patients underwent CABG, alongside age (P = 0.010) and LVEF ≤ 50% (P = 0.033).
Figure 3. Kaplan-Meier curve for overall survival stratified by postoperative skeletal muscle mass loss.
Based on the results of multivariate Cox regression analysis, four independent predictors were integrated to developed a novel nomogram for predicting OS (Figure 4). Each variable has a score on the point scale, and the estimated probability of 1-, 2-, and 3-year OS could easily be obtained by adding the total score and placing it on the total score scale. Patient characteristics in validation cohort were shown in Supplementary Table 2. The C-index for overall and validation cohort were 0.77 (95% CI: 0.69-0.86) and 0.82 (95% CI: 0.72-0.92), respectively. Furthermore, the nomogram yielded AUC values of 0.862, 0.819, 0.802 in the overall population and 0.856, 0.833, 0.863 in the validation cohort for predicting OS rates at 1, 2 and 3 years (Figure 5). The calibration curve of the nomogram for the survival probability at 1 and 2 years demonstrated good agreement between prediction and observation in the overall population and the validation cohort (Figure 6). In addition, when compared with EuroSCORE II, the novel nomogram with body composition-related parameters demonstrated higher predictive ability of long-term survival (Figure 7).
Figure 4. The nomogram developed to predict the overall survival. SMI, skeletal muscle index; %T12 SMI-change, T12 SMI percent change; LVEF, left ventricular ejection.
Figure 5. Area under the ROC curves (AUC) for survival prediction in the overall population (A) and validation cohort (B). ROC, receiver operator characteristic.
Figure 6. The calibration curve for survival prediction at (A) 1 year and (B) 2 years in the overall population and at (C) 1 year and (D) 2 years in the validation cohort. The nomogram-predicted probability of overall survival is plotted on the x-axis; the actual overall survival is plotted on the y-axis. OS, overall survival.
Figure 7. Time-dependent AUCs over time for EuroSCORE II and the nomogram for predicting the overall survival. EuroSCORE II, European System for Cardiac Operative Risk Evaluation II; AUC, area under the receiver operator characteristic curves.
To our knowledge, this is the first study to investigate the relationship between postoperative skeletal muscle loss and prognosis in patients underwent CABG. In the present study, we have demonstrated that about 20% of these patients have T12 SMI loss ≥ 5% 3 months after CABG, and postoperative ONS could rescue T12 SMI loss. Furthermore, we have presented evidence suggesting that %T12 SMI-change is an independent risk factor for OS of CABG. A nomogram incorporating %T12 SMI-change as well as the parameters such as age, preoperative low SMI, LVEF ≤ 50% was developed. Importantly, compared with EuroSCORE II, our novel nomogram showed stronger prediction efficiency for long-term outcomes.
Surgical trauma is frequently accompanied with hyperglycemia, accelerated systemic protein catabolism and amino acid oxidation, which results in nitrogen loss and negative protein balance (22, 23). A previous study has reported that the protein synthesis of skeletal muscle is severely inhibited after CABG surgery, with muscle protein synthesis rates decreasing by approximately 36% within the first 4 h after surgery (24). As an essential component of body composition, skeletal muscle mass (SMM) is most sensitive to changes in protein balance (25, 26). In the present study, 19.37% of patients experienced T12 SMI loss ≥ 5% at 3 months after CABG. In agreement with this finding, van Venrooij et al. also found that patients underwent cardiac surgery are more likely to suffer from skeletal muscle loss, especially in patients receiving CABG. Approximately 25% of those patients lose more than 5% of SMM within 2 months after surgery (18).
Among the several techniques to assess body composition, bioelectrical impedance analysis (BIA) is commonly used in primary care because of its portability and affordability and dual-energy X-ray absorptiometry (DXA) gain the popularity among some clinicians and researchers (27, 28). However, the muscle mass determined by BIA and DXA would sometimes fail to be consistent with each other due to the different bands of equipment and can be easily influenced by the body hydration status, such as the patients underwent CABG, among which fluid retention caused by unstable cardiac function often occurs (29). CT is the gold standard to assess muscle quantity/mass non-invasively (30). SMM at T12 level has good consistency with whole body muscle mass (17). Besides, the analysis based on the existing chest CT avoids extra radiation exposure and medical expenses of CABG patients. Based on the above considerations, chest CT was used to assess SMM in this study.
