- 1Esperer Onco Nutrition Pvt. Ltd., Mumbai, India
- 2Department of Biological Sciences, Birla Institute of Technology and Science – Pilani, Hyderabad, India
- 3Department of Clinical Haematology, Mahatma Gandhi Medical College and Hospital, Jaipur, India
Conveyance of pathogens between organisms causes communicable diseases. On the other hand, a non-communicable disease (NCD) was always thought to have no causative transmissible infective agents. Today, this clear distinction is increasingly getting blurred and NCDs are found to be associated with some transmissible components. The human microbiota carries a congregation of microbes, the majority and the most widely studied being bacteria in the gut. The adult human gut harbors ginormous inhabitant microbes, and the microbiome accommodates 150-fold more genes than the host genome. Microbial communities share a mutually beneficial relationship with the host, especially with respect to host physiology including digestion, immune responses, and metabolism. This review delineates the connection between environmental factors such as infections leading to gut dysbiosis and NCDs and explores the evidence regarding possible causal link between them. We also discuss the evidence regarding the value of appropriate therapeutic immunomodulatory nutritional interventions to reduce the development of such diseases. We behold such immunomodulatory effects have the potential to influence in various NCDs and restore homeostasis. We believe that the beginning of the era of microbiota-oriented personalized treatment modalities is not far away.
Introduction
As comprehended since centuries, an infectious (communicable) disease is a malady in which a particular infectious agent (or its toxins) gets transmitted from an individual to a susceptible host. On the other hand, a non-communicable disease (NCD) has no causative agents to be transmitted. As time rolled on, scrupulous scientific observation has stumbled upon an expanding number of NCDs that cognate with a contagious pathogenic risk factor. These findings actually have blurred the distinction between a communicable disease and a non-communicable disease, although there were clear demarcations between them earlier (1, 2). It is now well known that the human microbiota comprises a multitude of microorganisms, the majority and the most widely studied being bacteria residing in the gut. The adult gut harbors almost 100 trillion resident microbes, and the corresponding genome (microbiome) contains 150-fold more genes than the host genome itself. Needless to mention, these complex microbial communities have grown in the evolutionary pathway to maintain a symbiotic relation with the host physiology influencing digestion, immune responses, and metabolism. However, it is not yet absolutely clear to which magnitude microbial spread can contribute to the onset of NCDs rendering it as a subject for intense investigation. Nonetheless, it is assiduously arduous to decouple environment from microbiota, which renders the investigation of the transmissibility of NCDs a bit challenging (3).
Robert Koch’s postulates have been entrenched in microbiologists of a different era as necessary and sufficient conditions to be fulfilled – which states that a specific microorganism engenders specific disease. Though limited to some extent, these postulates can still be considered as a scaffold to decipher microbial causes of ailments (4). Similarly, a way to be able to establish some causal links beyond mere correlations between dysbiotic gut microbiota and occurrence of NCDs is therefore needed (5). For instance, in case of cardiovascular diseases (CVD), cogent evidence is accumulated, which emphasizes a correlation with the CutC enzyme (encoded by gut microbe), known to convert carnitine and phosphocholine compounds to trimethylamine (TMA). Thereafter, it reaches the host liver and gets oxidized into trimethylamine oxide (TMAO), which is proven to exert an impact on cholesterol metabolism and to enhance atherosclerosis development (6). It has become an exigent demand to identify various environmental risk factors (in addition to the genetic risk factors) to establish molecular pathways causally linking them with NCDs such as CVD, diabetes, osteoporosis, polycystic ovary disease (PCOD), and non-alcoholic fatty liver disease (NAFLD). Of many environmental components, microorganisms play crucial roles in pathogenesis and progression of cancer. Recent studies have proven that infectious agents can cause more than 20% of all cancers, such as Helicobacter pylori causes gastric cancer, hepatitis B and C virus can trigger the onset of liver cancer, while cervical cancer takes its origin from human papillomavirus (7, 8). Besides probing the effects of single contagious microorganism in tumorigenesis, scientists have also undertaken the investigation of tumor environment associated microbial pool which has been described as a crucial environmental factor for some cancers, including colorectum, liver, biliary tract, and breast cancer (9). Intestinal epithelium involves in a cross talk with trillions of bacteria sheltering in the colorectum (10). They have immense importance toward the maintenance of physiology of GI tract in terms of energy balance and immunity. It is obvious that alterations in their ratio of quantity can switch the equipoise, which can eventually result in intestinal and extraintestinal maladies (11, 12).
As ratiocinated from many preclinical and clinical evidences across the globe, most of the NCDs and cancers caused by infections are associated with systemic inflammation. Thus, it is counterintuitive that phytopharmaceuticals having anti-inflammatory properties would be deciphered as potential therapeutic arsenals against these bleak maladies. This review is an attempt to summarize all recent accomplishments of immunomodulatory nutraceuticals in the prevention or management of various NCDs mitigating the ominous effects of pathogenic infection. We also propose that phytonutrients should be accompanied by beneficial commensals (probiotics) while developing therapeutic nutraceutical formula to combat NCDs and some cancers. This will provide nutritional interventions to address the suboptimally treated infections to trigger the immunomodulation to combat the chronic inflammatory state caused by the pathogenic infection with subsequent disease regression. In the following sections, we will elaborately discuss the communicable part in the NCDs, gut dysbiosis, microbial origin of NCDs, and emergence of immunomodulatory nutraceuticals as a therapeutic modality.
Communicable diseases and non-communicable diseases
As we touched upon the origin of communicable diseases, various pathogens are transmitted in various ways (dermal, sexual, oral, fecal, respiratory, and bites) between various organisms, which have a tremendous potential to eventually make a multitude sick. To further probe into the details about the origin of the diseases, numerous chronological details can be cited. In 1546, Girolamo Fracastoro proposed that microorganisms might beget human ailments. Yet, it was not accepted for a lack of causal connection. A technology was discovered by Louis Pasteur in 1864 to associate microorganisms with transmissible maladies. This was followed by a demonstration of a microbial link for an illness of silkworms by the same scientist in 1870. Robert Koch proposed a theory in 1892, popularly known as Koch’s postulates, to emphatically establish the causal link between microbes and transmissible ailments, which were used for tuberculosis, the foremost reason of mortality at that time. Recognized as ‘germ theory,’ the postulates can be summarized as (i) the causative species will be present throughout the ailment (ii) which can be isolated and propagated in vitro, (iii) the cultured microbe must cause the disease in vivo, and (iv) it can be reisolated from the new host exhibiting identical properties as the original. This spawned an array of seminal contributions over the following two decades, understanding microbial origins for cholera, typhoid, diphtheria, tetanus, and bubonic pest and bacillary dysentery with the invention of modalities to treat them (vaccine, antibiotics, etc.). Subsequent scientific movements aptly confirmed ‘germ theory,’ the proposition that a specific microbe can cause a specific transmissible malady. The cornerstone of the concept was “pathogen-control” over the host, meaning thereby pathogens have the potential to defeat host defenses in individuals with an unimpaired immunity (4). Although there is no denying the fact that communicable diseases remain the leading causes of human mortality globally, still, in high-income countries, the foremost health challenges are NCDs, such as CVDs, some cancers, habitual respiratory conditions, diabetes, arthritis, and asthma. NCDs were initially conceived as maladies that are steered by rudimentary flaws in host physiology not transmittable directly from one person to another and are not caused by a contagious agent. Thus, the germ theory was not directly applied to NCDs. Strikingly, research exploration in the last few decades has tied the microbial pool inhabiting the large intestine to numerous NCDs. Therefore, as the germ theory lays down a theoretical framework for transmissible ailments, there has been an urgent exigency to enunciate a speculative scaffold to decipher the balance and imbalance between the host and microbial organ, correlating gut microbes with NCDs. A new terminology - ‘germ-organ theory’ is proposed to delineate the ideation. Unlike the ‘germ theory’ that portrays the pathogen in control, the cornerstone of the ‘germ-organ theory’ is the host-control over the microbial ecosystem. In order to build a theoretical overarching – Koch’s postulates need to be modified for the microbial origin of the NCDs, where the causative pathogenic invader would be replaced by a dysbiotic microbiota (DM). Thus, modified postulates will read like (i) DM is found in people having NCDs, (ii) which can be isolated and propagated in in vitro culture, (iii) it can originate the disease when transferred to a healthy host in vivo, and (iv) it can be reisolated from the new host. This set of principles can largely be applied to various NCDs such as CVDs, T2DM, IBD, and their risk factors such as obesity. In the following section, we shall map the connection between dysbiotic microbiota, host immune system, and NCDs (4, 13–17) (Figure 1).
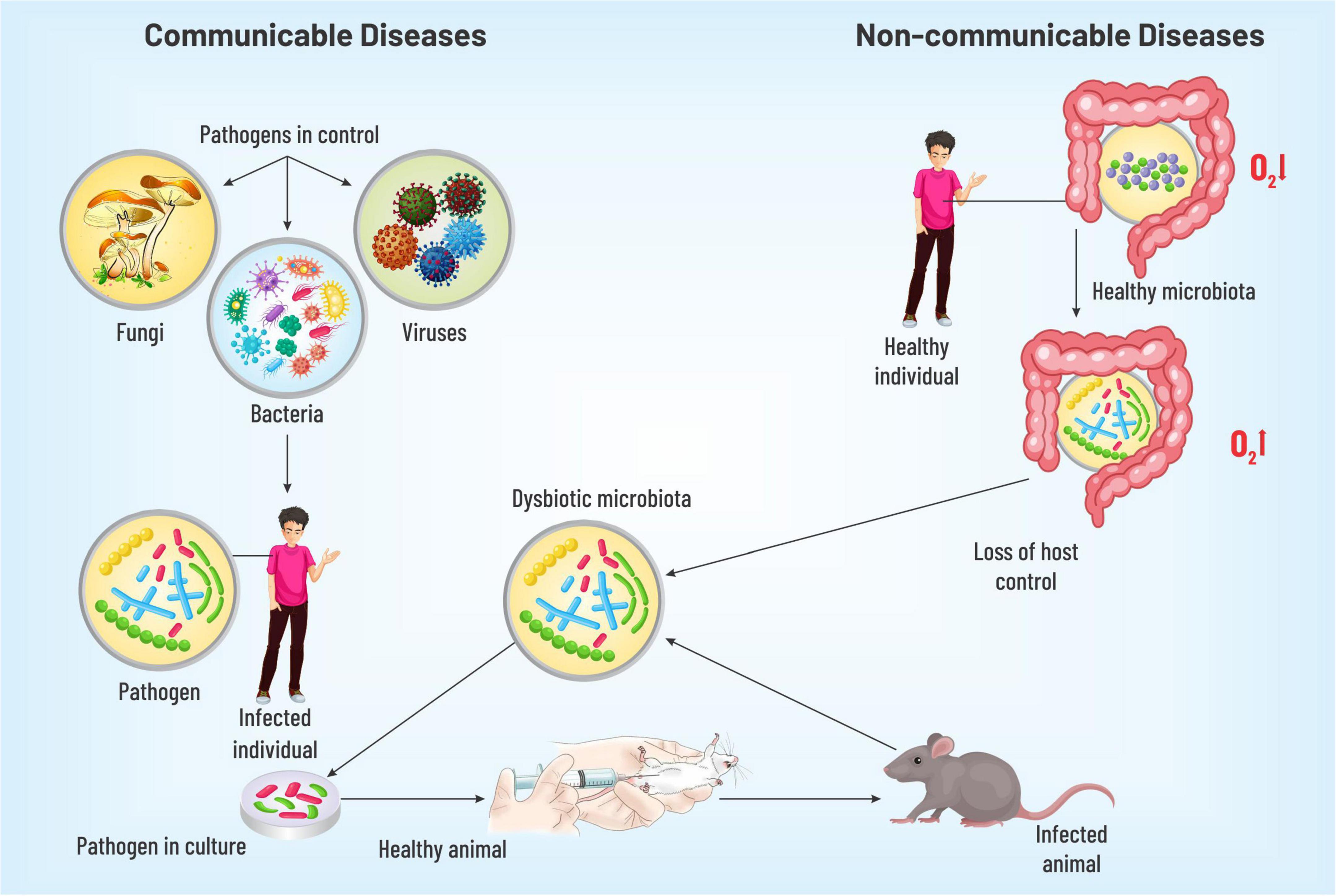
Figure 1. A diagrammatic representation discussing the identification of pathogenesis for communicable and non-communicable diseases. The dysbiotic gut microbiota on injection into the healthy model organism isolated from individuals with NCDs was observed to cause the respective disease thus verifying the hypothesis of gut microbiota playing a key role in disease pathogenesis.
Gut microbiota: Nutrition, immunity, and dysbiosis
Unlike invertebrates where a remarkable host control is manifested due to bacteria-specific peptides, diversified microbiota in mammalian gut does not advocate for species selection. Mammals control the composition of infant gut via milk oligosaccharides and of adult gut by secretion of antimicrobial proteins (e.g., defensins) (18–20). With growing knowledge, it is now evident that in order to maintain gut homeostasis, which is critically dependent on predominance of obligate anaerobes of Bacteroidetes phylum and Clostridia class, host limits the oxygen supply in the large intestine by keeping the colonic epithelium in hypoxia (21). This induces self-assembly of the coexisting microbial community with the formation of an anaerobic trophic network where every single location stands in for a nutrient niche to be occupied by a particular microbe having necessary metabolic activities creating similar metabolic patterns in individuals (22–24). Gut homeostasis, a stable equilibration maintained between host immunity and microbiota, is associated with hydrolysis and fermentation of complex structures of fibers by the obligate anaerobes into a bountiful of short-chain fatty acids (SCFA) metabolites – butyrate, propionate, acetate, and plentiful of other metabolites, where most of the SCFAs are assimilated by the host for its nutrition (25, 26). Thus, host-driven anaerobiosis inhibits the nutritional competition of the obligate anaerobes with the host for fiber metabolites, inhibits further catabolism of the metabolites to CO2 by facultative anaerobes, and steers its control over the microbial community without inflicting restriction on the composition. Moreover, these SCFA metabolites essentially are instrumental in immune development in various ways such as via inhibiting dendritic cell differentiation, promoting colonic and peripheral Treg production, and modulating function of intestinal macrophages. Importantly, homeostasis also requires a perpetual flow of SCFAs in epithelial cells of the colon to fuel PPARγ, the intracellular sensor for butyrate, and to stimulate Treg proliferation in colonic mucosa (27–29). These two mechanisms together propel the metabolism process in the direction of mitochondrial β-oxidation, which demands an ample amount of oxygen, and induce hypoxia in colonic epithelial cells (21, 30). To summarize epithelial hypoxia guarantees SCFA production by obligate anaerobes and SCFAs engender colonic epithelial hypoxia – thus, participating in a virtuous cycle that conserves gut homeostasis and preserves microbial ecosystem (Figure 2).
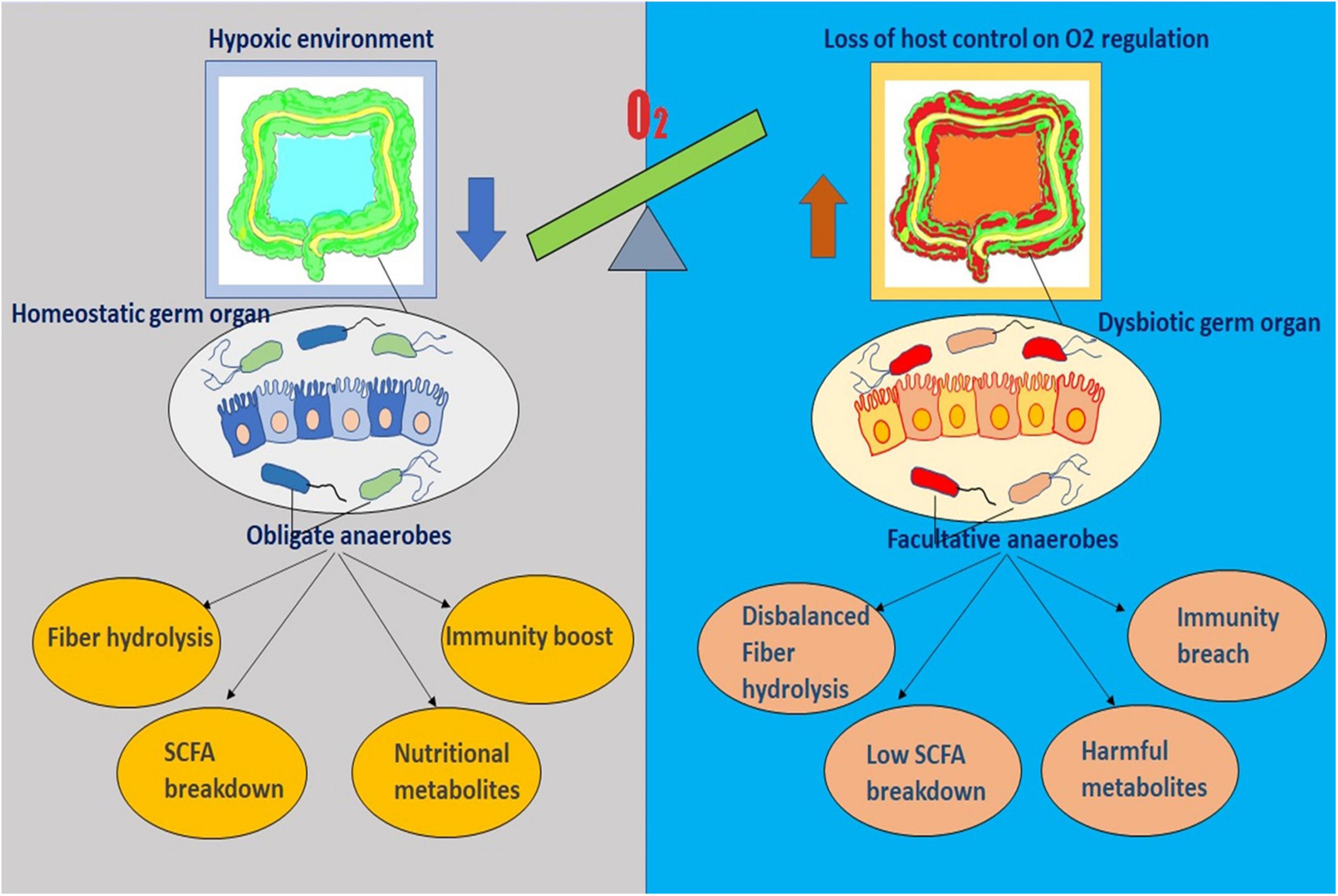
Figure 2. The diagram represents the sensitive interplay existing between the gut microenvironment and the residing microflora. It outlines the key role of oxygen imbalance in dysbiosis and diseased physiology.
