- 1School of Life Science and Engineering, Southwest University of Science and Technology, Mianyang, China
- 2Key Laboratory of Horticulture Science for Southern Mountainous Regions of Ministry of Education, College of Horticulture and Landscape Architecture, Southwest University, Chongqing, China
- 3The Southwest Institute of Fruits Nutrition, Chongqing, China
Although the Citrus tangerina cultivar “Dahongpao” (CTD) has been established as a rich source of polymethoxyflavones (PMFs) with anti-inflammatory and anti-cancer properties, their individual effects on cellular signaling remain to be elucidated. In this study, five major PMFs from the peel of CTD were isolated, including sinensetin, tetramethyl-O-scutellarin (5,6,7,4′-tetramethoxyflavone), nobiletin (5,6,7,8,3′, 4′-hexamethoxyflavone), tangeretin (5,6,7,8,4′-pentamethoxyflavone), and 5-demethylnobiletin (5-OH-6,7,8,3′,4′-pentamethoxyflavone). These PMFs were found to significantly (p < 0.05) inhibit the production of NO and biomarkers of chronic inflammation (TNF-α and IL-6). Additionally, they effectively suppressed mRNA biomarkers of acute inflammation (Cox-2 and iNOS), and to varying degrees promoted the activation of anti-inflammatory cytokines (IL-4, IL-13, TNF-β, and IL-10). Among the five PMFs, tangeretin was found to have a considerable anti-proliferative effect on tumor cell lines (PC-3 and DU145) and synergistically enhanced the cytotoxicity of mitoxantrone, partially via activation of the PTEN/AKT pathway. The findings of this study provide valuable insights into the activity of different PMF monomers and advance the understanding of the roles of PMFs in promoting apoptotic and anti-cancer effects.
Introduction
Citrus tangerina “Dahongpao” (CTD), a member of the Rutaceae family, is a tangerine cultivar widely grown in southwestern China. For centuries, the dried peels of mandarin/tangerine (C. reticulata L.), also referred to as “chenpi,” have been used to relieve and treat the symptoms of chronic or acute inflammation associated with digestive disorders (1), and recent pharmacological studies have shown that numerous components contained in aged citrus peel, particularly polymethoxylated flavones (PMFs), are the main bioactive constituents (2). PMFs are a subgroup of plant flavonoids that have attracted increasing attention on account of their broad-spectrum biological activities, including anti-cancer, anti-inflammatory, neuro-protective, anti-microbial, and anti-oxidative properties (3–5). With respect to these aforementioned properties of PMFs, the number and positions of methoxy groups have been identified as important factors contributing to their biological activity, with those containing a larger number of methoxy groups, being characterized by higher hydrophobicity, and thus stronger bioactivity (6). Although advanced phytochemical isolation and structural identification techniques have revealed more than 80 citrus PMFs (7), studies conducted to date have tended to focus on just two of these, nobiletin and tangeretin, whereas relatively little information is available regarding other PMFs (5, 7). Various PMFs showed differential effects on anti-inflammatory and anti-cancer, making it difficult to identify which activity was responsible for their protective effects.
In most acute or chronic diseases, inflammation always occurs at the beginning or end of diseases (8). Moderate inflammatory cytokines can help the body clear the invading pathogens, however, when homeostasis is disrupted, inflammation will develop into long-term diseases or acute injuries (9). Acute inflammations such as sepsis, endotoxemia, and some acute tissue damage release large amounts of pro-inflammatory cytokines and eventually break host immune tolerance (10). In response to acute infection or tissue damage, macrophages (M1 macrophages) initiate or increase the production of broad-spectrum pro-inflammatory cytokines (IL-1β, interleukin 1 beta; Cox-2, cyclooxygenase 2) and other inflammatory mediators (iNOS, inducible NO synthase) (11). Besides, tumor necrosis factor α (TNF-α) produced by macrophages also functions as a mediator of acute inflammation, platelet activation, and participation in the genesis of fever and anemia (12). Meanwhile, M2 macrophages, which are induced by anti-inflammatory cytokines including IL-4, IL-10, IL-13, and transforming growth factor β (TGF-β), are associated with anti-inflammation, wound healing, and tissue repair. Systemic chronic inflammation is a low-level, non-infectious chronic inflammatory state, which is closely related to cardiovascular disease, obesity, type 2 diabetes, various cancers, and neurodegenerative diseases. Monocytes are the precursors of the lipid-laden foam cells within atherosclerotic plaque and adipose tissue that produce sustainable levels of chronic pro-inflammatory cytokines such as TNF-α and IL-6 (13). Hence, attempts to identify anti-inflammatory agents and dietary regimens to prevent pro-inflammatory processes in the early stages of the expression of key pro-inflammatory mediators and cytokine genes have attracted a lot of interest.
Cancer has been a leading cause of morbidity and mortality worldwide for decades. Citrus PMF extracts have been used clinically to treat different types of cancer and exert anti-cancer activity in every stage. Citrus grandis Osbeck leaf extract, containing isosinensetin, sinensetin, tetramethyl-O-isoscutellarein, nobiletin, tangeretin and 5-demethylnobiletin, significantly reduced the survival rate of HeLa cells (14). Three major 5-hydroxy PMFs, namely: 5-demethylnobiletin, 5-hydroxy-3,6,7,8,3′,4′-hexamethoxyflavone, 5-hydroxy-6,7,8,4′-tetramethoxy-flavone, had overt inhibitory effects on human colon cancer HCT116 and HT29 cells (15). In addition, oral administration of 5-demethylnobiletin strongly lowered colon tumor incidence and reduced overall tumor size by decreasing the inflammatory mediators IL-1, IL-6, iNOS, and COX-2 in colonic tissue (16). Tangeretin inhibits cancer cells that lack the ability to repair DNA double-strand breaks (17). In addition, nobiletin arrested the cell cycle and reduced the cell viability of different cell lines. In terms of anti-cancer properties, PMFs can promote both intrinsic and extrinsic apoptotic pathways via the induction of p53 expression or the Bax: Bcl-2 protein ratio (7, 18), whereas different PMFs have been shown to have differential anti-proliferative activities against human cancer cell lines and circulating levels of NO (19). In addition, tangeretin can modulate energy production, inhibit cancer growth, induce apoptosis, and suppress cell cycle progression and migration in cancer cells (20). Moreover, it has recently been demonstrated that PMF monomers, including nobiletin, tangeretin, and 5-demethylnobiletin, can inhibit the proliferation of three gastric cancer cell lines and induce apoptosis (4). Interestingly, the findings of previous studies have identified a synergistic effect between certain anti-proliferative agents and tangeretin in enhancing anti-cancer activity (21). Prostate cancer is among the most prevalent malignancies and the second most prominent cause of cancer-related deaths in men (22, 23). Whereas, neuroendocrine prostate cancer accounts for ~2% of cases, it has been established that cells expressing neuroendocrine biomarkers can be detected in between 10 and 100% of prostate adenocarcinomas (24). However, although the bioactivities analyses of PMFs in different Citrus species have been reported (7, 19), the therapeutic efficacy of PMF monomers in prostate cancer remains poorly understood.
In this study, a macrophage-based system was used to compare the bioactivities of five major PMFs isolated from the peel of CTD on acute and chronic inflammation and anti-inflammatory factors. Moreover, the anti-proliferative properties of these compounds against human prostate cancer cell lines (PC-3 and DU145) were examined using a 3-(4,5-dimethylthiazol-2-yl)-2,5-diphenyltetrazolium bromide (MTT) assay, and the synergistic effects of co-administered mitoxantrone and tangeretin were also evaluated on these cancer cell lines.
Materials and methods
Standards and chemicals
Tangeretin, 3,5,6,7,8,3′,4′-heptamethoxyflavone, and 5-hydroxy-6,7,8,3′,4′-pentamethoxyflavone (5-demethylnobiletin) were obtained from ChromaDex (Santa Ana, CA, USA); isosinensetin, sinensetin, 5,6,7,4′-tetramethoxyflavone (tetramethyl-O-scutellarin), and nobiletin were obtained from SinoStandards (Chengdu, China); and HPLC-grade methanol, formic acid, dimethyl sulfoxide (DMSO), and MTT were obtained from Sigma (St. Louis, MO, USA). A Milli-Q system (Millipore, Bedford, MA, USA) was used to produce deionized water (18.20 M cm). All other analytical grade reagents were purchased from Chuandong Chemical Reagent Co., Ltd. (Chongqing, China).