Recently, preoperative sarcopenia has become a prognostic indicator for various types of surgery (31–34), whereas few studies investigated the impact of preoperative skeletal muscle loss on survival in patients receiving CABG. In our previous study, preoperative sarcopenia was proved to be an independent risk factor for postoperative complications and OS of CABG (10). Actually, postoperative skeletal muscle loss not only leads to a decrease in postoperative quality of life (18), but also causes decreased exercise capacity and impaired respiratory function (35). Therefore, comparing with preoperative sarcopenia, postoperative skeletal muscle loss is more sensitive for worse long-term prognosis, as it reflects the response to the surgical trauma and stress of patients. In this study, patients with postoperative T12 SMI loss ≥ 5% were more likely to have adverse OS (Figure 3). Multivariate Cox analysis demonstrated that preoperative low SMI, %T12 SMI-change, age and LVEF ≤ 50% remain independent risk factors of OS. For patients underwent CABG, reduced LVEF is frequently associated with ischemic cardiomyopathy and increases the risk of postoperative adverse events (36). Thus, we incorporated all four independent risk factors into an innovative nomogram for predicting long-term survival in CABG patients.
EuroSCORE II, the most commonly used risk assessment tool, has been shown to be effective in predicting postoperative risk in patients underwent cardiac surgery (37, 38). And EuroSCORE II is mainly based on clinical characteristics, surgical factors, and echocardiographic findings (39). In addition to the above factors, inflammation, left ventricular systolic dysfunction, nutritional status, and body composition can also affect patient outcomes. Even though EuroSCORE II is accurate in predicting short-term outcomes, its performance fades for mortality at follow-up longer than 30 days (40, 41). Therefore, it is necessary to develop a new risk stratification tool to predict long-term clinical outcomes. In this study, we exploited a nomogram for predicting OS in CABG patients, which showed favorable discrimination with AUC values consistently more than 0.8 and significantly higher than EuroSCORE II. The calibration curve of our nomogram for the survival probability at 1- and 2-year revealed good agreement between predicted and actual OS.
Exercise-based cardiac rehabilitation has been strongly recommended as an important adjunctive therapy after CABG in recent years (42, 43). Previous studies have demonstrated that exercise rehabilitation training can reduce muscle strength loss and improve postoperative quality of life after CABG (44–46). Whereas, some factors, such as postoperative pain, the risk of exercise, and the lack of local physiotherapy services make exercise-based cardiac rehabilitation programs less effective. The use of ONS can provide supplementary energy and nutrition in addition to normal food to improve energy expenditure from surgical trauma. Multiple previous studies have demonstrated that ONS can benefit patients by reducing postoperative skeletal muscle loss in many surgical procedures (47–49). As expected, ONS alleviated postoperative SMI loss in patients underwent CABG in our study, which adds a new strategy for cardiac rehabilitation programs. And as a reflection of systemic nutritional status, changes in SMI can be considered for evaluating the effects of ONS and guiding dietary recommendations.
There are some limitations to the present study. This is a single-center study and its findings need to be validated in international multi-center studies. Nonetheless, the present study still presents a large impact of postoperative SMI loss on OS. Else, due to the retrospective design of the study cohort, we only investigated changes in SMI in this study, and our future prospective studies intend to focus on the changes in skeletal muscle density and function after cardiac surgery. Finally, we only found that the application of ONS was associated with improved postoperative skeletal muscle loss in this study, and we are conducting a prospective study to further investigate the effect of ONS on clinical outcomes.
Postoperative SMI loss has an effective prognostic influence on long-term survival after CABG. ONS may be considered for patients underwent cardiac surgery to reduce skeletal muscle degradation and improve outcomes. The nomogram incorporating changes in SMI performs well in survival prediction.
The raw data supporting the conclusions of this article will be made available by the authors, without undue reservation.
The studies involving human participants were reviewed and approved by the Ethics Committee of Shanghai Tenth People’s Hospital. The patients/participants provided their written informed consent to participate in this study.
Z-LS, W-FZ, X-LY, and ZY designed the study. PZ, W-ZC, W-XD, W-HC, and FL collected the data. ZL did the analysis and interpretation of data. Z-LS wrote the article. ZY revised the article and took the decision to submit the article for publication. All authors gave final approval and agreed to take responsibility for all aspects of this work to ensuring integrity and accuracy.