In general, two major resident phyla in adult gut are Gram-negative Bacteroidetes and Gram-positive Firmicutes, with a lower abundance of actinobacteria, cyanobacteria, fusobacteria, proteobacteria, and verrucomicrobia (31). Lipopolysaccharides (LPS) present in the cell walls of Gram-negative strains trigger a strong inflammatory response in the host in order to defend against infectious invaders. As we discussed above, normalcy in tissue is maintained by well-orchestrated regulatory responses by impeding excess inflammation. It is worth mentioning here that the matrix of exposure to microbes in early life is of immense importance to build up a resilient immune regulation for the host and disruption of either host immune response or microbiota can induce chronic inflammation (23, 32–34). Unlike conventional comprehension of such as acute inflammation, chronic inflammation associated with obesity, atherosclerosis, diabetes, allergy, and asthma represents a chronic dysfunction of the tissue and a metabolic shift from homeostasis to an imbalanced state (35).
Gut dysbiosis can be stated as a disorder in the microbial organ as the host loses control over it. Microbial dysfunction causes imbalances in metabolite production affecting multiple organs and leading to various NCDs (26, 36, 37). This disruption incapacitates the host curbing the stream of oxygen into the colon – leading to a major switch in the microbial signature – a dominance of facultative over obligate anaerobes (38, 39). Augmentation of the facultative anaerobe (phylum proteobacteria) is observed in humans inclined to a western-style diet or receiving antibiotic therapy or suffering from IBS, IBD, metabolic syndrome, necrotizing enterocolitis, or colorectal cancer and also in various animal models of colitis and colorectal cancer (19). It is well known that antibiotics deplete the gut microbes lowering the concentration of SCFAs, which are pivotal for homeostasis. In addition, enteric facultative pathogens cause intestinal inflammation promoting transepithelial migration of neutrophils, which devour Clostridia resulting in a diminution in SCFAs (39, 40). Both the mechanisms corroborate a switch in energy metabolism from mitochondrial β-oxidation to anaerobic glycolysis, the latter escalates the oxygen levels exuding from the host colonic epithelium. Besides depletion of SCFAs, both pseudomembrane formation and colonic crypt hyperplasia contribute to an accretion of Proteobacteria via boosting the level of oxygen (and/or other respiratory electron acceptor) in the large intestine. In the following section, we will elaborately discuss how gut dysbiosis can lead to the occurrence of various NCDs.
Gut microbiome and various non-communicable diseases
As we have already started gathering, depending upon modulation of physiological, environmental, genetic, and behavioral factors for a prolonged time, various NCD conditions arise. Each year, 41 million human demises are caused by NCDs, and this death rate is 71% worldwide. More than 15 million individuals die from NCDs every year, and their ages are between 30 and 69 years. Almost 77% of all NCD deaths occur in low- and middle-income countries only. Below, we will attempt to create some causal links, beyond mere correlation, between alterations in gut microbial ecology with the onset of NCDs (3, 41). Table 1 summarizes the causative roles of microorganisms in the pathogenesis of various NCDs. Figure 3 depicts the detrimental effects produced by dysbiosis in diseased individuals in colorectal cancer, atherosclerosis, diabetes, and osteoporosis.
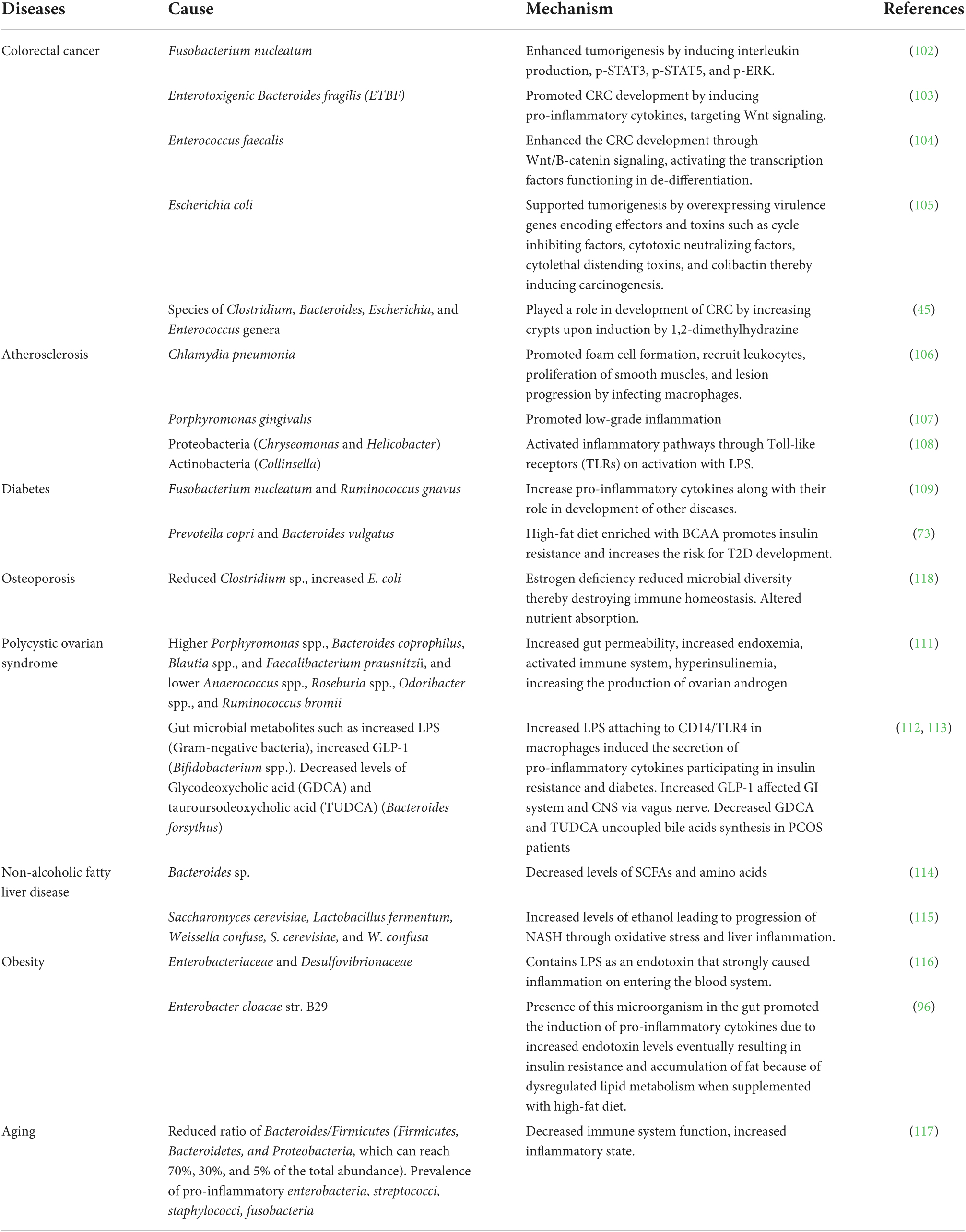
Table 1. Summary of causative microorganisms contributing to the pathophysiology of various NCDs and colorectal cancer.
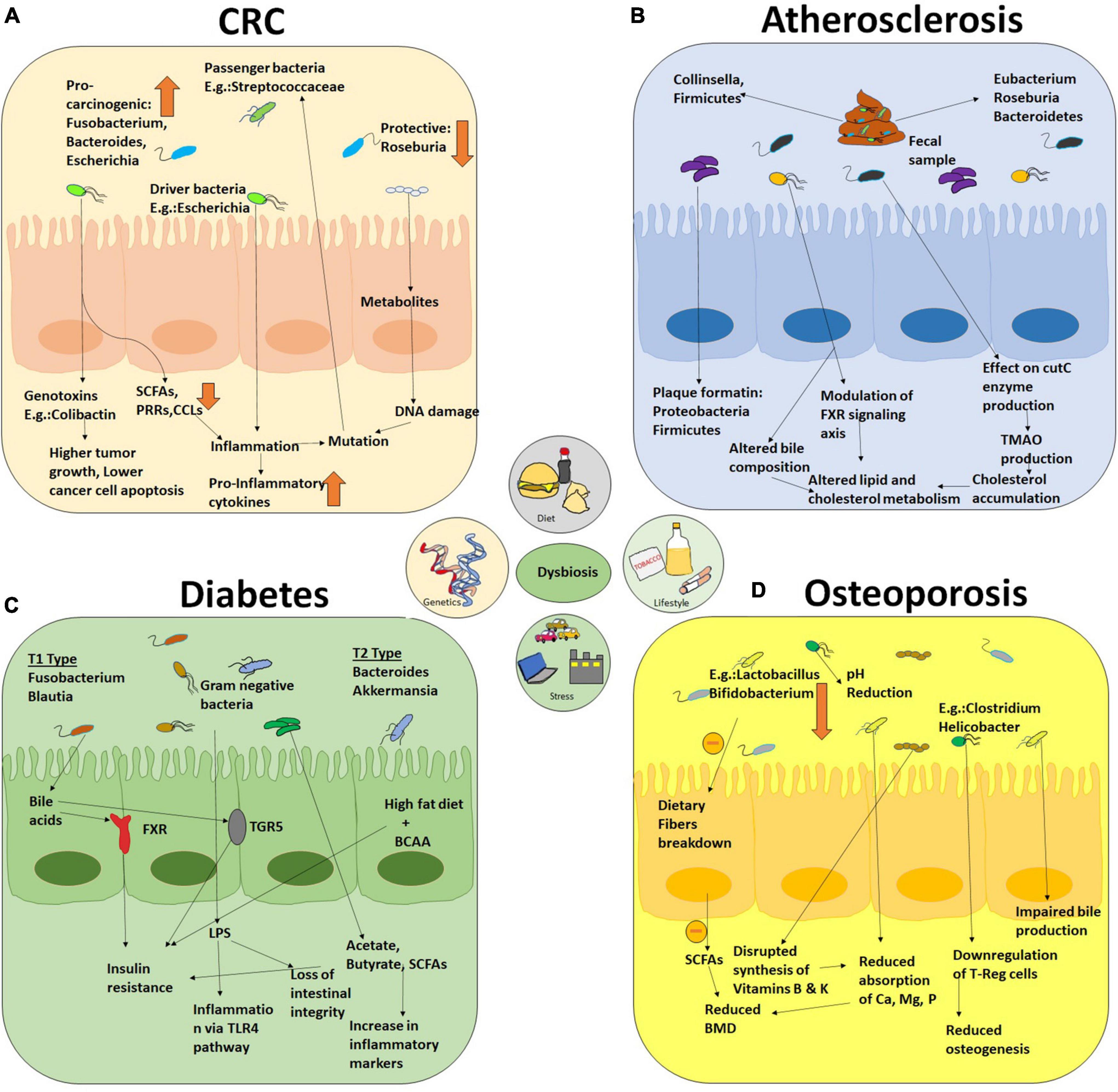
Figure 3. An overall holistic view of the workings of dysbiotic microbiota that contribute to disease pathophysiology of NCDs and some cancers. The figure includes four major diseases (A) colorectal cancer, (B) atherosclerosis, (C) diabetes, and (D) osteoporosis as examples of the detrimental effects produced by dysbiosis in diseased individuals.
Colorectal cancer
In 2018, colorectal cancer is the standard type of cancer ranked second in mortality and third in incidence globally (42). Approximately 70% of the human microbiome resides inside the colon (43). The genetic heritability of CRC is as low as 10–12%, which explains the role of microenvironment in the development of sporadic CRC (44). Several studies indicated the next part of gut microbes in CRC development. Microbial species such as Clostridium, Bacteroides, Enterococcus, and Escherichia genera were found to induce carcinogenesis by 1,2-dimethylhydrazine (45). A fecal transplant further established the role of gut microbiota in colorectum from patients with CRC to germ-free mice, which developed tumors when supplemented with azoxymethane to induce colon neoplasia (46). The studies showed the abundance of procarcinogens microbes belonging to Fusobacterium, Bacteroides, Porphyromonas, and Escherichia class with declined protective microbes such as Roseburia in patients with CRC (47–49).
The gut microbiome impacts on the development of CRC via different mechanisms, which include the microbial factors (genotoxins or metabolites). The drivers in this mechanistic pathway are SCFAs, PRRs, and several chemotactic factors (CCL17, CCL20, CXCL9, and CXCL10). Cytolethal distending toxin (CDT) and colibactin are commonly known toxins produced by Escherichia, Campylobacter, and Enterobacteriaceae spp. Some microbes may act as carcinogenic (driver microbes) and some as opportunistic bacteria (passenger bacteria) in the tumor microenvironment. This host microorganism interaction leads to the activation of several downstream signaling pathways, resulting in CRC development (50).
To describe the polymicrobial interaction in the tumor environment of CRC, Tjalsma et al. put forward the driver–passenger model. This model proposed that the driver bacterium such as Bacteroides fragilis and Escherichia coli in the tumor microenvironment releases genotoxins that promote inflammation causing premature transformations in adenocarcinoma cells thereby causing a favorable tumor microenvironment for the growth of passenger microorganisms. Passenger bacterium such as Streptococcaceae and Coriobacteriaceae proliferate in the tumor microenvironment in such a way that can outgrow the other bacterial species. The development of microbial biomarkers depends on different tumor stages. Identification of various bacterial strains surviving across different carcinogenic stages is also important in this aspect. While the driver bacteria can be directed against colorectal cancer, the passenger bacteria is associated with disease initiation, propagation, and response to treatment (51).
Ingestion of non-digestible carbohydrates leads to the production of SCFAs due to microbial fermentation in the colon. Butyrate is known as an anti-inflammatory and tumor-suppressive entity that induces apoptosis in CRC. Reduced levels of SCFAs are linked with a high risk of CRC, advanced colorectal adenoma, and ulcerative colitis development (52–54). For screening CRC, accurate biomarkers are needed, which if detected at early stages, can be treated with clinical therapies. The fecal immunochemical test (FIT) is currently used to detect CRC, with the significant drawback being 79% sensitive and 25–27% susceptible to detect advanced colorectal adenomas. Several studies have reported the utilization of microbial species to detect CRC using butyryl-Co-A dehydrogenase from F. nucleatum and RNA polymerase B subunit from P. micra quantified by PCR (49). Several reports established the association between oral microflora such as Prevotella and Streptococcus and CRC opening a new array for predicting CRC.
Atherosclerosis
In recent years, a new dimension of understanding has emerged on the pathogenesis of cardiovascular diseases, beyond the interaction of nutrition and genetic variation. Preclinical and clinical evidences have revealed that gut microbiota influences the pathogenesis of atherosclerosis (55). An involuted indication involves both inflammatory and metabolic pathways which are leveraged by the gut microbiota in three pathways: (i) acceleration of plaque formation via turning on the inflammatory immune response caused by local or distant infection, (ii) generation of ominous bacterial metabolites (viz., TMAO) from specific components of diet, and (iii) disruption of cholesterol and lipid metabolism routes of the host (56). As evident from the scientific studies discovering the presence of bacterial DNA in plaques, both direct and distant infections contribute to atherosclerotic plaques development. These bacteria are also found mostly in gut and oral cavity (57). To our intrigue, 16S rRNA analysis revealed a large abundance of Firmicutes and Proteobacteria phyla in the atherosclerotic plaques (58). Besides these, pathogenic Helicobacteraceae and Neisseriaceae were also found to be more prevalent in patients with symptomatic atherosclerosis (59). 16S shotgun sequencing experiment of fecal samples from healthy individuals, patients with atherosclerosis, and healthy volunteers with symptomatic atherosclerosis was found to vary in various aspects. Patients with disease symptoms were reported an enhancement in genus Collinsella, whereas Eubacterium and Roseburia were increased in controls. Moreover, gut dysbiosis in the patients was accompanied by an augmentation of an abundance of inflammatory genes (60). The abundance of Firmicutes got increased and Bacteroidetes got decreased when compared with healthy volunteer (61). Several preclinical and clinical studies have suggested strong causal links between gut microbiome and atherosclerosis. Compelling evidence is accumulated, which emphasizes a correlation with the CutC enzyme (encoded by gut microbe), known to convert carnitine and phosphocholine compounds to trimethylamine (TMA). Thereafter, it reaches the host liver and gets oxidized to trimethylamine oxide (TMAO), which was proven to affect cholesterol metabolism-enhancing cholesterol accumulation in macrophages and atherosclerosis development (6).
It is also evident from a few studies that patients with atherosclerosis have modified lipid metabolism, and plasma cholesterol levels are correlated with bacteria in the gut and oral cavity (57). Mechanistically, gut microbiota modulates cholesterol and lipid metabolism through altering bile acids and its farnesoid X nuclear receptor (FXR). It is now understood that bile acids help in the absorption of dietary lipids and fat-soluble vitamins. In the liver, primary bile acids are generated by being conjugated to taurine or glycine, formation of secondary bile acids is achieved by intestinal decoupling by bacterial bile salt hydrolase followed by colonic metabolism. However, gut microbial modulation of bile acid composition and signaling in FXR-expressing tissues in atherosclerosis is not been fully explored yet and remains as a matter of intense investigation (62). There is no denying the fact that a perpetual interaction between host and gut microbiota over a life span would determine the speed of disease propagation. To establish the causal relationship between a species or metabolites and atherosclerosis, it is, therefore, imperative to establish proper linkages of microbiota to distinct functions.