Plant materials
CTD fruits were harvested during the commercial maturity stage (in October) from the National Citrus Germplasm Repository (Chongqing, China), and authenticated by Professor Dong Jiang (Citrus Research Institute, Southwest University). A voucher specimen (voucher No. LR0094) has been deposited in Citrus Research Institute, Southwest University, Chongqing, China. The clean peel of these fruits was dried at 40°C in a circulating drying cabinet, and then ground and passed through a 60-mesh sieve.
Extraction, enrichment, and isolation of PMFs
PMFs were prepared using the procedure described in the previous study, with minor modifications (25). Briefly, the air-dried citrus peel powder (5.0 kg) was ultrasound (300 W)-extracted twice with 100 L of 90% ethanol (each for 34 min) at 41°C and concentrated in vacuo to obtain a crude extract. This extract was diluted in 25% ethanol prior to loading onto an HPD 300 macroporous resin-based column chromatography system. Following adsorption, the impurities were removed by eluting with distilled water and 7% ethanol. A PMF-rich extract was obtained by subsequent elution with 90% ethanol, and following rotary evaporation to remove all organic reagents, was frozen at −80°C and freeze-dried to obtain a lyophilized powder. The freeze-dried PMF-rich extract (5.15 g) was subsequently redissolved in methanol to a concentration of 100 mg/mL, from which individual PMFs were separated and isolated using a prep-HPLC system equipped with a 515 HPLC Pump, a Waters 2767 Sample Manager, an ACQUITY QDa detector, and an XBridge Shield RP18 column (19 × 250 mm, 5 μm; Milford, MA, USA).
PMF identification
To identify the constituents of the PMF-rich extract and determine the purity of individual PMFs, we performed ultra-high-performance liquid chromatography-photodiode array (UPLC-PDA) spectrometry (Waters, Milford, MA, USA). Samples were separated on an ACQUITY UPLC BEH C18 column (2.1 × 100 mm, 1.7 mm, UK) at a column temperature of 50°C. The mobile phases were water containing 0.05% formic acid (solvent A) and methanol (solvent B), with gradient elution performed as follows: 0–0.6 min, 90–80% A; 0.6–5 min, 80–30% A; 5–7 min, 30–10% A; 7–9 min, 10–90% A. The flow rate was set to 0.3 mL/min and the detection wavelength was 330 nm. Nuclear magnetic resonance (NMR) spectra were obtained using a Bruker NMR AVANCE III10600 spectrometer (Bruker BioS-pin, Karlsruhe, Germany).
Cell culture
Murine macrophage RAW264.7 cells (ATCC®, Chicago, USA) were incubated in Dulbecco's Modified Eagle's Medium (DMEM; Fisher) containing 10% (v/v) fetal bovine serum (FBS; BI, Israel) and 1% (v/v) penicillin-streptomycin (Gibco™, USA). The human prostate cancer cell lines PC-3 and DU145 (ATCC®, Chicago, USA) were maintained in 50% DMEM and 50% F-12K medium (Fisher) supplemented with 10% FBS and 1% penicillin-streptomycin. These cell lines were incubated at 37°C in a 5% CO2 humidified incubator. PMF samples used for cell assays were prepared as 1,000 × stock solutions in DMSO and unless otherwise stated, were maintained at 20°C until used.
Cell viability assay
RAW264.7 cells were seeded in 96-well plates at a density of 8 × 103 cells/well and left to stabilize for 24 h. The viability of cells treated with PMFs was determined after a 48-h incubation using an MTT assay, in which sextuplicate samples were quantified spectrophotometrically at 570 nm using a Synergy H1 microplate reader (BioTek, Winooski, Vt., USA). The concentrations of test reagents that promoted no changes in the viability of RAW264.7 cells compared with that of the vehicle were selected for further studies.
Anti-inflammatory activity assay
RAW264.7 cells (5 × 106 cells/3 mL/dish in 35-mm culture dishes) were pretreated with PMFs (25 μM) or DMSO for 24 h, after which the cells were treated with fresh medium containing 1 μg/mL LPS and incubated for a further 24 h. Dexamethasone (DEX, 10 μM) was employed as a positive control. NO production in the supernatant was determined using a Griess reagent system (Beyotime, Beijing, China) according to the manufacturer's instructions. Absorbance was determined at 540 nm. The levels of anti-inflammatory cytokines (IL-4, IL-10, IL-13, and TGF-β) and pro-inflammatory cytokines (IL-6, IL-1β, and TNF-α) in the supernatant were measured using enzyme-linked immunosorbent assay kits (Shanghai Optimization Biological Technology Co., LTD.) according to manufacturer's protocol.
Anti-proliferative activity assay
For the purposes of anti-proliferative activity assays, following cell stabilization, the medium was replaced with fresh media containing differing amounts of test compounds (6.25, 12.5, 25, 50, 100, and 150 μM), and the cell viability was determined after incubating for a further 48 h, as described in Section Cell viability assay. Anti-proliferative activity was determined using the following equation:
Morphological assay
Clonogenic assays were performed using cells (1 × 103 cells/well) seeded in 6-well plates and pretreated with tangeretin (12.5 μM), mitoxantrone (10 nM), or the combination thereof for 24 h. Thereafter, the reagent-containing medium was replaced with fresh medium and the treated cells were incubated for a further 7 days. The resulting colonies were stained with 0.25% crystal violet for counting.
For nuclear morphology assays, cells (1 × 104 cells/well) were seeded in 24-well plates and left for 24 h. Thereafter, the stabilized cells were treated with tangeretin (12.5 μM), mitoxantrone (10 nM), or the combination thereof for 48 h. The cells were then fixed with 4% paraformaldehyde for 30 min and stained with 1 μg/mL 4′,6′-diamidino-2-phenylindole for 15 min at room temperature. The cells were subsequently washed with phosphate-buffered saline and viewed under an Olympus BX53 upright fluorescence microscope (Olympus). Images were captured using a DP27 digital camera and cellSens Dimension software.
Total RNA extraction, purification, and cDNA synthesis
Cells were harvested in RNase-free EP tubes, from which total RNA was extracted using TRIzol reagent (Invitrogen). cDNA synthesis using a reverse transcription cDNA synthesis kit (TIANGEN Biotech, Beijing) and quantitative PCR were performed using a Step One Plus Real-Time PCR Systems under the following PCR cycling conditions: 95°C for 1 min; 95°C for 20 s (45 cycles), and 60°C for 60 s. The oligonucleotide primers used for amplification (Supplementary Table 1) were synthesized by Beijing Hua-da Genetic Engineering Company. β-actin was used as an internal reference gene, and relative gene expression was calculated using the 2−ΔΔCt method (26).
Western blotting
PC-3 cells (5 × 106 cells/well) were seeded in 80-mm dishes, left to stabilize for 24 h, and then treated with tangeretin, mitoxantrone, or the combination thereof for a further 48 h. Cytoplasmic proteins were extracted using the procedure described by Yoon and Liu (27) and a BCA kit (Beyotime, China) was used to determine protein contents. Equal amounts of isolated protein samples were separated using 10% SDS-PAGE gels, and the separated proteins were subsequently transferred to PVDF membranes (Merck Millipore). The membranes were then blocked for 1 h at room temperature with non-fat dry milk (5%) in tris buffer containing Tween-20 solution (TBST, 0.1%), prior to being probed with primary antibodies (Bcl-XL, Caspase-9, and Caspase-3) overnight at 4°C. The membranes were then incubated for 1 h at room temperature in TBST solution containing a horseradish peroxidase-conjugated second antibody. Protein bands were visualized using Odyssey CLX infrared imaging system (LICOR Bioscience).
Data analysis
Data are presented as the means ± standard deviation (SD). The data were analyzed by one-way analysis of variance (ANOVA) using the statistical package for social sciences software (SPSS 22.0; IBM, USA). A p-value < 0.05 was taken to be indicative of statistical significance.
Results and Discussion
Quantitative analysis and isolation of PMFs
The seven main PMF compounds, namely six OCH3-PMFs and one OH-PMF, were quantitatively detected in the peel extract of “Dahongpao” tangerine by UPLC–PAD (Supplementary Figure 1). The PMF-rich extract from CTD contained nobiletin (210.87 ± 0.57 mg/g extract), tangeretin (55.66 ± 0.62 mg/g), sinensetin (28.11 ± 1.11 mg/g), 5-demethylnobiletin (24.66 ± 0.33 mg/g), isosinensetin (12.90 ± 0.12 mg/g), tetramethyl-O-scutellarin (12.14 ± 0.36 mg/g), and 3,5,6,7,8,3′,4′- heptamethoxyflavone (7.68 ± 0.46 mg/g) as the major PMF components (Supplementary Table 2). Consistent with the findings of previous studies (25), nobiletin and tangeretin were the PMFs detected in the highest amounts in the tangerine peel. To further evaluate the activity of these metabolites, five PMFs from the peel of CTD were separated and purified using MS-directed prep-HPLC (Figure 1). The yields and spectroscopic data of these compounds are shown in Table 1 and Supplementary Figures S2–S11.