This work was supported by the National Natural Science Foundation of China (no. 81770884), the Science and Technology Commission of Shanghai Municipality (no. 21DZ2208300), and the Shanghai Association of Integrative Medicine (no. shcim202101).
The authors declare that the research was conducted in the absence of any commercial or financial relationships that could be construed as a potential conflict of interest.
All claims expressed in this article are solely those of the authors and do not necessarily represent those of their affiliated organizations, or those of the publisher, the editors and the reviewers. Any product that may be evaluated in this article, or claim that may be made by its manufacturer, is not guaranteed or endorsed by the publisher.
The Supplementary Material for this article can be found online at: https://www.frontiersin.org/articles/10.3389/fnut.2022.970729/full#supplementary-material
1. Tsao CW, Aday AW, Almarzooq ZI, Alonso A, Beaton AZ, Bittencourt MS, et al. Heart disease and stroke statistics-2022 update: a report from the American heart association. Circulation. (2022) 145:e153–639. doi: 10.1161/CIR.0000000000001052
2. Roth GA, Mensah GA, Johnson CO, Addolorato G, Ammirati E, Baddour LM, et al. Global burden of cardiovascular diseases and risk factors, 1990-2019: update from the GBD 2019 study. J Am Coll Cardiol. (2020) 76:2982–3021. doi: 10.1016/j.jacc.2020.11.010
3. Takahashi K, Serruys PW, Gao C, Ono M, Wang R, Thuijs D, et al. Ten-Year All-Cause death according to completeness of revascularization in patients with three-vessel disease or left main coronary artery disease: insights from the SYNTAX extended survival study. Circulation. (2021) 144:96–109. doi: 10.1161/CIRCULATIONAHA.120.046289
4. Doenst T, Haverich A, Serruys P, Bonow RO, Kappetein P, Falk V, et al. PCI and CABG for treating stable coronary artery disease: JACC review topic of the week. J Am Coll Cardiol. (2019) 73:964–76. doi: 10.1016/j.jacc.2018.11.053
5. Polzl L, Thielmann M, Cymorek S, Nagele F, Hirsch J, Graber M, et al. Impact of myocardial injury after coronary artery bypass grafting on long-term prognosis. Eur Heart J. (2022) 43:2407–17. doi: 10.1093/eurheartj/ehac054
6. Thuijs D, Davierwala P, Milojevic M, Deo SV, Noack T, Kappetein AP, et al. Long-term survival after coronary bypass surgery with multiple versus single arterial grafts. Eur J Cardiothorac Surg. (2022) 61:925–33. doi: 10.1093/ejcts/ezab392
7. Teman NR, Hawkins RB, Charles EJ, Mehaffey JH, Speir AM, Quader MA, et al. Minimally invasive vs open coronary surgery: a multi-institutional analysis of cost and outcomes. Ann Thorac Surg. (2021) 111:1478–84. doi: 10.1016/j.athoracsur.2020.06.136
8. Squiers JJ, Schaffer JM, Banwait JK, Ryan WH, Mack MJ, DiMaio JM. Long-Term survival after on-pump and off-pump coronary artery bypass grafting. Ann Thorac Surg. (2021) 113:1943–52. doi: 10.1016/j.athoracsur.2021.07.037
9. Gaudino M, Audisio K, Rahouma M, Chadow D, Cancelli G, Soletti GJ, et al. Comparison of long-term clinical outcomes of skeletonized vs pedicled internal thoracic artery harvesting techniques in the arterial revascularization trial. JAMA Cardiol. (2021) 6:1380–6. doi: 10.1001/jamacardio.2021.3866
10. Shen ZL, Liu Z, Zang WF, Zhang P, Zou HB, Dong WX, et al. Thoracic sarcopenia predicts clinical outcomes in patients underwent coronary artery bypass grafting: a 6-year cohort study. Asian J Surg. (2022). doi: 10.1016/j.asjsur.2022.03.096 [Epub ahead print].