Diabetes
The widespread presence of obesity in the majority of the population increases the development of other metabolic NCDs such as CVD and T2DM (63). These disorders alone contribute to approximately 80% of premature mortality, with diabetes mellitus affecting nearly 347 million people globally (64). Lifestyle, obesity, malnutrition, and other health-related disorders contribute majorly to CVD and T2D in the Indian population (65). Recent studies showed the link between diet and the microbiome inhabiting the gut and their contribution to food digestion and absorption. Studies reported that the Bifidobacteria, Prevotella, and Akkermansia majorly inhabit individuals consuming the plant-based fiber-rich diet. In T2DM, Bacteroides, Akkermansia, Ruminococcus, and Faecalibacterium were found in abundance, while Roseburia were found to be in lower concentrations. Intestinal microbiota, disruption in the intestinal barrier, and inflammation affect the development of diabetes and obesity. Alteration in gut microflora due to external factors such as diet affects the metabolism causing diabetes and insulin resistance. LPS secreted by Gram-negative gut microflora produces inflammatory cytokines causing inflammation through TLR4 signaling pathway (66). The increased LPS was also found to destroy the integrity of the intestine, causing high LPS absorption. While acetic acid and butyrate have been reported to enhance the intestinal barrier functioning linked to insulin resistance and inflammation, SCFAs are also known to regulate glucose homeostasis through G protein activation of the Langerhans cells (67, 68).
When fecal bacteria transplantation was performed in insulin-resistant patients from insulin-sensitive patients, significant insulin sensitivity was observed with an abundance of butyrate-producing bacteria, which was observed on analysis as Faecalibacterium prausnitzii (69). Gut microbes transform the bile acid synthesized in the liver into secondary bile acids through enzyme metabolism. These secondary bile acids regulate insulin sensitivity through Farnesoid X receptor (FXR) and the Takeda G protein-coupled receptor 5 (TGR5) (70). Branched-chain amino acids (BCAA) are a critical predictive marker for T2D and are associated with the risk of developing T2D (71, 72).
A high-fat diet with BCAA also leads to insulin resistance has also been reported. Other studies with humans supported that BCAA with a high fiber supplementation escalates the risk of T2D development. The microflora associated with BCAA synthesis is majorly Prevotella copri and Bacteroides vulgatus (73).
Osteoporosis
Osteoporosis is a disease often marked by decreased bone mass per unit volume and wear and tear of bone tissue microstructure, increasing the susceptibility to fracture. It can occur irrespective of age and sex and is common in postmenopausal women. Nutrition, heredity, lifestyle, and hormone levels are known to contribute toward the development of the malady. The four distinct phases of the bone remodeling cycle comprise initiation, resorption, reversal, and formation. Endosteal cells, osteoclasts, osteoblasts, and osteocytes together form the bone remodeling units that participate in the bone renewal process (74). The main regulators participating in bone metabolism include vitamin D, estrogen, inflammatory factors, and parathyroid hormones. Menopausal women often manifest primary osteoporosis thereby rendering menopause as a major risk factor of the same (75). In contrast, pathological factors such as inflammatory bowel disease, parathyroid disease, glucocorticoid therapy, type 1 diabetes, arthritis, and smoking lead to secondary osteoporosis’s onset (76).
The gut microbial composition can surely influence nutrient absorption. Upraised abundances of microbes such as lactobacillus and bifidobacteria were shown to inhabit the human intestinal tract, promoting the absorption of phosphorous, calcium, and magnesium, leading to increased bone mineral density (BMD) (77). It was also shown that the diversity of gut microbes could affect the pH of the gut, further affecting nutrient absorption, specifically, calcium absorption. These microbes also participate in bile acid metabolism and vitamin B and K synthesis, playing a crucial role in bone health and calcium absorption. The gut microbiota catabolizes the macromolecules into simpler components facilitating the ease of nutrient absorption by increasing the bone density and delaying the onset of osteoporosis (78).
Moreover, SCFAs produced by the microbial fermentation of dietary fibers regulate bone mass and osteocyte formation. Bone loss was prevented and bone mass was increased in mice fed with SCFA with ameliorated osteoporotic condition. The importance of a balanced diet in the maintenance of bone health was proved when the fecal transplantation was performed from a high-fat diet mouse to a healthy mouse that adopted the gut microflora, causing osteoporosis in healthy mice. These studies showed that the high-fat diet impairs the micro-environment of bone marrow, causing the poor reorganization of hematopoietic stem cells. HFD was also found to activate PPARg2, thereby enhancing bone marrow lipogenesis and impairing osteoblast formation (79).
According to a few recent studies, a commonality related to immune component is shared between osteoporosis and inflammatory joint disease. Function of gut microbiota may be regulated by Th17/T-reg cells. Several gut bacterial species such as Clostridium, Bifidobacterium, Helicobacter, Bacteroides, and Lactobacillus facilitate the generation of Treg cells in the colon. SCFAs, vitamin A, and estrogen are key players in maintaining the dynamic balance between Th17/T-reg cells inhibiting osteogenesis (80).
Polycystic ovarian syndrome
Polycystic ovarian syndrome (PCOS) is an endocrino-pathological disorder in which the ovaries produce exceptionally abnormal levels of the male hormone androgen. With hyperandrogenism, insulin resistance, menstrual disorders, hirsutism, infertility, and anovulation, the principal facets of the syndrome, PCOS is prevalent in 5–7% of women population in their reproductive age (81). PCOS is also accompanied by disorders such as hypertension, endometrial carcinoma, type 2 diabetes mellitus, glucose intolerance, dyslipidemia, insulin resistance, dysfunctional bleeding, cardiovascular disease, and pregnancy loss in the majority of the patients (82). Neuroendocrine, environmental factors, metabolic and immune dysfunctions, and genetics play crucial roles in developing PCOS. While polycystic appearing ovary (PAO) is preclinical, it does not fall under PCOS. In contrast, factors such as insulin resistance, obesity, dopaminergic dysregulation, and stress make the transition of PAO to PCOS in women (83).
Studies showed that the diversity of gut microbes in patients with PCOS is significantly reduced compared with control groups (84). Several genes participating in PCOS development are found to play a role in carbohydrate metabolism and steroid synthesis pathway, thus creating a link between metabolic factors and possible mechanism of PCOS development (85). The occurrence and progress of metabolic and endocrine imbalances in PCOS are affected by the intestinal microflora. In a pilot, clinical study that compared the microbiome from patients with PCOS to control patients, a decrease in Tenericutes bacterium (ML615 and S247) was observed (86). It was shown that the gut microbiota disorder in obese patients increases the energy intake of the host as the SCFAs produced from the glucose conversion stimulate the peptide YY release, which prohibits intestinal peristalsis, diminishes the pancreatic discharge, and stimulates energy absorption in the intestine (87, 88). Turnbaugh et al. showed that the Firmicutes population in the intestine was higher, and Bacteroides was lower in obese mice that increased the intake and absorption of energy and exhibited the symptoms of obesity (11). Butyrate, acetate, and propionate are the major SCFAs that activate peroxisome proliferator-activated receptor gamma (PPAR-γ), which regulates fatty acid and glucose uptake. SCFAs in the intestines, skeletal muscles, fat, immune system, nervous system, and liver are found to impede appetite-stimulating hormone (ASH) secreted by gastric mucosa that is known to inhibit the secretion of GnRH and other sex hormones. ASH is known to inhibit the CYP19A1 expression that inhibits the conversion of androgen to estrogen. Lower ASH correlates with enhanced androgen levels causing hyperandrogenemia (89). Figure 4 highlights various mechanisms responsible for PCOS development.
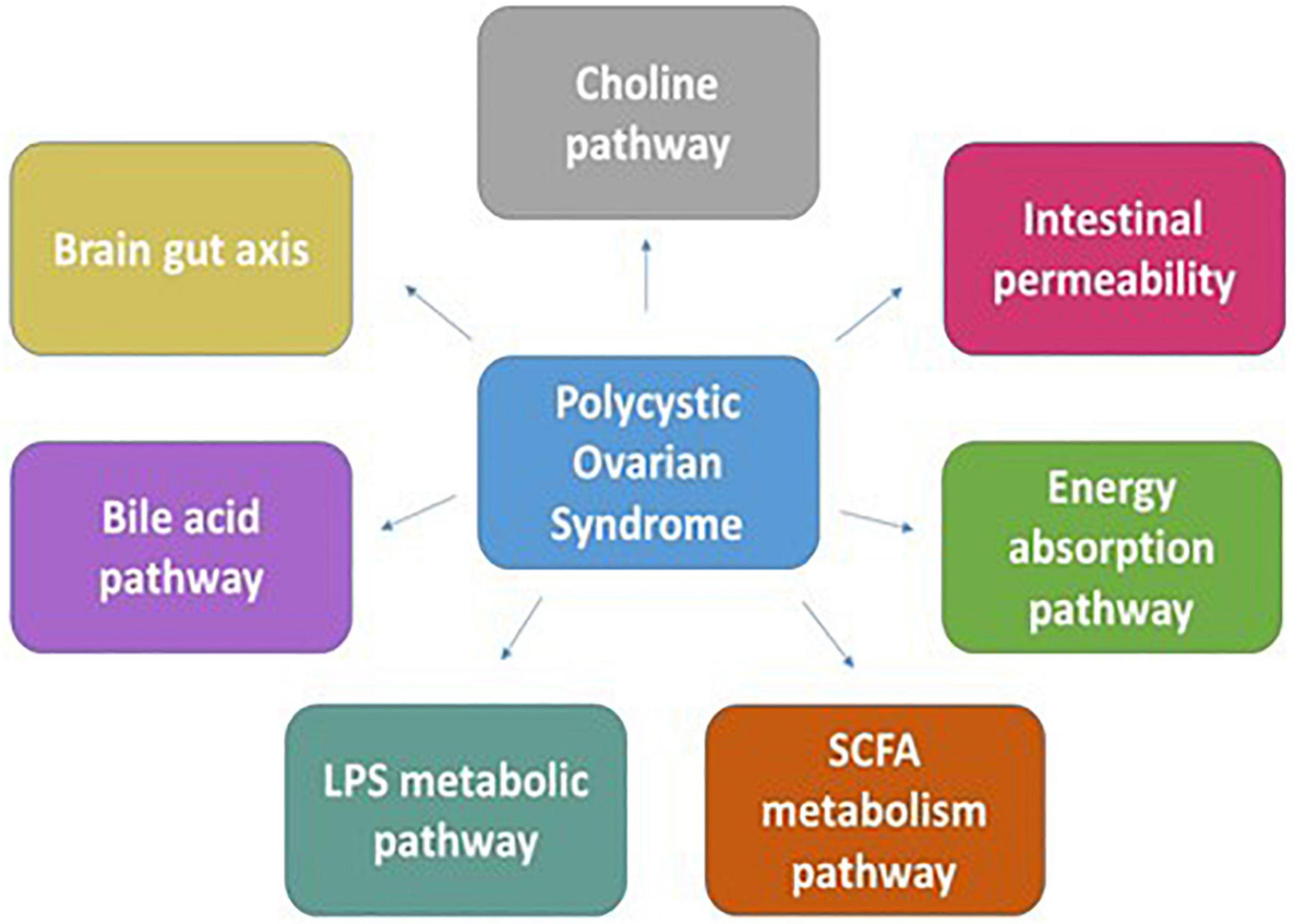
Figure 4. The figure discusses the mechanisms pertaining to the development of PCOS through the cross talk between the genes participating in pathways such as energy absorption, carbohydrate-metabolizing pathways, bile acid pathway, SCFA, and LPS metabolic pathways with the dysbiotic gut microbiota.
Non-alcoholic fatty liver disease
Non-alcoholic fatty liver disease (NAFLD) is the most common liver disease observed in the past few years. It is indicated by hepatic steatosis which may advance to liver cirrhosis, hepatocellular carcinoma, and non-alcoholic steatohepatitis. Obesity and type 2 diabetes are the major factors that lead to NAFLD development (90). It has been shown that gut dysbiosis correlates with NAFLD. The composition of the gut microbiome differs from NASH, cirrhosis, and fibrosis. The intestinal barrier is compromised due to nutrition stress in NAFLD, which leads to translocating of the gut microbe and their metabolites into the blood system causing hepatic inflammation and cirrhosis (91).
Obesity
WHO elucidated obesity as unrestrained body fat accumulation leading to increased body mass index (BMI) often caused by an imbalance between energy consumed and expended, measured in terms of calories. In general, BMI greater than 25 is considered as overweight and beyond 30 is known as obese (92, 93). A recent survey by National Health and Nutrition Examination (NHANES) found that more than two in three adults were overweight or had obesity (94). It is on a dramatic rise in low- and middle-income countries, mostly prevalent in urban areas. Nonetheless, obesity is a global burden for our health system and a leading cause of the development of metabolic diseases such as diabetes, atherosclerosis, osteoarthritis, gout, high blood pressure, and some cancers. The shifts in gut microbial composition have been found to play an important role in obesity (95). In 2016, Cindy D. Davis et al. demonstrated that obesity can be attributed to alterations in the composition of gut microbes such as diminished microbial diversity or mutations in bacterial genes and associated metabolic pathways. Obese mice exhibited a higher percentage of Firmicutes and half the amount of Bacteroidetes, with concurrent augmentation in microbial genes entailing polysaccharide breakdown compared to thin siblings (95).
Aging-related non-communicable diseases
The gut microbiome provides several benefits such as aiding in digestion and food absorption, metabolization of fibers to produce beneficial SCFAs, nutrient and vitamin synthesis, regulation of host immunity, and integrity of intestine. The gut microbial composition depends on the acidity of the small intestine, stomach and colon, lifestyle, and racial and geographical differences. The microbial composition shifts its function from beneficial to the host toward causing inflammation in individuals with morbid obesity. A similar trend was observed during aging that caused diseases such as CVD, Alzheimer’s disease, insulin resistance, T2DM, and frailty. Compositional differences between these gutbacterial species were observed more in aged population than young individuals. The reduction in gut microbiome diversity was observed concerning age in aged mice, having reduced bacterial biosynthesis of biotin and cobalamin, enhanced creatine degradation and SOS genes associated with DNA repair.
A model, hypothesized to examine the impact of the gut microbiome on aging, was later proved to be correct. It states that microbial dysbiosis increases the intestinal permeability triggering the inflammatory response of the host. This induction of inflammation affects the microbial composition, further promoting dysbiosis creating a feed-forward loop (96). Gut microbial population and its density have influenced systemic inflammation (97). Extrinsic elements including diet and lifestyle determine microbial composition and related dysbiosis (98). When tested on model organisms, the high-fat and meat-dominated diet correlate with microbial dysbiosis (99). In contrast, the Mediterranean diet was shown to induce a healthy gut microbiome (100).
Many gut microbiome and biomolecules synthesized by them, such as SCFAs, vitamins, gut-derived hormones, and other chemical compounds, affect life span and health (101). Table 1 mentions the microbes that modulates the life span.
Immunomodulatory nutraceuticals toward management of non-communicable diseases
It is discernible from our prior discussion that nutraceuticals can play various roles toward the prevention and management of the NCDs. In this section, we shall focus on their immunomodulatory aspects with a view to develop more efficacious formulations. Figure 5 depicts the therapeutic immunomodulatory role of various nutraceuticals (such as prebiotics, probiotics, and phytochemicals) toward the management of CRC, atherosclerosis, diabetes, and osteoporosis.
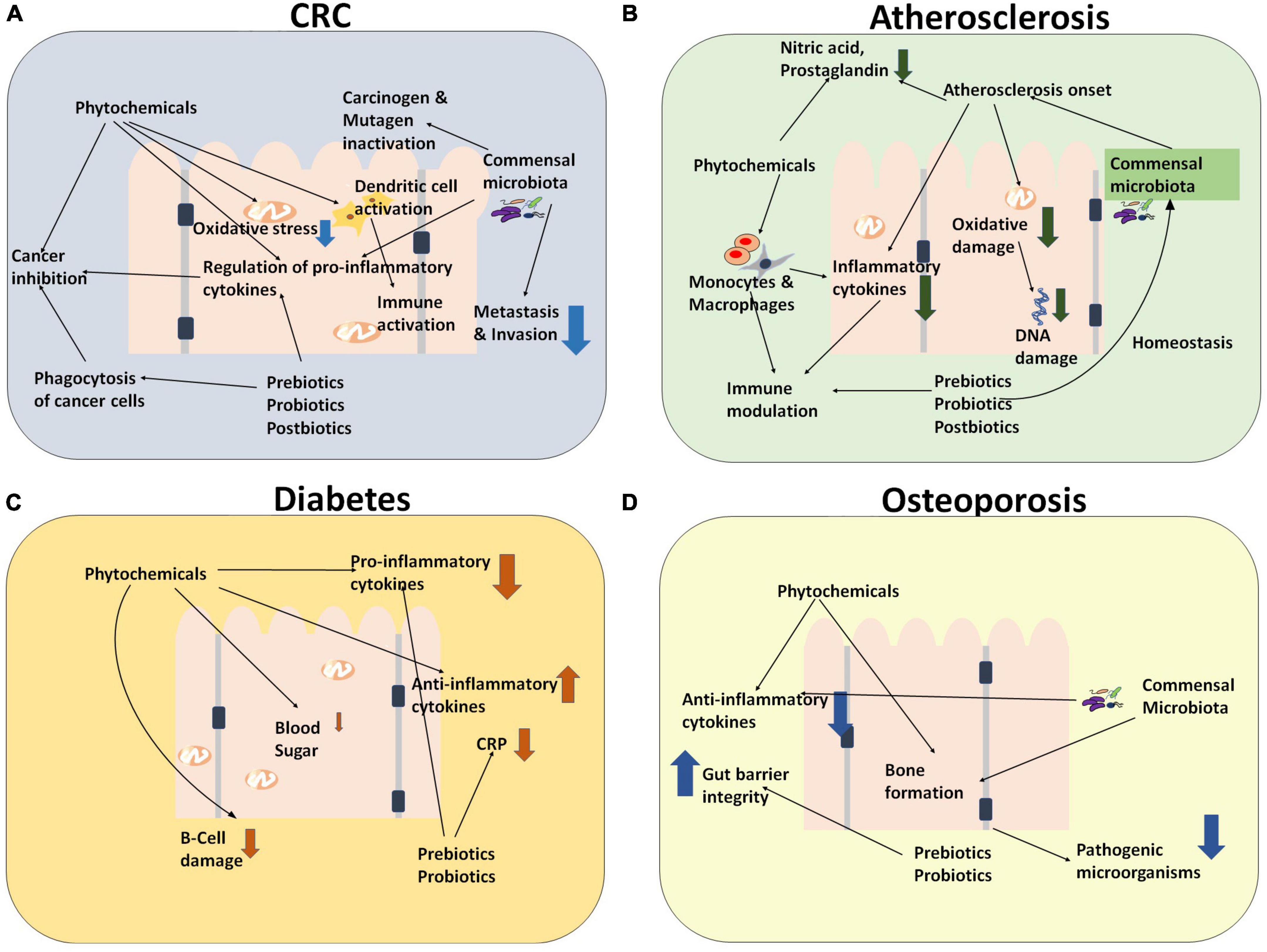
Figure 5. Diagrammatic representation for the immunomodulatory role of various nutraceuticals (such as prebiotics, probiotics, and phytochemicals) wherein they exert a combinatorial effect through several pathways toward the management of (A) CRC and NCDs [such as (B) Atherosclerosis, (C) diabetes, and (D) osteoporosis].