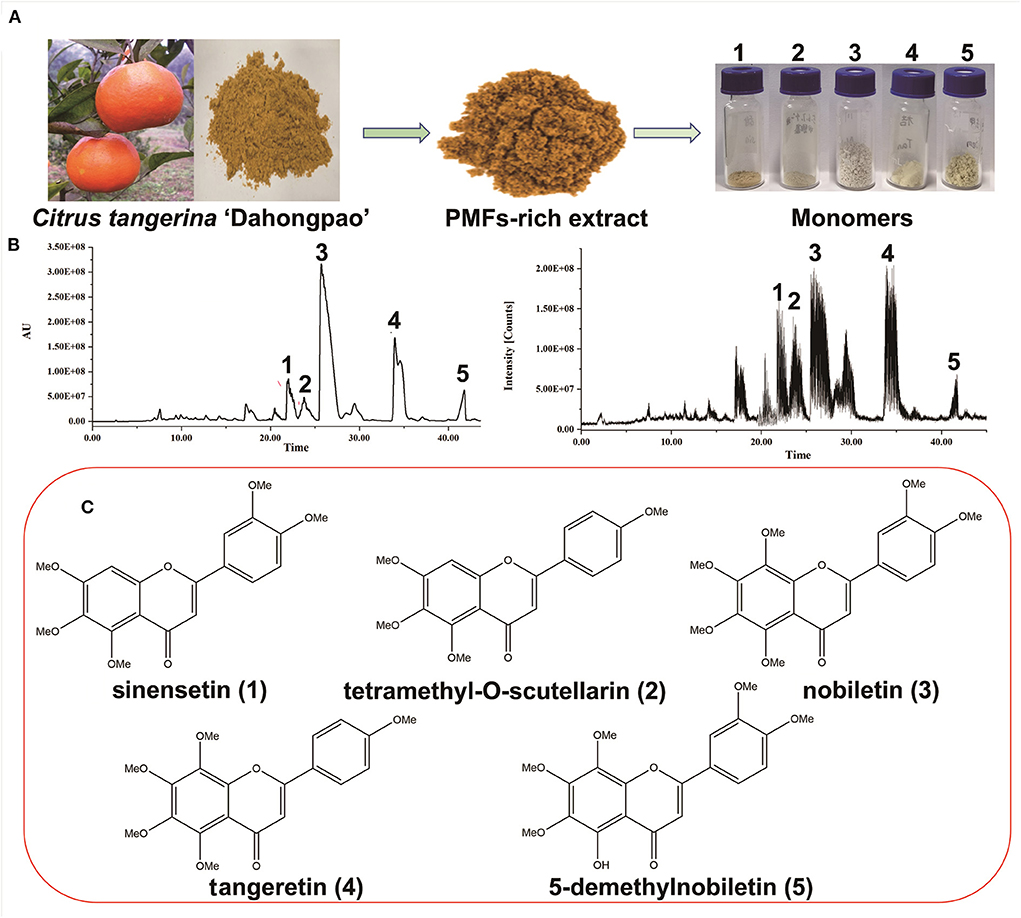
Figure 1. Purification and identification of polymethoxyflavones (PMFs) from Citrus reticulata “Dahongpao.” (A) The preparation of PMFs; (B) Chromatogram and MSE ion profile of PMFs; (C) Chemical structure of PMFs.
Effect of different citrus PMFs on the viability of RAW 264.7 cells
The cytotoxic effects of the five isolated PMFs on RAW 264.7 macrophage viability after 48 h of exposure were assessed using the MTT assay (Figure 2). The results revealed that none of these PMFs had any adverse effects regarding cell viability in the concentration range 0.625–25 μM, which we accordingly used in the subsequent studies. However, when applied at 50 μM, all five PMFs significantly reduced cell viability to levels below 90% and the inhibitory effects were enhanced at higher concentrations.
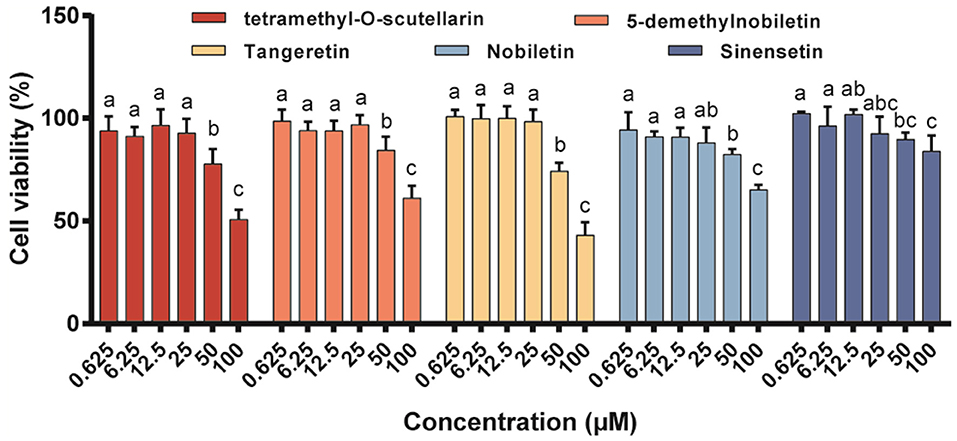
Figure 2. Effects of different PMFs on cell viability of murine RAW 264.7 macrophages. Data were presented as the means ± SD for three independent experiments. Means denoted by different lower-case letters were significantly different (One-way ANOVA, Dunnett's post-hoc test, p < 0.05).
Effect of different citrus PMFs on inflammatory biomarkers
The utility of LPS as a systemic inducer of inflammatory responses is based on its dual effects of inducing low-level chronic inflammation associated with gastrointestinal dysfunction and increased endotoxin flux (28), as well as endothelial cell damage and an acute inflammatory response (29). In the present study, individual PMFs were incubated with LPS-activated Raw 264.7 macrophages to ascertain cellular inflammatory activity. In this regard, nitric oxide (NO) is a highly reactive biological messenger that is overexpressed in inflammatory reactions and different diseases and can induce DNA damage (30), The control of intracellular NO production accordingly represents an effective approach for inhibiting inflammatory responses (19). When LPS was administrated alone, macrophages showed an approximately 2.5-fold increase in NO production compared with that observed in control cells (Figure 3A). In contrast, all assessed PMFs significantly inhibited the production of NO. Notably, when applied at a concentration of 25 μM, tetramethyl-O-scutellarin and nobiletin inhibited NO induction to the same level as the positive control (10 μM dexamethasone).