11. Hansen D, Linsen L, Verboven K, Hendrikx M, Rummens JL, van Erum M, et al. Magnitude of muscle wasting early after on-pump coronary artery bypass graft surgery and exploration of aetiology. Exp Physiol. (2015) 100:818–28. doi: 10.1113/EP085053
12. Szulc P, Munoz F, Marchand F, Chapurlat R, Delmas PD. Rapid loss of appendicular skeletal muscle mass is associated with higher all-cause mortality in older men: the prospective MINOS study. Am J Clin Nutr. (2010) 91:1227–36. doi: 10.3945/ajcn.2009.28256
13. Cossu AP, Suelzu S, Piu P, Orecchioni M, Bazzu G, Padua G, et al. Do on- and off-pump coronary bypass surgery differently affect perioperative peripheral tissue metabolism? Minerva Anestesiol. (2012) 78:26–33.
14. van Gassel RJJ, Baggerman MR, van de Poll MCG. Metabolic aspects of muscle wasting during critical illness. Curr Opin Clin Nutr Metab Care. (2020) 23:96–101. doi: 10.1097/MCO.0000000000000628
15. Okamura H, Kimura N, Mieno M, Yuri K, Yamaguchi A. Preoperative sarcopenia is associated with late mortality after off-pump coronary artery bypass grafting. Eur J Cardiothorac Surg. (2020) 58:121–9. doi: 10.1093/ejcts/ezz378
16. Clavien PA, Barkun J, de Oliveira ML, Vauthey JN, Dindo D, Schulick RD, et al. The Clavien-Dindo classification of surgical complications: five-year experience. Ann Surg. (2009) 250:187–96. doi: 10.1097/SLA.0b013e3181b13ca2
17. Derstine BA, Holcombe SA, Ross BE, Wang NC, Su GL, Wang SC. Skeletal muscle cutoff values for sarcopenia diagnosis using T10 to L5 measurements in a healthy US population. Sci Rep. (2018) 8:11369. doi: 10.1038/s41598-018-29825-5
18. van Venrooij LM, Verberne HJ, de Vos R, Borgmeijer-Hoelen MM, van Leeuwen PA, de Mol BA. Postoperative loss of skeletal muscle mass, complications and quality of life in patients undergoing cardiac surgery. Nutrition. (2012) 28:40–5. doi: 10.1016/j.nut.2011.02.007
19. Tan S, Zhuang Q, Zhang Z, Li S, Xu J, Wang J, et al. Postoperative loss of skeletal muscle mass predicts poor survival after gastric cancer surgery. Front Nutr. (2022) 9:794576. doi: 10.3389/fnut.2022.794576
20. Lee J, Lin JB, Wu MH, Chang CL, Jan YT, Chen YJ. Muscle loss after chemoradiotherapy as a biomarker of distant failures in locally advanced cervical cancer. Cancers. (2020) 12:3. doi: 10.3390/cancers12030595
21. Huang CY, Yang YC, Chen TC, Chen JR, Chen YJ, Wu MH, et al. Muscle loss during primary debulking surgery and chemotherapy predicts poor survival in advanced-stage ovarian cancer. J Cachexia Sarcopenia Muscle. (2020) 11:534–46. doi: 10.1002/jcsm.12524
22. Schricker T, Lattermann R. Perioperative catabolism. Can J Anaesth. (2015) 62:182–93. doi: 10.1007/s12630-014-0274-y
23. Weimann A, Braga M, Carli F, Higashiguchi T, Hubner M, Klek S, et al. ESPEN practical guideline: clinical nutrition in surgery. Clin Nutr. (2021) 40:4745–61. doi: 10.1016/j.clnu.2021.03.031
24. Caso G, Vosswinkel JA, Garlick PJ, Barry MK, Bilfinger TV, McNurlan MA. Altered protein metabolism following coronary artery bypass graft (CABG) surgery. Clin Sci. (2008) 114:339–46. doi: 10.1042/CS20070278
25. Wolfe RR. The underappreciated role of muscle in health and disease. Am J Clin Nutr. (2006) 84:475–82. doi: 10.1093/ajcn/84.3.475
26. Carbone JW, McClung JP, Pasiakos SM. Skeletal muscle responses to negative energy balance: effects of dietary protein. Adv Nutr. (2012) 3:119–26. doi: 10.3945/an.111.001792
27. Moore ML, Benavides ML, Dellinger JR, Adamson BT, Tinsley GM. Segmental body composition evaluation by bioelectrical impedance analysis and dual-energy X-ray absorptiometry: quantifying agreement between methods. Clin Nutr. (2020) 39:2802–10. doi: 10.1016/j.clnu.2019.12.009