Colorectal cancer
Colorectal cancer (CRC) still remains an arduous burden for our health system (118). In 2020, 935,000 deaths have been reported by WHO in both men and women. American Cancer Society (ACS) anticipated that number of new patients with CRC would reach around 2.4 million in the world until the year 2035 (119, 120). Global research would not deny that infection and dysbiosis are closely related to CRC and some nutraceutical compounds including phytochemicals, probiotics, prebiotics, and postbiotics have displayed prominent immunomodulatory roles in the prevention and management of the same. For instance, quercetin, silymarin, and curcumin have shown promising immunomodulatory effects against CRC management, viz., quercetin activated the dendritic cell and thereby antigen presentation, curcumin induced apoptosis and silymarin inhibited Wnt signaling in human colorectal cancer cells (121–124). Few research groups also found some phytonutrient and plant secondary metabolites which exhibited positive outcome toward growth inhibition of CRC by regulating pro-inflammatory cytokines (125, 126). It has been well understood that healthy commensals in gut microbiota such as L. casei, L. plantarum, L. bulgaricus, L. acidophilus, and Bifidobacterium longum can inactivate carcinogens or mutagens, alter cell differentiation and induce immunomodulatory effects toward growth inhibition of cancer cells (127). Several preclinical and clinical studies demonstrated that prebiotics, probiotics, and postbiotics can improve the immune response such as stimulation of cytokines, viz., IL-6, IL-17, and IL-23, and the downregulation of induction of pro-inflammatory Th17 cells (128). Recently, Yan Li et al. showed that the expression of GPR109A was increased and tumor counts were decreased when postbiotic butyrate got elevated in colon polyposis-bearing mice treated with prebiotic (129). The detailed effect of immunomodulatory nutraceuticals on CRC has been depicted in Figure 5 and Table 2.
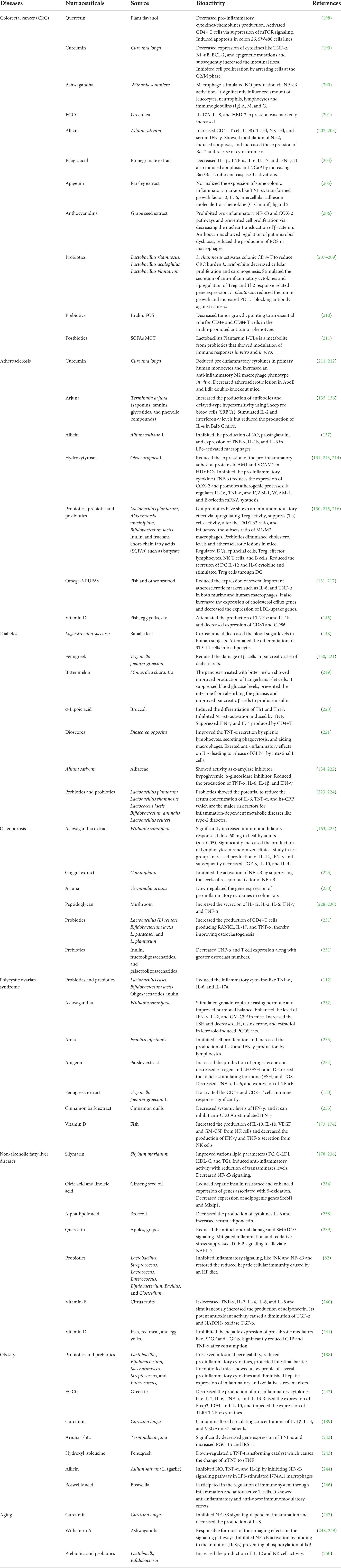
Table 2. Immunomodulatory roles of the nutraceuticals in CRC, and in various NCDs including atherosclerosis, diabetes, osteoporosis, polycystic ovarian syndrome, non-alcoholic fatty liver diseases, obesity, and aging.
Atherosclerosis
Atherosclerosis is elucidated as an accumulation of cholesterol and conscript of macrophages into artery walls yielding plaques (130). As mentioned in the previous section, gut dysbiosis has been proposed to be directly associated with acute or chronic dysfunctions of atherosclerosis in the host. Intriguingly, in the last two decades, a number of nutraceuticals have shown their potential toward the management of atherosclerosis (131, 132). Much to our interest, preclinical and clinical evidences have been accumulated for their immunomodulatory role in the disease management as well. For instance, curcumin inhibited the production of IL-8, MIP-1α, MCP-1, IL-1β, and TNF-α by LPS-stimulated on human peripheral blood monocytes and alveolar macrophages. It ameliorates experimental autoimmune myocarditis (EAM) by reduced the inflammation in inflammatory macrophages and polarized M0 and M1 macrophage to M3 phenotype (133, 134). In addition, other groups also revealed that phytochemicals such as Terminalia arjuna extract had a prominent effect on the management of atherosclerosis. For example, in 2009, Halder et al. reported that T. arjuna possesses anti-inflammatory potential against some phlogistic agents as well as antinociceptive activity plausibly mediated via opioid receptors (135, 136). Similarly, Da Yeon Lee et al. (137), demonstrated that Allicin minimized inflammatory cytokines expression in murine such as IL-6, IL-1β, and TNF-α in macrophages stimulated with LPS (138). It was also demonstrated that hydroxytyrosol has played a major role toward diminishing cytokines IL-12 and IL-23 and Th1 and Th17 activation inhibiting atherosclerosis progression (139, 140). Similarly, in Qiang Wan et al. demonstrated that Berberine has decreased serum levels of IL-6 and TNF-α, which played a key role in pathogenesis of atherosclerosis (141). Interestingly, green tea extract increased the production of nitric oxide leading to enhanced vasodilation. Besides that, flavanols exhibited vasodilation plus lessened circulating oxLDL levels after 5 weeks (128). Moreover, prebiotics, probiotics, and postbiotics (SCFAs) are showing promising results toward the homeostasis of gut microbiota which is leading to counter the onset of atherosclerosis. For example, Lactobacilli have shown immunomodulatory effects against atherogenesis such as enhancement of the activity of Tregs, suppression of Th1, Th17, modification of Th1/Th2 ratio, influencing the subsets ratio of M1/M2 macrophages (142, 143). Few other groups reported that Mediterranean diet, antioxidant phytonutrient like coenzyme Q10, bioactive compounds like vitamins D, E, A and C, polyunsaturated fatty acids (ω-3 and ω-6), etc. are showed prominent effects for management of CVD (131, 144, 145). Taken together, immunomodulatory nutraceuticals can be used alone or concurrent with other pharmaceutical treatment modalities to prevent and manage atherosclerosis and to ameliorate the QoL of the patients.
Diabetes
Diabetes mellitus (DM) is a metabolic disease, which takes its origin from several genetic and environmental factors and characterized by insulin resistance or impaired production of insulin hormone from pancreatic β-cell, which creates an enormous health burden with micro as well as macrovascular complications. As reported by International Diabetes Federation in 2019, diabetic population was estimated to be 463 million and it was predicted to increase up to 700 million by 2045 (146). As mentioned in the previous section, alteration in gut microbial composition can largely contribute to the onset of the disease. Herein, we aim to summarize some immunomodulatory nutraceuticals including botanicals and probiotics, which can help in the management of diabetes. In 2012, Toshihiro Miura et al. reported that Lagerstroemia speciosa L., a rich source of corosolic acid as well as ellagitannins, was responsible for blocking the activation of NF-κB in a dose- and time-dependent manner in H9c2 cell line, which modulated anti-inflammatory action resulting in inhibition of diabetes-induced cardiomyocyte hypertrophy (147, 148). Similarly, other research groups (Sneha J. Anarthe et al. and Neelam Makare et al.) demonstrated that 500 mg/kg of Trigonella foenum-graecum increased the population of lymphocytes and T cell (149–151). Bitter melon, α-lipoic acid, dioscorea, allium sativum, and amaranthus have also shown positive antidiabetic immunomodulatory effects (152–156). In Bahare Salehi et al. reported that α-lipoic acid has the potential to be used for the management of diabetes and other NCDs including Alzheimer (157). In addition, Giuseppe Derosa et al. recommended that L-carnitine, α-lipoic acid, berberine, and ω-3 fatty acids might be useful toward the management of diabetes (158). Probiotics (bifidobacteria, lactobacilli, and Streptococcus thermophilus) have been demonstrated to be a powerful arsenal to combat central components of metabolic syndrome, like T2D (159). The detailed immunomodulatory effects of the nutraceuticals toward the inhibition of diabetes are summarized in Table 2 and Figure 5.
Osteoporosis
Osteoporosis, mostly afflicting postmenopausal women, is a bone metabolic disorder characterized by bone loss leading to an enhanced risk of fracture (110). As reported by Jing Yan et al. in resident microbes promote bone formation and prolonged exposure results in net skeletal growth. Hormone IGF-1, produced by microbiota, promotes bone development and remodeling (160). Other groups also demonstrated that gut microbiota played a key role in bone homeostasis, regulated bone metabolism through various pathways, endocrine system and through immune system, and promote on calcium balance (110, 161). Importantly, with a continuous accumulation of knowledge, it was disclosed that the “brain–gut” axis may be a potential target for the bone, which affects the onset and propagation of osteoporosis. Yuan-Wei Zhang et al. demonstrated that the monitoring of TNF+ T and Th17 inflammatory cells in the bone marrow improved the overall inflammatory state. It may be called the “brain-gut–bone” axis (162). Accumulated preclinical evidence also account for the nutraceuticals playing a potential role in the management of bone loss past menopause. Some phyto-nutraceutical compound showed positive results against osteoporosis. For instance, in 2021, Tharakan and co-workers reported a positive immunomodulatory effect of Withania somnifera extract during preclinical studies which significantly increased the production of cytokines IFN-γ, IL-4, and CD45+, CD3+, CD4+, CD8+, and CD19+ NK cells (163). Moreover, Zaffar Azam et al. recently reported that guggul extract, arjuna, coriolus versicolor, and Punica granatum showed a promising immunomodulatory effect for the management of bone health (164). Similarly (165, 166) reported that probiotics and prebiotics can help to preserve gut barrier integrity, protect against pathogenic microorganisms, and promote alteration of CD4+ T cell activation, which can modulate osteoclastogenic cytokine production (165, 166). A research group demonstrated that vitamin D has played some role in the prevention and management of osteoporosis via regulating calcium–phosphorus homeostasis controlling Treg differentiation, reducing Th17 cell response, and inflammatory cytokines secretion (167, 168). The details immunomodulatory effect is depicted in below Figure 5 and Table 2.
Polycystic ovarian syndrome
Polycystic ovarian syndrome (PCOS) is a gynecologic endocrine metabolic disease, particularly affecting women of reproductive age (111). As evident from prior scientific research, gut microbiota can influence the pathogenesis and clinical manifestations of PCOS (84). Recently, Fang-fang He et al. reported that gut dysbiosis occurs in PCOS animal models and patients with PCOS which hint at an apparently ambiguous role in the prevalence of Prevotellaceae (169). To our intrigue, other nutraceutical compounds have also shown their potential toward the management of PCOS. For example, probiotics and prebiotics (Lactobacillus casei, Bifidobacterium lactis, Lactobacillus plantarum, Lactobacillus rhamnosus, oligosaccharides, inulin) have shown a reduction in inflammatory cytokines (TNF-α and IL-17a) in patients with PCOS as reported by Gamze Yurtdaş et al. (111, 170). Similarly, other research groups (Bilal Bin-Hafeez et al. and Sneha J. Anarthe et al.) demonstrated a dose-dependent immunomodulatory activity of methanolic extract of fenugreek (149, 150). It has also been demonstrated that phytonutrients such as ashwagandha, amla, apigenin, and cinnamon bark extract furnish promising immunomodulatory effects for the management of PCOS, the details are summarized in Table 2 (171, 172). Moreover, in Kuniaki Ota et al. demonstrated that vitamin D has shown an immunomodulatory effect inhibiting the proliferation of Th1 cells and limit their cytokine production, such as IFN-γ, IL-2, and TNF-α. Some contradictory behavior of vitamin D has also been recorded (173, 174). In summary, immunomodulatory nutritional intervention can be used alone to prevent and manage PCOS or concurrent with other pharmaceutical treatment modalities to ameliorate the quality of life of the patients.
Non-alcoholic fatty liver disease
Non-alcoholic fatty liver disease (NAFLD) is one of the leading causes of mortality and morbidity all over the world. The prevalence of NAFLD is projected in 2020 to increase up to 56% in the next 10 years (175). It is majorly caused by an accretion of fatty acid content greater than 5% of liver weight (176). Moreover, gut microbiome, its metabolites and their interactions with the immune system - together entails the pathogenesis of NAFLD and hepatocellular carcinoma (HCC) via gut–liver axis (177). Herein, we highlight some nutraceuticals, vitamins, prebiotics, and probiotic supplements, which showed positive outcome in the management of NAFLD in few preclinical and clinical settings. For instance, in Annalisa Curcio et al. demonstrated that Silybum marianum, having antioxidant and anti-inflammatory activity and comprising ∼70–80% of silymarin with a mixture of flavonolignans and silybin, improved steatosis and liver enzymes with patients with NAFLD (178). Similarly, other research groups showed that gut microbiota–derived tryptophan metabolites (I3A) weakened the expression of TNF-α, IL-1β, and MCP-1 on macrophages exposed to palmitate and lipopolysaccharide (179). In addition, probiotics/synbiotics are used as supportive supplement diets to reduce inflammation, hepatic steatosis, and liver stiffness as shown in meta-analysis (180, 181). Importantly, in 2017, Kelishadi and colleagues demonstrated that a probiotic blend containing Bifidobacterium lactis (DSMZ 32269), Lactobacillus acidophilus (ATCC B3208), Lactobacillus rhamnosus (DSMZ 21690), and Bifidobacterium bifidum (ATCC SD6576) had shown a positive result after 12 weeks of treatment on patients with pediatric NAFLD along with reduced liver injury compared to placebo treatment (180–182). Immunomodulatory effects of various nutraceuticals for the management of NAFLD are summarized in below Table 2.
Obesity
Though obesity cannot strictly be stated as NCD, it is a well-known risk factor that can beget several metabolic disorders such as CVD and T2DM. It is proven that certain gut bacterial genera are associated with obesity, making microbiome modulation an attractive tool for its management. In Aline Corado Gomes et al. demonstrated various molecular patterns that lead together to obesity, such as immune system, lipid metabolism, satiety hormones, nutrient metabolism, and microbiota–adipose tissue axis (183). Thus, maintaining homeostasis and attenuation of the exaggerated inflammatory reaction owing to dysbiosis is required in order to prohibit the onset of obesity which makes probiotics a therapeutic modality against the disorder. For example, Lactobacillus gasseri SBT2055, Bifidobacterium L66, and Bifidobacterium adolescentis drew attention as they altered the composition of gut microbiota and affected food intake, appetite, body weight, body composition, and metabolic functions involving GI pathways (184). A few other groups also evaluated the effects of prebiotics on obesity. For instance, prebiotic-fed mice showed a low profile of several pro-inflammatory cytokines and diminished hepatic expression of inflammatory and oxidative stress markers that could maintain gut homeostasis and control obesity (185–188). Phytonutrients have also shown some promise in obesity management. As reported by Shiva Ganjali et al. in (162), curcumin showed a promising role in obesity management. When obese individuals treated with 1 g curcumin/day in a 4-week long randomized crossover trial, the mean serum IL-1β (p = 0.042), IL-4 (p = 0.008), and VEGF (p = 0.01) were found to be significantly reduced (189). Similarly, other authors recently reported that phytonutrients such as green tee, arjunarishta, fenugreek, and boswellic acid played a major role toward controlling obesity (190, 191). Table 2 elucidates the immunomodulatory effects of these ingredients.
Aging
Aging is a complex phenomenon spawned out of the interaction of environmental, genetic, and/or epigenetic events interfering with body’s functions with time. Age-related chronic disorders are progressively increasing due to the increased life expectancy in the elderly population. These can be attributed to the compositional shift in the gut microbiota which remains associated with low-grade inflammation and innate immunity activation which can trigger many metabolic dysfunctions. Therefore, gut microbiota can be considered as a target for the elderly population to reverse aging as well as inhibit metabolic ailments (117). Here, we mention the effects of some phytonutrients, prebiotics, and postbiotics supplements which have demonstrated their potential to ameliorate the gut dysbiosis and to direct toward healthy aging (192). In preclinical studies, curcumin demonstrated symptomatic reduction in some age-related diseases such as CVD, T2DM, and cancer owing to its well-known anti-inflammatory property via inhibiting NF-αB signaling (193). Other research groups have also demonstrated that Shilajit, Withaferin A, and prebiotics and probiotics can play crucial roles in the management and prevention of aging-associated immune compromise and metabolic diseases including cancer, diabetes, and obesity (194, 195). Probiotic supplementation increased NK cell and phagocytic activity, mostly effective in elderly population. It also ameliorated the detrimental effects of malnutrition on immunity in elderly people by improving their nutritional and immune status, as demonstrated by increasing levels of serum albumin and intestinal immunoglobulin A (IgA) production (196). In 2021, Xin Fang et al. demonstrated that the antiaging effects of the probiotic has been regulated intestinal microbiota and inhibited TLR4/NFκB-induced inflammation in mouse model (197). The effect of a probiotic blend of two Bifidobacterium species in a South Korean elderly (65 years) population after 12 weeks was measured to have reduced abundance of Prevotellaceae family and Eubacterium, Clostridiales, and Allisonela order which results in improved cognitive function and stress management capacity further reinforcing the significance of gut–brain axis. The details of immunomodulatory effects of various nutraceuticals on aging are depicted in Table 2.