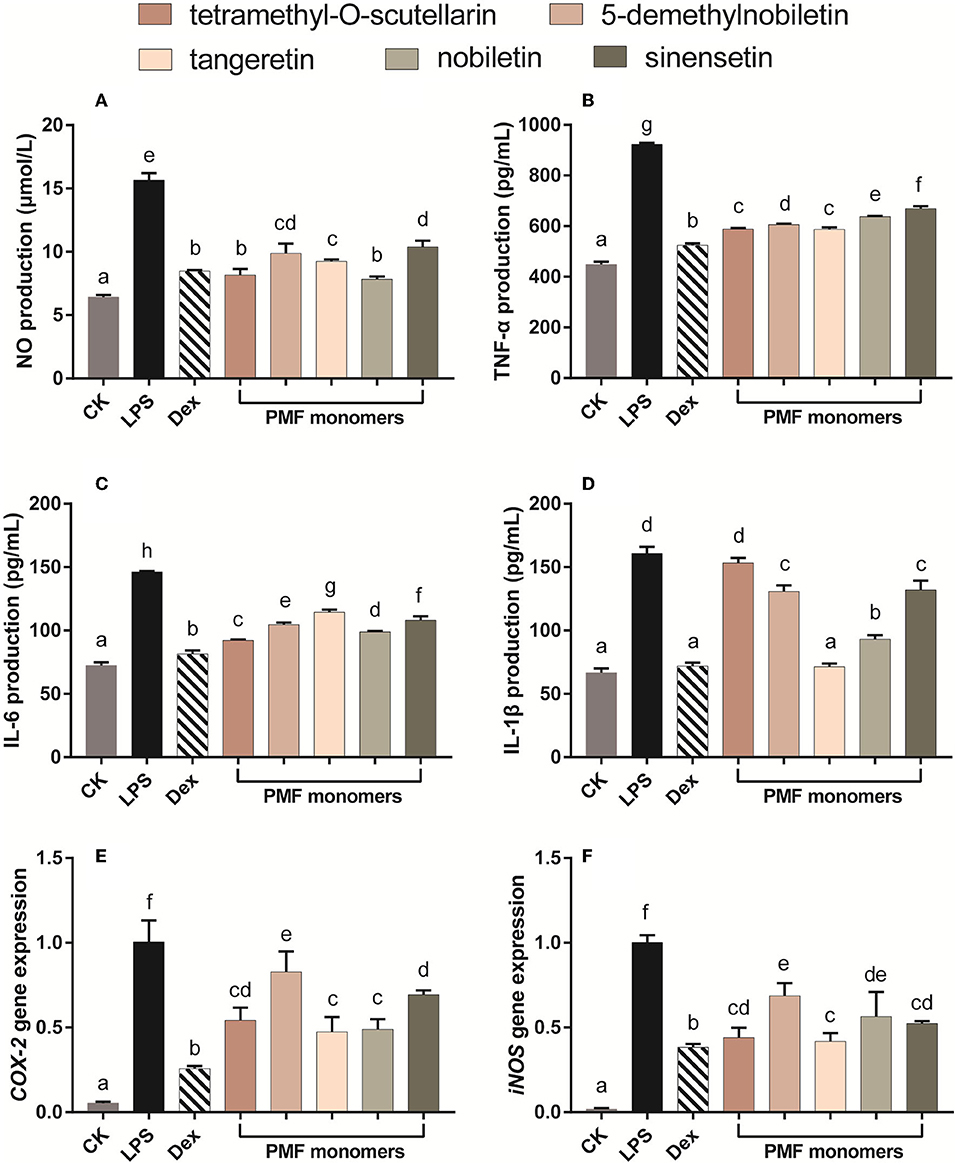
Figure 3. Effects of different PMFs on NO production (A), pro-inflammatory cytokine and gene expression profiles associated with chronic inflammatory (B,C) and acute inflammatory response (D–F) in the LPS-stimulated RAW264.7 macrophage cells. Results are expressed as means ± SD, n = 3 experiments. Means not sharing the same letter were significantly different (One-way ANOVA, Dunnett's post-hoc test, p < 0.05 was considered significant).
With the exception of the inhibitory effect of tetramethyl-O-scutellarin on IL-β, all assessed PMF monomers induced a significant suppression of all evaluated biomarkers of inflammation (Figures 3B–F). With respect to TNF-α production, the inhibitory capacity of the five PMFs could be ordered as follows: tetramethyl-O-scutellarin = tangeretin > 5-demethylnobiletin > nobiletin > sinensetin. Similarly, in the case of IL-6 production, the inhibitory effects could be ordered as follows: tetramethyl-O-scutellarin > nobiletin > 5-demethylnobiletin > sinensetin > tangeretin. These findings are comparable to those previously reported for three polymethoxyflavones (nobiletin, tangeretin, and 5-demethylnobiletin) isolated from ougan (C. reticulata Cv. Suavissima), which have been found to inhibit NO production, and the expressions of IL-1β, IL-6, and TNFα (31). Among these targets, IL-1β expression was found to be the most strongly affected by treatments, with the exception of tetramethyl-O-scutellarin, which showed no significant effect regarding the attenuation of LPS-induced IL-1β expression. Notably, tangeretin was found to be the only monomer showing borderline inhibition of IL-β at 25 μM (p < 0.05). In addition, the expression of the pro-inflammatory genes Cox-2 and iNOS was found to be strongly suppressed by all monomers, with tangeretin being the most effective in this regard. Nobiletin significantly inhibited LPS-induced PGE 2 production and IL-1α, IL-1β, TNF-α, and IL-6 in mouse J774A.1 macrophages. Especially, nobiletin selectively down-regulated the expression level of COX-2 instead of COX-1(32). The administration of tangeretin strongly alleviated the inflammatory injury in the kidney triggered by cisplatin in rats possibly through decreasing TNF-α level and augmenting IL-1α in molecular level (33). Moreover, oral administration of nobiletin effectively suppressed tumor formation and metastasis in the xenograft mice, and lowered the levels of NF-κB in the isolated tumors (34). It has been established that the anti-inflammatory activity of citrus flavonoids is closely associated with the structural properties of different flavonoids (35), and in this respect, it is speculated that the strong inhibitory capacities of tangeretin and nobiletin against IL-β and Cox-2 are correlated with the methoxy substituents at positions 5 and 8 (36). Consistent with the inhibitory effect of NO production, we speculate that the hydroxyl group may be associated with attenuating the inhibitory activity of PMFs on the expression of iNOS (36). In summary, these findings indicate that differences in the inhibitory effects of PMFs against biomarkers of inflammation could be attributable to difference their molecular structures (37).
Effect of different citrus PMFs on anti-inflammatory cytokines
Cytokines, which include both pro-inflammatory and anti-inflammatory types, are well-established regulators of host responses to infection, immune responses, inflammation, and trauma. Inflammatory processes are controlled by anti-inflammatory cytokines, including IL-10 and IL-4, the activity of which contributes to the inhibition of the production of pro-inflammatory cytokines such as IL-1, IL-6, and TNF-α (38). Exposure of murine RAW 264.7 macrophages cells to LPS leads to excessive production of pro-inflammatory cytokines, which in turn initiates the activation of inflammatory cascades (1). In the present study, the levels of the anti-inflammatory cytokines IL-4, IL-13, TNF-β, and IL-10 in LPS-activated Raw 264.7 macrophages were increase in response to incubation with most of the assessed individual PMF (Figure 4). IL-4 production was most strongly affected by sinensetin, which showed no difference with the positive control (Figure 4A). The activation capacity for IL-13 was in the order of tangeretin ≥ tetramethyl-O-scutellarin ≥ nobiletin ≥ 5-demethylnobiletin > sinensetin (Figure 4B). TGF-β plays an essential role in the chemotaxis of macrophage and fibroblast toward wounds, and the interaction between macrophages and TGF-β has been established to contribute to wound repair (39). In the present study, 5-demethylnobiletin, sinensetin, tetramethyl-O-scutellarin, and nobiletin were generally effective in promoting an increase in TGF-β (Figure 4C). It has also been established that the phenotype of macrophages undergoes a transition from M1 to M2 in response to the up-regulated expression of IL-10 (40). We found that the individual citrus PMFs had a significant promotive effect on IL-10, with tangeretin being observed to strongest capacity in this regard (Figure 4D). On the basis of the findings with respect to IL-10, we speculated that IL-10/STAT3 signal-mediated macrophage phenotypic transition is one of the pathways via which PMFs contribute to an alleviation of LPS-induced inflammation (40). In summary, on the basis of comparative analysis of the structures of the five assessed PMF monomers, the difference in the mechanisms of action could be associated with the number and position of methoxy groups and the presence of hydroxyl groups.