28. Lahav Y, Goldstein N, Gepner Y. Comparison of body composition assessment across body mass index categories by two multifrequency bioelectrical impedance analysis devices and dual-energy X-ray absorptiometry in clinical settings. Eur J Clin Nutr. (2021) 75:1275–82. doi: 10.1038/s41430-020-00839-5
29. Slight RD, Demosthenous N, Nzewi OC, Soliman AR, McClelland DB, Mankad PS. The effect of gain in total body water on haemoglobin concentration and body weight following cardiac surgery. Heart Lung Circ. (2006) 15:256–60. doi: 10.1016/j.hlc.2006.03.013
30. Cruz-Jentoft AJ, Bahat G, Bauer J, Boirie Y, Bruyère O, Cederholm T, et al. Sarcopenia: revised European consensus on definition and diagnosis. Age Ageing. (2019) 48:16–31. doi: 10.1093/ageing/afy169
31. Hu MH, Yen HK, Chen IH, Wu CH, Chen CW, Yang JJ, et al. Decreased psoas muscle area is a prognosticator for 90-day and 1-year survival in patients undergoing surgical treatment for spinal metastasis. Clin Nutr. (2022) 41:620–9. doi: 10.1016/j.clnu.2022.01.011
32. Klajda M, Trachtenberg B, Araujo R, Estep JD, Masotti M, Teigen L, et al. Pre-operative sarcopenia is predictive of recurrent gastrointestinal bleeding on left ventricular assist device support: a multicenter analysis. J Heart Lung Transplant. (2022) 41:757–62. doi: 10.1016/j.healun.2022.01.004
33. Gallone G, Depaoli A, D’Ascenzo F, Tore D, Allois L, Bruno F, et al. Impact of computed-tomography defined sarcopenia on outcomes of older adults undergoing transcatheter aortic valve implantation. J Cardiovasc Comput Tomogr. (2022) 16:207–14. doi: 10.1016/j.jcct.2021.12.001
34. Zou HB, Yan XL, Dong WX, Yu DY, Zhang FM, Zhou LP, et al. Sarcopenia is a predictive factor of poor quality of life and prognosis in patients after radical gastrectomy. Eur J Surg Oncol. (2021) 47:1976–84. doi: 10.1016/j.ejso.2021.03.004
35. Rajaei S, Dabbagh A. Risk factors for postoperative respiratory mortality and morbidity in patients undergoing coronary artery bypass grafting. Anesth Pain Med. (2012) 2:60–5. doi: 10.5812/aapm.5228
36. Vignon P. Assessment of pulmonary arterial pressure using critical care echocardiography: dealing with the yin and the yang? Crit Care Med. (2019) 47:126–8. doi: 10.1097/CCM.0000000000003491
37. Koo SK, Dignan R, Lo EYW, Williams C, Xuan W. Evidence-Based determination of cut-off points for increased cardiac-surgery mortality risk with EuroSCORE II and STS: the best-performing risk scoring models in a single-centre australian population. Heart Lung Circ. (2022) 31:590–601. doi: 10.1016/j.hlc.2021.08.026
38. Ad N, Holmes SD, Patel J, Pritchard G, Shuman DJ, Halpin L. Comparison of EuroSCORE II, Original EuroSCORE, and the society of thoracic surgeons risk score in cardiac surgery patients. Ann Thorac Surg. (2016) 102:573–9. doi: 10.1016/j.athoracsur.2016.01.105
39. Nashef SA, Roques F, Sharples LD, Nilsson J, Smith C, Goldstone AR, et al. EuroSCORE II. Eur J Cardiothorac Surg. (2012) 41:734–44. doi: 10.1093/ejcts/ezs043
40. Carino D, Denti P, Ascione G, Del Forno B, Lapenna E, Ruggeri S, et al. Is the EuroSCORE II reliable in surgical mitral valve repair? A single-centre validation study. Eur J Cardiothorac Surg. (2021) 59:863–8. doi: 10.1093/ejcts/ezaa403
41. Barili F, Pacini D, D’Ovidio M, Dang NC, Alamanni F, Di Bartolomeo R, et al. The impact of EuroSCORE II risk factors on prediction of long-term mortality. Ann Thorac Surg. (2016) 102:1296–303. doi: 10.1016/j.athoracsur.2016.04.017
42. Baman JR, Sekhon S, Maganti K. Cardiac rehabilitation. JAMA. (2021) 326:366. doi: 10.1001/jama.2021.5952