Summarizing the above facts, it is quite palpable to decipher the mechanistic roles of the vast majority of the nutraceuticals are playing, which can be validated and translated into beneficial formulations with better efficacy.
Future perspective
So far, the causative roles of microbes in non-communicable diseases, some cancers, and immunomodulation via nutraceutical management are recognizable. The prevalence of microbes in gut is dependent on several factors such as immunity, food, and external environment. Several other agents such as antibiotics, mutagens, or carcinogens can influence human microbiome via immunosuppression, oxygen deprivation, biofilm formation, etc. Though fecal microbiome transplant (FMT) technology from a healthy donor has gained popularity, the United States Food and Drug Administration (FDA) recently issued several restrictions on FMT and its trials after numerous infections and one death was reported (251–254). It is also important to study cohort for the health status, effect of food habits, and age of a person to harvest gut microbiome/fecal microbiome to get rid of accidental pathogenesis. Microbial biobanks can be established in various countries abiding by the law of the lands by collecting microbial communities from healthy donors at various stages of their lives. Considering the degree and frequency of the NCD occurrence, these preserved microbial communities can be transplanted. Periodical preservation of fecal microbiome and/or gut microbiota can be a probable substitute in order to develop a personalized FMT for dysbiosis but deciding timeline for harvesting fecal microbiome for preservation is the biggest challenge. Metagenomic analysis methods can guide both taxonomic and functional information from diverse microbial groups. Despite significant efforts directed toward culturing and classifying microbial diversity within gut ecosystem, it still remains difficult to identify biological role of some microbial community that are low in number or nutrient provided for culturing is insufficient. Correct understanding of this can be exploited further to formulate immunomodulatory nutraceutical products for the management of several non-communicable diseases affected by dysbiosis of human microbiome. Another important aspect that should be exhaustively explored in the future is the prediction of the occurrence of NCDs and some cancers by analyzing the gut microbiome or fecal microbiome. Considering varying demography, food habits, and ethnicity, different cohorts can be chosen for experimentation and analysis. Once precisely predicted, numerous preventive measures can be taken to avoid the incidence of the maladies. Personalized precision nutraceutical interventions can be suggested once the disease has been detected. Various nano-theranostics (therapy plus diagnostics) modalities can be discovered and utilized toward successful diagnosis and eradication of the disease. For effective execution of these kind of studies, close collaborations between academic institutes, hospitals, and industries are required. More of these collaborations should be encouraged and implemented by the regulatory agencies.
Conclusion
In summary, identification of the various microbes causing low-grade inflammation over a period of time in the human system which eventually leads to the onset of the pathogenesis of several NCDs and some cancers are of immense importance. It is also equally important to harness therapeutic benefits from the nutraceuticals in terms of their immunomodulatory activities toward inhibiting the pathogenesis and progression of the aforementioned maladies. Here, we attempted to present a broad summary of these two interconnected phenomena which can open new avenues to address the bleak ramifications of various NCDs and cancers. We envision that a plethora of novel therapeutics can be generated based on systematic analysis of the cause and effectuating the personalized precision medicine which can be begotten from the immunomodulatory nutraceuticals in recent future.
Author contributions
AM, KN, and RC contributed to the conceptualization. AK, KS, DB, KN, RC, PP, and AM contributed to the manuscript writing. AM contributed to the editing. All authors contributed to the article and approved the submitted version.
Funding
This study received no external funding. The APC was funded by Esperer Onco Nutrition Pvt. Ltd.
Acknowledgments
The authors acknowledge Esperer Onco Nutrition Management and BITS-Pilani (Hyderabad) Management for their support. The authors also acknowledge Centre for Human Diseases and Research (CHDR), BITS Hyderabad for their continuous support.
Conflict of interest
AK, RC, and AM were employed by the company Esperer Onco Nutrition Pvt. Ltd.
The remaining authors declare that the research was conducted in the absence of any commercial or financial relationships that could be construed as a potential conflict of interest.
Publisher’s note
All claims expressed in this article are solely those of the authors and do not necessarily represent those of their affiliated organizations, or those of the publisher, the editors and the reviewers. Any product that may be evaluated in this article, or claim that may be made by its manufacturer, is not guaranteed or endorsed by the publisher.
References
1. Ogoina D, Onyemelukwe GC. The role of infections in the emergence of non-communicable diseases (NCDs): compelling needs for novel strategies in the developing world. J Infect Public Health. (2009) 2:14–29. doi: 10.1016/j.jiph.2009.02.001
2. Vadjdi S, Farjam M. Communicable diseases and non-communicable diseases: which one is the priority in the health policies? Galen Med. J. (2017) 6:1–2. doi: 10.31661/gmj.v6i1.851
3. Selway CA, Sudarpa J, Weyrich LS. Moving beyond the gut microbiome: combining systems biology and multi-site microbiome analyses to combat non-communicable diseases. Med Microecol. (2022) 12:100052. doi: 10.1016/j.medmic.2022.100052
4. Byndloss MX, Baumler AJ. The germ-organ theory of non-communicable diseases. Nat Rev Microbiol. (2018) 16:103–10. doi: 10.1038/nrmicro.2017.158
5. Finlay BB. CIFAR humans. Are noncommunicable diseases communicable? Science. (2020) 367:250–1. doi: 10.1126/science.aaz3834
6. Tang WW, Hazen SL. The contributory role of gut microbiota in cardiovascular disease. J Clin Investig. (2014) 124:4204–11. doi: 10.1172/JCI72331
7. Masrour-Roudsari J, Ebrahimpour S. Causal role of infectious agents in cancer: an overview. Caspian J Intern Med. (2017) 8:153.
8. Plummer M, de Martel C, Vignat J, Ferlay J, Bray F, Franceschi S. Global burden of cancers attributable to infections in 2012: a synthetic analysis. Lancet Glob Health. (2016) 4:609–16. doi: 10.1016/S2214-109X(16)30143-7
9. Helmink BA, Khan MA, Hermann A, Gopalakrishnan V, Wargo JA. The microbiome, cancer, and cancer therapy. Nat Med. (2019) 3:377–88. doi: 10.1038/s41591-019-0377-7
10. Qin J, Li R, Raes J, Arumugam M, Burgdorf KS, Manichanh C, et al. A human gut microbial gene catalogue established by metagenomic sequencing. Nature. (2010) 464:59–65. doi: 10.1038/nature08821
11. Turnbaugh PJ, Ley RE, Mahowald MA, Magrini V, Mardis ER, Gordon JI. An obesity- associated gut microbiome with increased capacity for energy harvest. Nature. (2006) 444:1027–31. doi: 10.1038/nature05414
12. Chung H, Pamp SJ, Hill JA, Surana NK, Edelman SM, Troy EB, et al. Gut immune maturation depends on colonization with a host-specific microbiota. Cell. (2012) 149:1578–93. doi: 10.1016/j.cell.2012.04.037
13. Lopez CA, Kingsbury DD, Velazquez EM, Baumler AJ. Collateral damage: microbiota- derived metabolites and immune function in the antibiotic era. Cell Host Microbe. (2014) 16:156–63. doi: 10.1016/j.chom.2014.07.009
14. O’Hara AM, Shanahan F. The gut flora as a forgotten organ. EMBO Rep. (2006) 7:688–93. doi: 10.1038/sj.embor.7400731
15. Tap J, Mondot S, Levenez F, Pelletier E, Caron C, Furet JP, et al. Towards the human intestinal microbiota phylogenetic core. Environ Microbiol. (2009) 10:2574–84. doi: 10.1111/j.1462-2920.2009.01982.x
16. Noverr MC, Huffnagle GB. The ‘microflora hypothesis’ of allergic diseases. Clin Exp Allergy. (2005) 35:1511–20. doi: 10.1111/j.1365-2222.2005.02379.x
17. Packey CD, Sartor RB. Commensal bacteria, traditional and opportunistic pathogens, dysbiosis and bacterial killing in inflammatory bowel diseases. Curr Opin Infect Dis. (2009) 22:292. doi: 10.1097/QCO.0b013e32832a8a5d
18. Franzenburg S, Walter J, Kunzel S, Wang J, Baines JF, Bosch TC, et al. Distinct antimicrobial peptide expression determines host species-specific bacterial associations. Proc Natl Acad Sci USA. (2013) 110:3730–8. doi: 10.1073/pnas.1304960110
19. Garrido D, Dallas DC, Mills DA. Consumption of human milk glycoconjugates by infant- associated bifidobacteria: mechanisms and implications. Microbiology. (2013) 159:649. doi: 10.1099/mic.0.064113-0
20. Salzman NH, Hung K, Haribhai D, Chu H, Karlsson-Sjoberg J, Amir E, et al. Enteric defensins are essential regulators of intestinal microbial ecology. Nature Immunol. (2010) 11:76–82. doi: 10.1038/ni.1825
21. Byndloss MX, Olsan EE, Rivera-Chavez F, Tiffany CR, Cevallos SA, Lokken KL, et al. Microbiota-activated PPAR-y signaling inhibits dysbiotic Enterobacteriaceae expansion. Science. (2017) 357:570–5. doi: 10.1126/science.aam9949
22. Faith JJ, Guruge JL, Charbonneau M, Subramanian S, Seedorf H, Goodman AL, et al. The long-term stability of the human gut microbiota. Science. (2013) 34:1237439. doi: 10.1126/science.1237439
23. Rivera-Chavez F, Baumler AJ. The pyromaniac inside you: Salmonella metabolism in the host gut. Annu Rev Microbiol. (2015) 69:31–48. doi: 10.1146/annurev-micro-091014-104108
24. Freter R, Brickner H, Fekete J, Vickerman MM, Carey KE. Survival and implantation of Escherichia coli in the intestinal tract. Infect Immun. (1983) 39:686–703. doi: 10.1128/iai.39.2.686-703.1983
25. Roy CC, Kien CL, Bouthillier L, Levy E. Short-chain fatty acids: ready for prime time? Nutr Clin Pract. (2006) 21:351–66. doi: 10.1177/0115426506021004351
26. Nicholson JK, Holmes E, Kinross J, Burcelin R, Gibson G, Jia W, et al. Host-gut microbiota metabolic interactions. Science. (2012) 336:1262–7. doi: 10.1126/science.1223813
27. Wang B, Morinobu A, Horiuchi M, Liu J, Kumagai S. Butyrate inhibits functional differentiation of human monocyte-derived dendritic cells. Cell Immunol. (2008) 253:54–8. doi: 10.1016/j.cellimm.2008.04.016
28. Arpaia N, Campbell C, Fan X, Dikiy S, Van Der Veeken J, Deroos P, et al. Metabolites produced by commensal bacteria promote peripheral regulatory T-cell generation. Nature. (2013) 504:451–5. doi: 10.1038/nature12726
29. Furusawa Y, Obata Y, Fukuda S, Endo TA, Nakato G, Takahashi D, et al. Commensal microbe-derived butyrate induces the differentiation of colonic regulatory T cells. Nature. (2013) 504:446–50. doi: 10.1038/nature12721
30. Furuta GT, Turner JR, Taylor CT, Hershberg RM, Comerford K, Narravula S, et al. Hypoxia-inducible factor 1-dependent induction of intestinal trefoil factor protects barrier function during hypoxia. J Exp Med. (2001) 193:1027–34. doi: 10.1084/jem.193.9.1027
31. Lozupone CA, Stombaugh JI, Gordon JI, Jansson JK, Knight R. Diversity, stability and resilience of the human gut microbiota. Nature. (2012) 489:220–30. doi: 10.1038/nature11550
32. Renz H, Brandtzaeg P, Hornef M. The impact of perinatal immune development on mucosal homeostasis and chronic inflammation. Nat Rev Immunol. (2012) 12:9–23. doi: 10.1038/nri3112
33. Sommer F, Backhed F. The gut microbiota masters of host development and physiology. Nat Rev Microbiol. (2013) 11:227–38. doi: 10.1038/nrmicro2974
34. Rook GA. Regulation of the immune system by biodiversity from the natural environment: an ecosystem service essential to health. Proc Natl Acad Sci USA. (2013) 110:18360–7. doi: 10.1073/pnas.1313731110
35. Medzhitov R. Origin and physiological roles of inflammation. Nature. (2008) 454:428–35. doi: 10.1038/nature07201
36. Round JL, Mazmanian SK. The gut microbiota shapes intestinal immune responses during health and disease. Nat Rev Immunol. (2009) 9:313–23. doi: 10.1038/nri2515
37. Cani PD. Gut microbiota at the intersection of everything? Nat Rev Gastroenterol Hepatol. (2017) 14:321–2. doi: 10.1038/nrgastro.2017.54
38. Rigottier-Gois L. Dysbiosis in inflammatory bowel diseases: the oxygen hypothesis. ISME J. (2013) 7:1256–61. doi: 10.1038/ismej.2013.80
39. Rivera-Chavez F, Lopez CA, Baumler AJ. Oxygen as a driver of gut dysbiosis. Free Radic Biol Med. (2017) 105:93–101. doi: 10.1016/j.freeradbiomed.2016.09.022
40. Rivera-Chavez F, Zhang LF, Faber F, Lopez CA, Byndloss MX, Olsan EE, et al. Depletion of butyrate-producing Clostridia from the gut microbiota drives an aerobic luminal expansion of Salmonella. Cell Host Microbe. (2016) 19:443–54. doi: 10.1016/j.chom.2016.03.004
41. West CE, Renz H, Jenmalm MC, Kozyrskyj AL, Allen KJ, Vuillermin P, et al. The gut microbiota and inflammatory noncommunicable diseases: associations and potentials for gut microbiota therapies. J Allergy Clin Immunol. (2015) 135:3–13. doi: 10.1016/j.jaci.2014.11.012
42. Bray F, Ferlay J, Soeijomataram I, Siegel RL, Torre LA, Jemal A. Global cancer statistics 2018: GLOBOCAN estimates of incidence and mortality worldwide for 36 cancers in 185 countries. CA Cancer J Clin. (2018) 68:394–424. doi: 10.3322/caac.21492
43. Sekirov I, Russell SL, Antunes LC, Finlay BB. Gut microbiota in health and disease. Physiol Rev. (2010) 90:859–904. doi: 10.1152/physrev.00045.2009
44. Foulkes WD. Inherited susceptibility to common cancers. N Engl J Med. (2008) 359:2143–53. doi: 10.1056/NEJMra0802968
45. Onoue M, Kado S, Sakaitani Y, Uchida K, Morotomi M. Specific species of intestinal bacteria influence the induction of aberrant crypt foci by 1, 2-dimethylhydrazine in rats. Cancer Lett. (1997) 113:179–86. doi: 10.1016/S0304-3835(97)04698-3
46. Wong SH, Zhao L, Zhang X, Nakatsu G, Han J, Xu W, et al. Gavage of fecal samples from patients with colorectal cancer promotes intestinal carcinogenesis in germ-free and conventional mice. Gastroenterology. (2017) 153:1621–33. doi: 10.1053/j.gastro.2017.08.022
47. Castellarin M, Warren RL, Freeman JD, Dreolini L, Krzywinski M, Strauss J, et al. Fusobacterium nucleatum infection is prevalent in human colorectal carcinoma. Genome Res. (2012) 22:299–306. doi: 10.1101/gr.126516.111
48. Feng Q, Liang S, Jia H, Stadlmayr A, Tang L, Lan Z, et al. Gut microbiome development along the colorectal adenoma-carcinoma sequence. Nat Commun. (2015) 6:6528. doi: 10.1038/ncomms7528
49. Yu J, Feng Q, Wong SH, Zhang D, Liang QY, Qin Y, et al. Metagenomic analysis of faecal microbiome as a tool towards targeted non-invasive biomarkers for colorectal cancer. Gut. (2017) 66:70–8. doi: 10.1136/gutjnl-2015-309800
50. Sanchez-Alcoholado L, Ramos-Molina B, Otero A, Laborda-Illanes A, Ordonez R, Medina JA, et al. The role of the gut microbiome in colorectal cancer development and therapy response. Cancers. (2020) 12:1406. doi: 10.3390/cancers12061406
51. Wang Y, Zhang C, Hou S, Wu X, Liu J, Wan X. Analyses of potential driver and passenger bacteria in human colorectal cancer. Cancer Manag Res. (2020) 12:11553. doi: 10.2147/CMAR.S275316
52. Chen HM, Yu YN, Wang JL, Lin YW, Kong X, Yang CQ, et al. Decreased dietary fiber intake and structural alteration of gut microbiota in patients with advanced colorectal adenoma. Am Clin Nutr. (2013) 97:1044–52. doi: 10.3945/ajcn.112.046607
53. Vernia P, Gnaedinger A, Hauck W, Breuer RI. Organic anions and the diarrhea of inflammatory bowel disease. Digest Dis Sci. (1988) 33:1353–8. doi: 10.1007/BF01536987
54. Ou J, Carbonero F, Zoetendal EG, DeLany JP, Wang M, Newton K, et al. Diet, microbiota, and microbial metabolites in colon cancer risk in rural Africans and African Americans. Am J Clin Nutr. (2013) 98:111–20. doi: 10.3945/ajcn.112.056689
55. Joseph J, Loscalzo J. Nutri (meta) genetics and cardiovascular disease: novel concepts in the interaction of diet and genomic variation. Curr Atheroscler Rep. (2015) 17:505. doi: 10.1007/s11883-015-0505-x