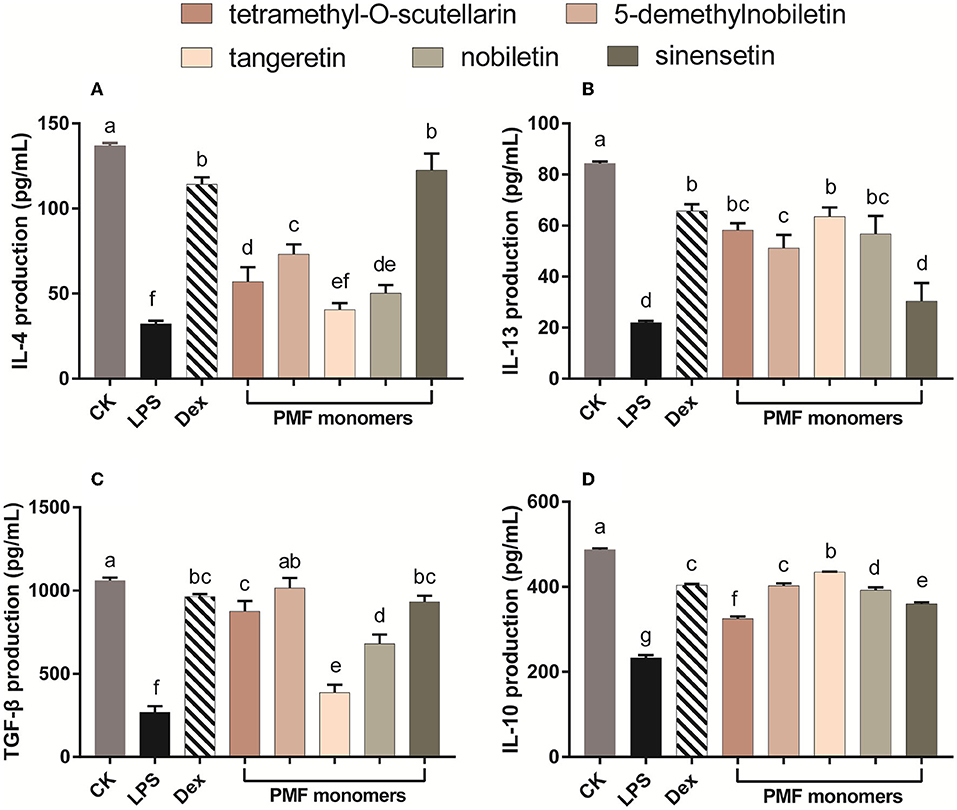
Figure 4. Effect of different PMFs on anti-inflammatory cytokines in the LPS-stimulated RAW264.7 macrophage cells. (A) IL-4; (B) IL-13; (C) TGF-â; (D) IL-10. Results were expressed as means ± SD, n = 3 experiments. Means denoted by different lower-case letters are significantly different (One-way ANOVA, Dunnett's post-hoc test, p < 0.05).
Effect of different citrus PMFs on anti-proliferative activity
Compared with that in normal cells, reactive oxygen species (ROS) homeostasis in cancer cells is maintained at a generally higher level and that the prostate cancer cells PC-3 and DU145 are characterized by higher ROS generation than normal prostate cells (41, 42). In addition, up to 25% of the lethal metastatic cancers have been shown to comprise neuroendocrine cells, and the PC-3 cell line is characterized by a neuroendocrine or small cell carcinoma type rather than an adenocarcinoma type (43, 44). In the present study, two different human prostate cancer cell lines (DU145 and PC-3) were used to evaluate the anti-proliferative activity of the different citrus PMFs (Figure 5), the IC50 values (concentration of the test compound that causes 50% inhibition) of which are listed in Supplementary Table 3. Among the assessed PMFs, tangeretin and 5-demethylnobiletin exhibited significant anti-proliferative activity against both these cell lines, whereas no appreciable inhibitory effects among cells treated with the other three PMFs (tetramethyl-O-scutellarin, nobiletin, and sinensetin) were observed at the assessed concentrations. In this context, the findings of previous studies have indicated that hydroxylation of PMFs at the 5-position of the A-ring is an essential pre-requisite for enhancing their anti-cancer activity (45), which can probably be ascribed to the formation of ionic bonds between the free phenolic hydroxyl group and charged amino acid residues (19). Indeed, these results confirmed that 5-demethylnobiletin was characterized by a stronger anti-proliferative activity than nobiletin. However, compared with 5-demethylnobiletin, tangeretin exhibited a notably stronger cytotoxic effect against the two assessed prostate cancer cell lines, with IC50 values of 22.12 and 46.60 μM for PC-3 (Figure 5A) and DU145 (Figure 5B), respectively. Available studies suggest that citrus PMFs can exert anticancer activity in six aspects, including reducing tumorigenesis by absorbing environmental carcinogens or eliminating mutations in vivo (17); promoting apoptosis by regulating apoptotic protein expression (20); inhibiting cancer cell proliferation or blocking nutrient and oxygen entry into cancer cells during the cell cycle; inhibiting tumor cell invasion or metastasis by suppressing matrix metalloproteinase expression; antagonizing multidrug resistance by inhibiting the activity of the ATP-binding cassette family of membrane transport proteins (46). Tangeretin and 5-demethylnobiletin. have been found to selectively upregulate expression of the RARB gene and activate Caspase-3 and−9 and PARP1 proteins to inhibit the proliferation of three gastric cancer cell lines and induce apoptosis. In addition to 5-demethylnobiletin and tangeretin upregulate the expression of cleaved Caspase 8, thereby implying that the pathway of 5-demethylnobiletin-induced apoptosis might differ slightly from that induced by tangeretin, which could be attributable to the hydroxy substitution at position 5 in the 5-demethylnobiletin molecule (4). Moreover, it has been established that PMFs are characterized by higher lipophilicity and greater permeability than hydroxy-PMFs, thereby tending to indicate that the potent bioactivity of PMFs may enhance cellular absorption (47). Collectively, our findings thus indicate that tangeretin is an effective anti-cancer agent that may potentially serve as a novel therapeutic option for the treatment of prostate cancer.
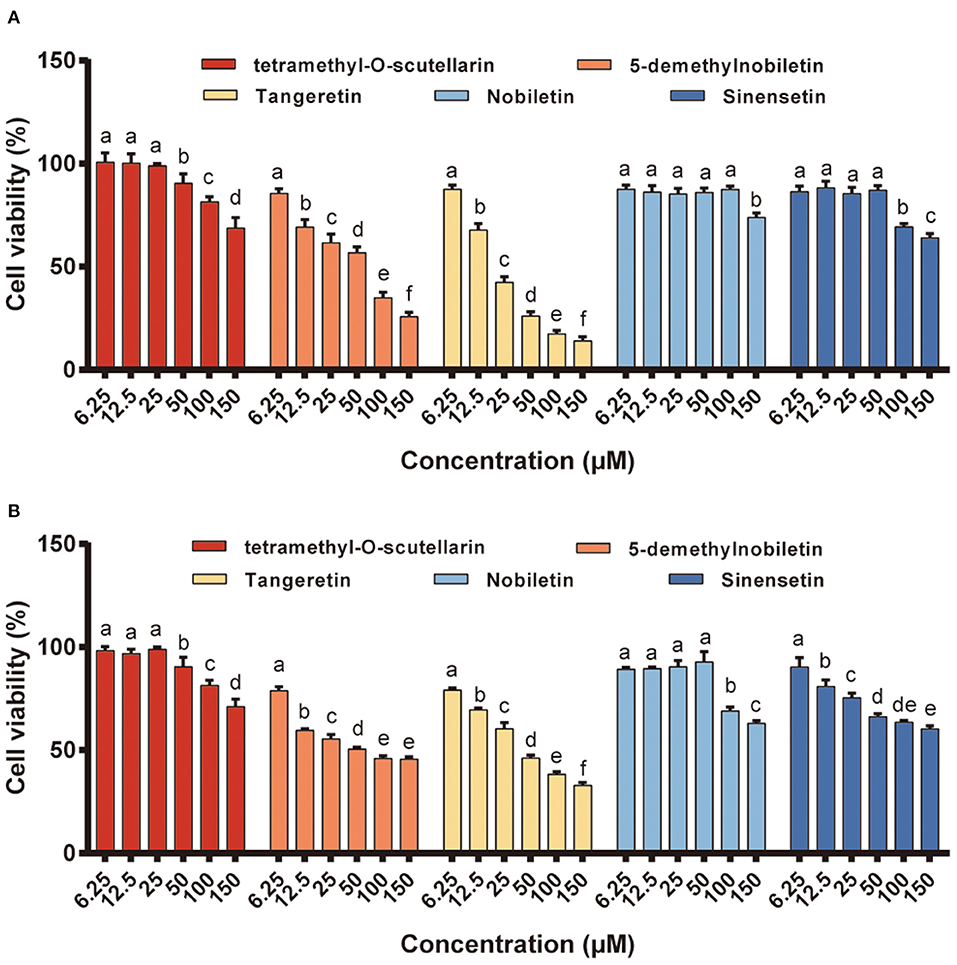
Figure 5. The anti-proliferation activity of different PMFs against two prostate cancer cell lines. (A) PC-3; (B) DU145. Results were expressed as the means ± SD, n = 3 experiments. Means denoted by different lower-case letters are significantly different (One-way ANOVA, Dunnett's post-hoc test, p < 0.05).