43. Heidenreich PA, Bozkurt B, Aguilar D, Allen LA, Byun JJ, Colvin MM, et al. 2022 AHA/ACC/HFSA guideline for the management of heart failure: a report of the American college of cardiology/american heart association joint committee on clinical practice guidelines. Circulation. (2022) 145:e895–1032. doi: 10.1161/CIR.0000000000001063
44. de Aquino TN, de Faria Rosseto S, Lucio Vaz J, de Faria Cordeiro Alves C, Vidigal FC, Galdino G. Evaluation of respiratory and peripheral muscle training in individuals undergoing myocardial revascularization. J Card Surg. (2021) 36:3166–73. doi: 10.1111/jocs.15698
45. Dos Santos TD, Pereira SN, Portela LOC, Cardoso DM, Lago PD, Dos Santos Guarda N, et al. Moderate-to-high intensity inspiratory muscle training improves the effects of combined training on exercise capacity in patients after coronary artery bypass graft surgery: a randomized clinical trial. Int J Cardiol. (2019) 279:40–6. doi: 10.1016/j.ijcard.2018.12.013
46. Nishitani M, Shimada K, Masaki M, Sunayama S, Kume A, Fukao K, et al. Effect of cardiac rehabilitation on muscle mass, muscle strength, and exercise tolerance in diabetic patients after coronary artery bypass grafting. J Cardiol. (2013) 61:216–21. doi: 10.1016/j.jjcc.2012.11.004
47. Tan S, Meng Q, Jiang Y, Zhuang Q, Xi Q, Xu J, et al. Impact of oral nutritional supplements in post-discharge patients at nutritional risk following colorectal cancer surgery: a randomised clinical trial. Clin Nutr. (2021) 40:47–53. doi: 10.1016/j.clnu.2020.05.038
48. Malafarina V, Uriz-Otano F, Malafarina C, Martinez JA, Zulet MA. Effectiveness of nutritional supplementation on sarcopenia and recovery in hip fracture patients. A multi-centre randomized trial. Maturitas. (2017) 101:42–50. doi: 10.1016/j.maturitas.2017.04.010
Keywords: skeletal muscle loss, coronary artery bypass grafting, nomogram, survival, oral nutritional supplement
Citation: Shen Z-L, Liu Z, Zhang P, Chen W-Z, Dong W-X, Chen W-H, Lin F, Zang W-F, Yan X-L and Yu Z (2022) Prognostic significance of postoperative loss of skeletal muscle mass in patients underwent coronary artery bypass grafting. Front. Nutr. 9:970729. doi: 10.3389/fnut.2022.970729
Received: 16 June 2022; Accepted: 10 August 2022;
Published: 02 September 2022.
Edited by:
Lilia Castillo-Martinez, Instituto Nacional de Ciencias Médicas y Nutrición Salvador Zubirán (INCMNSZ), MexicoReviewed by:
María Fernanda Bernal, National Autonomous University of Mexico, MexicoCopyright © 2022 Shen, Liu, Zhang, Chen, Dong, Chen, Lin, Zang, Yan and Yu. This is an open-access article distributed under the terms of the Creative Commons Attribution License (CC BY). The use, distribution or reproduction in other forums is permitted, provided the original author(s) and the copyright owner(s) are credited and that the original publication in this journal is cited, in accordance with accepted academic practice. No use, distribution or reproduction is permitted which does not comply with these terms.
*Correspondence: Wang-Fu Zang, emFuZ3dmQHRvbmdqaS5lZHUuY24=; Xia-Lin Yan, eWFueGlhbGluMjAxNUAxNjMuY29t; Zhen Yu, eXV6aGVuQHRvbmdqaS5lZHUuY24=
†These authors have contributed equally to this work and share first authorship
Disclaimer: All claims expressed in this article are solely those of the authors and do not necessarily represent those of their affiliated organizations, or those of the publisher, the editors and the reviewers. Any product that may be evaluated in this article or claim that may be made by its manufacturer is not guaranteed or endorsed by the publisher.
Research integrity at Frontiers
Learn more about the work of our research integrity team to safeguard the quality of each article we publish.