56. Jonsson AL, Backhed F. Role of gut microbiota in atherosclerosis. Nat Rev Cardiol. (2017) 14:79–87. doi: 10.1038/nrcardio.2016.183
57. Koren O, Spor A, Felin J, Fak F, Stombaugh J, Tremaroli V, et al. Human oral, gut, and plaque microbiota in patients with atherosclerosis. Proc Natl Acad Sci USA. (2011) 108:4592–8. doi: 10.1073/pnas.1011383107
58. Calandrini CA, Ribeiro AC, Gonnelli AC, Ota-Tsuzuki C, Rangel LP, Saba-Chujfi E, et al. Microbial composition of atherosclerotic plaques. Oral Dis. (2014) 20:128–34. doi: 10.1111/odi.12205
59. Mitra S, Drautz-Moses DI, Alhede M, Maw MT, Liu Y, Purbojati RW, et al. In silico analyses of metagenomes from human atherosclerotic plaque samples. Microbiome. (2015) 3:38. doi: 10.1186/s40168-015-0100-y
60. Karlsson FH, Fak F, Nookaew I, Tremaroli V, Fagerberg B, Petranovic D, et al. Symptomatic atherosclerosis is associated with an altered gut metagenome. Nat Commun. (2012) 3:1245. doi: 10.1038/ncomms2266
61. Emoto T, Yamashita T, Sasaki N, Hirota Y, Hayashi T, So A, et al. Analysis of gut microbiota in coronary artery disease patients: a possible link between gut microbiota and coronary artery disease. J Atheroscler Thromb. (2016) 23:32672. doi: 10.5551/jat.32672
62. Wahlström A, Sayin SI, Marschall HU, Backhed F. Intestinal crosstalk between bile acids and microbiota and its impact on host metabolism. Cell Metab. (2016) 24:41–50. doi: 10.1016/j.cmet.2016.05.005
63. Lewarne T. Understanding the role of nutrition in preventing non-communicable diseases and supporting planetary health. Nurs Stand. (2022) 2:37. doi: 10.7748/ns.2022.e11814
64. Roglic G, Varghese C, Thamarangsi T. Diabetes in South-East Asia: burden, gaps, challenges and ways forward. WHO South East Asia J Public Health. (2016) 5:1–4. doi: 10.4103/2224-3151.206546
65. Mehta SR, Kashyap AS, Das S. Diabetes mellitus in India: the modern scourge. Med J Armed Forces India. (2009) 65:50–4. doi: 10.1016/S0377-1237(09)80056-7
66. Boutagy NE, McMillan RP, Frisard MI, Hulver MW. Metabolic endotoxemia with obesity: is it real and is it relevant? Biochimie. (2016) 124:11–20. doi: 10.1016/j.biochi.2015.06.020
67. Kim YA, Keogh JB, Clifton PM. Probiotics, prebiotics, synbiotics and insulin sensitivity. Nutr Res Rev. (2018) 31:35–51. doi: 10.1017/S095442241700018X
68. Suzuki T, Yoshida S, Hara H. Physiological concentrations of short-chain fatty acids immediately suppress colonic epithelial permeability. Br J Nutr. (2008) 100:297–305. doi: 10.1017/S0007114508888733
69. Vrieze A, Van Nood E, Holleman F, Salojarvi J, Kootte RS, Bartelsman JF, et al. Transfer of intestinal microbiota from lean donors increases insulin sensitivity in individuals with metabolic syndrome. Gastroenterology. (2012) 143:913–6. doi: 10.1053/j.gastro.2012.06.031
70. Pathak P, Xie C, Nichols RG, Ferrell JM, Boehme S, Krausz KW, et al. Intestine farnesoid X receptor agonist and the gut microbiota activate G-protein bile acid receptor-1 signaling to improve metabolism. Hepatology. (2018) 68:157488. doi: 10.1002/hep.29857
71. Giesbertz P, Daniel H. Branched-chain amino acids as biomarkers in diabetes. Curr Opin Clin Nutr Metab Care. (2016) 19:48–54. doi: 10.1097/MCO.0000000000000235
72. Wang TJ, Larson MG, Vasan RS, Cheng S, Rhee EP, McCabe E, et al. Metabolite profiles and the risk of developing diabetes. Nat Med. (2011) 17:448–53. doi: 10.1038/nm.2307
73. Pedersen HK, Gudmundsdottir V, Nielsen HB, Hyotylainen T, Nielsen T, Jensen BA, et al. Human gut microbes impact host serum metabolome and insulin sensitivity. Nature. (2016) 535:376–81. doi: 10.1038/nature18646
74. Kohli N, Ho S, Brown SJ, Sawadkar P, Sharma V, Snow M, et al. Bone remodelling in vitro: where are we headed?:-A review on the current understanding of physiological bone remodelling and inflammation and the strategies for testing biomaterials in vitro. Bone. (2018) 110:38–46. doi: 10.1016/j.bone.2018.01.015
75. Manolagas SC. From estrogen-centric to aging and oxidative stress: a revised perspective of the pathogenesis of osteoporosis. Endocr Rev. (2010) 31:266–300. doi: 10.1210/er.2009-0024
76. Han GG, Lee JY, Jin GD, Park J, Choi YH, Kang SK, et al. Tracing of the fecal microbiota of commercial pigs at five growth stages from birth to shipment. Sci Rep. (2018) 8:6012. doi: 10.1038/s41598-018-24508-7
77. Palmer MF, Rolls BA. The absorption and secretion of calcium in the gastrointestinal tract of germ-free and conventional chicks. Br J Nutr. (1981) 46:549–58. doi: 10.1079/BJN19810064
79. Luo Y, Chen GL, Hannemann N, Ipseiz N, Kronke G, Bauerle T, et al. Microbiota from obese mice regulate hematopoietic stem cell differentiation by altering the bone niche. Cell Metab. (2015) 22:886–94. doi: 10.1016/j.cmet.2015.08.020
80. Chen X, Oppenheim JJ. Th17 cells and tregs: unlikely allies. J Leukoc Biol. (2014) 95:723–31. doi: 10.1189/jlb.1213633
81. Carmina E, Lobo RA. Polycystic ovary syndrome (PCOS): arguably the most common endocrinopathy is associated with significant morbidity in women. J Clin Endocrinol Metab. (1999) 84:1897–9. doi: 10.1210/jcem.84.6.5803
82. Azziz R, Carmina E, Dewailly D, Diamanti-Kandarakis E, Escobar-Morreale HF, Futterweit W, et al. The androgen excess and PCOS society criteria for the polycystic ovary syndrome: the complete task force report. Fertil Steril. (2009) 91:456–88. doi: 10.1016/j.fertnstert.2008.06.035
83. Lobo RA. A unifying concept for polycystic ovary syndrome. In: RJ Chang, editor. Polycystic Ovary Syndrome. Serono Symposia USA. New York, NY: Springer (1996). doi: 10.1007/978-1-4613-8483-0_23
84. Torres PJ, Siakowska M, Banaszewska B, Pawelczyk L, Duleba AJ, Kelley ST, et al. Gut microbial diversity in women with polycystic ovary syndrome correlates with hyperandrogenism. J Clin Endocrinol Metab. (2018) 103:1502-11. doi: 10.1210/jc.2017-02153
85. Li T, Wu K, You L, Xing X, Wang P, Cui L, et al. Common variant rs9939609 in gene FTO confers risk to polycystic ovary syndrome. PLoS One. (2013) 8:66250. doi: 10.1371/journal.pone.0066250
86. Lindheim L, Bashir M, Munzker J, Trummer C, Zachhuber V, Leber B, et al. Alterations in gut microbiome composition and barrier function are associated with reproductive and metabolic defects in women with polycystic ovary syndrome (PCOS): a pilot study. PLoS One. (2017) 12:e0168390. doi: 10.1371/journal.pone.0168390
87. Karaki SI, Mitsui R, Hayashi H, Kato I, Sugiya H, Iwanaga T, et al. Short-chain fatty acid receptor, GPR43, is expressed by enteroendocrine cells and mucosal mast cells in rat intestine. Cell Tissue Res. (2006) 324:353–60. doi: 10.1007/s00441-005-0140-x
88. Karra E, Chandarana K, Batterham RL. The role of peptide YY in appetite regulation and obesity. Physiol Soc. (2009) 587:19–25. doi: 10.1113/jphysiol.2008.164269
89. Novelle MG, Vazquez MJ, Martinello KD, Sanchez-Garrido MA, Tena-Sempere M, Dieguez C. Neonatal events, such as androgenization and postnatal overfeeding, modify the response to ghrelin. Sci Rep. (2014) 4:855. doi: 10.1038/srep04855
90. Castera L, Friedrich-Rust M, Loomba R. Noninvasive assessment of liver disease in patients with nonalcoholic fatty liver disease. Gastroenterology. (2019) 156:1264–81. doi: 10.1053/j.gastro.2018.12.036
91. Chen D, Le TH, Shahidipour H, Read SA, Ahlenstiel G. The role of gut-derived microbial antigens on liver fibrosis initiation and progression. Cells. (2019) 8:1324. doi: 10.3390/cells8111324
92. World Health Organization [WHO].Obesity. (2017). Available online at: https://www.who.int/health-topics/obesity (accessed January 10, 2022).
94. Jensen MD, Ryan DH, Apovian CM, Ard JD, Comuzzie AG, Donato KA, et al. AHA/ACC/TOS guideline for the management of overweight and obesity in adults: a report of the American college of cardiology. Circulation. (2014) 129:S102–38. doi: 10.1161/01.cir.0000437739.71477.ee
95. Li Y, Elmen L, Segota I, Xian Y, Tinoco R, Feng Y, et al. Prebiotic-induced anti-tumor immunity attenuates tumor growth. Cell Rep. (2020) 30:1753–66. doi: 10.1016/j.celrep.2020.01.035
96. Fei N, Zhao L. An opportunistic pathogen isolated from the gut of an obese human causes obesity in germfree mice. ISME J. (2013) 7:880–4. doi: 10.1038/ismej.2012.153
97. Belkaid Y, Naik S. Compartmentalized and systemic control of tissue immunity by commensals. Nat Immunol. (2013) 14:646–53. doi: 10.1038/ni.2604
98. Bäckhed F, Manchester JK, Semenkovich CF, Gordon JI. Mechanisms underlying the resistance to diet-induced obesity in germ-free mice. Proc Natl Acad Sci USA. (2007) 104:979–84. doi: 10.1073/pnas.0605374104
99. Cani PD, Bibiloni R, Knauf C, Waget A, Neyrinck AM, Delzenne NM, et al. Changes in gut microbiota control metabolic endotoxemia-induced inflammation in high-fat diet- induced obesity and diabetes in mice. Diabetes. (2008) 57:1470–81. doi: 10.2337/db07-1403
100. De Filippis F, Pellegrini N, Vannini L, Jeffery IB, La Storia A, Laghi L, et al. High-level adherence to a Mediterranean diet beneficially impacts the gut microbiota and associated metabolome. Gut. (2016) 65:1812–21. doi: 10.1136/gutjnl-2015-309957
101. Westfall S, Lomis N, Kahouli I, Dia SY, Singh SP, Prakash S. Microbiome, probiotics and neurodegenerative diseases: deciphering the gut brain axis. Cell Mol Life Sci. (2017) 74:3769–87. doi: 10.1007/s00018-017-2550-9
102. Yu YN, Yu TC, Zhao HJ, Sun TT, Chen HM, Chen HY, et al. Berberine may rescue Fusobacterium nucleatum-induced colorectal tumorigenesis by modulating the tumor microenvironment. Oncotarget. (2015) 6:32013. doi: 10.18632/oncotarget.5166
103. Allen J, Hao S, Sears CL, Timp W. Epigenetic changes induced by Bacteroides fragilis toxin. Infect Immun. (2019) 87:e447–418. doi: 10.1128/IAI.00447-18
104. Wang X, Yang Y, Huycke MM. Commensal-infected macrophages induce dedifferentiation and reprogramming of epithelial cells during colorectal carcinogenesis. Oncotarget. (2017) 8:102176. doi: 10.18632/oncotarget.22250
105. Khan AA, Khan Z, Malik A, Kalam MA, Cash P, Ashraf MT, et al. Colorectal cancer-inflammatory bowel disease nexus and felony of Escherichia coli. Life Sci. (2017) 180:60–7. doi: 10.1016/j.lfs.2017.05.016
106. Blessing E, Campbell LA, Rosenfeld ME, Chough N, Kuo CC. Chlamydia pneumoniae infection accelerates hyperlipidemia induced atherosclerotic lesion development in C57BL/6J mice. Atherosclerosis. (2001) 158:13–7. doi: 10.1016/S0021-9150(00)00758-9
107. Clearfield M. C-reactive protein levels and outcomes after statin therapy. Curr Atheroscler Rep. (2006) 8:8–9. doi: 10.1007/s11883-006-0058-0
108. Akira S, Uematsu S, Takeuchi O. Pathogen recognition and innate immunity. Cell. (2006) 124:783–801. doi: 10.1016/j.cell.2006.02.015
109. Yang Y, Weng W, Peng J, Hong L, Yang L, Toiyama Y, et al. Fusobacterium nucleatum increases proliferation of colorectal cancer cells and tumor development in mice by activating toll-like receptor 4 signaling to nuclear factor- KB, and up- regulating expression of microRNA-21. Gastroenterology. (2017) 152:851–66. doi: 10.1053/j.gastro.2016.11.018
110. Liu M, Zeng X, Ma C, Yi H, Ali Z, Mou X, et al. Injectable hydrogels for cartilage and bone tissue engineering. Bone Res. (2017) 5:1–20. doi: 10.1038/boneres.2017.14
111. Yurtdaş G, Akdevelioglu Y. A new approach to polycystic ovary syndrome: the gut microbiota. J Am Coll Nutr. (2020) 39:371–82. doi: 10.1080/07315724.2019.1657515
112. Chassaing B, Ley RE, Gewirtz AT. Intestinal epithelial cell toll-like receptor 5 regulates the intestinal microbiota to prevent low-grade inflammation and metabolic syndrome in mice. Gastroenterology. (2014) 147:1363–77. doi: 10.1053/j.gastro.2014.08.033
113. Shindo K, Machida M, Fukumura M, Koide K, Yamazaki R. Omeprazole induces altered bile acid metabolism. Gut. (1998) 42:266–71. doi: 10.1136/gut.42.2.266
114. Zhao Y, Wu J, Li JV, Zhou NY, Tang H, Wang Y. Gut microbiota composition modifies fecal metabolic profiles in mice. J Prot Res. (2013) 12:2987–99. doi: 10.1021/pr400263n
115. Elshaghabee FM, Bockelmann W, Meske D, De Vrese M, Walte HG, Schrezenmeir J, et al. Ethanol production by selected intestinal microorganisms and lactic acid bacteria growing under different nutritional conditions. Front Microbiol. (2016) 7:47. doi: 10.3389/fmicb.2016.00047
116. Lindberg AA, Weintraub A, Zahringer U, Rietschel ET. Structure-activity relationships in lipopolysaccharides of Bacteroides fragilis. Rev Infect Dis. (1990) 12:S133–41. doi: 10.1093/clinids/12.Supplement_2.S133
117. Juarez-Fernández M, Porras D, Garda-Mediavilla MV, Roman-Saguillo S, Gonzalez-Gallego J, Nistal E, et al. Aging, gut microbiota and metabolic diseases: management through physical exercise and nutritional interventions. Nutrients. (2020) 13:16. doi: 10.3390/nu13010016
118. Rossi M, Keshavarzian A, Bishehsari F. Nutraceuticals in colorectal cancer: a mechanistic approach. Eur J Pharmacol. (2018) 833:396–402. doi: 10.1016/j.ejphar.2018.06.027
119. World Health Orgenization [WHO].Cancer. Geneva: WHO (2020). Available online at: https://www.who.int/news-room/fact-sheets/detail/cancer (accessed January 5, 2022).
120. Rad AH, Aghebati-Maleki L, Kafil HS, Abbasi A. Molecular mechanisms of postbiotics in colorectal cancer prevention and treatment. Crit Rev Food Sci Nutr. (2021) 61:1787–803. doi: 10.1080/10408398.2020.1765310
121. Eo HJ, Park GH, Jeong JB. Inhibition of Wnt signaling by silymarin in human colorectal cancer cells. Biomol Ther. (2016) 24:380. doi: 10.4062/biomolther.2015.154
122. Hosseinzade A, Sadeghi O, Naghdipour Biregani A, Soukhtehzari S, Brandt GS, Esmaillzadeh A. Immunomodulatory effects of flavonoids: possible induction of T CD4+ regulatory cells through suppression of mTOR pathway signaling activity. Front Immunol. (2019) 10:51. doi: 10.3389/fimmu.2019.00051
123. Huang RY, Yu YL, Cheng WC, OuYang CN, Fu E, Chu CL. Immunosuppressive effect of quercetin on dendritic cell activation and function. J Immunol. (2010) 184:6815–21. doi: 10.4049/jimmunol.0903991
124. Pricci M, Girardi B, Giorgio F, Losurdo G, Ierardi E, Di Leo A. Curcumin and colorectal cancer: from basic to clinical evidences. Int J Mol Sci. (2020) 21:2364. doi: 10.3390/ijms21072364
125. Singh N, Bhalla M, de Jager P, Gilca M. An overview on ashwagandha: a Rasayana (rejuvenator) of Ayurveda. Afr J Tradit Complement Altern Med. (2011) 8:5. doi: 10.4314/ajtcam.v8i5S.9
126. Dharmawansa KS, Hoskin DW, Rupasinghe HV. Chemopreventive effect of dietary anthocyanins against gastrointestinal cancers: a review of recent advances and perspectives. Int J Mol Sci. (2020) 21:6555. doi: 10.3390/ijms21186555
127. Jandhyala SM, Talukdar R, Subramanyam C, Vuyyuru H, Sasikala M, Reddy DN. Role of the normal gut microbiota. World J Gastroenterol. (2015) 21:8787. doi: 10.3748/wjg.v21.i29.8787
128. Raskov H, Burcharth J, Pommergaard HC. Linking gut microbiota to colorectal cancer. J Cancer. (2017) 8:3378. doi: 10.7150/jca.20497
129. Rossi M, Mirbagheri SEYEDS, Keshavarzian A, Bishehsari F. Nutraceuticals in colorectal cancer: a mechanistic approach. Eur J Pharmacol. (2018) 833: 396–402. doi: 10.1016/j.ejphar.2018.06.027
130. Ma J, Li H. The role of gut microbiota in atherosclerosis and hypertension. Front Pharmacol. (2018) 9:1082. doi: 10.3389/fphar.2018.01082
131. Moss JW, Ramji DP. Nutraceutical therapies for atherosclerosis. Nat Rev Cardiol. (2016) 13:513–32. doi: 10.1038/nrcardio.2016.103
132. Sosnowska B, Penson P, Banach M. The role of nutraceuticals in the prevention of cardiovascular disease. Cardiovasc Diagn Ther. (2017) 7:S21. doi: 10.21037/cdt.2017.03.20
133. Peng Y, Ao M, Dong B, Jiang Y, Yu L, Chen Z, et al. Anti-inflammatory effects of curcumin in the inflammatory diseases: status, limitations and countermeasures. Drug Des Dev Ther. (2021) 15:4503. doi: 10.2147/DDDT.S327378
134. Abe Y, Hashimoto SH, Horie T. Curcumin inhibition of inflammatory cytokine production by human peripheral blood monocytes and alveolar macrophages. Pharmacol Res. (1999) 39:41–7. doi: 10.1006/phrs.1998.0404
135. Halder S, Bharal N, Mediratta PK, Kaur I, Sharma KK. Anti-inflammatory, immunomodulatory and antinociceptive activity of Terminalia arjuna Roxb bark powder in mice and rats. Ind J Exp Biol. (2009) 47:577–83.