Tangeretin enhances the cytotoxicity of mitoxantrone in human prostate cancer DU145 and PC3 cell lines
Most malignancies are challenged by intrinsic drug resistance, and the combination of nature products with anticancer drugs is an intriguing new approach for cancer chemotherapy (7). Currently, mitoxantrone is commonly used as an anti-tumor drug for the effective treatment of different types of malignant tumor, including breast and advanced prostate cancers, lymphoma, and leukemia (48). Consistent with our findings that among the five assessed PMFs, tangeretin is the most effective anti-prostate cancer constituent in CTD, it has previously been reported that this PMF can enhance the bioavailability of anti-cancer agents by inhibiting the activity of efflux transporters (49). This accordingly prompted us to assess the therapeutic effects of combinations of anti-cancer drugs and tangeretin on prostate cancer.
On the basis of the results of clonogenic assays, tangeretin (12.5 μM) and mitoxantrone (12.5 nM) inhibited the cell colony formation of PC3 and DU145 cells when applied alone, which is consistent with the previously reported inhibition of clonogenic survival by tangeretin or mitoxantrone (48, 50). Having established the individual activities of these two agents, co-treatments with tangeretin and mitoxantrone was performed to examine any potential synergistic inhibitory effects with respect the cell colony-forming ability of the PC3 and DU145 lines (Figure 6A). Compared with the individual treatment groups, cells exposed to a combination of tangeretin and mitoxantrone showed clear evidence of apoptotic effects, including morphological shrinkage and nuclear condensation (Figure 6A). Apoptosis is known to be primarily attributable to the caspase (cysteinyl, aspartate-specific proteases) family of proteases (51). The anti-cancer properties of tangeretin with respect to prostate cancer cells has be found to be mediated via the PI3K/Akt/mTOR signaling pathway, regulated by the Bax, Bcl-2, Bcl-xL, caspase 9, caspase 3, PTEN, AKT, and mTOR genes (46), and one of the hallmarks of apoptosis of apoptosis in numerous cell types is the activation of Caspase-3,−7,−8, and−9 (52). In both PC3 and DU145 cell lines, identical patterns of apoptosis-related genes expression in response to treatment with tangeretin (Figure 6B). Consistent with previously reported observations (53), the levels of PTEN expression in both tangeretin and mitoxantrone groups were significantly (p < 0.05) higher than those detected in control group cells, whereas in contrast, the expression of AKT1, AKT2, and Bcl-2 mRNA was found to be significantly (p < 0.05) inhibited by either of these two compounds. However, compared with that seen in the controls, no significant changes were detected in the mRNA levels of Bax or caspase-3 in cells exposed to either tangeretin or mitoxantrone. Interestingly, however, simultaneously exposing cells to both tangeretin and mitoxantrone was observed to promote significant changes in the expression of Bax and caspase-3, and western blot analysis further confirmed that when co-administered, tangeretin and mitoxantrone markedly enhanced their individual effects by suppressing the expression of Bcl-XL and upregulating the expression of Caspase-9 and−3 (Figure 6C). Accordingly, compared with their individual application, administering tangeretin and mitoxantrone in combination contributed to an enhancement of anti-cancer efficacy. Growing studies have proved that tangeretin develop as a secure and useful antagonist of multidrug resistance. For example. tangeretin has synergistic cytotoxicity with imatinib in K562 cells through an apoptotic mechanism (54). In addition, compared with doxorubicin alone, the combination of doxorubicin and tangeretin improved the cytotoxic effect on MCF-7 and T47D cells (55). The potential mechanism involves significant inhibition of the ABCB1 transporter activity and reduced efflux of ABCB1 substrates by tangeretin (56).
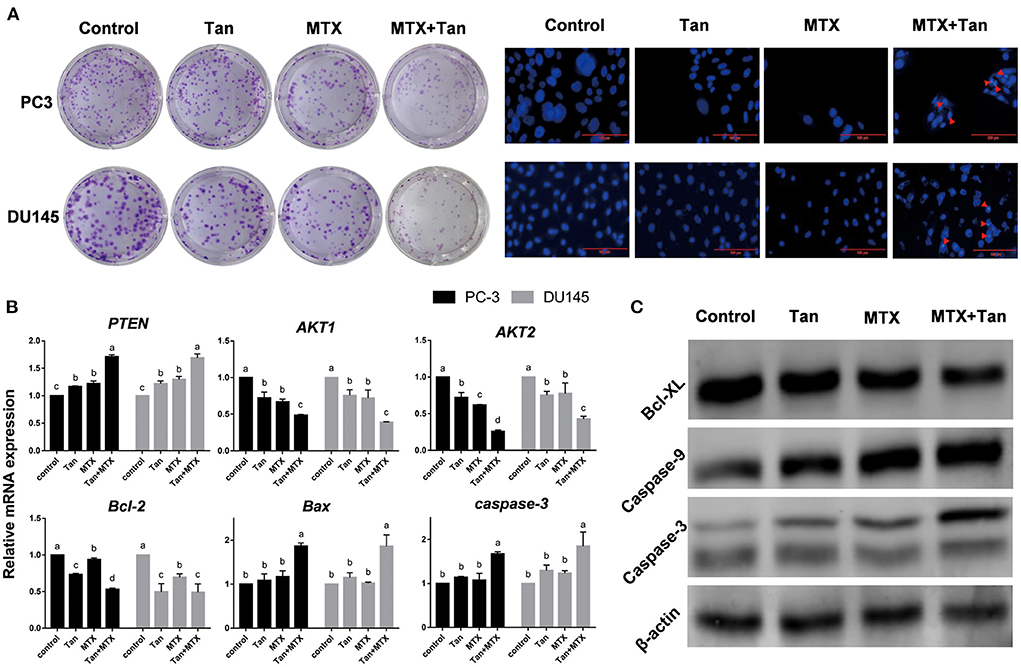
Figure 6. Morphology and gene and protein expression regulated by tangeretin (Tan), mitoxantrone (MTX), or their combination (MTX+Tan) in prostate cancer cell lines. (A) Images of clonogenic assay- and DAP-stained cells. The red arrowheads indicate apoptotic bodies. (B) Relative gene expression detected by qRT-PCR. (C) Protein expression detected by western blot analysis.
Conclusion
A distinctive citrus variety (Dahongpao) with a high content of PMFs was selected as the material to separate and purify five PMF monomers (sinensetin, 5,6,7,4′-tetramethoxyflavone, nobiletin, tangeretin, and 5-demethylnobiletin). All five PMFs were found to suppress LPS-induced NO release and reduced the production of chronic inflammation-related cytokines (TNF-α and IL-6) and the expression of acute inflammatory biomarkers (Cox-2 and iNOS). In addition, we demonstrated that these five compounds selectively activated different anti-inflammatory cytokines, with differential effects being ascribed to differences in molecular structure. Among the PMFs assessed, tangeretin showed the most pronounced anti-proliferative activity against prostate cancer cell lines (PC-3 and DU145) and was found to synergistically enhance the cytotoxic effects of mitoxantrone on these cells by modulating apoptosis-related genes and proteins. Collectively, our findings provide valuable information that will contribute to promoting the further utilization of CTD peel and individual PMFs as effective anti-inflammatory and anti-cancer agents.
Data availability statement
The original contributions presented in the study are included in the article/Supplementary material, further inquiries can be directed to the corresponding authors.
Author contributions
QC and ZZ: conceptualization. QC, CT, and YG: methodology and investigation. QC and DW: formal analysis, writing—original draft preparation, and writing—review and editing. BS and ZL: software and visualization. ZZ, QC, and DW: funding acquisition. All authors have read and agreed to the published version of the manuscript.
Funding
This research was funded by Applied Basic Research Program of Science and Technology Department of Sichuan Province (22NSFSC2544 and 22NSFSC2571), the Ph.D. Programs Foundation of Southwest University of Science and Technology (No. 22zx7111 and 22zx7112), and National Natural Science Foundation of China (No. 31772260).
Conflict of interest
The authors declare that the research was conducted in the absence of any commercial or financial relationships that could be construed as a potential conflict of interest.
Publisher's note
All claims expressed in this article are solely those of the authors and do not necessarily represent those of their affiliated organizations, or those of the publisher, the editors and the reviewers. Any product that may be evaluated in this article, or claim that may be made by its manufacturer, is not guaranteed or endorsed by the publisher.