136. Shivaprasad HN, Kharya MD, Rana AC, Mohan S. Preliminary immunomodulatory activities of the aqueous extract of terminalia chebula. Pharm Biol. (2006) 44:32–4. doi: 10.1080/13880200500530542
137. Lee DY, Li H, Lim HJ, Lee HJ, Jeon R, Ryu JH. Anti-inflammatory activity of sulfur- containing compounds from garlic. J Med Food. (2012) 15:992–9. doi: 10.1089/jmf.2012.2275
138. Shang A, Cao SY, Xu XY, Gan RY, Tang GY, Corke H, et al. Bioactive compounds and biological functions of garlic (Allium sativum L.). Foods. (2019) 8:246. doi: 10.3390/foods8070246
139. Santangelo C, Vari R, Scazzocchio B, De Sanctis P, Giovannini C, D’Archivio M, et al. Anti-inflammatory activity of extra virgin olive oil polyphenols: which role in the prevention and treatment of immune-mediated inflammatory diseases? Endocr Metab Immune Disord Drug Targets. (2018) 18:36–50. doi: 10.2174/1871530317666171114114321
140. Vilaplana-Perez C, Aunon D, Garda-Flores LA, Gil-Izquierdo A. Hydroxytyrosol and potential uses in cardiovascular diseases, cancer, and AIDS. Front Nutr. (2014) 1:18. doi: 10.3389/fnut.2014.00018
141. Wan Q, Liu Z, Yang Y, Cui X. Suppressive effects of berberine on atherosclerosis via downregulating visfatin expression and attenuating visfatin-induced endothelial dysfunction. Int J Mol Med. (2018) 41:1939–48. doi: 10.3892/ijmm.2018.3440
142. Wu H, Chiou J. Potential benefits of probiotics and prebiotics for coronary heart disease and stroke. Nutrition. (2021) 13:2878. doi: 10.3390/nu13082878
143. Ding YH, Qian LY, Pang J, Lin JY, Xu Q, Wang LH, et al. The regulation of immune cells by Lactobacilli: a potential therapeutic target for anti-atherosclerosis therapy. Oncotarget. (2017) 8:59915. doi: 10.18632/oncotarget.18346
144. Ruiz-León AM, Lapuente M, Estruch R, Casas R. Clinical advances in immunonutrition and atherosclerosis: a review. Front Immunol. (2019) 10:837. doi: 10.3389/fimmu.2019.00837
145. Almerighi C, Sinistro A, Cavazza A, Ciaprini C, Rocchi G, Bergamini A. 1a, 25- dihydroxyvitamin D3 inhibits CD40L-induced pro-inflammatory and immunomodulatory activity in human monocytes. Cytokine. (2009) 45:190–7. doi: 10.1016/j.cyto.2008.12.009
146. Ruff WE, Vieira SM, Kriegel MA. The role of the gut microbiota in the pathogenesis of antiphospholipid syndrome. Curr Rheumatol Rep. (2015) 17:472. doi: 10.1007/s11926-014-0472-1
147. Nunez-Sanchez MA, Karmokar A, Gonzalez-Sarrias A. In vivo relevant mixed urolithins and ellagic acid inhibit phenotypic and molecular colon cancer stem cell features: a new potentiality for ellagitannin metabolites against cancer. Food Chem Toxicol. (2016) 92:8–16. doi: 10.1016/j.fct.2016.03.011
148. Nguyen D, Zachariasova A, Spurna K, Hricko J, Phung H, Viktorova J, et al. Antidiabetic compounds in stem juice from banana. Czech J Food Sci. (2017) 35:407–13. doi: 10.17221/172/2017-CJFS
149. Anarthe SJ, Sunitha D, Raju MG. Immunomodulatory activity for methanolic extract of Trigonella foenum graecum whole plant in wistar albino rats. Am J Phytomed Clin Ther. (2014) 2:1081–92.
150. Bin-Hafeez B, Haque R, Parvez S, Pandey S, Sayeed I, Raisuddin S. Immunomodulatory effects of fenugreek (Trigonella foenum graecum L.) extract in mice. Int Immunopharmacol. (2003) 3:257–65. doi: 10.1016/S1567-5769(02)00292-8
151. Makare N, Bodhankar S, Rangari V. Immunomodulatory activity of alcoholic extract of Mangifera indica L. in mice. J Ethnopharmacol. (2001) 78:133–7. doi: 10.1016/S0378-8741(01)00326-9
152. Joseph B, Jini D. Antidiabetic effects of Momordica charantia (bitter melon) and its medicinal potency. Asian Pacif J Tropic Dis. (2013) 3:93–102. doi: 10.1016/S2222-1808(13)60052-3
153. Salehi B, Ata A, V Anil Kumar N, Sharopov F, Ramirez-Alarcon K, Ruiz-Ortega A, et al. Antidiabetic potential of medicinal plants and their active components. Biomolecules. (2019) 9:551. doi: 10.3390/biom9100551
154. Nani D, Proverawati A. Immunomodulatory effects of black solo garlic (Allium sativum L.) on streptozotocin-induced diabetes in Wistar rats. Heliyon. (2021) 7:08493. doi: 10.1016/j.heliyon.2021.e08493
155. Ayyanar M, Ignacimuthu S. Ethnobotanical survey of medicinal plants commonly used by Kani tribals in Tirunelveli hills of Western Ghats, India. J Ethnopharmacol. (2011) 134:851–64. doi: 10.1016/j.jep.2011.01.029
156. Zambrana S, Lundqvist LC, Veliz V, Catrina SB, Gonzales E, Ostenson CG. Amaranthus caudatus stimulates insulin secretion in goto-kakizaki rats, a model of diabetes mellitus type 2. Nutrients. (2018) 10:94. doi: 10.3390/nu10010094
157. Salehi B, Berkay Yilmaz Y, Antika G, Boyunegmez Tumer T, Fawzi Mahomoodally M, Lobine D, et al. Insights on the use of a- lipoic acid for therapeutic purposes. Biomolecules. (2019) 9:356. doi: 10.3390/biom9080356
158. Derosa G, Limas CP, Madas PC, Estrella A, Maffioli P. State of the art papers dietary and nutraceutical approach to type 2 diabetes. Arch Med Sci. (2014) 10:336–44. doi: 10.5114/aoms.2014.42587
159. Mallappa RH, Balasubramaniam C, Amarlapudi MR, Kelkar S, Adewumi GA, Kadyan S, et al. Role of probiotics in the prevention and management of diabetes and obesity. Probiotics in the Prevention and Management of Human Diseases. Cambridge, MA: Academic Press (2022). p. 321–36. doi: 10.1016/B978-0-12-823733-5.00006-4
160. Yan J, Herzog JW, Tsang K, Brennan CA, Bower MA, Garrett WS, et al. Gut microbiota induce IGF-1 and promote bone formation and growth. Proc Natl Acad Sci USA. (2016) 113:7554–63. doi: 10.1073/pnas.1607235113
161. Seely KD, Kotelko CA, Douglas H, Bealer B, Brooks AE. The human gut microbiota: a key mediator of osteoporosis and osteogenesis. Int J Mol Sci. (2021) 22:9452. doi: 10.3390/ijms22179452
162. Zhang YW, Li YJ, Lu PP, Dai GC, Chen XX, Rui YF. The modulatory effect and implication of gut microbiota on osteoporosis: from the perspective of “brain-gut-bone” axis. Food Funct. (2021) 12:5703–18. doi: 10.1039/D0FO03468A
163. Tharakan A, Shukla H, Benny IR, Tharakan M, George L, Koshy S. Immunomodulatory effect of Withania somnifera (Ashwagandha) extract—a randomized, double-blind, placebo controlled trial with an open label extension on healthy participants. J Clin Med. (2021) 10:3644. doi: 10.3390/jcm10163644
164. Azam Z, Pandey V, Gupta N, Sapra L, Dar HY, Shokeen N, et al. Phytoconstituents as novel osteo-protective agents: implications in bone health. Front Biosci. (2020) 25:1259–96. doi: 10.2741/4855
165. McCabe LR, Parameswaran N editors. Understanding the Gut-Bone Signaling Axis: Mechanisms and Therapeutic Implications. New York, NY: Springer (2017). doi: 10.1007/978-3-319-66653-2
166. Ibanez L, Rouleau M, Wakkach A, Blin-Wakkach C. Gut microbiome and bone. Joint Bone Spine. (2019) 86:43–7. doi: 10.1016/j.jbspin.2018.02.008
167. Sassi F, Tamone C, D’Amelio P. Vitamin D: nutrient, hormone, and immunomodulator. Nutrients. (2018) 10:1656. doi: 10.3390/nu10111656
168. Holick MF. Vitamin D: important for prevention of osteoporosis, cardiovascular heart disease, type 1 diabetes, autoimmune diseases, and some cancers. Southern Med J. (2005) 98:1024–8. doi: 10.1097/01.SMJ.0000140865.32054.DB
169. He FF, Li YM. Role of gut microbiota in the development of insulin resistance and the mechanism underlying polycystic ovary syndrome: a review. J Ovarian Res. (2020) 13:73. doi: 10.1186/s13048-020-00670-3
170. Zhou L, Ni Z, Cheng W, Yu J, Sun S, Zhai D, et al. Characteristic gut microbiota and predicted metabolic functions in women with PCOS. Endocr Connect. (2020) 9:63–73. doi: 10.1530/EC-19-0522
171. Pachiappan S, Ramalingam K, Balasubramanian A. A review on phytomedicine and their mechanism of action on PCOS. Int. J. Cur. Res. Rev. (2020) 12:81. doi: 10.31782/IJCRR.2020.122322
172. Nasimi Doost Azgomi R, Zomorrodi A, Nazemyieh H, Fazljou SM, Sadeghi Bazargani H, Nejatbakhsh F, et al. Effects of Withania somnifera on reproductive system: a systematic review of the available evidence. Biomed Res Int. (2018) 24:2018. doi: 10.1155/2018/4076430
173. Ota K, Dambaeva S, Han AR, Beaman K, Gilman-Sachs A, Kwak-Kim J. Vitamin D deficiency may be a risk factor for recurrent pregnancy losses by increasing cellular immunity and autoimmunity. Hum Reprod. (2014) 29:208–19. doi: 10.1093/humrep/det424
174. Zarnani AH. Vitamin D and human reproduction: past, present and future. Fertil Steril. (2010) 93:2738–43.
175. Younossi Z, Anstee QM, Marietti M, Hardy T, Henry L, Eslam M, et al. Global burden of NAFLD and NASH: trends, predictions, risk factors and prevention. Nat Rev Gastroenterol Hepatol. (2018) 15:11–20. doi: 10.1038/nrgastro.2017.109
176. Kneeman JM, Misdraji J, Corey KE. Secondary causes of nonalcoholic fatty liver disease. Ther Adv Gastroenterol. (2012) 5:199–207. doi: 10.1177/1756283X11430859
177. Behary J, Amorim N, Jiang XT, Raposo A, Gong L, McGovern E, et al. Gut microbiota impact on the peripheral immune response in non-alcoholic fatty liver disease related hepatocellular carcinoma. Nat Commun. (2021) 12:187. doi: 10.1038/s41467-020-20422-7
178. Curcio A, Romano A, Cuozzo S, Di Nicola A, Grassi O, Schiaroli D, et al. Silymarin in combination with vitamin C, vitamin E, coenzyme Q10 and selenomethionine to improve liver enzymes and blood lipid profile in NAFLD patients. Medicina. (2020) 56:544. doi: 10.3390/medicina56100544
179. Krishnan S, Ding Y, Saedi N, Choi M, Sridharan GV, Sherr DH, et al. Gut microbiota-derived tryptophan metabolites modulate inflammatory response in hepatocytes and macrophages. Cell Rep. (2018) 23:1099–111. doi: 10.1016/j.celrep.2018.03.109
180. Yang M, Khoukaz L, Qi X, Kimchi ET, Staveley-O’Carroll KF, Li G. Diet and gut microbiota interaction-derived metabolites and intrahepatic immune response in NAFLD development and treatment. Biomedicines. (2021) 9:1893. doi: 10.3390/biomedicines9121893
181. Sharpton SR, Maraj B, Harding-Theobald E, Vittinghoff E, Terrault NA. Gut microbiome- targeted therapies in nonalcoholic fatty liver disease: a systematic review, meta-analysis, and meta-regression. Am J Clin Nutr. (2019) 110:139–49. doi: 10.1093/ajcn/nqz042
182. Famouri F, Shariat Z, Hashemipour M, Keikha M, Kelishadi R. Effects of probiotics on nonalcoholic fatty liver disease in obese children and adolescents. J Pediatr Gastroenterol Nutr. (2017) 64:413–7. doi: 10.1097/MPG.0000000000001422
183. Gomes AC, Hoffmann C, Mota JF. The human gut microbiota: metabolism and perspective in obesity. Gut Microbes. (2018) 9:308–25. doi: 10.1080/19490976.2018.1465157
184. Kobyliak N, Conte C, Cammarota G, Haley AP, Styriak I, Gaspar L, et al. Probiotics in prevention and treatment of obesity: a critical view. Nutr Metab. (2016) 13:14. doi: 10.1186/s12986-016-0067-0
185. Sivamaruthi BS, Kesika P, Suganthy N, Chaiyasut C. A review on role of microbiome in obesity and antiobesity properties of probiotic supplements. Biomed Res Int. (2019) 2019:3291367. doi: 10.1155/2019/3291367
186. Cerdó T, Garda-Santos JA, G Bermudez M, Campoy C. The role of probiotics and prebiotics in the prevention and treatment of obesity. Nutrients. (2019) 11:635. doi: 10.3390/nu11030635
187. Cani PD, Possemiers S, Van de Wiele T, Guiot Y, Everard A, Rottier O, et al. Changes in gut microbiota control inflammation in obese mice through a mechanism involving GLP-2-driven improvement of gut permeability. Gut. (2009) 58:1091–103. doi: 10.1136/gut.2008.165886
188. Guo S, Gillingham T, Guo Y, Meng D, Zhu W, Walker WA, et al. Secretions of Bifidobacterium infantis and Lactobacillus acidophilus protect intestinal epithelial barrier function. J Pediatr Gastroenterol Nutr. (2017) 64:404–12. doi: 10.1097/MPG.0000000000001310
189. Esmaily H, Sahebkar A, Iranshahi M, Ganjali S, Mohammadi A, Ferns G, et al. An investigation of the effects of curcumin on anxiety and depression in obese individuals: a randomized controlled trial. Chin J Integr Med. (2015) 21:332–8. doi: 10.1007/s11655-015-2160-z
190. Wang S, Li Z, Ma Y, Liu Y, Lin CC, Li S, et al. Immunomodulatory effects of green tea polyphenols. Molecules. (2021) 26:3755. doi: 10.3390/molecules26123755
191. Gadisa E, Weldearegay G, Desta K, Tsegaye G, Hailu S, Jote K, et al. Combined antibacterial effect of essential oils from three most commonly used Ethiopian traditional medicinal plants on multidrug resistant bacteria. BMC Complement Altern Med. (2019) 19:24. doi: 10.1186/s12906-019-2429-4
192. Bielak-Zmijewska A, Grabowska W, Ciolko A, Bojko A, Mosieniak G, Bijoch L, et al. The role of curcumin in the modulation of ageing. Int J Mol Sci. (2019) 20:1239. doi: 10.3390/ijms20051239
193. Olszewska R, Jawien J, Gajda M, Mateuszuk L, Gebska A, Korabiowska M, et al. Effect of curcumin on atherosclerosis in apoE/LDLR-double knockout mice. J Physiol Pharmacol. (2005) 56:627–35.
194. Bhavsar SK, Thaker AM, Malik JK. Shilajit. In: RC Gupta, editor. Nutraceuticals: Efficacy, Safety and Toxicity. Cambridge, MA: Academic Press (2016). p. 707–16. doi: 10.1016/B978-0-12-802147-7.00051-6
195. Kadam A, Kadam D, Tungare K, Shah H. Probiotics and prebiotics in healthy ageing. Nutrition, Food and Diet in Ageing and Longevity. Cham: Springer (2021). doi: 10.1007/978-3-030-83017-5_5
196. Patel PJ, Singh SK, Panaich S, Cardozo L. The aging gut and the role of prebiotics, probiotics, and synbiotics: a review. J Clin Gerontol Geriatr. (2014) 5:3–6. doi: 10.1016/j.jcgg.2013.08.003
197. Fang X, Yue M, Wei J, Wang Y, Hong D, Wang B, et al. Evaluation of the anti-aging effects of a probiotic combination isolated from centenarians in a SAMP8 mouse model. Front Immunol. (2021) 2:5163. doi: 10.3389/fimmu.2021.792746
198. Shan BE, Wang MX, Li RQ. Quercetin inhibit human SW480 colon cancer growth in association with inhibition of cyclin D1 and survivin expression through Wnt/p-catenin signaling pathway. Cancer Investig. (2009) 27:604–12. doi: 10.1080/07357900802337191
199. Mosieniak G, Adamowicz M, Alster O, Jaskowiak H, Szczepankiewicz AA, Wilczynski GM, et al. Curcumin induces permanent growth arrest of human colon cancer cells: link between senescence and autophagy. Mech Ageing Dev. (2012) 133:444–55. doi: 10.1016/j.mad.2012.05.004
200. Muralikrishnan G, Dinda AK, Shakeel F. Immunomodulatory effects of Withania somnifera on azoxymethane induced experimental colon cancer in mice. Immunol Investig. (2010) 39:688–98. doi: 10.3109/08820139.2010.487083
201. Rahayu RP, Prasetyo RA, Purwanto DA, Kresnoadi U, Iskandar RP, Rubianto M. The immunomodulatory effect of green tea (Camellia sinensis) leaves extract on immunocompromised Wistar rats infected by Candida albicans. Vet World. (2018) 11:765. doi: 10.14202/vetworld.2018.765-770
202. Wang CJ, Wang C, Han J, Wang YK, Tang L, Shen DW, et al. Effect of combined treatment with recombinant interleukin-2 and allicin on pancreatic cancer. Mol Biol Rep. (2013) 40:6579–85. doi: 10.1007/s11033-013-2766-1
203. Sarvizadeh M, Hasanpour O, Naderi Ghale-Noie Z, Mollazadeh S, Rezaei M, Pourghadamyari H, et al. Allicin and digestive system cancers: from chemical structure to its therapeutic opportunities. Front Oncol. (2021) 11:650256. doi: 10.3389/fonc.2021.650256
204. Bessler H, Djaldetti M. On the link between ellagic acid and the immune balance between human mononuclear and colon carcinoma cells. Immunol Curr Res. (2017) 1:101.