Supplementary material
The Supplementary Material for this article can be found online at: https://www.frontiersin.org/articles/10.3389/fnut.2022.963662/full#supplementary-material
References
1. Chen XM, Tait AR, Kitts DD. Flavonoid composition of orange peel and its association with antioxidant and anti-inflammatory activities. Food Chem. (2017) 218:15–21. doi: 10.1016/j.foodchem.2016.09.016
2. Liu N, Li X, Zhao P, Zhang X, Qiao O, Huang L, et al. A review of chemical constituents and health-promoting effects of citrus peels. Food Chem. (2021) 365:130585. doi: 10.1016/j.foodchem.2021.130585
3. Ke Z, Zhao Y, Tan S, Chen H, Li Y, Zhou Z, et al. Citrus reticulata Blanco peel extract ameliorates hepatic steatosis, oxidative stress and inflammation in HF and MCD diet-induced NASH C57BL/6 J mice. J Nutr Biochem. (2020) 83:108426. doi: 10.1016/j.jnutbio.2020.108426
4. Wang Y, Chen Y, Zhang H, Chen J, Cao J, Chen Q, et al. Polymethoxyflavones from citrus inhibited gastric cancer cell proliferation through inducing apoptosis by upregulating RARbeta, both in vitro and in vivo. Food Chem Toxicol. (2020) 146:111811. doi: 10.1016/j.fct.2020.111811
5. Zhang M, Zhu SY, Ho CT, Huang QR. Citrus polymethoxyflavones as regulators of metabolic homoeostasis: recent advances for possible mechanisms. Trends Food Sci Tech. (2021) 110:743–53. doi: 10.1016/j.tifs.2021.02.046
6. Walle T. Methoxylated flavones, a superior cancer chemopreventive flavonoid subclass? Semin. Cancer Biol. (2007) 17:354–62. doi: 10.1016/j.semcancer.2007.05.002
7. Gao Z, Gao W, Zeng SL, Li P, Liu EH. Chemical structures, bioactivities and molecular mechanisms of citrus polymethoxyflavones. J Funct Foods. (2018) 40:498–509. doi: 10.1016/j.jff.2017.11.036
8. Gui Q, Jiang Z, Zhang L. Insights into the modulatory role of cyclosporine A and its research advances in acute inflammation. Int Immunopharmacol. (2021) 93:107420. doi: 10.1016/j.intimp.2021.107420
9. Ingersoll MA, Platt AM, Potteaux S, Randolph GJ. Monocyte trafficking in acute and chronic inflammation. Trends Immunol. (2011) 32:470–7. doi: 10.1016/j.it.2011.05.001
10. Xiao F, Farag MA, Xiao J, Yang X, Liu Y, Shen J, et al. The influence of phytochemicals on cell heterogeneity in chronic inflammation-associated diseases: the prospects of single cell sequencing. J Nutr Biochem. (2022) 108:109091. doi: 10.1016/j.jnutbio.2022.109091
11. Shi C, Pamer EG. Monocyte recruitment during infection and inflammation. Nat Rev Immunol. (2011) 11:762–74. doi: 10.1038/nri3070
12. Lacerda RB, Sales NM, da Silva LL, Tesch R, Miranda AL, Barreiro EJ, et al. Novel potent imidazo[1,2-a]pyridine-N-Glycinyl-hydrazone inhibitors of TNF-alpha production: in vitro and in vivo studies. PLoS ONE. (2014) 9:e91660. doi: 10.1371/journal.pone.0091660
13. Suganami T, Nishida J, Ogawa Y. A paracrine loop between adipocytes and macrophages aggravates inflammatory changes: role of free fatty acids and tumor necrosis factor alpha. Arterioscl Throm Vas. (2005) 25:2062–8. doi: 10.1161/01.ATV.0000183883.72263.13
14. Kim H, Moon JY, Mosaddik A, Cho SK. Induction of apoptosis in human cervical carcinoma HeLa cells by polymethoxylated flavone-rich Citrus grandis Osbeck (Dangyuja) leaf extract. Food Chem Toxicol. (2010) 48:2435–42. doi: 10.1016/j.fct.2010.06.006
15. Qiu P, Dong P, Guan H, Li S, Ho CT, Pan MH, et al. Inhibitory effects of 5-hydroxy polymethoxyflavones on colon cancer cells. Mol Nutr Food Res. (2010) 54(Suppl 2):S244–252. doi: 10.1002/mnfr.200900605
16. Song M, Wu X, Zheng J, Xiao H. 5-Demethylnobiletin inhibits colon carcinogenesis in azoxymethane/dextran sulfate sodium-treated mice. FASEB J. (2014) 28:3–4. doi: 10.1096/fasebj.28.1_supplement.123.3
17. Chong SY, Wu MY, Lo YC. Tangeretin sensitizes SGS1-deficient cells by inducing DNA damage. J Agric Food Chem. (2013) 61:6376–82. doi: 10.1021/jf401831e
18. Dong Y, Cao A, Shi J, Yin P, Wang L, Ji G, et al. Tangeretin, a citrus polymethoxyflavonoid, induces apoptosis of human gastric cancer AGS cells through extrinsic and intrinsic signaling pathways. Oncol Rep. (2014) 31:1788–94. doi: 10.3892/or.2014.3034
19. Duan L, Dou LL, Yu KY, Guo L, Chen BZ, Li P, et al. Polymethoxyflavones in peel of Citrus reticulata ‘Chachi' and their biological activities. Food Chem. (2017) 234:254–61. doi: 10.1016/j.foodchem.2017.05.018
20. Raza W, Luqman S, Meena A. Prospects of tangeretin as a modulator of cancer targets/pathways. Pharmacol Res. (2020) 161:105202. doi: 10.1016/j.phrs.2020.105202
21. Mdkhana B, Zaher DM, Abdin SM, Omar HA. Tangeretin boosts the anticancer activity of metformin in breast cancer cells via curbing the energy production. Phytomedicine. (2021) 83:153470. doi: 10.1016/j.phymed.2021.153470
22. Bray F, Ferlay J, Soerjomataram I, Siegel RL, Torre LA, Jemal A. Global cancer statistics 2018: GLOBOCAN estimates of incidence and mortality worldwide for 36 cancers in 185 countries. CA Cancer J Clin. (2018) 68:394–424. doi: 10.3322/caac.21492
23. Gundem G, Van Loo P, Kremeyer B, Alexandrov LB, Tubio JMC, Papaemmanuil E, et al. The evolutionary history of lethal metastatic prostate cancer. Nature. (2015) 520:353–7. doi: 10.1038/nature14347
24. Epstein JI, Amin MB, Beltran H, Lotan TL, Mosquera JM, Reuter VE, et al. Proposed morphologic classification of prostate cancer with neuroendocrine differentiation. Am J Surg Pathol. (2014) 38:756–67. doi: 10.1097/PAS.0000000000000208
25. Li ZQ, Zhao ZY, Zhou ZQ. Simultaneous separation and purification of five polymethoxylated flavones from “Dahongpao” tangerine (Citrus tangerina Tanaka) using macroporous adsorptive resins combined with Prep-HPLC. Molecules. (2018) 23:2660. doi: 10.3390/molecules23102660
26. Livak KJ, Schmittgen TD. Analysis of relative gene expression data using real-time quantitative pcr and the 2−ΔΔCT method. Methods. (2001) 25:402–8. doi: 10.1006/meth.2001.1262
27. Yoon H, Liu RH. Effect of selected phytochemicals and apple extracts on NF-κB activation in human breast cancer MCF-7 cells. J Agric Food Chem. (2007) 55:3167–73. doi: 10.1021/jf0632379
28. Dey P. Targeting gut barrier dysfunction with phytotherapies: Effective strategy against chronic diseases. Pharmacol Res. (2020) 161:105135. doi: 10.1016/j.phrs.2020.105135
29. Bannerman DD, Goldblum SE. Mechanisms of bacterial lipopolysaccharide-induced endothelial apoptosis. Am J Physiol Lung C. (2003) 284:L899–914. doi: 10.1152/ajplung.00338.2002
30. Heidari F, Rabizadeh S, Mansournia MA, Mirmiranpoor H, Salehi SS, Akhavan S, et al. Inflammatory, oxidative stress and anti-oxidative markers in patients with endometrial carcinoma and diabetes. Cytokine. (2019) 120:186–90. doi: 10.1016/j.cyto.2019.05.007
31. Wang Y, Zang W, Ji S, Cao J, Sun C. Three polymethoxyflavones purified from Ougan (Citrus reticulata Cv. Suavissima) inhibited LPS-induced NO elevation in the neuroglia BV-2 cell line via the JAK2/STAT3 pathway. Nutrients. (2019) 11:791. doi: 10.3390/nu11040791