205. Ghiţu A, Schwiebs A, Radeke HH, Avram S, Zupko I, Bor A, et al. A comprehensive assessment of apigenin as an antiproliferative, proapoptotic, antiangiogenic and immunomodulatory phytocompound. Nutrients. (2019) 114:858. doi: 10.3390/nu11040858
206. Ferrari D, Speciale A, Cristani M, Fratantonio D, Molonia MS, Ranaldi G, et al. Cyanidin-3-O-glucoside inhibits NF-kB signalling in intestinal epithelial cells exposed to TNF-a and exerts protective effects via Nrf2 pathway activation. Toxicol Lett. (2016) 264:51–8. doi: 10.1016/j.toxlet.2016.10.014
207. Owens JA, Saeedi BJ, Naudin CR, Hunter-Chang S, Barbian ME, Eboka RU, et al. Lactobacillus rhamnosus GG orchestrates an antitumor immune response. Cell Mol Gastroenterol Hepatol. (2021) 12:1311–27. doi: 10.1016/j.jcmgh.2021.06.001
208. Lee HA, Kim H, Lee KW, Park KY. Dead nano-sized Lactobacillus plantarum inhibits azoxymethane/dextran sulfate sodium-induced colon cancer in Balb/c mice. J Med Food. (2015) 18:1400–5. doi: 10.1089/jmf.2015.3577
209. Yue Y, Ye K, Lu J, Wang X, Zhang S, Liu L, et al. Probiotic strain Lactobacillus plantarum YYC-3 prevents colon cancer in mice by regulating the tumour microenvironment. Biomed Pharmacother. (2020) 127:110159. doi: 10.1016/j.biopha.2020.110159
210. Roller M, Clune Y, Collins K, Rechkemmer G, Watzl B. Consumption of prebiotic inulin enriched with oligofructose in combination with the probiotics Lactobacillus rhamnosus and Bifidobacterium lactis has minor effects on selected immune parameters in polypectomised and colon cancer patients. Br J Nutr. (2007) 97:676–84. doi: 10.1017/S0007114507450292
211. Dimitrovski D, Cencic A, Winkelhausen E, Langerholc T. Lactobacillus plantarum extracellular metabolites: in vitro assessment of probiotic effects on normal and cancerogenic human cells. Int Dairy J. (2014) 39:293–300. doi: 10.1016/j.idairyj.2014.07.009
212. Gao S, Zhou J, Liu N, Wang L, Gao Q, Wu Y, et al. Curcumin induces M2 macrophage polarization by secretion IL-4 and/or IL-13. J Mol Cell Cardiol. (2015) 85:131–9. doi: 10.1016/j.yjmcc.2015.04.025
213. Killeen MJ, Linder M, Pontoniere P, Crea R. NF-KP signaling and chronic inflammatory diseases: exploring the potential of natural products to drive new therapeutic opportunities. Drug Discov Today. (2014) 19:373–8. doi: 10.1016/j.drudis.2013.11.002
214. Dell’Agli M, Fagnani R, Mitro N, Scurati S, Masciadri M, Mussoni L, et al. Minor components of olive oil modulate proatherogenic adhesion molecules involved in endothelial activation. J Agricult Food Chem. (2006) 54:3259–64. doi: 10.1021/jf0529161
215. Li J, Lin S, Vanhoutte PM, Woo CW, Xu A. Akkermansia muciniphila protects against atherosclerosis by preventing metabolic endotoxemia-induced inflammation in Apoe-/- mice. Circulation. (2016) 133:2434–46. doi: 10.1161/CIRCULATIONAHA.115.019645
216. Li L, Wang M, Chen J, Xu Z, Wang S, Xia X, et al. Preventive effects of Bacillus licheniformis on heat stroke in rats by sustaining intestinal barrier function and modulating gut microbiota. Front Microbiol. (2021) 12:630841. doi: 10.3389/fmicb.2021.630841
217. Hughes DA, Southon S, Pinder AC. (n-3) Polyunsaturated fatty acids modulate the expression of functionally associated molecules on human monocytes in vitro. J Nutr. (1996) 126:603–10. doi: 10.1093/jn/126.3.603
218. Hamden K, Masmoudi H, Carreau S, Elfeki A. Immunomodulatory, P-cell, and neuroprotective actions of fenugreek oil from alloxan-induced diabetes. Immunopharmacol Immunotoxicol. (2010) 32:437–45. doi: 10.3109/08923970903490486
219. Ahmed I, Adeghate E, Cummings E, Sharma AK, Singh J. Beneficial effects and mechanism of action of Momordica charantia juice in the treatment of streptozotocin-induced diabetes mellitus in rat. Mol Cell Biochem. (2004) 261:63–70. doi: 10.1023/B:MCBI.0000028738.95518.90
220. Lee CK, Lee EY, Kim YG, Mun SH, Moon HB, Yoo B. Alpha-lipoic acid inhibits TNF-a induced NF-KB activation through blocking of MEKK1-MKK4-IKK signaling cascades. Int Immunopharmacol. (2008) 8:362–70. doi: 10.1016/j.intimp.2007.10.020
221. Go HK, Rahman MM, Kim GB, Na CS, Song CH, Kim JS, et al. Antidiabetic effects of yam (Dioscorea batatas) and its active constituent, allantoin, in a rat model of streptozotocin-induced diabetes. Nutrients. (2015) 7:8532–44. doi: 10.3390/nu7105411
222. Zinjarde SS, Bhargava SY, Kumar AR. Potent a-amylase inhibitory activity of Indian Ayurvedic medicinal plants. BMC Complement Altern Med. (2011) 11:5. doi: 10.1186/1472-6882-11-5
223. Lee YS, Lee D, Park GS, Ko SH, Park J, Lee YK, et al. Lactobacillus plantarum HAC01 ameliorates type 2 diabetes in high-fat diet and streptozotocin-induced diabetic mice in association with modulating the gut microbiota. Food Funct. (2021) 12:6363–73. doi: 10.1039/D1FO00698C
224. Vatanen T, Franzosa EA, Schwager R, Tripathi S, Arthur TD, Vehik K, et al. The human gut microbiome in early-onset type 1 diabetes from the TEDDY study. Nature. (2018) 562:589–94. doi: 10.1038/s41586-018-0620-2
225. Agarwal R, Diwanay S, Patki P, Patwardhan B. Studies on immunomodulatory activity of Withania somnifera (Ashwagandha) extracts in experimental immune inflammation. J Ethnopharmacol. (1999) 67:27–35. doi: 10.1016/S0378-8741(99)00065-3
226. Khan MK, Ansari IA, Khan MS, Arif JM. Dietary phytochemicals as potent chemotherapeutic agents against breast cancer: inhibition of NF-KB pathway via molecular interactions in rel homology domain of its precursor protein p105. Pharmacogn Mag. (2013) 9:51. doi: 10.4103/0973-1296.108140
227. Abiodun OO, Rodriguez-Nogales A, Algieri F, Gomez-Caravaca AM, Segura-Carretero A, Utrilla MP, et al. Antiinflammatory and immunomodulatory activity of an ethanolic extract from the stem bark of Terminalia catappa L. (Combretaceae): in vitro and in vivo evidence. J Ethnopharmacol. (2016) 192:309–19. doi: 10.1016/j.jep.2016.07.056
228. Luo KW, Yue GG, Ko CH, Lee JK, Gao S, Li LF, et al. In vivo and in vitro anti-tumor and anti-metastasis effects of Coriolus versicolor aqueous extract on mouse mammary 4T1 carcinoma. Phytomedicine. (2014) 21:1078–87. doi: 10.1016/j.phymed.2014.04.020
229. Ye L, Zheng X, Zhang J, Yang Y, Meng Y, Li J, et al. Composition analysis and immunomodulatory capacity of peptidoglycan from ling zhi or reishi medicinal mushroom, ganoderma lucidum (W. Curt.: Fr.) P. Karst. Strain 119 (Aphyllophoromycetideae). Int J Med Mushrooms. (2010) 12:2. doi: 10.1615/IntJMedMushr.v12.i2.60
230. Ivanov II, de Llanos Frutos R, Manel N, Yoshinaga K, Rifkin DB, Sartor RB, et al. Specific microbiota direct the differentiation of IL-17-producing T-helper cells in the mucosa of the small intestine. Cell Host Microbe. (2008) 4:337–49. doi: 10.1016/j.chom.2008.09.009
231. Tremaroli V, Backhed F. Functional interactions between the gut microbiota and host metabolism. Nature. (2012) 489:242–9. doi: 10.1038/nature11552
232. Saiyed A, Jahan N, Makbul SA, Ansari M, Bano H, Habib SH. Effect of combination of Withania somnifera Dunal and Tribulus terrestris Linn on letrozole induced polycystic ovarian syndrome in rats. Integr Med Res. (2016) 5:293–300. doi: 10.1016/j.imr.2016.10.002
233. Ram MS, Neetu D, Yogesh B, Anju B, Dipti P, Pauline T, et al. Cyto-protective and immunomodulating properties of Amla (Emblica officinalis) on lymphocytes: an in-vitro study. J Ethnopharmacol. (2002) 81:5–10. doi: 10.1016/S0378-8741(01)00421-4
234. Rani R, Hajam YA, Kumar R, Bhat RA, Rai S, Rather MA. A landscape analysis of the potential role of polyphenols for the treatment of polycystic ovarian syndrome (PCOS). Phytomed Plus. (2022) 2:100161. doi: 10.1016/j.phyplu.2021.100161
235. Niphade SR. Immunomodulatory Activity of Cinnamon Bark. Ph D. Thesis. Karnataka: Rajiv Gandhi University of Health Sciences (2006).
236. Ou Q, Weng Y, Wang S, Zhao Y, Zhang F, Zhou J, et al. Silybin alleviates hepatic steatosis and fibrosis in NASH mice by inhibiting oxidative stress and involvement with the Nf-KB pathway. Digest Dis Sci. (2018) 63:3398–408. doi: 10.1007/s10620-018-5268-0
237. Kim GW, Jo HK, Chung SH. Ginseng seed oil ameliorates hepatic lipid accumulation in vitro and in vivo. J Ginseng Res. (2018) 42:419–28. doi: 10.1016/j.jgr.2017.04.010
238. Hosseinpour-Arjmand S, Amirkhizi F, Ebrahimi-Mameghani M. The effect of alpha-lipoic acid on inflammatory markers and body composition in obese patients with non-alcoholic fatty liver disease: a randomized, double-blind, placebo-controlled trial. J Clin Pharm Ther. (2019) 44:258–67. doi: 10.1111/jcpt.12784
239. Qin G, Ma J, Huang Q, Yin H, Han J, Li M, et al. Isoquercetin improves hepatic lipid accumulation by activating AMPK pathway and suppressing TGF-P signaling on an HFD-induced nonalcoholic fatty liver disease rat model. Int J Mol Sci. (2018) 19:4126. doi: 10.3390/ijms19124126
240. Chalasani N, Younossi Z, Lavine JE, Diehl AM, Brunt EM, Cusi K, et al. The diagnosis and management of non-alcoholic fatty liver disease: practice guideline by the American Association for the Study of Liver Diseases, American College of Gastroenterology, and the American Gastroenterological Association. Hepatology. (2012) 55:2005–23. doi: 10.1002/hep.25762
241. Beilfuss A, Sowa JP, Sydor S, Beste M, Bechmann LP, Schlattjan M, et al. Vitamin D counteracts fibrogenic TGF-P signalling in human hepatic stellate cells both receptor-dependently and independently. Gut. (2015) 64:791–9. doi: 10.1136/gutjnl-2014-307024
242. Braun DP, Johnson DM, Katsantonis NG, Bhesaniya K, Staren ED. Apoptotic and immunomodulatory effects of green tea extracts (GTE) on chemoresistant human tumor cells. J Clin Oncol. (2009) 27:22101. doi: 10.1200/jco.2009.27.15_suppl.e22101
243. Ahmad G, Masoodi MH, Tabassum N, Mir SA, Iqbal MJ. In vivo hepatoprotective potential of extracts obtained from floral spikes of Prunella vulgaris L. J Ayurveda Integr Med. (2020) 11:502–7. doi: 10.1016/j.jaim.2019.08.003
244. Gao F, Du W, Zafar MI, Shafqat RA, Jian L, Cai Q, et al. 4-Hydroxyisoleucine ameliorates an insulin resistant-like state in 3T3-L1 adipocytes by regulating TACE/TIMP3 expression. Drug Des Dev Ther. (2015) 9:5727. doi: 10.2147/DDDT.S92355
245. Park SY, Seetharaman R, Ko MJ, Kim TH, Yoon MK, Kwak JH, et al. Ethyl linoleate from garlic attenuates lipopolysaccharide-induced pro-inflammatory cytokine production by inducing heme oxygenase-1 in RAW264.7 cells. Int Immunopharmacol. (2014) 19:253–61. doi: 10.1016/j.intimp.2014.01.017
246. Karoly P, Ruehlman LS. Psychological “resilience” and its correlates in chronic pain: findings from a national community sample. Pain. (2006) 123:90–7. doi: 10.1016/j.pain.2006.02.014
247. Gopas J, Stern E, Zurgil U, Ozer J, Ben-Ari A, Shubinsky G, et al. Reed-Sternberg cells in Hodgkin’s lymphoma present features of cellular senescence. Cell Death Dis. (2016) 7:2457. doi: 10.1038/cddis.2016.185
248. Kumar S, Seal CJ, Howes MJ, Kite GC, Okello EJ. In vitro protective effects of Withania somnifera (L.) dunal root extract against hydrogen peroxide and P-amyloid (1-42) -induced cytotoxicity in differentiated PC12 cells. Phytother Res. (2010) 24:1567–74. doi: 10.1002/ptr.3261
249. Kumano K, Kanak MA, Saravanan PB, Blanck JP, Liu Y, Vasu S, et al. Withaferin A inhibits lymphocyte proliferation, dendritic cell maturation in vitro and prolongs islet allograft survival. Sci Rep. (2021) 11:10661. doi: 10.1038/s41598-021-90181-y
250. Yaqoob P. Ageing, immunity and influenza: a role for probiotics? Proc Nutr Soc. (2014) 73:309–17. doi: 10.1017/S0029665113003777
251. US-FDA.Fecal Microbiota for Transplantation: Safety Communication- Risk of Serious Adverse Reactions Due to Transmission of Multi-Drug Resistant Organisms. Silver Spring, MD: US-FDA (2019). Available online at: https://www.fda.gov/safety/medical-product-safety-information/fecal-micro biota-transplantation-safety-communication-risk-serious-adverse-reactions-due (accessed March 23, 2022).
252. US-FDA.Important Safety Alert Regarding use of Fecal Microbiota for Transplantation and Risk of Serious Adverse Reactions Due to Transmission of Multi-Drug Resistant Organisms. Silver Spring, MD: US-FDA (2019). Available online at: https://www.fda.gov/vaccines-blood-biologics/safety-availability-biologics/important-safety-alert-regarding-use-fecal- microbiota-transplantation-and-risk-serious-adverse (accessed March 23, 2022).
253. US-FDA.Update to March 12, 2020, Safety Alert Regarding Use of Fecal Microbiota for Transplantation and Risk of Serious Adverse Events Likely Due to Transmission of Pathogenic Organisms. Silver Spring, MD: US-FDA (2020). Available online at: https://www.fda.gov/vaccines-blood-biologics/safety-availability-biologics/update-march-12-2020-safety-alert-regardi ng-use-fecal-microbiota-transplantation-and-risk-serious (accessed March 23, 2022).
Keywords: gut microbiota, dysbiosis, NCDs, immunomodulatory nutritional intervention, probiotics
Citation: Kumar A, Sakhare K, Bhattacharya D, Chattopadhyay R, Parikh P, Narayan KP and Mukherjee A (2022) Communication in non-communicable diseases (NCDs) and role of immunomodulatory nutraceuticals in their management. Front. Nutr. 9:966152. doi: 10.3389/fnut.2022.966152
Received: 13 June 2022; Accepted: 11 August 2022;
Published: 21 September 2022.
Edited by:
Balamurugan Ramadass, All India Institute of Medical Sciences Bhubaneswar, IndiaReviewed by:
Soumya Basu, Dr. D. Y. Patil Biotechnology & Bioinformatics Institute, IndiaBiswatrish Sarkar, Birla Institute of Technology, Mesra, India
Copyright © 2022 Kumar, Sakhare, Bhattacharya, Chattopadhyay, Parikh, Narayan and Mukherjee. This is an open-access article distributed under the terms of the Creative Commons Attribution License (CC BY). The use, distribution or reproduction in other forums is permitted, provided the original author(s) and the copyright owner(s) are credited and that the original publication in this journal is cited, in accordance with accepted academic practice. No use, distribution or reproduction is permitted which does not comply with these terms.
*Correspondence: Kumar P. Narayan, pranav@hyderabad.bits-pilani.ac.in; Anubhab Mukherjee, dranubhab@esperernutrition.com
†These authors have contributed equally to this work