32. Pan MH, Lai CS, Ho CT. Anti-inflammatory activity of natural dietary flavonoids. Food Funct. (2010) 1:15–31. doi: 10.1039/c0fo00103a
33. Arab HH, Mohamed WR, Barakat BM, Arafa el SA. Tangeretin attenuates cisplatin-induced renal injury in rats: Impact on the inflammatory cascade and oxidative perturbations. Chem Biol Interact. (2016) 258:205–13. doi: 10.1016/j.cbi.2016.09.008
34. Chien SY, Hsieh MJ, Chen CJ, Yang SF, Chen MK. Nobiletin inhibits invasion and migration of human nasopharyngeal carcinoma cell lines by involving ERK1/2 and transcriptional inhibition of MMP-2. Expert Opin Ther Tar. (2015) 19:1–14. doi: 10.1517/14728222.2014.992875
35. Bayat P, Farshchi M, Yousefian M, Mahmoudi M, Yazdian-Robati R. Flavonoids, the compounds with anti-inflammatory and immunomodulatory properties, as promising tools in multiple sclerosis (MS) therapy: a systematic review of preclinical evidence. Int Immunopharmacol. (2021) 95:107562. doi: 10.1016/j.intimp.2021.107562
36. Owis AI. Citrus polymethoxyflavones: biofunctional molecules of therapeutic interest. In: Rahman A, editor. Studies in Natural Products Chemistry. Amsterdam: Elsevier Science (2018). p. 509–30. doi: 10.1016/B978-0-444-64179-3.00015-3
37. Huang X, Zhu J, Wang L, Jing H, Ma C, Kou X, et al. Inhibitory mechanisms and interaction of tangeretin, 5-demethyltangeretin, nobiletin, and 5-demethylnobiletin from citrus peels on pancreatic lipase: kinetics, spectroscopies, and molecular dynamics simulation. Int J Biol Macromol. (2020) 164:1927–38. doi: 10.1016/j.ijbiomac.2020.07.305
38. Saad B, Abouatta BS, Basha W, Hmade A, Kmail A, Khasib S, et al. Hypericum triquetrifolium-derived factors downregulate the production levels of LPS-induced nitric oxide and tumor necrosis factor-alpha in THP-1 cells. Evid-Based Compl Alt. (2011) 2011:586470. doi: 10.1093/ecam/nen056
39. Qin S, Zheng JH, Xia ZH, Qian J, Deng CL, Yang SL. CTHRC1 promotes wound repair by increasing M2 macrophages via regulating the TGF-beta and notch pathways. Biomed Pharmacother. (2019) 113:108594. doi: 10.1016/j.biopha.2019.01.055
40. Sun Y, Tang J, Li C, Liu J, Liu H. Sulforaphane attenuates dextran sodium sulphate induced intestinal inflammation via IL-10/STAT3 signaling mediated macrophage phenotype switching. Food Sci Hum Well. (2022) 11:129–42. doi: 10.1016/j.fshw.2021.07.014
41. Sharma GP, Gurung SK, Inam A, Nigam L, Bist A, Mohapatra D, et al. CID-6033590 inhibits p38MAPK pathway and induces S-phase cell cycle arrest and apoptosis in DU145 and PC-3 cells. Toxicol In Vitro. (2019) 60:420–36. doi: 10.1016/j.tiv.2019.06.003
42. Koul HK, Pal M, Koul S. Role of p38 MAP kinase signal transduction in solid tumors. Genes Cancer. (2013) 4:342–59. doi: 10.1177/1947601913507951
43. Yadav SS, Li J, Stockert JA, Herzog B, O'Connor J, Garzon-Manco L, et al. Induction of neuroendocrine differentiation in prostate cancer cells by dovitinib (TKI-258) and its therapeutic implications. Transl Oncol. (2017) 10:357–66. doi: 10.1016/j.tranon.2017.01.011
44. Tai S, Sun Y, Squires JM, Zhang H, Oh WK, Liang CZ, et al. PC3 is a cell line characteristic of prostatic small cell carcinoma. Prostate. (2011) 71:1668–79. doi: 10.1002/pros.21383
45. Sergeev IN, Li S, Colby J, Ho CT, Dushenkov S. Polymethoxylated flavones induce Ca2+-mediated apoptosis in breast cancer cells. Life Sci. (2006) 80:245–53. doi: 10.1016/j.lfs.2006.09.006
46. Boye A, Ahmad I, Fakhri S, Hussain Y, Khan H. Incipient citrus polymethoxylated flavone Tangeretin as anticancer drug candidate: mechanistic insights, limitations and possible solutions. Adv Cancer Biol Metastasis. (2021) 3:100010. doi: 10.1016/j.adcanc.2021.100010
47. Hung WL, Chang WS, Lu WC, Wei GJ, Wang Y, Ho CT, et al. Pharmacokinetics, bioavailability, tissue distribution and excretion of tangeretin in rat. J Food Drug Anal. (2018) 26:849–57. doi: 10.1016/j.jfda.2017.08.003
48. Neuhaus O, Kieseier BC, Hartung HP. Therapeutic role of mitoxantrone in multiple sclerosis. Pharmacol Therapeut. (2006) 109:198–209. doi: 10.1016/j.pharmthera.2005.07.002
49. Suguro R, Pang XC, Yuan ZW, Chen SY, Zhu YZ, Xie Y. Combinational applicaton of silybin and tangeretin attenuates the progression of non-alcoholic steatohepatitis (NASH) in mice via modulating lipid metabolism. Pharmacol Res. (2020) 151:104519. doi: 10.1016/j.phrs.2019.104519
50. Roshini A, Jagadeesan S, Arivazhagan L, Cho YJ, Lim JH, Doh YH, et al. pH-sensitive tangeretin-ZnO quantum dots exert apoptotic and anti-metastatic effects in metastatic lung cancer cell line. Mater Sci Eng C. (2018) 92:477–88. doi: 10.1016/j.msec.2018.06.073
51. Li J, Yuan J. Caspases in apoptosis and beyond. Oncogene. (2008) 27:6194–206. doi: 10.1038/onc.2008.297
52. Lai SH, Jiang GB, Yao JH, Li W, Han BJ, Zhang C, et al. Cytotoxic activity, DNA damage, cellular uptake, apoptosis and western blot analysis of ruthenium(II) polypyridyl complex against human lung decarcinoma A549 cell. J Inorg Biochem. (2015) 152:1–9. doi: 10.1016/j.jinorgbio.2015.08.012
53. Morley KL, Ferguson PJ, Koropatnick J. Tangeretin and nobiletin induce G1 cell cycle arrest but not apoptosis in human breast and colon cancer cells. Cancer Lett. (2007) 251:168–78. doi: 10.1016/j.canlet.2006.11.016
54. Lust S, Vanhoecke B, Van Gele M, Philippe J, Bracke M, Offner F. The flavonoid tangeretin activates the unfolded protein response and synergizes with imatinib in the erythroleukemia cell line K562. Mol Nutr Food Res. (2010) 54:823–32. doi: 10.1002/mnfr.200900186
55. Meiyanto E, Fitriasari A, Hermawan A, Junedi S, Susidarti RA. The improvement of doxorubicin activity on breast cancer cell lines by tangeretin through cell cycle modulation. Oriental Pharm Exp M. (2011) 11:183–90. doi: 10.1007/s13596-011-0016-4
Keywords: Citrus tangerina “Dahongpao”, polymethoxylated flavone, tangeretin, anti-inflammation, anti-cancer
Citation: Chen Q, Gu Y, Tan C, Sundararajan B, Li Z, Wang D and Zhou Z (2022) Comparative effects of five polymethoxyflavones purified from Citrus tangerina on inflammation and cancer. Front. Nutr. 9:963662. doi: 10.3389/fnut.2022.963662
Received: 07 June 2022; Accepted: 11 July 2022;
Published: 08 September 2022.
Edited by:
Fabiana Ourique, Universidade Federal de Juiz de Fora, BrazilCopyright © 2022 Chen, Gu, Tan, Sundararajan, Li, Wang and Zhou. This is an open-access article distributed under the terms of the Creative Commons Attribution License (CC BY). The use, distribution or reproduction in other forums is permitted, provided the original author(s) and the copyright owner(s) are credited and that the original publication in this journal is cited, in accordance with accepted academic practice. No use, distribution or reproduction is permitted which does not comply with these terms.
*Correspondence: Dan Wang, wanda@swust.edu.cn; Zhiqin Zhou, fruitnutri@swu.edu.cn